- 1Department of Cardiology, Pulmonology, and Nephrology, Faculty of Medicine, Yamagata University, Yamagata, Japan
- 2Department of Pediatric Hematology/Oncology, University Michigan Medical School, Ann Arbor, MI, United States
- 3Department of Internal Medicine III, Division of Hematology and Cell Therapy, Faculty of Medicine, Yamagata University, Yamagata, Japan
Acute kidney injury (AKI) is a common complication of allogeneic hematopoietic cell transplantation (allo-HCT) and is associated with non-relapse mortality (NRM) and quality of life (QOL). Multiple factors may contribute to AKI during allo-HCT and are often present at the same time making it difficult to determine the cause of AKI in each patient. Nephrotoxic drugs, infections, thrombotic microangiopathy (TMA), and sinusoidal obstruction syndrome (SOS) are well described causes of AKI during allo-HCT. Acute graft-versus-host disease (aGVHD) is a major complication of allo-HCT that mainly targets the intestines, liver, and skin. However, recent studies suggest aGVHD may also attack the kidney and contribute to AKI following allo-HCT. For example, severe aGVHD is associated with AKI, suggesting a link between the two. In addition, animal models have shown donor immune cell infiltration and increased expression of inflammatory cytokines in recipient kidneys after allo-HCT. Therefore, aGVHD may also target the kidney and contribute to AKI following allo-HCT. Herein, we describe the etiology, diagnosis, risk factors, pathophysiology, prevention, and treatment of renal injury after allo-HCT. In addition, we highlight emerging evidence that aGVHD may contribute to the development of AKI after allo-HCT.
Introduction
Hematopoietic cell transplantation (HCT) is a curative therapy for hematologic malignancies and many non-malignant diseases (1). HCT is classified as either autologous (auto-HCT), when recipient hematopoietic stem cells are stored and then infused, or allogeneic (allo-HCT), when the infused hematopoietic stem cells are derived from a related or unrelated donor. Prior to transplantation, conditioning with chemotherapy and/or total body irradiation (TBI) is necessary to eradicate malignant residual tumors and inhibit rejection of donor hematopoietic cells. Myeloablative conditioning with high-dose cyclophosphamide (CY) and TBI or a combination of busulfan (BU) and CY are two common regimens. Non-myeloablative conditioning or reduced-intensity conditioning with less intense pretreatment is commonly utilized for elderly patients or those with comorbidities (2).
To prevent graft-versus-host disease (GVHD), immunosuppressive prophylaxis is necessary after transplantation (3). GVHD is caused by alloreactive donor T cells, attacking recipient tissues and is a major life-threatening complication of allo-HCT (4). Previously GVHD was classified into acute GVHD (aGVHD) if it developed within 100 days after transplantation or chronic GVHD (cGVHD) if it developed after 100 days. However, GVHD classification is now based on clinical and pathological characteristics (5).
The characteristic symptoms of aGVHD are rash, diarrhea, and jaundice (4). The pathophysiology causing these symptoms begins with tissue damage from conditioning regimens that results in the release of damage-associated molecular patterns (DAMPs). Injury to the intestinal mucosa and skin also causes a breakdown in barrier function. Barrier breakdown allows microbes to invade the body and release pathogen-associated molecular patterns (PAMPs). PAMPs and DAMPs are danger signals that activate the innate immune system to produce proinflammatory cytokines, such as tumor necrosis factor (TNF)-α and interleukin (IL)-1β, which amplify tissue damage and activate antigen-presenting cells (APCs). Activated host APCs then stimulate donor T cells, which in turn produce proinflammatory cytokines, such as interferon (IFN)-γ, that further activate the innate immune system. Finally, tissue damage caused by cytotoxic T lymphocytes and cytotoxic cytokines derived from activated T cells and innate immune cells, results in the development of clinically apparent aGVHD (3, 6).
The most common immunosuppressive regimen used to prevent GVHD after allo-HCT consists of a calcineurin inhibitor (tacrolimus or cyclosporine) in combination with a short-term course of methotrexate (MTX) (4). Systemic high-dose corticosteroids are the first-line treatment for patients who develop aGVHD (7).
The main organs affected by aGVHD are the skin, liver, and gastrointestinal (GI) tract, but a variety of other organs may also be affected (8). Classically, the kidney is not recognized as a main target organ of aGVHD and no renal aGVHD diagnostic criteria have been established (8). However, various factors related to conditioning and GVHD prophylaxis are known to cause renal injury after allo-HCT. In patients with aGVHD and renal dysfunction, it is often difficult to identify the cause of renal dysfunction due to the frequent co-occurrence of multiple possible etiologies. Renal biopsy is the gold-standard examination for deconvoluting multiple possible etiologies of renal injury; however, invasive renal biopsies are rarely safe during the acute phase of GVHD. Recently, animal studies suggest that the kidney may be a target of aGVHD. Here, we describe the pathophysiology and management of acute kidney injury (AKI) after allo-HCT and highlight the emerging association between AKI and aGVHD.
Criteria for Acute Kidney Injury
Although AKI is a common disease, there have been no internationally standardized criteria (9). In 2004, the Acute Dialysis Quality Initiative (ADQI) published the Risk, Injury, Failure, Loss, and End-stage renal disease (RIFLE) criteria (10). The ADQI defined acute renal failure (ARF) as elevated serum creatinine (sCr), decreased glomerular filtration rate (GFR), and decreased urine output. The AQDI also classified the severity of ARF based on the degree to which these parameters were altered (10). Later, the Acute Kidney Injury Network (AKIN) proposed the concept of acute kidney injury (AKI) in order to include early renal injury. The AKIN criteria, published in 2007, modified the RIEFLE criteria by including mild elevations of sCr (11). In 2012, the Kidney Disease Improving Global Outcomes (KDIGO) criteria were proposed, which integrated the RIEFLE and AKIN criteria. The KDIGO criteria for AKI include anyone of the following: 1) an increase in sCr by≧0.3 mg/dl within 48 hours, 2) an increase in sCr to ≧1.5 times baseline within the preceding 7 days, and 3) a urine volume <0.5 ml/kg/h for 6 hours. The severity of AKI is classified by the KDIGO as Stage 1 to 3 based on sCr or urine output (12). The details of each criterion are shown in Table 1. Importantly, the latest KDIGO criteria are as or more predictive of life expectancy than either the RIEFLE or AKIN criteria (13–15). The KDIGO criteria are frequently used in recent studies to measure the incidence of AKI after HCT (16–19).
Kidney Disease After HCT
According to a recently published meta-analysis of reports from 1995-2019, the incidence of AKI after HCT was 55.1%, with Stage 3, the most severe form, occurring in 8.3% of patients (20).
Factors known to contribute to the risk of AKI after HCT include pre-treatment factors such as being female (21), age 55 years or older (22), and underlying conditions such as diabetes (23), hypertension (21), and chronic kidney disease (CKD) (24). Risk factors for AKI associated with HCT include TBI conditioning (22), use of a calcineurin inhibitors (CNIs) for GVHD prevention (23, 25, 26), and use of MTX for GVHD prophylaxis (22, 23). Post-transplant stay in an intensive care unit (21) and the need for mechanical ventilation (27) are also risk factors. Several post-HCT complications also increase the risk for AKI including hepatic sinusoidal obstruction syndrome (SOS) (28), sepsis (28, 29), and cytomegalovirus infection (22). AKI risk can be further increased by agents used to treat post-HCT complications including amphotericin B (30), acyclovir (31), aminoglycosides (32), and the concomitant use of multiple nephrotoxic drugs (33).
AKI is more common in the early phase of HCT due to the risk of conditioning toxicity, sepsis, SOS, and drug-induced renal injury that are more common early post-HCT (23, 34). For these reasons, clinical studies typically assess post-HCT AKI at 100 days post-transplantation (35).
The incidence of AKI varies according to the type of HCT. In auto -HCT recipients, graft failure is less common, and CNIs are not required because there is no risk for GVHD. Less antibiotics are administrated to auto-HCT recipients than to allo-HCT recipients because duration of neutrophilia is shorter. Therefore, the incidence of AKI is less in auto-HCT versus allo-HCT recipients (36–39). AKI incidence is lower following nonmyeloablative compared to myeloablative conditioning due to decreased rates of infection, SOS, and organ failure (35, 40). Overall, the greatest risk of AKI is with myeloablative allo-HCT (21-73%), followed by nonmyeloablative allo-HCT (29-56%), and then autologous transplantation (10.4-19%) (36, 41, 42). Reports vary on whether the incidence of AKI is higher with cord blood or HLA mismatched donor transplantation (28, 43–47).
Whether the indication for HCT is for a malignant or nonmalignant disorders does not significantly affect the incidence of AKI (33, 48, 49). However, malignancies are a risk factor for post-transplant CKD (50), and these patients should be monitored carefully for changes in renal function. Multiple myeloma (MM) and immunoglobulin light-chain (AL) amyloidosis, which themselves cause AKI (see the section of “Etiologies of AKI after HCT”), have been reported to cause relatively little post-transplant AKI. However, patients with these disorders are typically treated with auto-HCT, which is less nephrotoxic than allo-HCT (19, 24).
AKI after allo-HCT is associated with all-cause (19, 20, 22, 28, 33, 39, 51) and non-relapse mortality (17, 23, 33), and the earlier the onset of AKI, the higher the mortality (52). The severity of AKI decreases overall survival, and mortality worsens to 55-100% with renal failure requiring dialysis (17, 22, 36, 37, 53, 54). AKI after allo-HCT is also a risk factor for CKD (33, 50). Representative studies that focus on the association of AKI with transplant outcomes are shown in Table 2. In pediatric HCT recipient, the incidence of AKI is similar to adults (44, 48, 49, 56–58), AKI worsens mortality after HCT (49, 57), and the 1-year survival rate is less than 10% in patients with renal failure requiring renal replacement therapy (56). Fortunately, HCT-related AKI has decreased in recent years due to the increased use of less toxic conditioning regimens, decreased rates of SOS, modified infection prophylaxis, less amphotericin B use, and declining rates of severe aGVHD (59, 60).
