- 1Virus and Microbiological Special Diagnostics, Statens Serum Institut, Copenhagen, Denmark
- 2Centre for HIV and STI’s, National Institute for Communicable Diseases, Johannesburg, South Africa
- 3Faculty of Health Sciences, University of the Witwatersrand, Johannesburg, South Africa
Receptors for the crystallisable fragment (Fc) of immunoglobulin (Ig) G, Fcγ receptors (FcγRs), link the humoral and cellular arms of the immune response, providing a diverse armamentarium of antimicrobial effector functions. Findings from HIV-1 vaccine efficacy trials highlight the need for further study of Fc-FcR interactions in understanding what may constitute vaccine-induced protective immunity. These include host genetic correlates identified within the low affinity Fcγ-receptor locus in three HIV-1 efficacy trials – VAX004, RV144, and HVTN 505. This perspective summarizes our present knowledge of FcγR genetics in the context of findings from HIV-1 efficacy trials, and draws on genetic variation described in other contexts, such as mother-to-child HIV-1 transmission and HIV-1 disease progression, to explore the potential contribution of FcγR variability in modulating different HIV-1 vaccine efficacy outcomes. Appreciating the complexity and the importance of the collective contribution of variation within the FCGR gene locus is important for understanding the role of FcγRs in protection against HIV-1 acquisition.
Introduction
Despite enormous research efforts over 30 years, a highly efficacious preventative HIV vaccine remains elusive. Nonetheless, each vaccine efficacy trial provided new insight. Only one HIV-1 vaccine trial has shown some level of protection against HIV-1 acquisition. The RV144 vaccine trial (1), conducted in Thailand, achieved modest vaccine efficacy at 31.2%, while 6 other efficacy trials – VAX003 (2), VAX004 (3), HVTN502 (the Step trial) (4), HVTN503 (the Phambili trial) (5), HVTN505 (6), and HVTN702 (the RV144 follow-on trial) (7) – failed to prevent HIV-1 acquisition in vaccinees, and even increased risk in some individuals (4, 8). Many differences could account for the efficacy outcomes, including the vaccine regimen (design, virus subtype, and adjuvant), diversity of circulating virus strains, sex, modes of transmission, different risk populations, geography, and host genetics.
The initial immune correlate analysis from RV144 (9) provided the impetus for more detailed study of immune correlates to better understand vaccine-induced immune protection against HIV-1. These subsequent studies and analyses have revealed the inordinately complex nature of immunological mechanisms that collectively act to provide protection against acquisition of HIV-1 [reviewed in (10)]. In particular, they have highlighted many HIV-specific antibody parameters as correlates of HIV-1 acquisition risk (9, 11–14), many of which bind FcγRs to mediate their functions. Indeed, FcγR-mediated effector functions associate with vaccine protection (9, 15). Host genetic correlates further implicating a role for FcγRs have been identified in three efficacy trials, VAX004 (16), RV144 (17), and HVTN505 (18); each conducted in different population groups with distinct allelic variability across FcγRs (19).
Here we summarize our present knowledge of FcγR genetics in the context of findings from HIV-1 efficacy trials, and include studies of mother-to-child HIV-1 transmission and HIV-1 disease progression. We highlight the complexity of the FCGR locus, the importance of using validated methods to aid interpretation, the inclusion of FCGR gene copy number determination, and population genetic differences, among other considerations outlined.
The Low Affinity FcγRs and Host Genetic Variability
IgG, elicited through active immunization (infection or vaccination) or transferred passively (intravenous infusion or transplacental), modulates an antiviral response through several mechanisms. The antigen binding fragment (Fab) may neutralize virus infection by binding viral surface proteins and preventing attachment to host receptors, while the antibody Fc domain direct immune mechanisms through the engagement of FcγRs. Cross-linking of FcγRs on the cell surface through multivalent interactions, initiates responses that include antibody-dependent cellular cytotoxicity (ADCC), antibody-dependent cellular phagocytosis (ADCP), oxidative burst, release of inflammatory mediators, and regulation of antibody production (Figure 1A) (21–24).
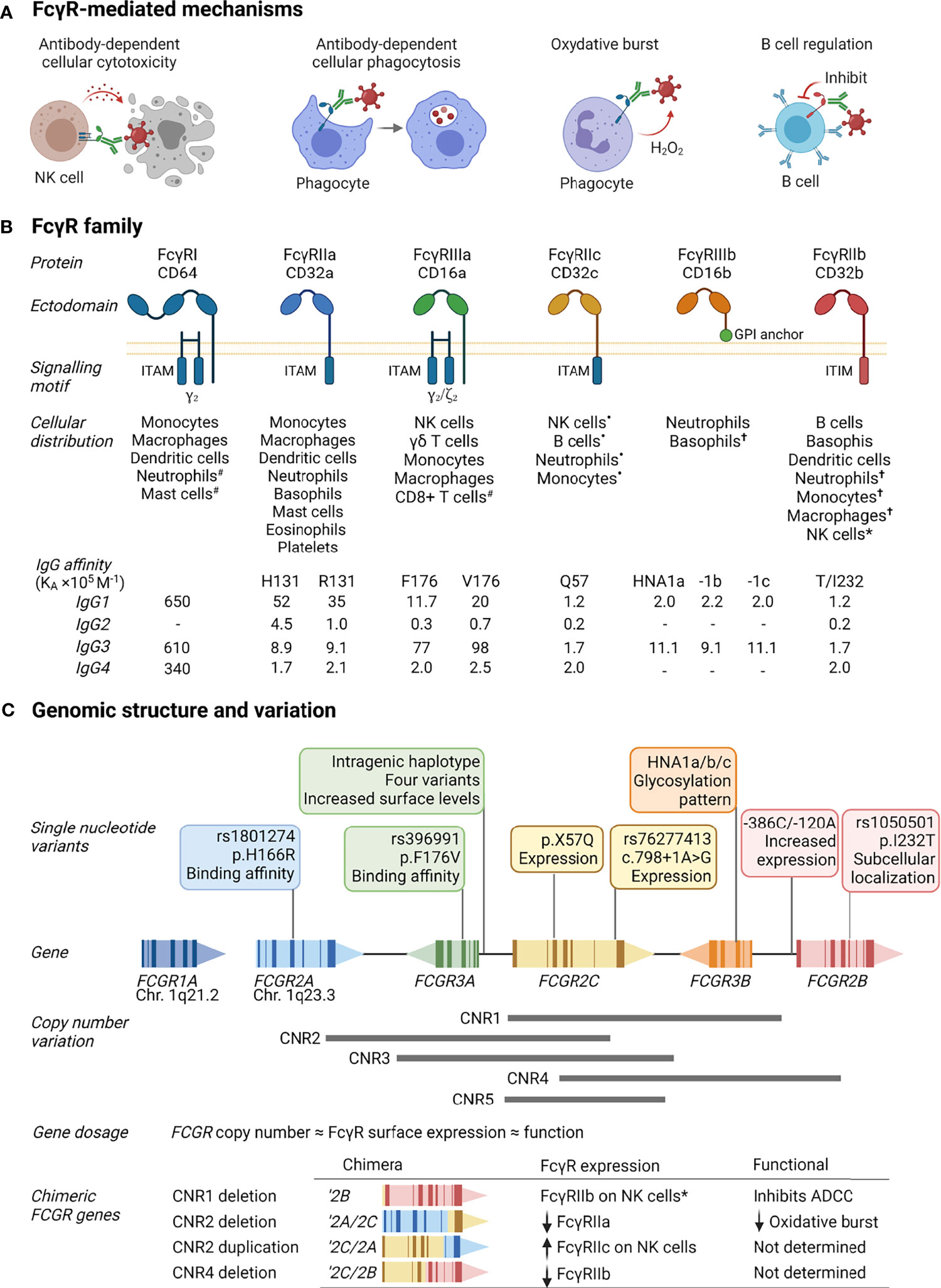
Figure 1 FcγR function, structure and variability. (A) FcγRs activate or inhibit immune mechanisms that include killing of infected cells through antibody-dependent cellular cytotoxicity, clearance of immune complexes through phagocytosis, release of reactive oxygen species [superoxide anion (O-2) and hydrogen peroxide (H2O2)], and regulation of B cell activation through co-engaging the B cell receptor and inhibitory FcγRIIb by immune complexes. (B) FcγRs comprise a family of receptors: FcγRI, FcγRIIa, FcγRIIb, FcγRIIc, FcγRIIIa, FcγRIIIb; also known by their cluster of differentiation (CD) markers CD64, CD32a, CD32b, CD32c, CD16a, and CD16b, respectively. The FcγRs IgG binding chain activates or regulates immune responses depending on its association with or inclusion of an immunoreceptor tyrosine activation motif (ITAM) or inhibitory motif (ITIM). Unique among FcγRs, FcγRIIIb attaches to the cell membrane with a glycosylphosphatidylinositol (GPI) anchor. Each receptor has a specific cell expression profile and affinity for IgG and its subtypes (IgG1-4), shown as affinity constants (KA×105 M-1); -, no binding. Expression patterns: #inducible expression; •in individuals bearing the FCGR2C expression variants (20); †very low expression or expressed by rare subsets; *expressed in individuals bearing a FCGR2C-FCGR3B gene deletion. (C) The cluster of FCGR2A/B/C and FCGR3A/B genes on chromosome 1q23.3 that encode FcγRIIa/b/c and FcγRIIIa/b are polymorphic. Variants include nonsynonymous single nucleotide polymorphisms that alter the receptor’s binding affinity for certain IgG subtypes, determine expression of an otherwise pseudogene, increase surface expression, glycosylation, and subcellular localization. Large segmental duplications and deletions in the FCGR cluster further modify FcγR expression levels and create chimeric genes that yield FcγRs with altered cellular distribution and/or function. Created with BioRender.com.
