- 1Shandong Provincial Key Laboratory for Rheumatic Disease and Translational Medicine, The First Affiliated Hospital of Shandong First Medical University & Shandong Provincial Qianfoshan Hospital, Jinan, China
- 2School of Clinical and Basic Medical Sciences, Shandong First Medical University & Shandong Academy of Medical Sciences, Jinan, China
- 3Department of Gastroenterology, The First Affiliated Hospital of Shandong First Medical University & Shandong Provincial Qianfoshan Hospital, Jinan, China
Inflammatory bowel disease (IBD), mainly including ulcerative colitis (UC) and Crohn’s disease (CD), is an autoimmune gastrointestinal disease characterized by chronic inflammation and frequent recurrence. Accumulating evidence has confirmed that chronic psychological stress is considered to trigger IBD deterioration and relapse. Moreover, studies have demonstrated that patients with IBD have a higher risk of developing symptoms of anxiety and depression than healthy individuals. However, the underlying mechanism of the link between psychological stress and IBD remains poorly understood. This review used a psychoneuroimmunology perspective to assess possible neuro-visceral integration, immune modulation, and crucial intestinal microbiome changes in IBD. Furthermore, the bidirectionality of the brain–gut axis was emphasized in the context, indicating that IBD pathophysiology increases the inflammatory response in the central nervous system and further contributes to anxiety- and depression-like behavioral comorbidities. This information will help accurately characterize the link between psychological stress and IBD disease activity. Additionally, the clinical application of functional brain imaging, microbiota-targeted treatment, psychotherapy and antidepressants should be considered during the treatment and diagnosis of IBD with behavioral comorbidities. This review elucidates the significance of more high-quality research combined with large clinical sample sizes and multiple diagnostic methods and psychotherapy, which may help to achieve personalized therapeutic strategies for IBD patients based on stress relief.
Introduction
Inflammatory bowel disease (IBD), mainly including ulcerative colitis (UC) and Crohn’s disease (CD), is an autoimmune gastrointestinal disease (1). This disease is a common condition affecting approximately 1.5 million people in the United States and 2.2 million people in Europe, with an increasing global incidence (2). Although the exact etiology of IBD remains elusive, the complex interaction between genetic factors, environmental factors, host immune regulation, intestinal microbes and microbial metabolites plays a vital role in the pathogenesis of IBD (3–6). The course of IBD is long and variable, often alternating between periods of quiescent disease and periods of relapsing disease with more active inflammatory episodes (7). Given that IBD is prone to relapse and difficult to treat, it can significantly impact patients’ quality of life (QoL) (8, 9).
In recent years, accumulating evidence has shown that the interaction between brain and gut is closely related to the occurrence and development of gastrointestinal (GI) diseases such as IBD and irritable bowel syndrome (IBS) (10). From this, the concept of brain–gut axis was proposed, which refers to the complex bidirectional communication network between the central nervous system and the intestine (11, 12). This axis enables the cross-talk between the nervous system (including the central nervous system, autonomic nervous system, and enteric nervous system), the endocrine system and the immune system (13–15). A dysregulation of this axis is arguably involved in the pathophysiology of IBD which has long been associated with mental conditions, such as stress, anxiety, and depression. It has been reported that psychological stress is involved in the permeability, motility, sensitivity, and secretion of the intestine, composition of gut microbes, and the promotion of the development and reactivation of intestinal inflammation in animal models of colitis (16–19). Furthermore, in some clinical studies, stress, anxiety, and depression have been considered triggers of IBD relapse and clinical deterioration (20, 21). IBD patients are at higher risk of depression than healthy individuals (22–24). Additionally, a recent meta-analysis has demonstrated that patients with active disease were more prone to experience symptoms of psychological disorders than those with inactive disease (22). Although the bidirectional effects of the brain–gut axis might help explain these observations (25, 26), the complex mechanisms underlying the interaction between psychological stress and the pathophysiology of IBD have not been fully understood.
A recent study suggested that stress resulting from the spread of the new coronavirus disease 2019 (COVID-19) has a strikingly positive relationship with CD activity (27). Therefore, in the context of the ongoing epidemic and spread of COVID-19, to elucidate the interplay between mental states and IBD evolution and better understand the bi-directional modulation through the brain–gut axis, it is essential to explore the exact mechanism of the link between mental health and IBD evolution and provide leads for therapeutic interventions. In this review, we used a psychoneuroimmunology perspective to discuss the mechanism by which chronic psychological stress impacts neuroendocrine immune regulation, damages the intestinal immune function and microbiota homeostasis, and subsequently aggravates IBD progression (Figure 1). Moreover, we found that disordered gut homeostasis in IBD was responsible for driving the brain pathology, exacerbating inflammatory response in the CNS, and contributing to anxiety- and depression-like behavior (Figure 2). Additionally, the application of neuroimaging studies in evaluating possible neuromechanisms involved in IBD and adjunctive effects of some promising microbiota-targeted treatment, psychological therapies and antidepressants are discussed.
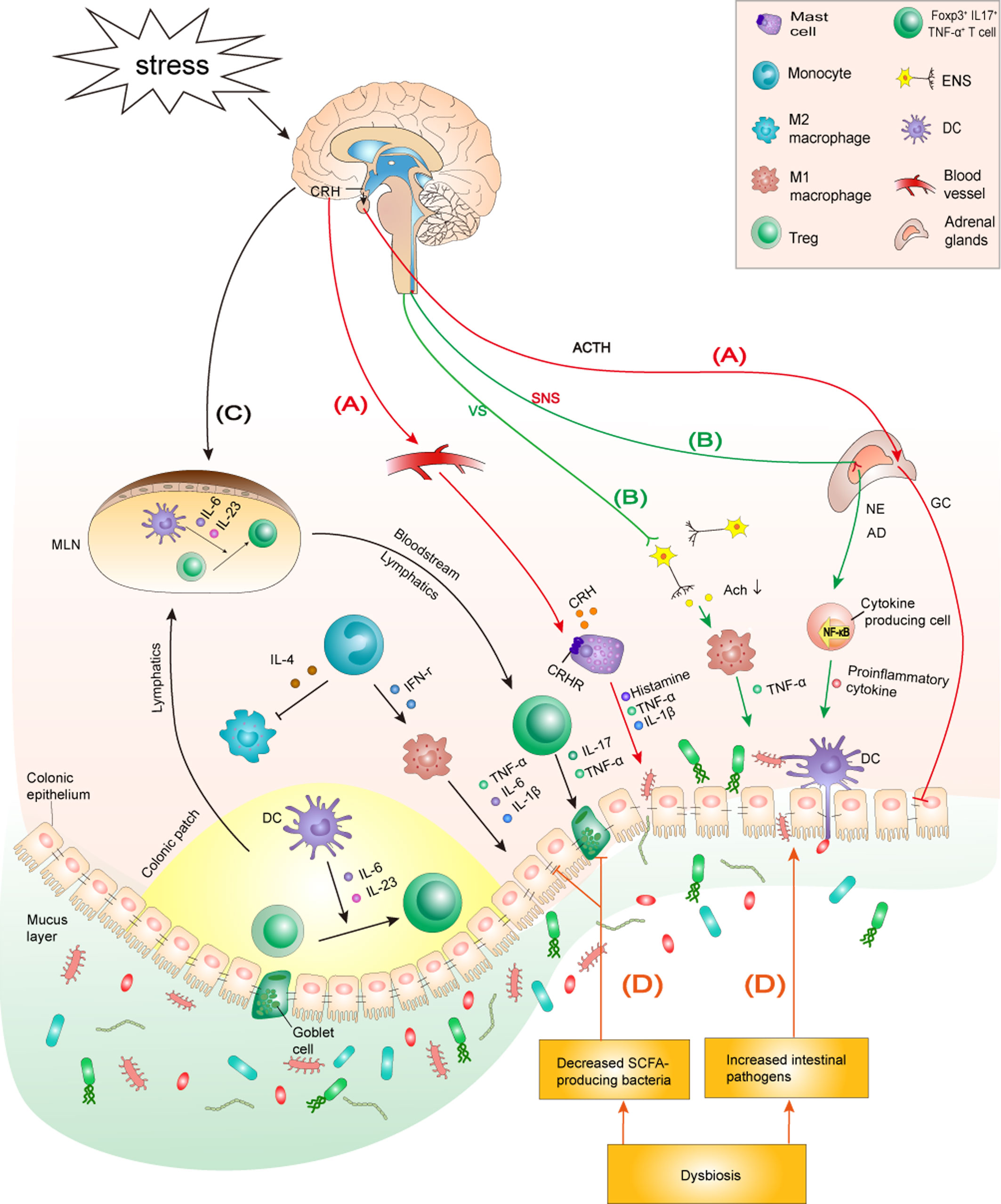
Figure 1 The mechanism by which chronic psychological stress aggravates IBD. (A) Stress activates the HPA axis: Stress induces the hypothalamus to release CRH, which triggers the release of ACTH from the hypophysis. ACTH subsequently initiates GC over-synthesis and release in the adrenal cortex. CRH reaches the colon through the blood and binds to CRHRs on the surface of MCs to promote degranulation and the production of proinflammatory cytokines IL-1β and TNF-α, which, in turn, increase the permeability of the colonic epithelial barrier. Furthermore, the overproduction of GC reduces tight junction protein expression between colonocytes, impairing the intestinal barrier function. (B) Stress activates the SNS but inhibits the vagus nerve: The sympathetic nervous system is stimulated by stress, which causes the adrenal medulla to secrete excessive AD and NE, promoting the activation of NF-κB signaling and mediating higher secretion of inflammatory cytokines in peripheral tissues. The inhibition of the anti-inflammatory effect of the vagus nerve promotes macrophage-induced TNF-α production, which aggravates the progress of colitis. (C) Stress is involved in innate and adaptive immune dysfunction by regulating specific immune cells and cytokine production: Stress induces macrophages to infiltrate the colon and polarize into an M1 phenotype. Additionally, stress promotes intestinal DCs to secrete IL-6 and IL-23, which induces Tregs to differentiate into a Foxp3+IL17+TNF-α+T cell phenotype. M1 macrophages and Foxp3+IL17+TNF-α+T cells secrete corresponding inflammatory cytokines to aggravate the colonic inflammatory response. (D) Stress induces microbiome community dysbiosis: Stress causes dysbiosis by increasing intestinal pathogens and decreasing SCFA-producing bacteria in the intestine. Intestinal pathogens can cause and exacerbate colitis by impairing the intestinal barrier and activating intestinal immunity. Decreased SCFAs lead to reduced tight junction protein expression and goblet cell numbers, which in turn lead to impaired intestinal barrier function and aggravated colonic inflammation. HPA axis, hypothalamic-pituitary-adrenal axis; CRH, corticotropin-releasing hormone; ACTH, adrenocorticotropic hormone; GC, glucocorticoids; CRHRs, CRH receptors; ANS, autonomic nervous system; SNS, sympathetic nervous system; AD, adrenaline; NE, noradrenaline; VS, vagus nerve; MCs, mast cells; SCFAs: short-chain fatty acids; MLN, mesenteric lymph node; ENS, enteric nervous system.
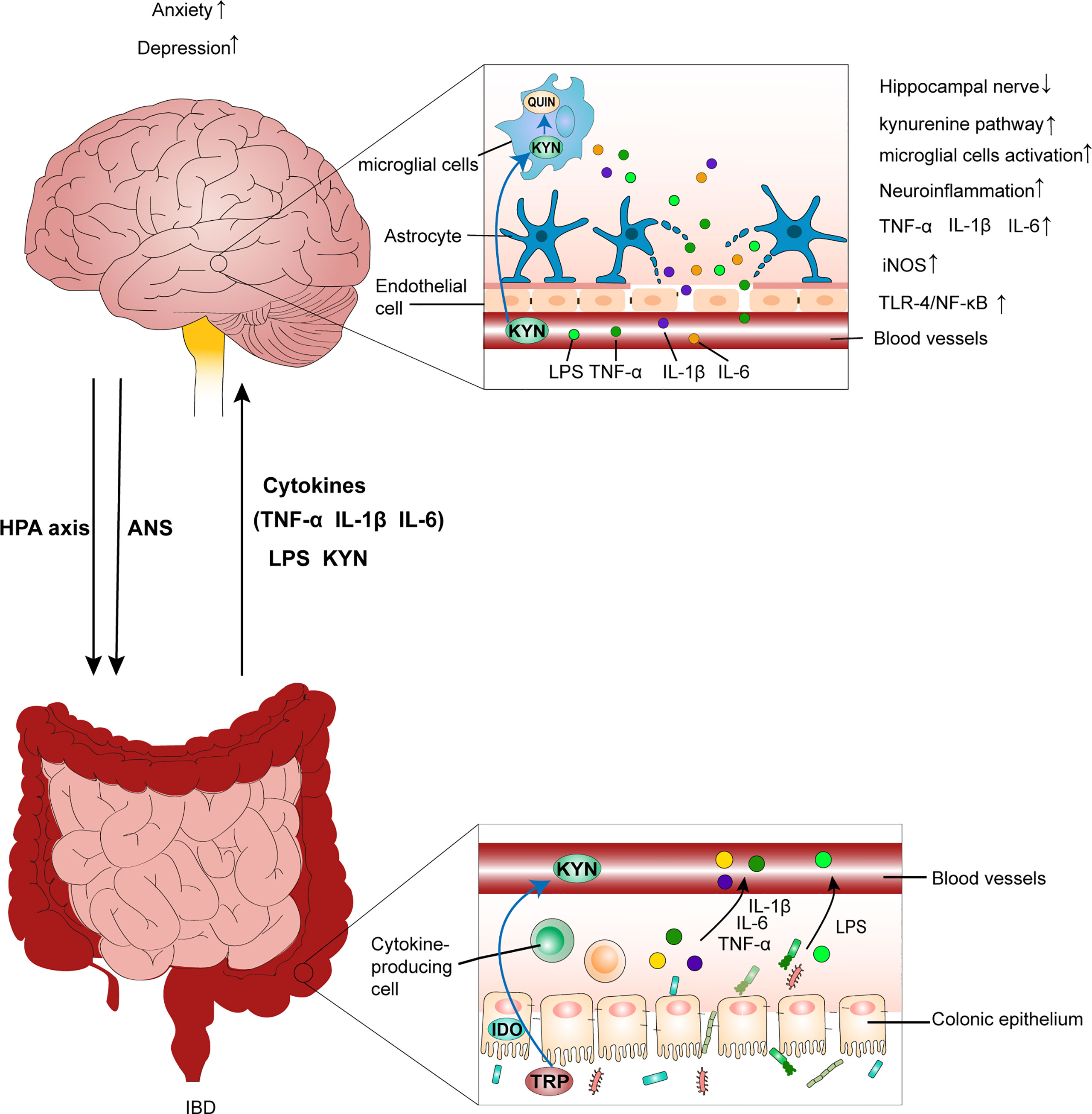
Figure 2 IBD leads to anxiety- and depression-like behavioral comorbidities by inducing neuroinflammation. Chronic psychological stress, such as anxiety and depression, aggravates colitis by activating the HPA axis and disrupting the ANS. This is accompanied by an increase in the production of LPS, pro-inflammatory cytokines (TNF-α, IL-1β, and IL-6), and gut leak. Additionally, peripheral inflammation causes a decrease in tight junction protein expression between endothelial cells in the BBB. Then, the disruption of BBB integrity predisposes the translocation of circulating inflammatory mediators into brain tissues, which leads to the inflammatory response in the CNS by increasing IL-1β, IL-6, and TNF-α levels, activating the TLR4/NF-κB inflammatory pathway, as well as increasing iNOS and nitrite levels in brain tissues. Further, circulating inflammatory mediators reaching the brain affect neuroglial networks and activate microglial cells, which further exacerbate the inflammatory response in the CNS. Additionally, activated microglial cells can promote neurodegeneration of the hippocampal nerve, which also contributes to mood disorders. Furthermore, overactivation of the kynurenine pathway generates excess QUIN in the brain, which impairs neurons and neuroglial cells, resulting in inflammation–mediated depression. IBD, inflammatory bowel disease; HPA axis, hypothalamic-pituitary-adrenal axis; ANS, autonomic nervous system; LPS, lipopolysaccharide; BBB, blood–brain barrier; CNS, central nervous system; TRP, tryptophan; KYN, kynurenine; QUIN, neurotoxic quinolinic acid.
