- 1Research and Development Immunoassays, Roche Diagnostics GmbH, Penzberg, Germany
- 2Biostatistics & Data Science, Roche Diagnostics GmbH, Penzberg, Germany
Background: Automated, high throughput assays are required to quantify the immune response after infection with or vaccination against severe acute respiratory syndrome coronavirus 2 (SARS-CoV-2). This study on the Roche Elecsys® Anti-SARS-CoV-2 S (ACOV2S) assay provides insights on the assay design and performance.
Methods: The ACOV2S assay quantifies antibodies to the receptor-binding domain of the SARS-CoV-2 spike protein. The assigned units and the underlying standardization were compared to the international reference standard in BAU/mL. Assay specificity was assessed in samples (n=5981) collected prior to the COVID-19 pandemic and in samples from patients with non-COVID-19 respiratory infections (n=697) or other infectious diseases (n=771). Sensitivity was measured in 1313 samples from patients with mild COVID-19 and 297 samples from patients hospitalized with COVID-19. Comparison of results was performed to a comparator semi-quantitative anti-S1 assay of indirect detection format as well as a commercially available and an in-house version of a surrogate neutralization assay (ACE2-RBD).
Results: The originally assigned units for the ACOV2S assay were shown to be congruent to the units of the First International WHO Standard for anti-SARS-CoV-2 immunoglobulins. Overall specificity was 99.98% with no geographical differences noted and no loss of specificity in samples containing potentially cross-reacting antibodies. High sensitivity was observed, with 98.8% of samples reported to be reactive >14 days after infection and sustained detection of antibodies over time. For all samples, ACOV2S titers and neutralization capacities developed with comparable dynamics. Robust standardization and assay setup enable excellent reproducibility of results, independent of lot or analyzer used.
Conclusion: The results from this study confirmed that ACOV2S is a highly sensitive and specific assay and correlates well with surrogate neutralization assays. The units established for ACOV2S are also interchangeable with the units of the First International WHO Standard for anti-SARS-CoV-2 immunoglobulins. Worldwide availability of the assay and analyzers render ACOV2S a highly practical tool for population-wide assessment and monitoring of the humoral response to SARS-CoV-2 infection or vaccination.
1. Introduction
The assessment of the humoral immune response to infection with respiratory viruses that cause common cold-type diseases is not necessarily a typical diagnostic request. However, with the emergence in late 2019 of SARS-CoV-2, a virus that can cause the severe acute respiratory syndrome COVID-19, a need for serologic monitoring of antibody responses arose. In the early phase of the pandemic, the highly specific and sensitive Elecsys® Anti-SARS-CoV-2 (ACOV2; Roche Diagnostics) assay, was developed for post-acute detection of infection in order to improve our understanding of virus circulation dynamics (1).
The SARS-CoV-2 virus is associated with considerable morbidity and mortality and has placed a substantial burden on healthcare systems. As a result, the development of an effective vaccine was prioritized, as herd immunity was considered to be key for the transition from pandemic to endemic and to limit the clinical burden of COVID-19 (2). However, prior to the emergence of SARS-CoV-2, protective immunity to infections with viruses of the Coronaviridae family had been considered challenging, as protection seemed to be associated with strong individual variation and, as for many respiratory diseases, appeared to be transient (2–4). The SARS-CoV-2 spike (S) protein was considered the most promising target for protective immunity as it is the most prominent structural protein on the surface of the virus. Consequently, the majority of SARS-CoV-2 vaccine candidates focused on the S protein or derivatives thereof (5). In addition, vaccines based on dead or attenuated viruses contained the S protein as a prominent immunogen (6).
Detection and monitoring of the humoral immune response by quantitation of antibody titers following vaccination is well established in routine diagnostic laboratories. Detected antibodies primarily reflect the degree of a mounted humoral immune response but can also be considered as a generic marker of activation of the immune system. Also, the generation of immunological memory can be deduced if the test is set up to reflect antibody affinity maturation. Appropriate antigen selection and tailoring can improve correlation of the test result to the neutralization potential of the detected antibodies. Titer monitoring over time requires quantitation of the polyclonal antibody response raised by the host. This implies that the assay has to be optimized for linear dilution of a rather heterogeneous analyte. Equally important, consistency of obtained results over time requires robust assay standardization in order to ensure reproducible results independent of manufacturing lot and time.
Quantifying antibodies to the receptor-binding domain (RBD) of the SARS-CoV-2 S protein was expected to provide a good positive predictive value for neutralization. The RBD binds to the angiotensin-converting enzyme-2 (ACE2) receptor and mediates the initial step of virus fusion with the host cell (7). Thus, antibodies against RBD have a high likelihood of interfering with binding to ACE2, i.e., they confer neutralizing effects (5). The RBD is a well-defined subdomain of the S protein and is composed of only 222 amino acids (8). Its size and structure rendered it a promising antigen candidate.
We developed the Elecsys Anti-SARS-CoV-2-S (ACOV2S) assay in order to quantitatively determine the humoral response to SARS-CoV-2 infection or vaccination. Here, we describe the design of the assay and present performance data, including standardization and correlation to the first international WHO standard on anti-SARS-CoV-2 immunoglobulins, specificity and sensitivity in a large cohort of samples acquired either pre-pandemic or from patients with a polymerase chain reaction (PCR)-confirmed SARS-CoV-2 infection, and correlation with a surrogate neutralization assay.
2. Methods
2.1 Study design
The performance of the Elecsys ACOV2S assay was evaluated at Roche Diagnostics (Penzberg, Germany). All samples were collected and tested in accordance with applicable regulations, including relevant European Union directives and regulations, and the principles of the Declaration of Helsinki. Anonymized frozen serum or plasma samples were used for this study and included residual samples from blood donation centers or routine laboratory diagnostics and commercially available samples. A statement was obtained from the Ethics Committee of the Landesärztekammer Bayern confirming that there are no objections to the transfer and coherent use of anonymized leftover samples. For specificity analyses, samples from blood donors (n=2713 from the USA and n=740 from Africa) or routine diagnostic samples (n=2528 from Europe) collected before December 2019, and therefore presumed to be negative for SARS-CoV-2, were tested. In addition, cross-reactivity of the ACOV2S assay was assessed in 697 samples from patients with a respiratory infection and 771 samples from patients with other infectious, auto-immune, or non-infectious hepatic disease. These samples were obtained prior to October 2019 and were therefore considered to be seronegative for SARS-CoV-2-specific antibodies. Presence of specific immunoglobulins for other infections was confirmed using an approved in vitro diagnostic test for this indication. The immunoglobulin isotype was IgG unless indicated otherwise. To assess sensitivity, 1610 PCR-confirmed single or sequential samples from 402 different patients with a native SARS-CoV-2 infection that occurred between February and April 2020 (i.e., infection with a strain of presumed Wuhan-Hu-1 phenotype) were tested. This included 1313 samples from 159 patients with mild COVID-19 (defined as not requiring hospitalization) and 297 samples taken from 243 patients who had been hospitalized with COVID-19. Hospitalization typically occurred in response to severe symptoms such as respiratory distress or critically low oxygenation of peripheral blood, with all clinical decision-making at the discretion of the local treating physician. All samples had a known time difference between positive PCR test and blood draw. The date of PCR diagnosis served as the reference point for infection as memory recall of symptom onset can vary significantly between individuals, particularly in cases with a mild course of disease. The majority of the samples from mild cases were taken >20 days post-PCR, conversely, the majority of the samples from hospitalized patients were taken within 20 days of a positive PCR result. A representative subset of the sensitivity samples were also tested using the Siemens SARS-CoV-2 immunoglobulin G (IgG) (COV2G) semi-quantitative assay according to the manufacturer’s instructions. Analytical studies on precision and reproducibility required considerable sample volumes. To fulfil these needs, pooled samples from two different donors with a native infection had to be used.
