- 1Department of Pharmaceutical Sciences, School of Pharmacy, College of Health Professions, North Dakota State University, Fargo, ND, United States
- 2Sol Sherry Thrombosis Research Center, Temple University School of Medicine, Temple University Hospital, Philadelphia, PA, United States
- 3Center for Inflammation and Lung Research, Department of Microbiology, Immunology and Inflammation, Lewis Katz School of Medicine, Temple University, Philadelphia, PA, United States
- 4Department of Microbiological Sciences, College of Agriculture, Food Systems and Natural Resources, North Dakota State University, Fargo, ND, United States
Sepsis, a complex clinical syndrome resulting from a serious infection, is a major healthcare problem associated with high mortality. Sex-related differences in the immune response to sepsis have been proposed but the mechanism is still unknown. Purinergic signaling is a sex-specific regulatory mechanism in immune cell physiology. Our studies have shown that blocking the ADP-receptor P2Y12 but not P2Y1 receptor was protective in male mice during sepsis, but not female. We now hypothesize that there are sex-related differences in modulating P2Y12 or P2Y1 signaling pathways during sepsis. Male and female wild-type (WT), P2Y12 knock-out (KO), and P2Y1 KO mice underwent sham surgery or cecal ligation and puncture (CLP) to induce sepsis. The P2Y12 antagonist ticagrelor or the P2Y1 antagonist MRS2279 were administered intra-peritoneally after surgery to septic male and female mice. Blood, lungs and kidneys were collected 24 hours post-surgery. Sepsis-induced changes in platelet activation, secretion and platelet interaction with immune cells were measured by flow cytometry. Neutrophil infiltration in the lung and kidney was determined by a myeloperoxidase (MPO) colorimetric assay kit. Sepsis-induced platelet activation, secretion and aggregate formation were reduced in male CLP P2Y12 KO and in female CLP P2Y1 KO mice compared with their CLP WT counterpart. Sepsis-induced MPO activity was reduced in male CLP P2Y12 KO and CLP P2Y1 KO female mice. CLP males treated with ticagrelor or MRS2279 showed a decrease in sepsis-induced MPO levels in lung and kidneys, aggregate formation, and platelet activation as compared to untreated male CLP mice. There were no differences in platelet activation, aggregate formation, and neutrophil infiltration in lung and kidney between female CLP mice and female CLP mice treated with ticagrelor or MRS2279. In human T lymphocytes, blocking P2Y1 or P2Y12 alters cell growth and secretion in vitro in a sex-dependent manner, supporting the data obtained in mice. In conclusion, targeting purinergic signaling represents a promising therapy for sepsis but drug targeting purinergic signaling is sex-specific and needs to be investigated to determine sex-related targeted therapies in sepsis.
Introduction
Sepsis, a complex clinical syndrome resulting from a serious infection, is a major healthcare problem associated with high morbidity and mortality (1). Current sepsis treatments are limited to supportive therapies (2), and specific pharmacologic treatments that could greatly improve patient outcomes have not yet been developed (2, 3). With hospital mortality rates of affected patients reportedly as high as 50%, there is a critical need for improved methods for treating sepsis (1).
Sex-related differences in the morbidity and mortality of sepsis have been observed in animal models and human diseases (4–8). To date, females has shown decreased mortality and organ failure in mice and humans compared to their male counterpart (4, 7). However, the lack of studies comparing both sexes limit our capacity to evaluate the extent of sex-related differences. Hence sex should be taken into account when identifying the optimal pharmacological intervention for sepsis. Sex-related differences have been observed in other diseases, such as cardiovascular diseases (4), Alzheimer’s (9), cancer (10), and ulcerative colitis (11). In some cases, such as ulcerative colitis (11) and cardiovascular diseases (4) sex-specific treatment has been identified, improving the patient outcome. But there is a gap in knowledge for other diseases.
Purinergic signaling represents a novel regulatory mechanism in immune cell physiology (12). Cells respond to activation with the release of cellular ATP, which regulates cell functions (13). In sepsis, large amounts of ADP are released by tissue damage and immune cells. This leads to over-activation of purinergic signaling contributing to immune dysfunction (13). As a result, regulating purinergic signaling can reveal new avenues in the treatment of sepsis.
Changes in purinergic receptors has been investigated within sexes. For instance, changes in purinergic signaling response may be hormone-dependent (14–17) and they vary in the sex organs (18–20). Indeed, in a murine menopause model, ovarian protein levels of purinergic receptor P2X2 increased with ageing, suggesting that the P2X2 receptor is involved with menopause/ageing-related decline in ovarian function in females (21). Beaucage and coworkers revealed that the P2X7 receptor subtype might be involved in an age- and sex-dependent regulation of adipogenesis and lipid metabolism (14, 16).
Platelet ADP-induced activation is regulated by the P2Y12 and P2Y1 signaling pathways (22, 23). P2Y12 is a Gi protein coupled receptor (24), while P2Y1 is a Gq protein coupled receptor (23, 24). Activation of the P2Y12 signaling pathway leads to platelet aggregation and potentiation of platelet secretion (25) while P2Y1 activation leads to shape change and aggregation (23, 24). We have previously shown that blockade of the P2Y12 signaling pathway in a murine model of sepsis results in improved outcomes in male mice (26), through decreased α-granule secretion of inflammatory mediators and reduced mobilization of P-selectin to the plasma membrane of platelets (26). We have shown that by blocking specific signaling pathways in platelets, we can regulate inflammation without compromising platelet functions. However, P2Y1 deficiency did not alter inflammation levels or lung injury in a murine model of sepsis in male mice. However, there are sex-related differences in how platelet respond to activation. In fact, female platelets are more reactive to agonists, especially ADP than male (27, 28) suggesting sex-related differences in ADP- induced platelet activation. Sex-related differences in the expression of P2Y1 or P2Y12 have been shown to vary within sexes in the murine brain (29) but information about the expression of these receptors in other organs is limited.
In this study, we aim to investigate whether either deficiency or blocking the ADP-receptors P2Y1 or P2Y12 alters inflammation levels in sepsis in a sex-specific manner. To achieve this aim, we used a well-recognized murine model of sepsis (cecal ligation and double puncture) and compared male and female mice upon P2Y1 or P2Y12 deficiency or blockade. Our data show that P2Y12 but not P2Y1 deficiency, decreased the activity of MPO in lungs and kidneys, platelet-leukocyte interaction and platelet activation in male but not female mice. Either P2Y12 or P2Y1 blockade could decrease activity of MPO in lungs and kidneys and platelet-leukocyte interaction in male mice. On the other hand, P2Y1 deficiency but not blockade decreased activity of MPO in lungs and platelet-leukocyte interaction in female mice. In human T lymphocytes, blocking P2Y1 or P2Y12 alters cell growth and secretion in vitro in a sex-dependent manner, supporting the data obtained in mice. Thus, drug targeting purinergic signaling appears to be sex-specific and needs to be investigated to determine sex-related targeted therapies in sepsis.
Materials and methods
Materials
All reagents were of analytical grade and were obtained from Thermo Fisher Scientific (Waltham, MA) unless stated otherwise. Ficoll-Paque was from GE Healthcare Bio-Sciences AB (Uppsala, SE). FITC-conjugated anti-mouse CD11b (clone M1/70) was obtained from Invitrogen (Waltham, MA). PE-conjugated anti-mouse CD4 (clone GK 1.5), FITC- conjugated anti-mouse CD41 (clone MWreg30) and FITC-conjugated mouse anti p-selectin (clone RB40.34) were obtained from BD Bioscience (San Jose, CA). FITC-conjugated anti-mouse CD14 (clone MEM-18) were obtained from Sigma-Aldrich (St. Louis, MO). PE-conjugated anti-human CD4 (clone OKT4) was purchased from BioLegend (San Diego, CA) and APC- conjugated anti-human CD8 (clone HIT8a) antibodies was obtained from eBioscience (San Diego, CA) Invitrogen (Waltham, MA). Rat IgG2a κ isotype control FITC [clone eBR2a), rat IgG2b K isotype control PE (clone eB149/10H5)] were purchased from eBioscience (San Diego, CA). Ticagrelor and MRS2279 was obtained from TOCRIS (Pittsburgh, PA).
Animals and treatments
Animal procedures and handling adhered to the National Institutes of Health standards and were approved by the Institutional Animal Care and Use Committee Protocol #A21040 at North Dakota State University (Fargo, ND, USA). Male and female wild-type and P2Y12 deficient pathogen-free C57BL/6 mice (weight, 25-30 g; 8-10 weeks of age) were obtained from Schering-Plough Corporation (Kenilworth, NJ)1-4. P2Y1 deficient pathogen-free C57BL/6 male and female mice were generated by subcontract with Lexicon Genetics Inc. (Woodlands, TX) through knockout constructs as described previously (22, 25, 30). Only 8-10-week homozygote animals were included. Male and female mice were housed in a climate-controlled pathogen free environment and given free access to food and water.
The cecal ligation and double puncture (CLP) procedures were performed on under anesthesia with isoflurane (2 ± 4% induction in chamber and 1 ± 2% maintenance in mask) as described previously (26, 31–33). Following midline laparotomy, the cecum was identified, the mesentery trimmed, and the stalk joining the cecum to the large intestine ligated. Care was taken to assure the intestinal continuity was not interrupted. The cecum was punctured twice with a 24-gauge needle on the anti-mesenteric border, stool expressed, and the cecum returned to the abdomen. Sham control animals underwent a laparotomy without ligation or double puncture. Experiments were performed in P2Y12, P2Y1 KO, and wild-type (WT) male and female mice that were randomly assigned to one of four groups for wild-type or KO: wild-type and KO sham control group (6 animals per group); wild-type and KO undergoing CLP (CLP group, 6 animals per group).
Ticagrelor and MRS2279 were administrated intraperitoneally to WT male and female mice (6 animals per group) with a dose of 30 mg/kg (ticagrelor) (34–36) and 50mg/kg (MRS2279) (37, 38) at the time of surgery. Sham mice received the same doses of Ticagrelor and MRS2279. After the procedure but prior to emergence, sham and CLP mice were fluid-resuscitated (1 ml/mouse sterile saline, subcutaneously).
At 24 hours post-surgery, mice were anesthetized and blood samples were collected by cardiac puncture (10:1 in 3.8% sodium citrate) for hematology studies (Hemavet® Multispecies Hematology System, Drew Scientific, Inc. Oxford, CT). All mice were euthanized by cardiac puncture and exsanguination. Lungs and kidneys were collected and fixed or frozen immediately in liquid nitrogen.
Myeloperoxidase activity
Lungs and kidneys were homogenized and sonicated. After centrifugation (10,000 for 10 minutes at 4°C), myeloperoxidase (MPO) levels were detected using a MPO calorimetric assay kit (BioVision, USA). The assay was performed as described by the manufacturer. Briefly, perfused lung and kidney tissues were homogenized rapidly on ice upon addition of myeloperoxidase (MPO) Assay buffer and the supernatant collected after centrifugation (10,000 for 10 minutes at 4°C). Resorufin standard and MPO positive control were prepared. Standards, positive controls, and samples were transferred into different wells of the 96-well plate. Reaction mix prepared with MPO assay buffer, MPO peroxidation substrate, and hydrogen peroxidase (0.88M) was added to each well and thoroughly mixed after which fluorescence (Ex/Em=535/587 nm) was kinetically measured at 37°C for 10 minutes. A resorufin standard curve was plotted and the MPO activity of the test samples was calculated.
Blood urea nitrogen and creatinine measurement
Plasma aliquots from each animal were obtained by blood centrifugation (2,000 x g for 10 minutes) and immediately frozen. Blood urea nitrogen levels were measured using the urea nitrogen (BUN) colorimetric detection kit (ThermoFisher Scientific, USA). The assay was performed as described by the manufacturer. The plasma samples were diluted with deionized water (1:30) prior to use. The urea nitrogen standard (100 mg/dL urea nitrogen) was used. Samples and standards were added to a 96-well plate. Acidic solutions coded as color reagents A and B in the kit were each added to all wells and incubated for 30 minutes, and the absorbance was immediately read at 450 nm using a microplate reader. Plasma creatinine was determined by an enzymatic assay kit (Mouse Creatinine Assay Kit no. 80350, Crystal Chem, Downers Grove, IL). The assay was performed as described by the manufacturer. Samples were added to a 96-well plate and incubated with the sarcosine oxidase solution provided. Following a 5-minute incubation at 37°C, a peroxidase solution was added to the samples and the plate was incubated for 5 minutes. The absorbance was immediately read at 550 nm using a microplate reader.
Platelet-leukocyte aggregate formation and P-selectin expression in whole blood
Murine blood samples were diluted 1:2 in PBS and incubated with either FITC-conjugated anti-mouse CD11b (dilution 1:100 in PBS) or CD4 (dilution 1:100 in PBS) or CD14 (dilution 1:100 in PBS) and PE-conjugated anti-mouse CD41 (dilution 1:100 in PBS) or with FITC-conjugated anti-mouse P-selectin (dilution 1:100 in PBS) for 20 minutes at 25°C. The reaction was stopped by adding BD FACSTM lysing solution (1:10 in PBS). Samples were kept in the dark and at 4°C prior to analysis. Flow cytometry was performed using Accuri-C6 System and data were analyzed with FlowJo software. Platelet (CD41+) and leukocyte (CD11b+) or T lymphocyte (CD4+) or monocyte (CD14+) aggregates were discriminated by forward and side light scatter and identified by their positive staining for PE-CD41 or FITC-CD11b, or FITC-CD4, or FITC-CD14 respectively. Events double positive for PE and FITC were identified aggregates and were recorded as a percentage of a total of 20,000 gated neutrophils or monocytes or T lymphocytes. Gating strategies are shown in Supplemental Figures 1A–C.