FcγRs are a complex family of activating and inhibitory receptors, comprising three classes of molecules and different isoforms: FcγRIa, FcγRIIa/b/c, and FcγRIIIa/b (Figure 1B). All FcγRs are glycoproteins belonging to the Ig superfamily and consist of a ligand-binding α-chain with two (FcγRII and FcγRIII) or three (FcγRI) extracellular Ig-like domains, a transmembrane domain, and intracytoplasmic domain. The activating or inhibitory signaling motifs are located either within the α-chain (FcγRII) or associated signaling subunits (FcγRI and FcγRIIIa) (25). Unique to the FcγR family, FcγRIIIb attaches to the cell membrane with a glycosylphosphatidylinositol anchor. Despite lacking intrinsic cytoplasmic signaling domains, FcγRIIIb induces several cell responses (26–28). Each FcγR is expressed on specific cell types, either constitutively or induced, and has particular affinities for IgG and its subtypes (IgG1-4). The genes that encode FcγRs – FCGR1A, FCGR2A/B/C, and FCGR3A/B – are further subject to considerable allelic variation, resulting from segmental genomic duplications/deletions or single nucleotide polymorphisms.
FCGR2C, FCGR3A, and FCGR3B occur at different gene copies due to the gain or loss of defined copy number regions (CNR1-5, Figure 1C). The number of FCGR genes per diploid genome directly correlate with FcγR surface density and function (29, 30). In addition to this gene dosage effect, duplications/deletions create chimeric FCGRs that alter the cellular distribution, expression, and function of FcγRs. A deletion of CNR1, present in 7.4-18.1% of individuals depending on ethnicity, juxtaposes the 5’-regulatory sequences of FCGR2C with the coding sequence of FCGR2B, creating the chimeric FCGR2B’ and expression of FcγRIIb on cytotoxic NK cells where it inhibits cell activation and ADCC (31, 32). A CNR2 deletion, present in <1.5% of individuals, leads to an FCGR2A/2C chimera that result in reduced FcγRIIa surface levels and oxidative burst response (32, 33). Conversely, a CNR2 duplication, present in 1.6-4.5% of individuals, leads to an FCGR2C/2A chimeric gene that increases FcγRIIc expression levels.
Allelic variation for FcγRI is low. In contrast, several single nucleotide variants with a known phenotypic or functional consequence exist for FcγRIIa/b/c and FcγRIIIa/b (34). Distinct amino acid changes in the membrane proximal Ig-like domain of FcγRIIa and FcγRIIIa alter their affinity for IgG subtypes and associated effector functions, including FcγRIIa-p.H166R (alias H131R, rs1801274) and FcγRIIIa-p.F176V (alias F158V, rs396991) (35–38). Conversely, in the transmembrane domain of FcγRIIb, the p.I232T variant (rs1050501) alters its inclusion in lipid rafts and inhibitory signaling (39). In FcγRIIIb, a combination of six amino acid changes determine the human neutrophil antigens (HNA) 1a/b/c – molecules that are antigenically distinct and modulate neutrophil phagocytosis and oxidative burst (40). Unlike other FCGRs, FCGR2C occurs predominantly as a pseudogene, where a combination of FCGR2C minor alleles – p.X57Q (alias X13Q) and c.798+1A>G (rs76277413) – determine its surface expression (20, 41). Other co-inherited single nucleotide variants (haplotypes) within the promotor region of FCGR2B/C and spanning FCGR3A modulate surface expression levels of FcγRIIb/c and FcγRIIIa, respectively (42–44).
Over the past few years, research identified several new FCGR variants of clinical relevance in the context of HIV-1 (described below). Although, linkage disequilibrium (co-occurring variants) in the FCGR locus has impeded identification of potential causal variants (19, 45, 46). Studying FCGR variants in different population groups in the same and/or different context may help define a role for specific variants, since linkage disequilibrium is inconsistent between geographical populations (19). Of note, describing new FCGR variants and assigning them to specific FcγRs warrants caution, since high nucleotide sequence homology between FCGRs could lead to inaccurate assignment of variants to specific genes (34); thus, highlighting the need for validated genotyping methods. In general, for the description of new and conventional FCGR variants, we encourage the use of a single international genotypic variation nomenclature as described by the Human Genome Variation Society (HGVS) to enable cross-referencing of FCGR variants between studies (34, 47). We include here the HGVS name for all variants.
FcγR Gene Variants and HIV Vaccine Efficacy Trials
In HIV-1 vaccine efficacy trials, studies have shown clear associations between FcγR-mediated effector functions and risk of HIV-1 acquisition following vaccination (9, 15, 16, 48). To dissect further, three vaccine efficacy studies to date have investigated FcγR variation as a modifier of antibody Fc-mediated effector functions and HIV-1 acquisition risk (Figure 2A).
