- 1Laboratory of Oral Histopathology, Faculty of Health Sciences, University of Brasília, Brasília, DF, Brazil
- 2Department of Analytical, Bioanalytical Sciences and Miniaturization, École Supérieure de Physique et de Chimie Industrielles (ESPCI) de la Ville de Paris, Paris, France
- 3Faculté de Chirurgie Dentaire, Université Paris Descartes Sorbonne 12 Rue de l’École de Médecine, Paris, France
Since the introduction of efficient vaccines anti-SARS-CoV-2, antibody quantification becomes increasingly useful for immunological monitoring and COVID-19 control. In several situations, saliva samples may be an alternative to the serological test. Thus, this rapid systematic review aimed to evaluate if saliva is suitable for SARS-CoV-2 detection after vaccination. For this purpose, search strategies were applied at EMBASE, PubMed, and Web of Science. Studies were selected by two reviewers in a two-phase process. After selection, 15 studies were eligible and included in data synthesis. In total, salivary samples of approximately 1,080 vaccinated and/or convalescent individuals were analyzed. The applied vaccines were mostly mRNA-based (BioNTech 162b2 mRNA/Pfizer and Spikevax mRNA-1273/Moderna), but recombinant viral-vectored vaccines (Ad26. COV2. S Janssen - Johnson & Johnson and Vaxzevria/Oxford AstraZeneca) were also included. Different techniques were applied for saliva evaluation, such as ELISA assay, Multiplex immunoassay, flow cytometry, neutralizing and electrochemical assays. Although antibody titers are lower in saliva than in serum, the results showed that saliva is suitable for antibody detection. The mean of reported correlations for titers in saliva and serum/plasma were moderate for IgG (0.55, 95% CI 0.38-9.73), and weak for IgA (0.28, 95% CI 0.12-0.44). Additionally, six out of nine studies reported numerical titers for immunoglobulins detection, from which the level in saliva reached their reference value in four (66%). IgG but not IgA are frequently presented in saliva from vaccinated anti-COVID-19. Four studies reported lower IgA salivary titers in vaccinated compared to previously infected individuals, otherwise, two reported higher titers of IgA in vaccinated. Concerning IgG, two studies reported high antibody titers in the saliva of vaccinated individuals compared to those previously infected and one presented similar results for vaccinated and infected. The detection of antibodies anti-SARS-CoV-2 in the saliva is available, which suggests this type of sample is a suitable alternative for monitoring the population. Thus, the results also pointed out the possible lack of mucosal immunity induction after anti-SARS-CoV-2 vaccination. It highlights the importance of new vaccination strategies also focused on mucosal alternatives directly on primary routes of SARS-CoV-2 entrance.
Systematic Review Registration: https://www.crd.york.ac.uk/prospero/display_record.php?ID=CRD42022336968, identifier CRD42022336968.
Introduction
Since the COVID-19 outbreak, the development of vaccines is the highest priority due to the rapid transmission and lethality of SARS-CoV-2. Although the development of a safe and effective vaccine is a long and complicated process that typically takes 10 to 15 years (1), the scientific community turned it into an active and powerful field to develop emerging vaccines at an unprecedented speed (2, 3). The main COVID-19 vaccine type currently available are messenger RNA (mRNA) based, including BioNTech 162b2 mRNA/Pfizer (BNT) and Spikevax mRNA-1273/Moderna (MOD). Soon there were also recombinant viral-vectored vaccines, as Ad26. COV2. S Janssen - Johnson & Johnson (JJ), Vaxzevria/Oxford AstraZeneca (AZD), and inactivated virus approaches, like CoronaVac (Sinovac/Butantan) and Covaxin (Bharat Biotech) (4).
From the urgent introduction of these vaccines anti-SARS-CoV-2 worldwide until now, more than 60% of the world population had already received a full initial protocol of vaccination (5). The public health effect was mostly in the reduction of symptomatic and severe cases, impacting also on proportionate mortality caused by COVID-19 (4, 6–8). In this regard, vaccines anti-COVID-19 proved to be effective in inducing humoral immunity (9). However, antibody titers declined over time after vaccination, with a subsequent reduction in neutralizing activity (10–13).
In this sense, frequent population follow-up on antibody quantification becomes increasingly useful for immunological monitoring and COVID-19 control after vaccination. Serological testing for SARS-CoV-2 antibody is the standard reference, being important to assess immunological responses after both vaccine and infection (14). Nevertheless, the invasive process needed for blood collection can limit its employment as a frequent method. As an alternative, saliva has been reported to be a rich biofluid in the assessment of immunity for several diseases, especially those in which the mouth is a route of infection (8, 15–17). Oral fluid, more commonly named “saliva”, is a complex mixture of salivary gland secretions, gingival crevicular exudate, oral microorganisms, and food debris. Thus, oral fluid is a potential source of immunoglobulins, such as immunoglobulin G (IgG) issued from the blood and reaching the oral cavity by the gingival crevicular fluid and immunoglobulin A (IgA) issued from the salivary glands. The production of secretory IgA reflects mucosal immunity, which may impact COVID-19 transmission in addition to the current reduction of symptomatic and severe cases (18–20). Moreover, saliva collection is easy, non-invasive, and requires relatively simple instructions, representing several advantages over blood samples (19, 21).
Thus, the characterization of coronavirus saliva-specific signatures could provide valuable information towards antibodies anti-SARS-CoV-2 in vaccinated individuals. So, this rapid systematic review aims to verify whether saliva is suitable for SARS-CoV-2 antibody (immunoglobulins) detection after vaccination.
Methods
A rapid systematic review was undertaken to evaluate whether saliva is suitable for SARS-CoV-2 antibodies detection after vaccination. Rapid systematic reviews are a knowledge generation strategy that provides high evidence in a short timeframe to support clinical and policy decision-making, especially during disease outbreaks (22, 23). Thus, the methodology was systematized as suggested by the PRISMA guideline (24) with some adaptations, such as a shorter search strategy, faster data extraction, and mostly qualitative synthesis (PROSPERO Protocol - CRD42022336968). The purpose is to provide urgent information with reference to the potential of saliva in assessing immunological response after vaccination anti-SARS-CoV-2. In addition, our evidence also contributes to the new discussion on the induction of mucosal immunity.
Search strategy and inclusion criteria
Electronic search strategies were developed and applied to Embase, PubMed, and Web of Science (Appendix 1). The search included all articles published until May 8, 2022, without language restrictions. The inclusion criteria were based on the PICOS strategy, in which Population (P): Human vaccinated for SARS-CoV-2, infected or uninfected; Intervention (I): Anti-SARS-CoV-2 vaccine; Comparator/control (C): Humans not vaccinated for COVID-19; Outcomes (O): detection of SARS-CoV-2 antibodies in saliva, type of vaccine, type of antibodies, methods and techniques for detection; and Studies (S): observational studies.
