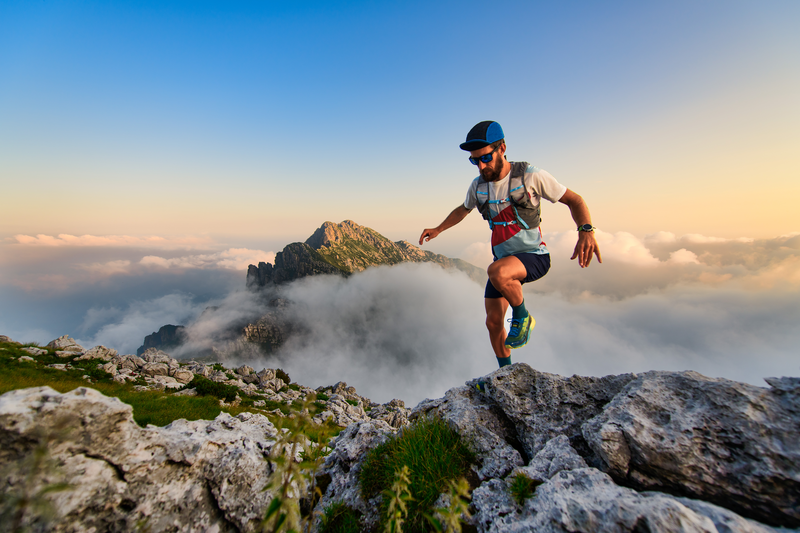
94% of researchers rate our articles as excellent or good
Learn more about the work of our research integrity team to safeguard the quality of each article we publish.
Find out more
REVIEW article
Front. Immunol. , 24 October 2022
Sec. Inflammation
Volume 13 - 2022 | https://doi.org/10.3389/fimmu.2022.1006434
Neuroinflammation is the fundamental immune response against multiple factors in the central nervous system and is characterized by the production of inflammatory mediators, activated microglia and astrocytes, and the recruitment of innate and adaptive immune cells to inflammatory sites, that contributes to the pathological process of related brain diseases, such as Alzheimer’s disease, Parkinson’s disease, depression, and stroke. Flavonoids, as a species of important natural compounds, have been widely revealed to alleviate neuroinflammation by inhibiting the production of pro-inflammatory mediators, elevating the secretion of anti-inflammatory factors, and modulating the polarization of microglia and astrocyte, mainly via suppressing the activation of NLRP3 inflammasome, as well as NF-κB, MAPK, and JAK/STAT pathways, promoting Nrf2, AMPK, BDNF/CREB, Wnt/β-Catenin, PI3k/Akt signals and SIRT1-mediated HMGB1 deacetylation. This review will provide the latest and comprehensive knowledge on the therapeutic benefits and mechanisms of natural flavonoids in neuroinflammation, and the natural flavonoids might be developed into food supplements or lead compounds for neuroinflammation-associated brain disorders.
Neuroinflammation generally refers to a complex immune response in the central nervous system (CNS) to various endogenous or exogenous stimuli, such as misfolded proteins, toxins and pathogen, leading to brain tissue inflammatory cell infiltration, gliosis, neuronal loss, etc. (1). Pro-inflammatory mediators, produced by microglia, astrocytes and other immune cells in the process of neuroinflammation, repress the differentiation and trigger apoptosis and necroptosis of neurons, increase the production of excitatory neurotransmitters and inhibit the transmission of monoamine neurotransmitter, ultimately resulting in neuronal degeneration (2–4). And amyloid-β (Aβ), tau, α-synuclein and other misfolded proteins aggregate in neurons or intercellular neurons during inflammation, that forms neurofibrillary tangles and senile plaques in cerebral cortex and hippocampus, and generates Lewy bodies in substantia nigra pars compacta (5, 6). Furthermore, the integrity of tight junctions of endothelial cells and the components of basal lamina are degraded in inflammatory states, which augments the permeability of blood-brain barrier (BBB) and subsequently leads leukocytes invading the brain parenchyma (7, 8). Therefore, intervening neuroinflammation may be an important strategy to the treatment of brain disorders.
The roles of neuroinflammation are different, normally, inflammation is a defense mechanism that initially protects the brain via clearing up pathogens, cell fragments, mis-folded proteins and other stimulus to maintain or restore the integrity of tissues (9). Nevertheless, uncontrolled neuroinflammation engenders neuronal degeneration and BBB disruption, that is marked by the secretion of pro-inflammatory cytokines, chemokines, and small-molecule messengers, which are primarily released by activated microglia and astrocytes (10).
Microglia are macrophages derived from erythromyeloid pro-genitors in the yolk sac, and exhibit a wide array of functions that include regulation of programmed cell death of neurons, striping excess synapses from developing neurons and promotion of neurite formation (11). With the change of brain microenvironment, microglia, like peripheral macrophages, are activated by various inflammatory stimuli, and polarize into classical M1 type and alternative M2 type (12). Specific as follows, M1 microglia are typically characterized by the secretion of pro-inflammatory cytokines and chemokines, such as IL-6, IL-1β, TNF-α, and MCP-1, bringing about unbridled and prolonged neuroinflammation (13). On the contrary, M2 microglia with the markers of Ym-1, FIZZ-1 and Arg-1, secrete anti-inflammatory cytokines, including IL-4, IL-10, and IL-13, to suppress inflammation (14).
Astrocytes are neural parenchymal cells derived from neural stem cells, and are able to regulate the extracellular balance of ions, fluid and transmitters, modulate cerebral blood flow and the formation and maintenance of the BBB (15). In the process of inflammation, astrocytes polarize into neurotoxic phenotype (A1), that is characterized by cellular hypertrophy, increased production of glial fibrillary acidic protein and complement, astrogliosis, and glial scars formation, in addition to pro-inflammatory factors secretion (16). Besides, A1 astrocytes directly influence vascular and perivascular cells leading to alterations in BBB permeability (17). In contrast, astrocytes are activated by protective factors in inflammation and polarize into neuroprotective phenotype (A2), that increase the release of neurotrophic factors, such as BDNF, NGF and VEGF, as well as thrombospondins like TSP-1, which promote outgrowth and survival of neurons (18, 19).
Cellular crosstalk among microglia, astrocytes and neurons poses feedback loops and brings maladjusted and self-magnifying neuroinflammation. Normally, astrocytes offer nutritional support molecules for microglia to promote their morphological and functional stability, while in the process of neuroinflammation, A1 astrocytes release inflammatory mediators and increase the permeability of BBB to activate M1 microglia accompanied by an enhanced ability to migrate (20, 21). Meanwhile, molecular factors secreted by M1 microglia also polarize astrocytes into A1 state inducing astrocytosis and the secretion of neurotoxic factors (22). Furthermore, proinflammatory mediators released by A1/M1 directly initiate neuronal apoptosis and necroptosis, and due to the decrease in A1/M1 uptake capacity, intercellular excitatory transmitters such as glutamate are increased, resulting in neuronal excitotoxicity (23, 24). Conversely, A2/M2 secrete anti-inflammatory cytokines, neurotrophic factors and other protective mediators to inhibit neuroinflammation and promote neuronal generation and survival (25). Therefore, the functional changes of microglia and astrocytes affect neuronal function and central nervous immune system, and bring about the occurrence or aggravation of various brain diseases.
Overall, an increase of inflammatory mediators, polarization of microglia and astrocytes, and crosstalk among microglia, astrocytes and neurons, are the key factors for the occurrence and development of neuroinflammation, thereby, adjusting the above changes are effective strategies to treat neuroinflammation-related brain disorders.
Flavonoids, natural compounds with a basic structural unit of 2-phenylchromone, are widely present in herbs and various dietary sources, such as fruits, vegetables, tea, and cereal in the form of glycosides or free state. Human interventions and experimental studies have shown a role of natural flavonoids in brain diseases, as evidenced by the reduction in multiple pro-inflammatory mediators. Therefore, understanding the effects and mechanisms of flavonoids in anti-neuroinflammation would be highly valuable for developing nutritional guidelines and therapeutic strategies to related brain disorders.
Flavones and flavonols are important groups of flavonoids that are widely studied. Luteolin (Figure 1), a flavone compound, is found in various herbs, vegetables, and fruits, such as perilla leaf, peppermint, celery, carrot, and apple. Luteolin is reported to down-regulate the secretion of IL-1β, TNF-α, and IL-6 (26–28), suppress p65 and p38 phosphorylation in lipopolysaccharide (LPS)-induced C6 cells (26), inhibit nucleus p65, ASC, NLRP3, and cleaved-Caspase-1 protein expression, and increase Nrf2 protein in oxyhemoglobin-induced primary cortical neurons and glia cells (27, 28). In LPS, Aβ1-42 and triple-transgenic-induced AD mice, luteolin ameliorates behavior impairment, inhibits overproduction of pro-inflammatory mediators (26, 29–31), as well as restrains GFAP, p38 protein expression and the phosphorylation of JNK and p65 (26, 31). In other studies, luteolin is reported to decrease the release of pro-inflammatory mediators (27, 28), decrease TRAF6, TLR4, and p-p65 expression as well as TRAF6 ubiquitination in the brain of intracerebral hemorrhage (ICH)-induced rats (27), suppress NLRP3 proteins expression and increase the activity of Nrf2 in the brain of subarachnoid hemorrhage-induced rats (28). Therefore, luteolin might inhibit the excessive production of pro-inflammatory cytokines through regulating TLR4/TRAF6/NF-κB, MAPK, Nrf2 pathways and NLRP3 inflammasome to treat neuroinflammation-related brain diseases.
Baicalin (Figure 1) and baicalein (Figure 1) are rich in Scutellaria baicalensis Georgi, an edible medicinal plant. Various disease models, such as neurodegenerative diseases and encephalomyelitis have proved the anti-neuroinflammatory effects of baicalin and baicalein. In ischemia-reperfusion (I/R) and chronic unpredictable mild stress (CUMS)-induced mice, baicalin supplementation leads a reduction of IL-6, IL-1β, and TNF-α (32, 33), a trend of decreased TLR4 protein expression, and an increase of phosphorylation of PI3k, Akt and FoxO1 in the hippocampus of mice (33). Moreover, baicalin decreases IL-18 and iNOS levels (34–37), suppresses the protein expression of Iba-1, GFAP, TLR4, p-p65, p-IκBα, NLRP3, and cleaved-Caspase-1 in the hippocampus of APP/PS1 mice (37), reduces the production of HMGB1 and NF-κB, and elevates SIRT1 expression in the cerebral cortices and hippocampus from LPS-induced mice (34–36). Thus, baicalin possesses the ability to attenuate neuroinflammation via adjusting NLRP3 inflammasome and PI3k/Akt/FoxO1, SIRT1/HMGB1, and TLR4/NF-κB signaling pathways.