2.2 Elecsys anti-SARS-CoV-2 S assay
The Elecsys Anti-SARS-CoV-2 S (ACOV2S) immunoassay is a quantitative electrochemiluminescence immunoassay (ECLIA) that detects antibodies to the SARS-CoV-2 S protein RBD. The candidate antigens were generated from HEK293 culture and transient transfection. The expression constructs included a leader signal for secretion and a His-tag for purification. Recombinant protein was then harvested from culture supernatants and purified by affinity and size exclusion chromatography. Labelling of proteins with biotin and ruthenium was realized by NHS-mediated amide bond formation of labels and protein of interest. The assay applies the double antigen sandwich format detecting immunoglobulins from the sample when bridged between two specifier antigens provided by the assay. Results are automatically read off a lot-specific standard curve and reported as the analyte concentration of each sample in U/mL. As the ACOV2S assay is a quantitative and standardized assay, no specific cut-off formula was applied. A medical decision point (MDP) indicative of the significant presence of RBD-specific antibodies was postulated at 0.8 U/mL based on a preliminary sample set from infected and non-infected samples (data not shown). This MDP was then found to fit well in the design verification studies shown in this manuscript and previous publications (9). Values between 0.40–250 represent the linear range. Samples above 250 U/mL can be automatically diluted into the linear range of the assay with Diluent Universal (Roche Diagnostics, Rotkreuz, Switzerland). The analyzer then multiplies diluted results with the dilution factor. Experimental setups as well as data acquisition and evaluation followed standard operation procedures, which were in alignment with the respective CLSI guidelines.
2.3 Comparator assays
Samples were also analyzed using a comparator immunoassay and a neutralizing assay. The Siemens SARS-CoV-2 IgG (COV2G) antibody test is an automated two-step indirect chemiluminescent sandwich immunoassay that detects IgG antibodies against the spike RBD of the SARS-CoV-2 virus. Here the detected antibodies bind with one or both of their paratopes to the immobilized antigen, while counterstaining with anti-human IgG then indirectly detects the bound antibodies. Indirect detection methods are less able to reflect antibody maturation compared to double antigen sandwich formats (10).
COV2G results are reported in index values, with <1.0 interpreted as non-reactive (negative) for anti-SARS-CoV-2 antibodies and ≥1.0 interpreted as reactive (positive) for anti-SARS-CoV-2 antibodies (11). Of note, the first generation COV2G assay was used in this study. This has since been replaced with an updated version with broadened dynamic range, but still utilizes an indirect detection format.
The GenScript cPass™ SARS-CoV-2 neutralization antibody detection kit is a blocking enzyme-linked immunosorbent assay (ELISA) intended for the qualitative and semi-quantitative direct detection of immunoglobulins that neutralize the interaction between RBD and hACE2. In samples that do not contain SARS-CoV-2 neutralizing antibodies, RBD conjugated to HRP can bind to ACE-2 without any impairment, generating a strong signal comparable to the reference reaction. If SARS-CoV-2 neutralizing antibodies are present, they will bind to RBD-HRP and prevent the interaction with ACE-2, resulting in impaired signal. Results are reported as ratio of sample result to reference result which equals percentage binding inhibition, with <30% interpreted as negative and ≥30% interpreted as positive for SARS-CoV-2 neutralizing antibodies (12).
We also developed an in-house version of a surrogate neutralization assay for application on cobas e analyzers (Elecsys ACE2-RBD assay). Similar to cPass, but performed in solution rather than solid phase, this method measures the potential of samples containing SARS-CoV-2 antibodies to compete with ACE2-RBD binding.
2.4 Interference Testing
Potential interference was investigated for commonly used drugs (13, 14), drugs often used in clinical treatment of acute COVID-19, and drugs that may interrupt the RBD-ACE2 interface (15). Potentially unspecific interference and dilution effects of the diluent used for solubilization of the drug were compensated by addition of the respective diluent without drug to a reference sample.
2.5 Statistical analyses
The software R, version 3.4.0, was used for regression analyses (Passing-Bablock) to the First International WHO Standard for anti-SARS-CoV-2 immunoglobulins and for the assessment of reproducibility and precision of results across different aliquots, days, analyzers, and assay lots. The experimental setup and statistical analyses followed the recommendations of the respective CLSI guideline (EP05-A3) (16). In brief, five aliquots of the indicated samples were measured over five days with three different lots on three different analyzer platforms. Observed variation was calculated in R for the indicated categories by standard procedures. All other analyses were performed using GraphPad Prism 9. For sensitivity, specificity, and precision, point estimates and 95% confidence intervals were calculated. RBD titers from sequential samples were displayed as spaghetti and smoothened median curves for the ACOV2S and comparator assays. Relative recovery was calculated for each participant as the titer measured at the last timepoint as a percentage of the highest titer measured. Qualitative agreement between the Genscript cPass and Elecsys ACE2-RBS neutralization assays was analyzed by positive predictive value (PPV) and relative specificity and sensitivity with exact 95% binomial CIs.
3. Results
3.1 Selection of antigen and assay format
The RBD antigen could be expressed in high yields and reproducibly high quality and was highly amenable to purification and labeling procedures. We also evaluated trimeric spike and monomeric spike S1 subdomain antigens, but these could be expressed with lower yields only, and additionally required higher purification stringency. Moreover, an initial functional assessment with pre-pandemic samples suggested that better specificity was achieved for antibodies targeting the RBD compared with the trimeric spike or monomeric spike S1 antigens (Figure 1).
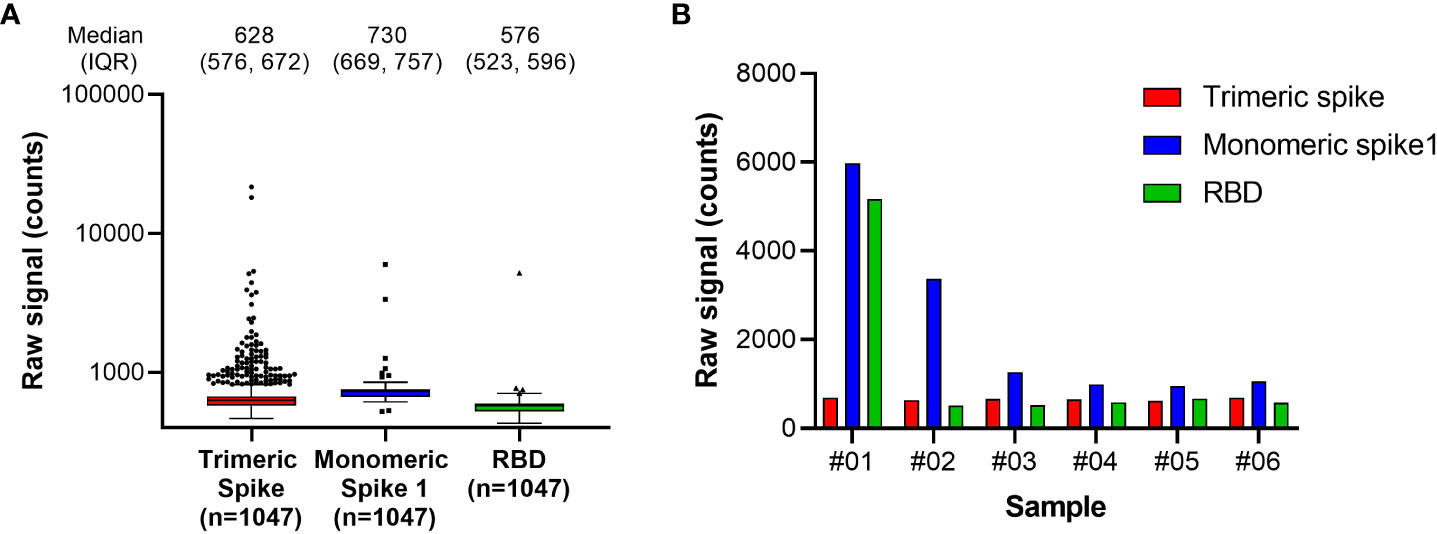
Figure 1 Antigen specificity in pre-pandemic samples. Assessment of Spike antigen specificity in pre-pandemic serum samples (n=1047) is shown in (A). Samples with results exceeding the 95% confidence interval of signal distribution in the assessment with Monomeric Spike 1 or RBD, as shown in (A), were resolved in detail in (B). RBD, receptor binding domain.