Study selection, data collection and synthesis
The selection was completed in a systematic two-phase process by VTC and JAS. A third author (ENSG) was involved when required to make a final decision. Final selection was always based on the full text of the publication. VTC and JAS collected the required information from each selected article and ENSG cross-checked all data to confirm its accuracy. Primary outcome was the detection of antibodies in saliva, considering correlation analysis of saliva and serum/plasma, and detectable capacity using titers reference values and proportions. Secondary outcomes included comparison of titers levels among variable characteristics. The qualitative synthesis was conducted by grouping and comparing data reported in included studies in relation to primary and secondary outcomes. Additionally, graphics were conducted to better illustrate the outcomes analyzed in each study. For correlation data, the coefficient of each study reporting this analysis were collected and grouped. Then, the mean with standard deviation were calculated without comparison or statistical tests. The GraphPad Prism software version 9.4.0 (GraphPad Software, La Jolla California USA) was used to construct the graphics.
Results
Selection and characteristics of studies
In the first phase, 178 studies were identified through databases, and after removing duplicates, 134 references remained for screening titles and abstracts. From that, 97 records were excluded and 37 were selected to phase 2. A full-text reading was conducted on 35 references since two were not available. Based on inclusion criteria, 20 articles were excluded, and 15 studies were selected for the synthesis of results (12, 16, 25–37). The flow diagram summarizing the selection process is presented in Figure 1.
Of the included articles, 11 are longitudinal studies (12, 16, 25–28, 30, 32, 35–37), and four are cross-sectional studies (29, 31, 33, 34). All studies were published in English between 2021 and 2022. Five of them were conducted in Italy (25, 27, 28, 31, 32), three in United States (12, 16, 33), three in Germany (30, 34, 35), two in Canada (26, 36), one in Croatia (29), and one in Australia (37).
Summary of results
Considering the 15 included studies, approximately a total of 1,080 vaccinated and/or convalescent individuals were analyzed. The most evaluated vaccine was the BioNTech 162b2 mRNA/Pfizer (BNT) (15 studies, around 637 vaccinated), followed by Spikevax mRNA-1273/Moderna (MOD) (five studies, about 77 vaccinated), Vaxzevria/Oxford AstraZeneca (AZD) (three studies, approximately 44 vaccinated), and Ad26. COV2. S Janssen (JJ) (one study, one vaccinated). Although the sample of main interest consisted of serum/plasma and saliva of vaccinated individuals, some studies also included healthy and previous infected ones as a comparison. From this, only two studies did not include participants previously infected with SARS-CoV-2 (25, 29).
Saliva samples were mainly collected using cotton devices (n=6), such as Salivette® and similar (26, 29, 32, 35–37), splitting methods (n=4) (12, 25, 30, 31) and aspiration (n=1) (27). Some papers did not report the collection method (n=4) (16, 28, 33, 34). The methods of analysis included Enzyme-linked immunosorbent assay (ELISA), Multiplex bead assays, Electro-Chemiluminescence immunoassay (ECLIA), Flow Cytometry (FC), and Chemiluminescence Immunoassay (CLIA). Additionally, five studies evaluated neutralizing activity anti-SARS-CoV-2 (Figure 2A).
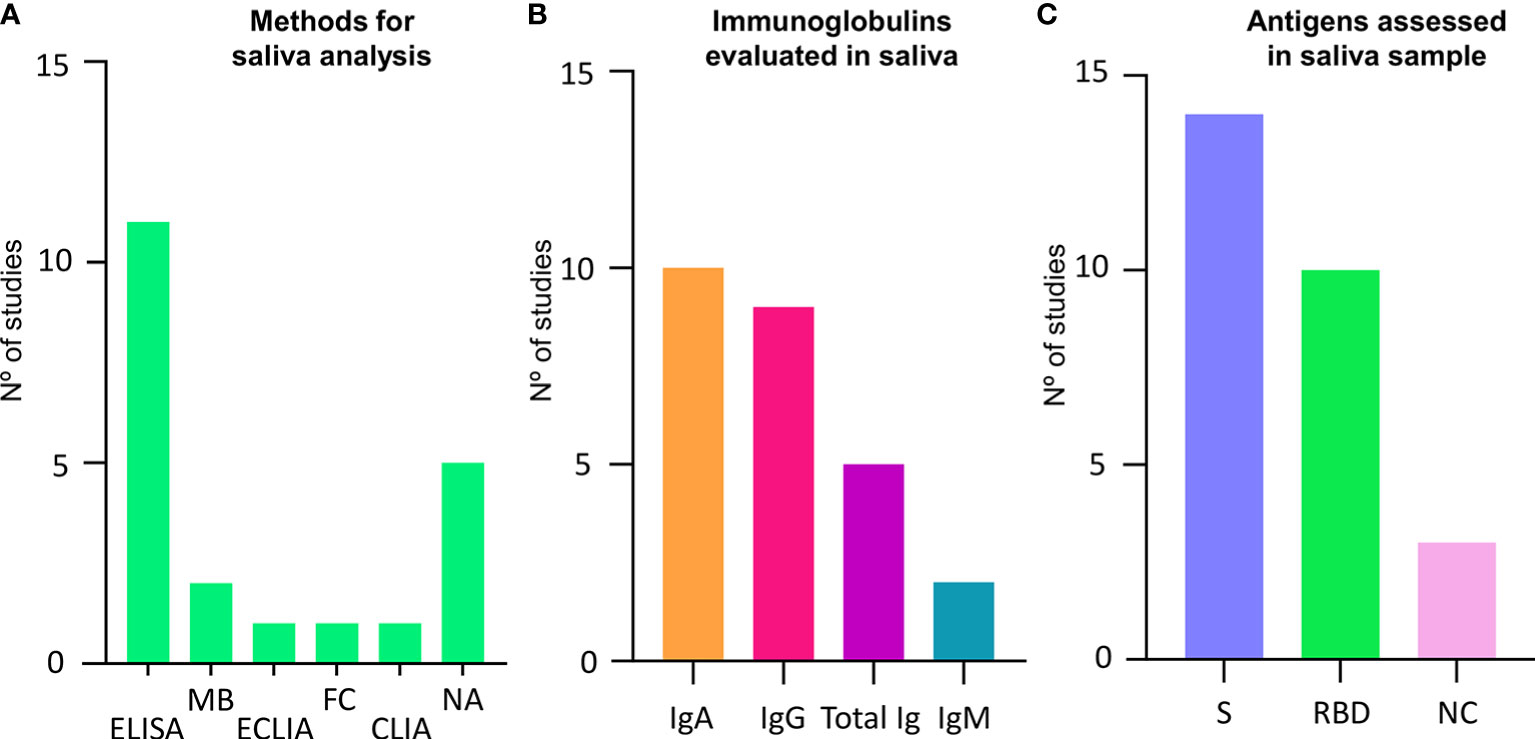
Figure 2 Characteristics of studies and antigen analysis in salivary samples. (A) Graphic showing the methods assessed and assays applied for saliva analysis and the number of studies using each type. (B) Graphic showing different immunoglobulins assessed and the number of studies assessing each type. (C) Graphic showing different antigen assessed for binding reaction and the number of studies assessing each type. CLIA, chemiluminescence immunoassay; ECLIA, electro-chemiluminescence immunoassay; ELISA, Enzyme-linked immunosorbent assay; FC, Flow Cytometry; IgA, Immunoglobulin A; IgG, immunoglobulin G; IgM,Immunoglobulin M; MB, Multiplex Bead; NA, Neutralizing activity; NC, nucleocapsid; NR, Not reported; BD, receptor binding domain; S, spike protein; Total Ig, Total immunoglobulins.