Etiologies of AKI After HCT
There are various causes of AKI after HCT. An overview is shown in Figure 1.
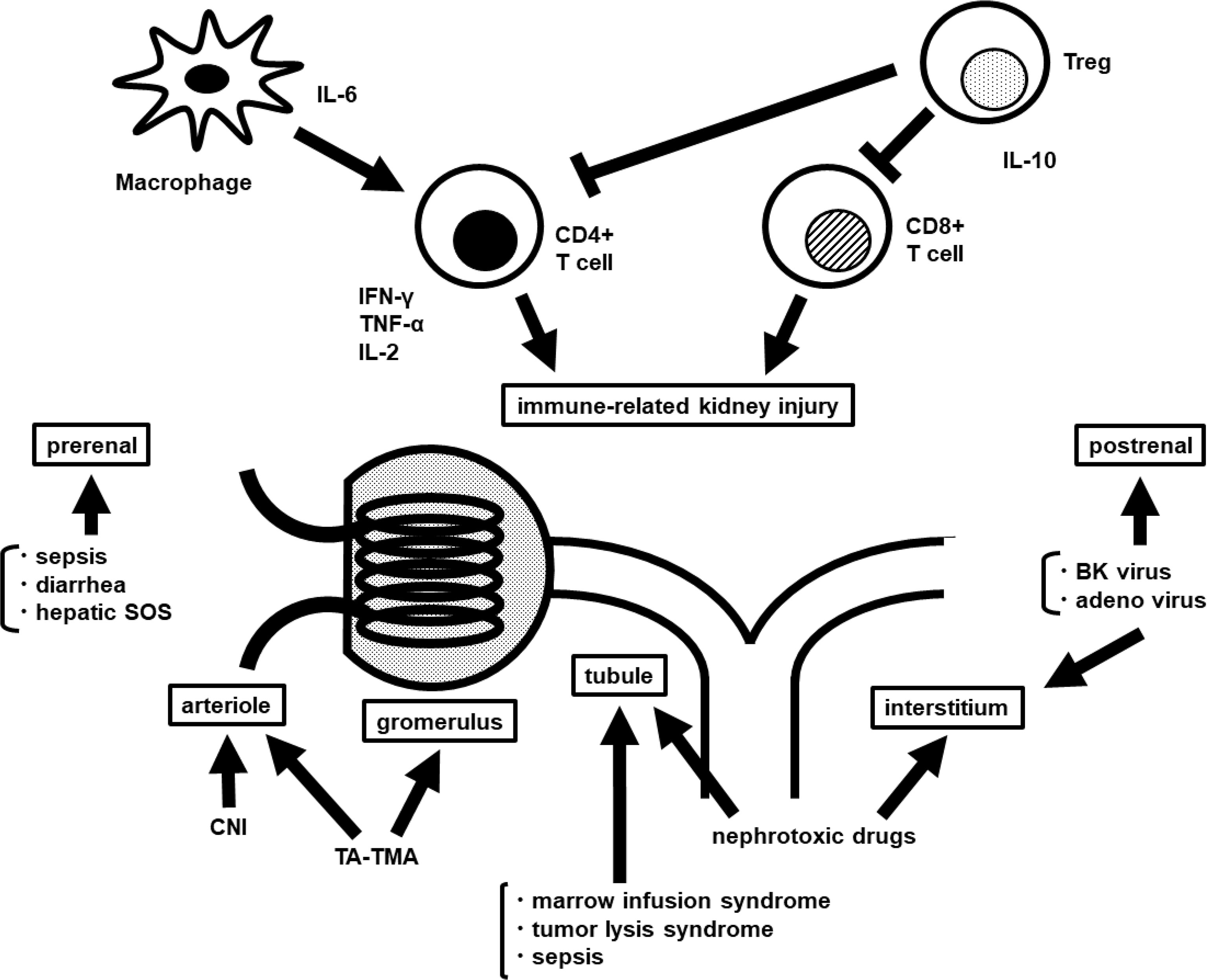
Figure 1 Overview of the pathophysiology of AKI after HCT. AKI, acute kidney injury; HCT, hematopoietic cell transplantation; IL, interleukin; IFN, interferon; TNF, tumor necrosis factor; Treg, regulatory T cell; SOS, sinusoidal obstruction syndrome; CNI, calcineurin inhibitor; TA-TMA, transplantation associated-thrombotic microangiopathy.
Nephrotoxic Drugs
Most of the renal injury after HCT is thought to be caused by nephrotoxic drugs, particularly CNIs given for GVHD prophylaxis (34). CNIs can cause both AKI and CKD (61); however, CNIs serum concentration does not always correlate with the severity of AKI (26). CNIs cause AKI through a variety of mechanisms. One way is by inhibiting the production of vasodilators and increasing the production of vasoconstrictors, resulting in the contraction of afferent and efferent arterioles. They also cause vacuolation and dysfunction of renal tubules (61), and they increase the levels of oxidative stress that damages the renal endothelium and contributes to the development of thrombotic microangiopathy (TMA) (62). Consistent with the vasoconstrictive effect of CNIs on afferent and efferent arterioles, inhibition of the renin-angiotensin-aldosterone system may be useful for preventing CNI nephrotoxicity (60).
MTX can also be nephrotoxic. The mechanism is thought to relate to direct tubular injury and/or its precipitation in the renal tubules. The risk for MTX-induced nephrotoxicity is increased by high dose intravenous administration, dehydration, and aciduria (63).
Chemotherapeutic agents used in conditioning (cytarabine and fludarabine) can be nephrotoxic and primarily cause acute tubular injury. Vomiting and diarrhea, which are common adverse events of chemotherapy, cause pre-renal AKI due to dehydration (35). CY and BU cause post-renal AKI due to hemorrhagic cystitis (35, 64).
Many antimicrobial agents may induce direct renal injury or acute interstitial nephritis due to allergic reactions leading to AKI (60). For example, aminoglycosides can cause Fanconi syndrome and Bartter-like syndrome (32). The antifungal agent, amphotericin B causes AKI in a dose-dependent manner via renal vasoconstriction and direct tubular injury (65). Fortunately, liposomal amphotericin B, which is now more commonly used, is far less nephrotoxic (66). Finally, acyclovir, an antiviral agent, has been associated with crystal-induced tubular injury and obstruction (60, 67).
Hematological Disease-Associated AKI
Hematologic diseases themselves can cause renal injury. For example, AKI occurs in 20-50% of patients with multiple myeloma (68). The most common cause of AKI in patients with multiple myeloma is cast nephropathy, in which large amounts of light chains bind to Tamm-Horsfall protein in the tubules and form insoluble casts, resulting in tubular obstruction and injury. Other causes include light chain deposition disease, AL amyloidosis, and hypercalcemia (69). Direct invasion of the kidney by lymphoma and leukemia can also cause AKI (70).
Complications of HCT
Recipients are immunocompromised and prone to sepsis after HCT (60). Gram-negative bacteria is more common in cord blood transplantation than in bone marrow transplantation (71), likely due to a longer period of neutropenia. Sepsis causes systemic vasodilatation, hypotension, and cytokine-induced endothelial damage, leading to AKI (72). As mentioned above, some of the antimicrobial agents used in the treatment of sepsis are nephrotoxic.
Adenovirus and BK virus infections are also common opportunistic infections following allo-HCT and often lead to AKI (35, 60). Adenoviruses may cause hemorrhagic cystitis and, rarely, necrotizing tubulointerstitial nephritis (73). Adenovirus infections are more common in transplants from unrelated donors and in pediatric patients. Severe adenovirus infection can cause hepatitis, pneumonitis, and encephalitis, and multi-organ failure (74). Reactivation of BK virus may lead to hemorrhagic cystitis, ureteral stricture, and tubulointerstitial nephritis (75). Acute GVHD, allo-HCT, and BK viremia are still associated with increased risk for hemorrhagic cystitis (76, 77).
Nephrotic syndrome, while rare (0.4-6.0%), may also develop following allo-HCT (34). Membranous glomerulonephritis (MGN) and minimal change disease (MCD) account for about two-thirds and one-quarter of nephrotic syndrome cases following allo-HCT, respectively (78). Intriguingly, the onset of nephrotic syndrome following allo-HCT has been associated with recent reduction in the dose of immunosuppressive drugs (78) and the onset of GVHD (79). Most cases occur more than 6 months after transplantation and are considered a rare manifestation of cGVHD (34, 60). However, de novo nephrotic syndrome without GVHD also occurs (80).
Marrow infusion syndrome is caused by hemolysis of erythrocytes that release hemoproteins into the recipient. These hemoproteins cause symptoms of hemolysis, such as fever and vomiting. They can also cause acute tubular injury by forming casts in the tubules. Hemolysis resulting in marrow infusion syndrome often occurs during the preservation of stem cells or upon infusion of grafts containing the cryoprotectant dimethyl sulfoxide (DMSO), which can cause hemolysis in recipients of DMSO-containing grafts. Marrow infusion syndrome is mitigated and treated by intravenous hydration and by rinsing or red blood cell-depleting the graft (81).
Tumor lysis syndrome (TLS) occurs when a large number of tumor cells lyse and release toxic cellular contents. It is characterized by hyperkalemia, hypocalcemia, hyperphosphatemia, hyperuricemia, and crystal-induced kidney injury (82). While more common during induction chemotherapy for leukemia, TLS is relatively rare following HCT because most patients come to transplant following multiple treatment courses that dramatically reduce tumor burden (82).