Baicalein, an aglycone of baicalin, is also widely studied in neuroinflammation. Baicalein inhibits microglia activation and polarization with decreasing TNF-α, iNOS, IL-1β, IL-6, CD16 and CD86 production, and enhancing Arg-1 and CD206 levels in LPS plus IFN-γ-induced BV2 cells through activating STAT1 expression and inhibiting TLR4/NF-κB pathway (38), and in ischemic penumbra from middle cerebral artery occlusion (MCAO)-induced rats through the inactivation of IκBα, JNK, ERK and p38, as well as nuclear translocation of p65 (38–40). In other studies, baicalein is reported to reduce IFN-γ, IL-5, and IL-12 secretion, as well as repress GFAP and Iba-1 expression in substantia nigra (SN) and midbrain from MPTP or rotenone-induced PD mice via downregulating cleaved-Caspase-1, cleaved-GSDMD, and NLRP3, as well as promoting PSD95, SYP, BDNF, p-TrkB, CREB, p-PI3k, p-Akt, and p-CaMK II expression (41, 42). In summary, baicalein restrain microglia activation and polarization through inhibiting NLRP3 inflammasome and regulating MAPKs, STAT1, TLR4/NF-κB, BDNF/TrkB/CREB signaling pathways.
Morin, 3,5,7,2’,4’-pentahydroxyflavone, is a bioactive flavonol compound that is extensively found in a variety of herbs, vegetables and fruits, like onion, orange, mulberries and almond hulls. Lots of researches have intensely demonstrated the anti-neuroinflammatory properties of morin (43, 44). Morin has been reported to decrease the secretion of NO, TNF-α, and IL-6, and suppress the protein expression of NF-κB in the striatum, prefrontal cortex and hippocampus from social defeat stress-induced mice (45) and in the hippocampus from Aβ1-42-induced AD rats (46). Besides, morin represses the production of nNOS and GFAP in brain of ifosfamide-induced neurotoxicity rats via decreasing the production of NF-κB and JNK, and increasing Nrf2 expression (47). These suggest that morin could suppress neuroinflammation via JNK, NF-κB and Nrf2 signaling pathways.
Quercetin (Figure 1), a natural flavonol is widely distributed in herbal medicines, fruits and vegetables, such as tea, apple, grape, and onion. In LPS-induced primary microglia or BV2 cells, quercetin decreases the ability of phagocytic, reduces the levels of inflammatory mediators including NO, TNF-α, IL-6, IL-1β, MCP-1, CXCL10, iNOS, COX-2, and lipocalin-2, and increases the secretion of IL-10 through activating AMPK and Nrf2 signaling pathways, as well as inhibiting NF-κB signaling pathway (48–50). In vivo studies, quercetin is reported to improve aging-, or LPS-induced behavior disorders, inhibit microglia and astrocytes activation, as well as decrease IL-1β levels via elevating SIRT1 protein expression and suppressing NLRP3, cleaved-Caspase-1 protein production in the brain of mice (49–51). Besides, in LPS-stimulated or traumatic brain injury rats, quercetin decreases the production of pro-inflammatory mediators in rat brain through suppressing NF-κB pathway, as well as initiating the Nrf2/HO-1 pathway (52). Thus, quercetin is a safe and effective dietary supplement to ameliorate neuroinflammation via increasing SIRT1 protein expression, inhibiting NLRP3 inflammasomes activation and adjusting NF-κB, Akt, AMPK, and Nrf2/HO-1 pathways.
Kaempferol (Figure 1), a dietary flavonol, presents in most plant-based foods, such as tea, broccoli, kale, cabbage and grapefruit, which has been described to possess resultful anti-neuroinflammatory effects. In LPS-induced BV2 cells, kaempferol reduces iNOS, IL-1β, IL-18, and TNF-α levels, suppresses CD32 production, and enhances Arg-1 and CD206 expression through down-regulating NLRP3, ASC, Caspase-1, p-p38 and p-NF-κB (53, 54). In vivo studies, kaempferol diminishes the production of COX-2, MCP-1, ICAM-1, IL-1β, IL-6, and TNF-α, and attenuates microglia activation in striatum of LPS-induced mice via suppressing the protein expression of HMGB1 and TLR4 (55), and in ischemic cortices from I/R rats through decreasing the phosphorylation and nuclear transposition of p65 (56). Kaempferol also decreases iNOS, COX-2 and IL-18 production through inhibiting NF-κB, p38 phosphorylation and NLRP3 inflammasome activation in the SN from PD rats induced by 6-hydroxydopamine (6-OHDA) (53). These researches suggest that kaempferol could regulate microglia polarization and reduce the pro-inflammatory mediators via suppressing NLRP3, HMGB1/TLR4, MAPKs, and NF-κB signaling pathways.
Icariin (Figure 1), a typical flavonol glycoside isolated from Epimedium brevicornu Maxim. has been studied to treat a variety of inflammation-related brain disorders. In LPS-treated glia, icariin down-regulates NO, TNF-α, IL-1β, IL-18, COX-2, and iNOS levels (57, 58). In MPTP- or 6-OHDA-induced PD mice, icariin alleviates dopaminergic neuronal damage, decreases the secretion of pro-inflammation cytokines and inhibits the protein expression of Iba-1 and GFAP in brain through suppressing NLRP3 inflammasome activation and promoting Nrf2, Keap1, HO-1 and NQO1 protein production (59, 60). In other study, icariin also reduces the levels of pro-inflammatory mediators including IFN-γ, MCP-1, IL-12, IL-17A, and GM-CSF in serum and brain of APP/PS1 mice (61). Thus, icariin shows great potentiality to attenuate neuroinflammation.
A natural flavonol glycoside, hyperoside (Figure 1) isolates from many herbs, such as Cuscuta chinensis Lam., Forsythia suspensa, and Crataegus pinnatifida Bge. Numerous studies have pointed out that hyperoside possesses anti-neuroinflammatory effects. Hyperoside supplement is reported to alleviate IL-1β, IL-6, IL-8, and TNF-α secretion through up-regulating SIRT1, Wnt1, β-Caspase, Shh, and Patch in LPS-treated HT22 cells (62). And in MPTP-induced PD mice, hyperoside reverses the motor dysfunction, reduces pro-inflammatory factors production and down-regulates Iba-1 and GFAP via reducing NLRP3, ASC, and P20 expression and increasing PACAP content and CREB phosphorylation in the SN (63). Furthermore, in streptozotocin plus high-fat diet-induced type 2 diabetic neuropathy rats, hyperoside alleviates cognitive dysfunction, and decrease the production of IL-1β, IL-6, TNF-α, and iNOS through suppressing NF-κB and Caspase-3 proteins expression in the brain (64). Therefore, hyperoside has great potential to alleviate neuroinflammation via inhibiting NLRP3 inflammasome activation, as well as regulating SIRT1/Wnt and NF-κB pathways.
Rutin (Figure 1), a flavonol glycoside abundantly distributed in tea, buckwheat, passion flower, and apple, exerts potent anti-neuroinflammatory properties. In LPS-treated BV2 cells, rutin promotes the phenotypic transformation of M1 to M2 with reducing IL-6, TNF-α, IL-1β, NO, iNOS, and CD86 levels, and up-regulating Arg-1, CD206 and IL-10 via inhibiting the expression of TLR4 and MyD88, and blocking NF-κB and IKKβ phosphorylation (65). Rutin is also found to improve Tau-P301S-induced memory deficits, suppress the activation of microglia and astrocytes, as well as decrease the levels of pro-inflammatory mediators, through the inactivation of IKKβ and p65 in the brain of AD mice (66). Thus, rutin shows great potential to ameliorate neuroinflammation via TLR4/MyD88/NF-κB signaling pathway.
Moreover, other flavones and flavonols compounds, such as hispidulin, cymaroside, myricitrin and troxerutin also exert anti-neuroinflammatory effects. They could restrain the activation and polarization of microglia, as well as inhibit the expression of pro-inflammatory mediators via suppressing NLRP3 inflammasome activation and regulating PI3k/Akt, MAPKs, Nrf2 or NF-κB signaling pathways in PD, AD, traumatic brain injury, depression and I/R injury, which are specifically showed in Table 1.
Pinocembrin is a kind of flavanone mainly extracted from honey and propolis. Pinocembrin inhibits microglia activation, reverses the up-regulation of TNF-α, iNOS, COX-2, IL-6, and IL-1β in the hippocampus from intermittent hypoxia-induced mice via suppressing the protein expression of NLRP3, ASC, and cleaved-Caspase-1, as well as enhancing BNIP3, LC3-II, ATG7, Beclin-1, and ATG5 expression (91). In other study, pinocembrin is found to alleviate CUMS-induced depressive-like behaviors, reduce pro-inflammatory cytokines and increase IL-10, TGF-β secretion in the hippocampus through the up-regulation of Nrf2 and HO-1, and the inactivation of NF-κB (92). Thus, pinocembrin has great potential to alleviate neuroinflammation through regulating NLRP3 inflammasome activation, BNIP3-mediated mitophagy, Nrf2/HO-1 along with NF-κB signaling pathways.
Farrerol (Figure 2), a type of 2,3-dihydroflavonoid, isolated from rhododendron leaves, down-regulates the expression of IL‐6, IL‐1β, TNF‐α, iNOS, COX‐2, NO, and PGE2 in LPS-induced BV2 cells through inhibiting p65 and Akt phosphorylation (93). And in MPP+‐treated BV2 cells, farrerol is also found to decrease pro-inflammatory mediators levels via suppressing TLR4 and MyD88 expression, as well as p65 and IκBα phosphorylation (94). Moreover, farrerol alleviates motor dysfunction and mitigates microglial activation in the SN of LPS-induced rats (93). Thus, farrerol exerts anti-neuroinflammatory effects through regulating Akt and TLR4/MyD88/NF-κB pathways.
Naringin and its aglycone naringenin (Figure 2) are widely found in citrus fruits as a biological neuroactive flavanones compound which has anti-neuroinflammatory activities. In social-defeat stress-induced neurobehavioral deficits mice and MCP-1-stimulated rats, naringin is reported to reverse behavioral impairments, and reduce TNF-α, IL−1β, IL-6, and NO secretion in striatum, prefrontal cortex, and hippocampus (95, 96). Moreover, naringin could also cut pro-inflammatory mediators down in the brain of haloperidol−revulsive or bisphenol-A-mediated rats (97, 98). Besides, in LPS-induced BV2 cells, naringenin, the aglycone of naringin, is found to inhibit pro-inflammatory factors such as NO, IL-1β, and IL-18 release, and up-regulate Arg-1, and IL-10 through suppressing NLRP3 and cleaved-Caspase-1 protein expression, and inhibiting JNK and ERK phosphorylation (99, 100). In other study, naringenin improves the cognitive deficiency, decreases pro-inflammatory cytokines secretion, and inhibits GFAP protein expression in the hippocampus from AD mice (101). These results suggest that naringin and naringenin play beneficial roles in neuroinflammation and related diseases.
