- 1Student Research Committee, Faculty of Medicine, Shahid Beheshti University of Medical Sciences, Tehran, Iran
- 2Faculty of Medicine, Medical University of Qom, Qom, Iran
- 3Liver and Pancreatobiliary Diseases Research Center, Digestive Disease Research Institute, Shariati Hospital, Tehran University of Medical Sciences, Tehran, Iran
- 4Department of Biology, Faculty of Science, University of British Columbia, Kelowna, BC, Canada
- 5Faculty of Medicine, Shahid Beheshti University of Medical Sciences, Tehran, Iran
- 6Critical Care Quality Improvement Research Center, Loghman Hakim Hospital, Shahid Beheshti University of Medical Sciences, Tehran, Iran
Background: Multiple sclerosis (MS) is a debilitating neurodegenerative disorder characterized by axonal damage, demyelination, and perivascular inflammatory lesions in the white matter of the central nervous system (CNS). Kynurenine pathway (KP), which is the major route of tryptophan (TRP) metabolism, generates a variety of neurotoxic as well as neuroprotective compounds, affecting MS pathology and the severity of impairments. Alterations in KP have been described not only in MS, but also in various psychiatric and neurodegenerative diseases. The purpose of this systematic review is to investigate the previously reported dysregulation of KP and differences in its metabolites and enzymes in patients with MS compared to healthy control subjects.
Method: Electronic databases of PubMed, Scopus, Cochrane Database of Systematic Reviews, and Web of Science were searched to identify studies measuring concentrations of KP metabolites and enzymes in MS patients and control subjects. The following metabolites and enzymes implicated in the KP were investigated: TRP, kynurenine (KYN), kynurenic acid (KYNA), quinolinic acid (QUIN), picolinic acid (PIC), hydroxyindoleacetic acid (HIAA), indoleamine 2,3-dioxygenase (IDO), kynurenine aminotransferase (KAT), and their related ratios.
Result: Ten studies were included in our systematic review. Our review demonstrates that IDO expression is reduced in the peripheral blood mononuclear cells (PBMCs) of MS patients compared to healthy controls. Also, increased levels of QUIN and QUIN/KYNA in the serum and cerebrospinal fluid (CSF) of MS patients is observed. Differences in levels of other metabolites and enzymes of KP are also reported in some of the reviewed studies, however there are discrepancies among the included reports.
Conclusion: The results of this investigation suggest a possible connection between alterations in the levels of KP metabolite or enzymes and MS. QUIN levels in CSF were higher in MS patients than in healthy controls, suggesting that QUIN may be involved in the pathogenesis of MS. The data indicate that differences in the serum/blood or CSF levels of certain KP metabolites and enzymes could potentially be used to differentiate between MS patients and control subjects.
Introduction
Multiple sclerosis (MS) is one of the most prevalent neurological disorders worldwide, with an annual incidence rate of approximately 2 per 100,000 (1). MS is a disabling neurodegenerative, autoimmune, inflammatory, and demyelinating disease of the central nervous system (CNS) (2), predominantly affecting young adults during their most productive years which is from 20 to 50 (3, 4).
MS is characterized by axonal damage, demyelination, and perivascular inflammatory lesions in the CNS white matter. T lymphocytes autoreactive against CNS antigens may initiate MS pathogenesis (5). Numerous proinflammatory factors and cytokines have been found to be altered in the blood, brain tissues, and cerebrospinal fluid (CSF) of MS patients (6). The kynurenine pathway (KP) in MS is induced by proinflammatory cytokine cascades resulting in altered levels of KP metabolites (7, 8).
The KP is critical for providing cellular energy to the immune system under physiological conditions, by generating nicotinamide adenine dinucleotide (NAD+). However, the metabolites of KP with neuroactive function, collectively referred to as “kynurenines” play an important role in chronic neuroinflammation. Under inflammatory conditions, these metabolites are typically considered neurotoxic and gliotoxic due to their adverse effects on glutamatergic neurotransmission and direct toxicity towards neurons and glial cells (9, 10). In addition, tryptophan (TRP) and some intermediate metabolites in the KP exhibit immunomodulatory properties. It is also well established that the indoleamine 2,3-dioxygenase (IDO) enzyme significantly contributes to immune regulation by depleting TRP and producing kynurenine (KYN) (11, 12). A link between the aryl hydrocarbon receptor and IDO is identified in the expansion of Th17 and regulatory T cells, which plays a significant role in various autoimmune disorders and cancer (13, 14). Kynurenic acid (KYNA), which is a metabolite produced through the KP, acts as a neuroprotective agent, while quinolinic acid (QUIN) is an established neurotoxic agent (15–20). Overall, alterations in the KP and in TRP metabolism are critical in MS pathogenesis, since abnormalities in TRP metabolism have been shown to impair regulation of T cell activity (21).
In the KP, TRP is the first substrate converted to KYN by two enzymes named IDO and tryptophan-2,3-dioxygenase (TDO). KYN is subsequently catalyzed by kynurenine aminotransferase (KAT) and kynurenine-3-monooxygenase (KMO) to produce two different metabolites, 3-hydroxykynurenine (3-HK) and KYNA, respectively. 3-HK is altered to 3-hydroxyanthranillic acid (3-HANA) by an enzyme called kynureninase, and the next metabolite produced from 3-HANA is QUIN. At last, NAD+ is the ultimate metabolite of TRP produced through the KP (22).
While activation of some KP enzymes have short-term benefits, such as decreased T cell proliferation and immunosuppression, their chronic activation results in the production of neurotoxic metabolites and impairs the innate repair mechanism of remyelination (23). In MS patients, proinflammatory cytokine levels rise in the serum, resulting in IDO activation (7). TRP levels are decreased in the CSF and serum of patients with MS, suggesting the role of KP metabolism in MS pathogenesis (24–26). In all stages of MS, changes in the balance between neurotoxic and neuroprotective kynurenine metabolites have been observed (27). The CSF levels of HIAA are lower in MS patients compared to healthy control subjects (28). Finally, any alteration in each of the KP metabolites and enzymes can affect neurons and contribute to the MS pathogenesis and neurodegeneration. Therefore, the purpose of this systematic review is to ascertain whether altered metabolites and enzymes of KP can be measured in MS.
Materials and methods
Search strategy
We searched the following four databases for relevant studies published up to March 2021: PubMed, Scopus, Cochrane Database of Systematic Reviews, and Web of Science. Two authors conducted an independent search using the following query: (tryptophan OR kynurenine OR kynurenate OR kynurenic OR anthranilic OR anthranilate OR quinolinate OR quinolinic OR picolinate OR picolinic OR xanthurenic OR xanthurenate) AND (multiple sclerosis OR disseminated sclerosis). Additionally, we searched the reference lists of related articles to avoid overlooking relevant studies. All 678 papers found during the search were inserted into the Endnote software for screening. Following that, 366 duplicate publications were deleted. Subsequently, the Newcastle-Ottawa scale was used to evaluate the included studies (Table 2).
Inclusion and exclusion criteria
We included all observational studies published in English that measured KP metabolites or enzymes in MS patients and corresponding control subjects. We excluded animal studies, those that lacked a control group, and those without randomized sampling. After excluding duplicates (366), two authors independently screened the initially identified articles based on their titles and abstracts (31 studies remained). They examined the full text of the selected studies and then shortlisted the studies that met the inclusion criteria (10). Potential disagreements were resolved by a third author (Figure 1).
