- 1Department of General Surgery, Taizhou Central Hospital (Taizhou University Hospital), Taizhou, China
- 2Department of General Surgery, Second Xiangya Hospital, Central South University, Changsha, China
- 3Department of Clinical Laboratory, Taizhou Central Hospital (Taizhou University Hospital), Taizhou, China
Hepatocellular carcinoma (HCC) is the third leading cause of cancer-related deaths worldwide and is increasing in incidence. Local ablative therapy plays a leading role in HCC treatment. Radiofrequency (RFA) is one of the first-line therapies for early local ablation. Other local ablation techniques (e.g., microwave ablation, cryoablation, irreversible electroporation, phototherapy.) have been extensively explored in clinical trials or cell/animal studies but have not yet been established as a standard treatment or applied clinically. On the one hand, single treatment may not meet the needs. On the other hand, ablative therapy can stimulate local and systemic immune effects. The combination strategy of immunotherapy and ablation is reasonable. In this review, we briefly summarized the current status and progress of ablation and immunotherapy for HCC. The immune effects of local ablation and the strategies of combination therapy, especially synergistic strategies based on biomedical materials, were discussed. This review is hoped to provide references for future researches on ablative immunotherapy to arrive to a promising new era of HCC treatment.
Introduction
Primary liver cancer is one of the most common malignant tumors in the digestive system and the third leading cause of cancer-related deaths (1). Hepatocellular carcinoma (HCC), which comprises ~90% of cases, is the most common type of primary liver cancer. The management of HCC lies on the Barcelona Clinic Liver Cancer (BCLC) staging system. Most clinical practices guidelines recommend resection, thermal ablation and transplantation for patients with early HCC (BCLC 0, A), whereas transarterial chemoembolization (TACE) and systemic therapies are preferred for patients with intermediate (BCLC B) and advanced (BCLC C) HCCs, respectively (2–5). Surgical resection and transplantation could offer the best chance for a cure in early HCC, but not all patients with early-stage HCC, especially those with cirrhosis, benefit from these treatments. Liver function and portal hypertension are the fatal selection criteria of resection, because 80%-90% HCCs develop from cirrhosis (6). Moreover, the recurrence rate after HCC resection reaches as high as 68% (7). Scientists and surgeons have exerted much effort into the removal of tumors (8). However, this task is still an insurmountable mountain, because HCC cannot be considered a local disease even in the early stage. The outcomes of liver transplantation are superior to that of hepatic resection. However, organ shortage, long waiting time, and high cost are deterred, except for the strict transplantation indication. Locoregional ablative therapy including radiofrequency ablation (RFA) and microwave ablation (MWA), is a potentially curative strategy for early HCC, coming into sight. The advent of the genomic era, as well as the increase in the understanding of the role of immunity in HCC progression, support targeted therapy and immunotherapy. The combination of ablative therapy and immunotherapy has been a subject of recent clinical and basic researches. Herein, we summarized ablative therapy and immunotherapy for HCC, discussed their synergistic anti-tumor effects, and envisaged the current trends and future prospects of their combination.
Ablation Therapy
Thermal ablation, demonstrates similar outcomes as hepatic resection in early HCC (tumors size < 2–3 cm) (9, 10). Other ablation therapies, such as cryoablation, have not established a standard clinic procedure and are therefore less used. In recent years, photodynamic therapy (PDT), photothermal therapy (PTT), magnetic hyperthermia therapy (MHT), and irreversible electroporation (IRE) have shown potential applications in HCC with the prevalence of biomaterials in medicine. The major mechanism of ablative therapies is to induce irreversible thermal (i.e., RFA, MWA, and PTT) or non-thermal tumor destruction (i.e., IRE and PDT) via electromagnetic or light energy. This section gives a brief retrospect of traditional ablative therapies for HCC, as well as novel ablative techniques (Figure 1), and discusses their immunological effects.
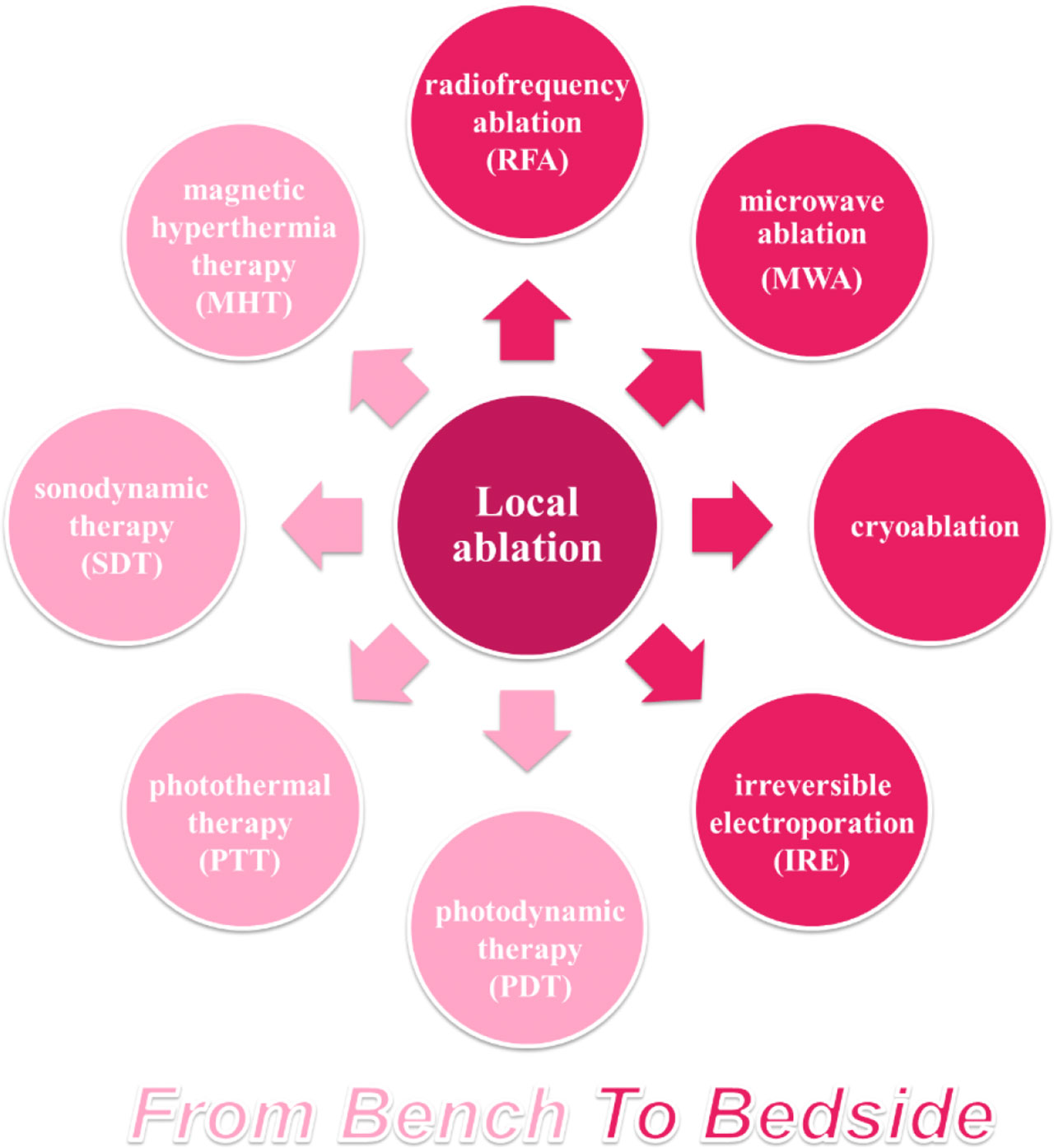
Figure 1 Overview of ablative techniques for HCC. Ablative strategy has occupied an important position among HCC therapies, based on thermal or non-thermal tumor destruction. RFA, the most common ablation technique applied for patients with HCC, has been developed as a standard treatment, while other ablative techniques have been explored in clinical or preclinical researches.
Clinical Applications
RFA, a standard ablative and first-line therapy for small-sized HCC, is more cost-effective than hepatic resection (10). RFA can achieve tumor necrosis at 375–480 kHz and > 60°C (11). However, traditional monopolar RFA is limited in tumors less than 2–3 cm or near vessels due to heat sink effect, which is also related to recurrence (12). Novel techniques are developed to improve ablation efficacy. No-touch multibipolar RFA can be used to tumors up to 5 cm with similar disease-free survival (DFS) and overall survival (OS) rates compared with resection (13). However, insufficient RFA (iRFA) is one of the major reasons for recurrence after RFA. iRFA could lead to HCC with a more aggressive phenotype, drug resistance and worse prognosis (Table 1). The ablative margin assessed by computed tomography (CT) after RFA can be an important predictor of local tumor progression (LTP) and overall recurrence. A study indicated that insufficient ablative margin (<5 mm) was associated with higher rates of LTP and overall recurrence in HCC, but the sensitivity values were only 36.4% and 46.2%, respectively (26). iRFA could promote the proliferation, migration, invasion, epithelial-mesenchymal transition (EMT), and angiogenesis of residual tumors through the transcriptional and epigenetic regulation. Some signaling pathways associated with tumor growth and progression, such as the Akt signaling pathway involved in cellular proliferation, survival and angiogenesis are activated after iRFA (20, 22, 24, 25).
Several strategies have been used to counter iRFA. One of which is to improve the accuracy of imaging guidance for the specific identification of tumor boundaries, especially with the application of nanotechnology. Jiang and colleagues developed a nanobubble conjugated with colony-stimulating factor 1 receptor (CSF-1R), called NBCSF-1R, for HCC margin detection (27). NBCSF-1R provided a non-invasive effective ultrasound imaging capabilities for evaluating therapy response of RFA through the high specificity targeting of CSF-1R-overexpressing macrophages and HCC tumor margin. Another strategy is the combination therapy for salvage. For instance, sorafenib and IFN-α combined with herbal compound inhibited the EMT of HCC cells after iRFA (28, 29); bevacizumab inhibited the tumor growth and angiogenesis induced by iRFA (30); and CTLA-4 blockade suppressed the growth of residual tumors and improved survival in a subcutaneous murine HCC model (31). Other agents include metformin (32) and hydroxychloroquine (HCQ) (33). However, one study demonstrated that ATPase inhibitory factor 1 (IF1) increased HCC cells’ resistance to sorafenib after iRFA (16). These results indicated that the application of systemic therapy or immunotherapy could cope with the adverse impacts of iRFA but the choice of agents could be limited by iRFA-induced resistance.
MWA could provide higher temperature with expanded ablation zone and shorter ablation time because of its higher frequency (900–2,450 MHz) (11). A recent study showed that MWA provided more excellent tumor control than RFA for patients with perivascular HCC (34, 35). In addition, a meta-analysis of randomized-controlled trials demonstrated that MWA seemed to benefit disease-free survivals at 5 years compared with RFA (36). New microwave thermosphere ablation (MTA) may provide a safer and more effective ablation with shorter time than RFA with the developments of novel MWA systems (37).
Cryoablation is also a thermal technique that could be more effective and safer for tumors not suitable for RFA or MWA, such as perivascular HCC. The goal of cryoablation is to destroy tumor tissue by alternating freezing and thawing on the basis of the Joule-Thomson effect, which benefits low risk vascular complications (38). Moreover, a multicenter randomized controlled trial demonstrated that cryoablation achieved lower local tumor progression than RFA with similar OS and DFS rates (39). IRE is a non-thermal ablative technique that mediates cell damage by changing cell permeability and cellular homeostasis, which lead to cell death (40). Although IRE is a relatively new technique and few clinical studies have been conducted, its safety and efficacy have been proven (41, 42). Similar to cryoablation, one of advantages of IRE is that this technique can be used for tumors not suitable for RFA or MWA, such as perivascular HCC (43).
In a word, ablation take an indispensable place in the clinical treatment of HCC. A series of new techniques have been developed to improve the ablation efficacy and zone to benefit more patients. However, these technologies are image-guided, and their efficacy is closely related to the skills of operators to some extent. This factor is a major barrier to application and an interfering factor that is difficult to eliminate in comparative studies.