Platelet factor 4 and soluble P-selectin measurement
Plasma aliquots from each animal were obtained by centrifugation (2,000g for 10 minutes) of the blood samples and the plasma was immediately frozen. To detect PF4 and soluble p-selectin concentrations, corresponding ELISA kits (Sigma) were used. The assay was performed as described by the manufacturer. Briefly, Standard protein of mouse PF4 and P-selectin were reconstituted and diluted accordingly to provide a standard stock solution. Samples and standard were added to a 96-well plate coated with either anti-human PF4 or anti-P-selectin antibody, covered and incubated at room temperature for 2.5 hours and overnight at 4°C respectively with gentle shaking. The plates were washed and biotinylated mouse P-selectin or biotinylated anti-mouse PF-4 detection antibodies was added to each well and incubated at room temperature for an hour with gentle shaking. After washing, HRP conjugated streptavidin was added to each well and incubated for 45 minutes at room temperature. Then 3,3,5,5’-tetramethylbenzidine (TMB) in buffer solution was added to each well and incubated in the dark at room temperature for 30 minutes. The reaction was stopped by adding stop solution (0.2M sulfuric acid) to each well and the absorbance immediately read at 450nm using a micro-plate reader.
Thromboxane generation assay
Plasma aliquots from each animal were obtained by blood centrifugation (2,000g for 10 minutes). Samples were used to evaluate thromboxane generation using a TXB2 EIA kit from Enzo Life Sciences (catalog no. ADI-901-002). The assay was performed as described by the manufacturer. Briefly, TXB2 standard was prepared using Assay Buffer (tris buffered saline solution supplemented with proteins and sodium azide). Standards and unknown samples were pipetted into the appropriate wells. Blue conjugate (alkaline phosphatase-conjugated with TXB2) and antibody solution (rabbit polyclonal antibody to TXB2) was pipetted into each well. The plate was incubated at room temperature for 2 hours and washed using a wash solution (tris buffered saline containing detergents). pNpp substrate solution (p-nitrophenyl phosphate in buffer) was added to each well and incubated at room temperature for 45 minutes. The reaction was stopped using trisodium phosphate in water and the optical density read immediately at 405nm.
Cytokine measurements
Plasma aliquots from each animal were obtained by blood centrifugation (2,000g for 10 minutes). Levels of RANTES, Il-1β, IL-17, TNF-α, and IFN-γ were determined using the high sensitivity mouse cytokine discovery array 32-plex (Eve Technologies, Calgary, Canada).
Human peripheral blood mononuclear cell isolation
Human blood was obtained from healthy volunteers following informed consent. The Institutional Review Board of North Dakota State University approved the study (#3735). The age of the donors is 39.4 ± 3.0 for females and 38.5 ± 3.6 for males. Blood was collected in 3.2% sodium citrate vacutainers. Blood was diluted with RPMI1640, added to 10 ml of Ficoll-Paque, and centrifuged at 300 g for 30 minutes at RT. Peripheral blood mononuclear cells (PBMC) were collected from the interphase and washed twice in PBS. Viable cell numbers were determined using Eve Automatic Cell counter (NanoEntek, Walthan, MA). Cells with viability higher than 85% were selected and viability was taken into account when the cell number was calculated. Cells (0.5x106 cells/mL) were then cultured at 37°C and 5% CO2 in RPMI 1640 media, fully supplemented with penicillin-streptomycin (each at 0.8 mM) and L-glutamine (2 mM).
Peripheral blood mononuclear cell culture and treatments
PBMC were co-cultured for 3 days at 37°C in the presence or absence of LPS (1μM). To block the P2Y12 signaling pathway in vitro, we used Ticagrelor (100μg/mL), a well-established selective P2Y12 antagonist, which has been used in multiple in vitro studies (32, 33, 39, 40). To block the P2Y1 signaling pathway in vitro, we used MRS2279 (MRS, 100μg/mL), a well-established selective P2Y1 antagonist, which has been used in multiple in vitro studies (41–44). Negative control cells received an equivalent amount of vehicle (saline). Three-days after LPS exposure, cells were collected and analyzed using flow cytometry. The supernatant was collected and stored at -20°C prior to analysis.
CD4+ and CD8+ cell population
CD4 and CD8 phenotyping were measured via flow cytometry. Isolated PBMC (0.5 x 106 cells) were incubated with FITC-conjugated anti-human CD4 (1:100 dilution in saline) and PE-conjugated anti-human CD8 antibodies (1:100 dilution in saline) for 1 hour at room temperature. Cells were washed in PBS and kept in PBS at 4°C prior to analysis. Cells were then acquired using Accuri-C6 System and analyzed using the Flow Jo software. The total number of events acquired was 20,000 for each sample. Data are shown as a % of positive events as compared to the total number of events acquired (20,000) (Figure 1). Rat IgG2a κ isotype control FITC (clone eBR2a), rat IgG2b κ isotype control PE (clone eB149/10H5) were included as negative isotype controls. Gating strategies are shown now in Supplemental Figures 1D, E.
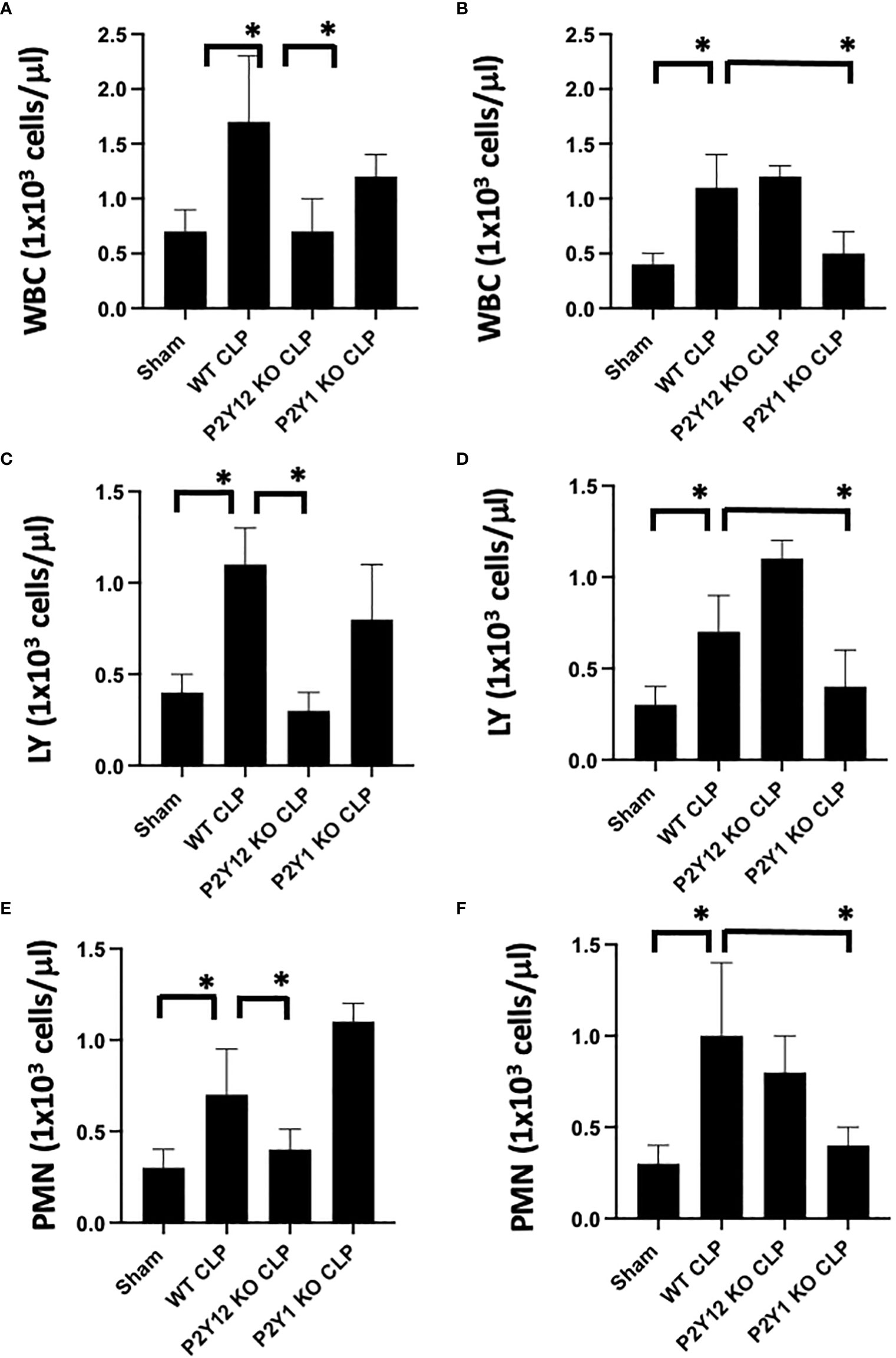
Figure 1 Inflammation-induced elevation in circulating white blood cell counts is decreased in male P2Y12 and female P2Y1 KO mice. Blood samples were collected by cardiac puncture in 3.8% sodium citrate (10:1), and hematology studies were performed. Graphs show counts of White blood cells (WBC, A, B) lymphocytes (LY; C, D), and neutrophils (PMN; E, F). Sham and CLP in wild-type (WT) and P2Y12 KO and P2Y1 mice of male (A, C E) and female (B, D, F) mice. Values are expressed as 1x103cells/μL, mean ± S.E.M. n = 5). (*P < 0.05). No differences between WT, P2Y12 KO, and P2Y1 KO sham were noted in both male and female mice (data not shown).
TNF-α measurement
Supernatant from human cell samples were collected by centrifugation (5,000g for 10 minutes) at day 3. TNF-α levels were determined by ELISA (Invitrogen - Waltham, MA). The assay was performed following manufacturer instructions. Briefly, samples and standards were incubated with Biotin-conjugate antibody (anti-human TNF-α polyclonal antibody) at room temperature for 2 hours and washed with assay buffer (PBS with 1% Tween 20, 10% BSA). Then samples were incubated with Streptavidin-HRP for 1 hour at room temperature. The wells were washed using the assay buffer and TMB substrate solution (tetramethyl-benzidine) was added to all wells and incubated at room temperature for 10 minutes. The reaction was stopped using Phosphoric acid (1M) and the absorbance was read immediately at 450 nm using a spectrophotometer.
IFN- γ measurement
Supernatant from human cell samples were collected by centrifugation (5,000g for 10 minutes) at day 3. IFN-γ concentrations were determined by ELISA (Invitrogen - Waltham, MA). The assay was performed following manufacturer instructions. Standards and samples were added to the wells, and incubated overnight at 4°C with gentle shaking. The wells were washed with the wash buffer provided and standards and samples were incubated with biotin conjugate for 1 hour at room temperature. After washing the plate, standards and samples were incubated with streptavidin-HRP for 45 minutes at room temperature. After washing the plate, standards and samples were incubated with TMB substrate solution (tetramethyl-benzidine) in the dark at room temperature for about 30 minutes. The stop solution was added, and the absorbance read immediately at 450 nm.
Statistical analysis
Differences among groups were statistically analyzed using one-way ANOVA; Bonferroni’s Multiple Comparison Test was used as a post-hoc analysis. Each treatment group included four or more experiments (n ≥ 4), based on power calculations and work performed previously (45–47). For human cell experiments: each independent experiment was performed using platelets and PBMCs isolated from one donor. PBMCs and platelets from 4 donors were isolated, co-cultured, stimulated and analyzed. Differences among groups were analyzed using a one-way ANOVA test. The analysis was performed in an unpaired fashion. P < 0.05 was considered to be significant. Data are reported as mean ± standard error of the mean (S.E.M.) for each group.
Results
Inflammation-induced elevation in circulating white blood cells counts is decreased in male P2Y12 and female P2Y1 null mice
First, we analyzed the number of circulating white blood cells (WBC), lymphocytes (LY) and neutrophils (PMN) in blood samples (Figure 1) from WT, P2Y1 KO and P2Y12 KO male and female mice. Upon CLP surgery, we observed a significant increase in leukocyte count (white blood cells (A-B), lymphocytes (C-D) and neutrophils (E-F) in septic mice as compared with the Sham group in both male (A-C-E) and female (B-D-F) mice (Figure 1, P<0.05; male CLP vs male sham or CLP vs female CLP vs female sham). Interestingly, the increase was more pronounced in male septic mice as compared with the female counterpart (Figure 1, P<0.05; male CLP vs female CLP) for white blood cells, lymphocytes, and neutrophils. However, the sepsis-induced leukocyte count (white blood cells, lymphocytes, and neutrophils) was not increased in the CLP P2Y12 KO male mice compared to WT CLP male mice (Figures 1A, C, E P<0.05; male CLP vs male P2Y12 KO CLP) but no change in CLP P2Y1 KO male mice was noted (Figures 1A, C, E). In female mice, no difference was noted between CLP P2Y12 KO mice and WT CLP mice, while leukocyte count (lymphocytes and neutrophils) was not increased in the CLP P2Y1 KO female mice compared to WT CLP female (Figures 1B, D, F) P<0.05; female CLP vs female P2Y1 KO CLP. Platelet count did not change in all the groups analyzed (data not shown).
Sepsis-induced increase of MPO activity in the lungs is reduced in male septic P2Y12 and female septic P2Y1 KO mice
As the lung is one of the most affected organs during sepsis (48–50), we investigated MPO activity in lung tissue as an indication of neutrophil infiltration in the tissue (Figure 2). Lung samples from Sham and CLP mice were analyzed for male and female mice in WT, P2Y1, and P2Y12 KO. Although MPO activity was significantly increased in both male (Figure 2A) and female (Figure 2B) WT mice following CLP as compared with the Sham counterpart (Figure 2, P<0.05; male CLP vs male sham and female CLP vs female sham), a higher increase in MPO activity was noted in male WT CLP mice as compared with female WT CLP mice (Figure 2, P<0.05; male CLP vs female CLP). MPO activity was not elevated in the lungs of KO P2Y12 CLP mice as compared to the activity in CLP WT mice in males (Figure 2. A P<0.05; male CLP vs male P2Y12 KO CLP), while no difference between WT and P2Y12 KO was seen in female mice (Figure 2B). These data suggest that P2Y12 deficiency alter pulmonary inflammation and inflammatory cell recruitment in male but not in female mice during sepsis. In P2Y1 mice, we did not see any difference in MPO activity in male P2Y1 KO CLP mice as compared with male WT CLP mice (Figure 2A). However, in female mice, MPO activity is significantly reduced in female P2Y1 KO CLP compared with WT CLP mice (Figure 2B, P<0.05; female CLP vs female P2Y1 KO CLP). These data suggest that P2Y1 deficiency alter sepsis-induced pulmonary inflammation and inflammatory cell recruitment in female mice but not male mice.