Hesperidin and its aglycone hesperetin (Figure 2) with anti-neuroinflammatory effects, are mainly distributed in citrus fruits such as oranges, grapefruit, and lemons. Hesperidin is found to inhibit the release of TNF-α, IL-1β, IL-6, as well as MCP-1 in LPS-stimulated BV2 cells and HT22 cells and the brain of N-methyl-D-aspartate-induced mice through inhibiting NF-κB pathway (102). Besides, hesperidin also down-regulates the levels of pro-inflammatory mediators, decreases HMGB1, RAGE, p-IκBα, and p-p65 protein expression, and increases the protein levels of BDNF and p-TRκB in corticosterone-stimulated PC12 cells and hippocampus of CUMS-processed mice (103).
In LPS-stimulated BV2 cells, hesperetin, an aglycone of hesperidin, inhibits the levels of NO, and iNOS via the inactivation of TLR4, ERK, p38, and p65 (104, 105). And in LPS-induced mice and Aβ1-42-induced AD mice, hesperetin is found to improve behavioral disorders, suppress astrocyte and microglia activation, and decrease iNOS and COX-2 production in the cortical and hippocampus of mice via inhibiting the protein expression of TLR4 and p-p65 (104–106). On the basis of their safety and effectiveness, hesperidin and hesperetin may be further researched to alleviate neuroinflammation as food supplements.
Dihydromyricetin (Figure 2) is a major bioactive flavanonol extracted from Ampelopsis grossedentata. In LPS-stimulated BV2 cells, dihydromyricetin reduces the levels of TNF-α, IL-6, IL-1β, COX-2, and iNOS through inhibiting NLRP3, ASC, Caspase-1, HIF1a, TLR4 and MyD88 protein expression, as well as Akt, p65, and IκBα phosphorylation (107, 108). Besides, in the brain from LPS-induced mice, dihydromyricetin is reported to down-regulate pro-inflammatory mediators, and suppress CD11b and CD14 expression through TLR4/Akt/HIF1a/NLRP3 pathway (107). Dihydromyricetin supplement reduces the secretion of IFN-γ, IL-1α, MIP-1β, CXCL2, CCL17, IL-2, and IL-7 in serum, and improves the loss and dystrophy of microglia in the hippocampus from social isolation-induced mice (109). Moreover, dihydromyricetin also ameliorates the memory deficiency and reduces the levels of pro-inflammatory mediators in the brain of Aβ1-42-processed AD rats (110). These results suggest that dihydromyricetin could alleviate neuroinflammation-related brain disorders.
In addition, other flavanones and flavanonols compounds, such as 7-Methoxyflavanone, dihydroquercetin, liquiritigenin exert anti-neuroinflammatory effects which are amply shown in Table 2.
Table 2 Effects of flavanones, flavanonols, isoflavones, chalcones, dihydrochalcone and other flavonoids on neuroinflammation.
Calycosin (Figure 3) is an active isoflavone isolated from Radix Astragali. Treatment of calycosin protects mice against ICH-induced damages, improves neurobehavior, reduces the secretion of TNF-α, IL-6, IL-1β and IL-18, as well as inhibits microglia activation in perihematomal tissue of the brain from ICH-induced mice through suppressing IκBα and p65 phosphorylation, and repressing both transcriptional and translational of NLRP3, ASC, Caspase-1 (128). Besides, calycosin mitigates the behavioral dysfunctions, protects TH neurons and down-regulates the levels of pro-inflammatory mediators in the brain from MPTP‐induced PD mice via suppressing TLR2, TLR4, and nuclear NF-κB expression, as well as inhibiting p38, JNK and ERK phosphorylation (129). Thus, calycosin can alleviate neuroinflammation through modulating NLRP3 inflammasome and MAPKs, TLR/NF-κB pathways.
Genistein (Figure 3) distributed in soy is an isoflavone and has anti-neuroinflammatory effects. Genistein ameliorates hypoxic-ischemic brain damage-induced neuroinflammation with reducing TNF-α, IL-1β, and IL-6 secretion by the up-regulation of Nrf2, HO-1 and IκBα, and the inactivation of IκBα and NF-κB (130). Moreover, genistein improves cognitive disorders, and reduces MCP-1 release, and elevates the levels of IL-10, IGF-1, BDNF, and CREB in the hippocampus of hypoxia-induced mice (131). In other study, genistein is reported to reduce the levels of IL-6, IL‐1β, TNF-α, IL-8, iNOS, and CD16, and increase CD206 and Arg-1 expression in isoflurane-mediated BV2 cells and hippocampal regions from isoflurane-induced rats through restraining TLR4, MyD88, and TRAF6 protein expression, as well as suppressing TAK1, p38, ERK, IκBα and NF-κB phosphorylation (132). These evidences demonstrate that genistein is able to repress neuroinflammation via promoting Nrf2 pathway, as well as suppressing MAPKs and NF-κB pathways.
Puerarin (Figure 3), distributed in Pueraria lobata, could reduce the secretion of IL-8, IL-18, MCP-1 and CCL2 in TNF-α plus IL-1β-induced primary nerve cells and the trigeminal ganglions from complete Freund’s adjuvant-treated mice through the inhibition of NLRP3, Caspase-1, TGF-β1, NLRP1 protein and Smad3 phosphorylation, and the up-regulation of SIRT1 (133). Another study shows that puerarin could alleviate ICH-induced behavioral defects, drop hematoma volume and histological injury, decrease IL-6, IL‐1β, and TNF-α secretion, as well as down-regulate 3-NT, 8-OHdG, and ROS levels in the perihematomal brain tissue of ICH-induced rats via promoting PI3k and Akt phosphorylation, and suppressing the phosphorylation of p65 and the nuclear accumulation of p65 (134). Thus, puerarin inhibits the activation of NLRP3 inflammasome, promotes the expression of SIRT1 and regulates TGF-β1/Smads, PI3k/Akt, and NF-κB pathways to ameliorate neuroinflammation.
In addition, daidzein, isoformononetin, ononin and other isoflavonoids compounds are also able to inhibit pro-inflammatory cytokines secretion via regulating Akt, ERK and NF-κB signaling pathways to exert anti-neuroinflammatory effects which are shown in Table 2.
Isoliquiritigenin (Figure 4), with a chalcone structure, is derived from licorice root. Isoliquiritigenin alleviates morphological changes and reduces the levels of COX-2, iNOS, NO, IL-1β, IL-6, and TNF-α via the up-regulation of Nrf2, HO-1 and NQO1, and the inhibition of p65 nuclear translocation in BV2 cells induced by Aβ oligomers, and increases the cell viability in co-culture of BV2 cells and N2a cells (135). In vivo study, isoliquiritigenin reverses LPS-induced cognitive deficits, promotes the expression of PSD-95, BDNF, and synaptophysin, and restrains the secretion of CCL3, TNF-α, IL-1β, and IL-6 in hippocampus from LPS-stimulated rats via increasing GSK-3β phosphorylation and Nrf2, HO-1, NQO1 expression, as well as suppressing the protein expression of NF-κB (136). Moreover, isoliquiritigenin is also reported to improve cognitive impairment, decrease TNF-α, IL-1β and IL-18 secretion, and suppress the activation of microglia and astrocytes in hippocampus from kainic acid-induced seizures rats through inhibiting cleaved-Caspase-3, cleaved-Caspase-9, and NLRP3 expression, and enhancing Nrf2, HO-1, and NQO1 production (137). Therefore, isoliquiritigenin shows great potential to attenuate neuroinflammation by regulating NLRP3 inflammasome, Nrf2/HO-1 and NF-κB pathways.
Hydroxysafflor yellow A (HSYA) (Figure 4) exists in Carthamus tinctorius L. with good effects of alleviating neuroinflammation. HSYA treatment inhibits NO, TNF-α, IL-1β, IL-6, and iNOS levels, suppresses CD16 and CD32 expression, as well as promotes Arg-1 and CD206 production in LPS-induced primary microglia through the up-regulation of Nrf2, HO-1 and SIRT1 (138). HSYA also reduces pro-inflammatory cytokines release, increases the secretion of IL-4, IL-10 and IL-13, as well as regulates microglia polarization in Aβ1-42-stimulated BV2 cells via suppressing the expression of TREM2, and TLR4, and the phosphorylation of p65 and IκBα (139). Administration of HSYA down-regulates the levels of iNOS and COX-2 in 6-OHDA-revulsive SH-SY5Y cells and SN from 6-OHDA-induced mice through suppressing IκBα production and the phosphorylation of p65, p38 and JNK (140). Furthermore, HSYA also lessens the cerebral infarction area, decreases the levels of NO and iNOS, and increases the production of eNOS in the cortical penumbra of I/R rats via elevating the expression of IκBα and p-GSK-3β, and suppressing cleaved-Capase-3 expression and p65 phosphorylation (141). Thus, HSYA could suppress the activation and polarization of microglia, and reduce pro-inflammatory mediators production through TREM2/TLR4/NF-κB, Nrf2/HO-1, and MAPK pathways.
Trilobatin (Figure 4) is a dihydrochalcone compound distributed in Lithocarpuspolystachyus Rehd., and has anti-neuroinflammatory effects. Trilobatin is reported to improve cognitive impairment, reduce activated microglia and astrocytes with decreasing Iba-1 and GFAP expression, and inhibit the secretion of TNF-α, IL-1β and IL-6 in the hippocampus from APP/PS1 and triple-transgenic-induced AD mice through the down-regulation of HMGB1, TLR4, MyD88, TRAF6 and p-p65 (142, 143). Moreover, in OGD/R-stimulated astrocytes and the brain of MACO-induced I/R rats, trilobatin reduces the production of iNOS, and suppresses the activation of microglia and astrocytes via up-regulating Nrf2, HO-1, NQO1 and SIRT3 protein, as well as suppressing p65 phosphorylation and the expression of Keap1, TLR4, MyD88, and TRAF6 (144). Thus, as a potential therapeutic drug, trilobatin can prevent and treat neuroinflammation-related brain disorders.
Phloretin (Figure 4), a dihydrochalcone flavonoid, is abundant in apple with anti-neuroinflammatory effects. Phloretin is reported to down-regulate the secretion of TNF-α in the brain of Aβ25-35-induced AD rats (145), decrease the levels of IL-6, IL-1β, iNOS and COX-2, and reduce activated microglia and astrocytes in the brain from MPTP-induced PD mice (146).
In addition to the above compounds, the other chalcones and dihydrochalcone compounds such as phloridzin, xanthohumol, isoliquiritin also have anti-neuroinflammatory effects via modulating mTOR, NF-κB, or Nrf2/HO-1 pathways, which are shown in Table 2.
There are others types of flavonoids, including flavanols, anthocyanidins, and bioflavonoids. Some of them also have anti-neuroinflammatory effects, such as flavanol epigallocatechin-3-O-gallate (EGCG), anthocyanidin cyanidin-3-O-glucocide, bioflavonoid isoginkgetin, etc.
