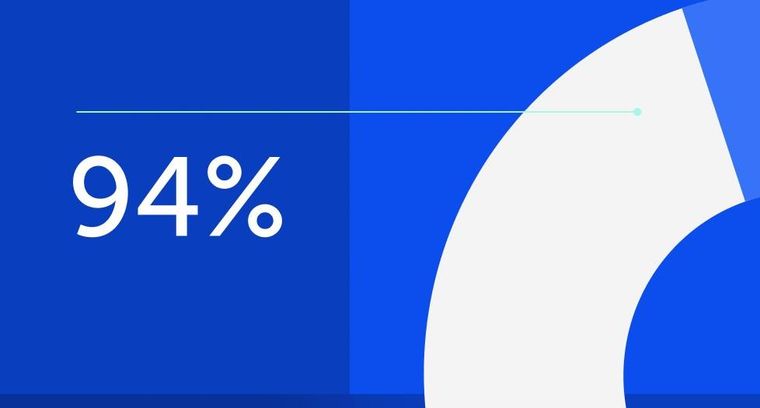
94% of researchers rate our articles as excellent or good
Learn more about the work of our research integrity team to safeguard the quality of each article we publish.
Find out more
SYSTEMATIC REVIEW article
Front. Immunol., 18 October 2022
Sec. Molecular Innate Immunity
Volume 13 - 2022 | https://doi.org/10.3389/fimmu.2022.1007102
This article is part of the Research TopicCurrent Challenges in Complement DiagnosticsView all 27 articles
Background: The complement system is an essential component of our innate defense and plays a vital role in the pathogenesis of many diseases. Assessment of complement activation is critical in monitoring both disease progression and response to therapy. Complement analysis requires accurate and standardized sampling and assay procedures, which has proven to be challenging.
Objective: We performed a systematic analysis of the current methods used to assess complement components and reviewed whether the identified studies performed their complement measurements according to the recommended practice regarding pre-analytical sample handling and assay technique. Results are supplemented with own data regarding the assessment of key complement biomarkers to illustrate the importance of accurate sampling and measuring of complement components.
Methods: A literature search using the Pubmed/MEDLINE database was performed focusing on studies measuring the key complement components C3, C5 and/or their split products and/or the soluble variant of the terminal C5b-9 complement complex (sTCC) in human blood samples that were published between February 2017 and February 2022. The identified studies were reviewed whether they had used the correct sample type and techniques for their analyses.
Results: A total of 92 out of 376 studies were selected for full-text analysis. Forty-five studies (49%) were identified as using the correct sample type and techniques for their complement analyses, while 25 studies (27%) did not use the correct sample type or technique. For 22 studies (24%), it was not specified which sample type was used.
Conclusion: A substantial part of the reviewed studies did not use the appropriate sample type for assessing complement activation or did not mention which sample type was used. This deviation from the standardized procedure can lead to misinterpretation of complement biomarker levels and hampers proper comparison of complement measurements between studies. Therefore, this study underlines the necessity of general guidelines for accurate and standardized complement analysis
Complement is a key innate immune system and is part of the first line of defense against pathogens. It is recognized as being evolutionary among the oldest pathogen recognition systems, and not only has this system a critical role in killing microbes, it also bridges innate and adaptive immune responses. The realization that an increasing number of human diseases are, at least partly, complement-mediated has driven a renewed interest in complement. Accurate analysis of complement components and complement activation is of utmost importance for diagnosing disease and/or therapy monitoring (1–5). However, reliably determining the complement status of individual patients has proven to be challenging (1, 5). Measured concentrations of complement system proteins vary widely among different laboratories due to non-unified protocols for pre-analytical sample handling, including collection, processing, and storage. Also, laboratories do not always use the correct sample type for the method or assay used. Other reasons for inconsistencies in concentrations are the lack of uniform and recognized calibrators, the use of different techniques, reagents, and antibodies recognizing different epitopes in the antigen. This indicates the high need for standardization of techniques, assays and biomaterials in combination with proper pre-analytical sample handling and storage (2, 3).
This review aimed to provide an overview and general guidelines for measuring complement activation in a reliable and standardized way. We performed a systematic analysis of the literature regarding current methods used to assess complement C3, C5 and/or their split products and/or the soluble terminal complement complex (sTCC; also referred to as sC5b-9 or membrane attack complex, MAC) in human blood. We reviewed whether the identified studies performed their complement measurements according to the recommended practice regarding pre-analytical sample handling and assay technique. Results were supplemented with own data, not included in the systematic review, to illustrate the importance of using the correct biomaterials, methods, and techniques for the assessment of complement components.
The complement system comprises of approximately 50 soluble and cell surface-bound proteins organized to eliminate structures recognized as dangerous. These danger signals include invading microorganisms, apoptotic and necrotic cells and immune complexes (6, 7). Furthermore, complement can link innate and adaptive immune responses by mediating regulation of T cell and B cell responses (8). More recently, several studies reported a role for intracellular complement (9–11).
The complement pathway is tightly regulated by a network of proteins to avoid uncontrolled activation (Figure 1) (12). The system is organized in three activation pathways: the classical pathway (CP), the lectin pathway (LP) and the alternative pathway (AP). Each pathway is activated by different molecules. The CP is activated by the binding of C1q to antigen-bound-IgM or -IgG hexamers forming immune complexes. The LP is activated by binding of one of the recognition molecules mannose-binding lectin (MBL), collectin 10, 11 or 12, or ficolin-1, -2, or -3 to microorganism-associated molecular patterns (MAMPs) or carbohydrate structures located on damaged cells. The AP is continuously activated at a low level as a result of spontaneous C3 hydrolysis allowing for activation on surfaces that lack proper complement regulation, typically non-host surfaces (12–15). Pathway activation results in the cleavage and activation of complement C3 and C5. Subsequently, this will lead to the generation of active fragments such as C3a, C3b, iC3b, C3dg, C4a, C4b and C5a and the formation of TCC (also known as MAC or C5b-9) (1). While TCC is inserted into pathogen cell membranes, resulting in cell death of sensitive cells, typically Gram-negative bacteria, the other activation fragments bind to their corresponding complement receptor expressed by various cell types. Binding of activation products to these receptors leads to a variety of biological responses such as phagocytosis, cell migration, chemotaxis, cytokine production, cell activation and modulation of pattern recognition receptor (PRR)-induced responses (Figure 1) (12, 13, 16–18).
Inadequate functioning of the complement system, e.g. by component deficiencies or exaggerated activation, has been linked to numerous diseases, both rare and common, and the list is growing continuously (Table 1) (13, 72–75). An imbalance in complement activation and regulation can lead to life-threatening conditions such as coagulation dysregulation, systemic inflammation and failure of organs such as the eyes, kidneys, skin, brain and vascular system (75–77). Because of the large number of complement-mediated diseases, together with recent results from large-scale genomics and proteomic studies (78, 79), the interest in the complement system has renewed, especially as a promising target for therapeutic intervention. This was demonstrated more than a decade ago by the first complement-specific drug, eculizumab (Soliris, Alexion), targeting the key complement component C5 (29). In recent years, human complement studies have become very important in drug delivery, nanomedicine and biomaterial fields, as elegantly summarized by Moghimi and coworkers (80, 81). Interestingly, assessment of complement within these fields is often performed using incorrect or suboptimal assays and samples that were inappropriate handled regarding collection, storage and pre-analytical preparations (80). As complement is involved in an increasing number of human diseases, standardized measurement of complement components is essential (1–5, 82). However, accurate determination of the complement status of individual patients has proven still to be challenging and needs to be performed in a uniform way.
To provide insight into the current practice in complement measurements at individual labs, we conducted a systematic literature search in which we reviewed which sample type and techniques were used for the assessment of complement activation in the past five years. The studies were assessed for their quality based on the recommended guidelines for studying complement activation as described below.
For accurate complement analysis, it is important that the complement activation is blocked at blood sampling since activation ex-vivo during sample processing may alter the results of interest (2, 12). Hence, appropriate pre-analytical sample handling is a necessity to avoid erroneous results. For complement analysis, samples may be assessed either for complement function or quantification of individual complement components (2, 3, 83). Both types of analysis require a specific approach regarding the sample type, pre-analytical sample handing and measurements.
For assessing the ability of complement activation, serum samples should be used (84). Whole blood should be collected in serum tubes. These collection tubes are available either with or without a clot activator (e.g. silica). Note that some complement analyses may be affected by the type of collection tube, e.g. ficolin 2 is depleted in serum retrieved from tubes with silica, which should especially be taken into account when assessing the lectin pathway (80, 85, 86). Let blood coagulate for approximately 30 min at RT. Please be aware that blood coagulation kinetics are slightly slower in tubes without a clot activator, so ensure complete clot retraction. Optimize if needed. For both type of serum collection tubes, separate the serum fraction by centrifugation (2000xg, 10 min) at 4°C. Process serum within 1 h. Complement activation is temperature-dependent so always keep serum on ice to avoid ex-vivo complement activation (12, 80, 81, 87, 88). Store serum samples at -80°C since storage at higher temperatures such as -20°C will create a slow freezing rate that allows for complement activation. Mechanical stress (rigorous shaking or vortexing) and repeated cycles of freezing and thawing should be minimized as much as possible since every cycle may increase complement activation (2, 84) (Figure 2A). Next to serum, also Hirudin-plasma (or Lepirudin; recombinant Hirudin) can be used for the assessment of complement function as Hirudin blocks coagulation but allows complement activation (when used in low concentrations: 0.2 IU/mL and 2 IU/mL) (89). As complement is also activated by a low pH (<7.1), it is advised to monitor and control it (with appropriate buffers) to avoid erroneous measurements (90).
Figure 2 Flowchart for the selection of the appropriate sample type and technique for complement analysis. Flowchart for selection of (A) the appropriate sample type or (B) the appropriate technique for the assessment of complement.
For studying complement function, it is of utmost importance that the appropriate buffers are used. For assessing CP and LP activation, buffers containing both Ca2+ and Mg2+ should be used. These cations are required in order to form protein complexes which initiate or mediate progression of complement activation. Ca2+ is essential for the activation of CP and LP protease complexes that cleave C4 and C2 (91, 92), whereas Mg2+ is needed for the binding of C2 to C4b to form CP/LP C3 convertases (93). For studying AP activation, CP and LP activity must be blocked by adding ethylene glycol tetraacetic acid (EGTA) to chelate Ca2+ (Figure 1). Additionally, an optimal concentration of Mg2+ is required since the binding of Factor B to C3b to form the AP C3 convertase is Mg2+-dependent (3, 94). Traditional complement buffers like GVB++ (gelatin veronal buffer) contain 0.15 mM Ca2+ and 0.5 mM Mg2+. Hirudin or lepirudin do not interfere with divalent cations.
For assessing complement function, several techniques and assays are available (Figure 2B). Traditionally, complement function of the CP and AP was assessed using respectively the CH50 and AH50 hemolytic assays. These assays detect reduction, absence and/or inactivity of several components of the complement pathway. The CH50 is based on the capacity of serum to activate complement in-vitro and subsequently lyse sheep erythrocytes opsonized with anti-sheep antibodies (usually rabbit IgG) via the CP. The AH50 is based on complement-dependent lysis of rabbit erythrocytes, which cannot recruit the soluble human complement regulator Factor H, thereby allowing for AP activation (95). If one or more complement components are absent (e.g. due to a genetic deficiency) or their levels decreased (e.g. due to overactivation leading to consumption), hemolytic activity is decreased. When both assays are performed side-by-side, they can indicate C3 and terminal pathway (TP) deficiencies (C5, C6, C7, C8, and C9; absence of hemolysis in both CH50 and AH50), CP deficiencies (C1, C2, C4; absence of lysis in CH50 only) and AP deficiencies (Factor B, D, H, I, properdin; absence of lysis in AH50 only). Factor H and Factor I deficiency can also result in C3 consumption as a result of uncontrolled AP activation, leading to low CH50.