Hepatic sinusoidal obstruction syndrome (SOS) is characterized by painful hepatomegaly, jaundice, and weight gain due to fluid retention (83). AKI often co-occurs with SOS (84, 85), which is more frequently seen after allo- than auto-HCT (81), and severe SOS may lead to multiple organ failure (86). SOS develops following sinusoidal endothelial damage from conditioning therapy, resulting in hepatic portal hypertension, ascites, and increased abdominal pressure. While the exact cause of renal injury in SOS is uncertain and likely multifactorial, decreased renal blood flow due to elevated abdominal pressure likely contributes to tubular injury, which further exacerbates fluid retention and multiorgan failure (86, 87).
Thrombotic Microangiopathy
Transplantation-associated-thrombotic microangiopathy (TA-TMA) is another complication of HCT associated with a substantial risk of mortality (88). It typically develops subacute or chronically (89), and can also lead to AKI (60). Vascular endothelial damage associated with transplantation results in thrombus formation and fibrin deposition in the capillaries and small arteries, microangiopathic hemolytic anemia, and consumptive thrombocytopenia (88). TBI, high-dose BU, CNIs, aGVHD Grade II-IV, infections (BK virus, cytomegalovirus, parvovirus B19, aspergillus species, adenovirus), peripheral blood stem cell transplantation, and use of unrelated donors are all risk factors for TA-TMA (62).
Diagnostic criteria for TA-TMA have been developed by the Blood and Marrow Transplant Clinical Trial Network (BMT-CTN) (90) and the European Group for Blood and Marrow Transplantation (EBMT) (91). Both sets of criteria require the presence of schistocytes and elevated lactate dehydrogenase (LDH). The BMT-CTN criteria also requires worsening renal function (90).
The Kidney is the most vulnerable organ to TA-TMA (62, 89). Renal TA-TMA presents as both AKI and CKD (62, 89) and is often accompanied by hypertension, proteinuria, and a decreased GFR (89). The histopathology of renal TA-TMA is characterized by fibrin deposition in the glomeruli, narrowing of the capillary lumen, presence of fragmented red blood cells, basement membrane duplication, mesangiolysis, and edema of the endothelium (89, 92).
Although endothelial damage plays a major role in the pathogenesis of TA-TMA (62), it is unclear whether it is a direct complication of transplantation or a manifestation of GVHD, infection, or drug toxicity (60). Factors known to cause endothelial damage include CNIs, mammalian target of rapamycin (mTOR) inhibitors, chemotherapy, and TBI (35). Recent studies have also suggested the involvement of complement activation (62, 88).
Several clinical studies have shown an association between TA-TMA and aGVHD (93, 94), but these were retrospective studies confounded by the use of CNIs (88). Nevertheless, clinical studies have suggested that vascular endothelial cells are targeted by donor T cells (95), and some studies suggest that TA-TMA may be caused by GVHD of the vascular endothelium (89, 96, 97).
Kidney Disease Associated With GVHD
Renal injury after allo-HCT is generally attributed to the etiologies describes above. However, aGVHD is a risk factor for AKI (23, 28, 53, 54, 98), and recent studies have suggested that the kidney may be a direct target of aGVHD (34). Traditionally, the kidney was not considered a target of aGVHD (35). However, diarrhea associated with severe GVHD can indirectly cause dehydration leading to AKI, and CNIs used for GVHD prophylaxis can also cause AKI. Hence, the association between kidney injury and aGVHD is controversial. In the following section, we review studies investigating the relationship between aGVHD and AKI.
Clinical Studies
Hingorani et al. (99) measured cytokines in the urine of patients who underwent allogeneic or autologous transplantation. Increased urine IL-6 and IL-15 levels after HCT were associated with an increased risk of developing proteinuria, and an increased urine MCP-1 level after HCT was associated with chronic kidney disease at 1 year. Thus, these data suggested kidney inflammation occurs after HCT.
Inflammatory cytokines are involved in the pathogenesis of GVHD, but they are not unique to GVHD and are elevated by other HCT-related complications and inflammatory disorders (100). In studies exploring GVHD-specific biomarkers, elafin was identified as a biomarker for cutaneous GVHD (101). Elafin is an elastase-specific protease inhibitor expressed mainly in epithelial tissues, is secreted in response to IL-1 and TNF-α, and has functions such as antibacterial activity, inflammatory cell recruitment, and dendritic cell activation (102, 103). In a study that measured urine elafin levels in patients after HCT (98), it was found that patients with AKI had higher urine elafin levels than those without AKI, and patients with albuminuria also had higher urine elafin levels than those without albuminuria. In addition, elafin was associated with increased risk of CKD and death. These data suggest that inflammation similar to cutaneous aGVHD may occur in the kidney.
Histological diagnosis of renal dysfunction shortly after HCT is rare, and pathological diagnostic criteria have not been established (35). Nonetheless, several renal histopathology studies using tissue obtained by biopsy or autopsy have been reported. For example, Girsberger et al. (104) reported that renal biopsy pathology was consistent with TA-TMA in 29%, CNI toxicity in 24%, and membranous glomerulonephritis in 18% of patients who presented with deterioration of kidney function or proteinuria after HCT (12 allo-HCT, 5 auto-HCT). In 137 autopsies (114 allo-HCT, 23 autologous HCT), the most common renal pathology was acute tubular damage (40%), followed by chronic vascular and interstitial change (11%), and TMA (10%). A small number of cases of membranous glomerulonephritis (1%) and acute interstitial nephritis (1%) were also observed. The median time from transplantation was 497 days for biopsies and 91 days for autopsies; therefore, cGVHD may have a greater association with kidney injury than aGVHD. Mii et al. (97) studied renal biopsy (two cases) and autopsy (5 cases) tissue from patients who developed renal TA-TMA. The median interval between HCT and renal biopsy or autopsy was 7 months. Five of the 7 patients underwent allo-HCT, all 7 patients underwent conditioning that included TBI, and all but one patient received a CNI for GVHD prophylaxis. In addition to TA-TMA changes, all patients had glomerulitis, tubulitis, and peritubular capillaritis with T cell infiltration. Based on these results, the authors concluded that the kidney is a potential target for GVHD.
Studies With Animal Models
Animal models have been important tools for studying the pathophysiology of HCT complications, most notably GVHD, and for developing new therapies to treat these complications (105). In addition to the above-described clinical studies, kidney injury associated with aGVHD has also been studied in animal models. These models revealed important insights into the relationship between kidney injury post allo-HCT and GVHD.
Two studies that measured renal function in mice after allo-HCT reported that sCr did not increase (106, 107). Because creatinine is a waste product of muscle metabolism (108), the lack of sCr elevation may have been due to loss of muscle mass after allo-HCT. By contrast, blood urea nitrogen (BUN), another marker of renal function, was elevated in a rat model (107).
In addition to markers of renal function, elevated markers of renal injury have also been observed in mouse models. These markers include urine protein, albumin (106), N-acetyl-beta-D-glucosaminidase (NAG) (106, 107), and neutrophil gelatinase-associated lipocalin (NGAL) (109), which mainly reflects tubular injury (110, 111). The expression of αKlotho, which is down-regulated in AKI and CKD (112), was also decreased in allo-HCT mice (109).
Higo et al. (107) evaluated renal lesions in a rat bone marrow transplantation (BMT) model. The kidneys in the allo-HCT group were infiltrated with donor leukocytes. Areas with mild inflammation were characterized by CD4+ T cell, CD8+ T cell, and CD68+ macrophage infiltration of the interstitium around small arteries. Whereas in lesions with moderate to severe inflammation, the cellular infiltrate extended into the interstitium surrounding the tubules. Peritubular capillaritis, tubulitis, acute glomerulitis, and endarteritis were also observed in lesions with moderate to severe inflammation. There was no renal deposition of immunoglobulin or complement. In a study using a mouse BMT model (106), similar results were reported. Specifically, allo-HCT recipient mice developed aGVHD within 4 weeks; renal tissue was infiltrated with CD4+ T cells, CD8+ T cells, FoxP3+ T cells, and macrophages; and endarteritis, interstitial nephritis, tubulitis, and glomerulitis were observed.
Ma et al. (113) observed the presence of renal TA-TMA in a TBI-conditioned murine BMT model. In the kidneys of allo-HCT recipients, in addition to tubulitis and interstitial nephritis, mesengiolysis, mesangial proliferation, mesangial edema, subendothelial thickening, endothelial thickening, lumen narrowing, fibrinoid necrosis of afferent arterioles, and microthrombi were observed. All of which are similar to patients with renal TA-TMA. Immunostaining showed C3 complement deposition in the glomeruli, and these glomerular lesions were attenuated in C3-deficient mice, suggesting that complement activation may also be involved in renal injury.
In the kidneys of mice following allo-HCT, increased expression of messenger RNAs for TNF-α, IFN-γ, IL-1α, IL-2, IL-6, and IL-10, as well as the adhesion molecules intercellular adhesion molecule 1 (ICAM-1) and vascular cell adhesion molecule 1 (VCAM-1) have been reported (106). Sadeghi et al. (114) performed BMT in mice using chemotherapy conditioning and compared kidney transcript expression patterns to those in the liver. Genes that were upregulated in the kidneys of allogeneic recipients, compared to syngeneic recipients and muscle without GVHD, included genes involved in antigen presentation, immune response, and leukocyte migration. These patterns were similar to those in the liver.
Collectively, these pre-clinical studies suggest that infiltration of donor-derived immune cells, changes in cytokines and chemokines, and activation of the complement system may be responsible for renal injury after allo-HCT.