Emerging Ablative Strategies
Phototherapy (e.g., PDT and PTT) is an emerging and prospective cancer therapeutic strategy. Phototherapy kills cancer cells through photochemical or photophysical effects to achieve therapeutic effects. Various photosensitizers (PSs), such as porphyrin-based PDT (44), 5-aminolaevulinic acid-PDT (45) and Radachlorin-PDT (46), could be applied for HCC. However, several factors need to be improved before these methods could be clinically used. First, light (laser) is one of the most indispensable elements in PDT and PTT, on which the therapeutic effect mostly depends. PSs and photothermal agents can be activated only when the light wavelength is in a specific range, known as therapeutic window. Moreover, light wavelength also determines the depth of tissue penetration, which limits percutaneous application of phototherapy to tumors in abdominal parenchymal organs, especially in deep parts. The rapid development of endoscopic techniques and biomedical materials gave rise to strategies to overcome the depth dependence. For example, Li et al. reported laparoscopic-assisted photothermal ablation method based on superparamagnetic iron oxide (SPIO) and new indocyanine green (ICG), called IR820@PEG-SPIO (47). More surprisingly, IR820@PEG-SPIO completely ablated orthotopic liver cancer in nude mice model, as well as detect early-stage HCC (diameter < 2 mm) via fluorescence, photoacoustic and magnetic resonance (MR) imaging. Compared with visible light, near infrared (NIR) light and X-Ray can provide deeper penetration (48–51). Besides, MHT, an alternative strategy, has been proposed to further overcome the limitation of penetration depth. Qian and colleagues developed a ferrimagnetic silk fibroin hydrogel (FSH) and demonstrated that FSH-mediated MHT, without depth limitation, could be more suitable for treating liver tumors compared with traditional PTT (52).
Nanoplatforms have stood out because they have improved therapeutic effects and reduced adverse effects, provide precise operation with optimized imaging guidance, and combine therapeutic strategies for synergistic anti-tumor effects. Zhu et al. designed a nanoparticle (ZnPc/SFB@BSA) that combined PDT, PTT and sorafenib with increased efficacy and decreased side effects of sorafenib (53). Jin and colleagues reported another nanoparticle loaded with sorafenib/indocyanine for PDT/PTT/chemotherapy, which could provide synergistic effects against HCC (54). Liu’s group has been devoted to designing different nanoplatforms for combined phototherapy/chemotherapy by aptamer (TLS11a) modification to enhance HCC-specific targeting (55–57). Nanoplatforms may provide more detailed and comprehensive information about tumor size, anatomical structure, and location and realize precise theranostic guidance by applying dual- or multimodal images that integrate optical and traditional medical images (e.g., CT and MR images). For instance, Qi et al. synthesized a NIR-II photoacoustic (PA) CT imaging-guided nanoagent for HCC theranostic strategy, called Pt@PDA-c (58). Pt@PDA-c had deep tissue penetration and high resolution, which provided accurate location of deep (~4 mm intraabdominal depth) and small (diameter < 5 mm) HCC lesions. Moreover, Pt@PDA-c-mediated PTT could eliminated HCC without recurrence under the guidance of real-time PACT.
Immunological Effects of Ablation
Ablation has long been considered a local treatment. However, growing evidence shows that ablation does more than physically eliminating tumors; it can also play a considerable role in distant lesions through immune effects, also known as abscopal effect. Changes in circulating immune cells/factors and tumor immune microenvironments have been explored by analyzing peripheral blood and tumor models. In 2005, Michael Geissler and colleagues found that local tumor ablation (percutaneous ethanol injection [PEI]/RFA) increased HCC immunogenicity in patients thus to promote endogenous adjuvants release and dendritic cell (DC) activation (59). Besides, RFA induced systemic immune variation in innate immune cells (including natural killer (NK) cells and plasmacytoid DCs) and adaptive immune cells (including tumor-specific T cells, antigen-presenting cells [APCs] and CD8 central memory T cells) (60–62). De novo or enhanced tumor-specific immune responses could be observed in patients with HCC after MWA (63). Wu and colleagues observed that neutrophil, monocyte and NK cell were increased to induce innate immune response and immunosuppressive lymphocyte was decreased in patients with HCC post-IRE (64). Moreover, their results indicated an ideal treatment window for immunotherapy (3–14 days post-IRE) to further control tumor recurrence and metastasis. Moreover, the expression of immune checkpoints (programmed cell death protein-1 [PD-1] and PD-1 cognate ligand [PD-L1]), which are associated with HCC tumor size, blood vessel invasion, and BCLC staging, can be downregulated by cryoablation but upregulated at recurrence (65).
The results observed in patients have also been further validated in various animal models. RFA increased CD8+ T cells, memory CD8+ T cells, and DCs and decreased regulatory T (Treg) cells in a unique murine model developed through a combination of intrasplenic inoculated oncogenic hepatocytes and carbon tetrachloride (66). Dai et al. reported that IRE could increase anti-tumor CD8+ T cells to prevent local tumor regrowth and distant metastasis and decrease immunosuppressive Treg and PD-1+ T cells in C57BL/6J mouse model bearing subcutaneous H22 hepatoma (67). Fong’s group demonstrated that IRE induced tumor antigens and facilitated granulocyte macrophage colony-stimulating factor plasmid transfer to achieve local and systemic anti-tumor responses in Yorkshire pig models (68, 69). Similarly, in other solid tumors, RFA can not only reduce the proportions of immunosuppressive cells (including Treg cells, tumor-associated macrophages and neutrophils), but increase the T cell infiltration as well as expression of the immune checkpoints (PD-1/PD-L1 and lymphocyte-activation gene 3 [LAG3]) in RFA-treated tumors and distant non-RFA tumors (70, 71). Moreover, serum and intra-tumoral cytokines, such as IFN-γ, IL-1α/β, IL-2/6/8/10, and TNFα/β, were also increased or activated (64, 72–75).
Increasing evidence have proved that ablation therapy could activate systemic anti-tumor immunological effects and inhibit immunosuppressive effects (Figure 2). However, RFA could also increase PD-1/PD-L1 expression, which was repressed by sunitinib with activation of immune response (66). This effect may facilitate checkpoint inhibitor therapy by constructing an immune-supportive microenvironment. Thus, combining ablation with immunotherapy is rational to achieve augmented and longer anti-tumor effects and prevent HCC progression with improved outcomes.
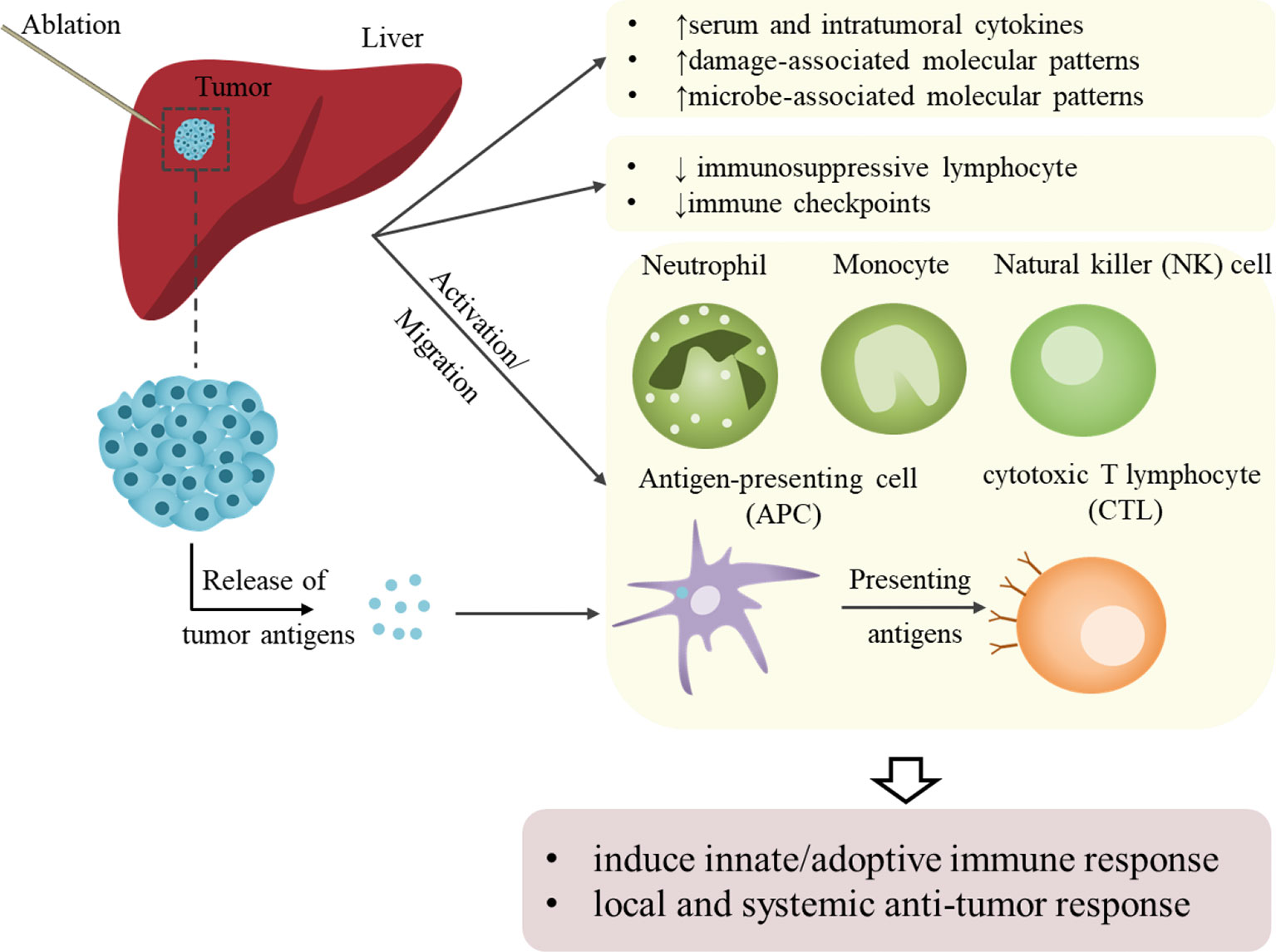
Figure 2 Schematic representation of ablation-induced immunological effects on HCC. Ablation assists local and systemic antitumor responses by activating antitumor immunity and suppressing immunosuppressive effects. On the one hand, the activation of or increase in innate immune cells and cytokines that kill tumor cells achieves non-specific tumor killing. The activation of or increase in adoptive immune cells and the release of tumor-associated or tumor-specific antigens mediates specific anti-tumor immunity. However, these immune effects brought by local ablation are relatively weak and could not meet the requirement needed to sustain anti-tumor effects and prevent recurrence.
Immunotherapy
The 5-year recurrence rates of early HCC with operation or ablation are as high as 70% (6). A retrospective study found that 64 of 103 patients with early/intermediate HCC who received RFA experienced recurrence (76). In addition to the pathophysiological characteristics of the HCC, incomplete treatment response results in the high post-operative recurrence rate, which negatively affects long-term survival. In a meta-analysis reviewing the recurrence rate of HCC after RFA over a ten-year period, the size, number, and location of tumors are partly responsible for incomplete treatment response, limiting the application of RFA in the early 2000s (77). With the introduction of multiple treatment modalities, such as RFA + PEI/TACE, these limitations have been broken and post-recurrence rates have been significantly reduced. However, to complicate matters further, recurrent tumors may be more aggressive (23, 78–80). Thus, adjuvant systemic therapy is taken in consideration. Sorafenib, a multi-tyrosine kinase inhibitor (TKI), has considerably improved the survival of patients with advanced HCC, whereas chemotherapy does not (81). Other emerging TKI drugs, including lenvatinib, regorafenib, cabozantinib and donafenib have been proved to improve the survival benefit of patients with advanced HCC (82–86). However, sorafenib, as an adjuvant therapy for HCC after resection or ablation, did not improved recurrence-free survival (RFS) (82). Furthermore, a phase III STORM trial established a predictive 146-gene signature, which comprised some genes involved in immune-related processes; however, the tested biomarkers and reported prognostic gene signatures lacked value in predicting adjuvant sorafenib on RFS (87). Surprisingly, iodine (131I)-labeled metuximab, an immunotherapeutic agent, proved to benefit RFS of post-operative or post-ablative patients with HCC, in particularly those with CD147+ (88, 89).
The immune system plays a critical role in HCC, particularly in the HCC development and progression, as well as the treatment response or tolerance (Figure 3). Bruno et al. elaborated the HCC immune microenvironment (e.g., antigens, molecular features, and immune cells), and reviewed HCC immunotherapies including immune checkpoint inhibitor (ICI)-based therapies, as well as others based on adoptive cells and vaccines (90). This section will not dwell on the above; instead, it will give a brief retrospect of the application of immune modulators and the advances in novel immunomodulatory strategies.
