- 1Biomolecular Mass Spectrometry and Proteomics, Bijvoet Center for Biomolecular Research and Utrecht Institute for Pharmaceutical Sciences, Utrecht University, Utrecht, Netherlands
- 2Netherlands Proteomics Centre, Utrecht University, Utrecht, Netherlands
Mass-spectrometry based immunopeptidomics has provided unprecedented insights into antigen presentation, not only charting an enormous ligandome of self-antigens, but also cancer neoantigens and peptide antigens harbouring post-translational modifications. Here we concentrate on the latter, focusing on the small subset of HLA Class I peptides (less than 1%) that has been observed to be post-translationally modified (PTM) by a O-linked N-acetylglucosamine (GlcNAc). Just like neoantigens these modified antigens may have specific immunomodulatory functions. Here we compiled from literature, and a new dataset originating from the JY B cell lymphoblastoid cell line, a concise albeit comprehensive list of O-GlcNAcylated HLA class I peptides. This cumulative list of O-GlcNAcylated HLA peptides were derived from normal and cancerous origin, as well as tissue specimen. Remarkably, the overlap in detected O-GlcNAcylated HLA peptides as well as their source proteins is strikingly high. Most of the O-GlcNAcylated HLA peptides originate from nuclear proteins, notably transcription factors. From this list, we extract that O-GlcNAcylated HLA Class I peptides are preferentially presented by the HLA-B*07:02 allele. This allele loads peptides with a Proline residue anchor at position 2, and features a binding groove that can accommodate well the recently proposed consensus sequence for O-GlcNAcylation, P(V/A/T/S)g(S/T), essentially explaining why HLA-B*07:02 is a favoured binding allele. The observations drawn from the compiled list, may assist in the prediction of novel O-GlcNAcylated HLA antigens, which will be best presented by patients harbouring HLA-B*07:02 or related alleles that use Proline as anchoring residue.
Introduction
Peptide antigen presentation by the human leukocyte antigen (HLA) to T-cells forms an integral part of the human immune surveillance (1). Characterizing the identity of these HLA peptide antigens is a critical first step to understand their immunomodulatory functions, and may guide important decisions in the development of vaccines used for immunotherapy (2, 3). A major source of therapeutic intervention for cancer patients comes from neoantigens in the HLA Class I ligandome, introduced by cancer induced mutations (4, 5). Mass spectrometry (MS) based immunopeptidomics provides means to identify disease or patient specific HLA antigen presentation, and chart the HLA bound peptide ligandome (6, 7). Next to the peptide sequence and protein of origin, mass spectrometry can also be used to identity post-translation modifications (PTMs) on the antigens. These may constitute another source of “neoantigens”, as several PTMs are recognized as hallmarks of specific diseases, including cancer and autoimmune diseases (8, 9). Peptide antigens carrying such PTMs have been shown to affect or even regulate immune system recognition of the HLA Class I peptides (10–12).
Of all the HLA peptides presented by cells or in a tissue, only a small fraction seems to harbor PTMs (13–18). Several studies have reported on phosphorylated HLA peptides, and even shown that these could be considered Tumor Associated Antigens (TAAs) (19). Since phosphorylation signalling can be drastically dysregulated in cancer, immunity to such modified epitopes can also be lost or gained under disease conditions (19, 20). Modification on serine and threonine residues with β N-acetylglucosamine (O-GlcNAc) is known to engage in reciprocal crosstalk with phosphorylation (21), and such a dynamic balance between phosphorylation and O-GlcNAcylation is particularly relevant in the nucleus and cytosol, where the enzymes coordinating the addition or removal of O-linked N-acetylglucosamine (O-GlcNAc) reside. When O-GlcNAcylated proteins are degraded via the proteasomal route and loaded onto the HLA Class I molecules, the presented peptides can retain the O-GlcNAc moiety from the source protein. Notably, aberrant O-GlcNAcylation has been shown to correlate with augmented cancer cell proliferation, survival, invasion, and metastasis (22). It has also been reported that HLA peptides can elicit glycopeptide specific T-cell responses (13, 14). Hence, it is important to characterize the HLA ligandome for O-GlcNAcylated class I peptides.
HLA class I peptides are generally more difficult to sequence and identify by mass spectrometry, compared to tryptic peptides, as they are relatively small, do not carry a charged C-terminus (R/K in tryptic peptides), and fragment less efficiently into complementary series of b- and y-ions. Recent advances in immunopeptidomics have substantially improved the identification of HLA peptides, notably the sensitivity and sequence specificity have been improved, making detection of neoantigens more feasible (23, 24). Using hybrid tandem MS fragmentation methods, Marino et al. and Malaker et al. independently identified so far some of the largest sets of O-GlcNAc modified HLA Class I peptides (25, 26). Both studies reported a few dozen of unique O-GlcNAc modified HLA Class I peptides, but also showed that some of these carried additional glycans, extended by Gal, Gal-NeuAc and even other monosaccharides. Moreover, Malaker et al. reported potent multifunctional T-cell responses to some of these O-GlcNAc modified HLA Class I peptides, but not to the unmodified HLA Class I peptide counterpart, and apparently also found more O-GlcNAc modified HLA Class I peptides in cancer cells and tissue, compared to non-cancerous cells. To further improve our understanding of the immunological role that O-GlcNAc modified HLA Class I peptides might have to play, it is important to examine the properties of these peptides, the source proteins of origin, the HLA loading specificity, and whether these peptides are functionally activating in immune surveillance.
In this report, using hybrid fragmentation MS strategies, we identified a new set of in total 23 O-GlcNAc HLA Class I peptides presented by the non-cancerous JY B-lymphoblastoid cell line. We compared this dataset with the earlier reported O-GlcNAc HLA Class I peptides described in the literature to collate a total list of 55 O-GlcNAcylated HLA Class I peptides. Our compilation includes the glycopeptide sequence, the likely subcellular localisation of the proteins of origin and the predicted HLA allele and their respective binding affinity. We observed substantial congruence between the glycopeptide sequences we report here and the existing datasets of Malaker et al, and Marino et al. While comparing these datasets we noticed that O-GlcNAc HLA Class I peptides were presented with a marked preference by HLA-B*07:02, which harbors a Pro anchoring site for the P2 position.
Materials and Methods
Cell Culture and Isolation of HLA Class I Associated Peptides
The B-lymphoblastoid cell line JY, homozygote in class I alleles (HLA-A*02:01, HLA-B*07:02, HLA-C*07:02) was cultured in RPMI 1640 supplemented with 10% fetal bovine serum, 50 U/mL penicillin, and 50 μg/mL streptomycin. HLA class I peptides were retrieved via immunoaffinity purification, as described previously (24, 27). Specifically, HLA class I complexes were immunoprecipitated using the pan-HLA class I mouse monoclonal IgG2a antibody W6/32 (28), and antigen peptides were separated from HLA molecules by elution with 10% (v/v) acetic acid, and filtration over a 10-kDa molecular weight cutoff membrane (Merck Millipore). The HLA class I peptide ligands was freeze-dried, and reconstituted in 0.1% formic acid for further cleanup by C18 STAGE tips (Thermo Fischer Scientific) before LC/MS-MS analysis.