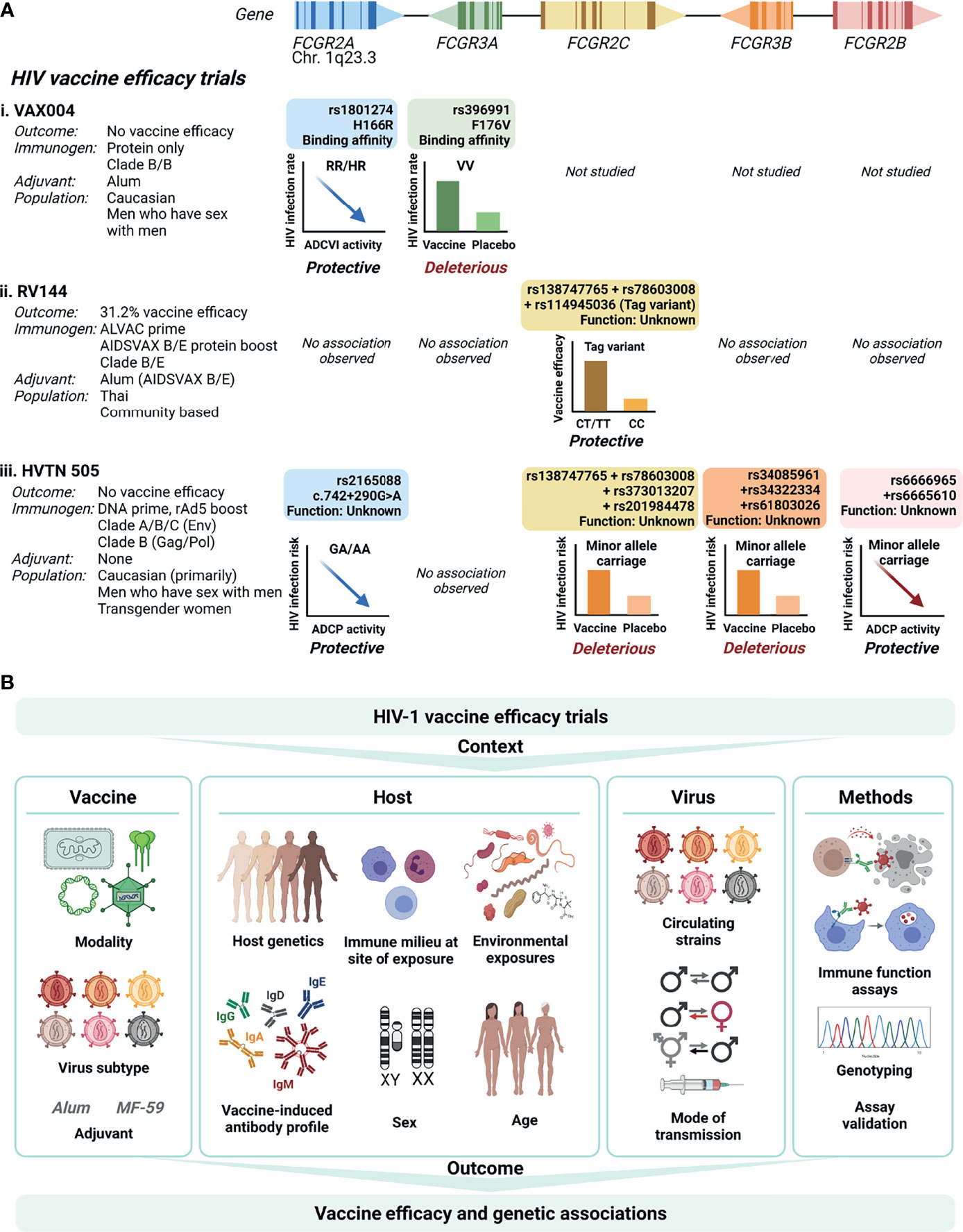
Figure 2 FCGR variant associations with HIV-1 vaccine efficacy trial outcomes. (A) To date, three HIV-1 vaccine efficacy trials investigated the association between FCGR variants and HIV-1 acquisition risk: VAX004, RV144 and HVTN505. The trials differed with regard to vaccine modalities, target HIV-1 subtypes, study populations, mode of HIV-1 transmission, and host ethnicities. In VAX004 and HVTN505 vaccinees bearing minor alleles within FCGR2A and FCGR2B, enhanced Fc-mediated effector functions [antibody-dependent cellular viral inhibition (ADCVI) and antibody-dependent cellular phagocytosis (ADCP), respectively] associated with reduced risk of HIV-1 acquisition. In VAX004, enhanced HIV-1 acquisition occurred in vaccinees homozygous for the FcγRIIIa-176V allele. Co-inherited intragenic minor alleles in FCGR2C enhanced vaccine efficacy in RV144, but increased HIV-1 acquisition risk in HVTN505. Similarly, co-inherited minor alleles in the 5’ untranslated region of FCGR3B associated with HIV-1 acquisition risk in HVTN505. (B) Defining FCGR genetic associations with HIV-1 vaccine efficacy is affected by several factors relating to the vaccine, the host, the virus and methodology used. Created with BioRender.com.
The VAX004 trial evaluated a recombinant envelope protein (AIDSVAX B/B) prime-boost regimen in predominantly Caucasian men who have sex with men (3). Vaccine recipients who remained uninfected had higher antibody-dependent cell-mediated virus inhibition (ADCVI) responses, which encompass ADCC, ADCP and the induction of soluble antiviral factors, than those who became infected (48). The magnitude of ADCVI responses inversely correlated with the HIV-1 acquisition rate, but only in individuals bearing low affinity alleles for FcγRIIa-p.H166R (HR/RR genotypes) and FcγRIIIa-p.F176V (FF genotype) (48) (Figure 2Ai). When adjusted for linkage disequilibrium between the two variants, an independent association with FcγRIIa-p.H166R remained. However, the FcγRIIa-p.H166R variant itself did not predict acquisition rate (16). Conversely, in the low risk behavioral group, vaccinees homozygous for the p.176V allele were at greater rate of acquiring HIV-1 compared to those who received the placebo (hazard ratio 4.51), suggesting enhanced infection from the use of AIDSVAX B/B in this genotype group (16).
The RV144 trial, which evaluated a heterologous ALVAC-HIV (vCP1521) canary pox vector prime and AIDSVAX B/E protein boost regimen, demonstrated modest vaccine efficacy (31.2%) in Thai individuals (1). The primary determinants of vaccine efficacy were binding IgG to the variable loops 1 and 2 (V1V2) region of gp120 and binding of plasma IgA to envelope (9). In a secondary analysis, the combination of high levels of ADCC and low plasma anti-HIV-1 envelope IgA antibodies inversely correlated with HIV-1 acquisition risk (9). Variants within FcγRIIIa, the major FcγR involved in NK cell-mediated ADCC, did not associate with HIV-1 acquisition risk (17) (Figure 2Aii). Conversely, three single nucleotide variants within FCGR2C significantly modified vaccine efficacy that include FCGR2C 126C>T (HVGS name: c.134-96C>T, rs114945036), c.353C>T (p. T118I, rs138747765), and c.391+111G>A (rs78603008) (17). All variants were in complete linkage disequilibrium in Thai RV144 trial participants, forming a haplotype. Possession of the haplotype associated with an estimated vaccine efficacy of 91% against CRF01_AE 169K HIV-1 and 64% against any HIV-1 strain, compared to 15% and 11% in the absence of the haplotype, respectively. The functional significance of the variant is unrelated to FcγRIIc surface expression, since only one study participant carried an FcγRIIc-p.57Q allele that predicts expression (17). Alternatively, the haplotype locates within a weak transcriptional enhancer (49). The minor alleles likely abrogate binding of repressor proteins within the regulatory motif and increase mRNA expression. Indeed, in Epstein-Barr virus transformed lymphoblastoid B-cell lines from European Caucasians, the minor allele haplotype associated with increased expression of FCGR2A and/or FCGR2C exon 7 (50). Other FCGR2C variants in complete linkage disequilibrium with the haplotype include c.113-1058T>C (rs2169052/rs115953596) and c.113-684C>T (rs111828362) (49) were not genotyped in RV144 participants and warrant further investigation. Of significance, two components of the haplotype, p.T118I (rs138747765) and c.391+111G>A (rs78603008), are rarely polymorphic in Africans (19), where the RV144 follow-up trial HVTN 702 failed to protect against HIV-1 infection (7).