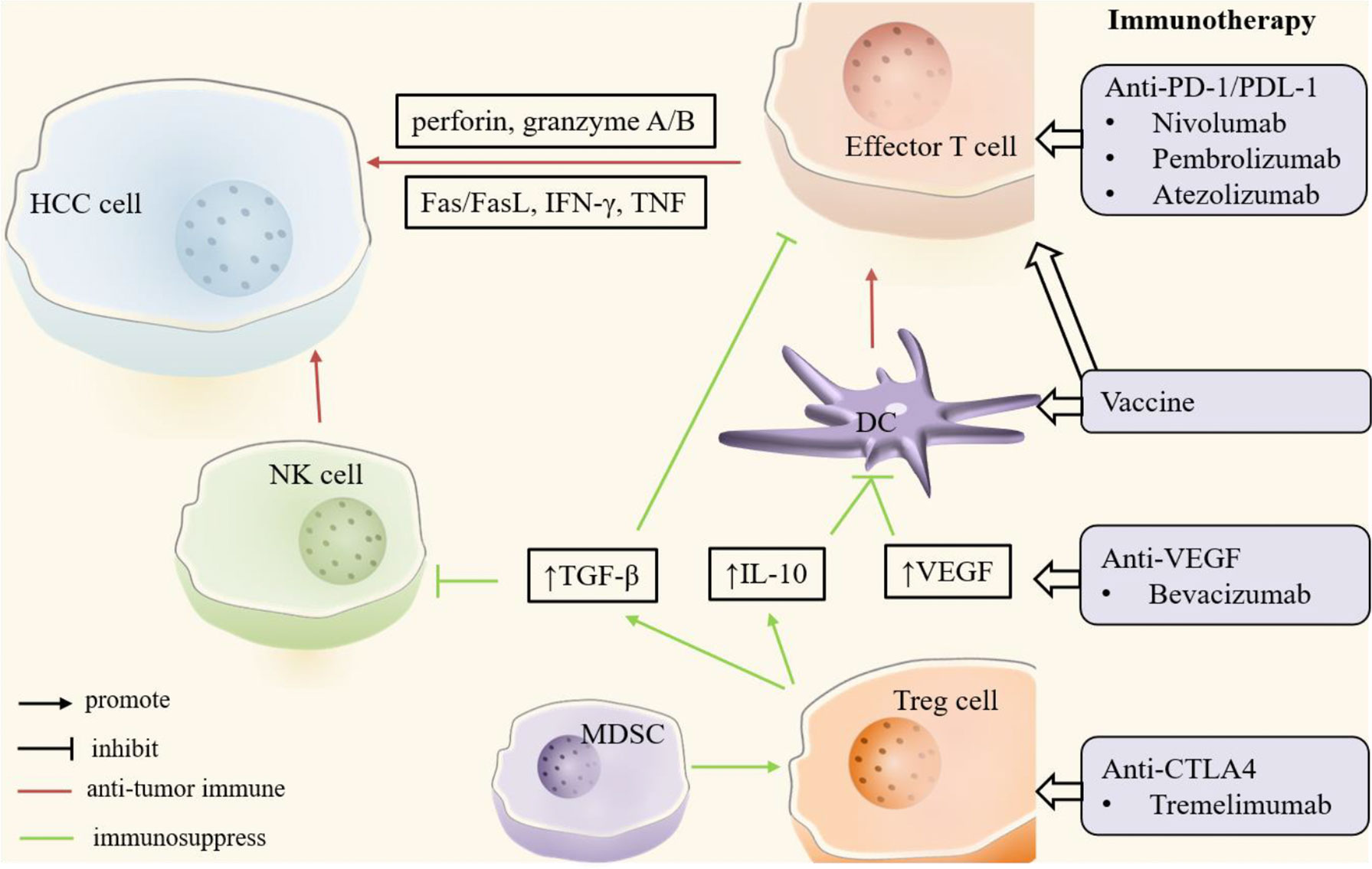
Figure 3 Key players in HCC immune microenvironment. In the HCC microenvironment, natural killer (NK) cells, dendritic cells (DCs) and effector T cells mainly play an anti-tumor immune role (red). Regulatory T (Treg) cells and myeloid-derived suppressor cells (MDSCs) promote tumor immune escape or drug resistance through immunosuppressive effects (green). In addition, tumor growth factor-β (TGF-β), interleukin-10 (IL-10) and other cytokines play an important role in tumor immunity. Immunotherapy enhances anti-tumor immunity or suppresses immunosuppression by targeting these critical cells and molecules. CTLA4, cytotoxic T lymphocyte-associated antigen 4; DC, dendritic cell; FasL, Fas ligand; HCC, hepatocellular carcinoma; IFN-γ, interferon-γ; IL-10, interleukin-10; MDSC, myeloid-derived suppressor cell; NK, natural killer; PD-1, programmed cell death protein-1; PDL-1, programmed cell death protein ligand -1; TGF-β, tumor growth factor-β; Treg, regulatory T; VEGF, vascular endothelial growth factor.
In short, the goal of immunomodulatory strategies is to activate anti-tumor immune response and/or suppress immune evasion. Immune checkpoints, the surface receptors expressed on immune system cells, include PD-1, PD-L1, cytotoxic T lymphocyte associated antigen 4 (CTLA4), LAG3, and T cell immunoglobulin and mucin domain containing-3 (TIM3) (91). Overexpressed PD-L1 in HCC cells can promote its binding with PD-1 on effector T cells, resulting in immune escape of tumor cells and apoptosis of T cells, which is conducive to the growth and progression of HCC (92). Overexpression of CTLA4 and TIM3 in Treg cells and overexpression of LAG3 and TIM3 in tumor infiltrating T lymphocytes can prevent the activation of effector T cells, also resulting in immune escape of tumor cells (90). The immune checkpoint is one of the immunosuppressive mechanisms that can help HCC immune escape by binding to corresponding ligands in HCC, which is also the rationale for the therapeutic application of ICIs. Recent clinical trials suggested that ICIs, whether alone or in combination with other agents, had a positive effect in HCC. Nivolumab (anti-PD-1), atezolizumab (anti-PD-L1), and tremelimumab (anti- CTLA4), have been proved to be safe and have effective anti-tumor responses for treating HCC (93–95). Notably, nivolumab and pembrolizumab well tolerated and effective in patients with advanced HCC after sorafenib failure with promising effects on long-term survival (96, 97). Atezolizumab, particularly in combination with bevacizumab (anti-VEGF), has superior performance compared with sorafenib in term of survival outcomes and the life quality of patients with unresectable HCC (98, 99).
Other immunotherapies, including adoptive immunotherapies (AITs) and immunotherapeutic vaccinations, activate anti-tumor immune response. AIT improves anti-tumor immunity by expanding or sensitizing lymphocytes in vitro and reinjecting them into patients, and cancer vaccines aim to enhance tumor-specific immune responses that are primarily activated by antigen-presenting cells (e.g., DCs) and produce endogenous TAAs. Although these treatments have not been studied as extensively as ICIs, they are under clinical studies. Clinical trials demonstrated the safety and efficacy of T cell- (100), DC- (101), and activated cytokine-induced killer (CIK) cell- (102) based adoptive immunotherapies, as well as oncolytic virus (103, 104) and peptide (105–107) vaccines for HCC. Glypican-3 (GPC3), a carcinoembryonic antigen ideal for immunotherapy target, has been studied extensively as an anti-tumor vaccine of HCC. Phase I/II clinical trials suggested that GPC3 peptide vaccine is effective in inducing cytotoxic T lymphocyte (CTL) killing cancer cells, reducing RFS, and improving OS, particularly in patients with GPC3-overexpressing HCC (105, 108, 109). An animal experiment demonstrated that the synergistic anti-tumor effects depended on increased GPC3-induced CTL though the combination of PD-1/PD-L1 blockade and GPC3 peptide vaccine (110). Moreover, a series of novel GPC3-targeting vaccine (111, 112) and antibodies (113–115) and cellular immunotherapeutic strategies (116–118) against GPC3 rely on the role of GPC3 in HCC and immunotherapy.
Strategies for enhancing therapeutic effects and monitoring immunotherapies have been developed based on advanced technologies. For instance, Liao et al. successfully applied NIR-II fluorescent imaging to NK cell-based immunotherapy for the real-time quantitative tracking and visualization of the viability of adoptive NK cells in vivo (119). The potency of immunotherapies can be enhanced by modification with specific antigens (120–122), mRNA optimization (123) and combination with adjuvants (124, 125).
Combined Ablative-Immunotherapy
As mentioned in Section 2.3, ablation techniques could induce local and systemic antitumor immune responses, but these responses are relatively weak, and cannot completely control the tumor. This reason explains the high local recurrence rates after treatment. RFA activated tumor-specific T cells, but it could not identify a new grown tumor or a recurrent tumor, which resulted in the tumor immune escape and recurrence in a HCC patient (60). Moreover, only 30% of patients with HCC achieved long-term remission and better DFS, because of the tumor-specific immune responses induced by MWA (63). The facts that the application of a single locoregional therapy has a high recurrence rate and locoregional ablation can induce anti-tumor immune responses, have led to the development of combined ablative and systemic therapy studies for recurrence reduction or treatment, as well as improved survival outcomes. Indeed, the advent of TKIs and immunotherapy have improved the outcomes of patients with HCC. Sorafenib, the most promising candidate for adjuvant chemotherapy, failed in patients with HCC after resection or ablation. Results from STORM trial in 2005 showed that compared with placebo, adjuvant sorafenib did not significantly improve RFS in patients with HCC post resection or ablation (126), which is consistent with the findings of existing randomized trials that showed no survival benefit for HCC patients after ablation with adjuvant sorafenib (127, 128). In addition, a study has shown that vitamin K combined with angiotensin converting enzyme inhibitors can inhibit the cumulative recurrence of HCC after treatment (129). A retrospective study has shown that angiotensin II receptor 1 blockers (sartans) can significantly improve overall survival and recurrence time in HCC patients after RFA (130), while another study have shown that this combination can only improve recurrence time (131). These results suggest that more rigorous randomized clinical trials are needed to verify the efficacy of this combination for HCC. On the other hand, the unsatisfying combinations indicated the emergence of immunotherapy as an adjuvant candidate.
In the VX2 tumor model, the combination of RFA and CpG-oligodeoxynuleotides vaccine prevented tumor progression and improved survival outcomes by enhancing anti-tumor T cell response and cytotoxicity (132). Using the CT26 tumor model, Liu et al. studied the roles of palliative RFA (pRFA) in T-cell immune responses and tumor recurrence, which could be more significant in combination with antibodies (74). Likewise, MWA combined with anti-PD-1/anti-CTLA-4 protected mice from recurrence with improved survival (133).
Clinical Combination on the Way
Table 2 reviews the finished clinical trials of the combinations of ablation and immunotherapy. Nivolumab and pembrolizumab, which are PD-1 blockades, received quick approvals as second line therapy for patients with HCC after sorafenib failure on the basis of CheckMate-040 (93) and KEYNOTE-224 (97). A recent proof-of-concept clinical trial suggested that the application of RFA or MWA enhanced the anti-tumor effects and response rates (from 10% to 24%) of nivolumab and pembrolizumab (135). The explanation for synergistic effects may be found in Section 2.3 in this review. In brief, the critical roles of RFA in T cell infiltration/response and PD-1 expression may be one of rationales for combining RFA with PD-1 blockade. Besides, the combination of RFA with tremelimumab (CTLA-4 blockade) have been also explored (134, 135).
Various studies have demonstrated the safe and effective to applicate adjuvant adoptive cellular immunotherapies to patients with HCC post-ablation with improved RFS and OS (102, 139–141). For patients with metastatic HCC, the combination of cryoablation and DC-CIK cell immunotherapy also achieved a significantly higher OS (median: 32 months) than cryoablation (median: 17.5 months) and the untreated group (median: 3 months) (144). Moreover, the multiple treatment modality for cryo-immunotherapy could provide better prognosis than the single one.
Notably, Tetsuya Nakatsura’s team found that RFA stood out among other locoregional therapies (including surgical resection and TACE) by referring to GPC3-specific T-cell-mediated immune response for HCC (145). Compared with resection, RFA significantly induced GPC3-specific CTLs, especially in patients with GPC3-overexpressing HCC. Consequently, the phase II study of GPC3 peptide vaccine for adjuvant immunotherapy was carried out, laying a foundation of antitumor efficacy of GPC3 peptide vaccine and induced GPC3-specific CTL (105). Although the combination of resection or RFA with GPC3 peptide vaccine decreased the 1-year recurrence rate (142), whether different local strategies had an impact on the prognosis of the combination treatment remained unclear. However, another randomized phase II study showed that adjuvant immunotherapy with tumor associated antigen (TAA)-pulsed DC vaccine prolonged the RFS of patients with complete remission in non-RFA (including surgical resection, TACE, and PEI) groups compared with those in the RFA group (102). These results suggest that combination strategy benefits patients, but the choice of optimal combinations is thought provoking.
