- 1Department of Medical Biochemistry, Faculty of Medicine, University of Sarajevo, Sarajevo, Bosnia and Herzegovina
- 2Department of Laboratory Medicine, Medical University of Vienna, Vienna, Austria
Cellular death, aging, and tissue damage trigger inflammation that leads to enzymatic and non-enzymatic lipid peroxidation of polyunsaturated fatty acids present on cellular membranes and lipoproteins. This results in the generation of highly reactive degradation products, such as malondialdehyde (MDA) and 4-hydroxynonenal (4-HNE), that covalently modify free amino groups of proteins and lipids in their vicinity. These newly generated neoepitopes represent a unique set of damage-associated molecular patterns (DAMPs) associated with oxidative stress termed oxidation-specific epitopes (OSEs). OSEs are enriched on oxidized lipoproteins, microvesicles, and dying cells, and can trigger sterile inflammation. Therefore, prompt recognition and removal of OSEs is required to maintain the homeostatic balance. This is partially achieved by various humoral components of the innate immune system, such as natural IgM antibodies, pentraxins and complement components that not only bind OSEs but in some cases modulate their pro-inflammatory potential. Natural IgM antibodies are potent complement activators, and 30% of them recognize OSEs such as oxidized phosphocholine (OxPC-), 4-HNE-, and MDA-epitopes. Furthermore, OxPC-epitopes can bind the complement-activating pentraxin C-reactive protein, while MDA-epitopes are bound by C1q, C3a, complement factor H (CFH), and complement factor H-related proteins 1, 3, 5 (FHR-1, FHR-3, FHR-5). In addition, CFH and FHR-3 are recruited to 2-(ω-carboxyethyl)pyrrole (CEP), and full-length CFH also possesses the ability to attenuate 4-HNE-induced oxidative stress. Consequently, alterations in the innate humoral defense against OSEs predispose to the development of diseases associated with oxidative stress, as shown for the prototypical OSE, MDA-epitopes. In this mini-review, we focus on the mechanisms of the accumulation of OSEs, the pathophysiological consequences, and the interactions between different OSEs and complement components. Additionally, we will discuss the clinical potential of genetic variants in OSE-recognizing complement proteins – the OSE complotype - in the risk estimation of diseases associated with oxidative stress.
1 Introduction
1.1 Generation of OSE
Increased oxidative stress, characterized by exalted levels of reactive oxygen species, leads to lipid peroxidation of polyunsaturated fatty acids localized in cellular membranes. Lipid peroxidation can be initiated by enzymatic or non-enzymatic mechanisms, resulting in the generation of reactive lipid mediators. Adduction of reactive lipid degradation products to free amino-groups on macromolecules generates novel neo-epitopes, termed oxidation-specific epitopes (OSEs) (1). Once adducted, OSEs have the capacity to alter the function of the affected biomolecule and tag their carrier as “altered-self”. Although there is a large amount of different lipid degradation byproducts that can create OSEs, the best studied examples are malondialdehyde and the more advanced malondialdehydeacetaldehyde, which we are collectively calling MDA (as umbrella term for different MDA-epitopes), 4-hydroxynonenal (4-HNE), 2-(ω-carboxyethyl)-pyrrole (CEP), oxidized cardiolipin (OxCL) and the phosphocholine head group-containing oxidized phospholipids (OxPC).
Within tissues or in the circulation, OSE-decorated structures are considered to be markers of oxidative stress and are found in many pathological conditions such as chronic inflammation, autoimmunity, infections, cancer, and neurological disorders (1–7).
1.2 Biological carriers and function of OSEs
The ubiquitous presence of lipids in living cells enables OSEs formation on versatile biological carriers. Major carriers are free biomolecules, oxidized lipoproteins, microvesicles, and apoptotic cells (1).
1.2.1 Free biomolecules
1.2.1.1 Proteins
On proteins, OSEs are attached to the amino groups of lysine and arginine side chains, but modifications of other amino acids have been shown (8–12). This irreversible adduction can alter protein carrier function, lead to aggregation, and increase its immunogenicity (13, 14). The ultimate fate of OSE-modified proteins in homeostasis is proteolytic degradation or clearance by immune responses.
1.2.1.2 Nucleic acids
Nuclear and mitochondrial DNA modifications by MDA and 4-HNE are mutagenic and cancerogenic by causing nucleotide exchange or forming cross-links (11, 15).
1.2.1.3 Phospholipids
Amino group-containing phospholipids, such as phosphatidylethanolamine (PE) and phosphatidylserine (PS), can be modified by MDA, 4-HNE, and CEP (16–21). OSEs on phospholipids alter their recognition by pattern recognition receptors (PRR) and their ability to serve as phospholipase substrates (22–24).
1.2.2 Lipoproteins
1.2.2.1 Low-density lipoprotein
Oxidized LDL has been discovered as the initial carrier of OSEs, where they are considered to be major drivers of atherosclerosis development (25, 26). Following the retention of plasma LDL in the intima of the arterial wall, both the lipid and protein components of LDL can become oxidized (OxLDL). Newly oxidized LDL is pro-inflammatory, chemotactic, and pro-coagulatory (2, 27). Upon OxLDL clearance, engulfing macrophages are converted into foam cells - hallmark cells of atherosclerotic plaques (28–30). Additionally, MDA-, 4-HNE-, OxPC-, and OxCL-epitopes have been documented in atherosclerotic plaques of mice and humans (25, 31–36).
1.2.2.2 High-density lipoprotein
Once modified by OSEs, HDL loses its function to remove cholesterol from cells via the ATP-binding cassette transporter A1 (ABCA1) pathway and, via CD36, promotes platelet aggregation (37–41).
1.2.3 Microvesicles
Microvesicles are extracellular vesicles (0,1 - 1 µm) with a phospholipid bilayer enriched in phosphatidylserine. They are generated by cellular activation or apoptosis and are pro-coagulatory and pro-inflammatory (2, 42, 43). As carriers of parental cells’ biological cargo, they play a role in inter-organ communication, and alterations in their numbers and content are associated with many pathologies (43, 44). The presence of OSEs, namely MDA- and OxPC-epitopes, has been demonstrated on a subset of circulating MVs and MVs from in vitro stimulated cells (45–47).
1.2.4 Dying cells
The presence of OSEs on dying cells and apoptotic blebs seems to be independent of the mechanism of apoptosis induction and the cell types undergoing apoptosis (33, 34, 48–52). Early experiments already proposed that the presence of OSEs on cells undergoing programmed cell death (apoptosis and necrosis) plays a role in their clearance by enhancing their ability to be recognized by phagocytic cells (48, 53).
1.3 The function of OSEs as danger-associated molecular pattern molecules
Among well-established DAMPs, like histones, cholesterol crystals, DNA, and others, OSEs represent a distinct group (1, 54). Exposure of OSEs mediates the recognition by innate immunity sensors and can trigger sterile inflammation (51, 52, 55–60). The main cellular innate sensors of OSEs are scavenger receptors and - typically in cooperation - toll-like receptors (TLRs), which are responsible not only for recognition but also for the initiation of downstream signaling events (1).
In vitro, treatment with MDA-modified proteins and MDA+ MVs induces cytokine secretion (e.g., interleukin-8 (IL-8), or its murine functional homologues chemokine ligand 1(CXCL1) and CXCL2) in various human or murine cell types and cell lines (47, 51, 52, 61). Moreover, in vivo, intravitreal injection of MDA-modified bovine serum albumin (BSA) led to an increase in CXCL1 expression in retinal pigment epithelial (RPE) cells (52). Similarly, in a mouse peritonitis model, injection of MDA-BSA resulted in secretion of CXCL1 and CXCL2 and recruitment of neutrophils and monocytes. Moreover, treatment with the anti-MDA IgM antibody (LR04) attenuated hepatic pro-inflammatory cytokine secretion and leukocyte infiltration induced by the western diet (51). Scavenger receptor A1 (SRA1), CD36, lectin-like OxLDL receptor (LOX1), and CD16 on monocytes and macrophages have been shown to act as sensors for MDA (51, 62–64).
4-HNE increased cytokine secretion, e.g., IL-8, recruitment of neutrophils and macrophages via TLR4/NFκB in animal models of atherosclerosis and chronic obstructive pulmonary disease (59, 60). Also, it stimulated the release of pro-coagulatory tissue factor-positive MVs from perivascular cells (65). LOX1 has been identified as the scavenger receptor for 4-HNE (66).
OxPC or OxPC-rich MVs have been reported to induce endothelial cell activation, monocyte recruitment, cytokine secretion (e.g., IL-6) by macrophages, and apoptosis in smooth muscle cells (3, 45, 49, 67). Furthermore, OxPC drives hypercholesterolemia-induced inflammation and atherogenesis and restrains bone formation in vivo (4, 35). SRB1, TLR2, and CD36-TLR4-TLR6 heterotrimeric signaling complex are required to recognize Ox-PC (68–70).
CEP has been shown to activate the NLRP3 inflammasome and stimulate the production of IL-1β (71). Moreover, injection of CEP into the mouse eye increased Th1 response and enhanced angiogenesis (72–74). Additionally, in a peritonitis model, CEP generated by neutrophils promotes infiltration of monocytes and macrophages by binding to β2 integrins on their surfaces (75). For CEP binding and clearance, the coordinated action of CD36 and TLR2 is required (76).
2 Recognition of OSEs by innate humoral immunity
Both innate and adaptive immune responses against OSEs have been demonstrated, and their functional implications are being elucidated. Thus, in this mini-review we will focus solely on representatives of soluble innate immune responses to OSEs: natural IgM antibodies, pentraxins, and several components of the complement cascade because their levels and genetic variants have been implicated in the development of diseases associated with increased oxidative stress (Table 1).