Saliva samples were evaluated for IgA in 10 studies and for IgG in nine. Total Igs were assessed in five, while IgM was assessed only in two studies (Figure 2B). Concerning specific antigens for antibodies detection, 14 studies assessed the spike protein (S) subunits 1 and 2 or whole trimer, 10 studies used the RBD region, and three the nucleocapsid (NC) (Figure 2C). Detailed information can be found in Table 1.
Correlation of immunoglobulins detection in serum/plasma and saliva
To assess the correlation between antibodies quantity in serum/plasma and saliva, seven analyses were reported for IgG, three for IgA, and two for Total Igs. All studies reported linear correlation; however, the results were heterogeneous. The mean coefficients reported for IgG were 0.55 (95% CI 0.38-9.73), indicating a moderated correlation that varies between weak and strong (Figure 3A). The mean correlation coefficient for IgA was 0.28 (95% CI 0.12-0.44), representing a weak correlation result (Figure 3A). A mean analysis for Total Igs correlation in serum/plasma and saliva was not feasible. The individual reports for Total Igs presented a strong correlation in Lapic et al. (29) study (Spearman correlation; r=0.66), and no correlation in Robinson et al. (36) study (Linearity analysis; p=0.90).
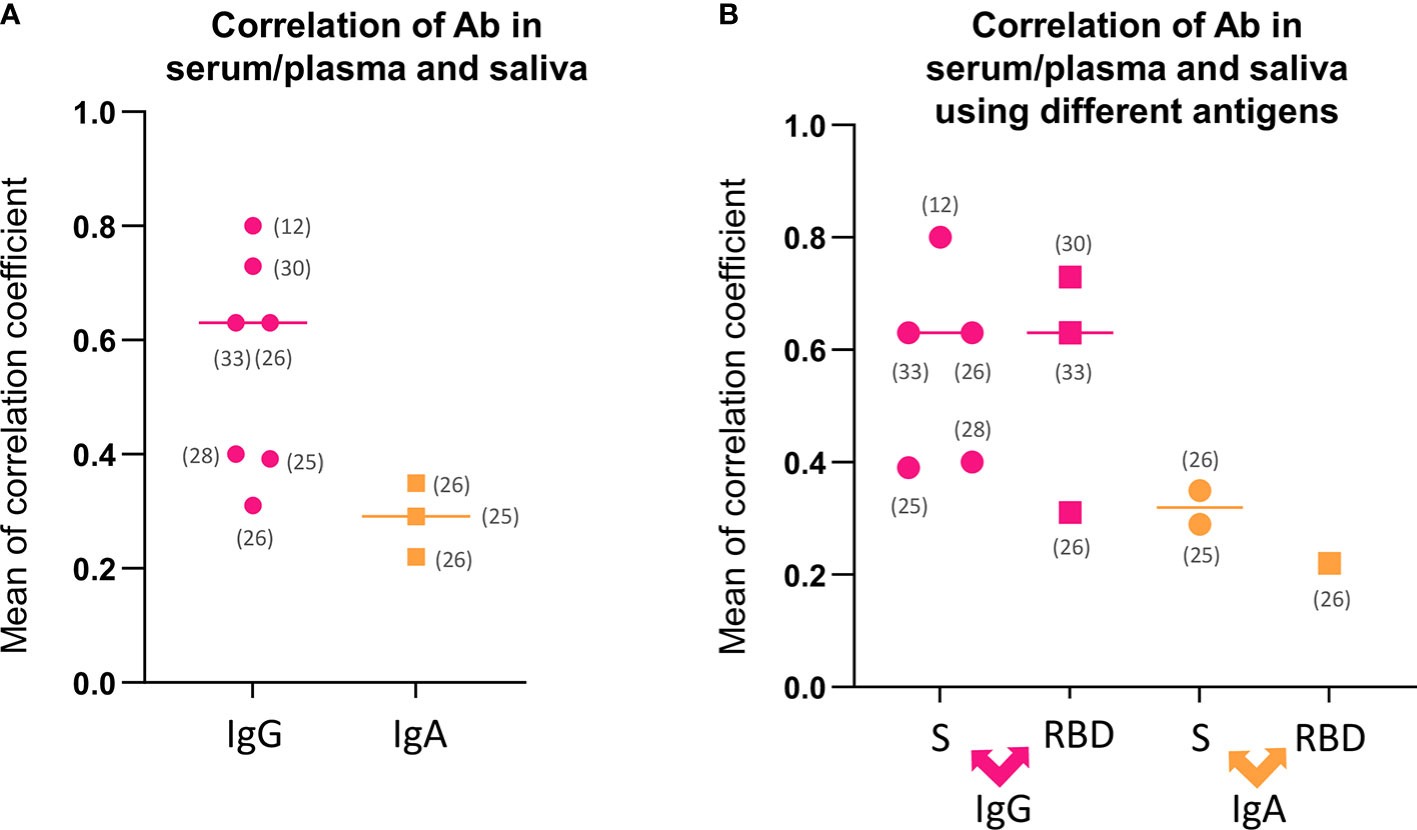
Figure 3 Correlations analysis regarding antibodies detection in serum/plasma and saliva. (A) Mean of correlation coefficients between antibodies detection in serum/plasma and saliva for IgG and IgA. (B) Mean of correlation coefficients between antibodies detection separated by antigen-antibody reaction for IgG and IgA. IgA, Immunoglobulin A; IgG, immunoglobulin G; RBD, receptor binding domain; S, spike protein; Total Ig, Total immunoglobulins.
These results lead to a question of whether there is a similarity in correlation strongness when the different antigen-antibody reaction was detected. Thus, the correlations performed with anti-S and anti-RBD were separated, and the mean coefficients were compared for IgG and IgA. The mean analysis showed 0.57 (95% CI 0.35-0.78) for anti-S IgG, 0.55 (95% CI 0.01-1.02) anti-RBD IgG, 0.32 (95% CI -0.06-0.70) for anti-S IgA, and 0.22 for anti-RBD IgA (Figure 3B), which suggests approximate results using different antigen binding.
Only three studies reported accuracy values for salivary analysis (Table 1). Sensitivity was higher than specificity in two studies (99% and 88%; 100% and 86.5%, respectively). The other study reported just the sensitivity value of 75% six months after the second dose.