Prevention and Treatment of AKI After HCT
Principles of Prevention and Treatment of AKI
AKI is triggered by a variety of factors related to HCT. Therefore, reducing these HCT-related complications is key to preventing AKI. One important strategy to reduce HCT-related complications is to tailor the choice of conditioning regimen and donor source according to each patient’s disease status and comorbidities. The largest contributor to AKI after HCT is drug-induced kidney injury, which can be mitigated by administrating appropriate doses of nephrotoxic agents, and using less nephrotoxic agents when possible. For example, limiting exposure to nephrotoxic antimicrobials decreases the incidence and severity of AKI (115).
Treatment of AKI depends on whether it is pre-renal, renal, or post-renal. Pre-renal AKI is caused by inadequate renal blood flow and responds to hydration. Hydration is also used to prevent renal injury from nephrotoxic agents such as IV contrast for imaging studies. However, care must be taken to avoid fluid overload and pulmonary edema in fluid-sensitive patients including those with decreased cardiac function. Renal AKI is unresponsive to hydration, and oliguria or anuria may persist for several weeks. Blood pressure and fluid balance should be tightly controlled, and nephrotoxic drugs should be discontinued. Depending on the cause of renal AKI, pharmacotherapy with furosemide, atrial natriuretic peptide, and low-dose dopamine may be used, but there is a lack of evidence for their efficacy in preventing or treating renal AKI. In the case of post-renal AKI, obstruction and hydronephrosis are diagnosed by imaging studies, and the main treatment is relief of the obstruction (12, 116).
Renal replacement therapy (RRT) is required for severe renal dysfunction. Patients with prolonged oliguria or anuria, for which RRT is essential for life support, are absolute indications for RRT. There is no consensus on whether earlier initiation of RRT improves the prognosis of severe AKI (117–120).
Disease-Specific Treatment
When the cause of AKI is determined, treatment should focus on correcting it. For example, AKI related to hepatic SOS should be treated with defibrotide, aggressive attempts to maintain fluid balance, and possibly methylprednisolone (121, 122). Prophylactic use of ursodeoxycholic acid (123), defibrotide (124), and fresh frozen plasma (125) should be considered in those at high risk of SOS.
There is no established treatment for TA-TMA, but potential contributing factors should be eliminated when possible. For example, if an infection is thought to contribute, then treatment should be directed toward the pathogen, and every effort should be made to avoid further kidney injury. If CNI therapy is thought to contribute, then CNI withdrawal or dose reduction should be considered (62). Plasma exchange may be performed for the treatment of severe TA-TMA, but the response is usually poor (126, 127). Other potentially efficacious treatments include recombinant thrombomodulin (128), defibrotide (129), rituximab (a monoclonal antibody against CD20) (130), and eculizumab (a monoclonal antibody against complement C5) (131). However, none of these have been investigated in large-scale prospective studies.
Hemorrhagic cystitis (caused by adenovirus or BK virus infection) may require surgical decompression with a nephrostomy tube if urinary tract obstruction cannot be relieved by bladder irrigation from a urinary catheter. The antiviral drug cidofovir is effective for hemorrhagic cystitis caused by adenovirus (132, 133) and may be effective for hemorrhagic cystitis caused by BK virus (134). Ganciclovir (135) and valganciclovir (136) have also been reported to be effective against hemorrhagic cystitis caused by adenovirus.
Conclusion
AKI is a common complication of HCT and an important determinant of HCT-related mortality. As described above, AKI after HCT can be caused by a variety of HCT complications and by many drugs commonly used before, during, and after HCT. Furthermore, the agents used to prevent and treat many HCT-related complications can contribute to kidney injury. In individual patients, it is common for several etiologies of AKI to be present at once. In fact, it is likely that these multiple etiologies act in combination. Due to the presence of multiple etiologies for AKI, it is often difficult to quantify the contribution of any one factor in individual patients. In addition, uncharacterized factors may also contribute to renal injury after HCT. For instance, the kidneys are not considered a primary aGVHD target organ, but recent data suggests that renal aGVHD may cause AKI.
Additional research is needed to identify the factors that cause AKI in HCT recipients. This research will hopefully improve the clinical ability to pinpoint specific causes of AKI in individual patients, and lead to therapies targeting each underlying pathologic etiology. Such advances in the diagnosis, prevention, and treatment of AKI in HCT recipients will improve the safety of HCT.
Author Contributions
MM, KI, and TT conceived of the concept and important topics to include in the article. All authors contributed to writing this review and critical appraisal and review of the final version.
Funding
This work was supported by JSPS KAKENHI Grant-in-Aid for Scientific Research (C) Grant Number JP20K08704 (TT), JP21K08410 (KI), The Japanese Society of Hematology Research Grant (TT), Takeda Science Foundation Research Grant (TT), The Hope from Harper St. Baldrick’s Foundation Fellowship (DP), and Hyundai Hope on Wheels Young Investigator Grant (DP).
Conflict of Interest
The authors declare that the research was conducted in the absence of any commercial or financial relationships that could be construed as a potential conflict of interest.
Publisher’s Note
All claims expressed in this article are solely those of the authors and do not necessarily represent those of their affiliated organizations, or those of the publisher, the editors and the reviewers. Any product that may be evaluated in this article, or claim that may be made by its manufacturer, is not guaranteed or endorsed by the publisher.
References
1. Copelan EA. Hematopoietic Stem-Cell Transplantation. N Engl J Med (2006) 354(17):1813–26. doi: 10.1056/NEJMra052638
2. Singh AK, McGuirk JP. Allogeneic Stem Cell Transplantation: A Historical and Scientific Overview. Cancer Res (2016) 76(22):6445–51. doi: 10.1158/0008-5472.CAN-16-1311
3. Ferrara JL, Levine JE, Reddy P, Holler E. Graft-Versus-Host Disease. Lancet (2009) 373(9674):1550–61. doi: 10.1016/S0140-6736(09)60237-3
4. Zeiser R, Blazar BR. Acute Graft-Versus-Host Disease - Biologic Process, Prevention, and Therapy. N Engl J Med (2017) 377(22):2167–79. doi: 10.1056/NEJMra1609337
5. Toubai T, Sun Y, Reddy P. GVHD Pathophysiology: Is Acute Different From Chronic? Best Pract Res Clin Haematol (2008) 21(2):101–17. doi: 10.1016/j.beha.2008.02.005
6. Ramadan A, Paczesny S. Various Forms of Tissue Damage and Danger Signals Following Hematopoietic Stem-Cell Transplantation. Front Immunol (2015) 6:14. doi: 10.3389/fimmu.2015.00014
7. Toubai T, Magenau J. Immunopathology and Biology-Based Treatment of Steroid-Refractory Graft-Versus-Host Disease. Blood (2020) 136(4):429–40. doi: 10.1182/blood.2019000953
8. Mariotti J, Penack O, Castagna L. Acute Graft-Versus-Host-Disease Other Than Typical Targets: Between Myths and Facts. Transplant Cell Ther (2021) 27(2):115–24. doi: 10.1016/j.bbmt.2020.09.033
9. Thomas ME, Blaine C, Dawnay A, Devonald MA, Ftouh S, Laing C, et al. The Definition of Acute Kidney Injury and Its Use in Practice. Kidney Int (2015) 87(1):62–73. doi: 10.1038/ki.2014.328
10. Bellomo R, Ronco C, Kellum JA, Mehta RL, Palevsky P, workgroup ADQI. Acute Renal Failure - Definition, Outcome Measures, Animal Models, Fluid Therapy and Information Technology Needs: The Second International Consensus Conference of the Acute Dialysis Quality Initiative (ADQI) Group. Crit Care (2004) 8(4):R204–12. doi: 10.1186/cc2872
