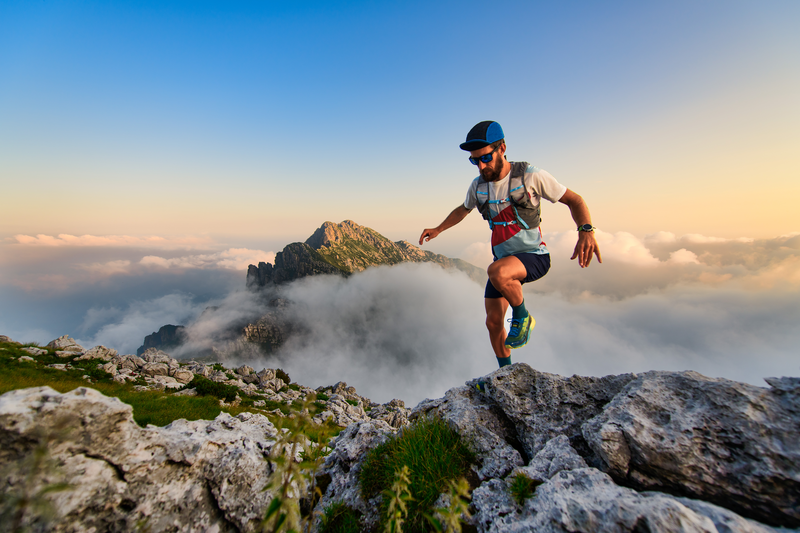
94% of researchers rate our articles as excellent or good
Learn more about the work of our research integrity team to safeguard the quality of each article we publish.
Find out more
PERSPECTIVE article
Front. Immunol. , 14 December 2021
Sec. Vaccines and Molecular Therapeutics
Volume 12 - 2021 | https://doi.org/10.3389/fimmu.2021.778028
A correction has been applied to this article in:
Corrigendum: A Perspective on the Success and Failure of BCG
TB continues to be one of the major public health threats. BCG is the only available vaccine against TB and confers significant protection against the childhood disease. However, the protective efficacy of BCG against adult pulmonary TB, which represents a larger burden of disease, is highly variable. It has been suggested that prior exposure to environmental mycobacteria (EMb) mitigates the anti-TB efficacy of BCG by blocking its duplication or masking its immunogenicity. However, its effectiveness against childhood TB and failure of repeated administration to provide additional benefit against pulmonary TB, suggest of some other mechanisms for the variable efficacy of BCG against the pulmonary disease. Importantly, TB is a heterogeneous disease occurring in different forms and having distinct mechanisms of pathogenesis. While inability of the immune system to contain the bacilli is responsible for TB pathogenesis in infants, an aggravated immune response to Mtb has been blamed for the development of adult pulmonary TB. Available data suggest that EMb play a key role in heightening the immune response against Mtb. In this article, differential efficacy of BCG against childhood and adult TB is explained by taking into account the heterogeneity of TB, mechanisms of TB pathogenesis, and the effect of EMb on anti-Mtb immunity. It is believed that a refined understanding of the success and failure of BCG will help in the development of effective anti-TB vaccines.
Tuberculosis (TB) continues to be one of the major public health threats, accounting for approximately 1.5 million deaths per year across the globe. BCG (Bacillus Calmette-Guerin), which was developed nearly 100 years ago, is the only available vaccine against TB. Despite conferring significant protection against childhood manifestations of TB, protective efficacy of BCG against adult pulmonary TB is highly variable (1, 2). Lowest efficacy of BCG against adult pulmonary TB has been observed in the tropics, which account for the major burden of the disease. Why BCG confers significant protection against childhood TB but fails against the adult pulmonary disease remains an open question.
Mycobacterium tuberculosis (Mtb), the causative agent of TB, shares an intricate relationship with the host immune system and leads to different clinical outcomes. Therefore, TB is considered a heterogeneous disease, occurring in different patterns and presentations and having distinct mechanisms of pathogenesis (3). In young children, Mtb infection leads to the primary disease commonly affecting extra-pulmonary sites. On the other hand, TB develops after reactivation of latent infection and preferentially affects lung tissue in most immunocompetent adults. Among various factors involved in defining the efficacy of BCG and the outcome of Mtb infection, exposure to environmental mycobacteria (EMb) has been shown to play a particularly important role (1).
