- National Vaccine and Serum Institute (NVSI), China National Biotech Group (CNBG), Beijing, China
Diabetes is the most prevalent metabolic disease in the world today. In addition to elevated blood glucose, it also causes serious complications, which has a significant effect on the quality of life of patients. Diabetic trauma is one of complications as a result of the interaction of diabetic neuropathy, peripheral vascular disease, infection, trauma, and other factors. Diabetic trauma usually leads to poor healing of the trauma and even to severe foot ulcers, wound gangrene, and even amputation, causing serious psychological, physical, and financial burdens to diabetic patients. Non-coding RNAs (ncRNAs) carried by exosomes have been demonstrated to be relevant to the development and treatment of diabetes and its complications. Exosomes act as vehicle, which contain nucleic acids such as mRNA and microRNA (miRNA), and play a role in the intercellular communication and the exchange of substances between cells. Because exosomes are derived from cells, there are several advantages over synthetic nanoparticle including good biocompatibility and low immunogenicity. Exosomal ncRNAs could serve as markers for the clinical diagnosis of diabetes and could also be employed to accelerate diabetic wound healing via the regulation of the immune response and modulation of cell function. ncRNAs in exosomes can be employed to promote diabetic wound healing by regulating inflammation and accelerating re-vascularization, re-epithelialization, and extracellular matrix remodeling. Herein, exosomes in terms of ncRNA (miRNA, lncRNA, and circRNA) to accelerate diabetic wounds healing were summarized, and we discussed the challenge of the loading strategy of ncRNA into exosomes.
Introduction
Diabetes mellitus (DM) is a class of metabolic disease with defective insulin secretion or when the body cannot utilize the insulin effectively, which is caused by two factors including both genetic and environmental factors. In addition to increasing blood glucose, various complications induced by diabetes include diabetic cardiomyopathy, diabetic nephropathy, diabetic cerebrovascular complications, and diabetic trauma malunion, which create a large burden to patients. Among these complications, wound healing is impaired due to impaired angiogenesis, neuropathy, unusual inflammatory response, and dysfunction of fibroblasts (1). Thus, there is an urgent need to develop a novel treatment for wound healing by regulating this complex pathophysiology.
There are several stages of wound healing such as hemostasis, inflammation, proliferation, contraction, and remodeling (2). The normal wound healing cascade is dependent on the coordination and synchronization of growth factors, matrix metalloproteinases (MMPs), cytokines, inflammatory cells, keratinocytes, fibroblasts, and endothelial cells. However, the healing process in diabetic wounds usually does not strictly follow the normal wound healing process described above and can be stalled at inflammation stage and cannot proceed to the next stage. In the diabetic state, absolute or relative insulin deficiency leads to an increase in the amount glucose and the interruption of lipid metabolism. In addition, abnormalities in energy metabolism could lead to abnormalities in immune cells and signal transduction, which play significant roles in chronic long-term inflammation of the wound and consequently in healing (Figure 1). A series of mechanisms such as polyol pathway, hexosamine pathway, protein kinase C (PKC) pathway, and nitric oxidase synthase pathway that lead to neuropathy impede diabetic wound healing. As a result, elevated levels of oxidative stress in diabetic patients are a major factor in impaired diabetic wound healing (3) Furthermore, diabetic wounds also suffer from hypoxia, abnormal vascularization, neurological damage, reduced number of epidermal nerves (4), reduction in the secretion of growth factors, and imbalance between accumulation of extracellular matrix components and matrix metalloproteinase remodeling. In all, these factors contributed to the delay in wound healing in diabetic patients through keeping the wound inflamed for a long period (3).
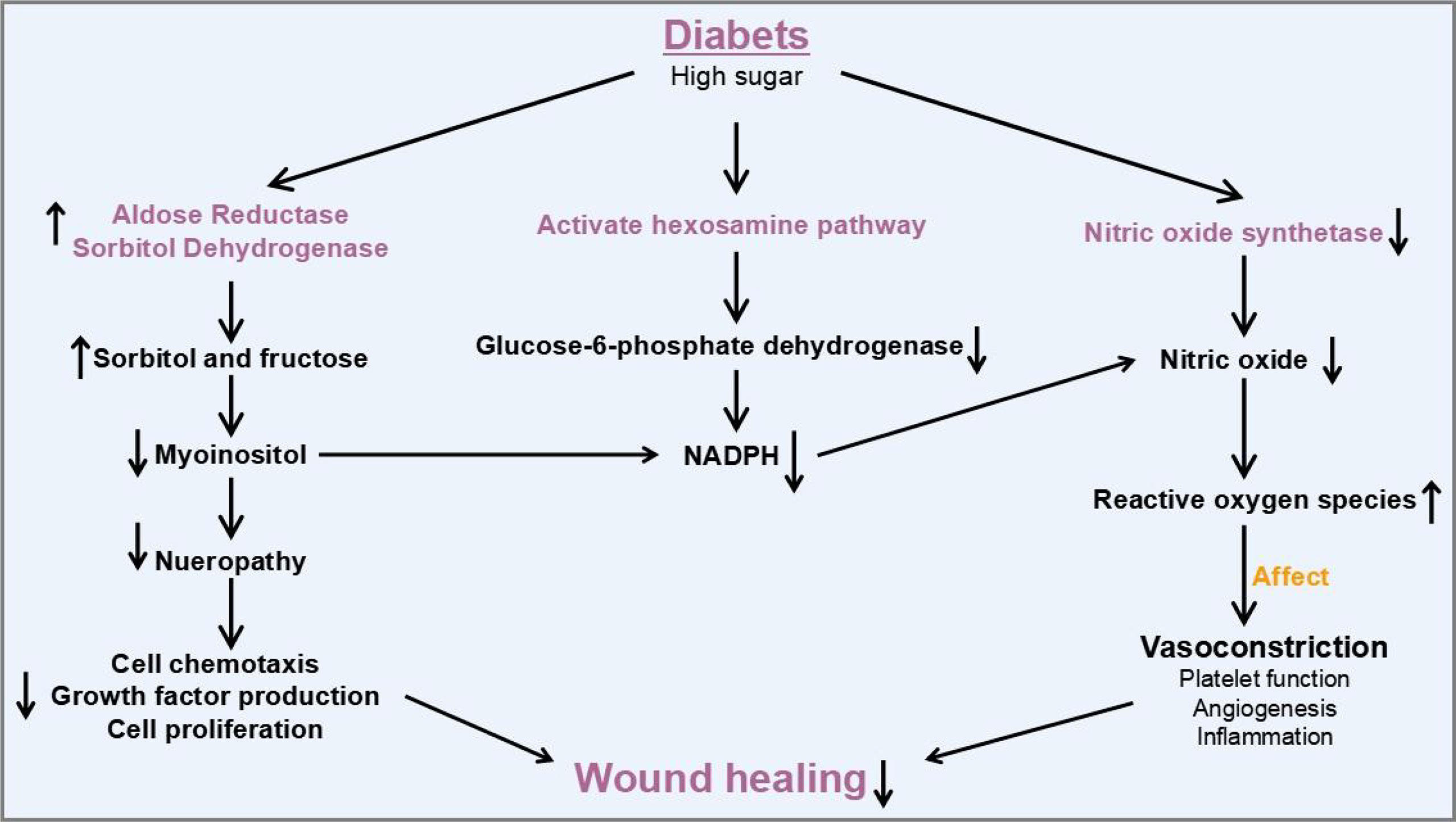
Figure 1 Molecular mechanisms and therapeutic potential of exosomal ncRNAs involved in diabetic wound healing.
Therefore, the promotion of wound healing is an effective strategy to significantly reduce amputations and deaths in diabetic patients. In recent years, surgery was considered as an efficient approach in the treatment of diabetic foot ulcer (DFU). Stem cells and related derivative biologics have been employed as alternative for diabetic wound healing. Nevertheless, there are many drawbacks in the use of stem cells such as the risk of rejection and carcinogenesis. Over the past decades, exosomes that are secreted by cells have received much attention as a cell-free alternative owing its possessing similar functions with cells without causing rejection and carcinogenesis (5).
Exosomes are extracellular vesicles with a diameter of about 30–150 nm, which serve as a vehicle to carry various substances, including various proteins, complexes, and non-coding RNAs. Exosomes play key roles in intercellular communication and the exchange of substances between cells. Exosomes contain a large amount of RNA species, including mRNA, miRNA, rRNA, lncRNA, tRNA, piRNA, snRNA, and snoRNA, which are involved in a variety of physiological and pathological processes (6, 7). Non-coding RNAs (ncRNAs) such as miRNA, lncRNA, and circRNA in exosomes are considered as active ingredients. ncRNAs are able to transcribe from the genome and perform their biological functions at the RNA level to regulate a variety of types of biological process. Particularly, ncRNAs delivered by exosomes may affect the occurrence and progression of various diseases via diverse mechanisms, such as diabetes. Some miRNA-containing exosomes regulated insulin sensitivity through targeting important protein key modulators and serving as pathological factors (8). There are strong associations between lncRNAs including ANARIL, MEG2, Sox2OT, MALAT1, and diabetes, especially type 2 diabetes (9). Additionally, circRNA and piRNA are known to promote the pathological process of diabetes (10). Abnormal expression of exosomal ncRNAs is observed in the wound of diabetic patients accompanied with elevated level of reactive oxygen species (ROS) and M1 macrophage polarization caused by high concentration of blood glucose. Therefore, these abnormal exosomal ncRNAs in exosomes could facilitate further the detection and treatment of diabetes. Garcia et al. have shown seven miRNAs with abnormal expression, which are isolated from plasma exosomes of diabetic patients. These miRNAs could be considered for the diagnosis of T1DM. On the contrary, some exosomal ncRNAs could be employed to treat diabetes and its complications by regulating several signaling pathways through regulating macrophage polarization toward M2 (11), accelerating fibroblast migration and proliferation, and promoting neovascularization (12) and angiogenesis (13). In addition, stem-cell-derived exosomes are well known to regulate inflammation and promote re-vascularization, re-epithelialization, and extracellular matrix remodeling in the repair of diabetic wounds via ncRNA. Besides miRNAs, lncRNAs and circRNAs, tRFs, which are tRNA-derived fragments, could regulate inflammatory response via the nuclear factor kappa B (NF-κB) signaling pathway. Liu and his colleagues have demonstrated that the nuclear translocation of p65 could be suppressed by decreasing the level of tRF5-AlaCGC, inhibiting NF-κB activity to reduce inflammation (14). More types of ncRNAs to accelerate the healing process will be discovered in the near future. In this review, ncRNAs in exosomes in terms of diabetic wound healing via anti-inflammatory, angiogenic, cell proliferation promoting, and matrix remodeling were reviewed (Scheme 1).