Data extraction
All relevant data from eligible studies were extracted, including first author name, country of origin, publication date, metabolite measurement methods, patient and control group characteristics, and measured levels of TRP, KP metabolites and enzymes. To this end, two authors extracted data independently and then compared their results. The current study was approved by the Shahid Beheshti University of Medical Sciences ethics committee IR.SBMU.RETECH.REC.1400.919.
Results
The current study has been performed based on PRISMA checklist. After screening the titles and abstracts of the initially searched studies, 41 potentially relevant studies remained, of which 17 articles were included in the systematic review following full-text screening (3, 8, 16–18, 29–39) (Figure 1). It should be regarded that we also excluded some potentially relevant studies due to their designs. For instance, some studies assessed KP metabolites only in animals, and some did not compare KP metabolites levels with a healthy control group (19, 40, 41). All studies included were published in English and published up to March 2021. Two studies assessed the variables in two distinct populations (32, 39). One study utilized two cohorts, one of which met our inclusion criteria and was included in the systematic review (16). Table 1 summarizes the study characteristics and significant findings from the included studies.
Kynurenine
Eleven studies (3, 8, 16, 29, 31, 33, 35, 37–39, 42) involving 730 individuals provided data on KYN levels (468 MS patients and 262 healthy controls). Three studies (8, 16, 33) used CSF as the sample source, four studies utilized serum (3, 29, 37, 38), one study employed peripheral blood mononuclear cells (PBMCs) (35), one study used urine as the sample source (31), and two studies utilized both CSF and serum (39, 42). Negrotto et al. (35) reported that RRMS patients in the remission phase had remarkably lower KYN levels than controls in PBMCs (P< 0.001). Moreover, according to a study by Gaetani et al. (31), RRMS patients had significantly lower KYN levels than controls in the urine sample (P= 0.010). In contrast, a study conducted by Rajda et al. (8) illustrated that MS patients had significantly higher levels of kynurenine in their CSF than healthy controls (P=0.049). Moreover, Sadowska-bartosz et al. (38) and Adamczyk-sowa et al. (29) stated in their studies that RRMS patients without treatment have considerably increased levels of KYN than healthy controls in their serum (P<0.05). Additionally, Sadowska-bartosz et al. (38) and Adamczyk-sowa et al. (29) reported significantly elevated levels of KYN in the serum of RRMS patients without treatment compared with RRMS patients treated with IFN-β1b (P<0.05 and P<0.01, respectively). Besides, Herman et al. (33) illustrated that SPMS patients had remarkably higher KYN levels in their CSF in comparison to healthy controls and RRMS patients (P<0.05). Also, in studies by Aeinehband et al. (16) on CSF (p>0.05), Mancuso et al. (3) on serum (P>0.05), Lim et al. (42) on serum and CSF, Olsson et al. on serum, and Tomosi et al. (39) on both CSF and serum samples (P=0.169 and P= 0.894, respectively), no significant difference in kynurenine levels was observed between MS patients and controls. ()
Tryptophan
Eight studies (3, 8, 16, 35, 37–39, 42) involving 559 individuals (361 MS patients and 198 healthy controls) provided data on TRP levels. Two studies (8, 16) used CSF as the sample source, three studies utilized serum (3, 37, 38), one study used PBMCs as the sample source (35), and two studies employed both CSF and serum (39, 42). Negrotto et al. (35) reported significantly higher TRP levels in the PBMCs of RRMS patients who are in the remission phase compared to controls (p=0.0007). However, in a study by Rajda et al. (8), CSF levels of TRP were insignificantly lower in MS patients than in healthy controls (p=0.12). Three other studies by Aeinehband et al. (16), Tomosi et al. (39), and Lim et al. (42) demonstrated no significant differences in the CSF levels of TRP in MS patients in comparison to controls (p>0.05, p=0.92, and P>0.05 respectively). Similarly, five studies conducted by Tomosi et al. (39), Mancuso et al. (3), Lim et al. (42), Olsson et al. (37), and Sadowska-bartosz et al. (38) reported that TRP levels in the serum samples of MS patients did not change significantly (p> 0.05).
Kynurenic acid
Eight studies (8, 16–18, 32, 37, 39, 42) collected comparative data on KYNA levels in 478 individuals (299 patients and 179 controls). Four studies (8, 16–18) used CSF as the source of the sample; two studies utilized both CSF and serum (39, 42), one study used serum samples (37), and one study employed both red blood cells (RBCs) and plasma as the source of the sample (32). Rejdak and his colleagues (17) reported in a study that KYNA levels in the CSF of MS patients in the remission phase are lower than in patients with non-inflammatory neurological disorders (P<0.01). However, in another study by Rejdak et al. (18), they reported significantly elevated KYNA levels in the CSF of RRMS patients who are in the relapse phase (P=0.01). Also, KYNA levels in the CSF were significantly lower in RRMS patients than in controls in the studies conducted by Tomosi et al. (39) (p=0.04). In a study done by Olsson et al. (37) it was shown that RRMS patients without any treatment had lower serum KYNA concentrations (P<0.05), but there were no significant differences between MS patients and controls in the studies performed by Aeinehband et al. (16) and Rajda et al. (8) (p>0.05 and p=0.42, respectively). On the other hand, Hartai et al. (32) discovered that MS patients had a remarkably higher level of KYNA in their plasma than controls (p<0.05). Additionally, Lim et al. revealed that RRMS patients have higher serum KYNA in comparison to healthy controls and progressive MS patients (P<0.0001). However, studies were done by Tomosi et al. (39) on serum, and Hartai et al. (32) on RBCs, reported no significant difference in KYNA levels between MS patients and healthy controls (p=0.16 and p>0.05, respectively).
Quinolinic acid
Three studies (8, 16, 39) collected data on QUIN levels in 151 individuals (95 MS patients and 56 healthy controls). In two studies (8, 16), the sample source was CSF; in one study, the sample source was both CSF and serum (39). Rajda et al. (8) and Tomosi et al. (39) showed that the QUIN levels in CSF were remarkably higher in MS patients than in healthy controls (p=0.001 and p<0.0001, respectively). Likewise, Tomosi et al. (39) reported a higher level of QUIN in serum samples of MS patients compared with controls (p=0.030). Conversely, there was no significant difference in the CSF levels of QUIN in a study conducted by Aeinehband et al. (16) (p>0.05).
Picolinic acid
Two studies (8, 39) collected data on the picolinic acid (PIC) levels of 93 individuals (75 MS patients and 18 controls). In one study (8), the sample was obtained from CSF, while in another study, the sample was obtained from both CSF and serum (39). Tomosi et al. found that PIC levels were significantly lower in the CSF sample of MS patients than in healthy controls (39) (p=0.02). However, according to Rajda et al. (8) and Tomosi et al. (39), there was no significant difference in PIC levels of CSF and serum samples, respectively, between MS patients and controls (p=0.59 and p=0.25, respectively).