In the present manuscript, I shed light on the variable efficacy of BCG and explained it by taking into account the heterogeneity of TB, mechanisms of TB pathogenesis, and the effect of EMb on anti-Mtb immunity. It is believed that a refined understanding of the success and failure of BCG will help in the development of effective anti-TB vaccines.
BCG was derived by French researchers Albert Calmette and Camille Guerin by in vitro passaging M. bovis for nearly 13 years. Clinical studies with BCG took place in France and Belgium in the 1920s and demonstrated its efficacy against childhood TB (4). As the effectiveness of BCG against childhood TB was observed in these and other European countries, World Health Organization (WHO) recommended expansion of the BCG vaccination program to the TB-endemic countries (1).
Despite more than 3 billion people receiving BCG, TB continues to be a devastating disease worldwide. A major drawback of BCG is its variable efficacy (ranging from nil to 80%) against adult TB. Palmer and colleagues were first to recognize that BCG is more effective against pulmonary TB at higher latitudes (1). Various factors including strain variations and poor cold-chain maintenance were suggested to be responsible for the variable efficacy of BCG against the adult disease. However, as the same BCG strains exhibited higher anti-TB efficacy in other countries, and were effective against leprosy in areas of their poor anti-TB efficacy, role of these factors in variable efficacy of BCG against adult TB was proved to be unfounded (5).
Currently, two hypotheses (masking hypothesis and blocking hypothesis) are considered most pertinent for explaining the failure of BCG against pulmonary TB. Masking hypothesis was based on the work by Palmer and colleagues, who observed in large-scale experiments in guinea pigs that immunization with EMb induces an appreciable level of protective immunity against Mtb and that the effects of subsequent BCG vaccination in these animals are markedly reduced (6). It was deduced that EMb, while imparting certain degree of protective immunity against Mtb, mask the efficacy of BCG to confer protection against the TB bacilli (6). Blocking hypothesis was based on the work by Anderson and colleagues, who demonstrated with a mouse model that prior exposure to EMb induces potent antimycobacterial immune responses, which block the duplication of BCG and thereby abrogate its efficacy to induce protective immunity against subsequent Mtb infection (7). As BCG is a live attenuated vaccine, its duplication in immunized people was considered as a precondition for induction of effective immunity against Mtb.
In either case, augmenting antimycobacterial immune response with booster doses of BCG could have been a potent strategy to enhance the anti-TB efficacy of BCG. However, repeated BCG administration has been found to not confer additional protection against pulmonary TB (although protection against leprosy is improved) (8, 9). Also, it is unlikely for the infants to receive significant EMb exposure before BCG vaccination, for they are immunized soon after birth. Moreover, the above hypotheses are based on animal studies, which have yielded contrasting result, with their applicability in humans difficult to verify (10–12). Therefore, why BCG fails to confer significant protection against adult pulmonary TB needs to be revisited.
TB is a heterogeneous disease occurring in different patterns and presentations (13). Two distinct presentations of TB can be seen in infants and immunocompetent adults (14, 15). In infants and young children, Mtb infection leads to primary disease with a high mortality rate. This form of disease commonly affects extra-pulmonary sites and in severe cases, can occur in disseminated form (miliary TB) (15). On the contrary, initial Mtb infection in immunocompetent adults is mostly contained as latent TB (LTB). This state of asymptomatic infection may persist lifelong in most but 5-10% of people, who would develop active disease during their lifetime (3). Active TB in immunocompetent adults preferentially affects lung tissue.
