- 1Department of Pediatrics and Adolescent Medicine, University Hospital Rigshospitalet, Copenhagen, Denmark
- 2Novo Nordisk Foundation Center for Basic Metabolic Research, Faculty of Health and Medical Sciences, University of Copenhagen, Copenhagen, Denmark
- 3Department of Biomedical Sciences, Faculty of Health and Medical Sciences, University of Copenhagen, Copenhagen, Denmark
- 4Institute for Inflammation Research, Center for Rheumatology and Spine Diseases, University Hospital Rigshospitalet, Copenhagen, Denmark
- 5The Children’s Obesity Clinic, Accredited European Centre for Obesity Management, Department of Pediatrics, Copenhagen University Hospital Holbæk, Holbæk, Denmark
- 6Department of Pediatrics, Kolding Hospital a Part of Lillebælt Hospital, Kolding, Denmark
Patients undergoing allogeneic hematopoietic stem cell transplantation (HSCT) are challenged with severe side effects, which are propagated by mucosal barrier disruption, and the related microbial translocation and systemic inflammation. Glucagon-like peptide-1 (GLP-1), a well-known incretin hormone, possesses anti-inflammatory properties and promotes regeneration of damaged intestinal epithelium in animal studies. We hypothesized that the immense inter-individual variation in the degree of mucosal damage and systemic inflammation, seen after HSCT is influenced by endogenous GLP-1 and could be related to acute post-transplant complications. In this prospective study we measured serial weekly fasting plasma GLP-1, along with C-reactive protein (CRP), and citrulline in 82 pediatric patients during allogeneic HSCT together with a fasting plasma GLP-1 in sex- and age-matched healthy controls. Overall, GLP-1 levels were increased in the patients during the course of HSCT compared with the controls, but tended to decrease post-transplant, most pronounced in patients receiving high-intensity conditioning regimen. The increase in CRP seen in the early post-transplant phase was significantly lower from day +8 to +13 in patients with GLP-1 above the upper quartile (>10 pmol/L) at day 0 (all P ≤ 0.03). Similar findings were seen for peak CRP levels after adjusting for type of conditioning (-47.0%; 95% CI, -8.1 – -69.4%, P = 0.02). Citrulline declined significantly following the transplantation illustrating a decrease in viable enterocytes, most evident in patients receiving high-intensity conditioning regimen. GLP-1 levels at day 0 associated with the recovery rate of citrulline from day 0 to +21 (34 percentage points (pp)/GLP-1 doubling; 95% CI, 10 – 58pp; P = 0. 008) and day 0 to day +90 (48 pp/GLP-1 doubling; 95% CI, 17 – 79pp; P = 0. 004), also after adjustment for type of conditioning. This translated into a reduced risk of acute graft-versus-host disease (aGvHD) in patients with highest day 0 GLP-1 levels (>10 pmol/L) (cause-specific HR: 0.3; 95% CI, 0.2 – 0.9, P = 0.02). In conclusion, this study strongly suggests that GLP-1 influences regeneration of injured epithelial barriers and ameliorates inflammatory responses in the early post-transplant phase.
Introduction
Allogeneic hematopoietic stem cell transplantation (HSCT) in children and adolescents is challenged by adverse events, which to a large extent are related to toxic reactions in the gastrointestinal tract (1). Oral and gastrointestinal mucositis are reported in up to 90 – 100% of patients during HSCT with myeloablative conditioning (2–4), and substantial evidence indicates that intestinal toxicity induces severe systemic inflammation and translocation of bacterial products, leading to increased risk of acute graft-versus-host-disease (aGvHD), invasive infections, multi-organ failure and treatment-related mortality (5–8).
The susceptibility of the patients to develop these complications is highly variable, which opens a window for personalized treatment, but further progress is hampered by the absence of predictive biomarkers. Moreover, there is no effective treatment preventing severe mucositis and the current treatment is symptomatic, based on parenteral hydration and nutrition, pain relief by morphine and use of broad-spectrum antibiotics.
However, sustained proliferation of intestinal epithelium is known to be elemental in healing of mucositis and maintenance of the intestinal barrier and involves growth factors produced in the gut (9, 10). Glucagon-like peptide-1 (GLP-1) is a peptide hormone secreted from enteroendocrine L-cells following enteral food intake, and is well-known for being essential in regulating blood glucose by the stimulation of insulin secretion (11, 12). Additionally, increased secretion of GLP-1 is seen after chemotherapy-induced intestinal injury in rodents (13, 14), and elevated plasma levels are observed in humans after chemotherapy (15) and during gut ischemia (16). Indeed, GLP-1 has intestinotrophic effects sustaining the integrity of intestinal mucosal barrier in animal studies (17, 18). Administration of GLP-1 analogs can ameliorate chemotherapy-induced intestinal injury (13), while ablation of L-cells in mice has led to severe mucositis as well as insufficient intestinal healing after chemotherapy (14, 19). In addition, GLP-1 acts as a direct anti-inflammatory mediator locally through GLP-1-receptors expressed on intestinal intraepithelial lymphocytes (20, 21).
In the present study, we measured fasting GLP-1 plasma levels in pediatric patients undergoing HSCT from before the start of conditioning and during the early post-transplant period to determine possible associations between GLP-1 and intestinal damage, measured by citrulline levels, systemic inflammation and post-HSCT complications.
Materials and Methods
Study Population
In this prospective population-based study, 82 children and adolescents (1–18 years of age) undergoing their first allogeneic HSCT were consecutively recruited at University Hospital Rigshospitalet, Copenhagen, Denmark, from March 2015 to November 2019. This patient cohort has previously been described in a different context (22).
Conditioning groups were defined as 1) high-intensity myeloablative conditioning (total body irradiation (TBI) 12Gy + etoposide, busulfan + cyclophosphamide, or busulfan + thiotepa + fludarabine) and 2) low-intensity myeloablative conditioning (other fludarabine-based regimens or cyclophosphamide + TBI 2Gy) (23) (Table 1).
Control Cohort
A control cohort matched by sex and nearest age option with a patient/control frequency ratio of 1:5 (N = 410 controls, Table 2) was included from a population-based cohort of Danish/North-European children and adolescents without obesity and diabetes, 6-18 years of age (N = 2,266), enrolled in The Danish Childhood Obesity Data- and Biobank from 2009 - 2019 and previously described (24, 25).

Table 2 Age and sex in the HSCT-patients and the sex- and age-matched control group, frequency ratio 1:5.
Blood Samples for Laboratory Analyses
Blood samples were collected at 6 AM at the following time points: before start of the conditioning regimen, at the day of transplantation (day 0), and at days +7, +14 and +21 post-transplantation. The control cohort had a single venous blood sample collected between 7 and 9 AM, following an overnight fast (26). EDTA anticoagulated blood was centrifuged up to two hours after collection and plasma was isolated and stored at -80°C.