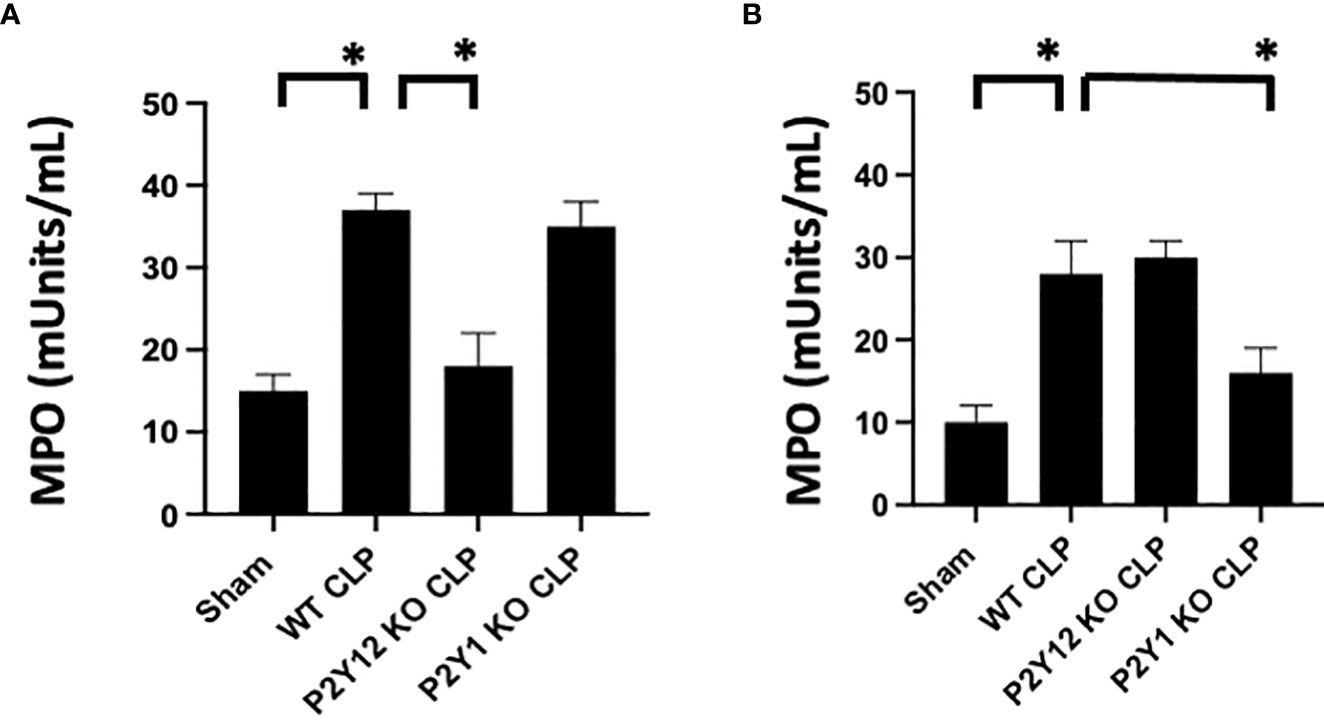
Figure 2 Sepsis-induced increase of activity of MPO in the lungs is reduced in male septic P2Y12 and female septic P2Y1 KO mice. MPO analysis was performed in lung samples of Sham and CLP in wild-type (WT) and P2Y12 KO and P2Y1 mice of male (A) and female (B) mice. Values are expressed as mUnits/mL, mean ± SEM. (*P < 0.05) (n = 5). No differences between WT, P2Y12 KO, and P2Y1 KO sham were noted in both male and female mice (data not shown).
Sepsis induced- platelet activation was decreased in male septic P2Y12 and female septic P2Y1 KO mice
To determine whether sepsis-induced platelet activation was sex-dependent or required P2Y12 or P2Y1 signaling, we measured p-selectin platelet surface expression in septic mice (WT, P2Y12 KO CLP and P2Y1 KO CLP) for both male (Figure 3A) and female (Figure 3B). P-selectin was increased in both WT CLP male and female mice as compared with Sham counterparts (Figure 3, P<0.05; male CLP vs male sham and female CLP vs female sham, P<0.05; male CLP vs female CLP). In P2Y12 KO CLP mice, sepsis-induced p-selectin values were lower than the WT CLP (Figure 3A P<0.05, male CLP vs male P2Y12 KO CLP) while no difference between WT CLP and P2Y12 KO CLP was noted in female mice. Similarly, to what was noted in Figures 1 and 2, no difference in p-selectin values was reported between WT CLP and P2Y1 KO CLP in male mice, but p-selectin was significantly reduced in P2Y1 KO CLP in female mice (Figure 3B, P<0.05, female CLP vs female P2Y1 KO CLP).
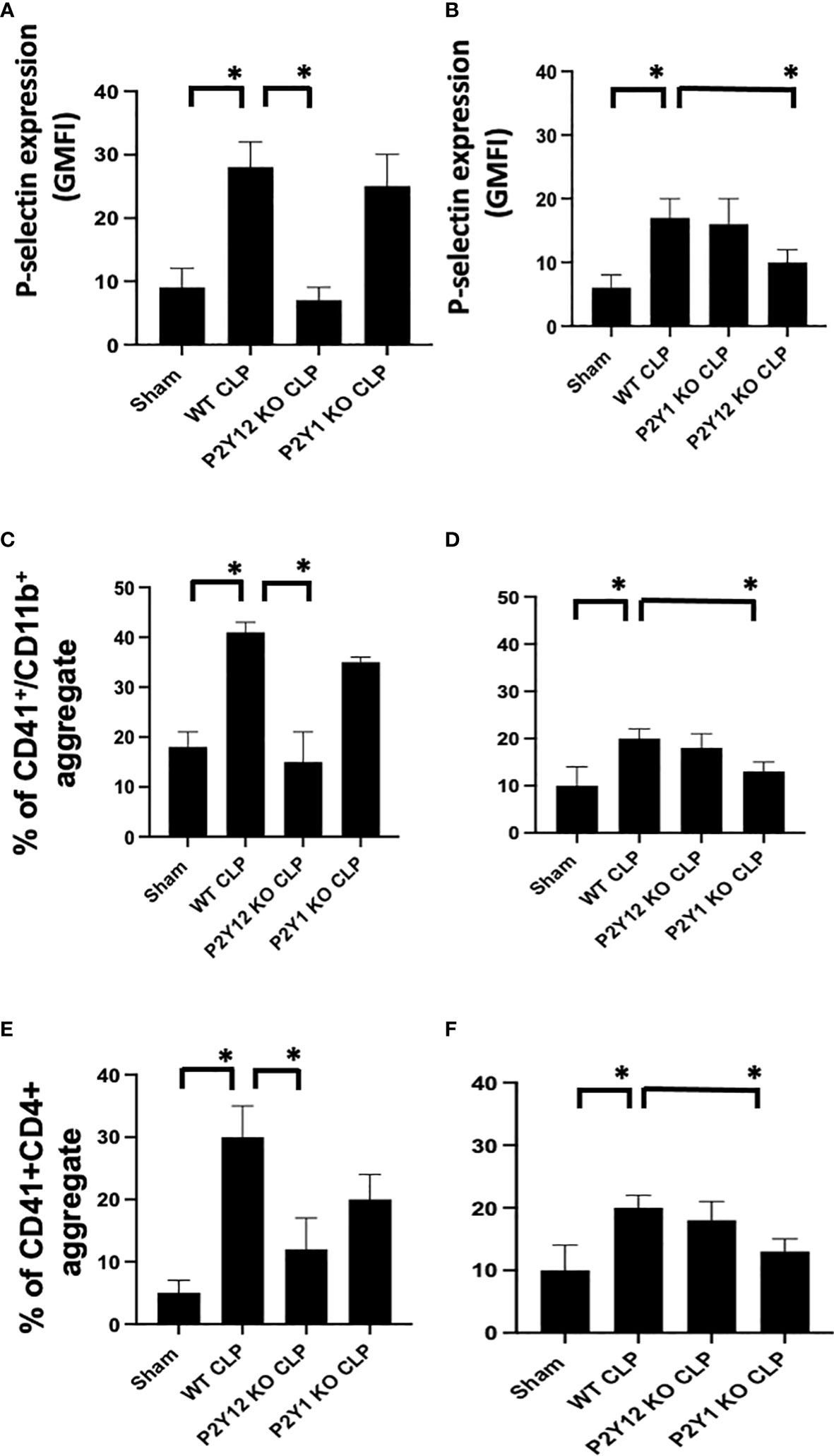
Figure 3 Sepsis-induced platelet activation and CD41+/CD11b or CD41+/CD4+ aggregates were decreased in male septic P2Y12 and female septic P2Y1 KO mice. Blood samples were collected by cardiac puncture in 3.8% sodium citrate (10:1) (A, B) P-selectin expression on the platelet surface was evaluated using flow cytometry in whole blood. Data were collected from WT, P2Y12 KO, and P2Y1 mice of male (A) and female (B) mice. Both Sham and CLP were analyzed. (n=6) (*P<0.05) Values are expressed as Geometric Mean of fluorescence intensity (GMFI), mean ± S.E.M No differences between WT, P2Y12 KO, and P2Y1 KO sham were noted in both male and female mice. (C, D) Peripheral blood collected from WT, P2Y12 KO, and P2Y1 mice of male (C) and female (D) mice were incubated with antibodies against CD41 (platelet marker) and CD11B (leukocyte marker). Activated leukocytes were gated based on CD11b expression, and cell shape and data were analyzed as a percentage of aggregates expressing both CD41 and CD11b. Values are expressed as the percentage of CD41+/CD11b+ cells, mean ± SEM, n=5). No differences between WT, P2Y12 KO, and P2Y1 KO sham were noted in both male and female mice. (*P<0.05). (E, F) Peripheral blood collected from WT, P2Y12 KO, and P2Y1 mice of male (E) and female (F) mice was incubated with antibodies against CD41 (platelet marker) and CD4 (T cell marker). T cells were gated based on CD4 expression and cell shape, and data were analyzed based on the percentage of aggregates that express both CD41 and CD4 (n = 6). No differences between WT, P2Y12 KO, and P2Y1 KO sham were noted in both male and female mice (data not shown). (*P < 0.05).
Sepsis induced- platelet-leukocyte aggregate formation in whole blood was reduced in male septic P2Y12 and female septic P2Y1 KO mice
As platelet-leukocyte interaction is a key step in sepsis, we analyzed platelet-leukocyte aggregate formation in the whole blood and we investigated whether targeting the receptor P2Y12 or P2Y1 can modulate platelet-leukocyte interaction differently in male and female mice (Figures 3C, D). First, we measured interaction between platelets (CD41) and leukocyte (CD11b). The percentage of CD41+/CD11b+ aggregate was increased in male CLP and female CLP as compared with their Sham counterpart (Figures 3C, D, P<0.05, male CLP vs male Sham and female CLP vs female sham). Female CLP-induced platelet-leukocyte aggregates was lower than CLP males (Figures 3C, D, P<0.05, male CLP vs female CLP). In P2Y12 KO CLP mice, sepsis-induced platelet-leukocyte aggregates values were lower than the WT CLP (Figure 3C; P<0.05, male CLP vs male P2Y12 KO CLP) while no difference between WT CLP and P2Y12 KO CLP was noted in female mice (Figure 3D). However, no difference in platelet-leukocyte aggregates was reported between WT CLP and P2Y1 KO CLP in male mice (Figure 3C), but CD41+/CD11b+ aggregates were significantly reduced in P2Y1 KO CLP in female mice (Figure 3D, P<0.05, female CLP vs female P2Y1 KO CLP).
Second, we analyzed the aggregate formation of circulating platelets (CD41+) and T helper (CD4+) cells. The percent of platelet-CD4 lymphocytes aggregate was increased during sepsis as compared with the sham control group in male and female mice (Figure 3C P<0.05, male CLP vs male Sham and female CLP vs female sham), but in P2Y12 KO male mice platelets-CD4+ T cells aggregates were diminished, suggesting decreased platelets and CD4+ T cells interactions (Figure 3C P<0.05, male CLP vs male P2Y12 KO CLP). In female mice, no change was noted in platelets and CD4+ T cells interaction upon P2Y12 deficiency. No change in platelets-CD4+ T cells aggregate was noted in male CLP P2Y1 KO mice as compared with male CLP WT mice. But in female mice, platelets and CD4+ T cells aggregate is reduced in female CLP P2Y1 KO mice as compared with female CLP WT mice (Figure 3C P<0.05, female CLP vs female P2Y1 KO CLP). These data suggest that P2Y12 and P2Y1 deficiency reduce platelet-leukocyte and T cell-platelet interactions in a sex-dependent manner.