11. Mehta RL, Kellum JA, Shah SV, Molitoris BA, Ronco C, Warnock DG, et al. Acute Kidney Injury Network: Report of an Initiative to Improve Outcomes in Acute Kidney Injury. Crit Care (2007) 11(2):R31. doi: 10.1186/cc5713
12. Kidney Disease. Improving Global Outcomes (KDIGO) Acute Kidney Injury Work Group. KDIGO Clinical Practice Guideline for Acute Kidney Injury. Kidney Int Suppl (2012) 2:1–138. doi: 10.1038/kisup.2012.1
13. Luo X, Jiang L, Du B, Wen Y, Wang M, Xi X, et al. A Comparison of Different Diagnostic Criteria of Acute Kidney Injury in Critically Ill Patients. Crit Care (2014) 18(4):R144. doi: 10.1186/cc13977
14. Fujii T, Uchino S, Takinami M, Bellomo R. Validation of the Kidney Disease Improving Global Outcomes Criteria for AKI and Comparison of Three Criteria in Hospitalized Patients. Clin J Am Soc Nephrol (2014) 9(5):848–54. doi: 10.2215/CJN.09530913
15. Zeng X, McMahon GM, Brunelli SM, Bates DW, Waikar SS. Incidence, Outcomes, and Comparisons Across Definitions of AKI in Hospitalized Individuals. Clin J Am Soc Nephrol (2014) 9(1):12–20. doi: 10.2215/CJN.02730313
16. Canet E, Lengline E, Zafrani L, Peraldi MN, Socié G, Azoulay E. Acute Kidney Injury in Critically Ill Allo-HSCT Recipients. Bone Marrow Transplant (2014) 49(8):1121–2. doi: 10.1038/bmt.2014.100
17. Piñana JL, Perez-Pitarch A, Garcia-Cadenas I, Barba P, Hernandez-Boluda JC, Esquirol A, et al. A Time-to-Event Model for Acute Kidney Injury After Reduced-Intensity Conditioning Stem Cell Transplantation Using a Tacrolimus- and Sirolimus-Based Graft-Versus-Host Disease Prophylaxis. Biol Blood Marrow Transplant (2017) 23(7):1177–85. doi: 10.1016/j.bbmt.2017.03.035
18. Mima A, Tansho K, Nagahara D, Tsubaki K. Incidence of Acute Kidney Disease After Receiving Hematopoietic Stem Cell Transplantation: A Single-Center Retrospective Study. PeerJ (2019) 7:e6467. doi: 10.7717/peerj.6467
19. Andronesi AG, Tanase AD, Sorohan BM, Craciun OG, Stefan L, Varady Z, et al. Incidence and Risk Factors for Acute Kidney Injury Following Autologous Stem Cell Transplantation for Multiple Myeloma. Cancer Med (2019) 8(6):3278–85. doi: 10.1002/cam4.2187
20. Kanduri SR, Cheungpasitporn W, Thongprayoon C, Bathini T, Kovvuru K, Garla V, et al. Incidence and Mortality of Acute Kidney Injury in Patients Undergoing Hematopoietic Stem Cell Transplantation: A Systematic Review and Meta-Analysis. QJM (2020) 113(9):621–32. doi: 10.1093/qjmed/hcaa072
21. Kersting S, Koomans HA, Hené RJ, Verdonck LF. Acute Renal Failure After Allogeneic Myeloablative Stem Cell Transplantation: Retrospective Analysis of Incidence, Risk Factors and Survival. Bone Marrow Transplant (2007) 39(6):359–65. doi: 10.1038/sj.bmt.1705599
22. Gutiérrez-García G, Villarreal J, Garrote M, Rovira M, Blasco M, Suárez-Lledó M, et al. Impact of Severe Acute Kidney Injury and Chronic Kidney Disease on Allogeneic Hematopoietic Cell Transplant Recipients: A Retrospective Single Center Analysis. Bone Marrow Transplant (2020) 55(7):1264–71. doi: 10.1038/s41409-020-0843-3
23. Piñana JL, Valcárcel D, Martino R, Barba P, Moreno E, Sureda A, et al. Study of Kidney Function Impairment After Reduced-Intensity Conditioning Allogeneic Hematopoietic Stem Cell Transplantation. A Single-Center Experience. Biol Blood Marrow Transplant (2009) 15(1):21–9. doi: 10.1016/j.bbmt.2008.10.011
24. Fadia A, Casserly LF, Sanchorawala V, Seldin DC, Wright DG, Skinner M, et al. Incidence and Outcome of Acute Renal Failure Complicating Autologous Stem Cell Transplantation for AL Amyloidosis. Kidney Int (2003) 63(5):1868–73. doi: 10.1046/j.1523-1755.2003.00936.x
25. Wolff D, Wilhelm S, Hahn J, Gentilini C, Hilgendorf I, Steiner B, et al. Replacement of Calcineurin Inhibitors With Daclizumab in Patients With Transplantation-Associated Microangiopathy or Renal Insufficiency Associated With Graft-Versus-Host Disease. Bone Marrow Transplant (2006) 38(6):445–51. doi: 10.1038/sj.bmt.1705454
26. Abramson MH, Gutgarts V, Zheng J, Maloy MA, Ruiz JD, Scordo M, et al. Acute Kidney Injury in the Modern Era of Allogeneic Hematopoietic Stem Cell Transplantation. Clin J Am Soc Nephrol (2021) 16(9):1318–27. doi: 10.2215/CJN.19801220
27. Liu H, Ding JH, Liu BC, Zhao G, Chen BA. Early Renal Injury After Nonmyeloablative Allogeneic Peripheral Blood Stem Cell Transplantation in Patients With Chronic Myelocytic Leukemia. Am J Nephrol (2007) 27(4):336–41. doi: 10.1159/000103213
28. Liu H, Li YF, Liu BC, Ding JH, Chen BA, Xu WL, et al. A Multicenter, Retrospective Study of Acute Kidney Injury in Adult Patients With Nonmyeloablative Hematopoietic SCT. Bone Marrow Transplant (2010) 45(1):153–8. doi: 10.1038/bmt.2009.99
29. Wanchoo R, Stotter BR, Bayer RL, Jhaveri KD. Acute Kidney Injury in Hematopoietic Stem Cell Transplantation. Curr Opin Crit Care (2019) 25(6):531–8. doi: 10.1097/MCC.0000000000000657
30. Hingorani SR, Guthrie K, Batchelder A, Schoch G, Aboulhosn N, Manchion J, et al. Acute Renal Failure After Myeloablative Hematopoietic Cell Transplant: Incidence and Risk Factors. Kidney Int (2005) 67(1):272–7. doi: 10.1111/j.1523-1755.2005.00078.x
31. Izzedine H, Launay-Vacher V, Deray G. Antiviral Drug-Induced Nephrotoxicity. Am J Kidney Dis (2005) 45(5):804–17. doi: 10.1053/j.ajkd.2005.02.010
32. Lopez-Novoa JM, Quiros Y, Vicente L, Morales AI, Lopez-Hernandez FJ. New Insights Into the Mechanism of Aminoglycoside Nephrotoxicity: An Integrative Point of View. Kidney Int (2011) 79(1):33–45. doi: 10.1038/ki.2010.337
33. Sakaguchi M, Nakayama K, Yamaguchi H, Mii A, Shimizu A, Inai K, et al. Risk Factors for Acute Kidney Injury and Chronic Kidney Disease Following Allogeneic Hematopoietic Stem Cell Transplantation for Hematopoietic Malignancies. Acta Haematol (2020) 143(5):452–64. doi: 10.1159/000504354
34. Hingorani S. Renal Complications of Hematopoietic-Cell Transplantation. N Engl J Med (2016) 374(23):2256–67. doi: 10.1056/NEJMra1404711
35. Lopes JA, Jorge S, Neves M. Acute Kidney Injury in HCT: An Update. Bone Marrow Transplant (2016) 51(6):755–62. doi: 10.1038/bmt.2015.357
36. Parikh CR, Coca SG. Acute Renal Failure in Hematopoietic Cell Transplantation. Kidney Int (2006) 69(3):430–5. doi: 10.1038/sj.ki.5000055
37. Sehgal B, George P, John MJ, Samuel C. Acute Kidney Injury and Mortality in Hematopoietic Stem Cell Transplantation: A Single-Center Experience. Indian J Nephrol (2017) 27(1):13–9. doi: 10.4103/0971-4065.177138
38. Khalil AA, Khalil LT, Awidi A. Incidence, Risk Factors and Prognosis of Acute Kidney Injury Following Hematopoietic Stem Cell Transplant: A Pilot Study. Int J Stem Cells (2019) 12(1):43–50. doi: 10.15283/ijsc18056
39. Bhasin B, Ber Ce P, Szabo A, Chhabra S, D’Souza A. Correlates and Outcomes of Early Acute Kidney Injury After Hematopoietic Cell Transplantation. Am J Med Sci (2021) 362(1):72–7. doi: 10.1016/j.amjms.2021.03.013
40. Parikh CR, Schrier RW, Storer B, Diaconescu R, Sorror ML, Maris MB, et al. Comparison of ARF After Myeloablative and Nonmyeloablative Hematopoietic Cell Transplantation. Am J Kidney Dis (2005) 45(3):502–9. doi: 10.1053/j.ajkd.2004.11.013
41. Lopes JA, Jorge S. Acute Kidney Injury Following HCT: Incidence, Risk Factors and Outcome. Bone Marrow Transplant (2011) 46(11):1399–408. doi: 10.1038/bmt.2011.46
42. Kogon A, Hingorani S. Acute Kidney Injury in Hematopoietic Cell Transplantation. Semin Nephrol (2010) 30(6):615–26. doi: 10.1016/j.semnephrol.2010.09.009
43. Yu ZP, Ding JH, Chen BA, Liu BC, Liu H, Li YF, et al. Risk Factors for Acute Kidney Injury in Patients Undergoing Allogeneic Hematopoietic Stem Cell Transplantation. Chin J Cancer (2010) 29(11):946–51. doi: 10.5732/cjc.010.10293
44. Hirano D, Kakegawa D, Miwa S, Umeda C, Takemasa Y, Tokunaga A, et al. Independent Risk Factors and Long-Term Outcomes for Acute Kidney Injury in Pediatric Patients Undergoing Hematopoietic Stem Cell Transplantation: A Retrospective Cohort Study. BMC Nephrol (2020) 21(1):373. doi: 10.1186/s12882-020-02045-8
