- 1Department of Endoscopy Center, China-Japan Union Hospital of Jilin University, Changchun, China
- 2Department of Immunology, College of Basic Medical Sciences, Jilin University, Changchun, China
Tumor microenvironment is the general term for all non-cancer components and their metabolites in tumor tissue. These components include the extracellular matrix, fibroblasts, immune cells, and endothelial cells. In the early stages of tumors, the tumor microenvironment has a tumor suppressor function. As the tumor progresses, tumor immune tolerance is induced under the action of various factors, such that the tumor suppressor microenvironment is continuously transformed into a tumor-promoting microenvironment, which promotes tumor immune escape. Eventually, tumor cells manifest the characteristics of malignant proliferation, invasion, metastasis, and drug resistance. In recent years, stress effects of the extracellular matrix, metabolic and phenotypic changes of innate immune cells (such as neutrophils, mast cells), and adaptive immune cells in the tumor microenvironment have been revealed to mediate the emerging mechanisms of immune tolerance, providing us with a large number of emerging therapeutic targets to relieve tumor immune tolerance. Gastric cancer is one of the most common digestive tract malignancies worldwide, whose mortality rate remains high. According to latest guidelines, the first-line chemotherapy of advanced gastric cancer is the traditional platinum and fluorouracil therapy, while immunotherapy for gastric cancer is extremely limited, including only Human epidermal growth factor receptor 2 (HER-2) and programmed death ligand 1 (PD-L1) targeted drugs, whose benefits are limited. Clinical experiments confirmed that cytotoxic T-lymphocyte-associated protein 4 (CTLA-4), vascular endothelial growth factor receptor (VEGFR) and other targeted drugs alone or in combination with other drugs have limited efficacy in patients with advanced gastric cancer, far less than in lung cancer, colon cancer, and other tumors. The failure of immunotherapy is mainly related to the induction of immune tolerance in the tumor microenvironment of gastric cancer. Therefore, solving the immune tolerance of tumors is key to the success of gastric cancer immunotherapy. In this study, we summarize the latest mechanisms of various components of the tumor microenvironment in gastric cancer for inducing immune tolerance and promoting the formation of the malignant phenotype of gastric cancer, as well as the research progress of targeting the tumor microenvironment to overcome immune tolerance in the treatment of gastric cancer.
Introduction
Gastric cancer (GC) is one of the most common digestive tract malignancies worldwide, ranking fifth in morbidity and fourth in mortality (1). With the development of early diagnosis technology, although the incidence of GC exhibits a certain downward trend, the fatality rate of patients at an advanced stage that is inoperable is very high, and there is no effective treatment plan to date. In recent years, the rise of tumor immunotherapy has fueled the last hope for patients with advanced GC. Currently, the only targeted immunotherapy regimens for GC are Human epidermal growth factor receptor 2 (HER-2) monoclonal antibody, programmed death 1 (PD-1) monoclonal antibody and programmed death ligand 1 (PD-L1) monoclonal antibody. However, in GC patients, only 15–30% of patients are HER-2 positive, and the benefits of the treatment are limited (2). Although the efficacy of the PD-L1 monoclonal antibody is superior to first-line chemotherapy, the overall median survival of patients is only extended by two months. This may be related to the existence of immune tolerance in some patients (3). Chimeric Antigen Receptor T-Cell (CAR-T) therapy for GC is currently limited to clinical trials and a few case reports. An effective anti-tumor immune response includes effective presentation of antigens by dendritic cell (DC) cells, the activation and proliferation of specific T cells, and the maintenance of a lasting immune response. Inhibition of any of these points will lead to immune tolerance of the tumor (4). Therefore, in-depth exploration of the mechanism of immune tolerance in GC will help develop more effective treatment options.
Tumor microenvironment (TME) is the general term for all non-cancer components and their metabolites and secretions in tumor, which includes a large number of immune infiltrating cells, such as immune infiltrating lymphocytes (TILs). These immune cells constitute the immune microenvironment of the tumor. Current studies confirmed that TME has an important impact on malignant phenotypes such as tumor growth, invasion, metastasis, drug resistance, and immune escape. Stomach has a strong acidic environment and a unique endocrine system, which also makes the tumor microenvironment of gastric cancer different. Tumor immune microenvironment has both tumor-promoting and tumor-suppressing effects. In the stage of tumorigenesis, TME has a tumor-suppressing effect. However, as the tumor progresses, components of the tumor-suppressing microenvironment are continuously inhibited, and the tumor-promoting microenvironment is constantly being suppressed, leading to immune tolerance and tumor progression. In the process of tumor progression, on the one hand, tumors inhibit the function, number, and distribution of cytotoxic immune cells in the tumor microenvironment by competing for metabolites, secreting extracellular vesicles and cytokines, reducing the expression of self-antigens, resulting in immune tolerance. A large number of cancer-promoting immune cells continue to dominate tumors, which accelerates tumor progression and further inhibits the function of cytotoxic immune cells. Targeting the tumor microenvironment to inhibit the positive feedback loop of tumor immune tolerance is expected to contribute to a better treatment of tumors. In this article, we summarize the latest mechanisms of cellular components in the tumor microenvironment of GC for inducing immune tolerance, promoting the formation of the malignant phenotype of GC, and targeting the components of the tumor microenvironment to reduce immune tolerance in the research progress on the treatment of GC.
The constitution of GC TME
The tumor microenvironment of GC is composed of extracellular matrix (ECM), fibroblasts, endothelial cells, mesenchymal stem cells, macrophages, lymphocytes, neutrophils and other cell components. The metabolites and cytokines secreted by these cell components (including GC cells) are also important components of TME. These components in GC TME play their own roles in inducing the immune tolerance to promote the GC progress.
Tumor-associated macrophages
Macrophages infiltrating the tumor microenvironment are called tumor-associated macrophages (TAMs), which have two polarization-activated states, namely, classical M1 polarization with tumor suppressor function and alternatively-activated M2 polarization with tumor-promoting function (5). In GC, M2 polarization of TAM is induced in the tumor microenvironment, and inhibition of M1 polarization is one of the important factors in the formation of immune tolerance (Figure 1A).
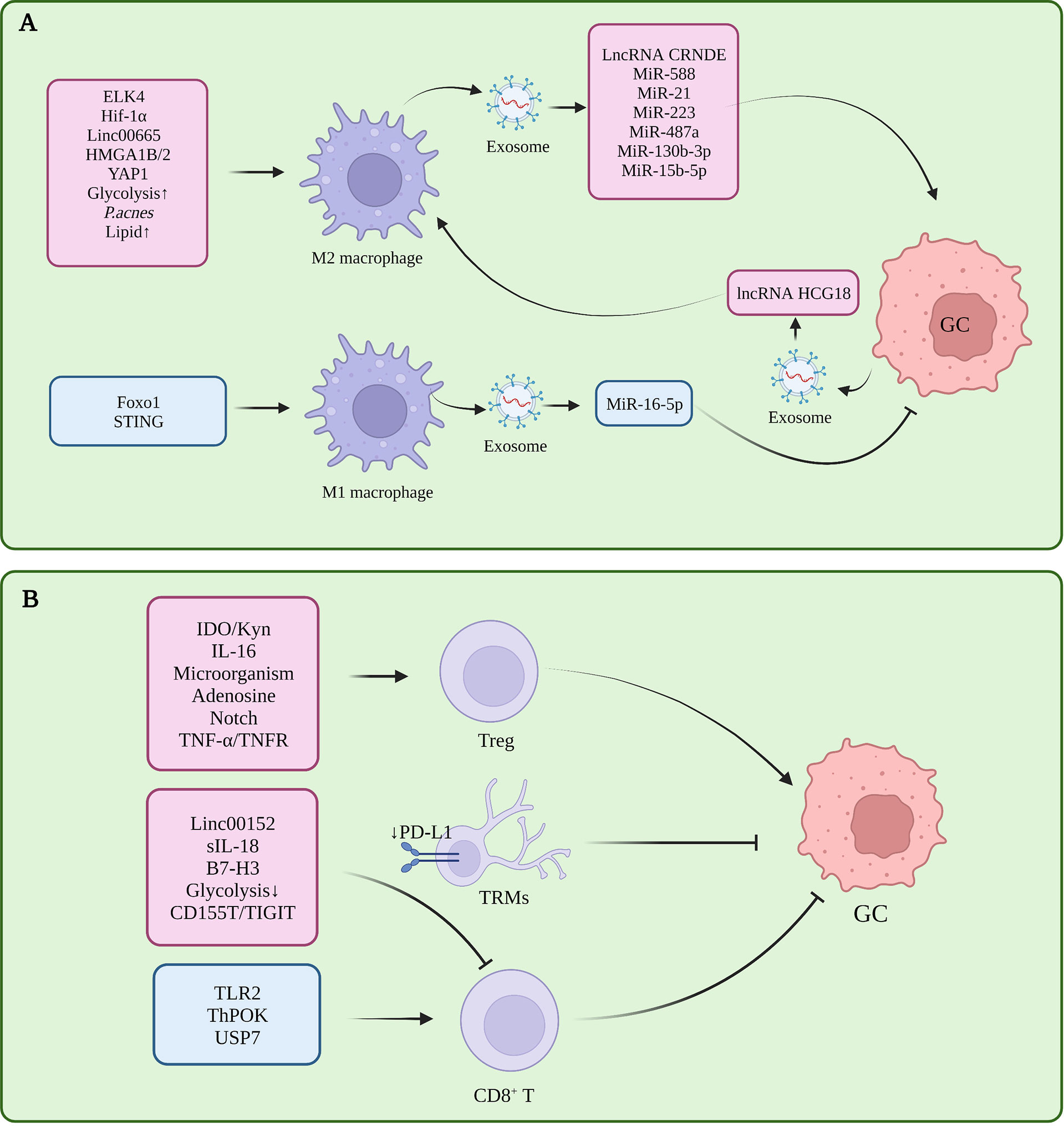
Figure 1 (A) Tumor-associated macrophages in gastric cancer immune tolerance. (B) Tumor-infiltrating T cells in gastric cancer immune tolerance.
Several studies had proved that several molecules participated in M2 polarization in TME, which is closely related to the progress of GC. Pentraxin-3 (PTX3) can inhibit the stemness of GC cells and M2 polarization of macrophages, and prevent the formation of papillary metastases in GC (the early stage of ascites metastasis) (6). ETS-like transcription factor 4 (ELK4) promotes M2 polarization of macrophages by activating lysine-specific demethylase 5A (KDM5A), which inhibits the expression of Praja2 (PJA2) by removing H3K4me3 of the PJA2 promoter, thereby promoting M2 polarization of macrophages (7). Cisplatin induced activation of hypoxia inducible factor 1 alpha subunit (HIF1α) signaling directly drives the transcription of tumor-derived leukemia inhibitory factor, activates the STAT3 signaling pathway, and stimulates M2 polarization of macrophages, thereby promoting the resistance of gastric tumors to chemotherapeutic drugs (8). POU class 1 homeobox 1 (POU1F1) upregulated by High mobility group A 1B/2 (HMGA1B/2) promotes GC metastasis by regulating macrophage M2 polarization in a Chemokine 12 (CXCL12)/CXC motif chemokine receptor type 4 (CXCR4) dependent manner (9). Propionibacterium acnes (P. acnes) promotes gastric cancer progression by promoting M2 polarization of macrophages through Toll-like Receptor 4 (TLR4)/phosphoinositide 3-kinase (PI3K)/protein kinase B (AKT) signaling (10). Calmodulin 2 (CALM2) in GC promotes M2 polarization of macrophages through the Adenylate kinase 2 (AK2)/Signal transducer and activator of transcription 3 (STAT3)/HIF-1/vascular endothelial growth factor A (VEGFA) axis, thereby promoting GC metastasis and angiogenesis (11). Therefore, these molecules or signal pathway related to the M2 polarization might be the potential targets for treating GC effectively.
In addition, CD68+ CD163- M1 macrophages are required for PD-1/PD-L1 monoclonal antibody using in GC treatment (12). Interestingly, the knockdown of STING in THP1 cell line or activation of STING via 2’3’-c-GAMP were shown to promote M1 polarization of macrophages and exert an anti-tumor effect, suggesting that the STING pathway has complex and meaningful regulatory roles in macrophages (13).In gastric cancer, macrophages can induce the transformation of mesenchymal stem cells (MSC) cells into fibroblasts, and then participate in the formation of immune tolerance (14).
In fact, macrophages are an emerging tumor therapeutic target, and current therapeutic modalities for TAM include the inhibition of macrophage recruitment in tumors, depletion of macrophages, induction of macrophage reprogramming to the M1 phenotype, and enhanced phagocytosis of macrophages (15). We look forward to future studies that can demonstrate the critical role of TAMs in GC. Currently, CAR-macrophages have entered the phase I clinical trial stage as the latest CAR cells, but their application in GC remains lacking (16).
T cells
T cells are highly heterogeneous. In TME, CD8+ T cells assume the role of killing tumor cells, while Treg is the most representative CD4+ immunosuppressive cell. In addition to memory T cells, γδ T cells, Nature killing (NK) T cells, and Th cells have been shown to play an important role in tumor progression and immune tolerance in gastric cancer (Figure 1B).
