- Shanghai Engineering Research Center of Food Microbiology, School of Health Science and Engineering, University of Shanghai for Science and Technology, Shanghai, China
The resistance of Lactobacillus plantarum to vancomycin depends on its peptidoglycan composition. Vancomycin has poor binding affinity with peptidoglycan precursors ending in D-alanyl-D-lactate (D-Ala-D-Lac) but binds strongly to peptidoglycan precursors ending in D-alanyl-D-alanine (D-Ala-D-Ala), resulting in resistance and sensitivity, respectively. The ligase Ddl, which generates D-Ala-D-Lac or D-Ala-D-Ala incorporated into the peptidoglycan precursor chain, is responsible for this specificity. To study the effect of peptidoglycan precursors on immunity, we constructed several strains of L. plantarum expressing the ddl gene of Lactococcus lactis to change their peptidoglycan precursors. The change in the termini of the peptidoglycan precursors was determined by the sensitivity of the strains to vancomycin. The overexpression of ddl increased the susceptibility of the strains to vancomycin. We further explored the regulation of the macrophage inflammatory response pathway by the wild-type and constructed strains, and found that these strains induced the MyD88-dependent TRAF6/MAPK pathway, and the increase in D-Ala L. plantarum peptidoglycan precursors increased the secretion of the inflammatory factors IL-6, IL-1β and TNF-α. These results indicate that D-Ala-ended peptidoglycan precursors play a central role in the variable immunomodulatory ability of L. plantarum.
Introduction
The cytoplasmic membrane in gram-positive bacteria is covered by a thick cell wall consisting of multiple layers of peptidoglycan, a polysaccharide composed of two alternating glucose derivatives, N-acetylglucosamine (NAG) and N-acetylmuramic acid (NAM). The chains are cross-linked to one another by a tetrapeptide that extends from the NAM sugar unit, allowing a lattice-like structure to form. The four amino acids that compose the tetrapeptide are L-alanine, D-glutamine, L-lysine or meso-diaminopimelic acid (DPA), and D-alanine (D-Ala) (1).
In peptidoglycan synthesis, the precursor pentapeptide Lipid II is generated with a terminal D-alanyl-D-alanine (D-Ala-D-Ala) or D-alanyl-D-lactate (D-Ala-D-Lac). This is the starting unit from which bacteria extend the sugar chain and crosslink the peptide chain outside the cell membrane to form a mature peptidoglycan. Under the action of transglycosylase, the Lipid II is linked to the sugar chain outside the cell membrane to make it extend horizontally. After the action of transpeptidase, peptide bonds are formed between the peptide tails of adjacent peptidoglycan monomers and terminal amino acid (D-alanine or D-lactate) is released, which leads to longitudinal cross-linking. Vancomycin combines with D-Ala-D-Ala-ended peptidoglycan precursors on the bacterial cell wall to form a stable non-covalent complex, thereby inhibiting the extension or/and cross-linking of peptidoglycan to repress cell wall synthesis of and eventually cause bacterial cell death (2, 3). Lactobacillus plantarum produces peptidoglycan precursors ending in D-lactate (D-Lac) instead of D-Ala, leading to intrinsic resistance to vancomycin. L. plantarum expresses the D-Ala-D-Lac ligase (DdlLp), which plays a central role in this specificity by synthesizing D-Ala-D-Lac depsipeptides that are added to the precursor peptide chain (4), and D-Lac dehydrogenase for D-Lac production, which affects peptidoglycan biosynthesis (5). In contrast, Lactobacillus lactis exclusively produces D-Ala-ended peptidoglycan precursors through D-Ala-D-Ala ligase (DdlLc) action, resulting in natural sensitivity to high levels of vancomycin (6).
L. plantarum can produce D-Lac or L-lactate (L-Lac) through the activity of D-Lac or L-Lac dehydrogenase, respectively (7). A mutant strain of L. plantarum lacking both D- and L-Lac dehydrogenase activities was reported to produce only trace amounts of D- and L-Lac, thereby seriously affecting the peptidoglycan synthesis pathway (2). Although the wild-type precursor was still present, the mutant strain substantially synthesized novel D-Ala-ended precursors and showed a highly enhanced sensitivity to vancomycin (2). D-Ala on the teichoic acid (TA) branch chain caused corresponding immunological responses and triggered the immune response pathway. Purification of lipoteichoic acid (LTA) from Staphylococcus aureus caused the loss of alanine substituents and weaker cytokine induction activity than that observed with unpurified LTA. The hydrolysis of active LTA alanine substituents also greatly reduced the induction of cytokines (8). In in-vivo experiments, the L. plantarum mutant was found to incorporate much less D-Ala in TAs than the wild-type strain. This deficiency significantly impacted bacteria-induced immunomodulatory reactions, with a significant decrease in proinflammatory cytokines secreted by peripheral blood mononuclear cells and monocytes stimulated by the mutant strain compared with the parental strain (9).
Toll-like receptors (TLRs) are the main pattern recognition receptors on the host cells that recognize microorganism, inducing a broad spectrum of extracellular and intracellular signaling pathways. Nuclear factors-kappa β (NF-κβ) is a downstream hub of TLR signaling pathways (10), and is present in almost all cells. When a cell is stimulated, pathogen-associated molecular patterns, in combination with TLRs, send a signal to intracellular receptor. The Toll/interleukin (IL)-1 receptors (TIR) region of TLRs binds to the carboxyl terminal of MyD88, which then recruits interleukin-1 receptor associated kinases (IRAK) and induces phosphorylation of IRAK1. IRAK1 interacts with TNF6 to activate the IKK complex and MAPK. The IKK complex phosphorylates IκB kinase, which is then ubiquitinated and degraded by ubiquitin ligase (11). NF-κβ, which binds to IκB, is activated, and enters the nucleus to regulate the transcriptional expression of target genes (12), and then leads to inflammation, immunity, and various pathological reactions (13). INOS is a key enzyme in NO synthesis and induces NO production. The high expression of iNOS can produce excessive NO and reactive nitrogen and cause cell inflammatory damage.