Neuroendocrine stress response pathways
Different regions of the brain, especially the hypothalamus, amygdala, and hippocampus, interact with each other through complex neural networks involved in the generation of stress responses. Stress-induced neuroendocrine changes occur by two effector pathways: the hypothalamic-pituitary-adrenal (HPA) axis and the autonomic nervous system (ANS) (28). The HPA axis is activated by various stressors and, in conjunction with the ANS, enables the body to mount coordinated responses to perceived threats.
Psychological stress and the HPA axis
The HPA axis is a classic neuroendocrine regulatory network involved in the adaptive response to psychological stress. Chronic psychological stress can induce the lasting activation of the HPA axis (29). The hypothalamus secrets excessive corticotropin-releasing hormone (CRH), triggering the release of adrenocorticotropic hormone (ACTH) from the anterior pituitary gland. ACTH itself stimulates the secretion of adrenal glucocorticoids (GCs) by the zona fasciculata of the adrenal cortex, such as cortisol in humans and corticosterone in rodents (28, 30, 31).
Recent studies have shown that the HPA axis plays a significant role in stress and intestinal diseases. Firstly, CRH might be involved in the regulation of the intestinal barrier function, intestinal peristalsis, and secretion function in some intestinal diseases, such as IBS and IBD (32). Some studies have indicated that corticotropin-releasing hormone receptors (CRHRs) on the surface of mast cells (MCs), dendritic cells (DCs), T cells, and other gastrointestinal immune cells bind to excessive CRH released by the hypothalamus during stress responses, inducing MC degranulation, T cell-related adaptive immune response activation, increased intestinal-epithelial permeability, and increased pro-inflammatory cytokine production (33–36). In a recent study conducted on humans, the stress induced by public speaking raised serum cortisol levels in some subjects, and it was only in these subjects that the intestinal barrier function was disrupted, suggesting that the HPA axis was activated. Moreover, exogenous CRH administration in humans reproduced the impact of public speaking-induced psychological stress, which increased small intestine permeability (37). Additionally, the overproduction of GCs and CRH induced by chronic psychological stress reduces the expression of tight junctions in the intestine, damaging the function of the intestinal mucosal barrier, which is closely related to IBD progression (38–40).
Psychological stress and the ANS
Stress controls the autonomic response by stimulating the sympathetic nervous system, which causes the adrenal medulla to secrete excess epinephrine and norepinephrine, subsequently inducing central and peripheral tissues to secrete excessive inflammatory mediators/proinflammatory cytokines and promote the activation of inflammatory nuclear factor-κB (NF-κB) signaling (41). The gut itself has a rich supply of nerves, called the enteric nervous system (ENS). The neurons of the sympathetic and parasympathetic nervous system communicate with enteric nerves to form a complex neural network that has been termed the brain–gut axis. The ENS contains a large number of neurons that are responsible for regulating many functions of the gastrointestinal tract (28, 42). The stress-induced activation of the sympathetic nervous system excites the enteric ENS and increases the density of nerve fibers and the number of cholinergic neurons in the intestinal mucosa, leading to higher permeability of the intestinal epithelium (43, 44). Clinical studies have shown that lesions in CD and UC patients have a higher number of submucosal nervous plexuses and increased epithelial permeability (45).
The vagus nerve is a parasympathetic nerve which can achieve bidirectional communication between the CNS and the gastrointestinal tract (46). The release of acetylcholine from the efferent fibers of the vagus nerve inhibits macrophage-induced tumor necrosis factor (TNF)-α production through interneurons of ENS synapses between efferent vagus nerve endings and macrophages. Secretions from the vagus nerve are released through synapses and can also inhibit TNF-α secretion by splenic macrophages (46). Given the impact of TNF-α on IBD progression, the vagal inhibition of TNF-α secretion could be a promising avenue for treatment (47–49). A newly-identified vasovagal liver–brain–gut reflex circuit could control the number of peripheral regulatory T cells and maintain gut homeostasis (50).
It has shown that psychological stress inhibits the anti-inflammatory effect of the vagus nerve as stress reduces nerve tension and accelerates the production of inflammatory cytokines in several diseases, such as treatment-refractory depression, posttraumatic stress disorder, and IBD (46, 51, 52). Meta-analyses have indicated that the expression levels of some proinflammatory cytokines, like interleukin (IL)-1, IL-6, and TNF-α are significantly upregulated during depressive episodes (53, 54). In both UC and CD patients, lower vagal activity was associated with systemic inflammatory manifestations, especially excessive TNF-α and C-reactive protein (CRP) production (49, 55). Systemic inflammation, by itself, can lead to a depressive state and promote IBD flare-ups (20, 56, 57). Excitedly, vagus nerve stimulation (VNS) has shown promise for modulating neuroinflammation and suppressing intestinal inflammation associated with IBD (58–63). Kibleur et al. (60) have found that chronic VNS decreased anxiety and resulted in general clinical improvement of CD. An additional study demonstrated that VNS improved the intestinal barrier function by promoting intestinal epithelial renewal (48). Thus, by restoring vagus nerve activity, VNS is potentially useful in the treatment of IBD (46).
Chronic psychological stress disturbs intestinal immune function during IBD
The dysfunction of innate and adaptive immune pathways is the primary cause of intestinal inflammation in patients with IBD (10, 64, 65). It is well known that the immunopathogenesis of IBD has been involved in mediation by various different cell types, including classic immune cells, like macrophages, MCs, neutrophils, DCs, monocytes, and adaptive immune cells, such as T cells, and nonimmune cells, like epithelial cells, endothelial cells, and mesenchymal cells (66, 67). However, it is still unclear to what extent psychological stress is involved in the regulation of the immunopathology of these immune cells during the aggravation/relapse of IBD or intestinal inflammation. In other words, spatiotemporal regulation of psychological stress on these immune cells and cytokine production is important to elucidate the mechanism underlying stress-mediated IBD/intestinal inflammation. Table 1 presents a summary of the stress-induced dysfunction of specific immune cells and the mechanisms involved in IBD progression/inflammatory aggravation.
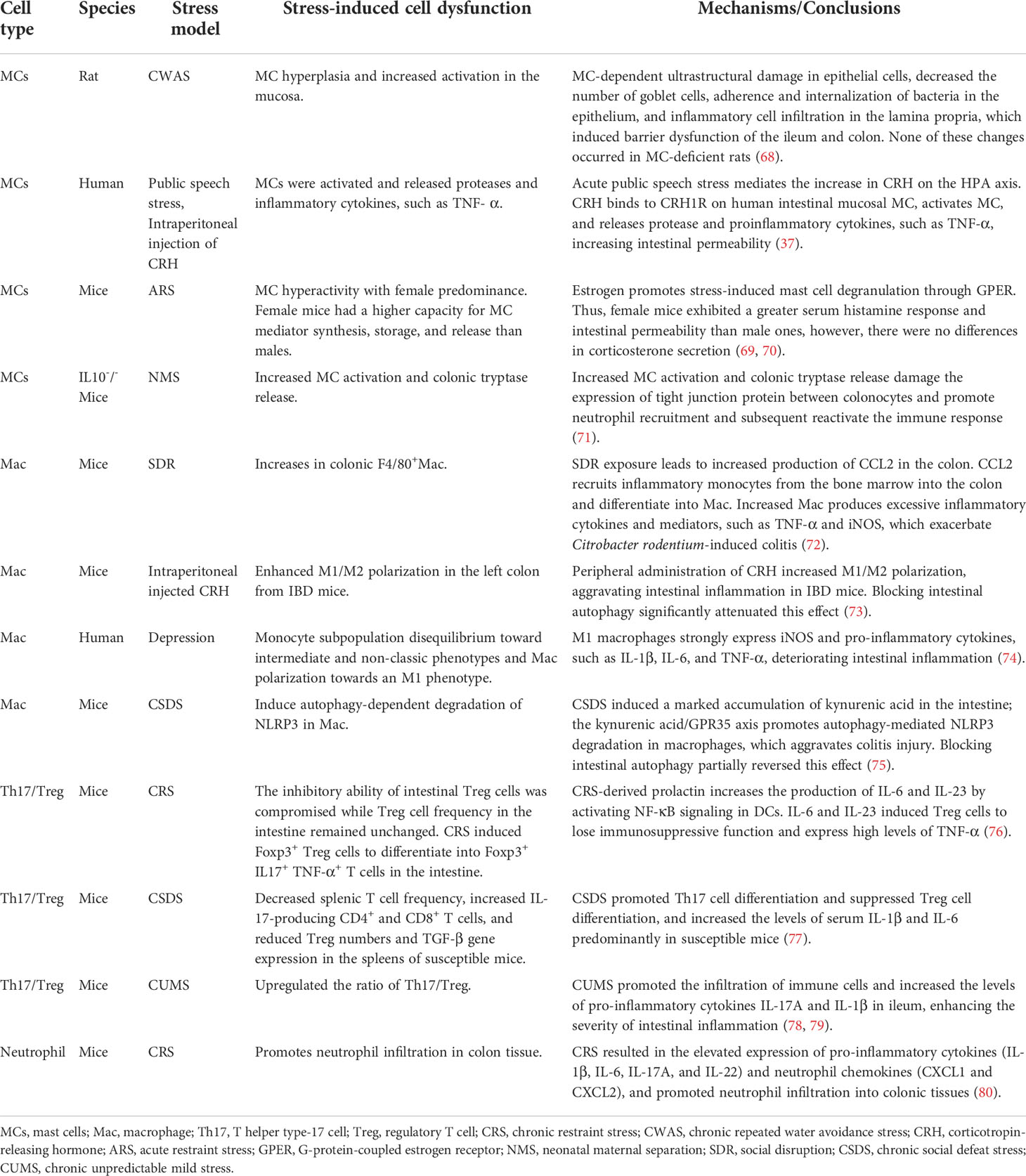
Table 1 Stress-induced dysfunction of specific immune cells and mechanisms promoting IBD development/inflammatory aggravation.
Chronic psychological stress activates intestinal mucosal MCs
MCs are effectors of the brain–gut axis that produce neurotransmitters and pro-inflammatory cytokines in response to stress signals to regulate the permeability of the intestinal epithelial barrier via the HPA axis (81). Animal experiments have shown that chronic psychological stress-induced CRH is released by the hypothalamus and binds to MC CRHR-1 to induce activation and degranulation of MC and increase the permeability of the intestinal epithelium while blocking CRH release inhibits MC degranulation and the production of TNF-α and protease, which delays the impairment of intestinal barrier. Intriguingly, in stressed rats with an MC defect, intestinal epithelial function and morphology were not markedly altered (68, 82). One Clinical study found that public speaking-caused psychological stress results in the activation of HPA axis and the elevated level of CRH only in some volunteers that the intestinal permeability was higher than the other volunteers, whereas, symptoms could be eliminated by MC stabilizers (37). These studies have implied that psychological stress aggravates the permeability of the intestinal epithelial barrier likely through an intestinal mucosal MC activation-dependent pathway (37). Additionally, the study of Mackey et al. (69) found that when C57BL/6 mice were exposed to restraint stress (RS), female mice had significantly higher intestinal permeability, serum histamine levels, and MC degranulation than male ones, suggesting that changes to the intestinal epithelial barrier induced by psychological stress through the regulation of MCs were sex-specific. This phenomenon might be caused by G-protein-coupled estrogen receptor (GPER) on MCs of the colonic mucosa. Estrogen promotes stress-induced MC degranulation through GPER (70). In a comorbid mice model of neonatal maternal separation (NMS) stress and colitis, the researchers have explored that NMS stress exacerbates colitis in IL10-/- mice by disrupting intestinal barrier. Notably, increased MC activation and colonic tryptase release were found which could damage the expression of tight junction protein between colonocytes and promote neutrophil recruitment and subsequent reactivate the immune response, thus like play a vital role in defects in intestinal barrier function (71).
Chronic psychological stress promotes M1 phenotype polarization and autophagy in macrophages
Macrophages play a key role in IBD pathogenesis, and macrophage infiltration, along with an imbalance in the macrophage M1/M2 ratio, is associated with IBD development (83, 84). Recent studies have indicated that macrophages are involved in the pathophysiology of psychological stress-induced IBD aggravation. Social disruption (SDR) stress has been shown to increase the number of F4/80+ macrophages in the colon and thus enhanced severity of infectious colitis in mice (72). Additionally, as the stress mediator, CRH can abnormally enhance the macrophage M1/M2 polarization in the left colon of mice with IBD (73). Similarly, in clinical studies, depression promoted the migration of monocytes from the blood to the intestinal mucosa, causing macrophages to infiltrate colonic tissues, which aggravates inflammation. CD patients with depression have a higher number of M1 macrophages and higher levels of their secreted proinflammatory cytokines and a lower number of M2 macrophages and lower levels of their secreted anti-inflammatory cytokines (e.g., IL-10) than those without depression, suggesting that depression promotes macrophage polarization into an M1 phenotype and accelerates IBD progression (74). Another recent study has shown that the stress-induced over-activation of macrophage autophagy might also aggravate IBD (75). The results indicated that chronic social defeat stress (CSDS) caused kynurenic acid accumulation in mouse colons, which, in turn, enhanced macrophage autophagy in the intestine. This induced the defective activation of NLRP3 inflammasomes, making mice more vulnerable to dextran sulfate sodium (DSS)-induced colitis (75). Thus, it is possible that chronic psychological stress is involved in IBD’s pathophysiology by promoting the infiltration of macrophages into the colon and polarization of macrophages into an M1 phenotype, which both enhance autophagy.
Chronic psychological stress regulates T cell differentiation, phenotype, and function
CD4+CD25+Foxp3+cells, which express the inhibitory receptor, CTLA-4, and produce high levels of IL-10 and TGF-β, are the key regulatory T cells (Tregs) responsible for inducing immune tolerance and preventing colitis by inhibiting immune effector cell activation through cell contact and inhibitory cytokine production (76, 85). The unbalanced T helper type-17 (Th17) cell/Treg ratio associated with intestinal inflammation is a typical feature of the disruption in mucosal immune homeostasis (86–88). Present studies indicate psychological stress-induced alterations in the differentiation frequency of Th17 and Treg subsets. In CSDS mouse model, stress enhanced splenic IL-17-producing Th17 differentiation, suppressed Treg cell differentiation and decreased the levels of TGF-β in the stress susceptible mice (77). Similarly, in CUMS mouse models, stress upregulated the ratio of Th17/Treg in the ileum, disrupted immune homeostasis by activating the inflammatory response and thereby enhance intestinal inflammation (78, 79). These studies have implied that an investigation of the precise mechanism by which chronic psychological stress impacts Th17 and Treg subset differentiation is imperative to understand the mechanism of stress-induced adaptive immunity response of intestinal T cells.
A recent study found that chronic psychological stress also induced phenotypic and functional changes in T cell subsets. In CRS mouse models, chronic stress did not change the frequency of Treg differentiation in the intestine but could induce phenotypic changes in Treg cells and damage their inhibitory function. In the study, chronic psychological stress stimulated serum prolactin secretion and, in turn, activated inflammatory NF-κB signaling to induce IL-6 and IL-23 production from DCs. This change in the intestinal DC phenotype induced Tregs to differentiate into Foxp3+ IL17+ TNF-α+ T cells that produced a lot of TNF-α and enhanced intestinal inflammation. The study also showed that chronic stress aggravated colitis symptoms in mice on DSS/TNBS treatment (76). These findings showed that chronic psychological stress regulates the differentiation frequency, phenotype, and function of Th17 and/or Treg cells in a complex manner, providing important research directions for the study of how chronic psychological stress regulates intestinal immune function and affects IBD development.
