- 1Division of Allergy, Pulmonary, and Critical Care Medicine, Vanderbilt University Medical Center, Nashville, TN, United States
- 2Department of Pathology, Microbiology, and Immunology, Vanderbilt University School of Medicine, Nashville, TN, United States
- 3Research Service, Tennessee Valley Healthcare System, United States Department of Veterans Affairs, Nashville, TN, United States
Innate lymphoid cells (ILCs) are a critical element of the innate immune system and are potent producers of pro-inflammatory cytokines. Recently, however, the production of the anti-inflammatory cytokine IL-10 has been observed in all ILC subtypes (ILC1s, ILC2s, and ILC3s) suggesting their ability to adopt a regulatory phenotype that serves to maintain lung and gut homeostasis. Other studies advocate a potential therapeutic role of these IL-10-expressing ILCs in allergic diseases such as asthma, colitis, and pancreatic islet allograft rejection. Herein, we review IL-10 producing ILCs, discussing their development, function, regulation, and immunotherapeutic potential through suppressing harmful inflammatory responses. Furthermore, we address inconsistencies in the literature regarding these regulatory IL-10 producing ILCs, as well as directions for future research.
Introduction
Innate lymphoid cells (ILCs) are an immune cell type that have cytokine production features of T lymphocytes but lack rearranged antigen receptors. As a result, ILCs lack antigen specificity and instead respond to alarmins released predominantly, but not exclusively, by epithelial and endothelial cells in response to damage caused by infection, injury, or disease. Currently, three groups of ILCs have been discovered and defined. Group 1 innate lymphoid cells (ILC1s), the counterpart to CD4+ T helper (Th) type 1 cells, produce interferon gamma (IFN-γ) and express the transcription factor T-bet (1, 2). Group 2 Innate Lymphoid cells (ILC2s), analogous to CD4+ Th2 cells, produce interleukin (IL)-5, IL-9, and IL-13, and express the transcription factor GATA binding protein 3 (GATA-3) (3–5). Group 3 innate lymphoid cells (ILC3s), that parallel CD4+ Th17 cells, produce IL-17 and IL-22, and express the transcription factor retinoid-related orphan receptor gamma t (RORγt) (6–9).
In the field of allergy, ILC2s are a primary focal point due to their double-edged sword nature in both the pathogenesis, and possibly prevention, of allergic disease. In the respiratory and gastrointestinal tracts, epithelial cells can be challenged by infectious agents or allergens that contain pathogen- or damage- associated molecular patterns, resulting in epithelial cell release of alarmins: IL-25, IL-33, and thymic stromal lymphopoietin (TSLP), which activate ILC2s (10–12). ILC2s respond by migrating to the challenged site where they proliferate and release the pro-inflammatory cytokines mentioned earlier at an amount that is 10-fold greater, on a per cell basis, than that released by their CD4+ Th2 counterpart (13). As a result, ILC2s can participate in host protective roles, such as the eradication of helminthic parasites through IL-5-induced eosinophil recruitment and IL-13-induced goblet hyperplasia and peristalsis (14–16). However, when ILC2s are activated by alarmins in the setting of asthma, the IL-5 they produce can lead to eosinophil activation whose products damage the airway and exacerbate bronchoconstriction. IL-13 is a central mediator of asthma by promoting bronchial hyperresponsiveness and airway remodeling, as shown in Figure 1 (17). Additionally, IL-13 disrupts the integrity of the epithelial barrier by breaking down tight junctions (18) and promoting TSLP release, leading to corticosteroid resistance in ILC2s (19).