The Double Antigen Sandwich (DAGS) immunoassay format is well established for the detection of immunoglobulins using Elecsys assays on cobas e analyzers. Elecsys assays have a short turnaround time of typically 18 minutes, which requires a highly sensitive detection system and, most importantly, rapid formation of detectable immunocomplexes including the analyte. Successful complex formation requires the provision of all assay components in solution (no precoated solid phase) to benefit from free diffusion. This supports low thresholds for detectable concentrations and, together with the sensitive electrochemiluminescence (ECL) detection method coming with a broad linear range, enables strong signal dynamics of the DAGS method used in Elecsys assays. The DAGS format per se does not differentiate between immunoglobulin classes. However, bridging of two antigens is required for signal generation, which leads by design to the predominant detection of high-affinity antibodies, i.e., IgG. We sought to further sustain this tendency by the provision of well-defined and monomeric antigens as well as rather stringent buffer conditions, such that single binding events, as mediated by IgG, are the major signal driver. RBD was ultimately selected as the ideal candidate to fulfill these requirements. By design, DAGS assays feature strong specificity and do not require sample predilution to reduce unspecific reactivity. The application of a highly specific antigen further corroborates this feature. Together with the adjustment of buffer stringency, these aspects serve to balance the required specificity versus sensitivity.
3.2 Standardization and correlation to WHO standard
At the time of the development and launch of the ACOV2S assay, no standard reference material existed for qualitative SARS-CoV-2 antibody assays. We developed an internal reference standard to enable reproducible and lot independent test results in arbitrary units. Parallel assessment of a medical decision point (MDP) for differentiation of samples being non-reactive or reactive for RBD-specific antibodies indicated that such an MDP might lie close to the numeric unit of 1 on this scale. In addition, established working calibrators based on pools of native human sample material were adjusted to cover critical supporting points enabling the generation of a robust standard curve. The primary measuring range of the ACOV2S assay was defined from 0.4 to 250 U/mL, determined by optimal linear detection of a dilution series of human samples and with the majority of results within this range following native infection with a mild course of disease. While retrospectively a larger measuring range had been desirable, automated dilution provides a convenient workaround. We subsequently observed that the units of the First International WHO Standard for anti-SARS-CoV-2 immunoglobulins (NIBSC code 20/136) were congruent to the originally assigned units based on the internal standard (Figure 2). Linearity was proven using a dilution series of the WHO standard as well as excellent value recovery. Robustness of the applied standardization procedure could be proven by the use of three different assay lots, one representing the initial assay lot undergoing reference standardization (DR lot), an additional lot from early phase of routine production (MP02) and another routine lot produced 3 production events later (MP05, 9 months after DR lot production). The data shown in Figure 2 were generated by parallel analysis of these three lots. The observed congruency of the results indicates good stability of the product over time as well as reliable standardization ensuring the same numeric results independent of lot and product age.
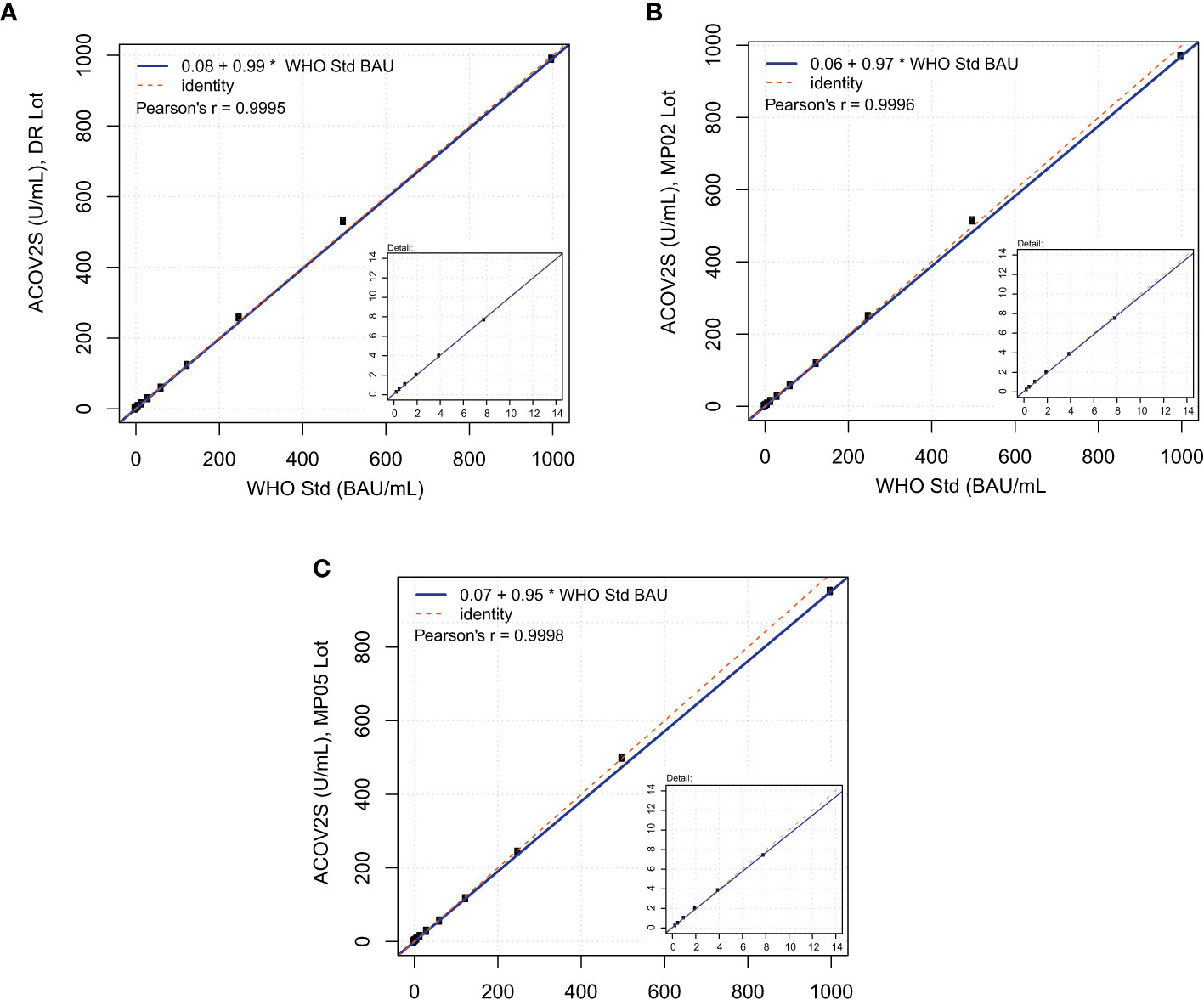
Figure 2 Correlation of ACOV2S units to the First International WHO Standard for anti-SARS-CoV-2 immunoglobulins. Linearity using a dilution series of the WHO standard for (A) lot DR, (B) lot MP02, and (C) lot MP05.
3.3 Specificity
Specificity was extensively assessed in pre-pandemic samples obtained from blood donors in the USA and Africa and routine diagnostic samples acquired in Europe. One presumably false-positive result was observed from >5000 samples in total, giving an overall specificity of 99.98%. No differences in specificity were observed between samples from different geographical locations and no differences were observed between different serological backgrounds (Table 1). Specificity of the ACOV2S assay was also confirmed in a cohort of pre-pandemic samples with potentially cross-reacting antibodies to related indications, i.e., respiratory diseases (Table 2) or less closely related clinical indications (Table S1). Potential assay interference from common drugs, special drugs used in COVID-19 treatment or drugs with postulated potential to interrupt the RBD-ACE2 interface (14) was also assessed. For the vast majority, no significant interference was observed, as analyte recovery in spiked samples (1–3x daily dose) was within acceptance range (90–110%) compared to the unspiked reference sample (Table S2). Analyte recovery was 69–80% using higher drug concentrations of ritonavir (0.360 and 0.480 mg/L) and 87–88% with highest itraconazole concentration (30 mg/L).

Table 1 Specificity in samples obtained from routine diagnostic laboratories and blood donors pre-pandemic.