Springing Synergistic Strategies Based on Nanoplatforms
While the clinical trials are in full force, the combination of ablation and immunotherapy is also attracting the attention of scientists in basic medicine and biomedical materials. The development and application of multi-functional nanoplatforms have enabled synergistic ablative-immunotherapy strategy to flourish, instead of the sequential combination. On the one hand, a nanoplatform can deliver multiple drugs with optimized drug performance and therapeutic efficiency, as well as reduced drug toxicity. On the other hand, nanoplatforms can apply imaging technology to identify and locate tumors, guide ablation procedures, as well as monitor drug responses and therapy efficacy. Moreover, such a combination strategy may maximize the synergistic anti-tumor effects and thus achieve a greater therapeutic efficacy than the mere sum of the parts.
First, nanoplatforms can improve the targeting ability of agents through the innate enhanced permeability and retention effect and modifications with specific targets to enhance the anti-tumor effects (8). A mesoporous silica based nanosystem co-loading ICG and sorafenib, named (ICG+S)@mSiO2, was developed for synergetic PTT/immuno-enhanced therapy (146). (ICG+S)@mSiO2 improved endocytosis of HCC cells and photothermal efficiency. Active targeting deliveries were achieved in SP94-PB-SF-Cy5.5 nanoparticles (NPs) (147) and PCN-ACF-CpG@HA NPs (148) by conjugated with HCC specific targeting peptide (such as SP94) and HA (targeting CD44 receptor-overexpressed HCC cells), respectively. Moreover, SP94-PB-SF-Cy5.5 and PCN-ACF-CpG@HA, in combination with PD-L1 blockade and an immunologic adjuvant (CpG), enhanced the PTT- and PDT-induced weak immunogenic cell death of cancer cells. Similarly, these strategies for enhanced immune responses also applied sonodynamic immunotherapy as recently reported by Tan et al. (149) and Lin et al. (150). Moreover, anti-TGF-β antibody modification is an active targeting strategy that enhances cell endocytosis for improved PTT and an immunotherapeutic strategy for immune activation (151). Besides, ICG/ICG-SF-Gel-based photothermal-immunotherapy inhibited primary and distal tumor growth, with improved survival time with the help of Ganoderma lucidum polysaccharides (GLP) for enhancing the antitumor immunity (152). These combined anti-tumor effects led to the application of TAAs in in situ vaccination to eliminate residual and distant lesions, as well as inhibit tumor recurrence and metastasis.
Biomimetic nanotechnology, which integrates advantages of nanoplatform delivery and cellular immunotherapy, provides novel strategies for synergistic ablative immunotherapy. On the one hand, biomimetic nanoplatforms are ideal for targeted drug delivery because of their superior biological characteristics. For instance, Wang et al. developed a photothermal immunotherapy nanoplatform based on synthetic high-density lipoprotein (sHDL) (153). The higher expression of sHDL in HCC cells than in other normal cells of liver facilitates the preferential delivery of agents into the cytosol of HCC cells. Ma and colleagues designed a CAR-T cell membrane-coated mesoporous silica NP, which specifically recognized GPC3+ HCC cells (154). On the other hand, a programmable therapeutic strategy based on engineered immune cells provide a possibility for the synergy of ablation and cellular immunotherapy. Zhang et al. constructed an artificial engineered NK cell decorated with TLS11a (a HCC-specific targeting aptamer) for photothermal immunotherapy (56).
Discussion
The development of science and technology and the deepening of researches on HCC have promoted vigorous developments of treatment strategies for HCC, including the local treatment represented by the clinical standard treatment (RFA) and the emerging phototherapy, and the systematic treatment represented by sorafenib and immune blockers. However, monotherapies have shown some limitations. RFA is a first-line ablative therapy with established technical standard for patients with HCC. However, over 30% of patients suffer from recurrence or metastasis after iRFA (27). The solutions to the problems after iRFA include two aspects: improving the efficiency of RFA and applying combination therapy. The former can be solved well with the development of imaging technology based on nanomaterials, but the process from new drug development to clinical application is long and slow. The latter provides a salvage alternative for residual tumors, but the choice of drugs is thought provoking because of possible drug resistance after iRFA. Moreover, high-quality evidence-based medicine are lacking to support these solutions.
In comparison, increasing evidence support combination therapy. Thus, the combination of ablation and immunotherapy is rationale. On the one hand, ablation can promote anti-tumor immune responses. However, these responses are not strong enough to completely control tumors. On the other hand, the addition of immunotherapy may synergistically amplify the anti-tumor immune effect. The application of nanotechnology and nanomaterials in ablative immunotherapy strengthens the combination; enhances therapeutic effects by improving the physical, chemical, and physiological properties of agents; and achieves a synergistic effect through theranostic nanoplatforms.
Of course, many controversies and challenges need to be resolved. How to develop individualized treatment strategies to obtain the best treatment effect needs to be taken into consideration in clinical research. First, most clinical trials of ablative immunotherapy apply adjuvant immunotherapy after ablation. The frequency of ablation and the optimal time of immunotherapy application need to be specified. For example, a study showed that the ideal time window for immunotherapy after IRE is 3-14 days post-ablation (64). Another study suggested that the frequency of cryoablation is related to prognosis (144). Second, the expression difference of specific genes, such as GPC3 (105, 108, 109), in some patients with HCC leads to different immunotherapy responses and outcomes. Third, the combinations of ablative immunotherapy are diverse. Although some studies demonstrated that ablative immunotherapy provides better outcomes than single ablation or immunotherapy, whether different combinations have differences is unknown. In addition, more multicenter, randomized clinical trials with large samples are needed to confirm the benefits of the ablative immunotherapy. With regard to basic researches, the animal models used in ablative therapy, especially phototherapy, and subcutaneous tumor transplantation model are not suitable because the penetration depth of such techniques is limited. Moreover, tumors in solid organs such as liver, are difficult to reach by percutaneous or laparoscopic ablative techniques, unless the tumor is on the surface of the organ. The development of new drugs based on nanomaterials (such as NIR/X-Ray activated PSs and photothermal drugs) and novel technologies (such as SDT), has been devoted to address these problems. Of course, the success of these advances in cell and animal levels is still a long way from clinical applications. Nonetheless, ablative immunotherapy is expected to gain a place in HCC therapy and benefit patients in the near future.
Author Contributions
KW and CJ contributed to conceive and design the study. CW, KW, and JM performed the article searching. HJ, WL, and YZ extracted the data. CW and KW wrote the manuscript. CJ and JM supervised the manuscript. All authors contributed to the article and approved the submitted version.
Funding
This project is supported by the grants from Zhejiang Province Public Welfare Technology Application Research Project (No. LGF21H160022), Natural Science Foundation of Zhejiang Province (No. LQ22H160055), Science and Technology Plan Project of Taizhou (No. 21ywb26 and 21ywb29), Medical Science and Technology Project of Zhejiang Province (No. 2017KY711), and Project of Taizhou University (No.2018PY057).
Conflict of Interest
The authors declare that the research was conducted in the absence of any commercial or financial relationships that could be construed as a potential conflict of interest.
Publisher’s Note
All claims expressed in this article are solely those of the authors and do not necessarily represent those of their affiliated organizations, or those of the publisher, the editors and the reviewers. Any product that may be evaluated in this article, or claim that may be made by its manufacturer, is not guaranteed or endorsed by the publisher.
References
1. Sung H, Ferlay J, Siegel RL, Laversanne M, Soerjomataram I, Jemal A, et al. Global Cancer Statistics 2020: GLOBOCAN Estimates of Incidence and Mortality Worldwide for 36 Cancers in 185 Countries. CA: Cancer J Clin (2021) 71(3):209–49. doi: 10.3322/caac.21660
2. Vogel A, Cervantes A, Chau I, Daniele B, Llovet JM, Meyer T, et al. Hepatocellular Carcinoma: ESMO Clinical Practice Guidelines for Diagnosis, Treatment and Follow-Up. Ann Oncol (2018) 29(Suppl 4):iv238–55. doi: 10.1093/annonc/mdy308
3. Chen LT, Martinelli E, Cheng AL, Pentheroudakis G, Qin S, Bhattacharyya GS, et al. Pan-Asian Adapted ESMO Clinical Practice Guidelines for the Management of Patients With Intermediate and Advanced/Relapsed Hepatocellular Carcinoma: A TOS-ESMO Initiative Endorsed by CSCO, ISMPO, JSMO, KSMO, MOS and SSO. Ann Oncol (2020) 31(3):334–51. doi: 10.1016/j.annonc.2019.12.001
4. Gordan JD, Kennedy EB, Abou-Alfa GK, Beg MS, Brower ST, Gade TP, et al. Systemic Therapy for Advanced Hepatocellular Carcinoma: ASCO Guideline. J Clin Oncol (2020) 38(36):4317–45. doi: 10.1200/JCO.20.02672
5. Benson AB, D'Angelica MI, Abbott DE, Abrams TA, Alberts SR, Anaya DA, et al. Guidelines Insights: Hepatobiliary Cancers, Version 2.2019. J Natl Compr Cancer Netw: JNCCN (2019) 17(4):302–10. doi: 10.6004/jnccn.2019.0019