Complement function can also be assessed using functionalized enzyme-linked immunosorbent assays (ELISA), which are considered more accurate and reproducible and are less prone to variation. This type of assays uses plates coated with either IgM, mannose or lipopolysaccharides (LPS) to examine CP, LP or AP activation respectively. In the presence of the appropriate buffers, complement is activated in the patient’s serum sample (at 37°C). Next, pathway activation is assessed by the detection of C5b-9 in the terminal pathway using a specific conjugate antibody directed to a neo-epitope in C5b-9 (12, 96, 97). These assays require a relatively high sample dilution to prevent cross-pathway interference. However, using a sample dilution that is too high might lead to false negative results. When concentrations for critical complement components needed for complement activation are too low, the pathway will not be activated. As the window for the correct sample dilution is rather small, it is easily missed. Therefore, this high sample dilution may be considered as a drawback when these types of assays are used.
A third type of assay for assessing complement function is the liposome immunoassay. This type of assay consists of liposomes coated with antigens (for instance with dinitrophenyl (DNP)) and containing a reporter molecule (signal-producing molecules). When serum is mixed with the liposomes and an antibody-containing reagent (in this case anti-DNP), the formed immune complex will trigger complement activation resulting in disruption of the liposomes and release of the entrapped reporter molecule from the lysed vesicles. The degree of reporter release corresponds to the degree of complement activation and can easily be measured (98, 99).
For analysis of individual complement components, EDTA-plasma should be used having at least a final concentration of 10 mM (82, 100), especially when measuring activation products. Using EDTA-plasma, any further activation of complement is minimized (but not completely excluded) since EDTA chelates Ca2+ and Mg2+ thereby blocking the function of the C1 complex and the C3 convertases (4, 83, 100–102). Of note, nafamostat mesylate (0.2 mg/mL) can be used in addition to EDTA to maximize inhibition of artificial complement activation (100). Heparin-plasma should be avoided as heparin interacts with many complement proteins and regulators such as C1, C1q, C1-INH, C2, C4, C4b, C4BP, MASP-1, MASP-2, C3, C3b, FB, FD, FH, properdin, C6, C8, C9 and vitronectin, thereby affecting complement function (84, 103). Low levels of heparin (<2 IU/mL) can activate complement whereas high levels (20 IU/mL) inhibit complement activation (89). If possible, we recommend to also avoid citrate-plasma for the assessment of complement components. Although differences in concentrations for markers such as C4d, Bb, C3a, C5a and sTCC are very small between freshly processed EDTA- and citrate-plasma samples, these markers are more stable in EDTA-plasma when stored longer at either RT or 4°C before processing. Citrate-plasma also tends to be more sensitive for freeze-thawing (83, 104). Serum cannot be used for the assessment of individual complement components, especially activation products. Due to the absence of complement inhibitors, artificial complement activation during collection, shipment, processing and/or pre-analytical sample handling will cause an increase of many complement components making reliable determination of these markers impossible (83, 104).
EDTA-whole blood should be kept on ice and centrifugated at 4°C within 60 minutes after collection. Keep transportation and logistics of samples from the patients to the lab as short as possible and handle samples with care (keep on ice, minimize mechanical stress). Isolated EDTA-plasma should be kept on ice and as soon as possible snap-frozen and placed in -80°C. Shipment of EDTA-plasma for complement analysis should always be done on dry ice. EDTA-plasma for complement marker analysis should be thawed and kept on ice and repeated freeze-thawing should be avoided. Different markers have various sensitivity to freeze-thawing (100). Note that EDTA-plasma should not be used for functional complement testing in the assays described above as these assays detect in-vitro complement activation which is inhibited by EDTA (1) (Figure 2A).
Several techniques for analysis of complement components are available (Figure 2B). In most clinical laboratories, analysis of individual complement components such as C3, C4 and sometimes C5 is performed by nephelometry or turbidimetry. These techniques are widely used in clinical laboratories because they are relatively easily automated and inexpensive. However, a major drawback of nephelometry and turbidimetry is that it cannot distinguish between the native non-activated complement protein and its activated split products. For instance, for C3 it will detect both the intact non-activated C3 and its activated proteolytic fragments C3a, C3b, iC3b, C3c and/or C3d (105). Another drawback of is that these methods use a relatively large sample volume. This is especially a challenge for complement analysis in young children, of whom often only small amounts of sample is available.
ELISA is a technique also widely used for quantification of individual complement components, its activated split products and complement regulators. When using well-defined antibodies, ELISA can quantify complement-derived split products next to the native intact protein, reflecting the actual state of complement activation (2, 3, 105). For measuring the specific complement activation products antibodies recognizing split-product-specific neoepitopes, i.e. neoepitopes that only become exposed after cleavage of the native protein, should be used. In addition, detection of split products generated via complement-independent bypass routes is possible (106).
Next to ELISA, a more recent technique being developed for the quantification of individual complement components and their split products is (in most cases bead-based) multiplex assays. Such assay is, in principle, a combination of immunoassays thereby able to analyze multiple analytes at the same time. Next to the advantages of saving time and sample volume, these assays provide a comprehensive overview of the patient’s complement activation status in a single run (107). Currently, the specificity of these commercial bead based multiplex complement assays is still under debate (107, 108) which argues thorough validation of its use in comparison to existing assay formats.
Our systematic review procedure was adopted from the Preferred Reporting Items for Systematic Reviews and Meta-Analyses (PRISMA) guidelines (109). Structured literature searches using the Pubmed/MEDLINE database were performed in February 2022. Search terms included the medical subject headings (MeSH) terms for: “Complement C3” OR “Complement C3a” OR “Complement C5” OR “Complement C5a” OR “Complement Membrane Attack Complex” AND “Complement activation” to retrieve all studies assessing these complement components. Supplementary Dataset 1 lists all keywords captured by the MeSH terms used. Next, titles and abstracts were screened by two independent researchers (ET and RB) to determine if the study met the inclusion criteria (see below). Studies potentially of interest were selected and full text papers were retrieved for in-depth review.
All studies that assessed the complement components C3, C5 and/or their split products C3a and C5a and/or sTCC (MAC, sC5b-9) in human blood samples (serum and plasma) that were published in peer-reviewed journals between February 2017 and February 2022 were included.
The following studies were excluded: not assessing complement components C3, C5 and/or their split products and/or sTCC in human blood samples, animal studies, ex-vivo stimulation studies, reviews or editorials, studies in languages other than English and no full text available. The selection process was performed by two authors (ET and RB), based on titles, abstracts, and subsequently full text papers.
Subsequently, studies that passed the inclusion criteria were categorized according to sample type and techniques used for complement assessment. Studies that were identified as using the correct sample type for their complement measurements (according to the guidelines addressed above and in Figure 2) were scored regarding overall quality standards for performing research, using the following criteria (110):
1. Did the study provide sufficient information regarding the experimental design and methods (e.g. appropriate method/approach to investigate study aim, clear which analyses were performed in which experimental groups/samples)?
2. Were the baseline characteristics sex and age checked and matched in the control cohorts used for the study?
3. Was sample collection, storage and handling performed according to the requirements for assessment of complement components as outlined in this paper?
4. Were measurements performed in a blinded fashion?
Using these criteria, the nominated studies were awarded a score ranging from 0 to 4, 1 point per met criterion.
Three experiments were performed to illustrate the importance of correct pre-analytical sample handling and complement assessment.
In the first experiment, we tested the effect of artificial complement activation in the tube induced by inappropriate pre-analytical sample handling. The components C3c and sTCC were measured in complement-preserved serum samples (n=5) and in plasma samples anticoagulated either with citrate, heparin, EDTA 10 mM (normal EDTA blood collection tube, no extra EDTA added) and EDTA ≥20 mM (normal EDTA blood collection tube with extra EDTA added) (n=5 for each anticoagulant). All samples used for this experiment were derived from healthy controls (HC). Plasma samples were put on ice immediately after collection and processed within 1 h (centrifuged for 10 min., 2000xg at 4°C and stored at -80°C until further use). Complement-preserved serum samples from were collected aseptically and processed within 1 h (clotted at room RT 30-45 min., centrifuged for 10 min., 2000xg at 4°C and stored at -80°C until further use). Aliquots for all samples were thawed and incubated either at RT or on ice for 10 min, 1 h, 2 h and 16 h before measurements. Subsequently, C3c and sTCC levels were measured by commercially available solid-phase ELISA assays according to the manufacturer’s instructions (Cat# HK368 (C3c), HK328 (sTCC), Hycult Biotech, Uden, The Netherlands). Assays are specific for these markers and do not detect other activation products (e.g. C3b, iC3b, C3d) or components not integrated in the TCC. The levels measured in the samples incubated for 10 min were used as a baseline and were set to 100%. All other timepoints were compared to baseline.
Secondly, we assessed C3 concentrations in EDTA-plasma derived from HC (n=6) using two in-house assay designs. These assays did not differ from each other, except for the capture antibody to test the effect of (differences in) antigenicity between antibodies. Samples were collected and handled as described above. Samples were thawed on ice and kept on ice during pre-analytical sample handling. Two distinct capture antibodies were used, recognizing a different epitope in the C3 protein, i.e., the monoclonal antibody clone 3E7 (cat# HM2286) versus the monoclonal antibody 1H8 (cat# HM2287; Hycult biotech, Uden, The Netherlands). Antibody concentrations and all other reagents and buffers (HK355; Hycult biotech, Uden, The Netherlands), as well as the calibrator (human native C3; cat#113, Complement Technology, TX, USA) were the same between the two assay designs and assays were performed by the same operator on the same day. C3 concentrations were measured using both setups in parallel according to manufacturer instructions. Briefly, microtiter wells (Nunc maxisorp cat# 468667, Thermo Fisher Scientific, Waltham, MA, USA) were coated either with clone 3E7 or 1H8 at 4°C overnight (5 µg/ml) in 1x PBS. After blocking with 1x PBS, 1% BSA for 1 – 1.5 hours, wells were washed four times using 1x PBS, 0.05% Tween 20. Calibrator was added to the wells in a 2x serial dilution ranging from 500 to 7.8 ng/ml in dilution buffer (DB; 1x PBS, 0.1% BSA). EDTA-plasma samples were added to the wells in a serial dilution ranging from 1:500 to 1:64000, also in DB. Both calibrator and samples were incubated 1 h at RT. For both assays the same detection antibody was used (monoclonal antibody clone 474; cat# HM2073, biotinylated; Hycult biotech, Uden, The Netherlands). After washing, wells were incubated with detection antibody (0.1 µg/ml in DB) for 1 h at RT. Again, wells were washed and tetramethylbenzidine substrate (TMB; K-blue aqueous; Neogen, Scotland, UK) was added starting an enzymatic reaction that produced a colored product that can be measured. Reaction was stopped after 30 min. by adding oxalic acid and the absorbance at 450 nm was measured.