The primary and extra-pulmonary nature of TB in infants/young children is suggestive of their incompetence to contain Mtb. In keeping with this, immunological milieu in young children has been shown to be skewed towards the TH2 side, with dampened TH1-type of immunity and inflammatory pathways (16). Moreover, CD4+ T cells in young children are recent thymic emigrants with defective functionality and differentiation bias towards TH2 effector cells (17). That is why most children would respond poorly to mycobacterial antigens during tuberculin skin testing (TST) and IFN-γ release assay (IGRA) (15). On the other hand, immune system is effective against Mtb in most adults. These people mount a robust TH1 type of immune response to Mtb, resulting in sequestration of infected macrophages into lung granulomas and containment of infection as LTB (18). Paradoxically, host immune system has also been implicated in the reactivation of latent infection into active TB in immunocompetent adults (19).
Host response to Mtb begins with its recognition by resident lung macrophages which, along with dendritic cells, induce adaptive immune responses to bacilli. TH1-polarized CD4+ T cells are the key orchestrators of protective immunity against Mtb (18). Critical role of these cells in protection against Mtb is demonstrated by increased risk of TB in CD4+ T cell-lymphopenic HIV/AIDS patients. Similarly, CD4+ T cell depletion from animal models has been shown to increase the susceptibility to mycobacterial disease. Antimycobacterial functions of CD4+ T cells are partly mediated by TNF-α and IFN-γ, which induce bactericidal mechanisms in infected macrophages and facilitate their sequestration in the granulomas (3). Other cell types including CD8+ T cells, NK, and NKT cells have also been shown to contribute towards host resistance to Mtb (3).
LTB, which follows initial Mtb infection in most adults, is considered a state of protection against Mtb (20). It is characterized by a moderate anti-Mtb immune response in comparison to active pulmonary TB, wherein an aggravated antimycobacterial immunity is commonly observed (19). Heightened IFN-γ+CD4+ T-cell response to mycobacterial antigens, as evidenced by tuberculin reaction, IGRA, and in vitro assays, occurs frequently in active TB patients and is an important parameter distinguishing active TB from latent infection (21–24). Indeed, increased IFN-γ levels in different tissues is one of the most common and consistent observations during active TB (3). Importantly, IFN-γ levels directly correlate with TB severity and subside with its successful treatment, suggesting that mycobacterial burden could also contribute towards the aggravation of anti-Mtb immune responses (3). It is worth mentioning that host genetics also plays an important role in tuberculin sensitivity and some people may not exhibit tuberculin reactivity despite active disease (25, 26). Interestingly, these people have been shown to exhibit fewer symptoms but a more advanced disease (25).
TB-associated immune reconstitution inflammatory syndrome (TB-IRIS), which develops with the reactivation of asymptomatic Mtb infection in a subset of antiretroviral therapy (ART)-treated HIV-infected people, provides direct evidence for the pathological role of aggravated immunity during Mtb infection. ART-mediated decline in viral load results in the over-expansion of Mtb-specific CD4+ T cells in HIV and Mtb-coinfected patients (27). Lower baseline CD4+ T-cell count and rapid increase in these cells number with ART are the major risk factors for the development of TB-IRIS (28). Importantly, ART-treated people who are destined to develop TB-IRIS exhibit a more strong TH1-type of CD4+ T-cell response to Mtb antigens (27). Similar results have been obtained from animal studies, wherein the human condition was mimicked by adoptively transferring CD4+ T cells in M. avium-infected, TCRα−/− mice (29). After receiving CD4+ T cells, these animals developed an aggravated immune response to the bacilli, exhibited wasting, lost weight, and eventually died of exacerbated immunity (29). Interestingly, development of immune reconstitution disease in these animals was mediated by hyperactive IFN-γ+CD4+ T cells.
The course of Mtb infection in PD-1−/− mice also implicates aggravated CD4+ T-cell response in TB pathogenesis. PD-1 is a cell-surface receptor involved in the negative regulation of T-cell responses, and its deficiency results in significantly increased susceptibility to mycobacterial diseases, compared with wild-type mice (30, 31). Mechanistic studies have shown that PD-1−/− mice mount a hyperactive CD4+ T-cell responses to mycobacteria, which drive mycobacterial pathogenesis in these animals (32). In agreement with animal studies, PD-1 blockade in cancer patients (a type of immunotherapy) has resulted in multiple cases of TB (33). It is noteworthy that PD-1 blockade-mediated TB development is associated with increased frequency of Mtb-specific IFN-γ+CD4+ T cells (33).
