- 1Neuroimmunology and Multiple Sclerosis (MS) Research Section (NIMS), Department of Neurology, University and University Hospital Zurich, Zurich, Switzerland
- 2Department of Medical Oncology and Hematology, University and University Hospital Zurich, Zurich, Switzerland
Multiple sclerosis (MS) is an autoimmune disease of the central nervous system, in which autoreactive T and B cells play important roles. Other lymphocytes such as NK cells and innate-like T cells appear to be involved as well. To name a few examples, CD56bright NK cells were described as an immunoregulatory NK cell subset in MS while innate-like T cells in MS were described in brain lesions and with proinflammatory signatures. Autologous hematopoietic stem cell transplantation (aHSCT) is a procedure used to treat MS. This procedure includes hematopoietic stem/progenitor cell (HSPC) mobilization, then high-dose chemotherapy combined with anti-thymocyte globulin (ATG) and subsequent infusion of the patients own HSPCs to reconstitute a functional immune system. aHSCT inhibits MS disease activity very effectively and for long time, presumably due to elimination of autoreactive T cells. Here, we performed multidimensional flow cytometry experiments in peripheral blood lymphocytes of 27 MS patients before and after aHSCT to address its potential influence on NK and innate-like T cells. After aHSCT, the relative frequency and absolute numbers of CD56bright NK cells rise above pre-aHSCT levels while all studied innate-like T cell populations decrease. Hence, our data support an enhanced immune regulation by CD56bright NK cells and the efficient reduction of proinflammatory innate-like T cells by aHSCT in MS. These observations contribute to our current understanding of the immunological effects of aHSCT in MS.
Introduction
Over 2.5 million people worldwide are affected with multiple sclerosis (MS), a chronic, demyelinating disease of the central nervous system (CNS), which is usually diagnosed at the young age of 20-40 years. Autoimmune inflammation is considered the main pathomechanism, which results in demyelination, neurodegeneration, glia activation and metabolic changes, the latter being more pronounced in progressive MS forms (1). A large body of evidence supports the importance of T cells in the pathogenesis of MS, especially of CD4+ T cells (1). In addition, recent data have shown that B lymphocytes, as another adaptive immune cell type, also play a crucial role (2–4).
Besides adaptive immune cells, available data indicate that innate immune cells including innate lymphoid cells (ILCs) and innate-like T and B lymphocytes are involved in MS pathogenesis (5). Amongst ILCs, natural killer (NK) cells are most important to mention (5). NK cells are a source of immunoregulatory cytokines, they interact with other immune cells and can directly kill target cells. For instance, the killing of mutated tumour cells or virus-infected cells can be triggered via downregulation or absence of MHC (“missing self”), or also via antibody-dependent cellular cytotoxicity (ADCC) (6, 7). In the healthy condition, NK cell activation and killing is controlled by multiple NK receptors with a balance between activating receptors e.g. for Fc region of IgG leading to ADCC, and inhibitory receptors recognizing e.g. MHC, resulting in the attack of cells with low or absent MHC expression (6). Certain NK receptor variants are associated with decreased MS risk, highlighting the importance of NK cells and their receptors in MS pathophysiology (8). However, how NK cells are implicated in the pathophysiology of MS is not fully understood. Certain populations might enhance disease progression by harming the myelin sheath via ADCC, while others can have immunoregulatory effects like killing activated T cells (9).
In the innate-like lymphocyte compartment, cells that have been shown to be involved in MS are γδ T cells, NKT cells and mucosa-associated invariant T (MAIT) cells (5). All these innate-like T cells share certain features. Their T cell receptor (TCR) repertoire diversity is limited (10), they show antigen specificity against a restricted number of antigens, are not restricted by classical MHC molecules, mostly reside in barrier organs like mucosal tissues, and they are able to respond faster after activation compared to adaptive immune cells (11). Similar to conventional T cells, innate-like T cells mature in the thymus and undergo comparable positive and negative selection processes (12). Mechanisms by which they contribute to the inflammation in MS include the migration to the inflammatory lesions in the brain, the secretion of proinflammatory cytokines, thereby enhancing local inflammation, and possibly also cytotoxicity towards oligodendrocytes (5).
Despite enormous advances in the last 25 years, the treatments of MS are only partially effective and a fraction of MS patients still develops disability over time. Autologous hematopoietic stem cell transplantation (aHSCT) is a long-established procedure that, besides its broad use in haematological malignancies and as a rescue treatment after cancer chemotherapy, has been applied in the last 30 year for the treatment of autoimmune diseases. Among these, aHSCT is most frequently used for treating MS, and, based on rapidly growing data, it appears superior to even the most effective, approved therapies (13). The proposed mechanism(s) of action of aHSCT include, first, the depletion of autoreactive immune cells and the subsequent reconstitution of a “new” immune system from the re-infused autologous hematopoietic stem/progenitor cells (HSPCs) (13–18). Additionally, in the context of the profoundly lymphopenic environment shortly after the aHSCT, increased numbers and/or enhanced function of immunoregulatory cell populations have been described. Therefore, the generation of a “new” TCR repertoire and enhanced immune regulation are believed to be important aspects for the efficacy of aHSCT in MS (13–16).
Since innate immune cells have received relatively less attention than adaptive immune cells in the field of aHSCT in MS, we have studied here the effects of aHSCT on NK and innate-like T cells to assess their potential involvement in the beneficial effects of this treatment in MS.
Material and Methods
Patients and Samples
All MS patients eligible for transplantation were asked for written informed consent and included into the local aHSCT-in-MS registry. Ethic protocols for the collection of biomaterials and to study aHSCT are in place (BASEC-No. 2018-01854). Patients were treated with aHSCT because of (1.) failure of highly active disease-modifying therapy as evident by (2.a.) clinical activity (relapses), (2.b.) and/or radiological (new and/or contrast enhancing lesions) inflammatory activity, (2.c.) and/or clinical progression. Further limitations to receive aHSCT included (3.) age (18-55 years), (4.) disease duration (max. 15 years) and (5.) neurological disability status as measured by expanded disease status scale (EDSS; 2.0-6.5).
The conditioning regimen consisted of 4 g/m² cyclophosphamide and 30 Mio units/day granulocyte-colony stimulating factor (G-CSF, filgrastim). The graft containing the mobilized CD34+ HSPCs was collected by leukapheresis. The ablative conditioning followed the BEAM-ATG protocol. BEAM contains 300 mg/m² BCNU (carmustine) i.v. day -6, 200 mg/m² etoposide i.v. day -5 to -2, 200 mg/m² Ara-C (cytarabine) i.v. day -5 to -2, 140 mg/m² melphalan i.v. day -1. Day 0 is defined as the day of the re-infusion of the graft containing the autologous HSPCs (4-8 x 106 CD34+ cells/kg body weight). For in vivo depletion of residual T cells both in the graft, but also in the patient, rabbit anti-thymocyte globulin (ATG) was given on day 1 and 2 at 3.75 mg/kg i.v.
EDTA-anticoagulated blood was collected from MS patients before and at several time points after aHSCT (months 1, 3, 6, 12, 24). Additionally, excess material of the apheresis product for HSPC collection was examined. A schematic representation of the aHSCT procedure in MS and sampling times are depicted in Figure 1. Details about the 27 MS patients included in this cohort are included in Table 1. Blood from a cohort of 12 age- and sex-matched healthy controls (HCs) and 10 untreated relapsing remitting MS (RRMS) patients (Table 1), as well as three HC leukapheresis products without prior mobilization, and one anonymised buffy coat from the “Blood donation center Zurich SRK” were included as controls. Table 2 depicts selected statistics about the cohort. Peripheral blood mononuclear cells (PBMCs) were freshly isolated from these materials using Ficoll-Paque™ density gradient. PBMCs were then cryopreserved for 24-48h at -80°C prior to long-term storage in liquid nitrogen, so all PBMCs underwent one single freeze/thaw cycle.
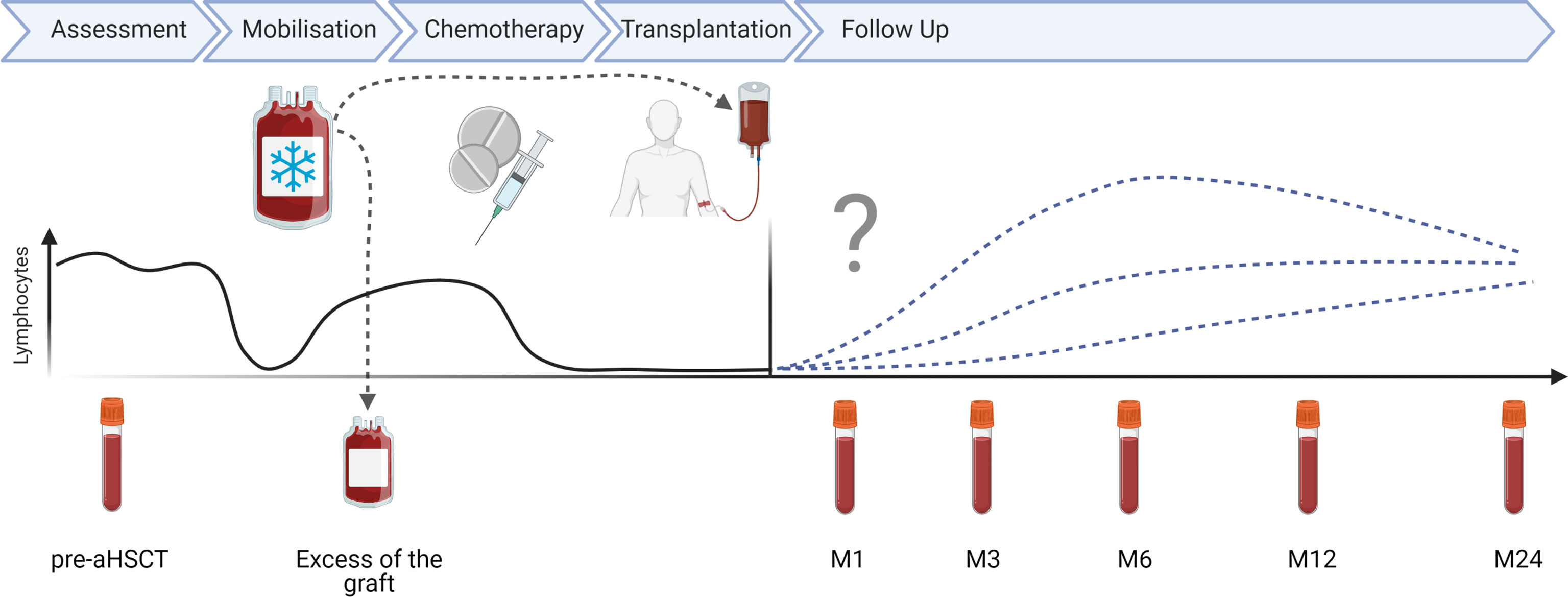
Figure 1 aHSCT in MS treatment scheme: schematic overview of aHSCT in MS procedure and sampling time points in months (M).