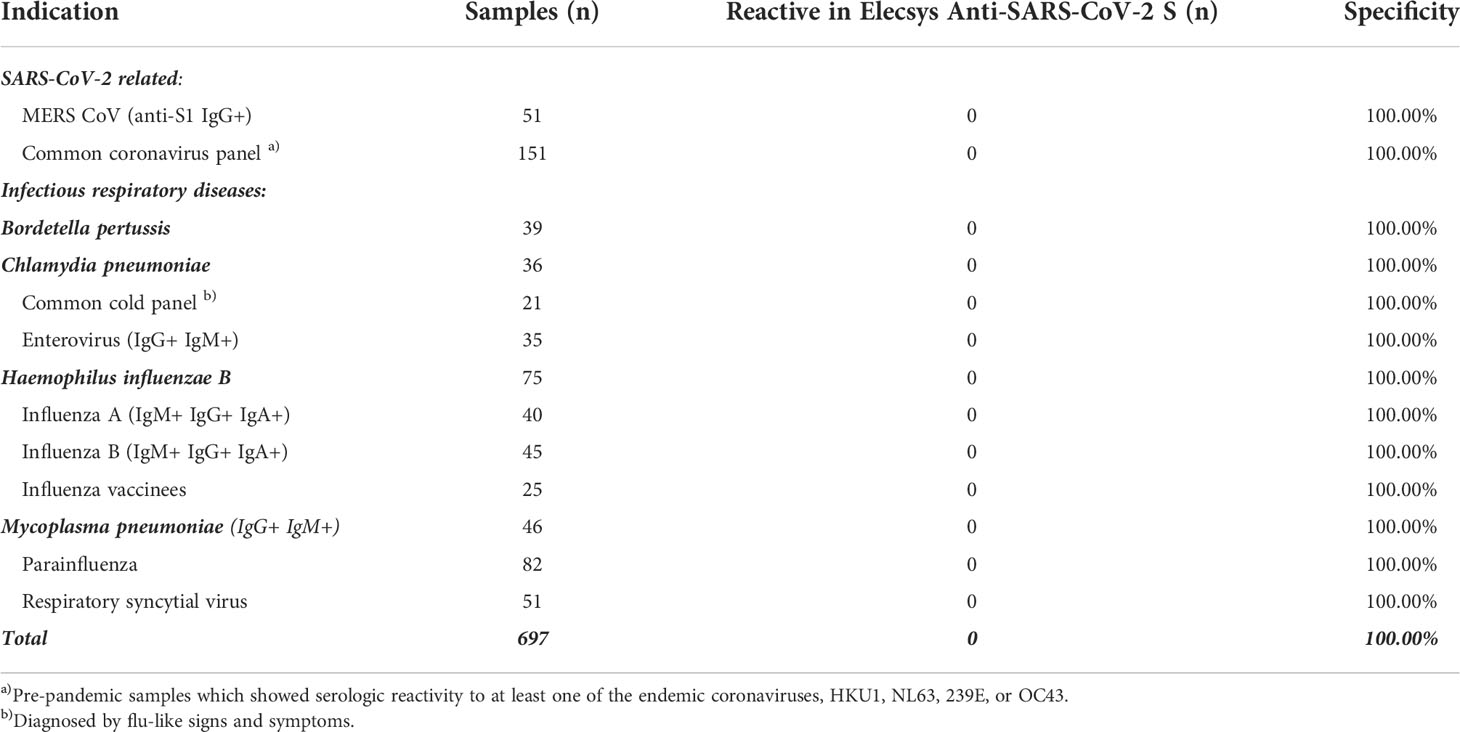
Table 2 Cross-reactivity of the ACOV2S assay in samples from participants with respiratory infections.
3.4 Sensitivity
Sensitive detection of seroconversion was observed for patients with a native infection with SARS-CoV-2, with most samples determined “reactive” by ACOV2S within 14 days of a positive PCR test. Sensitivity increased with the onset of the humoral immune response, with 98.8% of samples reported to be reactive >14 days after infection. By day 28, all samples for patients in this cohort were determined to be reactive (Figure 3).
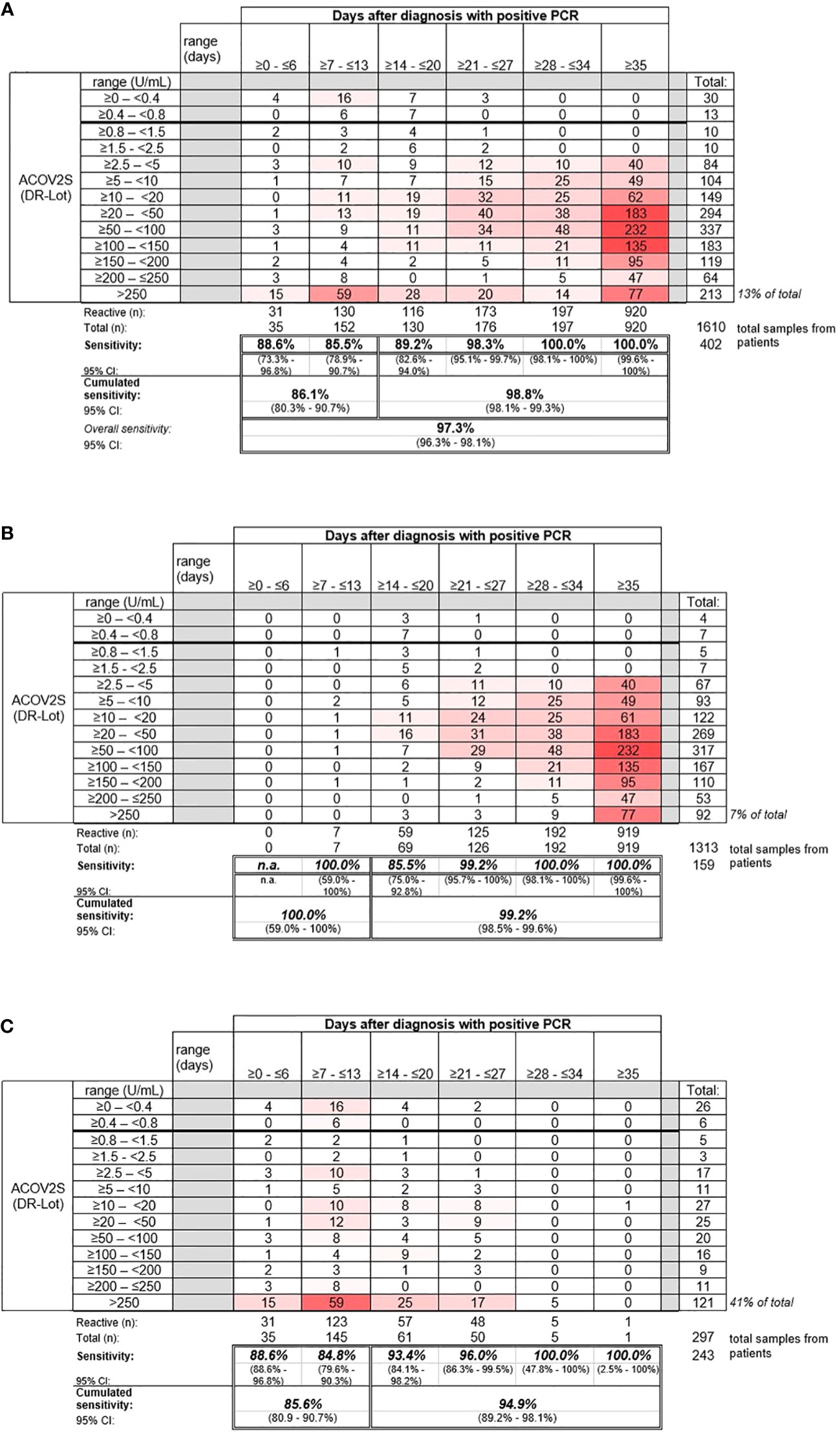
Figure 3 Sensitivity of the ACOV2S assay. (A) Sensitivity of the ACOV2S assay in samples from (A) all patients, (B) patients with mild symptoms of COVID-19, and (C) patients hospitalized due to COVID-19.
Samples from patients with mild COVID-19 exhibited average rising titers over time as indicated by the heat map (Figure 3B). In total, only 7% of all samples from patients with mild disease exceeded the primary measuring range (>250 U/mL). The patient with the latest seroconversion was part of the mild disease cohort. For patients with severe disease, i.e., hospitalized patients, higher titers were observed early after diagnostic PCR, with 41% of all samples exceeding the primary measuring range (most of them exceeded 250 U/mL within 14 days after diagnostic PCR; Figure 3C). Rapid development of relatively high titers was observed in case of severe course of disease, whereas moderate, still continuous antibody titer development seemed to be associated with mild disease.
A tendency for stable or even increasing anti-S titers over time following native infection was observed using the ACOV2S assay. This overall trend can be visualized in a smoothened median (50-day average) of all samples per timepoint (Figure 4A). Using the COV2G comparator method applying indirect antibody detection, the reverse effect was reported, i.e., antibody levels appear to be waning over time (Figure 4A).