The decrease in the number and dysfunction of CD8+T cells is one of the reasons for gastric cancer immune tolerance. In GC tissues with high expression levels of B7-H3 (CD276), the density of CD8+ T cells within the tumor was reduced, suggesting that B7-H3 may be involved in the mechanism of tumor evasion of immune responses (17). Toll-like receptor 2 (TLR2) was down-regulated in CD8+ T cells of gastric cancer patients, and TLR2 activation could increase the expression of perforin and granzyme B in CD8+ T cells and enhance CD8+ T cells cytotoxicity (18). The chromatin status of tumor-specific T cells is correlated with their dysfunction (19), and GC patients with high open circulating CD8+ T cell chromatin respond better to pembrolizumab (20). CD103+ CD4+ T cells exhibit an immunosuppressive phenotype and high retention capacity in GC tumor tissues, leading to CD8 + T cell dysfunction, and granzyme B (GZMB), interferon-γ (IFN-γ), tumor necrosis factor α (TNF-α), and perforin (PRF-1) reduction (21).
In an in vitro 3D culture model, Treg cells were enriched in early intestinal-type GC and could promote the growth of spheroids by inducing interleukin-2Rα (IL-2Rα) expression and activation of mitogen-activated protein kinases (MAPK) signaling pathway in tumor cells (22). The infiltration level of tumor necrosis factor receptor 2 (TNFR2)+ Tregs increases with the progression of GC. This is a prognostic marker and independent risk factor for GC, and activation of the TNF-α/TNFR2 pathway promotes the immunosuppressive phenotype and function of Tregs (23). Gastric mucosal microbial analysis found that Comamonas and Gaiella were negatively correlated with the number of pDCs and Tregs in GC, and Stenotrophomonas and Selenomonas were positively correlated with the number of pDCs and Tregs in GC, revealing the impact of microorganisms on tumor immunity (24). DAPT, a γ-secretase inhibitor that inactivates Notch signaling, can reduce the immunosuppressive capacity of CD4+CD25+CD127 dim/- Tregs after DAPT treatment in GC (25). CD4+ T cells in GC can promote the up-regulation of PD-L1 in mesenchymal stem cells through p-STAT3, thereby stimulating the proliferation of GC cells. This further stimulates the proliferation of GC cells. However, this study did not specifically explore the subset of CD4+T functions, and the role of Treg remains to be elucidated (26). Therefore, Treg cells infiltrated in GC tissue play an important role in the progression of the disease by inducing immune tolerance. By targeting the inhibition of Treg production or function, this may relieve the immune tolerance state of GC patients, leading to a more effective delay or treatment of the disease.
The zinc finger and BTB domain containing 7B (Zbtb7b, Alias ThPOK) as transcription factors can upregulate sperm tail PG-rich repeat containing 1 (STPG1) and downregulate Tumor necrosis factor receptor superfamily member 12A (TNFRSF12A) at the transcriptional level, inhibiting the proliferation of gastric cancer cells and promoting the proliferation of T cells (27, 28). The CXXC zinc finger protein 4 (CXXC4) can activate T cells by inhibiting the ETS-like transcription factor 1 (ELK1)/MIR100HG pathway, increase the IFN-γ secreted by CD3+ T cells, and relieve the immune tolerance of GC cells (29). Dexamethasone can inhibit immune evasion by inducing T cell glucocorticoid receptor (GR)/STAT3 pathway-mediated downregulation of PD-L1 and Indoleamine 2,3-dioxygenase 1 (IDO1) (30). In GC, ubiquitin-specific processing protease 7 (USP7) directly interacts with PD-L1 to stabilize it. USP7 inhibitors likewise inhibit tumor proliferation and promote PD-1/PD-L1 expression and immune response (31).
T cells are the executors of tumor immunity, as they directly exercise the tumor-killing function. In the context of inducing immune tolerance in the tumor microenvironment, CD8+ T cells appear dysfunction and exhausted, and immune checkpoint inhibitors against CD8+ T cells appear as an inefficient method. Therefore, reversing the immune tolerance microenvironment in TME and restoring the number, infiltration range, and function of CD8+T cells are the most popular solutions to reduce immune tolerance.
Neutrophils
Tumor-associated neutrophils (TAN) are functionally classified as tumor-suppressing N1 cells and tumor-promoting N2 cells. Transforming growth factor beta (TGF-β) induces N1 to N2 polarization (32). A retrospective study showed that a large number of tumor-associated neutrophils infiltrating GC tissue indicate a greater the risk of lymph node metastasis (33). TANs promote the progression of GC by promoting the polarization of IL-17A producing Th subset cells through the B7-H2-extracellular signal-regulated kinase (ERK) pathway (34). In human neutrophils and GC cells co-culturing experiments, blocking the formation of NETs regulates the expression of Bcl-2, Bax, and nuclear factor kappa B (NF-κB) in GC cells, promoting GC cell apoptosis and inhibiting their invasion (35, 36). Tumor-derived GM-CSF activates neutrophils and induces PD-L1 expression in neutrophils through the Janus kinase (JAK) signaling and activator of STAT3 signaling pathway. Activated PD-L1+ neutrophils effectively suppress normal T cell immunity in vitro and promote human GC growth and progression in vivo (37). The FasL (CD95L)+ PD-L2+ neutrophil subpopulation accounts for more than 20% of all neutrophils in advanced GC. This conditional neutrophil (TCN) was treated with FasL and/or PD-L2 antibodies. After treatment, the injection of CD8+ T cells into tumor-bearing mice constructed with SGC-7901 can significantly reduce the tumor volume and increase the infiltration of CD8+ T cells, indicating that this subset of neutrophils is involved in gastric cancer. This has a significant immunosuppressive effect against CD8+ T cells (38). In vitro co-culture experiments and IHC showed that TAN infiltrates PD-1+ T cells, inhibits T cell proliferation, up-regulates the expression of PD-L1, and promotes the formation of an immunosuppressive microenvironment (39). Current studies showed that TAN promotes tumor immune tolerance in tumors by remodeling the ECM, promoting angiogenesis, generating NETs, and interacting with other immune cells (40). Currently, there are also some therapeutic regimens targeting neutrophils to relieve immune tolerance. However, reducing the risk of infection caused by neutrophil levels is still the biggest obstacle to this regimen (Figure 2A).
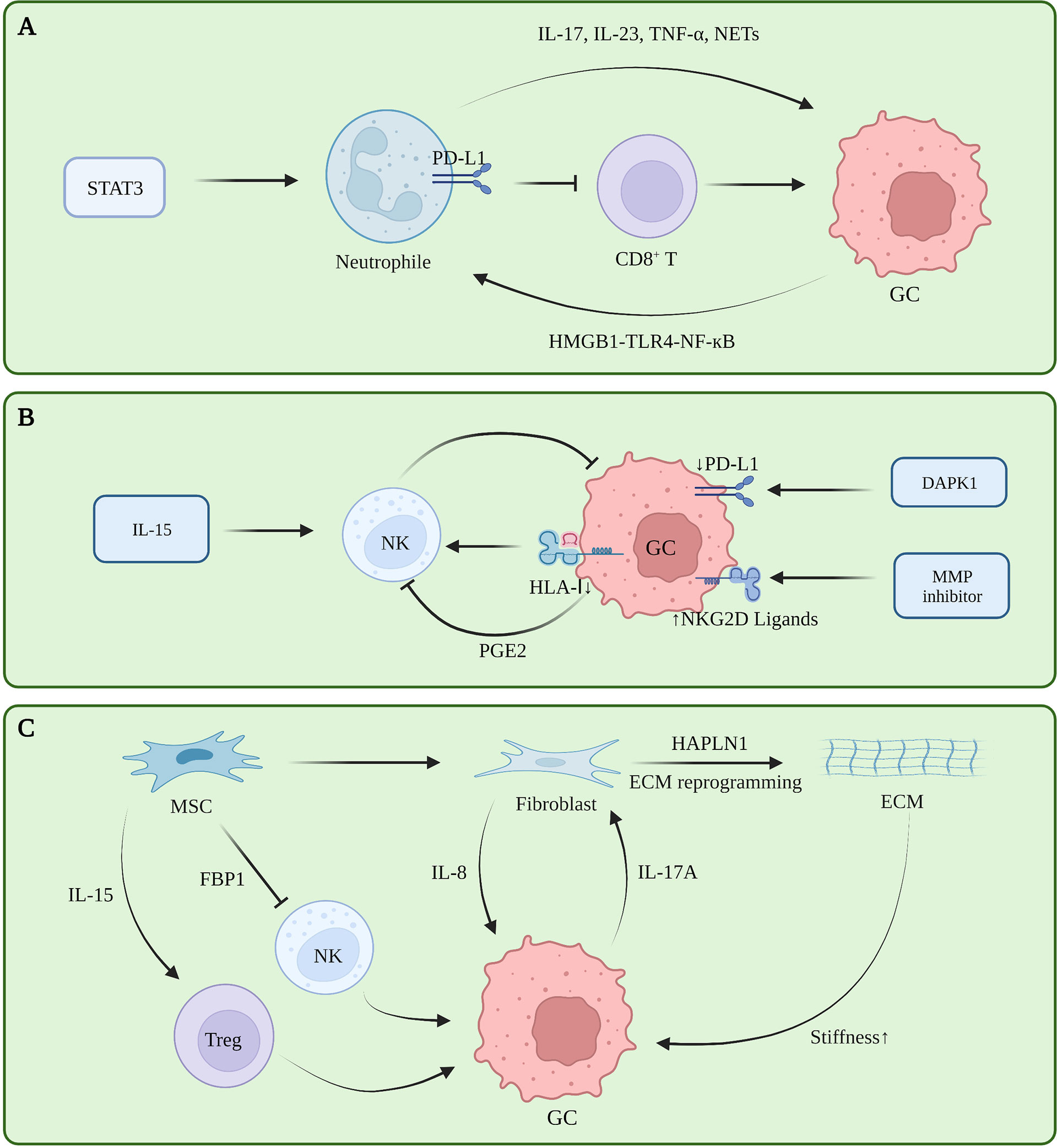
Figure 2 (A) Tumor-associated neutrophils in gastric cancer immune tolerance. (B) NK cells in gastric cancer immune tolerance. (C) ECM, fibroblasts and mesenchymal stem cells in gastric cancer immune tolerance.
NK cells
NK cells can directly kill target cells and recognize tumor cells that CD8+ T fails to recognize. However, NK cells exhibit dysfunctional behavior in TME (41). The infiltration level of NK cells in tumors and the level in peripheral blood are positively correlated with the prognosis of GC patients, and negatively correlated with the expression level of cyclooxygenase-2 (COX-2) (42, 43). The c-myc of NK cells in the peripheral blood of GC patients is down-regulated at the RNA and protein levels, and mitotic arrest is associated with NK dysfunction in GC patients (44). The expression level of the NK activating receptor NK Group 2 Member D (NKG2D) in GC patients is positively correlated with patient prognosis. Although NK cells in the resting state have little cytotoxicity against GC, NK cells induced by the K562-mb15-41BBL cell line in vitro have strong effects on GC cytotoxicity and strong antitumor activity in animal experiments (45). Decreased human leukocyte antigen class I (HLA-I) expression leads to decreased NK cell infiltration in GC and is insensitive to NK cell activity (46). Death-associated protein kinase 1 (DAPK1) downregulates the IKKβ/CSN5 axis in GC, inhibits PD-L1 expression, and activates the killing ability of NK cells (47). Matrix metalloproteinase (MMP)-2, MMP-9, and pan-MMP inhibitors can upregulate the expression of NKG2D ligands in GC, making GC cells more sensitive to NK cells (48). In in vitro experiments, prostaglandin 2 (PGE2) secreted by GC cells can inhibit the proliferation of NK cells and induce their apoptosis (42). IL-15 can activate the activity of NK cells and inhibit the formation of liver metastases in a mouse model of GC liver metastasis (49). iNKT cells are involved in the initial steps of anti-tumor immunity. However, the increased frequency of iNKT in peripheral blood of patients with GC does not bring good benefits to the patients. Subsequent experiments have shown that the ability of iNKT cells to degranulate and produce IFN-γ in patients with GC is impaired (50). Further follow-up studies are required to clarify the heterogeneity of NK cells in GC, and the factors that inhibit NK cell function in GC TME, to find an effective NK cell-based therapeutic regimen for GC (Figure 2B).
ECM
The ECM is a network of collagen, fibronectin, laminin, vitronectin, elastin, as well as growth factors, cytokines, and matrix metalloproteinases that support and maintain the epithelial cell structure (51). These components are mainly secreted by fibroblasts, although other cells in the microenvironment likewise have the ability to secrete these substances (52). In the stage of gastric carcinogenesis, ECM is considered to be an initiating factor of gastric carcinogenesis. Studies showed that different subtypes of GC have different ECM components, and that a lower degree of differentiation indicates a greater abundance of ECM components, higher cell metabolism, and higher degree of metabolic reprogramming (53). Proteomic analysis revealed that ECM components of tumor tissues were no different from normal tissues, whereas their levels varied greatly, which was mainly manifested as increased ECM proteins and decreased basement membrane components that were closely related to tumor angiogenesis, invasion and metastasis, i.e., closely related to the formation of malignant phenotypes (54). During the progression stage of GC, ECM deposits continuously and increases in density, directly interacting with receptors on the surface of tumor cells, reducing E-cadherin/β-catenin, and promoting the proliferation, invasion, and metastasis of GC cells (55). Further, enhanced environmental stress caused by the increased matrix density is likewise an important reason for ECM to promote tumor progression. The researchers cultured GC cells in hydrogels with different stress intensities and found that with the increase of stress, the CD44 expression of tumor cells reversibly became nonfunctional. isomers, promoting the metastasis of GC (56). In fact, the stress role of ECM in breast cancer has been confirmed, and high-strength ECM can promote the epithelial-mesenchymal transition (EMT) process of breast cancer, increase the infiltration of M2 macrophages, and inhibit the function of CD8+ T cells (57, 58). However, this mechanism must be further clarified in GC (Figure 2C).