2.1 Natural antibodies
Natural IgM antibodies are pre-existing antibodies that typically contain unmutated variable regions encoded by germline gene sequences. In mice, natural antibodies are secreted by B1 cells, but marginal zone B cells may also contribute to their production. Natural antibodies arise in newborns without infections or exposure to exogenous antigens and thus can be found in gnotobiotic mice. Thirty percent of all natural IgM antibodies have specificity for OSEs, such as MDA, OxPC, and 4-HNE, among which MDA is the predominant antigen (50). A series of OSE-specific natural IgM antibodies have been cloned, of which the best characterized are LR04, NA17, and E014 (recognizing MDA), T15/E06 (recognizing OxPC), and LR01 (recognizing OxCL) (25, 33, 34, 48, 50, 114). They bind to microbial antigens and altered-self structures, which allows them to mediate important functions in host defense, but also makes them essential in homeostasis maintenance, respectively (115). Natural IgM antibodies neutralize the pro-inflammatory effects of oxidized lipids and MVs, mediate apoptotic cell clearance, and are anti-atherogenic by blocking OxLDL uptake and foam cell formation (45, 47, 77–79). Additionally, we have shown that anti-MDA IgM hinders the binding of coagulation factors X/Xa on MVs, attenuating the propagation of coagulation and protecting from pulmonary thrombosis in mice (42). Moreover, MDA-targeted passive and active immunization strategies that increase the levels of MDA-specific IgM protect from atherosclerosis and hepatic inflammation (80–82). Furthermore, mice unable to secrete natural IgM antibodies display impaired clearance of apoptotic cells and develop arthritis and lupus-like disease, which can be in part explained by the lack of OSE-specific IgMs (83–85). Finally, studies in various human cohorts demonstrated that low levels of IgMs against OSEs are associated with an elevated risk of developing cardiovascular diseases (CVDs), confirming the beneficial role of anti-OSE IgMs (86–92).
2.2 Pentraxins
Pentraxins are acute-phase proteins and represent soluble innate pattern recognition proteins. As such, they facilitate the removal of invading microorganisms and damaged host cells. There are two types of pentraxins, short (C-reactive protein (CRP) and serum amyloid P (SAP)) and long ones (pentraxin 3 (PTX3)) (116). CRP has been shown to bind to OxPC on OxLDL, and they colocalize on the surface of apoptotic cells and in human atherosclerotic lesions (49, 93). When CRP is complexed with OxPC-epitopes, it recruits C1q and activates the C1 complex of the classical complement cascade (CCC) (94). In contrast to CRP, SAP and PTX3 do not bind to OSEs, although SAP competes out the binding of plasma IgM and CRP on late apoptotic cells (93). Interestingly, OxLDL enhanced the expression of PTX3, which promoted OxLDL uptake by macrophages and blocked cholesterol efflux (95, 96). Furthermore, PTX3 protects against 4-HNE-induced complement activation by recruiting CFH to the basal RPE and inner Bruch’s membrane in AMD (117).
2.3 Complement components
The complement cascade protects and orchestrates the removal of invading pathogens and altered self- or foreign-structures by employing three pathways. Its activity is steered by complement activators and regulators that prevent collateral damage to host tissues.
2.3.1 C1q
C1q is a multimeric protein, a part of the initiator complex of the CCC. With its globular head, it can recognize pathogen-associated molecular patterns (PAMPs), DAMPs, and immune complexes, and once bound, it activates the CCC with the collagen-like domain. It binds to OxLDL, which leads to the complement activation and deposition of C3b, facilitating OxLDL uptake by monocytes and macrophages (97, 98). Additionally, the engulfment of OxLDL with C1q suppresses macrophage NFκB and NLRP3 activation, resulting in an enhancement of IL-10 and a reduction in IL-1β secretion (99). The initial notion that the binding of C1q to oxidized lipoproteins is mediated through OSEs came from the finding that MDA-LDL binds C1q, resulting in the deposition of C4b and activation of the CCC, which can be inhibited by ApoE (100). Furthermore, C1q is found both on circulating MVs, and apoptotic cells; however, if this binding is (in part) OSE-dependent has not been investigated so far (118–120). Due to the impairment of apoptotic cell clearance, C1q deficiency in mice and men predisposes to the development of systemic lupus erythematosus (SLE) (118, 121).
2.3.2 C3a
Complement anaphylatoxin C3a is a small degradation product of C3 generated by C3 convertase. It is a chemotactic molecule of the immune system, and although classically considered a pro-inflammatory molecule, C3a has been shown to have some anti-inflammatory functions (122, 123). MDA-epitopes are ligands for C3a on OxLDL and apoptotic cells. Furthermore, this OxLDL-C3a interaction results in increased internalization of C3a by macrophages, thus making OxLDL a platform enhancing uptake of C3a (101). Myeloperoxidase-rich MVs can also be the carriers of C3a; however, if recruitment of C3a to MVs is mediated by OSEs has to be elucidated (124).
2.3.3 Complement factor H
Complement factor H is the regulator of the alternative complement pathway. It comprises 20 short consensus repeat (SCR) domains and acts as a sensor of PAMPs and DAMPs. Additionally, a splice variant of CFH exists – factor H-like protein 1 (FHL-1). Impairment of CFH functions contributes to the development of many diseases, with AMD and atypical hemolytic uremic syndrome as the most prominent examples (125, 126). Weismann et al. demonstrated that CFH and FHL-1 recognize MDA-epitopes and colocalize within the retina and atherosclerotic lesions. MDA recognition is achieved by SCR7 and SCR19-20, where SCR7 is the most critical, but SCR19-20 also matter (103–106). CFH protects from MDA-induced IL-8 secretion and inactivates C3b into iC3b on MDA-carrying surfaces (52). Furthermore, the CFH variant Tyr402His (rs1061170) within SCR7 that predisposes to AMD results in decreased binding to MDA-epitopes in healthy individuals and AMD patients (52, 105). Similarly, transgenic mice with human SCR6-8 402His inserted into a mouse CFH display an AMD-like phenotype (103). In addition, CFH binds CEP-decorated surfaces and this binding is attenuated in the presence of complement factor H-related protein 3 (FHR-3) (112). The observation that CFH binds to OxPC-epitopes requires further validation (52, 107, 108). Although CFH does not directly interact with 4-HNE-epitopes, it protects ARPE-19 cells from 4-HNE-induced cell death by attenuating apoptotic and necroptotic cell death pathways (52, 109). Next to OSEs, CFH binds many other DAMPs on the surface of dying cells, apoptotic blebs, and MVs (52, 110, 127, 128). There, CFH compensates for the loss of membrane-bound complement inhibitors by protecting cells from excessive complement activation and limiting inflammatory potential (127, 128).
2.3.4 Complement factor H-related proteins
FHRs are five plasma proteins that share high structural and functional similarities with CFH, among other recognition of OSEs by FHR-1, 3-, and -5. Interestingly, FHRs do not have potent complement regulatory activity like CFH. Their competition with CFH in recruitment to various ligands labels them as “deregulators of CFH activity” (129, 130).
Using a genome-wide association study, we identified FHR-1 as the main competitor to CFH for binding MDA-epitopes in a cohort of healthy individuals. Once bound to MDA-epitopes, FHR-1 blocks CFH-mediated C3b inactivation, allowing C3b and Bb deposition and propagation of the alternative complement pathway. MDA-epitopes on necrotic cells are recognized by FHR-1 via SCR1-2 (105, 110, 111). This activates monocytes in the vicinity via EMR2 receptors and NLRP3 pathway (111). Consequently, in necrotic cores of atherosclerotic lesions, FHR-1 colocalizes with macrophages and stimulates IL-1β and IL-8 secretion (110). Considering its property to bind MDA-epitopes, deletion of the gene encoding for FHR-1 (CFHR1) is assumed to be beneficial in chronic inflammation. Indeed, carriers of this deletion have a reduced risk of atherosclerotic CVDs and anti-neutrophil cytoplasmic antibody-associated vasculitis and display lower levels of inflammatory markers (110, 111). Therefore, on MDA-carrying host surfaces, FHR-1 is pro-inflammatory. Of note, FHR-1 does not bind any other OSEs (105).
The deletion of CFHR3&CFHR1 genes was shown to enhance CFH binding to MDA-epitopes because, as FHR-1, FHR-3 competes for them. Compared to FHR-1 and CFH, it displays the lowest affinity towards MDA-epitopes. Once bound to MDA-epitopes, FHR-3 does not cause deregulation of CFH function (105). Moreover, FHR-3 has been shown to also bind to CEP-epitopes but does not interact with OxPC- and 4-HNE-modifications (105, 112). Interestingly, when attached to the surface of polarized senescent ARPE-19 cells, FHR-3 is internalized. Engulfed FHR-3 drives pro-inflammatory responses of RPE cells by upregulating C3 and factor B expression and translocating newly generated C3a from the cytoplasm to the membrane (112). Although the relation between OSE-binding and FHR-3 has not been investigated in diseases associated with a high level of oxidative stress, in many of them, e.g., rheumatoid arthritis and SLE, FHR-3 serum levels are increased, and the lack of CFHR3 gene is protective (131).
Complement factor H-related protein 5, one of the largest members of the FHR family, attaches itself to apoptotic and necrotic cells through SCR5-7 domains. MDA-epitopes were shown to be the predominant ligands responsible for this recruitment, as demonstrated by the fact that the density of these epitopes determines the amount of FHR-5 bound. Like FHR-1 and FHR-3, when attached to MDA-epitopes, FHR-5 reduces CFH cofactor activity and enhances C3 deposition. Additionally, a hybrid protein FHR-21-2-FHR-5 obtained from the serum of a patient with C3 glomerulopathy binds to MDA-epitopes (113). When adhered to apoptotic and necrotic cells’ surfaces, FHR-5 and FHR-1 recruit CRP and PTX3 and activate classical and alternative complement pathways, facilitating opsonization (132).
2.4 The connection between natural IgM antibodies, pentraxins, and complement proteins
Although OSE-recognizing IgM antibodies and pentraxins have been shown to instruct phagocytic cells for clearance of damaged structures individually, they can also employ the complement cascade to ensure even more efficient and potent removal machinery (1, 49, 114, 133).