(-)-Epicatechin (Figure 5), a dietary flavanols, is widely distributed in foods such as tea, cocoa and grapes. Treatment of (-)-Epicatechin improves HFD-induced cognitive and memory impairment and inhibits the activation of microglia via decreasing the transcription of TLR4 and NOX4 in the hippocampus (147). In other study, (-)-Epicatechin represses the activation of microglia and astrocytes, reduces the levels of TNF-α, IFN-γ, IL-1β, IL-3, IL-5, IL-6, IL-15, and COX-2, as well as promotes IL-10 and IL-11 secretion in the hippocampus from aging mice through suppressing Caspase-3, Caspase-9 and NF-κB protein expression, and promoting Akt and GSK-3β phosphorylation (148). Therefore, (-)-Epicatechin possesses ability to lighten neuroinflammation through regulating TLR4/NOX4, Akt/GSK-3β, and NF-κB pathways.
EGCG (Figure 5) with anti-neuroinflammation effects is the major catechin component from green tea. EGCG supplementation down-regulates the levels of TNF-α, IL-6, and IL-1β in palmitic acid-stimulated BV2 cells and hypothalamus of HFD mice through suppressing the expression of JAK2 and STAT3 (149). In APP/PS1 transgenic mice, EGCG is reported to improve behavioral disorders, inhibit microglia activation, decrease IL-1β secretion and increase the release of IL-10 and IL-13 in the hippocampus (150). EGCG also down-regulates NO and TNF-α levels, as well as inhibits Caspase-3 and NF-κB protein expression in the cerebral cortex and hippocampus from ethanol-treated rats (151). Besides, in CUMS-induced rats and rotenone-stimulated PD rats, EGCG also ameliorates behavioral disorders, and reduces pro-inflammatory cytokines secretion (152, 153). Thus, EGCG, as a dietary compound, treats neuroinflammation-related brain diseases by modulating JAK2/STAT3 and NF-κB pathway.
Moreover, in PD, depression or ischemic stroke models, the other flavonoids compounds such as cyanidin-3-O-glucocide, agathisflavone and ginkgetin also exert anti-neuroinflammatory effects via PI3k/Akt, TLR4/NF-κB and MAPK pathways, which are shown in Table 2.
Neuroinflammation, a complex immune response, is a key hallmark of brain disorders. Following stimuli, activated microglia and astrocytes secrete massive pro-inflammatory cytokines, chemokines and small-molecule messengers, and cause further tissue dysfunction, which is the characteristic of neuroinflammation. Evidently, it is potential to develop therapeutic and preventive strategies to treat brain disorders targeting neuroinflammation. Based on the recent investigations, natural flavonoids exhibit plenty of beneficial anti-neuroinflammatory effects, such as down-regulating the expression of pro-inflammatory mediators, accelerating the secretion of anti-inflammatory cytokines, inhibiting astrocytosis, and suppressing the activation and polarization of microglia, and the main mechanisms of natural flavonoids against neuroinflammation include the inhibition of NLRP3 inflammasome activation and MAPKs, JAK/STAT, NF-κB and apoptotic pathways, as well as the activation of Nrf2, AMPK, BDNF/CREB, Wnt/β-Catenin, PI3k/Akt pathways and SIRT1-mediated HMGB1 deacetylation (Figure 6). And from the summary of current research, different types of natural flavonoids share with similar anti-neuroinflammatory mechanisms without obvious difference, among which, inflammatory and oxidative signaling pathways have been widely studied, while other pathways are less studied and need further study. Moreover, the current researches on natural flavonoids against neuroinflammation have some limitations. Firstly, the current researches mainly focus on flavones and flavonols, other types of flavonoids are less studied. Therefore, this is an area that warrants further investigation on rest of the flavonoids in related brain diseases. Secondly, the mechanism researches pay close attention to the activation and polarization of microglia, but ignore astrocytes and the crosstalk among microglia, astrocytes and neurons which requires further study. Overall, these preclinical data help us to further investigate natural flavonoids and offer ideas for finding new dietary supplements or lead compounds to treat neuroinflammation and related brain disorders.
Figure 6 Overview of the pathological course of neuroinflammation and the mechanisms of natural flavonoids against neuroinflammation. (A) Under neuroinflammation state, microglia and astrocytes are activated and secrete a lot of inflammatory cytokines, chemokines, along with small-molecule messengers which disturb the normal functioning of neurons and cause damage in brain tissue. (B) Natural flavonoids play anti-neuroinflammatory effects via inhibiting the activation of NLRP3 inflammasome and MAPKs, JAK/STAT, NF-κB pathways, promoting Nrf2, AMPK, BDNF/CREB, Wnt/β-Catenin, PI3k/Akt pathways and SIRT1-mediated HMGB1 deacetylation.
YC and FP contributed equally to the design and draft of the manuscript. YC and FP drafted the manuscript. YC, FP, ZX, and JC revised the manuscript. CP and DL conceived and designed the whole project. All authors reviewed and approved the final manuscript.
Financial support by National Natural Science Foundation of China (82104477, U19A2010 and 81891012), National Interdisciplinary Innovation Team of Traditional Chinese Medicine (ZYYCXTD-D-202209) are gratefully acknowledged.
The authors declare that the research was conducted in the absence of any commercial or financial relationships that could be construed as a potential conflict of interest.
All claims expressed in this article are solely those of the authors and do not necessarily represent those of their affiliated organizations, or those of the publisher, the editors and the reviewers. Any product that may be evaluated in this article, or claim that may be made by its manufacturer, is not guaranteed or endorsed by the publisher.
1. Stephenson J, Nutma E, van der Valk P, Amor S. Inflammation in CNS neurodegenerative diseases. Immunology (2018) 154(2):204–19. doi: 10.1111/imm.12922
2. Lyman M, Lloyd DG, Ji X, Vizcaychipi MP, Ma D. Neuroinflammation: The role and consequences. Neurosci Res (2014) 79:1–12. doi: 10.1016/j.neures.2013.10.004
3. Jayaraman A, Htike TT, James R, Picon C, Reynolds R. TNF-mediated neuroinflammation is linked to neuronal necroptosis in alzheimer's disease hippocampus. Acta Neuropathol Commun (2021) 9(1):159. doi: 10.1186/s40478-021-01264-w
4. Czapski GA, Strosznajder JB. Glutamate and GABA in microglia-neuron cross-talk in alzheimer's disease. Int J Mol Sci (2021) 22(21):11677. doi: 10.3390/ijms222111677
5. Calsolaro V, Edison P. Neuroinflammation in alzheimer's disease: Current evidence and future directions. Alzheimers Dement (2016) 12(6):719–32. doi: 10.1016/j.jalz.2016.02.010
6. Choi I, Zhang Y, Seegobin SP, Pruvost M, Wang Q, Purtell K, et al. Microglia clear neuron-released A-synuclein Via selective autophagy and prevent neurodegeneration. Nat Commun (2020) 11(1):1386. doi: 10.1038/s41467-020-15119-w
7. Bonney S, Seitz S, Ryan CA, Jones KL, Clarke P, Tyler KL, et al. Gamma interferon alters junctional integrity via rho kinase, resulting in blood-brain barrier leakage in experimental viral encephalitis. mBio (2019) 10(4):e01675–19. doi: 10.1128/mBio.01675-19
8. Zapata-Acevedo JF, García-Pérez V, Cabezas-Pérez R, Losada-Barragán M, Vargas-Sánchez K, González-Reyes RE. Laminin as a biomarker of blood-brain barrier disruption under neuroinflammation: A systematic review. Int J Mol Sci (2022) 23(12):6788. doi: 10.3390/ijms23126788
9. Xanthos DN, Sandkühler J. Neurogenic neuroinflammation: Inflammatory cns reactions in response to neuronal activity. Nat Rev Neurosci (2014) 15(1):43–53. doi: 10.1038/nrn3617
10. Gorji A. Neuroinflammation: The pathogenic mechanism of neurological disorders. Int J Mol Sci (2022) 23(10):5744. doi: 10.3390/ijms23105744
11. Cowan M, Petri WA. Microglia: Immune regulators of neurodevelopment. Front Immunol (2018) 9:2576. doi: 10.3389/fimmu.2018.02576
12. Li D, Yang C, Zhu JZ, Lopez E, Zhang T, Tong Q, et al. Berberine remodels adipose tissue to attenuate metabolic disorders by activating sirtuin 3. Acta Pharmacol Sin (2022) 43(5):1285–98. doi: 10.1038/s41401-021-00736-y
13. Guo S, Wang H, Yin Y. Microglia polarization from M1 to M2 in neurodegenerative diseases. Front Aging Neurosci (2022) 14:815347. doi: 10.3389/fnagi.2022.815347
14. Yang X, Xu S, Qian Y, Xiao Q. Resveratrol regulates microglia M1/M2 polarization Via pgc-1α in conditions of neuroinflammatory injury. Brain Behav Immun (2017) 64:162–72. doi: 10.1016/j.bbi.2017.03.003
15. Cherry JD, Olschowka JA, O'Banion MK. Neuroinflammation and M2 microglia: The good, the bad, and the inflamed. J Neuroinflamm (2014) 11:98. doi: 10.1186/1742-2094-11-98
16. Hasel P, Liddelow SA. Astrocytes. Curr Biol (2021) 31(7):R326–R7. doi: 10.1016/j.cub.2021.01.056
17. Pekny M, Nilsson M. Astrocyte activation and reactive gliosis. Glia (2005) 50(4):427–34. doi: 10.1002/glia.20207