Except for the immune cells mentioned above, neutrophils may also be one of the target cells of psychological stress-induced IBD. A recent study revealed that chronic psychological stress upregulated the levels of neutrophil chemokines (CXCL1 and CXCL2) which stimulated neutrophil mobilization and infiltration into colonic tissues, thereby secreted excessive proinflammatory cytokines (IL-1β, IL-6, IL-17A, and IL-22), so the robust inflammatory response aggravating colitis in a DSS-induced mice model (80). Obviously, there are many other immune cells involved in the occurrence of IBD, such as natural killer (NK) cells, natural killer T (NKT) cells, and innate lymphoid cells (ILCs) (67). However, whether these immune cells are involved in the mechanisms underlying the interaction of psychological disorders with the IBD progression has not been studied. Perhaps, such studies should be initiated under both experimental conditions and human clinical trials.
Neuroimmune modulation of biogenic amines with focus on Th17 Cells
It has shown that biogenic amines, mainly including catecholaminergic dopamine, norepinephrine, and adrenaline, 5-hydroxytryptamine (5-HT)/serotonin, and histamine, are direct neurotransmitters present in central and peripheral tissues with potential effects on neuroimmune interaction and brain–gut axis (89–92). Particularly, serotonin is one of the critical neurotransmitters that not only involves the pathogenesis of various psychological and psychotic diseases, but also alleviates neuroinflammation by manipulating immune cell activity and cytokine production (93). More than 90% of serotonin is contained in the gut and accumulating evidence has demonstrated that serotonin may be of relevance in relation to the psychopathology of IBD and IBD with psychological comorbidities by modulating the IL-17/Th17 signaling response (89, 90). It has demonstrated that serotonin follows a receptor-specific pattern to suppress the release of inflammatory mediators IL-1β, IL-6, TGF-β3 and IL-23 and thus inhibit the development of pathogenic Th17 cells (94–96).
Along with biogenic amines, recent studies have shown the critical pathogenetic role of Th17-cells and Th17-immune response in participation in the gut-brain axis to mediate chronic neuroinflammatory and autoimmune diseases (97). Intriguingly, studies have found that compared to that of healthy control, patients with depression have decreased Tregs and concentration of serotonin, as well as increased Th17 cells (92, 98). Th17-cells produce pro-inflammatory cytokines such as IL-17A, IL-21, IL-22, and interferon-γ (IFN-γ) (99, 100). IL-17A production and the expression of IL-6 and chemokine receptor-6 promote penetration of Th17-cells through the blood–brain barrier (BBB) into the CNS and induced the mental disorders and neuroinflammation (101, 102).
Although the precise mechanisms of action of Th17 cells in depression remain unclear, Th17 cells appear as a promising therapeutic target for depression. The neutralization of IL-17A by anti-IL-17A antibodies or supplement of serotonin to block the release of gut Th17 cells might represent a reasonable and feasible therapeutic approaches to improve depression symptoms (92).
In addition to regulating immune cells directly, psychological stress can also affect host immunity through altering the composition and abundance of the intestinal microbiota. The disturbed intestinal microbiota induced the increased expression of inflammatory cytokines both in the gut and in systemic tissues by promoting the colonization and amplification of pathogens, activating immune responses, and promoting intestinal inflammation (103, 104). Therefore, the precise mechanism by which stress manipulates intestinal microbiota and thus shapes host immunity has become a central segment to further analyzing the pathology of IBD with psychological comorbidities.
Chronic psychological stress disrupts the intestinal microbiome in IBD
The brain–gut axis is a sophisticated bidirectional communication network between the CNS and the intestine. Recently, the intestinal microbiome has been regarded as the third critical component of the brain–gut axis, and the concept of a brain–gut–microbiota axis has been proposed (105, 106). As mentioned above, psychological stressors can change to the composition of intestinal microbiota, and promoting intestinal inflammation (103, 104). In this context, increasing attention has been placed on the interactive regulatory mechanism of the brain and microbiota during disease progression (107, 108). Table 2 provides a summary of stress-induced microbiome community dysbiosis and the mechanisms involved in IBD progression/inflammatory aggravation.
A acute stress can reduce the abundance and diversity of bacterial species, increase colonic colonization/amplification of pathogens–, especially the Proteobacteria population, including Citrobacter rodentium, Helicobacter pylori, and Clostridium, and upregulate the expression of inflammatory cytokines in the colon and plasma, this exacerbating gastrointestinal inflammation (104, 109–112). Long-term chronic stimulation also changes the composition of intestinal microbiota, further damaging immune homeostasis in the intestinal mucosa and aggravating colitis (17, 113–115). Chronic stress induced spontaneous colitis or exacerbated chemically-induced colitis by promoting the expansion of pathogens and activating the mucosal immune response (17, 113–115). For example, in chronic subordinate colony housing (CSC) pretreated mice, the abundance of Helicobacter and Paraprevotella was remarkably increased and simultaneously activated the host’s immune response. After adding DSS, mouse mesenteric lymph node cells were further increased, more IFN-γ and IL-6 was produced, and intestinal tissue damage worsened (113). Similarly, male C57BL/6 mice that received CRS pretreatment and subsequent DSS intervention showed a more significantly increased abundance of pro-inflammatory bacteria, such as Peptostreptococcaceae, Helicobacter, Streptococcus, and Enterococcus faecalis, further increasing inflammation, activating the IL-6/STAT3 inflammatory signaling, and aggravating colitis. Unexpectedly, the stress sensitization of colitis was not stopped in IL-6 knockout mouse models, which suggestes that a high inflammatory response was not the main cause of the chronic stress-aggravated colitis. Conversely, antibiotic treatment eliminated the intestinal microbiota factor and the difference between the stressed group and the non-stressed group (17). Additionally, Clostridium perfringens and Clostridium sordellii are both pathogens producing phospholipase C, a major virulence mediator. The abundance of these bacteria was increased in water avoidance stress (WAS)-treated mice, and both strains of bacteria have been associated with colitis exacerbation (114, 115). These findings indicated that the key target of chronic stress-induced intestinal inflammation is intestinal microbiota (17, 113–115).
Researchers have also found that the change in microbial flora induced by CRS results in insufficient intestinal mucosal barrier-related protein, mucin-2, thus, disrupting the colonic mucus and aggravating colitis in murine models, suggesting that the microbial flora factor plays a synergetic role in damaging the intestinal barrier (16, 17). More especially, researchers have demonstrated that the population of Akkermansia muciniphila (A. muciniphila) was obviously reduced in CRS mice and UC patients with depressive symptoms. Remarkably, its abundance changes were positively correlated with mucin-2 expression. The administration of A. muciniphila could repair colonic mucus layer and modify the gut microbiota. Their results suggest that A. muciniphila plays a beneficial role in protecting the intestinal mucosa in IBD patients with psychological disorders (16). Interestingly, maternal stress during pregnancy also affects the offspring. Prenatal maternal stress (PNMS) can increase the abundance of pathogenic bacteria, especially proteobacterial groups, and decrease the abundance of beneficial bacteria, especially Bifidobacterium and Blautia, which has profound pathological impacts on the infant’s immune system and ultimately increases the susceptibility of adult mice offspring to colitis (116, 117).
While much of the current understanding of the link between stress and intestinal microbiome comes from animal models (121, 122), many human and patient-related studies of the brain–gut–microbiome axis are in progress (123–125). A few clinical studies have also associated stress with microbiome homeostasis and gastrointestinal health (118, 126, 127). Studies have shown that the richness and diversity of the fecal microbiome were dramatically reduced in IBD patients with anxiety or depression compared to patients without anxiety or depression (118, 126, 127). For patients with IBD in remission, Humbel et al. found that psychological disorders were negatively correlated with the abundance of Clostridia, Bacilli, Bacteroidia, and β- and γ-Proteobacteria. Although Proteobacteria is widely considered as pathogenic bacteria, the population of β- and γ-Proteobacteria was generally reduced with increasing anxiety in this study. Thus, the clinical trials with large samples are needed to further confirm the validation of this questionable finding. Psychological disorders have also been linked to a decrease in the composition and richness of some bacteria such as Lachnospiraceae, Fusobacteriaceae, Ruminococcaceae, and Veillonellaceae (127). Additionally, studies have demonstrated that the relative abundance of Desulfovibrio in patients with UC and Bifidobacterium in patients with CD is associated with depression, whereas the abundance of Sutterella, RF 32, and Lactococcus associated with patients’ QoL only among patients with CD (127). For patients with active UC, a prospective study of 240 Chinese patients has demonstrated that, in total, almost 50% of the patients had symptoms of anxiety or depression (118). The researchers have found that patients with UC and depression/anxiety (UCD/UCA) had more Lactobacillales, Sellimonas, Streptococcus, and Enterococcus but less Prevotella 9 and Lachnospira than those with UC without depression or anxiety (UCND/UCNA) (118). Correspondingly, the researchers also found an obvious increase in the abundance of the Enterococcus genus and a reduction in, among others, Bifidobacterium, Roseburia, Lachnospiraceae/Lachnospira, and Ruminococcus in IBD patients (119, 128, 129). From this point of view, these studies also suggested that psychological stress aggravating IBD might be related to the amplification of intestinal pathogens and the decrease in the number of beneficial bacteria. However, because the above-mentioned research comprise cross-sectional observational studies of gut microbiota, it remains to be clarified whether the observed changes in the gut microbiome are a cause or a consequence of IBD and psychological disorders, and a comprehensive dissection of the interactions between the microbiota and the host is needed. It is also necessary to use germ-free animal models to verify the validity of the conclusions. A recent Fecal microbiota transplantation (FMT) study has partially solved these problems (126). The results of this study showed that fecal transplantation in IBD patients with depression (IBD/D+-F) caused more severe IBD-like colitis in specific pathogen-free (SPF) recipient mice than in IBD patients without depression (IBD/D−-F). Additionally, it should be noted that the Enterococcaceae population was higher in IBD/D+-F than in IBD/D−-F (126). Unfortunately, the study used the SPF mice model rather than the germ-free mice model. Nevertheless, the above-discussed studies suggest that the alterations of the intestinal microbiota might be not only a vital factor in the appearance of psychological symptoms but also a target of future microbiota-modulating therapeutic strategies targeting at IBD patients or IBD patients with psychological comorbidities.
In addition to the intestinal microbiota itself, the microbial metabolites also participate in the pathogenesis of colitis. Short-chain fatty acids (SCFAs), one of the important metabolites of intestinal flora, play a major role in several physiological processes, including the maintenance of the intestinal barrier, the inhibition of opportunistic intestinal pathogen colonization, and the regulation of the Th17/RORγt+ Treg cell balance (130, 131). A recent study of the animal model revealed that CUMS causes a significant decline in the intestinal Prevotella-9 and Alloprevotella abundance (120). Furthermore, clinical studies also found that the relative abundance of Roseburia, the butyrate-producing bacterial strain, was negatively correlated with depression in CD (127, 132). As mentioned above, compared to UCND/UCNA, UCD/UCA had less Prevotella-9 and Lachnospira (118). Microbial dysbiosis and low abundance of butyrate-producing bacteria, including Prevotella-9 and Lachnospira, reduces butyrate levels in the intestine, lowers intestinal epithelial tight junction protein expression, and decreases the number of goblet cells, resulting in damage to the barrier function of the mucosal layer and aggravating the colonic inflammatory response (118, 120). Additionally, the levels of a few metabolites, such as 2’-deoxy-D-ribose and L-pipecolic acid, which were enriched in UCND/UCNA, were decreased, accompanied by a reduction in immunoglobulin proteins in UCD/UCA, implying that the two beneficial metabolites might play a significant role in the host immune response of UCD/UCA. Particularly, the prophylactic administration of these metabolites could significantly alleviate the depressive-like behaviors in mice with colitis and decrease central and peripheral circulating inflammatory cytokine levels (118). The above studies have strongly confirmed that stress can induce alterations to the fecal microbiota and metabolites, further aggravating intestinal inflammation. Interestingly, the modulation of the intestinal microbiota changes stress responses, further emphasizing the bidirectionality of the brain–gut axis. FMT has become an attractive therapeutic strategy (133). It has shown that the severity of anxiety, depression and obsession in IBD patients decreased after FMT (134). In a stress model that mimics social isolation, altering the intestinal flora with the administration of rifaximin could reduce stress responses and brain function deterioration (135). In another repeated psychosocial stress model, SCFAs supplementation alleviates increase in intestinal permeability (136). Such findings were complemented by those of studies assessing the protective effect of probiotics during stress. For example, it has been demonstrated that treatment with probiotics (i.e., Lactobacillus casei [LcS]) relieved stress-induced abdominal dysfunction (137). Probiotic supplements also may alleviate stress-induced colitis by suppressing gut bacterial LPS and subsequently inhibiting the intestinal inflammatory response (138).
IBD leads to anxiety- and depression-like behavioral comorbidities
Studies have shown that anxiety/depression-like behavior, as -comorbidity, is likely to evolve into one of the main features of IBD (139–141). Moreover, long-term follow-up of patients found that patients who were clinically active at baseline but had no symptoms of anxiety or depression at the start of the study had a significantly higher risk of developing new-onset anxiety or depression symptoms (142). However, the pathologic mechanisms by which intestinal inflammation induces cerebral structural changes and, thus, lead to behavioral comorbidities remain unclear. Based on gathered evidence, we proposed the potential mechanism by which IBD leads to anxiety/depression (Figure 2).
It has been shown that circulating pro-inflammatory mediators in IBD patients might be the main culprits promoting the development of CNS inflammation. Consequently, increasing evidence indicates that mood disorders are associated with altered inflammatory statuses in the CNS (143–145). In some studies involving the use of experimental colitis animal models, peripheral inflammation elevated the levels of proinflammatory cytokines such as TNF-α, IL-1β, and IL-6 in the hippocampus, cerebral cortex, and hypothalamus (146–153). Furthermore, researchers have also found the overexpression of TLR-4, phosphorylated-NF-κB p65, myeloid differentiation primary response 88 (Myd88), and NOD-like receptor protein 3 (NLRP3) in the hippocampi of animals in IBD groups compared with control animals, indicating the activation of the TLR4/NF-κB inflammatory pathway and NLRP3 inflammasomes in the CNS (148, 153, 154). That evidence suggests that colitis could lead to CNS inflammation, which culminates in the occurrence of abnormal behavior in animals. Additionally, peripheral inflammation also significantly increased inducible NO synthase (iNOS) and nitrite levels in the hippocampus and cerebral cortex, contributing to the occurrence of anxiety- and depression-like behavioral comorbidities (151, 154, 155). For example, compared with those in the control group, mice with colitis showed longer-lasting immobility in the forced swimming test (FST), and higher expression levels of pro-inflammatory mediators (including TNF-α), iNOS expression, and nitrite content in the hippocampus. However, when NOS inhibitors were used to prevent iNOS and nitrite production, mice with colitis had a shorter rest time in FST, lower TNF-α and nitrite levels in the hippocampus, and reduced inflammatory injury in the hippocampal region (155).
Hence, the crucial mechanism by which peripheral inflammation contributes to the progression of several CNS disorders remains unknown. Other studies demonstrated that colitis can increase the amounts of bacteria-derived toxic byproducts (LPS), pro-inflammatory cytokines (including TNF-α, IL-1β and IL-6), and gut leak (126, 153, 156). Moreover, the expression levels of tight junction proteins, including ZO-1, occludin, and zonulin, were significantly decreased in the cerebral tissues of experimental animals with colitis (153, 156, 157). These findings have confirmed that colitis can cause endothelial damage to the BBB. Furthermore, a broken BBB is beneficial in translocating circulating proinflammatory mediators into cerebral tissue, leading to the inflammatory response in the CNS and affecting neuroglial networks and activated microglial cells that further exacerbate the inflammatory response in the CNS (145). Additionally, activated microglial cells can impair the proliferation and differentiation of hippocampal progenitor cells by producing serotonin-depleting enzymes and mediators, and consequently, promoting neurodegeneration, which is associated with the pathophysiology of depression-like behaviors (158, 159).