Natural IgM antibodies, CRP, and SAP have been shown to recruit early components of the CCC, such as C1q, mannose-binding lectin, and ficolins in efferocytosis (49, 84, 93, 134–137). Additionally, on the surface of apoptotic cells, regulators of complement activity - C4-binding protein (C4BP) and CFH - can attach directly to DAMPs or indirectly, e.g., through CRP (108, 138, 139). Recruitment of these complement regulators prevents the assembly of the membrane attack complex and lysis, keeping efferocytosis immunosilent. After engulfment of apoptotic cells opsonized with CRP and complement, macrophages maintain an anti-inflammatory status (136, 140, 141). In contrast to other complement components, FHR-1, -3, and -5 have been shown to bind to the surface of necrotic cells via OSEs, CRP, or other DAMPs to enhance opsonization by complement activation and act pro-inflammatory (111, 113, 132, 142).
Importantly, it has to be kept in mind that the combined recognition of MDA and any other co-expose OSE by various humoral immune responses as well as the cellular receptors binding them and/or the cellular receptors binding OSE directly will ultimately determine the net biological effect. The elucidation of these functional responses will provide insights into the pathophysiological relevance of OSE recognition by humoral immunity.
3 OSEs, recognition of OSEs and its clinical potential
Structures modified by OSEs have been detected practically everywhere, in various tissues and body fluids (4, 10).
As markers of enhanced oxidative stress, OSEs occur early, contribute to disease development, and have strong biomarker potential that has not been explored enough. The most commonly used assay for lipid peroxidation, thiobarbituric acid reactive substances assay (TBARS), is not specific, while mass spectrometry, immunological, chromatography, and imaging techniques are much more specific and reliable but costly. Still, even today, TBA-based assays are used in many clinical studies (143, 144). In contrast, studies that monitor immune responses against OSEs and their effects have not been broadly performed. Many such investigations have been conducted for atherosclerosis as a prototypical OSE-driven pathology with a vital contribution of the immune system. Evidence obtained from this research supports the observation that IgG antibodies to OxLDL are pro-atherogenic and increase the risk of developing CVDs – though this association may be more complex and depend on the IgG isotype. In contrast, IgM antibodies to OxLDL have largely shown to be associated with atheroprotection (145, 146). An even more precise prediction for CVD events was observed in a prospective 15-year-long study when the multivariable prediction models, including levels of oxidized phospholipids (OxPL)/apolipoprotein B (apoB) as OSEs, OSE-specific IgM and IgG antibodies, and CRP were used (88). It is known that the levels of CRP correlate with the levels of OSEs (MDA and 4-HNE), but if this association depends on CRP genetic variants influencing CRP levels and/or recruitment to damaged surfaces have not been investigated so far (147).
Furthermore, OSE-recognizing complement components have been associated with the development of autoimmunity, emphasizing the importance of these proteins in the clearance of damaged cells and prevention of autoantigen spill-over. So far, the interaction between C1q and MDA has not been characterized; therefore, no polymorphisms in C1q were shown to influence MDA-binding or levels. However, congenital C1q deficiency is associated with the development of lupus-like autoimmunity due to the impaired clearance of apoptotic cells (118, 148). Interestingly, in SLE, the OSE levels (MDA, 4-HNE, and OxPC) and OSE-specific IgM and IgG antibodies are altered (149–151).
The most important modulators of CFH binding to MDA-epitopes – the CFH variant Tyr402His and the deletion of CFHR3&CFHR1 genes – are frequent in the population and affect the development of the two most common diseases, atherosclerosis and AMD (52, 105). Although these diseases affect different organs, they have a similar underlying pathology linked with increased oxidative stress. Deletion of CFHR3&CFHR1 genes offers protection in both of them, while Tyr402His is deleterious in AMD and possibly atherosclerosis, highlighting CFH’s importance in homeostatic responses (110, 152–155). Since CFH is a crucial player in regulating complement activation, variants affecting its activity have been used in combined genetic risk scores/haplotypes for some complementopathies (153).
Based on the available literature, it is evident that the early appearance of OSEs and innate humoral immune responses are critical players in the development and progression of pathologies caused by oxidative stress (Figure 1). Therefore, using individual genetic variants or levels of OSE-recognizing proteins in disease prediction models generates less accurate prediction scores. To obtain a more precise, personalized risk prediction score for the ability of the host to deal with increased oxidative stress, we suggest using integrative analysis that considers all individual OSE-related parameters combined (levels of OSE and OSE-recognizing proteins and genetic variant within OSE-recognizing proteins). A strong argument for such an approach comes from a study in which combining CEP levels with AMD risk alleles in ARMS2, HtrA serine peptidase 1 (HTRA1), CFH or C3 showed a twofold to threefold increased risk score compared to a genotype-based score alone (156).
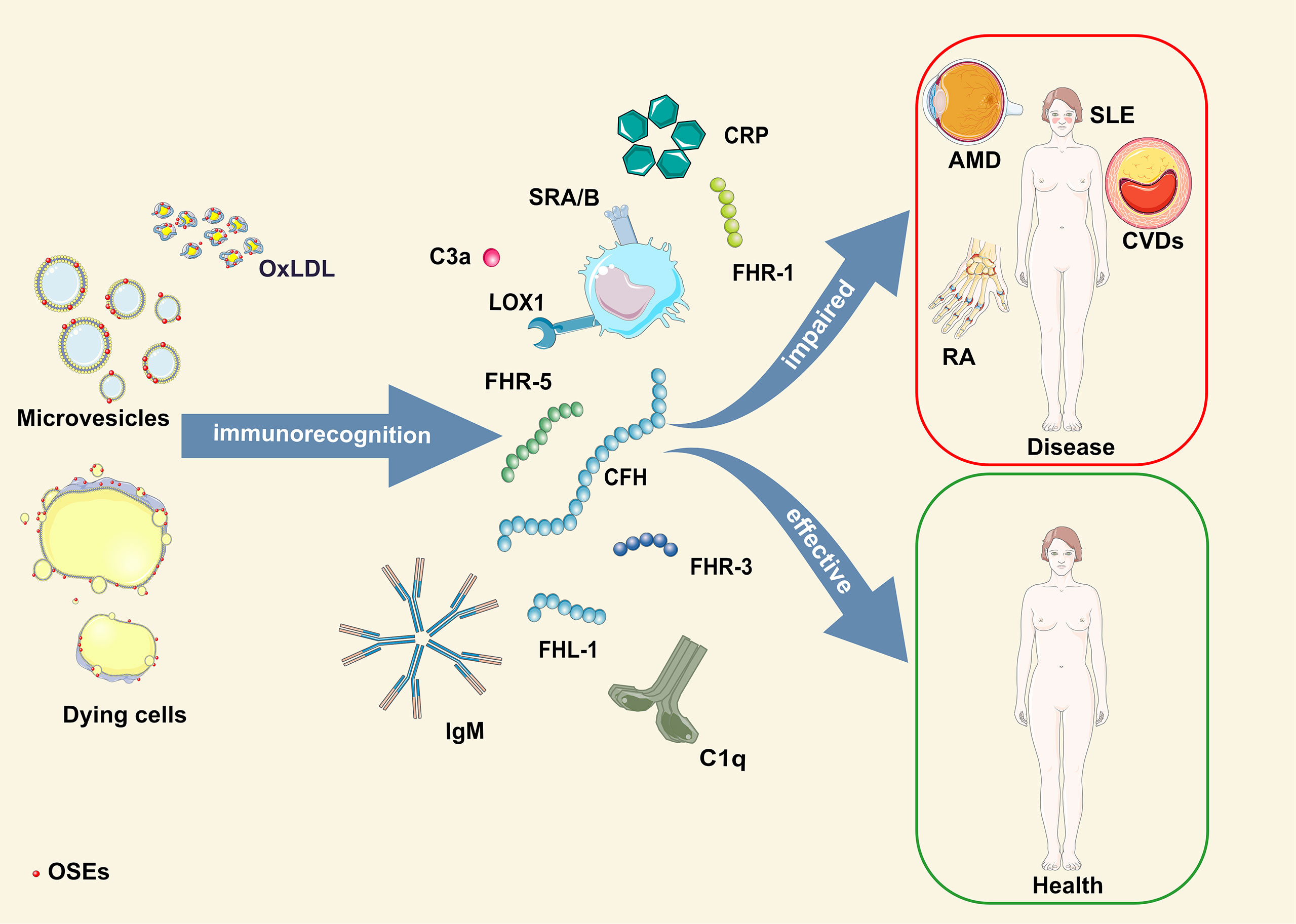
Figure 1 Schematic illustration of OSE humoral immunorecognition and consequences thereof. AMD, age-related macular degeneration; CFH, complement factor H-related protein; CRP, C-reactive protein; CVDs, cardiovascular diseases; FHL-1, factor H-like protein 1; FHR-1, -3, -5, complement factor H-related protein 1, 3, 5; OSE, oxidation-specific epitopes; OxLDL, oxidized low-density lipoprotein; RA, rheumatoid arthritis; SLE, systemic lupus erythematosus.
Thus, we propose a novel concept – the OSE complotype. The OSE complotype would include levels of specific OSEs, immune responses involved in their detection and clearance, and a repertoire of inherited genetic variants that modulate properties of OSE-recognizing proteins. This integrative method would allow for a more precision medicine-directed approach to evaluate the individual risk, progression, and therapeutic responses in oxidative stress-related diseases.
Author contributions
All authors listed have made a substantial, direct, and intellectual contribution to the work and approved it for publication.
Funding
CJB was supported by grants of the Austrian Science Fund (SFB F54) and the Leducq Foundation (TNE-20CVD03). NPM was supported by the Vienna Science and Technology Fund (WWTF LS20-081).