The third experiment focused on choosing the appropriate marker, accompanied by the correct technique and the added value of quantifying both native complement proteins and their split products in parallel in patients with a severe acute respiratory syndrome coronavirus-2 (SARS-CoV-2) infection. Results were adapted from a previously reported study (66). The complement markers C3, C3a, C3c, C5 and sTCC were assessed in EDTA-plasma samples from patients with PCR-proven or clinically presumed Corona Virus Disease 2019 (COVID-19) admitted to the intensive care unit (ICU) and in HC. Also here, EDTA-plasma samples were collected, processed, and handled as described above. Complement measurements were performed using commercially available solid-phase ELISA assays according to the manufacturer’s instructions [Cat# HK355 (C3), HK354 (C3a), HK368 (C3c), HK390 (C5), HK328 (TCC), Hycult Biotech, Uden, The Netherlands].
Continuous data were presented as the mean ± SEM following criteria for normal distribution. Data were analyzed using a (paired) student t-test, ANOVA or Mann-Whitney test where appropriate (Graphpad Prism 8.4.2, San Diego, CA, USA). A p-value <0.05 was considered significant.
The study protocol was approved by the local ethics committee (CMO 2020 6344 and CMO 2016 2963) and performed in accordance with the latest version of the declaration of Helsinki and guidelines for good clinical practice (GCP).
A systematic literature review was conducted to provide a comprehensive overview of the methods and sample types used for the assessment of complement markers during the period February 2017 – February 2022. Subsequently, we assessed whether the identified studies performed their complement analyses in a reliable manner regarding techniques and sample types. We focused on the key complement markers C3, C5 and their split products C3a and C5a, and sTCC.
In total, 441 records were identified through database searching using the indicated MeSH terms (Figure 3). After excluding non-English papers, animal studies, reviews/editorials and records of which no full text was available, 376 records remained (Supplementary Dataset 2). These remaining abstracts were screened based on whether levels of the complement components C3, C5 and/or their split products and/or TCC were assessed in human blood. Studies performing ex-vivo stimulation experiments, assessing complement deposition in tissues or using cultured cells were excluded. A total of 92 articles were selected for full text analysis. Study characteristics such as aim of the study, experimental set-up and used techniques were recorded (Supplementary Table 1).
Regarding the techniques used for measuring complement components in these 92 studies, 69 studies used ELISA (75%), 12 studies (13%) used turbidimetry/nephelometry and three studies (3%) used mass spectrometry. Nine studies (10%) used other techniques and 11 studies (12%) did not specify the technique that was used (Figure 4). Several studies used more than one technique for their measurements, hence the number of performed analyses is higher than the total number of the identified studies.
Figure 4 Pie charts showing the percentages regarding the used techniques and whether the correct sample types were used for complement assessment. A total of 92 studies were included in the analysis. (A) Percentages regarding the used techniques. Several studies used more than 1 technique for their measurements, hence the number of performed analyses is higher than the total number of the identified studies. (B) Percentages of studies that used/did not used the correct sample type for complement assessment.
These 92 identified studies were assessed on whether complement measurements were performed correctly and reliably concerning sample type, pre-analytical sample handling and techniques with regard to the complement components being investigated. This resulted in the identification of three distinct groups (Figure 4; Supplementary Table 1):
1. Studies identified as using the correct sample type for assessing complement activation products (mostly EDTA-plasma) (n=45; 49%) (Table 2).
2. Studies identified as not using the correct sample type (mostly serum) (n=25; 27%).
3. Studies that did not specify the sample matrix or the techniques used for measuring complement (n=22; 24%).
Table 2 Overview of the 45 selected studies that assessed complement components ex-vivo in the past 5 years (2017-2022).
For the 45 studies identified as using the correct sample type (mostly EDTA-plasma), ELISA was the method of choice (n=43; 96%), sometimes supplemented with other techniques such as nephelometry or rocket immuno-electrophoresis (n=6; 7%). In this first group, roughly two categories could be identified: studies investigating complement in health and disease (n=39; 87%) and studies with a technical aim such as development or improvement of new tools and assays (n=6; 13%). Regarding studies investigating complement in health and disease, 31 studies assessed complement markers in patients compared to controls in a wide range of infectious, autoimmune, inflammatory and inflammatory-associated diseases or conditions (66, 111–140). A total of 8 studies focused on complement regulation and activation after treatment in patients (141–148). Regarding the technical studies, 3 studies described the development of new tools or assays (149–151) and 3 studies focused on the development/improvement of new methods or procedures (152–154) (Table 2). Next, these 45 studies were scored regarding overall quality standards according to the criteria listed in the methods section. This resulted in a total score ranging from 0 to 4. All studies but one (score=2) reached a good score of 3, indicating that all the criteria were met except that analyses were not performed in a blinded fashion or that this was not mentioned (Table 2).
A total of 25 studies (27%) were identified as not using the correct sample type for the complement measurements (Figure 4; Supplementary Table 1). Most of these studies used serum samples for measuring individual complement components and their split products. This group also showed a larger variety in techniques used for complement analysis when compared to the group using the correct sample type. ELISA was used in 13 studies (52%), turbidimetry/nephelometry in 7 studies (28%), mass spectrometry in 2 studies (8%) and 5 studies (20%) used other techniques. A total of 5 studies (20%) did not specify the used techniques. Once again, several studies used more than one technique for their measurements, hence the number of performed analyses is higher than the total number of the identified studies.
The last group of 22 studies (24%) did not specify which sample type was used for their measurements. For 6 out of these 22 studies (27%), it is unclear whether serum or plasma was used. The remaining 16 studies (73%) used plasma samples for their measurements but did not specify which anticoagulant tubes were used. Regarding the techniques, 12 studies (55%) reported using ELISA, one study (5%), mass spectrometry and one study (5%) nephelometry. For the remaining eight studies (36%), it is unclear which technology was applied. In addition, little information was provided regarding pre-analytical sample handling and storage (Figure 4; Supplementary Table 1).
Overall, from the 92 selected studies for in-depth analyses, 45 studies (49%) were identified as using the correct sample type for their complement measurements. A total of 47 studies (51%) did not use the correct sample type or it was not clear which sample type was used.
To demonstrate the effect of artificial complement activation in the tube induced by inappropriate pre-analytical sample handling, we measured C3c and sTCC concentrations in complement-preserved serum samples and in plasma samples anticoagulated either with citrate, heparin, EDTA 10 mM and EDTA ≥20 mM. Samples were incubated at RT or on ice for 10 min, 1 h, 2 h and 16 h before measurements. C3c concentrations measured in all plasma samples at RT did not significantly differ from baseline concentrations (10 min) when measured after 1h and 2h. However, both C3c concentrations and the degree of variation between samples increased when plasma samples were measured after 16 h at RT (but this difference did not reach statistical significance due to large variation and low number of samples) (Figure 5A). In contrast, a strong increase in C3c concentrations was observed in complement-preserved serum measured after 1 h and 2 h at RT when compared to baseline and the difference reached statistical significance after 2 h (36342 ± 2685 ng/ml versus 15201 ± 2760 ng/ml; p=0.0005). Relative C3c values in serum increased 2.0 to 5.4-fold after 16 h when compared to baseline (Figure 5A). When plasma samples were kept on ice, no differences in C3c concentrations or much variation between samples was observed after 1 h, 2 h and 16 h (Figure 5B). Also for complement-preserved serum on ice, C3c concentrations did not differ at 1 h and 2 h but after 16 h relative C3c values ranged from 0.69 (decrease) to 4.3-fold when compared to baseline (Figure 5B). For sTCC, levels show the same pattern as observed for C3c. For plasma samples, sTCC concentrations at RT did not differ at 1 h and 2 h but after 16 h, both concentrations and degree of variation between samples increased (again this increase did not reach statistical significance due to large variation and low number of samples) (Figure 5C). Of note, sTCC concentrations in complement-preserved serum samples showed a different pattern as observed for C3c. Concentrations tended to be lower at 1 h, 2 h and 16 h at RT but this was not statistically significant (Figure 5C). When samples were kept on ice, all plasma samples showed no differences in sTCC concentrations at 1 h, 2 h and 16 h when compared to baseline (Figure 5D). Concentrations of sTCC in complement-preserved serum samples on ice tended to fluctuate between timepoints but this did not reach statistical significance (Figure 5D). Overall, EDTA-plasma samples showed most stable results regarding C3c and sTCC levels overtime for both RT and on ice. In addition, less variation in concentrations was observed between samples anticoagulated with 20 mM EDTA when compared to 10 mM EDTA (Figures 5A–D).
Figure 5 Benchtop stability of the components C3c and soluble TCC in serum samples and in plasma samples with different anticoagulants. C3c and sTCC levels were measured in complement-preserved serum samples (n=5) and in plasma samples anticoagulated either with citrate, heparin, EDTA 10 mM and EDTA ≥20 mM (n=5 for each anticoagulant). All samples were thawed and incubated at RT or on ice for 10 min, 1h, 2h and 16h before measurements. (A) C3c levels measured after incubation at RT and (B) on ice. (C) sTCC levels measured after incubation at RT and (D) on ice. Samples were analyzed using a serial dilution ranging from 1:50 to 1:400 and data are represented as mean ± SEM. Deviation from baseline ≤10% is highlighted in grey.
To illustrate the effect of antibodies that differ in antigenicity, we quantified C3 concentrations in EDTA-plasma samples from healthy controls using two ELISA setups capturing C3 at two different epitopes (Figures 6A, B). Mean C3 values were 2.7-fold higher in the assay in which HM2287 was used as capturing antibody compared to the assay in which HM2286 was used (1223 ± 120.9 µg/ml versus 449 ± 28.6 µg/ml; p<0.0001) (Figure 6B).
Figure 6 C3 EDTA-plasma concentrations in healthy individuals measured using two different assay designs. EDTA-plasma samples were analyzed in duplicates and data are represented as (A) individual values and (B) mean ± SEM.
To demonstrate that choosing the correct technique/approach for assessing complement activation is of major importance for correct interpretation of results, we quantified C3, C3a, C3c, C5 and sTCC using ELISA in COVID-19 patients admitted to the ICU. This technique was chosen because of its ability to quantify and distinguish between native complement proteins and their complement-activated split products. Results show that the EDTA-plasma concentrations for the split/activation products C3a, C3c and sTCC were significantly (p<0.0001) increased in ICU patients when compared to HC. In contrast, C3 and C5 concentrations did not differ between these two groups (Figure 7) (66).
Figure 7 C3 and C5 consumption in COVID-19 patients. EDTA-plasma sample values for the markers C3, C3a, C3c, C5 and soluble TCC. Samples were analyzed using a serial dilution ranging from 1:500 to 1:64000 and data are represented as individual values and mean ± SEM. NS, non-significant; HC, healthy volunteers; ICU, intensive care unit; COVID-19, Corona Virus Disease 2019.
In this review, we systematically reviewed the available literature in the period 2017-2022 regarding measurements for C3, C5 and/or their split products and/or sTCC in human blood samples and whether these measurements were performed correctly and reliably concerning sample type, pre-analytical sample handling and used techniques and assays.