Significantly higher risk of TB in the immunocompetent adults cured of its previous episode also implicates aggravated CD4+ T-cell responses in TB development (34). Mtb-specific CD4+ T-cell responses are elevated during active TB, and a proportion of these cells can persist as memory cells after successful treatment (35, 36). It has been estimated that ~70% of people cured of pulmonary TB exhibit positive tuberculin reaction and IGRA up to 30 years after treatment (37). With Mtb reinfection, these hosts are more likely to mount hyperactive CD4+ T-cell responses, leading to active TB.
The success of BCG against childhood TB and its variable efficacy against adult pulmonary disease are explained below by taking into account the heterogeneity of TB, protective versus pathological immunity during Mtb infection, and effect of EMb on anti-Mtb immune responses.
As discussed above, the immune system in children is poorly developed with dampened TH1 responses and inflammatory pathways (16). Infant CD4+ T cells exhibit defective functionality and differentiation bias towards TH2 effector cells (17). BCG vaccination alters the immune profile and promotes Mtb-specific TH1-polarized immune responses in these people (38). Vekemans et al. demonstrated that frequencies of IFN-γ-producing cells and the levels of IFN-γ produced in response to PPD in BCG-vaccinated infants are comparable with those in adults (39). As in the case of adults, CD4+ T lymphocytes are the main source of IFN-γ in BCG-vaccinated infants (39). Strong lympho-proliferative response and TH1 cytokine secretion in BCG-vaccinated infants have been demonstrated by the other researchers also (40, 41). BCG vaccination has also been shown to induce mycobacterium-specific cytotoxic T lymphocyte responses in neonates (40, 42). Soares et al. demonstrated that CD8+ T cells are an important source of IFN-γ, IL-2 and TNF-α in BCG-immunized infants (43). Besides CD4+ and CD8+ T cells, BCG can also activate unconventional γδ T cells, which play a bridging role between innate and adaptive immunity against Mtb and act as an important source of IFN-γ in vaccinated children (44, 45). In contrast to abundant TH1 cytokines, BCG-vaccinated newborns demonstrate relatively lower levels of IL-4 and IL-10 (43).
A few studies have also examined the status of immunological memory in BCG-vaccinated infants. It has been observed that newborn BCG vaccination leads to the development of memory CD4+ T-cell population with phenotypic characteristics of central memory cells and functional attributes of effector memory cells (46). In a follow-up study, levels of IFN-γ, IFN-γ+CD4+ T cells, and IFN-γ+ γδ T in <1-year-old BCG-vaccinated children were found to be comparable with those present at different time points till ≥5 years (47). Interestingly, Kagina et al. demonstrated a superior CD4+ T-cell memory response with BCG administered at 10 weeks (48). Owing to the induction of TH1-polarized responses and immunological memory by BCG, vaccinated infants are more likely to respond rapidly and optimally to Mtb infection, resulting in the effective containment of the bacilli. Accordingly, BCG confers significant protection against childhood manifestations of TB.
Host response to Mtb is more complex in adults. Although a small proportion of TB cases in them can be attributed to hereditary or acquired defects in immune system, a majority of adult pulmonary TB patients demonstrate an aggravated immune response to Mtb (23). Maximum burden of adult pulmonary TB lies in the tropics, which account for approximately 95% of TB cases and 98% of TB-related deaths (49). Importantly, people living in the tropics exhibit a stronger tuberculin reaction, compared with those in subtropical and temperate zones.