Immunoglobulins titers in saliva
Immunoglobulins levels in serum/plasma and saliva were assessed as an outcome in all included studies. Table 2 presents the summary of the nine studies that reported antibody titers or proportions detected in saliva versus serum/plasma. There were two ways of reporting: 1. Quantification of antibody titers, and 2. Proportion of individuals with positive detection. The studies presented a main increasing pattern of titers before vaccination, and after first and second doses. On the contrary, Sheikh-Mohamed et al. (26) reported higher detection after one dose than after two doses. Most studies reported higher antibody titers in serum/plasma than in saliva. Six out of nine studies reported the numerical quantification of antibody titers, from which titers’ level in saliva was able to reach the reference value for detection in four studies. The two reminding studies did not define an objective reference value for saliva standardized analysis. Three studies presented proportion values showing that saliva was suitable for antibody detection in all (100%) with superior percentages for IgG than IgA in two studies that assessed both immunoglobins.
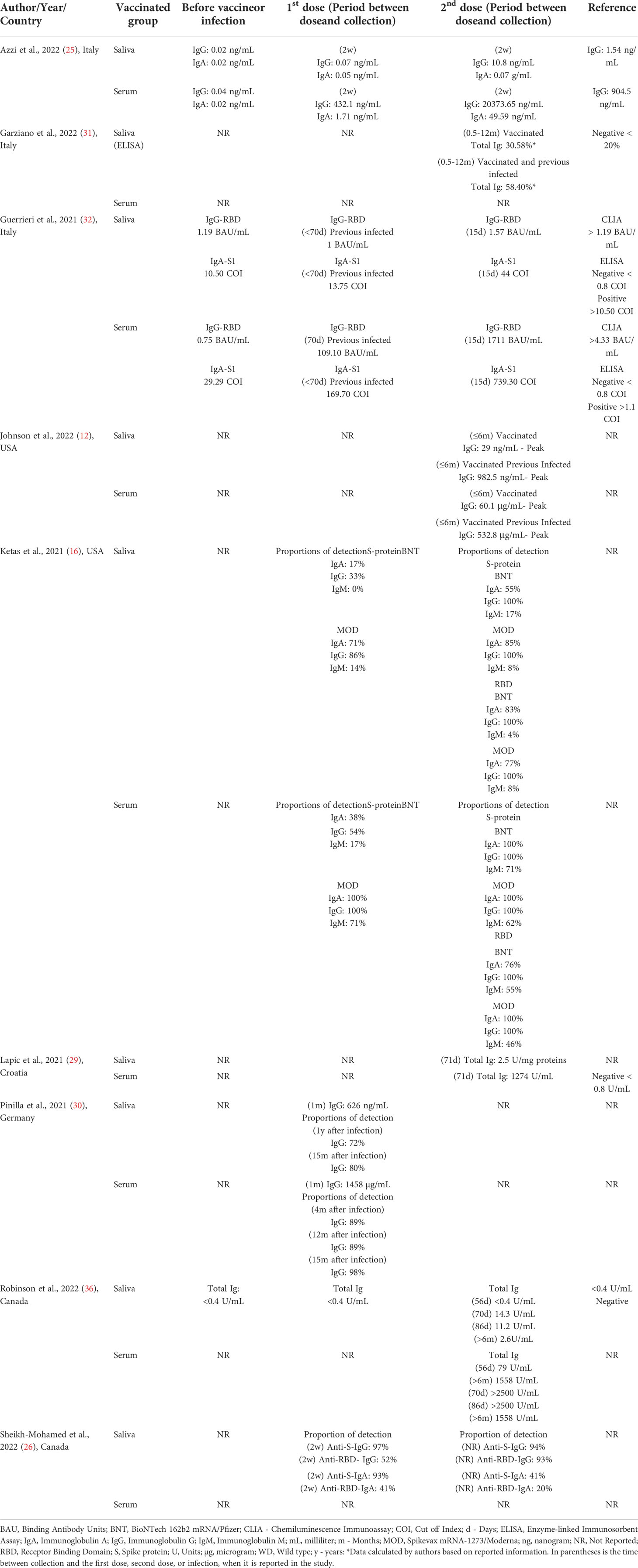
Table 2 Antibodies titers or proportion of detection in saliva versus serum/plasma of studies that expressly reported numerical data.
Furthermore, eight studies performed the comparison of IgA titers in saliva between vaccinated and previous infected individuals with or without vaccine doses. Four studies reported lower IgA salivary titers in vaccinated without previous infection (50%), otherwise, two reported higher titers of IgA in those individuals (25%%). One reported conflicting results showing higher titers in vaccinated compared to mild/moderate COVID-19 cases and lower titers compared to severe ones (12.5%). In addition, one study failed to detect values for both groups of individuals (12.5%) (Figure 4A). Toward IgG, two studies reported high levels in saliva of only vaccinated individuals compared to previous infected ones (66%) and one reported similar results for vaccinated and infected (33%) (Figure 4B).
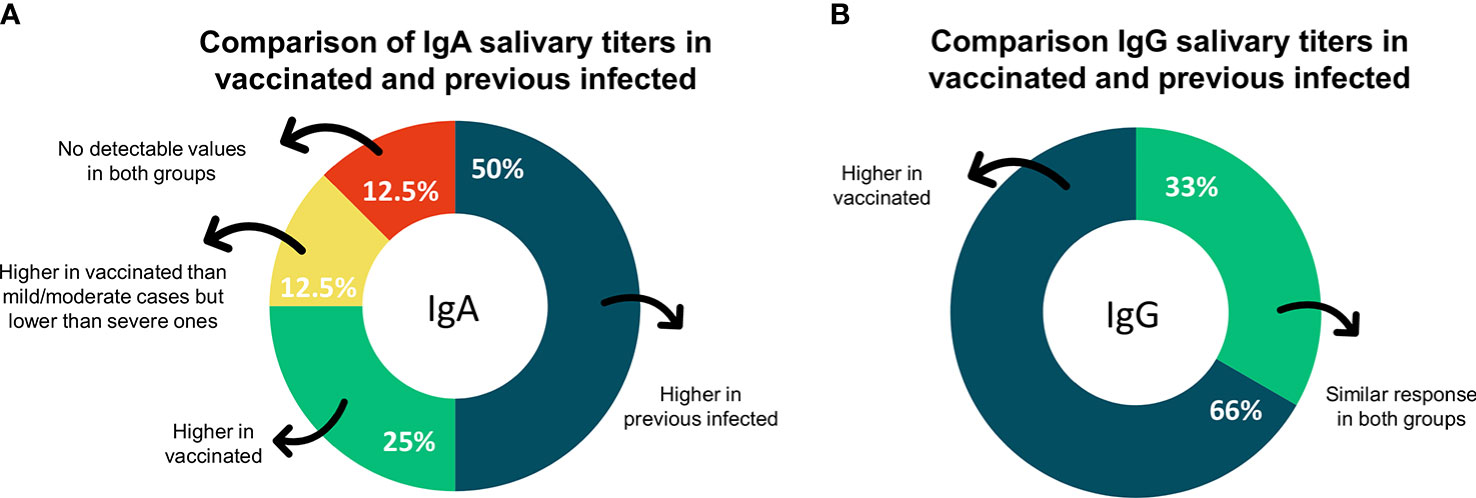
Figure 4 The proportion of studies on secondary outcomes. (A) Graphic showing the proportion of studies reporting results on IgA comparison between vaccinated and previous infected individuals (B) Graphic showing the proportion of studies reporting results on IgG comparison between vaccinated and previous infected individuals. IgA, immunoglobulin A; IgG, Immunoglobulin G.