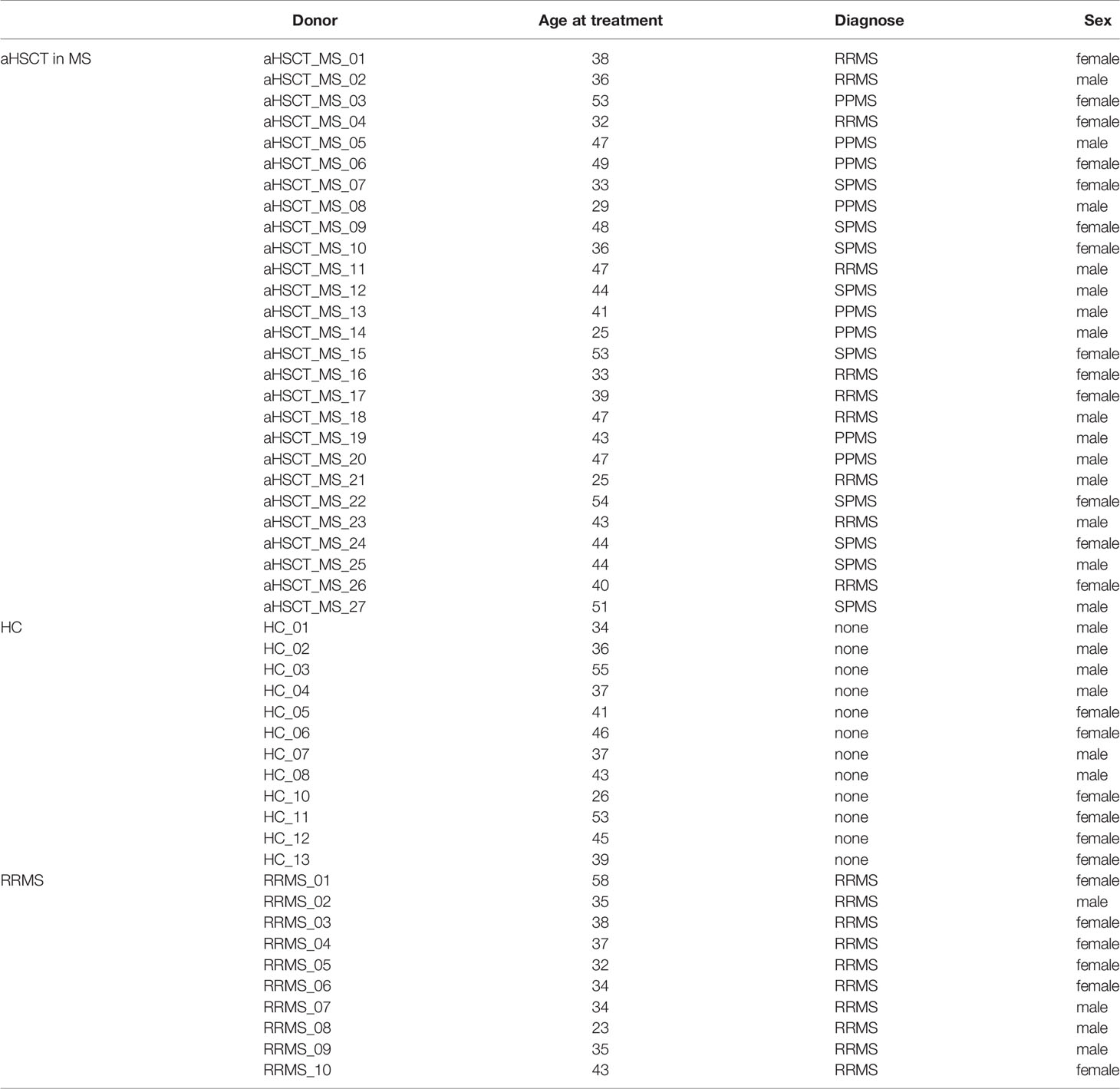
Table 1 Clinical and demographic characteristics of MS patients treated with aHSCT as well as healthy controls and RRMS disease controls.
Flow Cytometry
To immunophenotype the collected PBMCs, we developed three flow cytometry panels, a broad cell subset panel an NK and innate-like T cell panel and a CCR6 panel. For the broad cell subset panel and the CCR6 panel, samples from all 27 patients (pre = 26, M1 = 21, M3 = 19, M6 = 27, M12 = 25, M24 = 11), HC (= 12) and RRMS (= 10) were used, while 7 patients were used for the NK and innate-like T cell panel (patients no. 13, 17, 18, 19, 20, 24 and 26).
We used the following fluorophore-conjugated antibodies: anti-CD45 PerCP-Cy5.5, anti-CD3 BV786, anti-CD56 PE, anti-CD16 AF700, anti-Vα7.2 BV421, anti-Vδ2 BV711, anti-CD8 BV510 and anti-CCR6 BV785 (all from BioLegend®), anti-CD4 PE-Texas Red (Invitrogen™), anti-CD14 Pacific Blue, anti-TCRγδ FITC, anti-CD3 AF700 (all from BD) and anti-CD161 APC (Miltenyi Biotec). To exclude dead cells, we used the LIVE/DEAD™ fixable dyes Near-IR and Green (Invitrogen™). In brief, frozen PBMCs were thawed and counted. One million cells were first stained with the viability marker and blocked with purified human IgG (Sigma) and then stained with the respective surface markers. Samples were acquired with a BD LSR Fortessa flow cytometer and analysed using the software FlowJo (FlowJo LLC). Gating is shown in Supplementary Figures 1–3. Statistical tests included first a global test (ANOVA or Kruskal-Wallis) and in case of significance followed by pairwise comparisons (t-test or Wilcoxon). Post-aHSCT time points were usually compared to HC group whenever present, otherwise the pre-aHSCT time point was the reference group. Significance levels were ns for p > 0.05, * for p <= 0.05, ** for p <= 0.01, *** for p <= 0.001 and **** for p <= 0.0001. Statistical analysis as well as visualizations were performed in R Core Team (2020).
Results
To characterize NK and innate-like T cells before and after aHSCT in MS patients, three flow cytometry panels were used. For an overview of the studied NK and innate-like T cell populations and their nomenclature see Figure 2. We are aware that this is a simplification. Nevertheless, it should help understanding the variety of innate immune cells that we studied and their potential role in aHSCT.
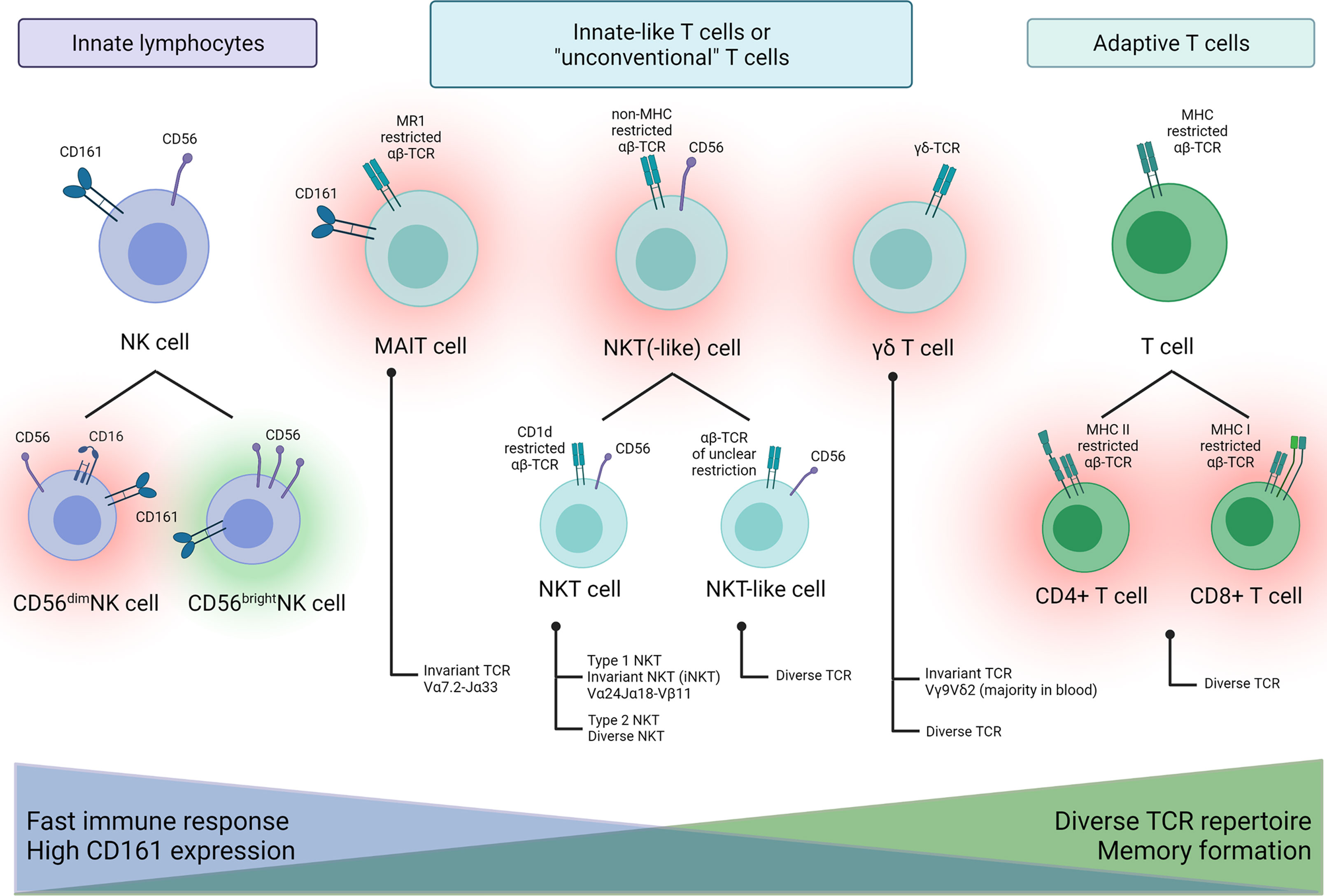
Figure 2 Schematic overview of NK and innate-like T cell populations characterized in this study and their possible proinflammatory (red halo) or beneficial (green halo) role in MS.
Within the hematopoietic system, the surface molecule CD56, also known as neural cell adhesion molecule (NCAM), in the absence of CD3 usually identifies NK cells (19). The degree of CD56 expression on NK cells subdivides them into a CD56bright and a CD56dim population. We first analysed PBMCs with a broad cell subset panel for major leucocyte subsets. Among NK cells, we differentiated CD56bright and CD56dim NK cells. Interestingly, the percentage of NK cells in the graft was not affected to a great extent by the mobilization regimen of cyclophosphamide and G-CSF (Figure 3A). However, compared to the matched pre-aHSCT sample, CD56bright NK cells and CD56+CD3+ cells are slightly decreased (Figure 3B).