Probiotics improving immunity (14, 15), enrich beneficial intestinal bacteria (16, 17) and inhibit the production of inflammatory factors related to intestinal diseases (18, 19). Certain probiotics can regulate the TLR NF-κβ signaling pathway and the expression of inflammatory factors, improving intestinal mucosal inflammation (20). Peptidoglycan, one of the most abundant microbe-associated molecular patterns (MAMPs) in lactic acid bacteria (LAB), activates the host’s antigen-presenting cells by binding to TLRs. This binding induces cell surface receptors expression to regulate cell functions, while stimulating the host cells to secrete cytokines and chemokines to regulate the immune response (21).
Peptidoglycan, wall TA and LTA have immunomodulatory effects, and a change in the D-Ala content of L. plantarum LTA was shown to significantly affect the immunomodulatory responses induced by the bacterium (22). It is not yet known whether D-Ala derived from peptidoglycans has a similar immunomodulatory effect. In this work, we overexpressed the gene encoding L. lactis dipeptide ligase (ddl) into L. plantarum AR113 and Lac dehydrogenase double knockout (AR113△ldhL△ldhD) strains. To validate changes in the peptidoglycan precursor termini, we investigated the sensitivity of these strains to high levels of vancomycin. The constructed strains were evaluated in-vitro to explore the effects of D-Ala-ended peptidoglycan precursors on immune reaction. This study helps to identify the role of D-Ala in the immunomodulator ability of L. plantarum, and provides a theoretical basis for the screening and application of probiotics.
Materials and Methods
Bacterial Strains, Media, and Culture Conditions
L. plantarum AR113, L. plantarum AR113△ldhL△ldhD and L. lactis NZ9000 were obtained from Shanghai Engineering Research Center of Food Microbiology, University of Shanghai for Science and Technology (Shanghai, China). L. plantarum AR113 and L. plantarum AR113△ldhL△ldhD were streaked on separate de Man, Rogosa and Sharpe (MRS, Oxoid Ltd, Basingstoke, UK) agar plates, and incubated in an anaerobic workstation at 37°C for 24 h before experimental use. L. plantarum were grown at 37°C in MRS broth in the presence of 50 mM L-lactate (98%, Shanghai yuanye Bio-Technology Co., Ltd) or D-lactate (90%, Shanghai yuanye Bio-Technology Co., Ltd) when minimum inhibitory concentration (MIC) was measured. L. lactis NZ9000 was grown in M17 medium (Difco Laboratories, MI, U.S.A) supplemented with 0.5% glucose (GM17) at 30°C for 36 h before experimental use. E. coli top10 was used as a host for molecular cloning and was grown at 37°C in Luris-Bertani broth (LB, Gibco, CA, USA).
DNA Manipulation and Cloning Procedure
Ddl was amplified by PCR using L. lactis NZ9000 chromosomal DNA as a template with the primers 5’- atatgaatgacaatga tgttggatccatgtcaaaagaa acT -3’ and 5’- cacgggaaaatcatctcttataactc gagatctatcgata agcttaag -3’. The PCR product was purified for sequencing by the BGI Company (Shenzhen, China). The correct sequence was cleaved by the restriction endonucleases BamHI and Xho I and subcloned into the pIB184 plasmid predigested at the same restriction sites. The resulting recombinant plasmid was named as pIB184-DdlLc.
Transformation by Electroporation
L. plantarum competent cells were prepared as previously described (23). Electro transformation of L. plantarum was performed as follows. Briefly, a 3-5% (v/v) inoculum from an overnight culture was inoculated into 50 mL of SGMRS (50 mL MRS with 0.3 M sucrose and 1% glycine), and incubated at 37°C until the mid-exponential phase was achieved (OD600 of 0.3-0.5). The cells were recovered by centrifugation at 4500 rpm and 4°C for 10 min. The cells were washed twice with SM buffer (952 mM sucrose, 5 mM MgCl2), and resuspended in 800 μL of SM buffer. The competent cells were aliquoted and stored at −80°C. Subsequently, 100 μL of the cell suspension and a maximum of 1μL of the plasmid DNA solution were electroporated using an Eporator electroporator (Bio-Rad, UK) in cuvettes with a 2-mm electroporation gap at 2.5 kV, capacitance of 4 ms, and parallel resistance of 400 Ω.
After electroporation, the cells were immediately resuspended in 800 μL of SGMRS and kept at 37°C for 3 h for recovery. Then, 100 μL of the cells were spread on MRS agar plates containing 200 μg/mL erythromycin and incubated at 37°C for 36-48 h. Transformants grown on resistant plates were further screened by plasmid isolation and restriction enzyme analysis.
Minimum Inhibitory Concentration of Vancomycin
The bacteria were streaked on separate MRS agar plates containing 200 μg/mL erythromycin (Sangon Biotech, Shanghai, China), and the plates were incubated in an anaerobic workstation at 37°C. After 2 days of culture, single colonies of bacteria were separately activated for two generations in MRS liquid medium containing 200 μg/mL erythromycin at 37°C for 16 h. Subsequently, a 3-5% (v/v) inoculum from an overnight culture was inoculated into 10 mL MRS liquid medium containing 0, 0.5, 2, 16, and 256 μg/mL of vancomycin.
Cell Culture Experiments
RAW264.7 mouse macrophages were activated and maintained using Dulbecco’s modified Eagle’s medium (DMEM, Gibco™, Thermo Fisher Scientific, Grand Island, USA) containing 10% fetal bovine serum, 100 U/mL penicillin, and 100 μg/mL streptomycin at 37°C under a humidified atmosphere of 5% CO2.