Neutralizing activity
Two studies reported the main conclusions on neutralization correlations (Table 1). Garziano et al. (31) assessed the correlation of neutralizing activity in plasma and saliva. The reported results showed stronger coefficients for individuals who were previously infected with (r=0.52) or without (r=0.55) vaccination, compared to those uninfected and vaccinated (r=0.03). Meyer-Ardnt et al. (34) evaluated the correlation of salivary secretory IgA and neutralizing activity, showing weak results for elderly vaccinated individuals (r=0.46 after 28 days; r=0.44 after 49 days), very weak for middle-aged vaccinated (r=0.38 after 28 days; r=0.08 after 49 days), and moderate for previous infected ones (r=0.66 after 28 days; r=0.59 after 49 days). Additionally in neutralizing fields, Nickel et al. (35) was the only study reporting neutralization after the third dose of vaccine. The results provided evidence of stronger neutralizing activity in the group receiving heterologous vaccination protocol (AZD-BNT) compared to homologous one (BTN-BNT). Thus, a combination of different SARS-CoV-2 vaccine classes seems to lead to a stronger humoral immune response which may result in a better protective effect.
Discussion
SARS-CoV-2 is an enveloped single-stranded RNA virus, of which surface glycoprotein spike mediates viral entrance into host cells, especially through angiotensin-converting enzyme 2 (ACE2) (38). Against it, antibodies modulate cell infection by neutralizing viral antigen binding (39). Potent neutralizing antibodies were readily isolated from convalescent individuals, suggesting that SARS-CoV-2 is a neutralization-sensitive virus. Those neutralizing antibodies are targeted against the RBD motif of the spike protein, which is a relevant antigen to vaccines goals (40, 41). In this context, vaccine based on mRNA anti-SARS-CoV-2 was the most reported in this review. It demonstrated the capability of inducing antibodies in both previously infected and not infected individuals, increasing humoral and cellular immunity after the second vaccination dose (42). Furthermore, mRNA vaccines encode trimerized RBD which is modified by adding a “foldon” trimerization domain to increase immunogenicity. The result is the induction of anti-RBD neutralizing antibodies specific for SARS-COV-2 in plasma, and a T cell response with Th1 cytokine and low-level CD8 T cell (43).
In this systematic review, the main outcome was the correlation between serum/plasma and saliva antibodies, with the purpose of comparing the two types of samples source. Among the techniques for detecting antibodies, ELISA is one of the most used for serological tests (44). For years, its use has been also reported for saliva samples in different disease responses, such as hepatitis A (45), leprosy (46), and autism spectrum disorder (47). Thus, ELISA is suggested as a method to detect anti-COVID-19 antibodies in saliva. In this view, as most SARS-CoV-2 vaccines in use or in advanced development are based on the viral spike protein subunits, the antigen used for antibody detection is the S protein or its RBD region. Protein S is present on virions as prefusion trimers, where RBD is arbitrarily open or closed (41). IgG, mainly IgG1, dominates S- and RBD-specific antibody responses, which are intended against structurally folded S and RBD and three distinct peptide epitopes in S2. Although immunity assessment assays may vary respecting antigen-antibodies reactions, the synthesis of results suggests that it does not impact antibody detection after vaccination.
From our results, the mean correlation coefficient between serum/plasma and saliva was stronger for IgG than IgA. Nevertheless, at mucosal sites secretory IgA act with an essential role in protection mucosal surfaces by preventing the binding of viruses to epithelial cells (48). Salivary IgG, as well as a very limited amount of monomeric IgA, are derived from plasma via gingival crevices and could participate in viral protection (28, 49). Some articles comparing the immune responses of vaccinated and previously infected individuals suggested that salivary IgA titers were higher in the saliva of the infected, whereas IgG presented a higher salivary titer in vaccinated individuals (25, 27, 28, 35). As the current anti-COVID-19 vaccines used a systemic injection, they predominantly induce circulatory IgG (20, 50) indicating that after a mRNA vaccination, the IgG are translocated into saliva in sufficient amounts to have a high predictive value of induced seroconversion. However, as the testing methods used only the S protein (or its RDB subunit) as antigens, it is difficult to compare the IgG titers obtained after vaccination or natural infection. Indeed, except for vaccines that used inactivated viruses, the vaccinal antigen is based on the S protein, and only anti-S antibodies were obtained after vaccination, whereas the natural infection induces various antibodies specific to the various proteins of the virus (39). Thus, the testing methods are built to evidence the performance of the vaccination rather than the complexity of the antibody response obtained after natural infection. For IgA, several reports concluded that SARS-CoV-2 infection was associated with a mucosal secretory IgA response (25, 48) whereas, in vaccinated individuals, the IgA present in saliva were from blood origin (28). Thus, salivary IgAs induced by vaccination seems to be mainly exuded from serum while in previous infected individuals it came from a local mucosal immunity response.
Neutralizing antibodies were also assessed in the included studies. Viral neutralization plays a key role in anti-viral immunity and assessing its capacity is an important strategy to measure protective immunity (43, 51). In serum, neutralizing activity seems to be similar in previously infected individuals, either vaccinated or not, and uninfected vaccinated ones. However, neutralizing activity in saliva was high in convalescents and scarcely detected after vaccination (31). This observation could be explained by the absence of mucosal immunity induction in vaccinated individuals, associated with a quantity of Ig issued from the crevicular fluid that was insufficient to be neutralizing. Furthermore, one dose of vaccine was able to boost an anti-SARS-CoV-2 response in previously infected individuals, whilst the third dose with a different vaccine type led to a significantly stronger response than only two doses (31, 35). Although a neutralizing activity was detected in saliva, intramuscular vaccines are not proved to be effective in producing salivary effects.