6. Llovet JM, Kelley RK, Villanueva A, Singal AG, Pikarsky E, Roayaie S, et al. Hepatocellular Carcinoma. Nat Rev Dis Primers (2021) 7(1):6. doi: 10.1038/s41572-020-00240-3
7. Roayaie S, Obeidat K, Sposito C, Mariani L, Bhoori S, Pellegrinelli A, et al. Resection of Hepatocellular Cancer ≤2 Cm: Results From Two Western Centers. Hepatol (Baltimore Md) (2013) 57(4):1426–35. doi: 10.1002/hep.25832
8. Wang C, Fan W, Zhang Z, Wen Y, Xiong L, Chen X. Advanced Nanotechnology Leading the Way to Multimodal Imaging-Guided Precision Surgical Therapy. Adv Mater (2019) 31(49):1904329. doi: 10.1002/adma.201904329
9. Peng Z-W, Liu F-R, Ye S, Xu L, Zhang Y-J, Liang H-H, et al. Radiofrequency Ablation Versus Open Hepatic Resection for Elderly Patients (> 65 Years) With Very Early or Early Hepatocellular Carcinoma. Cancer (2013) 119(21):3812–20. doi: 10.1002/cncr.28293
10. Cucchetti A, Piscaglia F, Cescon M, Colecchia A, Ercolani G, Bolondi L, et al. Cost-Effectiveness of Hepatic Resection Versus Percutaneous Radiofrequency Ablation for Early Hepatocellular Carcinoma. J Hepatol (2013) 59(2):300–7. doi: 10.1016/j.jhep.2013.04.009
11. Breen DJ, Lencioni R. Image-Guided Ablation of Primary Liver and Renal Tumours. Nat Rev Clin Oncol (2015) 12(3):175–86. doi: 10.1038/nrclinonc.2014.237
12. Lehmann KS, Poch FGM, Rieder C, Schenk A, Stroux A, Frericks BB, et al. Minimal Vascular Flows Cause Strong Heat Sink Effects in Hepatic Radiofrequency Ablation Ex Vivo. J Hepatobiliary Pancreat Sci (2016) 23(8):508–16. doi: 10.1002/jhbp.370
13. Mohkam K, Dumont P-N, Manichon A-F, Jouvet J-C, Boussel L, Merle P, et al. No-Touch Multibipolar Radiofrequency Ablation vs. Surgical Resection for Solitary Hepatocellular Carcinoma Ranging From 2 to 5 Cm. J Hepatol (2018) 68(6):1172–80. doi: 10.1016/j.jhep.2018.01.014
14. Su T, Huang M, Liao J, Lin S, Yu P, Yang J, et al. Insufficient Radiofrequency Ablation Promotes Hepatocellular Carcinoma Metastasis Through M A mRNA Methylation Dependent Mechanism. Hepatol (Baltimore Md) (2021) 74(3):1339–56. doi: 10.1002/hep.31766
15. Kong J, Yao C, Dong S, Wu S, Xu Y, Li K, et al. ICAM-1 Activates Platelets and Promotes Endothelial Permeability Through VE-Cadherin After Insufficient Radiofrequency Ablation. Adv Sci (Weinheim Baden-Wurttemberg Germany) (2021) 8(4):2002228. doi: 10.1002/advs.202002228
16. Kong J, Yao C, Ding X, Dong S, Wu S, Sun W, et al. ATPase Inhibitory Factor 1 Promotes Hepatocellular Carcinoma Progression After Insufficient Radiofrequency Ablation, and Attenuates Cell Sensitivity to Sorafenib Therapy. Front Oncol (2020) 10:1080. doi: 10.3389/fonc.2020.01080
17. Ma D, Gao X, Liu Z, Lu X, Ju H, Zhang N. Exosome-Transferred Long non-Coding RNA ASMTL-AS1 Contributes to Malignant Phenotypes in Residual Hepatocellular Carcinoma After Insufficient Radiofrequency Ablation. Cell Prolif (2020) 53(9):e12795. doi: 10.1111/cpr.12795
18. Li Z, Jiang M, Zhang T, Liu S. Promotes Hepatocellular Carcinoma via Axis Under Insufficient Radiofrequency Ablation Condition. Cancer Biother Radiopharm (2020). doi: 10.1089/cbr.2019.3541
19. Jia G, Li F, Tong R, Liu Y, Zuo M, Ma L, et al. C-Met/MAPK Pathway Promotes the Malignant Progression of Residual Hepatocellular Carcinoma Cells After Insufficient Radiofrequency Ablation. Med Oncol (Northwood Lond Engl) (2020) 37(12):117. doi: 10.1007/s12032-020-01444-z
20. Zhang N, Li H, Qin C, Ma D, Zhao Y, Zhu W, et al. Insufficient Radiofrequency Ablation Promotes the Metastasis of Residual Hepatocellular Carcinoma Cells via Upregulating Flotillin Proteins. J Cancer Res Clin Oncol (2019) 145(4):895–907. doi: 10.1007/s00432-019-02852-z
21. Xu W-L, Wang S-H, Sun W-B, Gao J, Ding X-M, Kong J, et al. Insufficient Radiofrequency Ablation-Induced Autophagy Contributes to the Rapid Progression of Residual Hepatocellular Carcinoma Through the HIF-1α/BNIP3 Signaling Pathway. BMB Rep (2019) 52(4):277–82. doi: 10.5483/BMBRep.2019.52.4.263
22. Zhang N, Ma D, Wang L, Zhu X, Pan Q, Zhao Y, et al. Insufficient Radiofrequency Ablation Treated Hepatocellular Carcinoma Cells Promote Metastasis by Up-Regulation Itgb3. J Cancer (2017) 8(18):3742–54. doi: 10.7150/jca.20816
23. Zhang N, Wang L, Chai Z-T, Zhu Z-M, Zhu X-D, Ma D-N, et al. Incomplete Radiofrequency Ablation Enhances Invasiveness and Metastasis of Residual Cancer of Hepatocellular Carcinoma Cell HCCLM3 via Activating β-Catenin Signaling. PLoS One (2014) 9(12):e115949. doi: 10.1371/journal.pone.0115949
24. Dong S, Kong J, Kong F, Kong J, Gao J, Ke S, et al. Insufficient Radiofrequency Ablation Promotes Epithelial-Mesenchymal Transition of Hepatocellular Carcinoma Cells Through Akt and ERK Signaling Pathways. J Transl Med (2013) 11:273. doi: 10.1186/1479-5876-11-273
25. Kong J, Kong L, Kong J, Ke S, Gao J, Ding X, et al. After Insufficient Radiofrequency Ablation, Tumor-Associated Endothelial Cells Exhibit Enhanced Angiogenesis and Promote Invasiveness of Residual Hepatocellular Carcinoma. J Transl Med (2012) 10:230. doi: 10.1186/1479-5876-10-230
26. Teng W, Liu K-W, Lin C-C, Jeng W-J, Chen W-T, Sheen IS, et al. Insufficient Ablative Margin Determined by Early Computed Tomography may Predict the Recurrence of Hepatocellular Carcinoma After Radiofrequency Ablation. Liver Cancer (2015) 4(1):26–38. doi: 10.1159/000343877
27. Jiang Q, Zeng Y, Xu Y, Xiao X, Liu H, Zhou B, et al. Ultrasound Molecular Imaging as a Potential Non-Invasive Diagnosis to Detect the Margin of Hepatocarcinoma via CSF-1r Targeting. Front Bioeng Biotechnol (2020) 8:783. doi: 10.3389/fbioe.2020.00783
28. Dong S, Kong J, Kong F, Kong J, Gao J, Ji L, et al. Sorafenib Suppresses the Epithelial-Mesenchymal Transition of Hepatocellular Carcinoma Cells After Insufficient Radiofrequency Ablation. BMC Cancer (2015) 15:939. doi: 10.1186/s12885-015-1949-7
29. Zhang N, Wang L-R, Li D-D, Ma D-N, Wang C-H, He X-G, et al. Interferon-α Combined With Herbal Compound "Songyou Yin" Effectively Inhibits the Increased Invasiveness and Metastasis by Insufficient Radiofrequency Ablation of Hepatocellular Carcinoma in an Animal Model. Integr Cancer Ther (2018) 17(4):1260–9. doi: 10.1177/1534735418801525
30. Kong J, Kong J, Pan B, Ke S, Dong S, Li X, et al. Insufficient Radiofrequency Ablation Promotes Angiogenesis of Residual Hepatocellular Carcinoma via HIF-1α/VEGFA. PLoS One (2012) 7(5):e37266. doi: 10.1371/journal.pone.0037266
31. Zhang L, Wang J, Jiang J, Zhang M, Shen J. CTLA-4 Blockade Suppresses Progression of Residual Tumors and Improves Survival After Insufficient Radiofrequency Ablation in a Subcutaneous Murine Hepatoma Model. Cardiovasc Intervent Radiol (2020) 43(9):1353–61. doi: 10.1007/s00270-020-02505-6
32. Zhang Q, Kong J, Dong S, Xu W, Sun W. Metformin Exhibits the Anti-Proliferation and Anti-Invasion Effects in Hepatocellular Carcinoma Cells After Insufficient Radiofrequency Ablation. Cancer Cell Int (2017) 17:48. doi: 10.1186/s12935-017-0418-6
33. Zhao Z, Wu J, Liu X, Liang M, Zhou X, Ouyang S, et al. Insufficient Radiofrequency Ablation Promotes Proliferation of Residual Hepatocellular Carcinoma via Autophagy. Cancer Lett (2018) 421:73–81. doi: 10.1016/j.canlet.2018.02.024
34. An C, Li W-Z, Huang Z-M, Yu X-L, Han Y-Z, Liu F-Y, et al. Small Single Perivascular Hepatocellular Carcinoma: Comparisons of Radiofrequency Ablation and Microwave Ablation by Using Propensity Score Analysis. Eur Radiol (2021) 31(7):4764–73. doi: 10.1007/s00330-020-07571-5
35. Feng Y, Wang L, Lv H, Shi T, Xu C, Zheng H, et al. Microwave Ablation Versus Radiofrequency Ablation for Perivascular Hepatocellular Carcinoma: A Propensity Score Analysis. HPB (2021) 23(4):512–9. doi: 10.1016/j.hpb.2020.08.006
36. Facciorusso A, Abd El Aziz MA, Tartaglia N, Ramai D, Mohan BP, Cotsoglou C, et al. Microwave Ablation Versus Radiofrequency Ablation for Treatment of Hepatocellular Carcinoma: A Meta-Analysis of Randomized Controlled Trials. Cancers (Basel) (2020) 12(12):3796–806. doi: 10.3390/cancers12123796
37. Tamai H, Okamura J. New Next-Generation Microwave Thermosphere Ablation for Small Hepatocellular Carcinoma. Clin Mol Hepatol (2021) 27(4):564–74. doi: 10.3350/cmh.2021.0136
38. Kim R, Kang TW, Cha DI, Song KD, Lee MW, Rhim H, et al. Percutaneous Cryoablation for Perivascular Hepatocellular Carcinoma: Therapeutic Efficacy and Vascular Complications. Eur Radiol (2019) 29(2):654–62. doi: 10.1007/s00330-018-5617-6
39. Wang C, Wang H, Yang W, Hu K, Xie H, Hu K-Q, et al. Multicenter Randomized Controlled Trial of Percutaneous Cryoablation Versus Radiofrequency Ablation in Hepatocellular Carcinoma. Hepatol (Baltimore Md) (2015) 61(5):1579–90. doi: 10.1002/hep.27548
40. Saini A, Breen I, Alzubaidi S, Pershad Y, Sheth R, Naidu S, et al. Irreversible Electroporation in Liver Cancers and Whole Organ Engineering. J Clin Med (2018) 8(1):22–32. doi: 10.3390/jcm8010022
41. Sutter O, Calvo J, N'Kontchou G, Nault J-C, Ourabia R, Nahon P, et al. Safety and Efficacy of Irreversible Electroporation for the Treatment of Hepatocellular Carcinoma Not Amenable to Thermal Ablation Techniques: A Retrospective Single-Center Case Series. Radiology (2017) 284(3):877–86. doi: 10.1148/radiol.2017161413
42. Frühling P, Nilsson A, Duraj F, Haglund U, Norén A. Single-Center Nonrandomized Clinical Trial to Assess the Safety and Efficacy of Irreversible Electroporation (IRE) Ablation of Liver Tumors in Humans: Short to Mid-Term Results. Eur J Surg Oncol (2017) 43(4):751–7. doi: 10.1016/j.ejso.2016.12.004
43. Niessen C, Igl J, Pregler B, Beyer L, Noeva E, Dollinger M, et al. Factors Associated With Short-Term Local Recurrence of Liver Cancer After Percutaneous Ablation Using Irreversible Electroporation: A Prospective Single-Center Study. J Vasc Intervent Radiol JVIR (2015) 26(5):694–702. doi: 10.1016/j.jvir.2015.02.001