LC-MS/MS Analysis
The HLA Class I peptides were analyzed using an Ultimate 3000 UHPLC (Thermo Fisher Scientific) coupled to an Orbitrap Fusion Lumos (Thermo Fischer Scientific). The peptides were trapped on (Thermo Fisher Scientific, µ-Precolumn, 300 µm i.d. x 5mm, C18 PepMap100, 5 µm, 100 Å) for 5 min in solvent A (0.1% formic acid in water) before being separated on an analytical column (Agilent Poroshell, EC-C18, 2.7 μm, 50 cm × 75 μm). Solvent B consisted of 80% acetonitrile in 0.1% formic acid. The gradient was as follows: first 5 min of trapping, followed by 100 min gradient from 5% to 40% solvent B. Subsequently, 10 min of washing with 99% solvent B and 10 min re-equilibration with 9% solvent A. The mass spectrometer operated in data-dependent mode. Full scan MS spectra from m/z 350 to 1400 were acquired at a resolution of 120,000 in the Orbitrap after accumulation to a target value of 4 × 105 or a maximum injection time of 50 ms.
For global acquisition of the JY immunopeptidome, higher-energy collisional dissociation (HCD) MS/MS spectra were acquired at a resolution of 60,000. Charge states of 2 up to 4 starting at m/z 120 were chosen for fragmentation using the MIPS algorithm with 1.2 Da isolation window. The fragmentation was performed using 30% normalized collision energy (NCE) on selected precursors with 30s dynamic exclusion after accumulation of 5×104 ions. Focused characterisation of the O-GlcNAcylated immunopeptidome was performed by electron transfer dissociation (ETD) and energy-stepped HCD, triggered when two of the six O-GlcNAc signature fragment ions (m/z 204, 186, 168, 144, 138, and 126) were detected at > 5% relative abundance, as described previously (29, 30).
Data Analysis
All RAW files were searched using the PEAKS Studio 10.5 against the Swiss-Prot human database (20,258 entries, downloaded in February 2018) edited with the JY-specific HLA proteins and 20 most abundant FBS contaminants, with no enzyme specificity in the search engine to identify the unmodified HLA ligandome. Precursor ion and MS/MS tolerances were set to 10 ppm and 0.03 Da. Methionine oxidation and cysteinylation were set as variable modifications. Peptides were filtered by precursor tolerance 5 ppm, < 1% FDR, XCorr > 1.7, and peptide rank 1. Only peptides between 8 and 12 amino acid long were selected for further analysis. Glycopeptide assignments were made by searching the same RAW files using Byonic 4.1. Precursor and MS/MS tolerance were set to 10 ppm and 0.03 Da. Peptide loading affinity was predicted with NetMHC 4.1 algorithm for the identified HLA peptide sequences against HLA-A*02:01, HLA-B*07:02 and HLA-C*07:02 allele, without considering potential changes in loading affinity due to O-GlcNAcylation. Peptides were considered binders when % Rank < 2. We classified the binding predictions for the O-GlcNAc HLA peptides as strong (IC50 ≤ 50 nM), regular (50 nM < IC50 ≤ 500 nM) and weak (500 nM < IC50 ≤ 5000 nM) binders for peptide sequence. Gene ontology (GO) analysis of the source proteins of the O-GlcNAcylated HLA class I peptides was performed by functional annotation tool DAVID (31). The mass spectrometry proteomics data have been deposited to the ProteomeXchange Consortium via the PRIDE (32) partner repository with the dataset identifier PXD028874.
Results and Discussion
Triggered MS Detection of O-GlcNAcylated HLA Peptide Ligands
Here, we first revisited the ligandome of the JY B-lymphoblastoid cell line, a model cell line used by several groups active in immunopeptidomics including ours (27, 33–35), as it has relative high expression of HLA complexes, and is homozygote in its HLA class I alleles, harbouring just HLA-A*02:01, HLA-B*07:02, and HLA-C*07:02 alleles. We have previously shown that several thousand of HLA peptides can be identified when analyzing JY cells. Here we attempted to characterize the glycosylated subset and report the detection of 23 unique O-GlcNAcylated HLA class I peptides. In our calculation we count each unique peptide just once, even when it is identified with multiple different PTMs. To boost the identifications we examined the occurrence of diagnostic oxonium ions (m/z 204, 186, 168, 144, 138) in the MS2 spectra, and used these features to trigger targeted analysis by ETD and stepped HCD, to boost the generation of fragment ions for identification of the glycopeptide backbone (17, 26). As described earlier (36), the relative abundance of the oxonium fragment ions was used to confirm O-GlcNAc assignment of all 23 O-GlcNAc HLA peptides identified, as opposed to being O-GalNAcylated. A representative EThcD spectrum from the O-GlcNAcylated TPASgSRAQTL peptide is shown in Figure 1, exposing both backbone fragments as well as glycan ions.
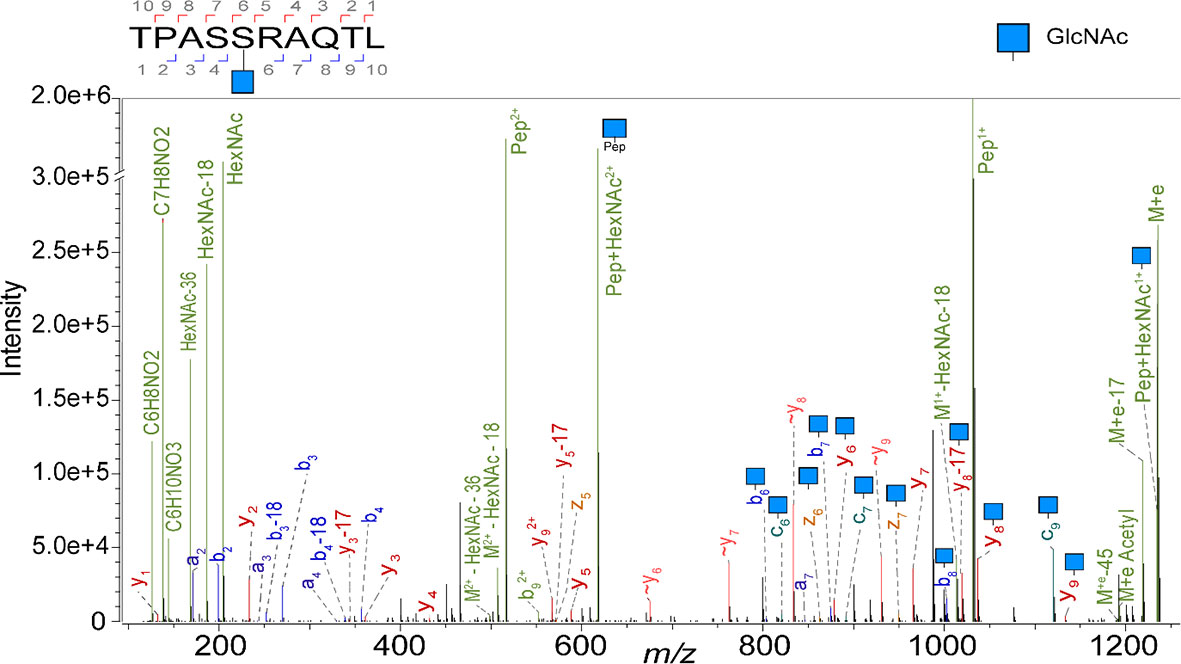
Figure 1 Illustrative EThcD mass spectrum of the peptide TPASgSRAQTL, originating from Spectrin, in its O-GlcNAcylated form, modified at P5. Highlighted in green are the oxonium ions. Fragment ions that elucidate the amino acid sequence and the glycosylation site are labelled as b, c, y and z. The fragment ions without modification are indicated with a wave dash.