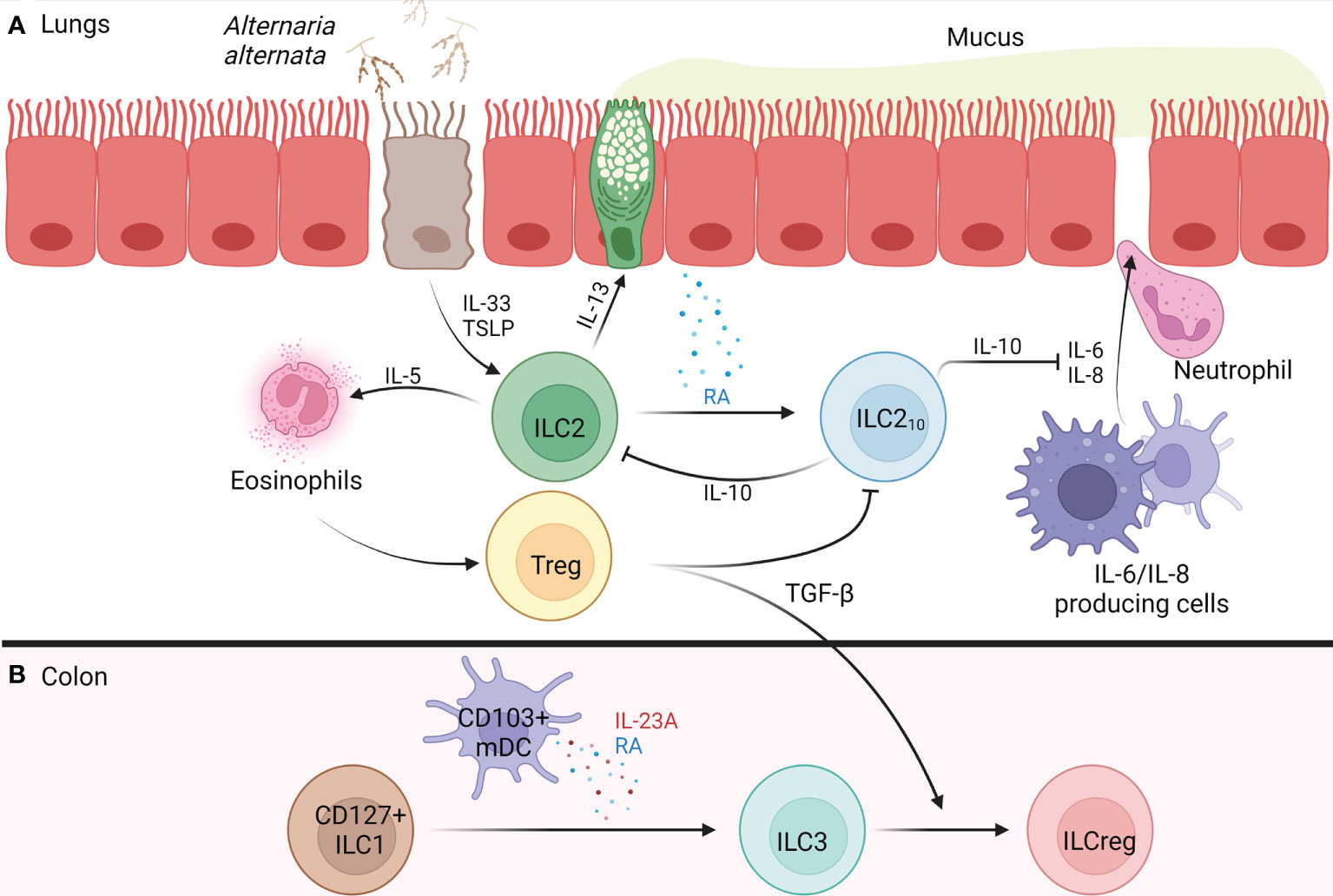
Figure 1 Development of IL-10+ ILCs in the Lung and Colon of Humans. (A) Alternaria alternata activates airway epithelium 2a) activated airway epithelium releases TSLP and IL-33 2a) IL-33 activates ILC2s, causing release of IL-5 and IL-13, while TSLP confers corticosteroid resistance 3a) IL-5 recruits and activates eosinophils 4a) IL-13 causes goblet cell hyperplasia, AHR, and release of RA from airway epithelium 5a) RA promotes the trans differentiation of ILC2s into ILC210s 6a) ILC210s release IL-10 which inhibits ILC2-mediated type 2 inflammation and maintains barrier integrity through the inhibition of IL-6 and IL-8 which function to increase barrier permeability, resulting in neutrophil transmigration 7a) Tregs form and regulate type 2 inflammation through release of TGF-β which blocks ILC210s. (B) In the colon, CD103+ mDCs release RA and IL-23A, promoting CD127+ ILC1s trans differentiation into ILC3s 2b) Tregs release TGF-β promoting the trans differentiation of ILC3s into ILCregs. AHR, airway hyperreactivity; ILC, innate lymphoid cell; ILC1, type 1 innate lymphoid cell; ILC2, type 2 innate lymphoid cell; ILC3, type 3 innate lymphoid cell; ILC210s, IL-10+ type 2 innate lymphoid cell; ILCreg, regulatory innate lymphoid cell; IL, interleukin; mDC, monocyte-derived dendritic cell; RA, retinoic acid; TGF-β, transforming growth factor beta; Treg, regulatory T cell; TSLP, thymic stromal lymphopoietin. Figure 1 was created using BioRender.com.
To promote immunologic tolerance, T regulatory cells (Tregs) derived in the thymus or extrathymically from CD4+ naïve T cells release the anti-inflammatory cytokines transforming growth factor beta (TGF-β) and IL-10 (20, 21). Interestingly, recent studies reveal a unique ability for ILCs to adopt a regulatory phenotype, similar to Tregs, through production of IL-10. Herein we review the development, function, regulation, pathogenic and potential immunotherapeutic roles of IL-10-producing ILCs, as well as address controversies and directions for future research.
Regulatory innate lymphoid cells (ILCregs)
Using IL-10-green fluorescent protein (GFP) reporter mice, a small subset of Lin- CD45+ CD127+ IL-10+ ILCs were identified in the small intestinal lamina propria (sLP) at baseline. Sample analysis of human intestinal biopsies using flow cytometry also confirmed the presence of these IL-10+ ILCs in the sLP of humans at baseline (22). These cells were named regulatory innate lymphoid cells (ILCregs) due to their absence of ILC1 markers NK1.1, NKp46, and Tbx21 (encodes T-bet); ILC2 markers ST2, killer cell lectin-like receptor subfamily G member 1 (KLRG1), and GATA-3; and ILC3 markers NKp46, CD4, and RORγt. Thus, these IL-10+ ILCs were deemed to be a new kind of ILC subset (22).