P2Y12 or P2Y1 antagonism attenuates lung and renal MPO activity in sepsis in a sex-related manner
Next, we investigated whether P2Y12 or P2Y1 antagonism alters sepsis-induced MPO activity similarly to receptor deficiency. Leukocyte trafficking to the lungs and kidneys are among the most affected organs during sepsis (51, 52). At 24 hours post-CLP, there is evidence of both lung and kidney damage associated with increased leukocyte influx (MPO activity) (31, 53, 54). Next, we determined whether blocking the P2Y12 or P2Y1 receptors alters MPO activity in the lung (Figures 4A, B) and kidney (Figures 4C, D) of septic male (4A and C) or female (4B and D) mice. Figure 4A shows that MPO activity was increased during sepsis in the lung (Figure 4A) in male mice as compared with the Sham group (P<0.05; male CLP vs male sham). However, when male mice were treated with either MRS2279 or ticagrelor, a significant reduction in MPO was noted in septic male mice as compared with untreated CLP mice (P<0.05; CLP vs CLP + MRS2279 or CLP vs CLP + ticagrelor). MPO activity was also increased during sepsis in the lung (Figure 4B) in female mice as compared with the Sham group (P<0.05; female CLP vs female sham). However, when female mice were treated with either MRS2279 or ticagrelor, no change was noted in MPO levels in the lung in septic female as compared with untreated female CLP (Figure 4B). Similarly in kidney samples (Figure 4C), when male mice were treated with either MRS2279 or ticagrelor, a significant reduction in MPO was noted in septic mice as compared with untreated mice (P<0.05; CLP vs CLP + MRS2279 or CLP vs CLP + ticagrelor). When female mice were treated with either MRS2279 or ticagrelor, no change was noted in MPO levels in the kidney in septic females as compared with untreated female CLP (Figure 4D). These data suggest that blocking either P2Y1 or P2Y12 receptors decreased activity of MPO in the lungs and kidneys of male, but not female mice.
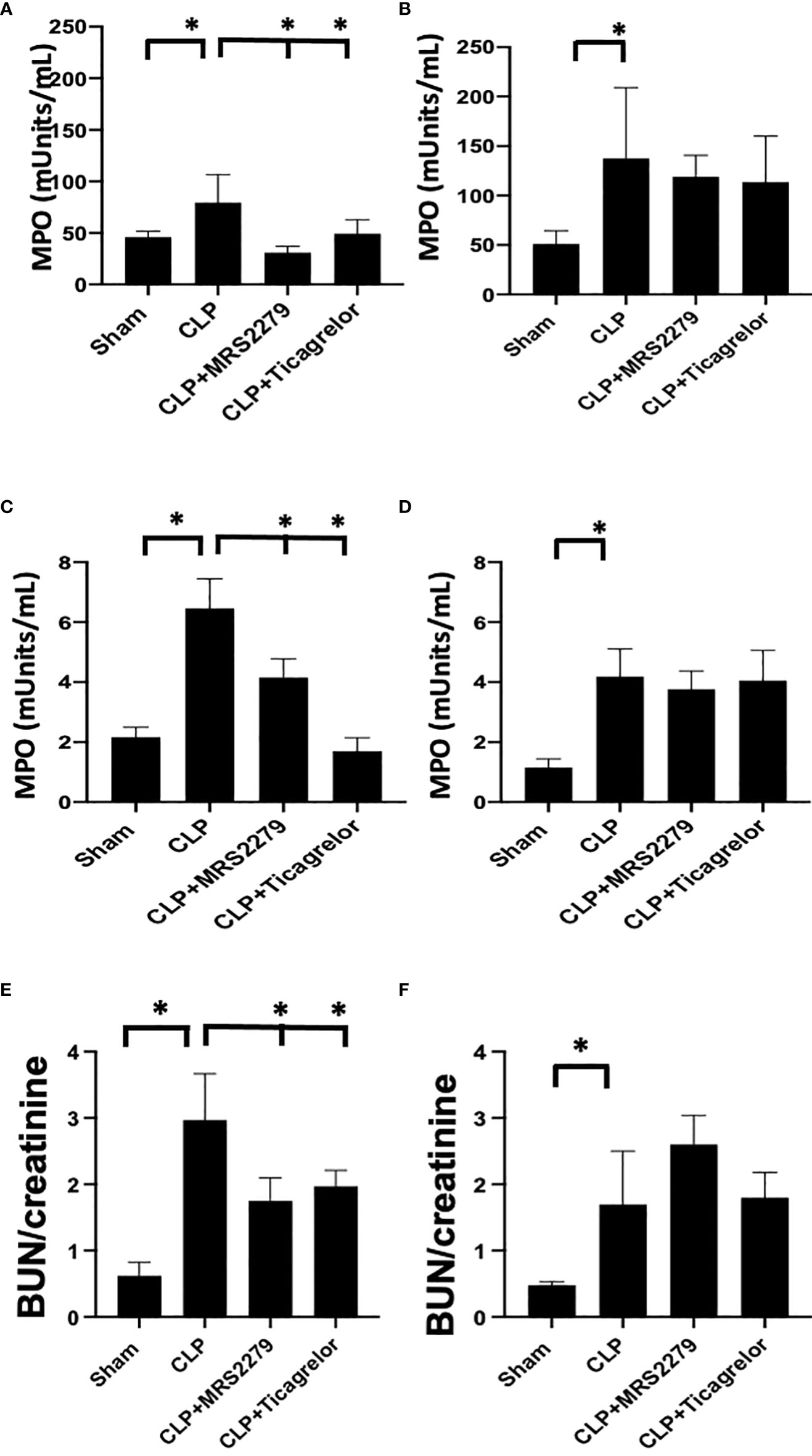
Figure 4 P2Y12 or P2Y1 antagonism attenuates lung and renal MPO activity in sepsis in a sex-related manner. (A–D) MPO analysis was performed in the lung (A, B) and kidney (C, D) samples of Sham, CLP, MRS2279-treated CLP, and ticagrelor-treated CLP in male (A, C) and female mice (B, D). Values are expressed as mUnits/mL, mean ± SEM. (n = 5). (*P < 0.05). (E, F) Blood urea nitrogen (BUN)/Creatinine ratios were determined 24 hours post-surgery in plasma samples of Sham, CLP + CLP, MRS2279-treated CLP, and ticagrelor-treated CLP in male (E) and female mice (F).
We also investigate a plasma indicator of kidney injury such as blood urea nitrogen (BUN)/creatinine ratios. The ratio was elevated in the CLP group in both males (Figure 4E) and females (Figure 4F) as compared to their sham control, demonstrating increased levels of blood urea nitrogen BUN/creatinine ratios upon sepsis. Importantly, in male mice (Figure 4E), exposure to ticagrelor and MRS2279 resulted in a significant decrease in BUN/creatinine ratios (P<0.05; CLP vs CLP + MRS2279 or CLP vs CLP + ticagrelor). No changes were noted in female mice upon treatments (Figure 4F).
P2Y12 or P2Y1 antagonism alters sepsis-induced platelet activation, and platelet–leukocyte aggregate formation in a sex-specific manner
We investigated whether P2Y12 or P2Y1 antagonism can influence platelet activation in male and female mice during sepsis. We analyzed P-selectin expression on the surface of circulating platelets (Figures 5A, B) and the levels of soluble p-selectin in the plasma (Figures 5C, D). In both male (Figure 5A) and female (Figure 5B) mice, sepsis augmented p-selectin expression on platelet surface as compared with their Sham control (P<0.05; male Sham vs male CLP or female Sham vs female CLP). P-selectin expression was higher in septic males as compared with septic female mice (P<0.05; male CLP vs female CLP). In male mice, treatment with MRS2279 p-selectin surface expression was not changed in response to CLP, while ticagrelor treatment significantly prevented the sepsis induced elevation of p-selectin surface expression as compared with untreated CLP (P<0.05; CLP vs CLP + ticagrelor). In female mice, no change was noted in p-selectin expression upon treatment with either MRS2279 or ticagrelor as compared with untreated CLP (Figure 5B). Similar results were noted for soluble p-selectin in the plasma of septic mice. (Figures 5C, D). Again, soluble p-selectin was higher in untreated septic male mice as compared with septic female mice (P<0.05; male CLP vs female CLP). In male mice, treatment with MRS2279 or ticagrelor lowered sepsis-elevated soluble p-selectin as compared with untreated CLP (Figure 5C; P<0.05; CLP vs CLP + MRS2279; CLP vs CLP + ticagrelor). In female mice, no change was noted in p-selectin expression upon treatment with either MRS2279 or ticagrelor as compared with untreated CLP (Figure 5D).
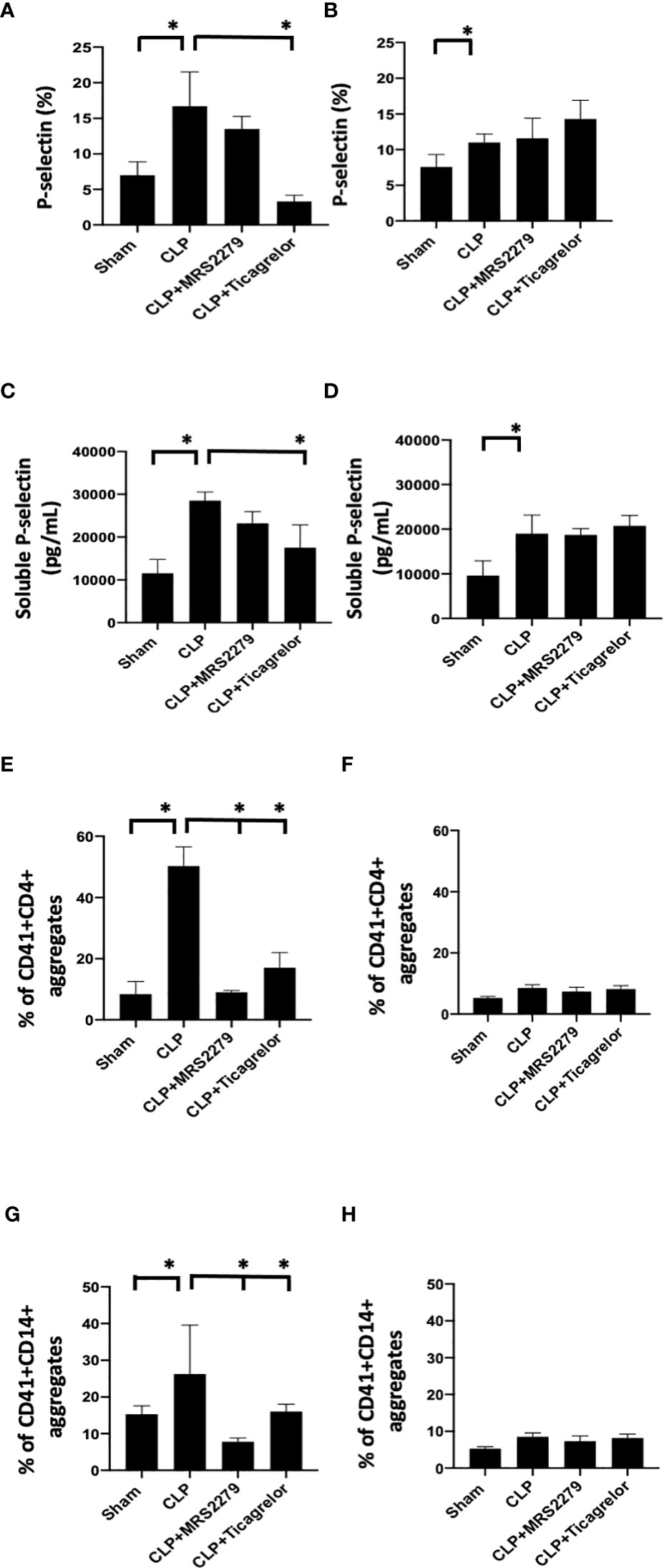
Figure 5 P2Y12 or P2Y1 antagonism alters sepsis-induced platelet activation, and platelet–leukocyte aggregate formation in a sex-specific manner. (A, B)_Blood samples were collected by cardiac puncture in 3.8% sodium citrate (10:1) P-selectin expression on platelet surface was evaluated using flow cytometry in whole blood. Data were collected from Sham and CLP in male (A) and female mice (B). Mice were untreated or treated with MRS (50mg/kg) or ticagrelor (30 mg/kg). Values are expressed as % of platelets positive to p-selectin, mean ± S.E.M. (*P < 0.05). (C, D) Soluble p-selectin was analyzed in the plasma collected from Sham and CLP male (C) and female (D) mice using an ELISA kit. Mice were untreated or treated with MRS (10mg/kg) or ticagrelor (10mg/kg). Values are expressed as pg/mL, mean ± S.E.M. (*P < 0.05). (E-H) Samples were collected from Sham and CLP male (E–G) and female (F–H) mice. Samples were incubated with antibodies against CD41 (platelet marker) and CD4 (T cell marker, E, F) or CD14 (monocyte marker, G, H). T cells or monocytes were gated based on CD4 or CD14 expression respectively and cell shape. Data were analyzed based on the percentage of aggregates that express both CD41 and CD4 (E, F) or CD14 (G, H). Values are expressed as the percentage of CD41+/CD11b+ cells, mean ± SEM n = 7).
Aggregates of platelets and other immune cells have been observed in peripheral whole blood during other diseases [22, 23], including sepsis (26, 31). Hence, we investigated the aggregates of platelets and CD4+ T cells (Figures 5E, F) or CD14+ cells (Figures 5G, H) circulating in the whole blood of sham control, CLP mice, and CLP mice treated with MRS2179 or ticagrelor in both male and female (Figure 5). Aggregates were analyzed using flow cytometry. CD4+ or CD14+ cells were gated, and the percent of aggregates as events positive for both CD41 and CD4 Figures 5E, F) or CD41 and CD14 (Figures 5G, H) respectively was determined. Platelet-CD4+ T cell aggregate formation was increased during sepsis as compared with the sham control group in both male and female mice (Figures 5E, F, P<0.05; male Sham vs male CLP, female Sham vs female CLP). The % of platelet-CD4+ T cell aggregate was significantly higher in male CLP mice as compared with CLP female mice (P<0.05; male CLP vs female CLP). Treatment with either MRS2279 or ticagrelor dramatically diminished aggregate formation in male mice (Figure 5E; P<0.05; CLP vs CLP + MRS2279; CLP vs CLP + ticagrelor), suggesting that blockade of either P2Y12 or P2Y1 signaling pathway contribute to decrease platelets and CD4+ T cells interactions in male mice. No change was noted in female mice when either P2Y12 or P2Y1 were blocked (Figure 5H).
