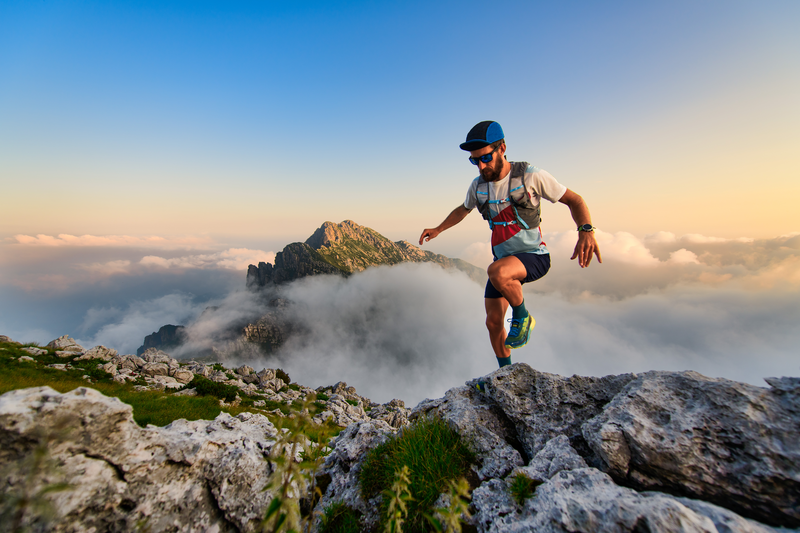
94% of researchers rate our articles as excellent or good
Learn more about the work of our research integrity team to safeguard the quality of each article we publish.
Find out more
SYSTEMATIC REVIEW article
Front. Immunol. , 14 September 2022
Sec. Cancer Immunity and Immunotherapy
Volume 13 - 2022 | https://doi.org/10.3389/fimmu.2022.1006860
This article is part of the Research Topic Current Research in Cardiovascular Pleiotropy: Beneficial and adverse effects of therapeutics View all 5 articles
Immune checkpoint inhibitors (ICIs) in combination withother anti-cancer treatments have been approved for a variety of cancers. While the difference in the incidence of cardiovascular adverse events has not been fully investigated. We aimed to assess the the differences in cardiotoxicity among cancer patients receiving different ICI therapies. PubMed, Embase, Web of Science, Cochrane Library, and ClinicalTrials.gov. websites were searched for all randomized controlled trials (RCTs) of ICI. The primary outcomes were any grade cardiotoxicity and Grade 3-5 cardiotoxicity, the secondary outcomes were any grade myocarditis and Grade 3-5 myocarditis, with sub-analyses based on cancer type and does of ICI. A systematic review and frequency network meta-analysis were then performed for cardiotoxicity events. 91 RCTs (n=52247) involving 12 treatment arms were finally included. We observed that PD-L1 + CTLA-4 had the highest risk among all therapies inducing any grade cardiotoxicity, and the differences were significant except PD-1 + CTLA-4, PD-1 + TTD and PD-L1 + TTD. In addition, CTLA-4 had a higher risk of Grade 3-5 cardiotoxicity than PD-1 and anit-PD-L1. For Grade 1-5 myocarditis and Grade 3-5 myocarditis, no significant difference was found among differences therapies. No differences were observed in subgroup analyses according to does and cancer type. There were differences in the incidence of cardiotoxicity among different ICI therapies. For ICI monotherapy, CTLA-4 may be linked to Grade 3-5 cardiotoxicity than PD-1 or PD-L1. For dual therapy, the cardiotoxicity of dual ICI therapy seems to be higher than that of chemotherapy or targeted therapy.
Recently, immune checkpoint inhibitors (ICIs) have greatly improved the outcomes of several cancers and have become the hotspot of cancer research (1). ICIs include anti-cytotoxic T-lymphocyte antigen-4 (CTLA-4), anti-programmed cell death 1 (PD-1), and anti-programmed cell death ligand 1 (PD-L1). Immune checkpoint inhibitors induce CD8-positive T cells to kill cancer cells and enhance the anti-tumor response of the immune system by blocking the inhibitory signal of T cell activation (2, 3). However, they may cause damage to normal tissues while attacking tumor cells and leading to the occurrence of immune-related adverse events (irAEs).
These irAEs can affect any organ system. The common irAEs include diarrhea, colitis, hepatitis, skin toxicities and endocrinopathies, with fewer irAEs in cardiovascular, hematologic, renal, neurologic and ophthalmologic (4). Although cardiotoxicity are uncommon, they are serious irAEs which may cause the interruption, termination of immunotherapy or even the death of the patient. Common cardiotoxicity include coronary artery disease, heart failure, myocarditis, atrial fibrillation, and pericardial disease. The mortality of myocarditis is up to 39.7% - 50%, ranking first among all irAEs (5–9).