Kynurenine, tryptophan, quinolinic acid ratio
Five studies measured KYN/TRP ratios (3, 16, 31, 39, 42) in 354 individuals (213 MS patients and 141 healthy controls). One study used urine as the sample source (31), one used CSF (16), two used both serum and CSF (39, 42), and the last study used serum samples (3). It has been elucidated by Lim et al. (42) that KYN/TRP was significantly increased in the serum samples of all the MS subtype groups (RRMS, PPMS, and SPMS) compared to healthy controls (P<0.0001). Also, Mancuso et al. (3) showed that KYN/TRP was higher in MS patients in the stable phase, MS patients in the acute phase before the initiation of glucocorticoids, and healthy controls compared with AMS patients after the initiation of glucocorticoids (P<0.05). Gaetani et al. (31) found that MS patients had remarkably lower KYN/TRP ratio in urine samples than the controls (p=0.04). In three studies were done by Aeinehband et al. (16), Mancuso et al. (3), and Tomosi et al. (39), no significant differences in KYN/TRP ratios were observed between MS patients and controls in CSF or serum samples (3, 16, 39).
Two studies (16, 39) assessed QUIN/KYNA ratios in 92 individuals (58 MS patients and 34 healthy controls). In one study, the sample source was CSF (16), while the other study used serum and CSF (39). According to the result of the study by Tomosi et al. (39), QUIN/KYNA ratios were significantly higher in MS patients compared to healthy controls in both CSF and serum samples (p=0.0015 and p=0.0183, respectively). However, Aeinehband et al. (16) reported that there was no significant difference in CSF QUIN/KYNA ratios between MS patients and controls (p>0.05).
Two studies (16, 39) involving 92 participants determined the KYNA/KYN ratio (58 MS patients and 34 healthy controls) in MS patients and controls. Tomosi et al. (39) discovered that MS patients had significantly lower KYNA/KYN ratios compared to controls when CSF samples were analyzed (p=0.0041), but there was no significant difference when serum samples were measured (p=0.0832). Aeinehband et al. (16) reported no statistically significant difference in KYNA/KYN ratios between MS patients and controls (p>0.05).
Indoleamine 2,3-dioxygenase
The expression of IDO mRNA was analyzed in six studies (3, 30, 34–37), including 1812 individuals (930 MS patients and 882 healthy controls). five studies (3, 30, 34–36) assessed IDO mRNA in mononuclear cells. One study (37) used whole blood for assessment. Four studies were done by Huang et al. (34), Mancuso et al. (3), Negrotto et al. (35), and Nejati et al. (36) reported reduced levels of IDO mRNA expression in MS patients in comparison to controls (p<0.05, p=0.01, p<0.001, and p<0.0001 respectively). However, Agliardi et al. (30) and Olsson et al. (37) showed no difference in IDO mRNA expression between MS patients and healthy controls. Additionally, Negrotto et al. (35) measured IDO1 protein levels in PBMCs and detected reduced IDO1 protein expression in MS patients when compared to healthy controls (p<0.001).
Kynurenine aminotransferase
One study, including 27 individuals (13 MS patients and 14 healthy controls) measured the enzymatic activity of both KAT I and KAT II in plasma and RBCs (32). It showed that the activities of both KAT I and KAT II enzymes are significantly higher in RBCs of MS patients compared with healthy controls (p<0.05). However, no significant difference in KAT I and KAT II plasma enzymatic activity could be detected between MS patients and healthy controls.
Discussion
In recent years, there has been mounting evidence that KP plays a significant role in neurodegenerative diseases such as MS (27). Inflammation or degeneration of the CNS induces the metabolism of TRP primarily through the production of KYN and related breakdown products (43). As MS progresses, levels of inflammatory cytokines, including interferon-γ (IFN-γ) and Tumor necrosis factor-α (TNF-α), increase, activating KP (44, 45). This study systematically reviewed 10 published primary research articles investigating differences between MS patients and healthy controls in serum, CSF, and urine levels of six major metabolites and two enzymes associated with the KP. We focused on TRP, KYN, KYNA, QUIN, and PIC levels as well as IDO mRNA expression and KAT activity.
MS pathogenesis likely involves several different mechanisms. One of the most popular hypotheses is that the infiltration of immune-activated macrophages and T cells causes death of oligodendrocytes that are responsible for myelinating axons in a healthy CNS (46, 47). KP metabolites have been suggested to promote both immune tolerance and autoimmunity according to this model of MS pathogenesis. Studies have revealed significantly lower TRP levels in the serum and CSF of MS patients, suggesting that KP activation may play a role in the disease pathogenesis (24, 25). In the human CNS, TRP is mostly metabolized through KP. Nevertheless, there are cells that do not express the entire enzymatic pathway. Only reactive microglia, infiltrating macrophages, and active neurons contain the complete pathway (43, 48). A study using urine samples from 47 Relapsing Remitting Multiple Sclerosis (RRMS) patients and 43 healthy controls reported that women had lower levels of urinary TRP and KYN than men (31). After adjusting for age and gender, urine concentrations of TRP did not show a significant difference between the RRMS and control group. Although the expanded disability scale (EDSS) has shown significant correlation with TRP urinary concentrations, disease duration has not been associated with KP metabolite levels (31). In contrast, another study reported significantly higher levels of TRP in PBMCs of RRMS patients compared to healthy controls (35). Aeinehband et al. (16) investigated cross-sectional cell-free CSF samples from patients with RRMS in both the relapse and remission phases, Primary-Progressive Multiple Sclerosis (PPMS), Secondary-Progressive Multiple Sclerosis (SPMS), for KP metabolites, using patients living with other neurological diseases, including syringomyelia, vertigo, anxiety, postcommotio syndrome, alcohol-related spastic paraparesis, neurasthenia, and unspecific sensory symptoms, as controls. They found that although there was no absolute difference in CSF concentrations of KP metabolites between PPMS and SPMS patients, PPMS patients displayed increased levels of all metabolites except for TRP in comparison to SPMS patients (16). In addition, disparities in TRP concentrations could be associated with variable characteristics of the enrolled patients reflecting the correlation between disease activity as well as disease courses with changes in KP metabolites. Moreover, inflammatory processes that initiate KP metabolism are associated with fluctuations in cytokine concentrations throughout the various phases of MS (3), which may contribute to the controversial results reported in recent publications. Future studies should compare concentrations of KP metabolites and MS disease activity in order to find novel therapeutic targets and prognostic markers.
The conversion of TRP to KYN is the first and rate-limiting step in KP metabolism, and is regulated by IDO-1 in most human tissues and TDO in liver cells (49). There have been multiple studies indicating that KYN influences the proliferation of several T cell subtypes, including CD4+ T lymphocytes and CD8+ T lymphocytes (50–52). In addition, it has been demonstrated that KYN can compromise the function of natural killer cells while simultaneously showing pro-apoptotic effects (53, 54). Therefore, KYN levels have been measured in the serum, CSF, PBMCs, and urine of MS patients and compared to healthy controls. There have been substantial differences among published results. RRMS patients had considerably lower urinary KYN concentrations when compared to healthy controls (31); however, KYN concentrations in CSF did not differ significantly between MS and non-inflammatory neurological disorders patients (16). When RRMS patients treated with IFN-β were compared to untreated RRMS patients, an increase in KYN level was observed (29, 55, 56). In contrast, another study did not find any alterations of KP activation resulting from IFN-β therapy in untreated MS patients (16).