Fibroblasts
Cancer associated fibroblasts (CAF) are the main cells that secrete and degrade ECM, and also secrete a large number of cytokines, chemokines, and exosomes. In gastric cancer, hyaluronan and proteoglycan link protein 1 (HAPLN1) were the most significantly upregulated genes in fibroblasts. Second harmonic generation imaging with a multi-photon microscope showed that the knockdown removal of HAPLN1 significantly reduces the density, length, width, and number of fibers in the ECM (59). A study on secretomes revealed that a Helicobacter pylori infection can lead to changes in the secretion of fibroblasts in the gastric mucosa, induce metabolic reprogramming and changes in the microenvironment of epithelial cells and tumor cells, and lead to type III EMT changes (especially tumors), where the epithelial-mesenchymal transition is closely related to the occurrence and development of gastric cancer (60, 61). Furthermore, fibroblasts exhibit complex immunomodulatory roles in other tumors. In breast cancer, the Yes-associated protein (YAP) pathway promotes fibroblast-induced ECM hardening, and the hardened ECM activates fibroblasts through YAP again, promoting breast cancer progression and immune tolerance (62). Moreover, various cytokines secreted by fibroblasts have complex regulatory effects on T cells, macrophages, and mast cells. Tumor therapy strategies targeting fibroblasts have also been tested in pancreatic cancer, breast cancer, and other tumors (63), Nevertheless, research in this area on GC remains insufficient (Figure 2C).
Endothelial cells
Angiogenesis provides nutrition and oxygen to the tumor microenvironment and promotes tumor growth. Endothelial cells play an important role in this process (64). Antiangiogenic vascular endothelial growth factor receptor (VGFR) monoclonal antibody and tyrosine kinase inhibitor (TKI) are also one of the treatment schemes for advanced GC. Single cell sequencing revealed the specificity of tumor endothelial cells (TEC) in TME in phenotype and metabolism. Some TECs have the potential to transform into mesenchymal cells in gastric cancer, and these endothelial cells play an important role in angiogenesis (65). Subsequent studies also showed that TEC participated in the formation of tumor immune tolerance under hypoxia (66). TEC can interact with CAF through VEGFA in GC (67).In other tumors, TEC can up regulate the immune checkpoint molecules of T cells and inhibit the activation of T cells (68). TEC expressing FasL can reduce the number of CD8+ T cells and increase the number of Treg (69).
Mesenchymal stem cells
As a type of pluripotent stem cells, mesenchymal stem cells (MSC) can differentiate into fibroblasts in tumors, and further exhibit a tumor suppressor function in some tumors, which is in contrast with the heterogeneity of MSCs and induced differentiation directions in different tumors (70). The heterogeneity of MSC in the tumor microenvironment of GC must be further clarified (Figure 2C).
Endocrine signaling in GC TME
Gastric has endocrine function and can secrete gastrin, cholecystokinin (CCK), secretin and other substances. This is a special characteristic of GC distinct from the other solid tumor, such as lung caner, liver cancer and so on. Which may determine its unique TME for the GC progress. The current research has confirmed that the high expression of gastrin precursor, gastrin, and gastrin downstream receptor CCK2R is an important factor in the occurrence and progression of GC (71). Targeting the gastrin peptide (polyclonal antibody stimulator-PAS) can increase CD8+ T lymphocytes and reduce the number of M2 macrophages in GC (72). Gastric endocrine system can promote gastric cancer, but its role in tumor microenvironment and regulation of immune tolerance of gastric cancer still need further research in the future.
Metabolic heterogeneity GC TME
In gastric cancer TME, the metabolic patterns of various cell components are different. The competition of metabolic substances leads to metabolic reprogramming, thus affecting the function of various cell components in TME, which is one of the reasons for immune tolerance (73).
Glycolysis
TME lacks nutrients, and glucose metabolism is necessary for cell survival. The glucose uptake capacity of gastric cancer cells is significantly higher than other cells in TME. This glucose deficiency will induce other cells in TME to undergo metabolic reprogramming and then lead to their redifferentiation (73). PTEN-induced kinase 1 (PINK1) deficiency in GC causes M2 polarization of TAMs, which is mainly related to the enhanced glycolysis level caused by PINK1 deletion (74). GC cells overexpressing YAP1 can promote M2 polarization by secreting IL-13, which activates the glucose transporter 3 (GLUT3) dependent glycolytic metabolic reprogramming of TAMs (75, 76). While lactate can further promote the M2 polarization of TAM, inhibition of monocarboxylate transporters (MCT) or HIF-1α can significantly reverse this effect (77), hypoxia-induced elevated glycolysis levels can also induce a decrease in M1 macrophages in GC (78), which suggests an important role of glucose metabolism reprogramming in the tumor microenvironment. Polymorphonucler myeloid-derived suppressor cells (PMN-MDSCs) accumulate in GC cells and inhibit CD8+ T cell glycolysis through the S100A8/A9-TLR4/AKT/mechanistic target of rapamycin (mTOR) axis, leading to CD8+ T cell exhaustion, and making GC susceptible to PD-1 therapy immune tolerance (79). In GC, the CD155T/TIGIT signaling pathway can inhibit the uptake of glucose by CD8+ T cells, thereby inhibiting its function (80). MSC can inhibit the glucose uptake and lactate production of NK cells by upregulating FBP1, thereby weakening their glycolytic metabolism and inhibiting the degranulation ability, perforin production, and cytotoxicity of NK cells (81). In GC, inhibition of Glycogen synthase kinase-3beta (GSK-3β) can increase the infiltration of CD8+ T memory stem cells (Tscm) in GC tissue, and promote their differentiation potential and anti-GC ability (82).
High level glycolysis in TME will lead to lactic acid accumulation, induce TME acidification, and inhibit the function of CD8+T cells (83). Increase lactic level not only directly limits the cytolytic function of NK cells, but also indirectly inhibits NK cells by increasing the number of MDSC (84). In addition, high level lactic can also induce M2 polarization of macrophages and enhance Treg function (85).
Lipid
Studies showed that lipid accumulation exists in TAM, and this accumulation of lipids can induce the M2 polarization of TAM, upregulate PD-L1, block the anti-tumor T cell response, and exert an immunosuppressive effect (86). About 30% of tumor-infiltrating lymphocytes (TILs) in the tumor microenvironment of gastric adenocarcinoma are CD69+CD103+ tissue-resident memory T cells (TRMs) cells. Fatty acid oxidation is necessary for the survival of Trm cells, but the uptake of fatty acids by Trm cells is far less than that of GC cells. The PD- L1 blocker can upregulate the expression of Fabp4/5 in gastric cancer Trm, increase its uptake of fatty acids, and thus enhance its anti-tumor activity (87). CD8+CD103+TRMs have stronger anti-tumor activity, but exhibit reduced infiltration in gastric cancer, and their cytolytic capacity can be restored by PD-1 blockade and 4-1BB co-stimulation in vitro (88).
Amino acid
Glutamine is necessary for tumor cells, and its metabolites can inhibit the proliferation of T cells and the secretion of cytokines (89). The IDO/Kyn pathway is a classic immunosuppressive pathway. Kyn derived from GC cells can increase the level of Treg infiltration and induce Treg cells to secrete IL-10, which further activates the STAT3/BCL2 pathway to induce GC resistance to chemotherapy (90). Adenosine is a key immunosuppressive metabolite in the tumor microenvironment (91), and Treg cells isolated from peripheral blood of GC patients have the ability to promote adenosine production, which in turn inhibits the activity of CD8+ T cells through the A2aR pathway (92).
Cytokines in GC TME
Cytokines are regulators of innate and adaptive immunity, and play an important role in the formation of tumor immune tolerance. GC cells can secrete IL-17A to promote the transformation of normal fibroblasts (NF) into tumor-associated fibroblasts (CAF). MSC in GC can upregulate the ratio of Tregs and increase the expression of PD-L1 by secreting IL-15, promoting the EMT process and immune tolerance in GC (93). Fibroblasts promote the proliferation of GC cells by secreting IL-8, forming a positive feedback to promote the malignant phenotype of GC access (94). Hepatocyte growth factor (HGF) secreted by CAF can promote angiogenesis of GC through PI3K/AKT and ERK1/2 pathways (95). Elevated serum interleukin 8 (sIL-8) levels are closely related to poor prognosis and lymph node metastasis in GC, sIL- 8 can promote GC metastasis by increasing the PD-1 expression of CD8+ T (96). GC-derived TGF-β1 promotes PD-1- independent CD8+ T cell dysfunction, and the restoration of CD8+ T cell function by combined blockade of PD-1 and TGF-β1 may benefit future GC immunotherapy (97). Cytokines and ECM reorganization in the tumor microenvironment are key factors in the transformation of macrophages from M1 to M2 (98). Studies have shown that TAM can inhibit the function of natural killer (NK) cells in the tumor microenvironment by secreting TGF-β1 (99). M2 macrophage secreted CHI3L1 can play the same role by binding to IL-13Rα2 (100). TAM can activate the NF-κB and STAT3 signaling pathways of GC cells by secreting TNF-α and IL-6, upregulate the expression of PD-L1, and promote the immune escape and proliferation of GC cells (101). The overexpression of secreted acidic cysteine-rich protein (SPARC) in M2 macrophages can inhibit its tumor-promoting effect (102). Studies have also shown that CXCL8 secreted by M2 macrophages can upregulate their own PD-L1 levels (103). IL-4-stimulated EGFR transactivation helps suppress M2 polarization in macrophages, and TAMs in patients with advanced GC have low epidermal growth factor receptor (EGFR) expression, which may be related to the resistance to EGFR monoclonal antibody therapy (104).Tumor-associated neutrophils activate AKT and p38 pathways in MSCs by secreting inflammatory molecules, such as IL-17, IL-23, and TNF-α, inducing their transformation into fibroblasts, and promoting the development of gastric cancer (105). IL-17a produced by TAN can also promote the EMT process of GC through the JAK2/STAT3 pathway (106).
Noncoding RNAs and exosomes in GC TME
Non coding RNA is a kind of RNA without coding function, including miRNA, lncRNA and circle RNA. Non coding RNA can regulate gene expression and protein function, and form a complex regulatory network. In addition, non coding RNA can also affect other cells in the tumor microenvironment through exosomes, which plays an important role in the formation of tumor immunity. The interaction between Linc00665 and BACH1 leads to the activation and binding of BACH1 to the Wnt1 promoter, promoting M2 polarization of TAMs and GC progression (107). LncRNA CRNDE (108), miR-588 (109), miR-21 (110) in M2-polarized TAM-derived exosomes can enhance the resistance of GC cells to cisplatin, while miR-223 enhances GC cell resistance to doxorubicin (111), Meanwhile, miR-487a, miR-130b-3p can promote the progression of GC (112, 113). M2 macrophage-derived miR-15b-5p can promote GC metastasis through the BRMS1/DAPK1 axis (114). M1-polarized TAM-derived exosomes can down-regulate the expression of PD-L1 in gastric cancer cells through miR-16-5p and activate T cell immunity (115). Linc00152 inhibits CD8 + T cell infiltration in GC by binding to enhancer of zeste homolog 2 (EZH2) and regulating the CXCL9, 10/CXCR3 axis (116).
Gastric cancer-derived exosomes can induce the PD-1+ phenotype in TAMs, most of which will differentiate into M2 macrophages. Furthermore, these exosomes can inhibit the proliferation of CD8+ T cells in the microenvironment promotes the secretion of IFN-γ, which in turn promotes the progression of GC (117). Docking protein-1 (DOK1) downregulates the expression of PD-L1 in TAM (118). Another study showed that gastric cancer-derived exosomes could promote the M2 polarization of TAMs through the lncRNA HCG18-miR-875-3p-Kruppel-like factor 4 (KLF4) pathway (119). The overexpression of lncRNA ANCR promotes GC cell invasion and metastasis by inhibiting the polarization of macrophages toward M1 by downregulating Foxo1 (120). In GC, the low expression of miR-128-3p is closely related to the poor prognosis of patients. The direct target of miR-128-3p is IL-16, which can reduce the infiltration of CD4+ CD25+ Foxp3+ Tregs in GC tissue by inhibiting the expression of IL-16 (121). MiR-105-5p is expressed in GC at low levels, and overexpression of miR-105-5p can directly inhibit the expression of PD-L1 and activate CD8+ T cells (122). γδ T cells are a class of T cells recently discovered to have important functions in tumor immune tolerance, Vγ9Vδ2 T cells are the main subset of γδ T, and gastric cancer-derived exosome miR-135b-5p can damage anti-tumor function of Vγ9Vδ2 T cells by targeting SP1 (123). MiR-451 in gastric cancer-derived exosomes can transfer to T cells and activate the mTOR pathway, inducing their differentiation into Th-17 cells (124). Gastric cancer-derived exosomal PD-L1 is upregulated in advanced GC patients treated with 5-FU, leading to systemic immune tolerance (125). Mir-1290 in gastric cancer-derived exosomes can inhibit T cell proliferation through the grainyhead-like 2 (Grhl2)/zinc finger E-box binding homeobox 1 (ZEB1)/PD-L1 axis and participate in immune tolerance (126). PD-L1 in exosomes has an immunosuppressive effect in tumors, and histone lysine-specific demethylase 1 (LSD1) can upregulate the level of PD-L1 in gastric cancer-derived exosomes and induce T cell immune resistance (127). GC cell-derived exosomes induce neutrophil autophagy and tumor-promoting activation through the high mobility group box-1 (HMGB1)/TLR4/NF-κB signaling pathway, ultimately promoting the proliferation and migration of GC cells (128). Gastric cancer-derived exosomes can upregulate PD-L1 expression in neutrophils by transporting HMGB1, thereby inhibiting T cell function (129).