Interestingly, while ILCregs exhibited similarities to Tregs, such as their ability to produce IL-10 and TGF-β, they lacked expression of the Treg transcription factor Foxp3 (23). Unlike ILC1s, ILC2s, and ILC3s, ILCregs originate from the common helper-like innate lymphoid precursor (CHILP)-α4β7+Id2high and express Id3 which is required for their development/maintenance (1, 22). Due to the constitutive presence of ILCregs in the intestines and their expansion seen during dextran sodium sulfate (DSS)-induced colitis in Rag-/- mice (22), ILCregs have been conjectured to maintain gut tolerance through production of IL-10. When activated ILC1s and ILC3s were adoptively transferred into DSS-induced colitis Rag1-/- Il2rg-/- (ILCreg deficient) mice, severe colitis resulted, an effect that was attenuated upon ILCreg reconstitution (22). However, severe colitis resulted upon transferring IL-10Rα-/- ILC1s and ILC3s into Rag1-/- Il2rg-/- mice reconstituted with WT ILCregs, revealing that ILCregs protect against colitis through IL-10 (22). Notably, Tregs isolated from Foxp3-DTR (human diphtheria toxin receptor)-GFP mice adoptively transferred into ILC1/ILC3 reconstituted Rag1-/- Il2rg-/- mice had no effect on intestinal inflammation even after the depletion of Foxp3+ Tregs using diphtheria toxin (DT) treatment (22). However, when ILCregDTR cells were depleted in the intestines of mice following DT treatment, severe inflammation ensued (22). Importantly, these studies distinguish ILCregs as having a unique protective function in the intestines of mice.
In addition to the sLP, ILCregs have been discovered residing in the kidney’s interstitium of both humans and mice at baseline. These ILCregs produce large amounts of IL-10 and TGF-β that protect against renal ischemia/reperfusion injury (IRI), an effect that was abolished by neutralizing IL-10 and TGF-β antibodies (24). Interestingly, administration of an IL-2/anti-IL-2 monoclonal antibody complex (IL-2c) expanded ILCregs in the kidney of IRI Rag-/- mice, reducing tubular epithelial cell apoptosis and improving renal function (24). Importantly, depletion of these renal ILCregs using PC61 (an anti-CD25 antibody) showed greater kidney injury in IRI Rag-/- mice, revealing their critical role in renal protection (24). Adoptive transfer of ILCregs expanded ex vivo with IL-2c into IRI C57BL/6 mice further confirmed their protective role by restoring kidney function through the suppression of ILC1 and neutrophil infiltration and enhancing M2 macrophage generation (24). Notably, ILCregs in the kidneys reduced the frequency of ILC1s but not ILC2s or ILC3s, suggesting a pathogenic role of ILC1s in renal IRI (24) as well as differences in ILCreg function dependent on anatomical location (see Table 1).
Regulatory phenotype of ILC2s
There is also evidence that ILC2s have the capacity to produce IL-10 and may have immunoinhibitory potential. For instance, the hypoxic microenvironment of pancreatic ductal adenocarcinoma tumors (PDAC) can promote ILC2s to become regulatory IL-10+ ILC2s through the upregulation of hypoxia-inducible factor 1-alpha (HIF-1α) which binds to the Il10 promoter (27). Importantly, reoxygenation or neoadjuvant chemotherapy caused IL-10+ ILC2s to convert back into ILC2s, suggesting a regulatory plasticity. Unlike the previously described ILCregs, IL-10+ ILC2s maintained their ILC2 phenotype through the expression of Il1rl1 (ST2), KLRG1 (26) and Gata3 (28); thus, they have been termed ILC210s (see Table 1).
ILC2s treated with the common Treg polarization factors TGF-β, vitamin D, or retinoic acid (RA), became ILC210s only in the presence of RA (25). Notably, administration of a pan- retinoic acid receptor (RAR) inhibitor blocked ILC210s generation in a dose-dependent manner, revealing that RA acts through RAR to induce the ILC210 phenotype (25). In an in vitro study using air liquid interface (ALI) cultures of primary bronchial epithelial cells treated with IL-5, IL-13, and IL-33, from patients with chronic rhinosinusitis with nasal polyps (CRSwNP), only IL-13 promoted RA generation (25). This result suggests that IL-13 derived from ILC2s upregulates ILC210s by promoting RA generation from epithelial cells, which in turn downregulates the ILC2-induced type 2 inflammatory response through IL-10 release (see Figure 1). This implies that ILC2s have a mechanism to autoregulate the inflammation that they induce.