The KYN/TRP ratio is indicative of the IDO activity as well as KP. IDO expression could potently be induced by several mediators including IFN-γ, TGF-β, toll-like receptor ligands, polyamines, TNF-α, platelet activating factor, and human immunodeficiency virus (HIV) proteins (51, 57–61). IDO mRNA expression was found to be lower in MS patients compared to healthy controls (3, 31, 34–36). Lower KYN along with a decreased urine KYN/TRP ratio in RRMS patients was found to be inversely related to the intensity of disability, suggesting a reduced TRP metabolism in the earliest stages of the disease (31, 62). Contrarily, some studies determined a significantly increased KYN/TRP ratio in MS patients compared to healthy controls (42), in addition to other studies demonstrating no meaningful difference in KYN/TRP ratios between MS patients and healthy subjects (3, 16, 39). These discrepancies could result from different biofluid samples analyzed, indicating different phases of TRP metabolism and variation in KP enzymes involved in each site. Moreover, the treatment that MS patients receive may affect the KP metabolites. For example, in the study conducted by Gaetani et al. (31), most MS patients received MS treatment, especially interferons. Thus, to elucidate the variation in KP metabolite concentrations in different organs and tissues, further controlled studies on different body fluids such as urine, blood, and CSF concurrently in the same subjects are needed.
Enzyme KAT converts KYN to KYNA (63). The KYNA/KYN ratio was increased in the CSF of MS patients compared to controls, while no difference was detected in serum ratios from the same subjects (39). Authors, in line with previous studies, also demonstrated lower CSF levels of KYNA among MS patients compared to healthy controls (17). Lim et al. demonstrated decreases in the levels of both enzymes that produce KYNA in the postmortem MS brain sections, correlating with lower levels of KYNA (64). In disagreement, KYNA concentrations were found to be significantly higher in the plasma and cerebrospinal fluid of patients with MS compared to healthy subjects. Additionally, researchers have stated that KYNA has a neuroprotective role in progressive MS (32, 65). PPMS patients are unique in having significantly increased levels of KYNA, which has been found to display neuroprotective effects both experimentally and clinically, decelerating disease progression (66, 67). Further research has revealed elevated KYNA levels during the acute relapse phase of MS (18). Conversely, SPMS patients show a decreased neuroprotection index (68), confirming the idea of altered KP activation among patients with different MS clinical courses.
In contrast to neuroprotective KYNA, QUIN is considered a neurotoxic metabolite of KP (6, 6, 19), thus, shifting KP toward KYNA instead of QUIN could be a potential therapeutic strategy. Although astrocytes do not utilize the full enzymatic pathway, they produce high levels of KYN that can be metabolized to QUIN by microglia, monocytes, or infiltrating macrophages and result in neurotoxicity (69–71). The higher QUIN/KYN ratio during the relapse phase of MS patients compared with remission phase, confirms that the QUIN-induced apoptosis of myelin producing oligodendrocytes as a sign of failed remyelination (16, 68, 69, 72). Furthermore, QUIN was found to be responsible for the impaired phosphorylation of tau protein in progressive MS (73). Indeed, QUIN could be a potential biomarker of active relapse and demyelinating phases of MS (Figure 2).
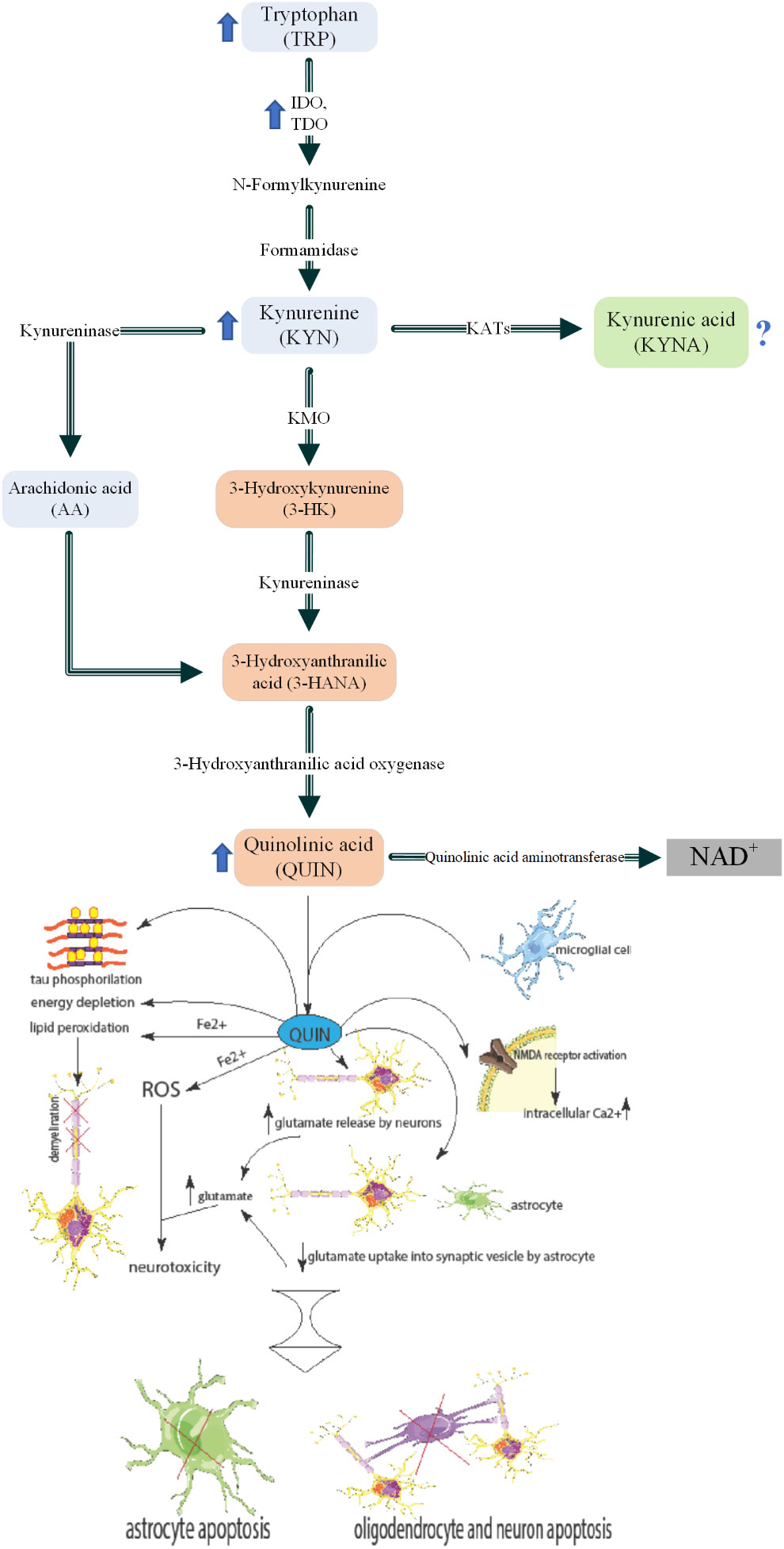
Figure 2 Kynurenine pathway and roles of QUIN in MS pathogenesis. Inflammatory cytokines such as TNF-α and INF-α which are increased in MS patients blood circulation. These induce increased activity and levels of IDO and KMO in macrophages. Higher activity of these enzymes leads to KP activation and thus high levels of QUIN is secreted to the blood. The increased QUIN can pass the BBB and enter the brain parenchyma. This QUIN is together with the QUIN secreted by microglia lead to several pathological mechanisms: 1) NMDA receptor activation in the cells and therefore higher intracellular calcium. 2) increased glutamate release by neurons and decreased glutamate uptake into synaptic vesicles by astrocytes. These cause higher glutamate levels in the micro-environment which cause neurotoxicity. 3) Increased ROS formation which causes neurotoxicity. 4) Lipid peroxidation that can lead to demyelination. 5) Energy depletion 6) Tau phosphorylation. The mentioned mechanisms, generally cause apoptosis of astrocytes, oligodendrocytes (an important cell in myelin production), and neurons. Parts of the figure were drawn by using pictures from Servier Medical Art. Servier Medical Art by Servier is licensed under a Creative Commons Attribution 3.0 Unported License (https://creativecommons.org/licenses/by/3.0/).