Exosomes
Exosomes are first discovered in 1983 in the supernatant of sheep erythrocytes cultured in vitro as vesicles within the lumen of the cell. Exosomes have a density of 1.10–1.18 g/ml; are flat, spherical, or cup shaped under electron microscopy; and are mainly spherical in structure in body fluids (15). Exosomes are the end-products of the endocytosis process, in which cells were cytosol zed to form endosomal vesicles (ILVs) with exogenous antibodies, which formed early endosomes (EEs) by the action of organelles such as the Golgi apparatus. The vesicle membrane of EEs is constantly invaginated and selectively receives intracellular LEs fused with the cell membrane and releases exosomes into the extracellular environment (16, 17). In addition, exosomes demonstrate property to fuse with the cytosolic membrane of neighboring cells and then release the nucleic acid molecules carried by exosomes, such as DNA, mRNAs, miRNAs, and lncRNAs. In the meanwhile, exosomes bind to receptors to involve signal transduction and alter cellular function. They can also regulate cell status directly by transporting proteins and lipids, causing a series of biological effects in the target cells. In diabetic wounds, the expression levels of inflammation-related genes including p53, HIF-1α, TNF-α, IL-6, IL-10, NF-κB, and STAT1 are abnormal, leading to the slowing of wound healing (18, 19).
Exosomal miRNA for diabetic wound healing
miRNAs are a class of small ncRNAs consisting of about 22 nucleotides that are widely found in plants, animals, and some viruses. They are involved in regulating physiological and pathological processes in the body by binding to the untranslated regions (UTRs) of target mRNAs and playing a role in transcriptional or post-transcriptional regulation (20); miRNAs can be encapsulated in exosomes, which carry and release them into target cells or tissues (21). In diabetic wounds, they are involved in regulating multiple fields in inflammatory response, angiogenesis and homeostasis, ECM generation, and re-epithelialization. Exosomal miRNAs are emerging key regulators of diabetes development and progression and have been demonstrated to play a vital role in regulating the progression of diabetic wound healing.
Exosomal miRNAs regulated the inflammatory response
In the process of diabetic wound healing, diabetic wound may stall at inflammation phase; the microenvironment for healing is thus disrupted, making wound healing poor. Monocytes and macrophages are widely considered to be responsible for the early inflammatory response to wounds, but they also facilitate in angiogenesis, wound contraction, and tissue remodeling. Macrophages are divided into two main subpopulations according to activities. M1 types promote inflammation and inhibit cell proliferation, while M2 types inhibit inflammation and promote cell proliferation. The transition from M1 to M2 type of wound macrophages to regulate the inflammatory response is the key factor in promoting wound healing. However, in diabetic wounds, there is an excessive impairment from M1 to M2 types, which resulted in these wounds not going through a normal healing state but always in a permanent inflammatory state (22). Mesenchymal stem cells (MSCs) are employed to treat various inflammatory responses in the wound healing process via the transformation of macrophage from M1 to M2. Currently, mesenchymal stem cell therapy (MSCT), a cell therapy, is considered as an attractively therapeutic method to enhance skin wound healing. During cutaneous wound healing, most of the therapeutic benefits of MSCT appear to because of paracrine signaling pathways through the stimulation of not only differentiation but also angiogenesis (23–25). However, the mechanisms by which bone marrow MSCs regulate inflammation remained unclear until 2019. He et al. found that inhibiting exosomes secretion that derive from MSCs resulted in the reduction in M2 macrophages in both in vitro co-culture systems and in vivo interaction sites. To sum up, MSCT is capable of inducing macrophage polarization to the M2 type by secreting exosomes. Further studies revealed that miRNA-223 in MSCT-EXO could modulate the polarization of macrophages to M2 by targeting the PHNOX1 gene, thereby regulating the inflammatory response in the wound and achieving a wound healing effect (11). Osteoarthritis (OA) pathology could be controlled by overexpression of miRNA because miRNA-210 can reduce the expression of proinflammatory cytokines (26). High-throughput sequencing results have revealed that the expression level of miRNA-210 is low in bone marrow mesenchymal stem-cell-derived exosomes (BMSCs-Exos). Hence, BMSCs-210-Exos-derived exosomes with overexpressing miRNA-210 could effectively inhibit chondrocyte apoptosis (27). In addition, MSCs can also be preconditioned with lipopolysaccharide (LPS), and treated MSCs show enhanced paracrine effects including increased nutritional support and improved reproductive and reparative properties. Ti et al. discovered that the exosomes secreted by MSCs that were pretreated with LPS have 40 significantly abnormal expressions of miRNAs, as compared to those without treatment (28). During these miRNAs, miR-let-7b have the highest expression levels of five unique miRNAs in hLPSpre-Exo. miR-let-7b in LPSpre-Exo could convert the polarization of macrophage via TLR4/NF-κB/STAT3/AKT signaling pathways. As a result, the inflammatory response was significantly attenuated, resulting in the promotion of diabetic wound healing (Figure 2A). Thus, miRNAs in exosomes could inhibit wound inflammation by modulating macrophage status, thereafter promoting wound healing.
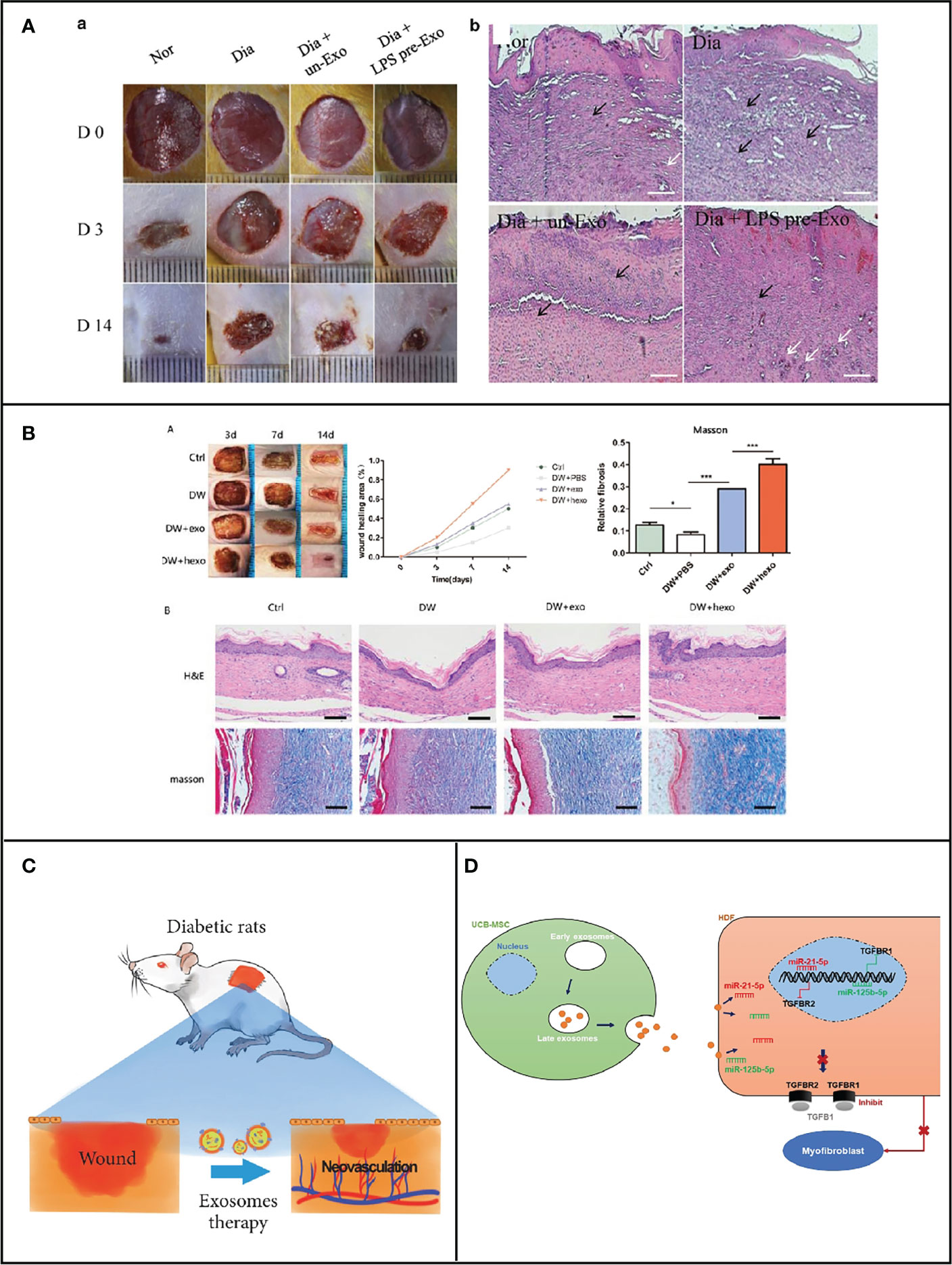
Figure 2 (A) miRNA carried by MSC-generated exosomes regulates macrophage polarization for the healing of cutaneous wounds in diabetic rats [Adapted from Ti et al., doi: 10.1186/s12967-015-0642-6, (28)]. (B) miRNA carried by ADSCs exosomes for diabetic wound healing [Adapted from Wang et al., doi: 10.1186/s12951-021-00942-0, (29)]. (C) Exosomes derived from marrow mesenchymal stem cells to accelerate cutaneous wound healing by promoting angiogenesis [Adapted from Ding et al., doi: 10.1155/2019/9742765, (30)]. (D) UCB-MSC-exo improves regenerative wound healing and suppresses scar formation by inhibiting the expression of TGF-β receptors [Adapted from Fang et al., doi: 10.5966/sctm.2015-0367, (31)].