Out of 376 records, a total of 92 studies were included. From those 92 studies, 45 studies (49%) were identified as using the correct sample type for their measurements. Using our scoring method to assess the overall quality of those 45 studies, most studies showed a total score of 3, indicating good quality. However, it is remarkable that performing a study in a blinded fashion is not standard procedure or is not mentioned. Although beyond the scope of this paper, we would like to stress the importance of basic guidelines and procedures for experiments to assure the quality and integrity of laboratory studies. For instance, ideally samples should be blinded for the operator performing the measurements and experimental groups should be randomly dispersed over the plates. If possible, run all samples on the same plate, especially when samples are paired. This is of course not always possible when the number of samples is too high. In that case, use assays/materials from the same batch to avoid batch-to-batch differences as much as possible. For each separate plate, QC samples with known values should be included on different spots throughout the plate to assess intra- and inter-variability. These procedures for conducting an experiment are often not mentioned.
Twenty-five (27%) studies did not use the correct sample type for their complement measurements. EDTA-plasma is the preferred sample type for measuring individual complement components and their activation products to minimize artificial complement activation. Most of these 25 studies chose serum for their analysis. This does not necessarily mean that the results presented in these 25 studies are unreliable and that these studies are invalid. However, complement components analyses in serum samples can be subjected to artificial complement activation during clotting leading to increased variation in concentrations between samples thereby introducing noise (155). In addition, the identified studies using serum samples did not mention exactly how serum was prepared and what type of collection tubes (with or without clot activator) were used. As also mentioned above, different serum collection tubes may affect complement analyses of the lectin pathway (80, 85). It has been shown that the use of silica as clot activator results in lower ficolin-2 levels and increased ficolin-1 levels (85, 86). In contrast, ficolin-2 depletion does not occur in serum prepared using collection tubes without a clot activator, but the study of Geno and coworkers showed that ficolin-2 inhibitors arise in serum after long-time storage at -80°C (156). These aspects make accurate measurements and identification of differences in concentrations between experimental groups difficult, especially when these differences are subtle. Complement split products are particularly sensitive to false results and measuring them in serum is not valid.
The third group identified consisted of 22 studies (24%) that did not specify which sample type was used for their measurements. Often, these studies also did not report the technology used for their analyses. Also here, this does not necessarily mean that these studies are of lower quality regarding the assessment of complement. However, for these studies it is very difficult to objectively assess and value the results as it is not clear how these results were obtained. Providing information regarding samples, collection tubes, shipment conditions, pre-analytical sample handling and storage, used techniques and methods is key, not only for assessing complement activation but for performing research in general. Providing this information is not only the responsibility of the authors but also of the reviewers during the peer-review process.
A systematic review is a powerful analytical tool to collect and analyze data in an objective and standardized way. However, as with all methods, there are limitations. One limitation of our study is that the research question did not include complement components other than the complement markers C3, C5, their split products and TCC. These markers were selected as these are key components of the complement system and, to date, the only approved complement-specific drug targets C5. Another drawback of our study is that systematic searches by using keywords is limited. If MeSH keywords do not match or are not mentioned in titles and/or abstracts, these studies are missed. Next to these ‘practical’ limitations, we are aware that our systematic search does not fully represent pre-analytical sample handling and complement assessment in a daily diagnostic setting. Complement measurements performed solely for diagnostic purposes are not reported in the Pubmed/MEDLINE database and are therefore missed.
As mentioned in the introduction, there is a high need for appropriate pre-analytical sample handling, storage of samples and standardization of complement testing to be able to compare results between studies and laboratories. Most of the twenty-five studies identified as not using not the correct sample type for their measurements, used serum samples to measure complement activation products.
To provide insight in the effect of artificial complement activation of serum samples in the tube, we measured C3c and sTCC levels in both serum and plasma samples incubated at RT or on ice for several hours. The results clearly show that in serum samples, both C3c levels and variation in levels between samples immediately increases when samples are ‘left on the bench’ at RT for a couple of hours. For plasma samples, this effect is completely absent for the first few hours and only after 16 h, also here some increase in variation and levels is observed. These effects in plasma after 16 h are expected as it is known that these anticoagulants minimize complement activation but not inhibit it completely (1). Incubating samples on ice most certainly slows down artificial complement activation, as the effects on C3c levels in serum are less pronounced when compared to RT. However, also here it is clear that levels and variation between samples changes over time, thereby obscuring the measurements. More or less the same effects were seen for the sTCC measurements, but it was not expected that levels would decrease in serum samples after incubation at RT. An explanation for this is currently lacking, although when also observing the serum sTCC levels incubated on ice, it is clear that levels varied a lot, making measurements difficult to interpret, especially with these low numbers. Regarding the different plasma samples analyzed in this experiment, results show that the concentrations for both C3c and sTCC remained stable for 2 h at RT and on ice in EDTA-plasma. Although concentrations and variations between samples shifted a little after 1 and 2 h in citrate and heparin plasma, they remained relatively stable when compared to baseline. However, it is well-known from literature that EDTA is more effective in inhibiting complement activation when compared to citrate and heparin (83, 157, 158). This holds true not only for benchtop stability but also after freeze-thawing of samples (83), although the magnitude of effects varies between the different complement components. Overall, these results re-confirm that EDTA-plasma is the most stable sample type for measuring complement activation products. Moreover, our results showed that levels in EDTA-plasma samples with extra EDTA (≥20 mM instead of 10 mM) were more stable when compared to plasma samples with no extra EDTA (normal EDTA collection tube). This might argue for the need of adding extra EDTA when samples are collected for the measurement of complement activation products. However, a larger study is needed to confirm these initial results.
Of note, our guidelines for sample handling are more strict than the actual limits that we (and others) have measured in our experimental set-up with healthy control samples (83). However, importantly, conditions in clinical practice are less controlled than in planned experimental settings of blood collection, as was the case in this study. In addition, samples in which there is already (low-rate) complement activation, in vitro activation may have faster kinetics, making them even more prone to false results after inappropriate handling.
To illustrate the effect of poorly standardized testing, we measured C3 levels in ETDA-plasma from HCs using two assay setups. Both assays were exact the same except that two distinct capture antibodies were used, recognizing different epitopes in the C3 protein. Recognizing alternative epitopes also means that binding affinity for the C3 antigen differs between the two antibodies. Variation in binding affinity will result in different amounts of antigen that can be detected in an assay. Indeed, results showed a 2.7-fold change in mean C3 levels between the two assay setups in this small cohort of healthy individuals. These results show that using two distinct antibodies, recognizing different epitopes of C3, leads to difficulties in assessing and comparing data and may have consequences for interpretation. Differences can be explained by the conformational changes of C3 during activation (159). Together with accessibility, small changes in conformational epitopes can lead to changes in affinity and antibody kinetics when applied in assays. This indicates the need for assays using antibodies that recognize the same epitope within the antigen being measured, especially in the light that antibody-based assays are among the most-preferred methods for complement assessment. Next to that, assays need to be calibrated against uniform protein standards in order to produce results that can be compared between different testing facilities.
Furthermore, our results show that selecting the correct markers, accompanied by the appropriate technique, is of high importance for measuring complement, especially in a diagnostic setting. Our measurements in COVID-19 patients, using dedicated ELISAs for both the native proteins as well as the split products, clearly showed that complement is activated in COVID-19. Levels for the complement activation markers C3a, C3c and sTCC were increased in patients when compared to controls whereas no differences in levels for the native proteins C3 and C5 were detected. Measuring only C3 and C5 plasma concentrations (and not the split products), as performed in most peripheral hospitals handling routine protocols, would have led to the incorrect conclusion that complement is not activated in these COVID-19 patients. Using nephelometry to measure complement activation would, in this case, not suffice as this technique will not distinguish between native complement proteins and its split products and therefore measure a mixture of C3 and its activation products C3a, C3b, iC3b, C3c and C3d. This would have obscured the outcome. Also using ELISAs for only the unprocessed markers C3 and C5 would not suffice is this case. The combination of measurement of native and activated complement fragments would yield more and earlier insights in unexpected protein levels, deficiencies and could aid to predict the remaining complement-activating potential or aid in staging of complement during disease.
These inconsistencies indicate the high need for well-characterized and standardized assays. This need for standardization and well-defined and characterized assays is well-known within the field. It is therefore that in 2009, the Sub-Committee for the Standardization and Quality Assessment of Complement Measurements (the ‘Complement EQA Group’) was formally recognized and became part of the IUIS (International Union of Immunological Societies) Quality Assessment and Standardization Committee (https://iuis.org/committees/qas/) (2, 82). Next to that, the Complement EQA Group is a standing committee also of the International Complement Society (ICS). The purpose of the EQA group is to facilitate standardized diagnostic complement analysis by developing calibrator materials and test recommendations (2, 82). Initial steps have been made as a serum standard to be used as calibrator for quantitative and functional complement analysis in normal, non-activated human serum (Standard 1; ICS#1) was prepared and evaluated. Next to ‘Standard 1’, also a complement activated standard (Standard 2; ICS#2) was prepared to be used as calibrator in assays for quantifying complement activation products. Both calibrators, together with single patient pathological samples were sent around to participating complement diagnostics laboratories for quality assessment rounds (or ring trials); an inter-laboratory test that allows to evaluate the performance of testing laboratories. Using the calibrators ICS#1 and #2, several complement factors were quantified in parallel in all participating laboratories. The between-laboratory coefficient of variation (CV%) ranged between 0.07 and 0.99 (2, 3, 82). Creating such calibrators with defined amounts of the individual complement components for complement assessment in a diagnostic setting will most certainly improve the quality and consistency of the test results and much progress has been made regarding this. However, as also stated by the Complement EQA Group, other assay components should be used in a standardized matter. For instance, recommendations for sample matrices and advice/descriptions regarding the preferred epitopes in antibody-based assays would most likely improve standardized complement measurements. Next, the EQA Group could provide concentration ranges for complement markers within diseases to be used as reference values for laboratories. This will provide each testing facility the opportunity to set up its own assay without infringing reproducibility in test results between different laboratories.
As the results of our systematic literature review have illustrated, 49% of the identified studies investigating complement activation uses the appropriate sample type for their analysis while 27% of the studies did not. For 24% of the studies, it was not clear what sample type or technique was used. Next, we provided examples that using inappropriate sample types and techniques might lead to misinterpretation of results. Complement biology is an emerging field given the prominent pathogenic involvement in many diseases and its potential for tailored therapeutic intervention. As a result, assessment of complement activation and regulation will become more important in the near future for both research and the daily diagnostic setting. Therefore, it is of utmost importance that complement analysis is performed in a robust and reliable manner. This holds not only for the assessment itself, but also for sample collection, shipment, pre-analytical sample handling, and storage. We underline the importance of awareness regarding these matters and that they may influence the outcome of complement studies.
For the future, we recommend that a uniform protocol is used for standardized sample collection, shipment, pre-analytical handling and storage for both the assessment of complement function as the measurements of individual complement components. Next to that, a more detailed description of the study design is needed regarding used methods and materials (e.g., type of collection tubes). For functional testing, complement-preserved serum (prepared as indicated in Figure 2) should be used. To assess individual complement components, EDTA-plasma must be used to minimize artificial complement activation. A first set-up for this uniform protocol is outlined in Figure 2. The assessment itself should be performed using standardized assays to produce results that are robust and comparable between diagnostic laboratories. Regarding the assays, standardized calibrators and reagents must be used. Antibody-based assays should preferably use antibodies recognizing the same epitope to minimize the variation in kinetics of the antibodies for the antigen. Standardization of complement analysis is organized and coordinated by the Sub-Committee for the Standardization and Quality Assessment of Complement Measurements (the ‘Complement EQA Group’). We believe that this group should be strongly embedded within and supported by the complement society so that they can have a leading role in providing clear directives for complement measurements.