18. Zhang Y, Meng T, Chen J, Zhang Y, Kang J, Li X, et al. Mir-21a-5p promotes inflammation following traumatic spinal cord injury through upregulation of neurotoxic reactive astrocyte (A1) polarization by inhibiting the CNTF/STAT3/Nkrf pathway. Int J Biol Sci (2021) 17(11):2795–810. doi: 10.7150/ijbs.60509
19. Matejuk A, Ransohoff RM. Crosstalk between astrocytes and microglia: An overview. Front Immunol (2020) 11:1416. doi: 10.3389/fimmu.2020.01416
20. Borst K, Dumas AA, Prinz M. Microglia: Immune and non-immune functions. Immunity (2021) 54(10):2194–208. doi: 10.1016/j.immuni.2021.09.014
21. Jha MK, Jo M, Kim J-H, Suk K. Microglia-astrocyte crosstalk: An intimate molecular conversation. Neuroscientist (2019) 25(3):227–40. doi: 10.1177/1073858418783959
22. Marinelli S, Basilico B, Marrone MC, Ragozzino D. Microglia-neuron crosstalk: Signaling mechanism and control of synaptic transmission. Semin Cell Dev Biol (2019) 94:138–51. doi: 10.1016/j.semcdb.2019.05.017
23. Colombo E, Cordiglieri C, Melli G, Newcombe J, Krumbholz M, Parada LF, et al. Stimulation of the neurotrophin receptor TrkB on astrocytes drives nitric oxide production and neurodegeneration. J Exp Med (2012) 209(3):521–35. doi: 10.1084/jem.20110698
24. Linnerbauer M, Wheeler MA, Quintana FJ. Astrocyte crosstalk in cns inflammation. Neuron (2020) 108(4):608–22. doi: 10.1016/j.neuron.2020.08.012
25. Jaeger BN, Parylak SL, Gage FH. Mechanisms of dietary flavonoid action in neuronal function and neuroinflammation. Mol Aspects Med (2018) 61:50–62. doi: 10.1016/j.mam.2017.11.003
26. Kou J-J, Shi J-Z, He Y-Y, Hao J-J, Zhang H-Y, Luo D-M, et al. Luteolin alleviates cognitive impairment in alzheimer's disease mouse model Via inhibiting endoplasmic reticulum stress-dependent neuroinflammation. Acta Pharmacol Sin (2022) 43(4):840–9. doi: 10.1038/s41401-021-00702-8
27. Yang Y, Tan X, Xu J, Wang T, Liang T, Xu X, et al. Luteolin alleviates neuroinflammation Via downregulating the TLR4/TRAF6/NF-κB pathway after intracerebral hemorrhage. BioMed Pharmacother (2020) 126:110044. doi: 10.1016/j.biopha.2020.110044
28. Zhang Z-H, Liu J-Q, Hu C-D, Zhao X-T, Qin F-Y, Zhuang Z, et al. Luteolin confers cerebroprotection after subarachnoid hemorrhage by suppression of NLPR3 inflammasome activation through Nrf2-dependent pathway. Oxid Med Cell Longev (2021) 2021:5838101. doi: 10.1155/2021/5838101
29. Achour M, Ferdousi F, Sasaki K, Isoda H. Luteolin modulates neural stem cells fate determination: Study on human neural stem cells, and study on lps-induced depression mice model. Front Cell Dev Biol (2021) 9:753279. doi: 10.3389/fcell.2021.753279
30. Zhou W, Hu M, Hu J, Du Z, Su Q, Xiang Z. Luteolin suppresses microglia neuroinflammatory responses and relieves inflammation-induced cognitive impairments. Neurotox Res (2021) 39(6):1800–11. doi: 10.1007/s12640-021-00426-x
31. Ahmad S, Jo MH, Ikram M, Khan A, Kim MO. Deciphering the potential neuroprotective effects of luteolin against aβ–induced alzheimer's disease. Int J Mol Sci (2021) 22(17):9583. doi: 10.3390/ijms22179583
32. Liu J, Zhang T, Wang Y, Si C, Wang X, Wang R-T, et al. Baicalin ameliorates neuropathology in repeated cerebral ischemia-reperfusion injury model mice by remodeling the gut microbiota. Aging (Albany NY) (2020) 12(4):3791–806. doi: 10.18632/aging.102846
33. Guo L-T, Wang S-Q, Su J, Xu L-X, Ji Z-Y, Zhang R-Y, et al. Baicalin ameliorates neuroinflammation-induced depressive-like behavior through inhibition of toll-like receptor 4 expression via the PI3k/Akt/Foxo1 pathway. J Neuroinflamm (2019) 16(1):95. doi: 10.1186/s12974-019-1474-8
34. Shah M-A, Park D-J, Kang J-B, Kim M-O, Koh P-O. Baicalin alleviates lipopolysaccharide-induced neuroglial activation and inflammatory factors activation in hippocampus of adult mice. Lab Anim Res (2020) 36:32. doi: 10.1186/s42826-020-00058-w
35. Li Y, Liu T, Li Y, Han D, Hong J, Yang N, et al. Baicalin ameliorates cognitive impairment and protects microglia from lps-induced neuroinflammation Via the Sirt1/HMGB1 pathway. Oxid Med Cell Longev (2020) 2020:4751349. doi: 10.1155/2020/4751349
36. Shah M-A, Park D-J, Kang J-B, Kim M-O, Koh P-O. Baicalin attenuates lipopolysaccharide-induced neuroinflammation in cerebral cortex of mice Via inhibiting nuclear factor kappa b (NF-κB) activation. J Vet Med Sci (2019) 81(9):1359–67. doi: 10.1292/jvms.19-0281
37. Jin X, Liu M-Y, Zhang D-F, Zhong X, Du K, Qian P, et al. Baicalin mitigates cognitive impairment and protects neurons from microglia-mediated neuroinflammation Via suppressing Nlrp3 inflammasomes and TLR4/NF-κB signaling pathway. CNS Neurosci Ther (2019) 25(5):575–90. doi: 10.1111/cns.13086
38. Ran Y, Qie S, Gao F, Ding Z, Yang S, Tian G, et al. Baicalein ameliorates ischemic brain damage through suppressing proinflammatory microglia polarization via inhibiting the TLR4/ NF-κB and Stat1 pathway. Brain Res (2021) 1770:147626. doi: 10.1016/j.brainres.2021.147626
39. Pan L, Sze YH, Yang M, Tang J, Zhao S, Yi I, et al. Baicalein-a potent pro-homeostatic regulator of microglia in retinal ischemic injury. Front Immunol (2022) 13:837497. doi: 10.3389/fimmu.2022.837497
40. Yang S, Wang H, Yang Y, Wang R, Wang Y, Wu C, et al. Baicalein administered in the subacute phase ameliorates ischemia-Reperfusion-Induced brain injury by reducing neuroinflammation and neuronal damage. BioMed Pharmacother (2019) 117:109102. doi: 10.1016/j.biopha.2019.109102
41. Rui W, Li S, Xiao H, Xiao M, Shi J. Baicalein attenuates neuroinflammation by inhibiting NLRP3/Caspase-1/Gsdmd pathway in mptp induced mice model of parkinson's disease. Int J Neuropsychopharmacol (2020) 23(11):762–73. doi: 10.1093/ijnp/pyaa060
42. Zhao X, Kong D, Zhou Q, Wei G, Song J, Liang Y, et al. Baicalein alleviates depression-like behavior in rotenone- induced parkinson's disease model in mice through activating the BDNF/TrkB/CREB pathway. BioMed Pharmacother (2021) 140:111556. doi: 10.1016/j.biopha.2021.111556
43. Xu X-E, Li M-Z, Yao E-S, Gong S, Xie J, Gao W, et al. Morin exerts protective effects on encephalopathy and sepsis-associated cognitive functions in a murine sepsis model. Brain Res Bull (2020) 159:53–60. doi: 10.1016/j.brainresbull.2020.03.019
44. Khamchai S, Chumboatong W, Hata J, Tocharus C, Suksamrarn A, Tocharus J. Morin protects the blood-brain barrier integrity against cerebral ischemia reperfusion through anti-inflammatory actions in rats. Sci Rep (2020) 10(1):13379. doi: 10.1038/s41598-020-70214-8
45. Ben-Azu B, Emokpae O, Ajayi AM, Jarikre TA, Orhode V, Aderibigbe AO, et al. Repeated psychosocial stress causes glutamic acid decarboxylase isoform-67, oxidative-Nox-2 changes and neuroinflammation in mice: Prevention by treatment with a neuroactive flavonoid, morin. Brain Res (2020) 1744:146917. doi: 10.1016/j.brainres.2020.146917
46. Mohammadi N, Asle-Rousta M, Rahnema M, Amini R. Morin attenuates memory deficits in a rat model of alzheimer's disease by ameliorating oxidative stress and neuroinflammation. Eur J Pharmacol (2021) 910:174506. doi: 10.1016/j.ejphar.2021.174506
47. Çelik H, Kucukler S, Çomaklı S, Özdemir S, Caglayan C, Yardım A, et al. Morin attenuates ifosfamide-induced neurotoxicity in rats via suppression of oxidative stress, neuroinflammation and neuronal apoptosis. Neurotoxicology (2020) 76:126–37. doi: 10.1016/j.neuro.2019.11.004
48. Tsai C-F, Chen G-W, Chen Y-C, Shen C-K, Lu D-Y, Yang L-Y, et al. Regulatory effects of quercetin on M1/M2 macrophage polarization and Oxidative/Antioxidative balance. Nutrients (2021) 14(1):67. doi: 10.3390/nu14010067
49. Kang S, Piao Y, Kang YC, Lim S, Pak YK. Qi-activating quercetin alleviates mitochondrial dysfunction and neuroinflammation in vivo and in vitro. Arch Pharm Res (2020) 43(5):553–66. doi: 10.1007/s12272-020-01238-x
50. Han X, Xu T, Fang Q, Zhang H, Yue L, Hu G, et al. Quercetin hinders microglial activation to alleviate neurotoxicity Via the interplay between Nlrp3 inflammasome and mitophagy. Redox Biol (2021) 44:102010. doi: 10.1016/j.redox.2021.102010
51. Li H, Chen F-J, Yang W-L, Qiao H-Z, Zhang S-J. Quercetin improves cognitive disorder in aging mice by inhibiting Nlrp3 inflammasome activation. Food Funct (2021) 12(2):717–25. doi: 10.1039/d0fo01900c
52. Lee B, Yeom M, Shim I, Lee H, Hahm D-H. Protective effects of quercetin on anxiety-like symptoms and neuroinflammation induced by lipopolysaccharide in rats. Evid Based Complement Alternat Med (2020) 2020:4892415. doi: 10.1155/2020/4892415
53. Cai M, Zhuang W, Lv E, Liu Z, Wang Y, Zhang W, et al. Kaemperfol alleviates pyroptosis and microglia-mediated neuroinflammation in parkinson's disease Via inhibiting P38mapk/ NF-κB signaling pathway. Neurochem Int (2022) 152:105221. doi: 10.1016/j.neuint.2021.105221
54. Chang S, Li X, Zheng Y, Shi H, Zhang D, Jing B, et al. Kaempferol exerts a neuroprotective effect to reduce neuropathic pain through TLR4/NF-κB signaling pathway. Phytother Res (2022) 36(4):1678–91. doi: 10.1002/ptr.7396