Distribution of O-GlcNAcylated HLA Class I Peptides
In the present ligandome analysis of the JY cells, we detected around ~ 8800 unique HLA class I peptides, and thus these 23 O-GlcNAcylated HLA peptides constitute in numbers just about 0.26% of the total ligandome. By examining the binding affinity to HLA-A*02:01, HLA-B*07:02 and HLA-C*07:02, we observed that 21 out of the 23 (91%) were predicted to be strong binders of the HLA-B*07:02 allele (Figure 2A and Table 1). This prevalence for HLA-B*07:02 was in striking contrast to the distribution of the unmodified HLA antigens, where 41% of all HLA peptides were predicted to be strong binders to HLA-B*07:02 (Figure 2A). The O-GlcNAcylated peptides in our JY dataset originated from just 21 non-redundant source proteins. We found this low number of detected O-GlcNAcylated peptides and source proteins quite surprising, as a recent compilation, termed the human ‘O-GlcNAc-ome’ database (37), described that in the human proteome at least 7000 different O-GlcNAcylation sites on cumulatively 5000 proteins could be present. Evidently, not many of these lead to an abundant presentation of O-GlcNAcylated HLA class I peptides. Around three quarters of the source proteins were of nuclear origin. We also observed that quite a few of the source proteins have been functionally annotated as binding to either DNA or RNA. This observation, although based on a small number of O-GlcNAcylated peptides detected in our dataset, seems to agree with the suggestion that O-GlcNAc modifications are critical in the regulation of transcriptional events (38).
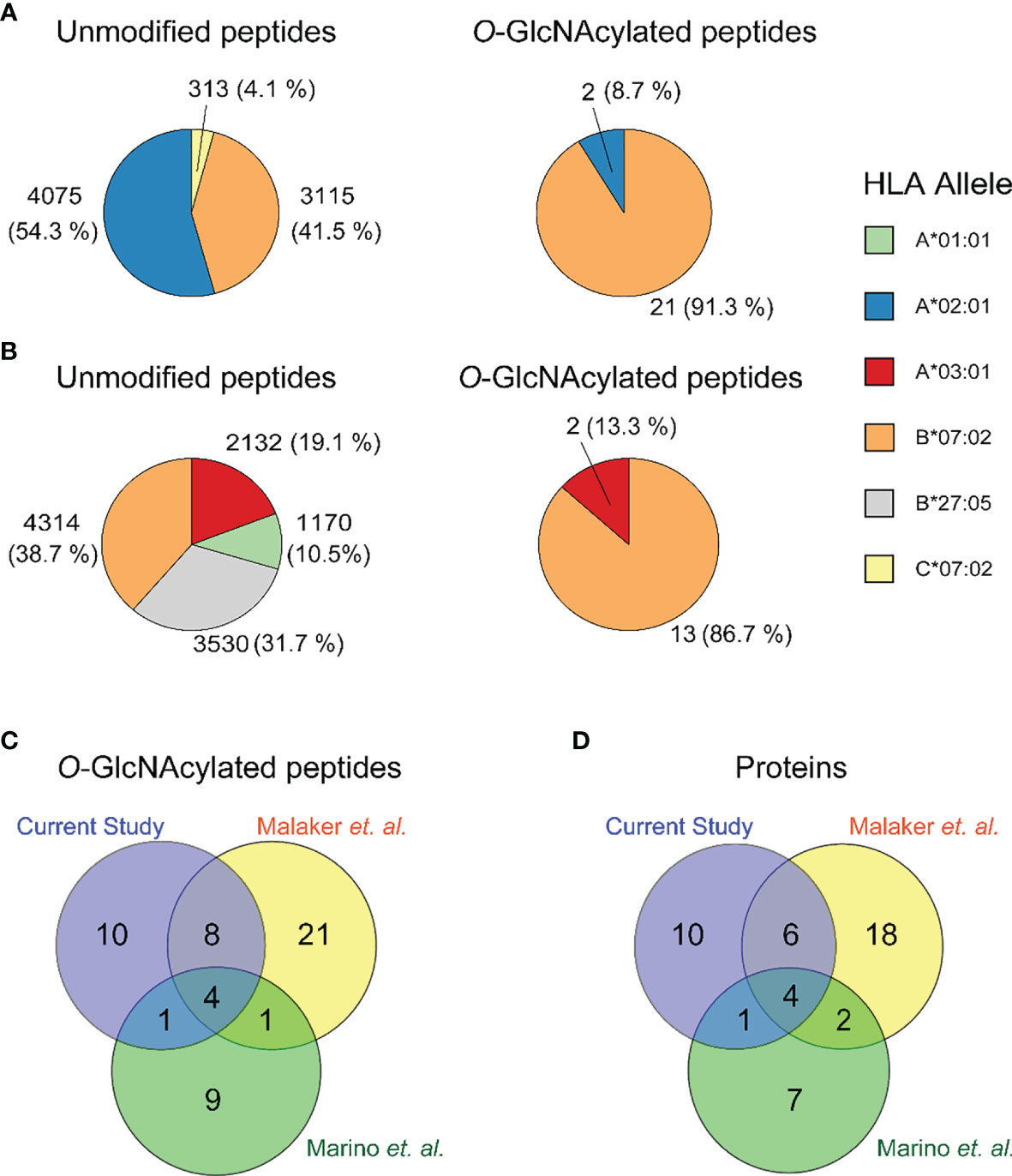
Figure 2 Distribution of the identified unmodified (left) and O-GlcNAcylated (right) HLA class I peptides over (A) the JY HLA alleles in the current study, and (B) all HLA alleles in the GR-LCL cell line (C) Overlap in identified peptides in the current study (purple), Malaker et al. (yellow) and Marino et al. (green). (D) Overlap in identified proteins in the current study (purple), Malaker et al. (yellow) and Marino et al. (green).