Currently, ICIs are often used in combination with chemotherapeutic drugs or targeted therapeutic drugs (10). And more and more evidence supports the clinical value of combining appropriately chemotherapies or targeted therapies with ICI (11, 12). However, the effects of the combination therapy on cardiotoxicity are not clear. Due to its relatively low incidence, it is often easily ignored by clinicians. Although there is no strong evidence from clinical trials, early surveillance and identification of cardiotoxicity, timely discontinuation of ICI, and the use of corticosteroid therapy are essential to improve the prognosis (13). Therefore, this study conducted a network meta-analysis to compare the risk of cardiotoxicity with different combinations of ICI therapies and to provide a reference for the safety of clinical treatment with ICI.
The incidence and mortality of cardiotoxicity of different ICI therapies were analyzed. The primary objective was to compare the risk of Grade 1-5 cardiotoxicity and Grade 3-5 cardiotoxicity associated with different ICI therapies. The secondary objective was to compare the risk of Grade 1-5 myocarditis and Grade 3-5 myocarditis associated with different ICI therapies. Subgroup analyses were performed to evaluate the impact of tumour type and doses of ICI.
This network meta-analysis was conducted according to PRISMA guidelines (14). The RCTs comparing all ICI (alone or in combination with standard therapy) with other non-ICI therapy or single-agent ICI with double-agent ICI was comprehensively searched in PubMed, Embase, Web of Science, Cochrane Library and ClincalTrials.gov from January 1, 2014 to January 1, 2022. The keywords included “PD‐1”, “PD-L1”, “immune checkpoint inhibitors”, “atezolizumab”, “avelumab”, “durvalumab”, “ipilimumab”, “nivolumab”, “pembrolizumab”, “camrelizumab”, “tremelimumab”, “toripalimab”, “sintilimab”, “cancer” (Supplementary Table 1).
The inclusion criteria for this study were as follows (1): Patients: those with cancer whether they received basic treatment or not (2); Interventions: the treatment group received ICI, either alone or in combination. The control group received placebo, non-ICI based therapy or different doses of the same ICI (3); Study type: randomized controlled trials, the language was limited to English (4); Outcomes: we used common terminology criteria for adverse events (CTCAE) version 5 to determine cardiotoxicity. We excluded (1): single-arm trials (2); studies with incomplete data or without full text available.
Two researchers (XTC and RPN) independently extracted the data and evaluated the quality of the included studies. If there was any disagreement, a consensus should be reached after consultation with a third reviewer (JY). For each eligible study, the extracted data included (1): study information (first author, study name, publication year, National Clinical Trial number) (2); patient baseline information (sample size, median age, follow-up, tumor type, lines of treatment) (3); treatment group and control group (treatments in each arm, type of ICI, cardiovascular adverse events in each arm).
Risk of bias assessment was undertaken by two independent investigators (XTC and RPN) using RevMan5.4 software, according to the RCT bias risk assessment tool recommended by the Cochrane Collaboration Handbook (15).
Stata version17.0 software was used. Freeman Tukey double inverse sine transform method was used to calculate the incidence and mortality of cardiotoxicity. We used risk ratios (RRs) and 95% confidence intervals (95% CI) as summary statistics to quantify the risk of cardiotoxicity. The network meta-analysis was undertaken by the Frequency model (16). The data were fitted with an inconsistent model and a consistent model respectively to test the overall consistency. The node splitting method and ring inconsistency test were utilized to test the local consistency. If the P-value > 0.05, it indicated that there was no inconsistency, and the consistency model could be employed for analysis (17). The surface under the cumulative ranking (SUCRA) curve was calculated to rank the cardiotoxicity with different regimens. The smaller the SUCRA, the greater the risk of cardiotoxicity and the lower the safety (18). The publication bias and small sample effect were detected by Begg’ test.
8,589 related literatures were obtained after a comprehensive search. Finally, 91 studies (52,247 subjects) were included, after reading 158 potential studies (Figure 1). The characteristics of the included studies is show in Supplementary Table 2. All RCTs reported Grade 1-5 cardiotoxicity. 75 RCTs reported Grade 3-5 cardiotoxicity. 36 RCTs reported Grade 1-5 myocarditis, and 24 RCTs reported Grade 3-5 myocarditis. The included studies involved 17 cancer types, of which lung cancer accounted for 33%. Among the 91 articles, 58% compared ICI to chemotherapy. The network plot of Grade 1-5 cardiotoxicity is shown in Figure 2.
Figure 2 Network plots for Grade 1-5 cardiotoxicity. The area of the circle represents the sample size of the treatment measure, the line represents the study and the line thickness represents the number of studies.