Acknowledgments
The figure was created using Servier Medical Art (https://servier.com/en/brochure/servier-medical-art/).
Conflict of interest
The authors declare that the research was conducted in the absence of any commercial or financial relationships that could be construed as a potential conflict of interest.
Publisher’s note
All claims expressed in this article are solely those of the authors and do not necessarily represent those of their affiliated organizations, or those of the publisher, the editors and the reviewers. Any product that may be evaluated in this article, or claim that may be made by its manufacturer, is not guaranteed or endorsed by the publisher.
References
1. Binder CJ, Papac-Milicevic N, Witztum JL. Innate sensing of oxidation-specific epitopes in health and disease. Nat Rev Immunol (2016) 16(8):485–97. doi: 10.1038/nri.2016.63
2. Obermayer G, Afonyushkin T, Binder CJ. Oxidized low-density lipoprotein in inflammation-driven thrombosis. J Thromb Haemost (2018) 16(3):418–28. doi: 10.1111/jth.13925
3. Imai Y, Kuba K, Neely GG, Yaghubian-Malhami R, Perkmann T, van Loo G, et al. Identification of oxidative stress and toll-like receptor 4 signaling as a key pathway of acute lung injury. Cell (2008) 133(2):235–49. doi: 10.1016/j.cell.2008.02.043
4. Ambrogini E, Que X, Wang S, Yamaguchi F, Weinstein RS, Tsimikas S, et al. Oxidation-specific epitopes restrain bone formation. Nat Commun (2018) 9(1):1–11. doi: 10.1038/s41467-018-04047-5
5. Maes M, Mihaylova I, Kubera M, Leunis JC, Geffard M. IgM-mediated autoimmune responses directed against multiple neoepitopes in depression: New pathways that underpin the inflammatory and neuroprogressive pathophysiology. J Affect Disord (2011) 135(1–3):414–8. doi: 10.1016/j.jad.2011.08.023
6. Furnkranz A, Schober A, Bochkov VN, Bashtrykov P, Kronke G, Kadl A, et al. Oxidized phospholipids trigger atherogenic inflammation in murine arteries. Arterioscler Thromb Vasc Biol (2005) 25(3):633–8. doi: 10.1161/01.ATV.0000153106.03644.a0
7. Palmieri M, Kim H, Gomez-Acevedo H, Que X, Tsimikas S, Jilka RL, et al. A neutralizing antibody targeting oxidized phospholipids promotes bone anabolism in chow-fed young adult mice. J Bone Miner Res (2021) 36(1):170–85. doi: 10.1002/jbmr.4173
8. Esterbauer H, Schaur RJ, Zollner H. Chemistry and biochemistry of 4-hydroxynonenal, malonaldehyde and related aldehydes. Free Radic Biol Med (1991) 11(1):81–128. doi: 10.1016/0891-5849(91)90192-6
9. Papac-Milicevic N, Busch CJL, Binder CJ. Malondialdehyde epitopes as targets of immunity and the implications for atherosclerosis. In: Advances in immunology, 1st ed. Cambridge, MA, USA: Elsevier Inc (2016). p. 1–59. doi: 10.1016/bs.ai.2016.02.001
10. Lesgards JF, Frayne IR, Comte B, Busseuil D, Rhéaume É, Tardif JC, et al. Differential distribution of 4-hydroxynonenal adducts to sulfur and nitrogen residues in blood proteins as revealed using raney nickel and gas chromatography-mass spectrometry. Free Radic Biol Med (2009) 47(10):1375–85. doi: 10.1016/j.freeradbiomed.2009.08.002
11. Salomon RG. Carboxyethylpyrroles: From hypothesis to the discovery of biologically active natural products. Chem Res Toxicol (2017) 30(1):105–13. doi: 10.1021/acs.chemrestox.6b00304
12. Reis A, Domingues P, Ferrer-Correia AJV, Domingues MRM. Peptide-phospholipid cross-linking reactions: Identification of leucine enkephalin-Alka(e)nal-Glycerophosphatidylcholine adducts by tandem mass spectrometry. J Am Soc Mass Spectrom (2006) 17(5):657–60. doi: 10.1016/j.jasms.2006.01.006
13. Domingues RM, Domingues P, Melo T, Pérez-Sala D, Reis A, Spickett CM. Lipoxidation adducts with peptides and proteins: Deleterious modifications or signaling mechanisms? J Proteomics (2013) 92:110–31. doi: 10.1016/j.jprot.2013.06.004
14. Nakashima I, Liu W, Akhand AA, Takeda K, Kawamoto Y, Kato M, et al. 4-hydroxynonenal triggers multistep signal transduction cascades for suppression of cellular functions. Mol Aspects Med (2003) 24(4–5):231–8. doi: 10.1016/S0098-2997(03)00018-9
15. Xu J, Zhong Y, Jing D, Wu Z. Preoperative enteral immunonutrition improves postoperative outcome in patients with gastrointestinal cancer. World J Surg (2006) 30(7):1284–9. doi: 10.1007/s00268-005-0756-8
16. Bhuyan DK, Master RWP, Bhuyan KC. Crosslinking of aminophospholipids in cellular membranes of lens by oxidative stress in vitro. Biochim Biophys Acta - Biomembr (1996) 1285(1):21–8. doi: 10.1016/S0005-2736(96)00142-3
17. Guo L, Chen Z, Amarnath V, Davies SS. Identification of novel bioactive aldehyde-modified phosphatidylethanolamines formed by lipid peroxidation. Free Radic Biol Med (2012) 53(6):1226–38. doi: 10.1016/j.freeradbiomed.2012.07.077
18. Xiao M, Zhong H, Xia L, Tao Y, Yin H. Pathophysiology of mitochondrial lipid oxidation: Role of 4-hydroxynonenal (4-HNE) and other bioactive lipids in mitochondria. Free Radic Biol Med (2017) 111:316–27. doi: 10.1016/j.freeradbiomed.2017.04.363
19. Guo J, Wang H, Hrinczenko B, Salomon RG. Efficient quantitative analysis of carboxyalkylpyrrole ethanolamine phospholipids: Elevated levels in sickle cell disease blood. Chem Res Toxicol (2016) 29(7):1187–97. doi: 10.1021/acs.chemrestox.6b00152
20. Wang H, Guo J, West XZ, Bid HK, Lu L, Hong L, et al. Detection and biological activities of carboxyethylpyrrole ethanolamine phospholipids (CEP-EPs). Chem Res Toxicol (2014) 27(12):2015–22. doi: 10.1021/tx500216a
21. Biswas S, Xin L, Panigrahi S, Zimman A, Wang H, Yakubenko VP, et al. Novel phosphatidylethanolamine derivatives accumulate in circulation in hyperlipidemic ApoE-/- mice and activate platelets via TLR2. Blood (2016) 127(21):2618–29. doi: 10.1182/blood-2015-08-664300
22. Borisenko GG, Iverson SL, Ahlberg S, Kagan VE, Fadeel B. Milk fat globule epidermal growth factor 8 (MFG-E8) binds to oxidized phosphatidylserine: Implications for macrophage clearance of apoptotic cells. Cell Death Differ (2004) 11(8):943–5. doi: 10.1038/sj.cdd.4401421
23. Guichardant M, Bernoud-Hubac N, Chantegrel B, Deshayes C, Lagarde M. Aldehydes from n-6 fatty acid peroxidation. effects on aminophospholipids. Prostaglandins Leukot Essent Fat Acids (2002) 67(2–3):147–9. doi: 10.1054/plef.2002.0412
24. Uderhardt S, Herrmann M, Oskolkova OV, Aschermann S, Bicker W, Ipseiz N, et al. 12/15-lipoxygenase orchestrates the clearance of apoptotic cells and maintains immunologic tolerance. Immunity (2012) 36(5):834–46. doi: 10.1016/j.immuni.2012.03.010
25. Palinski W, Hörkkö S, Miller E, Steinbrecher UP, Powell HC, Curtiss LK, et al. Cloning of monoclonal autoantibodies to epitopes of oxidized lipoproteins from apolipoprotein e-deficient mice: Demonstration of epitopes of oxidized low density lipoprotein in human plasma. J Clin Invest (1996) 98:800–14. doi: 10.1172/JCI118853
26. Steinberg D, Witztum JL. History of discovery: Oxidized low-density lipoprotein and atherosclerosis. Arterioscler Thromb Vasc Biol (2010) 30(12):2311–6. doi: 10.1161/ATVBAHA.108.179697
27. Roy P, Orecchioni M, Ley K. How the immune system shapes atherosclerosis: roles of innate and adaptive immunity. Nat Rev Immunol (2022) 22(4):251–65. doi: 10.1038/s41577-021-00584-1
28. Quinn MT, Parthasarathy S, Fong LG, Steinberg D. Oxidatively modified low density lipoproteins: A potential role in recruitment and retention of monocyte/macrophages during atherogenesis. Proc Natl Acad Sci USA (1987) 84(9):2995–8. doi: 10.1073/pnas.84.9.2995
29. Glass CK, Witztum JL. Atherosclerosis. Cell (2001) 104(4):503–16. doi: 10.1016/S0092-8674(01)00238-0
30. Leibundgut G, Witztum JL, Tsimikas S. Oxidation-specific epitopes and immunological responses: Translational biotheranostic implications for atherosclerosis. Curr Opin Pharmacol (2013) 13(2):168–79. doi: 10.1016/j.coph.2013.02.005
31. Hörkkö S, Bird DA, Miller E, Itabe H, Leitinger N, Subbanagounder G, et al. Monoclonal autoantibodies specific for oxidized phospholipids or oxidized phospholipid-protein adducts inhibit macrophage uptake of oxidized low-density lipoproteins. J Clin Invest (1999) 103(1):117–28. doi: 10.1172/JCI4533
32. Jurgens G, Chen Q, Esterbauer H, Mair S, Ledinski G, Dinges HP. Immunostaining of human autopsy aortas with antibodies to modified apolipoprotein b and apoprotein(a). Arterioscler Thromb (1993) 13(11):1689–99. doi: 10.1161/01.atv.13.11.1689