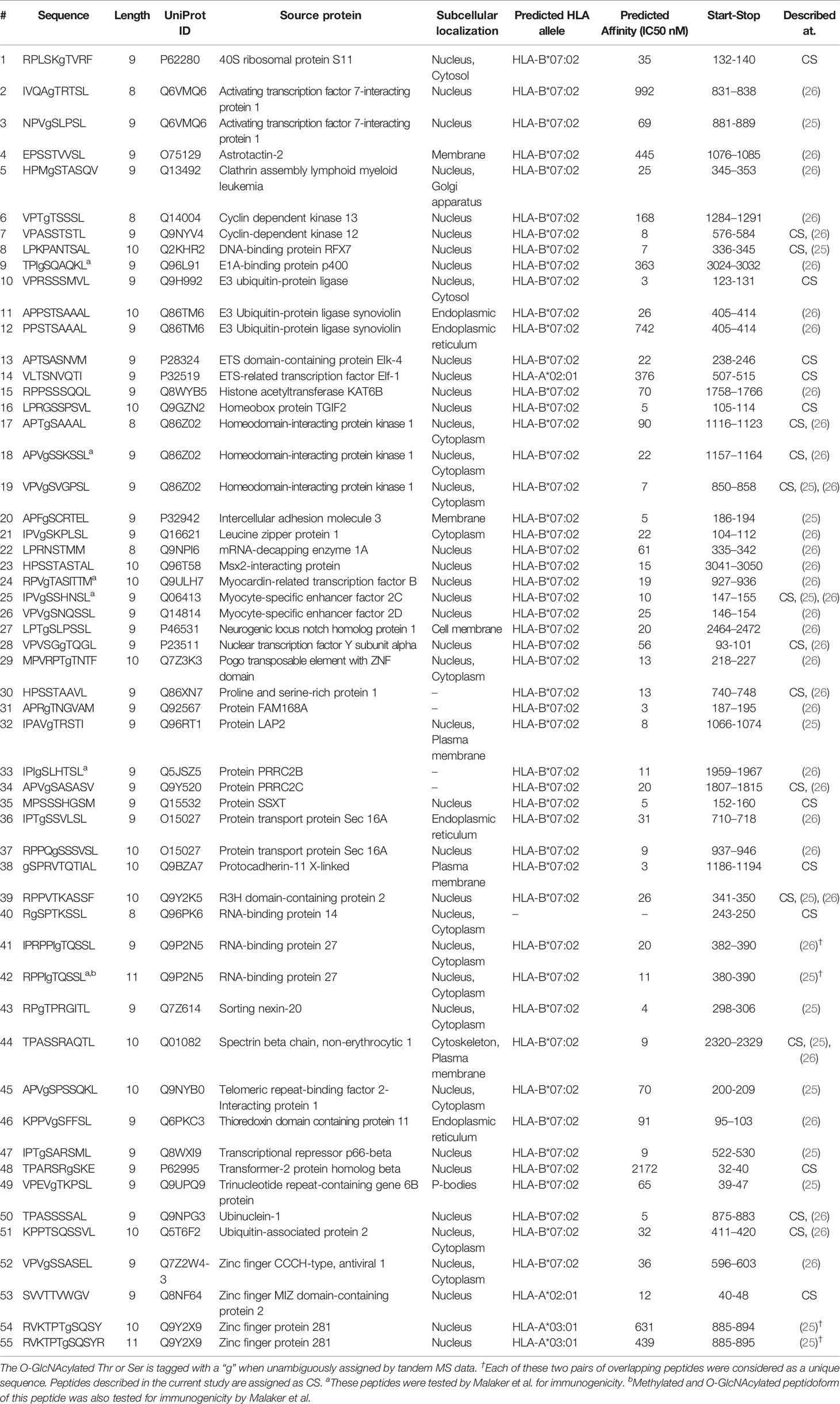
Table 1 Compilation of O-GlcNAcylated HLA class I peptides identified in the current study by Marino et al. and Malaker et al.
Earlier, Marino et al. and Malaker et al. have reported on O-GlcNAcylated HLA class I peptides. We therefore consolidated our identifications, with their earlier data, to compile a comprehensive compendium of experimentally detected O-GlcNAcylated HLA class I peptides. Marino et al. were the first to report on a variety of glycosylated HLA class I peptides, immunoprecipitated and enriched using the pan-HLA-I-specific antibody (W6/32), from the GR B-lymphoblastoid cell line (25). This GR-LCL cell line is heterozygote in HLA class I alleles and harbors the HLA-A*01:01, A*03:01, B*07:02, B*27:05, C*02:02, C*07:02 alleles. In total Marino et al. detected 15 O-GlcNAcylated peptides with unique backbone sequences, in a full dataset of ∼15,000 HLA class I peptides (17, 25). Therefore, also in their data O-GlcNAcylated peptides constituted just around 0.1% of all HLA peptides. They observed that approximately 87% of the detected O-GlcNAcylated peptides were predicted to bind to HLA-B*07:02, whereas for the unmodified peptides this was just ~39% (Figure 2B). Another key feature of these O-GlcNAc HLA peptides was that most of them were derived from nuclear source proteins. All these findings are very much in line with our observations, although here we used the JY cell line.
Malaker et al. analyzed the HLA class I immunopeptidomes from blood cells of primary leukemia (chronic myeloid leukemia, acute myeloid leukemia and acute lymphoblastic leukemia) patients, relying on a HLA-B*07-specific antibody (ME1) to pull down HLA Class I complexes (26). Healthy T and B cells were isolated from normal spleen and tonsil biopsies from healthy donors. Additionally, they also included the JY cell line in their analysis, as a reference system. To optimize the detection of O-GlcNAcylated peptides they combined several different approaches, including HCD-MS/MS analysis of the HLA ligandome, making use of glycan specific neutral losses, an HCD triggered ETD approach like Marino et al., and a selective enrichment of esterified glycopeptides using an amino phenylboronic acid-derivatized affinity matrix to capture the glycopeptides. As in the other mentioned studies and ours, the relative glycan fragment ion intensities were used to confirm that all peptides identified were indeed O-GlcNAcylated. Notwithstanding these thorough and admirable efforts, and considering the multiple tissues, biopsies and cell lines investigated, Malaker et al. could identify and characterize only 34 unique glycosylated HLA class I peptides in total. Interestingly, most of the O-GlcNAcylated peptides (33/34) were found by them exclusively in the leukemia samples, and they reported solely one O-GlcNAcylated HLA peptide detected in the JY cells (as opposed to the 23 we report here). This increase in identifications of O-GlcNAcylated HLA Class-I peptides, when compared to the study of Malaker et al., could be due to multiple issues, such as the use of a more sensitive mass spectrometer, refined bioinformatics tools and a combination of hybrid fragmentation triggering methods. Moreover, Malaker et al. studied the JY cells just as a model system, focusing more on the primary cancer cells and tissues. As in the work of Marino et al., several (10 out of 34) O-GlcNAc peptides were also detected to carry one or more monosaccharide extensions (Gal and Gal-NeuAc).
Given the small number of O-GlcNAcylated HLA class I peptides detected in each of these studies, as well as in the current study, we had expected a priori that such small number of identifications might render the overlap in between these studies to be close to zero. Nonetheless, this was not the case, and instead, there was a substantial overlap in detected O-GlcNAcylated HLA class I peptides (Figure 2C) and their source proteins (Figure 2D). This was even more surprising, given that the source material in these three studies was completely different. Cumulatively, from the datasets described above we compiled a list of 55 unique O-GlcNAcylated HLA class I peptides (Table 1).
The potential role of O-GlcNAcylated peptides as neoantigens was investigated in depth by Malaker et al. Using a subset of both unmodified as well as O-GlcNAcylated HLA Class I peptides they assessed seven of the O-GlcNAcylated peptides detected in the leukemia cells. Five out of these seven HLA-B*07:02 glycopeptides were immunogenic. All healthy donors had immunity to at least one of the glycopeptides with strong responses similar to chronic viral antigens. They further assessed cytotoxicity responses in healthy donors towards a specific peptide harboring both methylation and O-GlcNAcylation. These latter HLA peptides invoked a significant T-cell activation. In contrast, no T-cell response was observed for the unmodified HLA peptide counterpart. Notably, we identified two out of the seven O-GlcNAcylated HLA-I peptides that were tested for immunogenicity by Malaker et al. also in our study on non-cancerous JY cells. These two peptides are APVgSSKSSL and IPVgSSHNSL In summary, these findings suggest that the specific T-cell response towards O-GlcNAcylated HLA-B*07:02 peptides may potentially represent an autologous immunoprotective mechanism against leukemia.