More recently, new mechanisms leading to these behavioral comorbidities in IBD have been proposed. Carloni et al. (160) described a vascular barrier existing in the brain choroid plexus (PVB), which is able to respond to intestinal inflammation through bacteria-derived LPS. An in vitro experimental model of choroid plexus endothelial cells has shown that PVB closure is associated with short-term memory and mental deficits. Speculation that the dysfunction of the gut-brain vascular axis might be a factor worthy of attention leading to IBD-related psychiatric comorbidities. Additionally, Chen et al. (161) reported that IBD-related psychological disorders could be related to the activation of the kynurenine pathway (KP) due to chronic inflammation. After the activation of KP, excessive neurotoxic quinolinic acid and less neuroprotective kynerunic acid were produced in cerebral tissue, ultimately resulting in inflammation-induced depression.
Understanding the mechanisms of how IBD leads to psychiatric comorbidities and exploring the causal relationship between these comorbidities and IBD are important for the prevention, treatment, and prognosis of IBD. Although the current studies are mostly limited to animal models, many of the findings provide valuable clues to clarify the potential link between IBD and anxiety- and depression-like behaviors in humans. With the development of functional brain imaging technology that can monitor the changes in brain signaling and morphology, the mechanism of the brain–gut bidirectional communications and novel avenues for intervention can be further clarified.
Application of neuroimaging studies in evaluating the possible neuromechanisms of IBD
Brain–gut interactions may be able to explain the increased prevalence of psychiatric symptoms in IBD, however, studies on the pathological changes in the brain caused by IBD remain equivocal. In recent years, functional brain imaging mainly including positron emission tomography (PET) and functional magnetic resonance imaging (fMRI), has enabled in vivo analyses of the interactions and neurological mechanisms between the brain and digestive tract, advancing the scientific understanding of this relationship. Compared with PET, fMRI is non-invasive, safer and easier to operate. Therefore, it is more widely used to image changes in brain function in IBD (162–165).
Agostini et al. (166) used fMRI to investigate the neural emotional changes in UC patients in remission versus healthy controls. They found that patients with UC manifest a decrease in sensitivity to positive emotions of joy and well-being. Because all patients enrolled in their study were in remission, the emotional dysfunction was not strictly associated with current inflammatory activity. Nevertheless, their findings represent a preliminary but interesting result that might reveal novel avenues for the study of the brain–gut axis and for understanding the relationship between mood and intestinal chronic inflammation (166). More recently, research has found that changes in gray matter volume (GMV) in regions, such as the insula, pregenual anterior cingulated cortex, thalamus, amygdala, supplementary motor area, periaqueductal gray, hypothalamus, and precentral gyrus, which were involved in visceral sensory pathways, were more commonly observed in UC patients using structural MRI than in healthy controls. Meanwhile, compared to patients with UC in remission, those in the active phase have a much greater chance of developing GMV changes, thus, the researchers claimed that changes in the brain could be partially connected with the clinical stage in patients with UC (167). Interestingly, multimodal brain MRI analysis has shown that behavioral symptoms are strongly associated with structural and functional changes in deep gray matter that modulate emotional, cognitive, and stress responses in IBD patients. Compared to healthy controls, the patients with UC or CD exhibited the increased volumes of amygdale and hypothalamus, the neurodegeneration of putamen and pallidum, and significantly increased activity and functional connectivity (FC) in cognitive and emotional processing brain regions, including the hypothalamus, basal ganglia, and limbic system. Particularly, an increase in the volume of the thalamus can reflect an exacerbated inflammatory state in the brain. Meanwhile, the researchers also found that the hippocampal nerve activity is increased with IBD in the active phase compared with those in remission (168). Additionally, a dynamic brain functional connectome analysis using fMRI has revealed medial prefrontal cortex dysfunction, which is involved in the deterioration of depression and anxiety in patients with UC (169). Those studies provide a promising perspective and convincing neuroimaging evidence of potential neuromechanisms of UC.
fMRI can also be used to provide additional information about changes in the brain structure of CD patients. CD patients display altered grey matter structures and grey matter structural connectome, which just to a certain extent gives the reason why these patients showed higher levels of anxiety and depression (163, 170). Stress-induced hyperactivity has been found in particular brain regions of CD patients, including the amygdala and midcingulate cortex (171, 172). These alterations might represent a disruption of the brain–gut axis connection that could predispose IBD patients to psychological comorbidities and need to be considered during treatment. It is worthnoting that recent studies have focused on brain functional alterations in CD patients during the resting-state (173–177). Compared to healthy controls, fMRI analysis demonstrated that the CD patients during the resting-state of brain show the altered FC of pre-frontal cortex by increasing FC between the cognitive control and salience network and decreasing within the default mode network (174). The findings of another fMRI analysis explored the aberrant FC of the amygdala with the multiple regions, including and insula, parahippocampus, as well as anterior middle cingulate cortex/dorsal anterior cingulate cortex (173). Additonally, a recent meta-analysis study for the existing neuroimaging data indicated that, compared to healthy controls, patients with CD during the resting-state of brain had decreased FC in the paracentral lobule and cingulated gyrus as well as reduced GMV in the medial frontal gyrus (175). These brain functional alterations suggested that the aberrant FC may be associated with high sensitivity in negative emotion and reduced inhibition on processing of visceral pain and sensation in CD patients during the resting-state of brain. Excitingly, it has indicated that the improved cognitive deficits in major depressive disorder were linked to alterations in limbic (amygdala) function following anti-TNF-α treatment (178). Thus, the urgent issues should investigate not only potential network alterations during different CD states especially the period of active disease and even after treatment but also combine various neuroimaging and psychometric approaches to clarify how these respective alterations are related to each other.
Although a growing number of neuroimaging studies have shown significant neurological changes in IBD, small sample sizes and cross-sectional studies might undermine the credibility of these results. Furthermore, since UC and CD respectively share distinct pathological features, it is unclear whether such distinct pathological features lead to different neuroanatomical changes. Therefore, future studies should examine more patients with CD and UC to determine whether there are significant neurological differences between the two specific diseases. Additionally, to determine whether brain structural alterations correlate with disease activity, researchers should include patients with different disease states to investigate separately in a longitudinal design.
Psychotherapy and antidepressants for patients with IBD
Recent research findings have suggested that psychological interventions can improve the treatment effect of gastrointestinal diseases, which will improve the QoL of patients (179–184). Among a variety of psychological interventions, cognitive behavioral therapy (CBT) is recognized as the most effective psychotherapy for managing IBD, which can reduce the rate of psychological disorder and improve QoL in IBD patients (185–189). Furthermore, in a benchmarking study, CBT not only significantly reduced scores of anxiety and low mood and significantly increased sores of QoL but also decreased disease activity in the specific group of IBD patients who also had anxiety and low mood (186). Similarly, treatment with CBT has been associated with a significantly greater improvement in the depressive severity in the overall sample of young people with depression and CD and correlated with a significantly greater improvement in the pediatric CD activity in the subset with active IBD (190). Additionally, a randomized trial indicated that multicomponent CBT (MCBT) is highly effective in alleviating low mood and improving QoL in stress-prone IBD patients. Noticeably, this program could effectively reduce the relapse rate of patients; however, no differences in disease activity indexes were found (191). As far as the current study is concerned, a recent systematic review has claimed that the positive effects of CBT on improving the psychological status of IBD patients are not long-lasting, and there is insufficient evidence that CBT improves disease activity or reduces inflammation levels (192).
Mindfulness-based interventions (MBIs), which primarily manifest as attention to one’s physical sensations, thoughts, and emotions, have also been shown to have a positive impact on relieving stress levels and improving QoL in IBD patients (193–196). For example, patients with inactive UC demonstrated significantly lower stress and higher QoL after mindfulness-based stress reduction treatment (197). Recently, a randomized controlled trial has also demonstrated that MBIs had a positive impact on alleviating psychological disorders, fatigue, and improving the QoL of patients with CD. Particularly, CD patients with severe baseline psychological symptoms were the greatest beneficiaries of MBIs (198). Additionally, some studies have indicated that MBIs likely represented a promising intervention to reduce inflammation in IBD patients because MBIs could effectively decrease the levels of mucosal inflammatory biomarkers, including IL-6, fecal calprotectin, and CRP levels (194, 196, 198). Thus far, limited support is available for the effects of MBI on disease-related and physiologic outcomes. Therefore, future studies should pay more attention to the clarification of the physiological effects of MBIs on IBD, and find reliable physiological indicators to evaluate its effects.
Currently, various types of psychotherapy interventions–, such as gut-directed hypnotherapy, participation in the breath-body-mind workshop, advanced combination treatment, specialized educational and psychological counseling, and acceptance and commitment therapy–, are available (184, 199–202). The effectiveness of these psychological interventions for managing IBD is also being studied; however, there is still a paucity of trial evidence of efficacy for most of them. For example, it is not certain that hypnotherapy can relieve IBD symptoms and prolong the remission period during UC (203, 204). Although the effect of psychological therapy on IBD is controversial, 30%–50% of- IBD patients with reduced QoL are expected to receive complementary psychological interventions (205, 206). Additionally, it has been demonstrated that patients with active disease, especially during a flare, have higher anxiety/depression and lower QoL scores and appear to benefit more from psychotherapy than those in remission (207, 208). Therefore, more high-quality studies are needed to design personalized psychotherapy whose early intervention would improve coping strategies and QoL scores for IBD patients in the future.
Accompanied by psychotherapy, antidepressants have been considered as potential adjunctive treatments for IBD with psychological comorbidities. Firstly, some studies have indicated that antidepressants such as Tianeptine and Duloxetine have positive influence on the improvement of severity of psychological and physical symptoms of patients with IBD (209). Furthermore, it has shown that antidepressants can help mitigate coexisting functional gastrointestinal symptoms in IBD. Some studies have demonstrated that Tricyclic antidepressants (TCAs) have efficacy in treating residual gastrointestinal symptoms and slowing colonic transit in IBD patients (210, 211). Excitingly, antidepressants may also have a positive effect on the inflammatory state of IBD. In animal models of IBD, both desipramine and fluoxetine significantly attenuated colonic pathological damage and lowered serum concentrations of TNF-α and IL-1β (212, 213). Several human trials have observed that antidepressant fluoxetine show modest effects on immune functions (214), and antidepressants tianeptine and duloxetine can significantly improve the disease activity (209). Additionally, antidepressants might relieve chronic pain during remission and improve impaired sleep quality in patients with IBD (215, 216).
Antidepressants may be beneficial in the treatment of IBD, however, the evidence supporting this conclusion is insufficient, the quality of the evidence is very low to moderate and requires further investigation. Limited preliminary studies has shown the potential of antidepressants for IBD, which needs to be validated by a large number of well-designed clinical randomizedcontrolledtrial (RCT) trials with a longer follow-up in the future. Although no major adverse events have been reported with antidepressants for IBD, there is a potential risk of gastrointestinal-specific adverse effects, especially when more than one antidepressant is used (214, 217). At present, it is not clear which class or specific drug might be the most effective for patients with IBD alone or for those with psychological comorbidities (218). Despite the studies in this area is still deficient, accumulating evidence suggests that antidepressant treatments have the potential to mitigate the risk of IBD, ameliorate mental health and QoL in some IBD patients, and alter the natural history of the disease (219–221).
Conclusion
Chronic psychological stress accelerates IBD progression by affecting the neuroendocrine-immune regulatory network and disturbing intestinal mucosal immunity and microbiome homeostasis. Of note, IBD might also lead to anxiety- and depression-like behavioral comorbidities by over-activating brain immune regulation. Thus, there is clear bidirectionality of the brain–gut axis in IBD and psychological stress. Brain–gut interactions could explain the increased prevalence of psychological disorders in IBD patients; however, the pathological changes in the cerebral structures in IBD patients cannot be ignored. Functional brain imaging is a valuable method of investigating the possible neuromechanisms of IBD underlying visceral disturbances. Current findings have suggested that IBD might have a negative impact on cerebral structure networks that manifest as widespread neuroanatomical changes associated with stress. A deeper study of brain structural networks in IBD patients will help us develop adjuvant therapies to treat extraintestinal comorbid conditions, such as depression or anxiety.
Although we are now starting to understand the underlying mechanism of the link between psychological stress and IBD, we do not know how often psychological disorders co-occur with IBD, nor do we know the causal relationship between them. Despite the current study is limited, fortunately microbiome manipulations by using fecal microbiota transplantation or administration of microbial metabolites or probiotics can target specific pathways in IBD pathogenesis and promise to play a crucial role in the treatment of IBD and its concomitant psychiatric disorders. The development of microbiota-targeted treatment strategies will change the disease course and improve the mental health and QoL of patients with IBD (133, 134, 136, 138, 222, 223). Futhermore, psychotherapy and antidepressants have also become promising areas. Until now there is not enough evidence to prove that psychotherapy and antidepressants are effective, however, the early intervention of the designed personalized psychotherapy and appropriate use of antidepressants will be expected to improve coping strategies and QoL scores for IBD patients in the future. Additonally, current studies have also largely relied on murine models, and validation using other advanced preclinical IBD models and IBD patients is needed. Comprehensive clinical studies with large sample sizes combined with more high-quality research are needed to create personalized treatment strategies for IBD patients. In conclusion, there is increasing evidence that the brain–gut axis regulates disease progression.Translating these discoveries into psychoneuroimmunology will be essential for clarifying the pathological mechanism of IBD with psychological comorbidities and designing clinical treatment protocols.
Author contributions
LG and SuL were involved in writing the manuscript. SL and JY performed the majority of literature retrieval work. GH designed the tables and figures. CX and WS were responsible for proposing the framework and revising the manuscript. All authors contributed to the article and approved the submitted version.
Funding
This work was supported by the National Natural Science Foundation of China (82271803), the Natural Science Foundation of Shandong Province (ZR2019MH123), the Academic Promotion Programme of Shandong First Medical University (2019QL007, YS22-0001817), the Open Project of Shandong Provincial Key Laboratory of Animal Biotechnology and Disease Control and Prevention (ABDC-201901), and the Shandong Provincial Integrated Traditional Chinese and Western Medicine Special Disease Prevention Project (YXH2019ZXY003).
Acknowledgments
The manuscript has been reviewed, and the syntax and grammar have been edited by Charlesworth Author Services. Additionally, we thank Shanghai Sensichip Infotech Co. Ltd, Shanghai, China, for their mapping services.
Conflict of interest
The authors declare that the research was conducted in the absence of any commercial or financial relationships that could be construed as a potential conflict of interest.
Publisher’s note
All claims expressed in this article are solely those of the authors and do not necessarily represent those of their affiliated organizations, or those of the publisher, the editors and the reviewers. Any product that may be evaluated in this article, or claim that may be made by its manufacturer, is not guaranteed or endorsed by the publisher.