Quantification of GLP-1
The plasma concentration of total GLP-1 in both patients and controls was measured in duplicates using a GLP-1 ELISA kit (Mercodia, Uppsala, Sweden) according to the manufacturer’s instructions (27). Both active GLP-1 (7-36) amide and the degraded isoform GLP-1 (9-36) amide were measured and reflects the secretion of GLP-1 because amidated isoforms of GLP-1 are highly predominant in humans (28). Measurement range was 0.9 to 940 pmol/L.
Citrulline
As a marker of enterocyte damage, plasma citrulline was measured at the same time points as GLP-1 and at follow-up day +90 post-HSCT. A Waters Acquity™ Ultra-Performance Liquid Chromatography system with a Tandem Quadrupole detector was used for the analysis (29).
CRP
All patients had CRP monitored daily during the first three weeks after transplantation. When more than one measurement per day was available, the mean was calculated to represent the CRP level of that day. CRPmax was defined as maximum CRP value from day +1 to day +21. CRP was analyzed using Modular P Module (Roche, Basel, Switzerland) (upper normal limit, 10 mg/L) at the Department of Clinical Biochemistry, University Hospital Rigshospitalet. Blood cultures were routinely collected on all patients with fever and results were registered from day -14 to day +30.
Statistical Analyses
A mixed model with a compound symmetry covariance matrix was used to investigate associations of GLP-1 and citrulline over time with patient-specific characteristics and the association between CRP over time and dichotomized GLP-1 level at day 0. GLP-1 and CRPmax were log-transformed due to their skewness.
Correlation analyses were performed using Spearman’s rank order correlation analysis. The Mann-Whitney U-test or the Kruskal–Wallis univariate test were used for comparisons between groups. Simple and multiple linear regression models were used to determine the association between GLP-1 and CRPmax. All potential risk factors listed in Tables 1, 2 were tested in univariate analyses and included in the multivariate model if they showed statistically significant associations with outcome variables and/or GLP-1 as indicated under results. Interaction between GLP-1 and conditioning group stratification on CRPmax was included as a covariate in the initial multivariate model and tested with ANOVA. Logistic simple and multiple regressions were used to determine variables associated with occurrence of aGvHD and sinusoidal obstruction syndrome (SOS). Cumulative incidences, cause-specific Cox regression models and Gray’s test (30) were used to estimate the risk of aGvHD.
Statistical significance was defined as a two-sided P < 0.05. All statistical analyses were performed using R statistical software version 3.6.1 [R Foundation for Statistical Computing, Vienna, Austria (31)] and RStudio version 1.2.1335 (RStudio, Boston, MA).
Ethics Statement
The study was approved by Capital Region of Denmark’s Ethical Committee (H-7-2014-016) and conducted in accordance with the Declaration of Helsinki. Written informed consent was obtained from all included patients and/or their legal guardians.
Results
Clinical characteristics of patients and controls are presented in Tables 1, 2. Before start of conditioning, 44 out of the 82 included patients had a fasting sample available for GLP-1 measurement. These patients did not differ from the rest of the cohort regarding sex, age, or diagnosis. At the remaining time points all patients had fasting GLP-1 measurements available.
GLP-1 in Patients and Healthy Controls
Median fasting GLP-1 levels in the patients were higher than in the controls at all time points but decreased during the first weeks of the transplantation, most pronounced in patients receiving high-intensity myeloablative conditioning (Figure 1).
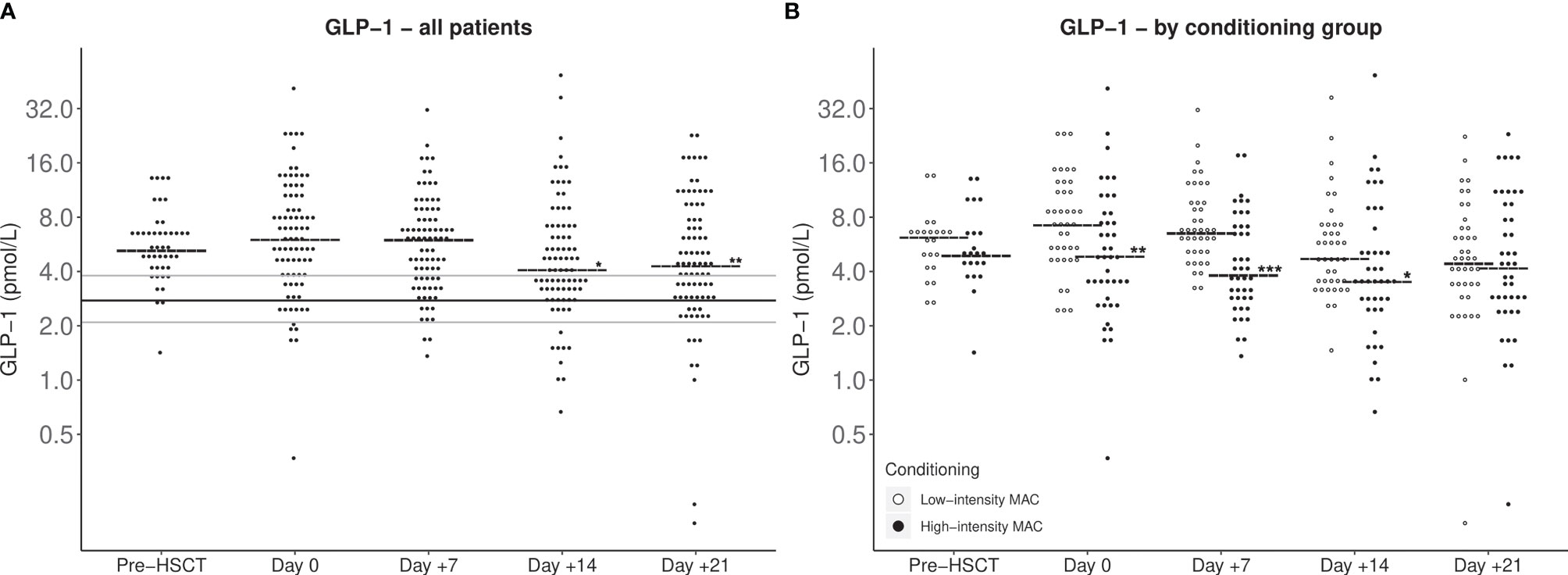
Figure 1 GLP-1 fasting plasma levels during pediatric HSCT from before conditioning until day +21 post-HSCT. Horizontal short lines: Median GLP-1. (A) All included patients. Consistent lines: Median (black), lower and upper quartile (grey) for fasting GLP-1 for healthy control cohort. Statistical evaluation indicates increased GLP-1 levels of HSCT pediatric patients compared with the control cohort at all time points (P < 0.001). A mixed model analysis showed GLP-1 levels at day +14 and day +21 to be lower than day 0 GLP-1 levels (*P < 0.05; **P < 0.005). (B) Patients stratified by conditioning group. Patients treated with high-intensity conditioning regimens had generally lower post-HSCT GLP-1 levels than patients treated with low-intensity conditioning evaluated with a mixed model analysis (*P < 0.05; **P < 0.005; ***P < 0.001).