RNA Extraction and RT-PCR Gene Expression Analysis
RT-PCR was performed using a LightCycler®96 instrument (Roche China). cDNA was synthesized using a HiScript ®III RT SuperMix for qPCR (+gDNA wiper) from 500 ng of total RNA extracted from RAW264.7 cells. Then, qPCR was performed using a UNICON™qPCR SYBR®Green Master Mix (Yeasen Biotechnology, Shanghai, China) and β-actin was used as an internal reference gene. The procedure of quantitative PCR was as follows: 95°C for 30 s, 95°C for 5 s, 40 cycles, at optimum annealing temperature for 30 s. The results were analyzed by the method of 2-ΔΔCt. The primer sequences are shown in Table 1.
Statistical Analysis
The gene sequencing result were analyzed using Vector NTI (Thermo Fisher Scientific, Waltham, MA, U.S.A). GraphPad Prism 8 (GraphPad Software, San Diego, CA, USA) was used for the figures, and SPSS (IBM Corp., Armonk, NY, U.S.A) software was used for statistical analysis. Shapiro–Wilk test was used to verify the normality of data and Levene’s test was used to assess equal variance of data. Statistical comparison of two groups was performed using Student’s t-test, or Wilcoxon–Mann–Whitney test (when normality test fails). Multiple groups were compared with one-way ANOVA. The statistical analyses were assessed using one-way ANOVA. Data are presented as the means with standard deviations and differ significantly as presented with different letters at P < 0.05.
Results
Construction of Recombinant Strains and Sensitivity to Vancomycin
L. plantarum AR113△ldhL△ldhD is a mutant of L. plantarum AR113 in which the ldhL and ldhD genes are knocked out. These key genes regulate the production of L-Lac dehydrogenase and D-Lac dehydrogenase, which catalyze pyruvate to produce L-Lac and D-Lac respectively. The correct ddl fragment was digested by BamHI and XhoI, and ligated into a pIB184 vector, containing the constitutive promoter P23 from LAB to produce the recombinant plasmid pIB184-DdlLc. The recombinant plasmid was verified by PCR and double digestion. pIB184-DdlLc was transformed into L. plantarum AR113 and L. plantarum AR113△ldhL△ldhD competent cells by electroporation to yield the strains AR113-DdlLc and AR113△ldhL△ldhD-DdlLc (Figure 1). The ldhL and ldhD knockout strain we constructed barely produced lactic acid and consequently provided little D-Lac for peptidoglycan precursor production. Therefore, exogenous D-LAC was added to remedy this defect. We tested the sensitivity of the wild-type strain and Ddl overexpressing strains to vancomycin to observe any effects related to the change in peptidoglycan precursor termini. L. plantarum AR113△ldhL△ldhD showed high sensitivity to vancomycin, which was mitigated by supplementation with exogenous D-Lac. Similar results were observed for L. plantarum AR113△ldhL△ldhD-DdlLc. These results indicated that L. plantarum AR113△ldhL△ldhD and AR113△ldhL△ldhD-DdlLc produced peptidoglycan precursors ending in D-Lac, like those produced by the wild-type AR113 strain, after supplementation with exogenous D-Lac, thus reducing sensitivity to vancomycin (Table 2).
Analysis of the Immunomodulatory Signaling Pathway Induced by Ddl-Overexpressing Strains
Excessive secretion of inducible nitric oxide synthase (iNOS) can induce the production of large amounts of nitric oxide (NO), resulting in inflammatory cell damage. Compared with the wild-type L. plantarum AR113 strain, the three strains engineered to overexpress Ddl led to greater increases in the expression of iNOS mRNA. The increase in D-Ala-ended peptidoglycan on the bacterial cell wall increased the expression of the gene encoding iNOS (Figure 2).
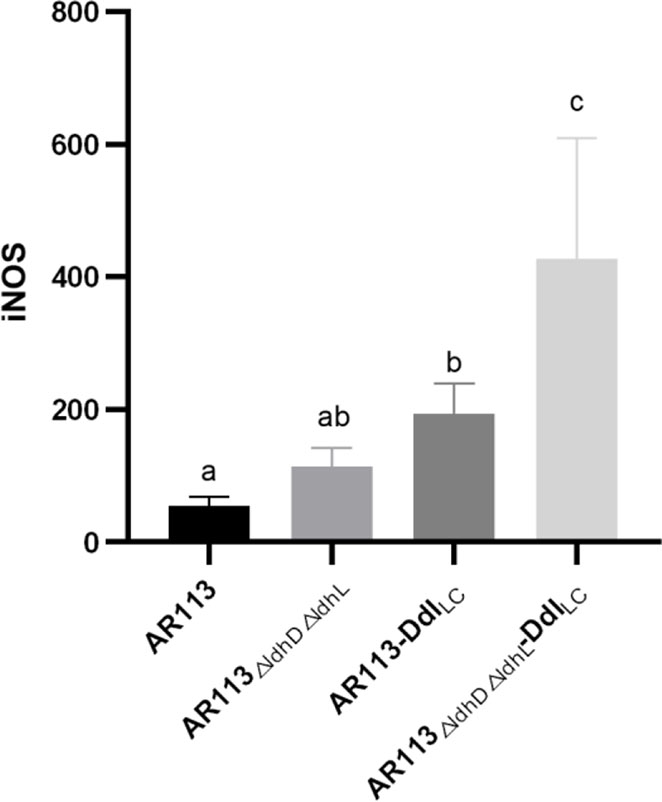
Figure 2 Effect of Ddl-overexpressing L. plantarum strains on iNOS production in macrophages. The statistical analyses were assessed using one-way ANOVA. Data are represented as means ± standard deviations (n = 7). Data indicated with different letters differ significantly (P < 0.05).