References
1. Ungaro R, Mehandru S, Allen PB, Peyrin-Biroulet L, Colombel J-F. Ulcerative colitis. Lancet (2017) 389(10080):1756–70. doi: 10.1016/s0140-6736(16)32126-2
2. Jairath V, Feagan BG. Global burden of inflammatory bowel disease. Lancet Gastroenterol Hepatol (2020) 5(1):2–3. doi: 10.1016/s2468-1253(19)30358-9
3. Amoroso C, Perillo F, Strati F, Fantini MC, Caprioli F, Facciotti F. The role of gut microbiota biomodulators on mucosal immunity and intestinal inflammation. Cells (2020) 9(5):1234. doi: 10.3390/cells9051234
4. Caldera F, Hillman L, Saha S, Wald A, Grimes I, Zhang Y, et al. Immunogenicity of high dose influenza vaccine for patients with inflammatory bowel disease on anti-tnf monotherapy: A randomized clinical trial. Inflammation Bowel Dis (2020) 26(4):593–602. doi: 10.1093/ibd/izz164
5. Ho SM, Lewis JD, Mayer EA, Plevy SE, Chuang E, Rappaport SM, et al. Challenges in ibd research: Environmental triggers. Inflammation Bowel Dis (2019) 25(Suppl 2):S13–23. doi: 10.1093/ibd/izz076
6. McGovern DP, Kugathasan S, Cho JH. Genetics of inflammatory bowel diseases. Gastroenterology (2015) 149(5):1163–76.e2. doi: 10.1053/j.gastro.2015.08.001
7. Jordi SBU, Lang BM, Auschra B, von Kanel R, Biedermann L, Greuter T, et al. Depressive symptoms predict clinical recurrence of inflammatory bowel disease. Inflammation Bowel Dis (2022) 28(4):560–71. doi: 10.1093/ibd/izab136
8. Ng SC, Shi HY, Hamidi N, Underwood FE, Tang W, Benchimol EI, et al. Worldwide incidence and prevalence of inflammatory bowel disease in the 21st century: A systematic review of population-based studies. Lancet (2017) 390(10114):2769–78. doi: 10.1016/s0140-6736(17)32448-0
9. Ng SC, Tang W, Ching JY, Wong M, Chow CM, Hui AJ, et al. Incidence and phenotype of inflammatory bowel disease based on results from the Asia-pacific crohn's and colitis epidemiology study. Gastroenterology (2013) 145(1):158–65.e2. doi: 10.1053/j.gastro.2013.04.007
10. Powell N, Walker MM, Talley NJ. The mucosal immune system: Master regulator of bidirectional gut-brain communications. Nat Rev Gastroenterol Hepatol (2017) 14(3):143–59. doi: 10.1038/nrgastro.2016.191
11. Sudo N, Chida Y, Aiba Y, Sonoda J, Oyama N, Yu XN, et al. Postnatal microbial colonization programs the hypothalamic-Pituitary-Adrenal system for stress response in mice. J Physiol (2004) 558(Pt 1):263–75. doi: 10.1113/jphysiol.2004.063388
12. Skonieczna-Zydecka K, Marlicz W, Misera A, Koulaouzidis A, Loniewski I. Microbiome-the missing link in the gut-brain axis: Focus on its role in gastrointestinal and mental health. J Clin Med (2018) 7(12):521. doi: 10.3390/jcm7120521
13. Duvallet C, Gibbons SM, Gurry T, Irizarry RA, Alm EJ. Meta-analysis of gut microbiome studies identifies disease-specific and shared responses. Nat Commun (2017) 8(1):1784. doi: 10.1038/s41467-017-01973-8
14. Blacher E, Bashiardes S, Shapiro H, Rothschild D, Mor U, Dori-Bachash M, et al. Potential roles of gut microbiome and metabolites in modulating als in mice. Nature (2019) 572(7770):474–80. doi: 10.1038/s41586-019-1443-5
15. Burberry A, Wells MF, Limone F, Couto A, Smith KS, Keaney J, et al. C9orf72 suppresses systemic and neural inflammation induced by gut bacteria. Nature (2020) 582(7810):89–94. doi: 10.1038/s41586-020-2288-7
16. Chen T, Wang R, Duan Z, Yuan X, Ding Y, Feng Z, et al. Akkermansia muciniphila protects against psychological disorder-induced gut microbiota-mediated colonic mucosal barrier damage and aggravation of colitis. Front Cell Infect Microbiol (2021) 11:723856. doi: 10.3389/fcimb.2021.723856
17. Gao X, Cao Q, Cheng Y, Zhao D, Wang Z, Yang H, et al. Chronic stress promotes colitis by disturbing the gut microbiota and triggering immune system response. Proc Natl Acad Sci U.S.A. (2018) 115(13):E2960–E9. doi: 10.1073/pnas.1720696115
18. Komoto M, Asada A, Ohshima Y, Miyanaga K, Morimoto H, Yasukawa T, et al. Dextran sulfate sodium-induced colitis in C57bl/6j mice increases their susceptibility to chronic unpredictable mild stress that induces depressive-like behavior. Life Sci (2022) 289:120217. doi: 10.1016/j.lfs.2021.120217
19. Seifi M, Rodaway S, Rudolph U, Swinny JD. Gabaa receptor subtypes regulate stress-induced colon inflammation in mice. Gastroenterology (2018) 155(3):852–64.e3. doi: 10.1053/j.gastro.2018.05.033
20. Mikocka-Walus A, Pittet V, Rossel JB, von Kanel R, Swiss IBDCSG. Symptoms of depression and anxiety are independently associated with clinical recurrence of inflammatory bowel disease. Clin Gastroenterol Hepatol (2016) 14(6):829–35.e1. doi: 10.1016/j.cgh.2015.12.045
21. Sun Y, Li L, Xie R, Wang B, Jiang K, Cao H. Stress triggers flare of inflammatory bowel disease in children and adults. Front Pediatr (2019) 7:432. doi: 10.3389/fped.2019.00432
22. Barberio B, Zamani M, Black CJ, Savarino EV, Ford AC. Prevalence of symptoms of anxiety and depression in patients with inflammatory bowel disease: A systematic review and meta-analysis. Lancet Gastroenterol Hepatol (2021) 6(5):359–70. doi: 10.1016/s2468-1253(21)00014-5
23. Bernstein CN, Hitchon CA, Walld R, Bolton JM, Sareen J, Walker JR, et al. Increased burden of psychiatric disorders in inflammatory bowel disease. Inflammation Bowel Dis (2019) 25(2):360–8. doi: 10.1093/ibd/izy235
24. Neuendorf R, Harding A, Stello N, Hanes D, Wahbeh H. Depression and anxiety in patients with inflammatory bowel disease: A systematic review. J Psychosom Res (2016) 87:70–80. doi: 10.1016/j.jpsychores.2016.06.001
25. Dinan TG, Cryan JF. The microbiome-Gut-Brain axis in health and disease. Gastroenterol Clin North Am (2017) 46(1):77–89. doi: 10.1016/j.gtc.2016.09.007
26. Gracie DJ, Guthrie EA, Hamlin PJ, Ford AC. Bi-directionality of brain-gut interactions in patients with inflammatory bowel disease. Gastroenterology (2018) 154(6):1635–46.e3. doi: 10.1053/j.gastro.2018.01.027
27. Conti C, Rosa I, Zito L, Grossi L, Efthymakis K, Neri M, et al. Influence of the covid-19 outbreak on disease activity and quality of life in inflammatory bowel disease patients. Front Psychiatry (2021) 12:664088. doi: 10.3389/fpsyt.2021.664088
28. Mawdsley JE, Rampton DS. Psychological stress in ibd: New insights into pathogenic and therapeutic implications. Gut (2005) 54(10):1481–91. doi: 10.1136/gut.2005.064261
29. Labanski A, Langhorst J, Engler H, Elsenbruch S. Stress and the brain-gut axis in functional and chronic-inflammatory gastrointestinal diseases: A transdisciplinary challenge. Psychoneuroendocrinology (2020) 111:104501. doi: 10.1016/j.psyneuen.2019.104501
30. Tsigos C, Chrousos GP. Hypothalamic-Pituitary-Adrenal axis, neuroendocrine factors and stress. J Psychosom Res (2002) 53(4):865–71. doi: 10.1016/s0022-3999(02)00429-4
31. Ulrich-Lai YM, Herman JP. Neural regulation of endocrine and autonomic stress responses. Nat Rev Neurosci (2009) 10(6):397–409. doi: 10.1038/nrn2647
32. Tache Y, Larauche M, Yuan PQ, Million M. Brain and gut crf signaling: Biological actions and role in the gastrointestinal tract. Curr Mol Pharmacol (2018) 11(1):51–71. doi: 10.2174/1874467210666170224095741
33. Li B, Lee C, Filler T, Hock A, Wu RY, Li Q, et al. Inhibition of corticotropin-releasing hormone receptor 1 and activation of receptor 2 protect against colonic injury and promote epithelium repair. Sci Rep (2017) 7:46616. doi: 10.1038/srep46616
34. Hill LT, Kidson SH, Michell WL. Corticotropin-releasing factor: A possible key to gut dysfunction in the critically ill. Nutrition (2013) 29(7-8):948–52. doi: 10.1016/j.nut.2012.12.023
35. Zheng PY, Feng BS, Oluwole C, Struiksma S, Chen X, Li P, et al. Psychological stress induces eosinophils to produce corticotrophin releasing hormone in the intestine. Gut (2009) 58(11):1473–9. doi: 10.1136/gut.2009.181701
36. Bauguil SC, Cousin B, Bour S, Casteilla L, Penicaud L, Carpéné C. Adipose tissue lymphocytes: Types and roles. J Physiol Biochem (2009) 65(4):423–36. doi: 10.1007/BF03185938
37. Vanuytsel T, van Wanrooy S, Vanheel H, Vanormelingen C, Verschueren S, Houben E, et al. Psychological stress and corticotropin-releasing hormone increase intestinal permeability in humans by a mast cell-dependent mechanism. Gut (2014) 63(8):1293–9. doi: 10.1136/gutjnl-2013-305690
38. Xu W, Lu J, Chen Y, Wang Z, Cao J, Dong Y. Impairment of crh in the intestinal mucosal epithelial barrier of pregnant bama miniature pig induced by restraint stress. Endocr J (2021) 68(4):485–502. doi: 10.1507/endocrj.EJ20-0332
39. Zheng G, Victor Fon G, Meixner W, Creekmore A, Zong Y, KD M, et al. Chronic stress and intestinal barrier dysfunction: Glucocorticoid receptor and transcription repressor Hes1 regulate tight junction protein claudin-1 promoter. Sci Rep (2017) 7(1):4502. doi: 10.1038/s41598-017-04755-w
40. Zong Y, Zhu S, Zhang S, Zheng G, Wiley JW, Hong S. Chronic stress and intestinal permeability: Lubiprostone regulates glucocorticoid receptor-mediated changes in colon epithelial tight junction proteins, barrier function, and visceral pain in the rodent and human. Neurogastroenterol Motil (2019) 31(2):e13477. doi: 10.1111/nmo.13477
41. Johnson JD, Barnard DF, Kulp AC, Mehta DM. Neuroendocrine regulation of brain cytokines after psychological stress. J Endocr Soc (2019) 3(7):1302–20. doi: 10.1210/js.2019-00053
42. Goyal RK, Hirano I. The enteric nervous system. N Engl J Med (1996) 334(17):1106–15. doi: 10.1056/NEJM199604253341707
43. Barreau F, Salvador-Cartier C, Houdeau E, Bueno L, Fioramonti J. Long-term alterations of colonic nerve-mast cell interactions induced by neonatal maternal deprivation in rats. Gut (2008) 57(5):582–90. doi: 10.1136/gut.2007.126680
44. Gareau MG, Jury J, Perdue MH. Neonatal maternal separation of rat pups results in abnormal cholinergic regulation of epithelial permeability. Am J Physiol Gastrointest Liver Physiol (2007) 293(1):G198–203. doi: 10.1152/ajpgi.00392.2006
45. Villanacci V, Bassotti G, Nascimbeni R, Antonelli E, Cadei M, Fisogni S, et al. Enteric nervous system abnormalities in inflammatory bowel diseases. Neurogastroenterol Motil (2008) 20(9):1009–16. doi: 10.1111/j.1365-2982.2008.01146.x
46. Bonaz B, Sinniger V, Pellissier S. Therapeutic potential of vagus nerve stimulation for inflammatory bowel diseases. Front Neurosci (2021) 15:650971. doi: 10.3389/fnins.2021.650971
47. Eberhardson M, Hedin CRH, Carlson M, Tarnawski L, Levine YA, Olofsson PS. Towards improved control of inflammatory bowel disease. Scand J Immunol (2019) 89(3):e12745. doi: 10.1111/sji.12745
48. Meroni E, Stakenborg N, Gomez-Pinilla PJ, Stakenborg M, Aguilera-Lizarraga J, Florens M, et al. Vagus nerve stimulation promotes epithelial proliferation and controls colon monocyte infiltration during dss-induced colitis. Front Med (Lausanne) (2021) 8:694268. doi: 10.3389/fmed.2021.694268
49. Pellissier S, Dantzer C, Mondillon L, Trocme C, Gauchez AS, Ducros V, et al. Relationship between vagal tone, cortisol, tnf-alpha, epinephrine and negative affects in crohn's disease and irritable bowel syndrome. PloS One (2014) 9(9):e105328. doi: 10.1371/journal.pone.0105328
50. Teratani T, Mikami Y, Nakamoto N, Suzuki T, Harada Y, Okabayashi K, et al. The liver-Brain-Gut neural arc maintains the treg cell niche in the gut. Nature (2020) 585(7826):591–6. doi: 10.1038/s41586-020-2425-3
51. Breit S, Kupferberg A, Rogler G, Hasler G. Vagus nerve as modulator of the brain-gut axis in psychiatric and inflammatory disorders. Front Psychiatry (2018) 9:44. doi: 10.3389/fpsyt.2018.00044
52. Kolacz J, Kovacic KK, Porges SW. Traumatic stress and the autonomic brain-gut connection in development: Polyvagal theory as an integrative framework for psychosocial and gastrointestinal pathology. Dev Psychobiol (2019) 61(5):796–809. doi: 10.1002/dev.21852
53. Hiles SA, Baker AL, de Malmanche T, Attia J. A meta-analysis of differences in il-6 and il-10 between people with and without depression: Exploring the causes of heterogeneity. Brain Behav Immun (2012) 26(7):1180–8. doi: 10.1016/j.bbi.2012.06.001
54. Kohler CA, Freitas TH, Maes M, de Andrade NQ, Liu CS, Fernandes BS, et al. Peripheral cytokine and chemokine alterations in depression: A meta-analysis of 82 studies. Acta Psychiatr Scand (2017) 135(5):373–87. doi: 10.1111/acps.12698
55. Gunterberg V, Simren M, Ohman L, Friberg P, Jones MP, Van Oudenhove L, et al. Autonomic nervous system function predicts the inflammatory response over three years in newly diagnosed ulcerative colitis patients. Neurogastroenterol Motil (2016) 28(11):1655–62. doi: 10.1111/nmo.12865
56. Mikocka-Walus A, Knowles SR, Keefer L, Graff L. Controversies revisited: A systematic review of the comorbidity of depression and anxiety with inflammatory bowel diseases. Inflammation Bowel Dis (2016) 22(3):752–62. doi: 10.1097/MIB.0000000000000620
57. Abbott A. The inflamed mind: A radical new approach to depression. Nature (2018) 557(May 31 TN.7707):633–4. doi: 10.1038/d41586-018-05261-3
58. Lin YY, Chang CC, Huang CC, Tzeng NS, Kao YC, Chang HA. Efficacy and neurophysiological predictors of treatment response of adjunct bifrontal transcranial direct current stimulation (Tdcs) in treating unipolar and bipolar depression. J Affect Disord (2021) 280(Pt A):295–304. doi: 10.1016/j.jad.2020.11.030
59. Carrington EV, Evers J, Grossi U, Dinning PG, Scott SM, O'Connell PR, et al. A systematic review of sacral nerve stimulation mechanisms in the treatment of fecal incontinence and constipation. Neurogastroenterol Motil (2014) 26(9):1222–37. doi: 10.1111/nmo.12388
60. Kibleur A, Pellissier S, Sinniger V, Robert J, Gronlier E, Clarencon D, et al. Electroencephalographic correlates of low-frequency vagus nerve stimulation therapy for crohn's disease. Clin Neurophysiol (2018) 129(5):1041–6. doi: 10.1016/j.clinph.2018.02.127
61. Payne SC, Furness JB, Burns O, Sedo A, Hyakumura T, Shepherd RK, et al. Anti-inflammatory effects of abdominal vagus nerve stimulation on experimental intestinal inflammation. Front Neurosci (2019) 13:418. doi: 10.3389/fnins.2019.00418
62. Meroni E, Stakenborg N, Gomez-Pinilla PJ, De Hertogh G, Goverse G, Matteoli G, et al. Functional characterization of oxazolone-induced colitis and survival improvement by vagus nerve stimulation. PloS One (2018) 13(5):e0197487. doi: 10.1371/journal.pone.0197487
63. Jin H, Guo J, Liu J, Lyu B, Foreman RD, Yin J, et al. Anti-inflammatory effects and mechanisms of vagal nerve stimulation combined with electroacupuncture in a rodent model of tnbs-induced colitis. Am J Physiol Gastrointest Liver Physiol (2017) 313(3):G192–202. doi: 10.1152/ajpgi.00254.2016
64. Hu D, Wan L, Chen M, Caudle Y, LeSage G, Li Q, et al. Essential role of il-10/Stat3 in chronic stress-induced immune suppression. Brain Behav Immun (2014) 36:118–27. doi: 10.1016/j.bbi.2013.10.016
65. Li C, Li H, Jiang K, Li J, Gai X. Tlr4 signaling pathway in mouse Lewis lung cancer cells promotes the expression of tgf-Beta1 and il-10 and tumor cells migration. BioMed Mater Eng (2014) 24(1):869–75. doi: 10.3233/BME-130879
66. Geremia A, Biancheri P, Allan P, Corazza GR, Di Sabatino A. Innate and adaptive immunity in inflammatory bowel disease. Autoimmun Rev (2014) 13(1):3–10. doi: 10.1016/j.autrev.2013.06.004
67. Ray K. Immunopathogenesis of ibd: Current state of the art. Nat Rev Gastroenterol Hepatol (2017) 14(1):3. doi: 10.1038/nrgastro.2016.186
68. Soderholm JD, Yang PC, Ceponis P, Vohra A, Riddell R, Sherman PM, et al. Chronic stress induces mast cell-dependent bacterial adherence and initiates mucosal inflammation in rat intestine. Gastroenterology (2002) 123(4):1099–108. doi: 10.1053/gast.2002.36019