Assess risk bias according to the Cochrane Collaboration Handbook, and all included studies had a low risk of bias. We utilized the Begg’s test to evaluate the publication bias. The results are as follows: Grade 1-5 cardiotoxicity (P=0.696)>0.05, Grade 3-5 cardiotoxicity (P=0.547)>0.05, Grade 1-5 myocarditis (P=0.139)>0.05, and Grade 3-5 myocarditis (P=0.088)>0.05. The scatter distribution on the Begg funnel is basically symmetrical, suggesting that there was no significant publication bias of the included studies (Supplementary Figure 1).
The incidence of Grade 1-5 cardiotoxicity, Grade 3-5 cardiotoxicity, Grade 1-5 myocarditis and Grade 3-5 myocarditis were 3.23% (839/25998), 0.97% (220/22718), 0.35% (43/12270) and 0.33% (25/7528), respectively. Different therapies had separate incidences of cardiotoxicity. The incidence of Grade 1-5 cardiotoxicity, Grade 3-5 cardiotoxicity, Grade 1-5 myocarditis and Grade 3-5 myocarditis with PD-1 + Chemo, PD-1 + TTD and PD-1 + CTLA-4 were relatively high. The highest incidences of Grade 1-5 cardiotoxicity, Grade 3-5 cardiotoxicity, Grade 1-5 myocarditis and Grade 3-5 myocarditis were from PD-1 + TTD, up to 6.39% (95% CI: 3.45-10.14), 4.10% (95% CI: 3.00-5.40), 1.10% (95% CI: 0.56-1.82) and 0.93% (95% CI: 0.44-1.60) respectively (Figure 3).
Figure 3 Incidence of cardiotoxicity among different therapies. (A): Incidence of Grade 1-5 cardiotoxicity among different therapies, (B): Incidence of Grade 3-5 cardiotoxicity among different therapies, (C): Incidence of Grade 1-5 myocarditis among different therapies, (D): Incidence of Grade 3-5 myocarditis among different therapies.
A total of 66 fatal cardiovascular adverse events occurred (17 cardiac arrest, 12 myocardial infarction, 12 cardiac failure, 8 myocarditis, 17 others). The combined mortality was calculated by using the random effect model Freeman Tukey double inverse sine conversion. The total mortality of cardiotoxicity with ICI was 0.55% (95% CI: 0.40%-0.73%). The highest mortality of cardiotoxicity with PD-1 + TTD was 1.84% (95% CI: 0.30%-4.68%), followed by PD-1 + CTLA-4 1.55% (95% CI: 0.06%-5.02%). There was no significant difference between ICI therapy and non-ICI therapy (RR=1.35, 95% CI: 0.91-2.01) (Table 1).
Overall, ICI treatment increased the risk of cardiotoxicity compared with non-ICI treatment (Grade 1-5 cardiotoxicity: RR = 1.45, 95% CI: 1.26-1.65; Grade 3-5 cardiotoxicity: RR = 1.55, 95% CI: 1.21-2.00; Grade 1-5 myocarditis: RR = 2.58, 95% CI: 1.54-4.31; Grade 3-5 myocarditis: RR = 2.76, 95% CI: 1.45-5.24; Figures 4, 5). For Grade 1-5 cardiotoxicity, PD-1 (RR=2.03, 95% CI: 1.16-3.56) and PD-L1 (RR=3.12, 95% CI: 3.12-7.40) were statistically significant compared with placebo, but CTLA-4 showed no significant difference. PD-L1 (RR=1.70, 95% CI: 1.04-2.77) and PD-1+ CTLA-4 (RR=2.82, 95% CI: 1.08-7.34) were statistically significant compared with chemotherapy. PD-1 + TTD (RR=1.94, 95% CI: 1.18-3.18) was statistically significant compared with TTD. For Grade 3-5 cardiotoxicity, there was no significant difference between PD-1 and PD-L1 compared with placebo, whereas CTLA-4 (RR=2.34, 95% CI: 1.26-4.36) was statistically significant. PD-1 + TTD (RR=2.20, 95% CI: 1.29-3.75) was statistically significant compared with TTD. There was no significant difference between the other group treated with ICI and the control group. For Grade 1-5 myocarditis and Grade 3-5 myocarditis, only PD-1 + TTD vs. TTD was statistically significant (RR=5.67, 95% CI: 1.25-25.67; RR=4.71, 95% CI: 1.02-21.85).
Figure 4 Results of traditional pairwise meta-analysis for Grade 1-5 cardiotoxicity and Grade 3-5 cardiotoxicity. (A): Forestplot for Grade 1-5 cardiotoxicity, (B): Forestplot for Grade 3-5 cardiotoxicity, N: mumber of head to head comparison groups, NA, no available, a, Tau2 represents between-study heterogeneity characterized by standard deviation; b, Bold values denote statistical significance.
Figure 5 Results of traditional pairwise meta-analysis for Grade 1-5 myocarditis and Grade 3-5 myocarditis. (A): Forestplot for Grade 1-5 myocarditis, (B): Forestplot for Grade 3-5 myocarditis, N: mumber of head to head comparison groups, NA, no available; a, Tau2 represents between-study heterogeneity characterized by standard deviation; b, Bold values denote statistical significance.