Exosomal miRNAs for fibroblast/keratinocytes proliferation
In chronic non-healing wounds, an abnormal phenotype including reduced proliferation, early senescence, and altered cytokine release patterns is observed in fibroblasts and keratinocytes (32). Fibroblasts have been found to promote granulation tissue formation by secreting extracellular matrix, and microfilaments and microtubules within fibroblasts can form a skeleton that acts as a fibrous scaffolding structure for wound repair (33). A loose connective tissue contains a large number of fibroblasts that produce elastic, collagenous, and reticular fibers, which play an essential role in wound healing. Therefore, the proliferation and migration of fibroblasts through exosomal miRNAs are effective tactics to accelerate wound healing. The proliferation of cells is enhanced after transplantation of stem cells. The generated stem cell exosomes show a similar function with stem cells to improve cell proliferation.
Fibroblast proliferation and migration could be improved when subjected to hypoxic adipose stem-cell-derived exosomes. Under hypoxic condition, the activity and proliferation of adipose stem cells are significantly enhanced compared to normoxia, which was reported by Wang and his colleagues (29). The expression of 215 microRNAs (miRNAs) was increased, in which 369 miRNAs were decreased in hypoxia adipose stem cell exosomes (HypADSCs-exo) in comparison with adipose stem cell exosomes (ADSCs-exo) on the basis of high-throughput sequencing. An increase in miR-21-3p, miR-126-5p, and miR-31-5p and a decrease in miR-99b and miR-146-a were observed. Furthermore, the inflammation was inhibited after treatment with the above-mentioned exosomes via PI3K/AKT signaling pathway. Meanwhile, cell metabolism, differentiation, and TGF-β function were also regulated. As can be seen in Figure 2B, the process of diabetic wound healing was accelerated when subjected to HypADSCs-exo. Therefore, stem cell exosomes are an alternative approach in the promotion of diabetic wound healing. Besides ADSCs-exo, human amniotic mesenchymal stem cell (hAMSC)-derived exosomes could strengthen the migrating ability of fibroblast. Importantly, Chen et al. have demonstrated that the ability to improve wound healing in exosomes secreted by hAMSC was higher than that of unmodified hAMSC, while the above-mentioned ability of hAMSC-Exo was reduced after knockdown of miR-135a, suggesting that miR-135a in hAMSC-Exo can promote fibroblast proliferation and migration (34), which further indicated that exosomal miRNA is capable of helping wound shut. Moreover, miRNA also could be loaded into exosomes to corresponding function. Chen et al. indicated that these stem cell exosomes could promote the closeup of rat dorsal wounds. First, miRNA-146a was loaded into adipose stem cell exosomes. Subsequently, wound healing was improved because those exosomes could enhance the migration and proliferation of fibroblasts and accelerate neovascularization through the regulation of the level of SERPINH1 and p-ERK (35). Exosome cargo miRNA-146a was demonstrated to inhibit the leukocyte adhesion molecules expression and the level of proinflammatory cytokines, causing suppressive effect toward the inflammation of endothelial cells, which was activated by IL-1β. miRNA in exosomes could play multiple roles including the promotion of cell proliferation, migration, tube formation, and angiogenesis and the suppression of inflammatory behavior to the contribution of diabetic wound healing (12).
Exosomal miRNAs for vascular growth
Chronic wounds caused by diabetes often have microangiopathy, accompanied with alterations such as decreasing production of pro-angiogenic factors and lessened endothelial progenitor cells, ultimately leading to abnormal function of the vascular network (36). Revascularization and angiogenesis are important manifestations of wound healing. miRNA-221-3p could improve the function of cells through regulating HIF-1α. Macrophages are polarized into proinflammatory by inhibiting JAK3 signal pathway (37). Xu et al. have reported the high expression of miRNA-221-3p in exosomes generated from endothelial progenitor cell (EPCs). Exosomal miRNA-221-3p can downregulate the expression of p27 and p57 proteins, which are involved in the cell cycle signaling pathway. According to immunohistochemistry results, the angiogenesis-related proteins VEGF and CD31 and the cell proliferation marker Ki67 had higher expression levels after treatment with EPC-derived exosomes with high miRNA-221-3p expression. Moreover, it has been revealed that miRNA-221-3p may be engaged in the cell cycle, AGE-RAGE, and p53 signaling pathway in diabetic complications. Therefore, miRNA-221-3p may encourage the proliferation of vascular cells by blocking cell cycle negative regulators (38). Additionally, miRNA-221-3p in stem cell exosomes activated Akt/endothelial nitric oxide synthase pathway to promote angiogenesis. Subsequently, the proliferation and migration of endothelial cell was enhanced, resulting in the improvement of diabetic wound healing (39). Other signaling pathway such as HIPK2 was targeted by exosomal miRNA-221-3p to improve the function of HUVEC and accelerate angiogenesis, contributing to concrescence of the wound in diabetic rats (40). On the other hand, Ding et al. found a significant increase in miR-126 levels upon treatment with deferoxamine exosomes (DFO-Exos) from BMSCs pretreated with deferoxamine (Figure 2C). It was also observed that when DFO-Exos was given, the amount of the phosphatase and tensin homolog (PTEN) in endothelial cells, one target of miRNA, was decreased. PTEN was a proangiogenic pathway in endothelial cells via the PI3K/AKT signaling pathway. When miR-126 was inhibited, the level of PTEN was increased. Thus, miR-126 can promote angiogenesis by decreasing the level of PTEN (30). Similarly, Xiong et al. observed a distinct increase in miR-20b-5p in exosomes, which was obtained from plasma of patients with T2DM. Angiogenesis in human umbilical vein endothelial cell was inhibited by miR-20b-5p through Wnt9b/β signaling pathway. Either miR-20b-5p or diabetic exosomes, which was exposed to the wound site, was sufficient to slow down both wound healing and angiogenesis. Therefore, diabetic wound healing could be improved after treatment with mir-20b-5p inhibitors including ncRNA (41). Engineered exosomes with miRNA-21-5p from human adipocytes accelerate wound healing in a model of full-thickness skin defects in diabetic rats; the results of diabetic rats and keratinocytes indicated that miRNA-21-5p functions through the Wnt/b-catenin pathway and promotes angiogenesis and collagen remodeling in the wound area (42). miRNA-21-5p in exosomes derived from human mesenchymal stem cells promotes the proliferation of HUVECs and enhances angiogenesis by activating Akt and mitogen-activated protein kinase (MAPK) and upregulating VEGFR1. Meanwhile, miRNA-21-5p accelerates the wound healing of foot ulcers in diabetic rats (43). In addition, Yan et al. have utilized low-cost milk exosomes, which served as vehicle to load miR-31-5p by electroporation. the exosomal miR-31-5p dramatically improved endothelial cell function, leading to enhanced diabetic wound healing by downregulating the expression of HIF1AN process (13).
Exosomal miRNAs for matrix remodeling
The body avoided pathogen infection and rehydrated in the presence of epidermal cells. The extracellular matrix is mainly composed of collagen, fibronectin, elastin, laminin, etc. It provides an appropriate microenvironment for cells, thus inducing cell adhesion, migration, and differentiation (44). During wound repair process, the extracellular matrix is an essential constituent. The related components secreted by keratinocytes are indispensable parts for the skin to function normally (45). In the early stages of healing, collagen deposition is more significant. Fibroblast could produce large quantities of collagen and other matrix components to construct the microenvironment for epidermal cell coverage (46). However, in the later period of healing, matrix remodeling is more important. The remodeling of the extracellular matrix usually takes 2 weeks or even 1 year, and the production and remodeling of the extracellular matrix are critical in the extent of scar formation. Because of similar functions with stem cells, stem cell-derived exosomes could be employed to regulate the extracellular matrix and promote collagen production, thereby reducing scarring. As demonstrated in Figure 2D, Fang et al. indicated that umbilical-cord-derived bone marrow mesenchymal stem cells (uMSCs) reduced scar formation and myofibroblast accumulation in a mouse model of skin defects. By utilizing miRNA omics, the uMSC-Exos group, enriched in specific miRNAs (miR-21, miR-23a, miR-125b, and miR-145), played a key role in inhibiting myofibroblast formation through suppressing the TGF-β/SMAD2 pathway (31). Exosomes secreted by human amniotic fluid stem cells also prevent myofibroblast differentiation. Zhang et al. assessed the impact of hAFSC-derived exosomes (hAFSC-exo) on anti-fibrotic scarring during wound healing by employing a rat model of complete skin damage. Through exosome-specific miRNAs, hAFSCs aid in the healing of wounds and inhibit the development of fibrotic scars. Additionally, hAFSC-exo-specific miRNAs enhance scar-free wound healing by the downregulation of TGF-R1 and miR-21-5p, miR-22-3p, and miR-27a-3p and the inhibition of myofibroblast production (47).