One of the most striking features examining all identified O-GlcNAc HLA class I peptides is that approximately 90% (50/55) peptides are predicted to be strong binders to HLA-B*07:02 allele. This finding was expected for the dataset of Malaker et al. as they used an antibody specific for HLA-B*07, but in Marino et al. and in the current study a pan-HLA antibody was used that has no specific preference for the HLA-B*07 allele. Thus, the compiled data hint at an overall preference for O-GlcNAcylated HLA class I peptides to be presented by HLA-B*07:02, regardless of the immunopeptidome isolation strategy.
Preferential Loading of O-GlcNAcylated HLA Class I Peptides on HLA-B*07:02
To further investigate a potential basis for the observed preferential presentation of O-GlcNAcylated peptides by the HLA-B*07:02 allele, we evaluated the peptide amino acid sequences of the 55 O-GlcNAcylated HLA peptides and compared them against the sequences of the unmodified peptides observed to bind the HLA-B*07:02 allele in the current dataset. Considering the site of modification, most O-GlcNAc sites were found in between position 3 to position 7 (P3-P7), with the highest incidence at position 4 (P4). Peptides presented by HLA-B*07:02 have a high preference for a proline residue at P2 position (Figure 3A). The peptide sequences of the 9-mer O-GlcNAcylated peptides (32 peptides in the compiled list) predominantly confirmed the presence of a proline residues at P2 along with preference for a valine residue at P3, a serine at P4 (72%, p-value < 0.05) and a serine or threonine residue at P5 (Ser 36%, p-value < 0.05) as illustrated in Figure 3B. Similarly sequences for the 10-mer O-GlcNAcylated peptides (12 peptides) were also rather consistent with a proline residue at P2 or P3, valine in P4 and serine/threonine residues at P4 to P6 (Table 1).
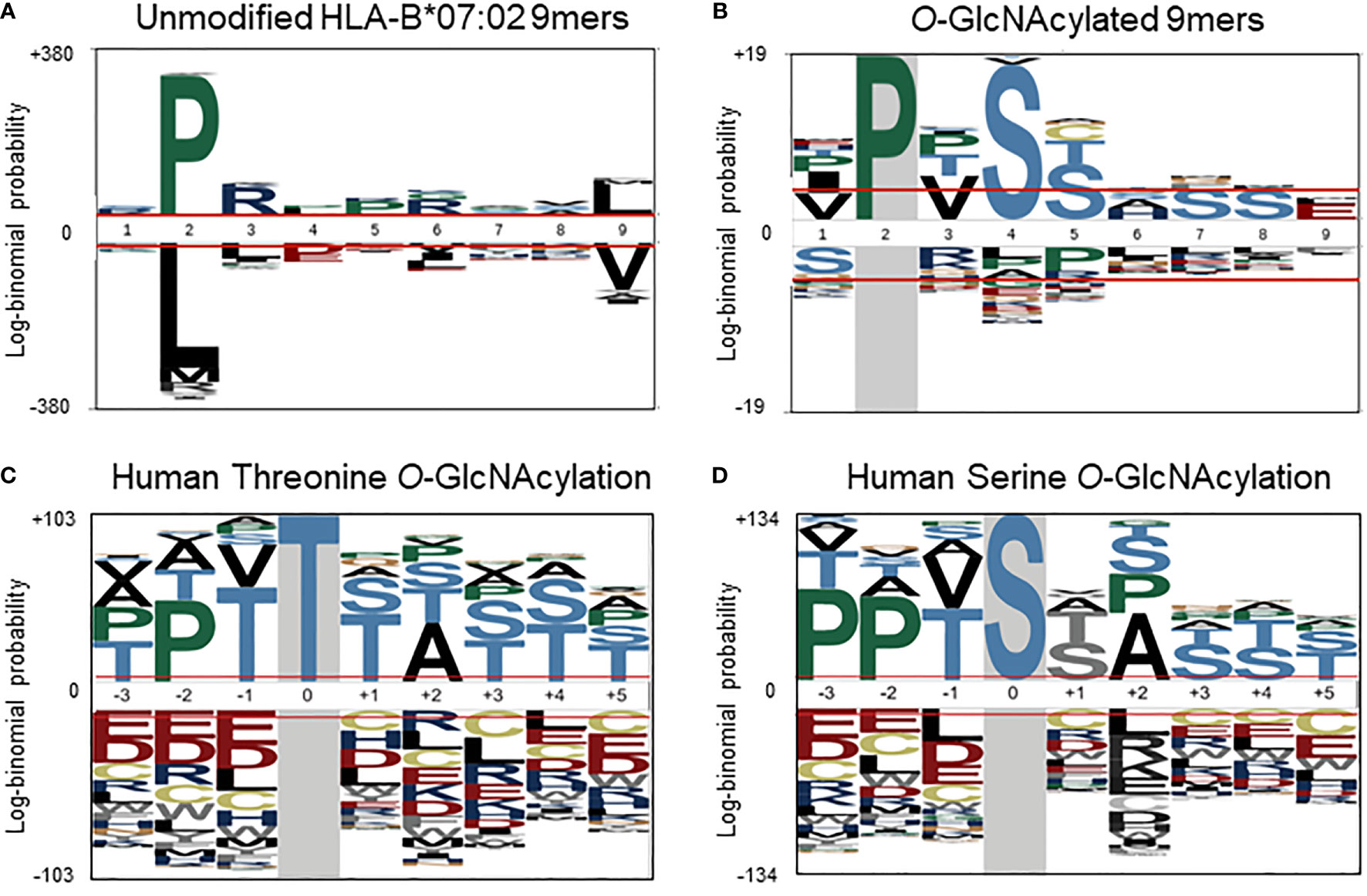
Figure 3 (A) Sequence motif (pLogo) of the in the current study detected unmodified HLA-B*07:02 peptides (9-mers) predicted as binders (% Rank < 2), with the clear anchoring positions Pro at P2 and Leu at P9. (B) Sequence motif of the O-GlcNAcylated HLA peptides (9-mers), with next to Pro at P2, Val at P3 and Ser at P4 and P5. Semi-consensus sequences based on the 7002 human O-GlcNAcylation sites for (C) Threonine and (D) Serine O-GlcNAcylation. (adapted from Wuff-Fuentes et al. (https://creativecommons.org/licenses/by/4.0/legalcode).
Although protein O-GlcNAcylation has been studied for decades, for a long time it was not clear that there could be a conserved substrate sequence motif. However, more recent structural (21, 39) and bioinformatic studies (37) have revealed a semi-consensus substrate motif for protein O-GlcNAcylation, namely P-P-(V/T)-g(S/T)-(S/T)-A (Figures 3C, D) (37). This motif matches well with the sequence motif compiled from our compendium of O-GlcNAcylated HLA class I peptides, where a dominant proline is observed (Figures 3B, D). It is quite conceivable that HLA-B*07 peptide antigens also naturally over-represent the O-GlcNAcylation motif, thereby resulting in preferential presentation of O-GlcNAcylated HLA class I peptides by the HLA-B*07 allele.
Compiling the combined dataset of O-GlcNAcylated HLA class I peptides from three recent studies, the substantial overlap in peptides detected in all or at least two of these studies was striking. Moreover, some other peculiar features were also repeatedly observed in all studies, for instance, the frequent co-occurrence of other PTMs, and the observation of glycan extensions next to the O-GlcNAcylation.