The HVTN 505 trial that evaluated another heterologous prime-boost regimen – a multigene, multiclade DNA prime and recombinant adenovirus 5 (rAd5) boost – did not show any efficacy in a cohort of predominantly Caucasian men who have sex with men (6). However, ADCP responses and binding of immune complexes to recombinant FcγRIIa-p.166H inversely correlated with HIV-1 acquisition risk (15) (Figure 2Aiii). The associations increased for individuals without HIV-1 envelope IgA. Intriguingly, in a phase IIa clinical trial of the same DNA/rAd5 regimen (HVTN 204) (51), a different group did not detect ADCP responses (52). The cause of the distinct observations is unclear; both groups used the same assay albeit a different antibody source (isolated IgG vs. serum) and antigen (vaccine clade-specific gp120 vs. Con S gp140) (52). In the HVTN 505 trial participants, targeted sequencing of regions encoding the extracellular domains of FcγRs identified several variants that associated with HIV-1 acquisition risk or Fc-mediated effector functions. An FCGR2A intronic variant modified HIV-1 acquisition risk, FCGR2A-intron13-645-G/A (HGVS name: c.742+290G>A, rs2165088) (15). In vaccine recipients bearing the minor allele of c.742+290G>A, the magnitude of ADCP responses and FcγRIIa-p.166H binding to antibody-rgp140 complexes associated with reduced risk of HIV-1 acquisition (15). The functional consequence of FCGR2A c.742+290G>A is unknown and it does not appear to be in complete or high linkage disequilibrium with other variants in, or flanking, FCGR2A. Inverse correlations between ADCP with HIV-1 acquisition risk similarly occurred for participants bearing minor alleles of two FCGR2B variants (synonymous FCGR2B-exon5-523-G/A; HGVS name: c.336G>A, rs6665610 and FCGR2B-intron14-352-T/G; HGVS name: c.760+26T>G, rs6666965) (18). c.336G>A is in high linkage disequilibrium with seven other FCGR2B variants and associated with decreased expression of FCGR2A (18).
Furthermore, in HVTN 505 participants, a four-variant FCGR2C haplotype and three-variant FCGR3B haplotype associated with increased HIV-1 acquisition risk (hazard ratio 9.79 and 2.78, respectively) (18) (Figure 2Aiii). The FCGR2C haplotype comprise two of the three FCGR2C variants identified as protective in the RV144 vaccine trial (p.T118I, rs138747765; and c.391+111G>A, rs78603008). The lack of association with the third FCGR2C variant (c.134-96C>T, rs114945036) is likely due to incomplete linkage disequilibrium of the three FCGR2C variants in Caucasians (49), the predominant ethnicity of HVTN 505 participants. Additional FCGR2C variants were in complete linkage disequilibrium in HVTN 505 participants, FCGR2C-intron15-403-C/T (HGVS name: c.760+81C>T, rs373013207] and FCGR2C-intron15-433-G/A (HGVS name: c.760+111G>A, rs201984478). The functional consequences of these variants remains to be determined. The haplotype within FCGR3B that also associated with increased HIV-1 acquisition comprise three variants in the 5’ untranslated region of FCGR3B, 111 to 126 nucleotides upstream of the transcription start site and potentially in the gene promoter region. These include FCGR3B-5’utr222-G/A (HGVS name: c.-111G>T; rs34085961), FCGR3B-5’utr44-T/A (HGVS name: c.-181T>A, rs34322334), and FCGR3B-5’utr99-C/G (HGVS name: c.-126C>G, rs61803026). In individuals with the FCGR3B haplotype, vaccination was less likely to induce potentially protective envelope-specific IgG and/or CD8+ T-cell responses than for individuals without the FCGR3B haplotype.
FcγR Variants in Other HIV Infection and Disease Contexts
Mother-to-child-transmission. Investigations of FCGR variants and mother-to-child-transmission risk are limited to two Kenyan cohorts and one South African cohort (53–55). In a Kenyan cohort of grouped perinatal HIV-1 transmission routes (in utero, intrapartum, and breastfeeding), infants with the FcγRIIa-p.166HH genotype were at increased risk of acquiring HIV-1 compared with infants bearing the p.166HR genotype (53). Studies of a Kenyan cohort with a large representation of breastfeeding HIV-1 transmission and our South African cohort with predominantly in utero and intrapartum HIV-1 transmission, did not replicate these findings (55, 56). In the latter two cohorts, the maternal FcγRIIIa-F176V variant associated with HIV-1 transmission, although with contrasting findings. In the Kenyan cohort of predominantly breastfeeding women, heterozygous mothers (FV) had an increased risk of transmitting HIV-1 compared to homozygous mothers (combined FF/VV); however, carriage of the 176V allele did not predict HIV-1 transmission (56). If adjustment for multiple comparisons were applied in the study, the association would not have been statistically significant. In contrast, our South African cohort revealed a protective role for the 176V allele in in utero transmission, where the association remained significant after adjustment for multiple comparisons (55). A recent study of FCGR2C variability in South African children revealed a protective role for a single gene copy of FCGR2C/3B per diploid genome (57). In contrast, children bearing the minor allele of the FCGR2C variant c.134-96C>T (rs114945036) – identified as protective in Thai RV144 vaccine recipients (17) – were more likely to acquire HIV-1 compared to children homozygous for the c.134-96C allele (57).
Disease progression. The FcγRIIa low affinity genotype, p.166RR, predicted a faster CD4 decline compared to p.166RH/HH in the Multicenter AIDS Cohort Study (MACS) of predominantly Caucasian men who have sex with men (58). A similar analysis in Kenyan women – a different host genetic background, sex and route of transmission – showed no effect (59). In addition, the variant did not modify natural control of HIV-1 infection in African Americans (60, 61). Despite convincing evidence for a role for ADCC in natural HIV-1 control [reviewed in (62)], the FcγRIIIa-p.F176V variant does not appear to modify HIV-1 disease course in Caucasians (58) or African Americans (60) (after adjusting for multiple comparisons). Neither FcγRIIa-p.H166R, FcγRIIIa-p.F176V, nor FcγRIIb-p.I232T associated with HIV-1 control in the French multicentric CODEX cohort (63). Of note, the potential for FcγR variants to modify HIV-1 control may only become apparent when considering variability within the ligand, such as IgG γ chain phenotypes (GM allotypes). For example, in individuals bearing the FcγRIIa p.166HH or FcγRIIIa p.176FV/VV genotypes, HIV-1 viraemic control was more likely in the absence of the IgG GM21 allotype (61). Beyond the protein-coding region, a variant located 3.1 kilobases upstream of FCGR2A, g.1954 A>G (rs10800309), modified HIV-1 disease progression in a cohort of predominantly Caucasian men and women (63). In particular, homozygosity for g.1954A allele, which associates with increased FcγRIIa surface expression on myeloid cells, predicted natural control of HIV-1 independent of HLA-B57 and HLA-B27 (63). Another non-coding variant, the FCGR2C variant c.134-96C>T (rs114945036), predicted HIV-1 disease progression in South Africans (49), the same population where the RV144 follow-on trial, HVTN702, failed to show efficacy (7). However, in the French multicentric CODEX cohort of predominantly Caucasian individuals, the same FCGR2C variant did not associate with disease progression (63). It is unclear whether the different outcomes of RV144 and HVTN702 result from diverse population genetics, that include FCGR2C, or vaccine-associated factors that include differences in HIV-1 subtype envelopes, mismatched circulating strains, adjuvant or additional booster vaccination. Regardless, the collective findings further emphasize the importance of the FCGR2C locus, and additional study in different contexts will help elucidate the underlying protective/deleterious mechanisms.