Inflammation and GLP-1
To explore potential anti-inflammatory protective effects of GLP-1, we investigated associations between GLP-1 and CRP. CRP increased significantly during the course of transplantation reaching a maximum at day +9. Peak CRP levels were significantly higher in patients receiving high-intensity vs low-intensity conditioning (median CRPmax 35 vs. 89 mg/L (P < 0.001) but were not associated with other patient- and transplant-related characteristics. Stratifying patients in two groups according to GLP-1 levels at day 0, patients with GLP-1 levels in the upper quartile (>10 pmol/L) had significantly lower CRPmax levels (-56%; 95% CI, -20– -76%, P = 0.007). This association remained significant after adjusting for type of conditioning (-47.0%; 95% CI, -8.1 – -69.4%, P = 0.02). There was no interaction between GLP-1 levels and conditioning group on CRPmax. Likewise, when looking at daily CRP measurements post-HSCT, GLP-1 levels above the upper quartile at day 0 were associated with lower levels of CRP from day +8 to day +13 (all P ≤ 0.03) (Figure 2).
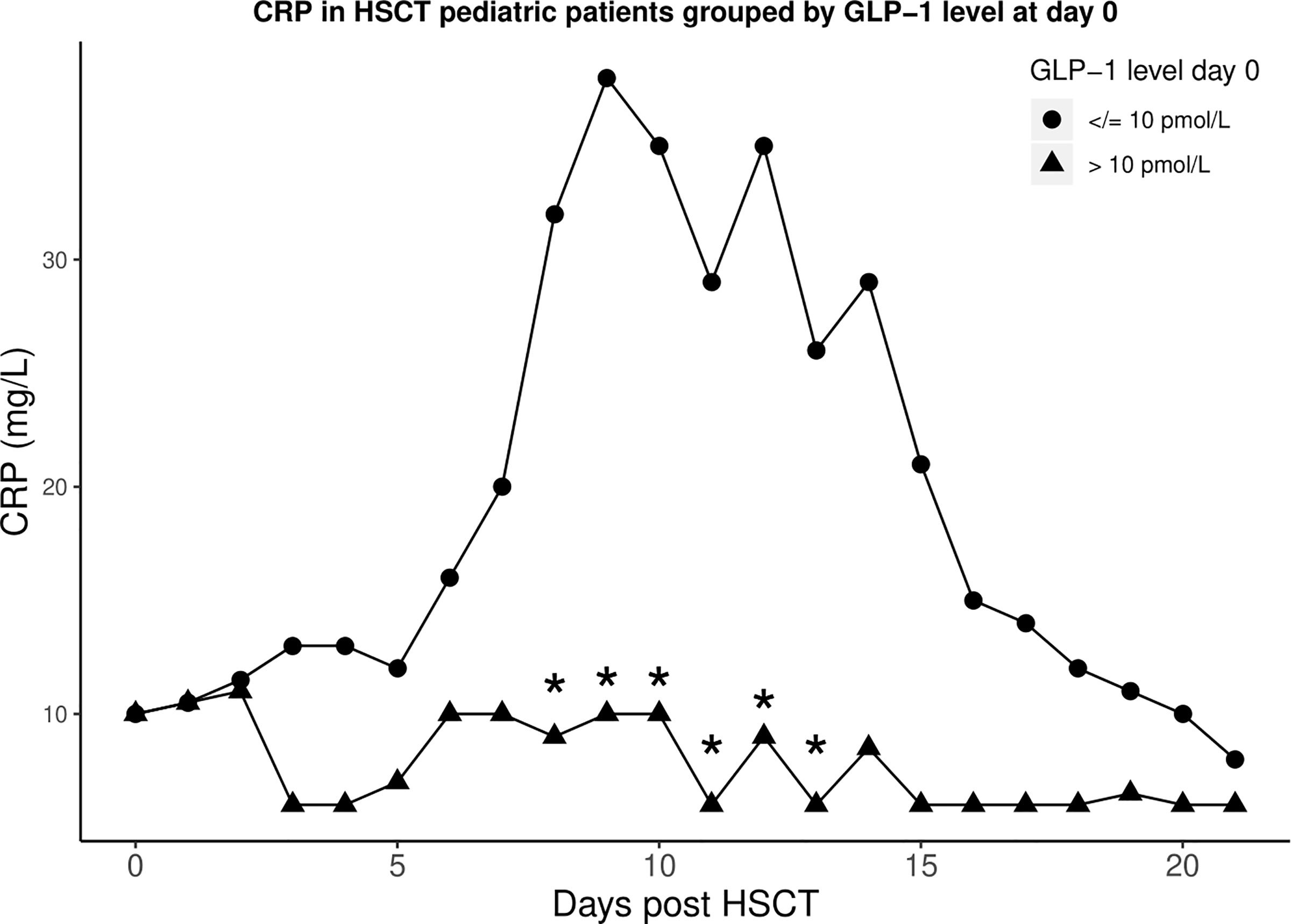
Figure 2 Median CRP levels after HSCT in two groups stratified by GLP-1 level at day 0 [≤10 pmol/L (circles) and >10 pmol/L (triangles)]. *CRP levels significantly differ between the two groups (all P ≤ 0.03).
Citrulline and GLP-1
The systemic inflammatory response during the aplastic phase of HSCT has to a large extent been related to disintegration of the intestinal mucosal barrier, which can be effectively monitored by plasma citrulline, being a marker of the total population of viable enterocytes (32). Citrulline levels decreased significantly after conditioning therapy reaching nadir at day +7, coinciding with the timepoint of maximum CRP levels. At day +90, citrulline had again risen close to pre-HSCT levels [median (IQR): 19.0 (16.0 - 22.0) vs 20.7 (16.5 - 27.2) μmol/L] (Figure 3A). The citrulline decrease was most pronounced in patients receiving high-intensity myeloablative conditioning and with a delayed recovery compared with those undergoing low-intensity conditioning (significantly lower levels at day +14 and +21, but comparable levels at day +90).