Very few studies have measured PIC in MS patients and controls. PIC induces inflammatory macrophage proteins in association with IFN-γ at low concentrations and acts as an activator of macrophages (74–76). This process of macrophages co-activation by PIC emphasizes the importance of PIC neuroprotection in neurodegenerative conditions (77). Decreased PIC levels in MS are consistent with its protective role in this and other degenerative disorders. Notably, another study demonstrated the inverse relationship between PIC and QUIN, with PIC being higher in RRMS groups but lower in PPMS groups (78).
In summary, the importance of KP metabolites as prognostic, diagnostic, and therapeutic biomarkers is commonly known. It is still unknown whether KP is beneficial in the pathogenesis of MS by acting as a protective pathway or whether its activation is a sign of deterioration; however, it is well established that prolonged KP metabolism and the accumulation of neurotoxic metabolites accelerate the progression of MS. More controlled studies on specific fluid samples from particular disease phases are needed to unravel the changes the KP undergoes during MS pathogenesis. Further research is necessary to evaluate the KP metabolism rate and its possible subtypes in patients with MS at all stages while also considering demographic data of patients, including age and sex. Moreover, due to changes in KP during different phases of MS and in different types of MS and the effect of MS treatment on KP, it is suggested to report data about the type and phase of MS in patients and the treatment that they have been received when measuring the KP metabolites. Moreover, given the effect of disease-modifying therapies such as IFN-β1 on KP metabolite levels and the effect of KP activation on treatment efficacy, additional research should focus on the effect of available therapies on KP metabolite concentrations and their effects on treatment efficacy.
Limitations
Our study has important limitations. First, reported details of patient characteristics were limited, consequently, findings could not be conclusively extrapolated to MS in general. Second, only a small number of selected articles met our criteria for covering all MS stages. This could be one of the reasons for the discrepancies mentioned above. Third, the studies that were investigated included samples collected from different tissues, which made it difficult to comprehensively compare the results.
Conclusion
In conclusion, this study established a potential link between altered KP metabolite levels and MS disease progression. Based on our systematic review, different KYN metabolites can be measured in MS, highlighting the potential role of KP in the pathophysiology of MS. This finding is critical for future research, which would benefit from larger scale studies comparing KP metabolites in individuals with MS. QUIN has previously been suggested to contribute to neurodegeneration. In this review we found that QUIN levels in the CSF of MS patients was higher compared to healthy controls, indicating that QUIN may play a role in MS pathogenesis. Although it was suggested that KYNA is neuroprotective and have beneficial effects in MS, the difference of KYNA levels between MS patients and controls was not significant. Also, different levels of other KP metabolites, including KYN, TRP, PIC and their ratio were also found between MS patients and controls; however, there were discrepancies between studies. Further high-quality studies on peripheral and central KP metabolite concentrations are required to better understand the dynamics of these metabolite levels in MS. Further research is also necessary to overcome our study limitations and to evaluate the rate of KP metabolism and its possible subtypes in patients with MS at all stages and ages. Moreover, given the effect of disease-modifying therapies such as IFN-β1 on KP metabolite levels and the effect of KP activation on treatment efficacy, additional research should focus on the effect of available therapies on KP metabolite concentrations and their likely effects on treatment efficacy.
Data availability statement
The original contributions presented in the study are included in the article/supplementary material. Further inquiries can be directed to the corresponding authors.
Author contributions
MF, KV, and SY contributed to the conception and design of the study. MH and FS contributed to the supervision of the manuscript. SY organized the database. AK and AMc edited the paper scientifically. All authors contributed to the article and approved the submitted version.
Acknowledgments
The authors of this study are thankful to the Clinical Research Development Center (CRDC) and Skull Base Research Center of Loghman Hakim Hospital, Shahid Beheshti University of Medical Sciences, Tehran, Iran for their support, cooperation, and assistance throughout the period of study.
Conflict of interest
The authors declare that the research was conducted in the absence of any commercial or financial relationships that could be construed as a potential conflict of interest.
Publisher’s note
All claims expressed in this article are solely those of the authors and do not necessarily represent those of their affiliated organizations, or those of the publisher, the editors and the reviewers. Any product that may be evaluated in this article, or claim that may be made by its manufacturer, is not guaranteed or endorsed by the publisher.
Abbreviations
CSF, cerebrospinal fluid; MS, multiple sclerosis; RBC, red blood cell; PBMC, peripheral blood mononuclear cell; TRP, tryptophan; KYN, kynurenine; KYNA, kynurenic acid; QUIN, quinolinic acid; PIC, picolinic acid; HIAA, hydroxyindoleacetic acid; LC, Liquid chromatography; GC, gas chromatography; IDO,Indolaeamine 2,3-Dioxygenase; HK,3-hydroxykynurenine; AA, anthranilic acid; XA, xanthurenic acid; KMO, Kynurenine 3 Monooxygenase; ROS, Reactive oxygen species; BBB, Blood brain barrier.