Discussion
Many factors affect the host immunological response to immunization and to the pathogen (HIV) encountered. These include i) the route of inoculation and of HIV-1 acquisition, ii) immunogen/virus variability, iii) vaccine regimen (modality, dose, timing, adjuvant), iv) other prior exposures (related or unrelated), comorbidities and pre-existing infections, v) age, vi) sex, vii) geography (population genetics), and viii) genetic variation of the host (Figure 2B). The immune milieu present at antigen encounter is affected by all these factors, which collectively define what could be called “an immunological founder effect” – a measure of an individual’s immune capability that dictates the likelihood of producing a protective response to vaccination or infection. As context matters, the antibody Fc-FcγR axis, implicated in protection from acquisition of HIV-1 in vaccine recipients, would be expected to be modulated by these factors.
Investigations of FcγRs and their variants warrant several considerations. i) There are no association studies of FCGR copy number variation and HIV vaccine outcome. In RV144, ADCC was a correlate of protection. It is therefore plausible that a CNR1 deletion, which results in the expression of the inhibitory FcγRIIb on NK cells and subsequent inhibition of ADCC, may have an effect on vaccine efficacy. ii) Investigations of single nucleotide variants need to adjust for FCGR gene copy number. Certain minor alleles are more prevalent in individuals with more than two gene copies and may confound quantitative trait loci studies of FCGR variants (49). iii) Investigations of Fc-mediated effector functions should consider the autologous FcγR variants since they modulate binding of the receptor to antibodies, surface expression levels of the receptor, and/or cell activation/inhibition (64). iv) FCGR genes are highly homologous. Assigning single nucleotide variants to specific FCGRs requires validated methods. v) Considerable linkage disequilibrium between single nucleotide variants exist across the FCGR gene region (19, 45, 46), complicating identification of potential causal variants. vi) Increasing evidence suggest a clinical significance for non-coding FCGR variants highlighting potential complex cis- or transgene regulation that warrants characterization and investigation in other contexts. vii) FcγRs often co-occur on the same cell type. Elucidating the role of a single variant requires adjusting for allelic variants in co-expressed FcγRs, since the collective function of all co-expressed FcγRs will determine the effector response. Furthermore, phenotypic and functional analyses of FCGR genotype combinations are highly relevant, as demonstrated by an association of the FCGR2A rs1801274:rs10800309 diplotype with cell-type specific FcγRII expression (65) and FcγRIIa: FcγRIIIb haplotypes with neutrophil function (66). viii) FCGR variation – gene copy number variation, single nucleotide variants, and linkage disequilibrium – differ significantly between population groups and genetic association cannot necessarily be extrapolated between groups. ix) Phenotypic and functional consequences of allelic variants should be studied in the disease context and immune milieu of the condition under study, since disease may alter allelic function (67).
In summary, FCGR genetic variants have been associated with protective or deleterious infection and disease outcomes. Much insight can be gained into the potential functional significance of these variants by testing samples from other efficacy trials. For example, HVTN 702, which was non-efficacious in South Africans immunized with subtype C envelope ALVAC-HIV (vCP2438) prime and an MF59-adjuvanted subtype C bivalent envelope protein boost (7). Similarly, individuals passively immunized with broadly neutralizing antibody (VRC01) in the Antibody Mediated Prevention (AMP) trials (68) provide another informative study model. Harnessing host genetic variation between populations, and studying the collective contribution of FCGR variants in different infection/disease contexts, will provide much needed insights into what constitutes protective immunity to HIV-1. Importantly, the considerations discussed here extend beyond the context of HIV, bearing relevance to other infections and vaccination strategies that encompass endemic [e.g. malaria (69)], epidemic [e.g. influenza and respiratory syncytial virus (70–72)], pandemic [e.g. severe acute respiratory syndrome coronavirus 2 (SARS-CoV-2) (73)], and emerging/re-emerging infectious diseases [e.g. Ebola (74, 75)].
Data Availability Statement
The original contributions presented in the study are included in the article/supplementary material. Further inquiries can be directed to the corresponding author.
Author Contributions
RL and CT conceptualized and wrote the article. Figures were generated by RL. All authors contributed to the article and approved the submitted version.
Funding
CT receives funding as part of the South African Research Chairs Initiative of the Department of Science and Innovation and National Research Foundation (84177).
Conflict of Interest
The authors declare that the research was conducted in the absence of any commercial or financial relationships that could be construed as a potential conflict of interest.
Publisher’s Note
All claims expressed in this article are solely those of the authors and do not necessarily represent those of their affiliated organizations, or those of the publisher, the editors and the reviewers. Any product that may be evaluated in this article, or claim that may be made by its manufacturer, is not guaranteed or endorsed by the publisher.
References
1. Rerks-Ngarm S, Pitisuttithum P, Nitayaphan S, Kaewkungwal J, Chiu J, Paris R, et al. Vaccination With ALVAC and AIDSVAX to Prevent HIV-1 Infection in Thailand. N Engl J Med (2009) 361:2209–20. doi: 10.1056/NEJMoa0908492
2. Pitisuttithum P, Gilbert P, Gurwith M, Heyward W, Martin M, Van Griensven F, et al. Randomized, Double-Blind, Placebo-Controlled Efficacy Trial of a Bivalent Recombinant Glycoprotein 120 HIV-1 Vaccine Among Injection Drug Users in Bangkok, Thailand. J Infect Dis (2006) 194:1661–71. doi: 10.1086/508748