In the sensitivity analysis, the pooled RR estimates were stable, with only minimal fluctuations when excluding each study sequentially.
We ranked the probabilities of cardiovascular adverse events for all treatments by estimating the SUCRA value (Figure 6). Detailed probabilities of cardiovascular adverse events are showed in Supplementary Figure 2. A lower SUCRA value indicated a higher probability of cardiotoxicity. For Grade 1-5 cardiotoxicity, the corresponding ranking of incidences of the twelve groups from high to low was: PD-L1 + CTLA-4 (2.1%), PD-1 + CTLA-4 (22.6%), PD-1 + TTD (25.6%), PD-L1 (29.3%), PD-L1+TTD (33.2%), PD-1+Chemo (46.4%), PD-1 (55.2%), CTLA-4 (66.9%), PD-L1+Chemo (71.2%), Chemo (73.3%), TTD (76.5%), Placebo (97.5%). For Grade 3-5 cardiotoxicity, the corresponding ranking of incidences of the twelve groups from high to low was: PD-1+TTD (11.1%), PD-1+CTLA-4 (33.0%), TTD (33.1%), PD-L1+CTLA-4 (35.4%), CTLA-4 (41.5%), PD-1+Chemo (45.0%), PD-L1+TTD (45.0%), PD-1 (54.3%), PD-L1(58.2%), Chemo (72.5%), Placebo (80.1%), PD-L1+Chemo (90.9%). For Grade 1-5 myocarditis, the corresponding ranking of incidences of the twelve groups from high to low was: PD-1+CTLA-4 (20.9%), PD-1+TTD (28.3%), CTLA-4 (37.0%), PD-1 (45.4%), PD-1+Chemo (46.7%), PD-L1 (47.5%), Placebo (49.7%), PD-L1+CTLA-4 (52.6%), Chemo (61.8%), PD-L1+Chemo (65.7%), PD-L1+TTD (65.8%), TTD (78.4%). For Grade 3-5 myocarditis, the corresponding ranking of incidences of the twelve groups from high to low was: PD-1+TTD (21.9%), PD-1+CTLA-4 (27.4%), CTLA-4 (36.8%), PD-1+Chemo (47.4%), Placebo (48.0%), PD-1 (51.0%), PD-L1+CTLA-4 (55.7%), PD-L1+TTD (60.0%), Chemo (60.4%), PD-L1 (61.4%), TTD (64.4%), PD-L1+Chemo (65.6%).
Figure 6 Rankings of SUCRA for the risk of cardiotoxicity. (A) Grade 1-5 cardiotoxicity, (B) Grade 3-5 cardiotoxicity, (C) Grade 1-5 myocarditis, (D) Grade 3-5 myocarditis. SUCRA, surface under the cumulative ranking.
There was not any significant difference in terms of the risk of Grade 1-5 cardiotoxicity between different ICI monotherapy. In addition, the risk of Grade 1-5 cardiotoxicity in ICI monotherapy was not higher than that in single chemotherapy or single targeted therapy, except PD-L1 vs. Chemo (RR=1.53, 95% CI: 1.06-2.20). According to the ranking of SUCRA, PD-L1 + CTLA-4 ranked first among all therapies inducing Grade 1-5 cardiotoxicity, and the differences were significant except PD-1 + CTLA-4, PD-1 + TTD and PD-L1 + TTD (PD-1: RR=0.35, 95% CI: 0.17-0.72; PD-L1: RR=0.48, 95% CI: 0.26-0.89; CTLA-4: RR=3.19, 95% CI: 1.46-6.96; PD-1+Chemo: RR=0.42, 95% CI: 0.20-0.87; PD-L1 + TTD: RR=2.54, 95% CI: 1.15-5.61; TTD: RR=3.52, 95% CI: 1.54-8.05; Chemo: RR=3.19, 95% CI: 1.61-6.32; Table 2). PD-1 + CTLA-4 ranked first for Grade 1-5 myocarditis, PD-1 + TTD ranked first for Grade 3-5 cardiotoxicity and Grade 3-5 myocarditis, but there was no significant difference between other therapies except PD-1 + TTD vs. TTD (Table 3).
Table 2 Network estimates of treatment comparisons for Grade 1-5 cardiotoxicity (on the lower triangle) and Grade 3-5 cardiotoxicity (on the upper triangle).
Table 3 Network estimates of treatment comparisons for Grade 1-5 myocarditis (on the lower triangle) and Grade 3-5 myocarditis (on the upper triangle).