Immunotolerance targeting therapies in GC
Immune cells and non-immune cell components in the microenvironment induce tumor immune tolerance through a variety of mechanisms, which plays an important role in the occurrence and development of GC. Therefore, targeted intervention in the key links of immune tolerance in the microenvironment of GC is expected to become an effective strategy for its treatment. To date, this treatment mainly includes CAR modified cell therapy, herbal medicines, monomer drugs, oncolytic viruses, and other biological agents (Table 1).
CAR-cell
In recent years, CAR-cell, including CAR-T and CAR-NK had been developed for treating GC. Mesothelin (MSLN)-CAR-T cells can effectively inhibit the growth of GC cells in the Patient-derived tumor xenograft (PDX) model (130). Mesenchymal-epithelial transition factor (cMet)-PD1/CD28 CAR-T is a second-generation CAR. The researchers constructed a PD1/CD28 chimeric switch receptor by fusing the extracellular domain of PD-1 with the transmembrane and intracellular domains of CD28. Converting the inhibitory signal of PD-1 into the activation signal of CD28 can effectively inhibit the growth of GC in vitro and in vivo, and increase the infiltration of central memory T cells, prolong the long-term anti-tumor effect, and reducing the secretion of inflammatory factor IL-6 (131). The intercellular adhesion molecule 1 (ICAM-1) is expressed in nearly 50% of GC patients. In mouse models, CAR-T cells targeting ICAM-1 can target both primary and metastatic gastric cancers, exhibiting a good therapeutic effect (132). In a mouse model, anti-CD133 chimeric antigen receptor T (CAR-T) can selectively target cisplatin-resistant GC stem cells, and the combined use of cisplatin can improve the therapeutic effect (133). Nanobody VHH1-driven CAR-T (CDH17CART) targeting CDH17 can effectively treat gastrointestinal tumors without affecting normal epithelial cells in mouse experiments (134). Claudin18.2 (CLDN18.2) is a gastric-specific membrane protein, and CLDN18.2-specific CAR-T cells can effectively partially or completely eliminate GC in the PDX model. To date, this treatment passed phase I clinical trials (135, 136). Bispecific trophoblast cell surface antigen 2 (Trop2)/PD-L1-specific third-generation CAR-T cells were developed through lentiviral infection, which can effectively kill GC cells in vitro (137). A phase I clinical trial showed that in vitro expanded NK cells combined with trastuzumab or cetuximab had a certain therapeutic effect on GC, which was well tolerated by patients (138). A dual-targeting chimeric receptor (DTCR) PD1-DAP10/NKG2D increases the expression of PD1 and NKG2D on the surface of NK92 cells by viral transfection, and has comparable anti-tumor properties in a mouse tumor-bearing model constructed with SGC-7901 activity (139). MSLN-CAR NK cells constructed based on NK-92 cells can effectively kill MSLN-positive GC cells in vitro and inhibit tumor growth in the PDX model, with a large number of NK cells infiltrating the tumor (140). CAR cells constructed from various immune cells have shown a certain curative effect in animal models of GC. However, whether these treatments are effective for GC patients, and whether the selection of patients is targeted for their application, still needs extensive research.
Monomer drug
Studies have shown that the antiallergic drug Tranilast can inhibit the secretory function of fibroblasts in peritoneal metastatic GC tissue, effectively improve the tumor microenvironment, increase the infiltration of CD8+ T cells, as well as reduce the infiltration of M2 macrophages and mast cells. This leads to reduced proliferation and fibrosis of GC cells (141). Futibatinib is a novel FGFR1-4 inhibitor that exhibits broad-spectrum antitumor effects in various tumors, including gastric cancer (142). Metformin can promote the secretion of calmodulin-like protein 3 (Calml3) from CAF cells and inhibit the progression of GC (143). In patients with advanced GC, the application of large doses of PPI can inhibit the exosome secretion function of fibroblasts, improve the tumor microenvironment, and inhibit the malignant degree of GC (144). Nevertheless, the effect of PPI on gastric cancer remains controversial. Itraconazole can inhibit the activity of endothelial cells and fibroblasts in GC and alleviate the resistance of GC cells to bevacizumab (145). A selective inhibitor of PI3K-γ isoenzyme, IPI549, restores macrophage function and promotes anti-tumor T cell responses (86). Experiments in vivo show that methionine enkephalin (MENK) can promote M1 polarization of macrophages and upregulate the expression of opioid receptor (OGFr) by blocking the PI3K/AKT/mTOR signaling pathway, which inhibits GC cells (146). In CD8+ cells isolated from peripheral blood of tumor patients, the TLR2 agonist Pam3Csk4 enhanced the cytolytic activation of peripheral and tumor-infiltrating CD8+ T cells from GCs (18). De novo DNA methylation is acquired by PD1+CD8+ tumor-infiltrating T cells (TILs), which results in graded downregulation of cytokines, such as interferon-γ (IFN-γ), while 5-Aza-2’-deoxycytidine (DAC) de novo blocks DNA methylation in activated PD1+CD8+ TILs (147). The CCL28 blockade inhibits Treg cell infiltration and tumor progression in the mouse model (148). The single drugs mentioned above affect tumor immune tolerance through different mechanisms, thus playing a certain role in the treatment of GC. However, the targeting problem of monomeric drugs in vivo may be dangerous, diminishing the therapeutic effect and even causing serious side effects. Improving the targeting precision of their actions, such as by combining them with monoclonal antibodies, may solve this problem.
Herbal medicine
Herbs are widely used as tumor immune regulators and chemotherapeutic sensitizers. Although the therapeutic effect of herbs and their natural compounds is not as significant as that of classical drugs, their advantages of low toxicity and low side effects endow them with potential in tumor treatment (172, 173). In addition, some herbal medicines play an important role in the metabolic regulation of gastrointestinal tumors (174).
Berberine has complex functions in gastrointestinal tumors, including including autophagy, immunity, inflammation, modification of the gut microbiota and miRNA. Berberine is an inhibitor of CD47, which can enhance the phagocytosis of macrophages, and enhance the therapeutic effects of CD47 antibody and rituximab (149). Paeoniflorin improves the immune microenvironment of GC and inhibits the invasion and metastatic ability of GC by inhibiting the secretion of IL-6 in fibroblasts in GC tissue (175). Astragaloside IV and Triptonide can inhibit the cancer-promoting function of fibroblasts in GC (150, 151). Sophoridine inhibits M2-TAM polarization via the TLR4/IRF3 axis, increases CD8+ T proliferation and cytotoxic function, and downregulates the expression of CD8+ T cell exhaustion markers PD-1, Tim-3, and Lag-3 (152). Oleanolic acid can promote the balance of Treg/Th17 cells in GC by targeting IL-6 through miR-98-5p, and is a potential drug for the treatment of GC (153, 154).
Other biological agents
Several researchers constructed an oncolytic virus carrying relaxin (RLX), which can degrade ECM components in tumors (155). Results showed that in in vivo experiments, the oncolytic virus carrying RLX could effectively degrade the ECM of gastric cancer and increase the activated ECM. The accumulation of cytotoxic T cells and trastuzumab and PD-1 mAbs in gastric cancer tissues yielded significant anti-tumor effects (155). A fiber-modified hexon chimeric recombinant oncolytic adenovirus targeting CAFs can relatively specifically kill gastric CAFs and inhibit GC cell growth in vivo (156). G47Δ, a third-generation oncolytic herpes simplex virus type 1 virus, has passed phase II clinical trials in glioma, and has demonstrated significant anticancer effects in orthotopic tumor models and peritoneal dissemination models of GC. M2 macrophages were decreased, while M1 macrophages and NK cells were increased (157). A research group developed an injectable shear-thinning hydrogel, co-loaded with polyphyllin II (PP2) and resiquimod (R848) (PR-Gel for short), which induces TAM cell M2 in a mouse model of GC. Enhanced repolarization and CD8+ T cell infiltration to M1 exhibited favorable tumor suppressive effects (158). In in vitro experiments, the CD137 antibody can effectively induce apoptosis in primary GC cells by enhancing CD8+ T cells via activation of NF-κB signaling (159). A novel tumor-penetrating peptide, iRGD-anti-CD3, can immobilize iRGD on the surface of T cells through CD3 binding, promote T cell infiltration, and increase T cell activation and cytotoxicity to target cancer cells in 3D culture models and in vivo experiments (160, 161). Replacing the PH20 signal peptide with the tPA signal peptide and linking the IgG2 Fc fragment to construct human hyaluronidase PH20 (referred to as sPH20-IgG2) can enhance the cytotoxicity of MSLN CAR-T against GC in a mouse model (162, 163). Targeting Lewis Y and CD3 (m3s193 BsAb), a formatted novel T cell-binding bispecific antibody with IgG-[L]-scfv exhibited promising anti-GC activity in a mouse huPBMCs/GC co-transplantation model (164). Hydroxypropyl cellulose photocrosslinked hydrogel incorporating IFN-α2b can ensure the activity of IFN-α2b, stably release IFN-α2b to stimulate T cells over a long time, and combined with low-dose radiation of 5 Gy can induce activated T cells into tumor tissue, increasing the immunotherapy effect (165). The conjugation of αPD1 (i.e., nivolumab) to poly(ethylene glycol) (PEG) and poly(ϵ-caprolactone) (PCL) copolymers with PEG as linker (αPD1-PEG-PCL) by double emulsion solvent evaporation, encapsulating DAC in αPD1-PEG-PCL, this drug can better target PD1+CD8+ TIL to inhibit and kill GC cells (166). Anti-TGF-β/PD-L1 bispecific antibody YM101 is superior to anti-TGF-β and anti-PD-L1 monotherapies, increasing the numbers of tumor infiltrating lymphocytes and dendritic cells, elevating the ratio of M1/M2, and enhancing cytokine production in T cells (176). As an agonist of STING, bivalent manganese (Mn2+) can cooperate with YM101 to produce more lasting anti-tumor effect and enhance the presentation of tumor antigen (177). M7824 (MSB0011359C) is a bifunctional fusion protein composed of a monoclonal antibody against PD-L1 fused to the extracellular domain of TGF-β receptor II, the dual anti-immunosuppressive function of M7824 resulted in activation of both the innate and adaptive immune systems, which contributed to M7824’s antitumor activity relative to monotherapies (178). The above three bioagents had been confirmed that they could suppress multiple tumor cell lines, including colon cancer, lung cancer, breast cancer. Although it is not reported that their roles in GC, it provides potential therapy to GC in future. The DC cell vaccine loaded with MG-7 antigen (MG-7Ag) significantly activates specific cytotoxic T lymphocytes in the GC PDX model (167). Polylactic-co-glycolic acid nanoparticles (NPs) are encapsulated by DC cells, and the GC cell soluble lysate can enhance the differentiation of T cells to Th1 in tumors and enhance the effect of the DC vaccine (168). Dendritic cells modified by the SLC gene can promote DC maturation, enhance the ability of DCs to T cell chemotaxis and T cell stimulation, and induce specific anti-GC cellular immunity (169). The heat shock protein (HSP)-glycoprotein (gp) 96 can enhance the antigen-presenting ability of DC cells and the activity of NK cells in vitro (170). The fusion protein dsNKG2D-IL-15 can recruit and activate NK cells and inhibit the growth of GC in a nude mouse model (171).
Conclusion
In fact, there is no lack of immune cells in tumors, including immune cells with tumor suppressor functions. However, the exhaustion and functional inhibition of these cells are fundamental reasons for tumor immune tolerance and immune escape. In-depth research is particularly important for tumors such as GC, which have a large patient base and few treatment options. However, current research on tumor immunity of GC is significantly less than that of lung, colon, and other cancers, and numerous classical mechanisms of action have not been confirmed in GC. This may be related to the unique anatomical characteristics of gastric tissue. As an important part of the digestive system, the stomach is in close contact with various foods ingested from the outside world, thus maintaining its immune tolerance to food. At the same time, due to the long-term high gastric acid environment, Whether it also constitutes a unique immune microenvironment in the stomach may be inseparable from the occurrence and development of GC. The immune escape of gastric cancer is closely related to its tumor microenvironment, especially considering the changes in metabolic patterns and metabolites of each cell component in it, as well as the role of the secretion of each cell component. The dynamic changes in the tumor microenvironment in the tumor-promoting direction during tumor progression are of great significance. Reversing the reprogramming of the tumor microenvironment to counter the tumor-promoting direction can effectively solve the immunosuppression of tumors. Numerous traditional medicines have also been found to exert anti-tumor effects, which suggests that we must pay attention to the possibility of traditional medicines and traditional Chinese medicines as immunomodulators and sensitizers in tumor treatment. Considering the currently popular CAR-T, the main problems involve making CAR-T effectively infiltrate solid tumor tissue, making CAR-T play a lasting role in the immunosuppressive tumor microenvironment, and finding effective chimeric targets in as many tumor cells as possible, newer modifications and gene targets remain to be developed. NK cells and neutrophils as emerging therapeutic directions in GC must also be further studied.
With the development of omics technology, the heterogeneity of GC and various cellular components in GC TME are well known to us. Their differences are the only way to find solutions to the immune tolerance of GC and achieve precision medicine. Future research must explore the relationship and differences between GC and TME from a holistic perspective, find targets for the overall tumor microenvironment, and determine novel directions for solving GC immune tolerance.
Author contributions
CFL and WY conceptualized the study. YDL drafted the manuscript. YPL and CL conducted the literature review. All authors contributed to the article and approved the submitted version.