3. Flynn NM, Forthal DN, Harro CD, Judson FN, Mayer KH, Para MF, et al. Placebo-Controlled Phase 3 Trial of a Recombinant Glycoprotein 120 Vaccine to Prevent HIV-1 Infection. J Infect Dis (2005) 191:654–65. doi: 10.1086/428404
4. Buchbinder SP, Mehrotra DV, Duerr A, Fitzgerald DW, Mogg R, Li D, et al. Efficacy Assessment of a Cell-Mediated Immunity HIV-1 Vaccine (the STEP Study): A Double-Blind, Randomised, Placebo-Controlled, Test-Of-Concept Trial. Lancet (2008) 372:1881–93. doi: 10.1016/S0140-6736(08)61591-3
5. Gray GE, Allen M, Moodie Z, Churchyard G, Bekker LG, Nchabeleng M, et al. Safety and Efficacy of the HVTN 503/Phambili Study of a Clade-B-Based HIV-1 Vaccine in South Africa: A Double-Blind, Randomised, Placebo-Controlled Test-Of-Concept Phase 2b Study. Lancet Infect Dis (2011) 11:507–15. doi: 10.1016/S1473-3099(11)70098-6
6. Hammer SM, Sobieszczyk ME, Janes H, Karuna ST, Mulligan MJ, Grove D, et al. Efficacy Trial of a DNA/rAd5 HIV-1 Preventive Vaccine. N Engl J Med (2013) 369:2083–92. doi: 10.1056/NEJMoa1310566
7. Gray GE, Bekker LG, Laher F, Malahleha M, Allen M, Moodie Z, et al. Vaccine Efficacy of ALVAC-HIV and Bivalent Subtype C Gp120-MF59 in Adults. N Engl J Med (2021) 384:1089–100. doi: 10.1056/NEJMoa2031499
8. Gray GE, Moodie Z, Metch B, Gilbert PB, Bekker LG, Churchyard G, et al. Recombinant Adenovirus Type 5 HIV Gag/Pol/Nef Vaccine in South Africa: Unblinded, Long-Term Follow-Up of the Phase 2b HVTN 503/Phambili Study. Lancet Infect Dis (2014) 14:388–96. doi: 10.1016/S1473-3099(14)70020-9
9. Haynes BF, Gilbert PB, McElrath MJ, Zolla-Pazner S, Tomaras GD, Alam SM, et al. Immune-Correlates Analysis of an HIV-1 Vaccine Efficacy Trial. N Engl J Med (2012) 366:1275–86. doi: 10.1056/NEJMoa1113425
10. Tomaras GD, Plotkin SA. Complex Immune Correlates of Protection in HIV-1 Vaccine Efficacy Trials. Immunol Rev (2017) 275:245–61. doi: 10.1111/imr.12514
11. Gottardo R, Bailer RT, Korber BT, Gnanakaran S, Phillips J, Shen X, et al. Plasma IgG to Linear Epitopes in the V2 and V3 Regions of HIV-1 Gp120 Correlate With a Reduced Risk of Infection in the RV144 Vaccine Efficacy Trial. PloS One (2013) 8:e75665. doi: 10.1371/journal.pone.0075665
12. Zolla-Pazner S, Decamp A, Gilbert PB, Williams C, Yates NL, Williams WT, et al. Vaccine-Induced IgG Antibodies to V1V2 Regions of Multiple HIV-1 Subtypes Correlate With Decreased Risk of HIV-1 Infection. PloS One (2014) 9:e87572. doi: 10.1371/journal.pone.0087572
13. Yates NL, Liao HX, Fong Y, Decamp A, Vandergrift NA, Williams WT, et al. Vaccine-Induced Env V1-V2 IgG3 Correlates With Lower HIV-1 Infection Risk and Declines Soon After Vaccination. Sci Transl Med (2014) 6:228ra239. doi: 10.1126/scitranslmed.3007730
14. Tomaras GD, Ferrari G, Shen X, Alam SM, Liao HX, Pollara J, et al. Vaccine-Induced Plasma Iga Specific for the C1 Region of the HIV-1 Envelope Blocks Binding and Effector Function of IgG. Proc Natl Acad Sci USA (2013) 110:9019–24. https://doi.org/10.1073/pnas.1301456110
15. Neidich SD, Fong Y, Li SS, Geraghty DE, Williamson BD, Young WC, et al. Antibody Fc Effector Functions and IgG3 Associate With Decreased HIV-1 Risk. J Clin Invest (2019) 129:4838–49. doi: 10.1172/JCI126391
16. Forthal DN, Gabriel EE, Wang A, Landucci G, Phan TB. Association of Fcgamma Receptor IIIa Genotype With the Rate of HIV Infection After Gp120 Vaccination. Blood (2012) 120:2836–42. doi: 10.1182/blood-2012-05-431361
17. Li SS, Gilbert PB, Tomaras GD, Kijak G, Ferrari G, Thomas R, et al. FCGR2C Polymorphisms Associate With HIV-1 Vaccine Protection in RV144 Trial. J Clin Invest (2014) 124:3879–90. doi: 10.1172/JCI75539
18. Li SS, Gilbert PB, Carpp LN, Pyo CW, Janes H, Fong Y, et al. Fc Gamma Receptor Polymorphisms Modulated the Vaccine Effect on HIV-1 Risk in the HVTN 505 HIV Vaccine Trial. J Virol (2019) 93(21):e02041–18. doi: 10.1128/JVI.02041-18
19. Lassauniere R, Tiemessen CT. Variability at the FCGR Locus: Characterization in Black South Africans and Evidence for Ethnic Variation in and Out of Africa. Genes Immun (2016) 17:93–104. doi: 10.1038/gene.2015.60
20. Van Der Heijden J, Breunis WB, Geissler J, De Boer M, Van Den Berg TK, Kuijpers TW. Phenotypic Variation in IgG Receptors by NonclassicalFCGR2C Alleles. J Immunol (2012) 188:1318–24. doi: 10.4049/jimmunol.1003945
21. Hogarth PM. Fc Receptors Are Major Mediators of Antibody Based Inflammation in Autoimmunity. Curr Opin Immunol (2002) 14:798–802. doi: 10.1016/S0952-7915(02)00409-0
22. Amigorena S, Bonnerot C, Drake JR, Choquet D, Hunziker W, Guillet JG, et al. Cytoplasmic Domain Heterogeneity and Functions of IgG Fc Receptors in B Lymphocytes. Science (1992) 256:1808–12. doi: 10.1126/science.1535455
23. Muta T, Kurosaki T, Misulovin Z, Sanchez M, Nussenzweig MC, Ravetch JV. A 13-Amino-Acid Motif in the Cytoplasmic Domain of Fc Gamma RIIb Modulates B-Cell Receptor Signalling. Nature (1994) 368:70–3. doi: 10.1038/368070a0
24. Tzeng SJ, Bolland S, Inabe K, Kurosaki T, Pierce SK. The B Cell Inhibitory Fc Receptor TrIgGers Apoptosis by a Novel C-Abl Family Kinase-Dependent Pathway. J Biol Chem (2005) 280:35247–54. doi: 10.1074/jbc.M505308200
25. Lanier LL, Yu G, Phillips JH. Analysis of Fc Gamma RIII (CD16) Membrane Expression and Association With CD3 Zeta and Fc Epsilon RI-Gamma by Site-Directed Mutation. J Immunol (1991) 146:1571–6. https://www.jimmunol.org/content/146/5/1571
26. Rosales C, Brown EJ. Signal Transduction by Neutrophil Immunoglobulin G Fc Receptors. Dissociation of Intracytoplasmic Calcium Concentration Rise From Inositol 1,4,5-Trisphosphate. J Biol Chem (1992) 267:5265–71. doi: 10.1016/S0021-9258(18)42761-5
27. Salmon JE, Brogle NL, Edberg JC, Kimberly RP. Fc Gamma Receptor III Induces Actin Polymerization in Human Neutrophils and Primes Phagocytosis Mediated by Fc Gamma Receptor Ii. J Immunol (1991) 146:997–1004. https://www.jimmunol.org/content/146/3/997
28. Garcia-Garcia E, Nieto-Castaneda G, Ruiz-Saldana M, Mora N, Rosales C. FcgammarIIa and FcgammarIIIb Mediate Nuclear Factor Activation Through Separate Signaling Pathways in Human Neutrophils. J Immunol (2009) 182:4547–56. doi: 10.4049/jimmunol.0801468