55. Yang Y-L, Cheng X, Li W-H, Liu M, Wang Y-H, Du G-H. Kaempferol attenuates LPS-induced striatum injury in mice involving anti-neuroinflammation, maintaining bbb integrity, and down-regulating the HMGB1/TLR4 pathway. Int J Mol Sci (2019) 20(3):491. doi: 10.3390/ijms20030491
56. Li W-H, Cheng X, Yang Y-L, Liu M, Zhang S-S, Wang Y-H, et al. Kaempferol attenuates neuroinflammation and blood brain barrier dysfunction to improve neurological deficits in cerebral Ischemia/Reperfusion rats. Brain Res (2019) 1722:146361. doi: 10.1016/j.brainres.2019.146361
57. Zheng Y, Zhu G, He J, Wang G, Li D, Zhang F. Icariin targets Nrf2 signaling to inhibit microglia-mediated neuroinflammation. Int Immunopharmacol (2019) 73:304–11. doi: 10.1016/j.intimp.2019.05.033
58. Zhang W-D, Li N, Du Z-R, Zhang M, Chen S, Chen W-F. IGF-1 receptor is involved in the regulatory effects of icariin and icaritin in astrocytes under basal conditions and after an inflammatory challenge. Eur J Pharmacol (2021) 906:174269. doi: 10.1016/j.ejphar.2021.174269
59. Wu H, Liu X, Gao Z-Y, Lin M, Zhao X, Sun Y, et al. Icaritin provides neuroprotection in parkinson's disease by attenuating neuroinflammation, oxidative stress, and energy deficiency. Antioxidants (Basel) (2021) 10(4):529. doi: 10.3390/antiox10040529
60. Zhang B, Wang G, He J, Yang Q, Li D, Li J, et al. Icariin attenuates neuroinflammation and exerts dopamine neuroprotection via an Nrf2-dependent manner. J Neuroinflamm (2019) 16(1):92. doi: 10.1186/s12974-019-1472-x
61. Zhu T, Zhang F, Li H, He Y, Zhang G, Huang N, et al. Long-term icariin treatment ameliorates cognitive deficits via Cd4 T cell-mediated immuno-inflammatory responses in App/Ps1 mice. Clin Interv Aging (2019) 14:817–26. doi: 10.2147/CIA.S208068
62. Huang J, Zhou L, Chen J, Chen T, Lei B, Zheng N, et al. Hyperoside attenuate inflammation in Ht22 cells via upregulating Sirt1 to activities wnt/-catenin and sonic hedgehog pathways. Neural Plast (2021) 2021:8706400. doi: 10.1155/2021/8706400
63. Wang K, Lu C, Wang T, Qiao C, Lu L, Wu D, et al. Hyperoside suppresses NLRP3 inflammasome in parkinson's disease Via pituitary adenylate cyclase-activating polypeptide. Neurochem Int (2022) 152:105254. doi: 10.1016/j.neuint.2021.105254
64. Chen X, Famurewa AC, Tang J, Olatunde OO, Olatunji OJ. Hyperoside attenuates neuroinflammation, cognitive impairment and oxidative stress via suppressing TNF-α/ NF-κB/Caspase-3 signaling in type 2 diabetes rats. Nutr Neurosci (2021) 25(8):1774–84. doi: 10.1080/1028415X.2021.1901047
65. Lang G-P, Li C, Han Y-Y. Rutin pretreatment promotes microglial M1 to M2 phenotype polarization. Neural Regener Res (2021) 16(12):2499–504. doi: 10.4103/1673-5374.313050
66. Sun X-Y, Li L-J, Dong Q-X, Zhu J, Huang Y-R, Hou S-J, et al. Rutin prevents tau pathology and neuroinflammation in a mouse model of alzheimer's disease. J Neuroinflamm (2021) 18(1):131. doi: 10.1186/s12974-021-02182-3
67. Yu C-I, Cheng C-I, Kang Y-F, Chang P-C, Lin I-P, Kuo Y-H, et al. Hispidulin inhibits neuroinflammation in lipopolysaccharide-activated Bv2 microglia and attenuates the activation of akt, NF-κB, and Stat3 pathway. Neurotox Res (2020) 38(1):163–74. doi: 10.1007/s12640-020-00197-x
68. Liu B, Huang B, Hu G, He D, Li Y, Ran X, et al. Isovitexin-mediated regulation of microglial polarization in lipopolysaccharide-induced neuroinflammation Via activation of the Camkkβ/Ampk-Pgc-1α signaling axis. Front Immunol (2019) 10:2650. doi: 10.3389/fimmu.2019.02650
69. Lu L, Yang L-K, Yue J, Wang X-S, Qi J-Y, Yang F, et al. Scutellarin alleviates depression-like behaviors induced by LPS in mice partially through inhibition of astrocyte-mediated neuroinflammation. Neurosci Lett (2021) 765:136284. doi: 10.1016/j.neulet.2021.136284
70. Bian H-T, Wang G-H, Huang J-J, Liang L, Xiao L, Wang H-L. Scutellarin protects against lipopolysaccharide-induced behavioral deficits by inhibiting neuroinflammation and microglia activation in rats. Int Immunopharmacol (2020) 88:106943. doi: 10.1016/j.intimp.2020.106943
71. Wang H, Guo Y, Qiao Y, Zhang J, Jiang P. Nobiletin ameliorates Nlrp3 inflammasome-mediated inflammation through promoting autophagy Via the AMPK pathway. Mol Neurobiol (2020) 57(12):5056–68. doi: 10.1007/s12035-020-02071-5
72. Qi G, Mi Y, Fan R, Li R, Liu Z, Liu X. Nobiletin protects against systemic inflammation-stimulated memory impairment Via MAPK and NF-κB signaling pathways. J Agric Food Chem (2019) 67(18):5122–34. doi: 10.1021/acs.jafc.9b00133
73. Wirianto M, Wang C-Y, Kim E, Koike N, Gomez-Gutierrez R, Nohara K, et al. The clock modulator nobiletin mitigates astrogliosis-associated neuroinflammation and disease hallmarks in an alzheimer's disease model. FASEB J (2022) 36(3):e22186. doi: 10.1096/fj.202101633R
74. Tan X, Liang Z, Li Y, Zhi Y, Yi L, Bai S, et al. Isoorientin, a GSK-3β inhibitor, rescues synaptic dysfunction, spatial memory deficits and attenuates pathological progression in App/Ps1 model mice. Behav Brain Res (2021) 398:112968. doi: 10.1016/j.bbr.2020.112968
75. Habib CN, Mohamed MR, Tadros MG, Tolba MF, Menze ET, Masoud SI. The potential neuroprotective effect of diosmin in rotenone-induced model of parkinson's disease in rats. Eur J Pharmacol (2022) 914:174573. doi: 10.1016/j.ejphar.2021.174573
76. Cao W, Dong Y, Zhao W, Lu X, Sun L. Mulberrin attenuates 1-Methyl-4-Phenyl-1,2,3,6- tetrahydropyridine (Mptp)-induced parkinson's disease by promoting Wnt/B-catenin signaling pathway. J Chem Neuroanat (2019) 98:63–70. doi: 10.1016/j.jchemneu.2019.04.003
77. Zhang Y, Jiang Y, Lu D. Diosmetin suppresses neuronal apoptosis and inflammation by modulating the phosphoinositide 3-kinase (PI3k)/Akt/Nuclear factor-κB (NF-κB) signaling pathway in a rat model of pneumococcal meningitis. Med Sci Monit (2019) 25:2238–45. doi: 10.12659/MSM.911860
78. Guo X, Wang J, Wang N, Mishra A, Li H, Liu H, et al. Wogonin preventive impact on hippocampal neurodegeneration, inflammation and cognitive defects in temporal lobe epilepsy. Saudi J Biol Sci (2020) 27(8):2149–56. doi: 10.1016/j.sjbs.2020.05.030
79. Zhao F, Dang Y, Zhang R, Jing G, Liang W, Xie L, et al. Apigenin attenuates acrylonitrile-induced neuro-inflammation in rats: Involved of inactivation of the TLR4/ NF-κB signaling pathway. Int Immunopharmacol (2019) 75:105697. doi: 10.1016/j.intimp.2019.105697
80. Li Q, Tian Z, Wang M, Kou J, Wang C, Rong X, et al. Luteoloside attenuates neuroinflammation in focal cerebral ischemia in rats Via regulation of the PPARγ/Nrf2/ NF-κB signaling pathway. Int Immunopharmacol (2019) 66:309–16. doi: 10.1016/j.intimp.2018.11.044
81. Yang T, Feng C, Wang D, Qu Y, Yang Y, Wang Y, et al. Neuroprotective and anti-inflammatory effect of tangeretin against cerebral ischemia-reperfusion injury in rats. Inflammation (2020) 43(6):2332–43. doi: 10.1007/s10753-020-01303-z
82. Gao C, Song Y, Dou T, Jiang S, Wu H, Seshadri VD, et al. Troxerutin abrogates Ischemic/Reperfusion-induced brain injury through ameliorating oxidative stress and neuronal inflammation by inhibiting the expression of NLRP3 in sprague dawley rats. J Environ Pathol Toxicol Oncol (2021) 40(4):11–9. doi: 10.1615/JEnvironPatholToxicolOncol.2021038860
83. Jamali-Raeufy N, Kardgar S, Baluchnejadmojarad T, Roghani M, Goudarzi M. Troxerutin exerts neuroprotection against lipopolysaccharide (Lps) induced oxidative stress and neuroinflammation through targeting Sirt1/Sirt3 signaling pathway. Metab Brain Dis (2019) 34(5):1505–13. doi: 10.1007/s11011-019-00454-9
84. Jang J-H, Lee SH, Jung K, Yoo H, Park G. Inhibitory effects of myricetin on lipopolysaccharide-induced neuroinflammation. Brain Sci (2020) 10(1):32. doi: 10.3390/brainsci10010032
85. Yang Y-L, Liu M, Cheng X, Li W-H, Zhang S-S, Wang Y-H, et al. Myricitrin blocks activation of NF-κB and mapk signaling pathways to protect nigrostriatum neuron in lps-stimulated mice. J Neuroimmunol (2019) 337:577049. doi: 10.1016/j.jneuroim.2019.577049
86. Gong J, Luo S, Zhao S, Yin S, Li X, Mou T. Myricitrin attenuates memory impairment in a rat model of sepsis-associated encephalopathy Via the NLRP3/Bax/Bcl pathway. Folia Neuropathol (2019) 57(4):327–34. doi: 10.5114/fn.2019.89856
87. Ding H, Li Y, Chen S, Wen Y, Zhang S, Luo E, et al. Fisetin ameliorates cognitive impairment by activating mitophagy and suppressing neuroinflammation in rats with sepsis-associated encephalopathy. CNS Neurosci Ther (2022) 28(2):247–58. doi: 10.1111/cns.13765
88. Elizabeth A, Adegbuyi A, Olusegun A, Benneth B-A, Anthony E, Abayomi A, et al. Morin hydrate attenuates chronic stress-induced memory impairment and degeneration of hippocampal subfields in mice: The role of oxidative, nitrergic and neuroinflammatory pathways. Metab Brain Dis (2020) 35(7):1145–56. doi: 10.1007/s11011-020-00595-2
89. Sun Y, Zhang H, Wu Z, Yu X, Yin Y, Qian S, et al. Quercitrin rapidly alleviated depression-like behaviors in lipopolysaccharide-treated mice: The involvement of PI3k/Akt/NF-κB signaling suppression and CREB/BDNF signaling restoration in the hippocampus. ACS Chem Neurosci (2021) 12(18):3387–96. doi: 10.1021/acschemneuro.1c00371