The subgroup analysis for Grade 1-5 cardiotoxicity by cancer types and does of ICI. There was no significant difference in cardiotoxicity with different therapies for different cancer types (Supplementary Table 3). In the subgroup analysis based on dose (Supplementary Figure 3), compared with targeted therapy, Atezolizumab 1200 mg (q3w) treatment regimen increased the risk of cardiotoxicity (RR = 2.39, 95% CI: 1.04-5.49), while Atezolizumab 840 mg (q2w) regimen did not (RR = 0.51, 95% CI: 0.55-5.68). Through indirect comparison, there is no significant difference between Atezolizumab 1200 mg (q3w) and 840 mg (q2w) (RR = 0.44, 95% CI: 0.15-1.32).
In the point splitting method and ring inconsistency test (Supplementary Figure 4), the P values of the four outcome indicators were greater than 0.05, suggesting that there was no inconsistency in the whole and local.
In our systematic review and network meta-analysis, we analyzed the cardiotoxicity risk of different cancer treatment regimens and the effects of different cancer types or different doses of ICI on cardiotoxicity. Currently, whether ICI therapies will increase the risk of cardiotoxicity remains controversial. Some studies have shown that ICI treatment is not associated with a higher risk of cardiotoxicity (19, 20). However, this result may be due to the low incidence of ICI related cardiotoxicity and the limited analysis data included. In our study, we performed a comprehensive analysis of 91 RCTs (including 52247 patients and 12 treatment arms) and found that the use of ICI was associated with a higher risk of cardiotoxicity compared with non-ICI treatments. At present, the mechanism of immune related cardiotoxicity is unclear, but studies have shown that ICI blocking CTLA-4, PD-1 and PD-L1 signaling pathways may be one of the causes of autoimmune heart disease (21, 22).
For comparison among single ICI therapies, studies have shown that the incidence of high-grade toxicity in patients with metastatic melanoma receiving ipilimumab 10mg/kg is 57.9%. In contrast, PD-1 or PD-L1 caused high-grade adverse events in only 10%-15% of patients (23, 24). In our study, it was also found that CTLA-4 seems to be associated with Grade 3-5 cardiotoxicity compared with PD-1 and PD-L1, but the mechanism remains unclear.
For comparison of dual ICI therapy with single therapy, the risk of cardiotoxicity was higher with dual ICI therapy than with chemotherapy, targeted therapy and single ICI therapy. Based on the evidence network, the risk of cardiotoxicity with PD-L1 + CTLA-4 was higher than that with PD-1,PD-L1 and CTLA-4. The risk of cardiotoxicity of PD-1 + CTLA-4 is only higher than that with PD-1. Although PD-1 + CTLA-4 vs. PD-1, PD-L1 + CTLA-4 vs. PD-L1 and PD-L1 + CTLA-4 vs. CTLA-4 are not statistically significant in the traditional meta-analysis, this may be due to few included studies in this comparison groups and subsequently wide confidence intervals. According to the consistency of network meta-analysis, the results of network meta-analysis still have credibility (25).
For comparison among dual therapies, except that the risk of cardiotoxicity of PD-L1 + CTLA-4 was significantly higher than that of PD-L1 + Chemo, there was no significant difference in the risk of cardiotoxicity between dual ICI therapy and single ICI combined with chemotherapy or targeted therapy. Studies have shown that the incidence of cardiotoxicity is higher with dual ICI therapy than the incidence treated with ICI monotherapy, either as single treatment or in combination with chemotherapy (26). The explanation of the increased cardiotoxicity of dual ICI therapy likely obeys the different signaling pathways inhibited by PD-1 and CTLA-4 molecules on T Cells (27). On the one hand, it prevents the binding of CTLA-4 and B7 molecules. On the other hand, it blocks the inhibitory signal produced by the binding of PD-1 with PD-L1 (28, 29).
Although this study did not find significant differences in the risk of myocarditis between different ICI treatments, special attention should be paid to dual ICI therapy. It has been reported that the risk of myocarditis in patients treated with ICI therapy increases dramatically and dual ICI therapy has a higher incidence of myocarditis, with more serious symptoms and higher mortality (30). The mechanism of ICI-related myocarditis is unclear, but several preclinical studies have made some assumptions, such as inflammation caused by over activation of T cells (21, 22). The specific mechanism behind it needs to be further studied.
Of the 52,247 treated patients, 66 cardiovascular-related fatal events were reported. Cardiovascular iRAEs occur with an incidence of 1.14%–5%, but have the highest mortality rate among iRAEs up to 50% (31). The mortality of ICI-related cardiotoxicity cannot be ignored. In our study, there was no significant difference in the mortality of cardiotoxicity between the groups treated with ICI and the control group. The mortality of cardiotoxicity with PD-1 + TTD was the highest (1.84%). However, due to the rarity of cardiovascular-related fatal events with ICI and the difference in the number of therapies included in the study, this conclusion was uncertain. Although we did not find that the risk of cardiotoxicity was related to cancer type and dose of ICI. Previous studies indicated that the occurrence of irAEs induced by PD-1/PD-L1 agents is related to cancer types and is dose-independent (32, 33). So far, the mechanism underlying this result has not been well elucidated.