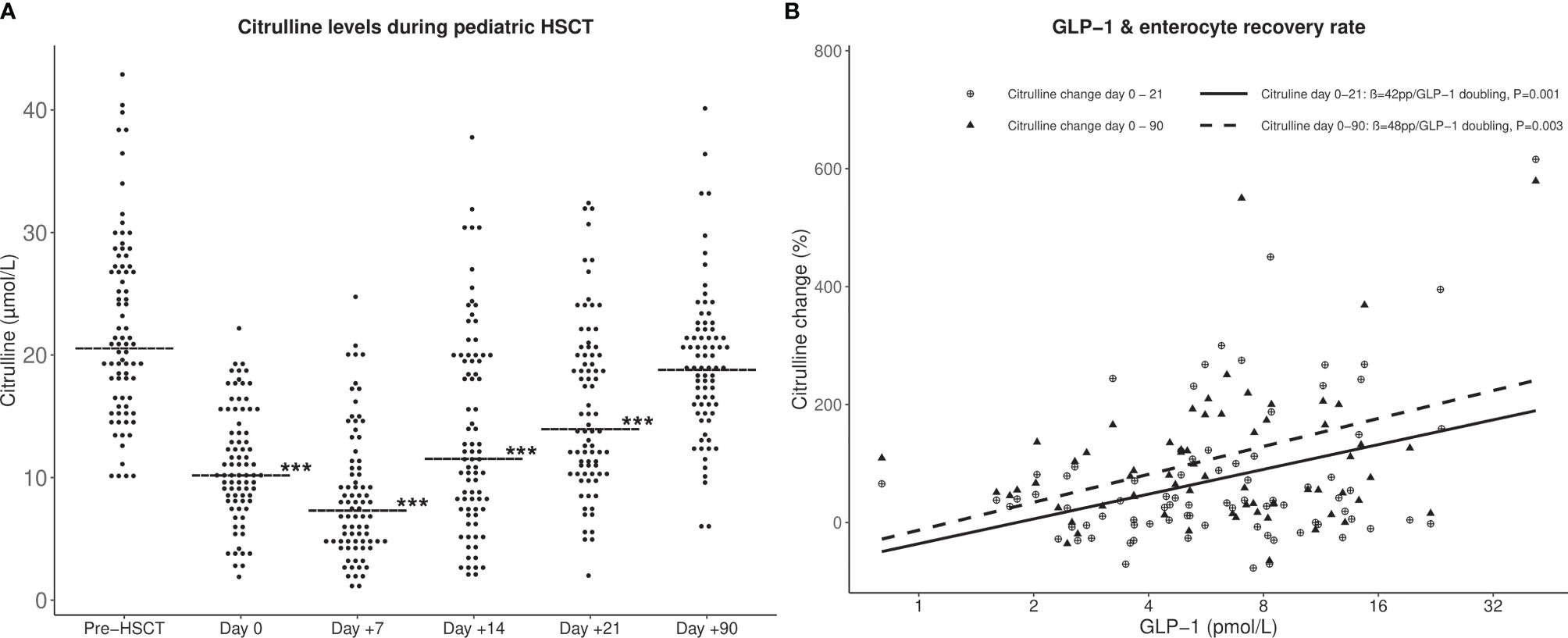
Figure 3 (A) Citrulline levels during HSCT from before conditioning until 90 days post-HSCT. Horizontal line: median citrulline. ***Citrulline levels significantly lower than pre-HSCT levels (P < 0.001). (B) Associations between GLP-1 levels at day of transplant (day 0) and relative citrulline increase from day 0 to day +21 (solid line) and from day 0 to day +90 (dashed line). P-values by simple linear regression models. pp, percentage point increase in citrulline.
To further investigate an effect of GLP-1 on epithelial repair, associations between GLP-1 levels and the rate of citrulline recovery were explored. GLP-1 levels at day 0 were significantly associated with the relative increase in citrulline levels from day 0 to +21 (42 percentage point (pp)/GLP-1 doubling; 95% CI, 18 – 66pp; P = 0. 001) and from day 0 to +90 (48 pp/GLP-1 doubling; 95% CI, 17 – 77pp, P = 0. 003) (Figure 3B). This remained significant in multivariate analyses adjusting for conditioning regimen (34; 95% CI, 10 – 58; P = 0. 008, and 48 pp/GLP-1 doubling; 95% CI, 17 – 79pp; P = 0. 004, respectively).
Clinical Outcomes
A total of 35 patients (43%) developed acute graft-versus-host disease (aGvHD) with onset at median day +14 (range: +5 to +34); grades III–IV aGvHD were seen in nine patients (11%). Patients with day 0 GLP-1 levels above the upper quartile showed a reduced risk of developing aGvHD (cause-specific HR: 0.3; 95% CI, 0.2-0.9, P = 0.02) (Figure 4A). In a multivariate analysis adjusting for the conditioning regimen this remained significant (HR: 0.4; 95% CI, 0.2 – 1.0, P = 0.04). Three and two patients developed steroid-dependent and steroid-refractory aGvHD, respectively. Although this limited number did not allow for conclusions regarding association with GLP-1 levels we noticed that none of these five patients had GLP-1 levels in the upper quartile (Figure 4B).
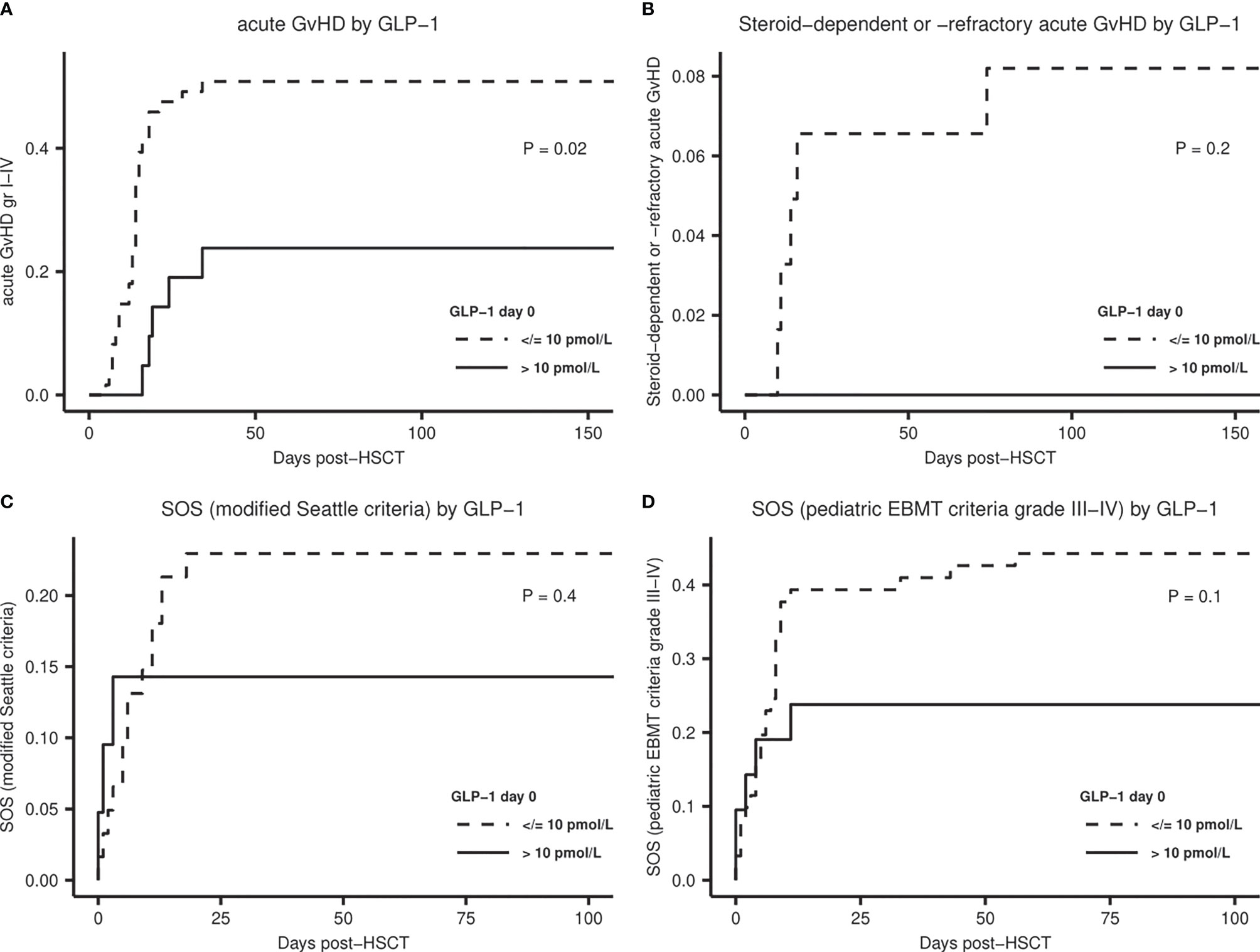
Figure 4 Cumulative incidence plots for acute treatment-related complications stratified by GLP-1 level at day 0 (≤10 pmol/L). (A) Acute GvHD, (B) Steroid-dependent or steroid-refractory acute GvHD, (C) Sinusoidal obstruction syndrome (SOS) diagnosed according to the modified Seattle criteria. (D) SOS diagnosed according to the pediatric EBMT criteria, severity grade III-IV. P-values by Gray’s test.