33. Tuominen A, Miller YI, Hansen LF, Kesäniemi YA, Witztum JL, Hörkkö S. A natural antibody to oxidized cardiolipin binds to oxidized low-density lipoprotein, apoptotic cells, and atherosclerotic lesions. Arterioscler Thromb Vasc Biol (2006) 26(9):2096–102. doi: 10.1161/01.ATV.0000233333.07991.4a
34. Amir S, Hartvigsen K, Gonen A, Leibundgut G, Que X, Jensen-Jarolim E, et al. Peptide mimotopes of malondialdehyde epitopes for clinical applications in cardiovascular disease. J Lipid Res (2012) 53(7):1316–26. doi: 10.1194/jlr.M025445
35. Que X, Hung MY, Yeang C, Gonen A, Prohaska TA, Sun X, et al. Oxidized phospholipids are proinflammatory and proatherogenic in hypercholesterolaemic mice. Nature (2018) 558(7709):301–6. doi: 10.1038/s41586-018-0198-8
36. Sawada N, Obama T, Mizuno M, Fukuhara K, Iwamoto S, Aiuchi T, et al. Transfer and enzyme-mediated metabolism of oxidized phosphatidylcholine and lysophosphatidylcholine between low-and high-density lipoproteins. Antioxidants (2020) 9(11):1–13. doi: 10.3390/antiox9111045
37. Shao B, Tang C, Heinecke JW, Oram JF. Oxidation of apolipoprotein a-I by myeloperoxidase impairs the initial interactions with ABCA1 required for signaling and cholesterol export. J Lipid Res (2010) 51(7):1849–58. doi: 10.1194/jlr.M004085
38. Salmon S, Maziére C, Auclair M, Theron L, Santus R, Maziére JC. Malondialdehyde modification and copper-induced autooxidation of high-density lipoprotein decrease cholesterol efflux from human cultured fibroblasts. Biochim Biophys Acta (BBA)/Lipids Lipid Metab (1992) 1125(2):230–5. doi: 10.1016/0005-2760(92)90050-6
39. Florens N, Calzada C, Lemoine S, Boulet MM, Guillot N, Barba C, et al. CKD increases carbonylation of HDL and is associated with impaired antiaggregant properties. J Am Soc Nephrol (2020) 31(7):1462–77. doi: 10.1681/ASN.2019111205
40. Lê QH, El Alaoui M, Véricel E, Ségrestin B, Soulère L, Guichardant M, et al. HDL enriched with oxidized phospholipids and HDL from diabetic patients inhibit platelet function. J Clin Endocrinol Metab (2015) 100(5):2006–14. doi: 10.1210/jc.2014-4214
41. Assinger A, Koller F, Schmid W, Zellner M, Babeluk R, Koller E, et al. Specific binding of hypochlorite-oxidized HDL to platelet CD36 triggers proinflammatory and procoagulant effects. Atherosclerosis (2010) 212(1):153–60. doi: 10.1016/j.atherosclerosis.2010.05.010
42. Obermayer G, Afonyushkin T, Göderle L, Puhm F, Schrottmaier WC, Taqi S, et al. Natural IgM antibodies inhibit microvesicle-driven coagulation and thrombosis. Blood (2020) 137(10):1406–15. doi: 10.1182/blood.2020007155
43. Van Niel G, D’Angelo G, Raposo G. Shedding light on the cell biology of extracellular vesicles. Nat Rev Mol Cell Biol (2018) 19(4):213–28. doi: 10.1038/nrm.2017.125
44. Witwer KW, Buzás EI, Bemis LT, Bora A, Lässer C, Lötvall J, et al. Standardization of sample collection, isolation and analysis methods in extracellular vesicle research. J Extracell Vesicles (2013) 2(1):20360. doi: 10.3402/jev.v2i0.20360
45. Huber J, Vales A, Mitulovic G, Blumer M, Schmid R, Witztum JL, et al. Oxidized membrane vesicles and blebs from apoptotic cells contain biologically active oxidized phospholipids that induce monocyte-endothelial interactions. Arterioscler Thromb Vasc Biol (2002) 22(1):101–7. doi: 10.1161/hq0102.101525
46. Liu ML, Scalia R, Mehta JL, Williams KJ. Cholesterol-induced membrane microvesicles as novel carriers of damage-associated molecular patterns: Mechanisms of formation, action, and detoxification. Arterioscler Thromb Vasc Biol (2012) 32(9):2113–21. doi: 10.1161/ATVBAHA.112.255471
47. Tsiantoulas D, Perkmann T, Afonyushkin T, Mangold A, Prohaska TA, Papac-Milicevic N, et al. Circulating microparticles carry oxidation-specific epitopes and are recognized by natural IgM antibodies. J Lipid Res (2015) 56(2):440–8. doi: 10.1194/jlr.P054569
48. Chang MK, Bergmark C, Laurila A, Hörkkö S, Han KH, Friedman P, et al. Monoclonal antibodies against oxidized low-density lipoprotein bind to apoptotic cells and inhibit their phagocytosis by elicited macrophages: Evidence that oxidation-specific epitopes mediate macrophage recognition. P Natl Acad Sci USA (1999) 96(May):6353–8. doi: 10.1073/pnas.96.11.6353
49. Chang MK, Binder CJ, Torzewski M. Witztum JL. c-reactive protein binds to both oxidized LDL and apoptotic cells through recognition of a common ligand: Phosphorylcholine of oxidized phospholipids. Proc Natl Acad Sci USA (2002) 99(20):13043–8. doi: 10.1073/pnas.192399699
50. Chou MY, Fogelstrand L, Hartvigsen K, Hansen LF, Woelkers D, Shaw PX, et al. Oxidation-specific epitopes are dominant targets of innate natural antibodies in mice and humans. J Clin Invest (2009) 119(5):1335–49. doi: 10.1172/JCI36800
51. Busch CJL, Hendrikx T, Weismann D, Jäckel S, Walenbergh SMA, Rendeiro AF, et al. Malondialdehyde epitopes are sterile mediators of hepatic inflammation in hypercholesterolemic mice. Hepatology (2017) 65(4):1181–95. doi: 10.1002/hep.28970
52. Weismann D, Hartvigsen K, Lauer N, Bennett KL, Scholl HPN, Issa PC, et al. Complement factor h binds malondialdehyde epitopes and protects from oxidative stress. Nature (2011) 478(7367):76–81. doi: 10.1038/nature10449
53. Terpstra V, Bird DA, Steinberg D. Evidence that the lipid moiety of oxidized low density lipoprotein plays a role in its interaction with macrophage receptors. Proc Natl Acad Sci USA (1998) 95(4):1806–11. doi: 10.1073/pnas.95.4.1806
54. Chen GY, Nuñez G. Sterile inflammation: sensing and reacting to damage. Nat Rev Immunol (2010) 10(12):826–37. doi: 10.1038/nri2873
55. Palinski W, Rosenfeld ME, Ylä-Herttuala S, Gurtner GC, Socher SS, Butler SW, et al. Low density lipoprotein undergoes oxidative modification in vivo. Proc Natl Acad Sci USA (1989) 86(4):1372–6. doi: 10.1073/pnas.86.4.1372
56. Thiele GM, Duryee MJ, Anderson DR, Klassen LW, Mohring SM, Young KA, et al. Malondialdehyde-acetaldehyde adducts and anti-Malondialdehyde-Acetaldehyde antibodies in rheumatoid arthritis. Arthritis Rheumatol (2015) 67(3):645–55. doi: 10.1002/art.38969
57. Moretto N, Facchinetti F, Southworth T, Civelli M, Singh D, Patacchini R. α,β-unsaturated aldehydes contained in cigarette smoke elicit IL-8 release in pulmonary cells through mitogen-activated protein kinases. Am J Physiol - Lung Cell Mol Physiol (2009) 296(5):839–49. doi: 10.1152/ajplung.90570.2008
58. Facchinetti F, Amadei F, Geppetti P, Tarantini F, Di Serio C, Dragotto A, et al. A,B-unsaturated aldehydes in cigarette smoke release inflammatory mediators from human macrophages. Am J Respir Cell Mol Biol (2007) 37(5):617–23. doi: 10.1165/rcmb.2007-0130OC
59. Gargiulo S, Gamba P, Testa G, Rossin D, Biasi F, Poli G, et al. Relation between TLR4/NF-κB signaling pathway activation by 27-hydroxycholesterol and 4-hydroxynonenal, and atherosclerotic plaque instability. Aging Cell (2015) 14(4):569–81. doi: 10.1111/acel.12322
60. Takimoto T, Yoshida M, Hirata H, Kashiwa Y, Takeda Y, Goya S, et al. 4-Hydroxy-2-nonenal induces chronic obstructive pulmonary disease-like histopathologic changes in mice. Biochem Biophys Res Commun (2012) 420(1):84–90. doi: 10.1016/j.bbrc.2012.02.119
61. Duryee MJ, Klassen LW, Freeman TL, Willis MS, Tuma DJ, Thiele GM. Lipopolysaccharide is a cofactor for malondialdehyde-acetaldehyde adduct-mediated cytokine/chemokine release by rat sinusoidal liver endothelial and kupffer cells. Alcohol Clin Exp Res (2004) 28(12):1931–8. doi: 10.1097/01.ALC.0000148115.90045.C5
62. Shechter I, Fogelman AM, Haberland ME, Seager J, Hokom M, Edwards PA. The metabolism of native and malondialdehyde-altered low density lipoproteins by human monocyte-macrophages. J Lipid Res (1981) 22(1):63–71. doi: 10.1016/s0022-2275(20)34741-6
63. Miller YI, Choi SH, Wiesner P, Fang L, Harkewicz R, Hartvigsen K, et al. Oxidation-specific epitopes are danger-associated molecular patterns recognized by pattern recognition receptors of innate immunity. Circ Res (2011) 108(2):235–48. doi: 10.1161/CIRCRESAHA.110.223875