L. plantarum cell wall peptidoglycan has an immune stimulatory effect and thus can bind to TLR2 and other receptors on macrophages, activate cell signal transduction, and induce downstream related to immune. Using PCR, the ability of D-Ala to induce the TLR2, TLR4 and MAPK signal transduction pathways was verified, whereas activity through the TRIF and Iκκ pathways was not verified (Figure 3A). This indicated that the strains bound to TLR2 and TLR4 on the surfaces of macrophages and entered the cells, and then triggered MyD88-dependent pathways. The ability of L. plantarum AR113, AR113△ldhL△ldhD and Ddl overexpressing strains binding to TLR2 and TLR4 was not significantly different (Figures 3B–D).
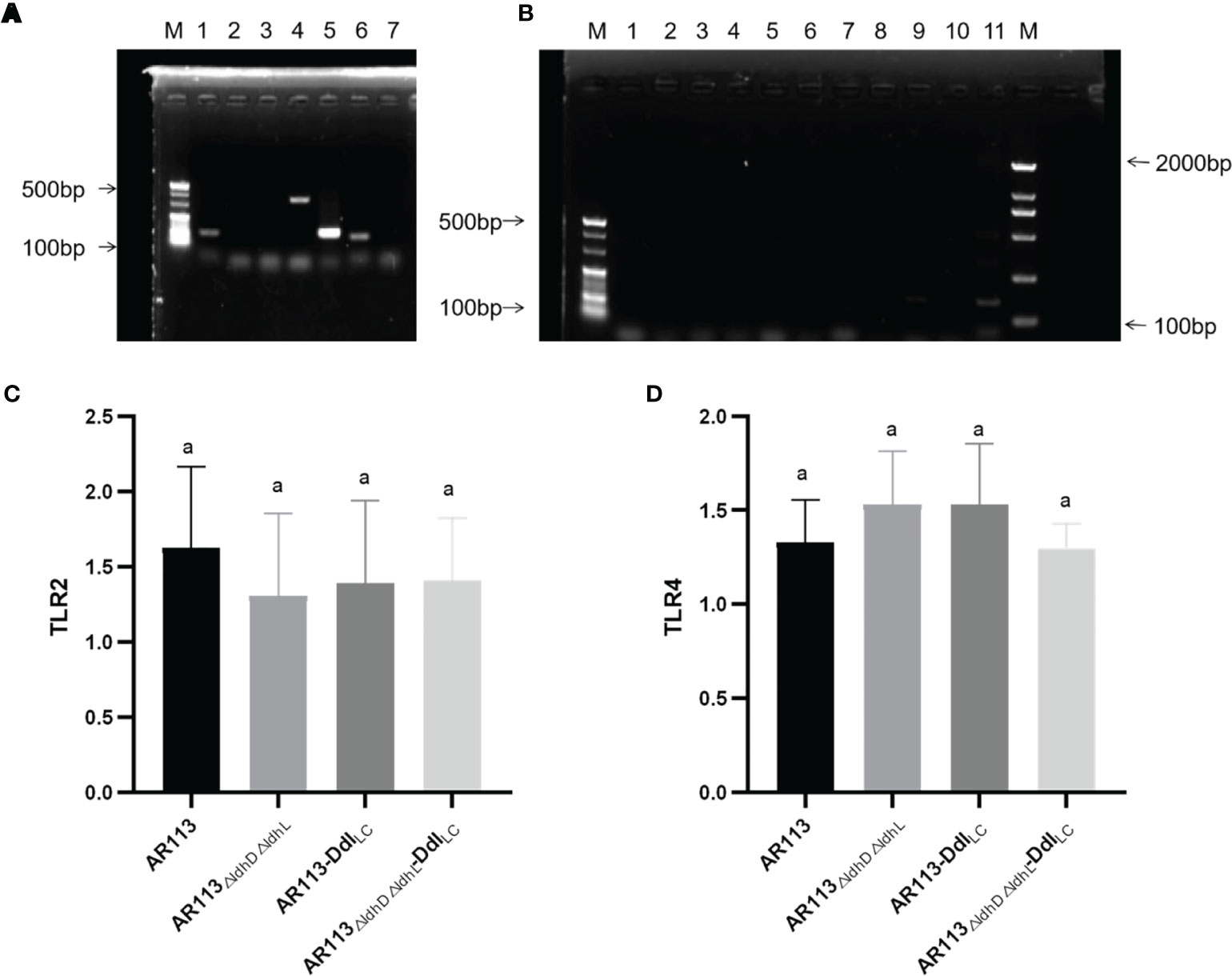
Figure 3 Analysis of immunomodulatory signaling pathways affected by Ddl-overexpressing strains. (A) lane 1: p38, lane2: JNK, lane 3: IRAK1, lane 4: p38MAPK, lane 5: TRAF6, lane6: ERK; (B) lane 1: TLR1, lane 2: TLR3, lane 3: TLR5, lane 4: TLR6, lane 5: TLR7, lane 6: TLR8, lane 7: TLR9, lane 8: Iκκ-β, lane 9: Iκκ-γ, lane 10: TRIF, lane 11: NF-κβP56; (C) RT-PCR of TLR2; (D) RT-PCR of TLR4. The statistical analyses were assessed using one-way ANOVA. Data are represented as means ± standard deviations (n = 7). Data indicated with different letters differ significantly (P < 0.05).