P2Y12 or P2Y1 antagonism decreased sepsis-induced platelet secretion in both male and female mice
We and other groups have previously observed an increase in platelet secretion during sepsis in animal models and patient samples (26, 31, 55, 56). Hence, we determined platelet secretion in septic male and female mice with and without blockade of either P2Y12 or P2Y1 signaling pathway (Figure 6). We measured plasma levels of platelet-factor 4 (PF-4) and Thromboxane (TBX-B2). As previously observed, both PF-4 and Thromboxane were elevated in male or female septic mice as compared with Sham control (Figure 6) P<0.05; Sham vs CLP in male and female). Interestingly, in contrast with the data obtained in KO mice (Figure 4), levels of both PF-4 and Thromboxane upon sepsis were comparable between male and female mice. Septic-induced Thromboxane increase was not noted in CLP male mice when mice were treated with either MRS2279 or ticagrelor as compared with untreated male CLP mice (Figure 6A, P<0.05; CLP vs CLP + MRS2279; CLP vs CLP + ticagrelor). Similar data were noted in female mice (Figure 6B), where either MRS2279 or ticagrelor treatment prevented the sepsis- elevated level of Thromboxane (P<0.05; CLP vs CLP + MRS2279; CLP vs CLP + ticagrelor). Similarly, PF-4 levels were not elevated in CLP male mice when mice were treated with either MRS2279 or ticagrelor as compared with untreated male CLP mice (Figure 6C; P<0.05; CLP vs CLP + MRS2279; CLP vs CLP + ticagrelor). Similar data were noted in female mice, where either MRS2279 or ticagrelor treatment prevented the elevated level of PF-4 (Figure 6D; P<0.05; CLP vs CLP + MRS2279; CLP vs CLP + ticagrelor). These data suggest that blocking either P2Y12 or P2Y1 signaling pathway prevents sepsis-elevated platelet secretion in a sex-independent manner.
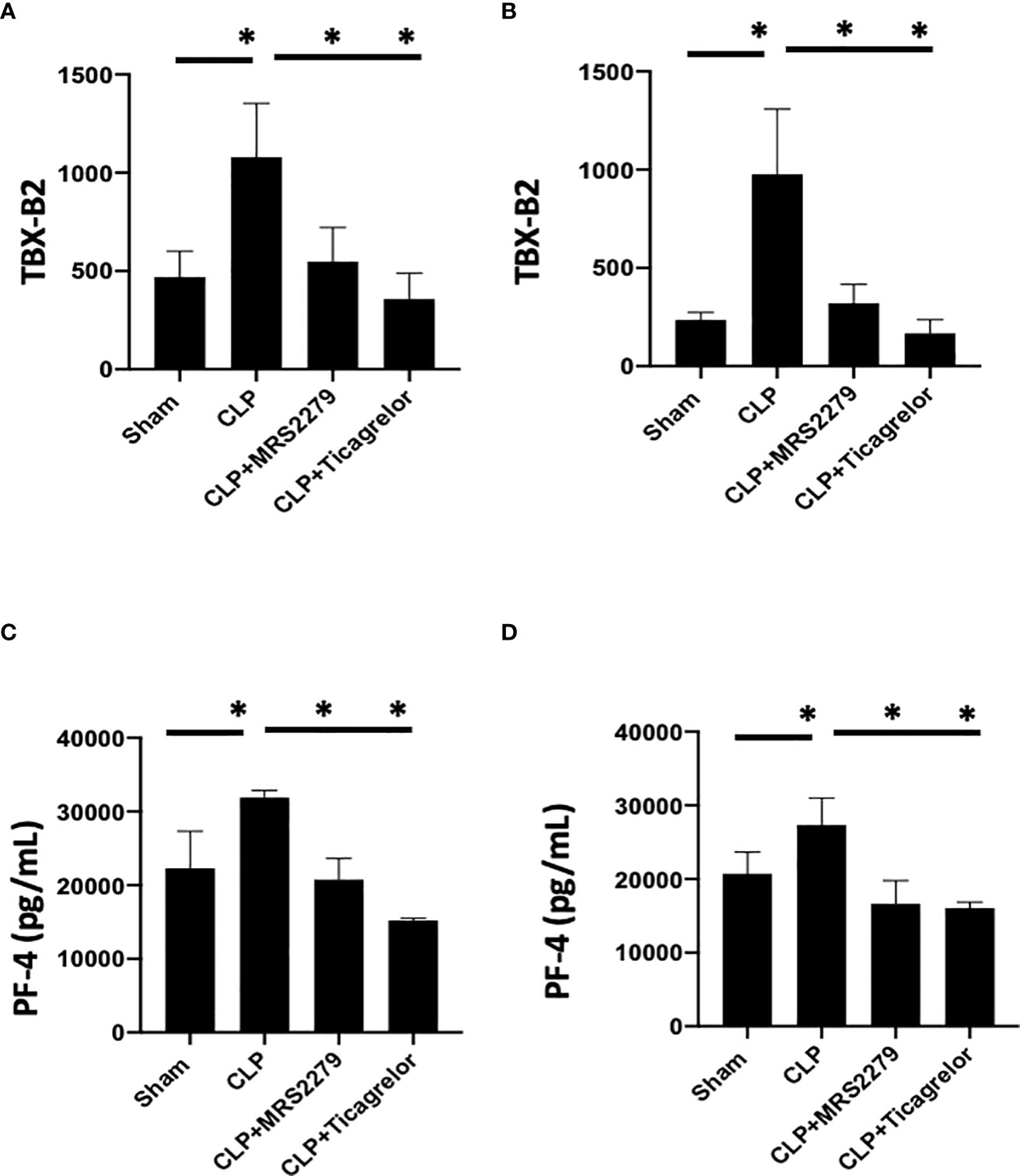
Figure 6 P2Y12 or P2Y1 antagonism decreased sepsis-induced platelet secretion in both male and female mice. Plasma levels of Thromboxane (TBX-B2) (A, B) or PF4 (C, D) were evaluated in female (left) and male (right) mice that underwent Sham or CLP surgery. Mice were treated with MRS2279 (MRS) or ticagrelor. Values are expressed as pmol/mL (*p < 0.05, n = 6).
P2Y12 or P2Y1 antagonism selectively alter cytokine levels in the plasma in a sex-specific manner
As an increase in circulating cytokines has been observed in patient samples (49, 57) and it has been related to the severity of the disease (58, 59), we investigated levels of RANTES, IL-10, IL-1β, IL-17, TNF-α and IFN-γ in plasma samples (Figure 7). As expected, all cytokines’ levels were significantly higher in septic mice as compared with their Sham counterpart for males (Figures 7A, C, E, G, I, K) and female (Figures 7B, D, F, H, J, L) mice.
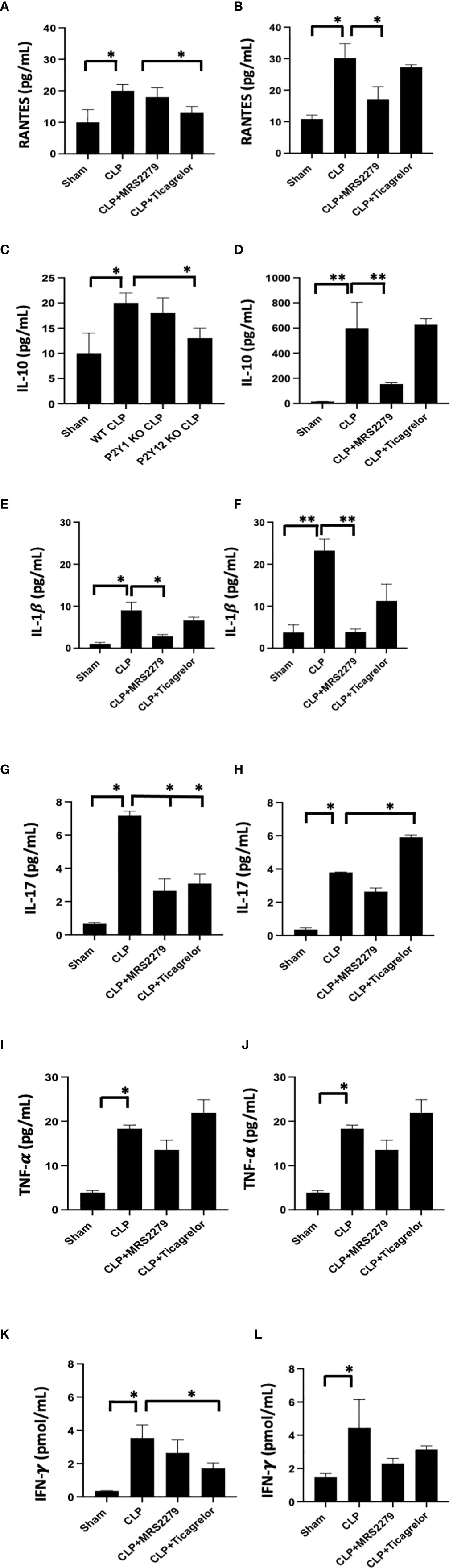
Figure 7 P2Y12 or P2Y1 antagonism selectively alters cytokine plasma levels in a sex-specific manner. Plasma samples obtained from each animal were used for detection levels of RANTES (A, B), IL-10 (C, D), IL-1β (E, F), IL-17 (G, H), TNF-α (I,J), and IFN-γ (K, L). Both Sham and CLP samples were analyzed for male and female mice. Mice were treated with MRS2279 (MRS) or ticagrelor. Values are expressed as pg/mL (*P < 0.05; **P < 0.01; n = 5).
For male mice, the sepsis-induced increase in RANTES plasma levels was decreased in mice treated with ticagrelor (Figure7A, P<0.05; CLP vs CLP + ticagrelor), while no change was noted when septic male mice were exposed to MRS2279. On the other hand, in female mice, the sepsis-induced increase in RANTES plasma levels was decreased when mice were exposed to MRS2279 (Figure 7B, P<0.05; CLP vs CLP MRS2279), while no change between septic mice was noted when mice were exposed to ticagrelor. Similar data were observed for IL-10 (Figures 7C, D) and IL-1β (E-F).
On the contrary, IL-17 levels were non increased in septic male mice treated with either ticagrelor or MRS2279 (Figure 7G, P<0.05; CLP vs CLP MRS2279 and CLP vs CLP + ticagrelor). On the other hand, in female mice, the sepsis-induced increase in IL-1β plasma levels was noted while mice were exposed to MRS2279 (Figure 7F, P<0.05; CLP vs CLP MRS2279), while an increase in IL-17 was noted in septic female mice exposed to ticagrelor as compared with septic untreated female mice (Figure 7H, P<0.05; CLP vs CLP MRS2279 and CLP vs CLP + ticagrelor).
No change was noted in both male and female mice in TNF-α levels in any of the treated groups as compared with septic males and females (Figures 7I, J).
Similarly, for male mice, the sepsis-induced increase in IFN-γ plasma levels were noted while mice were exposed to ticagrelor (Figure 7K, P<0.05; CLP vs CLP + ticagrelor), while no change between septic mice was noted when mice were exposed to MRS2279. On the other hand, in female mice, no change between treated septic mice and their treated counterpart was noted (Figure 7L).
P2Y1 antagonism selectively alters CD4 and CD8 cell populations in female but not male human PBMCs
To determine whether the sex-specific effects of purinergic signaling blockage that we noted in mice during sepsis is also observed in human cells, we investigated whether blocking P2Y1 or P2Y12 signaling pathways influence CD4 and CD8 differentiation when PBMCs cells are stimulated with LPS (Figure 8). PBMCs were isolated from male and female donors and P2Y1 or P2Y12 signaling pathways were blocked using MRS2179 and ticagrelor respectively (Figure 8, 100μg/mL). PBMCs were incubated with LPS (1μM) for 72 hours. Percentage of CD4 and CD8 cells (Figures 8A, B) was determined using flow cytometry and cytokine secretion was determined by ELISA (Figures 8C, D).
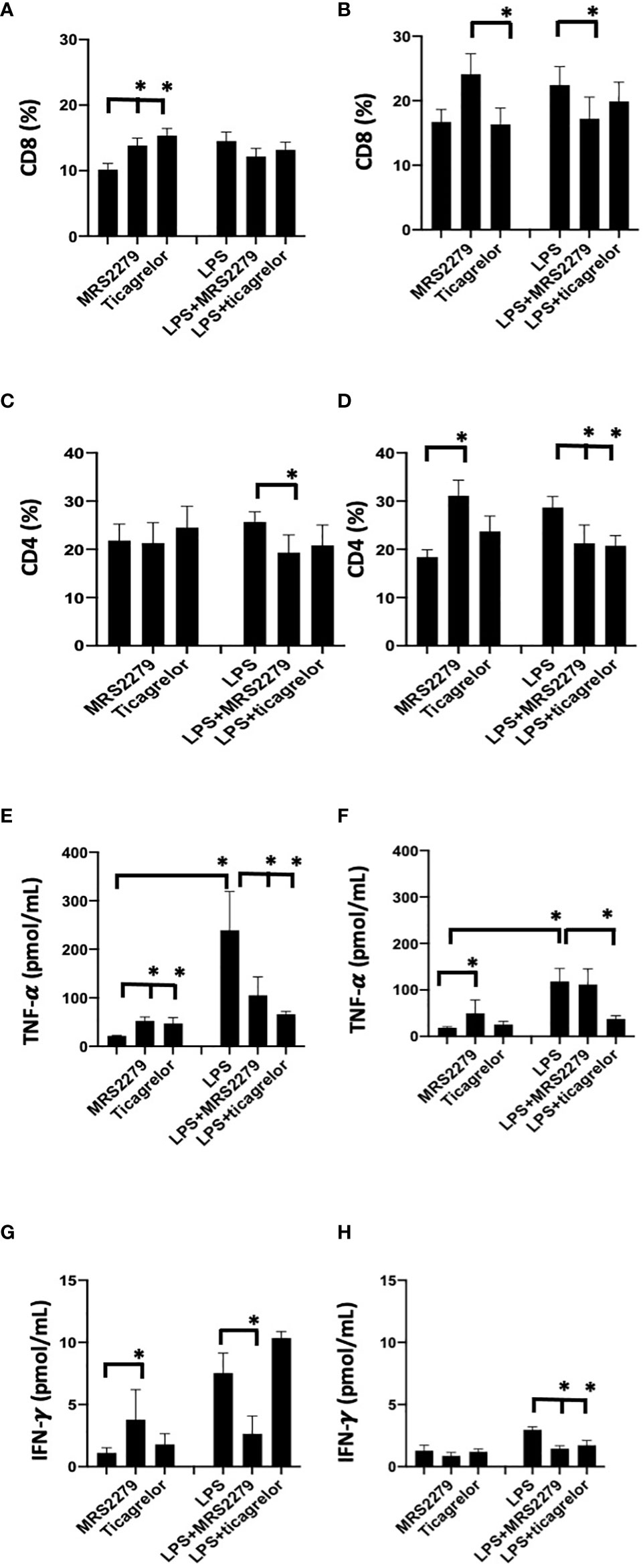
Figure 8 P2Y12 or P2Y1 antagonism selectively alter CD4 and CD8 cell populations and cytokine secretion in human PBMCs. Cells isolated from female (black) and male (white) donors were stimulated with LPS (right panel) or left unstimulated (left panel) for 72 h. Cells isolated from male (A-C-E-G) and female (B-D-F-H) donors were stimulated with LPS (right panel) or left unstimulated (left panel) for 72 hours. Unstimulated cells were cultured without stimuli. Cells were exposed MRS2279 (100μg/mL) or ticagrelor (100μg/mL). Negative control did not receive any treatment. Cell populations positive to CD8 (A-B) or CD4 (C-D) were determined using flow cytometry. Data are expressed as % of expression ± S.E.M. (*p < 0.05, n=6). Cytokine levels in the culture supernatants were determined for TNF-alpha (E-F) and IFN-γ (G-H). The groups analyzed were: negative control, MR2279-treated, and ticagrelor-treated cells. Cells were stimulated with LPS (right panel) or left unstimulated (left panel) for 72 h. Values are expressed in pg/ml; means ± S.E.M. are plotted (*p < 0.05, n = 6).