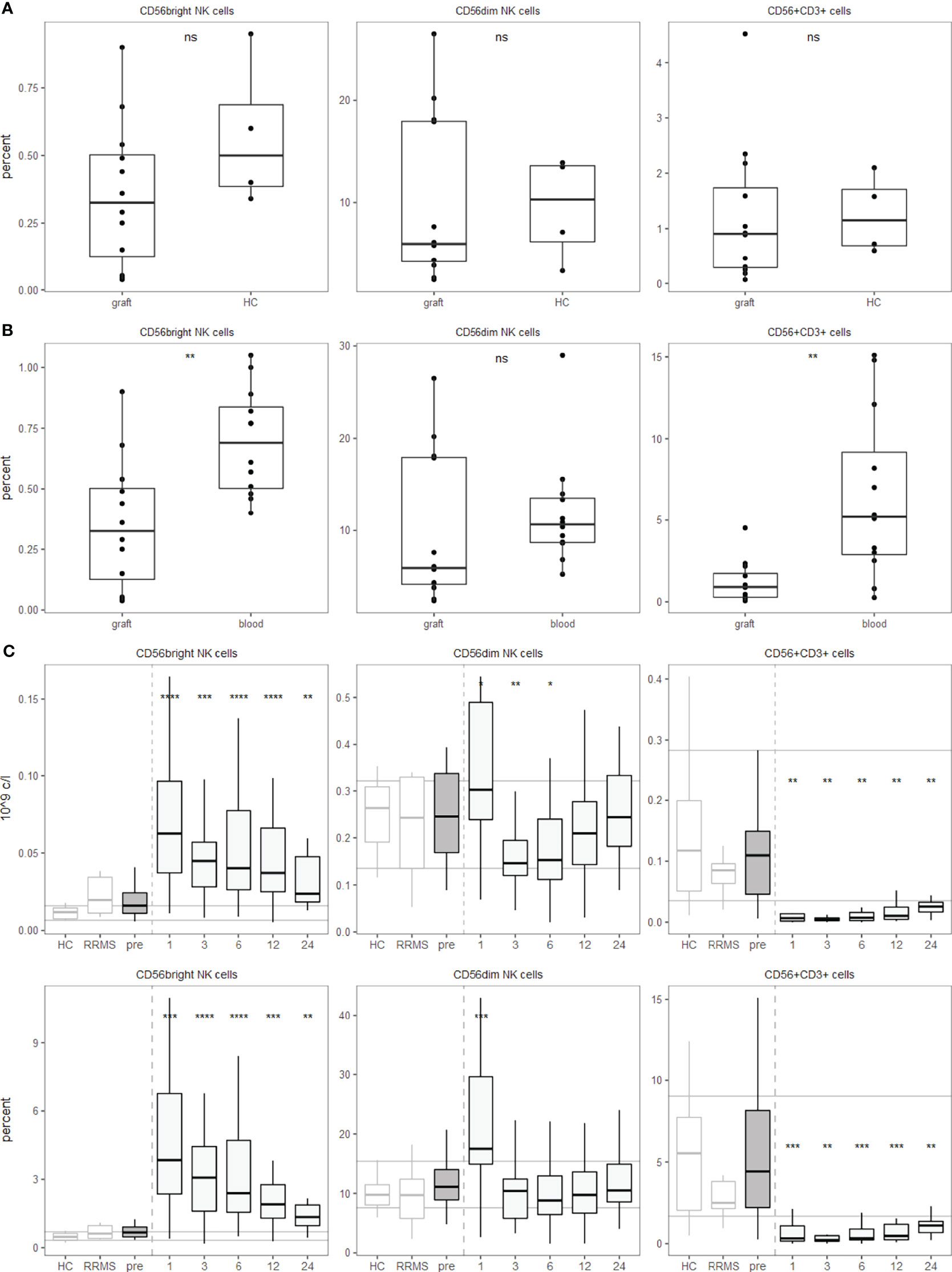
Figure 3 aHSCT but not the mobilization regimen is followed by a shift from CD56dim towards CD56bright NK cells and a strong decrease in innate-like CD56+CD3+ T cells. PBMCs were stained with surface fluorophore-conjugated antibodies and analyzed by flow cytometry. Percentages are fractions of total PBMCs and absolute cell numbers were calculated from lymphocyte numbers measured in clinical routine. (A) NK and innate-like T cell percentages in the graft after the mobilization regimen, but before aHSCT compared to three leukapheresis and one buffy coat. Number of samples were graft = 12, HC =4. (B) NK and innate-like T cell percentages in the graft after the mobilization regimen, but before aHSCT compared to the pre-aHSCT PBMCs of the respective patients. Number of samples were graft = 12, blood = 12. (C) Absolute (top) and relative (bottom) NK and innate-like T cell dynamics pre- and post-aHSCT. Horizontal lines reflect the median and 10th/90th percentile of age- and sex-matched HCs. Number of samples were pre = 26, M1 = 21, M3 = 19, M6 = 27, M12 = 25, M24 = 11, HC = 12, RRMS = 10. As a global test, ANOVA was used, and in case of significance followed by pairwise comparisons (HC vs 1, 3, 6, 12 and 24 months) using t-test.
After BEAM-ATG and the transplantation, we observed a significant increase in the abundance of CD56bright NK cells after aHSCT, while CD56dim NK cells peaked one month post-aHSCT, then declined with slow recovery over the two years of observation (Figure 3C). Interestingly, older individuals and progressive patients recovered with higher percentages of NK cells of both CD56bright and CD56dim phenotype (Supplementary Figures 4A, B).
Expression of CD56 is not limited to NK cells, but also observed on certain T cells. A subset of myelin-reactive CD4+ T cells from MS patients expresses CD56 and can lyse target cells expressing CD56 by homotypic interactions (20). This mechanism could be involved in oligodendrocyte damage (21). In αβ T cells, expression of CD56 is mostly associated with CD8+ T cells, but CD4+ T cells as well as γδ T cells can also express this marker (19). Hence, CD3+CD56+ double positive cells are a population containing several innate-like T cell populations, but are not entirely composed of them. Most importantly, bona fide αβ TCR expressing activated myelin-specific CD4+ T cells can express CD56 (20). Interestingly, we saw a very efficient and highly significant depletion of CD56+CD3+ double positive cells below pre-aHSCT and HC levels for at least two years (Figure 3C), and this depletion was not observed to this extent after the mobilization regimen (Figures 3A, B).
These findings, together with studies showing an involvement of NK and innate-like T cells in MS, led us to examine these populations in the setting of aHSCT in MS. With a panel that allowed us to differentiate innate-like T cells like MAIT cells, NKT(-like) cells and γδ T cells from the innate NK cell subpopulations, we studied their immune reconstitution in MS patients post-aHSCT in seven individuals of our cohort.
We used CD3 in combination with the invariant TCR Vα7.2 to identify MAIT cells, the γδ TCR to identify γδ T cells and exclusion of these TCRs and positivity for CD56 and CD3 to identify NKT and NKT-like cells. NKT cells are identified by the restriction of their αβ TCR to CD1d, but beyond that, specific markers for NKT cells are lacking (22). For this reason, co-expression of CD3 and CD56 as NK cell marker are often used for their identification. The analysis of MAIT cells, γδ T cells and NKT(-like) cells showed that all of them decreased after aHSCT and remained depleted for at least one year (Figure 4A). To further examine innate-like T cells and their capacity to enter specific tissues, we studied tissue-homing receptors. First, we focused on the tissue-homing receptor CD161, which is expressed on NK cells but also on several T cell subsets (23). We found a lower percentage of innate-like T cells expressing CD161 post-aHSCT than pre-aHSCT (Figure 4B).
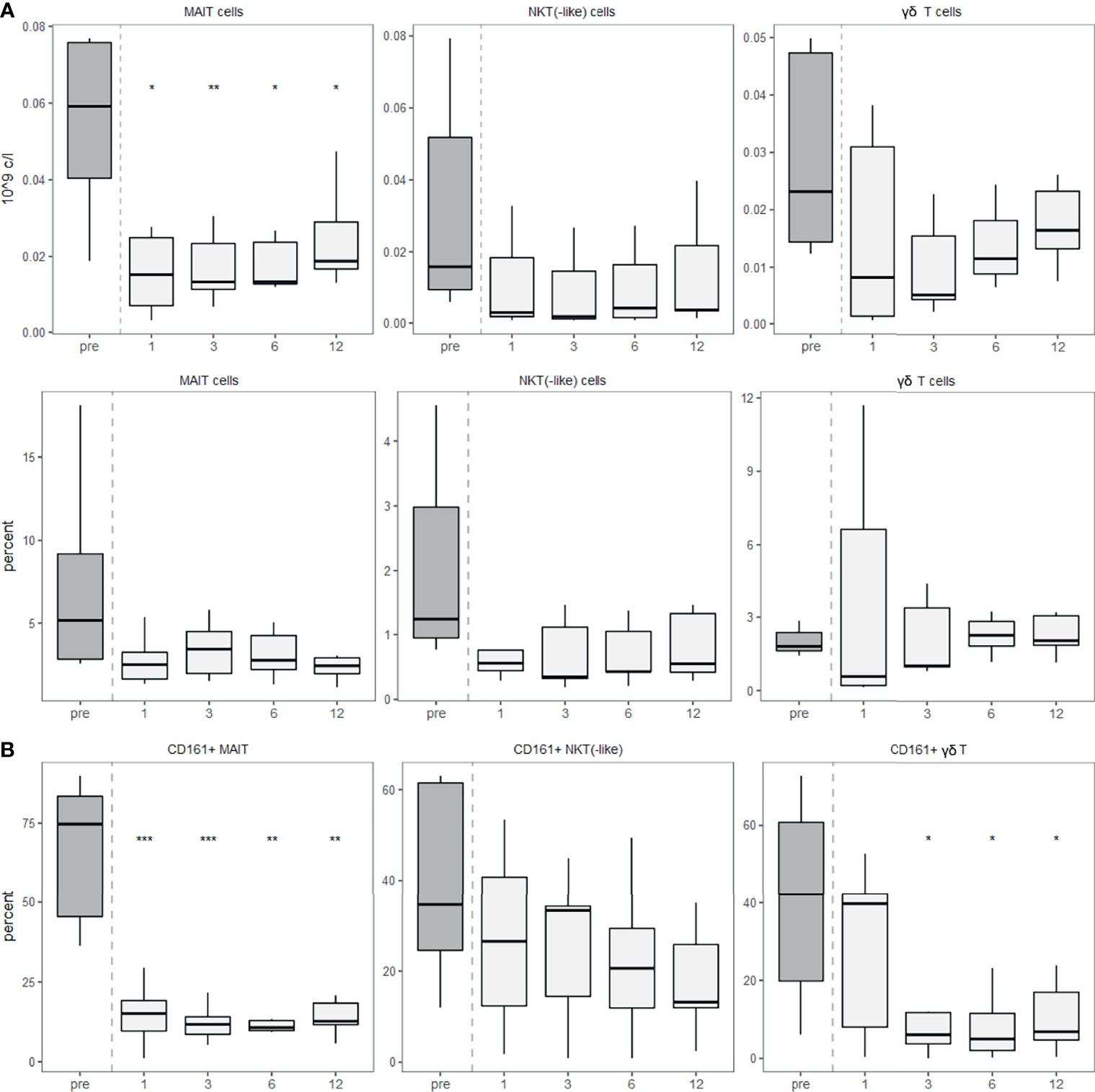
Figure 4 Innate-like T cells recover slowly after aHSCT in MS and show a lower expression of CD161. PBMCs were stained with fluorophore-conjugated surface antibodies and analyzed by flow cytometry. Percentages are fractions of total lymphocytes and absolute cell numbers were calculated from lymphocyte numbers measured in clinical routine. (A) Absolute (top) and relative (bottom) recovery of MAIT, NKT(-like) and γδ T cells post-aHSCT in MS. (B) Percentage of CD161-expressing cells within MAIT cells (left), percentage of CD161-expressing cells within NKT(-like) cells (middle) and percentage of CD161-expressing cells within γδ T cells (right). Number of samples were pre = 7, M1 = 7, M3 = 7, M6 = 7, M12 = 7. As a global test, Kruskal-Wallis was used, and in case of significance followed by pairwise comparisons (pre vs 1, 3, 6 and 12 months) using Wilcoxon signed-rank test.