Based on the current network evidence, we suggest that patients at high risk of cardiotoxicity (such as pre-existing cardiovascular conditions, previous and concomitant cardiotoxic treatments) with monotherapy choose PD-1/PD-L1 or appropriately reduce the dose of CTLA-4 within the effective dose range. For patients with no response to monotherapy or poor cardiac function, it is recommended to give priority to single ICI combined with chemotherapy.
There are two limitations in the network meta-analysis of this study. One limitation is that the incidence of cardiotoxicity with ICI is low. The trials included single-arm zero events and double-arm zero events, which may interfere with the statistical inference of the effect size and complicate the calculation. However, if the studies of double-arm zero events are excluded from the meta-analysis, the estimation of the overall effect size may be biased (34). This study did not include double-arm zero events, which may have a certain impact on the results. Another limitation is that we only classified and compared the drug categories of the treatment group and the control group, and did not identify the drugs in detail, which may affect the accuracy of the result analysis.
Our study has shown that different ICI treatment regimens show differents in the risk of cardiotoxicity. For ICI monotherapy, CTLA-4 may be linked to higher levels of cardiotoxicity than PD-1 or PD-L1. For dual therapy, the risk of cardiotoxicity associated with dual ICI therapy was higher than that with single chemotherapy or single targeted therapy. This study also gives evidence for the incidence and risk ratio of ICI-related cardiotoxicity and provide reference for individualized treatment of cancer patients.
Concept and Design: JY, XC and ML. Collection and assembly of data: XC and RN. Data analysis and interpretation: JY, XC, RN and BZ. Manuscript writing: ML, JY and XC. Manuscript revision: ML and SH. All authors contributed to the article and approved the submitted version.
This work was supported by The Startup Fund for Scientific Research, Fujian Undergraduate Education and teaching reform research Major project (Grant number: FBJG20210119), Fujian Provincial health technology project (Grant number: Fujian Provincial health technology project) and Natural Science Foundation of Fujian Province (Grant number: 2021J011304). The Joint Fund for the Innovation of Science and Technology, Fujian Province (Grant number 2018Y9037).
The authors declare that the research was conducted in the absence of any commercial or financial relationships that could be construed as a potential conflict of interest.
All claims expressed in this article are solely those of the authors and do not necessarily represent those of their affiliated organizations, or those of the publisher, the editors and the reviewers. Any product that may be evaluated in this article, or claim that may be made by its manufacturer, is not guaranteed or endorsed by the publisher.
The Supplementary Material for this article can be found online at: https://www.frontiersin.org/articles/10.3389/fimmu.2022.1006860/full#supplementary-material
Chemo, chemotherapy; CTLA-4, anti-cytotoxic T-lymphocyte antigen-4; CTCAEs, common terminology criteria for adverse events; CI(s), confidence interval(s); ICI(s), immune checkpoint inhibitor(s); irAEs, immune-related adverse events; PD-1, anti-programmed cell death 1; PD-L1, anti-programmed cell death ligand 1; RCTs, randomized clinical trials; RR(s), relative risk(s); SUCRA, surface under the cumulative ranking; TTD, targeted therapy drug.
1. Liu Z, Han C, Fu YX. Targeting innate sensing in the tumor microenvironment to improve immunotherapy. Cell Mol Immunol (2020) 17(1):13–26. doi: 10.1038/s41423-019-0341-y
2. Krummel MF. CTLA-4 engagement inhibits IL-2 accumulation and cell cycle progression upon activation of resting T cells. J Exp Med (1996) 183(6):2533–40. doi: 10.1084/jem.183.6.2533
3. Boussiotis VA. Molecular and biochemical aspects of the PD-1 checkpoint pathway. N Engl J Med (2016) 375(18):1767–78. doi: 10.1056/NEJMra1514296