45. Mae H, Ooi J, Takahashi S, Tomonari A, Tsukada N, Konuma T, et al. Early Renal Injury After Myeloablative Cord Blood Transplantation in Adults. Leuk Lymphoma (2008) 49(3):538–42. doi: 10.1080/10428190701824577
46. Mae H, Ooi J, Takahashi S, Kato S, Kawakita T, Ebihara Y, et al. Acute Kidney Injury After Myeloablative Cord Blood Transplantation in Adults: The Efficacy of Strict Monitoring of Vancomycin Serum Trough Concentrations. Transpl Infect Dis (2013) 15(2):181–6. doi: 10.1111/tid.12038
47. Gutgarts V, Sathick IJ, Zheng J, Politikos I, Devlin SM, Maloy MA, et al. Incidence and Risk Factors for Acute and Chronic Kidney Injury After Adult Cord Blood Transplantation. Biol Blood Marrow Transplant (2020) 26(4):758–63. doi: 10.1016/j.bbmt.2019.12.768
48. Musiał K, Kałwak K, Zwolińska D. The Impact of Allogeneic Hematopoietic Stem Cell Transplantation on Kidney Function in Children-A Single Center Experience. J Clin Med (2021) 10(5):1113. doi: 10.3390/jcm10051113
49. Matsuoka D, Hirabayashi K, Murase T, Saito S, Nakazawa Y. Impact of Acute Kidney Injury on Overall Survival in Children and Young Adults Undergoing Allogeneic Hematopoietic Stem Cell Transplantation. Pediatr Blood Cancer (2021) 68(9):e29167. doi: 10.1002/pbc.29167
50. Lugthart G, Jordans CCE, de Pagter APJ, Bresters D, Jol-van der Zijde CM, Bense JE, et al. Chronic Kidney Disease Ten Years After Pediatric Allogeneic Hematopoietic Stem Cell Transplantation. Kidney Int (2021) 100(4):906–14. doi: 10.1016/j.kint.2021.05.030
51. Coca SG, Yusuf B, Shlipak MG, Garg AX, Parikh CR. Long-Term Risk of Mortality and Other Adverse Outcomes After Acute Kidney Injury: A Systematic Review and Meta-Analysis. Am J Kidney Dis (2009) 53(6):961–73. doi: 10.1053/j.ajkd.2008.11.034
52. Shingai N, Morito T, Najima Y, Kobayashi T, Doki N, Kakihana K, et al. Early-Onset Acute Kidney Injury Is a Poor Prognostic Sign for Allogeneic SCT Recipients. Bone Marrow Transplant (2015) 50(12):1557–62. doi: 10.1038/bmt.2015.188
53. Kersting S, Dorp SV, Theobald M, Verdonck LF. Acute Renal Failure After Nonmyeloablative Stem Cell Transplantation in Adults. Biol Blood Marrow Transplant (2008) 14(1):125–31. doi: 10.1016/j.bbmt.2007.09.016
54. Mori J, Ohashi K, Yamaguchi T, Ando M, Hirashima Y, Kobayashi T, et al. Risk Assessment for Acute Kidney Injury After Allogeneic Hematopoietic Stem Cell Transplantation Based on Acute Kidney Injury Network Criteria. Intern Med (2012) 51(16):2105–10. doi: 10.2169/internalmedicine.51.7418
55. Liu Y, Xu L, Zhang X, Wang Y, Liu K, Chen H, et al. Acute Kidney Injury Following Haplo Stem Cell Transplantation: Incidence, Risk Factors and Outcome. Bone Marrow Transplant (2018) 53(4):483–6. doi: 10.1038/s41409-017-0030-3
56. Koh KN, Sunkara A, Kang G, Sooter A, Mulrooney DA, Triplett B, et al. Acute Kidney Injury in Pediatric Patients Receiving Allogeneic Hematopoietic Cell Transplantation: Incidence, Risk Factors, and Outcomes. Biol Blood Marrow Transplant (2018) 24(4):758–64. doi: 10.1016/j.bbmt.2017.11.021
57. Daraskevicius J, Azukaitis K, Dziugeviciute-Tupko J, Peciulyte M, Planciunaite R, Vaitkeviciene G, et al. Phenotypes and Baseline Risk Factors of Acute Kidney Injury in Children After Allogeneic Hematopoietic Stem Cell Transplantation. Front Pediatr (2020) 8:499. doi: 10.3389/fped.2020.00499
58. Prasad M, Jain NG, Radhakrishnan J, Jin Z, Satwani P. Risk Factors for Chronic Kidney Disease Following Acute Kidney Injury in Pediatric Allogeneic Hematopoietic Cell Transplantation. Bone Marrow Transplant (2021) 56(7):1665–73. doi: 10.1038/s41409-021-01228-7
59. Gooley TA, Chien JW, Pergam SA, Hingorani S, Sorror ML, Boeckh M, et al. Reduced Mortality After Allogeneic Hematopoietic-Cell Transplantation. N Engl J Med (2010) 363(22):2091–101. doi: 10.1056/NEJMoa1004383
60. Renaghan AD, Jaimes EA, Malyszko J, Perazella MA, Sprangers B, Rosner MH. Acute Kidney Injury and CKD Associated With Hematopoietic Stem Cell Transplantation. Clin J Am Soc Nephrol (2020) 15(2):289–97. doi: 10.2215/CJN.08580719
61. Naesens M, Kuypers DR, Sarwal M. Calcineurin Inhibitor Nephrotoxicity. Clin J Am Soc Nephrol (2009) 4(2):481–508. doi: 10.2215/CJN.04800908
62. Wanchoo R, Bayer RL, Bassil C, Jhaveri KD. Emerging Concepts in Hematopoietic Stem Cell Transplantation-Associated Renal Thrombotic Microangiopathy and Prospects for New Treatments. Am J Kidney Dis (2018) 72(6):857–65. doi: 10.1053/j.ajkd.2018.06.013
63. Abelson HT, Fosburg MT, Beardsley GP, Goorin AM, Gorka C, Link M, et al. Methotrexate-Induced Renal Impairment: Clinical Studies and Rescue From Systemic Toxicity With High-Dose Leucovorin and Thymidine. J Clin Oncol (1983) 1(3):208–16. doi: 10.1200/JCO.1983.1.3.208
64. Perazella MA. Pharmacology Behind Common Drug Nephrotoxicities. Clin J Am Soc Nephrol (2018) 13(12):1897–908. doi: 10.2215/CJN.00150118
65. Botero Aguirre JP, Restrepo Hamid AM. Amphotericin B Deoxycholate Versus Liposomal Amphotericin B: Effects on Kidney Function. Cochrane Database Syst Rev (2015) 11:CD010481. doi: 10.1002/14651858.CD010481.pub2
66. Mistro S, Maciel IEM, de Menezes RG, Maia ZP, Schooley RT, Badaró R. Does Lipid Emulsion Reduce Amphotericin B Nephrotoxicity? A Systematic Review and Meta-Analysis. Clin Infect Dis (2012) 54(12):1774–7. doi: 10.1093/cid/cis290
67. Perazella MA. Crystal-Induced Acute Renal Failure. Am J Med (1999) 106(4):459–65. doi: 10.1016/s0002-9343(99)00041-8
68. Finkel KW, Cohen EP, Shirali A, Abudayyeh A, Forum ASoNO-N. Paraprotein-Related Kidney Disease: Evaluation and Treatment of Myeloma Cast Nephropathy. Clin J Am Soc Nephrol (2016) 11(12):2273–9. doi: 10.2215/CJN.01640216
69. Hutchison CA, Batuman V, Behrens J, Bridoux F, Sirac C, Dispenzieri A, et al. The Pathogenesis and Diagnosis of Acute Kidney Injury in Multiple Myeloma. Nat Rev Nephrol (2011) 8(1):43–51. doi: 10.1038/nrneph.2011.168
70. Rosner MH, Perazella MA. Acute Kidney Injury in Patients With Cancer. N Engl J Med (2017) 376(18):1770–81. doi: 10.1056/NEJMra1613984
71. Averbuch D, Tridello G, Hoek J, Mikulska M, Pabst T, Yaňez San Segundo L, et al. Intercontinental Study on Pre-Engraftment and Post-Engraftment Gram-Negative Rods Bacteremia in Hematopoietic Stem Cell Transplantation Patients: Risk Factors and Association With Mortality. J Infect (2020) 81(6):882–94. doi: 10.1016/j.jinf.2020.11.002
72. Schrier RW, Wang W. Acute Renal Failure and Sepsis. N Engl J Med (2004) 351(2):159–69. doi: 10.1056/NEJMra032401
73. Ito M, Hirabayashi N, Uno Y, Nakayama A, Asai J. Necrotizing Tubulointerstitial Nephritis Associated With Adenovirus Infection. Hum Pathol (1991) 22(12):1225–31. doi: 10.1016/0046-8177(91)90104-w
74. Baldwin A, Kingman H, Darville M, Foot AB, Grier D, Cornish JM, et al. Outcome and Clinical Course of 100 Patients With Adenovirus Infection Following Bone Marrow Transplantation. Bone Marrow Transplant (2000) 26(12):1333–8. doi: 10.1038/sj.bmt.1702716
75. Hirsch HH, Steiger J. Polyomavirus Bk. Lancet Infect Dis (2003) 3(10):611–23. doi: 10.1016/s1473-3099(03)00770-9
76. Leung AY, Mak R, Lie AK, Yuen KY, Cheng VC, Liang R, et al. Clinicopathological Features and Risk Factors of Clinically Overt Haemorrhagic Cystitis Complicating Bone Marrow Transplantation. Bone Marrow Transplant (2002) 29(6):509–13. doi: 10.1038/sj.bmt.1703415
77. Erard V, Kim HW, Corey L, Limaye A, Huang ML, Myerson D, et al. BK DNA Viral Load in Plasma: Evidence for an Association With Hemorrhagic Cystitis in Allogeneic Hematopoietic Cell Transplant Recipients. Blood (2005) 106(3):1130–2. doi: 10.1182/blood-2004-12-4988
78. Brukamp K, Doyle AM, Bloom RD, Bunin N, Tomaszewski JE, Cizman B. Nephrotic Syndrome After Hematopoietic Cell Transplantation: Do Glomerular Lesions Represent Renal Graft-Versus-Host Disease? Clin J Am Soc Nephrol (2006) 1(4):685–94. doi: 10.2215/CJN.00380705