References
1. Walton C, King R, Rechtman L, Kaye W, Leray E, Marrie RA, et al. Rising prevalence of multiple sclerosis worldwide: Insights from the atlas of MS, third edition. Mult Scler (2020) 26(14):1816–21. doi: 10.1177/1352458520970841
2. Thompson AJ, Banwell BL, Barkhof F, Carroll WM, Coetzee T, Comi G, et al. Diagnosis of multiple sclerosis: 2017 revisions of the McDonald criteria. Lancet Neurol (2018) 17(2):162–73. doi: 10.1016/S1474-4422(17)30470-2
3. Mancuso R, Hernis A, Agostini S, Rovaris M, Caputo D, Fuchs D, et al. Indoleamine 2, 3 dioxygenase (IDO) expression and activity in relapsing-remitting multiple sclerosis. PloS One (2015) 10(6):e0130715. doi: 10.1371/journal.pone.0130715
4. Stovner LJ, Zwart JA, Hagen K, Terwindt G, Pascual J. Epidemiology of headache in Europe. Eur J Neurol (2006) 13(4):333–45. doi: 10.1111/j.1468-1331.2006.01184.x
5. Stinissen P, Hellings N. Activation of myelin reactive T cells in multiple sclerosis: A possible role for T cell degeneracy? Eur J Immunol (2008) 38(5):1190–3. doi: 10.1002/eji.200838371
6. Biernacki T, Sandi D, Bencsik K, Vécsei L. Kynurenines in the pathogenesis of multiple sclerosis: Therapeutic perspectives. Cells (2020) 9(6):1564. doi: 10.3390/cells9061564
7. Beck J, Rondot P, Catinot L, Falcoff E, Kirchner H, Wietzerbin J. Increased production of interferon gamma and tumor necrosis factor precedes clinical manifestation in multiple sclerosis: do cytokines trigger off exacerbations? Acta Neurol Scand (1988) 78(4):318–23. doi: 10.1111/j.1600-0404.1988.tb03663.x
8. Rajda C, Galla Z, Polyák H, Maróti Z, Babarczy K, Pukoli D, et al. Cerebrospinal fluid neurofilament light chain is associated with kynurenine pathway metabolite changes in multiple sclerosis. Int J Mol Sci (2020) 21(8):2665. doi: 10.3390/ijms21082665
9. Penberthy WT, Tsunoda I. The importance of NAD in multiple sclerosis. Curr Pharm design (2009) 15(1):64–99. doi: 10.2174/138161209787185751
10. Tan VX, Guillemin GJ. Kynurenine pathway metabolites as biomarkers for amyotrophic lateral sclerosis. Front Neurosci (2019) 13. doi: 10.3389/fnins.2019.01013
11. Huang Y-S, Ogbechi J, Clanchy FI, Williams RO, Stone TW. IDO and kynurenine metabolites in peripheral and CNS disorders. Front Immunol (2020) 11:388. doi: 10.3389/fimmu.2020.00388
12. Majláth Z, Annus A, Vecsei L. Kynurenine system and multiple sclerosis, pathomechanism and drug targets with an emphasis on laquinimod. Curr Drug Targets (2018) 19(7):805–14. doi: 10.2174/1389450117666161223125417
13. Masoudi S, Nemati AH, Fazli HR, Beygi S, Moradzadeh M, Pourshams A, et al. An increased level of aryl hydrocarbon receptor in patients with pancreatic cancer. Middle East J digestive Dis (2019) 11(1):38. doi: 10.15171/mejdd.2018.126
14. Nguyen NT, Nakahama T, Le DH, Van Son L, Chu HH, Kishimoto T. Aryl hydrocarbon receptor and kynurenine: recent advances in autoimmune disease research. Front Immunol (2014) 5:551. doi: 10.3389/fimmu.2014.00551
15. Joisten N, Rademacher A, Warnke C, Proschinger S, Schenk A, Walzik D, et al. Exercise diminishes plasma neurofilament light chain and reroutes the kynurenine pathway in multiple sclerosis. Neurol-Neuroimmunol Neuroinflamm (2021) 8(3):1–11. doi: 10.1212/NXI.0000000000000982
16. Aeinehband S, Brenner P, Ståhl S, Bhat M, Fidock MD, Khademi M, et al. Cerebrospinal fluid kynurenines in multiple sclerosis; relation to disease course and neurocognitive symptoms. Brain beh Immun (2016) 51:47–55. doi: 10.1016/j.bbi.2015.07.016
17. Rejdak K, Bartosik-Psujek H, Dobosz B, Kocki T, Grieb P, Giovannoni G, et al. Decreased level of kynurenic acid in cerebrospinal fluid of relapsing-onset multiple sclerosis patients. Neurosci Lett (2002) 331(1):63–5. doi: 10.1016/S0304-3940(02)00710-3
18. Rejdak K, Petzold A, Kocki T, Kurzepa J, Grieb P, Turski W, et al. Astrocytic activation in relation to inflammatory markers during clinical exacerbation of relapsing-remitting multiple sclerosis. J Neural Transm (2007) 114(8):1011. doi: 10.1007/s00702-007-0667-y
19. Sundaram G, Brew BJ, Jones SP, Adams S, Lim CK, Guillemin GJ. Quinolinic acid toxicity on oligodendroglial cells: relevance for multiple sclerosis and therapeutic strategies. J Neuroinflamm (2014) 11(1):1–11. doi: 10.1186/s12974-014-0204-5
20. Addae JI, Stone TW. Quinolinic acid and related excitotoxins: Mechanisms of neurotoxicity and disease relevance. In: Handbook of neurotoxicity. Springer Nature (2021) 1–22.
21. Sakurai K, Zou J-P, Tschetter JR, Ward JM, Shearer GM. Effect of indoleamine 2, 3-dioxygenase on induction of experimental autoimmune encephalomyelitis. J neuroimmunol (2002) 129(1-2):186–96. doi: 10.1016/S0165-5728(02)00176-5
22. Maddison DC, Giorgini F. The kynurenine pathway and neurodegenerative disease. Semin Cell Dev Biol (2015) 40:134–41. doi: 10.1016/j.semcdb.2015.03.002
23. Vécsei L, Szalárdy L, Fülöp F, Toldi J. Kynurenines in the CNS: recent advances and new questions. Nat Rev Drug Discovery (2013) 12(1):64–82. doi: 10.1038/nrd3793
24. Monaco F, Fumero S, Mondino A, Mutani R. Plasma and cerebrospinal fluid tryptophan in multiple sclerosis and degenerative diseases. J Neurol Neurosurg Psychiatry (1979) 42(7):640–1. doi: 10.1136/jnnp.42.7.640
25. Rudzite V, Berzinsh J, Grivane I, Fuchs D, Baier-Bitterlich G, Wachter H. Serum tryptophan, kynurenine, and neopterin in patients with Guillain-barre-syndrome (GBS) and multiple sclerosis (MS). In: Recent advances in tryptophan research. Springer, Boston, MA: Springer (1996). p. 183–7.
26. Sandyk R. Tryptophan availability and the susceptibility to stress in multiple sclerosis: A hypothesis. Int J Neurosci (1996) 86(1-2):47–53. doi: 10.3109/00207459608986697
27. Füvesi J, Rajda C, Bencsik K, Toldi J, Vécsei L. The role of kynurenines in the pathomechanism of amyotrophic lateral sclerosis and multiple sclerosis: therapeutic implications. J Neural Transm (2012) 119(2):225–34. doi: 10.1007/s00702-012-0765-3
28. Dorszewska J, Florczak-Wyspianska J, Kowalska M, Stanski M, Kowalewska A, Kozubski W. Serotonin in neurological diseases. In: Serotonin: A chemical messenger between all types of living cells. InTech (2017) 219.