Furthermore, we investigated the tissue-homing receptor CCR6 since its ligand CCL20 (also known as MIP-3α) is abundantly expressed in the gastrointestinal tract (24), where many innate-like T cells preferentially reside. We quantified the expression of CCR6 on T cells before and after aHSCT. CCR6 expression was reduced on CD8+ T cells after aHSCT, while CD4+ T cells expressed similar levels as healthy controls (Figure 5A). Absolute numbers of CD8+ CCR6+ T cells were decreased over a prolonged period of at least two years, while CD4+ CCR6+ T cells dropped only transiently and then recovered (Figure 5B).
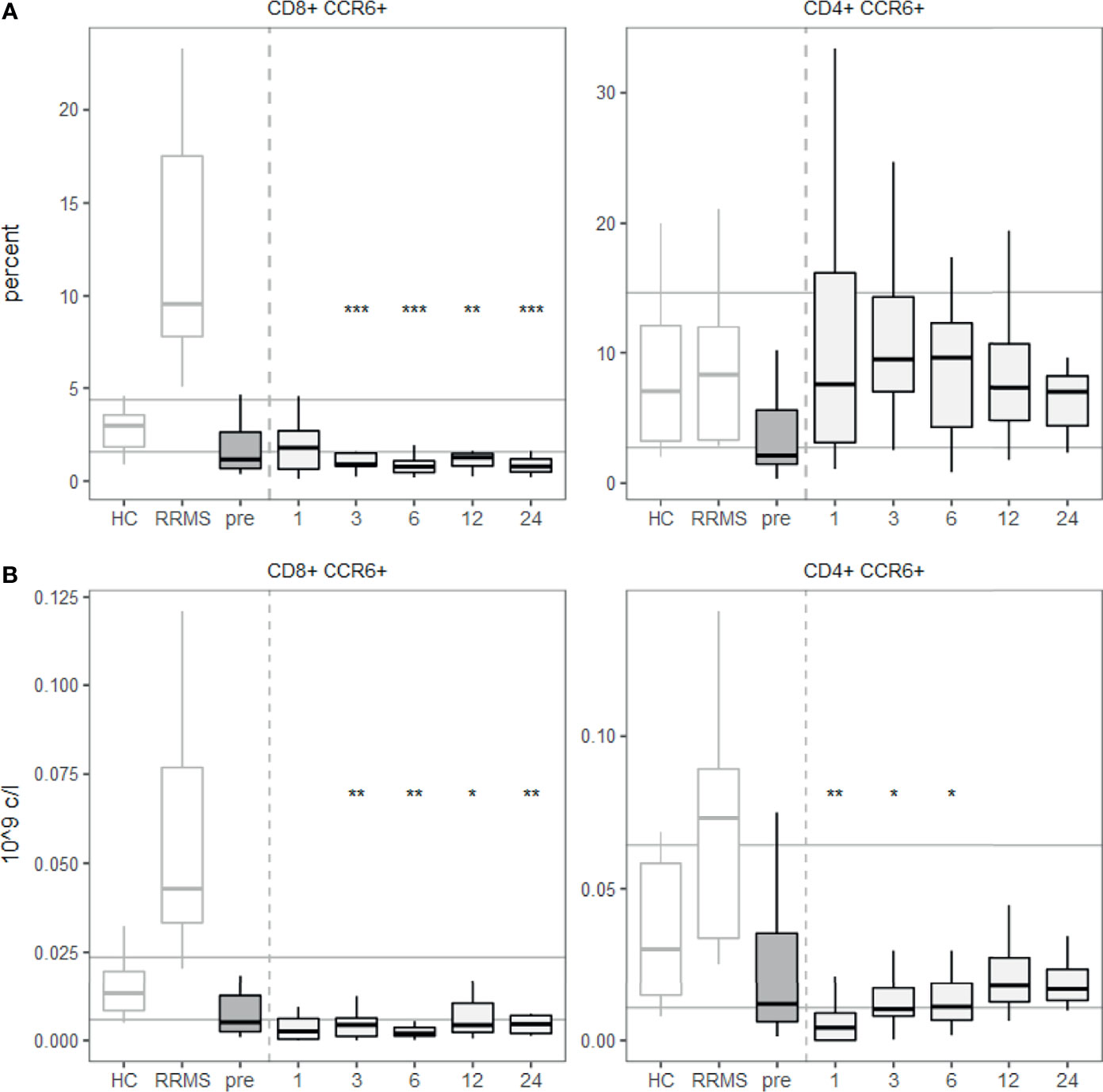
Figure 5 A lower percentage of CD8+ T cells express CCR6 from 3 months post aHSCT onwards. PBMCs were stained with fluorophore-conjugated surface antibodies and analyzed by flow cytometry. (A) Percentages are fractions of CD8+ (left) or CD4+ (right) T cells respectively. (B) Absolute number of CD8+ CCR6+ T cells (left) and CD4+ CCR6+ T cells (right). Number of samples were pre = 26, M1 = 21, M3 = 19, M6 = 27, M12 = 25, M24 = 11, HC = 12, RRMS = 10. As a global test, ANOVA was used, and in case of significance followed by pairwise comparisons (pre vs 1, 3, 6 and 12 months) using t-test.
Although γδ T cells could theoretically have a higher diversity than the αβ TCR repertoire (25), the diversity of the γδ TCR repertoire is usually smaller and shows use of invariant TCRs similar to other innate-like T cells including MAIT and NKT cells (26). The most commonly used V gene in the blood of humans is the Vδ2 chain, usually pairing with the Vγ9 (26). By staining for this TCR variable chain, we detected a strongly decreased Vδ2 usage post-aHSCT. While both Vδ2+ and Vδ2- populations expressed less CD161 after the transplantation, this effect was significant in the Vδ2+ cells (Figure 6A). As expected, the absolute number of Vδ2+ γδ T cells as well as CD161+ Vδ2+ γδ T cells and CD161+ Vδ2- γδ T cells decreased after aHSCT (Figure 6B).
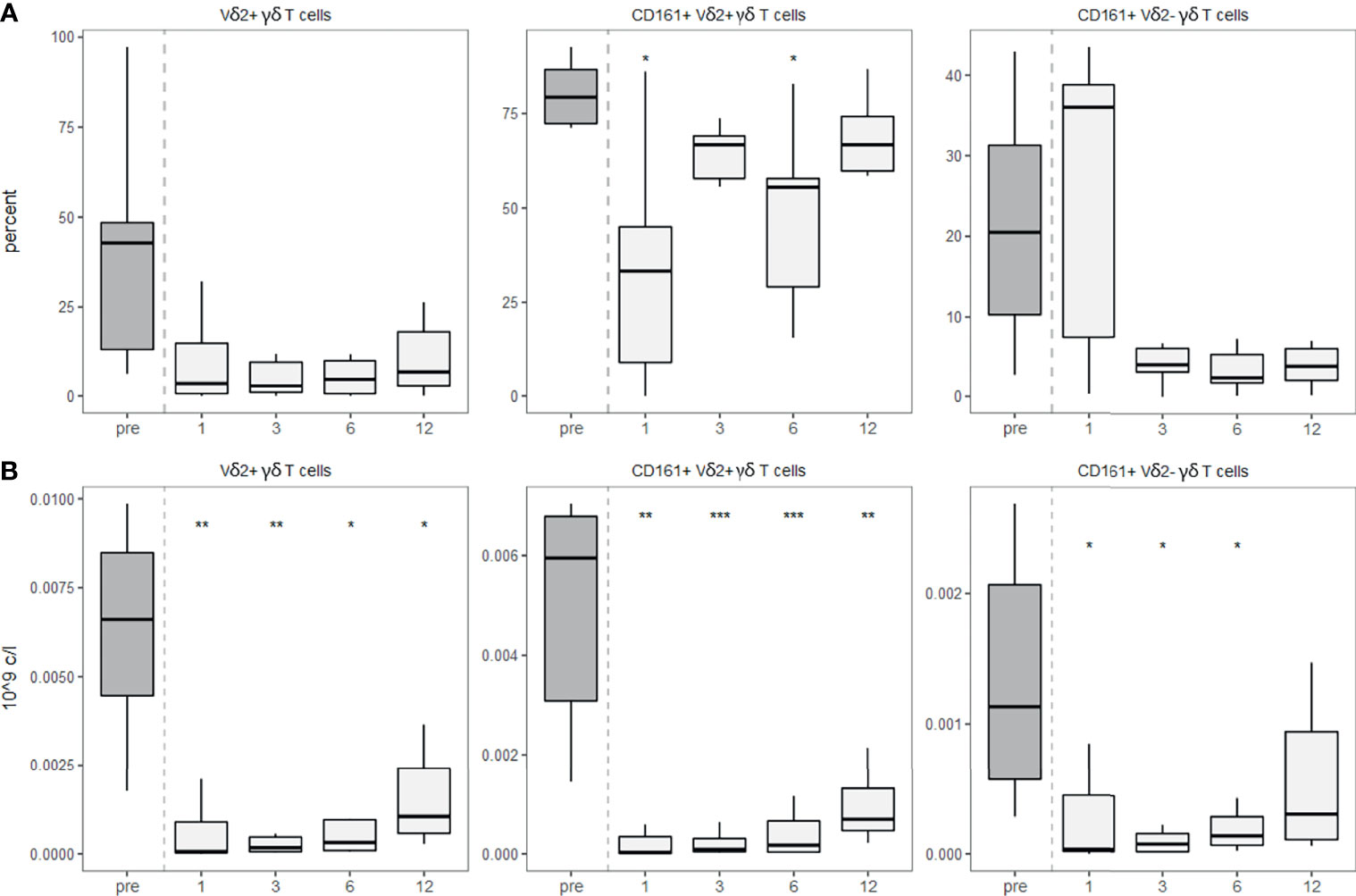
Figure 6 γδ T cells show decreased usage of Vδ2 after aHSCT in MS. PBMCs were stained with fluorophore-conjugated surface antibodies and analyzed by flow cytometry. (A) Percentage of Vδ2 fraction of γδ T cells (left), percentage of CD161+ cells within Vδ2+ γδ T cells (middle), percentage of CD161+ cells within Vδ2- γδ T cells (right). (B) Absolute numbers of Vδ2+ γδ T cells (right), absolute number of CD161+ Vδ2+ γδ T cells (middle), absolute number of CD161+ Vδ2- γδ T cells. Number of samples were pre = 7, M1 = 7, M3 = 7, M6 = 7, M12 = 7. As a global test, Kruskal-Wallis was used, and in case of significance followed by pairwise comparisons (pre vs 1, 3, 6 and 12 months) using Wilcoxon signed-rank test.