Co-Occurring PTMs on O-GlcNAcylated Peptides (N-(di)Methylation, Phosphorylation)
Both Marino et al. and Malakar et al. observed glycan extensions on quite a few O-GlcNAcylated HLA peptides. For instance, the most immunogenic O-GlcNAcylated peptide identified by Malaker et al. was me-RPPIgTQSSL, originating from the RNA-binding protein 27 (aa 382–390), which contains both a methylated N-terminal arginine and an O-GlcNAcylated threonine at P5. Interestingly, killing of autologous B cells pulsed with methylated, O-GlcNAcylated, and the doubly modified HLA peptide was observed in their study, however not with the unmodified peptide. Potent T-cell response with the doubly modified peptide suggests that methylation may potentially further boost immunogenicity. This HLA peptide was detected in quite a few different peptidoforms in their studies Figure 4. Besides the GlcNAc (T5), mono-methyl (R1), they also observed the GlcNAc (T5), symmetric di-methyl (R1) and GlcNAc (T5), and asymmetric di-methyl (R1) + acetylGlcNAc (T5). Marino et al. also detected quite a few different O-GlcNAcylated peptides from the RNA-binding protein 27, albeit all within the N-terminal extended backbone IPRPPIgTQSSL (aa 380–390) Figure 4. They reported five different forms: non-O-GlcNAc, one GlcNAc (Thr5), hexose-GlcNAc and even more extended glycans with mass shifts of 730 and 1021 Da. Moreover, arginine (Arg3) was observed to be modified with a mono-methyl (Arg3) or asymmetric di-methyl (Arg3), both with and without the glycan modifications. Thus, although we define RPPgTQSSL and IPRPPIgTQSSL as one unique peptide in Table 1, these are presented as HLA ligands in a dozen of different “peptidoforms”. In general, not much is known about the RNA-binding protein 27, and the specific sequence motif presented as HLA peptides. Moreover, whether there is any cross-talk in between these PTMs is also not known.
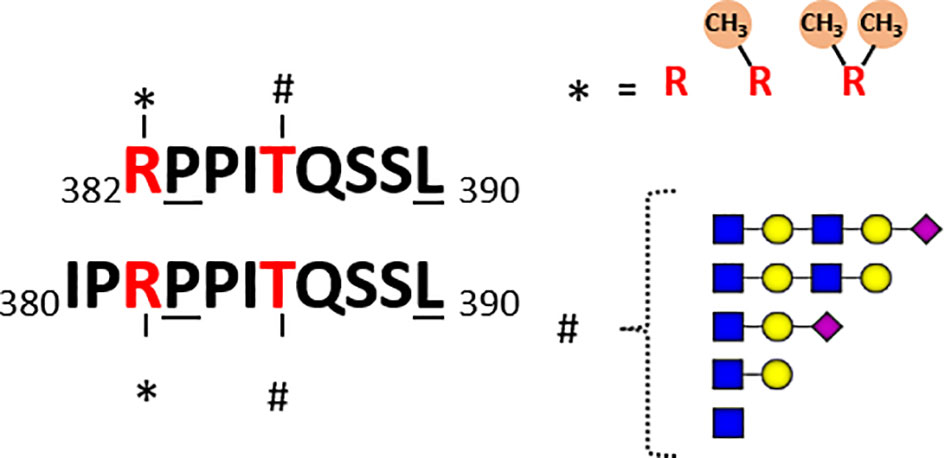
Figure 4 Schematic of detected co-occurring peptidoforms for the two overlapping unique HLA peptides detected, RPPITQSSL and IPRPPITQSSL, originating from RNA-binding protein 27. In the datasets of both Marino et al. and Malaker et al. several O-GlcNAcylated HLA peptides originating from the RNA binding protein 27 were reported. In Marino et al. the backbone sequence covered aa 380-390, whereas Malaker et al. observed the alike peptide covering aa 382-390. These peptides are likely HLA-B*07:02 binders, due to the underlined anchoring positions Pro at 2 and Leu at C-terminal position. In both datasets peptides were found with the Arg at 1 (3) being non-methylated, mono-methylated or di-methylated. The Thr at P5 (P7) was detected in several different glycoforms: non-O-GlcNAc, one GlcNAc, hexose-GlcNAc and even more extended glycans with mass shifts of 730 and 1021 Da.
Next, the peptides RVKpTPTgSQSY and RVKpTPTgSQSYR of the zinc-finger protein ZNF218 were also detected with both a phosphorylated Thr886 and an (extended) O-GlcNAc on Ser891. These peptides were also detected with mass extensions of 0, 203, 365, 656, 730, and 1021 Da, all indicative of a peptide backbone with extensions from 0 up to 6 glycan moieties. Both PTM sites have been reported previously, although no report is known if they co-occur in the source protein (40, 41). The proximity of O-GlcNAcylation and phosphorylation in RVKTPTSQSY may also hint at potential PTM crosstalk.
Finally, Malaker et al. reported glycosylation on the peptide IPVSSHNSL from the Myocyte-specific enhancer factor 2C protein, a transcription factor implicated in leukemogenesis (42), that was detected in 4 different glycoforms: GlcNAc (Ser4), double GlcNAc (Ser4, Ser5), single hexose-GlcNAc (Ser4), and an acetyl-GlcNAc (Ser4). This same peptide was also detected in Marino et al., although only in its O-GlcNAcylated form.
The origin of these additional glycan moieties is still puzzling. Most of the proteins observed to be decorated with these glycan extensions find their origin in the nucleus. Computational modelling of O-GlcNAcylated RPPVgTKASSF in complex with HLA molecule by Marino et al. revealed the glycan moiety was solvent exposed and not directly involved in the binding to the HLA Class I molecule groove. It is therefore plausible, as suggested earlier by Marino et al., that they may be first formed by proteasomal degradation as O-GlcNAcylated peptide, and then loaded onto an HLA class I molecule and extended by glycosyltransferases, starting with β1, 4 galactosyltransferase, in the Golgi, but this needs further validation. In general, the co-occurrence of so many peptidoforms of O-GlcNAcylated HLA Class I peptides raises the question whether all these forms have specific T-cell response and whether they are differentially immunogenic.
Summary
Combining data from our new dataset of O-GlcNAcylated HLA peptides from JY cells, with two recent related studies, we were able to compile a concise list of bone fide O-GlcNAcylated HLA peptides, presented by a whole array of different cancer and non-cancerous cells. Our comprehensive analysis of the sequences of all these peptides revealed preferential presentation of O-GlcNAcylated HLA peptides by the HLA-B*07 allele. The proline at P2 position of this allele allows the semi-consensus sequence motif of O-GlcNAcylation to be presented prominently. This preferred rule of presentation by HLA-B*07 and HLA-B*07-like alleles may also be useful in predicting putative O-GlcNAcylated HLA peptide antigens. Moreover, although the number of O-GlcNAcylated HLA peptides detected are small in each of the three studies evaluated, the overlap between these diverse studies were substantially high. Several of the reported O-GlcNAcylated HLA peptides are presented in multiple peptidoforms, carrying either additional phosphorylation or arginine-(di)methylation, along with glycan extensions of between 0 to 6 more carbohydrate moieties. Whether such distinct peptidoforms have differential functionality in immune surveillance needs to be further addressed.
Data Availability Statement
The datasets presented in this study can be found in online repositories. The names of the repository/repositories and accession number(s) can be found below: www.ebi.ac.uk/pride, PXD028874.
Author Contributions
AH conceived the idea. AS-B performed the MS experiments. SM and AS-B analyzed the data and wrote the initial draft of the manuscript along with AH. SM and AS-B contributed equally to this manuscript. All the authors contributed to the article and approved on the submitted version.