SOS was diagnosed in 17 (21%) of the patients according to the modified Seattle criteria (33) and in 41 (50%) according to the pediatric EBMT criteria (34), with severity grades III–IV in 32 (39%) of the patients. The frequency of SOS was not related to pre-transplant diagnosis. CRPmax was significantly associated with risk of SOS, also in multivariate analyses, both according to the modified Seattle criteria (OR = 2.1 per CRPmax doubling; 95% CI, 1.3 – 3.9; P = 0. 009) and to the pediatric EBMT grade III-IV (OR = 1.7 per CRPmax doubling; 95% CI, 1.1 – 2.7; P = 0.02). Cumulative incidence plots showed a tendency to lower incidences of SOS over time in patients with GLP-1 levels above the upper quartile (Figures 4C, D), even though not reaching statistical significance.
Ten patients (12%) developed bacteremia at median day +22 (0 to +84) post-HSCT. We were not able to make any conclusions regarding associations between bacteremia and GLP-1 or citrulline levels, most likely due to the limited incidence.
Median follow-up time was 2.0 years (1.0 – 4.7) from transplantation. Three patients died after HSCT, two of these due to treatment-related complications and one patient did not engraft. Of the 48 patients transplanted for a malignant disease, two patients relapsed. These limited numbers did not allow conclusions regarding prediction of survival by GLP-1 levels.
Discussion
In the present study, we investigated fasting levels of GLP-1 during the course of allogeneic HSCT to achieve new insights into the potentially protective effects of GLP-1 on treatment-related toxicity.
Although increased before start of conditioning in comparison with sex- and age-matched healthy children and adolescents, GLP-1 levels tended to decline during the course of transplant, most pronounced in patients receiving high-intensity conditioning therapy. A high GLP-1 level at day 0, the start of the toxic-aplastic phase, was associated with less systemic inflammation and with a faster recovery of enterocytes in terms of citrulline increase, even within the same conditioning group, indicating a protective and restorative effect of GLP-1. These findings translated into a reduced risk of aGvHD in patients with high GLP-1 levels.
Various animal studies have substantiated the intestinotrophic effects of GLP-1, which have been found comparable in size with the well-known effects of the closely related and co-secreted GLP-2 (13, 17, 19). Endogenous GLP-1 has been shown to be important for intestinal mucosal recovery in mice treated with chemotherapy (13, 14). The underlying mechanism of the intestinotrophic effects of both GLP-1 and GLP-2 are poorly understood. A signal interaction where GLP-1 potentiates the trophic effect of GLP-2 has been suggested (35), and co-treatment with the two peptides showed superior effects in recovery of chemotherapy-induced mucositis in mice compared with GLP-1 or GLP-2 monotherapy (14). Additional protective effects of GLP-1 have been suggested by studies demonstrating GLP-1-induced secretion of antimicrobial peptides from Paneth cells (36) and activated production of the barrier-protective mucus layer by Brunner’s glands (37). Furthermore, GLP-1 seems capable of directly modulating local inflammation in the gut by its interaction with intestinal epithelial lymphocytes leading to reduced pro-inflammatory cytokine secretion (20). A mucosal restorative effect of GLP-1 was observed in the present study, as GLP-1 levels at day of HSCT positively associated with post-HSCT citrulline increase, reflecting accelerated recovery of functioning enterocytes in patients with higher GLP-1. Together, the pleiotropic effects of GLP-1 could potentially contribute to the protection against a harmful systemic inflammatory response following the toxic impact on the intestinal epithelium and its down-stream effects in terms of non-infectious organ toxicities. Our data indicates that the ability to maintain GLP-1 levels after chemotherapy is important for protecting the gut against the damaging effects of the cytotoxic treatment and the resulting severe systemic inflammatory response. In other words, we suggest that GLP-1 may be defined as a factor enhancing “tissue tolerance”, which has appeared to be critical for disease severity in general (38). The demonstrated association between GLP-1 levels and aGvHD, even within the same group of conditioning regimen, in the present study, lend support to a protective role of GLP-1 against treatment-related complications and in line with our findings, a recent study by Norona et al. indicated L cells being a target of aGvHD and showed that lower numbers of L-cells were associated with increased mortality risk in adult patients with aGvHD (39). Despite a significant association between peak CRP levels and SOS, we were unable to demonstrate any significant association between GLP-1 and SOS, most likely due to lack of power in combination with the complex mechanisms behind SOS involving several other predisposing factors.
The factors that determine the rate of GLP-1 secretion and the resulting circulating GLP-1 levels during intestinal traumas are not fully understood. Indeed, a number of studies including both animal and clinical studies have suggested that the gut may respond to cytotoxic treatment by increasing GLP-1 secretion (13–16), thereby potentially counterbalancing the effects of chemotherapy-induced loss of epithelial cells, possibly including the GLP-1 secreting L-cells (39). The data of the present study, however, does not indicate that such putative mechanism could be adequately effective to maintain sufficient plasma GLP-1 levels in the subgroup undergoing the most intensive myeloablative conditioning therapy, as GLP-1 levels tended to decline after the transplant along with a more pronounced loss of enterocyte mass (shown as more pronounced citrulline decline) and increased inflammation.