Besides the protection against the severe form of COVID-19, it is also essential to understand whether and how vaccination can decrease SARS-CoV-2 transmission (52). According to included studies, available data provided a weak response of intramuscular vaccines to elicit readily detectable mucosal immunity, suggesting the importance of local induction. Considering the respiratory tropism of the SARS-CoV-2 virus, a vaccine delivered intranasally would be useful to induce mucosal immunity directly at the port of virus entrance, also preventing transmission (53). Furthermore, nasal immunization is better than parenteral routes when seeking to achieve mucosal immunity, since the capability to induce IgA specific for SARS-CoV-2 in the respiratory tract may avoid virus spreading to the lung and avert respiratory problems (54). In this field, orally and intranasally administered vaccines have already been approved for humans against various mucosal pathogens (55). Currently, at least 12 projects are presenting intranasal candidates anti-SARS-CoV-2 at pre-clinical or clinical phases (56). In addition to potentially inducing sterilizing immunity, intranasal alternatives for COVID-19 vaccines are predominantly focused on viral vectors and protein subunits, representing safer delivery platforms than the whole pathogens used in all the licensed mucosal vaccines (55, 56). However, the development of a new safe, and an efficient mucosal vaccine is a complex process and several factors including antigen doses, formulation, administration route and adjuvants should be considered (54, 57). Thus, the kinetics and durability of the mucosal responses are also key factors in vaccine development. As mucosal vaccines seem to be potential alternatives for decreasing SARS-CoV-2 transmission, many efforts may focus on those strategies development turning into a current field of interest.
There are some limitations to be highlighted. First, the used techniques for antibodies detection in saliva were validated for serum analysis, resulting in difficulties to establish reference values for saliva quantification in some studies. Second, the included studies are highly heterogeneous with respect to samples, collection intervals, and strategies for reporting data. Third, in some studies, the sample size was not well defined for all reported results, varying during the collection and analysis period process. Lastly, as COVID-19 is an urgent field, especially on vaccination at this time, we also included pre-print studies and letters for the editor, which impact detailed collection and quality.
Conclusion
Saliva is a suitable biofluid alternative for anti-SARS-CoV-2 antibodies detection in vaccinated and in previously infected individuals. Although salivary antibody titers are lower than serum titers, the detection of anti-SARS-CoV-2 immunoglobulins in saliva are satisfactory. Concerning specific immunoglobulins in vaccinated individuals, saliva seems to frequently present IgG but not uniformly IgA. The mean correlations in serum/plasma and saliva were moderate for IgG and weak for IgA. Thus, the results also suggest and pointed out the possible lack of mucosal immunity induction after anti-SARS-CoV-2 vaccination. It highlights the importance of new vaccination strategies focused also on mucosal alternatives directly on primary routes of SARS-CoV-2 entrance.
Data availability statement
The original contributions presented in the study are included in the article/Supplementary Material. Further inquiries can be directed to the corresponding author.
Author contributions
EG, AA, and HC designed the study. EG, VC, and JA wrote the first draft of the manuscript. JA conducted the search strategy. VC and JA selected the articles. EG was involved when required to make a final decision. VC and JA collected the required information from each selected article and EG cross-checked all data to confirm its accuracy. AA and HC reviewed and revised the draft. All authors contributed to the article and approved the submitted version.
Funding
This study received financial support by COPEI-DPI/DEX, University of Brasilia. It was supported by FAPDF (Foundation for Research Support of the Federal District, Brazil, Edital 04/2021 (PI: Guerra - 00193-00001145/2021-12). VC and JA are supported by CAPES (Coordination for the Improvement of Higher Education Personnel), Brazil. EG is supported by the CNPq (National Council for Scientific and Technological Development), Ministry of Education, Brazil.
Conflict of interest
The authors declare that the research was conducted in the absence of any commercial or financial relationships that could be construed as a potential conflict of interest.
Publisher’s note
All claims expressed in this article are solely those of the authors and do not necessarily represent those of their affiliated organizations, or those of the publisher, the editors and the reviewers. Any product that may be evaluated in this article, or claim that may be made by its manufacturer, is not guaranteed or endorsed by the publisher.
Supplementary material
The Supplementary Material for this article can be found online at: https://www.frontiersin.org/articles/10.3389/fimmu.2022.1006040/full#supplementary-material
References
1. Rahim F, Khakimova A, Ebrahimi A, Zolotarev O, Nasab FR. Global scientific research on sars-cov-2 vaccines: A bibliometric analysis. Cell J (2021) 23(5):523–31. doi: 10.22074/cellj.2021.7794
2. Su S, Du L, Jiang S. Learning from the past: Development of safe and effective COVID-19 vaccines. Nat Rev Microbiol (2021) 19(3):211–9. doi: 10.1038/s41579-020-00462-y
3. Mistry P, Barmania F, Mellet J, Peta K, Strydom A, Viljoen IM, et al. SARS-CoV-2 variants, vaccines, and host immunity. Front Immunol (2022) 12:809244. doi: 10.3389/fimmu.2021.809244
5. Ritchie H, Mathieu E, Rodés-Guirao L, Appel C, Giattino C, Ortiz-Ospina E, et al. “Coronavirus pandemic (COVID-19).” Our world in data. OurWorldInData.org (2020). Available at: https://ourworldindata.org/coronavirus.
6. Victora CG, Castro MC, Gurzenda S, Medeiros AC, França GVA, Barros AJD. Estimating the early impact of vaccination against COVID-19 on deaths among elderly people in Brazil: Analyses of routinely-collected data on vaccine coverage and mortality. eClinicalMedicine (2021) 38:1-6. doi: 10.1016/j.eclinm.2021.101036
7. Suthar AB, Wang J, Seffren V, Wiegand RE, Griffing S, Zell E. Public health impact of covid-19 vaccines in the US: Observational study. BMJ (2022) 377:e069317. doi: 10.1136/bmj-2021-069317
8. Huang N, Pérez P, Kato T, Mikami Y, Okuda K, Gilmore RC, et al. SARS-CoV-2 infection of the oral cavity and saliva. Nat Med (2021) 27(5):892–903. doi: 10.1038/s41591-021-01296-8
9. Becker M, Dulovic A, Junker D, Ruetalo N, Kaiser PD, Pinilla YT, et al. Immune response to SARS-CoV-2 variants of concern in vaccinated individuals. Nat Commun (2021) 12(1):1–8. doi: 10.1038/s41467-021-23473-6
10. Dan JM, Mateus J, Kato Y, Hastie KM, Yu ED, Faliti CE, et al. Immunological memory to SARS-CoV-2 assessed for up to 8 months after infection. Sci (2021) 371(6529):1-22. doi: 10.1126/science.abf4063
11. Ibarrondo FJ, Hofmann C, Fulcher JA, Goodman-Meza D, Mu W, Hausner MA, et al. Primary, recall, and decay kinetics of SARS-CoV-2 vaccine antibody responses. ACS Nano (2021) 15(7):11180–91. doi: 10.1021/acsnano.1c03972
12. Johnson JM, Fernandes SC, Wuelfing DL, Baillargeon AR, MacLure EL, Hwang S, et al. Quantifying post-vaccination protective anti-SARS-CoV-2 IgG antibodies in blood and saliva with a fully automated, high throughput digital immunoassay. medRxiv (2022). doi: 10.1101/2022.01.21.22269165
13. Wheatley AK, Juno JA, Wang JJ, Selva KJ, Reynaldi A, Tan HX, et al. Evolution of immune responses to SARS-CoV-2 in mild-moderate COVID-19. Nat Commun (2021) 12(1):1–11. doi: 10.1038/s41467-021-21444-5
14. Peterhoff D, Glück V, Vogel M, Schuster P, Schütz A, Neubert P, et al. A highly specific and sensitive serological assay detects SARS-CoV-2 antibody levels in COVID-19 patients that correlate with neutralization. Infection (2021) 49(1):75–82. doi: 10.1007/s15010-020-01503-7
15. Varadhachary A, Chatterjee D, Garza J, Garr RP, Foley C, Letkeman AF, et al. Salivary anti-SARS-CoV-2 IgA as an accessible biomarker of mucosal immunity against COVID-19. medRxiv Prepr Serv Heal Sci (2020), 1–26. doi: 10.1101/2020.08.07.20170258
16. Ketas TJ, Chaturbhuj D, Cruz Portillo VM, Francomano E, Golden E, Chandrasekhar S, et al. Antibody responses to SARS-CoV-2 mrna vaccines are detectable in saliva. Pathog Immun (2021) 6(1):116–34. doi: 10.20411/pai.v6i1.441
17. Isho B, Abe KT, Zuo M, Jamal AJ, Rathod B, Wang JH, et al. Evidence for sustained mucosal and systemic antibody responses to SARS-CoV-2 antigens in COVID-19 patients. medRxiv (2020). doi: 10.1101/2020.08.01.20166553. 2020.08.01.20166553.