In PBMCs isolated from male donors, in unstimulated PBMCs the % of CD8+ cells increased in unstimulated cells when treated with either MRS2179 or ticagrelor as compared with untreated unstimulated cells (Figure 8A, P<0.05; Unstim vs Unstim + MRS2279; Unstim vs Unstim + ticagrelor). No changes were noted in LPS-stimulated PBMCs isolated from male donors. Interestingly in unstimulated PBMC from female subjects, exposure to MRS2279 increased the % of CD8+ cells as compared with untreated cells (Figure 8B, P<0.05; Unstim vs Unstim + MRS2279; LPS vs LPS + MRS2279). No change was noted when unstimulated cells from female donors were incubated with ticagrelor (Figure 8B). Similar results were noted in LPS-stimulated PBMC from female donors (Figure 8B). Indeed, exposure to MRS2279 increased the % of CD8+ cells as compared with untreated cells (Figure 8B, P<0.05; LPS vs LPS + MRS2279). No change was noted when LPS-stimulated cells from female donors were incubated with ticagrelor (Figure 8B).
In PBMC isolated from male donors, no change was noted in the CD4+ population in unstimulated PBMCs cells when the cells were incubated with MRS2279 or ticagrelor (Figure 8C). However, in LPS-stimulated male PBMCs, the % of CD4+ cells decreased in LPS-stimulated cells when treated with MRS2179 but not ticagrelor compared with untreated LPS-stimulated cells (Figure 8C, P<0.05; LPS vs LPS + MRS2279). When female PBMCs were incubated with MRS2279, the % of CD4+ cells increased in unstimulated cells as compared with untreated cells (Figure 8D, P<0.05; Unstim vs Unstim + MRS2279). In contrast, in LPS-stimulated PBMCs the % of CD4+ cells decreased in LPS-stimulated cells when treated with either MRS2179 or ticagrelor as compared with untreated LPS-stimulated cells (Figure 8D, P<0.05; LPS vs LPS + MRS2279; LPS vs LPS + ticagrelor). These data indicate that blocking purinergic signaling alters the % of the CD8 and CD4 population depending on LPS stimulation in a sex-specific manner.
P2Y1 antagonism selectively alters IFN-γ and TNF-α levels in female but not male PBMCs
Next, we measured the concentration of TNF-α (Figure 8C) and IFN-γ (Figure 8D) in the supernatant of PBMCs cultured in the presence and absence of LPS (1μM - 72 hours) (Figures 8E–H). In male unstimulated cells, TNF-α content was significantly increased when PBMCs were exposed to either MRS or ticagrelor (Figure 8E). In female unstimulated cells, we noted a significant increase TNF-α when cells were treated with MRS, but not ticagrelor (Figure 8E, P<0.05; Unstim vs Unstim + MRS; Unstim vs Unstim + ticagrelor). In male LPS-stimulated cells, a significant decrease was noted when cells were incubated with either MRS or ticagrelor (Figure 8E, P<0.05; LPS vs LPS + MRS; LPS vs LPS + ticagrelor). In female LPS-stimulated cells, no change was noted in TNF-α in the supernatant when cells were treated with MRS, but a significant decrease was noted when cells were incubated with ticagrelor (Figure 8F, P<0.05; LPS vs LPS + ticagrelor). In unstimulated cells from male donors, INF-γ content was significantly increased when PBMCs were exposed to either MRS or ticagrelor (Figure 8G, P<0.05; Unstim vs Unstim + MRS, Unstim vs Unstim + ticagrelor). In unstimulated cells from female donors, no change was noted in INF-γ content between the supernatant collected from unstimulated cells as compared with unstimulated cells treated with either MRS or ticagrelor (Figure 8H). In LPS-stimulated cells from male donors, a significant increase was noted when cells were incubated with MRS but not ticagrelor as compared with the LPS-treated control (Figure 8G, P<0.05; LPS vs LPS + MRS). In LPS-stimulated cells from female donors, INF-γ was significantly decreased in cells treated with either MRS or ticagrelor as compared with untreated LPS control (Figure 7H, P<0.05; LPS vs LPS + MRS; LPS vs LPS + ticagrelor).
Discussion
Sex differences in the morbidity and mortality of sepsis have been observed in animal models and human diseases (4–8). To date, females have shown decreased mortality and organ failure in mice and humans compared to their male counterparts (4, 7). However, the lack of studies comparing both sexes limits our capacity to evaluate the extent of sex-related differences and to determine a sex-specific treatment as a result. Our previous studies revealed that platelets are important in sepsis and blocking purinergic signaling in platelets alters the outcome of sepsis in male mice (23, 26, 31). However, we are aware of sex-related differences in purinergic signaling responses (41, 60–63) and in platelet activation (20). Hence, it is essential to investigate how female mice respond to sepsis and whether blocking purinergic signaling alters platelets’ response in a sex-dependent manner. Our data suggest for the first time that the purinergic receptor P2Y12 and P2Y1 influence sepsis-induced activity of MPO in lungs and kidneys, circulating cytokines, platelet activation and platelet-leukocyte interaction differently in male and female mice. Hence targeting platelets and the purinergic receptor P2Y12 and P2Y1 may be an appropriate therapeutic strategy that is sex dependent.
In our previous studies in male mice using a CLP model of sepsis (19, 21), P2Y12 but not P2Y1 deficiency diminished platelet activation and ameliorated the outcome of sepsis (26). When we compared these data with data obtained from female mice, we noted that P2Y1 but not P2Y12 deficiency could improve the outcome of sepsis, in terms of neutrophil infiltration in the lungs and kidney, platelet activation, and platelet interaction with other immune cells. Previous data have also shown that purinergic signaling is activated differently in male and female mice (27, 28), and the expression of P2Y1 and P2Y12 receptor varies between sexes (29). Ticagrelor is currently used as anti-platelet therapy (64–66) and clinical studies have confirmed that the effects of ticagrelor in preventing cardio-vascular diseases in patients were comparable between men and women (67–69), hence a sex-related therapy may not be required for cardiovascular disease treatment. However, other studies identified significant changes between male and female patients suggesting a discrepancy in the literature that needs to be clarified (70, 71). The effects of ticagrelor on inflammatory conditions have been studied in a variety of animal models (35, 72–75), although most of the studies were performed exclusively on male mice (36, 73–75). Moreover, clinical trials investigating the effects of ticagrelor in patients during inflammatory conditions, such as inflammatory factors during myocardial infarction (76) or pneumonia (75) has started but they have not provided definitive answers. So far, our data suggest that targeting P2Y12 may not be the most appropriate approach to treating females in sepsis, and more data are required to determine whether there is any sex-specificity.
Interestingly, in male mice, blocking P2Y1 shows a decrease in activity of MPO in lungs and kidney and platelet-leukocyte interaction, similarly, to blocking P2Y12. This was observed in animal models of other diseases, such as colitis (38), Alzheimer (42) and multiple sclerosis (44) when blocking P2Y1 improved the outcome of the disease. However, the data are different from what we observed upon P2Y1 deficiency. In previous studies, P2Y1 blockade and P2Y1 deficiency did show comparable results (38, 77, 78), suggesting that the discrepancy we have observed now could be due to sepsis in general or the CLP model. As previous studies investigating either P2Y1 deficiency or antagonism were performed almost exclusively in male mice (37, 38, 44, 77), our experiments were the first to investigate changes in inflammation levels in sepsis in female mice upon P2Y1 blockade and compare them with the male counterpart. In this model of sepsis, P2Y1 blockade did not improve activity of MPO in lungs and kidneys nor altered platelet interaction with other cells. Surprisingly, this is different from what we observed upon P2Y1 deficiency. This discrepancy could be due to the dose of MRS2279 used or the off-target effects of MRS2279. We have previously noted P2Y12-independent effects upon P2Y12 antagonism in vivo (26) and in vitro (79, 80) but so far, no studies have reported similar results when targeting P2Y1. Moreover, the discrepancy could be due to the fact that the P2Y1 antagonist is administered at surgery, while P2Y1 deficiency may alter organ and/or tissue during development. However, it is interesting to notice that the data appear to be sex-specific as we noted the opposite in male mice. Sex-related differences in the immune response have been reported, indicating that some sex-related differences may be germline encoded. Sex-related differences in purinergic signaling expression and responses in female mice as compared with male mice have been reported (41, 60–63). Cytokine secretion upon inflammation has shown to vary between sexes (81). Indeed, we have observed that cytokine levels such as RANTES, IL-1β, IL-10, and IL-17 are altered by blocking P2Y1 or P2Y12 differently in septic male and female mice. Hence, purinergic signaling may regulate cytokine secretion in sepsis in a sex-related manner. Blocking P2Y12 or P2Y1 has shown to change cytokine secretion in several previous studies (26, 31, 74, 82, 83) but changes between male and female mice have not been measured. It would be interesting to investigate thoroughly whether P2Y1 and P2Y12 are expressed differently in the immune system of males and females. One study investigated LPS-induced lung injury in female mice that revealed platelet activation and neutrophil infiltration is dependent on P2Y1 (41). This is a different sepsis model than the one used in this study, but it may suggest that modulating the P2Y1 receptor alters platelet response during LPS-induced inflammation. Furthermore, changes in purinergic signaling may be hormonal-dependent. Indeed, other purinergic receptors such as a P2X7 appeared to be hormonal-dependent (14, 16), hence it would be interesting to determine whether the expression of P2Y1 or P2Y12 is related to hormone secretion.
To determine whether this sex-specificity of P2Y1 and P2Y12 activation was observed in human cells, we investigated whether PBMCs respond to purinergic signaling blockade in vitro when stimulated to LPS. We have previously investigated P2Y12 signaling pathways in human T cells and reported that human T cells express P2Y12 and P2Y12 receptors and blockade altered T cell proliferation and activation in a stimuli-dependent manner (80). However, no experiments have compared P2Y1 or P2Y12 blockade in LPS-activated PBMCs obtained from male and female donors. Our data exhibited that PBMCs obtained from male donors blocked by either P2Y1 or P2Y12 inhibitors similarly altered CD4+ and CD8+ populations, and these changes depended on whether the cells had been activated with LPS. These observations are supportive of our in vivo data, where blocking either P2Y1 or P2Y12 improved the outcome of sepsis similarly. However, in PBMCs obtained from female donors, the CD8+ population growth was altered only when P2Y1 was blocked. This is in line with the data we obtained in septic female P2Y1 deficient mice. Data on female mice have shown that P2Y1 blockade but not P2Y12 could decrease leukocyte chemotaxis and platelet-leukocyte interaction (41). Taken together the data support that blocking P2Y1 could be a more effective strategy to modulate the immune response in female mice. In future studies, it will be important to evaluate whether these changes are due to sex-related differences in P2Y1 and P2Y12 receptor expression. There are studies investigating how P2Y1 can regulate CD4+ differentiation in vivo and in vitro. P2Y1 deficiency decreased CD4+ population growth and in particular Th17 differentiation during colonic inflammation (77). In vitro experiments in human PBMCs have shown that blocking P2Y1 could modulate CD4+ cell activation (84). Indeed, mRNA levels of P2Y1 are higher than P2Y12 (84). In all the studies, no differentiation between male and female cells was analyzed so more data are required before we can identify the most appropriate therapeutic strategy for treating sepsis in both sexes.
The current study has several limitations. First, we have selected the time point of 24 hours post-CLP. It has been shown that at 24 hours organ injury (such as lungs, kidneys, and heart) (48, 50, 85–87), and cytokine levels increase in blood samples of CLP mice (48, 85) are comparable to that noted in septic patients (49, 57). However, to deepen our understanding of sex-related differences in sepsis, exploring a variety of time points is essential. Second, it is true that sepsis can occur at any age, but infants, people with chronic conditions, people with weakened immune systems, and older adults are at high risk (as stated by the Center for Disease Control and Prevention). Indeed, the incidence of sepsis increases with age and is associated with extremely high mortality rates (88, 89). Here, we have selected 8-10-week-old mice, which is comparable to a fertile adult in humans, however, it would be interesting to investigate older or younger male and female mice. These experiments could also investigate whether hormonal changes could be related to P2Y1 and P2Y12 expression. Finally, exploring a range of doses for both antagonists will also be an essential future study to determine whether the sex-related effects are dose-dependent.