The wild-type and Ddl overexpressing strains were co-cultured with macrophages, and the expression of transcription factors was measured in the latter cells. MyD88 plays a crucial role in signal transduction pathways involving IL-1 and TLRs. RT-PCR analysis showed that L. plantarum AR113△ldhL△ldhD-DdlLc intensely induced MyD88 mRNA expression, whereas L. plantarum AR113 and AR113-DdlLc induced weaker MyD88 mRNA expression. Ddl-overexpressing strains increased the expression of MyD88 mRNA in macrophages downstream of the TLR2 pathway (P < 0.05). Compared with L. plantarum AR113, AR113ΔldhL△ldhD did not significantly increase the expression of MyD88 mRNA (Figure 4A). TRAF6 plays a key role in innate and adaptive immune responses. The expression of TRAF6 mRNA was stimulated by Ddl-overexpressing AR113 strains (P < 0.05), and the strain lacking ldhL and ldhD induced activation of TRAF6 to a greater degree than MyD88 (Figure 4B). The activation of ERK, p38, and JNK in MAPK pathways were observed. All four L. plantarum strains induced comparable levels of p38, ERK, and JNK phosphorylation, suggesting that these strains can induce MyD88-dependent TRAF6/MAPK pathway signal transductions (Figures 4C–E).
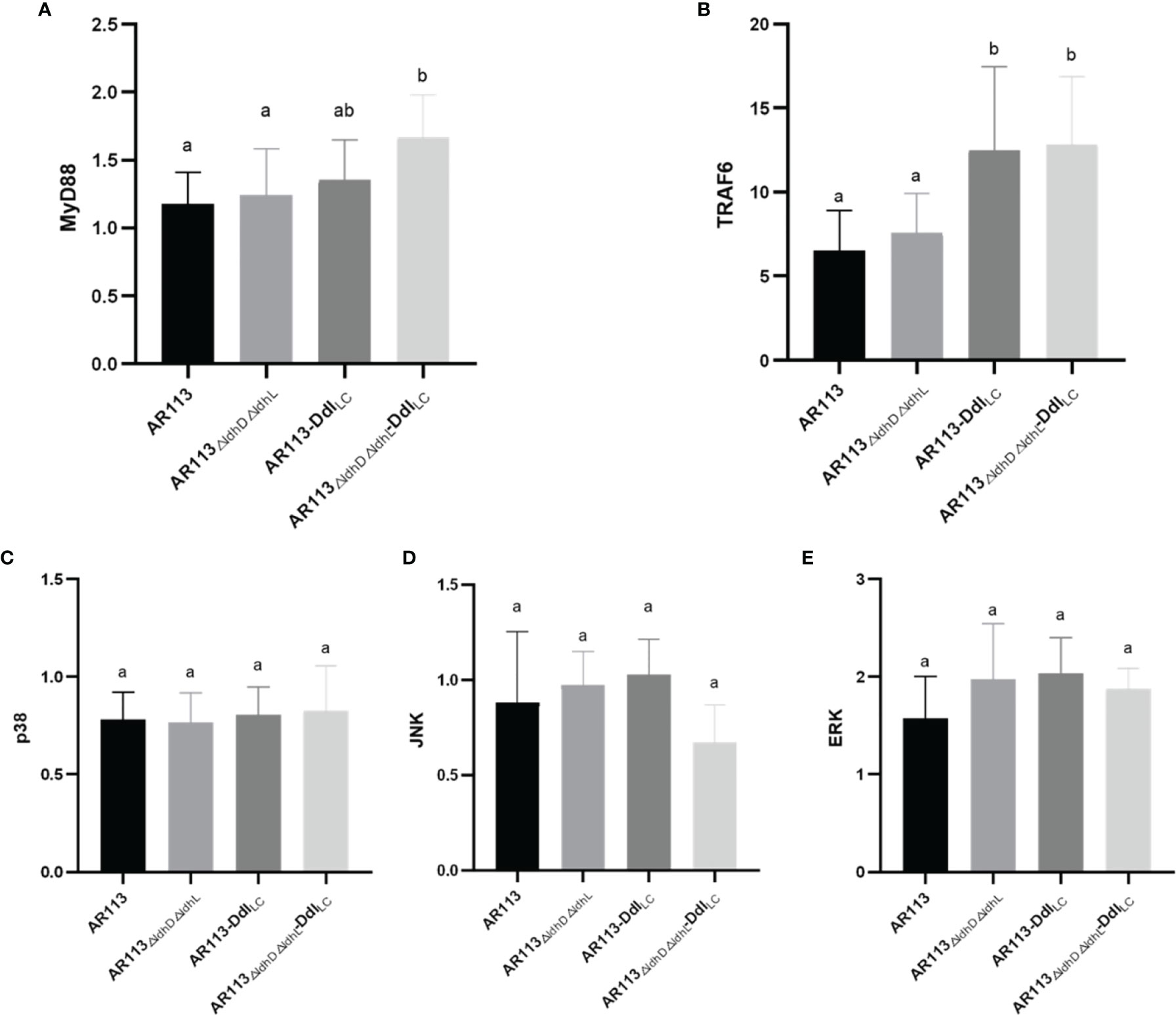
Figure 4 Analysis of the immunomodulatory signaling pathway affected by Ddl-overexpressed strains. (A) MyD88; (B) TRAF6; (C) p38; (D) JNK; (E) ERK. The statistical analyses were assessed using one-way ANOVA. Data are represented as means ± standard deviations (n = 7). Data indicated with different letters differ significantly (P < 0.05).
Effects of D-Ala-ended L. plantarum Peptidoglycan Precursors on Cytokine Production
To investigate the functional role of D-Ala at the termini of the of L. plantarum peptidoglycan precursors in the production of cytokine and expression of other factors, RAW264.7 macrophages were stimulated with L. plantarum AR113, AR113-DdlLc, AR113△ldhL△ldhD, and AR113△ldhL△ldhD-DdlLc. As shown in Figure 5, all four strains induced the expression of mRNAs encoding the cytokines IL-6, IL-1β, COX-2, IL-18 R, TGF-β, TNF-α, although relatively lower levels of gene expression were induced by the AR113 and AR113△ldhL△ldhD strains. These results indicate that D-Ala can induce the expression of genes encoding cytokines IL-6, IL-1β, COX-2 (Figure 5).