69. Mackey E, Ayyadurai S, Pohl CS, DC S, Li Y, Moeser AJ. Sexual dimorphism in the mast cell transcriptome and the pathophysiological responses to immunological and psychological stress. Biol Sex Differ (2016) 7:60. doi: 10.1186/s13293-016-0113-7
70. Xu S, Wang X, Zhao J, Yang S, Dong L, Qin B. Gper-mediated, oestrogen-dependent visceral hypersensitivity in stressed rats is associated with mast cell tryptase and histamine expression. Fundam Clin Pharmacol (2020) 34(4):433–43. doi: 10.1111/fcp.12537
71. Lennon EM, Maharshak N, Elloumi H, Borst L, Plevy SE, Moeser AJ. Early life stress triggers persistent colonic barrier dysfunction and exacerbates colitis in adult il-10-/- mice. Inflammation Bowel Dis (2013) 19(4):712–9. doi: 10.1097/MIB.0b013e3182802a4e
72. Mackos AR, Galley JD, Eubank TD, Easterling RS, Parry NM, Fox JG, et al. Social stress-enhanced severity of citrobacter rodentium-induced colitis is Ccl2-dependent and attenuated by probiotic lactobacillus reuteri. Mucosal Immunol (2016) 9(2):515–26. doi: 10.1038/mi.2015.81
73. Wang SL, Shao BZ, Zhao SB, Chang X, Wang P, Miao CY, et al. Intestinal autophagy links psychosocial stress with gut microbiota to promote inflammatory bowel disease. Cell Death Dis (2019) 10(6):391. doi: 10.1038/s41419-019-1634-x
74. Tang Y, Zhao L, Lei N, Chen P, Zhang Y. Crohn's disease patients with depression exhibit alterations in Monocyte/Macrophage phenotype and increased proinflammatory cytokine production. Dig Dis (2020) 38(3):211–21. doi: 10.1159/000501122
75. Zheng X, Hu M, Zang X, Fan Q, Liu Y, Che Y, et al. Kynurenic Acid/Gpr35 axis restricts Nlrp3 inflammasome activation and exacerbates colitis in mice with social stress. Brain Behav Immun (2019) 79:244–55. doi: 10.1016/j.bbi.2019.02.009
76. Wu W, Sun M, Zhang HP, Chen T, Wu R, Liu C, et al. Prolactin mediates psychological stress-induced dysfunction of regulatory T cells to facilitate intestinal inflammation. Gut (2014) 63(12):1883–92. doi: 10.1136/gutjnl-2013-306083
77. Ambree O, Ruland C, Zwanzger P, Klotz L, Baune BT, Arolt V, et al. Social defeat modulates T helper cell percentages in stress susceptible and resilient mice. Int J Mol Sci (2019) 20(14):3512. doi: 10.3390/ijms20143512
78. Westfall S, Caracci F, Estill M, Frolinger T, Shen L, Pasinetti GM. Chronic stress-induced depression and anxiety priming modulated by gut-Brain-Axis immunity. Front Immunol (2021) 12:670500. doi: 10.3389/fimmu.2021.670500
79. Westfall S, Caracci F, Zhao D, Wu QL, Frolinger T, Simon J, et al. Microbiota metabolites modulate the T helper 17 to regulatory T cell (Th17/Treg) imbalance promoting resilience to stress-induced anxiety- and depressive-like behaviors. Brain Behav Immun (2021) 91:350–68. doi: 10.1016/j.bbi.2020.10.013
80. Deng Q, Chen H, Liu Y, Xiao F, Guo L, Liu D, et al. Psychological stress promotes neutrophil infiltration in colon tissue through adrenergic signaling in dss-induced colitis model. Brain Behav Immun (2016) 57:243–54. doi: 10.1016/j.bbi.2016.04.017
81. Ballout J, Diener M. Interactions between rat submucosal neurons and mast cells are modified by cytokines and neurotransmitters. Eur J Pharmacol (2019) 864:172713. doi: 10.1016/j.ejphar.2019.172713
82. De Zuani M, Dal Secco C, Frossi B. Mast cells at the crossroads of microbiota and ibd. Eur J Immunol (2018) 48(12):1929–37. doi: 10.1002/eji.201847504
83. Lissner D, Schumann M, Batra A, Kredel LI, Kuhl AA, Erben U, et al. Monocyte and M1 macrophage-induced barrier defect contributes to chronic intestinal inflammation in ibd. Inflammation Bowel Dis (2015) 21(6):1297–305. doi: 10.1097/MIB.0000000000000384
84. Xu YW, Xing RX, Zhang WH, Li L, Wu Y, Hu J, et al. Toxoplasma Rop16i/Iii ameliorated inflammatory bowel diseases Via inducing M2 phenotype of macrophages. World J Gastroenterol (2019) 25(45):6634–52. doi: 10.3748/wjg.v25.i45.6634
85. Li MO, Rudensky AY. T Cell receptor signalling in the control of regulatory T cell differentiation and function. Nat Rev Immunol (2016) 16(4):220–33. doi: 10.1038/nri.2016.26
86. Britton GJ, Contijoch EJ, Mogno I, Vennaro OH, Llewellyn SR, Ng R, et al. Microbiotas from humans with inflammatory bowel disease alter the balance of gut Th17 and rorgammat(+) regulatory T cells and exacerbate colitis in mice. Immunity (2019) 50(1):212–24.e4. doi: 10.1016/j.immuni.2018.12.015
87. Zheng K, Jia J, Yan S, Shen H, Zhu P, Yu J. Paeoniflorin ameliorates ulcerative colitis by modulating the dendritic cell-mediated Th17/Treg balance. Inflammopharmacology (2020) 28(6):1705–16. doi: 10.1007/s10787-020-00722-6
88. Li J, Ueno A, Iacucci M, Fort Gasia M, Jijon HB, Panaccione R, et al. Crossover subsets of Cd4(+) T lymphocytes in the intestinal lamina propria of patients with crohn's disease and ulcerative colitis. Dig Dis Sci (2017) 62(9):2357–68. doi: 10.1007/s10620-017-4596-9
89. Wang Y, Balvers MGJ, Hendriks HFJ, Wilpshaar T, van Heek T, Witkamp RF, et al. Docosahexaenoyl serotonin emerges as most potent inhibitor of il-17 and ccl-20 released by blood mononuclear cells from a series of n-acyl serotonins identified in human intestinal tissue. Biochim Biophys Acta Mol Cell Biol Lipids (2017) 1862(9):823–31. doi: 10.1016/j.bbalip.2017.05.008
90. Poland M, Ten Klooster JP, Wang Z, Pieters R, Boekschoten M, Witkamp R, et al. Docosahexaenoyl serotonin, an endogenously formed n-3 fatty acid-serotonin conjugate has anti-inflammatory properties by attenuating il-23-Il-17 signaling in macrophages. Biochim Biophys Acta (2016) 1861(12 Pt A):2020–8. doi: 10.1016/j.bbalip.2016.09.012
91. Melnikov M, Sviridova A, Rogovskii V, Oleskin A, Boziki M, Bakirtzis C, et al. Serotoninergic system targeting in multiple sclerosis: The prospective for pathogenetic therapy. Mult Scler Relat Disord (2021) 51:102888. doi: 10.1016/j.msard.2021.102888
92. Beurel E, Medina-Rodriguez EM, Jope RS. Targeting the adaptive immune system in depression: Focus on T helper 17 cells. Pharmacol Rev (2022) 74(2):373–86. doi: 10.1124/pharmrev.120.000256
93. Wan M, Ding L, Wang D, Han J, Gao P. Serotonin: A potent immune cell modulator in autoimmune diseases. Front Immunol (2020) 11:186. doi: 10.3389/fimmu.2020.00186
94. Lee Y, Awasthi A, Yosef N, Quintana FJ, Xiao S, Peters A, et al. Induction and molecular signature of pathogenic Th17 cells. Nat Immunol (2012) 13(10):991–9. doi: 10.1038/ni.2416
95. Burkett PR, Meyer zu Horste G, Kuchroo VK. Pouring fuel on the fire: Th17 cells, the environment, and autoimmunity. J Clin Invest (2015) 125(6):2211–9. doi: 10.1172/JCI78085
96. Yang XO, Panopoulos AD, Nurieva R, Chang SH, Wang D, Watowich SS, et al. Stat3 regulates cytokine-mediated generation of inflammatory helper T cells. J Biol Chem (2007) 282(13):9358–63. doi: 10.1074/jbc.C600321200
97. Milovanovic J, Arsenijevic A, Stojanovic B, Kanjevac T, Arsenijevic D, Radosavljevic G, et al. Interleukin-17 in chronic inflammatory neurological diseases. Front Immunol (2020) 11:947. doi: 10.3389/fimmu.2020.00947
98. Chiu YC, Yang BH, Yang KC, Liu MN, Hu LY, Liou YJ, et al. A study of tryptophan, kynurenine and serotonin transporter in first-episode drug-naive major depressive disorder. Psychiatry Res Neuroimaging (2021) 312:111296. doi: 10.1016/j.pscychresns.2021.111296
99. Moser T, Akgun K, Proschmann U, Sellner J, Ziemssen T. The role of Th17 cells in multiple sclerosis: Therapeutic implications. Autoimmun Rev (2020) 19(10):102647. doi: 10.1016/j.autrev.2020.102647
100. Schinocca C, Rizzo C, Fasano S, Grasso G, La Barbera L, Ciccia F, et al. Role of the il-23/Il-17 pathway in rheumatic diseases: An overview. Front Immunol (2021) 12:637829. doi: 10.3389/fimmu.2021.637829
101. Reboldi A, Coisne C, Baumjohann D, Benvenuto F, Bottinelli D, Lira S, et al. C-c chemokine receptor 6-regulated entry of Th-17 cells into the cns through the choroid plexus is required for the initiation of eae. Nat Immunol (2009) 10(5):514–23. doi: 10.1038/ni.1716
102. Balasa R, Barcutean L, Balasa A, Motataianu A, Roman-Filip C, Manu D. The action of Th17 cells on blood brain barrier in multiple sclerosis and experimental autoimmune encephalomyelitis. Hum Immunol (2020) 81(5):237–43. doi: 10.1016/j.humimm.2020.02.009
103. Li N, Wang Q, Wang Y, Sun A, Lin Y, Jin Y, et al. Fecal microbiota transplantation from chronic unpredictable mild stress mice donors affects anxiety-like and depression-like behavior in recipient mice Via the gut microbiota-Inflammation-Brain axis. Stress (2019) 22(5):592–602. doi: 10.1080/10253890.2019.1617267
104. Bailey MT, Dowd SE, Galley JD, Hufnagle AR, Allen RG, Lyte M. Exposure to a social stressor alters the structure of the intestinal microbiota: Implications for stressor-induced immunomodulation. Brain Behav Immun (2011) 25(3):397–407. doi: 10.1016/j.bbi.2010.10.023
105. Cristofori F, Dargenio VN, Dargenio C, Miniello VL, Barone M, Francavilla R. Anti-inflammatory and immunomodulatory effects of probiotics in gut inflammation: A door to the body. Front Immunol (2021) 12:578386. doi: 10.3389/fimmu.2021.578386
106. Adamantidis A. How the gut talks to the brain. Science (2022) 376(6590):248–9. doi: 10.1126/science.abo7933
107. Cryan JF, O'Riordan KJ, Cowan CSM, Sandhu KV, Bastiaanssen TFS, Boehme M, et al. The microbiota-Gut-Brain axis. Physiol Rev (2019) 99(4):1877–2013. doi: 10.1152/physrev.00018.2018
108. Morais LH, HLt S, Mazmanian SK. The gut microbiota-brain axis in behaviour and brain disorders. Nat Rev Microbiol (2021) 19(4):241–55. doi: 10.1038/s41579-020-00460-0
109. Bailey MT, Dowd SE, Parry NM, Galley JD, Schauer DB, Lyte M. Stressor exposure disrupts commensal microbial populations in the intestines and leads to increased colonization by citrobacter rodentium. Infect Immun (2010) 78(4):1509–19. doi: 10.1128/IAI.00862-09
110. Konturek PC, Konturek K, Brzozowski T, Wojcik D, Magierowski M, Targosz A, et al. Participation of the intestinal microbiota in the mechanism of beneficial effect of treatment with synbiotic syngut on experimental colitis under stress conditions. J Physiol Pharmacol (2020) 71(3):329–42. doi: 10.26402/jpp.2020.3.03
111. Jang HM, Lee KE, Kim DH. The preventive and curative effects of lactobacillus reuteri Nk33 and bifidobacterium adolescentis Nk98 on immobilization stress-induced Anxiety/Depression and colitis in mice. Nutrients (2019) 11(4):819. doi: 10.3390/nu11040819
112. Kim JK, Han SK, Joo MK, Kim DH. Buspirone alleviates anxiety, depression, and colitis; and modulates gut microbiota in mice. Sci Rep (2021) 11(1):6094. doi: 10.1038/s41598-021-85681-w
113. Reber SO, Siebler PH, Donner NC, Morton JT, Smith DG, Kopelman JM, et al. Immunization with a heat-killed preparation of the environmental bacterium mycobacterium vaccae promotes stress resilience in mice. Proc Natl Acad Sci U.S.A. (2016) 113(22):E3130–9. doi: 10.1073/pnas.1600324113
114. Watanabe Y, Arase S, Nagaoka N, Kawai M, Matsumoto S. Chronic psychological stress disrupted the composition of the murine colonic microbiota and accelerated a murine model of inflammatory bowel disease. PloS One (2016) 11(3):e0150559. doi: 10.1371/journal.pone.0150559
115. Arase S, Watanabe Y, Setoyama H, Nagaoka N, Kawai M, Matsumoto S. Disturbance in the mucosa-associated commensal bacteria is associated with the exacerbation of chronic colitis by repeated psychological stress; is that the new target of probiotics? PloS One (2016) 11(8):e0160736. doi: 10.1371/journal.pone.0160736
116. Sun Y, Xie R, Li L, Jin G, Zhou B, Huang H, et al. Prenatal maternal stress exacerbates experimental colitis of offspring in adulthood. Front Immunol (2021) 12:700995. doi: 10.3389/fimmu.2021.700995
117. Zijlmans MA, Korpela K, Riksen-Walraven JM, de Vos WM, de Weerth C. Maternal prenatal stress is associated with the infant intestinal microbiota. Psychoneuroendocrinology (2015) 53:233–45. doi: 10.1016/j.psyneuen.2015.01.006
118. Yuan X, Chen B, Duan Z, Xia Z, Ding Y, Chen T, et al. Depression and anxiety in patients with active ulcerative colitis: Crosstalk of gut microbiota, metabolomics and proteomics. Gut Microbes (2021) 13(1):1987779. doi: 10.1080/19490976.2021.1987779
119. He XX, Li YH, Yan PG, Meng XC, Chen CY, Li KM, et al. Relationship between clinical features and intestinal microbiota in Chinese patients with ulcerative colitis. World J Gastroenterol (2021) 27(28):4722–37. doi: 10.3748/wjg.v27.i28.4722
120. Wei L, Li Y, Tang W, Sun Q, Chen L, Wang X, et al. Chronic unpredictable mild stress in rats induces colonic inflammation. Front Physiol (2019) 10:1228. doi: 10.3389/fphys.2019.01228
121. Osadchiy V, Martin CR, Mayer EA. The gut-brain axis and the microbiome: Mechanisms and clinical implications. Clin Gastroenterol Hepatol (2019) 17(2):322–32. doi: 10.1016/j.cgh.2018.10.002
122. Elsenbruch S, Enck P. The stress concept in gastroenterology: From selye to today. F1000Res (2017) 6:2149. doi: 10.12688/f1000research.12435.1
123. Pittayanon R, Lau JT, Yuan Y, Leontiadis GI, Tse F, Surette M, et al. Gut microbiota in patients with irritable bowel syndrome-a systematic review. Gastroenterology (2019) 157(1):97–108. doi: 10.1053/j.gastro.2019.03.049
124. Martin CR, Osadchiy V, Kalani A, Mayer EA. The brain-Gut-Microbiome axis. Cell Mol Gastroenterol Hepatol (2018) 6(2):133–48. doi: 10.1016/j.jcmgh.2018.04.003
125. Kennedy PJ, Cryan JF, Dinan TG, Clarke G. Kynurenine pathway metabolism and the microbiota-Gut-Brain axis. Neuropharmacology (2017) 112(Pt B):399–412. doi: 10.1016/j.neuropharm.2016.07.002
126. Jang HM, Kim JK, Joo MK, Shin YJ, Lee CK, Kim HJ, et al. Transplantation of fecal microbiota from patients with inflammatory bowel disease and depression alters immune response and behavior in recipient mice. Sci Rep (2021) 11(1):20406. doi: 10.1038/s41598-021-00088-x
127. Humbel F, Rieder JH, Franc Y, Juillerat P, Scharl M, Misselwitz B, et al. Association of alterations in intestinal microbiota with impaired psychological function in patients with inflammatory bowel diseases in remission. Clin Gastroenterol Hepatol (2020) 18(9):2019–29.e11. doi: 10.1016/j.cgh.2019.09.022
128. Lo Presti A, Zorzi F, Del Chierico F, Altomare A, Cocca S, Avola A, et al. Fecal and mucosal microbiota profiling in irritable bowel syndrome and inflammatory bowel disease. Front Microbiol (2019) 10:1655. doi: 10.3389/fmicb.2019.01655
129. Kowalska-Duplaga K, Gosiewski T, Kapusta P, Sroka-Oleksiak A, Wedrychowicz A, Pieczarkowski S, et al. Differences in the intestinal microbiome of healthy children and patients with newly diagnosed crohn's disease. Sci Rep (2019) 9(1):18880. doi: 10.1038/s41598-019-55290-9
130. Liu YJ, Tang B, Wang FC, Tang L, Lei YY, Luo Y, et al. Parthenolide ameliorates colon inflammation through regulating Treg/Th17 balance in a gut microbiota-dependent manner. Theranostics (2020) 10(12):5225–41. doi: 10.7150/thno.43716
131. van der Beek CM, Dejong CHC, Troost FJ, Masclee AAM, Lenaerts K. Role of short-chain fatty acids in colonic inflammation, carcinogenesis, and mucosal protection and healing. Nutr Rev (2017) 75(4):286–305. doi: 10.1093/nutrit/nuw067
132. Louis P, Young P, Holtrop G, Flint HJ. Diversity of human colonic butyrate-producing bacteria revealed by analysis of the butyryl-Coa:Acetate coa-transferase gene. Environ Microbiol (2010) 12(2):304–14. doi: 10.1111/j.1462-2920.2009.02066.x
133. Sokol H, Landman C, Seksik P, Berard L, Montil M, Nion-Larmurier I, et al. Fecal microbiota transplantation to maintain remission in crohn's disease: A pilot randomized controlled study. Microbiome (2020) 8(1):12. doi: 10.1186/s40168-020-0792-5
134. Kilinçarslan S, Evrensel A. The effect of fecal microbiota transplantation on psychiatric symptoms among patients with inflammatory bowel disease: An experimental study. Actas Esp Psiquiatr (2020) 48(1):1–7.