Finally, we also analysed NK cells in more detail by staining for CD16 and the expression of CD161 on the different NK cell subsets. These data largely are in line with the results shown in Figure 1 (Figure 7A). We detected a slight decrease in the percentage of CD56bright NK cells expressing CD161 one year after the aHSCT. Interestingly, one month post-aHSCT, the CD56dim cells showed a significant decrease in CD161 expression, while CD56-CD16+ NK cells did not change at month 12 after transient increases in the prior months (Figure 7B).
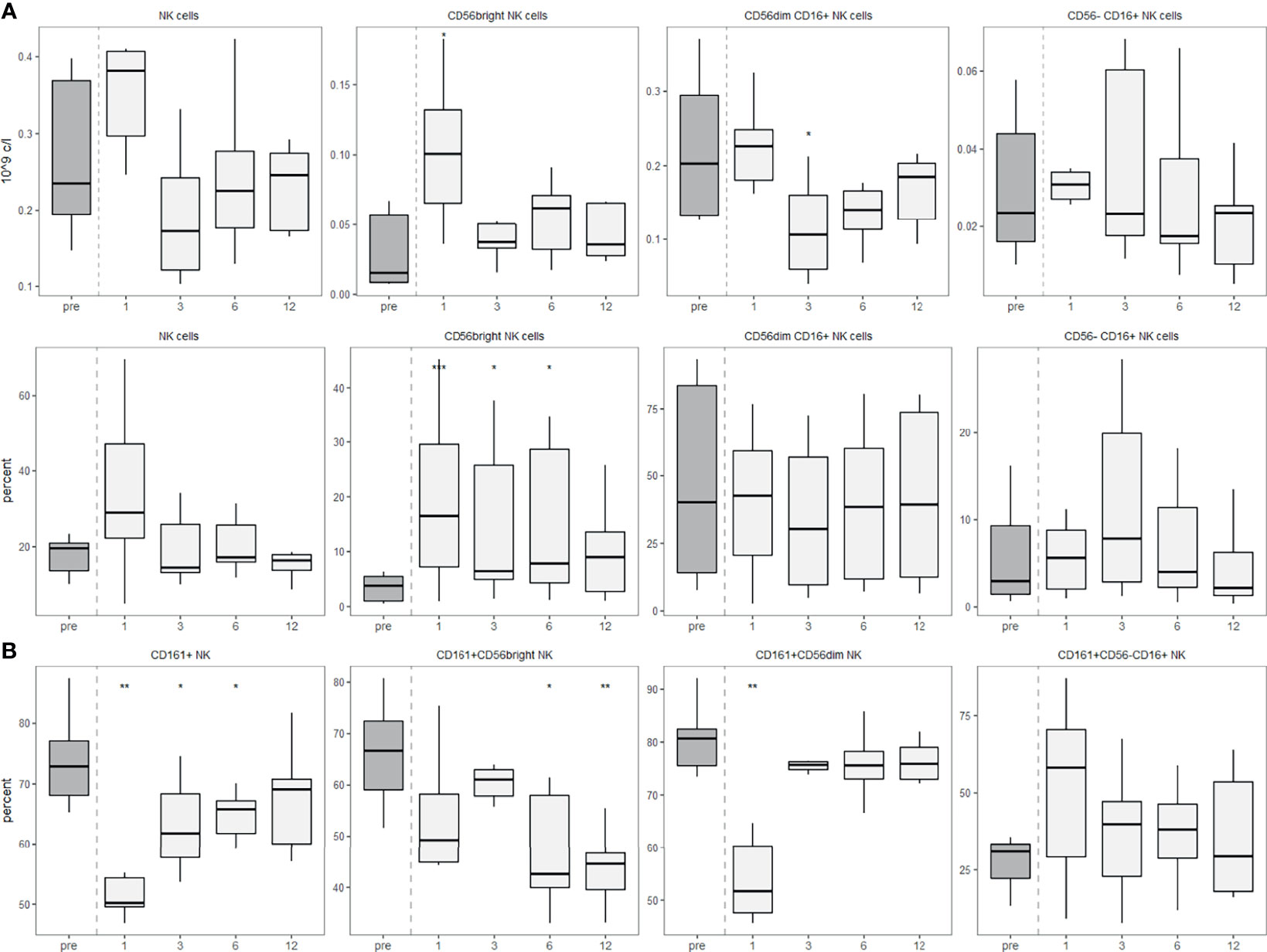
Figure 7 CD56dim NK cells express permanently less surface CD161 after aHSCT in MS. PBMCs were stained with fluorophore-conjugated surface antibodies and analyzed by flow cytometry. (A) Absolute (top) and relative (bottom) recovery of NK cells (left, percentage of lymphocytes) and CD56bright, CD56dim CD16+ and CD56-CD16+ NK cell subsets (percentage of NK cells). (B) Expression of CD161 by all NK cells (left) and their subpopulations. Number of samples were pre = 7, M1 = 7, M3 = 7, M6 = 7, M12 = 7. As a global test, Kruskal-Wallis was used, and in case of significance followed by pairwise comparisons (pre vs 1, 3, 6 and 12 months) using Wilcoxon signed-rank test.
Discussion
In this study we describe the immune reconstitution dynamics of NK and innate-like T cells after aHSCT in MS patients. With the help of our broad cell subset panel, we found a strong increase in CD56bright NK cells, while CD56dim NK cells had an overshoot at one month post-aHSCT and then a drop below HC levels. The different functions and roles of the CD56bright and CD56dim subsets have been discussed previously. Some authors stress the role of CD56 as an activation marker (19), while others focus on its differential expression during NK cell differentiation (27, 28). In detail, CD56bright NK cells are thought to be the least differentiated and “youngest” NK cell subset and mount responses mostly, but not entirely via producing cytokines. Subsequently, they can mature into CD56dim NK cells, which mediate their lytic activity following NK receptor-mediated activation (29). CD56bright NK cells show immunoregulatory functions like cytokine secretion with low cytotoxicity, while CD56dim cells usually express CD16 (Fcγ receptor III), have predominantly cytotoxic functions and can lyse, amongst others, activated T cells (19, 27, 28, 30). Moreover, there is a NK cell subset lacking CD56 expression while being positive for CD16. These CD56-CD16+ NK cells seem to be similar to the CD56dim NK cells, at least in a proteomics-focussed analysis (31).
The role of NK cells in MS is not clear. A dysregulation of CD56bright NK cells has been reported for MS patients (32, 33). The treatment of MS with the anti-CD25 (IL-2Rα) monoclonal antibody daclizumab identified the expansion of immunoregulatory CD56bright NK cells and the latter effectively blocked disease activity (30, 34, 35). Subsequent studies showed that this cell population is likely affected by other MS treatments as well (30, 36–40). The mechanism(s) of action of CD56bright NK cells in MS includes a dysregulation in their ability to lyse activated, but not resting CD4+ T cells (30, 39). This dysregulation is attributable to an impairment in the DNAM-1 interaction of NK cells with CD155 on T cells (39). IL-2 can stimulate NK cells to lyse T cells, which might be the mechanism by which daclizumab restores this impairment (30, 38, 41). CD56bright NK cells were also reported to be increased after aHSCT in MS (42–44). On the other hand, myelin damage in MS might partially be driven by ADCC via Fcγ-Receptors present mostly on CD56dim NK cells (45), which could indicate a pathogenic role of CD56dim NK cells in MS. Furthermore, a previous study observing NK cells in aHSCT in MS demonstrated a negative correlation of NK cells with Th1 and Th17 cells (44). They depleted post-aHSCT PBMCs of CD56+ NK cells ex vivo, stimulated them and analysed the Th1 and Th17 response, and reported increased Th1 and Th17 responses in case of NK cell depletion (44), presumably due to NKG2D-mediated killing of T cells in the presence of NK cells (44, 46). Our data shows that CD56bright NK cells are significantly increased, while CD56dim NK cells are stable/decreased except for one month post-aHSCT. Moreover, NK cells were shown to be more sensitive to clinically relevant concentrations of ATG than T cells in vitro (47). Therefore, “old” cytotoxic CD56dim NK cells are likely to be depleted by the aHSCT, and afterwards “new” immunomodulatory CD56bright NK cells emerge and predominate, an outcome that might positively influence a pathological NK cell-related disturbance in MS. In one study, the cytotoxicity of NK cells after high dose chemotherapy (BECH or BEAC protocol) was decreased in patients with non-Hodgkins’s lymphoma (48), indicating that this could be part of the immunological effects of aHSCT in MS.
Another interesting population are CD161+ NK cells. Prior data showed that CD161 expression on NK cells is associated with IFNγ production (49) but also with inhibition of cytolytic activity (50), which might allow NK cells to support a proinflammatory environment while reducing their lytic activity towards e.g. autoreactive CD4+ T cells. Our observation of lower expression of CD161 in NK cells post-aHSCT might therefore indicate a beneficial reduction in the secretion of proinflammatory cytokines together with an increased potential to kill autoreactive T cells.
To interpret our results on the innate-like T cells, it is important to consider their functions and properties in healthy individuals as well as their role in MS, or more specifically in the context of aHSCT in MS. Here, we give a very brief profile of the studied innate-like T cells. Figure 2 attempts to depict the different roles of these cells graphically.