Higher prevalence of skin test reactivity to PPD-B (M. avium-intracellulare antigen) suggests the greater abundance of EMb in the tropics, compared with temperate zones. In the Chingleput trial area, for example, ~90% of 10 to 14-year-old participants exhibited strong reactivity to PPD-B (50). For they carry multiple cross-reactive antigens, EMb can directly modulate the host response to Mtb. Accordingly, a more strong response to Mtb is observed in the tropics and in elder people, who are more likely to have received greater EMb exposure, compared with younger ones. In the south India trial, 62.0%/48.4% tuberculin positivity (>12 mm with 3 IU of PPD-S) was observed in 15 to 24-year-old male/female participants, which reached 81.8%/64% in 25 to 30-year-old male/female participants, respectively (51). In Karonga prevention study, tuberculin positivity (skin induration of ≥10) was found to increase from ~20% in 20-year-old male participants to 60% in 45-year-old male participants (8). Similar results have been obtained in other studies in tropical Africa (52). On the other hand, no participants in the 5-14 years age group developed grade II or III Heaf reactions (with PPD-S) and only ~15%/~20% of 15 to 24-year-old participants exhibited grade II/grade III Heaf reactions, respectively, in a clinical study in the United Kingdom (53).
It can be inferred that, putatively due to frequent EMb exposure, a large proportion of immunocompetent adults mount a heightened immune response to mycobacterial antigens in the tropics. As an aggravated antimycobacterial immune response leads to TB pathogenesis, many of these people are bound to undergo LTB reactivation into the active disease. Supporting this, people with stronger tuberculin reaction have been found to carry a higher risk of active TB in different clinical trials. Similarly, elevated risk of active TB has been observed in household contacts (of active TB patients), who develop stronger tuberculin reactions (19, 23). Interestingly, male participants have exhibited a more intense tuberculin reaction in clinical studies, which correlates with the higher incidence of adult TB in them (8, 50). It is plausible that frequent EMb exposure and resulting augmentation of antimycobacterial immune response increases the risk of active TB by mechanisms analogous to those in people cured of the previous episode of the disease.
The host immunological differences also provide an explanation for the variable efficacy of BCG against pulmonary TB in tropics and temperate zones. As most immunocompetent adults would develop a heightened antimycobacterial immune response in the tropics, BCG, which acts by promoting TH1-type of anti-Mtb immunity, cannot be of much use against adult pulmonary TB in these regions. On the contrary, lesser abundant EMb has a limited effect on antimycobacterial immunity at higher latitudes. Therefore, BCG-mediated immune response persists without significant modulation and vaccinated people exhibit higher protection against adult pulmonary TB in these areas. Supporting this explanation, percentages of tuberculin reactors have been found to be low in the northern temperate countries where BCG confers significant protection, and high in tropical areas where BCG exhibits poor efficacy against adult TB (54). The effects of EMb on anti-Mtb immune response and efficacy of BCG are depicted in Figure 1. However, it is worth mentioning that factors such as malnourishment and air pollution can also modulate the host antimycobacterial immunity and play a role in defining the vaccine efficacy.
Figure 1 Effects of environmental mycobacteria (EMb) and BCG on the host response to Mtb and the cross-talk thereof. Host response to Mtb is complex and heterogeneous. Infants and young children have a poorly developed immune system, which is incompetent in containing Mtb infection. BCG vaccination in these people promotes TH1 responses to Mtb, resulting in the effective containment of the bacilli and significant protection against childhood TB (dashed blue and red lines). Owing to the presence of cross-reactive antigens, EMb also activate a degree of immunity against Mtb and therefore, confer some protection against childhood TB (blue line). However, frequent EMb exposure leads to the aggravation of anti-Mtb immunity in immunocompetent adults, which drives TB pathogenesis and results in higher incidence of pulmonary TB in the tEMb-abundant areas (normal blue line). Similar aggravation of anti-Mtb immunity occurs in BCG-vaccinated adults in the EMb-abundant areas and leads to higher incidence of adult pulmonary TB and low efficacy of BCG in these places (dashed blue line). On the other hand, owing to low EMb exposure, BCG-mediated immunity against Mtb is not substantially modulated in the adult inhabitants in the areas of lower EMb abundance (dashed red line). Accordingly, vaccinated adults exhibit a moderately intense anti-Mtb immune response, which confers significant protection against adult pulmonary TB in these areas.