29. Willcocks LC, Lyons PA, Clatworthy MR, Robinson JI, Yang W, Newland SA, et al. Copy Number of FCGR3B, Which Is Associated With Systemic Lupus Erythematosus, Correlates With Protein Expression and Immune Complex Uptake. J Exp Med (2008) 205:1573–82. doi: 10.1084/jem.20072413
30. Breunis WB, Van Mirre E, Geissler J, Laddach N, Wolbink G, van der Schoot E, et al. Copy Number Variation at the FCGR Locus Includes FCGR3A, FCGR2C and FCGR3B But Not FCGR2A and FCGR2B. Hum Mutat (2009) 30:E640–650. doi: 10.1002/humu.20997
31. Mueller M, Barros P, Witherden AS, Roberts AL, Zhang Z, Schaschl H, et al. Genomic Pathology of Sle-Associated Copy-Number Variation at the FCGR2C/FCGR3B/FCGR2B Locus. Am J Hum Genet (2013) 92:28–40. doi: 10.1016/j.ajhg.2012.11.013
32. Niederer HA, Willcocks LC, Rayner TF, Yang W, Lau YL, Williams TN, et al. Copy Number, Linkage Disequilibrium and Disease Association in the FCGR Locus. Hum Mol Genet (2010) 19:3282–94. doi: 10.1093/hmg/ddq216
33. Nagelkerke SQ, Tacke CE, Breunis WB, Geissler J, Sins JW, Appelhof B, et al. Nonallelic Homologous Recombination of the FCGR2/3 Locus Results in Copy Number Variation and Novel Chimeric FCGR2 Genes With Aberrant Functional Expression. Genes Immun (2015) 16:422–9. doi: 10.1038/gene.2015.25
34. Nagelkerke SQ, Schmidt DE, De Haas M, Kuijpers TW. Genetic Variation in Low-To-Medium-Affinity Fcgamma Receptors: Functional Consequences, Disease Associations, and Opportunities for Personalized Medicine. Front Immunol (2019) 10:2237. doi: 10.3389/fimmu.2019.02237
35. Bruhns P, Iannascoli B, England P, Mancardi DA, Fernandez N, Jorieux S, et al. Specificity and Affinity of Human Fcγ Receptors and Their Polymorphic Variants for Human IgG Subclasses. Blood (2009) 113:3716–25. doi: 10.1182/blood-2008-09-179754
36. Sanders LA, Feldman RG, Voorhorst-Ogink MM, De Haas M, Rijkers GT, Capel PJ, et al. Human Immunoglobulin G (IgG) Fc Receptor Iia (Cd32) Polymorphism and IgG2-Mediated Bacterial Phagocytosis by Neutrophils. Infection Immun (1995) 63:73–81. doi: 10.1128/iai.63.1.73-81.1995
37. Warmerdam PA, Van De Winkel JG, Vlug A, Westerdaal NA, Capel PJ. A Single Amino Acid in the Second Ig-Like Domain of the Human Fc Gamma Receptor II Is Critical for Human IgG2 Binding. J Immunol (1991) 147:1338–43. https://www.jimmunol.org/content/147/4/1338.long
38. Wu J, Edberg JC, Redecha PB, Bansal V, Guyre PM, Coleman K, et al. A Novel Polymorphism of Fcgammariiia (Cd16) Alters Receptor Function and Predisposes to Autoimmune Disease. J Clin Invest (1997) 100:1059–70. doi: 10.1172/JCI119616
39. Floto RA, Clatworthy MR, Heilbronn KR, Rosner DR, Macary PA, Rankin A, et al. Loss of Function of a Lupus-Associated Fcγriib Polymorphism Through Exclusion From Lipid Rafts. Nat Med (2005) 11:1056–8. doi: 10.1038/nm1288
40. Salmon JE, Edberg JC, Kimberly RP. Fc Gamma Receptor III on Human Neutrophils. Allelic Variants Have Functionally Distinct Capacities. J Clin Invest (1990) 85:1287–95. doi: 10.1172/JCI114566
41. Li X, Wu J, Ptacek T, Redden David T, Brown Elizabeth E, Alarcón Graciela S, et al. Allelic-Dependent Expression of an Activating Fc Receptor on B Cells Enhances Humoral Immune Responses. Sci Transl Med (2013) 5:216ra175–216ra175. doi: 10.1126/scitranslmed.3007097
42. Su K, Wu J, Edberg JC, Li X, Ferguson P, Cooper GS, et al. A Promoter Haplotype of the Immunoreceptor Tyrosine-Based Inhibitory Motif-Bearing Fcγriib Alters Receptor Expression and Associates With Autoimmunity. I. Regulatory FCGR2B Polymorphisms and Their Association With Systemic Lupus Erythematosus. J Immunol (2004) 172:7186–91. doi: 10.4049/jimmunol.172.11.7186
43. Su K, Li X, Edberg JC, Wu J, Ferguson P, Kimberly RP. A Promoter Haplotype of the Immunoreceptor Tyrosine-Based Inhibitory Motif-Bearing Fcγriib Alters Receptor Expression and Associates With Autoimmunity. Ii. Differential Binding of Gata4 and Yin-Yang1 Transcription Factors and Correlated Receptor Expression and Function. J Immunol (2004) 172:7192–9. doi: 10.4049/jimmunol.172.11.7192
44. Lassaunière R, Shalekoff S, Tiemessen CT. A Novel FCGR3A Intragenic Haplotype Is Associated With Increased Fcγriiia/CD16a Cell Surface Density and Population Differences. Hum Immunol (2013) 74:627–34. doi: 10.1016/j.humimm.2013.01.020
45. Van Der Pol WL, Jansen MD, Sluiter WJ, Van De Sluis B, Leppers-Van De Straat FG, Kobayashi T, et al. Evidence for Non-Random Distribution of Fcgamma Receptor Genotype Combinations. Immunogenetics (2003) 55:240–6. doi: 10.1007/s00251-003-0574-9
46. Lejeune J, Piegu B, Gouilleux-Gruart V, Ohresser M, Watier H, Thibault G. FCGR2c Genotyping by Pyrosequencing Reveals Linkage Disequilibrium With FCGR3A V158F and FCGR2A H131R Polymorphisms in a Caucasian Population. MAbs (2012) 4:784–7. doi: 10.4161/mabs.22287
47. Den Dunnen JT, Antonarakis SE. Mutation Nomenclature Extensions and Suggestions to Describe Complex Mutations: A Discussion. Hum Mutat (2000) 15:7–12. doi: 10.1002/(SICI)1098-1004(200001)15:1<7::AID-HUMU4>3.0.CO;2-N
48. Forthal DN, Gilbert PB, Landucci G, Phan T. Recombinant Gp120 Vaccine-Induced Antibodies Inhibit Clinical Strains of HIV-1 in the Presence of Fc Receptor-Bearing Effector Cells and Correlate Inversely With HIV Infection Rate. J Immunol (2007) 178:6596–603. doi: 10.4049/jimmunol.178.10.6596
49. Lassauniere R, Paximadis M, Ebrahim O, Chaisson RE, Martinson NA, Tiemessen CT. The FCGR2C Allele That Modulated the Risk of HIV-1 Infection in the Thai Rv144 Vaccine Trial Is Implicated in HIV-1 Disease Progression. Genes Immun (2019) 20:651–9. doi: 10.1038/s41435-018-0053-9
50. Peng X, Li SS, Gilbert PB, Geraghty DE, Katze MG. FCGR2C Polymorphisms Associated With HIV-1 Vaccine Protection Are Linked to Altered Gene Expression of Fc-Gamma Receptors in Human B Cells. PloS One (2016) 11:e0152425. https://doi.org/10.1371/journal.pone.0152425
51. Churchyard GJ, Morgan C, Adams E, Hural J, Graham BS, Moodie Z, et al. A Phase IIa Randomized Clinical Trial of a Multiclade HIV-1 DNA Prime Followed by a Multiclade Rad5 HIV-1 Vaccine Boost in Healthy Adults (Hvtn204). PloS One (2011) 6:e21225. doi: 10.1371/journal.pone.0021225