79. Beyar-Katz O, Davila EK, Zuckerman T, Fineman R, Haddad N, Okasha D, et al. Adult Nephrotic Syndrome After Hematopoietic Stem Cell Transplantation: Renal Pathology Is the Best Predictor of Response to Therapy. Biol Blood Marrow Transplant (2016) 22(6):975–81. doi: 10.1016/j.bbmt.2015.12.014
80. Numata A, Morishita Y, Mori M, Saito O, Takemoto F, Ando Y, et al. De Novo Postallogeneic Hematopoietic Stem Cell Transplant Membranous Nephropathy. Exp Clin Transplant (2013) 11(1):75–8. doi: 10.6002/ect.2012.0078
81. Zager RA. Acute Renal Failure in the Setting of Bone Marrow Transplantation. Kidney Int (1994) 46(5):1443–58. doi: 10.1038/ki.1994.417
82. Mughal TI, Ejaz AA, Foringer JR, Coiffier B. An Integrated Clinical Approach for the Identification, Prevention, and Treatment of Tumor Lysis Syndrome. Cancer Treat Rev (2010) 36(2):164–76. doi: 10.1016/j.ctrv.2009.11.001
83. McDonald GB. Hepatobiliary Complications of Hematopoietic Cell Transplantation, 40 Years on. Hepatology (2010) 51(4):1450–60. doi: 10.1002/hep.23533
84. Gruss E, Bernis C, Tomas JF, Garcia-Canton C, Figuera A, Motellón JL, et al. Acute Renal Failure in Patients Following Bone Marrow Transplantation: Prevalence, Risk Factors and Outcome. Am J Nephrol (1995) 15(6):473–9. doi: 10.1159/000168889
85. Merouani A, Shpall EJ, Jones RB, Archer PG, Schrier RW. Renal Function in High Dose Chemotherapy and Autologous Hematopoietic Cell Support Treatment for Breast Cancer. Kidney Int (1996) 50(3):1026–31. doi: 10.1038/ki.1996.405
86. Bearman SI. The Syndrome of Hepatic Veno-Occlusive Disease After Marrow Transplantation. Blood (1995) 85(11):3005–20. doi: 10.1182/blood.V85.11.3005.bloodjournal85113005
87. Coppell JA, Richardson PG, Soiffer R, Martin PL, Kernan NA, Chen A, et al. Hepatic Veno-Occlusive Disease Following Stem Cell Transplantation: Incidence, Clinical Course, and Outcome. Biol Blood Marrow Transplant (2010) 16(2):157–68. doi: 10.1016/j.bbmt.2009.08.024
88. Jodele S, Laskin BL, Dandoy CE, Myers KC, El-Bietar J, Davies SM, et al. A New Paradigm: Diagnosis and Management of HSCT-Associated Thrombotic Microangiopathy as Multi-System Endothelial Injury. Blood Rev (2015) 29(3):191–204. doi: 10.1016/j.blre.2014.11.001
89. Mii A, Shimizu A, Yamaguchi H, Tsuruoka S. Renal Complications After Hematopoietic Stem Cell Transplantation: Role of Graft-Versus-Host Disease in Renal Thrombotic Microangiopathy. J Nippon Med Sch (2020) 87(1):7–12. doi: 10.1272/jnms.JNMS.2020_87-102
90. Ho VT, Cutler C, Carter S, Martin P, Adams R, Horowitz M, et al. Blood and Marrow Transplant Clinical Trials Network Toxicity Committee Consensus Summary: Thrombotic Microangiopathy After Hematopoietic Stem Cell Transplantation. Biol Blood Marrow Transplant (2005) 11(8):571–5. doi: 10.1016/j.bbmt.2005.06.001
91. Ruutu T, Barosi G, Benjamin RJ, Clark RE, George JN, Gratwohl A, et al. Diagnostic Criteria for Hematopoietic Stem Cell Transplant-Associated Microangiopathy: Results of a Consensus Process by an International Working Group. Haematologica (2007) 92(1):95–100. doi: 10.3324/haematol.10699
92. Siami K, Kojouri K, Swisher KK, Selby GB, George JN, Laszik ZG. Thrombotic Microangiopathy After Allogeneic Hematopoietic Stem Cell Transplantation: An Autopsy Study. Transplantation (2008) 85(1):22–8. doi: 10.1097/01.tp.0000297998.33418.7e
93. Changsirikulchai S, Myerson D, Guthrie KA, McDonald GB, Alpers CE, Hingorani SR. Renal Thrombotic Microangiopathy After Hematopoietic Cell Transplant: Role of GVHD in Pathogenesis. Clin J Am Soc Nephrol (2009) 4(2):345–53. doi: 10.2215/CJN.02070508
94. Epperla N, Li A, Logan B, Fretham C, Chhabra S, Aljurf M, et al. Incidence, Risk Factors for and Outcomes of Transplant-Associated Thrombotic Microangiopathy. Br J Haematol (2020) 189(6):1171–81. doi: 10.1111/bjh.16457
95. Luft T, Dietrich S, Falk C, Conzelmann M, Hess M, Benner A, et al. Steroid-Refractory GVHD: T-Cell Attack Within a Vulnerable Endothelial System. Blood (2011) 118(6):1685–92. doi: 10.1182/blood-2011-02-334821
96. Tichelli A, Gratwohl A. Vascular Endothelium as ’Novel’ Target of Graft-Versus-Host Disease. Best Pract Res Clin Haematol (2008) 21(2):139–48. doi: 10.1016/j.beha.2008.02.002
97. Mii A, Shimizu A, Kaneko T, Fujita E, Fukui M, Fujino T, et al. Renal Thrombotic Microangiopathy Associated With Chronic Graft-Versus-Host Disease After Allogeneic Hematopoietic Stem Cell Transplantation. Pathol Int (2011) 61(9):518–27. doi: 10.1111/j.1440-1827.2011.02704.x
98. Hingorani S, Finn LS, Pao E, Lawler R, Schoch G, McDonald GB, et al. Urinary Elafin and Kidney Injury in Hematopoietic Cell Transplant Recipients. Clin J Am Soc Nephrol (2015) 10(1):12–20. doi: 10.2215/CJN.01840214
99. Hingorani S, Gooley T, Pao E, Sandmaier B, McDonald G. Urinary Cytokines After HCT: Evidence for Renal Inflammation in the Pathogenesis of Proteinuria and Kidney Disease. Bone Marrow Transplant (2014) 49(3):403–9. doi: 10.1038/bmt.2013.197
100. Chen YB, Cutler CS. Biomarkers for Acute GVHD: Can We Predict the Unpredictable? Bone Marrow Transplant (2013) 48(6):755–60. doi: 10.1038/bmt.2012.143
101. Paczesny S, Braun TM, Levine JE, Hogan J, Crawford J, Coffing B, et al. Elafin Is a Biomarker of Graft-Versus-Host Disease of the Skin. Sci Transl Med (2010) 2(13):13ra2. doi: 10.1126/scitranslmed.3000406
102. Williams SE, Brown TI, Roghanian A, Sallenave JM. SLPI and Elafin: One Glove, Many Fingers. Clin Sci (Lond) (2006) 110(1):21–35. doi: 10.1042/CS20050115
103. Shaw L, Wiedow O. Therapeutic Potential of Human Elafin. Biochem Soc Trans (2011) 39(5):1450–4. doi: 10.1042/BST0391450
104. Girsberger M, Halter JP, Hopfer H, Dickenmann M, Menter T. Kidney Pathology After Hematologic Cell Transplantation-A Single-Center Observation Study of Indication Biopsies and Autopsies. Biol Blood Marrow Transplant (2018) 24(3):571–80. doi: 10.1016/j.bbmt.2017.11.008
105. Boieri M, Shah P, Dressel R, Inngjerdingen M. The Role of Animal Models in the Study of Hematopoietic Stem Cell Transplantation and GvHD: A Historical Overview. Front Immunol (2016) 7:333. doi: 10.3389/fimmu.2016.00333
106. Schmid PM, Bouazzaoui A, Schmid K, Birner C, Schach C, Maier LS, et al. Acute Renal Graft-Versus-Host Disease in a Murine Model of Allogeneic Bone Marrow Transplantation. Cell Transplant (2017) 26(8):1428–40. doi: 10.1177/0963689717720295
107. Higo S, Shimizu A, Masuda Y, Nagasaka S, Kajimoto Y, Kanzaki G, et al. Acute Graft-Versus-Host Disease of the Kidney in Allogeneic Rat Bone Marrow Transplantation. PloS One (2014) 9(12):e115399. doi: 10.1371/journal.pone.0115399
108. Pasala S, Carmody JB. How to Use… Serum Creatinine, Cystatin C and GFR. Arch Dis Child Educ Pract Ed (2017) 102(1):37–43. doi: 10.1136/archdischild-2016-311062
109. Amin R, He R, Gupta D, Zheng W, Burmakin M, Mohammad DK, et al. The Kidney Injury Caused by the Onset of Acute Graft-Versus-Host Disease Is Associated With Down-Regulation of αklotho. Int Immunopharmacol (2020) 78:106042. doi: 10.1016/j.intimp.2019.106042
110. Bazzi C, Petrini C, Rizza V, Arrigo G, Napodano P, Paparella M, et al. Urinary N-Acetyl-Beta-Glucosaminidase Excretion Is a Marker of Tubular Cell Dysfunction and a Predictor of Outcome in Primary Glomerulonephritis. Nephrol Dial Transplant (2002) 17(11):1890–6. doi: 10.1093/ndt/17.11.1890
111. Paragas N, Qiu A, Zhang Q, Samstein B, Deng SX, Schmidt-Ott KM, et al. The Ngal Reporter Mouse Detects the Response of the Kidney to Injury in Real Time. Nat Med (2011) 17(2):216–22. doi: 10.1038/nm.2290
112. Hu MC, Moe OW. Klotho as a Potential Biomarker and Therapy for Acute Kidney Injury. Nat Rev Nephrol (2012) 8(7):423–9. doi: 10.1038/nrneph.2012.92
113. Ma Q, Li D, Vasquez HG, You MJ, Afshar-Kharghan V. Kidney Injury in Murine Models of Hematopoietic Stem Cell Transplantation. Biol Blood Marrow Transplant (2019) 25(10):1920–4. doi: 10.1016/j.bbmt.2019.06.027