In conclusion, modulating P2Y12 or P2Y1 receptors can be effective in improving sepsis outcomes, depending on the sex. Targeting purinergic signaling represents a promising therapy for sepsis and identifying sex-specific purinergic signaling may lead to more sex-related targeted therapies in sepsis.
Data availability statement
The raw data supporting the conclusions of this article will be made available by the authors, without undue reservation.
Ethics statement
The Institutional Review Board of North Dakota State University approved the study (#3735). The patients/participants provided their written informed consent to participate in this study. The animal study was reviewed and approved by Institutional Animal Care and Use Committee Protocol #A21040 at North Dakota State University (Fargo, ND, USA).
Author contributions
EA collected materials, performed experiments, and analyzed data. PE collected materials performed experiments and analyzed data. SA analyzed data and wrote the manuscript. GPD analyzed data and wrote the manuscript. SPK analyzed data and wrote the manuscript. LEK analyzed data and wrote the manuscript. EL designed the research study, analyzed data, and wrote the manuscript. All authors contributed to the article and approved the submitted version.
Funding
This work was supported by the National Institute of Health, grant AI156627-01 to EL, grant HL155694 to SPK, and by the Defense Threat Reduction Agency (Grant #HDTRA11910012) to LEK.
Acknowledgments
We would like to thank Jamie Brown and Kimberly Peterson from Lillestol Research LLC (Fargo, ND) for collaborating with us in obtaining the human blood samples.
Conflict of interest
The authors declare that the research was conducted in the absence of any commercial or financial relationships that could be construed as a potential conflict of interest.
Publisher’s note
All claims expressed in this article are solely those of the authors and do not necessarily represent those of their affiliated organizations, or those of the publisher, the editors and the reviewers. Any product that may be evaluated in this article, or claim that may be made by its manufacturer, is not guaranteed or endorsed by the publisher.
Supplementary material
The Supplementary Material for this article can be found online at: https://www.frontiersin.org/articles/10.3389/fimmu.2022.1015577/full#supplementary-material
Supplementary Figure 1 | Flow cytometric gating strategy. Gating strategies are shown to define (A) platelet-CD4+ T cell aggregates, (B) platelet-CD41+ cell aggregates (C) p-selectin surface expression, and (D, E) T cell populations.
Abbreviations
CLP, cecal ligation and double puncture; IL-1β, interleukin-1 β; MPO, myeloperoxidase; PBMCs, peripheral blood mononuclear cells; PBS, phosphate-buffered saline; PF4, platelet factor 4.
References
1. Singer M, Deutschman CS, Seymour CW, Shankar-Hari M, Annane D, Bauer M, et al. The third international consensus definitions for sepsis and septic shock (Sepsis-3). JAMA (2016) 315(8):801–10. doi: 10.1001/jama.2016.0287
2. Martin-Loeches I, Levy MM, Artigas A. Management of severe sepsis: advances, challenges, and current status. Drug Des Devel Ther (2015) 9:2079–88. doi: 10.2147/DDDT.S78757
3. Dejager L, Pinheiro I, Dejonckheere E, Libert C. Cecal ligation and puncture: the gold standard model for polymicrobial sepsis? Trends Microbiol (2011) 19(4):198–208. doi: 10.1016/j.tim.2011.01.001
4. Angele MK, Pratschke S, Hubbard WJ, Chaudry IH. Gender differences in sepsis: cardiovascular and immunological aspects. Virulence (2014) 5(1):12–9. doi: 10.4161/viru.26982
5. Diodato MD, Knoferl MW, Schwacha MG, Bland KI, Chaudry IH. Gender differences in the inflammatory response and survival following haemorrhage and subsequent sepsis. Cytokine (2001) 14(3):162–9. doi: 10.1006/cyto.2001.0861
6. Eachempati SR, Hydo L, Barie PS. Gender-based differences in outcome in patients with sepsis. Arch Surg (1999) 134(12):1342–7. doi: 10.1001/archsurg.134.12.1342
7. Failla KR, Connelly CD. Systematic review of gender differences in sepsis management and outcomes. J Nurs Scholarsh. (2017) 49(3):312–24. doi: 10.1111/jnu.12295
8. Schroder J, Kahlke V, Book M, Stuber F. Gender differences in sepsis: genetically determined? Shock (2000) 14(3):307–10. doi: 10.1097/00024382-200014030-00011
9. Ferretti MT, Iulita MF, Cavedo E, Chiesa PA, Schumacher Dimech A, Santuccione Chadha A, et al. Sex differences in Alzheimer disease - the gateway to precision medicine. Nat Rev Neurol (2018) 14(8):457–69. doi: 10.1038/s41582-018-0032-9
10. Dorak MT, Karpuzoglu E. Gender differences in cancer susceptibility: an inadequately addressed issue. Front Genet (2012) 3:268. doi: 10.3389/fgene.2012.00268
11. Sceats LA, Morris AM, Bundorf MK, Park KT, Kin C. Sex differences in treatment strategies among patients with ulcerative colitis: A retrospective cohort analysis of privately insured patients. Dis Colon Rectum. (2019) 62(5):586–94. doi: 10.1097/DCR.0000000000001342
12. Cekic C, Linden J. Purinergic regulation of the immune system. Nat Rev Immunol (2016) 16(3):177–92. doi: 10.1038/nri.2016.4
13. Ledderose C, Bao Y, Kondo Y, Fakhari M, Slubowski C, Zhang J, et al. Purinergic signaling and the immune response in sepsis: A review. Clin Ther (2016) 38(5):1054–65. doi: 10.1016/j.clinthera.2016.04.002
14. Beaucage KL, Xiao A, Pollmann SI, Grol MW, Beach RJ, Holdsworth DW, et al. Loss of P2X7 nucleotide receptor function leads to abnormal fat distribution in mice. Purinergic Signal (2014) 10(2):291–304. doi: 10.1007/s11302-013-9388-x
15. Lu Y, Jiang Q, Yu L, Lu ZY, Meng SP, Su D, et al. 17beta-estradiol rapidly attenuates P2X3 receptor-mediated peripheral pain signal transduction via ERalpha and GPR30. Endocrinol (2013) 154(7):2421–33. doi: 10.1210/en.2012-2119
16. Madec S, Rossi C, Chiarugi M, Santini E, Salvati A, Ferrannini E, et al. Adipocyte P2X7 receptors expression: a role in modulating inflammatory response in subjects with metabolic syndrome? Atherosclerosis (2011) 219(2):552–8. doi: 10.1016/j.atherosclerosis.2011.09.012
17. Sivcev S, Slavikova B, Rupert M, Ivetic M, Nekardova M, Kudova E, et al. Synthetic testosterone derivatives modulate rat P2X2 and P2X4 receptor channel gating. J Neurochem (2019) 150(1):28–43. doi: 10.1111/jnc.14718
18. Bjorkgren I, Lishko PV. Purinergic signaling in testes revealed. J Gen Physiol (2016) 148(3):207–11. doi: 10.1085/jgp.201611676
19. Burnstock G. Purinergic signalling in endocrine organs. Purinergic Signal (2014) 10(1):189–231. doi: 10.1007/s11302-013-9396-x
20. Martinez-Ramirez AS, Vazquez-Cuevas FG. Purinergic signaling in the ovary. Mol Reprod Dev (2015) 82(11):839–48. doi: 10.1002/mrd.22537
21. Zimon A, Erat A, Von Wald T, Bissell B, Koulova A, Choi CH, et al. Genes invoked in the ovarian transition to menopause. Nucleic Acids Res (2006) 34(11):3279–87. doi: 10.1093/nar/gkl387
22. Kim S, Kunapuli SP. P2Y12 receptor in platelet activation. Platelets (2011) 22(1):56–60. doi: 10.3109/09537104.2010.497231
23. Liverani E, Kilpatrick LE, Tsygankov AY, Kunapuli SP. The role of P2Y(1)(2) receptor and activated platelets during inflammation. Curr Drug Targets. (2014) 15(7):720–8. doi: 10.2174/1389450115666140519162133
24. Murugappa S, Kunapuli SP. The role of ADP receptors in platelet function. Front Biosci (2006) 11:1977–86. doi: 10.2741/1939
25. Kahner BN, Shankar H, Murugappan S, Prasad GL, Kunapuli SP. Nucleotide receptor signaling in platelets. J Thromb Haemost (2006) 4(11):2317–26. doi: 10.1111/j.1538-7836.2006.02192.x
26. Liverani E, Rico MC, Tsygankov AY, Kilpatrick LE, Kunapuli SP. P2Y12 receptor modulates sepsis-induced inflammation. Arterioscler Thromb Vasc Biol (2016) 36(5):961–71. doi: 10.1161/ATVBAHA.116.307401
27. Coleman JR, Moore EE, Kelher MR, Samuels JM, Cohen MJ, Sauaia A, et al. Female platelets have distinct functional activity compared with male platelets: Implications in transfusion practice and treatment of trauma-induced coagulopathy. J Trauma Acute Care Surg (2019) 87(5):1052–60. doi: 10.1097/TA.0000000000002398
28. Leng XH, Hong SY, Larrucea S, Zhang W, Li TT, Lopez JA, et al. Platelets of female mice are intrinsically more sensitive to agonists than are platelets of males. Arterioscler Thromb Vasc Biol (2004) 24(2):376–81. doi: 10.1161/01.ATV.0000110445.95304.91
29. Crain JM, Nikodemova M, Watters JJ. Expression of P2 nucleotide receptors varies with age and sex in murine brain microglia. J Neuroinflammation. (2009) 6:24. doi: 10.1186/1742-2094-6-24
30. Quinton TM, Murugappan S, Kim S, Jin J, Kunapuli SP. Different G protein-coupled signaling pathways are involved in alpha granule release from human platelets. J Thromb Haemost. (2004) 2(6):978–84. doi: 10.1111/j.1538-7836.2004.00741.x
31. Albayati S, Vemulapalli H, Tsygankov AY, Liverani E. P2Y12 antagonism results in altered interactions between platelets and regulatory T cells during sepsis. J Leukoc Biol (2021) 110(1):141–53. doi: 10.1002/JLB.3A0220-097R
32. Bentur OS, Li J, Jiang CS, Martin LH, Kereiakes DJ, Coller BS. Application of auxiliary VerifyNow point-of-Care assays to assess the pharmacodynamics of RUC-4, a novel alphaIIbbeta3 receptor antagonist. TH Open (2021) 5(3):e449–e60. doi: 10.1055/s-0041-1732343
33. Pavlovic N, Kopsida M, Gerwins P, Heindryckx F. Inhibiting P2Y12 in macrophages induces endoplasmic reticulum stress and promotes an anti-tumoral phenotype. Int J Mol Sci (2020) 21(21):8177. doi: 10.3390/ijms21218177
34. Huang B, Qian Y, Xie S, Ye X, Chen H, Chen Z, et al. Ticagrelor inhibits the NLRP3 inflammasome to protect against inflammatory disease independent of the P2Y12 signaling pathway. Cell Mol Immunol (2021) 18(5):1278–89. doi: 10.1038/s41423-020-0444-5
35. Ye Y, Birnbaum GD, Perez-Polo JR, Nanhwan MK, Nylander S, Birnbaum Y. Ticagrelor protects the heart against reperfusion injury and improves remodeling after myocardial infarction. Arterioscler Thromb Vasc Biol (2015) 35(8):1805–14. doi: 10.1161/ATVBAHA.115.305655
36. Yu C, Gao CM, Xie N, Wang XQ, Ma YQ. Effect of ticagrelor on acute kidney injury in septic rats and its underlying mechanism. Exp Ther Med (2021) 21(5):475. doi: 10.3892/etm.2021.9906
37. Tian G, Zhou J, Quan Y, Kong Q, Wu W, Liu X. P2Y1 receptor agonist attenuates cardiac fibroblasts activation triggered by TGF-beta1. Front Pharmacol (2021) 12:627773. doi: 10.3389/fphar.2021.627773
38. Zhang C, Qin J, Zhang S, Zhang N, Tan B, Siwko S, et al. ADP/P2Y1 aggravates inflammatory bowel disease through ERK5-mediated NLRP3 inflammasome activation. Mucosal Immunol (2020) 13(6):931–45. doi: 10.1038/s41385-020-0307-5
39. Banka AL, Eniola-Adefeso O. Method article: an in vitro blood flow model to advance the study of platelet adhesion utilizing a damaged endothelium. Platelets (2022) 33(5):692–9. doi: 10.1080/09537104.2021.1988550
40. Wang P, Hu XX, Li YH, Gao NY, Chen GQ, Chen JL. Inhibitory effect of resveratrol on the pharmacokinetics of ticagrelor in vivo and in vitro. Can J Physiol Pharmacol (2021) 99(8):821–6. doi: 10.1139/cjpp-2020-0512
41. Amison RT, Arnold S, O'Shaughnessy BG, Cleary SJ, Ofoedu J, Idzko M, et al. Lipopolysaccharide (LPS) induced pulmonary neutrophil recruitment and platelet activation is mediated via the P2Y1 and P2Y14 receptors in mice. Pulm Pharmacol Ther (2017) 45:62–8. doi: 10.1016/j.pupt.2017.05.005
42. Delekate A, Fuchtemeier M, Schumacher T, Ulbrich C, Foddis M, Petzold GC. Metabotropic P2Y1 receptor signalling mediates astrocytic hyperactivity in vivo in an alzheimer's disease mouse model. Nat Commun (2014) 5:5422. doi: 10.1038/ncomms6422
43. Martorell A, Wellmann M, Guiffa F, Fuenzalida M, Bonansco C. P2Y1 receptor inhibition rescues impaired synaptic plasticity and astroglial Ca(2+)-dependent activity in the epileptic hippocampus. Neurobiol Dis (2020) 146:105132. doi: 10.1016/j.nbd.2020.105132