18. Heaney CD, Pisanic N, Randad PR, Kruczynski K, Howard T, Zhu X, et al. Comparative performance of multiplex salivary and commercially available serologic assays to detect SARS-CoV-2 IgG and neutralization titers. J Clin Virol (2021) 145:104997. doi: 10.1101/2021.01.28.21250717
19. Pisanic N, Randad PR, Kruczynski K, Manabe YC, Thomas DL, Pekosz A, et al. COVID-19 serology at population scale: SARS-CoV-2-specific antibody responses in saliva. J Clin Microbiol (2021) 59(1):1–13. doi: 10.1128/JCM.02204-20
20. Russell MW, Moldoveanu Z, Ogra PL, Mestecky J. Mucosal immunity in COVID-19: A neglected but critical aspect of SARS-CoV-2 infection. Front Immunol (2020) 11:611337. doi: 10.3389/fimmu.2020.611337
21. To KKW, Tsang OTY, Leung WS, Tam AR, Wu TC, Lung DC, et al. Temporal profiles of viral load in posterior oropharyngeal saliva samples and serum antibody responses during infection by SARS-CoV-2: an observational cohort study. Lancet Infect Dis (2020) 20(5):565–74. doi: 10.1016/S1473-3099(20)30196-1
22. Langlois EV, Straus SE, Antony J, King VJ, Tricco AC. Using rapid reviews to strengthen health policy and systems and progress towards universal health coverage. BMJ Glob Heal (2019) 4(1):1–4. doi: 10.1136/bmjgh-2018-001178
23. Tricco AC, Langlois EV, Straus SE. Rapid reviews to strengthen health policy and systems: a practical guide. Geneva: World Health Organization (2017). Licence: CC BY-NC-SA 3.0 IGO.
24. Page MJ, McKenzie JE, Bossuyt PM, Boutron I, Hoffmann TC, Mulrow CD, et al. The PRISMA 2020 statement: An updated guideline for reporting systematic reviews. BMJ (2021) 372:1-11. doi: 10.1136/bmj.n71
25. Azzi L, Dalla Gasperina D, Veronesi G, Shallak M, Ietto G, Iovino D, et al. Mucosal immune response in BNT162b2 COVID-19 vaccine recipients. eBioMedicine (2022) 75:1-10. doi: 10.1016/j.ebiom.2021.103788
26. Sheikh-Mohamed S, Isho B, Chao GYC, Zuo M, Cohen C, Lustig Y, et al. Systemic and mucosal IgA responses are variably induced in response to SARS-CoV-2 mRNA vaccination and are associated with protection against subsequent infection. Mucosal Immunol (2022) 15(5):799–808. doi: 10.1038/s41385-022-00511-0
27. Terreri S, Mortari EP, Vinci MR, Perno CF, Zaffina S, Carsetti R. Persistent b cell memory after SARS-CoV-2 vaccination is functional during breakthrough infections. Cell Host Microbe (2020) 3:400–8. doi: 10.1016/j.chom.2022.01.003
28. Darwich A, Pozzi C, Fornasa G, Lizier M, Azzolini E, Spadoni I, et al. BNT162b2 vaccine induces antibody release in saliva: a possible role for mucosal viral protection? EMBO Mol Med (2022) 14(5):1–9. doi: 10.15252/emmm.202115326
29. Lapić I, Šegulja D, Rogić D. Assessment of salivary antibody response to BNT162b2 mRNA COVID-19 vaccination. J Med Virol (2021) 93(9):5257–9. doi: 10.1002/jmv.27096
30. Pinilla YT, Heinzel C, Caminada LF, Consolaro D, Esen M, Kremsner PG, et al. SARS-CoV-2 antibodies are persisting in saliva for more than 15 months after infection and become strongly boosted after vaccination. Front Immunol (2021) 12:798859. doi: 10.3389/fimmu.2021.798859
31. Garziano M, Utyro O, Poliseno M, Santantonio TA, Saulle I, Strizzi S, et al. Natural SARS-CoV-2 infection affects neutralizing activity in saliva of vaccinees. Front Immunol (2022) 13:820250. doi: 10.3389/fimmu.2022.820250
32. Guerrieri M, Francavilla B, Fiorelli D, Nuccetelli M, Passali FM, Coppeta L, et al. Nasal and salivary mucosal humoral immune response elicited by mrna bnt162b2 covid-19 vaccine compared to sars-cov-2 natural infection. Vaccines (2021) 9(12):1–14. doi: 10.3390/vaccines9121499
33. Klingler J, Lambert GS, Itri V, Liu S, Bandres JC, Enyindah-Asonye G, et al. Detection of antibody responses against SARS-CoV-2 in plasma and saliva from vaccinated and infected individuals. Front Immunol (2021) 12:759688. doi: 10.3389/fimmu.2021.759688
34. Meyer-Arndt L, Schwarz T, Loyal L, Henze L, Kruse B, Dingeldey M, et al. Cutting edge: Serum but not mucosal antibody responses are associated with pre-existing SARS-CoV-2 spike cross-reactive CD4 + T cells following BNT162b2 vaccination in the elderly. J Immunol (2022) 208(5):1001–5. doi: 10.4049/jimmunol.2100990
35. Nickel O, Rockstroh A, Wolf J, Landgraf S, Kalbitz S, Kellner N, et al. Evaluation of the systemic and mucosal immune response induced by COVID-19 and the BNT162b2 mRNA vaccine for SARS-CoV-2. medRxiv (2022) 49(341):1–22. doi: 10.1101/2022.01.29.22270066. 2022.01.29.22270066.