29. Adamczyk-Sowa M, Galiniak S, Żyracka E, Grzesik M, Naparło K, Sowa P, et al. Oxidative modification of blood serum proteins in multiple sclerosis after interferon beta and melatonin treatment. Oxid Med Cell Longevity (2017) 2017:7905148. doi: 10.1155/2017/7905148
30. Agliardi C, Guerini FR, Zanzottera M, Rovaris M, Caputo D, Clerici M. Indoleamine-2, 3-dioxygenase (IDO) 2 polymorphisms are not associated with multiple sclerosis in italians. J Neurol Sci (2017) 377:31–4. doi: 10.1016/j.jns.2017.03.048
31. Gaetani L, Boscaro F, Pieraccini G, Calabresi P, Romani L, Di Filippo M, et al. Host and microbial tryptophan metabolic profiling in multiple sclerosis. Front Immunol (2020) 11:157. doi: 10.3389/fimmu.2020.00157
32. Hartai Z, Klivenyi P, Janaky T, Penke B, Dux L, Vecsei L. Kynurenine metabolism in multiple sclerosis. Acta Neurol Scand (2005) 112(2):93–6. doi: 10.1111/j.1600-0404.2005.00442.x
33. Herman S, Åkerfeldt T, Spjuth O, Burman J, Kultima K. Biochemical differences in cerebrospinal fluid between secondary progressive and relapsing–remitting multiple sclerosis. Cells (2019) 8(2):84. doi: 10.3390/cells8020084
34. Huang Y-M, Liu X, Steffensen K, Sanna A, Arru G, Fois ML, et al. Immunological heterogeneity of multiple sclerosis in Sardinia and Sweden. Multiple Sclerosis J (2005) 11(1):16–23. doi: 10.1191/1352458505ms1127oa
35. Negrotto L, Correale J. Amino acid catabolism in multiple sclerosis affects immune homeostasis. J Immunol (Baltimore Md 1950) (2017) 198(5):1900–9. doi: 10.4049/jimmunol.1601139
36. Nejati A, Shoja Z, Shahmahmoodi S, Tafakhori A, Mollaei-Kandelous Y, Rezaei F, et al. EBV and vitamin d status in relapsing-remitting multiple sclerosis patients with a unique cytokine signature. Med Microbiol Immunol (2016) 205(2):143–54. doi: 10.1007/s00430-015-0437-7
37. Olsson A, Gustavsen S, Nguyen TD, Nyman M, Langkilde AR, Hansen TH, et al. Serum short-chain fatty acids and associations with inflammation in newly diagnosed patients with multiple sclerosis and healthy controls. Front Immunol (2021) 12:661493. doi: 10.3389/fimmu.2021.661493
38. Sadowska-Bartosz I, Adamczyk-Sowa M, Galiniak S, Mucha S, Pierzchala K, Bartosz G. Oxidative modification of serum proteins in multiple sclerosis. Neurochem Int (2013) 63(5):507–16. doi: 10.1016/j.neuint.2013.08.009
39. Tömösi F, Kecskeméti G, Cseh EK, Szabó E, Rajda C, Kormány R, et al. A validated UHPLC-MS method for tryptophan metabolites: Application in the diagnosis of multiple sclerosis. J Pharm Biomed Anal (2020) 185:113246. doi: 10.1016/j.jpba.2020.113246
40. Kayaoğlu MY, Girgin G, Solmaz I, Baydar T, Anlar B. Urine neopterin in childhood acute demyelinating diseases: Potential for differential diagnosis. Multiple sclerosis related Disord (2022) 59:103662. doi: 10.1016/j.msard.2022.103662
41. Sundaram G, Lim CK, Brew BJ, Guillemin GJ. Kynurenine pathway modulation reverses the experimental autoimmune encephalomyelitis mouse disease progression. J Neuroinflamm (2020) 17(1):1–14. doi: 10.1186/s12974-020-01844-y
42. Lim CK, Bilgin A, Lovejoy DB, Tan V, Bustamante S, Taylor BV, et al. Kynurenine pathway metabolomics predicts and provides mechanistic insight into multiple sclerosis progression. Sci Rep (2017) 7:41473. doi: 10.1038/srep41473
43. Lovelace MD, Varney B, Sundaram G, Franco NF, Ng ML, Pai S, et al. Current evidence for a role of the kynurenine pathway of tryptophan metabolism in multiple sclerosis. Front Immunol (2016) 7(246). doi: 10.3389/fimmu.2016.00246
44. Rajda C, Majláth Z, Pukoli D, Vécsei L. Kynurenines and multiple sclerosis: The dialogue between the immune system and the central nervous system. Int J Mol Sci (2015) 16(8):18270–82. doi: 10.3390/ijms160818270
45. Lovelace MD, Varney B, Sundaram G, Lennon MJ, Lim CK, Jacobs K, et al. Recent evidence for an expanded role of the kynurenine pathway of tryptophan metabolism in neurological diseases. Neuropharmacology (2017) 112(Pt B):373–88. doi: 10.1016/j.neuropharm.2016.03.024
46. Raine CS, Scheinberg LC. On the immunopathology of plaque development and repair in multiple sclerosis. J neuroimmunol (1988) 20(2-3):189–201. doi: 10.1016/0165-5728(88)90160-9
47. O'Connor RA, Prendergast CT, Sabatos CA, Lau CW, Leech MD, Wraith DC, et al. Cutting edge: Th1 cells facilitate the entry of Th17 cells to the central nervous system during experimental autoimmune encephalomyelitis. J Immunol (2008) 181(6):3750–4. doi: 10.4049/jimmunol.181.6.3750
48. Guillemin GJ, Smith DG, Kerr SJ, Smythe GA, Kapoor V, Armati PJ, et al. Characterisation of kynurenine pathway metabolism in human astrocytes and implications in neuropathogenesis. Redox Rep (2000) 5(2-3):108–11. doi: 10.1179/135100000101535375
49. Sforzini L, Nettis MA, Mondelli V, Pariante CM. Inflammation in cancer and depression: A starring role for the kynurenine pathway. Psychopharmacology (2019) 236(10):2997–3011. doi: 10.1007/s00213-019-05200-8
50. Frumento G, Rotondo R, Tonetti M, Damonte G, Benatti U, Ferrara GB. Tryptophan-derived catabolites are responsible for inhibition of T and natural killer cell proliferation induced by indoleamine 2, 3-dioxygenase. J Exp Med (2002) 196(4):459–68. doi: 10.1084/jem.20020121
51. Grohmann U, Bronte V. Control of immune response by amino acid metabolism. Immunol Rev (2010) 236(1):243–64. doi: 10.1111/j.1600-065X.2010.00915.x
52. Fallarino F, Grohmann U, Vacca C, Bianchi R, Orabona C, Spreca A, et al. T Cell apoptosis by tryptophan catabolism. Cell Death Differentiation (2002) 9(10):1069–77. doi: 10.1038/sj.cdd.4401073
53. Chiesa MD, Carlomagno S, Frumento G, Balsamo M, Cantoni C, Conte R, et al. The tryptophan catabolite l-kynurenine inhibits the surface expression of NKp46-and NKG2D-activating receptors and regulates NK-cell function. Blood (2006) 108(13):4118–25. doi: 10.1182/blood-2006-03-006700
54. Terness P, Bauer TM, Roüse L, Dufter C, Watzlik A, Simon H, et al. Inhibition of allogeneic T cell proliferation by indoleamine 2, 3-dioxygenase–expressing dendritic cells: mediation of suppression by tryptophan metabolites. J Exp Med (2002) 196(4):447–57. doi: 10.1084/jem.20020052
55. Guillemin GJ, Kerr SJ, Pemberton LA, Smith DG, Smythe GA, Armati PJ, et al. IFN-β 1b induces kynurenine pathway metabolism in human macrophages: potential implications for multiple sclerosis treatment. J Interferon Cytokine Res (2001) 21(12):1097–101. doi: 10.1089/107999001317205231