In a model of allergic lung inflammation in mice induced by either four daily intranasal administrations of IL-33 or chronic papain exposure, a population of IL-10 producing Lin- Thy1.1+ ILC2s emerged (26). Interestingly, the same population of IL-10+ ILC2s was induced by treating ILC2s in vivo with IL-2c (26). However, ILC2 production of IL-10 is not restricted to the lungs. When treating small intestinal ILC2s from naïve mice with IL-2, IL-4, IL-10, IL-27, and neuromedin U (NMU) together, these ILC2s began producing IL-10 (29). Interestingly, IL-2 and IL-4 enhanced IL-10 production by ILC2 when these cytokines were administered individually in culture (29). As a result, these experiments collectively suggest that ILC2 trans-differentiation into ILC210s is a self-amplifying process instructed by their cytokine milieu and environment.
Regulation of IL-10 producing ILCregs and ILC2s
Immune suppression is not always beneficial, as in the case of PDAC tumors where IL-10+ ILCs promote tumor growth (27). As a result, regulation of IL-10 by ILCs is crucial. A study conducted on ILC2 from WT and C3a receptor knockout (C3ar-/-) mice reported that genetic deletion of the C3a receptor resulted in significantly less IL-13, IL-5, and granulocyte-macrophage colony-stimulating factor (GM-CSF) production, while C3a signaling inhibited IL-33-induced IL-10 production from ILC210s (30). Thus, the anaphylatoxin C3a combined with IL-33 stimulation enhanced the pro-inflammatory ILC2 phenotype through inhibiting Il10 transcription and promoted ILC2 antigen-presentation to CD4+ T cells, resulting in Th2 differentiation (30). Additionally, tumor necrosis factor-like cytokine 1A (TL1A) strongly abrogated IL-10 production in ILC210s while increasing IL-5 and IL-13 production (29). Collectively, these results reveal that the regulatory phenotype adopted by ILC2s is reversible and influenced by environmental conditions.
Cytokines can also downregulate IL-10-expressing ILCs. In human ILC210s from patients with systemic sclerosis (SSc), treatment with TGF-β dramatically decreased the production of IL-10 and reduced KLRG1 expression, an ILC2 surface marker found to be required for IL-10 production (29, 31, 32). However, unlike ILC210s, ILCregs rely on TGF-β signaling for their survival and expansion (see Table 1), as seen through the effects of deleting TGF-β receptors on ILCs using Tgfbr2flox/flox;CreERT2 mice (22, 33). This finding reveals differences between ILCregs and ILC210s, potentially revealing the presence of two regulatory ILC subtypes.
Regulatory phenotype of ILC3s and ex-ILC1s
Several pieces of evidence suggest that ILC3s are plastic and can become ILCregs. A study investigating colorectal cancer (CRC) tumor infiltrating ILCs from azoxymethane/dextran sodium sulfate (AOM/DSS)-induced colitis models revealed that ILC3 numbers decreased, while ILCreg numbers increased, during CRC tumor progression (34). At the late-stage of CRC tumors, fate mapping using Rosa26-STOP-tdTomato;Rorc-Cre;IL-10-GFP lineage tracing mice followed by AOM/DSS treatment revealed former ILC3s (exILC3s) producing IL-10 and expressing Id3 (34). Using TGF-β receptor knockout mice treated with AOM/DSS, ILCreg numbers decreased while ILC3 numbers increased, causing tumor growth suppression (34). Furthermore, ILC3 treatment with a TGF-β inhibitor prevented the conversion of ILC3s to ILCregs, a result that was consistent in both the AOM/DSS-induced CRC mice and patient derived xenograft (PDX) tumors (34). Collectively, TGF-β drives the trans-differentiation of ILC3s towards ILCregs in both humans and mice. This important finding reveals that IL-10 production from ILCs is not limited to KLRG1+ ILC2s, as previously thought (29, 32), and brings to question whether ILC1s can adopt a regulatory phenotype.
CD127+ ILC1s that lost their ability to proliferate contained the capacity to reversibly differentiate into ILC3s (exILC1s) in the presence of IL-2, IL-23, and IL-1β when administered together (35). Further analysis revealed that exILC1s lost their T-bet expression and IFN-γ production, but began expressing RORγt and producing IL-22, committing to an ILC3 phenotype (35). Notably, in the presence of IL-2 and IL-12, ILC3s and exILC1s lost their RORγt and IL-22 expression while upregulating T-bet expression and IFN-γ production, committing to an ILC1 phenotype (35). In addition to the mentioned cytokines, RA signals through its receptors (RARA, RARG, and RXRG) present on CD127+ ILC1s to accelerate the differentiation of ILC1s into ILC3s (35, 36). Human monocyte derived dendritic cells (mDCs) treated with RA upregulated CD103 expression and began producing RA and IL-23A under basal conditions and lipopolysaccharide (LPS) stimulation, revealing a role CD103+ mDCs play in CD127+ ILC1s differentiation toward ILC3s (35). As a result, it is possible ILC1s can become ILCregs through their commitment to an ILC3 phenotype in the presence of CD103+ mDCs (Figure 1). However, ex vivo stimulation with IL-12/IL-15 markedly increased IL-10 production in human ILC1s revealing their direct ability to adopt a regulatory phenotype (33). These findings reveal a regulatory plasticity within all ILC subtypes, and potential crosstalk between DCs and ILCs which should be further investigated in future research.