Exosomal lncRNAs regulated the inflammatory response
For diabetes, lncRNA plays significant roles in regulating wound inflammation via several pathways. ROS overproduction triggers neutrophil inflammatory infiltration, increased protease release, and an abundance of oxidative intermediate products, which ultimately result in cell death, affecting the healing up of wound in diabetic patients (48). The activity of transcription factors is directly regulated by low amounts of ROS as a signal molecule, which reduces apoptosis. Cells subjected to H2O2 showed a strong capacity to promote tube formation, migration, and proliferation of EPCs in a dose-dependent manner. In diabetic wounds, the expression level of inflammation-related genes including p53 was abnormal, leading to slow wound healing. PI3K/AKT was reported to act as a protective role against apoptosis caused by high glucose. PTENs have been demonstrated as a target gene of miRNA-152-3p, and lncRNA-H19 was a transcript antisense RNA affecting the level of miRNA-152-3p. PTEN serves as antagonist of PI3K. In 2019, Li and his colleagues found a high expression of miRNA-152-3p and low expression of lncRNA-H19 in the fibroblast with diabetic foot ulcer. After treatment with lncRNA-H19-overexpressed MSC-derived exosomes, miRNA-152-3p decreased in fibroblast accompanied by not only apoptosis but also inflammation (Figure 3A). In addition, proliferation and migration in fibroblast were promoted. As a consequence, the process of wound healing was accelerated upon injection with overexpressed lncRNA-H19 exosomes (52). Upregulation of lncRNA-H19 can also elevate the expression of FBN1 through competitively binding to miR-29b, which enhances the proliferation, migration, and inhibits apoptosis of fibroblasts, thus facilitating the wound healing of DFU (49). Exosomes that are secreted by the above-mentioned cells also have similar functions to cells. Furthermore, lncRNA NEAT1 in exosomes shows overexpression stimulated by oxidative stress on EPC cells, which is the effective therapeutic ingredient of exosomes (Figure 3C) (51). Evidence suggested that ROS concentration in macrophages could be modulated by lncRNA Lethe and lncRNA NTF3-5. The biochemical mechanism is that lncRNA NTF3-5 suppresses the level of NOX2 gene by activating NF-κB signaling on account of negative feedback loop between lncRNA Lethe and NF-κB (53). Similarly, increasing the expression of lncRNA MALAT1 is an alternative strategy to promote wound healing in diabetic patients (9). These lncRNAs, which could be cargoes by exosomes via various methods, would be employed as therapeutic drugs for the acceleration of the process of wound healing.
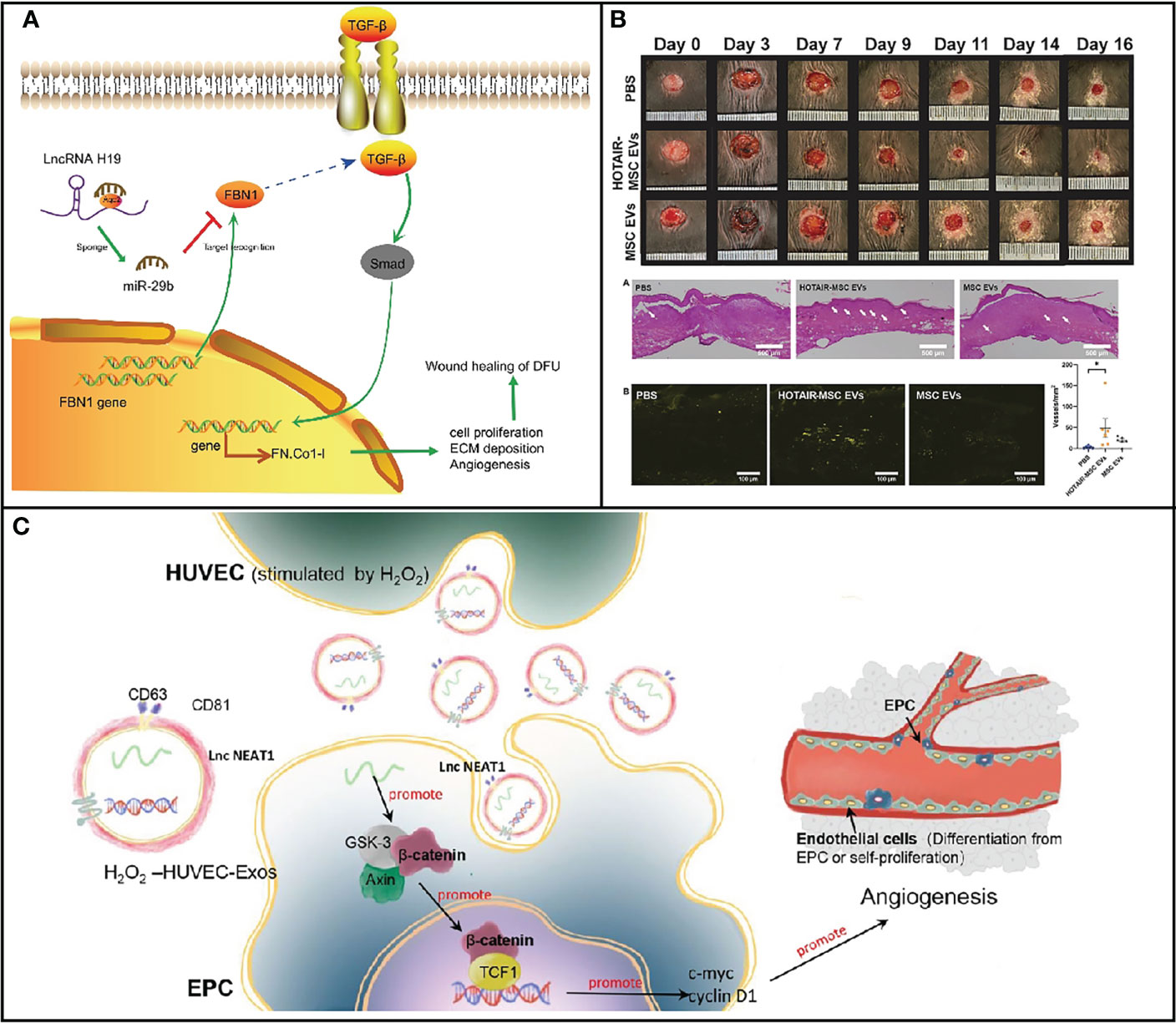
Figure 3 (A) Map of molecular mechanisms involved in lncRNA H19 modulation in wound healing of DFU by binding to miR-29b to regulate FBN1 expression [Adapted from Li et al., doi: 10.1096/fj.201900076RRRRR, (49)]. (B) HOTAIR-MSC EVs improve wound healing in db/db mice via increasing angiogenesis [Adapted from Born et al., doi: 10.1002/adhm.202002070, (50)]. (C) Schematic illustration of the function and mechanisms of HUVEC-Exos on EPCs [Adapted from Guo et al., doi: 10.1186/s13287-022-03013-9, (51)]. *p < 0.05.
Exosomal lncRNA for diabetic wound healing
The lncRNA is a loosely classified group of long RNA transcripts with no apparent protein-coding role with a length >200 nucleotides. LncRNAs play a role in several significant biological processes, including the imprinting of genomic loci, sculpting of chromosome shape, and allosteric control of enzyme function. LncRNA performs the functions through regulating target genes with different mechanisms such as scaffolds, decoys, signals, and guide. Thus, lncRNAs are associated with cell differentiation, status, development, and illness. Among these lncRNAs, X-inactive-specific transcript (XIST; in X chromosome inactivation), HOX transcript antisense RNA (HOTAIR; in positional identity), and telomerase RNA component (TERC; in telomere elongation) were well-explored lncRNAs in functional roles and mechanisms.
Exosomal lncRNAs for fibroblast/keratinocytes proliferation
He and coworkers demonstrated that the content of lncRNA CASC2 in the tissue was reduced in diabetic foot ulcers. The wound healed speedily when the lncRNA CASC2 was overexpressed. LncRNA CASC2 abolished fibroblasts apoptosis and promoted cell proliferation and migration. Mechanically, lncRNA CASC2 interacted with miRNA 155 to upregulate the level of its target gene HIF-1α. Thus, overexpression of lncRNA CASC2 is a novel approach for diabetic wound healing. Increasing HIF-1α level by lncRNAs is considered as an effective strategy to improve healing process. LncRNA-H19 and lncRNA MIR31HG have been employed to regulate the expression of HIF-1α to enhance the proliferation of fibroblast (54). In addition, lncRNA FAM83A-AS1 involved several signaling pathways such as glycolysis, hypoxia, and OXPHOS signaling. Chen et al. proved that lncRNA FAM83A-AS1 could enhance cell migration, invasion, and proliferation (55). LncRNA FAM83A-AS1 may be applied to strengthen the proliferation of fibroblast/keratinocytes for the treatment of diabetic wound. Although there is no literature on exosomal lncRNAs for cell proliferation in wound healing, lncRNAs delivered by exosomes would be explored in the near future.