Funding
This review was supported by grants from the Natural Science Foundation of China (No. 81872323, No. 82073299), Finance Department of Jilin Province (2021SCZ12, 2019SCZ013) and project of Health Commission of Jilin Provincial (No. 2021JL035).
Conflict of interest
The authors declare that the research was conducted in the absence of any commercial or financial relationships that could be construed as a potential conflict of interest.
Publisher’s note
All claims expressed in this article are solely those of the authors and do not necessarily represent those of their affiliated organizations, or those of the publisher, the editors and the reviewers. Any product that may be evaluated in this article, or claim that may be made by its manufacturer, is not guaranteed or endorsed by the publisher.
References
1. Sung H, Ferlay J, Siegel RL, Laversanne M, Soerjomataram I, Jemal A, et al. Global cancer statistics 2020: Globocan estimates of incidence and mortality worldwide for 36 cancers in 185 countries. CA Cancer J Clin (2021) 71(3):209–49. doi: 10.3322/caac.21660
2. Van Cutsem E, Bang Y-J, Feng-yi F, Xu JM, Lee K-W, Jiao S-C, et al. Her2 screening data from toga: Targeting Her2 in gastric and gastroesophageal junction cancer. Gastric Cancer (2015) 18(3):476–84. doi: 10.1007/s10120-014-0402-y
3. Janjigian YY, Shitara K, Moehler M, Garrido M, Salman P, Shen L, et al. First-line nivolumab plus chemotherapy versus chemotherapy alone for advanced gastric, gastro-oesophageal junction, and oesophageal adenocarcinoma (Checkmate 649): A randomised, open-label, phase 3 trial. Lancet (2021) 398(10294):27–40. doi: 10.1016/S0140-6736(21)00797-2
4. Makkouk A, Weiner GJ. Cancer immunotherapy and breaking immune tolerance: New approaches to an old challenge. Cancer Res (2015) 75(1):5–10. doi: 10.1158/0008-5472.can-14-2538
5. Chen D, Zhang X, Li Z, Zhu B. Metabolic regulatory crosstalk between tumor microenvironment and tumor-associated macrophages. Theranostics (2021) 11(3):1016–30. doi: 10.7150/thno.51777
6. Cui X, Qin T, Zhao Z, Yang G, Sanches JGP, Zhang Q, et al. Pentraxin-3 inhibits milky spots metastasis of gastric cancer by inhibiting M2 macrophage polarization. J Cancer (2021) 12(15):4686–97. doi: 10.7150/jca.58698
7. Zheng L, Xu H, Di Y, Chen L, Liu J, Kang L, et al. Elk4 promotes the development of gastric cancer by inducing M2 polarization of macrophages through regulation of the Kdm5a-Pja2-Ksr1 axis. J Transl Med (2021) 19(1):342. doi: 10.1186/s12967-021-02915-1
8. Yu S, Li Q, Wang Y, Cui Y, Yu Y, Li W, et al. Tumor-derived lif promotes chemoresistance Via activating tumor-associated macrophages in gastric cancers. Exp Cell Res (2021) 406(1):112734. doi: 10.1016/j.yexcr.2021.112734
9. Tang C, Lei X, Xiong L, Hu Z, Tang B. Hmga1b/2 transcriptionally activated-Pou1f1 facilitates gastric carcinoma metastasis Via Cxcl12/Cxcr4 axis-mediated macrophage polarization. Cell Death Dis (2021) 12(5):422. doi: 10.1038/s41419-021-03703-x
10. Li Q, Wu W, Gong D, Shang R, Wang J, Yu H. Propionibacterium acnes overabundance in gastric cancer promote M2 polarization of macrophages Via a Tlr4/Pi3k/Akt signaling. Gastric Cancer (2021) 24(6):1242–53. doi: 10.1007/s10120-021-01202-8
11. Mu G, Zhu Y, Dong Z, Shi L, Deng Y, Li H. Calmodulin 2 facilitates angiogenesis and metastasis of gastric cancer Stat3/Hif-1a/Vegf-a mediated macrophage polarization. Front Oncol (2021) 11:727306. doi: 10.3389/fonc.2021.727306
12. Zhao R, Wan Q, Wang Y, Wu Y, Xiao S, Li Q, et al. M1-like tams are required for the efficacy of pd-L1/Pd-1 blockades in gastric cancer. Oncoimmunology (2020) 10(1):1862520. doi: 10.1080/2162402X.2020.1862520
13. Miao L, Qi J, Zhao Q, Wu Q-N, Wei D-L, Wei X-L, et al. Targeting the sting pathway in tumor-associated macrophages regulates innate immune sensing of gastric cancer cells. Theranostics (2020) 10(2):498–515. doi: 10.7150/thno.37745
14. Zhang Q, Chai S, Wang W, Wan C, Zhang F, Li Y, et al. Macrophages activate mesenchymal stem cells to acquire cancer-associated fibroblast-like features resulting in gastric epithelial cell lesions and malignant transformation. Oncol Lett (2019) 17(1):747–56. doi: 10.3892/ol.2018.9703
15. Xia Y, Rao L, Yao H, Wang Z, Ning P, Chen X. Engineering macrophages for cancer immunotherapy and drug delivery. Adv Mater (2020) 32(40):e2002054. doi: 10.1002/adma.202002054
16. Pan K, Farrukh H, Chittepu VCSR, Xu H, Pan C-X, Zhu Z. Car race to cancer immunotherapy: From car T, car nk to car macrophage therapy. J Exp Clin Cancer Res (2022) 41(1):119. doi: 10.1186/s13046-022-02327-z
17. Ulase D, Behrens H-M, Krüger S, Zeissig S, Röcken C. Gastric carcinomas with stromal B7-H3 expression have lower intratumoural Cd8+ T cell density. Int J Mol Sci (2021) 22(4):2129. doi: 10.3390/ijms22042129
18. Xu J, Guo R, Jia J, He Y, He S. Activation of toll-like receptor 2 enhances peripheral and tumor-infiltrating Cd8 T cell cytotoxicity in patients with gastric cancer. BMC Immunol (2021) 22(1):67. doi: 10.1186/s12865-021-00459-z
19. Philip M, Fairchild L, Sun L, Horste EL, Camara S, Shakiba M, et al. Chromatin states define tumour-specific T cell dysfunction and reprogramming. Nature (2017) 545(7655):452–6. doi: 10.1038/nature22367
20. Shin HM, Kim G, Kim S, Sim JH, Choi J, Kim M, et al. Chromatin accessibility of circulating Cd8 T cells predicts treatment response to pd-1 blockade in patients with gastric cancer. Nat Commun (2021) 12(1):975. doi: 10.1038/s41467-021-21299-w
21. Gu Y, Chen Y, Jin K, Cao Y, Liu X, Lv K, et al. Intratumoral Cd103cd4 T cell infiltration defines immunoevasive contexture and poor clinical outcomes in gastric cancer patients. Oncoimmunology (2020) 9(1):1844402. doi: 10.1080/2162402X.2020.1844402
22. Rocha S, Basto AP, Ijsselsteijn ME, Teles SP, Azevedo MM, Gonçalves G, et al. Immunophenotype of gastric tumors unveils a pleiotropic role of regulatory T cells in tumor development. Cancers (Basel) (2021) 13(3):421. doi: 10.3390/cancers13030421
23. Qu Y, Wang X, Bai S, Niu L, Zhao G, Yao Y, et al. The effects of tnf-α/Tnfr2 in regulatory T cells on the microenvironment and progression of gastric cancer. Int J Cancer (2022) 150(8):1373–91. doi: 10.1002/ijc.33873
24. Ling Z, Shao L, Liu X, Cheng Y, Yan C, Mei Y, et al. Regulatory T cells and plasmacytoid dendritic cells within the tumor microenvironment in gastric cancer are correlated with gastric microbiota dysbiosis: A preliminary study. Front Immunol (2019) 10:533. doi: 10.3389/fimmu.2019.00533
25. Yang L, Zhao KL, Qin L, Ji DX, Zhang B, Zheng PF, et al. Notch signaling pathway regulates Cd4(+)Cd25(+)Cd127(Dim/-) regulatory T cells and T helper 17 cells function in gastric cancer patients. Biosci Rep (2019) 39(5):BSR20182044. doi: 10.1042/bsr20182044
26. Xu R, Zhao X, Zhao Y, Chen B, Sun L, Xu C, et al. Enhanced gastric cancer growth potential of mesenchymal stem cells derived from gastric cancer tissues educated by Cd4 T cells. Cell Prolif (2018) 51(2):e12399. doi: 10.1111/cpr.12399
27. Xia L, Jiang L, Chen Y, Zhang G, Chen L. Thpok transcriptionally inactivates Tnfrsf12a to increase the proliferation of T cells with the involvement of the nf-kb pathway. Cytokine (2021) 148:155658. doi: 10.1016/j.cyto.2021.155658
28. Chen Y, Jiang L, Xia L, Zhang G, Chen L. Thpok inhibits the immune escape of gastric cancer cells by inducing Stpg1 to inactivate the erk pathway. BMC Immunol (2022) 23(1):16. doi: 10.1186/s12865-022-00485-5
29. Li P, Ge D, Li P, Hu F, Chu J, Chen X, et al. Cxxc finger protein 4 inhibits the Cdk18-Erk1/2 axis to suppress the immune escape of gastric cancer cells with involvement of Elk1/Mir100hg pathway. J Cell Mol Med (2020) 24(17):10151–65. doi: 10.1111/jcmm.15625
30. Xiang Z, Zhou Z, Song S, Li J, Ji J, Yan R, et al. Dexamethasone suppresses immune evasion by inducing Gr/Stat3 mediated downregulation of pd-L1 and Ido1 pathways. Oncogene (2021) 40(31):5002–12. doi: 10.1038/s41388-021-01897-0
31. Wang Z, Kang W, Li O, Qi F, Wang J, You Y, et al. Abrogation of Usp7 is an alternative strategy to downregulate pd-L1 and sensitize gastric cancer cells to T cells killing. Acta Pharm Sin B (2021) 11(3):694–707. doi: 10.1016/j.apsb.2020.11.005
32. Fridlender ZG, Sun J, Kim S, Kapoor V, Cheng G, Ling L, et al. Polarization of tumor-associated neutrophil phenotype by tgf-β: “N1” versus “N2” tan. Cancer Cell (2009) 16(3):183–94. doi: 10.1016/j.ccr.2009.06.017
33. Wang Y, Zhai J, Zhang T, Han S, Zhang Y, Yao X, et al. Tumor-associated neutrophils can predict lymph node metastasis in early gastric cancer. Front Oncol (2020) 10:570113. doi: 10.3389/fonc.2020.570113
34. Shan Z-G, Chen J, Liu J-S, Zhang J-Y, Wang T-T, Teng Y-S, et al. Activated neutrophils polarize protumorigenic interleukin-17a-Producing T helper subsets through tnf-α-B7-H2-Dependent pathway in human gastric cancer. Clin Transl Med (2021) 11(6):e484. doi: 10.1002/ctm2.484
35. Li R, Zou X, Zhu T, Xu H, Li X, Zhu L. Destruction of neutrophil extracellular traps promotes the apoptosis and inhibits the invasion of gastric cancer cells by regulating the expression of bcl-2, bax and nf-κb. Onco Targets Ther (2020) 13:5271–81. doi: 10.2147/OTT.S227331
36. Zhu T, Zou X, Yang C, Li L, Wang B, Li R, et al. Neutrophil extracellular traps promote gastric cancer metastasis by inducing Epithelial-Mesenchymal transition. Int J Mol Med (2021) 48(1):127. doi: 10.3892/ijmm.2021.4960
37. Wang T-T, Zhao Y-L, Peng L-S, Chen N, Chen W, Lv Y-P, et al. Tumour-activated neutrophils in gastric cancer foster immune suppression and disease progression through gm-Csf-Pd-L1 pathway. Gut (2017) 66(11):1900–11. doi: 10.1136/gutjnl-2016-313075
38. Shan Z-G, Zhao Y-L, Zhang J-Y, Yan Z-B, Wang T-T, Mao F-Y, et al. Fasl pd-L2 identifies a novel immunosuppressive neutrophil population in human gastric cancer that promotes disease progression. Adv Sci (Weinh) (2022) 9(5):e2103543. doi: 10.1002/advs.202103543
39. Hiramatsu S, Tanaka H, Nishimura J, Yamakoshi Y, Sakimura C, Tamura T, et al. Gastric cancer cells alter the immunosuppressive function of neutrophils. Oncol Rep (2020) 43(1):251–9. doi: 10.3892/or.2019.7410
40. Zhao Y, Rahmy S, Liu Z, Zhang C, Lu X. Rational targeting of immunosuppressive neutrophils in cancer. Pharmacol Ther (2020) 212:107556. doi: 10.1016/j.pharmthera.2020.107556
41. Du Y, Wei Y. Therapeutic potential of natural killer cells in gastric cancer. Front Immunol (2018) 9:3095. doi: 10.3389/fimmu.2018.03095
42. Li T, Zhang Q, Jiang Y, Yu J, Hu Y, Mou T, et al. Gastric cancer cells inhibit natural killer cell proliferation and induce apoptosis Via prostaglandin E2. Oncoimmunology (2016) 5(2):e1069936. doi: 10.1080/2162402X.2015.1069936
43. Xie M-Z, Tang Y-P, Hu B-L, Li K-Z, Li J-L, Liang X-Q. Percentage of natural killer (Nk) cells in peripheral blood is associated with prognosis in patients with gastric cancer: A retrospective study from a single center. Med Sci Monit (2021) 27:e927464. doi: 10.12659/MSM.927464