Immunotherapeutic potential of IL-10 producing ILCs through stimulation or inhibition
Through in vivo generation and stimulation in the lungs, ILC210s show promise as potential therapeutics for allergic airway inflammation. Using CRSwNP patient nasal epithelial cells, ALI cultures co-cultured with ILC210s and challenged with grass-pollen allergen revealed that the addition of the ILC210s prevented allergen-induced epithelial barrier disintegration, an effect that was diminished upon the addition of anti-IL-10 neutralizing antibodies (32). Elevation of IL-10R surface expression on epithelial cells occurred upon allergen exposure, enhancing the ILC210-induced epithelial barrier restoration (32). As a result of this restoration, grass-pollen sublingual allergen immunotherapy (GP-SLIT) was investigated in allergic individuals. In groups treated with GP-SLIT, frequencies of ILC210s increased compared to the placebo-treated group, negatively correlating with clinical symptoms (32). This result shows promise in using GP-SLIT to induce ILC210s in atopic individuals, which function to restore barrier integrity and attenuate type 2 inflammation through IL-10 production. Furthermore, an in vitro study on nasal epithelium from allergic individuals co-cultured with ILC210s revealed that IL-10 served to maintain epithelial and endothelial barrier integrity by blocking IL-6 and IL-8, both of which promote neutrophil translocation by increasing barrier permeability as shown in Figure 1 (37, 38). In mice, IL-10 attenuated Th2-mediated allergic airway inflammation by downregulating Th2 survival through restoring granzyme B expression in CD4+ IL-10-/- cells (39).
To further investigate the immunosuppressive role of ILC210s in allergic diseases such as asthma, ILC210s and ILC2s in a 1:1 mix were adoptively transferred into Rag-/- γc-/- (T-cell, B-cell, and NK cell deficient) mice intranasally challenged with IL-33. In doing so, ILC2-dependent allergic airway hyperreactivity (AHR) was downregulated, a result that was abrogated upon the intraperitoneal administration of anti-IL-10R (40). ILC210s-induced AHR attenuation was further confirmed in mice challenged with Alternaria alternata that were adoptively transferred the same 1:1 ILC210s/ILC2s mix. The role of IL-10 was confirmed when administration of anti-IL-10R antibodies abrogated this effect (40). Collectively, in vivo generation of ILC210s in the lungs attenuates type 2 allergic responses through IL-10 production.
Another potential therapeutic role of ILC210s is the promotion of islet allograft survival in mice as measured through improved glucose tolerance (41). ILC210s were delivered to recipient mice either intravenously or through co-transplantation with the graft. Interestingly, allograft survival was increased in only the co-transplantation group, revealing a need for ILC210s to be within the graft to achieve maximal graft protection (41). Further investigation is needed to determine how these findings translate into clinical practice.
ILCregs and ILC210s – The same cell or are they different?
In this review we discussed ILCregs as those cells that express Id3, are stimulated by TGF-β, and arise from the α4β7+Id2high CHILPs or from ILC3s in the presence of TGF-β. Separately, we defined IL-10+ ILC2s as ILC210s as a consequence of their sustained expression of GATA-3 and suppression by TGF-β (see Table 1). However, whether these cells are the same or different remains to be fully defined. Notably, ILCregs arose in the gut and kidneys at steady state and during inflammation (22), while ILC210s arose in both the gut and lungs in the presence of inflammation only (25, 27). As such, further studies should be directed towards the molecular comparison of ILCregs and ILC210s to determine if their GATA-3 expression and response to TGF-ß is cell type specific or influenced by their environment/location.
As previously discussed, ILCregs devoid of all ILC markers were expressed in the sLP of mice (22). However, upon repeat of this experiment by a different group, no such cell population was found (29). Interestingly, this group discovered that only Lin- CD127+ Thy1- ILC2s expressed IL-10 in the small intestine (29). This finding revealed inconsistencies surrounding the presence and identification of ILCregs in the sLP. One reason for the inconsistent result was suggested to be caused by genetics and/or environmental factors. However, even controlling for these factors by purchasing C57BL/6 mice from three different vendors, no ILCregs were identified (29). As a result, the existence of ILCregs in mice are non-generalizable. Further studies need to investigate the contributions of other environmental influences such as inflammation or autoimmunity on the presence of ILCregs, in both the intestines of mice and humans.
Through studying the suppressive function of ILCregs in a mouse model of colitis, IL-10 inhibited the activation of both ILC1s and ILC3s, as previously discussed. However, in an in vitro study investigating the suppressive role of TGF-β and IL-10 in human ILC subsets, IL-10 inhibited cytokine production from pre-stimulated ILC2s while having no effect on pre-stimulated ILC1s (33). As a result, further studies are needed to determine the differential role of ILCregs in repressing the function of ILC1s, ILC2s, and ILC3s between mice and humans, and to determine whether this difference is influenced by the inflammatory environment.