90. Mulati A, Zhang X, Zhao T, Ren B, Wang L, Liu X, et al. Isorhamnetin attenuates high-fat and high-fructose diet induced cognitive impairments and neuroinflammation by mediating MAPK and NF-κB signaling pathways. Food Funct (2021) 12(19):9261–72. doi: 10.1039/d0fo03165h
91. Gong L-J, Wang X-Y, Gu W-Y, Wu X. Pinocembrin ameliorates intermittent hypoxia-induced neuroinflammation through Bnip3-dependent mitophagy in a murine model of sleep apnea. J Neuroinflamm (2020) 17(1):337. doi: 10.1186/s12974-020-02014-w
92. Wang W, Zheng L, Xu L, Tu J, Gu X. Pinocembrin mitigates depressive-like behaviors induced by chronic unpredictable mild stress through ameliorating neuroinflammation and apoptosis. Mol Med (2020) 26(1):53. doi: 10.1186/s10020-020-00179-x
93. Li Y, Zeng Y, Meng T, Gao X, Huang B, He D, et al. Farrerol protects dopaminergic neurons in a rat model of lipopolysaccharide-induced parkinson's disease by suppressing the activation of the akt and NF-κB signaling pathways. Int Immunopharmacol (2019) 75:105739. doi: 10.1016/j.intimp.2019.105739
94. Cui B, Guo X, You Y, Fu R. Farrerol attenuates mpp-induced inflammatory response by TLR4 signaling in a microglia cell line. Phytother Res (2019) 33(4):1134–41. doi: 10.1002/ptr.6307
95. Oladapo OM, Ben-Azu B, Ajayi AM, Emokpae O, Eneni A-EO, Omogbiya IA, et al. Naringin confers protection against psychosocial defeat stress-induced neurobehavioral deficits in mice: Involvement of glutamic acid decarboxylase isoform-67, oxido-nitrergic stress, and neuroinflammatory mechanisms. J Mol Neurosci (2021) 71(3):431–45. doi: 10.1007/s12031-020-01664-y
96. Long J-Y, Chen J-M, Liao Y-J, Zhou Y-J, Liang B-Y, Zhou Y. Naringin provides neuroprotection in Ccl2-induced cognition impairment by attenuating neuronal apoptosis in the hippocampus. Behav Brain Funct (2020) 16(1):4. doi: 10.1186/s12993-020-00166-6
97. Akintunde JK, Akintola TE, Adenuga GO, Odugbemi ZA, Adetoye RO, Akintunde OG. Naringin attenuates bisphenol-a mediated neurotoxicity in hypertensive rats by abrogation of cerebral nucleotide depletion, oxidative damage and neuroinflammation. Neurotoxicology (2020) 81:18–33. doi: 10.1016/j.neuro.2020.08.001
98. Wang M-H, Yang C-C, Tseng H-C, Fang C-H, Lin Y-W, Soung H-S. Naringin ameliorates haloperidol-induced neurotoxicity and orofacial dyskinesia in a rat model of human tardive dyskinesia. Neurotox Res (2021) 39(3):774–86. doi: 10.1007/s12640-021-00333-1
99. Chen C, Wei Y-Z, He X-M, Li D-D, Wang G-Q, Li J-J, et al. Naringenin produces neuroprotection against lps-induced dopamine neurotoxicity Via the inhibition of microglial Nlrp3 inflammasome activation. Front Immunol (2019) 10:936. doi: 10.3389/fimmu.2019.00936
100. Zhang B, Wei Y-Z, Wang G-Q, Li D-D, Shi J-S, Zhang F. Targeting mapk pathways by naringenin modulates microglia M1/M2 polarization in lipopolysaccharide-stimulated cultures. Front Cell Neurosci (2018) 12:531. doi: 10.3389/fncel.2018.00531
101. Zhou T, Liu L, Wang Q, Gao Y. Naringenin alleviates cognition deficits in high-fat diet-fed Samp8 mice. J Food Biochem (2020) 44(9):e13375. doi: 10.1111/jfbc.13375
102. Sato K, Sato T, Ohno-Oishi M, Ozawa M, Maekawa S, Shiga Y, et al. Chop deletion and anti-neuroinflammation treatment with hesperidin synergistically attenuate nmda retinal injury in mice. Exp Eye Res (2021) 213:108826. doi: 10.1016/j.exer.2021.108826
103. Fu H, Liu L, Tong Y, Li Y, Zhang X, Gao X, et al. The antidepressant effects of hesperidin on chronic unpredictable mild stress-induced mice. Eur J Pharmacol (2019) 853:236–46. doi: 10.1016/j.ejphar.2019.03.035
104. Jo SH, Kim ME, Cho JH, Lee Y, Lee J, Park Y-D, et al. Hesperetin inhibits neuroinflammation on microglia by suppressing inflammatory cytokines and MAPK pathways. Arch Pharm Res (2019) 42(8):695–703. doi: 10.1007/s12272-019-01174-5
105. Muhammad T, Ikram M, Ullah R, Rehman SU, Kim MO. Hesperetin, a citrus flavonoid, attenuates LPS-induced neuroinflammation, apoptosis and memory impairments by modulating TLR4/NF-κB signaling. Nutrients (2019) 11(3):648. doi: 10.3390/nu11030648
106. Ikram M, Muhammad T, Rehman SU, Khan A, Jo MG, Ali T, et al. Hesperetin confers neuroprotection by regulating Nrf2/TLR4/NF-κB signaling in an aβ mouse model. Mol Neurobiol (2019) 56(9):6293–309. doi: 10.1007/s12035-019-1512-7
107. Wei Y, Hu Y, Qi K, Li Y, Chen J, Wang R. Dihydromyricetin improves lps-induced sickness and depressive-like behaviors in mice by inhibiting the TLR4/Akt/Hif1a/NLRP3 pathway. Behav Brain Res (2022) 423:113775. doi: 10.1016/j.bbr.2022.113775
108. Jing N, Li X. Dihydromyricetin attenuates inflammation through TLR4/ NF-κB pathway. Open Med (Wars) (2019) 14:719–25. doi: 10.1515/med-2019-0083
109. Al Omran AJ, Shao AS, Watanabe S, Zhang Z, Zhang J, Xue C, et al. Social isolation induces neuroinflammation and microglia overactivation, while dihydromyricetin prevents and improves them. J Neuroinflamm (2022) 19(1):2. doi: 10.1186/s12974-021-02368-9
110. Wang Y, Lv W, Li Y, Liu D, He X, Liu T. Ampelopsin improves cognitive impairment in alzheimer's disease and effects of inflammatory cytokines and oxidative stress in the hippocampus. Curr Alzheimer Res (2020) 17(1):44–51. doi: 10.2174/1567205016666191203153447
111. Qu Z, Chen Y, Luo Z-H, Shen X-L, Hu Y-J. 7-methoxyflavanone alleviates neuroinflammation in lipopolysaccharide-stimulated microglial cells by inhibiting TLR4/MyD88/MAPK signalling and activating the Nrf2/Nqo-1 pathway. J Pharm Pharmacol (2020) 72(3):385–95. doi: 10.1111/jphp.13219
112. Wang Y, Yang Z, Wang Q, Ren Y, Wang Q, Li Z. Bavachin exerted anti-neuroinflammatory effects by regulation of A20 ubiquitin-editing complex. Int Immunopharmacol (2021) 100:108085. doi: 10.1016/j.intimp.2021.108085
113. Chen M, Zhang Q-P, Zhu J-X, Cheng J, Liu Q, Xu G-H, et al. Involvement of fgf-2 modulation in the antidepressant-like effects of liquiritin in mice. Eur J Pharmacol (2020) 881:173297. doi: 10.1016/j.ejphar.2020.173297
114. Du Y, Luo M, Du Y, Xu M, Yao Q, Wang K, et al. Liquiritigenin decreases aβ levels and ameliorates cognitive decline by regulating microglia M1/M2 transformation in ad mice. Neurotox Res (2021) 39(2):349–58. doi: 10.1007/s12640-020-00284-z
115. Gu L, Sun M, Li R, Zhang X, Tao Y, Yuan Y, et al. Didymin suppresses microglia pyroptosis and neuroinflammation through the Asc/Caspase-1/Gsdmd pathway following experimental intracerebral hemorrhage. Front Immunol (2022) 13:810582. doi: 10.3389/fimmu.2022.810582
116. Chaturvedi S, Tiwari V, Gangadhar NM, Rashid M, Sultana N, Singh SK, et al. Isoformononetin, a dietary isoflavone protects against streptozotocin induced rat model of neuroinflammation through inhibition of NLRP3/Asc/Il-1 axis activation. Life Sci (2021) 286(3):119989. doi: 10.1016/j.lfs.2021.119989
117. Berköz M, Krośniak M, Özkan-Yılmaz F, Özlüer-Hunt A. Prophylactic effect of biochanin a in lipopolysaccharide-stimulated Bv2 microglial cells. Immunopharmacol Immunotoxicol (2020) 42(4):330–9. doi: 10.1080/08923973.2020.1769128
118. Chen X, Zhang M, Ahmed M, Surapaneni KM, Veeraraghavan VP, Arulselvan P. Neuroprotective effects of ononin against the aluminium chloride-induced alzheimer's disease in rats. Saudi J Biol Sci (2021) 28(8):4232–9. doi: 10.1016/j.sjbs.2021.06.031
119. Liu Y, Fu Y, Zhang Y, Liu F, Rose GM, He X, et al. Butein attenuates the cytotoxic effects of LPS-stimulated microglia on the sh-Sy5y neuronal cell line. Eur J Pharmacol (2020) 868:172858. doi: 10.1016/j.ejphar.2019.172858
120. Li Z, Wang Q, Luan H, Yang M, Li Y, Tian G, et al. A novel target Tax1bp1 and P38/Nrf2 pathway independently involved in the anti-neuroinflammatory effect of isobavachalcone. Free Radic Biol Med (2020) 153:132–9. doi: 10.1016/j.freeradbiomed.2020.04.011
121. Sun X-L, Zhang J-B, Guo Y-X, Xia T-S, Xu L-C, Rahmand K, et al. Xanthohumol ameliorates memory impairment and reduces the deposition of B-amyloid in App/Ps1 mice via regulating the Mtor/Lc3ii and Bax/Bcl-2 signalling pathways. J Pharm Pharmacol (2021) 73(9):1230–9. doi: 10.1093/jpp/rgab052
122. Rahman SU, Ali T, Hao Q, He K, Li W, Ullah N, et al. Xanthohumol attenuates lipopolysaccharide-induced depressive like behavior in mice: Involvement of NF-κB/Nrf2 signaling pathways. Neurochem Res (2021) 46(12):3135–48. doi: 10.1007/s11064-021-03396-w
123. Li Y, Song W, Tong Y, Zhang X, Zhao J, Gao X, et al. Isoliquiritin ameliorates depression by suppressing Nlrp3-mediated pyroptosis Via mirna-27a/Syk/NF-κB axis. J Neuroinflamm (2021) 18(1):1. doi: 10.1186/s12974-020-02040-8
124. Kaewmool C, Udomruk S, Phitak T, Pothacharoen P, Kongtawelert P. Cyanidin-3-O-Glucoside protects Pc12 cells against neuronal apoptosis mediated by LPS-stimulated Bv2 microglial activation. Neurotox Res (2020) 37(1):111–25. doi: 10.1007/s12640-019-00102-1