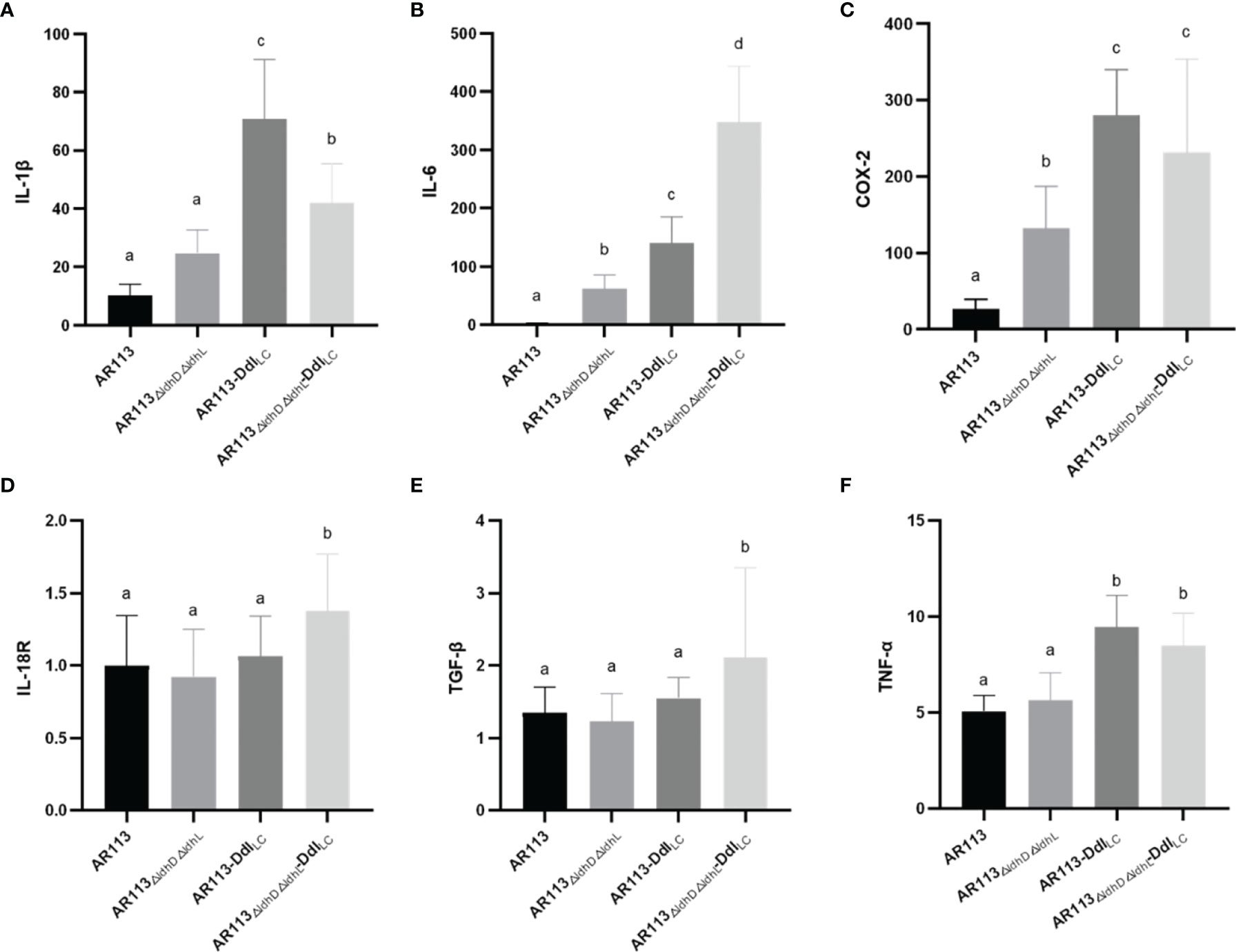
Figure 5 Effects of D-Ala-ended peptidoglycan precursors on the expression of cytokine/inflammatory factor genes (A) IL-1β, (B) IL-6, (C) COX-2, (D) IL-18R, (E) TGF-β and (F) TNF-α in macrophages. The statistical analyses were assessed using one-way ANOVA. Data are represented as means ± standard deviations (n = 7). Data indicated with different letters differ significantly (P < 0.05).
Discussion
Ddl synthesizes D-Alanyl-D-lactate or D-alanyl-D-alanine, which are added to the precursor peptide chain by the enzyme MurF and contribute to the sensitivity of L. lactis to vancomycin. An active site phenylalanine or tyrosine in the Ddl determines depsipeptide or dipeptide activity, which results in resistance and sensitivity to vancomycin, respectively. Ddl from L. plantarum encodes a depsipeptide ligase which synthesizing D-Ala-D-Lac depsipeptides, while L. lactis expresses the D-Ala-D-Ala ligase (DdlLp). In addition, the resistance of these strains to vancomycin was related to the amount of D-Ala at the termini of the peptidoglycan precursors. The loss of D-Lac dehydrogenase, the key enzyme in the formation of lactate from pyruvate, reduced D-Lac production and affected the sensitivity of the strain to vancomycin. Our study found DdlLc was overexpressed in L. plantarum to induce D-Ala-D-Ala dipeptide production and render the strain sensitive to vancomycin. When the strain was provided with exogenous D-Lac, the results were reversed, indicating that DdlLp promoted the production of large amounts of D-Ala-D-Lac for the synthesis of D-Lac-ended peptidoglycan precursors. Although D-Lac is also produced by the racemization of L-Lac in L. plantarum (24), the tested strains still showed vancomycin sensitivity when supplemented with exogenous L-Lac. This shows that only a certain amount of D-lac can change the sensitive to vancomycin. This also indicates that we have indeed changed the structure of the peptidoglycan precursor by knocking out the gene of Lac dehydrogenase or overexpressing the gene of DdlLc.