Other roles of ILC210s remains to be investigated, such as its ability to suppress lung eosinophilia. Through treating Rag-/- mice with IL-33 and IL-2c, a significant reduction in IL-33-induced lung eosinophilia occurred with extensive generation of ILC210s (26). However, no inhibitory studies using anti-IL-10 antibodies or IL-10-/- ILC2s were performed to prove the role of ILC210s in attenuating eosinophil migration to the lungs. As a result, in vivo delivery of IL-2c should be further investigated in its efficacy as an immune-targeted therapy that could reduce eosinophilia in atopic patients as well as protect against renal IRI, colitis, allergic airway inflammation, and allograft rejection due to its ability to generate ILC210s both in vivo and in vitro.
Interestingly, a cross-sectional study comparing grass-pollen allergic (GPA) and house dust mite-allergic (HDMA) individuals to a non-atopic healthy control (NAC) revealed that ILC2s from atopic individuals fail to adopt an IL-10-producing regulatory phenotype (32). This finding reveals a possible limitation in treating allergic disease through ILC210 generation. As a result, the regulation of the IL-10 promoter in ILC2s from GPA and HDMA patients should be investigated as it could further explain the lack of immune regulation seen in atopic patients.
Conclusion
There is increasing evidence that the IL-10 produced by ILCs suppresses immune responses and could be helpful, such as in allergic disease, or harmful, such as in the setting of cancer, to patients. However, due to the limitations regarding the specific deletion of IL-10+ ILCs in vivo, these cells remain an enigma as their exact role in human or mouse disease remains unknown. For instance, there are no specific surface markers for ILCregs for which antibody depletion could target to determine their role in regulating inflammatory processes. This is an emerging field that is certainly ripe for further investigation to understand the full nature and importance of these suppressive ILCs in human health.
Author contributions
CT wrote all drafts of the manuscript and RP edited the manuscript drafts. All authors contributed to the article and approved the submitted version.
Funding
This study was supported by NIH/NIAID 5RO1 AI124456, 5RO1 AI145265, 5R21 AI145397, 2U19 AI095227, and U.S. Department of Veterans Affairs 5I01BX004299
Conflict of interest
The authors declare that the research was conducted in the absence of any commercial or financial relationships that could be construed as a potential conflict of interest.
Publisher’s note
All claims expressed in this article are solely those of the authors and do not necessarily represent those of their affiliated organizations, or those of the publisher, the editors and the reviewers. Any product that may be evaluated in this article, or claim that may be made by its manufacturer, is not guaranteed or endorsed by the publisher.
References
1. Klose CSN, Flach M, Möhle L, Rogell L, Hoyler T, Ebert K, et al. Differentiation of type 1 ILCs from a common progenitor to all helper-like innate lymphoid cell lineages. Cell (2014) 157:340–56. doi: 10.1016/J.CELL.2014.03.030
2. Szabo SJ, Kim ST, Costa GL, Zhang X, Fathman CG, Glimcher LH. A novel transcription factor, T-bet, directs Th1 lineage commitment. Cell (2000) 100:655–69. doi: 10.1016/S0092-8674(00)80702-3
3. Neill DR, Wong SH, Bellosi A, Flynn RJ, Daly M, Langford TKA, et al. Nuocytes represent a new innate effector leukocyte that mediates type-2 immunity. Nature (2010) 464:1367–70. doi: 10.1038/nature08900
4. Yagi R, Zhong C, Northrup DL, Yu F, Bouladoux N, Spencer S, et al. The transcription factor GATA3 is critical for the development of all IL-7Rα-expressing innate lymphoid cells. Immunity (2014) 40:378–88. doi: 10.1016/j.immuni.2014.01.012
5. Mjösberg JM, Trifari S, Crellin NK, Peters CP, van Drunen CM, Piet B, et al. Human IL-25-and IL-33-responsive type 2 innate lymphoid cells are defined by expression of CRTH2 and CD161. Nat Immunol (2011) 12:1055–62. doi: 10.1038/ni.2104
6. Ivanov II, McKenzie BS, Zhou L, Tadokoro CE, Lepelley A, Lafaille JJ, et al. The orphan nuclear receptor RORγt directs the differentiation program of proinflammatory IL-17+ T helper cells. Cell (2006) 126:1121–33. doi: 10.1016/J.CELL.2006.07.035