The original contributions presented in the study are included in the article/Supplementary Material. Further inquiries can be directed to the corresponding author.
ET, RB and WB designed and supervised the study. ET, MR and AN performed the experiments. ET analyzed the data and wrote the manuscript. RB, MM, AN, PN and WB critically reviewed the manuscript. All authors discussed and interpreted the results, critically read the article and approved the final version.
ET received funding from European Union’s Horizon 2020 research and innovation programme under grant agreement No. 899163 “Screening of inFlammation to enable personalized Medicine” (SciFiMed, https://scifimed.eu/) and under grant agreement No. 860044 “COmplement Regulation and Variations in Opportunistic infectionS” (CORVOS; EU-H2020-MSCA-ITN-EJD; https://www.corvos.eu). RB received funding from the Dutch Kidney foundation (DKF) / Top Consortia for Knowledge and Innovation’s (TKI) & Life Sciences & Health (LSH) joint project “Improved Risk Stratification prior to Kidney Transplantation by Discriminating Pathogenic from Clinically Irrelevant Donor Epitope Specific HLA antibodies in relation to complement activation” (STRIDE.COM) under grant agreement No. DKF PPS09 / TKI-LSH19014-H026.
We thank Alexander Hoischen for his help and advice during this study.
RB, MR, WB and ET are employees of Hycult Biotech b.v.
The remaining authors declare that the research was conducted in the absence of any commercial or financial relationships that could be construed as a potential conflict of interest.
All claims expressed in this article are solely those of the authors and do not necessarily represent those of their affiliated organizations, or those of the publisher, the editors and the reviewers. Any product that may be evaluated in this article, or claim that may be made by its manufacturer, is not guaranteed or endorsed by the publisher.
The Supplementary Material for this article can be found online at: https://www.frontiersin.org/articles/10.3389/fimmu.2022.1007102/full#supplementary-material
1. Nilsson B, Ekdahl KN. Complement diagnostics: Concepts, indications, and practical guidelines. Clin Dev Immunol (2012), 962702. doi: 10.1155/2012/962702
2. Prohaszka Z, Nilsson B, Frazer-Abel A, Kirschfink M, et al. Complement analysis 2016: Clinical indications, laboratory diagnostics and quality control. Immunobiology (2016) 221(11):1247–58. doi: 10.1016/j.imbio.2016.06.008
3. Prohaszka Z, Kirschfink M, Frazer-Abel A. Complement analysis in the era of targeted therapeutics. Mol Immunol (2018) 102:84–8. doi: 10.1016/j.molimm.2018.06.001
4. Kirschfink M, Mollnes TE. Modern complement analysis. Clin Diagn Lab Immunol (2003) 10(6):982–9. doi: 10.1128/CDLI.10.6.982-989.2003
5. Mohebnasab M, Eriksson O, Persson B, Sandholm K, Mohlin C, Huber-Lang M, et al. Current and future approaches for monitoring responses to anti-complement therapeutics. Front Immunol (2019) 10:2539. doi: 10.3389/fimmu.2019.02539
6. Walport MJ. Complement. Frst of two parts. N Engl J Med (2001) 344(14):1058–66. doi: 10.1056/NEJM200104053441406
7. Walport MJ. Complement. Second of two parts. N Engl J Med (2001) 344(15):1140–4. doi: 10.1056/NEJM200104123441506
8. Dempsey PW, Allison ME, Akkaraju S, Goodnow CC, Fearon DT. C3d of complement as a molecular adjuvant: Bridging innate and acquired immunity. Science (1996) 271(5247):348–50. doi: 10.1126/science.271.5247.348
9. Elvington M, Liszewski MK, Bertram P, Kulkarni HS, Atkinson JP. A C3(H20) recycling pathway is a component of the intracellular complement system. J Clin Invest (2017) 127(3):970–81. doi: 10.1172/JCI89412
10. Liszewski MK, Kolev M, Le Friec G, Leung M, Bertram PG, Fara AF, et al. Intracellular complement activation sustains T cell homeostasis and mediates effector differentiation. Immunity (2013) 39(6):1143–57. doi: 10.1016/j.immuni.2013.10.018
11. Tam JC, Bidgood SR, McEwan WA, James LC. Intracellular sensing of complement C3 activates cell autonomous immunity. Science (2014) 345(6201):1256070. doi: 10.1126/science.1256070
12. Ekdahl KN, Persson B, Mohlin C, Sandholm K, Skattum L, Nilsson B. Interpretation of serological complement biomarkers in disease. Front Immunol (2018) 9:2237. doi: 10.3389/fimmu.2018.02237
13. Luo S, Hu D, Wang M, Zipfel PF, Hu Y. Complement in hemolysis- and thrombosis- related diseases. Front Immunol (2020) 11:1212. doi: 10.3389/fimmu.2020.01212
14. Hajishengallis G, Reis ES, Mastellos DC, Ricklin D, Lambris JD. Novel mechanisms and functions of complement. Nat Immunol (2017) 18(12):1288–98. doi: 10.1038/ni.3858
15. Ricklin D, Reis ES, Mastellos DC, Gros P, Lambris JD. Complement component C3 - the "Swiss army knife" of innate immunity and host defense. Immunol Rev (2016) 274(1):33–58. doi: 10.1111/imr.12500
16. Lachmann PJ. Genetic deficiencies of the complement system. In: Boll ist sieroter Milan (Milano, Italy: Istituto Sieroterapico Milanese), vol. 53. (1974). p. suppl:195–207.
17. Ling M, Murali M. Analysis of the complement system in the clinical immunology laboratory. Clin Lab Med (2019) 39(4):579–90. doi: 10.1016/j.cll.2019.07.006
18. Ricklin D, Mastellos DC, Reis ES, Lambris JD. The renaissance of complement therapeutics. Nat Rev Nephrol (2018) 14(1):26–47. doi: 10.1038/nrneph.2017.156
19. Geerlings MJ, de Jong EK, den Hollander AI. The complement system in age-related macular degeneration: A review of rare genetic variants and implications for personalized treatment. Mol Immunol (2017) 84:65–76. doi: 10.1016/j.molimm.2016.11.016
20. Yang LB, Li R, Meri S, Rogers J, Shen Y. Deficiency of complement defense protein CD59 may contribute to neurodegeneration in alzheimer's disease. J Neurosci (2000) 20(20):7505–9. doi: 10.1523/JNEUROSCI.20-20-07505.2000
21. Krance SH, Wu CY, Zou Y, Mao H, Toufighi S, He X, et al. The complement cascade in alzheimer's disease: a systematic review and meta-analysis. Mol Psychiatry (2019) 26(10):5532–41. doi: 10.1038/s41380-019-0536-8
22. Woodruff TM, Costantini KJ, Crane JW, Atkin JD, Monk PN, Taylor SM, et al. The complement factor C5a contributes to pathology in a rat model of amyotrophic lateral sclerosis. J Immunol (2008) 181(12):8727–34. doi: 10.4049/jimmunol.181.12.8727
23. Kallenberg CG, Heeringa P. Complement system activation in ANCA vasculitis: A translational success story? Mol Immunol (2015) 68(1):53–6. doi: 10.1016/j.molimm.2015.06.005
24. Wester Trejo MAC, Trouw LA, Bajema IM. The role of complement in antineutrophil cytoplasmic antibody-associated vasculitis. Curr Opin Rheumatol (2019) 31(1):3–8. doi: 10.1097/BOR.0000000000000557
25. Ma R, Cui Z, Liao YH, Zhao MH. Complement activation contributes to the injury and outcome of kidney in human anti-glomerular basement membrane disease. J Clin Immunol (2013) 33(1):172–8. doi: 10.1007/s10875-012-9772-2
26. Hu SY, Jia XY, Yang XW, Yu F, Cui Z, Zhao MH. Glomerular C1q deposition and serum anti-C1q antibodies in anti-glomerular basement membrane disease. BMC Immunol (2013) 14:42. doi: 10.1186/1471-2172-14-42
27. Unsworth DJ. Complement deficiency and disease. J Clin Pathol (2008) 61(9):1013–7. doi: 10.1136/jcp.2008.056317
28. Raina R, Krishnappa V, Blaha T, Kann T, Hein W, Burke L, et al. Atypical hemolytic-uremic syndrome: An update on pathophysiology, diagnosis, and treatment. Ther Apher Dial (2019) 23(1):4–21. doi: 10.1111/1744-9987.12763
29. Mastellos DC, Ricklin D, Lambris JD. Clinical promise of next-generation complement therapeutics. Nat Rev Drug Discovery (2019) 18(9):707–29. doi: 10.1038/s41573-019-0031-6
30. Berentsen S. Complement activation and inhibition in autoimmune hemolytic anemia: Focus on cold agglutinin disease. Semin Hematol (2018) 55(3):141–9. doi: 10.1053/j.seminhematol.2018.04.002
31. Shim K, Begum R, Yang C, Wang H. Complement activation in obesity, insulin resistance, and type 2 diabetes mellitus. World J Diabetes (2020) 11(1):1–12. doi: 10.4239/wjd.v11.i1.1
32. Schartz ND, Tenner AJ. The good, the bad, and the opportunities of the complement system in neurodegenerative disease. J Neuroinflamm (2020) 17(1):354. doi: 10.1186/s12974-020-02024-8
33. Michels M, van de Kar NCAJ, Okrój M, Blom AM, van Kraaij SAW, Volokhina EB, et al. Overactivity of alternative pathway convertases in patients with complement-mediated renal diseases. Front Immunol (2018) 9:612. doi: 10.3389/fimmu.2018.00612
34. Merinero HM, García SP, García-Fernández J, Arjona E, Tortajada A, Rodríguez de Córdoba S, et al. Complete functional characterization of disease-associated genetic variants in the complement factor h gene. Kidney Int (2018) 93(2):470–81. doi: 10.1016/j.kint.2017.07.015
35. Medjeral-Thomas NR, Pickering MC, Cook HT. Complement and kidney disease, new insights. Curr Opin Nephrol Hypertens (2021) 30(3):310–6. doi: 10.1097/MNH.0000000000000705
36. Gavriilaki M, Kimiskidis VK, Gavriilaki E. Precision medicine in neurology: The inspirational paradigm of complement therapeutics. Pharm (Basel) (2020) 13(11):341. doi: 10.3390/ph13110341
37. Lin Q, Min Y, Li Y, Zhu Y, Song X, Xu Q, et al. Henoch-schonlein purpura with hypocomplementemia. Pediatr Nephrol (2012) 27(5):801–6. doi: 10.1007/s00467-011-2070-z
38. Dumont C, Mérouani A, Ducruet T, Benoit G, Clermont MJ, Lapeyraque AL, et al. Clinical relevance of membrane attack complex deposition in children with IgA nephropathy and henoch-schonlein purpura. Pediatr Nephrol (2020) 35(5):843–50. doi: 10.1007/s00467-019-04445-x
39. Buck A, Christensen J, McCarty M. Hypocomplementemic urticarial vasculitis syndrome: a case report and literature review. J Clin Aesthet Dermatol (2012) 5(1):36–46.