64. Zhu X, Ng HP, Lai YC, Craigo JK, Nagilla PS, Raghani P, et al. Scavenger receptor function of mouse fcγ receptor III contributes to progression of atherosclerosis in apolipoprotein e hyperlipidemic mice. J Immunol (2014) 193(5):2483–95. doi: 10.4049/jimmunol.1303075
65. Ansari SA, Keshava S, Pendurthi UR, Rao LVM. Oxidative stress product, 4-Hydroxy-2-Nonenal, induces the release of tissue factor-positive microvesicles from perivascular cells into circulation. Arterioscler Thromb Vasc Biol (2021) 41(1):250–65. doi: 10.1161/ATVBAHA.120.315187
66. Kumano-Kuramochi M, Xie Q, Kajiwara S, Komba S, Minowa T, Machida S. Lectin-like oxidized LDL receptor-1 is palmitoylated and internalizes ligands via caveolae/raft-dependent endocytosis. Biochem Biophys Res Commun (2013) 434(3):594–9. doi: 10.1016/j.bbrc.2013.03.120
67. Loidl A, Sevcsik E, Riesenhuber G, Deigner HP, Hermetter A. Oxidized phospholipids in minimally modified low density lipoprotein induce apoptotic signaling via activation of acid sphingomyelinase in arterial smooth muscle cells. J Biol Chem (2003) 278(35):32921–8. doi: 10.1074/jbc.M306088200
68. Gao D, Ashraf MZ, Kar NS, Lin D, Sayre LM, Podrez EA. Structural basis for the recognition of oxidized phospholipids in oxidized low density lipoproteins by class b scavenger receptors CD36 and SR-BI. J Biol Chem (2010) 285(7):4447–54. doi: 10.1074/jbc.M109.082800
69. Kadl A, Sharma PR, Chen W, Agrawal R, Meher AK, Rudraiah S, et al. Oxidized phospholipid-induced inflammation is mediated by toll-like receptor 2. Free Radic Biol Med (2011) 51(10):1903–9. doi: 10.1016/j.freeradbiomed.2011.08.026
70. Stewart CR, Stuart LM, Wilkinson K, Van Gils JM, Deng J, Halle A, et al. CD36 ligands promote sterile inflammation through assembly of a toll-like receptor 4 and 6 heterodimer. Nat Immunol (2010) 11(2):155–61. doi: 10.1038/ni.1836
71. Doyle SL, Campbell M, Ozaki E, Salomon RG, Mori A, Kenna PF, et al. NLRP3 has a protective role in age-related macular degeneration through the induction of IL-18 by drusen components. Nat Med (2012) 18(5):791–8. doi: 10.1038/nm.2717
72. Cruz-Guilloty F, Saeed AM, Echegaray JJ, Duffort S, Ballmick A, Tan Y, et al. Infiltration of proinflammatory m1 macrophages into the outer retina precedes damage in a mouse model of age-related macular degeneration. Int J Inflam (2013) 2013:1–12. doi: 10.1155/2013/503725
73. West XZ, Malinin NL, Merkulova AA, Tischenko M, Kerr BA, Borden EC, et al. Oxidative stress induces angiogenesis by activating TLR2 with novel endogenous ligands. Nature (2010) 467(7318):972–6. doi: 10.1038/nature09421.Oxidative
74. Salomon RG, Hong L, Hollyfield JG. Discovery of carboxyethylpyrroles (CEPs): Critical insights into AMD, autism, cancer, and wound healing from basic research on the chemistry of oxidized phospholipids. Chem Res Toxicol (2011) 24(11):1803–16. doi: 10.1021/tx200206v
75. Yakubenko VP, Cui K, Ardell CL, Brown KE, West XZ, Gao D, et al. Oxidative modifications of extracellular matrix promote the second wave of inflammation via b2 integrins. Blood (2018) 132(1):78–88. doi: 10.1182/blood-2017-10-810176
76. Kim YW, Yakubenko VP, West XZ, Gugiu GB, Renganathan K, Biswas S, et al. Receptor-mediated mechanism controlling tissue levels of bioactive lipid oxidation products. Circ Res (2015) 117(4):321–32. doi: 10.1161/CIRCRESAHA.117.305925
77. Boullier A, Gillotte KL, Hörkkö S, Green SR, Friedman P, Dennis EA, et al. The binding of oxidized low density lipoprotein to mouse CD36 is mediated in part by oxidized phospholipids that are associated with both the lipid and protein moieties of the lipoprotein. J Biol Chem (2000) 275(13):9163–9. doi: 10.1074/jbc.275.13.9163
78. Chang MK, Binder CJ, Miller YI, Subbanagounder G, Silverman GJ, Berliner JA, et al. Apoptotic cells with oxidation-specific epitopes are immunogenic and proinflammatory. J Exp Med (2004) 200(11):1359–70. doi: 10.1084/jem.20031763
79. Gruber S, Hendrikx T, Tsiantoulas D, Ozsvar-Kozma M, Göderle L, Mallat Z, et al. Sialic acid-binding immunoglobulin-like lectin G promotes atherosclerosis and liver inflammation by suppressing the protective functions of b-1 cells. Cell Rep (2016) 14(10):2348–61. doi: 10.1016/j.celrep.2016.02.027
80. Faria-Neto JR, Chyu KY, Li X, Dimayuga PC, Ferreira C, Yano J, et al. Passive immunization with monoclonal IgM antibodies against phosphorylcholine reduces accelerated vein graft atherosclerosis in apolipoprotein e-null mice. Atherosclerosis (2006) 189(1):83–90. doi: 10.1016/j.atherosclerosis.2005.11.033
81. Gonen A, Hansen LF, Turner WW, Montano EN, Que X, Rafia A, et al. Atheroprotective immunization with malondialdehyde modified LDL is hapten-specific and dependant on advanced MDA-adducts: Implication for development of an atheroprotective vaccine. J Lipid Res (2014) 55(10):2137–55. doi: 10.1194/jlr.M053256
82. Freigang S, Hörkkö S, Miller E, Witztum JL, Palinski W. Immunization of LDL receptor-deficient mice with homologous malondialdehyde-modified and native LDL reduces progression of atherosclerosis by mechanisms other than induction of high titers of antibodies to oxidative neoepitopes. Arterioscler Thromb Vasc Biol (1998) 18(12):1972–82. doi: 10.1161/01.ATV.18.12.1972
83. Notley CA, Brown MA, Wright GP, Ehrenstein MR. Natural IgM is required for suppression of inflammatory arthritis by apoptotic cells. J Immunol (2011) 186(8):4967–72. doi: 10.4049/jimmunol.1003021
84. Chen Y, Khanna S, Goodyear CS, Park YB, Raz E, Thiel S, et al. Regulation of dendritic cells and macrophages by an anti-apoptotic cell natural antibody that suppresses TLR responses and inhibits inflammatory arthritis. J Immunol (2009) 183(2):1346–59. doi: 10.4049/jimmunol.0900948
85. Boes M, Schmidt T, Linkemann K, Beaudette BC, Marshak-Rothstein A, Chen J. Accelerated development of IgG autoantibodies and autoimmune disease in the absence of secreted IgM. Proc Natl Acad Sci USA (2000) 97(3):1184–9. doi: 10.1073/pnas.97.3.1184
86. Karvonen J, Päivänsalo M, Kesäniemi YA, Hörkkö S. Immunoglobulin m type of autoantibodies to oxidized low-density lipoprotein has an inverse relation to carotid artery atherosclerosis. Circulation (2003) 108:2107–12. doi: 10.1161/01.CIR.0000092891.55157.A7
87. Tsimikas S, Brilakis ES, Lennon RJ, Miller ER, Witztum JL, McConnell JP, et al. Relationship of IgG and IgM autoantibodies to oxidized low density lipoprotein with coronary artery disease and cardiovascular events. J Lipid Res (2007) 48(2):425–33. doi: 10.1194/jlr.M600361-JLR200
88. Tsimikas S, Willeit P, Willeit J, Santer P, Mayr M, Xu Q, et al. Oxidation-specific biomarkers, prospective 15-year cardiovascular and stroke outcomes, and net reclassification of cardiovascular events. J Am Coll Cardiol (2012) 60(21):2218–29. doi: 10.1016/j.jacc.2012.08.979
89. Khamis RY, Hughes AD, Caga-anan M, Chang CL, Boyle JJ, Kojima C, et al. High serum immunoglobulin G and m levels predict freedom from adverse cardiovascular events in Hypertension : A nested case-control substudy of the Anglo-Scandinavian cardiac outcomes trial. EBioMedicine (2016) 9:372–80. doi: 10.1016/j.ebiom.2016.06.012
90. Eichinger S, Kyrle PA, Kammer M, Eischer L, Ozsvar-Kozma M, Binder CJ. Natural antibodies to oxidation-specific epitopes: Innate immune response and venous thromboembolic disease. JTH (2016) 38(1):42–9. doi: 10.1111/ijlh.12426
91. Frostegård J, Su J, Sing S, Hua X, Vikström M, Leander K, et al. IgM antibodies to oxidized phosphatidylserine as protection markers in cardiovascular disease among 60-year olds. PloS One (2017) 12(4):1–13. doi: 10.1371/journal.pone.0171195
92. van den Berg VJ, Haskard DO, Fedorowski A, Hartley A, Kardys I, Caga-Anan M, et al. IgM anti-malondialdehyde low density lipoprotein antibody levels indicate coronary heart disease and necrotic core characteristics in the Nordic diltiazem (NORDIL) study and the integrated imaging and biomarker study 3 (IBIS-3). EBioMedicine (2018) 36:63–72. doi: 10.1016/j.ebiom.2018.08.023
93. Ciurana CLF, Hack CE. Competitive binding of pentraxins and IgM to newly exposed epitopes on late apoptotic cells. Cell Immunol (2006) 239:14–21. doi: 10.1016/j.cellimm.2006.02.006
94. Bíró A, Rovó Z, Papp D, Cervenak L, Varga L, Füst G, et al. Studies on the interactions between c-reactive protein and complement proteins. Immunology (2007) 121(1):40–50. doi: 10.1111/j.1365-2567.2007.02535.x