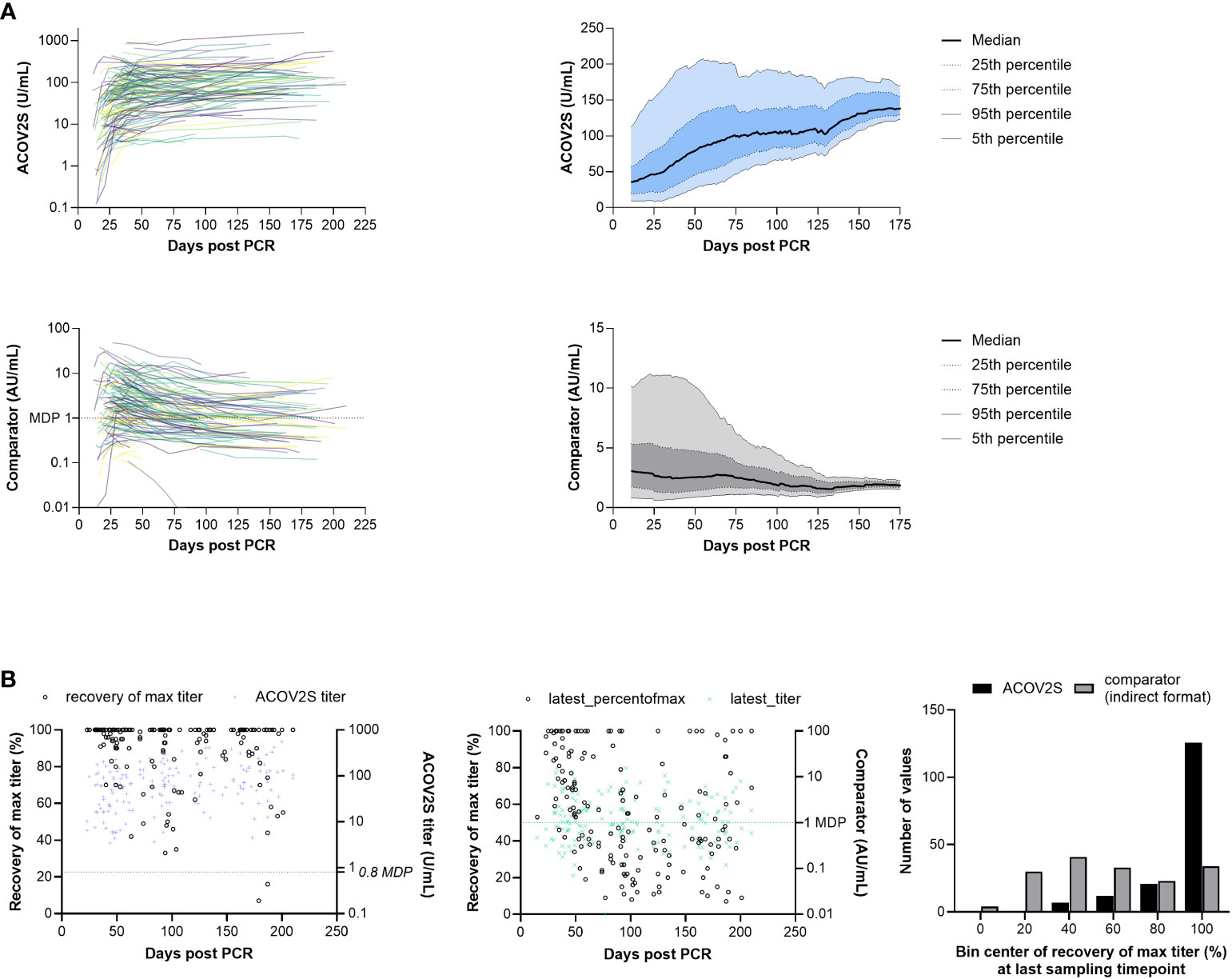
Figure 4 RBD titer dynamics. (A) Spaghetti and smoothened median curves for RBD titers over time for the ACOV2S and comparator assay, and (B) relative recovery at the last sampling timepoint for the ACOV2S and comparator assay (absolute titer values at the last sampling timepoint are plotted in color [right y-axis] and relative recovery is plotted in black [left y-axis]).
Dynamics of different methods can be difficult to compare, in particular when different antigens are used for detection (17). So absolute numeric values cannot be directly related. Also, variation in individual humoral immune responses complicates appropriate scaling. However, relative titer development over time can serve as a comparator. We therefore normalized the observed titers of a donor over time to their maximally observed titer. This procedure was independently applied to both methods (ACOV2S and COV2G). Figure 4B shows the plot of the normalized titer ratio (%, left y-axis) at the last sampling date of a donor. We also included the absolute titer for information (right y-axis). 100% recovery indicates maximal titer levels at the last time point, representing either still rising or no titer waning for this individual within the investigated period. The bar chart shows the cumulative frequency distribution of results. Using the ACOV2S assay, the majority of the patients exhibited 100% relative recovery at the last sampling timepoint, indicating sustained antibody titers. In contrast, relative recovery for the comparator assay was more variable and results of this method waned in a considerable proportion of the patients.
3.5 Correlation with neutralization assays
The correlation of ACOV2S with a surrogate neutralization assay (cPass™, Genscript) has already been described (18). Here, we investigated the correlation of the development of neutralizing potential over time as determined with cPass and antibody titer development as determined with ACOV2S using samples from patients with severe and mild course of disease from our sensitivity cohort. The samples included representative time points of longitudinal sample panels from individual donors with mild disease (n=22) to additionally resolve cPass and ACOV2S. In these longitudinal panels, ACOV2S titers and neutralization capacities developed with comparable dynamics (Figure 5A and S1). The correlation of the numeric value of inhibition as determined with cPass to the ACOV2S titer was very good in the individually resolved time buckets, despite cPass inhibition appearing less pronounced at later time points compared to ACOV2S antibody titers (Figure 5B). As in vitro determination of inhibition intentionally allows for saturation and hence does not reflect antibody affinity, but the ACOV2S method does, this might be a likely reason for the observed quantitative correlation dynamics. However, cPass is approved for qualitative interpretation only and the majority of ACOV2S reactive samples qualified “inhibitory” also over time. The exploratory application of 15 U/mL in ACOV2S as the decision point for correlation to inhibition was observed to lead to an improved PPV of the ACOV2S result on presence of inhibition in all investigated time buckets (Figure 5C). In general, all samples with an ACOV2S titer of ≥150 U/mL or higher seemed to saturate the inhibition potential in cPass at all addressed time buckets (Figure 5B).