114. Sadeghi B, Al-Chaqmaqchi H, Al-Hashmi S, Brodin D, Hassan Z, Abedi-Valugerdi M, et al. Early-Phase GVHD Gene Expression Profile in Target Versus Non-Target Tissues: Kidney, A Possible Target? Bone Marrow Transplant (2013) 48(2):284–93. doi: 10.1038/bmt.2012.120
115. Benoit SW, Goldstein SL, Dahale DS, Haslam DB, Nelson A, Truono K, et al. Reduction in Nephrotoxic Antimicrobial Exposure Decreases Associated Acute Kidney Injury in Pediatric Hematopoietic Stem Cell Transplant Patients. Biol Blood Marrow Transplant (2019) 25(8):1654–8. doi: 10.1016/j.bbmt.2019.04.022
116. Ostermann M, Bellomo R, Burdmann EA, Doi K, Endre ZH, Goldstein SL, et al. Controversies in Acute Kidney Injury: Conclusions From a Kidney Disease: Improving Global Outcomes (KDIGO) Conference. Kidney Int (2020) 98(2):294–309. doi: 10.1016/j.kint.2020.04.020
117. Gaudry S, Hajage D, Schortgen F, Martin-Lefevre L, Pons B, Boulet E, et al. Initiation Strategies for Renal-Replacement Therapy in the Intensive Care Unit. N Engl J Med (2016) 375(2):122–33. doi: 10.1056/NEJMoa1603017
118. Zarbock A, Kellum JA, Schmidt C, Van Aken H, Wempe C, Pavenstädt H, et al. Effect of Early vs Delayed Initiation of Renal Replacement Therapy on Mortality in Critically Ill Patients With Acute Kidney Injury: The ELAIN Randomized Clinical Trial. JAMA (2016) 315(20):2190–9. doi: 10.1001/jama.2016.5828
119. Barbar SD, Clere-Jehl R, Bourredjem A, Hernu R, Montini F, Bruyère R, et al. Timing of Renal-Replacement Therapy in Patients With Acute Kidney Injury and Sepsis. N Engl J Med (2018) 379(15):1431–42. doi: 10.1056/NEJMoa1803213
120. Bagshaw SM, Wald R, Adhikari NKJ, Bellomo R, da Costa BR, Dreyfuss D, et al. Timing of Initiation of Renal-Replacement Therapy in Acute Kidney Injury. N Engl J Med (2020) 383(3):240–51. doi: 10.1056/NEJMoa2000741
121. Richardson PG, Riches ML, Kernan NA, Brochstein JA, Mineishi S, Termuhlen AM, et al. Phase 3 Trial of Defibrotide for the Treatment of Severe Veno-Occlusive Disease and Multi-Organ Failure. Blood (2016) 127(13):1656–65. doi: 10.1182/blood-2015-10-676924
122. Al Beihany A, Al Omar H, Sahovic E, Chaudhri N, Al Mohareb F, Al Sharif F, et al. Successful Treatment of Hepatic Veno-Occlusive Disease After Myeloablative Allogeneic Hematopoietic Stem Cell Transplantation by Early Administration of a Short Course of Methylprednisolone. Bone Marrow Transplant (2008) 41(3):287–91. doi: 10.1038/sj.bmt.1705896
123. Tay J, Tinmouth A, Fergusson D, Huebsch L, Allan DS. Systematic Review of Controlled Clinical Trials on the Use of Ursodeoxycholic Acid for the Prevention of Hepatic Veno-Occlusive Disease in Hematopoietic Stem Cell Transplantation. Biol Blood Marrow Transplant (2007) 13(2):206–17. doi: 10.1016/j.bbmt.2006.09.012
124. Corbacioglu S, Cesaro S, Faraci M, Valteau-Couanet D, Gruhn B, Rovelli A, et al. Defibrotide for Prophylaxis of Hepatic Veno-Occlusive Disease in Paediatric Haemopoietic Stem-Cell Transplantation: An Open-Label, Phase 3, Randomised Controlled Trial. Lancet (2012) 379(9823):1301–9. doi: 10.1016/S0140-6736(11)61938-7
125. Matsumoto M, Kawa K, Uemura M, Kato S, Ishizashi H, Isonishi A, et al. Prophylactic Fresh Frozen Plasma may Prevent Development of Hepatic VOD After Stem Cell Transplantation via ADAMTS13-Mediated Restoration of Von Willebrand Factor Plasma Levels. Bone Marrow Transplant (2007) 40(3):251–9. doi: 10.1038/sj.bmt.1705724
126. Laskin BL, Goebel J, Davies SM, Jodele S. Small Vessels, Big Trouble in the Kidneys and Beyond: Hematopoietic Stem Cell Transplantation-Associated Thrombotic Microangiopathy. Blood (2011) 118(6):1452–62. doi: 10.1182/blood-2011-02-321315
127. Li A, Makar RS, Hurwitz S, Uhl L, Kaufman RM, Stowell CP, et al. Treatment With or Without Plasma Exchange for Patients With Acquired Thrombotic Microangiopathy Not Associated With Severe ADAMTS13 Deficiency: A Propensity Score-Matched Study. Transfusion (2016) 56(8):2069–77. doi: 10.1111/trf.13654
128. Fujiwara H, Maeda Y, Sando Y, Nakamura M, Tani K, Ishikawa T, et al. Treatment of Thrombotic Microangiopathy After Hematopoietic Stem Cell Transplantation With Recombinant Human Soluble Thrombomodulin. Transfusion (2016) 56(4):886–92. doi: 10.1111/trf.13437
129. Corti P, Uderzo C, Tagliabue A, Della Volpe A, Annaloro C, Tagliaferri E, et al. Defibrotide as a Promising Treatment for Thrombotic Thrombocytopenic Purpura in Patients Undergoing Bone Marrow Transplantation. Bone Marrow Transplant (2002) 29(6):542–3. doi: 10.1038/sj.bmt.1703414
130. Ostronoff M, Ostronoff F, Calixto R, Florêncio R, Florêncio M, Domingues MC, et al. Life-Threatening Hemolytic-Uremic Syndrome Treated With Rituximab in an Allogeneic Bone Marrow Transplant Recipient. Bone Marrow Transplant (2007) 39(10):649–51. doi: 10.1038/sj.bmt.1705657
131. Jodele S, Fukuda T, Mizuno K, Vinks AA, Laskin BL, Goebel J, et al. Variable Eculizumab Clearance Requires Pharmacodynamic Monitoring to Optimize Therapy for Thrombotic Microangiopathy After Hematopoietic Stem Cell Transplantation. Biol Blood Marrow Transplant (2016) 22(2):307–15. doi: 10.1016/j.bbmt.2015.10.002
132. Ljungman P, Ribaud P, Eyrich M, Matthes-Martin S, Einsele H, Bleakley M, et al. Cidofovir for Adenovirus Infections After Allogeneic Hematopoietic Stem Cell Transplantation: A Survey by the Infectious Diseases Working Party of the European Group for Blood and Marrow Transplantation. Bone Marrow Transplant (2003) 31(6):481–6. doi: 10.1038/sj.bmt.1703798
133. Nagafuji K, Aoki K, Henzan H, Kato K, Miyamoto T, Eto T, et al. Cidofovir for Treating Adenoviral Hemorrhagic Cystitis in Hematopoietic Stem Cell Transplant Recipients. Bone Marrow Transplant (2004) 34(10):909–14. doi: 10.1038/sj.bmt.1704682
134. Philippe M, Ranchon F, Gilis L, Schwiertz V, Vantard N, Ader F, et al. Cidofovir in the Treatment of BK Virus-Associated Hemorrhagic Cystitis After Allogeneic Hematopoietic Stem Cell Transplantation. Biol Blood Marrow Transplant (2016) 22(4):723–30. doi: 10.1016/j.bbmt.2015.12.009
135. Nakazawa Y, Suzuki T, Fukuyama T, Katsuyama Y, Tanaka M, Yanagisawa R, et al. Urinary Excretion of Ganciclovir Contributes to Improvement of Adenovirus-Associated Hemorrhagic Cystitis After Allogeneic Bone Marrow Transplantation. Pediatr Transplant (2009) 13(5):632–5. doi: 10.1111/j.1399-3046.2008.01027.x
136. Yanagisawa T, Saito S, Katsuyama Y, Hirabayashi K, Shigemura T, Tanaka M, et al. Successful Induction of Therapeutic Urinary Concentration by Intravenous Ganciclovir and Oral Valganciclovir With Remission of Adenoviral Hemorrhagic Cystitis After Cord Blood Transplantation. Pediatr Transplant (2018) e13241. doi: 10.1111/petr.13241
Keywords: acute kidney injury, allogeneic hematologic stem cell transplantation, GvHD, experimental BMT, cytokine, calcinurin inhibitors, thrombotic microagiopathy
Citation: Miyata M, Ichikawa K, Matsuki E, Watanabe M, Peltier D and Toubai T (2022) Recent Advances of Acute Kidney Injury in Hematopoietic Cell Transplantation. Front. Immunol. 12:779881. doi: 10.3389/fimmu.2021.779881
Received: 20 September 2021; Accepted: 02 December 2021;
Published: 04 January 2022.
Edited by:
Daniel Wolff, University Hospital Regensburg, GermanyReviewed by:
Ying-Jun Chang, Peking University People’s Hospital, ChinaLuisa Giaccone, University of Turin, Italy
Copyright © 2022 Miyata, Ichikawa, Matsuki, Watanabe, Peltier and Toubai. This is an open-access article distributed under the terms of the Creative Commons Attribution License (CC BY). The use, distribution or reproduction in other forums is permitted, provided the original author(s) and the copyright owner(s) are credited and that the original publication in this journal is cited, in accordance with accepted academic practice. No use, distribution or reproduction is permitted which does not comply with these terms.
*Correspondence: Tomomi Toubai, dG91YmFpQG1lZC5pZC55YW1hZ2F0YS11LmFjLmpw; Kazunobu Ichikawa, aWNoaWthd2Eta0BtZWQuaWQueWFtYWdhdGEtdS5hYy5qcA==