Previous studies have suggested the use of GLP-1 as a possible treatment of chemotherapy-induced mucositis (13, 18). Administration of GLP-1 analogs in mice treated with chemotherapy ameliorated mucositis and accelerated healing of the intestinal injury (13, 14). In patients with diabetes, obesity and psoriasis, treatment with GLP-1 analogs has been found to cause reduction in chronic low-grade inflammation (40–43). Although the mechanism is debated and probably confounded by the metabolic-improving effects of GLP-1 treatment (43), amelioration of the putative dysfunctional intestinal barrier might play a role in these conditions (44).
Although the results of the present study point to the relevance of further exploring the clinical potential of GLP-1 to ameliorate side effects of cytotoxic treatment and transplantation, it should be emphasized that GLP-1 treatment is known to cause anorexia and nausea (45), which are already unwanted side-effects for patients receiving chemotherapy. Further investigations including oral glucose tolerance test and clinical trials are needed to evaluate the tolerance of GLP-1 analogs in HSCT patients. Additionally, supplementary data on mucositis scoring and nutritional intake were not available in the present study but would be of interest in future studies.
Previous studies in animals and humans have indicated that GLP-1 is secreted in response to intestinal injury (13, 14, 16). An enhanced GLP-1-secretion may, according to both in vitro and rodent studies, be mediated by toll-like receptors at the basolateral sides of the L-cells, which become exposed to endotoxins due to mucosal barrier disruption (16, 46, 47). Such mechanisms might be active in critically ill patients where fasting GLP-1 levels have been found elevated (25, 47). In this study, GLP-1 levels in the patients, including pre-transplant levels, were generally higher than in healthy controls, suggesting that similar mechanisms may, to some extent, be activated already at the timepoint of referral in these often severely affected patients. Our findings, however, appear to be in contrast to a study by Skoczén et al., reporting decreased GLP-1 levels before conditioning in pediatric HSCT patients (48). The reason for this discrepancy is unclear and difficult to judge based on the limited number of participants in their study (27 patients; of those nine had a non-malignant diagnosis) and with an absence of observations in the early post-transplant period.
A strength of the present study is the meticulous design with numerous, consecutive and precise time points for GLP-1 measurements, both pre- and post-HSCT, as well as the inclusion of a large control cohort. Yet, this study is limited by its lack of data on mucositis scoring and nutritional intake, which potentially could have strengthened our conclusions. Moreover, a larger cohort is needed to obtain statistical power to detect possible associations between GLP-1 and risk of inflammatory treatment-related complications, including SOS and aGvHD.
In conclusion, we have presented evidence of an association between endogenous GLP-1 and enterocyte recovery rate and the degree of systemic inflammation in pediatric patients undergoing HSCT. These findings lend support to the notion that GLP-1 may contribute to the well-known large inter-individual variability in the tolerance to chemotherapy and irradiation and suggest new potential therapeutic strategies to prevent toxicities related to chemotherapy in HSCT.
Data Availability Statement
The raw data supporting the conclusions of this article will be made available by the authors, without undue reservation.
Ethics Statement
The studies involving human participants were reviewed and approved by the Capital Region of Denmark’s Ethical Committee (H-7-2014-016). Written informed consent to participate in this study was provided by the participants’ legal guardian/next of kin.
Author Contributions
ME contributed to data collection, performed laboratory and statistical analyses and interpretation and drafted the manuscript. HL, BH, and JH contributed to project design, laboratory analyses and data interpretation. KK and KS established sample collection design and contributed to sample collection. SS, CF-B, CF, J-CH, and TH contributed to sample collection and data interpretation. KM designed the project, established the collaboration and contributed to data interpretation. All authors critically revised the manuscript and gave their final approval of the version to be published.
Funding
Financial support was obtained from The Research Council at Rigshospitalet, The Childhood Cancer Foundation (#2017-1997), the NNF Center for Basic Metabolic Research, University of Copenhagen and the BRIDGE – Translational Excellence Programme (bridge.ku.dk) at the Faculty of Health and Medical Sciences, University of Copenhagen, funded by the Novo Nordisk Foundation (#NNF18SA0034956).
Conflict of Interest
The authors declare that the research was conducted in the absence of any commercial or financial relationships that could be construed as a potential conflict of interest.
Publisher’s Note
All claims expressed in this article are solely those of the authors and do not necessarily represent those of their affiliated organizations, or those of the publisher, the editors and the reviewers. Any product that may be evaluated in this article, or claim that may be made by its manufacturer, is not guaranteed or endorsed by the publisher.
References
1. Thornley I, Lehmann LE, Sung L, Holmes C, Spear JM, Brennan L, et al. A Multiagent Strategy to Decrease Regimen-Related Toxicity in Children Undergoing Allogeneic Hematopoietic Stem Cell Transplantation. Biol Blood Marrow Transplant (2004) 10(9):635–44. doi: 10.1016/j.bbmt.2004.06.004
2. Bowen JM, Wardill HR. Advances in the Understanding and Management of Mucositis During Stem Cell Transplantation. Curr Opin Support Palliat Care (2017) 11(4):341–6. doi: 10.1097/SPC.0000000000000310
3. Takahashi K, Soga Y, Murayama Y, Udagawa M, Nishimoto H, Sugiura Y, et al. Oral Mucositis in Patients Receiving Reduced-Intensity Regimens for Allogeneic Hematopoietic Cell Transplantation: Comparison With Conventional Regimen. Support Care Cancer (2010) 18(1):115–9. doi: 10.1007/s00520-009-0637-z