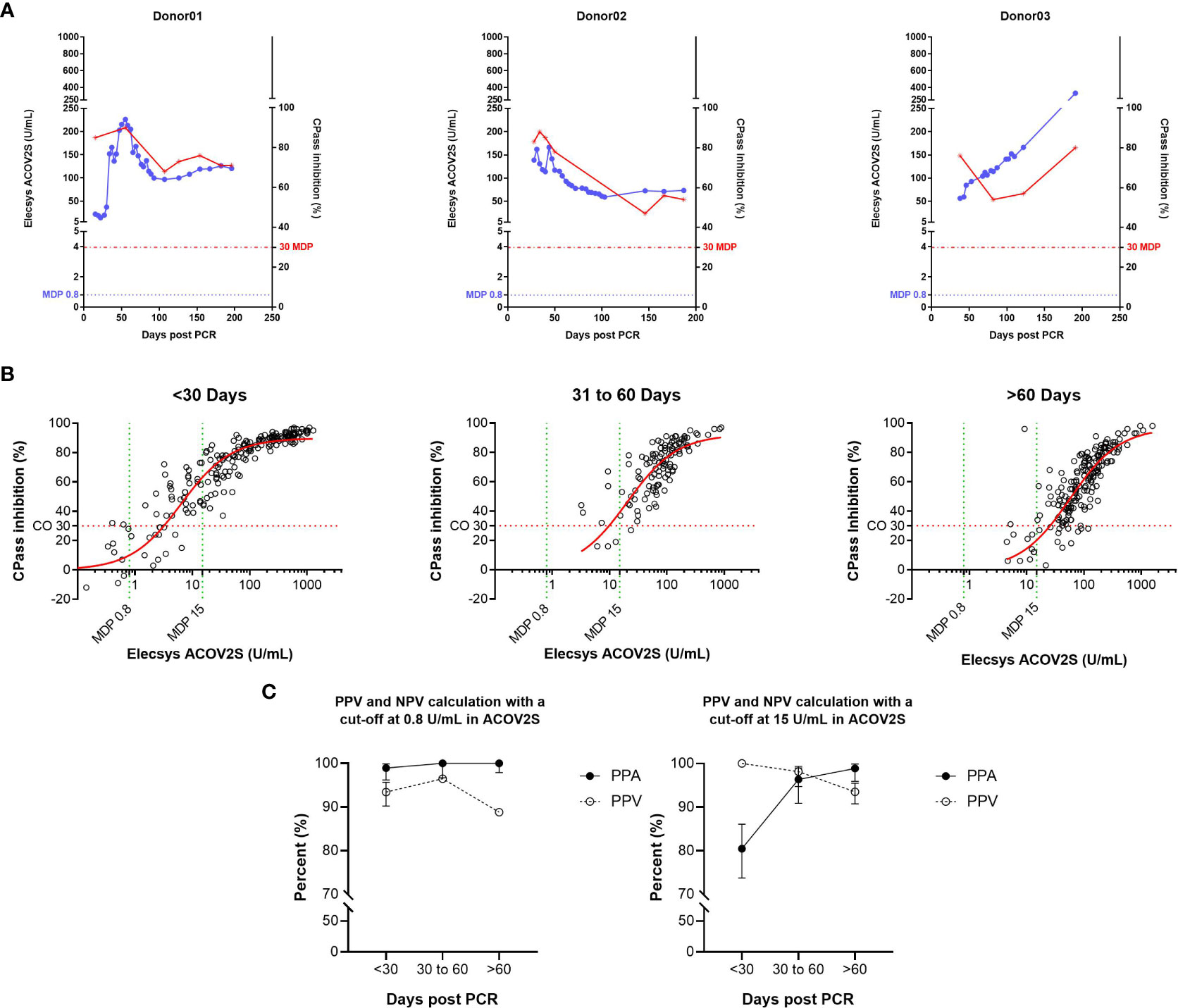
Figure 5 Correlation of the ACOV2S assay with results from the cPass neutralization assay. (A) ACOV2S titers and percentage inhibition from the cPass assay from longitudinal samples from exemplary selected individual donors, and (B) comparison of the ACOV2S and cPass results stratified in time buckets after diagnostic PCR and (C) derived qualitative agreements. ACOV2S reactivity in samples with cPass inhibitory capacities is shown as positive percent agreement (PPA), cPass inhibitory capacity ACOV2S reactive samples is shown as positive predictive value (PPV). Analyses were carried out applying both, 0.8 U/mL and 15 U/mL as decision point for relevant ACOV2S reactivity. This analysis used native samples collected from convalescent donors at different timepoints after infection.
We observed a good correlation of cPass results with the in-house Elecsys ACE2-RBD surrogate neutralization assay (Figure S2). A qualitative cutoff of 25% inhibition in the Elecsys ACE2-RBD method indicated the presence of neutralizing activity at a comparable level to the cPass method when applying the cPass cut off of 30%. A relative sensitivity of 93.4% was determined for the Elecsys ACE2-RBD assay and a ROC analysis resulted in an area of 0.9599 under the curve (Figure S2).
The internal competitive assay format correlated well with the titers determined with ACOV2S (Figure S3). Quantitative result correlation, as well as qualitative result interpretation, showed excellent comparability of the internal ACE-RBD method with ACOV2S.
Of note, the investigated sample cohort did not include a balanced amount of defined negative samples, but was conducted with samples from patients with confirmed SARS-CoV-2 infection. For this reason, a negative predictive value was not considered as the accuracy of the indicated negative agreement might be limited.
3.6 Precision and reproducibility
The ACOV2S assay demonstrated excellent precision (both within run and within lab) independently of the analyzer used, with the high throughput analyzer of the latest generation (cobas e 801) having slightly better precision than a benchtop analyzer of the previous generation (cobas e 411; Table 3). Reproducibility of the ACOV2S assay was investigated by assessment of differences in the result for aliquots of the same samples (n=5) measured on different days (n=5), on analyzers of different types (e411, e601, e801; n=3) and with different lots (n=3). In the worst case setting, only a marginal coefficient of variation was observed with 6.7% at LLOQ level and 3.2% at the high end of the primary measuring range (Table 4).
These data confirm that the applied standardization ensures excellent result recovery and lot comparability as well as independence of results from the type of analyzer used.
4. Discussion
ACOV2S is a highly sensitive and specific assay for the detection of antibodies to the SARS-CoV-2 S protein. The RBD was chosen as the antigen for this assay as it is highly immunogenic, part of all vaccines registered so far, and a prominent target for neutralizing antibodies due to its high functional relevance for the virus (5, 7). Moreover, the use of the RBD antigen resulted in higher specificity compared to using larger antigens and the well. Also, the well-defined and relatively small RBD supports linear dilution of the polyclonal and heterogeneous antibody mixture of a sample to the best extent.
The ACOV2S assay offers both excellent sensitivity and specificity for the detection of anti-RBD antibodies following a native SARS-CoV-2 infection, as shown here, as well as following vaccination with mRNA-1273 (Spikevax; Moderna, Cambridge, MA) (9, 19, 20). In this analysis, the high specificity of ACOV2S was confirmed in a large set of samples collected prior to the emergence of SARS-CoV-2. Strong specificity was critical in the beginning of the pandemic and low prevalence of disease and absence of vaccination. However, strong specificity is also advantageous in vaccinated populations, e.g., ruling out false-positive results and preventing non-convalescent or unvaccinated individuals from being put at risk of infection. The ACOV2S assay demonstrated no cross-reactivity to other respiratory or non-respiratory infections, including in samples from participants from Africa who are likely to have a different disease and immunological background compared to samples from Western participants. This is in contrast to reports in the literature, that identified possible false-positive SARS-CoV-2 serology (using non-ACOV2S assays) in pre-pandemic samples from malaria- or dengue fever-infected patients (21–23). With the growing proportion of infected and, most prominently, vaccinated individuals, the strong specificity of ACOV2S is a reliable tool to identify naïve, i.e., vulnerable, individuals.
Assays that measure antibodies against the RBD, such as ACOV2S, are by design expected to provide a strong positive predictive value for neutralization compared with assays that measure antibodies to the full S protein (expected to detect a large number of antibodies to other epitopes that are less likely to be neutralizing). In this and previous studies, the results from the ACOV2S assay have been shown to correlate well with in vitro and surrogate neutralization assays (19, 20, 24). Application of in vitro neutralization assays with high throughput is cost-and-labor intensive as laboratory sources providing this service are scarce. Also, considerable result variation is typically inevitable with assays involving live cell culture. Surrogate neutralization assays measuring interference with ACE-RBD interaction circumvent these disadvantages to a certain extent but apply a competitive assay format that typically comes with trade-offs in terms of dynamic range and robustness. The strong correlation of ACOV2S results with surrogate neutralization assays applied in this study renders the obtained ACOV2S results a suitable alternative to surrogate NT testing, with ACOV2S offering convincing performance, dynamics, and robustness.
No standard reference material existed for quantitative SARS-CoV-2 antibody assays at the time that the ACOV2S assay was initially developed. However, we have shown here that the units that were established for ACOV2S are interchangeable with the units of the First International WHO Standard for anti-SARS-CoV-2 immunoglobulins, signifying that data generated with ACOV2S can be assessed retrospectively without the need for restandardization.
Longitudinal monitoring of patients with mild course disease shows relatively stable or rising antibody titers over time when determined with ACOV2S and in contrast to the competitor method. These findings are in line with other reports on reduced waning of antibody titers with DAGS format assays (10, 25). Reinfection or consistent restimulation due to lack of viral clearance cannot be ruled out but would be detectable with an indirect method as well. However, DAGS format assays are able to reflect both and translate raising antibody concentration as well as raising antibody affinity into raising signals. This implies that low concentrations of high avidity antibodies can lead to the same result in the ACOV2S assay as high concentrations of low avidity antibodies; the biological function and the likelihood of detecting foreign target antigens in vivo are probably also comparable in both scenarios. In contrast, indirect formats do not differentiate if an antibody is bound with one or both paratopes to the antigen; either leads to the same signal. Antibody quality in terms of affinity is therefore more difficult to reflect in an indirect format assay.