135. Wang H, Braun C, Enck P. Effects of rifaximin on central responses to social stress-a pilot experiment. Neurotherapeutics (2018) 15(3):807–18. doi: 10.1007/s13311-018-0627-2
136. van de Wouw M, Boehme M, Lyte JM, Wiley N, Strain C, O'Sullivan O, et al. Short-chain fatty acids: Microbial metabolites that alleviate stress-induced brain-gut axis alterations. J Physiol (2018) 596(20):4923–44. doi: 10.1113/JP276431
137. Kataoka AK, Nishida K, Takada M, Kawai M, Hayakawa HK, Suda K, et al. Fermented milk containing lactobacillus casei strain shirota preserves the diversity of the gut microbiota and relieves abdominal dysfunction in healthy medical students exposed to academic stress. Appl Environ Microbiol (2016) 82(12):3649–58. doi: 10.1128/AEM.04134-15
138. Yoo JW, Shin YJ, Ma X, Son YH, Jang HM, Lee CK, et al. The alleviation of gut microbiota-induced depression and colitis in mice by anti-inflammatory probiotics Nk151, Nk173, and Nk175. Nutrients (2022) 14(10):2080. doi: 10.3390/nu14102080
139. Fairbrass KM, Lovatt J, Barberio B, Yuan Y, Gracie DJ, Ford AC. Bidirectional brain-gut axis effects influence mood and prognosis in ibd: A systematic review and meta-analysis. Gut (2022) 71(9):1773–80. doi: 10.1136/gutjnl-2021-325985
140. Bhamre R, Sawrav S, Adarkar S, Sakaria R, S JB. Psychiatric comorbidities in patients with inflammatory bowel disease. Indian J Gastroenterol (2018) 37(4):307–12. doi: 10.1007/s12664-018-0870-9
141. Byrne G, Rosenfeld G, Leung Y, Qian H, Raudzus J, Nunez C, et al. Prevalence of anxiety and depression in patients with inflammatory bowel disease. Can J Gastroenterol Hepatol (2017) 2017:6496727. doi: 10.1155/2017/6496727
142. Vigano CA, Beltrami MM, Bosi MF, Zanello R, Valtorta M, Maconi G. Alexithymia and psychopathology in patients suffering from inflammatory bowel disease: Arising differences and correlations to tailoring therapeutic strategies. Front Psychiatry (2018) 9:324. doi: 10.3389/fpsyt.2018.00324
143. Frenkel S, Bernstein CN, Sargent M, Jiang W, Kuang Q, Xu W, et al. Copy number variation-based gene set analysis reveals cytokine signalling pathways associated with psychiatric comorbidity in patients with inflammatory bowel disease. Genomics (2020) 112(1):683–93. doi: 10.1016/j.ygeno.2019.05.001
144. Abautret-Daly A, Dempsey E, Riestra S, de Francisco-Garcia R, Parra-Blanco A, Rodrigo L, et al. Association between psychological measures with inflammatory anddisease-related markers of inflammatory bowel disease. Int J Psychiatry Clin Pract (2017) 21(3):221–30. doi: 10.1080/13651501.2017.1306081
145. Craig CF, Filippone RT, Stavely R, Bornstein JC, Apostolopoulos V, Nurgali K. Neuroinflammation as an etiological trigger for depression comorbid with inflammatory bowel disease. J Neuroinflamm (2022) 19(1):4. doi: 10.1186/s12974-021-02354-1
146. Wang K, Yuan CP, Wang W, Yang ZQ, Cui W, Mu LZ, et al. Expression of interleukin 6 in brain and colon of rats with tnbs-induced colitis. World J Gastroenterol (2010) 16(18):2252–9. doi: 10.3748/wjg.v16.i18.2252
147. Riazi K, Galic MA, Kuzmiski JB, Ho W, Sharkey KA, Pittman QJ. Microglial activation and tnf-A production mediate altered cns excitability following peripheral inflammation. Proc Natl Acad Sci U.S.A. (2008) 105(44):17151–6. doi: 10.1073/pnas.0806682105
148. Jang SE, Lim SM, Jeong JJ, Jang HM, Lee HJ, Han MJ, et al. Gastrointestinal inflammation by gut microbiota disturbance induces memory impairment in mice. Mucosal Immunol (2018) 11(2):369–79. doi: 10.1038/mi.2017.49
149. Zonis S, Pechnick RN, Ljubimov VA, Mahgerefteh M, Wawrowsky K, Michelsen KS, et al. Chronic intestinal inflammation alters hippocampal neurogenesis. J Neuroinflamm (2015) 12:65. doi: 10.1186/s12974-015-0281-0
150. Takahashi K, Nakagawasai O, Nemoto W, Odaira T, Sakuma W, Onogi H, et al. Effect of enterococcus faecalis 2001 on colitis and depressive-like behavior in dextran sulfate sodium-treated mice: Involvement of the brain-gut axis. J Neuroinflamm (2019) 16(1):201. doi: 10.1186/s12974-019-1580-7
151. Dempsey E, Abautret-Daly A, Docherty NG, Medina C, Harkin A. Persistent central inflammation and region specific cellular activation accompany depression- and anxiety-like behaviours during the resolution phase of experimental colitis. Brain Behav Immun (2019) 80:616–32. doi: 10.1016/j.bbi.2019.05.007
152. Reichmann F, Hassan AM, Farzi A, Jain P, Schuligoi R, Holzer P. Dextran sulfate sodium-induced colitis alters stress-associated behaviour and neuropeptide gene expression in the amygdala-hippocampus network of mice. Sci Rep (2015) 5:9970. doi: 10.1038/srep09970
153. Zhou F, Jiang H, Kong N, Lin J, Zhang F, Mai T, et al. Electroacupuncture attenuated anxiety and depression-like behavior Via inhibition of hippocampal inflammatory response and metabolic disorders in tnbs-induced ibd rats. Oxid Med Cell Longev (2022) 2022:8295580. doi: 10.1155/2022/8295580
154. Haj-Mirzaian A, Amiri S, Amini-Khoei H, Hosseini MJ, Haj-Mirzaian A, Momeny M, et al. Anxiety- and depressive-like behaviors are associated with altered hippocampal energy and inflammatory status in a mouse model of crohn's disease. Neuroscience (2017) 366:124–37. doi: 10.1016/j.neuroscience.2017.10.023
155. Heydarpour P, Rahimian R, Fakhfouri G, Khoshkish S, Fakhraei N, Salehi-Sadaghiani M, et al. Behavioral despair associated with a mouse model of crohn's disease: Role of nitric oxide pathway. Prog Neuropsychopharmacol Biol Psychiatry (2016) 64:131–41. doi: 10.1016/j.pnpbp.2015.08.004
156. Han Y, Zhao T, Cheng X, Zhao M, Gong SH, Zhao YQ, et al. Cortical inflammation is increased in a dss-induced colitis mouse model. Neurosci Bull (2018) 34(6):9. doi: 10.1007/s12264-018-0288-5
157. Lv WJ, Liu C, Yu LZ, Zhou JH, Li Y, Xiong Y, et al. Melatonin alleviates neuroinflammation and metabolic disorder in dss-induced depression rats. Oxid Med Cell Longev (2020) 2020:1241894. doi: 10.1155/2020/1241894
158. Zhang J, He H, Qiao Y, Zhou T, He H, Yi S, et al. Priming of microglia with ifn-gamma impairs adult hippocampal neurogenesis and leads to depression-like behaviors and cognitive defects. Glia (2020) 68(12):2674–92. doi: 10.1002/glia.23878
159. Kwidzinski E, Bechmann I. Ido expression in the brain: A double-edged sword. J Mol Med (Berl) (2007) 85(12):1351–9. doi: 10.1007/s00109-007-0229-7
160. Carloni S, Bertocchi A, Mancinelli S, Bellini M, Erreni M, Borreca A, et al, et al. Identification of a choroid plexus vascular barrier closing during intestinal inflammation. Science (2021) 374(6566):439–48. doi: 10.1126/science.abc6108
161. Agus A, Planchais J, Sokol H. Gut microbiota regulation of tryptophan metabolism in health and disease. Cell Host Microbe (2018) 23(6):716–24. doi: 10.1016/j.chom.2018.05.003
162. Skrobisz K, Piotrowicz G, Drozdowska A, Markiet K, Sabisz A, Naumczyk P, et al. Use of functional magnetic resonance imaging in patients with irritable bowel syndrome and functional dyspepsia. Prz Gastroenterol (2019) 14(3):163–7. doi: 10.5114/pg.2019.88163
163. Thomann AK, Reindl W, Wustenberg T, Kmuche D, Ebert MP, Szabo K, et al. Aberrant brain structural Large-scale connectome in crohn's disease. Neurogastroenterol Motil (2019) 31(6):e13593. doi: 10.1111/nmo.13593
164. Thomann AK, Schmitgen MM, Kmuche D, Ebert MP, Thomann PA, Szabo K, et al. Exploring joint patterns of brain structure and function in inflammatory bowel diseases using multimodal data fusion. Neurogastroenterol Motil (2021) 33(6):e14078. doi: 10.1111/nmo.14078
165. Tillisch K, Labus JS. Neuroimaging the microbiome-Gut-Brain axis. Adv Exp Med Biol (2014) 817:405–16. doi: 10.1007/978-1-4939-0897-4_18
166. Agostini A, Filippini N, Cevolani D, Agati R, Leoni C, Tambasco R, et al. Brain functional changes in patients with ulcerative colitis: A functional magnetic resonance imaging study on emotional processing. Inflammation Bowel Dis (2011) 17(8):1769–77. doi: 10.1002/ibd.21549
167. Zhang S, Chen F, Wu J, Liu C, Yang G, Piao R, et al. Regional Gray matter volume changes in brains of patients with ulcerative colitis. Inflammation Bowel Dis (2022) 28(4):599–610. doi: 10.1093/ibd/izab252
168. Goodyear BG, Heidari F, Ingram RJM, Cortese F, Sharifi N, Kaplan GG, et al. Multimodal brain mri of deep Gray matter changes associated with inflammatory bowel disease. Inflammation Bowel Dis (2022):izac089. doi: 10.1093/ibd/izac089
169. Wang H, Labus JS, Griffin F, Gupta A, Bhatt RR, Sauk JS, et al. Functional brain rewiring and altered cortical stability in ulcerative colitis. Mol Psychiatry (2022) 27(3):1792–804. doi: 10.1038/s41380-021-01421-6
170. Bao CH, Liu P, Liu HR, Wu LY, Shi Y, Chen WF, et al. Alterations in brain grey matter structures in patients with crohn's disease and their correlation with psychological distress. J Crohns Colitis (2015) 9(7):532–40. doi: 10.1093/ecco-jcc/jjv057
171. Agostini A, Ballotta D, Righi S, Moretti M, Bertani A, Scarcelli A, et al. Stress and brain functional changes in patients with crohn's disease: A functional magnetic resonance imaging study. Neurogastroenterol Motil (2017) 29(10):1–10. doi: 10.1111/nmo.13108
172. Rubio A, Pellissier S, Van Oudenhove L, Ly HG, Dupont P, Tack J, et al. Brain responses to uncertainty about upcoming rectal discomfort in quiescent crohn's disease - a fmri study. Neurogastroenterol Motil (2016) 28(9):1419–32. doi: 10.1111/nmo.12844
173. Fan Y, Bao C, Wei Y, Wu J, Zhao Y, Zeng X, et al. Altered functional connectivity of the amygdala in crohn's disease. Brain Imaging Behav (2020) 14(6):2097–106. doi: 10.1007/s11682-019-00159-8
174. Kornelsen J, Wilson A, Labus JS, Witges K, Mayer EA, Bernstein CN. Brain resting-state network alterations associated with crohn's disease. Front Neurol (2020) 11:48. doi: 10.3389/fneur.2020.00048
175. Yeung AWK. Structural and functional changes in the brain of patients with crohn's disease: An activation likelihood estimation meta-analysis. Brain Imaging Behav (2021) 15(2):807–18. doi: 10.1007/s11682-020-00291-w
176. Thomann AK, Griebe M, Thomann PA, Hirjak D, Ebert MP, Szabo K, et al. Intrinsic neural network dysfunction in quiescent crohn's disease. Sci Rep (2017) 7(1):11579. doi: 10.1038/s41598-017-11792-y
177. Bao C, Liu P, Liu H, Jin X, Shi Y, Wu L, et al. Difference in regional neural fluctuations and functional connectivity in crohn's disease: A resting-state functional mri study. Brain Imaging Behav (2018) 12(6):1795–803. doi: 10.1007/s11682-018-9850-z
178. Gray MA, Chao CY, Staudacher HM, Kolosky NA, Talley NJ, Holtmann G. Anti-tnfalpha therapy in ibd alters brain activity reflecting visceral sensory function and cognitive-affective biases. PloS One (2018) 13(3):e0193542. doi: 10.1371/journal.pone.0193542
179. Black CJ, Thakur ER, Houghton LA, Quigley EMM, Moayyedi P, Ford AC. Efficacy of psychological therapies for irritable bowel syndrome: Systematic review and network meta-analysis. Gut (2020) 69(8):1441–51. doi: 10.1136/gutjnl-2020-321191
180. Farver-Vestergaard I, Jacobsen D, Zachariae R. Efficacy of psychosocial interventions on psychological and physical health outcomes in chronic obstructive pulmonary disease: A systematic review and meta-analysis. Psychother Psychosom (2015) 84(1):37–50. doi: 10.1159/000367635
181. Hanlon I, Hewitt C, Bell K, Phillips A, Mikocka-Walus A. Systematic review with meta-analysis: Online psychological interventions for mental and physical health outcomes in gastrointestinal disorders including irritable bowel syndrome and inflammatory bowel disease. Aliment Pharmacol Ther (2018) 48(3):244–59. doi: 10.1111/apt.14840
182. Jiang Y, Shorey S, Seah B, Chan WX, Tam WWS, Wang W. The effectiveness of psychological interventions on self-care, psychological and health outcomes in patients with chronic heart failure-a systematic review and meta-analysis. Int J Nurs Stud (2018) 78:16–25. doi: 10.1016/j.ijnurstu.2017.08.006