NKT cells preferentially reside in the colon (51) and the liver (52). An important subpopulation of NKT cells carry the invariant TCR chains Vα24-Jα18 and Vβ11 that recognize α-galactosylceramide (αGC) and are referred to as type I, classical NKT or invariant NKT (iNKT) cells. NKT cells with other TCRs are called type II or non-classical (22). Since not only NKT cells but also classical T cells can express CD56 (53), the non-CD1d-restricted CD3+CD56+ double positive population is referred to as NKT-like (22). The name “NKT-like cells” reflects the heterogeneity of this non-CD1d restricted CD56+CD3+ population, containing conventional T cells (e.g. CD56+CD4+ autoreactive and HLA class II-restricted T cells as described by Vergelli and colleagues (20)), MAIT cells and γδ T cells. MAIT cells are commonly defined as T cells expressing the invariant TCR chain Vα7.2 recognizing a riboflavin-derivative presented by a molecule called MHC-related 1 (MR1) (54). As their name already suggests, they are enriched in the mucosa of organs like the gut (54). There, they might be involved in the sensing of microbiota, providing barrier protection and the initiation of tissue repair responses (55). While both NKT as well as MAIT cells express an αβ TCR, there is another T cell subset expressing a γδ TCR. This population is characterized by their early activation following stimulation with conserved stress-induced ligands and is greatly enriched in epithelial tissues like the skin and the gastrointestinal tract (26), but also in the liver (52).
The role of these innate-like T cell populations in MS remains to be studied in more detail. We describe part of the evidence suggesting an involvement in the pathophysiology of MS. Innate-like T cells might directly contribute to the demyelination in the CNS, since the heterogeneous population of CD56+CD4+ T cells but also γδ T cells were reported to lyse oligodendrocytes in MS (56, 57). The local role of innate-like T cells in the CNS is further supported by the presence of MAIT cells (58), and clonally expanded γδ T cells (59, 60) within the CNS of MS patients. Especially the γδ T cells using the Vδ2 chain showed higher expression of CD161 in MS, which was associated with better transendothelial migration (61). Hence, the decreased usage of the Vδ2 chain likely represents the reduction of a γδ T cell subpopulation that seems more prone for CNS infiltration. Moreover, innate-like T cells might contribute to a proinflammatory environment by the secretion of Th1/Th17 cytokines as described for iNKT cells (62) and MAIT cells (63). Notably, iNKT cells are able to recognize a lipid called α-GC, and also polyacetylated GC, a derivative of GC present in the myelin sheath (64, 65). In MS, iNKTs were found to be hyporesponsive towards these myelin antigens (64, 65). The authors hypothesize that this is a consequence of their stimulation and subsequent anergy, possibly as a consequence of prior myelin destruction (66). Hence, most findings suggest that innate-like T cells are probably drivers of the disease and their efficient reduction after the transplantation may contribute to the treatment effects of aHSCT in MS.
There are only limited data about the influence of cyclophosphamide, BEAM or ATG on innate-like T cells. Nevertheless, there is one study that showed a faster recovery of MAIT cells than we describe here after various myeloablative regimens in haematological malignancies (67), indicating that ATG possibly has not only a cytotoxic effect on NK cells but also on MAIT cells.
NKR-P1A, also referred to as CD161, is a homodimer belonging to the C-type lectin family and is expressed by most NK cells (68). CD161 expression on T cells can be inhibitory, stimulatory, or act as a survival signal (23). Furthermore, it has been associated with tissue homing, cytotoxicity and a memory phenotype (23). CD161 expression distinguishes conventional and innate-like T cells, with low expression on conventional T cells and high expression on innate-like T cells. In other words, CD161 has been discussed as a marker of “innateness” (69). Interestingly, MAIT cells greatly overlap with CD161+ CD8+ cells and mostly show a Th17 cytokine profile (70, 71). In MS, CD161 expression on CD8+ T cells has been reported to be increased (72). In line with that, a proinflammatory IL-17-producing CD161+CD8+ T cell population was reported to be decreased after aHSCT in MS (42, 43). The authors mention this reduction of MAIT cells, however, one has to consider that immature MAIT cells mostly do not express CD161 (73). Hence, our observation of a reduced CD161 expression on innate-like T cells post-aHSCT could indicate the normalization of surface CD161 towards a physiological level or even a beneficial decrease below that. Functionally, this might point at a lower CNS-infiltrating capacity, reduced cytotoxicity (e.g. against oligodendrocytes), a more immature or adaptive cell population.
Finally, we studied CCR6 expression on CD3+ T cells and found less CD8+ T cells expressing it post-aHSCT. CCR6+ CD4+ T cells are mostly associated with the Th17 phenotype, while the CCR6+ CD8+ T cell phenotype is less well characterised (74). CCR6 is preferentially expressed by memory T cells (75), and drives mostly the migration of CD8+ T cells and NKT cells towards CCL20, while it is a less potent migration stimulus for CD4+ T cells (53). The decreased surface expression of CCR6 on CD8+ T cells post-aHSCT might point towards a reduced recruitment of bona fide CD8+ T cells and CD8+ expressing NKT cells to CCL20 producing tissues like the intestines. Whether the potentially reduced tissue homing to the gastro-intestinal tract (GIT) post-aHSCT is relevant for post-aHSCT outcome remains to be clarified. Furthermore, lymphocyte numbers might already be reduced in the GIT because of the strong reduction in the abundance of innate-like T cells. In this regard it might be also important to take into account the reported microbiota dysregulation in MS (76), though the precise interplay between lymphocytes and microbiota in MS pathophysiology remains to be elucidated in more detail.
Our results indicate that effects beyond the renewal of adaptive immune cells, namely beyond CD4+ and CD8+ T cells and B cells, may contribute to the excellent treatment effects of aHSCT in MS. Regarding the mechanism(s) of action of aHSCT in MS, the increased abundance of regulatory CD56bright NK cells, a long-term reduction of cytotoxic CD56dim NK cells combined with a reduction of proinflammatory innate-like T cell populations appear to play a role. Moreover, phenotypic changes such as a lower surface expression of tissue-homing receptors in innate-like T cells or a diminished usage of the Vδ2 chain in γδ T cells might add to the inhibitory effects on disease activity in MS.
Data Availability Statement
The raw data supporting the conclusions of this article will be made available by the authors, without undue reservation.
Ethics Statement
The studies involving human participants were reviewed and approved by Kantonale Ethikkommission Zürich. The patients/participants provided their written informed consent to participate in this study. Written informed consent was obtained from the individual(s) for the publication of any potentially identifiable images or data included in this article.
Author Contributions
JRu: Conceptualization, establishment of techniques, performing experiments, data curation, data analysis and interpretation, writing - first draft, revision and editing. JRe: Isolation of biomaterials, editing. SO: Data acquisition, editing. MD: Establishment of techniques, editing. AM: Investigation, clinical aspects, editing. US: Investigation, clinical aspects, editing. IJ: Investigation, clinical aspects, editing. RM: Conceptualization, data interpretation, funding acquisition, validation, writing – review and editing. All authors contributed to the article and approved the submitted version.
Funding
This study was supported by a Swiss National Science Foundation (SNF) grant (323530_183985) for MD/PhD students awarded to JRu, and SNF grant (32003B_185003) to RM.
Conflict of Interest
RM received unrestricted grant support from Biogen, Novartis, Hoffman La Roche and Third Rock, and compensation for advice or lecturing by Biogen, Novartis, Sanofi Genzyme, Merck, Hoffmann La Roche, Neuway, CellProtect, and Abata. RM is employed part-time by Cellerys, a startup company outfounded from the University of Zurich. He is a co-founder and stockholder of Cellerys and a co-founder of Abata Therapeutics. RM is listed as an inventor on patents of the University of Zurich about target antigens in multiple sclerosis. RM is further listed as inventor and received remuneration for a NIH-held patent on the use of daclizumab to treat multiple sclerosis. None of which has impact on the submitted work.
The remaining authors declare that the research was conducted in the absence of any commercial or financial relationships that could be construed as a potential conflict of interest.
Publisher’s Note
All claims expressed in this article are solely those of the authors and do not necessarily represent those of their affiliated organizations, or those of the publisher, the editors and the reviewers. Any product that may be evaluated in this article, or claim that may be made by its manufacturer, is not guaranteed or endorsed by the publisher.
Acknowledgments
We thank M. Mikolin, M. Morax and Z. Marti (NIMS) for processing blood samples and/or technical support, members of the clinical and laboratory teams at NIMS, M. Manz (Hematology Department, University Hospital Zurich) for performing leukaphereses, A. Thesenvitz (NIMS) and K. Ritter (Hematology Department) for contacting patients and collecting blood samples, and the staff of the MS Outpatient Clinic and Day Hospital, Neurology Clinic, as well as the staff of the Transplant Unit, Hematology Department, for participation in patient care-related aspects of the study. Figures 1, 2 were created with BioRender.com.