Taking the results from both formats together, the rapid decline in SARS-CoV-2-specific antibody levels, as reported by indirect assays format, appears to be counteracted by rapid affinity maturation as reflected by ACOV2S and resulting stable or rising test results. Of note, the degree of antibody waning in indirect assay formats seems to exceed the loss of in vitro neutralization capacities and even the in vivo efficacy of immunity (26–28). Although it is not strictly necessary to distinguish between immunoglobulin classes during serology screening, field observations have demonstrated that individuals may have a robust and protective immune response to re-infection that is longer lasting than indirect antibody detection formats would suggest (29, 30). Whether the ACOV2S antibody titer shows a greater correlation with a reduced risk of reinfection remains to be investigated. Another open question is whether neutralization assays also reflect the important aspect of antibody affinity in addition to antibody concentrations. Also, the correlation of antibody mediated neutralization as measured in vitro to a reduced risk of infection or protection from severe disease is, to our knowledge, not yet reliably established.
In general, there is a risk that assay results waning below cut-off or even LOQ may suggest that individuals are immune naïve; this can hamper titer monitoring. Differentiation of naïve patients from patients with presumed critically low immunity becomes more challenging.
Following ACOV2S titer development in immunocompromised patients will enable insights into their general humoral immune response and its correlation with the risk of developing severe disease. And, at the same time, understand antibody maturation under immunosuppression based on the observed ACOV2S titer dynamics.
The results presented here emphasize that antibody results generated using different detection methods and/or different detection antigens should not be considered interchangeable. Despite attempts at harmonization by application of uniform reference material, the individual humoral immune response and its polyclonal nature together with individual differences in antibody maturation and selection are likely to lead to significantly different results using assays that utilize different methods of detection.
Absolute antibody titers and dynamics may be a marker of disease severity (19, 31). In this study, in patients with mild COVID-19, antibody titers increased gradually over time. In contrast, antibody titers increased more rapidly and to a higher level in patients who were hospitalized. Although data are currently limited, some evidence is beginning to emerge supporting the medical value of antibody testing in the acute management of patients infected with SARS-CoV-2 (32).
Our study utilized commercially available sample collections from volunteers and retrospective analysis of leftover samples. As all donors were anonymized, detailed clinical data were not available for this study; therefore we cannot determine the presence or extent of bias in the presented results.
Straightforward and well-established workflows combined with reliable detectability render antibody levels an appealing marker of immune response to viral infection or vaccination. It remains to be elucidated as to what extent they can be considered a direct protective effector or merely a surrogate marker for activation of the immune system. In both scenarios, the role of antibody dynamics should be further investigated to elaborate if certain titer limits correlate with a reduced risk of severe course of disease and which co-variates are to be taken into account, e.g., age, immune status, and putative markers. The SARS-CoV-2 pandemic, which resulted in population-wide immunization campaigns and detailed clinical characterization of the disease course, has provided a rich field to investigate possible correlations in detail and for robust statistics to be generated. Emerging new variants of the SARS-CoV-2 virus add an additional level of complexity to these attempts. However, obtaining reliable numeric results over time is key to additionally taking this layer into account using putative correction factors, adaptation of thresholds, and adjusting the expectable level of confidence of predictive values (although currently still theoretical). In this study, the ACOV2S assay demonstrated excellent precision and reproducibility, which are prerequisites for reliable titer monitoring over time. Virtually worldwide availability of the assay and analyzers renders ACOV2S a suitable candidate for population-wide assessment and monitoring of the humoral response elicited by the SARS-CoV-2 virus.
5. Conclusion
ACOV2S is a highly sensitive and specific assay for the detection of antibodies to the RBD of the SARS-CoV-2 S protein. In this analysis, we have demonstrated standardization and correlation to the first international WHO standard of anti-SARS-CoV-2 immunoglobulins and correlation with surrogate neutralization assays. An analysis of absolute antibody titers and dynamics suggested that the assay can have value in determining disease severity. Furthermore, the DAGS format allows for the detection of low concentrations of high avidity antibodies, meaning that the ACOV2S assay may retain sensitivity during the process of antibody maturation. The ability of ACOV2S to predict the risk of severe disease in vaccinated or convalescent patients remains unknown but is of great interest in the current phase of the pandemic.
Data availability statement
The study was conducted in accordance with applicable regulations. For more information on the study and data sharing, qualified researchers may contact the corresponding author, simon.jochum@roche.com.
Ethics statement
All samples were collected and tested in accordance with applicable regulations, including relevant European Union directives and regulations, and the principles of the Declaration of Helsinki. Anonymized frozen serum or plasma samples were used for this study and included residual samples from blood donation centers or routine laboratory diagnostics and commercially available samples. Approval was granted by the Ethics Committee of the Landesaerztekammer Bayern who confirmed that there are no objections to the transfer and coherent use of anonymized leftover samples.
Author contributions
Study concept/design: SJ, MW, PM, and KT. Data acquisition: KT, JK, MW, and PM. Data analysis and interpretation: KT, MW, PM, JK, SJ, and SP. Review and final approval of manuscript: SJ, MW, KT, and SP. All authors contributed to the article and approved the submitted version.
Acknowledgments
ELECSYS and COBAS are trademarks of Roche. All other product names and trademarks are the property of their respective owners. The Elecsys Anti-SARS-CoV-2 S assay is approved under Emergency Use Authorisation in the US. Editorial support was provided by Steph Carter and Jade Drummond of inScience Communications, Springer Healthcare Ltd, UK, and was funded by Roche Diagnostics International Ltd (Rotkreuz, Switzerland).
Conflict of interest
This study received funding from Roche Diagnostics. The funder was involved in the study design, analysis, interpretation of data, the writing of this article and the decision to submit it for publication. Editorial support was provided by Steph Carter and Jade Drummond of inScience Communications, Springer Healthcare Ltd, UK, and was funded by Roche Diagnostics International Ltd (Rotkreuz, Switzerland). ELECSYS and COBAS are trademarks of Roche. All other product names and trademarks are the property of their respective owners. All authors (KT, MW, PM, JK, SP, and SJ) are employees of Roche Diagnostics.
Publisher's note
All claims expressed in this article are solely those of the authors and do not necessarily represent those of their affiliated organizations, or those of the publisher, the editors and the reviewers. Any product that may be evaluated in this article, or claim that may be made by its manufacturer, is not guaranteed or endorsed by the publisher.
Supplementary material
The Supplementary Material for this article can be found online at: https://www.frontiersin.org/articles/10.3389/fimmu.2022.1002576/full#supplementary-material
References
1. Muench P, Jochum S, Wenderoth V, Ofenloch-Haehnle B, Hombach M, Strobl M, et al. Development and validation of the ELECSYS anti-SARS-CoV-2 immunoassay as a highly specific tool for determining past exposure to SARS-CoV. J Clin Microbiol (2020) 58(10):e01694–20. doi: 10.1128/jcm.01694-20
2. Antia R, Halloran ME. Transition to endemicity: Understanding COVID-19. Immunity (2021) 54(10):2172–6. doi: 10.1016/j.immuni.2021.09.019
3. Hamady A, Lee J, Loboda ZA. Waning antibody responses in COVID-19: What can we learn from the analysis of other coronaviruses? Infection (2022) 50(1):11–25. doi: 10.1007/s15010-021-01664-z
4. Huang AT, Garcia-Carreras B, Hitchings MDT, Yang B, Katzelnick LC, Rattigan SM, et al. A systematic review of antibody mediated immunity to coronaviruses: Kinetics, correlates of protection, and association with severity. Nat Commun (2020) 11(1):4704. doi: 10.1038/s41467-020-18450-4
5. Dai L, Gao GF. Viral targets for vaccines against covid-19. Nat Rev Immunol (2021) 21(2):73–82. doi: 10.1038/s41577-020-00480-0
6. Heinz FX, Stiasny K. Distinguishing features of current covid-19 vaccines: Knowns and unknowns of antigen presentation and modes of action. NPJ Vaccines (2021) 6(1):104. doi: 10.1038/s41541-021-00369-6
7. Almehdi AM, Khoder G, Alchakee AS, Alsayyid AT, Sarg NH, Soliman SSM. SARS-CoV-2 spike protein: Pathogenesis, vaccines, and potential therapies. Infection (2021) 49(5):855–76. doi: 10.1007/s15010-021-01677-8
8. Huang Y, Yang C, Xu XF, Xu W, Liu SW. Structural and functional properties of sars-Cov-2 spike protein: Potential antivirus drug development for covid-19. Acta Pharmacol Sin (2020) 41(9):1141–9. doi: 10.1038/s41401-020-0485-4
9. Riester E, Findeisen P, Hegel JK, Kabesch M, Ambrosch A, Rank CM, et al. Performance evaluation of the Roche elecsys anti-SARS-CoV-2 S immunoassay. J Virol Methods (2021) 297:114271. doi: 10.1016/j.jviromet.2021.114271
10. Theel ES, Slev P, Wheeler S, Couturier MR, Wong SJ, Kadkhoda K. The role of antibody testing for SARS-CoV-2: Is there one? J Clin Microbiol (2020) 58(8). doi: 10.1128/JCM.00797-20