Conflict of Interest
The authors declare that the research was conducted in the absence of any commercial or financial relationships that could be construed as a potential conflict of interest.
Publisher’s Note
All claims expressed in this article are solely those of the authors and do not necessarily represent those of their affiliated organizations, or those of the publisher, the editors and the reviewers. Any product that may be evaluated in this article, or claim that may be made by its manufacturer, is not guaranteed or endorsed by the publisher.
Acknowledgments
We would like to acknowledge support for this research through the NWO funded Netherlands Proteomics Centre through the National Road Map for Large-scale Infrastructures program X-Omics (Project 184.034.019). LD and AH are further supported by the NWO Gravitation program Institute for Chemical Immunology (ICI00003). We acknowledge Dr. Stefan Stevanović (University of Tubingen, Germany) for providing the pan-HLA antibody W6/32.
References
1. Croft NP. Peptide Presentation to T Cells: Solving the Immunogenic Puzzle: Systems Immunology Profiling of Antigen Presentation for Prediction of CD8(+) T Cell Immunogenicity. Bioessays (2020) 42(3):e1900200. doi: 10.1002/bies.201900200
2. Parmiani G, Castelli C, Dalerba P, Mortarini R, Rivoltini L, Marincola FM, et al. Cancer Immunotherapy With Peptide-Based Vaccines: What Have We Achieved? Where are We Going? J Natl Cancer Inst (2002) 94(11):805–18. doi: 10.1093/jnci/94.11.805
3. Kowalewski DJ, Schuster H, Backert L, Berlin C, Kahn S, Kanz L, et al. HLA Ligandome Analysis Identifies the Underlying Specificities of Spontaneous Antileukemia Immune Responses in Chronic Lymphocytic Leukemia (CLL). Proc Natl Acad Sci USA (2015) 112(2):E166–75. doi: 10.1073/pnas.1416389112
4. Ott PA, Hu Z, Keskin DB, Shukla SA, Sun J, Bozym DJ, et al. An Immunogenic Personal Neoantigen Vaccine for Patients With Melanoma. Nature (2017) 547(7662):217–21. doi: 10.1038/nature22991
5. Sahin U, Derhovanessian E, Miller M, Kloke BP, Simon P, Lower M, et al. Personalized RNA Mutanome Vaccines Mobilize Poly-Specific Therapeutic Immunity Against Cancer. Nature (2017) 547(7662):222–6. doi: 10.1038/nature23003
6. Hunt DF, Henderson RA, Shabanowitz J, Sakaguchi K, Michel H, Sevilir N, et al. Characterization of Peptides Bound to the Class I MHC Molecule HLA-A2.1 by Mass Spectrometry. Science (1992) 255(5049):1261–3. doi: 10.1126/science.1546328
7. Wilhelm M, Zolg DP, Graber M, Gessulat S, Schmidt T, Schnatbaum K, et al. Deep Learning Boosts Sensitivity of Mass Spectrometry-Based Immunopeptidomics. Nat Commun (2021) 12(1):3346. doi: 10.1038/s41467-021-23713-9
8. Engelhard VH, Altrich-Vanlith M, Ostankovitch M, Zarling AL. Post-Translational Modifications of Naturally Processed MHC-Binding Epitopes. Curr Opin Immunol (2006) 18(1):92–7. doi: 10.1016/j.coi.2005.11.015
9. Doyle HA, Mamula MJ. Post-Translational Protein Modifications in Antigen Recognition and Autoimmunity. Trends Immunol (2001) 22(8):443–9. doi: 10.1016/S1471-4906(01)01976-7
10. Petersen J, Purcell AW, Rossjohn J. Post-Translationally Modified T Cell Epitopes: Immune Recognition and Immunotherapy. J Mol Med (Berl) (2009) 87(11):1045–51. doi: 10.1007/s00109-009-0526-4
11. Marcilla M, Alpizar A, Lombardia M, Ramos-Fernandez A, Ramos M, Albar JP. Increased Diversity of the HLA-B40 Ligandome by the Presentation of Peptides Phosphorylated at Their Main Anchor Residue. Mol Cell Proteomics (2014) 13(2):462–74. doi: 10.1074/mcp.M113.034314
12. Mohammed F, Stones DH, Zarling AL, Willcox CR, Shabanowitz J, Cummings KL, et al. The Antigenic Identity of Human Class I MHC Phosphopeptides Is Critically Dependent Upon Phosphorylation Status. Oncotarget (2017) 8(33):54160–72. doi: 10.18632/oncotarget.16952
13. Haurum JS, Hoier IB, Arsequell G, Neisig A, Valencia G, Zeuthen J, et al. Presentation of Cytosolic Glycosylated Peptides by Human Class I Major Histocompatibility Complex Molecules In Vivo. J Exp Med (1999) 190(1):145–50. doi: 10.1084/jem.190.1.145
14. Kastrup IB, Stevanovic S, Arsequell G, Valencia G, Zeuthen J, Rammensee HG, et al. Lectin Purified Human Class I MHC-Derived Peptides: Evidence for Presentation of Glycopeptides In Vivo. Tissue Antigens (2000) 56(2):129–35. doi: 10.1034/j.1399-0039.2000.560203.x
15. Zarling AL, Ficarro SB, White FM, Shabanowitz J, Hunt DF, Engelhard VH. Phosphorylated Peptides Are Naturally Processed and Presented by Major Histocompatibility Complex Class I Molecules In Vivo. J Exp Med (2000) 192(12):1755–62. doi: 10.1084/jem.192.12.1755
16. Yague J, Vazquez J, Lopez de Castro JA. A Post-Translational Modification of Nuclear Proteins, N(G),N(G)-Dimethyl-Arg, Found in a Natural HLA Class I Peptide Ligand. Protein Sci (2000) 9(11):2210–7. doi: 10.1110/ps.9.11.2210
17. Mommen GP, Frese CK, Meiring HD, van Gaans-van den Brink J, de Jong AP, van Els CA, et al. Expanding the Detectable HLA Peptide Repertoire Using Electron-Transfer/Higher-Energy Collision Dissociation (EThcD). Proc Natl Acad Sci U S A (2014) 111(12):4507–12. doi: 10.1073/pnas.1321458111
18. Alpizar A, Marino F, Ramos-Fernandez A, Lombardia M, Jeko A, Pazos F, et al. A Molecular Basis for the Presentation of Phosphorylated Peptides by HLA-B Antigens. Mol Cell Proteomics (2017) 16(2):181–93. doi: 10.1074/mcp.M116.063800
19. Cobbold M, de la Pena H, Norris A, Polefrone JM, Qian J, English AM, et al. MHC Class I-Associated Phosphopeptides Are the Targets of Memory-Like Immunity in Leukemia. Sci Transl Med (2013) 5(203):203ra125. doi: 10.1126/scitranslmed.3006061
20. Hanahan D, Weinberg RA. Hallmarks of Cancer: The Next Generation. Cell (2011) 144(5):646–74. doi: 10.1016/j.cell.2011.02.013
21. Leney AC, El Atmioui D, Wu W, Ovaa H, Heck AJR. Elucidating Crosstalk Mechanisms Between Phosphorylation and O-GlcNAcylation. Proc Natl Acad Sci USA (2017) 114(35):E7255–61. doi: 10.1073/pnas.1620529114