Exosomal lncRNAs for vascular growth
LncRNA in exosomes is capable of promoting angiogenesis to accelerate wound healing. HOTAIR has been shown to promote angiogenesis in several setting. Jay’s group has constructed exosomes with increased HOTAIR content to enhance angiogenesis for the promotion of healing of chronic wounds. On account of its intrinsic angiogenic properties, exosomes that were isolated from MSC with overexpressed HOTAIR demonstrated high level of HOTAIR compared with MSC without treatment. After treatment with HOTAIT-MSC exosomes, HOTAIR content was as much as 21-fold in human umbilical vein endothelial cells (HUVECs), resulting in about 11-fold increase in VEGFA within HUVECs. Moreover, gap closure was distinctly improved when exposed to HOTAIR-MSC exosomes (Figure 3B). As a consequence, there was an increase in the number of vessels in healed tissue when subjected to HOTAIR-MSC exosomes, demonstrating that lncRNA delivered by exosomes could be employed as nanomedicine for wound healing. Therefore, stimulation of angiogenesis and VEGFA are general therapeutic strategies to promote wound healing through lncRNA carried by exosomes (50). Similarly, Han et al. constructed MSC-derived exosomes to deliver lncRNA KLF3-AS1 to accelerate wound healing by the upregulation of VEGFA. KLF3-AS1 acted as a competing endogenous RNA for MiR-383 to increase the VEGFA signaling for diabetic wound healing. Under diabetic condition, exosomal KLF3-AS1 significantly promoted the proliferation of HUVESs. The content of proapoptotic proteins markedly upregulated, while anti-apoptotic proteins reduced after treatment with lncRNA delivered by exosomes due to the increasing VEGFA expression level. Interestingly, inflammation was also suppressed in the KLF3-AS1-Exos group, which further improved curative effects. Thus, improving angiogenesis and suppressing inflammation are effective strategies to treat diabetic wound (56).
Exosomal lncRNAs for matrix remodeling
A research by Herter at al. demonstrated that the expression of WAKMAR2 (lncRNA) was decreased in human chronic wounds as compared to normal wounds (57). WAKMAR2 is an RNA polymerase, which is observed in the cytoplasm and in the nucleus of keratinocytes. In order to investigate the molecular mechanisms modulation, the level of WAKMAR2, a variety of biomolecules, was used to treat keratinocytes in the wound, indicating that WAKMAR2 was regulated by TGF-β signaling pathways. Furthermore, the migration of keratinocytes was enhanced, and inflammation was suppressed in the presence of WAKMAR. As a consequence, diabetic wound repair could be improved when subjected to WAKMAR2, especially WAKMAR2 delivered by exosomes. Proteins in ECM could be degraded and modified by enzymes such as MMPs, serine proteinases, and disintegrant (58). Ye’s group indicated that cancer cell proliferation and migration were accelerated by lncRNA P73 antisense RNA 1T (lncRNA TP73-AS1) through regulating the expression of MMP2 and MMP9. By utilizing lncRNA TP73-AS1 carried by exosomes, the healing process may be accelerated via downregulating the proteins level of MMP2 and MMP9 to decrease degradation of ECM proteins.
Exosomal circRNA for diabetic wound healing
CircRNAs are a class of coding/ncRNA molecules that form a closed loop of exons and/or introns by covalently binding at the 3′and 5′ends (59, 60). Compared to linear mRNAs, circRNAs contain more abundant transcripts and are capable of regulating gene expression at the transcriptional and post-transcriptional levels. circRNAs are components of competing ceRNAs that could regulate gene expression by inhibiting miRNA activity to perform functions in physiological processes such as cell cycle or aging (61–63). They have tissue cell selectivity, structural stability, and sequence conservation and are widely expressed in mammalian cells.
Exosomal lncRNAs regulated the inflammatory response
Circ-Snhg11 from hypoxia-pretreated ADSC exosome promoted diabetic wound healing by increasing vascular differentiation function of EPCs by activation of the miR-144–3p/HIF-1α/VEGF signaling pathway. The result also found that circ-Snhg11 can also promote M2-like macrophage polarization under HG conditions by the regulation of miR-144–3p/HIF-1α/STAT3 signaling pathway (64). A research by Wang at al. discovered that the decrease in circRNA 001372 could upregulate IL-1, IL-6, IL-17, and IL-18, resulting in damage and apoptosis of neurocyte (65), while the inflammatory response was reduced when circRNA 001372 was overexpressed. Furthermore, they revealed that circRNA 001372 targeted miRNA-148b-3p to suppresses inflammation via the PI3K/Akt/NF-κB signaling pathway.
Exosomal circRNAs for fibroblast/keratinocytes proliferation
Si et al. have found that circRNA_100797 expression was decreased in human fibroblast after exposure to ultraviolet B. miR-23a-5p was the target of circRNA_100797, as analyzed by Gene Ontology and quantitative real-time PCR (qRT-PCR). The proliferation of fibroblast was enhanced when circRNA_100797 was highly expressed, and the cell cycle arrest was alleviated (66). In addition, the level of circRNA_000203 in cardiac fibroblast in diabetic mouse was increased accompanied by the increase in Col1a2, Col3a1, and α-SMA (67). circRNA_000203 could especially bind to miR-26b-5p for the suppression of the interaction between miR-26b-5p and collagen. Although circRNA_000203 exerted negative function in cardiac fibroblast, it could promote the healing of wound on account of promoting the expression of collagen. Therefore, circRNA serves as a sponge of miRNA to improve fibroblast/keratinocytes proliferation for the promotion of diabetic wound healing, especially delivered by exosomes by taking advantage of its perfect biocompatibility.
Exosomal circRNAs for vascular growth
It has been noted that hyperglycemia (HG) levels can affect the amounts of circRNA expression in HUVECs. Three of these circRNAs (hsa circ 0008360, hsa circ 0000109, and hsa circ 0002317) showed the largest upregulation, according to the miRNA genomics, which identified 214 circRNAs with differential expression. Based on bioinformatics, these circRNAs control the expression of genes involved in angiogenesis and vascular endothelial function (68). Chen et al. compared the differences in exosomal circRNAs isolated from serum between diabetic and non-diabetic patients. There were 67 circRNA differences in the level of expression between the two groups, and in the DFU group, 28 circular RNAs were upregulated and 39 were downregulated (69), circRNA not only could be used as serum biomarker for the clinical diagnosis of DFU, but it also plays a role in diabetic wound healing. Angiogenesis is an important cause of diabetic wound healing. One potential cause of diabetic ulcers is the damage to vascular endothelial cells, and EPC transplantation is considered as a promising approach for the treatment of hypovascular chronic wounds (70). In their study, Shang et al. revealed that transplantation of hypoxia-pretreated pre-endothelial progenitor cells (EPCs), which were under hypoxic condition before use, was more therapeutically effective than EPCs without treatment in accelerating diabetic wound healing. The overexpression of circ-Klhl8 inhibited hyperglycemia, which caused the injury to endothelial cells via activating autophagy. miR-212-3p was verified as the sole target of circKlhl8 by employing bioinformatics. miR-212-3p in diabetes causes pancreatic β-cell dysfunction and inhibits autophagy (71). In contrast, a certain level of autophagy promotes EPC migration and angiogenesis, and EPCs overexpressing circ-Klhl8 can promote wound repair by sponging miR-212-3p to reduce its expression and activate autophagy (Figure 4A). circ-Klhl8 can also regulate autophagy to accelerate wound healing by promoting SIRT5 overexpression. Additionally, it has been demonstrated that ADSC-exo carrying mmu circ 0000250 stimulates the production of miR-128-downstream 3p’s gene SIRT1 by binding to it and then activates cellular autophagy. Exosomes with high concentrations of mmu circ 0000250 sped up the healing of diabetic wounds (Figure 4B). It has been demonstrated that exosomes could increase angiogenesis and autophagy in Exosomes derived from human umbilical cord blood mesenchymal stem cells stimulate skin wounds to accelerate its healing (72).
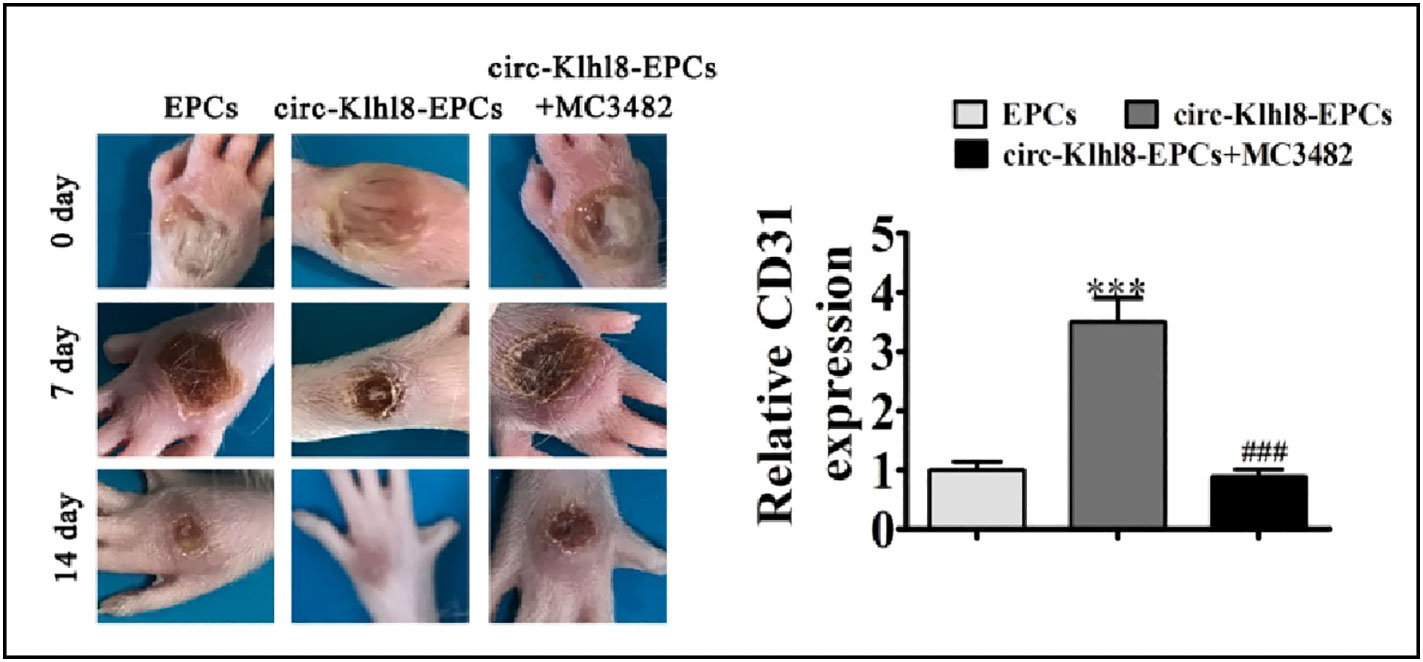
Figure 4 Hypoxic pretreatment endothelial progenitor cell (EPCs) accelerates diabetic wound closure and promotes angiogenic [Adapted from Shang et al., doi: 10.1016/j.jdiacomp.2021.108020, (71)]. ***p < 0.001 vs EPCs. ###P < 0.001 vs circ-Klhl8-EPCs.