44. Zakiryanova GK, Kustova E, Urazalieva NT, Baimuchametov ET, Nakisbekov NN, Shurin MR. Abnormal expression of c-myc oncogene in nk cells in patients with cancer. Int J Mol Sci (2019) 20(3):756. doi: 10.3390/ijms20030756
45. Mimura K, Kamiya T, Shiraishi K, Kua L-F, Shabbir A, So J, et al. Therapeutic potential of highly cytotoxic natural killer cells for gastric cancer. Int J Cancer (2014) 135(6):1390–8. doi: 10.1002/ijc.28780
46. Xing R, Li L, Chen L, Gao Z, Wang H, Li W, et al. Copy number variations of hla-I and activation of Nkp30 pathway determine the sensitivity of gastric cancer cells to the cytotoxicity of natural killer cells. Oncogene (2016) 35(20):2584–91. doi: 10.1038/onc.2015.324
47. Guo Z, Zhou C, Zhou L, Wang Z, Zhu X, Mu X. Overexpression of Dapk1-mediated inhibition of Ikkβ/Csn5/Pd-L1 axis enhances natural killer cell killing ability and inhibits tumor immune evasion in gastric cancer. Cell Immunol (2022) 372:104469. doi: 10.1016/j.cellimm.2021.104469
48. Shiraishi K, Mimura K, Kua L-F, Koh V, Siang LK, Nakajima S, et al. Inhibition of mmp activity can restore Nkg2d ligand expression in gastric cancer, leading to improved nk cell susceptibility. J Gastroenterol (2016) 51(12):1101–11. doi: 10.1007/s00535-016-1197-x
49. Wang W, Jin J, Dai F, Long Z, Liu X, Cai H, et al. Interleukin-15 suppresses gastric cancer liver metastases by enhancing natural killer cell activity in a murine model. Oncol Lett (2018) 16(4):4839–46. doi: 10.3892/ol.2018.9303
50. Ascui G, Gálvez-Jirón F, Kramm K, Schäfer C, Siña J, Pola V, et al. Decreased invariant natural killer T-Cell-Mediated antitumor immune response in patients with gastric cancer. Immunol Cell Biol (2020) 98(6):500–13. doi: 10.1111/imcb.12331
51. Moreira AM, Pereira J, Melo S, Fernandes MS, Carneiro P, Seruca R, et al. The extracellular matrix: An accomplice in gastric cancer development and progression. Cells (2020) 9(2):394. doi: 10.3390/cells9020394
52. Mohan V, Das A, Sagi I. Emerging roles of ecm remodeling processes in cancer. Semin Cancer Biol (2020) 62:192–200. doi: 10.1016/j.semcancer.2019.09.004
53. Sung J-Y, Cheong J-H. The matrisome is associated with metabolic reprograming in stem-like phenotypes of gastric cancer. Cancers (Basel) (2022) 14(6):756. doi: 10.3390/cancers14061438
54. Moreira AM, Ferreira RM, Carneiro P, Figueiredo J, Osório H, Barbosa J, et al. Proteomic identification of a gastric tumor ecm signature associated with cancer progression. Front Mol Biosci (2022) 9:818552. doi: 10.3389/fmolb.2022.818552
55. Jang M, Koh I, Lee JE, Lim JY, Cheong J-H, Kim P. Increased extracellular matrix density disrupts e-Cadherin/β-Catenin complex in gastric cancer cells. Biomater Sci (2018) 6(10):2704–13. doi: 10.1039/c8bm00843d
56. Branco da Cunha C, Klumpers DD, Koshy ST, Weaver JC, Chaudhuri O, Seruca R, et al. Cd44 alternative splicing in gastric cancer cells is regulated by culture dimensionality and matrix stiffness. Biomaterials (2016) 98:152–62. doi: 10.1016/j.biomaterials.2016.04.016
57. Acerbi I, Cassereau L, Dean I, Shi Q, Au A, Park C, et al. Human breast cancer invasion and aggression correlates with ecm stiffening and immune cell infiltration. Integr Biol quantitative Biosci Nano To Macro (2015) 7(10):1120–34. doi: 10.1039/c5ib00040h
58. Kuczek DE, Larsen AMH, Thorseth M-L, Carretta M, Kalvisa A, Siersbæk MS, et al. Collagen density regulates the activity of tumor-infiltrating T cells. J Immunother Cancer (2019) 7(1):68. doi: 10.1186/s40425-019-0556-6
59. Zhang T, Li X, He Y, Wang Y, Shen J, Wang S, et al. Cancer-associated fibroblasts-derived Hapln1 promotes tumour invasion through extracellular matrix remodeling in gastric cancer. Gastric Cancer (2022) 25(2):346–59. doi: 10.1007/s10120-021-01259-5
60. Krzysiek-Maczka G, Targosz A, Wrobel T, Paw M, Szczyrk U, Opila J, et al. Time-extended exposure of gastric epithelial cells to secretome of -activated fibroblasts induces reprogramming of gastric epithelium towards pre-cancerogenic and pro-invasive phenotype. Am J Cancer Res (2022) 12(3):1337–71.
61. Zhang Q, Wang M, Huang F, Yang T, Cai J, Zhang X, et al. H. pylori infection-induced msc differentiation into cafs promotes epithelial-mesenchymal transition in gastric epithelial cells. Int J Mol Med (2013) 32(6):1465–73. doi: 10.3892/ijmm.2013.1532
62. Calvo F, Ege N, Grande-Garcia A, Hooper S, Jenkins RP, Chaudhry SI, et al. Mechanotransduction and yap-dependent matrix remodelling is required for the generation and maintenance of cancer-associated fibroblasts. Nat Cell Biol (2013) 15(6):637–46. doi: 10.1038/ncb2756
63. Mao X, Xu J, Wang W, Liang C, Hua J, Liu J, et al. Crosstalk between cancer-associated fibroblasts and immune cells in the tumor microenvironment: New findings and future perspectives. Mol Cancer (2021) 20(1):131. doi: 10.1186/s12943-021-01428-1
64. Butler JM, Kobayashi H, Rafii S. Instructive role of the vascular niche in promoting tumour growth and tissue repair by angiocrine factors. Nat Rev Cancer (2010) 10(2):138–46. doi: 10.1038/nrc2791
65. Li X, Sun Z, Peng G, Xiao Y, Guo J, Wu B, et al. Single-cell rna sequencing reveals a pro-invasive cancer-associated fibroblast subgroup associated with poor clinical outcomes in patients with gastric cancer. Theranostics (2022) 12(2):620–38. doi: 10.7150/thno.60540
66. Nagl L, Horvath L, Pircher A, Wolf D. Tumor endothelial cells (Tecs) as potential immune directors of the tumor microenvironment - new findings and future perspectives. Front Cell Dev Biol (2020) 8:766. doi: 10.3389/fcell.2020.00766
67. Hoft SG, Pherson MD, DiPaolo RJ. Discovering immune-mediated mechanisms of gastric carcinogenesis through single-cell rna sequencing. Front Immunol (2022) 13:902017. doi: 10.3389/fimmu.2022.902017
68. Georganaki M, van Hooren L, Dimberg A. Vascular targeting to increase the efficiency of immune checkpoint blockade in cancer. Front Immunol (2018) 9:3081. doi: 10.3389/fimmu.2018.03081
69. Motz GT, Santoro SP, Wang LP, Garrabrant T, Lastra RR, Hagemann IS, et al. Tumor endothelium fasl establishes a selective immune barrier promoting tolerance in tumors. Nat Med (2014) 20(6):607–15. doi: 10.1038/nm.3541
70. Ridge SM, Sullivan FJ, Glynn SA. Mesenchymal stem cells: Key players in cancer progression. Mol Cancer (2017) 16(1):31. doi: 10.1186/s12943-017-0597-8
71. Oya Y, Hayakawa Y, Koike K. Tumor microenvironment in gastric cancers. Cancer Sci (2020) 111(8):2696–707. doi: 10.1111/cas.14521
72. Smith JP, Cao H, Chen W, Mahmood K, Phillips T, Sutton L, et al. Gastrin vaccine alone and in combination with an immune checkpoint antibody inhibits growth and metastases of gastric cancer. Front Oncol (2021) 11:788875. doi: 10.3389/fonc.2021.788875
73. Zhao L, Liu Y, Zhang S, Wei L, Cheng H, Wang J, et al. Impacts and mechanisms of metabolic reprogramming of tumor microenvironment for immunotherapy in gastric cancer. Cell Death Dis (2022) 13(4):378. doi: 10.1038/s41419-022-04821-w
74. Xu Y, Lu J, Tang Y, Xie W, Zhang H, Wang B, et al. Pink1 deficiency in gastric cancer compromises mitophagy, promotes the warburg effect, and facilitates M2 polarization of macrophages. Cancer Lett (2022) 529:19–36. doi: 10.1016/j.canlet.2021.12.032
75. He Z, Chen D, Wu J, Sui C, Deng X, Zhang P, et al. Yes associated protein 1 promotes resistance to 5-fluorouracil in gastric cancer by regulating Glut3-dependent glycometabolism reprogramming of tumor-associated macrophages. Arch Biochem Biophys (2021) 702:108838. doi: 10.1016/j.abb.2021.108838
76. Yao X, He Z, Qin C, Deng X, Bai L, Li G, et al. Slc2a3 promotes macrophage infiltration by glycolysis reprogramming in gastric cancer. Cancer Cell Int (2020) 20:503. doi: 10.1186/s12935-020-01599-9
77. Zhang L, Li S. Lactic acid promotes macrophage polarization through mct-Hif1α signaling in gastric cancer. Exp Cell Res (2020) 388(2):111846. doi: 10.1016/j.yexcr.2020.111846
78. Zhihua Y, Yulin T, Yibo W, Wei D, Yin C, Jiahao X, et al. Hypoxia decreases macrophage glycolysis and M1 percentage by targeting microrna-30c and mtor in human gastric cancer. Cancer Sci (2019) 110(8):2368–77. doi: 10.1111/cas.14110
79. Zhou X, Fang D, Liu H, Ou X, Zhang C, Zhao Z, et al. Pmn-mdscs accumulation induced by Cxcl1 promotes Cd8 T cells exhaustion in gastric cancer. Cancer Lett (2022) 532:215598. doi: 10.1016/j.canlet.2022.215598
80. He W, Zhang H, Han F, Chen X, Lin R, Wang W, et al. Cd155t/Tigit signaling regulates Cd8 T-cell metabolism and promotes tumor progression in human gastric cancer. Cancer Res (2017) 77(22):6375–88. doi: 10.1158/0008-5472.CAN-17-0381
81. Han F, Guo S, Huang C, Cui L, Zhao Y, Ma J, et al. Gastric cancer mesenchymal stem cells inhibit natural killer cell function by up-regulating Fbp1. Cent Eur J Immunol (2021) 46(4):427–37. doi: 10.5114/ceji.2021.111753
82. Zhang JY, Zhao YL, Lv YP, Cheng P, Chen W, Duan M, et al. Modulation of Cd8(+) memory stem T cell activity and glycogen synthase kinase 3β inhibition enhances anti-tumoral immunity in gastric cancer. Oncoimmunology (2018) 7(4):e1412900. doi: 10.1080/2162402x.2017.1412900
83. Ippolito L, Morandi A, Giannoni E, Chiarugi P. Lactate: A metabolic driver in the tumour landscape. Trends Biochem Sci (2019) 44(2):153–66. doi: 10.1016/j.tibs.2018.10.011
84. Husain Z, Seth P, Sukhatme VP. Tumor-derived lactate and myeloid-derived suppressor cells: Linking metabolism to cancer immunology. Oncoimmunology (2013) 2(11):e26383. doi: 10.4161/onci.26383
85. Wang ZH, Peng WB, Zhang P, Yang XP, Zhou Q. Lactate in the tumour microenvironment: From immune modulation to therapy. EBioMedicine (2021) 73:103627. doi: 10.1016/j.ebiom.2021.103627
86. Luo Q, Zheng N, Jiang L, Wang T, Zhang P, Liu Y, et al. Lipid accumulation in macrophages confers protumorigenic polarization and immunity in gastric cancer. Cancer Sci (2020) 111(11):4000–11. doi: 10.1111/cas.14616
87. Lin R, Zhang H, Yuan Y, He Q, Zhou J, Li S, et al. Fatty acid oxidation controls Cd8 tissue-resident memory T-cell survival in gastric adenocarcinoma. Cancer Immunol Res (2020) 8(4):479–92. doi: 10.1158/2326-6066.CIR-19-0702
88. Shen Y, Li X-L, Li Y-X, Shan Z-G, Zhao Y-L, Cheng P, et al. Distribution, phenotype, functional and clinical relevance of Cd8cd103 tissue-resident memory T cells in human gastric cancer. Cancer Immunol Immunother (2022) 71(7):1645–54. doi: 10.1007/s00262-021-03105-0
89. Carr EL, Kelman A, Wu GS, Gopaul R, Senkevitch E, Aghvanyan A, et al. Glutamine uptake and metabolism are coordinately regulated by Erk/Mapk during T lymphocyte activation. J Immunol (2010) 185(2):1037–44. doi: 10.4049/jimmunol.0903586
90. Wu D, Wang Z. Gastric cancer cell-derived kynurenines hyperactive regulatory T cells to promote chemoresistance Via the signaling pathway. DNA Cell Biol (2022) 41(4):447–55. doi: 10.1089/dna.2021.0936