40. Bigler C, Schaller M, Perahud I, Osthoff M, Trendelenburg M. Autoantibodies against complement C1q specifically target C1q bound on early apoptotic cells. J Immunol (2009) 183(5):3512–21. doi: 10.4049/jimmunol.0803573
41. Woodruff TM, Crane JW, Proctor LM, Buller KM, Shek AB, de Vos K, et al. Therapeutic activity of C5a receptor antagonists in a rat model of neurodegeneration. FASEB J (2006) 20(9):1407–17. doi: 10.1096/fj.05-5814com
42. Torina A, Villari S, Blanda V, Vullo S, La Manna MP, Shekarkar Azgomi M, et al. Innate immune response to tick-borne pathogens: Cellular and molecular mechanisms induced in the hosts. Int J Mol Sci (2020) 21(15):5437. doi: 10.3390/ijms21155437
43. Ermert D, Ram S, Laabei M. The hijackers guide to escaping complement: Lessons learned from pathogens. Mol Immunol (2019) 114:49–61. doi: 10.1016/j.molimm.2019.07.018
44. Sina C, Kemper C, Derer S. The intestinal complement system in inflammatory bowel disease: Shaping intestinal barrier function. Semin Immunol (2018) 37:66–73. doi: 10.1016/j.smim.2018.02.008
45. Miao Z, Ding K, Jin S, Dai L, Dai C, Li X. Using serum peptidomics to discovery the diagnostic marker for different stage of ulcerative colitis. J Pharm BioMed Anal (2021) 193:113725. doi: 10.1016/j.jpba.2020.113725
46. Zhou W, Farrar CA, Abe K, Pratt JR, Marsh JE, Wang Y, et al. Predominant role for C5b-9 in renal ischemia/reperfusion injury. J Clin Invest (2000) 105(10):1363–71. doi: 10.1172/JCI8621
47. Howard MC, Nauser CL, Farrar CA, Sacks SH. Complement in ischaemia-reperfusion injury and transplantation. Semin Immunopathol (2021) 43(6):789–97. doi: 10.1007/s00281-021-00896-3
48. Arias-Cabrales CE, Riera M, Pérez-Sáez MJ, Gimeno J, Benito D, Redondo D, et al. Activation of final complement components after kidney transplantation as a marker of delayed graft function severity. Clin Kidney J (2021) 14(4):1190–6. doi: 10.1093/ckj/sfaa147
49. Doreille A, Dieude M, Cardinal H. The determinants, biomarkers, and consequences of microvascular injury in kidney transplant recipients. Am J Physiol Renal Physiol (2019) 316(1):F9–F19. doi: 10.1152/ajprenal.00163.2018
50. Franzin R, Stasi A, Fiorentino M, Stallone G, Cantaluppi V, Gesualdo L, et al. Inflammaging and complement system: A link between acute kidney injury and chronic graft damage. Front Immunol (2020) 11:734. doi: 10.3389/fimmu.2020.00734
51. Howard JF Jr. Myasthenia gravis: The role of complement at the neuromuscular junction. Ann N Y Acad Sci (2018) 1412(1):113–28. doi: 10.1111/nyas.13522
52. Vogel CW. The role of complement in myocardial infarction reperfusion injury: An underappreciated therapeutic target. Front Cell Dev Biol (2020) 8:606407. doi: 10.3389/fcell.2020.606407
53. Uzonyi B, Szabó Z, Trojnár E, Hyvärinen S, Uray K, Nielsen HH, et al. Autoantibodies against the complement regulator factor h in the serum of patients with neuromyelitis optica spectrum disorder. Front Immunol (2021) 12:660382. doi: 10.3389/fimmu.2021.660382
54. Kielar M, Gala-Błądzińska A, Dumnicka P, Ceranowicz P, Kapusta M, Naumnik B, et al. Complement components in the diagnosis and treatment after kidney transplantation-is there a missing link? Biomolecules (2021) 11(6). doi: 10.3390/biom11060773
55. Loeffler DA, Camp DM, Conant SB. Complement activation in the parkinson's disease substantia nigra: an immunocytochemical study. J Neuroinflamm (2006) 3:29. doi: 10.1186/1742-2094-3-29
56. Yamada T, McGeer PL, McGeer EG. Lewy bodies in parkinson's disease are recognized by antibodies to complement proteins. Acta Neuropathol (1992) 84(1):100–4. doi: 10.1007/BF00427222
57. Rozemuller AJ, Eikelenboom P, Theeuwes JW, Jansen Steur EN, de Vos RA. Activated microglial cells and complement factors are unrelated to cortical lewy bodies. Acta Neuropathol (2000) 100(6):701–8. doi: 10.1007/s004010000225
58. Kitamura Y, Kojima M, Kurosawa T, Sasaki R, Ichihara S, Hiraku Y, et al. Proteomic profiling of exosomal proteins for blood-based biomarkers in parkinson's disease. Neuroscience (2018) 392:121–8. doi: 10.1016/j.neuroscience.2018.09.017
59. Shah N, Bhatt H. Paroxysmal nocturnal hemoglobinuria. In: StatPearls. FL: Treasure Island (2021).
60. Damgaard C, Holmstrup P, Van Dyke TE, Nielsen CH. The complement system and its role in the pathogenesis of periodontitis: Current concepts. J Periodontal Res (2015) 50(3):283–93. doi: 10.1111/jre.12209
61. Burk AM, Martin M, Flierl MA, Rittirsch D, Helm M, Lampl L, et al. Early complementopathy after multiple injuries in humans. Shock (2012) 37(4):348–54. doi: 10.1097/SHK.0b013e3182471795
62. Huber-Lang M, Kovtun A, Ignatius A. The role of complement in trauma and fracture healing. Semin Immunol (2013) 25(1):73–8. doi: 10.1016/j.smim.2013.05.006
63. Amara U, Flierl MA, Rittirsch D, Klos A, Chen H, Acker B, et al. Molecular intercommunication between the complement and coagulation systems. J Immunol (2010) 185(9):5628–36. doi: 10.4049/jimmunol.0903678
64. Bemis EA, Norris JM, Seifert J, Frazer-Abel A, Okamoto Y, Feser ML, et al. Complement and its environmental determinants in the progression of human rheumatoid arthritis. Mol Immunol (2019) 112:256–65. doi: 10.1016/j.molimm.2019.05.012
65. Nimgaonkar VL, Prasad KM, Chowdari KV, Severance EG, Yolken RH. The complement system: a gateway to gene-environment interactions in schizophrenia pathogenesis. Mol Psychiatry (2017) 22(11):1554–61. doi: 10.1038/mp.2017.151
66. de Nooijer AH, Grondman I, Janssen NAF, Netea MG, Willems L, van de Veerdonk FL, et al. Complement activation in the disease course of COVID-19 and its effects on clinical outcomes. J Infect Dis (2020) 223(2):214–24. doi: 10.1093/infdis/jiaa646.
67. Karasu E, Nilsson B, Köhl J, Lambris JD, Huber-Lang M. Targeting complement pathways in polytrauma- and sepsis-induced multiple-organ dysfunction. Front Immunol (2019) 10:543. doi: 10.3389/fimmu.2019.00543
68. Unnewehr H, Rittirsch D, Sarma JV, Zetoune F, Flierl MA, Perl M, et al. Changes and regulation of the C5a receptor on neutrophils during septic shock in humans. J Immunol (2013) 190(8):4215–25. doi: 10.4049/jimmunol.1200534
69. Panelius J, Meri S. Complement system in dermatological diseases - fire under the skin. Front Med (Lausanne) (2015) 2:3. doi: 10.3389/fmed.2015.00003
70. Reti M, Farkas P, Csuka D, Rázsó K, Schlammadinger Á, Udvardy ML, et al. Complement activation in thrombotic thrombocytopenic purpura. J Thromb Haemost (2012) 10(5):791–8. doi: 10.1111/j.1538-7836.2012.04674.x
71. Jha P, Bora PS, Bora NS. The role of complement system in ocular diseases including uveitis and macular degeneration. Mol Immunol (2007) 44(16):3901–8. doi: 10.1016/j.molimm.2007.06.145
72. Jodele S, Kohl J. Tackling COVID-19 infection through complement-targeted immunotherapy. Br J Pharmacol (2020) 178(14):2832–48. doi: 10.22541/au.158880110.01220133
73. Thurman JM. Complement and the kidney: An overview. Adv Chronic Kidney Dis (2020) 27(2):86–94. doi: 10.1053/j.ackd.2019.10.003
74. Reis ES, Mastellos DC, Ricklin D, Mantovani A, Lambris JD. Complement in cancer: untangling an intricate relationship. Nat Rev Immunol (2018) 18(1):5–18. doi: 10.1038/nri.2017.97
75. Reis ES, Mastellos DC, Hajishengallis G, Lambris JD. New insights into the immune functions of complement. Nat Rev Immunol (2019) 19(8):503–16. doi: 10.1038/s41577-019-0168-x
76. Ricklin D, Reis ES, Lambris JD. Complement in disease: A defence system turning offensive. Nat Rev Nephrol (2016) 12(7):383–401. doi: 10.1038/nrneph.2016.70
77. Cedzynski M, Thielens NM, Mollnes TE, Vorup-Jensen T. Editorial: The role of complement in health and disease. Front Immunol (2019) 10:1869. doi: 10.3389/fimmu.2019.01869
78. Montaldo C, Messina F, Abbate I, Antonioli M, Bordoni V, Aiello A, et al. Multi-omics approach to COVID-19: a domain-based literature review. J Transl Med (2021) 19(1):501. doi: 10.1186/s12967-021-03168-8
79. Yu L, Shen H, Ren X, Wang A, Zhu S, Zheng Y, et al. Multi-omics analysis reveals the interaction between the complement system and the coagulation cascade in the development of endometriosis. Sci Rep (2021) 11(1):11926. doi: 10.1038/s41598-021-90112-x
80. Moghimi SM, Dmitri S. Critical issues and pitfalls in serum and plasma handling for complement analysis in nanomedicine and bionanotechnology. Nano Today (2022) 44:101479. doi: 10.1016/j.nantod.2022.101479
81. Moghimi SM, Simberg D, Papini E, Farhangrazi ZS. Complement activation by drug carriers and particulate pharmaceuticals: Principles, challenges and opportunities. Adv Drug Delivery Rev (2020) 157:83–95. doi: 10.1016/j.addr.2020.04.012
82. Frazer-Abel A, Kirschfink M, Prohaszka Z. Expanding horizons in complement analysis and quality control. Front Immunol (2021) 12:697313. doi: 10.3389/fimmu.2021.697313
83. Mollnes TE, Garred P, Bergseth G. Effect of time, temperature and anticoagulants on in vitro complement activation: consequences for collection and preservation of samples to be examined for complement activation. Clin Exp Immunol (1988) 73(3):484–8.