95. Gustin C, Delaive E, Dieu M, Calay D, Raes M. Upregulation of pentraxin-3 in human endothelial cells after lysophosphatidic acid exposure. Arterioscler Thromb Vasc Biol (2008) 28(3):491–7. doi: 10.1161/ATVBAHA.107.158642
96. Liu W, Jiang J, Yan D, Li D, Li W, Ma Y, et al. Pentraxin 3 promotes oxLDL uptake and inhibits cholesterol efflux from macrophage-derived foam cells. Exp Mol Pathol (2014) 96(3):292–9. doi: 10.1016/j.yexmp.2014.03.007
97. Biró A, Thielens NM, Cervenák L, Prohászka Z, Füst G, Arlaud GJ. Modified low density lipoproteins differentially bind and activate the C1 complex of complement. Mol Immunol (2007) 44(6):1169–77. doi: 10.1016/j.molimm.2006.06.013
98. Fraser DA, Tenner AJ. Innate immune proteins C1q and mannan-binding lectin enhance clearance of atherogenic lipoproteins by human monocytes and macrophages. J Immunol (2010) 185(7):3932–9. doi: 10.4049/jimmunol.1002080
99. Spivia W, Magno PS, Le P, Fraser DA. Complement protein C1q promotes macrophage anti-inflammatory M2-like polarization during the clearance of atherogenic lipoproteins. Inflammation Res (2014) 63(10):885–93. doi: 10.1007/s00011-014-0762-0
100. Yin C, Ackermann S, Ma Z, Mohanta SK, Zhang C, Li Y, et al. ApoE attenuates unresolvable inflammation by complex formation with activated C1q. Nat Med (2019) 25(3):496–506. doi: 10.1038/s41591-018-0336-8
101. Veneskoski M, Turunen SP, Kummu O, Nissinen A, Rannikko S, Levonen AL, et al. Specific recognition of malondialdehyde and malondialdehyde acetaldehyde adducts on oxidized LDL and apoptotic cells by complement anaphylatoxin C3a. Free Radic Biol Med (2011) 51(4):834–43. doi: 10.1016/j.freeradbiomed.2011.05.029
102. Mogilenko DA, Kudriavtsev IV, Trulioff AS, Shavva VS, Dizhe EB, Missyul BV, et al. Modified low density lipoprotein stimulates complement C3 expression and secretion via liver X receptor and toll-like receptor 4 activation in human macrophages. J Biol Chem (2012) 287(8):5954–68. doi: 10.1074/jbc.M111.289322
103. Aredo B, Li T, Chen X, Zhang K, Wang CXZZ, Gou D, et al. A chimeric cfh transgene leads to increased retinal oxidative stress, inflammation, and accumulation of activated subretinal microglia in mice. Investig Opthalmol Vis Sci (2015) 56(6):3427. doi: 10.1167/iovs.14-16089
104. Hebecker M, Alba-Dominguez M, Roumenina LT, Reuter S, Hyvarinen S, Dragon-Durey MA, et al. An engineered construct combining complement regulatory and surface-recognition domains represents a minimal-size functional factor h. J Immunol (2013) 191:912–21. doi: 10.4049/jimmunol.1300269
105. Alic L, Papac-Milicevic N, Czamara D, Rudnick RB, Ozsvar-Kozma M, Hartmann A, et al. A genome-wide association study identifies key modulators of complement factor h binding to malondialdehyde-epitopes. Proc Natl Acad Sci USA (2020) 117(18):9942–51. doi: 10.1073/pnas.1913970117
106. Hyvärinen S, Uchida K, Varjosalo M, Jokela R, Jokiranta TS. Recognition of malondialdehyde-modified proteins by the c terminus of complement factor h is mediated via the polyanion binding site and impaired by mutations found in atypical hemolytic uremic syndrome. J Biol Chem (2014) 289(7):4295–306. doi: 10.1074/jbc.M113.527416
107. Shaw PX, Zhang L, Zhang M, Du H, Zhao L, Lee C, et al. Complement factor h genotypes impact risk of age-related macular degeneration by interaction with oxidized phospholipids. Proc Natl Acad Sci USA (2012) 109(34):13757–62. doi: 10.1073/pnas.1121309109
108. Sjöberg AP, Trouw LA, Clark SJ, Sjölander J, Heinegård D, Sim RB, et al. The factor h variant associated with age-related macular degeneration (His-384) and the non-disease-associated form bind differentially to c-reactive protein, fibromodulin, DNA, and necrotic cells. J Biol Chem (2007) 282(15):10894–900. doi: 10.1074/jbc.M610256200
109. Borras C, Canonica J, Jorieux S, Abache T, El Sanharawi M, Klein C, et al. CFH exerts anti-oxidant effects on retinal pigment epithelial cells independently from protecting against membrane attack complex. Sci Rep (2019) 9(1):1–12. doi: 10.1038/s41598-019-50420-9
110. Irmscher S, Zipfel SLH, Halder LD, Ivanov L, Gonzalez-Delgado A, Waldeyer C, et al. Factor h-related protein 1 (FHR-1) is associated with atherosclerotic cardiovascular disease. Sci Rep (2021) 11(1):1–15. doi: 10.1038/s41598-021-02011-w
111. Irmscher S, Brix SR, Zipfel SLH, Halder LD, Mutlutürk S, Wulf S, et al. Serum FHR1 binding to necrotic-type cells activates monocytic inflammasome and marks necrotic sites in vasculopathies. Nat Commun (2019) 10(1):1–14. doi: 10.1038/s41467-019-10766-0
112. Schäfer N, Rasras A, Ormenisan DM, Amslinger S, Enzmann V, Jägle H, et al. Complement factor h-related 3 enhanced inflammation and complement activation in human RPE cells. Front Immunol (2021) 12:769242(November). doi: 10.3389/fimmu.2021.769242
113. Rudnick RB, Chen Q, Stea ED, Hartmann A, Papac-Milicevic N, Person F, et al. FHR5 binds to laminins, uses separate C3b and surface-binding sites, and activates complement on malondialdehyde-acetaldehyde surfaces. J Immunol (2018) 200(7):2280–90. doi: 10.4049/jimmunol.1701641
114. Shaw PX, Hörkkö S, Chang M kyung K, Curtiss LK, Palinski W, Silverman GJ, et al. Natural antibodies with the T15 idiotype may act in atherosclerosis, apoptotic clearance, and protective immunity. J Clin Invest (2000) 105(12):1731–40. doi: 10.1172/JCI8472
115. Binder CJ. Natural IgM antibodies against oxidation-specific epitopes. J Clin Immunol (2010) 30(April):56–60. doi: 10.1007/s10875-010-9396-3
116. Haapasalo K, Meri S. Regulation of the complement system by pentraxins. Front Immunol (2019) 10:1750(August). doi: 10.3389/fimmu.2019.01750
117. Wang L, Cano M, Datta S, Wei H, Ebrahimi KB, Gorashi Y, et al. Pentraxin 3 recruits complement factor h to protect against oxidative stress-induced complement and inflammasome overactivation. J Pathol (2016) 240(4):495–506. doi: 10.1002/path.4811
118. Botto M, Dell’ Agnola C, Bygrave AE, Thompson EM, Cook HT, Petry F, et al. Homozygous C1q deficiency causes glomerulonephritis associated with multiple apoptotic bodies. Nat Genet (1998) 19(1):56–9. doi: 10.1038/ng0598-56
119. Nauta AJ, Trouw LA, Daha MR, Tijsma O, Nieuwland R, Schwaeble WJ, et al. Direct binding of C1q to apoptotic cells and cell blebs induces complement activation. Eur J Immunol (2002) 32(6):1726–36. doi: 10.1002/1521-4141(200206)32:6<1726::AID-IMMU1726>3.0.CO;2-R
120. Gasser O, Hess C, Miot S, Deon C, Sanchez JC, Schifferli JA. Characterisation and properties of ectosomes released by human polymorphonuclear neutrophils. Exp Cell Res (2003) 285(2):243–57. doi: 10.1016/S0014-4827(03)00055-7
121. Stegert M, Bock M, Trendelenburg M. Clinical presentation of human C1q deficiency: How much of a lupus? Mol Immunol (2015) 67(1):3–11. doi: 10.1016/j.molimm.2015.03.007
122. Merle NS, Noe R, Halbwachs-Mecarelli L, Fremeaux-Bacchi V, Roumenina LT. Complement system part II: Role in immunity. Front Immunol (2015) 6:257. doi: 10.3389/fimmu.2015.00257
123. Coulthard LG, Woodruff TM. Is the complement activation product C3a a proinflammatory molecule? re-evaluating the evidence and the myth. J Immunol (2015) 194(8):3542–8. doi: 10.4049/jimmunol.1403068
124. Antovic A, Mobarrez F, Manojlovic M, Soutari N, De Porta Baggemar V, Nordin A, et al. Microparticles expressing myeloperoxidase and complement C3a and C5a as markers of renal involvement in antineutrophil cytoplasmic antibody-associated vasculitis. J Rheumatol (2020) 47(5):714–21. doi: 10.3899/jrheum.181347
125. Ferreira V, Pangburn M, Cortés C. Complement control protein factor h: the good, the bad, and the inadequate. Mol Immunol (2010) 47(13):2187–97. doi: 10.1016/j.molimm.2010.05.007.Complement
126. Meri S, Haapasalo K. Function and dysfunction of complement factor h during formation of lipid-rich deposits. Front Immunol (2020) 11:611830(December). doi: 10.3389/fimmu.2020.611830
127. Trouw LA, Bengtsson AA, Gelderman KA, Dahlbäck B, Sturfelt G, Blom AM. C4b-binding protein and factor h compensate for the loss of membrane-bound complement inhibitors to protect apoptotic cells against excessive complement attack. J Biol Chem (2007) 282(39):28540–8. doi: 10.1074/jbc.M704354200