36. Robinson JL, German GJ. Salivary antibodies are detected with a commercial anti-SARS-CoV-2 assay only after two doses of vaccine using serum thresholds. Clin Biochem (2022) 104:66–9. doi: 10.1016/j.clinbiochem.2022.02.002
37. Selva KJ, Davis SK, Haycroft ER, Lee WS, Lopez E, Reynaldi A, et al. Tear antibodies to SARS-CoV-2: Implications for transmission. Clin Transl Immunol (2021) 10(11):1–8. doi: 10.1002/cti2.1354
38. Jain S, Batra H, Yadav P, Chand S. Covid-19 vaccines currently under preclinical and clinical studies, and associated antiviral immune response. Vaccines (2020) 8(4):1–16. doi: 10.3390/vaccines8040649
39. Poland GA, Ovsyannikova IG, Kennedy RB. SARS-CoV-2 immunity: review and applications to phase 3 vaccine candidates. Lancet (2020) 396(10262):1595–606. doi: 10.1016/S0140-6736(20)32137-1
40. Castro Dopico X, Ols S, Loré K, Karlsson Hedestam GB. Immunity to SARS-CoV-2 induced by infection or vaccination. J Intern Med (2022) 291(1):32–50. doi: 10.1111/joim.13372
41. Carnell GW, Ciazynska KA, Wells DA, Xiong X, Aguinam ET, McLaughlin SH, et al. SARS-CoV-2 spike protein stabilized in the closed state induces potent neutralizing responses. J Virol (2021) 95(15):1–16. doi: 10.1128/JVI.00203-21
42. Lozano-Ojalvo D, Camara C, Lopez-Granados E, Nozal P, del Pino-Molina L, Bravo-Gallego LY, et al. Differential effects of the second SARS-CoV-2 mRNA vaccine dose on T cell immunity in naive and COVID-19 recovered individuals. Cell Rep (2021) 36(8):1-5. doi: 10.1016/j.celrep.2021.109570
43. Flanagan KL, Best E, Crawford NW, Giles M, Koirala A, Macartney K, et al. Progress and pitfalls in the quest for effective SARS-CoV-2 (COVID-19) vaccines. Front Immunol (2020) 11:579250. doi: 10.3389/fimmu.2020.579250
44. Ong DSY, Fragkou PC, Schweitzer VA, Chemaly RF, Moschopoulos CD, Skevaki C. How to interpret and use COVID-19 serology and immunology tests. Clin Microbiol Infect (2021) 27(7):981–6. doi: 10.1016/j.cmi.2021.05.001
45. Oba IT, Spina AMM, Saraceni CP, Lemos MF, Senhoras RDCFA, Moreira RC, et al. Detection of hepatitis a antibodies by ELISA using saliva as clinical samples. Rev Inst Med Trop Sao Paulo (2000) 42(4):197–200. doi: 10.1590/s0036-46652000000400004
46. Calheira MC, Trindade SC, Falcão MML, Barbosa LSC, Carvalho GRB, Machado PRL, et al. Immunoassay standardization for the detection of immunoglobulin a (IgA) against porphyromonas gingivalis antigens in saliva of individuals with and without leprosy. AMB Express (2021) 11(1):152 1–7. doi: 10.1186/s13568-021-01312-7
47. Bhat SS, Kalal BS, Veena KM, Kakunje A, Sahana KSR, Rekha PD, et al. Serum and salivary immunoglobulin G4 levels in children with autism spectrum disorder from south India: A case-control study. Am J Clin Exp Immunol (2021) 10(4):103–11.
48. Sterlin D, Malaussena A, Gorochov G. IgA dominates the early neutralizing antibody response to SARS-CoV-2 virus. Medecine/Sciences (2021) 37(11):968–70. doi: 10.1126/scitranslmed.abd2223
49. Brandtzaeg P. Secretory immunity with special reference to the oral cavity. J Oral Microbiol (2013) 5(2013):1–24. doi: 10.3402/jom.v5i0.20401
50. Healy K, Pin E, Chen P, Söderdahl G, Nowak P, Mielke S, et al. Salivary IgG to SARS-CoV-2 indicates seroconversion and correlates to serum neutralization in mRNA-vaccinated immunocompromised individuals. Med (2022) 3(2):137–153.e3. doi: 10.1016/j.medj.2022.01.001
51. Favresse J, Gillot C, Di Chiaro L, Eucher C, Elsen M, Van Eeckhoudt S, et al. Neutralizing antibodies in covid-19 patients and vaccine recipients after two doses of bnt162b2. Viruses (2021) 13(7):1–13. doi: 10.3390/v13071364
52. Vitiello A, Ferrara F, Troiano V, La Porta R. COVID-19 vaccines and decreased transmission of SARS-CoV-2. Inflammopharmacology (2021) 29(5):1357–60. doi: 10.1007/s10787-021-00847-2
53. van der Ley PA, Zariri A, van Riet E, Oosterhoff D, Kruiswijk CP. An intranasal OMV-based vaccine induces high mucosal and systemic protecting immunity against a SARS-CoV-2 infection. Front Immunol (2021) 12:781280. doi: 10.3389/fimmu.2021.781280
54. Moreno-Fierros L, García-Silva I, Rosales-Mendoza S. Development of SARS-CoV-2 vaccines: Should we focus on mucosal immunity? Expert Opin Biol Ther (2020) 20(8):831–6. doi: 10.1080/14712598.2020.1767062
55. Lavelle EC, Ward RW. Mucosal vaccines — fortifying the frontiers. Nat Rev Immunol (2022) 22(4):236–50. doi: 10.1038/s41577-021-00583-2
56. Alu A, Chen L, Lei H, Wei Y, Tian X, Wei X. Intranasal COVID-19 vaccines: From bench to bed. eBioMedicine (2022) 76:103841. doi: 10.1016/j.ebiom.2022.103841
Keywords: SARS-CoV-2, antibodies, saliva, IgG, IgA, COVID-19 vaccines
Citation: Guerra ENS, Castro VT, Amorim dos Santos J, Acevedo AC and Chardin H (2022) Saliva is suitable for SARS-CoV-2 antibodies detection after vaccination: A rapid systematic review. Front. Immunol. 13:1006040. doi: 10.3389/fimmu.2022.1006040
Received: 28 July 2022; Accepted: 31 August 2022;
Published: 20 September 2022.
Edited by:
George Kenneth Lewis, University of Maryland, United StatesReviewed by:
Lok Bahadur Shrestha, University of New South Wales, AustraliaRille Pullerits, Sahlgrenska University Hospital, Sweden
Copyright © 2022 Guerra, Castro, Amorim dos Santos, Acevedo and Chardin. This is an open-access article distributed under the terms of the Creative Commons Attribution License (CC BY). The use, distribution or reproduction in other forums is permitted, provided the original author(s) and the copyright owner(s) are credited and that the original publication in this journal is cited, in accordance with accepted academic practice. No use, distribution or reproduction is permitted which does not comply with these terms.
*Correspondence: Eliete Neves Silva Guerra, ZWxpZXRlbmV2ZXNAdW5iLmJy