Challenge and perspective
At present, most of the research on ncRNA in diabetic wound repair is based on the role of naked nucleic acid. However, due to various shortcomings such as poor stability and deficiency of poor targeting, ncRNA have limited efficacy in clinical application and cannot be used for further applications. Conversely, exosomes containing nucleic acid can remain in the body for a longer time and have a stronger effect than naked nucleic acid drugs. However, the crucial problem is that rare sources of these natural exosomes cannot meet clinical needs. In addition, the content of ncRNA in exosomes does not suffice for clinical application. Therefore, ncRNA in engineered exosomes serves as cargo, which is loaded into exosomes. By taking advantage of biological, physical, chemical, and other methods, ncRNA could be packaged into exosomes with the same pharmacokinetic behavior with exosomes. However, the low envelopment rate is achieved by utilizing these passive loading methods. Active loading method will improve the envelopment rate for exosomes to load ncRNA. In addition, most of above approaches are inapplicable for large-scale production due to low yield and high cost. To address the above problems, biomimetic exosomes that possess similar structures with exosomes and liposomes can also be used as a substitute for natural exosomes to delivery ncRNA. Generally, biomimetic exosomes could be prepared via top–down and down–top strategy by employing lipids, proteins, and RNA or cells. Biomimetic exosome drugs have the characteristics of clear source, stable physicochemical properties, low cost, and good biocompatibility as compared to liposomes. Furthermore, compared with natural exosomes, biomimetic exosomes are easier to be synthesized on a large scale in comparison with natural exosomes isolated from cells. Nevertheless, clinical trials of engineered exosomes have just begun, and the synthetically biomimetic exosome have not yet been approved for further clinical application on account of lack of standard approaches for production, characterization, and quality control. In addition, some of the ncRNAs could involve several signaling pathway with multiple targets, which is not beneficial as a drug. In the future, ncRNA carried by novel multifunctional engineered exosomes and biomimetic exosomes will be generated by biotechnology, providing a new avenue for diabetic wound therapy.
Conclusion
Due to the significant impact on the quality of life of complications induced by the diabetes especially diabetic trauma, diabetic wound healing is urgently needed to improve patients’ life quality. Diabetic wound healing is affected by inflammation, cell proliferation, angiogenesis, and ECM remodeling. ncRNA could regulate these conditions to promote diabetic wound healing (Table 1). In comparison with synthetic vehicles, exosomes as a vehicle are employed to deliver ncRNA owing to high biocompatibility and lower toxicity. The level of specific ncRNA in natural exosomes does not fulfill clinical application such as diabetic wound healing. To date, a few exosomes for diabetic wound healing is undergoing clincal trials (Table 2). Therefore, engineered exosomes are constructed by various strategies including biological, physical, chemical, and other methods to carry ncRNA. Although several challenges need to be overcome in terms of ncRNA delivered by exosomes for clinical applications, there will is a broad prospect for this medicine in wound healing.
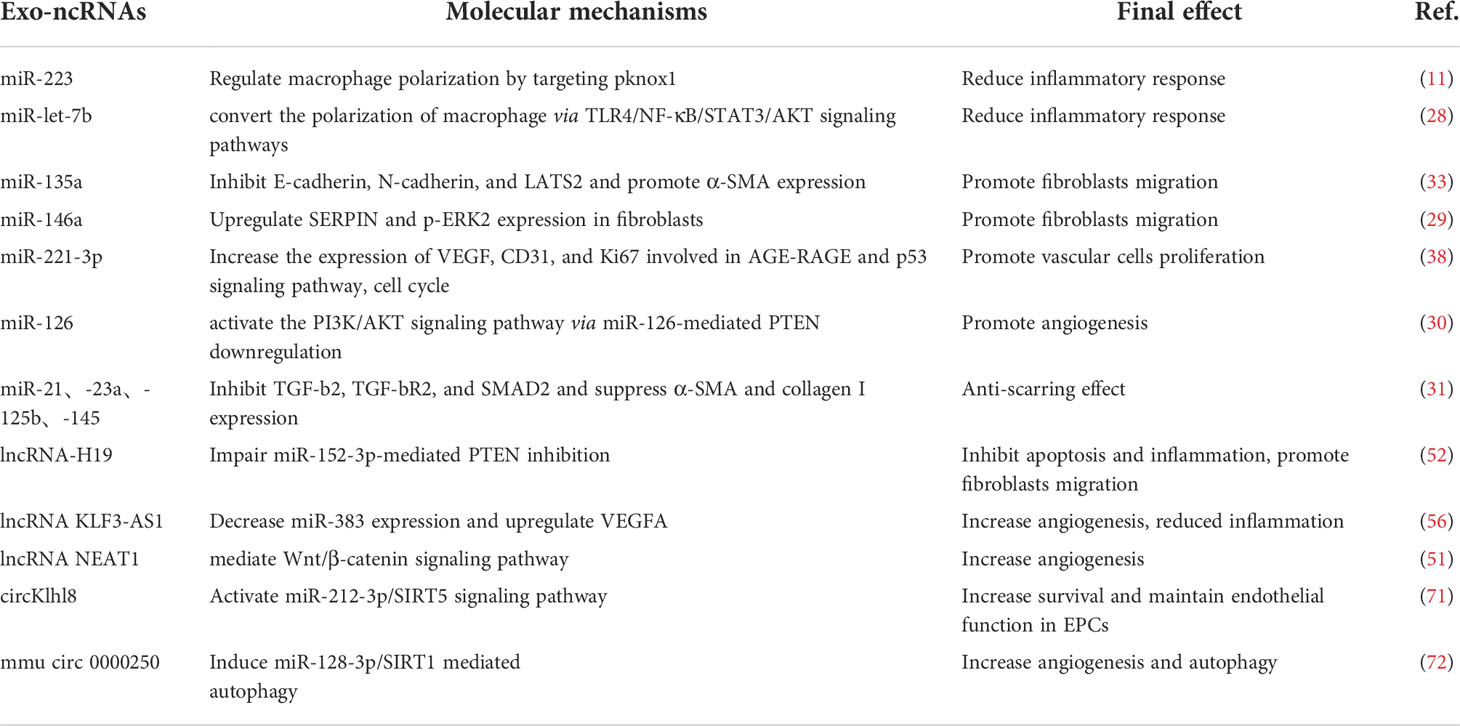
Table 1 Molecular mechanisms and therapeutic potential of exosomal ncRNAs involved in diabetic wound healing.
Author contributions
JS, XDW, XZ, and WA developed the review outline and drafted and wrote the manuscript. YZ and PY developed the figures. JS conceived the idea and supervised the content and writing. PG and XW critically contributed to the content and reviewed the manuscript to ensure accuracy and completeness. All authors contributed to the article and approved the submitted version.
Conflict of interest
All authors were employed by China National Biotech Group.
Publisher’s note
All claims expressed in this article are solely those of the authors and do not necessarily represent those of their affiliated organizations, or those of the publisher, the editors and the reviewers. Any product that may be evaluated in this article, or claim that may be made by its manufacturer, is not guaranteed or endorsed by the publisher.
References
1. Zhu D, Society CD. Guideline for the prevention and treatment of type 2 diabetes mellitus in China (2020 edition). Chin J Pract Intern Med (2021) 41(05):482–548. doi: 10.19538/j.nk2021090106
2. Rodrigues M, Kosaric N, Bonham CA, Gurtner GC. Wound Healing: A Cellular Perspective. Physiol Rev (2019) 99(1):665–706. Epub 2018/11/27. doi: 10.1152/physrev.00067.2017.