Supplementary Material
The Supplementary Material for this article can be found online at: https://www.frontiersin.org/articles/10.3389/fimmu.2021.794077/full#supplementary-material
References
1. Sospedra M, Martin R. Immunology of Multiple Sclerosis. Semin Neurol (2016) 36(2):115–27. doi: 10.1055/s-0036-1579739
2. Li R, Patterson KR, Bar-Or A. Reassessing B Cell Contributions in Multiple Sclerosis. Nat Immunol (2018) 19(7):696–707. doi: 10.1038/s41590-018-0135-x
3. Jelcic I, Al Nimer F, Wang J, Lentsch V, Planas R, Jelcic I, et al. Memory B Cells Activate Brain-Homing, Autoreactive CD4(+) T Cells in Multiple Sclerosis. Cell (2018) 175(1):85–100.e23. doi: 10.1016/j.cell.2018.08.011
4. Thi Cuc B, Pohar J, Fillatreau S. Understanding Regulatory B Cells in Autoimmune Diseases: The Case of Multiple Sclerosis. Curr Opin Immunol (2019) 61:26–32. doi: 10.1016/j.coi.2019.07.007
5. Van Kaer L, Postoak JL, Wang C, Yang G, Wu L. Innate, Innate-Like and Adaptive Lymphocytes in the Pathogenesis of MS and EAE. Cell Mol Immunol (2019) 16(6):531–9. doi: 10.1038/s41423-019-0221-5
6. Farag SS, Caligiuri MA. Human Natural Killer Cell Development and Biology. Blood Rev (2006) 20(3):123–37. doi: 10.1016/j.blre.2005.10.001
7. Morvan MG, Lanier LL. NK Cells and Cancer: You can Teach Innate Cells New Tricks. Nat Rev Cancer (2016) 16(1):7–19. doi: 10.1038/nrc.2015.5
8. Jelcić I, Hsu KC, Kakalacheva K, Breiden P, Dupont B, Uhrberg M, et al. Killer Immunoglobulin-Like Receptor Locus Polymorphisms in Multiple Sclerosis. Mult Scler (2012) 18(7):951–8. doi: 10.1177/1352458511431726
9. Mimpen M, Smolders J, Hupperts R, Damoiseaux J. Natural Killer Cells in Multiple Sclerosis: A Review. Immunol Lett (2020) 222:1–11. doi: 10.1016/j.imlet.2020.02.012
10. Porcelli S, Yockey CE, Brenner MB, Balk SP. Analysis of T Cell Antigen Receptor (TCR) Expression by Human Peripheral Blood CD4-8- Alpha/Beta T Cells Demonstrates Preferential Use of Several V Beta Genes and an Invariant TCR Alpha Chain. J Exp Med (1993) 178(1):1–16. doi: 10.1084/jem.178.1.1
11. Lanier LL. Shades of Grey–the Blurring View of Innate and Adaptive Immunity. Nat Rev Immunol (2013) 13(2):73–4. doi: 10.1038/nri3389
12. Pellicci DG, Koay HF, Berzins SP. Thymic Development of Unconventional T Cells: How NKT Cells, MAIT Cells and γδ T Cells Emerge. Nat Rev Immunol (2020) 20(12):756–70. doi: 10.1038/s41577-020-0345-y
13. Muraro PA, Martin R, Mancardi GL, Nicholas R, Sormani MP, Saccardi R. Autologous Haematopoietic Stem Cell Transplantation for Treatment of Multiple Sclerosis. Nat Rev Neurol (2017) 13(7):391–405. doi: 10.1038/nrneurol.2017.81
14. Malmegrim KCR, Lima-Junior JR, Arruda LCM, de Azevedo JTC, de Oliveira GLV, Oliveira MC. Autologous Hematopoietic Stem Cell Transplantation for Autoimmune Diseases: From Mechanistic Insights to Biomarkers. Front Immunol (2018) 9:2602. doi: 10.3389/fimmu.2018.02602
15. Arruda LC, Clave E, Moins-Teisserenc H, Douay C, Farge D, Toubert A. Resetting the Immune Response After Autologous Hematopoietic Stem Cell Transplantation for Autoimmune Diseases. Curr Res Transl Med (2016) 64(2):107–13. doi: 10.1016/j.retram.2016.03.004
16. Massey JC, Sutton IJ, Ma DDF, Moore JJ. Regenerating Immunotolerance in Multiple Sclerosis With Autologous Hematopoietic Stem Cell Transplant. Front Immunol (2018) 9:410. doi: 10.3389/fimmu.2018.00410
17. Darlington PJ, Touil T, Doucet JS, Gaucher D, Zeidan J, Gauchat D, et al. Diminished Th17 (Not Th1) Responses Underlie Multiple Sclerosis Disease Abrogation After Hematopoietic Stem Cell Transplantation. Ann Neurol (2013) 73(3):341–54. doi: 10.1002/ana.23784
18. Lünemann JD, Ruck T, Muraro PA, Bar-Or A, Wiendl H. Immune Reconstitution Therapies: Concepts for Durable Remission in Multiple Sclerosis. Nat Rev Neurol (2020) 16(1):56–62. doi: 10.1038/s41582-019-0268-z
19. Van Acker HH, Capsomidis A, Smits EL, Van Tendeloo VF. CD56 in the Immune System: More Than a Marker for Cytotoxicity? Front Immunol (2017) 8:892. doi: 10.3389/fimmu.2017.00892
20. Vergelli M, Hemmer B, Muraro PA, Tranquill L, Biddison WE, Sarin A, et al. Human Autoreactive CD4+ T Cell Clones Use Perforin- or Fas/Fas Ligand-Mediated Pathways for Target Cell Lysis. J Immunol (1997) 158(6):2756–61. doi: 10.1046/j.1365-2249.1999.01010.x
21. Antel JP, McCrea E, Ladiwala U, Y.-f. Qin and B. Becher: Non-MHC-Restricted Cell-Mediated Lysis of Human Oligodendrocytes In Vitro: Relation With CD56 Expression. J Immunol (1998) 160(4):1606–11.
22. Godfrey DI, MacDonald HR, Kronenberg M, Smyth MJ, Van Kaer L. NKT Cells: What's in a Name? Nat Rev Immunol (2004) 4(3):231–7. doi: 10.1038/nri1309
23. Konduri V, Oyewole-Said D, Vazquez-Perez J, Weldon SA, Halpert MM, Levitt JM, et al. CD8(+)CD161(+) T-Cells: Cytotoxic Memory Cells With High Therapeutic Potential. Front Immunol (2020) 11:613204. doi: 10.3389/fimmu.2020.613204
24. Ito T, t. Carson WF, Cavassani KA, Connett JM, Kunkel SL. CCR6 as a Mediator of Immunity in the Lung and Gut. Exp Cell Res (2011) 317(5):613–9. doi: 10.1016/j.yexcr.2010.12.018
25. Davis MM, Bjorkman PJ. T-Cell Antigen Receptor Genes and T-Cell Recognition. Nature (1988) 334(6181):395–402. doi: 10.1038/334395a0
26. Bonneville M, O'Brien RL, Born WK. Gammadelta T Cell Effector Functions: A Blend of Innate Programming and Acquired Plasticity. Nat Rev Immunol (2010) 10(7):467–78. doi: 10.1038/nri2781
27. Montaldo E, Del Zotto G, Della Chiesa M, Mingari MC, Moretta A, De Maria A, et al. Human NK Cell Receptors/Markers: A Tool to Analyze NK Cell Development, Subsets and Function. Cytometry A (2013) 83(8):702–13. doi: 10.1002/cyto.a.22302
28. Orange JS. Unraveling Human Natural Killer Cell Deficiency. J Clin Invest (2012) 122(3):798–801. doi: 10.1172/jci62620
29. Björkström NK, Strunz B, Ljunggren HG. Natural Killer Cells in Antiviral Immunity. Nat Rev Immunol (2021) 21:1–12. doi: 10.1038/s41577-021-00558-3
30. Bielekova B, Catalfamo M, Reichert-Scrivner S, Packer A, Cerna M, Waldmann TA, et al. Regulatory CD56(bright) Natural Killer Cells Mediate Immunomodulatory Effects of IL-2Ralpha-Targeted Therapy (Daclizumab) in Multiple Sclerosis. Proc Natl Acad Sci USA (2006) 103(15):5941–6. doi: 10.1073/pnas.0601335103
31. Voigt J, Malone DFG, Dias J, Leeansyah E, Björkström NK, Ljunggren HG, et al. Proteome Analysis of Human CD56(neg) NK Cells Reveals a Homogeneous Phenotype Surprisingly Similar to CD56(dim) NK Cells. Eur J Immunol (2018) 48(9):1456–69. doi: 10.1002/eji.201747450
32. Laroni A, Armentani E, Kerlero de Rosbo N, Ivaldi F, Marcenaro E, Sivori S, et al. Dysregulation of Regulatory CD56(bright) NK Cells/T Cells Interactions in Multiple Sclerosis. J Autoimmun (2016) 72:8–18. doi: 10.1016/j.jaut.2016.04.003
33. Lünemann A, Tackenberg B, DeAngelis T, Silva R, Messmer B, Vanoaica L, et al. Impaired IFN- Production and Proliferation of NK Cells in Multiple Sclerosis. Int Immunol (2011) 23:139–48. doi: 10.1093/intimm/dxq463
34. Bielekova B, Richert N, Howard T, Blevins G, Markovic-Plese S, McCartin J, et al. Humanized Anti-CD25 (Daclizumab) Inhibits Disease Activity in Multiple Sclerosis Patients Failing to Respond to Interferon Beta. Proc Natl Acad Sci USA (2004) 101(23):8705–8. doi: 10.1073/pnas.0402653101
35. Kappos L, Wiendl H, Selmaj K, Arnold DL, Havrdova E, Boyko A, et al. Daclizumab HYP Versus Interferon Beta-1a in Relapsing Multiple Sclerosis. N Engl J Med (2015) 373(15):1418–28. doi: 10.1056/NEJMoa1501481
36. Saraste M, Irjala H, Airas L. Expansion of CD56Bright Natural Killer Cells in the Peripheral Blood of Multiple Sclerosis Patients Treated With Interferon-Beta. Neurological Sci (2007) 28(3):121–6. doi: 10.1007/s10072-007-0803-3
37. Putzki N, Baranwal MK, Tettenborn B, Limmroth V, Kreuzfelder E. Effects of Natalizumab on Circulating B Cells, T Regulatory Cells and Natural Killer Cells. Eur Neurol (2010) 63(5):311–7. doi: 10.1159/000302687
38. Gross CC, Schulte-Mecklenbeck A, Wiendl H, Marcenaro E, Kerlero de Rosbo N, Uccelli A, et al. Regulatory Functions of Natural Killer Cells in Multiple Sclerosis. Front Immunol (2016) 7:606. doi: 10.3389/fimmu.2016.00606
39. Gross CC, Schulte-Mecklenbeck A, Rünzi A, Kuhlmann T, Posevitz-Fejfár A, Schwab N, et al. Impaired NK-Mediated Regulation of T-Cell Activity in Multiple Sclerosis is Reconstituted by IL-2 Receptor Modulation. Proc Natl Acad Sci USA (2016) 113(21):E2973–82. doi: 10.1073/pnas.1524924113
40. Sakuishi K, Miyake S, Yamamura T. Role of NK Cells and Invariant NKT Cells in Multiple Sclerosis. Results Probl Cell Differ (2010) 51:127–47. doi: 10.1007/400_2009_11
41. Martin JF, Perry JS, Jakhete NR, Wang X, Bielekova B. An IL-2 Paradox: Blocking CD25 on T Cells Induces IL-2-Driven Activation of CD56(bright) NK Cells. J Immunol (2010) 185(2):1311–20. doi: 10.4049/jimmunol.0902238
42. Moore JJ, Massey JC, Ford CD, Khoo ML, Zaunders JJ, Hendrawan K, et al. Prospective Phase II Clinical Trial of Autologous Haematopoietic Stem Cell Transplant for Treatment Refractory Multiple Sclerosis. J Neurol Neurosurg Psychiatry (2019) 90(5):514–21. doi: 10.1136/jnnp-2018-319446
43. Abrahamsson SV, Angelini DF, Dubinsky AN, Morel E, Oh U, Jones JL, et al. Non-Myeloablative Autologous Haematopoietic Stem Cell Transplantation Expands Regulatory Cells and Depletes IL-17 Producing Mucosal-Associated Invariant T Cells in Multiple Sclerosis. Brain 136(Pt (2013) 9):2888–903. doi: 10.1093/brain/awt182
44. Darlington PJ, Stopnicki B, Touil T, Doucet JS, Fawaz L, Roberts ME, et al. Natural Killer Cells Regulate Th17 Cells After Autologous Hematopoietic Stem Cell Transplantation for Relapsing Remitting Multiple Sclerosis. Front Immunol (2018) 9:834. doi: 10.3389/fimmu.2018.00834
45. Lagumersindez-Denis N, Wrzos C, Mack M, Winkler A, van der Meer F, Reinert MC, et al. Differential Contribution of Immune Effector Mechanisms to Cortical Demyelination in Multiple Sclerosis. Acta Neuropathol (2017) 134(1):15–34. doi: 10.1007/s00401-017-1706-x
46. Nielsen N, Ødum N, Ursø B, Lanier LL, Spee P. Cytotoxicity of CD56(bright) NK Cells Towards Autologous Activated CD4+ T Cells Is Mediated Through NKG2D, LFA-1 and TRAIL and Dampened via CD94/NKG2A. PloS One (2012) 7(2):e31959. doi: 10.1371/journal.pone.0031959
47. Penack O, Fischer L, Gentilini C, Nogai A, Muessig A, Rieger K, et al. The Type of ATG Matters – Natural Killer Cells Are Influenced Differentially by Thymoglobulin, Lymphoglobulin and ATG-Fresenius. Transpl Immunol (2007) 18(2):85–7. doi: 10.1016/j.trim.2007.05.001
48. Bierman PJ, Abe F, Buyukberber S, Ino K, Talmadge JE. Partial Review of Immunotherapeutic Pharmacology in Stem Cell Transplantation. In Vivo (2000) 14(1):221–36.