4. Gibson RJ, Bowen JM. Biomarkers of Regimen-Related Mucosal Injury. Cancer Treat Rev (2011) 37:487–93. doi: 10.1016/j.ctrv.2011.05.007
5. Blijlevens NMA, Donnelly JP, de Pauw BE. Prospective Evaluation of Gut Mucosal Barrier Injury Following Various Myeloablative Regimens for Haematopoietic Stem Cell Transplant. Bone Marrow Transplant (2005) 35(7):707–11. doi: 10.1038/sj.bmt.1704863
6. van der Velden WJFM, Herbers AHE, Feuth T, Schaap NPM, Donnelly JP, Blijlevens NMA. Intestinal Damage Determines the Inflammatory Response and Early Complications in Patients Receiving Conditioning for a Stem Cell Transplantation. PloS One (2010) 5(12):e15156. doi: 10.1371/journal.pone.0015156
7. Jordan K, Pontoppidan P, Uhlving HH, Kielsen K, Burrin DG, Weischendorff S, et al. Gastrointestinal Toxicity, Systemic Inflammation, and Liver Biochemistry in Allogeneic Hematopoietic Stem Cell Transplantation. Biol Blood Marrow Transplant (2017) 23(7):1170–6. doi: 10.1016/j.bbmt.2017.03.021
8. Takatsuka H, Takemoto Y, Yamada S, Wada H, Tamura S, Fujimori Y, et al. Complications After Bone Marrow Transplantation Are Manifestations of Systemic Inflammatory Response Syndrome. Bone Marrow Transplant (2000) 26:419–26. doi: 10.1038/sj.bmt.1702517
9. Sonis ST. Pathobiology of Mucositis. Nat Rev Cancer (2004) 20:11–5. doi: 10.1053/j.soncn.2003.10.003
11. Elliott RM, Morgan LM, Tredger JA, Deacon S, Wright J, Marks V. Glucagon-Like Peptide-1(7-36)Amide and Glucose-Dependent Insulinotropic Polypeptide Secretion in Response to Nutrient Ingestion in Man: Acute Post-Prandial and 24-H Secretion Patterns. J Endocrinol (1993) 138(1):159–66. doi: 10.1677/joe.0.1380159
12. Orskov C, Wettergren A, Holst JJ. Secretion of the Incretin Hormones Glucagon-Like Peptide-1 and Gastric Inhibitory Polypeptide Correlates With Insulin Secretion in Normal Man Throughout the Day. Scand J Gastroenterol (1996) 31(7):665–70. doi: 10.3109/00365529609009147
13. Kissow H, Hartmann B, Holst JJ, Poulsen SS. Glucagon-Like Peptide-1 as a Treatment for Chemotherapy-Induced Mucositis. Gut (2013) 62(12):1724–33. doi: 10.1136/gutjnl-2012-303280
14. Hytting-andreasen R, Balk-m E, Hartmann B, Kissow H. Endogenous Glucagon-Like Peptide- 1 and 2 Are Essential for Regeneration After Acute Intestinal Injury in Mice. PloS One (2018) 1–14. doi: 10.1371/journal.pone.0198046
15. Ebbesen MS, Kissow H, Hartmann B, Grell K, Gørløv JS, Kielsen K, et al. Glucagon-Like Peptide-1 Is a Marker of Systemic Inflammation in Patients Treated With High-Dose Chemotherapy and Autologous Stem Cell Transplantation. Biol Blood Marrow Transplant (2019) 25(6):1085–91. doi: 10.1016/j.bbmt.2019.01.036
16. Lebrun LJ, Lenaerts K, Kiers D, Drucker DJ, Lagrost L, Grober J. Enteroendocrine L Cells Sense LPS After Gut Barrier Injury to Enhance GLP-1 Secretion. Cell Rep (2017) 21:1160–8. doi: 10.1016/j.celrep.2017.10.008
17. Kissow H, Hartmann B, Holst JJ, Viby NE, Hansen LS, Rosenkilde MM, et al. Glucagon-Like Peptide-1 (GLP-1) Receptor Agonism or DPP-4 Inhibition Does Not Accelerate Neoplasia in Carcinogen Treated Mice. Regul Pept (2012) 179(1–3):91–100. doi: 10.1016/j.regpep.2012.08.016
18. Hunt JE, Holst JJ, Jeppesen PB, Kissow H. Glp-1 and Intestinal Diseases. Biomedicines (2021) 9(4):1–14. doi: 10.3390/biomedicines9040383
19. Dahlgren D, Sjöblom M, Hellström PM, Lennernäs H. Chemotherapeutics-Induced Intestinal Mucositis: Pathophysiology and Potential Treatment Strategies. Front Pharmacol (2021) 12:1–12. doi: 10.3389/fphar.2021.681417
20. Yusta B, Baggio LL, Koehler J, Holland D, Cao X, Pinnell LJ, et al. GLP-1R Agonists Modulate Enteric Immune Responses Through the Intestinal Intraepithelial Lymphocyte GLP-1r. Diabetes (2015) 64(7):2537–49. doi: 10.2337/db14-1577
21. Anbazhagan AN, Thaqi M, Priyamvada S, Jayawardena D, Kumar A, Gujral T, et al. GLP-1 Nanomedicine Alleviates Gut Inflammation. Nanomedicine Nanotechnol Biol Med (2017) 179(110):95–105. doi: 10.1016/j.nano.2016.08.004
22. Kammersgaard MB, Kielsen K, Heilmann C, Ifversen M, Müller K. Assessment of the Proposed EBMT Pediatric Criteria for Diagnosis and Severity Grading of Sinusoidal Obstruction Syndrome. Bone Marrow Transplant (2019) 54:1406–18. doi: 10.1038/s41409-018-0426-8
23. Bacigalupo A, Ballen K, Rizzo D, Giralt S, Lazarus H, Ho V, et al. Defining the Intensity of Conditioning Regimens: Working Definitions. Biol Blood Marrow Transplant (2009) 15:1628–33. doi: 10.1016/j.bbmt.2009.07.004
24. Lausten-Thomsen U, Christiansen M, Fonvig CE, Trier C, Pedersen O, Hansen T, et al. Reference Values for Serum Total Adiponectin in Healthy Non-Obese Children and Adolescents. Clin Chim Acta (2015) 450:11–4. doi: 10.1016/j.cca.2015.07.012
25. Stinson SE, Jonsson AE, Lund MAV, Frithioff-Bøjsøe C, Holm LA, Pedersen O, et al. Fasting Plasma GLP-1 Is Associated With Overweight/Obesity and Cardiometabolic Risk Factors in Children and Adolescents. J Clin Endocrinol Metab (2021) 106(6):1718–27. doi: 10.1210/clinem/dgab098
26. Nielsen TRH, Lausten-Thomsen U, Fonvig CE, Bøjsøe C, Pedersen L, Bratholm PS, et al. Dyslipidemia and Reference Values for Fasting Plasma Lipid Concentrations in Danish/North-European White Children and Adolescents. BMC Pediatr (2017) 17(1):116. doi: 10.1186/s12887-017-0868-y
27. Bak MJ, Wewer Albrechtsen NJ, Pedersen J, Knop FK, Vilsbøll T, Jørgensen NB, et al. Specificity and Sensitivity of Commercially Available Assays for Glucagon-Like Peptide-1 (GLP-1): Implications for GLP-1 Measurements in Clinical Studies. Diabetes Obes Metab (2014) 16(11):1155–64. doi: 10.1111/dom.12352
28. Orskov C, Rabenhoj L, Wettergren A, Kofod H, Holst JJ. Tissue and Plasma-Concentrations of Amidated and Glycine-Extended Glucagon-Like Peptide-I in Humans. Diabetes (1994) 43(4):535–9. doi: 10.2337/diab.43.4.535
29. Chaimbault P, Petritis K, Elfakir C, Dreux M. Determination of 20 Underivatized Proteinic Amino Acids by Ion-Pairing Chromatography and Pneumatically Assisted Electrospray Mass Spectrometry. J Chromatogr A (1999) 855(1):191–202. doi: 10.1016/S0021-9673(99)00685-8
30. Gray RJ. A Class of $K$-Sample Tests for Comparing the Cumulative Incidence of a Competing Risk. Ann Stat (1988) 3:1141–54.