3. Patel S, Srivastava S, Singh MR, Singh D. Mechanistic insight into diabetic wounds: Pathogenesis, molecular targets and treatment strategies to pace wound healing. BioMed Pharmacother (2019) 112:108615. doi: 10.1016/j.biopha.2019.108615
4. Armstrong DG, Boulton AJM, Bus SA. Diabetic foot ulcers and their recurrence. N Engl J Med (2017) 376(24):2367–75. doi: 10.1056/NEJMra1615439
5. Chen B, Cai J, Wei Y, Jiang Z, Desjardins HE, Adams AE, et al. Exosomes are comparable to source adipose stem cells in fat graft retention with up-regulating early inflammation and angiogenesis. Plast Reconstr Surg (2019) 144(5):816e–27e. doi: 10.1097/PRS.0000000000006175
6. Huang X, Yuan T, Tschannen M, Sun Z, Jacob H, Du M, et al. Characterization of human plasma-derived exosomal rnas by deep sequencing. BMC Genomics (2013) 14:319. doi: 10.1186/1471-2164-14-319
7. Huang G, Lin G, Zhu Y, Duan W, Jin D. Emerging technologies for profiling extracellular vesicle heterogeneity. Lab Chip (2020) 20(14):2423–37. doi: 10.1039/d0lc00431f
8. Katayama M, Wiklander OPB, Fritz T, Caidahl K, El-Andaloussi S, Zierath JR, et al. Circulating exosomal miR-20b-5p is elevated in type 2 diabetes and could impair insulin action in human skeletal muscle. Diabetes (2019) 68(3):515–26. doi: 10.2337/db18-0470
9. Chang W, Wang J. Exosomes and their noncoding RNA cargo are emerging as new modulators for diabetes mellitus. Cells. (2019) 8(8):853. doi: 10.3390/cells8080853
10. Fang Y, Wang X, Li W, Han J, Jin J, Su F, et al. Screening of circular RNAs and validation of circANKRD36 associated with inflammation in patients with type 2 diabetes mellitus. Int J Mol Med (2018) 42:1865–74. doi: 10.3892/ijmm.2018.3783
11. He X, Dong Z, Cao Y, Wang H, Liu S, Liao L, et al. Msc-derived exosome promotes M2 polarization and enhances cutaneous wound healing. Stem Cells Int (2019) 2019:7132708. doi: 10.1155/2019/7132708
12. Waters R, Subham S, Pacelli S, Modaresi S, Chakravarti AR, Paul A. Development of microrna-146a-Enriched stem cell secretome for wound-healing applications. Mol Pharm (2019) 16(10):4302–12. doi: 10.1021/acs.molpharmaceut.9b00639
13. Yan C, Chen J, Wang C, Yuan M, Kang Y, Wu Z, et al. Milk exosomes-mediated mir-31-5p delivery accelerates diabetic wound healing through promoting angiogenesis. Drug Delivery (2022) 29(1):214–28. doi: 10.1080/10717544.2021.2023699
14. Liu S, Chen Y, Ren Y, Zhou J, Ren J, Lee I, et al. A trna-derived rna fragment plays an important role in the mechanism of arsenite -induced cellular responses. Sci Rep (2018) 8(1):16838. doi: 10.1038/s41598-018-34899-2
15. Pan BT, Johnstone RM. Fate of the transferrin receptor during maturation of sheep reticulocytes in vitro: Selective externalization of the receptor. Cell (1983) 33(3):967–78. doi: 10.1016/0092-8674(83)90040-5
16. Stahl PD, Raposo G. Exosomes and extracellular vesicles: The path forward. Essays Biochem (2018) 62(2):119–24. doi: 10.1042/EBC20170088
17. Tkach M, Thery C. Communication by extracellular vesicles: Where we are and where we need to go. Cell (2016) 164(6):1226–32. doi: 10.1016/j.cell.2016.01.043
18. Yu P, Guo J, Li J, Shi X, Xu N, Jiang Y, et al. lncRNA-H19 in fibroblasts promotes wound healing in diabetes. Diabetes. (2022) 71(7):1562–78. doi: 10.2337/db21-0724
19. Yan C, Chen J, Yang X, Li W, Mao R, Chen Z. Emerging roles of long non-coding RNAs in diabetic foot ulcers. Diabetes Metab Syndr Obes (2021) 14:2549–60. doi: 10.2147/DMSO.S310566
20. Nie H, Wang Y, Liao Z, Zhou J, Ou C. The function and mechanism of circular rnas in gastrointestinal tumours. Cell Prolif (2020) 53(7):e12815. doi: 10.1111/cpr.12815
21. Cortez MA, Bueso-Ramos C, Ferdin J, Lopez-Berestein G, Sood AK, Calin GA. Micrornas in body fluids–the mix of hormones and biomarkers. Nat Rev Clin Oncol (2011) 8(8):467–77. doi: 10.1038/nrclinonc.2011.76
22. Las Heras K, Igartua M, Santos-Vizcaino E, Hernandez RM. Chronic wounds: Current status, available strategies and emerging therapeutic solutions. J Control Release (2020) 328:532–50. doi: 10.1016/j.jconrel.2020.09.039
23. Liu S, Jiang L, Li H, Shi H, Luo H, Zhang Y, et al. Mesenchymal stem cells prevent hypertrophic scar formation Via inflammatory regulation when undergoing apoptosis. J Invest Dermatol (2014) 134(10):2648–57. doi: 10.1038/jid.2014.169
24. Qi Y, Jiang D, Sindrilaru A, Stegemann A, Schatz S, Treiber N, et al. Tsg-6 released from intradermally injected mesenchymal stem cells accelerates wound healing and reduces tissue fibrosis in murine full-thickness skin wounds. J Invest Dermatol (2014) 134(2):526–37. doi: 10.1038/jid.2013.328
25. Wu Y, Chen L, Scott PG, Tredget EE. Mesenchymal stem cells enhance wound healing through differentiation and angiogenesis. Stem Cells (2007) 25(10):2648–59. doi: 10.1634/stemcells.2007-0226
26. Zhang D, Cao X, Li J, Zhao G. Mir-210 inhibits nf-kappab signaling pathway by targeting Dr6 in osteoarthritis. Sci Rep (2015) 5:12775. doi: 10.1038/srep12775
27. He L, Chen Y, Ke Z, Pang M, Yang B, Feng F, et al. Exosomes derived from mirna-210 overexpressing bone marrow mesenchymal stem cells protect lipopolysaccharide induced chondrocytes injury Via the nf-kappab pathway. Gene (2020) 751:144764. doi: 10.1016/j.gene.2020.144764
28. Ti D, Hao H, Tong C, Liu J, Dong L, Zheng J, et al. Lps-preconditioned mesenchymal stromal cells modify macrophage polarization for resolution of chronic inflammation Via exosome-shuttled let-7b. J Transl Med (2015) 13:308. doi: 10.1186/s12967-015-0642-6
29. Wang J, Wu H, Peng Y, Zhao Y, Qin Y, Zhang Y, et al. Hypoxia adipose stem cell-derived exosomes promote high-quality healing of diabetic wound involves activation of Pi3k/Akt pathways. J Nanobiotechnology (2021) 19(1):202. doi: 10.1186/s12951-021-00942-0
30. Ding J, Wang X, Chen B, Zhang J, Xu J. Exosomes derived from human bone marrow mesenchymal stem cells stimulated by deferoxamine accelerate cutaneous wound healing by promoting angiogenesis. BioMed Res Int (2019) 2019:9742765. doi: 10.1155/2019/9742765
31. Zhang Y, Pan Y, Liu Y, Li X, Tang L, Duan M, et al. Exosomes derived from human umbilical cord blood mesenchymal stem cells stimulate regenerative wound healing via transforming growth factor-β receptor inhibition. Stem Cell Res Ther (2021) 12(1):434. doi: 10.1186/s13287-021-02517-0
32. desJardins-Park HE, Foster DS, Longaker MT. Fibroblasts and wound healing: An update. Regener Med (2018) 13(5):491–5. doi: 10.2217/rme-2018-0073
33. Hormozi M, Baharvand P. Achillea biebersteinni afan may inhibit scar formation: In vitro study. Mol Genet Genomic Med (2019) 7(5):e640. doi: 10.1002/mgg3.640
34. Chen T, Gao S, Hao Y, Zhang F, Tang X, Wei Z, et al. [Experimental study of human amniotic mesenchymal stem cell exosome promoting fibroblasts migration through microrna-135a]. Zhongguo Xiu Fu Chong Jian Wai Ke Za Zhi (2020) 34(2):234–9. doi: 10.7507/1002-1892.201907136
35. Chen G, Wu Y, Zou L, Zeng Y. (2021). Effect of microrna-146a modified adipose-derived stem cell exosomes on rat back wound healing. Int J LowExtrem Wounds. doi: 10.1177/15347346211038092
36. Okonkwo UA, DiPietro LA. Diabetes and wound angiogenesis. Int J Mol Sci (2017) 18(7):1419. doi: 10.3390/ijms18071419
37. Quero L, Tiaden AN, Hanser E, Roux J, Laski A, Hall J, et al. Mir-221-3p drives the shift of M2-macrophages to a pro-inflammatory function by suppressing Jak3/Stat3 activation. Front Immunol (2019) 10:3087. doi: 10.3389/fimmu.2019.03087
38. Xu J, Bai S, Cao Y, Liu L, Fang Y, Du J, et al. Mirna-221-3p in endothelial progenitor cell-derived exosomes accelerates skin wound healing in diabetic mice. Diabetes Metab Syndr Obes (2020) 13:1259–70. doi: 10.2147/DMSO.S243549
39. Yu M, Liu W, Li J, Lu J, Lu H, Jia W, et al. Exosomes derived from atorvastatin-pretreated msc accelerate diabetic wound repair by enhancing angiogenesis Via Akt/Enos pathway. Stem Cell Res Ther (2020) 11(1):350. doi: 10.1186/s13287-020-01824-2
40. Yu Q, Liu L, Zhang X, Chang H, Ma S, Xie Z, et al. Mir-221-3p targets Hipk2 to promote diabetic wound healing. Microvasc Res (2022) 140:104306. doi: 10.1016/j.mvr.2021.104306
41. Xiong Y, Chen L, Yan C, Zhou W, Endo Y, Liu J, et al. Circulating exosomal mir-20b-5p inhibition restores Wnt9b signaling and reverses diabetes-associated impaired wound healing. Small (2020) 16(3):e1904044. doi: 10.1002/smll.201904044