Tuberculin reactions and TB incidence in rural versus urban population also provide firm support to the above explanation for the variable efficacy of BCG against pulmonary TB. Studies have shown that within the same geographical region, higher prevalence of non-specific tuberculin sensitivity is observed in the rural population (55–57). In an only trial of its kind, which compared vaccine efficacy in rural versus urban settings, BCG efficacy was found to be 18% in rural areas, compared with 42% in urban areas (58). In fact, lowest efficacy of BCG against pulmonary TB has been observed in the studies carried out in rural areas (50, 59, 60). It can be deduced that for aggravated antimycobacterial immunity drives pulmonary TB pathogenesis in the tropics, BCG (which acts by inducing anti-Mtb immune response) turns out to be ineffective against adult pulmonary TB in these areas.
TB is a heterogeneous disease occurring in different patterns/presentations with distinct mechanisms of pathogenesis (3). In infants and young children, the immune system is poorly developed with dampened TH1 responses and inflammatory pathways, rendering them susceptible to Mtb (16, 17). BCG vaccination promotes a TH1-type of anti-Mtb immunity in these people and therefore, confers significant protection against childhood TB (38). In immunocompetent adults, development of pulmonary TB has been attributed to an aggravated anti-Mtb immune responses (18, 19). Since a more intense antimycobacterial immune response is observed in the tropics, it is plausible that higher incidence of adult pulmonary TB would be reported from these areas. Also, BCG, which acts by inducing anti-Mtb immunity, would not be effective against pulmonary TB in these places. On the contrary, BCG-vaccinated people demonstrate a moderately intense antimycobacterial immune response at higher latitudes. For a moderate antimycobacterial response is protective against Mtb, BCG exhibits higher protective efficacy against adult TB in these areas. Firm support for the above explanation of the variable efficacy of BCG is provided by its effectiveness in rural versus urban settings (58). A more intense antimycobacterial immune response is observed in rural areas, wherein the efficacy of BCG has been found to be lower, compared with that in urban places (56, 57).
It can be proposed that a different vaccination approach against adult TB is required in the tropics. Most likely, an effective vaccine against adult TB in these areas would focus on moderating Mtb-specific IFN-γ+CD4+ T-cell responses and balancing pro- and anti-inflammatory pathways. That’s probably how we can prevent adult pulmonary TB and save millions of lives.
The original contributions presented in the study are included in the article/supplementary material. Further inquiries can be directed to the corresponding author.
The author confirms being the sole contributor of this work and has approved it for publication.
The author declares that the research was conducted in the absence of any commercial or financial relationships that could be construed as a potential conflict of interest.
All claims expressed in this article are solely those of the authors and do not necessarily represent those of their affiliated organizations, or those of the publisher, the editors and the reviewers. Any product that may be evaluated in this article, or claim that may be made by its manufacturer, is not guaranteed or endorsed by the publisher.
TB, tuberculosis; Mtb, Mycobacterium tuberculosis; BCG, Bacillus Calmette-Guerin; EMb, Environmental mycobacteria; LTB, latent tuberculosis; IFN, interferon.
1. Andersen P, Doherty TM. Opinion: The Success and Failure of BCG–Implications for a Novel Tuberculosis Vaccine. Nature Reviews. Microbiology (2005) 3:656. doi: 10.1038/nrmicro1211
2. Dockrell HM, Smith SG. What Have We Learnt About BCG Vaccination in the Last 20 Years? Front Immunol (2017) 8:1134. doi: 10.3389/fimmu.2017.01134
3. O’Garra A, Redford PS, McNab FW, Bloom CI, Wilkinson RJ, Berry MP. The Immune Response in Tuberculosis. Annu Rev Immunol (2013) 31:475–527. doi: 10.1146/annurev-immunol-032712-095939
5. Fine PE. Variation in Protection by BCG: Implications of and for Heterologous Immunity. Lancet (1995) 346:1339–45. doi: 10.1016/S0140-6736(95)92348-9
6. Palmer CE, Long MW. Effects of Infection With Atypical Mycobacteria on BCG Vaccination and Tuberculosis. Am Rev Respir Dis (1966) 94:553–68. doi: 10.1164/arrd.1966.94.4.553
7. Brandt L, Cunha JF, Olsen AW, Chilima B, Hirsch P, Appelberg R, et al. Failure of the Mycobacterium Bovis BCG Vaccine: Some Species of Environmental Mycobacteria Block Multiplication of BCG and Induction of Protective Immunity to Tuberculosis. Infect Immun (2002) 70:672–8. doi: 10.1128/IAI.70.2.672-678.2002
8. Crampin AC, Glynn JR, Fine PE. What has Karonga Taught Us? Tuberculosis Studied Over Three Decades. Int J Tuberc Lung Dis: Off J Int Union Against Tuberc Lung Dis (2009) 13:153–64.