31. R Core Team.. R: A Language and Environment for Statistical Computing [Internet]. Vienna, Austria: R Foundation for Statistical Computing (2019). Available at: https://www.r-project.org/.
32. Fragkos KC, Forbes A. Citrulline as a Marker of Intestinal Function and Absorption in Clinical Settings: A Systematic Review and Meta-Analysis. United Eur Gastroenterol J (2018) 6:181–91. doi: 10.1177/2050640617737632
33. Mcdonald GB, Sharma P, Matthews DE, Shulman HM, Thomas ED. Venocclusive Disease of the Liver After Bone Marrow Transplantation: Diagnosis, Incidence, and Predisposing Factors. Hepatology (1984) 4(1):116–22. doi: 10.1002/hep.1840040121
34. Corbacioglu S, Carreras E, Ansari M, Balduzzi A, Cesaro S, Dalle JH, et al. Diagnosis and Severity Criteria for Sinusoidal Obstruction Syndrome/Veno-Occlusive Disease in Pediatric Patients: A New Classification From the European Society for Blood and Marrow Transplantation. Bone Marrow Transplant (2018) 53(2):138–45. doi: 10.1038/bmt.2017.161
35. Koehler JA, Baggio LL, Yusta B, Longuet C, Rowland KJ, Cao X, et al. GLP-1R Agonists Promote Normal and Neoplastic Intestinal Growth Through Mechanisms Requiring Fgf7. Cell Metab (2015) 21(3):379–91. doi: 10.1016/j.cmet.2015.02.005
36. Kedees MH, Guz Y, Grigoryan M, Teitelman G. Functional Activity of Murine Intestinal Mucosal Cells Is Regulated by the Glucagon-Like Peptide-1 Receptor. Peptides (2013) 48:36–44. doi: 10.1016/j.peptides.2013.07.022
37. Bang-Berthelsen CH, Holm TL, Pyke C, Simonsen L, Søkilde R, Pociot F, et al. GLP-1 Induces Barrier Protective Expression in Brunner’s Glands and Regulates Colonic Inflammation. Inflamm Bowel Dis (2016) 22(9):2078–97. doi: 10.1097/MIB.0000000000000847
38. Wu S-R, Reddy P. Regulating Damage From Sterile Inflammation: A Tale of Two Tolerances. Trends Immunol (2017) 38(4):231–5. doi: 10.1016/j.it.2017.02.005
39. Norona J, Apostolova P, Schmidt D, Ihlemann R, Reischmann N, Taylor G, et al. Glucagon-Like Peptide 2 for Intestinal Stem Cell and Paneth Cell Repair During Graft-Versus-Host Disease in Mice and Humans. Blood (2020) 136(12):1442–55. doi: 10.1182/blood.2020005957
40. He L, Wong CK, Cheung KK, Yau HC, Fu A, Zhao H, et al. Anti-Inflammatory Effects of Exendin-4, a Glucagon-Like Peptide-1 Analog, on Human Peripheral Lymphocytes in Patients With Type 2 Diabetes. J Diabetes Investig (2013) 4(4):382–92. doi: 10.1111/jdi.12063
41. Ceriello A, Novials A, Ortega E, Canivell S, La Sala L, Pujadas G, et al. Glucagon-Like Peptide 1 Reduces Endothelial Dysfunction, Inflammation, and Oxidative Stress Induced by Both Hyperglycemia and Hypoglycemia in Type 1 Diabetes. Diabetes Care (2013) 36(8):2346–50. doi: 10.2337/dc12-2469
42. Hogan AE, Gaoatswe G, Lynch L, Corrigan MA, Woods C, O’Connell J, et al. Glucagon-Like Peptide 1 Analogue Therapy Directly Modulates Innate Immune-Mediated Inflammation in Individuals With Type 2 Diabetes Mellitus. Diabetologia (2014) 57(4):781–4. doi: 10.1007/s00125-013-3145-0
43. Drucker DJ, Rosen CF. Glucagon-Like Peptide-1 (GLP-1) Receptor Agonists, Obesity and Psoriasis: Diabetes Meets Dermatology. Diabetologia (2011) 54(11):2741–4. doi: 10.1007/s00125-011-2297-z
44. De Kort S, Keszthelyi D, Masclee AAM. Leaky Gut and Diabetes Mellitus: What Is the Link? Obes Rev (2011) 12(6):449–58. doi: 10.1111/j.1467-789X.2010.00845.x
45. Drucker DJ. Mechanisms of Action and Therapeutic Application of Glucagon-Like Peptide-1. Cell Metab (2018) 27(4):740–56. doi: 10.1016/j.cmet.2018.03.001
46. Nguyen AT, Mandard S, Dray C, Deckert V, Valet P, Besnard P, et al. Lipopolysaccharides-Mediated Increase in Glucose-Stimulated Insulin Secretion: Involvement of the GLP-1 Pathway. Diabetes (2014) 63(2):471–82. doi: 10.2337/db13-0903
47. Kahles F, Meyer C, Möllmann J, Diebold S, Findeisen HM, Lebherz C, et al. GLP-1 Secretion Is Increased by Inflammatory Stimuli in an IL-6-Dependent Manner, Leading to Hyperinsulinemia and Blood Glucose Lowering. Diabetes (2014) 63(10):3221–9. doi: 10.2337/db14-0100
Keywords: hematopoietic stem cell transplantation, high-dose chemotherapy, glucagon-like peptide-1, toxicity, systemic inflammation, pediatrics, growth factors
Citation: Ebbesen M, Kissow H, Hartmann B, Kielsen K, Sørensen K, Stinson SE, Frithioff-Bøjsøe C, Esmann Fonvig C, Holm J-C, Hansen T, Holst JJ and Müller KG (2021) Glucagon-Like Peptide-1 Is Associated With Systemic Inflammation in Pediatric Patients Treated With Hematopoietic Stem Cell Transplantation. Front. Immunol. 12:793588. doi: 10.3389/fimmu.2021.793588
Received: 12 October 2021; Accepted: 22 November 2021;
Published: 08 December 2021.
Edited by:
Daniela Novick, Weizmann Institute of Science, IsraelReviewed by:
Shigeo Fuji, Osaka International Cancer Institute, JapanMalgorzata Wojcik, Jagiellonian University Medical College, Poland
Copyright © 2021 Ebbesen, Kissow, Hartmann, Kielsen, Sørensen, Stinson, Frithioff-Bøjsøe, Esmann Fonvig, Holm, Hansen, Holst and Müller. This is an open-access article distributed under the terms of the Creative Commons Attribution License (CC BY). The use, distribution or reproduction in other forums is permitted, provided the original author(s) and the copyright owner(s) are credited and that the original publication in this journal is cited, in accordance with accepted academic practice. No use, distribution or reproduction is permitted which does not comply with these terms.
*Correspondence: Maria Ebbesen, maria.schou.ebbesen@regionh.dk