4. Joseph A, Simonaggio A, Stoclin A, Vieillard-Baron A, Geri G, Oudard S, et al. Immune-related adverse events: a retrospective look into the future of oncology in the intensive care unit. Ann Intensive Care (2022) 10(1):143. doi: 10.1186/s13613-020-00761-w
5. Master SR, Robinson A, Mills GM, Mansour RP. Cardiovascular complications of immune checkpoint inhibitor therapy. J Clin Oncol (2019) 37(15):2568–8. doi: 10.1200/JCO.2019.37.15_suppl.2568
6. Lyon AR, Yousaf N, Battisti NML, Moslehi J, Larkin J. Immune checkpoint inhibitors and cardiovascular toxicity. Lancet Oncol (2018) 19(9):e447–e58. doi: 10.1016/S1470-2045(18)30457-1
7. Salem JE, Manouchehri A, Moey M, Lebrun-Vignes B, Bastarache L, Pariente A, et al. Cardiovascular toxicities associated with immune checkpoint inhibitors: an observational, retrospective, pharmacovigilance study. Lancet Oncol (2018) 19(12):1579–89. doi: 10.1016/S1470-2045(18)30608-9
8. Wang DY, Salem JE, Cohen JV, Chandra S, Menzer C, Ye F, et al. Fatal toxic effects associated with immune checkpoint inhibitors: A systematic review and meta-analysis. JAMA Oncol (2018) 4(12):1721–8. doi: 10.1001/jamaoncol.2018.3923
9. Moslehi JJ, Salem JE, Sosman JA, Lebrun-Vignes B, Johnson DB. Increased reporting of fatal immune checkpoint inhibitor-associated myocarditis. Lancet (2018) 391(10124):933. doi: 10.1016/S0140-6736(18)30533-6
10. Vilgelm AE, Johnson DB, Richmond A. Combinatorial approach to cancer immunotherapy: strength in numbers. J Leukoc Biol (2016) 100(2):275–90. doi: 10.1189/jlb.5RI0116-013RR
11. Galluzzi L, Humeau J, Buqué A, Zitvogel L, Kroemer G. Immunostimulation with chemotherapy in the era of immune checkpoint inhibitors. Nat Rev Clin Oncol (2020) 17(12):725–41. doi: 10.1038/s41571-020-0413-z
12. Sharma P, Siddiqui BA, Anandhan S, Yadav SS, Subudhi SK, Gao J, et al. The next decade of immune checkpoint therapy. Cancer Discovery (2021) 11(4):838–57. doi: 10.1158/2159-8290.CD-20-1680
13. Zhang L, Zlotoff DA, Awadalla M, Mahmood SS, Nohria A, Hassan MZO, et al. Major adverse cardiovascular events and the timing and dose of corticosteroids in immune checkpoint inhibitor-associated myocarditis. Circulation (2020) 141(24):2031–4. doi: 10.1161/CIRCULATIONAHA.119.044703
14. Liberati A, Altman DG, Tetzlaff J, Mulrow C, Gøtzsche PC, Ioannidis JPA, et al. The PRISMA statement for reporting systematic reviews and meta-analyses of studies that evaluate health care interventions: explanation and elaboration. PLoS Med (2009) 6(7):e1000100. doi: 10.1371/journal.pmed.1000100
15. Higgins JP, Altman DG, Gotzsche PC, Juni P, Moher D, Oxman AD, et al. The cochrane collaboration's tool for assessing risk of bias in randomised trials. BMJ (2011) 343:d5928. doi: 10.1136/bmj.d5928
16. Bucher HC, Guyatt GH, Griffith LE, Walter SD. The results of direct and indirect treatment comparisons in meta-analysis of randomized controlled trials. J Clin Epidemiol (1997) 50(6):683–91. doi: 10.1016/s0895-4356(97)00049-8
17. Donegan S, Williamson P, D'Alessandro U, Tudur Smith C. Assessing key assumptions of network meta-analysis: A review of methods. Res Synth Methods (2013) 4(4):291–323. doi: 10.1002/jrsm.1085
18. Tonin FS, Rotta I, Mendes AM, Pontarolo R. Network meta-analysis: a technique to gather evidence from direct and indirect comparisons. Pharm Pract (Granada) (2017) 15(1):943. doi: 10.18549/PharmPract.2017.01.943
19. Rahouma M, Karim NA, Baudo M, Yahia M, Kamel M, Eldessouki I, et al. Cardiotoxicity with immune system targeting drugs: a meta-analysis of anti-PD/PD-L1 immunotherapy randomized clinical trials. Immunotherapy (2019) 11(8):725–35. doi: 10.2217/imt-2018-0118
20. Agostinetto E, Eiger D, Lambertini M, Ceppi M, Bruzzone M, Ponde N, et al. Cardiotoxicity of immune checkpoint inhibitors: A systematic review and meta-analysis of randomised clinical trials. Eur J Cancer (2021) 148:76–91. doi: 10.1016/j.ejca.2021.01.043
21. Lichtman AH. The heart of the matter: protection of the myocardium from T cells. J Autoimmun (2013) 45:90–6. doi: 10.1016/j.jaut.2013.05.004
22. Tarrio ML, Grabie N, Bu DX, Sharpe AH, Lichtman AH. PD-1 protects against inflammation and myocyte damage in T cell-mediated myocarditis. J Immunol (2012) 188(10):4876–84. doi: 10.4049/jimmunol