125. de Almeida MMA, Souza CDS, Dourado NS, da Silva AB, Ferreira RS, David JM, et al. Phytoestrogen agathisflavone ameliorates neuroinflammation-induced by LPS and IL-1β and protects neurons in cocultures of Glia/Neurons. Biomolecules (2020) 10(4):562. doi: 10.3390/biom10040562
126. Li P, Zhang F, Li Y, Zhang C, Yang Z, Zhang Y, et al. Isoginkgetin treatment attenuated lipopolysaccharide-induced monoamine neurotransmitter deficiency and depression-like behaviors through downregulating P38/NF-κB signaling pathway and suppressing microglia-induced apoptosis. J Psychopharmacol (2021) 35(10):1285–99. doi: 10.1177/02698811211032473
127. Xu B, He X, Sui Y, Wang X, Wang X, Ren L, et al. Ginkgetin aglycone attenuates neuroinflammation and neuronal injury in the rats with ischemic stroke by modulating Stat3/Jak2/Sirt1. Folia Neuropathol (2019) 57(1):16–23. doi: 10.5114/fn.2019.83827
128. Chen C, Cui J, Ji X, Yao L. Neuroprotective functions of calycosin against intracerebral hemorrhage-induced oxidative stress and neuroinflammation. Future Med Chem (2020) 12(7):583–92. doi: 10.4155/fmc-2019-0311
129. Yang J, Jia M, Zhang X, Wang P. Calycosin attenuates mptp-induced parkinson's disease by suppressing the activation of Tlr/NF-κB and mapk pathways. Phytother Res (2019) 33(2):309–18. doi: 10.1002/ptr.6221
130. Li Y, Zhang J-J, Chen R-J, Chen L, Chen S, Yang X-F, et al. Genistein mitigates oxidative stress and inflammation by regulating Nrf2/HO-1 and NF-κB signaling pathways in hypoxic-ischemic brain damage in neonatal mice. Ann Transl Med (2022) 10(2):32. doi: 10.21037/atm-21-4958
131. Rumman M, Pandey S, Singh B, Gupta M, Ubaid S, Mahdi AA. Genistein prevents hypoxia-induced cognitive dysfunctions by ameliorating oxidative stress and inflammation in the hippocampus. Neurotox Res (2021) 39(4):1123–33. doi: 10.1007/s12640-021-00353-x
132. Jiang T, Xu S, Shen Y, Xu Y, Li Y. Genistein attenuates isoflurane-induced neuroinflammation by inhibiting Tlr4-mediated microglial-polarization in vivo and in vitro. J Inflammation Res (2021) 14:2587–600. doi: 10.2147/JIR.S304336
133. Du K, Wu W, Feng X, Ke J, Xie H, Chen Y. Puerarin attenuates complete freund's adjuvant-induced trigeminal neuralgia and inflammation in a mouse model Via Sirt1-mediated tgf-B1/Smad3 inhibition. J Pain Res (2021) 14:2469–79. doi: 10.2147/JPR.S323588
134. Zeng J, Zheng S, Chen Y, Qu Y, Xie J, Hong E, et al. Puerarin attenuates intracerebral hemorrhage-induced early brain injury possibly by PI3k/Akt signal activation-mediated suppression of NF-κB pathway. J Cell Mol Med (2021) 25(16):7809–24. doi: 10.1111/jcmm.16679
135. Fu Y, Jia J. Isoliquiritigenin confers neuroprotection and alleviates amyloid-B42-Induced neuroinflammation in microglia by regulating the Nrf2/NF-κB signaling. Front Neurosci (2021) 15:638772. doi: 10.3389/fnins.2021.638772
136. Zhu X, Liu J, Chen S, Xue J, Huang S, Wang Y, et al. Isoliquiritigenin attenuates lipopolysaccharide-induced cognitive impairment through antioxidant and anti-inflammatory activity. BMC Neurosci (2019) 20(1):41. doi: 10.1186/s12868-019-0520-x
137. Zhu X, Liu J, Huang S, Zhu W, Wang Y, Chen O, et al. Neuroprotective effects of isoliquiritigenin against cognitive impairment Via suppression of synaptic dysfunction, neuronal injury, and neuroinflammation in rats with kainic acid-induced seizures. Int Immunopharmacol (2019) 72:358–66. doi: 10.1016/j.intimp.2019.04.028
138. Qin X, Chen J, Zhang G, Li C, Zhu J, Xue H, et al. Hydroxysafflor yellow a exerts anti-inflammatory effects mediated by Sirt1 in lipopolysaccharide-induced microglia activation. Front Pharmacol (2020) 11:1315. doi: 10.3389/fphar.2020.01315
139. Ren M, Zhang M, Zhang X, Wang C, Zheng Y, Hu Y. Hydroxysafflor yellow a inhibits aβ-induced neuroinflammation by modulating the phenotypic transformation of microglia Via TREM2/TLR4/NF-κB pathway in bv-2 cells. Neurochem Res (2022) 47(3):748–61. doi: 10.1007/s11064-021-03484-x
140. Yang X, Li Y, Chen L, Xu M, Wu J, Zhang P, et al. Protective effect of hydroxysafflor yellow a on dopaminergic neurons against 6-hydroxydopamine, activating anti-apoptotic and anti-neuroinflammatory pathways. Pharm Biol (2020) 58(1):686–94. doi: 10.1080/13880209.2020.1784237
141. Yang X, Chen L, Li Y, Gao F, Yan Z, Zhang P, et al. Protective effect of hydroxysafflor yellow a on cerebral ischemia reperfusion-injury by regulating GSK-3β-Mediated pathways. Neurosci Lett (2020) 736:135258. doi: 10.1016/j.neulet.2020.135258
142. Gao J-M, Zhang X, Shu G-T, Chen N-N, Zhang J-Y, Xu F, et al. Trilobatin rescues cognitive impairment of alzheimer's disease by targeting Hmgb1 through mediating Sirt3/SOD2 signaling pathway. Acta Pharmacol Sin (2022) 43(10):2482–94. doi: 10.1038/s41401-022-00888-5
143. Ding J, Huang J, Yin D, Liu T, Ren Z, Hu S, et al. Trilobatin alleviates cognitive deficits and pathologies in an alzheimer's disease mouse model. Oxid Med Cell Longev (2021) 2021:3298400. doi: 10.1155/2021/3298400
144. Gao J, Chen N, Li N, Xu F, Wang W, Lei Y, et al. Neuroprotective effects of trilobatin, a novel naturally occurring Sirt3 agonist from rehd., mitigate cerebral Ischemia/Reperfusion injury: Involvement of TLR4/NF-κB and Nrf2/Keap-1 signaling. Antioxid Redox Signal (2020) 33(2):117–43. doi: 10.1089/ars.2019.7825
145. Ghumatkar PJ, Patil SP, Peshattiwar V, Vijaykumar T, Dighe V, Vanage G, et al. The modulatory role of phloretin in aβ induced sporadic alzheimer's disease in rat model. Naunyn Schmiedebergs Arch Pharmacol (2019) 392(3):327–39. doi: 10.1007/s00210-018-1588-z
146. Zhang G, Yang G, Liu J. Phloretin attenuates behavior deficits and neuroinflammatory response in mptp induced parkinson's disease in mice. Life Sci (2019) 232:116600. doi: 10.1016/j.lfs.2019.116600
147. Kang J, Wang Z, Oteiza PI. (-)-Epicatechin mitigates high fat diet-induced neuroinflammation and altered behavior in mice. Food Funct (2020) 11(6):5065–76. doi: 10.1039/d0fo00486c
148. Navarrete-Yañez V, Garate-Carrillo A, Rodriguez A, Mendoza-Lorenzo P, Ceballos G, Calzada-Mendoza C, et al. Effects of (-)-Epicatechin on neuroinflammation and hyperphosphorylation of tau in the hippocampus of aged mice. Food Funct (2020) 11(12):10351–61. doi: 10.1039/d0fo02438d
149. Mao L, Hochstetter D, Yao L, Zhao Y, Zhou J, Wang Y, et al. Green tea polyphenol (-)-Epigallocatechin gallate (Egcg) attenuates neuroinflammation in palmitic acid-stimulated bv-2 microglia and high-fat diet-induced obese mice. Int J Mol Sci (2019) 20(20):5081. doi: 10.3390/ijms20205081
150. Bao J, Liu W, Zhou H-Y, Gui Y-R, Yang Y-H, Wu M-J, et al. Epigallocatechin-3-Gallate alleviates cognitive deficits in App/Ps1 mice. Curr Med Sci (2020) 40(1):18–27. doi: 10.1007/s11596-020-2142-z
151. Uniyal A, Akhilesh, Tiwari V, Gadepalli A, Ummadisetty O, Tiwari V. Epigallocatechin-3-Gallate improves chronic alcohol-induced cognitive dysfunction in rats by interfering with neuro-inflammatory, cell death and oxido-nitrosative cascade. Metab Brain Dis (2021) 36(7):2141–53. doi: 10.1007/s11011-021-00794-5
152. Wang JP, Xu S, Chen XH, Wang L, Li JY, Li GJ, et al. Antidepressant effect of egcg through the inhibition of hippocampal neuroinflammation in chronic unpredictable mild stress-induced depression rat model. J Funct Foods (2020) 73:104106. doi: 10.1016/j.jff.2020.104106
Keywords: natural flavonoids, neuroinflammation, microglia, astrocytes, brain disorders
Citation: Chen Y, Peng F, Xing Z, Chen J, Peng C and Li D (2022) Beneficial effects of natural flavonoids on neuroinflammation. Front. Immunol. 13:1006434. doi: 10.3389/fimmu.2022.1006434
Received: 29 July 2022; Accepted: 03 October 2022;
Published: 24 October 2022.
Edited by:
Jason C. O’Connor, The University of Texas Health Science Center at San Antonio, United StatesReviewed by:
Shathiswaran N. Andy, University of Malaya, MalaysiaCopyright © 2022 Chen, Peng, Xing, Chen, Peng and Li. This is an open-access article distributed under the terms of the Creative Commons Attribution License (CC BY). The use, distribution or reproduction in other forums is permitted, provided the original author(s) and the copyright owner(s) are credited and that the original publication in this journal is cited, in accordance with accepted academic practice. No use, distribution or reproduction is permitted which does not comply with these terms.
*Correspondence: Cheng Peng, cGVuZ2NoZW5nX2NkQDEyNi5jb20=; Dan Li, bGlkYW5AY2R1dGNtLmVkdS5jbg==
†These authors have contributed equally to this work and share first authorship
Disclaimer: All claims expressed in this article are solely those of the authors and do not necessarily represent those of their affiliated organizations, or those of the publisher, the editors and the reviewers. Any product that may be evaluated in this article or claim that may be made by its manufacturer is not guaranteed or endorsed by the publisher.
Research integrity at Frontiers
Learn more about the work of our research integrity team to safeguard the quality of each article we publish.