91. Young A, Ngiow SF, Gao Y, Patch A-M, Barkauskas DS, Messaoudene M, et al. A2ar adenosine signaling suppresses natural killer cell maturation in the tumor microenvironment. Cancer Res (2018) 78(4):1003–16. doi: 10.1158/0008-5472.CAN-17-2826
92. Shi L, Feng M, Du S, Wei X, Song H, Yixin X, et al. Adenosine generated by regulatory T cells induces Cd8 T cell exhaustion in gastric cancer through A2ar pathway. BioMed Res Int (2019) 2019:4093214. doi: 10.1155/2019/4093214
93. Sun L, Wang Q, Chen B, Zhao Y, Shen B, Wang X, et al. Human gastric cancer mesenchymal stem cell-derived Il15 contributes to tumor cell epithelial-mesenchymal transition Via upregulation tregs ratio and pd-1 expression in Cd4t cell. Stem Cells Dev (2018) 27(17):1203–14. doi: 10.1089/scd.2018.0043
94. Cao X-K, Xie B, Shao Y, Lin J. Cytokine-driven positive feedback loop organizes fibroblast transformation and facilitates gastric cancer progression. Clin Transl Oncol (2022) 24:1354–64. doi: 10.1007/s12094-022-02777-z
95. Ding X, Xi W, Ji J, Cai Q, Jiang J, Shi M, et al. Hgf derived from Cancer-Associated fibroblasts promotes vascularization in gastric cancer Via Pi3k/Akt and Erk1/2 signaling. Oncol Rep (2018) 40(2):1185–95. doi: 10.3892/or.2018.6500
96. Li X, Zhai J, Shen Y, Zhang T, Wang Y, He Y, et al. Tumor-derived il-8 facilitates lymph node metastasis of gastric cancer Via pd-1 up-regulation in Cd8 T cells. Cancer Immunol Immunother (2022). doi: 10.1007/s00262-022-03223-3
97. Shen Y, Teng Y, Lv Y, Zhao Y, Qiu Y, Chen W, et al. Pd-1 does not mark tumor-infiltrating Cd8+ T cell dysfunction in human gastric cancer. J Immunother Cancer (2020) 8(2):e000422. doi: 10.1136/jitc-2019-000422
98. Gambardella V, Castillo J, Tarazona N, Gimeno-Valiente F, Martínez-Ciarpaglini C, Cabeza-Segura M, et al. The role of tumor-associated macrophages in gastric cancer development and their potential as a therapeutic target. Cancer Treat Rev (2020) 86:102015. doi: 10.1016/j.ctrv.2020.102015
99. Peng L-S, Zhang J-Y, Teng Y-S, Zhao Y-L, Wang T-T, Mao F-Y, et al. Tumor-associated Monocytes/Macrophages impair nk-cell function Via Tgfβ1 in human gastric cancer. Cancer Immunol Res (2017) 5(3):248–56. doi: 10.1158/2326-6066.CIR-16-0152
100. Chen Y, Zhang S, Wang Q, Zhang X. Tumor-recruited M2 macrophages promote gastric and breast cancer metastasis Via M2 macrophage-secreted Chi3l1 protein. J Hematol Oncol (2017) 10(1):36. doi: 10.1186/s13045-017-0408-0
101. Ju X, Zhang H, Zhou Z, Chen M, Wang Q. Tumor-associated macrophages induce pd-L1 expression in gastric cancer cells through il-6 and tnf-α signaling. Exp Cell Res (2020) 396(2):112315. doi: 10.1016/j.yexcr.2020.112315
102. Hu J, Ma Y, Ma J, Chen S, Zhang X, Guo S, et al. Macrophage-derived sparc attenuates M2-mediated pro-tumour phenotypes. J Cancer (2020) 11(10):2981–92. doi: 10.7150/jca.39651
103. Lin C, He H, Liu H, Li R, Chen Y, Qi Y, et al. Tumour-associated macrophages-derived Cxcl8 determines immune evasion through autonomous pd-L1 expression in gastric cancer. Gut (2019) 68(10):1764–73. doi: 10.1136/gutjnl-2018-316324
104. Zhao G, Liu L, Peek RM, Hao X, Polk DB, Li H, et al. Activation of epidermal growth factor receptor in macrophages mediates feedback inhibition of M2 polarization and gastrointestinal tumor cell growth. J Biol Chem (2016) 291(39):20462–72. doi: 10.1074/jbc.M116.750182
105. Zhang J, Ji C, Li W, Mao Z, Shi Y, Shi H, et al. Tumor-educated neutrophils activate mesenchymal stem cells to promote gastric cancer growth and metastasis. Front Cell Dev Biol (2020) 8:788. doi: 10.3389/fcell.2020.00788
106. Li S, Cong X, Gao H, Lan X, Li Z, Wang W, et al. Tumor-associated neutrophils induce emt by il-17a to promote migration and invasion in gastric cancer cells. J Exp Clin Cancer Res (2019) 38(1):6. doi: 10.1186/s13046-018-1003-0
107. Yang B, Su K, Sha G, Bai Q, Sun G, Chen H, et al. Linc00665 interacts with Bach1 to activate Wnt1 and mediates the M2 polarization of tumor-associated macrophages in gc. Mol Immunol (2022) 146:1–8. doi: 10.1016/j.molimm.2022.03.120
108. Xin L, Zhou L-Q, Liu C, Zeng F, Yuan Y-W, Zhou Q, et al. Transfer of lncrna crnde in Tam-derived exosomes is linked with cisplatin resistance in gastric cancer. EMBO Rep (2021) 22(12):e52124. doi: 10.15252/embr.202052124
109. Cui H-Y, Rong J-S, Chen J, Guo J, Zhu J-Q, Ruan M, et al. Exosomal microrna-588 from M2 polarized macrophages contributes to cisplatin resistance of gastric cancer cells. World J Gastroenterol (2021) 27(36):6079–92. doi: 10.3748/wjg.v27.i36.6079
110. Zheng P, Chen L, Yuan X, Luo Q, Liu Y, Xie G, et al. Exosomal transfer of tumor-associated macrophage-derived mir-21 confers cisplatin resistance in gastric cancer cells. J Exp Clin Cancer Res (2017) 36(1):53. doi: 10.1186/s13046-017-0528-y
111. Gao H, Ma J, Cheng Y, Zheng P. Exosomal transfer of macrophage-derived mir-223 confers doxorubicin resistance in gastric cancer. Onco Targets Ther (2020) 13:12169–79. doi: 10.2147/OTT.S283542
112. Yang X, Cai S, Shu Y, Deng X, Zhang Y, He N, et al. Exosomal mir-487a derived from M2 macrophage promotes the progression of gastric cancer. Cell Cycle (2021) 20(4):434–44. doi: 10.1080/15384101.2021.1878326
113. Zhang Y, Meng W, Yue P, Li X. M2 macrophage-derived extracellular vesicles promote gastric cancer progression Via a microrna-130b-3p/Mll3/Grhl2 signaling cascade. J Exp Clin Cancer Res (2020) 39(1):134. doi: 10.1186/s13046-020-01626-7
114. Cao Y, Tu Y, Xiong J, Tan S, Luo L, Wu A, et al. Microrna-15b-5p encapsulated by M2 macrophage-derived extracellular vesicles promotes gastric cancer metastasis by targeting Brms1 and suppressing Dapk1 transcription. J Exp Clin Cancer Res (2022) 41(1):152. doi: 10.1186/s13046-022-02356-8
115. Li Z, Suo B, Long G, Gao Y, Song J, Zhang M, et al. Exosomal mirna-16-5p derived from M1 macrophages enhances T cell-dependent immune response by regulating pd-L1 in gastric cancer. Front Cell Dev Biol (2020) 8:572689. doi: 10.3389/fcell.2020.572689
116. Ou J, Lei P, Yang Z, Yang M, Luo L, Mo H, et al. Linc00152 mediates Cd8 T-cell infiltration in gastric cancer through binding to Ezh2 and regulating the Cxcl9, 10/Cxcr3 axis. J Mol Histol (2021) 52(3):611–20. doi: 10.1007/s10735-021-09967-z
117. Wang F, Li B, Wei Y, Zhao Y, Wang L, Zhang P, et al. Tumor-derived exosomes induce Pd1 macrophage population in human gastric cancer that promotes disease progression. Oncogenesis (2018) 7(5):41. doi: 10.1038/s41389-018-0049-3
118. Li T, Li B, Sara A, Ay C, Leung WY, Zhang Y, et al. Docking protein-1 promotes inflammatory macrophage signaling in gastric cancer. Oncoimmunology (2019) 8(11):e1649961. doi: 10.1080/2162402X.2019.1649961
119. Xin L, Wu Y, Liu C, Zeng F, Wang J-L, Wu D-Z, et al. Exosome-mediated transfer of lncrna Hcg18 promotes M2 macrophage polarization in gastric cancer. Mol Immunol (2021) 140:196–205. doi: 10.1016/j.molimm.2021.10.011
120. Xie C, Guo Y, Lou S. Lncrna ancr promotes invasion and migration of gastric cancer by regulating Foxo1 expression to inhibit macrophage M1 polarization. Dig Dis Sci (2020) 65(10):2863–72. doi: 10.1007/s10620-019-06019-1
121. Fang W, Shi C, Wang Y, Song J, Zhang L. Microrna-128-3p inhibits Cd4+ regulatory T cells enrichment by targeting interleukin 16 in gastric cancer. Bioengineered (2022) 13(1):1025–38. doi: 10.1080/21655979.2021.2017566
122. Miliotis C, Slack FJ. Mir-105-5p regulates pd-L1 expression and tumor immunogenicity in gastric cancer. Cancer Lett (2021) 518:115–26. doi: 10.1016/j.canlet.2021.05.037
123. Li J, Sun L, Chen Y, Zhu J, Shen J, Wang J, et al. Gastric cancer-derived exosomal mir-135b-5p impairs the function of Vγ9vδ2 T cells by targeting specificity protein 1. Cancer Immunol Immunother (2022) 71(2):311–25. doi: 10.1007/s00262-021-02991-8
124. Liu F, Bu Z, Zhao F, Xiao D. Increased T-helper 17 cell differentiation mediated by exosome-mediated microrna-451 redistribution in gastric cancer infiltrated T cells. Cancer Sci (2018) 109(1):65–73. doi: 10.1111/cas.13429
125. Zhang M, Fan Y, Che X, Hou K, Zhang C, Li C, et al. 5-Fu-Induced upregulation of exosomal pd-L1 causes immunosuppression in advanced gastric cancer patients. Front Oncol (2020) 10:492. doi: 10.3389/fonc.2020.00492
126. Liang Y, Liu Y, Zhang Q, Zhang H, Du J. Tumor-derived extracellular vesicles containing microrna-1290 promote immune escape of cancer cells through the Grhl2/Zeb1/Pd-L1 axis in gastric cancer. Transl Res (2021) 231:102–12. doi: 10.1016/j.trsl.2020.12.003
127. Shen D-D, Pang J-R, Bi Y-P, Zhao L-F, Li Y-R, Zhao L-J, et al. Lsd1 deletion decreases exosomal pd-L1 and restores T-cell response in gastric cancer. Mol Cancer (2022) 21(1):75. doi: 10.1186/s12943-022-01557-1
128. Zhang X, Shi H, Yuan X, Jiang P, Qian H, Xu W. Tumor-derived exosomes induce N2 polarization of neutrophils to promote gastric cancer cell migration. Mol Cancer (2018) 17(1):146. doi: 10.1186/s12943-018-0898-6
129. Shi Y, Zhang J, Mao Z, Jiang H, Liu W, Shi H, et al. Extracellular vesicles from gastric cancer cells induce pd-L1 expression on neutrophils to suppress T-cell immunity. Front Oncol (2020) 10:629. doi: 10.3389/fonc.2020.00629
130. Zhang Q, Liu G, Liu J, Yang M, Fu J, Liu G, et al. The antitumor capacity of mesothelin-Car-T cells in targeting solid tumors in mice. Mol Ther Oncolytics (2021) 20:556–68. doi: 10.1016/j.omto.2021.02.013
131. Chen C, Gu Y-M, Zhang F, Zhang Z-C, Zhang Y-T, He Y-D, et al. Construction of Pd1/Cd28 chimeric-switch receptor enhances anti-tumor ability of c-met car-T in gastric cancer. Oncoimmunology (2021) 10(1):1901434. doi: 10.1080/2162402X.2021.1901434
132. Jung M, Yang Y, McCloskey JE, Zaman M, Vedvyas Y, Zhang X, et al. Chimeric antigen receptor T cell therapy targeting icam-1 in gastric cancer. Mol Ther Oncolytics (2020) 18:587–601. doi: 10.1016/j.omto.2020.08.009
133. Han Y, Sun B, Cai H, Xuan Y. Simultaneously target of normal and stem cells-like gastric cancer cells Via cisplatin and anti-Cd133 car-T combination therapy. Cancer Immunol Immunother (2021) 70(10):2795–803. doi: 10.1007/s00262-021-02891-x
134. Feng Z, He X, Zhang X, Wu Y, Xing B, Knowles A, et al. Potent suppression of neuroendocrine tumors and gastrointestinal cancers by Cdh17car T cells without toxicity to normal tissues. Nat Cancer (2022) 3(5):581–94. doi: 10.1038/s43018-022-00344-7
135. Osterweil NGastric cancer car T-cell target antigen id'd. Cancer Discovery (2021) 11(12):2954. doi: 10.1158/2159-8290.CD-NB2021-0390
136. Xu B, Chen F, Zhang X, Wang Z, Che K, Wu N, et al. Antigen-specific T cell immunotherapy targeting Claudin18.2 in gastric cancer. Cancers (Basel) (2022) 14(11):2758. doi: 10.3390/cancers14112758
137. Zhao W, Jia L, Zhang M, Huang X, Qian P, Tang Q, et al. The killing effect of novel bi-specific Trop2/Pd-L1 car-T cell targeted gastric cancer. Am J Cancer Res (2019) 9(8):1846–56.