44. Egger NG, Schoenecker JA, Gourley WK, Motamedi M, Anderson KE, Weinman SA. Photosensitization of Experimental Hepatocellular Carcinoma With Protoporphyrin Synthesized From Administered Delta-Aminolevulinic Acid: Studies With Cultured Cells and Implanted Tumors. J Hepatol (1997) 26(4):913–20. doi: 10.1016/S0168-8278(97)80260-7
45. Otake M, Nishiwaki M, Kobayashi Y, Baba S, Kohno E, Kawasaki T, et al. Selective Accumulation of ALA-Induced PpIX and Photodynamic Effect in Chemically Induced Hepatocellular Carcinoma. Br J Cancer (2003) 89(4):730–6. doi: 10.1038/sj.bjc.6601135
46. Mirzaei H, Djavid GE, Hadizadeh M, Jahanshiri-Moghadam M, Hajian P. The Efficacy of Radachlorin-Mediated Photodynamic Therapy in Human Hepatocellular Carcinoma Cells. J Photochem Photobiol B (2015) 142:86–91. doi: 10.1016/j.jphotobiol.2014.11.007
47. Li Q, Chen K, Huang W, Ma H, Zhao X, Zhang J, et al. Minimally Invasive Photothermal Ablation Assisted by Laparoscopy as an Effective Preoperative Neoadjuvant Treatment for Orthotopic Hepatocellular Carcinoma. Cancer Lett (2021) 496:169–78. doi: 10.1016/j.canlet.2020.09.024
48. Fan W, Tang W, Lau J, Shen Z, Xie J, Shi J, et al. Breaking the Depth Dependence by Nanotechnology-Enhanced X-Ray-Excited Deep Cancer Theranostics. Adv Mater (Deerfield Beach Fla) (2019) 31(12):e1806381. doi: 10.1002/adma.201806381
49. Sun W, Zhou Z, Pratx G, Chen X, Chen H. Nanoscintillator-Mediated X-Ray Induced Photodynamic Therapy for Deep-Seated Tumors: From Concept to Biomedical Applications. Theranostics (2020) 10(3):1296–318. doi: 10.7150/thno.41578
50. Jin G, He R, Liu Q, Lin M, Dong Y, Li K, et al. Near-Infrared Light-Regulated Cancer Theranostic Nanoplatform Based on Aggregation-Induced Emission Luminogen Encapsulated Upconversion Nanoparticles. Theranostics (2019) 9(1):246–64. doi: 10.7150/thno.30174
51. Li S, Deng Q, Zhang Y, Li X, Wen G, Cui X, et al. Rational Design of Conjugated Small Molecules for Superior Photothermal Theranostics in the NIR-II Biowindow. Adv Mater (Deerfield Beach Fla) (2020) 32(33):e2001146. doi: 10.1002/adma.202001146
52. Qian K-Y, Song Y, Yan X, Dong L, Xue J, Xu Y, et al. Injectable Ferrimagnetic Silk Fibroin Hydrogel for Magnetic Hyperthermia Ablation of Deep Tumor. Biomaterials (2020) 259:120299. doi: 10.1016/j.biomaterials.2020.120299
53. Yu X-N, Deng Y, Zhang G-C, Liu J, Liu T-T, Dong L, et al. Sorafenib-Conjugated Zinc Phthalocyanine Based Nanocapsule for Trimodal Therapy in an Orthotopic Hepatocellular Carcinoma Xenograft Mouse Model. ACS Appl Mater Interfaces (2020) 12(15):17193–206. doi: 10.1021/acsami.0c00375
54. Wu H, Wang C, Sun J, Sun L, Wan J, Wang S, et al. Self-Assembled and Self-Monitored Sorafenib/Indocyanine Green Nanodrug With Synergistic Antitumor Activity Mediated by Hyperthermia and Reactive Oxygen Species-Induced Apoptosis. ACS Appl Mater Interfaces (2019) 11(47):43996–4006. doi: 10.1021/acsami.9b18086
55. Zhang D, Zheng A, Li J, Wu M, Wu L, Wei Z, et al. Smart Cu(II)-Aptamer Complexes Based Gold Nanoplatform for Tumor Micro-Environment Triggered Programmable Intracellular Prodrug Release, Photodynamic Treatment and Aggregation Induced Photothermal Therapy of Hepatocellular Carcinoma. Theranostics (2017) 7(1):164–79. doi: 10.7150/thno.17099
56. Zhang D, Zheng Y, Lin Z, Lan S, Zhang X, Zheng A, et al. Artificial Engineered Natural Killer Cells Combined With Antiheat Endurance as a Powerful Strategy for Enhancing Photothermal-Immunotherapy Efficiency of Solid Tumors. Small (Weinheim an der Bergstrasse Germany) (2019) 15(42):e1902636. doi: 10.1002/smll.201902636
57. Lan S, Lin Z, Zhang D, Zeng Y, Liu X. Photocatalysis Enhancement for Programmable Killing of Hepatocellular Carcinoma Through Self-Compensation Mechanisms Based on Black Phosphorus Quantum-Dot-Hybridized Nanocatalysts. ACS Appl Mater Interfaces (2019) 11(10):9804–13. doi: 10.1021/acsami.8b21820
58. Qi S, Zhang Y, Liu G, Chen J, Li X, Zhu Q, et al. Plasmonic-Doped Melanin-Mimic for CXCR4-Targeted NIR-II Photoacoustic Computed Tomography-Guided Photothermal Ablation of Orthotopic Hepatocellular Carcinoma. Acta Biomater (2021) 129:245–57. doi: 10.1016/j.actbio.2021.05.034
59. Ali MY, Grimm CF, Ritter M, Mohr L, Allgaier H-P, Weth R, et al. Activation of Dendritic Cells by Local Ablation of Hepatocellular Carcinoma. J Hepatol (2005) 43(5):817–22. doi: 10.1016/j.jhep.2005.04.016
60. Zerbini A, Pilli M, Penna A, Pelosi G, Schianchi C, Molinari A, et al. Radiofrequency Thermal Ablation of Hepatocellular Carcinoma Liver Nodules can Activate and Enhance Tumor-Specific T-Cell Responses. Cancer Res (2006) 66(2):1139–46. doi: 10.1158/0008-5472.CAN-05-2244
61. Zerbini A, Pilli M, Fagnoni F, Pelosi G, Pizzi MG, Schivazappa S, et al. Increased Immunostimulatory Activity Conferred to Antigen-Presenting Cells by Exposure to Antigen Extract From Hepatocellular Carcinoma After Radiofrequency Thermal Ablation. J Immunother (Hagerstown Md 1997) (2008) 31(3):271–82. doi: 10.1097/CJI.0b013e318160ff1c
62. Rochigneux P, Nault J-C, Mallet F, Chretien A-S, Barget N, Garcia AJ, et al. Dynamic of Systemic Immunity and its Impact on Tumor Recurrence After Radiofrequency Ablation of Hepatocellular Carcinoma. Oncoimmunology (2019) 8(8):1615818. doi: 10.1080/2162402X.2019.1615818
63. Leuchte K, Staib E, Thelen M, Gödel P, Lechner A, Zentis P, et al. Microwave Ablation Enhances Tumor-Specific Immune Response in Patients With Hepatocellular Carcinoma. Cancer Immunol Immunother (2021) 70(4):893–907. doi: 10.1007/s00262-020-02734-1
64. Guo X, Du F, Liu Q, Guo Y, Wang Q, Huang W, et al. Immunological Effect of Irreversible Electroporation on Hepatocellular Carcinoma. BMC Cancer (2021) 21(1):443. doi: 10.1186/s12885-021-08176-x
65. Zeng Z, Shi F, Zhou L, Zhang M-N, Chen Y, Chang X-J, et al. Upregulation of Circulating PD-L1/PD-1 is Associated With Poor Post-Cryoablation Prognosis in Patients With HBV-Related Hepatocellular Carcinoma. PLoS One (2011) 6(9):e23621. doi: 10.1371/journal.pone.0023621
66. Qi X, Yang M, Ma L, Sauer M, Avella D, Kaifi JT, et al. Synergizing Sunitinib and Radiofrequency Ablation to Treat Hepatocellular Cancer by Triggering the Antitumor Immune Response. J Immunother Cancer (2020) 8(2):1038–51. doi: 10.1136/jitc-2020-001038
67. Dai Z, Wang Z, Lei K, Liao J, Peng Z, Lin M, et al. Irreversible Electroporation Induces CD8 T Cell Immune Response Against Post-Ablation Hepatocellular Carcinoma Growth. Cancer Lett (2021) 503:1–10. doi: 10.1016/j.canlet.2021.01.001
68. Au JT, Wong J, Mittra A, Carpenter S, Haddad D, Carson J, et al. Irreversible Electroporation Is a Surgical Ablation Technique That Enhances Gene Transfer. Surgery (2011) 150(3):474–9. doi: 10.1016/j.surg.2011.07.007
69. Au JT, Mittra A, Song TJ, Cavnar M, Jun K, Carson J, et al. Irreversible Electroporation Facilitates Gene Transfer of a GM-CSF Plasmid With a Local and Systemic Response. Surgery (2013) 154(3):496–503. doi: 10.1016/j.surg.2013.06.005
70. Shi L, Chen L, Wu C, Zhu Y, Xu B, Zheng X, et al. PD-1 Blockade Boosts Radiofrequency Ablation-Elicited Adaptive Immune Responses Against Tumor. Clin Cancer Res (2016) 22(5):1173–84. doi: 10.1158/1078-0432.CCR-15-1352
71. Fei Q, Pan Y, Lin W, Zhou Y, Yu X, Hou Z, et al. High-Dimensional Single-Cell Analysis Delineates Radiofrequency Ablation Induced Immune Microenvironmental Remodeling in Pancreatic Cancer. Cell Death Dis (2020) 11(7):589. doi: 10.1038/s41419-020-02787-1
72. Chauhan A, Midha S, Kumar R, Meena R, Singh P, Jha SK, et al. Rapid Tumor Inhibition via Magnetic Hyperthermia Regulated by Caspase 3 With Time-Dependent Clearance of Iron Oxide Nanoparticles. Biomater Sci (2021) 9(8):2972–90. doi: 10.1039/d0bm01705a
73. Muñoz NM, Dupuis C, Williams M, Dixon K, McWatters A, Avritscher R, et al. Molecularly Targeted Photothermal Ablation Improves Tumor Specificity and Immune Modulation in a Rat Model of Hepatocellular Carcinoma. Commun Biol (2020) 3(1):783. doi: 10.1038/s42003-020-01522-y
74. Wu H, Li S-S, Zhou M, Jiang A-N, He Y, Wang S, et al. Palliative Radiofrequency Ablation Accelerates the Residual Tumor Progression Through Increasing Tumor-Infiltrating MDSCs and Reducing T-Cell-Mediated Anti-Tumor Immune Responses in Animal Model. Front Oncol (2020) 10:1308. doi: 10.3389/fonc.2020.01308
75. Zhao J, Li Q, Muktiali M, Ren B, Hu Y, Li D, et al. Effect of Microwave Ablation Treatment of Hepatic Malignancies on Serum Cytokine Levels. BMC Cancer (2020) 20(1):812. doi: 10.1186/s12885-020-07326-x
76. Facciorusso A, Del Prete V, Antonino M, Crucinio N, Neve V, Di Leo A, et al. Post-Recurrence Survival in Hepatocellular Carcinoma After Percutaneous Radiofrequency Ablation. Dig Liver Dis (2014) 46(11):1014–9. doi: 10.1016/j.dld.2014.07.012
77. Tiong L, Maddern GJ. Systematic Review and Meta-Analysis of Survival and Disease Recurrence After Radiofrequency Ablation for Hepatocellular Carcinoma. Br J Surg (2011) 98(9):1210–24. doi: 10.1002/bjs.7669
78. Yoshida S, Kornek M, Ikenaga N, Schmelzle M, Masuzaki R, Csizmadia E, et al. Sublethal Heat Treatment Promotes Epithelial-Mesenchymal Transition and Enhances the Malignant Potential of Hepatocellular Carcinoma. Hepatol (Baltimore Md) (2013) 58(5):1667–80. doi: 10.1002/hep.26526
79. Zhang R, Ma M, Lin X-H, Liu H-H, Chen J, Chen J, et al. Extracellular Matrix Collagen I Promotes the Tumor Progression of Residual Hepatocellular Carcinoma After Heat Treatment. BMC Cancer (2018) 18(1):901. doi: 10.1186/s12885-018-4820-9
80. Su T, Liao J, Dai Z, Xu L, Chen S, Wang Y, et al. Stress-Induced Phosphoprotein 1 Mediates Hepatocellular Carcinoma Metastasis After Insufficient Radiofrequency Ablation. Oncogene (2018) 37(26):3514–27. doi: 10.1038/s41388-018-0169-4
81. Bruix J, Raoul J-L, Sherman M, Mazzaferro V, Bolondi L, Craxi A, et al. Efficacy and Safety of Sorafenib in Patients With Advanced Hepatocellular Carcinoma: Subanalyses of a Phase III Trial. J Hepatol (2012) 57(4):821–9. doi: 10.1016/j.jhep.2012.06.014
82. Kudo M, Finn RS, Qin S, Han K-H, Ikeda K, Piscaglia F, et al. Lenvatinib Versus Sorafenib in First-Line Treatment of Patients With Unresectable Hepatocellular Carcinoma: A Randomised Phase 3 Non-Inferiority Trial. Lancet (Lond Engl) (2018) 391(10126):1163–73. doi: 10.1016/S0140-6736(18)30207-1