11. Siemens Healthineers. Sars-Cov-2 igg (Scovg) (2022). Available at: https://www.fda.gov/media/146931/download.
12. GenScript. Cpass sars-Cov-2 neutralization antibody detection kit (2022). Available at: https://www.fda.gov/media/143583/download.
13. Sonntag O, Scholer A. Drug interference in clinical chemistry: Recommendation of drugs and their concentrations to be used in drug interference studies. Ann Clin Biochem (2001) 38(Pt 4):376–85. doi: 10.1258/0004563011900696
14. CLSI. Supplemental tables for interference testing in clinical chemistry. In: CLSI guideline EP37 Wayne, 1st Edition. PA: Clinical and Laboratory Standards Institute (2018).
15. Awad IE, Abu-Saleh AA-AA, Sharma S, Yadav A, Poirier RA. High-throughput virtual screening of drug databanks for potential inhibitors of sars-Cov-2 spike glycoprotein. J Biomol Struct Dynamics (2022) 40(5):2099–112. doi: 10.1080/07391102.2020.1835721
16. CLSI. Evaluation of precision of quantitative measurement procedures. In: Clinical and laboratory standards institute document EP05-A3, 3rd Edition (2014).
17. Ferrari D, Clementi N, Spanò SM, Albitar-Nehme S, Ranno S, Colombini A, et al. Harmonization of six quantitative sars-Cov-2 serological assays using sera of vaccinated subjects. Clin Chim Acta (2021) 522:144–51. doi: 10.1016/j.cca.2021.08.024
18. Kennedy NA, Lin S, Goodhand JR, Chanchlani N, Hamilton B, Bewshea C, et al. Infliximab is associated with attenuated immunogenicity to BNT162b2 and ChAdOx1 nCoV-19 sars-Cov-2 vaccines in patients with ibd. Gut (2021) 70(10):1884–93. doi: 10.1136/gutjnl-2021-324789
19. Jochum S, Kirste I, Hortsch S, Grunert VP, Legault H, Eichenlaub U, et al. Clinical utility of elecsys anti-Sars-Cov-2 s assay in covid-19 vaccination: An exploratory analysis of the mrna-1273 phase 1 trial. Front Immunol (2021) 12:798117. doi: 10.3389/fimmu.2021.798117
20. Rubio-Acero R, Castelletti N, Fingerle V, Olbrich L, Bakuli A, Wolfel R, et al. In search of the sars-Cov-2 protection correlate: Head-to-Head comparison of two quantitative S1 assays in pre-characterized oligo-/Asymptomatic patients. Infect Dis Ther (2022) 10(3):1505–18. doi: 10.1007/s40121-021-00475-x
21. Lustig Y, Keler S, Kolodny R, Ben-Tal N, Atias-Varon D, Shlush E, et al. Potential antigenic cross-reactivity between severe acute respiratory syndrome coronavirus 2 (Sars-Cov-2) and dengue viruses. Clin Infect Dis (2021) 73(7):e2444-e9. doi: 10.1093/cid/ciaa1207
22. Manning J, Zaidi I, Lon C, Rosas LA, Park JK, Ponce A, et al. Sars-Cov-2 cross-reactivity in prepandemic serum from rural malaria-infected persons, Cambodia. Emerg Infect Dis (2022) 28(2):440–4. doi: 10.3201/eid2802.211725
23. Steinhardt LC, Ige F, Iriemenam NC, Greby SM, Hamada Y, Uwandu M, et al. Cross-reactivity of two sars-Cov-2 serological assays in a setting where malaria is endemic. J Clin Microbiol (2021) 59(7):e0051421. doi: 10.1128/jcm.00514-21
24. Kennedy NA, Goodhand JR, Bewshea C, Nice R, Chee D, Lin S, et al. Anti-Sars-Cov-2 antibody responses are attenuated in patients with IBD treated with infliximab. Gut (2021) 70(5):865–75. doi: 10.1136/gutjnl-2021-324388
25. Chansaenroj J, Yorsaeng R, Puenpa J, Wanlapakorn N, Chirathaworn C, Sudhinaraset N, et al. Long-term persistence of severe acute respiratory syndrome coronavirus 2 (Sars-Cov-2) spike protein-specific and neutralizing antibodies in recovered covid-19 patients. PloS One (2022) 17(4):e0267102. doi: 10.1371/journal.pone.0267102
26. Levin EG, Lustig Y, Cohen C, Fluss R, Indenbaum V, Amit S, et al. Waning immune humoral response to BNT162b2 covid-19 vaccine over 6 months. N Engl J Med (2021) 385(24):e84. doi: 10.1056/NEJMoa2114583
27. Muecksch F, Wise H, Templeton K, Batchelor B, Squires M, McCance K, et al. Longitudinal variation in SARS-CoV-2 antibody levels and emergence of viral variants: A serological analysis. Lancet Microbe (2022) 3(7):e493–502. doi: 10.1016/S2666-5247(22)00090-8
28. Muecksch F, Wise H, Batchelor B, Squires M, Semple E, Richardson C, et al. Longitudinal serological analysis and neutralizing antibody levels in coronavirus disease 2019 convalescent patients. J Infect Dis (2021) 223(3):389–98. doi: 10.1093/infdis/jiaa659
29. Gussarow D, Bonifacius A, Cossmann A, Stankov MV, Mausberg P, Tischer-Zimmermann S, et al. Long-lasting immunity against sars-Cov-2: Dream or reality? Front Med (Lausanne) (2021) 8:770381. doi: 10.3389/fmed.2021.770381
30. Pilz S, Theiler-Schwetz V, Trummer C, Krause R, Ioannidis JPA. Sars-Cov-2 reinfections: Overview of efficacy and duration of natural and hybrid immunity. Environ Res (2022) 209:112911. doi: 10.1016/j.envres.2022.112911
31. Yang Y, Yang M, Peng Y, Liang Y, Wei J, Xing L, et al. Longitudinal analysis of antibody dynamics in covid-19 convalescents reveals neutralizing responses up to 16 months after infection. Nat Microbiol (2022) 7(3):423–33. doi: 10.1038/s41564-021-01051-2
Keywords: SARS-CoV-2, COVID-19, quantitative serology, neutralization, sensitivity, specificity
Citation: Taffertshofer K, Walter M, Mackeben P, Kraemer J, Potapov S and Jochum S (2022) Design and performance characteristics of the Elecsys anti-SARS-CoV-2 S assay. Front. Immunol. 13:1002576. doi: 10.3389/fimmu.2022.1002576
Received: 25 July 2022; Accepted: 10 October 2022;
Published: 02 December 2022.
Edited by:
Richard Kennedy, Mayo Clinic, United StatesReviewed by:
Chang Kyung Kang, Seoul National University Hospital, South KoreaAna Mayanga, Universidad Científica del Sur, Peru
Tao Chen, Huazhong University of Science and Technology, China
Copyright © 2022 Taffertshofer, Walter, Mackeben, Kraemer, Potapov and Jochum. This is an open-access article distributed under the terms of the Creative Commons Attribution License (CC BY). The use, distribution or reproduction in other forums is permitted, provided the original author(s) and the copyright owner(s) are credited and that the original publication in this journal is cited, in accordance with accepted academic practice. No use, distribution or reproduction is permitted which does not comply with these terms.
*Correspondence: Simon Jochum, simon.jochum@roche.com