Large molecular substances in the L. plantarum cell wall and cell membrane surface induce a series of immune responses by binding to TLRs on the surfaces of macrophages and entering the cells, where they can trigger both MYD88-dependent and independent pathways. In our study, we co-cultured L. plantarum AR113, AR113-DdlLc, AR113△ldhL△ldhD, AR113△ldhL△ldhD-DdlLc with RAW264.7 macrophage. Endogenous NO, a small molecule with a wide range of complex biological activities, is involved in a variety of pathophysiological processes and exerts certain cytotoxic side effects at high concentrations. iNOS is a key enzyme in the synthesis of NO (25), and thus associated with inflammatory cell damage (26). L. plantarum AR113△ldhL△ldhD, which theoretically produced the highest level of D-Ala-ended peptidoglycan precursors among the tested strains, significantly promoted the expression of iNOS mRNA, which was measured at levels more than four times higher than those induced by the wild-type L. plantarum AR113 strain. We further confirm the expression of pathway-related factors in the macrophages by PCR. Only TLR2 and TLR4 had DNA bands. This result indicated that these four strains (AR113, AR113-DdlLc, AR113△ldhL△ldhD and AR113△ldhL△ldhD-DdlLc) induced TLR2 and TLR4 pathway activity and thus induced the TRAF6/MAPK pathway but not TRIF or Iκκ pathways. This is exactly in line with the immune response caused by Lactobacillus plantarum AR113 (27). The overexpression of DdlLC increased the level of D-Ala at the peptidoglycan termini of potently induced the production of proinflammatory cytokines by macrophages in co-culture. As previously reported, the loss of D-Ala on LTA branch chains of L. plantarum resulted in certain anti-inflammatory effects, indicating that D-Ala itself has pro-inflammatory effects (6). Therefore, the content of D-ala in the cell wall may be used to characterize the immunomodulatory ability of the strain. Ddl or Lac dehydrogenase can determine the change of D-ala content in the strain. Therefore, they may be used to quickly screen for immunomodulatory strains. Overall, our research provides a potential theoretical basis for the application or screening of probiotics.
Data Availability Statement
The original contributions presented in the study are included in the article/supplementary material. Further inquiries can be directed to the corresponding author.
Author Contributions
Methodology: XS, FL, and MZ. Data curation: FL, MZ, and GW. Investigation: XS, FL, YX, and MZ. Resources: YX, and LA. Writing—Original draft: XS, FL, MZ, and GW. Editing: LA. Writing-Review and Editing: XS, LA, and GW. All authors contributed to the article and approved the submitted version.
Funding
This study was funded by Shanghai Agriculture Applied Technology Development Program, China (grant 2019-02-08-00-07-F01152), National Science Foundation for Distinguished Young Scholars of China (No. 32025029).
Conflict of Interest
The authors declare that the research was conducted in the absence of any commercial or financial relationships that could be construed as a potential conflict of interest.
Publisher’s Note
All claims expressed in this article are solely those of the authors and do not necessarily represent those of their affiliated organizations, or those of the publisher, the editors and the reviewers. Any product that may be evaluated in this article, or claim that may be made by its manufacturer, is not guaranteed or endorsed by the publisher.
References
1. Waldemar V, Didier B, De PMA. Peptidoglycan Structure and Architecture. FEMS Microbiol Rev (2008) 2:149–67. doi: 10.1111/j.1574-6976.2007.00094.x
2. Nagarajan R. Antibacterial Activities and Modes of Action of Vancomycin and Related Glycopeptides. Antimicrob Agents Chemother (1991) 35(4):605–9. doi: 10.1128/AAC.35.4.605
3. Kahne D, Leimkuhler C, Lu W, Walsh C. Glycopeptide and Lipoglycopeptide Antibiotics. ChemInform (2005) 36(24):425–48. doi: 10.1002/chin.200524268
4. Deghorain M, Goffin P, Fontaine L, Mainardi JL, Daniel R, Errington J, et al. Selectivity for D-Lactate Incorporation Into the Peptidoglycan Precursors of Lactobacillus Plantarum: Role of Aad, a VanX-Like D-Alanyl-D-Alanine Dipeptidase. J Bacteriol (2007) 189(11):4332–7. doi: 10.1128/JB.01829-06
5. Ferain T, Hobbs JN, Richardson J, Bernard N, Delcour J. Knockout of the Two Ldh Genes has a Major Impact on Peptidoglycan Precursor Synthesis in Lactobacillus Plantarum. J Bacteriol (1996) 178(18):5431–7. doi: 10.1128/jb.178.18.5431-5437.1996
6. Grangette C, Nutten S, Palumbo E, Morath S, Mercenier A. From The Cover: Enhanced Antiinflammatory Capacity of a Lactobacillus Plantarum Mutant Synthesizing Modified Teichoic Acids. Proc Natl Acad Sci (2005) 102(29):10321–6. doi: 10.1073/pnas.0504084102
7. Stetter KO, Kandler O. Formation of Dl -Lactic Acid by Lactobacilli and Characterization of a Lactic Acid Racemase From Several Streptobacteria. Archiv für Mikrobiologie (1973) 94(3):221–47. doi: 10.1007/BF00417453