22. de Queiroz RM, Carvalho E, Dias WB. O-GlcNAcylation: The Sweet Side of the Cancer. Front Oncol (2014) 4:132. doi: 10.3389/fonc.2014.00132
23. Purcell AW, Ramarathinam SH, Ternette N. Mass Spectrometry-Based Identification of MHC-Bound Peptides for Immunopeptidomics. Nat Protoc (2019) 14(6):1687–707. doi: 10.1038/s41596-019-0133-y
24. Demmers LC, Heck AJR, Wu W. Pre-Fractionation Extends But Also Creates a Bias in the Detectable HLA Class Iota Ligandome. J Proteome Res (2019) 18(4):1634–43. doi: 10.1021/acs.jproteome.8b00821
25. Marino F, Bern M, Mommen GPM, Leney AC, van Gaans-van den Brink JAM, Bonvin A, et al. Extended O-GlcNAc on HLA Class-I-Bound Peptides. J Am Chem Soc (2015) 137(34):10922–5. doi: 10.1021/jacs.5b06586
26. Malaker SA, Penny SA, Steadman LG, Myers PT, Loke JC, Raghavan M, et al. Identification of Glycopeptides as Posttranslationally Modified Neoantigens in Leukemia. Cancer Immunol Res (2017) 5(5):376–84. doi: 10.1158/2326-6066.CIR-16-0280
27. Demmers LC, Wu W, Heck AJR. HLA Class II Presentation Is Specifically Altered at Elevated Temperatures in the B-Lymphoblastic Cell Line Jy. Mol Cell Proteomics (2021) 20:100089. doi: 10.1016/j.mcpro.2021.100089
28. Barnstable CJ, Bodmer WF, Brown G, Galfre G, Milstein C, Williams AF, et al. Production of Monoclonal Antibodies to Group A Erythrocytes, HLA and Other Human Cell Surface Antigens-New Tools for Genetic Analysis. Cell (1978) 14(1):9–20. doi: 10.1016/0092-8674(78)90296-9
29. Yang H, Yang C, Sun T. Characterization of Glycopeptides Using a Stepped Higher-Energy C-Trap Dissociation Approach on a Hybrid Quadrupole Orbitrap. Rapid Commun Mass Spectrom (2018) 32(16):1353–62. doi: 10.1002/rcm.8191
30. Caval T, Zhu J, Tian W, Remmelzwaal S, Yang Z, Clausen H, et al. Targeted Analysis of Lysosomal Directed Proteins and Their Sites of Mannose-6-Phosphate Modification. Mol Cell Proteomics (2019) 18(1):16–27. doi: 10.1074/mcp.RA118.000967
31. Huang da W, Sherman BT, Lempicki RA. Systematic and Integrative Analysis of Large Gene Lists Using DAVID Bioinformatics Resources. Nat Protoc (2009) 4(1):44–57. doi: 10.1038/nprot.2008.211
32. Perez-Riverol Y, Csordas A, Bai J, Bernal-Llinares M, Hewapathirana S, Kundu DJ, et al. The PRIDE Database and Related Tools and Resources in 2019: Improving Support for Quantification Data. Nucleic Acids Res (2019) 47(D1):D442–50. doi: 10.1093/nar/gky1106
33. Chong C, Marino F, Pak H, Racle J, Daniel RT, Muller M, et al. High-Throughput and Sensitive Immunopeptidomics Platform Reveals Profound Interferongamma-Mediated Remodeling of the Human Leukocyte Antigen (HLA) Ligandome. Mol Cell Proteomics (2018) 17(3):533–48. doi: 10.1074/mcp.TIR117.000383
34. Ghosh M, Gauger M, Marcu A, Nelde A, Denk M, Schuster H, et al. Guidance Document: Validation of a High-Performance Liquid Chromatography-Tandem Mass Spectrometry Immunopeptidomics Assay for the Identification of HLA Class I Ligands Suitable for Pharmaceutical Therapies. Mol Cell Proteomics (2020) 19(3):432–43. doi: 10.1074/mcp.C119.001652
35. Bauza-Martinez J, Heck AJR, Wu W. HLA-B and Cysteinylated Ligands Distinguish the Antigen Presentation Landscape of Extracellular Vesicles. Commun Biol (2021) 4(1):825. doi: 10.1038/s42003-021-02364-y
36. Halim A, Westerlind U, Pett C, Schorlemer M, Ruetschi U, Brinkmalm G, et al. Assignment of Saccharide Identities Through Analysis of Oxonium Ion Fragmentation Profiles in LC-MS/MS of Glycopeptides. J Proteome Res (2014) 13(12):6024–32. doi: 10.1021/pr500898r
37. Wulff-Fuentes E, Berendt RR, Massman L, Danner L, Malard F, Vora J, et al. The Human O-GlcNAcome Database and Meta-Analysis. Sci Data (2021) 8(1):25. doi: 10.1038/s41597-021-00810-4
38. Hart GW. Nutrient Regulation of Signaling and Transcription. J Biol Chem (2019) 294(7):2211–31. doi: 10.1074/jbc.AW119.003226
39. Pathak S, Alonso J, Schimpl M, Rafie K, Blair DE, Borodkin VS, et al. The Active Site of O-GlcNAc Transferase Imposes Constraints on Substrate Sequence. Nat Struct Mol Biol (2015) 22(9):744–50. doi: 10.1038/nsmb.3063
40. Wang Z, Udeshi ND, Slawson C, Compton PD, Sakabe K, Cheung WD, et al. Extensive Crosstalk Between O-GlcNAcylation and Phosphorylation Regulates Cytokinesis. Sci Signal (2010) 3(104):ra2. doi: 10.1126/scisignal.2000526
41. Rigbolt KT, Prokhorova TA, Akimov V, Henningsen J, Johansen PT, Kratchmarova I, et al. System-Wide Temporal Characterization of the Proteome and Phosphoproteome of Human Embryonic Stem Cell Differentiation. Sci Signal (2011) 4(164):rs3. doi: 10.1126/scisignal.2001570
Keywords: O-GlcNAcylation modification, HLA, MHC, immunopeptidome, HLA-B*07:02, neo-antigen
Citation: Mukherjee S, Sanchez-Bernabeu A, Demmers LC, Wu W and Heck AJR (2021) The HLA Ligandome Comprises a Limited Repertoire of O-GlcNAcylated Antigens Preferentially Associated With HLA-B*07:02. Front. Immunol. 12:796584. doi: 10.3389/fimmu.2021.796584
Received: 17 October 2021; Accepted: 15 November 2021;
Published: 01 December 2021.
Edited by:
Parameswaran Ramakrishnan, Case Western Reserve University, United StatesReviewed by:
Anastasia Mpakali, National Centre of Scientific Research Demokritos, GreeceFrank Momburg, German Cancer Research Center (DKFZ), Germany
Patrick E Fields, University of Kansas Medical Center, United States
Copyright © 2021 Mukherjee, Sanchez-Bernabeu, Demmers, Wu and Heck. This is an open-access article distributed under the terms of the Creative Commons Attribution License (CC BY). The use, distribution or reproduction in other forums is permitted, provided the original author(s) and the copyright owner(s) are credited and that the original publication in this journal is cited, in accordance with accepted academic practice. No use, distribution or reproduction is permitted which does not comply with these terms.
*Correspondence: Albert J. R. Heck, a.j.r.heck@uu.nl
†These authors have contributed equally to this work