183. Prothero L, Barley E, Galloway J, Georgopoulou S, Sturt J. The evidence base for psychological interventions for rheumatoid arthritis: A systematic review of reviews. Int J Nurs Stud (2018) 82:20–9. doi: 10.1016/j.ijnurstu.2018.03.008
184. Wynne B, McHugh L, Gao W, Keegan D, Byrne K, Rowan C, et al. Acceptance and commitment therapy reduces psychological stress in patients with inflammatory bowel diseases. Gastroenterology (2019) 156(4):935–45.e1. doi: 10.1053/j.gastro.2018.11.030
185. Li C, Hou Z, Liu Y, Ji Y, Xie L. Cognitive-behavioural therapy in patients with inflammatory bowel diseases: A systematic review and meta-analysis. Int J Nurs Pract (2019) 25(1):e12699. doi: 10.1111/ijn.12699
186. Jordan C, Hayee B, Chalder T. Cognitive behaviour therapy for distress in people with inflammatory bowel disease: A benchmarking study. Clin Psychol Psychother (2019) 26(1):14–23. doi: 10.1002/cpp.2326
187. Hunt MG, Loftus P, Accardo M, Keenan M, Cohen L, Osterman MT. Self-help cognitive behavioral therapy improves health-related quality of life for inflammatory bowel disease patients: A randomized controlled effectiveness trial. J Clin Psychol Med Settings (2020) 27(3):467–79. doi: 10.1007/s10880-019-09621-7
188. Bennebroek Evertsz F, Sprangers MAG, Sitnikova K, Stokkers PCF, Ponsioen CY, Bartelsman J, et al. Effectiveness of cognitive-behavioral therapy on quality of life, anxiety, and depressive symptoms among patients with inflammatory bowel disease: A multicenter randomized controlled trial. J Consult Clin Psychol (2017) 85(9):918–25. doi: 10.1037/ccp0000227
189. Dubinsky MC, Dotan I, Rubin DT, Bernauer M, Patel D, Cheung R, et al. Burden of comorbid anxiety and depression in patients with inflammatory bowel disease: A systematic literature review. Expert Rev Gastroenterol Hepatol (2021) 15(9):985–97. doi: 10.1080/17474124.2021.1911644
190. Szigethy E, Youk AO, Gonzalez-Heydrich J, Bujoreanu SI, Weisz J, Fairclough D, et al. Effect of 2 psychotherapies on depression and disease activity in pediatric crohn's disease. Inflammation Bowel Dis (2015) 21(6):1321–8. doi: 10.1097/MIB.0000000000000358
191. Giuliani C. The flavonoid quercetin induces ap-1 activation in frtl-5 thyroid cells. Antioxid (Basel) (2019) 8(5):112. doi: 10.3390/antiox8050112
192. Chen J, Chen X, Sun Y, Xie Y, Wang X, Li R, et al. The physiological and psychological effects of cognitive behavior therapy on patients with inflammatory bowel disease before covid-19: A systematic review. BMC Gastroenterol (2021) 21(1):469. doi: 10.1186/s12876-021-02003-0
193. Hood MM, Jedel S. Mindfulness-based interventions in inflammatory bowel disease. Gastroenterol Clin North Am (2017) 46(4):859–74. doi: 10.1016/j.gtc.2017.08.008
194. Gonzalez-Moret R, Cebolla A, Cortes X, Banos RM, Navarrete J, de la Rubia JE, et al. The effect of a mindfulness-based therapy on different biomarkers among patients with inflammatory bowel disease: A randomised controlled trial. Sci Rep (2020) 10(1):6071. doi: 10.1038/s41598-020-63168-4
195. Berrill JW, Sadlier M, Hood K, Green JT. Mindfulness-based therapy for inflammatory bowel disease patients with functional abdominal symptoms or high perceived stress levels. J Crohns Colitis (2014) 8(9):945–55. doi: 10.1016/j.crohns.2014.01.018
196. Ewais T, Begun J, Kenny M, Hay K, Houldin E, Chuang KH, et al. Mindfulness based cognitive therapy for youth with inflammatory bowel disease and depression - findings from a pilot randomised controlled trial. J Psychosom Res (2021) 149:110594. doi: 10.1016/j.jpsychores.2021.110594
197. Jedel S, Hoffman A, Merriman P, Swanson B, Voigt R, Rajan KB, et al. A randomized controlled trial of mindfulness-based stress reduction to prevent flare-up in patients with inactive ulcerative colitis. Digestion (2014) 89(2):142–55. doi: 10.1159/000356316
198. Goren G, Schwartz D, Friger M, Banai H, Sergienko R, Regev S, et al. Randomized controlled trial of cognitive-behavioral and mindfulness-based stress reduction on the quality of life of patients with crohn disease. Inflammation Bowel Dis (2022) 28(3):393–408. doi: 10.1093/ibd/izab083
199. Keefer L, Taft TH, Kiebles JL, Martinovich Z, Barrett TA, Palsson OS. Gut-directed hypnotherapy significantly augments clinical remission in quiescent ulcerative colitis. Aliment Pharmacol Ther (2013) 38(7):761–71. doi: 10.1111/apt.12449
200. Danese S, Solitano V, Jairath V, Peyrin-Biroulet L. The future of drug development for inflammatory bowel disease: The need to act (Advanced combination treatment). Gut (2022) 14:2022–327025. doi: 10.1136/gutjnl-2022-327025
201. Gavrilescu O, Prelipcean CC, Dranga M, Soponaru C, Mihai C. The specialized educational and psychological counseling in inflammatory bowel disease patients - a target or a challenge? Turk J Gastroenterol (2020) 31(11):760–6. doi: 10.5152/tjg.2020.19669
202. Gerbarg PL, Jacob VE, Stevens L, Bosworth BP, Chabouni F, DeFilippis EM, et al. The effect of breathing, movement, and meditation on psychological and physical symptoms and inflammatory biomarkers in inflammatory bowel disease: A randomized controlled trial. Inflammation Bowel Dis (2015) 21(12):2886–96. doi: 10.1097/MIB.0000000000000568
203. Torres J, Ellul P, Langhorst J, Mikocka-Walus A, Barreiro-de Acosta M, Basnayake C, et al. European Crohn's and colitis organisation topical review on complementary medicine and psychotherapy in inflammatory bowel disease. J Crohns Colitis (2019) 13(6):673–85e. doi: 10.1093/ecco-jcc/jjz051
204. Regueiro M, Greer JB, Szigethy E. Etiology and treatment of pain and psychosocial issues in patients with inflammatory bowel diseases. Gastroenterology (2017) 152(2):430–9.e4. doi: 10.1053/j.gastro.2016.10.036
205. Klag T, Mazurak N, Fantasia L, Schwille-Kiuntke J, Kirschniak A, Falch C, et al. High demand for psychotherapy in patients with inflammatory bowel disease. Inflammation Bowel Dis (2017) 23(10):1796–802. doi: 10.1097/MIB.0000000000001216
206. Kutschera M, Waldhoer T, Groechenig H, Haas T, Wenzl H, Steiner P, et al. The need for psychological and psychotherapeutic interventions in Austrian patients with inflammatory bowel disease. Z für Gastroenterol (2020) 58(05):e76. doi: 10.1055/s-0040-1712247
207. Knowles SR, Keefer L, Wilding H, Hewitt C, Graff LA, Mikocka-Walus A. Quality of life in inflammatory bowel disease: A systematic review and meta-Analyses-Part ii. Inflammation Bowel Dis (2018) 24(5):966–76. doi: 10.1093/ibd/izy015
208. Leone D, Gilardi D, Corro BE, Menichetti J, Vegni E, Correale C, et al. Psychological characteristics of inflammatory bowel disease patients: A comparison between active and nonactive patients. Inflammation Bowel Dis (2019) 25(8):1399–407. doi: 10.1093/ibd/izy400
209. Daghaghzadeh H, Naji F, Afshar H, Sharbafchi MR, Awat Feizi3 MM, Tabatabaeeyan M, et al. Efficacy of duloxetine add on in treatment of inflammatory bowel disease patients: A double-blind controlled study. J Res Med Sci (2015) 20(6):595–601. doi: 10.4103/1735-1995.165969
210. Gorard DA, Libby GW, Farthing MJ. Influence of antidepressants on whole gut and orocaecal transit times in health and irritable bowel syndrome. Aliment Pharmacol Ther (1994) 8(2):159–66. doi: 10.1111/j.1365-2036.1994.tb00273.x
211. Iskandar HN, Cassell B, Kanuri N, Gyawali CP, Gutierrez A, Dassopoulos T, et al. Tricyclic antidepressants for management of residual symptoms in inflammatory bowel disease. J Clin Gastroenterol (2014) 48(5):423–9. doi: 10.1097/MCG.0000000000000049
212. Varghese AK, Verdu EF, Bercik P, Khan WI, Blennerhassett PA, Szechtman H, et al. Antidepressants attenuate increased susceptibility to colitis in a murine model of depression. Gastroenterology (2006) 130(6):1743–53. doi: 10.1053/j.gastro.2006.02.007
213. Guemei AA, El Din NM, Baraka AM, El Said Darwish I. Do desipramine [10,11-Dihydro-5-[3-(Methylamino) propyl]-5h-Dibenz[B,F]Azepine monohydrochloride] and fluoxetine [N-Methyl-3-Phenyl-3-[4-(Trifluoromethyl)Phenoxy]-Propan-1-Amine] ameliorate the extent of colonic damage induced by acetic acid in rats? J Pharmacol Exp Ther (2008) 327(3):846–50. doi: 10.1124/jpet.108.141259
214. Mikocka-Walus A, Hughes PA, Bampton P, Gordon A, Campaniello MA, Mavrangelos C, et al. Fluoxetine for maintenance of remission and to improve quality of life in patients with crohn's disease: A pilot randomized placebo-controlled trial. J Crohns Colitis (2017) 11(4):509–14. doi: 10.1093/ecco-jcc/jjw165
215. Wichniak A, Wierzbicka A, Walecka M, Jernajczyk W. Effects of antidepressants on sleep. Curr Psychiatry Rep (2017) 19(9):63. doi: 10.1007/s11920-017-0816-4
216. Ruepert .L, Quartero AO, de Wit NJ, Heijden GJvd, Rubin G, Muris JW. Bulking agents, antispasmodics and antidepressants for the treatment of irritable bowel syndrome (Review). Cochrane Database Syst Rev (2011) 10(8):CD003460. doi: 10.1002/14651858.CD003460.pub3
217. Mikocka-Walus A, Ford AC, Drossman DA. Antidepressants in inflammatory bowel disease. Nat Rev Gastroenterol Hepatol (2020) 17(3):184–92. doi: 10.1038/s41575-019-0259-y
218. Mikocka-Walus A, Prady SL, Pollok J, Esterman AJ, Gordon AL, Knowles S, et al. Adjuvant therapy with antidepressants for the management of inflammatory bowel disease. Cochrane Database Syst Rev (2019) 4:CD012680. doi: 10.1002/14651858.CD012680.pub2
219. Frolkis AD, Vallerand IA, Shaheen AA, Lowerison MW, Swain MG, Barnabe C, et al. Depression increases the risk of inflammatory bowel disease, which may be mitigated by the use of antidepressants in the treatment of depression. Gut (2019) 68(9):1606–12. doi: 10.1136/gutjnl-2018-317182
220. Hall BJ, Hamlin PJ, Gracie DJ, Ford AC. The effect of antidepressants on the course of inflammatory bowel disease. Can J Gastroenterol Hepatol (2018) 2018:2047242. doi: 10.1155/2018/2047242
221. Macer BJ, Prady SL, Mikocka-Walus A. Antidepressants in inflammatory bowel disease: A systematic review. Inflammation Bowel Dis (2017) 23(4):534–50. doi: 10.1097/MIB.0000000000001059
222. Zhang XF, Guan XX, Tang YJ, Sun JF, Wang XK, Wang WD, et al. Clinical effects and gut microbiota changes of using probiotics, prebiotics or synbiotics in inflammatory bowel disease: A systematic review and meta-analysis. Eur J Nutr (2021) 60(5):2855–75. doi: 10.1007/s00394-021-02503-5
Keywords: psychological stress, inflammatory bowel disease, psychoneuroimmunology, brain-gut axis, anxiety, depression, psychiatric comorbidities, psychotherapy
Citation: Ge L, Liu S, Li S, Yang J, Hu G, Xu C and Song W (2022) Psychological stress in inflammatory bowel disease: Psychoneuroimmunological insights into bidirectional gut–brain communications. Front. Immunol. 13:1016578. doi: 10.3389/fimmu.2022.1016578
Received: 11 August 2022; Accepted: 20 September 2022;
Published: 06 October 2022.
Edited by:
Kevin P. Mollen, School of Medicine, University of Pittsburgh, United StatesReviewed by:
Mikhail Melnikov, Federal Medical & Biological Agency of Russia, RussiaHang Yu, China Academy of Chinese Medical Sciences, China
Copyright © 2022 Ge, Liu, Li, Yang, Hu, Xu and Song. This is an open-access article distributed under the terms of the Creative Commons Attribution License (CC BY). The use, distribution or reproduction in other forums is permitted, provided the original author(s) and the copyright owner(s) are credited and that the original publication in this journal is cited, in accordance with accepted academic practice. No use, distribution or reproduction is permitted which does not comply with these terms.
*Correspondence: Wengang Song, cy5jb21AMTYzLmNvbQ==
†These authors have contributed equally to this work and share last authorship