84. Lachmann PJ. Preparing serum for functional complement assays. J Immunol Methods (2010) 352(1-2):195–7. doi: 10.1016/j.jim.2009.11.003
85. Hein E, Bay JT, Munthe-Fog L, Garred P. Ficolin-2 reveals different analytical and biological properties dependent on different sample handling procedures. Mol Immunol (2013) 56(4):406–12. doi: 10.1016/j.molimm.2013.05.233
86. Brady AM, Spencer BL, Falsey AR, Nahm MH. Blood collection tubes influence serum ficolin-1 and ficolin-2 levels. Clin Vaccine Immunol (2014) 21(1):51–5. doi: 10.1128/CVI.00607-13
87. Ekdahl KN, Norberg D, Bengtsson AA, Sturfelt G, Nilsson UR, Nilsson B. Use of serum or buffer-changed EDTA-plasma in a rapid, inexpensive, and easy-to-perform hemolytic complement assay for differential diagnosis of systemic lupus erythematosus and monitoring of patients with the disease. Clin Vaccine Immunol (2007) 14(5):549–55. doi: 10.1128/CVI.00486-06
88. Moghimi SM, Simberg D, Skotland T, Yaghmur A, Hunter AC, et al. The interplay between blood proteins, complement, and macrophages on nanomedicine performance and responses. J Pharmacol Exp Ther (2019) 370(3):581–92. doi: 10.1124/jpet.119.258012
89. Mollnes TE, Brekke OL, Fung M, Fure H, Christiansen D, Bergseth G, et al. Essential role of the C5a receptor in e coli-induced oxidative burst and phagocytosis revealed by a novel lepirudin-based human whole blood model of inflammation. Blood (2002) 100(5):1869–77.
90. Kenawy HI, Boral I, Bevington A. Complement-coagulation cross-talk: A potential mediator of the physiological activation of complement by low pH. Front Immunol (2015) 6:215. doi: 10.3389/fimmu.2015.00215
91. Almitairi JOM, Venkatraman Girija U, Furze CM, Simpson-Gray X, Badakshi F, Marshall JE, et al. Structure of the C1r-C1s interaction of the C1 complex of complement activation. Proc Natl Acad Sci U.S.A. (2018) 115(4):768–73. doi: 10.1073/pnas.1718709115
92. Wallis R, Mitchell DA, Schmid R, Schwaeble WJ, Keeble AH. Paths reunited: Initiation of the classical and lectin pathways of complement activation. Immunobiology (2010) 215(1):1–11. doi: 10.1016/j.imbio.2009.08.006
93. Mortensen S, Jensen JK, Andersen GR. Solution structures of complement C2 and its C4 complexes propose pathway-specific mechanisms for control and activation of the complement proconvertases. J Biol Chem (2016) 291(32):16494–507. doi: 10.1074/jbc.M116.722017
94. Nilsson UR, Nilsson B. Simplified assays of hemolytic activity of the classical and alternative complement pathways. J Immunol Methods (1984) 72(1):49–59. doi: 10.1016/0022-1759(84)90432-0
95. Platts-Mills TA, Ishizaka K. Activation of the alternate pathway of human complements by rabbit cells. J Immunol (1974) 113(1):348–58.
96. Gadjeva M, Thiel S, Jensenius JC. Assays for the mannan-binding lectin pathway. Curr Protoc Immunol (2004), 13 6. doi: 10.1002/0471142735.im1306s58
97. Seelen MA, Roos A, Wieslander J, Mollnes TE, Sjöholm AG, Wurzner R, et al. Functional analysis of the classical, alternative, and MBL pathways of the complement system: Standardization and validation of a simple ELISA. J Immunol Methods (2005) 296(1-2):187–98. doi: 10.1016/j.jim.2004.11.016
98. Hendrickson OD, Skopinskaya SN, Yarkov SP, Zherdev AV, Dzantiev BB. Development of liposome immune lysis assay for the herbicide atrazine. J Immunoassay Immunochem (2004) 25(3):279–94. doi: 10.1081/IAS-200028084
99. Jaskowski TD, Martins TB, Litwin CM, Hill HR. Comparison of three different methods for measuring classical pathway complement activity. Clin Diagn Lab Immunol (1999) 6(1):137–9. doi: 10.1128/CDLI.6.1.137-139.1999
100. Bergseth G, Ludviksen JK, Kirschfink M, Giclas PC, Nilsson B, Mollnes TE. An international serum standard for application in assays to detect human complement activation products. Mol Immunol (2013) 56(3):232–9. doi: 10.1016/j.molimm.2013.05.221
101. Levine L, Osler AG, Mayer MM. Studies on the role of ca++ and mg++ in complement fixation and immune hemolysis. III. the respective roles of ca++ and mg++ in immune hemolysis. J Immunol (1953) 71(5):374–9.
102. Levine L, Cowan KM, Osler AG, Mayer MM. Studies on the role of ca++ and mg++ in complement fixation and immune hemolysis. i. uptake of complement nitrogen by specific precipitates and its inhibition by ethylenediamine tetraacetate. J Immunol (1953) 71(5):359–66.
103. Zaferani A, Talsma D, Richter MK, Daha MR, Navis GJ, Seelen MA, et al. Heparin/heparan sulphate interactions with complement–a possible target for reduction of renal function loss? Nephrol Dial Transplant (2014) 29(3):515–22. doi: 10.1093/ndt/gft243
104. Yang S, McGookey M, Wang Y, Cataland SR, Wu HM. Effect of blood sampling, processing, and storage on the measurement of complement activation biomarkers. Am J Clin Pathol (2015) 143(4):558–65. doi: 10.1309/AJCPXPD7ZQXNTIAL
105. Mollnes TE, Kirschfink M. Complement analysis in clinic and research. Adv Exp Med Biol (2006) 586:361–80. doi: 10.1007/0-387-34134-X_24
106. Harboe M, Thorgersen EB, Mollnes TE. Advances in assay of complement function and activation. Adv Drug Delivery Rev (2011) 63(12):976–87. doi: 10.1016/j.addr.2011.05.010
107. Sandholm K, Garred P, Bergseth G.. Discrepancies in plasma levels of complement components measured by a newly introduced commercially available magnetic bead technique compared to presently available clinical reference intervals. Scand J Immunol (2020) 91(2):e12831. doi: 10.1111/sji.12831
108. Sandholm K, Persson B, Abdalla S, Mohlin C, Nilsson B, Ekdahl KN. Quantification of complement proteins with special reference to C1q: Multiplex versus ELISA versus rocket immunoelectrophoresis versus nephelometry. Methods Mol Biol 2021 (2227) p:33–41. doi: 10.1007/978-1-0716-1016-9_3
109. Moher D, Liberati A, Tetzlaff J, Altman DG. Preferred reporting items for systematic reviews and meta-analyses: the PRISMA statement. In: Ann Intern med, vol. 151. (2009). p. 264–9, W64. doi: 10.7326/0003-4819-151-4-200908180-00135
110. Young JM, Solomon MJ. How to critically appraise an article. Nat Clin Pract Gastroenterol Hepatol (2009) 6(2):82–91. doi: 10.1038/ncpgasthep1331
111. Liu M, Luo X, Xu Q, Yu H, Gao L, Zhou R, Wang T, et al. Adipsin of the alternative complement pathway is a potential predictor for preeclampsia in early pregnancy. Front Immunol (2021) 12:702385. doi: 10.3389/fimmu.2021.702385
112. Pache F, Ringelstein M, Aktas O, Kleiter I, Jarius S, Siebert N, et al. C3 and C4 complement levels in AQP4-IgG-positive NMOSD and in MOGAD. J Neuroimmunol (2021) 360:577699. doi: 10.1016/j.jneuroim.2021.577699
113. Michels M, van de Kar NCAJ, van Kraaij SAW, Sarlea SA, Gracchi V, Engels FAPT, et al. Different aspects of classical pathway overactivation in patients with C3 glomerulopathy and immune complex-mediated membranoproliferative glomerulonephritis. Front Immunol (2021) 12:715704. doi: 10.3389/fimmu.2021.715704
114. Prens LM, Ardon CB, van Straalen KR, van der Zee HH, Seelen MAJ, Laman JD, et al. No evident systemic terminal complement pathway activation in hidradenitis suppurativa. J Invest Dermatol (2021), 2966–9, 141(12). doi: 10.1016/j.jid.2021.03.037
115. Chiu YL, Lin WC, Shu KH, Fang YW, Chang FC, Chou YH, et al. Alternative complement pathway is activated and associated with galactose-deficient IgA1 antibody in IgA nephropathy patients. Front Immunol (2021) 12:638309. doi: 10.3389/fimmu.2021.638309
116. Dhooge PPA, Runhart EH, Li CHZ, de Kat Angelino CM, Hoyng CB, van der Molen RG, et al. Systemic complement activation levels in stargardt disease. PloS One (2021) 16(6):e0253716. doi: 10.1371/journal.pone.0253716
117. Sinkovits G, Mező B, Réti M, Müller V, Iványi Z, Gál J, et al. Complement overactivation and consumption predicts in-hospital mortality in SARS-CoV-2 infection. Front Immunol (2021) 12:663187. doi: 10.3389/fimmu.2021.663187
118. Rognes IN, Pischke SE, Ottestad W, Røislien J, Berg JP, Johnson C, et al. Increased complement activation 3 to 6 h after trauma is a predictor of prolonged mechanical ventilation and multiple organ dysfunction syndrome: A prospective observational study. Mol Med (2021) 27(1):35. doi: 10.1186/s10020-021-00286-3
119. Denzinger M, Held M, Daigeler A, Krajewski S, Link A. Complement activation at the interface of wound dressings and blood does not influence keratinocyte migration/proliferation in vitro. Wound Repair Regener (2020) 28(4):573–5. doi: 10.1111/wrr.12817
120. Grinde D, Øverland T, Lima K, Schjalm C, Mollnes TE, Abrahamsen TG. Complement activation in 22q11.2 deletion syndrome. J Clin Immunol (2020) 40(3):515–23. doi: 10.1007/s10875-020-00766-x
121. Kristensen MK, Hansen MB, Madsen MB, Hansen CB, Pilely K, Hyldegaard O, et al. Complement activation is associated with mortality in patients with necrotizing soft-tissue infections-a prospective observational study. Front Immunol (2020) 11:17. doi: 10.3389/fimmu.2020.00017
122. Chauvet S, Berthaud R, Devriese M, Mignotet M, Vieira Martins P, Robe-Rybkine T, et al. Anti-factor b antibodies and acute postinfectious GN in children. J Am Soc Nephrol (2020) 31(4):829–40. doi: 10.1681/ASN.2019080851
123. Abe T, Kubo K, Izumoto S, Shimazu S, Goan A, Tanaka T, et al. Complement activation in human sepsis is related to sepsis-induced disseminated intravascular coagulation. Shock (2020) 54(2):198–204. doi: 10.1097/SHK.0000000000001504
124. Tjernberg AR, Woksepp H, Sandholm K, Johansson M, Dahle C, Ludvigsson JF, et al. Celiac disease and complement activation in response to streptococcus pneumoniae. Eur J Pediatr (2020) 179(1):133–40. doi: 10.1007/s00431-019-03490-w
125. Scambi C, Ugolini S, Tonello M, Bortolami O, De Franceschi L, Castagna A, et al. Complement activation in the plasma and placentas of women with different subsets of antiphospholipid syndrome. Am J Reprod Immunol (2019) 82(6):e13185. doi: 10.1111/aji.13185
126. Schein TN, Blackburn TE, Heath SL, Barnum SR. Plasma levels of soluble membrane attack complex are elevated despite viral suppression in HIV patients with poor immune reconstitution. Clin Exp Immunol (2019) 198(3):359–66. doi: 10.1111/cei.13366