56. Amirkhani A, Rajda C, Arvidsson B, Bencsik K, Boda K, Seres E, et al. Interferon-β affects the tryptophan metabolism in multiple sclerosis patients. Eur J Neurol (2005) 12(8):625–31. doi: 10.1111/j.1468-1331.2005.01041.x
57. Guillemin GJ, Smythe GA, Veas LA, Takikawa O, Brew BJ. Aβ1-42 induces production of quinolinic acid by human macrophages and microglia. Neuroreport (2003) 14(18):2311–5. doi: 10.1097/00001756-200312190-00005
58. O'Connor JC, André C, Wang Y, Lawson MA, Szegedi SS, Lestage J, et al. Interferon-γ and tumor necrosis factor-α mediate the upregulation of indoleamine 2, 3-dioxygenase and the induction of depressive-like behavior in mice in response to bacillus calmette-guerin. J Neurosci (2009) 29(13):4200–9. doi: 10.1523/JNEUROSCI.5032-08.2009
59. Smith DG, Guillemin GJ, Pemberton L, Kerr S, Nath A, Smythe GA, et al. Quinolinic acid is produced by macrophages stimulated by platelet activating factor, nef and tat. J neurovirol (2001) 7(1):56–60. doi: 10.1080/135502801300069692
60. Mondanelli G, Ugel S, Grohmann U, Bronte V. The immune regulation in cancer by the amino acid metabolizing enzymes ARG and IDO. Curr Opin Pharmacol (2017) 35:30–9. doi: 10.1016/j.coph.2017.05.002
61. Mondanelli G, Iacono A, Allegrucci M, Puccetti P, Grohmann U. Immunoregulatory interplay between arginine and tryptophan metabolism in health and disease. Front Immunol (2019) 10:1565. doi: 10.3389/fimmu.2019.01565
62. Puccetti P, Grohmann U. IDO and regulatory T cells: A role for reverse signalling and non-canonical NF-κB activation. Nat Rev Immunol (2007) 7(10):817–23. doi: 10.1038/nri2163
63. Han Q, Li J, Li J. pH dependence, substrate specificity and inhibition of human kynurenine aminotransferase I. Eur J Biochem (2004) 271(23-24):4804–14. doi: 10.1111/j.1432-1033.2004.04446.x
64. Lim CK, Brew BJ, Sundaram G, Guillemin GJ. Understanding the roles of the kynurenine pathway in multiple sclerosis progression. Int J Tryptophan Res (2010) 3:S4294. doi: 10.4137/IJTR.S4294
65. Kepplinger B, Baran H, Kainz A, Ferraz-Leite H, Newcombe J, Kalina P. Age-related increase of kynurenic acid in human cerebrospinal fluid–IgG and β2-microglobulin changes. Neurosignals (2005) 14(3):126–35. doi: 10.1159/000086295
66. Andiné P, Lehmann A, Ellrén K, Wennberg E, Kjellmer I, Nielsen T, et al. The excitatory amino acid antagonist kynurenic acid administered after hypoxic-ischemia in neonatal rats offers neuroprotection. Neurosci Lett (1988) 90(1-2):208–12. doi: 10.1016/0304-3940(88)90813-0
67. Atlas A, Franzen-Röhl E, Söderlund J, Jönsson EG, Samuelsson M, Schwieler L, et al. Sustained elevation of kynurenic acid in the cerebrospinal fluid of patients with herpes simplex virus type 1 encephalitis. Int J Tryptophan Res (2013) 6:S13256. doi: 10.4137/IJTR.S13256
68. Myint A-M, Kim YK, Verkerk R, Scharpé S, Steinbusch H, Leonard B. Kynurenine pathway in major depression: evidence of impaired neuroprotection. J Affect Disord (2007) 98(1-2):143–51. doi: 10.1016/j.jad.2006.07.013
69. Sas K, Robotka H, Toldi J, Vécsei L. Mitochondria, metabolic disturbances, oxidative stress and the kynurenine system, with focus on neurodegenerative disorders. J neurol Sci (2007) 257(1-2):221–39. doi: 10.1016/j.jns.2007.01.033
70. Guillemin GJ, Cullen KM, Lim CK, Smythe GA, Garner B, Kapoor V, et al. Characterization of the kynurenine pathway in human neurons. J Neurosci (2007) 27(47):12884–92. doi: 10.1523/JNEUROSCI.4101-07.2007
71. Lim CK, Smythe GA, Stocker R, Brew BJ, Guillemin GJ. Characterization of the kynurenine pathway in human oligodendrocytes. In: International congress series. Netherlands: Elsevier (2007).
72. Cammer W. Oligodendrocyte killing by quinolinic acid in vitro. Brain Res (2001) 896(1-2):157–60. doi: 10.1016/S0006-8993(01)02017-0
73. Anderson J, Hampton D, Patani R, Pryce G, Crowther R, Reynolds R, et al. Abnormally phosphorylated tau is associated with neuronal and axonal loss in experimental autoimmune encephalomyelitis and multiple sclerosis. Brain (2008) 131(7):1736–48. doi: 10.1093/brain/awn119
74. Bosco MC, Rapisarda A, Massazza S, Melillo G, Young H, Varesio L. The tryptophan catabolite picolinic acid selectively induces the chemokines macrophage inflammatory protein-1α and-1β in macrophages. J Immunol (2000) 164(6):3283–91. doi: 10.4049/jimmunol.164.6.3283
75. Melillo G, Cox GW, Radzioch D, Varesio L. Picolinic acid, a catabolite of l-tryptophan, is a costimulus for the induction of reactive nitrogen intermediate production in murine macrophages. J Immunol (1993) 150(9):4031–40.
76. Melillo G, Cox GW, Biragyn A, Sheffler LA, Varesio L. Regulation of nitric-oxide synthase mRNA expression by interferon-gamma and picolinic acid. J Biol Chem (1994) 269(11):8128–33. doi: 10.1016/S0021-9258(17)37169-7
77. Guillemin GJ, Kerr SJ, Smythe GA, Smith DG, Kapoor V, Armati PJ, et al. Kynurenine pathway metabolism in human astrocytes: A paradox for neuronal protection. J neurochem (2001) 78(4):842–53. doi: 10.1046/j.1471-4159.2001.00498.x
Keywords: multiple sclerosis, kynurenine pathway, kynurenine, tryptophan, picolinic acid, hydroxyindoleacetic acid, kynurenic acid, quinolinic acid
Citation: Fathi M, Vakili K, Yaghoobpoor S, Tavasol A, Jazi K, Mohamadkhani A, Klegeris A, McElhinney A, Mafi Z, Hajiesmaeili M and Sayehmiri F (2022) Dynamic changes in kynurenine pathway metabolites in multiple sclerosis: A systematic review. Front. Immunol. 13:1013784. doi: 10.3389/fimmu.2022.1013784
Received: 07 August 2022; Accepted: 04 October 2022;
Published: 02 November 2022.
Edited by:
Robert Weissert, University of Regensburg, GermanyReviewed by:
Bruce James Brew, St. Vincent’s Hospital Sydney, AustraliaChai K. Lim, Macquarie University, Australia
Trevor William Stone, University of Oxford, United Kingdom
Copyright © 2022 Fathi, Vakili, Yaghoobpoor, Tavasol, Jazi, Mohamadkhani, Klegeris, McElhinney, Mafi, Hajiesmaeili and Sayehmiri. This is an open-access article distributed under the terms of the Creative Commons Attribution License (CC BY). The use, distribution or reproduction in other forums is permitted, provided the original author(s) and the copyright owner(s) are credited and that the original publication in this journal is cited, in accordance with accepted academic practice. No use, distribution or reproduction is permitted which does not comply with these terms.
*Correspondence: Mohammadreza Hajiesmaeili, mrhajiesmaeili@sbmu.ac.ir; Fatemeh Sayehmiri, Fsayehmiri@yahoo.com
†These authors have contributed equally to this work and share first authorship