83. Cheon J, Chon HJ, Bang Y, Park NH, Shin JW, Kim KM, et al. Real-World Efficacy and Safety of Lenvatinib in Korean Patients With Advanced Hepatocellular Carcinoma: A Multicenter Retrospective Analysis. Liver Cancer (2020) 9(5):613–24. doi: 10.1159/000508901
84. Qin S, Bi F, Gu S, Bai Y, Chen Z, Wang Z, et al. Donafenib Versus Sorafenib in First-Line Treatment of Unresectable or Metastatic Hepatocellular Carcinoma: A Randomized, Open-Label, Parallel-Controlled Phase II-III Trial. J Clin Oncol (2021) 39(27):3002–11. doi: 10.1200/JCO.21.00163
85. Bruix J, Qin S, Merle P, Granito A, Huang Y-H, Bodoky G, et al. Regorafenib for Patients With Hepatocellular Carcinoma Who Progressed on Sorafenib Treatment (RESORCE): A Randomised, Double-Blind, Placebo-Controlled, Phase 3 Trial. Lancet (Lond Engl) (2017) 389(10064):56–66. doi: 10.1016/S0140-6736(16)32453-9
86. Kelley RK, Ryoo B-Y, Merle P, Park J-W, Bolondi L, Chan SL, et al. Second-Line Cabozantinib After Sorafenib Treatment for Advanced Hepatocellular Carcinoma: A Subgroup Analysis of the Phase 3 CELESTIAL Trial. ESMO Open (2020) 5(4):714–22. doi: 10.1136/esmoopen-2020-000714
87. Pinyol R, Montal R, Bassaganyas L, Sia D, Takayama T, Chau G-Y, et al. Molecular Predictors of Prevention of Recurrence in HCC With Sorafenib as Adjuvant Treatment and Prognostic Factors in the Phase 3 STORM Trial. Gut (2019) 68(6):1065–75. doi: 10.1136/gutjnl-2018-316408
88. Bian H, Zheng J-S, Nan G, Li R, Chen C, Hu C-X, et al. Randomized Trial of [131I] Metuximab in Treatment of Hepatocellular Carcinoma After Percutaneous Radiofrequency Ablation. J Natl Cancer Inst (2014) 106(9):239–43. doi: 10.1093/jnci/dju239
89. Li J, Xing J, Yang Y, Liu J, Wang W, Xia Y, et al. Adjuvant I-Metuximab for Hepatocellular Carcinoma After Liver Resection: A Randomised, Controlled, Multicentre, Open-Label, Phase 2 Trial. Lancet Gastroenterol Hepatol (2020) 5(6):548–60. doi: 10.1016/S2468-1253(19)30422-4
90. Sangro B, Sarobe P, Hervás-Stubbs S, Melero I. Advances in Immunotherapy for Hepatocellular Carcinoma. Nat Rev Gastroenterol Hepatol (2021) 18(8):525–43. doi: 10.1038/s41575-021-00438-0
91. Pinter M, Jain RK, Duda DG. The Current Landscape of Immune Checkpoint Blockade in Hepatocellular Carcinoma: A Review. JAMA Oncol (2021) 7(1):113–23. doi: 10.1001/jamaoncol.2020.3381
92. Abd El Aziz MA, Facciorusso A, Nayfeh T, Saadi S, Elnaggar M, Cotsoglou C, et al. Immune Checkpoint Inhibitors for Unresectable Hepatocellular Carcinoma. Vaccines (Basel) (2020) 8(4):616–44. doi: 10.3390/vaccines8040616
93. El-Khoueiry AB, Sangro B, Yau T, Crocenzi TS, Kudo M, Hsu C, et al. Nivolumab in Patients With Advanced Hepatocellular Carcinoma (CheckMate 040): An Open-Label, Non-Comparative, Phase 1/2 Dose Escalation and Expansion Trial. Lancet (Lond Engl) (2017) 389(10088):2492–502. doi: 10.1016/S0140-6736(17)31046-2
94. Lee MS, Ryoo B-Y, Hsu C-H, Numata K, Stein S, Verret W, et al. Atezolizumab With or Without Bevacizumab in Unresectable Hepatocellular Carcinoma (GO30140): An Open-Label, Multicentre, Phase 1b Study. Lancet Oncol (2020) 21(6):808–20. doi: 10.1016/S1470-2045(20)30156-X
95. Sangro B, Gomez-Martin C, de la Mata M, Iñarrairaegui M, Garralda E, Barrera P, et al. A Clinical Trial of CTLA-4 Blockade With Tremelimumab in Patients With Hepatocellular Carcinoma and Chronic Hepatitis C. J Hepatol (2013) 59(1):81–8. doi: 10.1016/j.jhep.2013.02.022
96. Yau T, Hsu C, Kim T-Y, Choo S-P, Kang Y-K, Hou M-M, et al. Nivolumab in Advanced Hepatocellular Carcinoma: Sorafenib-Experienced Asian Cohort Analysis. J Hepatol (2019) 71(3):543–52. doi: 10.1016/j.jhep.2019.05.014
97. Zhu AX, Finn RS, Edeline J, Cattan S, Ogasawara S, Palmer D, et al. Pembrolizumab in Patients With Advanced Hepatocellular Carcinoma Previously Treated With Sorafenib (KEYNOTE-224): A Non-Randomised, Open-Label Phase 2 Trial. Lancet Oncol (2018) 19(7):940–52. doi: 10.1016/S1470-2045(18)30351-6
98. Finn RS, Qin S, Ikeda M, Galle PR, Ducreux M, Kim T-Y, et al. Atezolizumab Plus Bevacizumab in Unresectable Hepatocellular Carcinoma. N Engl J Med (2020) 382(20):1894–905. doi: 10.1056/NEJMoa1915745
99. Galle PR, Finn RS, Qin S, Ikeda M, Zhu AX, Kim T-Y, et al. Patient-Reported Outcomes With Atezolizumab Plus Bevacizumab Versus Sorafenib in Patients With Unresectable Hepatocellular Carcinoma (IMbrave150): An Open-Label, Randomised, Phase 3 Trial. Lancet Oncol (2021) 22(7):991–1001. doi: 10.1016/S1470-2045(21)00151-0
100. Takayama T, Sekine T, Makuuchi M, Yamasaki S, Kosuge T, Yamamoto J, et al. Adoptive Immunotherapy to Lower Postsurgical Recurrence Rates of Hepatocellular Carcinoma: A Randomised Trial. Lancet (London Engl) (2000) 356(9232):802–7. doi: 10.1016/S0140-6736(00)02654-4
101. Palmer DH, Midgley RS, Mirza N, Torr EE, Ahmed F, Steele JC, et al. A Phase II Study of Adoptive Immunotherapy Using Dendritic Cells Pulsed With Tumor Lysate in Patients With Hepatocellular Carcinoma. Hepatol (Baltimore Md) (2009) 49(1):124–32. doi: 10.1002/hep.22626
102. Lee JH, Lee J-H, Lim Y-S, Yeon JE, Song T-J, Yu SJ, et al. Adjuvant Immunotherapy With Autologous Cytokine-Induced Killer Cells for Hepatocellular Carcinoma. Gastroenterology (2015) 148(7):1383–91. doi: 10.1053/j.gastro.2015.02.055
103. Heo J, Reid T, Ruo L, Breitbach CJ, Rose S, Bloomston M, et al. Randomized Dose-Finding Clinical Trial of Oncolytic Immunotherapeutic Vaccinia JX-594 in Liver Cancer. Nat Med (2013) 19(3):329–36. doi: 10.1038/nm.3089
104. Cripe TP, Ngo MC, Geller JI, Louis CU, Currier MA, Racadio JM, et al. Phase 1 Study of Intratumoral Pexa-Vec (JX-594), an Oncolytic and Immunotherapeutic Vaccinia Virus, in Pediatric Cancer Patients. Mol Ther (2015) 23(3):602–8. doi: 10.1038/mt.2014.243
105. Sawada Y, Yoshikawa T, Nobuoka D, Shirakawa H, Kuronuma T, Motomura Y, et al. Phase I Trial of a Glypican-3-Derived Peptide Vaccine for Advanced Hepatocellular Carcinoma: Immunologic Evidence and Potential for Improving Overall Survival. Clin Cancer Res (2012) 18(13):3686–96. doi: 10.1158/1078-0432.CCR-11-3044
106. Butterfield LH, Ribas A, Meng WS, Dissette VB, Amarnani S, Vu HT, et al. T-Cell Responses to HLA-A*0201 Immunodominant Peptides Derived From Alpha-Fetoprotein in Patients With Hepatocellular Cancer. Clin Cancer Res (2003) 9(16 Pt 1):5902–8.
107. Shang X-Y, Chen H-S, Zhang H-G, Pang X-W, Qiao H, Peng J-R, et al. The Spontaneous CD8+ T-Cell Response to HLA-A2-Restricted NY-ESO-1b Peptide in Hepatocellular Carcinoma Patients. Clin Cancer Res (2004) 10(20):6946–55. doi: 10.1158/1078-0432.CCR-04-0502
108. Yoshikawa T, Nakatsugawa M, Suzuki S, Shirakawa H, Nobuoka D, Sakemura N, et al. HLA-A2-Restricted Glypican-3 Peptide-Specific CTL Clones Induced by Peptide Vaccine Show High Avidity and Antigen-Specific Killing Activity Against Tumor Cells. Cancer Sci (2011) 102(5):918–25. doi: 10.1111/j.1349-7006.2011.01896.x
109. Lee J-H, Lee Y, Lee M, Heo MK, Song J-S, Kim K-H, et al. A Phase I/IIa Study of Adjuvant Immunotherapy With Tumour Antigen-Pulsed Dendritic Cells in Patients With Hepatocellular Carcinoma. Br J Cancer (2015) 113(12):1666–76. doi: 10.1038/bjc.2015.430
110. Sawada Y, Yoshikawa T, Shimomura M, Iwama T, Endo I, Nakatsura T. Programmed Death-1 Blockade Enhances the Antitumor Effects of Peptide Vaccine-Induced Peptide-Specific Cytotoxic T Lymphocytes. Int J Oncol (2015) 46(1):28–36. doi: 10.3892/ijo.2014.2737
111. Wu Q, Pi L, Le Trinh T, Zuo C, Xia M, Jiao Y, et al. A Novel Vaccine Targeting Glypican-3 as a Treatment for Hepatocellular Carcinoma. Mol Ther (2017) 25(10):2299–308. doi: 10.1016/j.ymthe.2017.08.005
112. Chen K, Wu Z, Zhao H, Wang Y, Ge Y, Wang D, et al. / Fusion Gene Immunization Generates Potent Antitumor Cellular Immunity and Enhances Anti-PD-1 Efficacy. Cancer Immunol Res (2020) 8(1):81–93. doi: 10.1158/2326-6066.CIR-19-0210
113. Yu L, Yang X, Huang N, Lang Q-L, He Q-L, Jian-Hua W, et al. A Novel Targeted GPC3/CD3 Bispecific Antibody for the Treatment Hepatocellular Carcinoma. Cancer Biol Ther (2020) 21(7):597–603. doi: 10.1080/15384047.2020.1743158
114. Liu X, Gao F, Jiang L, Jia M, Ao L, Lu M, et al. 32A9, a Novel Human Antibody for Designing an Immunotoxin and CAR-T Cells Against Glypican-3 in Hepatocellular Carcinoma. J Transl Med (2020) 18(1):295. doi: 10.1186/s12967-020-02462-1
115. Du K, Li Y, Liu J, Chen W, Wei Z, Luo Y, et al. A Bispecific Antibody Targeting GPC3 and CD47 Induced Enhanced Antitumor Efficacy Against Dual Antigen-Expressing HCC. Mol Ther (2021) 29(4):1572–84. doi: 10.1016/j.ymthe.2021.01.006
116. Li D, Li N, Zhang Y-F, Fu H, Feng M, Schneider D, et al. Persistent Polyfunctional Chimeric Antigen Receptor T Cells That Target Glypican 3 Eliminate Orthotopic Hepatocellular Carcinomas in Mice. Gastroenterology (2020) 158(8):2250–65. doi: 10.1053/j.gastro.2020.02.011
117. Sun L, Gao F, Gao Z, Ao L, Li N, Ma S, et al. Shed Antigen-Induced Blocking Effect on CAR-T Cells Targeting Glypican-3 in Hepatocellular Carcinoma. J Immunother Cancer (2021) 9(4):1875–88. doi: 10.1136/jitc-2020-001875
118. Yu M, Luo H, Fan M, Wu X, Shi B, Di S, et al. Development of GPC3-Specific Chimeric Antigen Receptor-Engineered Natural Killer Cells for the Treatment of Hepatocellular Carcinoma. Mol Ther (2018) 26(2):366–78. doi: 10.1016/j.ymthe.2017.12.012
119. Liao N, Su L, Zheng Y, Zhao B, Wu M, Zhang D, et al. In Vivo Tracking of Cell Viability for Adoptive Natural Killer Cell-Based Immunotherapy by Ratiometric NIR-II Fluorescence Imaging. Angew Chem Int Ed Engl (2021). doi: 10.1002/anie.202106730
120. Liu Q, Tian Y, Li Y, Zhang W, Cai W, Liu Y, et al. In Vivo Therapeutic Effects of Affinity-Improved-TCR Engineered T-Cells on HBV-Related Hepatocellular Carcinoma. J Immunother Cancer (2020) 8(2):1748–60. doi: 10.1136/jitc-2020-001748