49. Mathew PA, Chuang SS, Vaidya SV, Kumaresan PR, Boles KS, Pham HT. The LLT1 Receptor Induces IFN-Gamma Production by Human Natural Killer Cells. Mol Immunol (2004) 40(16):1157–63. doi: 10.1016/j.molimm.2003.11.024
50. Rosen DB, Bettadapura J, Alsharifi M, Mathew PA, Warren HS, Lanier LL. Cutting Edge: Lectin-Like Transcript-1 Is a Ligand for the Inhibitory Human NKR-P1A Receptor. J Immunol (2005) 175(12):7796–9. doi: 10.4049/jimmunol.175.12.7796
51. O'Keeffe J, Doherty DG, Kenna T, Sheahan K, O'Donoghue DP, Hyland JM, et al. Diverse Populations of T Cells With NK Cell Receptors Accumulate in the Human Intestine in Health and in Colorectal Cancer. Eur J Immunol (2004) 34(8):2110–9. doi: 10.1002/eji.200424958
52. Norris S, Doherty DG, Collins C, McEntee G, Traynor O, Hegarty JE, et al. Natural T Cells in the Human Liver: Cytotoxic Lymphocytes With Dual T Cell and Natural Killer Cell Phenotype and Function Are Phenotypically Heterogenous and Include Valpha24-JalphaQ and Gammadelta T Cell Receptor Bearing Cells. Hum Immunol (1999) 60(1):20–31. doi: 10.1016/s0198-8859(98)00098-6
53. Kim CH, Johnston B, Butcher EC. Trafficking Machinery of NKT Cells: Shared and Differential Chemokine Receptor Expression Among V Alpha 24(+)V Beta 11(+) NKT Cell Subsets With Distinct Cytokine-Producing Capacity. Blood (2002) 100(1):11–6. doi: 10.1182/blood-2001-12-0196
54. Godfrey DI, Koay HF, McCluskey J, Gherardin NA. The Biology and Functional Importance of MAIT Cells. Nat Immunol (2019) 20(9):1110–28. doi: 10.1038/s41590-019-0444-8
55. Mechelli R, Romano S, Romano C, Morena E, Buscarinu MC, Bigi R, et al. MAIT Cells and Microbiota in Multiple Sclerosis and Other Autoimmune Diseases. Microorganisms (2021) 9(6):1132. doi: 10.3390/microorganisms9061132
56. Zaguia F, Saikali P, Ludwin S, Newcombe J, Beauseigle D, McCrea E, et al. Cytotoxic NKG2C+ CD4 T Cells Target Oligodendrocytes in Multiple Sclerosis. J Immunol (2013) 190(6):2510–8. doi: 10.4049/jimmunol.1202725
57. Zeine R, Pon R, Ladiwala U, Antel JP, Filion LG, Freedman MS. Mechanism of Gammadelta T Cell-Induced Human Oligodendrocyte Cytotoxicity: Relevance to Multiple Sclerosis. J Neuroimmunol 87(1-2). doi: 10.1016/s0165-5728(98)00047-2
58. Illés Z, Shimamura M, Newcombe J, Oka N, Yamamura T. Accumulation of Valpha7.2-Jalpha33 Invariant T Cells in Human Autoimmune Inflammatory Lesions in the Nervous System. Int Immunol (2004) 16(2):223–30. doi: 10.1093/intimm/dxh018
59. Wucherpfennig KW, Newcombe J, Li H, Keddy C, Cuzner ML, Hafler DA. Gamma Delta T-Cell Receptor Repertoire in Acute Multiple Sclerosis Lesions. Proc Natl Acad Sci USA (1992) 89(10):4588–92. doi: 10.1073/pnas.89.6.2110
60. Shimonkevitz R, Colburn C, Burnham JA, Murray RS, Kotzin BL. Clonal Expansions of Activated Gamma/Delta T Cells in Recent-Onset Multiple Sclerosis. Proc Natl Acad Sci USA (1993) 90(3):923–7. doi: 10.1073/pnas.90.3.923
61. Poggi A, Zocchi MR, Costa P, Ferrero E, Borsellino G, Placido R, et al. IL-12-Mediated NKRP1A Up-Regulation and Consequent Enhancement of Endothelial Transmigration of V Delta 2+ TCR Gamma Delta+ T Lymphocytes From Healthy Donors and Multiple Sclerosis Patients. J Immunol (1999) 162(7):4349–54.
62. De Biasi S, Simone AM, Nasi M, Bianchini E, Ferraro D, Vitetta F, et al. iNKT Cells in Secondary Progressive Multiple Sclerosis Patients Display Pro-Inflammatory Profiles. Front Immunol (2016) 7:555. doi: 10.3389/fimmu.2016.00555
63. Willing A, Jäger J, Reinhardt S, Kursawe N, Friese MA. Production of IL-17 by MAIT Cells Is Increased in Multiple Sclerosis and Is Associated With IL-7 Receptor Expression. J Immunol (2018) 200(3):974–82. doi: 10.4049/jimmunol.1701213
64. O'Keeffe J, Gately CM, Counihan T, Hennessy M, Leahy T, Moran AP, et al. T-Cells Expressing Natural Killer (NK) Receptors Are Altered in Multiple Sclerosis and Responses to Alpha-Galactosylceramide are Impaired. J Neurol Sci (2008) 275(1-2):22–8. doi: 10.1016/j.jns.2008.07.007
65. Gately CM, Podbielska M, Counihan T, Hennessy M, Leahy T, Moran AP, et al. Invariant Natural Killer T-Cell Anergy to Endogenous Myelin Acetyl-Glycolipids in Multiple Sclerosis. J Neuroimmunol (2013) 259(1-2):1–7. doi: 10.1016/j.jneuroim.2013.02.020
66. Podbielska M, O'Keeffe J, Hogan EL. Autoimmunity in Multiple Sclerosis: Role of Sphingolipids, Invariant NKT Cells and Other Immune Elements in Control of Inflammation and Neurodegeneration. J Neurol Sci (2018) 385:198–214. doi: 10.1016/j.jns.2017.12.022
67. Novak J, Dobrovolny J, Brozova J, Novakova L, Kozak T. Recovery of Mucosal-Associated Invariant T Cells After Myeloablative Chemotherapy and Autologous Peripheral Blood Stem Cell Transplantation. Clin Exp Med (2016) 16(4):529–37. doi: 10.1007/s10238-015-0384-z
68. Lanier LL, Chang C, Phillips JH. Human NKR-P1A. A Disulfide-Linked Homodimer of the C-Type Lectin Superfamily Expressed by a Subset of NK and T Lymphocytes. J Immunol (1994) 153(6):2417–28.
69. Gao Y, Williams AP. Role of Innate T Cells in Anti-Bacterial Immunity. Front Immunol (2015) 6:302. doi: 10.3389/fimmu.2015.00302
70. Dusseaux M, Martin E, Serriari N, Péguillet I, Premel V, Louis D, et al. Human MAIT Cells Are Xenobiotic-Resistant, Tissue-Targeted, CD161hi IL-17-Secreting T Cells. Blood (2011) 117(4):1250–9. doi: 10.1182/blood-2010-08-303339
71. Billerbeck E, Kang Y-H, Walker L, Lockstone H, Grafmueller S, Fleming V, et al. Analysis of CD161 Expression on Human CD8+ T Cells Defines a Distinct Functional Subset With Tissue-Homing Properties. Proc Natl Acad Sci USA (2010) 107(7):3006–11. doi: 10.1073/pnas.0914839107
72. Annibali V, Ristori G, Angelini DF, Serafini B, Mechelli R, Cannoni S, et al. Cd161highcd8+T Cells Bear Pathogenetic Potential in Multiple Sclerosis. Brain (2011) 134(2):542–54. doi: 10.1093/brain/awq354
73. Koay HF, Gherardin NA, Enders A, Loh L, Mackay LK, Almeida CF, et al. A Three-Stage Intrathymic Development Pathway for the Mucosal-Associated Invariant T Cell Lineage. Nat Immunol (2016) 17(11):1300–11. doi: 10.1038/ni.3565
74. Lee AYS, Körner H. The CCR6-CCL20 Axis in Humoral Immunity and T-B Cell Immunobiology. Immunobiology (2019) 224(3):449–54. doi: 10.1016/j.imbio.2019.01.005
75. Liao F, Rabin RL, Smith CS, Sharma G, Nutman TB, Farber JM. CC-Chemokine Receptor 6 Is Expressed on Diverse Memory Subsets of T Cells and Determines Responsiveness to Macrophage Inflammatory Protein 3 Alpha. J Immunol (1999) 162(1):186–94.
Keywords: aHSCT, multiple sclerosis, innate-like T cells, NK cells, CD56bright NK cells, atypical T cells, MAIT cells, NKT cells
Citation: Ruder J, Rex J, Obahor S, Docampo MJ, Müller AMS, Schanz U, Jelcic I and Martin R (2021) NK Cells and Innate-Like T Cells After Autologous Hematopoietic Stem Cell Transplantation in Multiple Sclerosis. Front. Immunol. 12:794077. doi: 10.3389/fimmu.2021.794077
Received: 13 October 2021; Accepted: 29 November 2021;
Published: 16 December 2021.
Edited by:
Antoine Toubert, Université Paris Diderot, FranceReviewed by:
Tobias Alexander, Charité University Medicine Berlin, GermanyGirdhari Lal, National Centre for Cell Science, India
Copyright © 2021 Ruder, Rex, Obahor, Docampo, Müller, Schanz, Jelcic and Martin. This is an open-access article distributed under the terms of the Creative Commons Attribution License (CC BY). The use, distribution or reproduction in other forums is permitted, provided the original author(s) and the copyright owner(s) are credited and that the original publication in this journal is cited, in accordance with accepted academic practice. No use, distribution or reproduction is permitted which does not comply with these terms.
*Correspondence: Roland Martin, cm9sYW5kLm1hcnRpbkB1c3ouY2g=