128. Martin M, Leffler J, Smoląg KI, Mytych J, Björk A, Chaves LD, et al. Factor H uptake regulates intracellular C3 activation during apoptosis and decreases the inflammatory potential of nucleosomes. Cell Death Differ (2016) 23(5):903–11. doi: 10.1038/cdd.2015.164
129. Skerka C, Chen Q, Fremeaux-Bacchi V, Roumenina LT. Complement factor h related proteins (CFHRs). Mol Immunol (2013) 56(3):170–80. doi: 10.1016/j.molimm.2013.06.001
130. Jozsi M, Tortajada A, Uzonyi B, Goicoechea de Jorge E, Rodriguez de Cordoba S. Factor h-related proteins determine complement-activating surfaces. Trends Immunol (2015) 36(6):374–84. doi: 10.1016/j.it.2015.04.008
131. Schäfer N, Grosche A, Reinders J, Hauck SM, Pouw RB, Kuijpers TW, et al. Complement regulator FHR-3 is elevated either locally or systemically in a selection of autoimmune diseases. Front Immunol (2016) 7:542(November). doi: 10.3389/fimmu.2016.00542
132. Kárpáti É, Papp A, Schneider AE, Hajnal D, Cserhalmi M, Csincsi ÁI, et al. Interaction of the factor h family proteins FHR-1 and FHR-5 with DNA and dead cells: Implications for the regulation of complement activation and opsonization. Front Immunol (2020) 11:1297(July). doi: 10.3389/fimmu.2020.01297
133. Nauta AJ, Daha MR, Van Kooten C, Roos A. Recognition and clearance of apoptotic cells: A role for complement and pentraxins. Trends Immunol (2003) 24(3):148–54. doi: 10.1016/S1471-4906(03)00030-9
134. Chen Y, Park YB, Patel E, Silverman GJ. IgM antibodies to apoptosis-associated determinants recruit C1q and enhance dendritic cell phagocytosis of apoptotic cells. J Immunol (2009) 182(10):6031–43. doi: 10.4049/jimmunol.0804191.IgM
135. Ogden CA, Kowalewski R, Peng Y, Montenegro V, Elkon KB. IGM is required for efficient complement mediated phagocytosis of apoptotic cells in vivo. Autoimmunity (2005) 38(4):259–64. doi: 10.1080/08916930500124452
136. Gershov D, Kim SJ, Brot N, Elkon KB. C-reactive protein binds to apoptotic cells, protects the cells from assembly of the terminal complement components, and sustains an antiinflammatory innate immune response: Implications for systemic autoimmunity. J Exp Med (2000) 192(9):1353–63. doi: 10.1084/jem.192.9.1353
137. Joseph K, Kulik L, Coughlin B, Kunchithapautham K, Bandyopadhyay M, Thiel S, et al. Oxidative stress sensitizes retinal pigmented epithelial (RPE) cells to complement-mediated injury in a natural antibody-, lectin pathway-, and phospholipid epitope-dependent manner. J Biol Chem (2013) 288(18):12753–65. doi: 10.1074/jbc.M112.421891
138. Laine M, Jarva H, Seitsonen S, Haapasalo K, Lehtinen MJ, Lindeman N, et al. Y402H polymorphism of complement factor h affects binding affinity to c-reactive protein. J Immunol (2007) 178(6):3831–6. doi: 10.4049/jimmunol.178.6.3831
139. Swinkels M, Zhang JH, Tilakaratna V, Black G, Mcharg S, Inforzato A, et al. C-reactive protein and pentraxin-3 binding of factor h-like protein 1 differs from complement factor H : implications for retinal inflammation. Sci Rep (2018) 8:1–12. doi: 10.1038/s41598-017-18395-7
140. Trouw LA, Bengtsson AA, Gelderman KA, Dahlbäck B, Sturfelt G, Blom AM. C4b-binding protein and factor h compensate for the loss of membrane-bound complement inhibitors to protect apoptotic cells against excessive complement attack. J Biol Chem (2007) 282(39):28540–8. doi: 10.1074/jbc.M704354200
141. Merle NS, Church SE, Fremeaux-Bacchi V, Roumenina LT. Complement system part I – molecular mechanisms of activation and regulation. Front Immunol (2015) 6:262(June). doi: 10.3389/fimmu.2015.00262
142. Csincsi ÁI, Szabó Z, Bánlaki Z, Uzonyi B, Cserhalmi M, Kárpáti É, et al. FHR-1 binds to c-reactive protein and enhances rather than inhibits complement activation. J Immunol (2017) 199(1):292–303. doi: 10.4049/jimmunol.1600483
143. Tsikas D. Assessment of lipid peroxidation by measuring malondialdehyde (MDA) and relatives in biological samples: Analytical and biological challenges. Anal Biochem (2017) 524:13–30. doi: 10.1016/j.ab.2016.10.021
144. Briley-Saebo K, Yeang C, Witztum JL, Tsimikas S. Imaging of oxidation-specific epitopes with targeted nanoparticles to detect high-risk atherosclerotic lesions: Progress and future directions. J Cardiovasc Transl Res (2014) 7(8):719–36. doi: 10.1007/s12265-014-9590-4
145. Ravandi A, Boekholdt SM, Mallat Z, Talmud PJ, Kastelein JJP, Wareham NJ, et al. Relationship of IgG and IgM autoantibodies and immune complexes to oxidized LDL with markers of oxidation and inflammation and cardiovascular events: results from the EPIC-Norfolk study. J Lipid Res (2011) 52:1829–36. doi: 10.1194/jlr.M015776
146. Gounopoulos P, Merki E, Hansen LF, Choi SH, Tsimikas S. Antibodies to oxidized low density lipoprotein: Epidemiological studies and potential clinical applications in cardiovascular disease. Minerva Cardioangiol (2007) 55(6):821–37.
147. Atik N, Putri Pratiwi S, Hamijoyo L. Correlation between c-reactive protein with malondialdehyde in systemic lupus erythematosus patients. Int J Rheumatol (2020) 2020:1–5. doi: 10.1155/2020/8078412
148. Botto M, Walport MJ. C1q, autoimmunity and apoptosis. Immunobiology (2002) 205(4–5):395–406. doi: 10.1078/0171-2985-00141
149. Svenungsson E, Jensen-Urstad K, Heimburger M, Silveira A, Hamsten A, de Faire U, et al. Risk factors for cardiovascular disease in systemic lupus erythematosus. Circulation (2001) 104(16):1887–93. doi: 10.1161/hc4101.097518
150. Wu R, Svenungsson E, Gunnarsson I, Haegerstrand-Gillis C, Andersson B, Lundberg I, et al. Antibodies to adult human endothelial cells cross-react with oxidized low-density lipoprotein and beta 2-glycoprotein I (beta 2-GPI) in systemic lupus erythematosus. Clin Exp Immunol (1999) 115:561–6. doi: 10.1046/j.1365-2249.1999.00830.x
151. Thiagarajan D, Oparina N, Lundström S, Zubarev R, Sun J, Beretta L, et al. IgM antibodies against malondialdehyde and phosphorylcholine in different systemic rheumatic diseases. Sci Rep (2020) 10(1):1–13. doi: 10.1038/s41598-020-66981-z
152. Hughes AE, Orr N, Esfandiary H, Diaz-Torres M, Goodship T, Chakravarthy U. A common CFH haplotype, with deletion of CFHR1 and CFHR3, is associated with lower risk of age-related macular degeneration. Nat Genet (2006) 38(10):1173–7. doi: 10.1038/ng1890
153. Hageman GS, Hancox LS, Taiber AJ, Gehrs KM, Anderson DH, Johnson LV, et al. Extended haplotypes in the complement factor h (CFH) and CFH-related (CFHR) family of genes protect against age-related macular degeneration: characterization, ethnic distribution and evolutionary implications. Ann Med (2006) 38(8):592–604. doi: 10.1080/07853890601097030
154. Kiss MG, Binder CJ. The multifaceted impact of complement on atherosclerosis. Atherosclerosis (2022) 351:29–40. doi: 10.1016/j.atherosclerosis.2022.03.014
155. Kardys I, Klaver CCW, Despriet DDG, Bergen AAB, Uitterlinden AG, Hofman A, et al. A common polymorphism in the complement factor h gene is associated with increased risk of myocardial infarction. Rotterdam Study J Am Coll Cardiol (2006) 47(8):1568–75. doi: 10.1016/j.jacc.2005.11.076
Keywords: oxidation-specific epitopes, oxidative stress, DAMPs (damage-associated molecular patterns), complement - immunological terms, natural antibodies (NAbs), pentraxins, immune recognition
Citation: Alic L, Binder CJ and Papac-Milicevic N (2022) The OSE complotype and its clinical potential. Front. Immunol. 13:1010893. doi: 10.3389/fimmu.2022.1010893
Received: 03 August 2022; Accepted: 12 September 2022;
Published: 30 September 2022.
Edited by:
Per Nilsson, Linnaeus University, SwedenReviewed by:
Xuchu Que, University of California, San Diego, United StatesCopyright © 2022 Alic, Binder and Papac-Milicevic. This is an open-access article distributed under the terms of the Creative Commons Attribution License (CC BY). The use, distribution or reproduction in other forums is permitted, provided the original author(s) and the copyright owner(s) are credited and that the original publication in this journal is cited, in accordance with accepted academic practice. No use, distribution or reproduction is permitted which does not comply with these terms.
*Correspondence: Nikolina Papac-Milicevic, bmlrb2xpbmEucGFwYWNAbWVkdW5pd2llbi5hYy5hdA==