138. Ishikawa T, Okayama T, Sakamoto N, Ideno M, Oka K, Enoki T, et al. Phase I clinical trial of adoptive transfer of expanded natural killer cells in combination with Igg1 antibody in patients with gastric or colorectal cancer. Int J Cancer (2018) 142(12):2599–609. doi: 10.1002/ijc.31285
139. Li M, Zhi L, Yin M, Guo C, Zhang H, Lu C, et al. A novel bispecific chimeric Pd1-Dap10/Nkg2d receptor augments Nk92-cell therapy efficacy for human gastric cancer sgc-7901 cell. Biochem Biophys Res Commun (2020) 523(3):745–52. doi: 10.1016/j.bbrc.2020.01.005
140. Cao B, Liu M, Huang J, Zhou J, Li J, Lian H, et al. Development of mesothelin-specific car nk-92 cells for the treatment of gastric cancer. Int J Biol Sci (2021) 17(14):3850–61. doi: 10.7150/ijbs.64630
141. Nakamura Y, Kinoshita J, Yamaguchi T, Aoki T, Saito H, Hamabe-Horiike T, et al. Crosstalk between cancer-associated fibroblasts and immune cells in peritoneal metastasis: Inhibition in the migration of M2 macrophages and mast cells by tranilast. Gastric Cancer (2022) 25(3):515–26. doi: 10.1007/s10120-021-01275-5
142. Sootome H, Fujita H, Ito K, Ochiiwa H, Fujioka Y, Ito K, et al. Futibatinib is a novel irreversible fgfr 1-4 inhibitor that shows selective antitumor activity against fgfr-deregulated tumors. Cancer Res (2020) 80(22):4986–97. doi: 10.1158/0008-5472.CAN-19-2568
143. Chen G, Yu C, Tang Z, Liu S, An F, Zhu J, et al. Metformin suppresses gastric cancer progression through Calmodulin-Like protein 3 secreted from Tumor-Associated fibroblasts. Oncol Rep (2019) 41(1):405–14. doi: 10.3892/or.2018.6783
144. Guan X-W, Zhao F, Wang J-Y, Wang H-Y, Ge S-H, Wang X, et al. Tumor microenvironment interruption: A novel anti-cancer mechanism of proton-pump inhibitor in gastric cancer by suppressing the release of microrna-carrying exosomes. Am J Cancer Res (2017) 7(9):1913–25.
145. Hara M, Nagasaki T, Shiga K, Takeyama H. Suppression of cancer-associated fibroblasts and endothelial cells by itraconazole in bevacizumab-resistant gastrointestinal cancer. Anticancer Res (2016) 36(1):169–77.
146. Wang X, Jiao X, Meng Y, Chen H, Griffin N, Gao X, et al. Methionine enkephalin (Menk) inhibits human gastric cancer through regulating tumor associated macrophages (Tams) and Pi3k/Akt/Mtor signaling pathway inside cancer cells. Int Immunopharmacol (2018) 65:312–22. doi: 10.1016/j.intimp.2018.10.023
147. Sharma P, Allison JP. Immune checkpoint targeting in cancer therapy: Toward combination strategies with curative potential. Cell (2015) 161(2):205–14. doi: 10.1016/j.cell.2015.03.030
148. Ji L, Qian W, Gui L, Ji Z, Yin P, Lin GN, et al. Blockade of β-Catenin-Induced Ccl28 suppresses gastric cancer progression Via inhibition of treg cell infiltration. Cancer Res (2020) 80(10):2004–16. doi: 10.1158/0008-5472.CAN-19-3074
149. He L, Zhong Z, Chen M, Liang Q, Wang Y, Tan W. Current advances in coptidis rhizoma for gastrointestinal and other cancers. Front Pharmacol (2021) 12:775084. doi: 10.3389/fphar.2021.775084
150. Wang Z-F, Ma D-G, Zhu Z, Mu Y-P, Yang Y-Y, Feng L, et al. Astragaloside iv inhibits pathological functions of gastric cancer-associated fibroblasts. World J Gastroenterol (2017) 23(48):8512–25. doi: 10.3748/wjg.v23.i48.8512
151. Wang Z, Ma D, Wang C, Zhu Z, Yang Y, Zeng F, et al. Triptonide inhibits the pathological functions of gastric cancer-associated fibroblasts. BioMed Pharmacother (2017) 96:757–67. doi: 10.1016/j.biopha.2017.10.046
152. Zhuang H, Dai X, Zhang X, Mao Z, Huang H. Sophoridine suppresses macrophage-mediated immunosuppression through Tlr4/Irf3 pathway and subsequently upregulates Cd8 T cytotoxic function against gastric cancer. BioMed Pharmacother (2020) 121:109636. doi: 10.1016/j.biopha.2019.109636
153. Xu Q-F, Peng H-P, Lu X-R, Hu Y, Xu Z-H, Xu J-K. Oleanolic acid regulates the Treg/Th17 imbalance in gastric cancer by targeting il-6 with mir-98-5p. Cytokine (2021) 148:155656. doi: 10.1016/j.cyto.2021.155656
154. Lu X, Li Y, Yang W, Tao M, Dai Y, Xu J, et al. Inhibition of nf-κb is required for oleanolic acid to downregulate pd-L1 by promoting DNA demethylation in gastric cancer cells. J Biochem Mol Toxicol (2021) 35(1):e22621. doi: 10.1002/jbt.22621
155. Jung B-K, Ko HY, Kang H, Hong J, Ahn HM, Na Y, et al. Relaxin-expressing oncolytic adenovirus induces remodeling of physical and immunological aspects of cold tumor to potentiate pd-1 blockade. J Immunother Cancer (2020) 8(2):e000763. doi: 10.1136/jitc-2020-000763
156. Pang T, Wang X, Gao J, Chen W, Shen XJ, Nie MM, et al. Fiber-modified hexon-chimeric oncolytic adenovirus targeting cancer associated fibroblasts inhibits tumor growth in gastric carcinoma. Oncotarget (2017) 8(44):76468–78. doi: 10.18632/oncotarget.20273
157. Sugawara K, Iwai M, Yajima S, Tanaka M, Yanagihara K, Seto Y, et al. Efficacy of a third-generation oncolytic herpes virus G47δ in advanced stage models of human gastric cancer. Mol Ther Oncolytics (2020) 17:205–15. doi: 10.1016/j.omto.2020.03.022
158. Yang Y, Yang Y, Chen M, Chen J, Wang J, Ma Y, et al. Injectable shear-thinning polylysine hydrogels for localized immunotherapy of gastric cancer through repolarization of tumor-associated macrophages. Biomater Sci (2021) 9(19):6597–608. doi: 10.1039/d1bm01053k
159. Hu B-S, Tang T, Jia J-L, Xie B-C, Wu T-L, Sheng Y-Y, et al. Cd137 agonist induces gastric cancer cell apoptosis by enhancing the functions of Cd8 T cells Via nf-κb signaling. Cancer Cell Int (2020) 20:513. doi: 10.1186/s12935-020-01605-0
160. Zhou S, Meng F, Du S, Qian H, Ding N, Sha H, et al. Bifunctional irgd-Anti-Cd3 enhances antitumor potency of T cells by facilitating tumor infiltration and T-cell activation. J Immunother Cancer (2021) 9(5):e001925. doi: 10.1136/jitc-2020-001925
161. Zhu M, Wang H, Zhou S, Wei J, Ding N, Shao J, et al. Combination therapy with irgd-Anticd3 and pd-1 blockade enhances antitumor potency of cord blood-derived T cells. Onco Targets Ther (2021) 14:835–44. doi: 10.2147/OTT.S291086
162. Zhao R, Cui Y, Zheng Y, Li S, Lv J, Wu Q, et al. Human hyaluronidase Ph20 potentiates the antitumor activities of mesothelin-specific car-T cells against gastric cancer. Front Immunol (2021) 12:660488. doi: 10.3389/fimmu.2021.660488
163. Lv J, Zhao R, Wu D, Zheng D, Wu Z, Shi J, et al. Mesothelin is a target of chimeric antigen receptor T cells for treating gastric cancer. J Hematol Oncol (2019) 12(1):18. doi: 10.1186/s13045-019-0704-y
164. Chen J, Pan Z, Han L, Zhou Y, Zong H, Wang L, et al. A novel bispecific antibody targeting Cd3 and Lewis y with potent therapeutic efficacy against gastric cancer. Biomedicines (2021) 9(8):1059. doi: 10.3390/biomedicines9081059
165. Liu Q, Zhang D, Qian H, Chu Y, Yang Y, Shao J, et al. Superior antitumor efficacy of ifn-α2b-Incorporated photo-Cross-Linked hydrogels combined with T cell transfer and low-dose irradiation against gastric cancer. Int J Nanomed (2020) 15:3669–80. doi: 10.2147/IJN.S249174
166. Hu N, Li W, Hong Y, Zeng Z, Zhang J, Wu X, et al. A Pd1 targeted nano-delivery system based on epigenetic alterations of T cell responses in the treatment of gastric cancer. Mol Ther Oncolytics (2022) 24:148–59. doi: 10.1016/j.omto.2021.12.006
167. Zhu B, Sun Y, Wei X, Zhou H, Cao J, Li C, et al. Dendritic cell vaccine loaded with mg-7 antigen induces cytotoxic T lymphocyte responses against gastric cancer. J Healthc Eng (2022) 2022:1964081. doi: 10.1155/2022/1964081
168. Kohnepoushi C, Nejati V, Delirezh N, Biparva P. Poly lactic-Co-Glycolic acid nanoparticles containing human gastric tumor lysates as antigen delivery vehicles for dendritic cell-based antitumor immunotherapy. Immunol investigations (2019) 48(8):794–808. doi: 10.1080/08820139.2019.1610889
169. Xue G, Cheng Y, Ran F, Li X, Huang T, Yang Y, et al. Slc gene-modified dendritic cells mediate T cell-dependent anti-gastric cancer immune responses in vitro. Oncol Rep (2013) 29(2):595–604. doi: 10.3892/or.2012.2154
170. Lu W-W, Zhang H, Li Y-M, Ji F. Gastric cancer-derived heat shock protein-Gp96 peptide complex enhances dendritic cell activation. World J Gastroenterol (2017) 23(24):4390–8. doi: 10.3748/wjg.v23.i24.4390
171. Chen Y, Chen B, Yang T, Xiao W, Qian L, Ding Y, et al. Human fused Nkg2d-Il-15 protein controls xenografted human gastric cancer through the recruitment and activation of nk cells. Cell Mol Immunol (2017) 14(3):293–307. doi: 10.1038/cmi.2015.81
172. Zhong Z, Vong CT, Chen F, Tan H, Zhang C, Wang N, et al. Immunomodulatory potential of natural products from herbal medicines as immune checkpoints inhibitors: Helping to fight against cancer Via multiple targets. Med Res Rev (2022) 42(3):1246–79. doi: 10.1002/med.21876
173. Tan W, Pan T, Wang S, Li P, Men Y, Tan R, et al. Immunometabolism modulation, a new trick of edible and medicinal plants in cancer treatment. Food Chem (2021) 376:131860. doi: 10.1016/j.foodchem.2021.131860
174. Guo W, Cao P, Wang X, Hu M, Feng Y. Medicinal plants for the treatment of gastrointestinal cancers from the metabolomics perspective. Front Pharmacol (2022) 13:909755. doi: 10.3389/fphar.2022.909755
175. Wang Z-F, Ma D-G, Wang L, Feng L, Fu J-W, Li Y, et al. Paeoniflorin inhibits migration- and invasion-promoting capacities of gastric cancer associated fibroblasts. Chin J Integr Med (2019) 25(11):837–44. doi: 10.1007/s11655-018-2985-3
176. Yi M, Zhang J, Li A, Niu M, Yan Y, Jiao Y, et al. The construction, expression, and enhanced anti-tumor activity of Ym101: A bispecific antibody simultaneously targeting tgf-β and pd-L1. J Hematol Oncol (2021) 14(1):27. doi: 10.1186/s13045-021-01045-x
177. Yi M, Niu M, Zhang J, Li S, Zhu S, Yan Y, et al. Combine and conquer: Manganese synergizing anti-Tgf-β/Pd-L1 bispecific antibody Ym101 to overcome immunotherapy resistance in non-inflamed cancers. J Hematol Oncol (2021) 14(1):146. doi: 10.1186/s13045-021-01155-6
Keywords: immune tolerance, tumor microenvironment, gastric cancer, immunotherapy, tumor-infiltrating immune cells
Citation: Liu Y, Li C, Lu Y, Liu C and Yang W (2022) Tumor microenvironment-mediated immune tolerance in development and treatment of gastric cancer. Front. Immunol. 13:1016817. doi: 10.3389/fimmu.2022.1016817
Received: 11 August 2022; Accepted: 07 October 2022;
Published: 20 October 2022.
Edited by:
Jinyan Wang, China Medical University, ChinaReviewed by:
Zhangfeng Zhong, University of Macau, ChinaTie Fu Liu, Fudan University, China
Ming Yi, Zhejiang University, China
Copyright © 2022 Liu, Li, Lu, Liu and Yang. This is an open-access article distributed under the terms of the Creative Commons Attribution License (CC BY). The use, distribution or reproduction in other forums is permitted, provided the original author(s) and the copyright owner(s) are credited and that the original publication in this journal is cited, in accordance with accepted academic practice. No use, distribution or reproduction is permitted which does not comply with these terms.
*Correspondence: Changfeng Li, cfli@jlu.edu.cn; Wei Yang, ywei@jlu.edu.cn