8. Morath S. Structure–Function Relationship of Cytokine Induction by Lipoteichoic Acid Fromstaphylococcus Aureus. J Exp Med (2001) 193(3):393–8. doi: 10.1084/jem.193.3.393
9. Handwerger S, Pucci MJ. Vancomycin-Resistant Leuconostoc Mesenteroides and Lactobacillus Casei Synthesize Cytoplasmic. J Bacteriol (1994) 176(1):260–4. doi: 10.1128/jb.176.1.260-264.1994
10. Ghosh S, May MJ, Kopp EB. NF-κb AND REL PROTEINS: Evolutionarily Conserved Mediators of Immune Responses. Annu Rev Immunol (1998) 16(1):225. doi: 10.1146/annurev.immunol.16.1.225
11. DiDonato JA, Hayakawa M. A Cytokine-Responsive IkB Kinase That Activates the Transcription Factor NF-kB. Nature (1997) 388:548–54. doi: 10.1038/41493
12. Malinin NL, Boldin MP, Kovalenko AV, Wallach D. NF-κb Stimulatory Pathways Linked by a Common Kinase. Immunol Today (1997) 18(4):148–8. doi: 10.1016/S0167-5699(97)84658-0
13. Kaji R, Kiyoshima-Shibata J, Nagaoka M, Nanno M, Shida K. Bacterial Teichoic Acids Reverse Predominant IL-12 Production Induced by Certain Lactobacillus Strains Into Predominant IL-10 Production via TLR2-Dependent ERK Activation in Macrophages. J Immunol (2010) 184(7):3505–13. doi: 10.4049/jimmunol.0901569
14. Kozakova H, Schwarzer M, Tuckova L, Srutkova D, Czarnowska E, Rosiak I, et al. Colonization of Germ-Free Mice With a Mixture of Three Lactobacillus Strains Enhances the Integrity of Gut Mucosa and Ameliorates Allergic Sensitization. Cell Mol Immunol (2016) 13(002):251–62. doi: 10.1038/cmi.2015.09
15. Hazebrouck S, Przybylski-Nicaise L, Ah-Leung S, Adel-Patient K, Corthier G, Langella P, et al. Influence of the Route of Administration on Immunomodulatory Properties of Bovine Beta-Lactoglobulin-Producing Lactobacillus Casei. Vaccine (2009) 27(42):5800–5. doi: 10.1016/j.vaccine.2009.07.064
16. Shripada R, Gayatri A-J, Girish D, Simmer K. Probiotic Supplementation and Late-Onset Sepsis in Preterm Infants: A Meta-Analysis. Pediatrics (2016) 137(3):e20153684. doi: 10.1542/peds.2015-3684
17. Guangqiang W, Ying Z, Xin S, Yongjun X, Phoency F-H. Lactobacillus Casei LC2W can Inhibit the Colonization of Escherichia Coli O157:H7 In Vivo and Reduce the Severity of Colitis. Food Funct (2009) 10(9):5843–52. doi: 10.1039/c9fo01390c
18. Menard. Lactic Acid Bacteria Secrete Metabolites Retaining Anti-Inflammatory Properties After Intestinal Transport. Gut (2004) 53(6):821. doi: 10.1136/gut.2003.026252
19. Chen X, Yang G, Song JH, Hua X, Li D, Jeffrey G, et al. Probiotic Yeast Inhibits VEGFR Signaling and Angiogenesis in Intestinal Inflammation. PloS One (2013) 8(5):e64227. doi: 10.1371/journal.pone.0064227
21. Wells JM. Immunomodulatory Mechanisms of Lactobacilli. Microb Cell Factories (2011) 10(Suppl 1):S17. doi: 10.1186/1475-2859-10-S1-S17
22. Whun KK, Kang SS, Sun-Je W, Ok-Jin P, Bum AK, Song KD, et al. Lipoteichoic Acid of Probiotic Lactobacillus Plantarum Attenuates Poly I:C-Induced IL-8 Production in Porcine Intestinal Epithelial Cells. Front Microbiol (2017) 8:1827–. doi: 10.3389/fmicb.2017.01827
23. Yang P, Wang J, Qi Q. Prophage Recombinases-Mediated Genome Engineering in Lactobacillus Plantarum. Microb Cell Factories (2015) 14(1):1–11. doi: 10.1186/s12934-015-0344-z
24. Goffin P, Deghorain M, Mainardi JL, Tytgat I, Champomier-Verges MC, Kleerebezem M, et al. Lactate Racemization as a Rescue Pathway for Supplying D-Lactate to the Cell Wall Biosynthesis Machinery in Lactobacillus Plantarum. J Bacteriol (2005) 187(19):6750–61. doi: 10.1128/JB.187.19.6750-6761.2005
25. Karpuzoglu E, Ahmed SA. Estrogen Regulation of Nitric Oxide and Inducible Nitric Oxide Synthase (iNOS) in Immune Cells: Implications for Immunity, Autoimmune Diseases, and Apoptosis. Nitric Oxide Biol Chem (2006) 15(3):177–86. doi: 10.1016/j.niox.2006.03.009
26. Xue Q, Yan Y, Zhang R, Xiong H. Regulation of iNOS on Immune Cells and Its Role in Diseases. Int J Mol Sci (2018) 19(12). doi: 10.3390/ijms19123805
Keywords: Ddl ligase, Lactobacillus plantarum, D-alanine, vancomycin, immune pathways
Citation: Song X, Li F, Zhang M, Xia Y, Ai L and Wang G (2022) Effect of D-Ala-Ended Peptidoglycan Precursors on the Immune Regulation of Lactobacillus plantarum Strains. Front. Immunol. 12:825825. doi: 10.3389/fimmu.2021.825825
Received: 30 November 2021; Accepted: 20 December 2021;
Published: 19 January 2022.
Edited by:
Zhihong Sun, Inner Mongolia Agricultural University, ChinaCopyright © 2022 Song, Li, Zhang, Xia, Ai and Wang. This is an open-access article distributed under the terms of the Creative Commons Attribution License (CC BY). The use, distribution or reproduction in other forums is permitted, provided the original author(s) and the copyright owner(s) are credited and that the original publication in this journal is cited, in accordance with accepted academic practice. No use, distribution or reproduction is permitted which does not comply with these terms.
*Correspondence: Guangqiang Wang, 1015wanggq@163.com