127. Zhang MF, Huang J, Zhang YM, Qu Z, Wang X, Wang F, et al. Complement activation products in the circulation and urine of primary membranous nephropathy. BMC Nephrol (2019) 20(1):313. doi: 10.1186/s12882-019-1509-5
128. Gavriilaki E, Chrysanthopoulou A, Sakellari I, Batsis I, Mallouri D, Touloumenidou T, et al. Linking complement activation, coagulation, and neutrophils in transplant-associated thrombotic microangiopathy. Thromb Haemost (2019) 119(9):1433–40. doi: 10.1055/s-0039-1692721
129. Lynch AM, Mandava N, Patnaik JL, Frazer-Abel AA, Wagner BD, Palestine AG, et al. Systemic activation of the complement system in patients with advanced age-related macular degeneration. Eur J Ophthalmol (2020) 30(5):1061–8. doi: 10.1177/1120672119857896
130. Hoiland II, Liang RA, Braekkan SK, Pettersen K, Ludviksen JK, Latysheva N, et al. Complement activation assessed by the plasma terminal complement complex and future risk of venous thromboembolism. J Thromb Haemost (2019) 17(6):934–43. doi: 10.1111/jth.14438
131. Burwick RM, Velásquez JA, Valencia CM, Gutiérrez-Marín J, Edna-Estrada F, Silva JL, et al. Terminal complement activation in preeclampsia. Obstet Gynecol (2018) 132(6):1477–85. doi: 10.1097/AOG.0000000000002980
132. Rodriguez E, Gimeno J, Arias-Cabrales C, Barrios C, Redondo-Pachón D, Soler MJ, et al. Membrane attack complex and factor h in humans with acute kidney injury. Kidney Blood Press Res (2018) 43(5):1655–65. doi: 10.1159/000494680
133. Chauvet S, Roumenina LT, Aucouturier P, Marinozzi MC, Dragon-Durey MA, Karras A, et al. Both monoclonal and polyclonal immunoglobulin contingents mediate complement activation in monoclonal gammopathy associated-C3 glomerulopathy. Front Immunol (2018) 9:2260. doi: 10.3389/fimmu.2018.02260
134. Bavia L, Lidani KCF, Andrade FA, Sobrinho MIAH, Nisihara RM, de Messias-Reason IJ. Complement activation in acute myocardial infarction: An early marker of inflammation and tissue injury? Immunol Lett (2018) 200:18–25. doi: 10.1016/j.imlet.2018.06.006
135. Kanni T, Zenker O, Habel M, Riedemann N, Giamarellos-Bourboulis EJ. Complement activation in hidradenitis suppurativa: A new pathway of pathogenesis? Br J Dermatol (2018) 179(2):413–9. doi: 10.1111/bjd.16428
136. Siljan WW, Holter JC, Nymo SH, Husebye E, Ueland T, Aukrust P. Cytokine responses, microbial aetiology and short-term outcome in community-acquired pneumonia. Eur J Clin Invest (2018) 48(1):e12865. doi: 10.1111/eci.12865
137. Trendelenburg M, Stallone F, Pershyna K, Eisenhut T, Twerenbold R, Wildi K, et al. Complement activation products in acute heart failure: Potential role in pathophysiology, responses to treatment and impacts on long-term survival. Eur Heart J Acute Cardiovasc Care (2018) 7(4):348–57. doi: 10.1177/2048872617694674
138. Togarsimalemath SK, Sethi SK, Duggal R, Le Quintrec M, Jha P, Daniel R, et al. A novel CFHR1-CFHR5 hybrid leads to a familial dominant C3 glomerulopathy. Kidney Int (2017) 92(4):876–87. doi: 10.1016/j.kint.2017.04.025
139. Suffritti C, Tobaldini E, Schiavon R, Strada S, Maggioni L, Mehta S, et al. Complement and contact system activation in acute congestive heart failure patients. Clin Exp Immunol (2017) 190(2):251–7. doi: 10.1111/cei.13011
140. Grosso G, Vikerfors A, Woodhams B, Adam M, Bremme K, Holmström M, et al. Thrombin activatable fibrinolysis inhibitor (TAFI) - a possible link between coagulation and complement activation in the antiphospholipid syndrome (APS). Thromb Res (2017) 158:168–73. doi: 10.1016/j.thromres.2017.06.028
141. Witczak BJ, Pischke SE, Reisæter AV, Midtvedt K, Ludviksen JK, Heldal K, et al. Elevated terminal C5b-9 complement complex 10 weeks post kidney transplantation was associated with reduced long-term patient and kidney graft survival. Front Immunol (2021) 12:738927. doi: 10.3389/fimmu.2021.738927
142. Milosevits G, Mészáros T, Őrfi E, Bakos T, Garami M, Kovács G. Complement-mediated hypersensitivity reactions to an amphotericin b-containing lipid complex (Abelcet) in pediatric patients and anesthetized rats: Benefits of slow infusion. Nanomedicine (2021) 34:102366. doi: 10.1016/j.nano.2021.102366
143. Mastellos DC, Pires da Silva BGP, Fonseca BAL, Fonseca NP, Auxiliadora-Martins M, Mastaglio S, et al. Complement C3 vs C5 inhibition in severe COVID-19: Early clinical findings reveal differential biological efficacy. Clin Immunol (2020) 220:108598. doi: 10.1016/j.clim.2020.108598
144. Sartain S, Shubert S, Wu MF, Wang T, Martinez C. The alternative complement pathway activation product ba as a marker for transplant-associated thrombotic microangiopathy. Pediatr Blood Cancer (2020) 67(3):e28070. doi: 10.1002/pbc.28070
145. Faria B, Gaya da Costa M, Poppelaars F, Franssen CFM, Pestana M, Berger SP, et al. Administration of intravenous iron formulations induces complement activation in-vivo. Front Immunol (2019) 10:1885. doi: 10.3389/fimmu.2019.01885
146. Qi J, Wang J, Chen J, Su J, Tang Y, Wu X, et al. Plasma levels of complement activation fragments C3b and sC5b-9 significantly increased in patients with thrombotic microangiopathy after allogeneic stem cell transplantation. Ann Hematol (2017) 96(11):1849–55. doi: 10.1007/s00277-017-3092-9
147. Nilsson PH, Thomas AM, Bergseth G, Gustavsen A, Volokhina EB, van den Heuvel LP, et al. Eculizumab-C5 complexes express a C5a neoepitope in vivo: Consequences for interpretation of patient complement analyses. Mol Immunol (2017) 89:111–4. doi: 10.1016/j.molimm.2017.05.021
148. Wehling C, Amon O, Bommer M, Hoppe B, Kentouche K, Schalk G, et al. Monitoring of complement activation biomarkers and eculizumab in complement-mediated renal disorders. Clin Exp Immunol (2017) 187(2):304–15. doi: 10.1111/cei.12890
149. Troldborg A, Halkjær L, Pedersen H, Hansen A, Loft AG, Lindegaard H, et al. Complement activation in human autoimmune diseases and mouse models; employing a sandwich immunoassay specific for C3dg. J Immunol Methods (2020) 486:112866. doi: 10.1016/j.jim.2020.112866
150. Halkjaer L, Troldborg A, Pedersen H, Jensen L, Hansen AG, Hansen TK, et al. Complement receptor 2 based immunoassay measuring activation of the complement system at C3-level in plasma samples from mice and humans. Front Immunol (2020) 11:774. doi: 10.3389/fimmu.2020.00774
151. Elvington M, Liszewski MK, Liszewski AR, Kulkarni HS, Hachem RR, Mohanakumar T, et al. Development and optimization of an ELISA to quantitate C3(H 2 O) as a marker of human disease. Front Immunol (2019) 10:703. doi: 10.3389/fimmu.2019.00703
152. Nilsson PH, Johnson C, Quach QH, Macpherson A, Durrant O, Pischke SE, et al. A conformational change of complement C5 is required for thrombin-mediated cleavage, revealed by a novel ex vivo human whole blood model preserving full thrombin activity. J Immunol (2021) 207(6):1641–51. doi: 10.4049/jimmunol.2001471
153. Vercauteren KOA, Lambrecht S, Delanghe J. Preanalytical classical and alternative complement pathway activity loss. Biochem Med (Zagreb) (2019) 29(3):030701. doi: 10.11613/BM.2019.030701
154. Mansur S, Othman MHD, Ismail AF, Kadir SHSA, Goh PS, Hasbullah H, et al. Synthesis and characterisation of composite sulphonated polyurethane/polyethersulphone membrane for blood purification application. Mater Sci Eng C Mater Biol Appl (2019) 99:491–504. doi: 10.1016/j.msec.2019.01.092
155. Hamad OA, Ekdahl KN, Nilsson PH, Andersson J, Magotti P, Lambris JD, et al. Complement activation triggered by chondroitin sulfate released by thrombin receptor-activated platelets. J Thromb Haemost (2008) 6(8):1413–21. doi: 10.1111/j.1538-7836.2008.03034.x
156. Geno KA, Kennedy RE, Sawyer P, Brown CJ, Nahm MH. Ficolin-2 inhibitors are present in sera after prolonged storage at -80 degrees c. PeerJ (2016) 4:e2705. doi: 10.7717/peerj.2705
157. Henno LT, Storjord E, Christiansen D, Bergseth G, Ludviksen JK, Fure H, et al. Effect of the anticoagulant, storage time and temperature of blood samples on the concentrations of 27 multiplex assayed cytokines - consequences for defining reference values in healthy humans. Cytokine (2017) 97:86–95. doi: 10.1016/j.cyto.2017.05.014
158. Strobel L, Johswich KO. Anticoagulants impact on innate immune responses and bacterial survival in whole blood models of neisseria meningitidis infection. Sci Rep (2018) 8(1):10225. doi: 10.1038/s41598-018-28583-8
Keywords: complement system, complement diagnostics, systematic review, immunoassays, standardization of measurements, complement activation
Citation: Brandwijk RJMGE, Michels MAHM, van Rossum M, de Nooijer AH, Nilsson PH, de Bruin WCC and Toonen EJM (2022) Pitfalls in complement analysis: A systematic literature review of assessing complement activation. Front. Immunol. 13:1007102. doi: 10.3389/fimmu.2022.1007102
Received: 29 July 2022; Accepted: 13 September 2022;
Published: 18 October 2022.
Edited by:
Christian Marcel Karsten, University of Lübeck, GermanyReviewed by:
Michael Kirschfink, Heidelberg University, GermanyCopyright © 2022 Brandwijk, Michels, van Rossum, de Nooijer, Nilsson, de Bruin and Toonen. This is an open-access article distributed under the terms of the Creative Commons Attribution License (CC BY). The use, distribution or reproduction in other forums is permitted, provided the original author(s) and the copyright owner(s) are credited and that the original publication in this journal is cited, in accordance with accepted academic practice. No use, distribution or reproduction is permitted which does not comply with these terms.
*Correspondence: Erik J. M. Toonen, ZS50b29uZW5AaHljdWx0YmlvdGVjaC5jb20=
†These authors contributed equally to this work
Disclaimer: All claims expressed in this article are solely those of the authors and do not necessarily represent those of their affiliated organizations, or those of the publisher, the editors and the reviewers. Any product that may be evaluated in this article or claim that may be made by its manufacturer is not guaranteed or endorsed by the publisher.
Research integrity at Frontiers
Learn more about the work of our research integrity team to safeguard the quality of each article we publish.