44. Nobili P, Shen W, Milicevic K, Bogdanovic Pristov J, Audinat E, Nikolic L. Therapeutic potential of astrocyte purinergic signalling in epilepsy and multiple sclerosis. Front Pharmacol (2022) 13:900337. doi: 10.3389/fphar.2022.900337
45. Gerdes N, Zhu L, Ersoy M, Hermansson A, Hjemdahl P, Hu H, et al. Platelets regulate CD4(+) T-cell differentiation via multiple chemokines in humans. Thromb Haemost (2011) 106(2):353–62. doi: 10.1160/TH11-01-0020
46. Hamzeh-Cognasse H, Cognasse F, Palle S, Chavarin P, Olivier T, Delezay O, et al. Direct contact of platelets and their released products exert different effects on human dendritic cell maturation. BMC Immunol (2008) 9:54. doi: 10.1186/1471-2172-9-54
47. Tan S, Li S, Min Y, Gistera A, Moruzzi N, Zhang J, et al. Platelet factor 4 enhances CD4(+) T effector memory cell responses via akt-PGC1alpha-TFAM signaling-mediated mitochondrial biogenesis. J Thromb Haemost (2020) 18(10):2685–700. doi: 10.1111/jth.15005
48. Fang Z, Zhang X, Huang Y, Zhou H, Lu Y, Sun Y, et al. Piperlongumin improves survival in the mouse model of sepsis: Effect on coagulation factors and lung inflammation. Inflammation (2022). doi: 10.1007/s10753-022-01709-x
49. Gong W, Wen H. Sepsis induced by cecal ligation and puncture. Methods Mol Biol (2019) 1960:249–55. doi: 10.1007/978-1-4939-9167-9_22
50. Qiu W, An S, Wang T, Li J, Yu B, Zeng Z, et al. Melatonin suppresses ferroptosis via activation of the Nrf2/HO-1 signaling pathway in the mouse model of sepsis-induced acute kidney injury. Int Immunopharmacol (2022) 112:109162. doi: 10.1016/j.intimp.2022.109162
51. Kim WY, Hong SB. Sepsis and acute respiratory distress syndrome: Recent update. Tuberc Respir Dis (Seoul). (2016) 79(2):53–7. doi: 10.4046/trd.2016.79.2.53
52. Peerapornratana S, Manrique-Caballero CL, Gomez H, Kellum JA. Acute kidney injury from sepsis: current concepts, epidemiology, pathophysiology, prevention and treatment. Kidney Int (2019) 96(5):1083–99. doi: 10.1016/j.kint.2019.05.026
53. Khan AA, Alsahli MA, Rahmani AH. Myeloperoxidase as an active disease biomarker: Recent biochemical and pathological perspectives. Med Sci (Basel). (2018) 6(2):33. doi: 10.3390/medsci6020033
54. Liverani E, Mondrinos MJ, Sun S, Kunapuli SP, Kilpatrick LE. Role of protein kinase c-delta in regulating platelet activation and platelet-leukocyte interaction during sepsis. PloS One (2018) 13(4):e0195379. doi: 10.1371/journal.pone.0195379
55. Russwurm S, Vickers J, Meier-Hellmann A, Spangenberg P, Bredle D, Reinhart K, et al. Platelet and leukocyte activation correlate with the severity of septic organ dysfunction. Shock (2002) 17(4):263–8. doi: 10.1097/00024382-200204000-00004
56. Shimoyama Y, Umegaki O, Kadono N, Minami T. Presepsin and platelet to lymphocyte ratio predict the progression of septic subclinical acute kidney injury to septic acute kidney injury: a pilot study. BMC Res Notes. (2022) 15(1):212. doi: 10.1186/s13104-022-06103-2
57. Wen H. Sepsis induced by cecal ligation and puncture. Methods Mol Biol (2013) 1031:117–24. doi: 10.1007/978-1-62703-481-4_15
58. Fajgenbaum DC, June CH. Cytokine storm. N Engl J Med (2020) 383(23):2255–73. doi: 10.1056/NEJMra2026131
59. Moriyama K, Nishida O. Targeting cytokines, pathogen-associated molecular patterns, and damage-associated molecular patterns in sepsis via blood purification. Int J Mol Sci (2021) 22(16):8882. doi: 10.3390/ijms22168882
60. Amison RT, Jamshidi S, Rahman KM, Page CP, Pitchford SC. Diverse signalling of the platelet P2Y1 receptor leads to a dichotomy in platelet function. Eur J Pharmacol (2018) 827:58–70. doi: 10.1016/j.ejphar.2018.03.014
61. Burnstock G. Pathophysiology and therapeutic potential of purinergic signaling. Pharmacol Rev (2006) 58(1):58–86. doi: 10.1124/pr.58.1.5
62. Burnstock G. Purinergic signalling in the reproductive system in health and disease. Purinergic Signal (2014) 10(1):157–87. doi: 10.1007/s11302-013-9399-7
63. Crain JM, Watters JJ. Estrogen and P2 purinergic receptor systems in microglia: Therapeutic targets for neuroprotection. Open Drug Discovery J (2010) 2:148–67. doi: 10.2174/1877381801002010148
64. Ozaki AF, Jackevicius CA, Chong A, Sud M, Fang J, Austin PC, et al. Hospital-level variation in ticagrelor use in patients with acute coronary syndrome. J Am Heart Assoc (2022) 11(13):e024835. doi: 10.1161/JAHA.121.024835
65. Lee YJ, Suh Y, Kim JS, Cho YH, Yun KH, Kim YH, et al. Ticagrelor monotherapy after 3-month dual antiplatelet therapy in acute coronary syndrome by high bleeding risk: The subanalysis from the TICO trial. Korean Circ J (2022) 52(4):324–37. doi: 10.4070/kcj.2021.0321
66. Gargiulo G, Serino F, Esposito G. Cardiovascular mortality in patients with acute and chronic coronary syndrome: insights from the clinical evidence on ticagrelor. Eur Rev Med Pharmacol Sci (2022) 26(7):2524–42. doi: 10.26355/eurrev_202204_28490
67. Vogel B, Baber U, Cohen DJ, Sartori S, Sharma SK, Angiolillo DJ, et al. Sex differences among patients with high risk receiving ticagrelor with or without aspirin after percutaneous coronary intervention: A subgroup analysis of the TWILIGHT randomized clinical trial. JAMA Cardiol (2021) 6(9):1032–41. doi: 10.1001/jamacardio.2021.1720
68. Schreuder MM, Badal R, Boersma E, Kavousi M, Roos-Hesselink J, Versmissen J, et al. Efficacy and safety of high potent P2Y12 inhibitors prasugrel and ticagrelor in patients with coronary heart disease treated with dual antiplatelet therapy: A sex-specific systematic review and meta-analysis. J Am Heart Assoc (2020) 9(4):e014457. doi: 10.1161/JAHA.119.014457
69. Husted S, James SK, Bach RG, Becker RC, Budaj A, Heras M, et al. The efficacy of ticagrelor is maintained in women with acute coronary syndromes participating in the prospective, randomized, PLATelet inhibition and patient outcomes (PLATO) trial. Eur Heart J (2014) 35(23):1541–50. doi: 10.1093/eurheartj/ehu075
70. Ranucci M, Aloisio T, Di Dedda U, Menicanti L, de Vincentiis C, Baryshnikova E, et al. Gender-based differences in platelet function and platelet reactivity to P2Y12 inhibitors. PloS One (2019) 14(11):e0225771. doi: 10.1371/journal.pone.0225771
71. Waissi F, Dekker M, Bank IEM, Korporaal SJA, Urbanus RT, de Borst GJ, et al. Sex differences in flow cytometry-based platelet reactivity in stable outpatients suspected of myocardial ischemia. Res Pract Thromb Haemost. (2020) 4(5):879–85. doi: 10.1002/rth2.12344
72. Mansour A, Bachelot-Loza C, Nesseler N, Gaussem P, Gouin-Thibault I. P2Y12 inhibition beyond thrombosis: Effects on inflammation. Int J Mol Sci (2020) 21(4):174793. doi: 10.3390/ijms21041391
73. Mansour SM, Abd El-Aal SA, El-Abhar HS, Ahmed KA, Awny MM. Repositioning of ticagrelor: Renoprotection mediated by modulating renin-angiotensin system, inflammation, autophagy and galectin-3. Eur J Pharmacol (2022) 918:174793. doi: 10.1016/j.ejphar.2022.174793
74. Rahman M, Gustafsson D, Wang Y, Thorlacius H, Braun OO. Ticagrelor reduces neutrophil recruitment and lung damage in abdominal sepsis. Platelets (2014) 25(4):257–63. doi: 10.3109/09537104.2013.809520
75. Sexton TR, Zhang G, Macaulay TE, Callahan LA, Charnigo R, Vsevolozhskaya OA, et al. Ticagrelor reduces thromboinflammatory markers in patients with pneumonia. JACC Basic Transl Sci (2018) 3(4):435–49. doi: 10.1016/j.jacbts.2018.05.005
76. Gao CZ, Ma QQ, Wu J, Liu R, Wang F, Bai J, et al. Comparison of the effects of ticagrelor and clopidogrel on inflammatory factors, vascular endothelium functions and short-term prognosis in patients with acute ST-segment elevation myocardial infarction undergoing emergency percutaneous coronary intervention: a pilot study. Cell Physiol Biochem (2018) 48(1):385–96. doi: 10.1159/000491768
77. Chang YY, Huan QC, Peng J, Bi WC, Zhai LX, Chen Y, et al. P2Y1R ligation suppresses Th17 cell differentiation and alleviates colonic inflammation in an AMPK-dependent manner. Front Immunol (2022) 13:820524. doi: 10.3389/fimmu.2022.820524
78. Gao YY, Gao ZY. Extracellular adenosine diphosphate stimulates CXCL10-mediated mast cell infiltration through P2Y1 receptor to aggravate airway inflammation in asthmatic mice. Front Mol Biosci (2021) 8:621963. doi: 10.3389/fmolb.2021.621963
79. Liverani E, Rico MC, Garcia AE, Kilpatrick LE, Kunapuli SP. Prasugrel metabolites inhibit neutrophil functions. J Pharmacol Exp Ther (2013) 344(1):231–43. doi: 10.1124/jpet.112.195883
80. Vemulapalli H, Albayati S, Patwa VC, Tilley DG, Tsygankov AY, Liverani E. ADP exerts P2Y12 -dependent and P2Y12 -independent effects on primary human T cell responses to stimulation. J Cell Commun Signal (2020) 14(1):111–26. doi: 10.1007/s12079-019-00540-8
81. Takahashi T, Iwasaki A. Sex differences in immune responses. Science (2021) 371(6527):347–8. doi: 10.1126/science.abe7199
82. Kuboyama K, Harada H, Tozaki-Saitoh H, Tsuda M, Ushijima K, Inoue K. Astrocytic P2Y(1) receptor is involved in the regulation of cytokine/chemokine transcription and cerebral damage in a rat model of cerebral ischemia. J Cereb Blood Flow Metab (2011) 31(9):1930–41. doi: 10.1038/jcbfm.2011.49
83. Noguchi Y, Shinozaki Y, Fujishita K, Shibata K, Imura Y, Morizawa Y, et al. Astrocytes protect neurons against methylmercury via ATP/P2Y(1) receptor-mediated pathways in astrocytes. PloS One (2013) 8(2):e57898. doi: 10.1371/journal.pone.0057898
84. Woehrle T, Ledderose C, Rink J, Slubowski C, Junger WG. Autocrine stimulation of P2Y1 receptors is part of the purinergic signaling mechanism that regulates T cell activation. Purinergic Signal (2019) 15(2):127–37. doi: 10.1007/s11302-019-09653-6
85. Anter A, Ahmed AF, Hammad ASA, Almalki WH, Abdel Hafez SMN, Kasem AW, et al. The severity of acute kidney and lung injuries induced by cecal ligation and puncture is attenuated by menthol: Role of proliferating cell nuclear antigen and apoptotic markers. Front Med (Lausanne) (2022) 9:904286. doi: 10.3389/fmed.2022.904286
86. Mao S, Lv J, Chen M, Guo N, Fang Y, Tong J, et al. Serinc2 deficiency causes susceptibility to sepsis-associated acute lung injury. J Inflamm (Lond) (2022) 19(1):9. doi: 10.1186/s12950-022-00306-x
87. Mohammad S, O'Riordan CE, Verra C, Aimaretti E, Alves GF, Dreisch K, et al. RG100204, a novel aquaporin-9 inhibitor, reduces septic cardiomyopathy and multiple organ failure in murine sepsis. Front Immunol (2022) 13:900906. doi: 10.3389/fimmu.2022.900906
88. Bicen C, Akdemir M, Turken MA, Cekok K, Ekin A, Turan AC. Analysis of risk factors affecting mortality in elderly patients operated on for hip fractures: A retrospective comparative study. Acta Orthop Traumatol Turc (2021) 55(6):493–9. doi: 10.5152/j.aott.2021.21004
Keywords: platelets (PLT), purinergic signaling, sex-related differences, sepsis, platelet activation
Citation: Amoafo EB, Entsie P, Albayati S, Dorsam GP, Kunapuli SP, Kilpatrick LE and Liverani E (2022) Sex-related differences in the response of anti-platelet drug therapies targeting purinergic signaling pathways in sepsis. Front. Immunol. 13:1015577. doi: 10.3389/fimmu.2022.1015577
Received: 09 August 2022; Accepted: 10 October 2022;
Published: 02 November 2022.
Edited by:
Christoph Thiemermann, Queen Mary University of London, United KingdomReviewed by:
Borna Relja, Otto von Guericke University, GermanyBasilia Zingarelli, Cincinnati Children’s Hospital Medical Center, United States
Copyright © 2022 Amoafo, Entsie, Albayati, Dorsam, Kunapuli, Kilpatrick and Liverani. This is an open-access article distributed under the terms of the Creative Commons Attribution License (CC BY). The use, distribution or reproduction in other forums is permitted, provided the original author(s) and the copyright owner(s) are credited and that the original publication in this journal is cited, in accordance with accepted academic practice. No use, distribution or reproduction is permitted which does not comply with these terms.
*Correspondence: Elisabetta Liverani, ZWxpc2FiZXR0YS5saXZlcmFuaUBuZHN1LmVkdQ==