42. Lv Q, Deng J, Chen Y, Wang Y, Liu B, Liu J. Engineered human adipose stem-Cell-Derived exosomes loaded with mir-21-5p to promote diabetic cutaneous wound healing. Mol Pharm (2020) 17(5):1723–33. doi: 10.1021/acs.molpharmaceut.0c00177
43. Huang C, Luo W, Wang Q, Ye Y, Fan J, Lin L, et al. Human mesenchymal stem cells promote ischemic repairment and angiogenesis of diabetic foot through exosome mirna-21-5p. Stem Cell Res (2021) 52:102235. doi: 10.1016/j.scr.2021.102235
44. Guo Hy, Li Wq, Liu Ky, Xiao Rf, Sun Dl, Xi Jy. Recent research progress in construction of biomimetic engineered cardiac tissue based on extracellular matrix. Zhongguo Zuzhi Gongcheng Yanjiu. (2021) 25(10):1577–84. doi: 10.3969/j.issn
45. Pastar I, Stojadinovic O, Yin NC, Ramirez H, Nusbaum AG, Sawaya A, et al. Epithelialization in wound healing: A comprehensive review. Adv Wound Care (New Rochelle) (2014) 3(7):445–64. doi: 10.1089/wound.2013.0473
46. Li Q, Zhao H, Chen W, Huang P, Bi J. Human keratinocyte-derived microvesicle mirna-21 promotes skin wound healing in diabetic rats through facilitating fibroblast function and angiogenesis. Int J Biochem Cell Biol (2019) 114:105570. doi: 10.1016/j.biocel.2019.105570
47. Zhang Y, Yan J, Liu Y, Chen Z, Li X, Tang L, et al. Human amniotic fluid stem cell-derived exosomes as a novel cell-free therapy for cutaneous regeneration. Front Cell Dev Biol (2021) 9:685873. doi: 10.3389/fcell.2021.685873
48. Li X, Li N, Li B, Feng Y, Zhou D, Chen G. Noncoding rnas and rna-binding proteins in diabetic wound healing. Bioorg Med Chem Lett (2021) 50:128311. doi: 10.1016/j.bmcl.2021.128311
49. Li B, Zhou Y, Chen J, Wang T, Li Z, Fu Y, et al. Long noncoding rna H19 acts as a mir-29b sponge to promote wound healing in diabetic foot ulcer. FASEB J (2021) 35(1):e20526. doi: 10.1096/fj.201900076RRRRR
50. Born LJ, Chang KH, Shoureshi P, Lay F, Bengali S, Hsu ATW, et al. Hotair-loaded mesenchymal Stem/Stromal cell extracellular vesicles enhance angiogenesis and wound healing. Adv Healthc Mater (2022) 11(5):e2002070. doi: 10.1002/adhm.202002070
51. Guo L, Chen Y, Feng X, Sun D, Sun J, Mou S, et al. Oxidative stress-induced endothelial cells-derived exosomes accelerate skin flap survival through lnc Neat1-mediated promotion of endothelial progenitor cell function. Stem Cell Res Ther (2022) 13(1):325. doi: 10.1186/s13287-022-03013-9
52. Li B, Luan S, Chen J, Zhou Y, Wang T, Li Z, et al. The msc-derived exosomal lncrna H19 promotes wound healing in diabetic foot ulcers by upregulating pten Via microrna-152-3p. Mol Ther Nucleic Acids (2020) 19:814–26. doi: 10.1016/j.omtn.2019.11.034
53. Zgheib C, Hodges MM, Hu J, Liechty KW, Xu J. Long non-coding rna Lethe regulates hyperglycemia-induced reactive oxygen species production in macrophages. PloS One (2017) 12(5):e0177453. doi: 10.1371/journal.pone.0177453
54. He M, Tu L, Shu R, Meng Q, Du S, Xu Z, et al. Long noncoding rna Casc2 facilitated wound healing through mirna-155/Hif-1alpha in diabetic foot ulcers. Contrast Media Mol Imaging (2022) 2022:6291497. doi: 10.1155/2022/6291497
55. Chen Z, Hu Z, Sui Q, Huang Y, Zhao M, Li M, et al. Lncrna Fam83a-As1 facilitates tumor proliferation and the migration Via the hif-1alpha/ glycolysis axis in lung adenocarcinoma. Int J Biol Sci (2022) 18(2):522–35. doi: 10.7150/ijbs.67556
56. Han ZF, Cao JH, Liu ZY, Yang Z, Qi RX, Xu HL. Exosomal lncrna Klf3-As1 derived from bone marrow mesenchymal stem cells stimulates angiogenesis to promote diabetic cutaneous wound healing. Diabetes Res Clin Pract (2022) 183:109126. doi: 10.1016/j.diabres.2021.109126
57. Herter EK, Li D, Toma MA, Vij M, Li X, Visscher D, et al. Wakmar2, a long noncoding rna downregulated in human chronic wounds, modulates keratinocyte motility and production of inflammatory chemokines. J Invest Dermatol (2019) 139(6):1373–84. doi: 10.1016/j.jid.2018.11.033
58. Lu P, Takai K, Weaver VM, Werb Z. Extracellular matrix degradation and remodeling in development and disease. Cold Spring Harb Perspect Biol (2011) 3(12):a005058. doi: 10.1101/cshperspect.a005058
59. Patop IL, Wust S, Kadener S. Past, present, and future of circrnas. EMBO J (2019) 38(16):e100836. doi: 10.15252/embj.2018100836
60. Salzman J. Circular rna expression: Its potential regulation and function. Trends Genet (2016) 32(5):309–16. doi: 10.1016/j.tig.2016.03.002
61. Cortés-López M, Miura P. Emerging functions of circular rnas. Yale J Biol Med (2016) 89(4):527–37.
62. Qi X, Zhang DH, Wu N, Xiao JH, Wang X, Ma W. Cerna in cancer: Possible functions and clinical implications. J Med Genet (2015) 52(10):710–8. doi: 10.1136/jmedgenet-2015-103334
63. Zhong Y, Du Y, Yang X, Mo Y, Fan C, Xiong F, et al. Circular rnas function as cernas to regulate and control human cancer progression. Mol Cancer (2018) 17(1):79. doi: 10.1186/s12943-018-0827-8
64. Shi R, Jin Y, Zhao S, Yuan H, Shi J, Zhao H. Hypoxic adsc-derived exosomes enhance wound healing in diabetic mice Via delivery of circ-Snhg11 and induction of M2-like macrophage polarization. BioMed Pharmacother (2022) 153:113463. doi: 10.1016/j.biopha.2022.113463
65. Wang M, Suo L, Yang S, Zhang W. Circrna 001372 reduces inflammation in propofol-induced neuroinflammation and neural apoptosis through Pik3ca/Akt/Nf-kappab by mirna-148b-3p. J Invest Surg (2021) 34(11):1167–77. doi: 10.1080/08941939.2020.1771639
66. Si C, Wang J, Ma W, Hua H, Zhang M, Qian W, et al. Circular rna expression profile in human fibroblast premature senescence after repeated ultraviolet b irradiations revealed by microarray. J Cell Physiol (2019) 234(10):18156–68. doi: 10.1002/jcp.28449
67. Tang CM, Zhang M, Huang L, Hu ZQ, Zhu JN, Xiao Z, et al. CircRNA_000203 enhances the expression of fibrosis-associated genes by derepressing targets of miR-26b-5p, Col1a2 and CTGF, in cardiac fibroblasts. Sci Rep (2017) 7:40342. doi: 10.1038/srep40342
68. Wang A, Toma MA, Ma J, Li D, Vij M, Chu T, et al. Circular rna Hsa_Circ_0084443 is upregulated in diabetic foot ulcer and modulates keratinocyte migration and proliferation. Adv Wound Care (New Rochelle) (2020) 9(4):145–60. doi: 10.1089/wound.2019.0956
69. Chen ZJ, Shi XJ, Fu LJ, Liu J, Shi K, Zhang WB, et al. Serum and exosomal Hsa_Circ_0000907 and Hsa_Circ_0057362 as novel biomarkers in the early diagnosis of diabetic foot ulcer. Eur Rev Med Pharmacol Sci (2020) 24(15):8117–26. doi: 10.26355/eurrev_202008_22498
70. Kaushik K, Das A. Endothelial progenitor cell therapy for chronic wound tissue regeneration. Cytotherapy (2019) 21(11):1137–50. doi: 10.1016/j.jcyt.2019.09.002
71. Shang B, Xu T, Hu N, Mao Y, Du X. Circ-Klhl8 overexpression increased the therapeutic effect of epcs in diabetic wound healing Via the mir-212-3p/Sirt5 axis. J Diabetes Complications (2021) 35(11):108020. doi: 10.1016/j.jdiacomp.2021.108020
72. Shi R, Jin Y, Hu W, Lian W, Cao C, Han S, et al. Exosomes derived from Mmu_Circ_0000250-modified adipose-derived mesenchymal stem cells promote wound healing in diabetic mice by inducing mir-128-3p/Sirt1-Mediated autophagy. Am J Physiol Cell Physiol (2020) 318(5):C848–C56. doi: 10.1152/ajpcell.00041.2020
Keywords: exosomes, wound healing, non-coding RNA, diabetes mellitus, delivery
Citation: Shen J, Zhao X, Zhong Y, Yang P, Gao P, Wu X, Wang X and An W (2022) Exosomal ncRNAs: The pivotal players in diabetic wound healing. Front. Immunol. 13:1005307. doi: 10.3389/fimmu.2022.1005307
Received: 28 July 2022; Accepted: 06 October 2022;
Published: 07 November 2022.
Edited by:
Yuanzeng Min, University of Science and Technology of China, ChinaCopyright © 2022 Shen, Zhao, Zhong, Yang, Gao, Wu, Wang and An. This is an open-access article distributed under the terms of the Creative Commons Attribution License (CC BY). The use, distribution or reproduction in other forums is permitted, provided the original author(s) and the copyright owner(s) are credited and that the original publication in this journal is cited, in accordance with accepted academic practice. No use, distribution or reproduction is permitted which does not comply with these terms.
*Correspondence: Xudong Wang, eGR3YW5nMTNAMTYzLmNvbQ==; Wenlin An, YW53ZW5saW5Ac2lub3BoYXJtLmNvbQ==