7. Cupedo T, Crellin NK, Papazian N, Rombouts EJ, Weijer K, Grogan JL, et al. Human fetal lymphoid tissue-inducer cells are interleukin 17-producing precursors to RORC + CD127 + natural killer-like cells. Nat Immunol (2008) 10:66–74. doi: 10.1038/ni.1668
8. Cella M, Fuchs A, Vermi W, Facchetti F, Otero K, Lennerz JKM, et al. A human NK cell subset provides an innate source of IL-22 for mucosal immunity. Nature (2009) 457:722–5. doi: 10.1038/nature07537
9. Artis D, Spits H. The biology of innate lymphoid cells. Nature (2015) 517:293–301. doi: 10.1038/nature14189
10. Mjösberg J, Bernink J, Golebski K, Karrich JJ, Peters CP, Blom B, et al. The transcription factor GATA3 is essential for the function of human type 2 innate lymphoid cells. Immunity (2012) 37:649–59. doi: 10.1016/J.IMMUNI.2012.08.015
11. Salimi M, Barlow JL, Saunders SP, Xue L, Gutowska-Owsiak D, Wang X, et al. A role for IL-25 and IL-33-driven type-2 innate lymphoid cells in atopic dermatitis. J Exp Med (2013) 210:2939–50. doi: 10.1084/jem.20130351
12. Nagarkar DR, Poposki JA, Tan BK, Comeau MR, Peters AT, Hulse KE, et al. Thymic stromal lymphopoietin activity is increased in nasal polyps of chronic rhinosinusitis. J Allergy Clin Immunol (2013) 132:593–600. doi: 10.1016/j.jaci.2013.04.005
13. Klein Wolterink RG J, KleinJan A, van Nimwegen M, Bergen I, de Bruijn M, Levani Y, et al. Frontline pulmonary innate lymphoid cells are major producers of IL-5 and IL-13 in murine models of allergic asthma. Eur J Immunol (2012) 42:1106–16. doi: 10.1002/eji.201142018
14. Campbell L, Hepworth MR, Whittingham-Dowd J, Thompson S, Bancroft AJ, Hayes KS, et al. ILC2s mediate systemic innate protection by priming mucus production at distal mucosal sites. J Exp Med (2019) 216:2714–23. doi: 10.1084/jem.20180610
15. Oliphant CJ, Hwang YY, Walker JA, Salimi M, Wong SH, Brewer JM, et al. MHCII-mediated dialog between group 2 innate lymphoid cells and CD4+ T cells potentiates type 2 immunity and promotes parasitic helminth expulsion. Immunity (2014) 41:283–95. doi: 10.1016/J.IMMUNI.2014.06.016
16. Oliphant CJ, Hwang YY, Walker JA, Salimi M, Wong SH, Brewer JM, et al. Article MHCII-mediated dialog between group 2 innate lymphoid cells and CD4 + T cells potentiates type 2 immunity and promotes parasitic helminth expulsion. Immunity (2014) 41:283–95. doi: 10.1016/j.immuni.2014.06.016
17. Martin LB, Kita H, Leiferman KM, Gleich GJ. Eosinophils in allergy: Role in disease, degranulation, and cytokines. Int Arch Allergy Immunol (1996) 109:207–15. doi: 10.1159/000237239
18. Sugita K, Steer CA, Martinez-Gonzalez I, Altunbulakli C, Morita H, Castro-Giner F, et al. Type 2 innate lymphoid cells disrupt bronchial epithelial barrier integrity by targeting tight junctions through IL-13 in asthmatic patients. J Allergy Clin Immunol (2018) 141:300–310.e11. doi: 10.1016/J.JACI.2017.02.038
19. Liu S, Verma M, Michalec L, Liu W, Sripada A, Rollins D, et al. Steroid resistance of airway type 2 innate lymphoid cells from patients with severe asthma: The role of thymic stromal lymphopoietin. J Allergy Clin Immunol (2018) 141:257–268.e6. doi: 10.1016/J.JACI.2017.03.032
20. Liu M, Li S, Li MO. TGF-β control of adaptive immune tolerance: A break from treg cells. Bioessays (2018) 40:1–10. doi: 10.1002/bies.201800063
21. Shevach EM, Thornton AM. tTregs, pTregs, and iTregs: Similarities and differences. Immunol Rev (2014) 259:88–102. doi: 10.1111/imr.12160
22. Wang S, Xia P, Chen Y, Yin Z, Xu Z, Correspondence ZF. Regulatory innate lymphoid cells control innate intestinal inflammation. Cell (2017) 171:201–16. doi: 10.1016/j.cell.2017.07.027
23. Serafini N, Vosshenrich CAJ, di Santo JP. Transcriptional regulation of innate lymphoid cell fate. Nat Rev Immunol (2015) 15:415–28. doi: 10.1038/nri3855
24. Cao Q, Wang R, Wang Y, Niu Z, Chen T, Wang C, et al. Regulatory innate lymphoid cells suppress innate immunity and reduce renal ischemia/reperfusion injury. Kidney Int (2020) 97:130–42. doi: 10.1016/j.kint.2019.07.019
25. Morita H, Kubo T, Tec S, Ravindran A, Soyka MB, Ottavio Rinaldi A, et al. Induction of human regulatory innate lymphoid cells from group 2 innate lymphoid cells by retinoic acid. J Allergy Clin Immunol (2019) 143:2190–201. doi: 10.1016/j.jaci.2018.12.1018
26. Seehus CR, Kadavallore A, de la Torre B, Yeckes AR, Wang Y, Tang J, et al. Alternative activation generates IL-10 producing type 2 innate lymphoid cells. Nat Commun (2017) 8:1–13. doi: 10.1038/s41467-017-02023-z