23. Eggermont AMM, Blank CU, Mandala M, Long GV, Atkinson VG, Dalle S, et al. Longer follow-up confirms recurrence-free survival benefit of adjuvant pembrolizumab in high-risk stage III melanoma: Updated results from the EORTC 1325-MG/KEYNOTE-054 trial. J Clin Oncol (2021) 38(33):3925–36. doi: 10.1200/JCO.20.02110
24. Weber J, Mandala M, Del Vecchio M, Gogas HJ, Arance AM, Cowey CL, et al. Adjuvant nivolumab versus ipilimumab in resected stage III or IV melanoma. N Engl J Med (2017) 377(19):1824–35. doi: 10.1056/NEJMoa1709030
25. Nikolakopoulou A, Mavridis D, Furukawa TA, Cipriani A, Tricco AC, Straus SE, et al. Living network meta-analysis compared with pairwise meta-analysis in comparative effectiveness research: empirical study. BMJ (2018) 360:k585. doi: 10.1136/bmj.k585
26. Rubio-Infante N, Ramírez-Flores YA, Castillo EC, Lozano O, García-Rivas G, Torre-Amione G. Cardiotoxicity associated with immune checkpoint inhibitors therapy: A meta-analysis. Eur J Heart Fail (2021) 23(10):1739–47. doi: 10.1002/ejhf.2289
27. Rubio-Infante N, Ramirez-Flores YA, Castillo EC, Lozano O, Garcia-Rivas G, Torre-Amione G. A systematic review of the mechanisms involved in immune checkpoint inhibitors cardiotoxicity and challenges to improve clinical safety. Front Cell Dev Biol (2022) 10:851032. doi: 10.3389/fcell.2022.851032
28. Lee HT, Lee JY, Lim H, Lee SH, Moon YJ, Pyo HJ, et al. Molecular mechanism of PD-1/PD-L1 blockade via anti-PD-L1 antibodies atezolizumab and durvalumab. Sci Rep (2017) 7(1):5532. doi: 10.1038/s41598-017-06002-8
29. Hui E, Cheung J, Zhu J, Su X, Taylor MJ, Wallweber HA, et al. T Cell costimulatory receptor CD28 is a primary target for PD-1-mediated inhibition. Science (2016) 355(6332):1428–33. doi: 10.1126/science.aaf1292
30. Puzanov I, Diab A, Abdallah K, Bingham CO, Brogdon C, Dadu R, et al. Managing toxicities associated with immune checkpoint inhibitors: consensus recommendations from the society for immunotherapy of cancer (SITC) toxicity management working group. J Immunother Cancer (2017) 5(1):95. doi: 10.1186/s40425-017-0300-z
31. Stein-Merlob AF, Rothberg MV, Ribas A, Yang EH. Cardiotoxicities of novel cancer immunotherapies. Heart (2021) 107(21):1694–703. doi: 10.1136/heartjnl-2020-318083
32. Wang PF, Chen Y, Song SY, Wang TJ, Ji WJ, Li SW, et al. Immune-related adverse events associated with anti-PD-1/PD-L1 treatment for malignancies: A meta-analysis. Front Pharmacol (2017) 8:730. doi: 10.3389/fphar.2017.00730
33. Michot JM, Bigenwald C, Champiat S, Collins M, Carbonnel F, Postel-Vinay S, et al. Immune related adverse events with immune checkpoint blockade: a comprehensive review. Eur J Cancer (2016) 54:139–48. doi: 10.1016/j.ejca.2015.11.016
Keywords: cancer, cardiovascular adverse events, myocarditis, immune checkpoint inhibitors, network meta-analysis
Citation: Liu M, Cheng X, Ni R, Zheng B, Huang S and Yang J (2022) Cardiotoxicity of immune checkpoint inhibitors: A frequency network meta-analysis. Front. Immunol. 13:1006860. doi: 10.3389/fimmu.2022.1006860
Received: 29 July 2022; Accepted: 26 August 2022;
Published: 14 September 2022.
Edited by:
Ping-Yen Liu, National Cheng Kung University, TaiwanReviewed by:
Mu Shiang Huang, National Cheng Kung University Hospital, TaiwanCopyright © 2022 Liu, Cheng, Ni, Zheng, Huang, Yang. This is an open-access article distributed under the terms of the Creative Commons Attribution License (CC BY). The use, distribution or reproduction in other forums is permitted, provided the original author(s) and the copyright owner(s) are credited and that the original publication in this journal is cited, in accordance with accepted academic practice. No use, distribution or reproduction is permitted which does not comply with these terms.
*Correspondence: Jing Yang, ODI1NTgwMTI1QHFxLmNvbQ==
†These authors have contributed equally to this work
Disclaimer: All claims expressed in this article are solely those of the authors and do not necessarily represent those of their affiliated organizations, or those of the publisher, the editors and the reviewers. Any product that may be evaluated in this article or claim that may be made by its manufacturer is not guaranteed or endorsed by the publisher.
Research integrity at Frontiers
Learn more about the work of our research integrity team to safeguard the quality of each article we publish.