52. Chung AW, Kumar MP, Arnold KB, Yu WH, Schoen MK, Dunphy LJ, et al. Dissecting Polyclonal Vaccine-Induced Humoral Immunity Against HIV Using Systems Serology. Cell (2015) 163:988–98. doi: 10.1016/j.cell.2015.10.027
53. Brouwer KC, Lal RB, Mirel LB, Yang C, Van Eijk AM, Ayisi J, et al. Polymorphism of Fc Receptor IIa for IgG in Infants Is Associated With Susceptibility to Perinatal HIV-1 Infection. AIDS (2004) 18:1187–94. doi: 10.1097/00002030-200405210-00012
54. Milligan C, Overbaugh J. The Role of Cell-Associated Virus in Mother-To-Child HIV Transmission. J Infect Dis (2014) 210(Suppl 3):S631–640. doi: 10.1093/infdis/jiu344
55. Lassauniere R, Musekiwa A, Gray GE, Kuhn L, Tiemessen CT. Perinatal HIV-1 Transmission: Fc Gamma Receptor Variability Associates With Maternal Infectiousness and Infant Susceptibility. Retrovirology (2016) 13:40. doi: 10.1186/s12977-016-0272-y
56. Milligan C, Richardson BA, John-Stewart G, Nduati R, Overbaugh J. FCGR2A and FCGR3A Genotypes in Human Immunodeficiency Virus Mother-To-Child Transmission. Open Forum Infect Dis (2015) 2:ofv149. doi: 10.1093/ofid/ofv149
57. Ebonwu J, Lassaunière R, Paximadis M, Goosen M, Strehlau R, Gray GE, et al. An HIV Vaccine Protective Allele in FCGR2C Associates With Increased Odds of Perinatal HIV Acquisition. Front Immunol (2021) 12:760571. doi: 10.3389/fimmu.2021.760571
58. Forthal DN, Landucci G, Bream J, Jacobson LP, Phan TB, Montoya B. FcgammaRIIa Genotype Predicts Progression of HIV Infection. J Immunol (2007) 179:7916–23. doi: 10.4049/jimmunol.179.11.7916
59. Weis JF, Mcclelland RS, Jaoko W, Mandaliya KN, Overbaugh J, Graham SM. Short Communication: Fc Gamma Receptors IIa and IIIa Genetic Polymorphisms Do Not Predict HIV-1 Disease Progression in Kenyan Women. AIDS Res Hum Retroviruses (2015) 31:288–92. doi: 10.1089/aid.2014.0209
60. Poonia B, Kijak GH, Pauza CD. High Affinity Allele for the Gene of FCGR3a Is Risk Factor for HIV Infection and Progression. PloS One (2010) 5:e15562. doi: 10.1371/journal.pone.0015562
61. Deepe RN, Kistner-Griffin E, Martin JN, Deeks SG, Pandey JP. Epistatic Interactions Between Fc (GM) and Fcγr Genes and the Host Control of Human Immunodeficiency Virus Replication. Hum Immunol (2012) 73:263–6. doi: 10.1016/j.humimm.2011.12.008
62. Lewis GK. Role of Fc-Mediated Antibody Function in Protective Immunity Against HIV-1. Immunology (2014) 142:46–57. doi: 10.1111/imm.12232
63. Carapito R, Mayr L, Molitor A, Verniquet M, Schmidt S, Tahar O, et al. A FcɣRIIa Polymorphism Has a HLA-B57 and HLA-B27 Independent Effect on HIV Disease Outcome. Genes Immun (2020) 21:263–8. doi: 10.1038/s41435-020-0106-8
64. Su B, Dispinseri S, Iannone V, Zhang T, Wu H, Carapito R, et al. Update on Fc-Mediated Antibody Functions Against HIV-1 Beyond Neutralization. Front Immunol (2019) 10.2968 doi: 10.3389/fimmu.2019.02968
65. Roederer M, Quaye L, Mangino M, Beddall Margaret h, Mahnke Y, Chattopadhyay P, et al. The Genetic Architecture of the Human Immune System: A Bioresource for Autoimmunity and Disease Pathogenesis. Cell (2015) 161:387–403. doi: 10.1016/j.cell.2015.02.046
66. Van Der Heijden J, Nagelkerke S, Zhao X, Geissler J, Rispens T, Van Den Berg TK, et al. Haplotypes of Fcγriia and Fcγriiib Polymorphic Variants Influence IgG-Mediated Responses in Neutrophils. J Immunol (2014) 192:2715–21. doi: 10.4049/jimmunol.1203570
67. Phaahla NG, Lassauniere R, Da Costa Dias B, Waja Z, Martinson NA, Tiemessen CT. Chronic HIV-1 Infection Alters the Cellular Distribution of FcgammaRIIIa and the Functional Consequence of the FcgammaRIIIa-F158V Variant. Front Immunol (2019) 10:735. doi: 10.3389/fimmu.2019.00735
68. Corey L, Gilbert PB, Juraska M, Montefiori DC, Morris L, Karuna ST, et al. Two Randomized Trials of Neutralizing Antibodies to Prevent HIV-1 Acquisition. N Engl J Med (2021) 384:1003–14. doi: 10.1056/NEJMoa2031738
69. Teo A, Feng G, Brown GV, Beeson JG, Rogerson SJ. Functional Antibodies and Protection Against Blood-Stage Malaria. Trends Parasitol (2016) 32:887–98. doi: 10.1016/j.pt.2016.07.003
70. Boudreau CM, Alter G. Extra-Neutralizing FcR-Mediated Antibody Functions for a Universal Influenza Vaccine. Front Immunol (2019) 10:440. doi: 10.3389/fimmu.2019.00440
71. Vanderven HA, Kent SJ. The Protective Potential of Fc-Mediated Antibody Functions Against Influenza Virus and Other Viral Pathogens. Immunol Cell Biol (2020) 98:253–63. doi: 10.1111/imcb.12312
72. Van Erp EA, Luytjes W, Ferwerda G, Van Kasteren PB. Fc-Mediated Antibody Effector Functions During Respiratory Syncytial Virus Infection and Disease. Front Immunol (2019) 10:548. doi: 10.3389/fimmu.2019.00548
73. Natarajan H, Crowley AR, Butler SE, Xu S, Weiner JA, Bloch EM, et al. Markers of Polyfunctional SARS-CoV-2 Antibodies in Convalescent Plasma. mBio (2021) 12:e00765–00721. doi: 10.1128/mBio.00765-21
74. Liu Q, Fan C, Li Q, Zhou S, Huang W, Wang L, et al. Antibody-Dependent-Cellular-Cytotoxicity-Inducing Antibodies Significantly Affect the Post-Exposure Treatment of Ebola Virus Infection. Sci Rep (2017) 7:45552. doi: 10.1038/srep45552
Keywords: FCGR genes, Fc gamma receptor (FcγR), variant, polymorphism, copy number, HIV - human immunodeficiency virus, vaccine, disease progression
Citation: Lassaunière R and Tiemessen CT (2021) FcγR Genetic Variation and HIV-1 Vaccine Efficacy: Context And Considerations. Front. Immunol. 12:788203. doi: 10.3389/fimmu.2021.788203
Received: 01 October 2021; Accepted: 29 November 2021;
Published: 15 December 2021.
Edited by:
Gabriella Scarlatti, San Raffaele Hospital (IRCCS), ItalyReviewed by:
Bin Su, Capital Medical University, ChinaChristiane Moog, Institut National de la Santé et de la Recherche Médicale (INSERM), France
Copyright © 2021 Lassaunière and Tiemessen. This is an open-access article distributed under the terms of the Creative Commons Attribution License (CC BY). The use, distribution or reproduction in other forums is permitted, provided the original author(s) and the copyright owner(s) are credited and that the original publication in this journal is cited, in accordance with accepted academic practice. No use, distribution or reproduction is permitted which does not comply with these terms.
*Correspondence: Caroline T. Tiemessen, carolinet@nicd.ac.za; Ria Lassaunière, mlas@ssi.dk