27. Ye L, Jin K, Liao Z, Xiao Z, Xu H, Lin X, et al. Hypoxia-reprogrammed regulatory group 2 innate lymphoid cells promote immunosuppression in pancreatic cancer. EBioMedicine (2022) 79:1–15. doi: 10.1016/j.ebiom.2022.104016
28. Hoyler T, Klose CSN, Souabni A, Turqueti-Neves A, Pfeifer D, Rawlins EL, et al. The transcription factor GATA3 controls cell fate and maintenance of type 2 innate lymphoid cells Europe PMC funders group. Immunity (2012) 37:634–48. doi: 10.1016/j.immuni.2012.06.020
29. Bando JK, Gilfillan S, di Luccia B, Fachi JL, Sécca C, Cella M, et al. ILC2s are the predominant source of intestinal ILC-derived IL-10. J Exp Med (2019) 217:1–9. doi: 10.1084/jem.20191520
30. Gour N, Smole U, Yong H-M, Lewkowich IP, Yao N, Singh A, et al. C3a is required for ILC2 function in allergic airway inflammation. Mucosal Immunol (2018) 11:1653–62. doi: 10.1038/s41385-018-0064-x
31. Laurent P, Allard B, Manicki P, Jolivel V, Levionnois E, Jeljeli M, et al. TGFβ promotes low IL10-producing ILC2 with profibrotic ability involved in skin fibrosis in systemic sclerosis. Ann Rheum Dis (2021) 80:1594–603. doi: 10.1136/annrheumdis-2020-219748
32. Golebski K, Layhadi JA, Sahiner U, Steveling-Klein EH, Lenormand MM, Li RCY, et al. Induction of IL-10-producing type 2 innate lymphoid cells by allergen immunotherapy is associated with clinical response. Immunity (2021) 54:291–307.e7. doi: 10.1016/J.IMMUNI.2020.12.013
33. Bonne-Année S, Bush MC, Nutman TB. Differential modulation of human innate lymphoid cell (ILC) subsets by IL-10 and TGF-β. Sci Rep (2019) 9:1–10. doi: 10.1038/s41598-019-50308-8
34. Wang S, Qu Y, Xia P, Chen Y, Zhu X, Zhang J, et al. Transdifferentiation of tumor infiltrating innate lymphoid cells during progression of colorectal cancer. Cell Res (2020) 30:610–22. doi: 10.1038/s41422-020-0312-y
35. Bernink JH, Krabbendam L, Germar K, de Jong E, Gronke K, Kofoed-Nielsen M, et al. Interleukin-12 and -23 control plasticity of Cd127+ group 1 and group 3 innate lymphoid cells in the intestinal lamina propria. Immunity (2015) 43:146–60. doi: 10.1016/J.IMMUNI.2015.06.019
36. Mielke LA, Jones SA, Raverdeau M, Higgs R, Stefanska A, Groom JR, et al. Retinoic acid expression associates with enhanced IL-22 production by γδ T cells and innate lymphoid cells and attenuation of intestinal inflammation. J Exp Med (2013) 210:1117–24. doi: 10.1084/jem.20121588
37. Yu H, Huang X, Ma Y, Gao M, Wang O, Gao T, et al. Interleukin-8 regulates endothelial permeability by down-regulation of tight junction but not dependent on integrins induced focal adhesions. Int J Biol Sci (2013) 9:966–79. doi: 10.7150/ijbs.6996
38. Al-Sadi R, Ye D, Boivin M, Guo S, Hashimi M. Interleukin-6 modulation of intestinal epithelial tight junction permeability is mediated by JNK pathway activation of claudin-2 gene. PloS One (2014) 9:85345. doi: 10.1371/journal.pone.0085345
39. Coomes SM, Kannan Y, Pelly VS, Entwistle LJ, Guidi R, Perez-Lloret J, et al. CD4+ Th2 cells are directly regulated by IL-10 during allergic airway inflammation. Nature (2017) 10:150–61. doi: 10.1038/mi.2016.47
40. Howard E, Lewis G, Galle-Treger L, Hurrell BP, Helou DG, Shafiei-Jahani P, et al. IL-10 production by ILC2s requires blimp-1 and cMaf, modulates cellular metabolism, and ameliorates airway hyperreactivity. J Allergy Clin Immunol (2021) 147:1281–1295.e5. doi: 10.1016/J.JACI.2020.08.024
Keywords: regulatory, innate, lymphoid, IL-10, cell
Citation: Thomas CM and Peebles RS Jr (2022) Development and function of regulatory innate lymphoid cells. Front. Immunol. 13:1014774. doi: 10.3389/fimmu.2022.1014774
Received: 08 August 2022; Accepted: 20 September 2022;
Published: 04 October 2022.
Edited by:
Fu-Dong Shi, Tianjin Medical University General Hospital, ChinaReviewed by:
Hirohito Kita, Mayo Clinic, United StatesMinshu Li, Tianjin Medical University General Hospital, China
Wenyan He, Beijing Tiantan Hospital, Capital Medical University, China
Copyright © 2022 Thomas and Peebles. This is an open-access article distributed under the terms of the Creative Commons Attribution License (CC BY). The use, distribution or reproduction in other forums is permitted, provided the original author(s) and the copyright owner(s) are credited and that the original publication in this journal is cited, in accordance with accepted academic practice. No use, distribution or reproduction is permitted which does not comply with these terms.
*Correspondence: R. Stokes Peebles Jr, c3Rva2VzLnBlZWJsZXNAdmFuZGVyYmlsdC5lZHU=