121. Tseng H-C, Xiong W, Badeti S, Yang Y, Ma M, Liu T, et al. Efficacy of Anti-CD147 Chimeric Antigen Receptors Targeting Hepatocellular Carcinoma. Nat Commun (2020) 11(1):4810. doi: 10.1038/s41467-020-18444-2
122. Rettman P, Blunt MD, Fulton RJ, Vallejo AF, Bastidas-Legarda LY, España-Serrano L, et al. Peptide: MHC-Based DNA Vaccination Strategy to Activate Natural Killer Cells by Targeting Killer Cell Immunoglobulin-Like Receptors. J Immunother Cancer (2021) 9(5):1912–22. doi: 10.1136/jitc-2020-001912
123. Yang T, Li C, Wang X, Zhao D, Zhang M, Cao H, et al. Efficient Hepatic Delivery and Protein Expression Enabled by Optimized mRNA and Ionizable Lipid Nanoparticle. Bioact Mater (2020) 5(4):1053–61. doi: 10.1016/j.bioactmat.2020.07.003
124. Li G, Liu D, Kimchi ET, Kaifi JT, Qi X, Manjunath Y, et al. Nanoliposome C6-Ceramide Increases the Anti-Tumor Immune Response and Slows Growth of Liver Tumors in Mice. Gastroenterology (2018) 154(4):1024–36. doi: 10.1053/j.gastro.2017.10.050
125. Zuo B, Qi H, Lu Z, Chen L, Sun B, Yang R, et al. Alarmin-Painted Exosomes Elicit Persistent Antitumor Immunity in Large Established Tumors in Mice. Nat Commun (2020) 11(1):1790. doi: 10.1038/s41467-020-15569-2
126. Bruix J, Takayama T, Mazzaferro V, Chau G-Y, Yang J, Kudo M, et al. Adjuvant Sorafenib for Hepatocellular Carcinoma After Resection or Ablation (STORM): A Phase 3, Randomised, Double-Blind, Placebo-Controlled Trial. Lancet Oncol (2015) 16(13):1344–54. doi: 10.1016/S1470-2045(15)00198-9
127. Lencioni R, Llovet JM, Han G, Tak WY, Yang J, Guglielmi A, et al. Sorafenib or Placebo Plus TACE With Doxorubicin-Eluting Beads for Intermediate Stage HCC: The SPACE Trial. J Hepatol (2016) 64(5):1090–8. doi: 10.1016/j.jhep.2016.01.012
128. Meyer T, Fox R, Ma YT, Ross PJ, James MW, Sturgess R, et al. Sorafenib in Combination With Transarterial Chemoembolisation in Patients With Unresectable Hepatocellular Carcinoma (TACE 2): A Randomised Placebo-Controlled, Double-Blind, Phase 3 Trial. Lancet Gastroenterol Hepatol (2017) 2(8):565–75. doi: 10.1016/S2468-1253(17)30156-5
129. Yoshiji H, Noguchi R, Toyohara M, Ikenaka Y, Kitade M, Kaji K, et al. Combination of Vitamin K2 and Angiotensin-Converting Enzyme Inhibitor Ameliorates Cumulative Recurrence of Hepatocellular Carcinoma. J Hepatol (2009) 51(2):315–21. doi: 10.1016/j.jhep.2009.04.011
130. Facciorusso A, Del Prete V, Crucinio N, Muscatiello N, Carr BI, Di Leo A, et al. Angiotensin Receptor Blockers Improve Survival Outcomes After Radiofrequency Ablation in Hepatocarcinoma Patients. J Gastroenterol Hepatol (2015) 30(11):1643–50. doi: 10.1111/jgh.12988
131. Facciorusso A, Abd El Aziz MA, Cincione I, Cea UV, Germini A, Granieri S, et al. Angiotensin Receptor 1 Blockers Prolong Time to Recurrence After Radiofrequency Ablation in Hepatocellular Carcinoma Patients: A Retrospective Study. Biomedicines (2020) 8(10):399–410. doi: 10.3390/biomedicines8100399
132. Behm B, Di Fazio P, Michl P, Neureiter D, Kemmerling R, Hahn EG, et al. Additive Antitumour Response to the Rabbit VX2 Hepatoma by Combined Radio Frequency Ablation and Toll Like Receptor 9 Stimulation. Gut (2016) 65(1):134–43. doi: 10.1136/gutjnl-2014-308286
133. Duan X, Wang M, Han X, Ren J, Huang G, Ju S, et al. Combined Use of Microwave Ablation and Cell Immunotherapy Induces Nonspecific Immunity of Hepatocellular Carcinoma Model Mice. Cell Cycle (Georgetown Tex) (2020) 19(24):3595–607. doi: 10.1080/15384101.2020.1853942
134. Duffy AG, Ulahannan SV, Makorova-Rusher O, Rahma O, Wedemeyer H, Pratt D, et al. Tremelimumab in Combination With Ablation in Patients With Advanced Hepatocellular Carcinoma. J Hepatol (2017) 66(3):545–51. doi: 10.1016/j.jhep.2016.10.029
135. Agdashian D, ElGindi M, Xie C, Sandhu M, Pratt D, Kleiner DE, et al. The Effect of Anti-CTLA4 Treatment on Peripheral and Intra-Tumoral T Cells in Patients With Hepatocellular Carcinoma. Cancer Immunol Immunother (2019) 68(4):599–608. doi: 10.1007/s00262-019-02299-8
136. Wang X, Liu G, Chen S, Bi H, Xia F, Feng K, et al. Combination Therapy With PD-1 Blockade and Radiofrequency Ablation for Recurrent Hepatocellular Carcinoma: A Propensity Score Matching Analysis. Int J Hperthermia (2021) 38(1):1519–28. doi: 10.1080/02656736.2021.1991011
137. Lyu N, Kong Y, Li X, Mu L, Deng H, Chen H, et al. Ablation Reboots the Response in Advanced Hepatocellular Carcinoma With Stable or Atypical Response During PD-1 Therapy: A Proof-Of-Concept Study. Front Oncol (2020) 10:580241. doi: 10.3389/fonc.2020.580241
138. Ma H, Zhang Y, Wang Q, Li Y, He J, Wang H, et al. Therapeutic Safety and Effects of Adjuvant Autologous RetroNectin Activated Killer Cell Immunotherapy for Patients With Primary Hepatocellular Carcinoma After Radiofrequency Ablation. Cancer Biol Ther (2010) 9(11):903–7. doi: 10.4161/cbt.9.11.11697
139. Cui J, Wang N, Zhao H, Jin H, Wang G, Niu C, et al. Combination of Radiofrequency Ablation and Sequential Cellular Immunotherapy Improves Progression-Free Survival for Patients With Hepatocellular Carcinoma. Int J Cancer (2014) 134(2):342–51. doi: 10.1002/ijc.28372
140. Yoon JS, Song BG, Lee J-H, Lee HY, Kim SW, Chang Y, et al. Adjuvant Cytokine-Induced Killer Cell Immunotherapy for Hepatocellular Carcinoma: A Propensity Score-Matched Analysis of Real-World Data. BMC Cancer (2019) 19(1):523. doi: 10.1186/s12885-019-5740-z
141. Kitahara M, Mizukoshi E, Terashima T, Nakagawa H, Horii R, Iida N, et al. Safety and Long-Term Outcome of Intratumoral Injection of OK432-Stimulated Dendritic Cells for Hepatocellular Carcinomas After Radiofrequency Ablation. Transl Oncol (2020) 13(7):100777. doi: 10.1016/j.tranon.2020.100777
142. Sawada Y, Yoshikawa T, Ofuji K, Yoshimura M, Tsuchiya N, Takahashi M, et al. Phase II Study of the GPC3-Derived Peptide Vaccine as an Adjuvant Therapy for Hepatocellular Carcinoma Patients. Oncoimmunology (2016) 5(5):e1129483. doi: 10.1080/2162402X.2015.1129483
143. Zhou P, Liang P, Dong B, Yu X, Han Z, Xu Y. Phase I Clinical Study of Combination Therapy With Microwave Ablation and Cellular Immunotherapy in Hepatocellular Carcinoma. Cancer Biol Ther (2011) 11(5):450–6. doi: 10.4161/cbt.11.5.14669
144. Niu L-Z, Li J-L, Zeng J-Y, Mu F, Liao M-T, Yao F, et al. Combination Treatment With Comprehensive Cryoablation and Immunotherapy in Metastatic Hepatocellular Cancer. World J Gastroenterol (2013) 19(22):3473–80. doi: 10.3748/wjg.v19.i22.3473
145. Nobuoka D, Motomura Y, Shirakawa H, Yoshikawa T, Kuronuma T, Takahashi M, et al. Radiofrequency Ablation for Hepatocellular Carcinoma Induces Glypican-3 Peptide-Specific Cytotoxic T Lymphocytes. Int J Oncol (2012) 40(1):63–70. doi: 10.3892/ijo.2011.1202
146. Yang H, Liu H-S, Hou W, Gao J-X, Duan Y, Wei D, et al. An NIR-Responsive Mesoporous Silica Nanosystem for Synergetic Photothermal-Immunoenhancement Therapy of Hepatocellular Carcinoma. J Mater Chem B (2020) 8(2):251–9. doi: 10.1039/c9tb01891c
147. Zhou T, Liang X, Wang P, Hu Y, Qi Y, Jin Y, et al. A Hepatocellular Carcinoma Targeting Nanostrategy With Hypoxia-Ameliorating and Photothermal Abilities That, Combined With Immunotherapy, Inhibits Metastasis and Recurrence. ACS Nano (2020) 14(10):12679–96. doi: 10.1021/acsnano.0c01453
148. Cai Z, Xin F, Wei Z, Wu M, Lin X, Du X, et al. Photodynamic Therapy Combined With Antihypoxic Signaling and CpG Adjuvant as an In Situ Tumor Vaccine Based on Metal-Organic Framework Nanoparticles to Boost Cancer Immunotherapy. Adv Healthc Mater (2020) 9(1):e1900996. doi: 10.1002/adhm.201900996
149. Tan Y, Yang S, Ma Y, Li J, Xie Q, Liu C, et al. Nanobubbles Containing sPD-1 and Ce6 Mediate Combination Immunotherapy and Suppress Hepatocellular Carcinoma in Mice. Int J Nanomed (2021) 16:3241–54. doi: 10.2147/IJN.S305857
150. Lin X, Huang R, Huang Y, Wang K, Li H, Bao Y, et al. Nanosonosensitizer-Augmented Sonodynamic Therapy Combined With Checkpoint Blockade for Cancer Immunotherapy. Int J Nanomed (2021) 16:1889–99. doi: 10.2147/IJN.S290796
151. Cai H, Dai X, Guo X, Zhang L, Cao K, Yan F, et al. Ataxia Telangiectasia Mutated Inhibitor-Loaded Copper Sulfide Nanoparticles for Low-Temperature Photothermal Therapy of Hepatocellular Carcinoma. Acta Biomater (2021) 127:276–86. doi: 10.1016/j.actbio.2021.03.051
152. Xia Q-H, Lu C-T, Tong M-Q, Yue M, Chen R, Zhuge D-L, et al. Ganoderma Lucidum Polysaccharides Enhance the Abscopal Effect of Photothermal Therapy in Hepatoma-Bearing Mice Through Immunomodulatory, Anti-Proliferative, Pro-Apoptotic and Anti-Angiogenic. Front Pharmacol (2021) 12:648708. doi: 10.3389/fphar.2021.648708
153. Wang J, Meng J, Ran W, Lee RJ, Teng L, Zhang P, et al. Hepatocellular Carcinoma Growth Retardation and PD-1 Blockade Therapy Potentiation With Synthetic High-Density Lipoprotein. Nano Lett (2019) 19(8):5266–76. doi: 10.1021/acs.nanolett.9b01717
Keywords: hepatocellular carcinoma, ablation, immunotherapy, synergistic therapy, multifunctional nanoplatform, nanomedicine
Citation: Wang K, Wang C, Jiang H, Zhang Y, Lin W, Mo J and Jin C (2021) Combination of Ablation and Immunotherapy for Hepatocellular Carcinoma: Where We Are and Where to Go. Front. Immunol. 12:792781. doi: 10.3389/fimmu.2021.792781
Received: 11 October 2021; Accepted: 25 November 2021;
Published: 15 December 2021.
Edited by:
Xuesong Gu, Beth Israel Deaconess Medical Center and Harvard Medical School, United StatesReviewed by:
James Ahodantin, University of Maryland, Baltimore, United StatesAntonio Facciorusso, University of Foggia, Italy
Copyright © 2021 Wang, Wang, Jiang, Zhang, Lin, Mo and Jin. This is an open-access article distributed under the terms of the Creative Commons Attribution License (CC BY). The use, distribution or reproduction in other forums is permitted, provided the original author(s) and the copyright owner(s) are credited and that the original publication in this journal is cited, in accordance with accepted academic practice. No use, distribution or reproduction is permitted which does not comply with these terms.
*Correspondence: Chong Jin, jinc@tzzxyy.com; Jinggang Mo, mojg@tzzxyy.com