9. Glynn JR, Fielding K, Mzembe T, Sichali L, Banda L, McLean E, et al. BCG Re-Vaccination in Malawi: 30-Year Follow-Up of a Large, Randomised, Double-Blind, Placebo-Controlled Trial. Lancet Global Health (2021) 9:e1451–9. doi: 10.1016/S2214-109X(21)00309-0
10. Howard CJ, Kwong LS, Villarreal-Ramos B, Sopp P, Hope JC. Exposure to Mycobacterium Avium Primes the Immune System of Calves for Vaccination With Mycobacterium Bovis BCG. Clin Exp Immunol (2002) 130:190–5. doi: 10.1046/j.1365-2249.2002.01987.x
11. Thom M, Howard C, Villarreal-Ramos B, Mead E, Vordermeier M, Hope J. Consequence of Prior Exposure to Environmental Mycobacteria on BCG Vaccination and Diagnosis of Tuberculosis Infection. Tuberc (Edinburgh Scotland) (2008) 88:324–34. doi: 10.1016/j.tube.2007.12.002
12. Demangel C, Garnier T, Rosenkrands I, Cole ST. Differential Effects of Prior Exposure to Environmental Mycobacteria on Vaccination With Mycobacterium Bovis BCG or a Recombinant BCG Strain Expressing RD1 Antigens. Infect Immun (2005) 73:2190–6. doi: 10.1128/IAI.73.4.2190-2196.2005
13. Lenaerts A, Barry CE, Dartois V. Heterogeneity in Tuberculosis Pathology, Microenvironments and Therapeutic Responses. Immunol Rev (2015) 264:288–307. doi: 10.1111/imr.12252
14. Alcaïs A, Fieschi C, Abel L, Casanova J-L. Tuberculosis in Children and Adults. J Exp Med (2005) 202:1617–21. doi: 10.1084/jem.20052302
15. Marais BJ, Schaaf HS. Tuberculosis in Children. Cold Spring Harbor Perspect Med (2014) 4:a017855. doi: 10.1101/cshperspect.a017855
16. Saso A, Kampmann B. Vaccine Responses in Newborns. Semin Immunopathol (2017) 39:627–42. doi: 10.1007/s00281-017-0654-9
17. White GP, Watt PM, Holt BJ, Holt PG. Differential Patterns of Methylation of the IFN-Gamma Promoter at CpG and Non-CpG Sites Underlie Differences in IFN-Gamma Gene Expression Between Human Neonatal and Adult CD45RO- T Cells. J Immunol (Baltimore Md: 1950) (2002) 168:2820–7. doi: 10.4049/jimmunol.168.6.2820
18. Kumar P. Ifnγ-Producing CD4+ T Lymphocytes: The Double-Edged Swords in Tuberculosis. Clin Trans Med (2017) 6:1–7. doi: 10.1186/s40169-017-0151-8
19. Kumar P. Adult Pulmonary Tuberculosis as a Pathological Manifestation of Hyperactive Antimycobacterial Immune Response. Clin Trans Med (2016) 5:38. doi: 10.1186/s40169-016-0119-0
20. Mack U, Migliori G, Sester M, Rieder H, Ehlers S, Goletti D, et al. LTBI: Latent Tuberculosis Infection or Lasting Immune Responses to M. Tuberculosis? A TBNET Consensus Statement. Eur Respir J (2009) 33:956–73. doi: 10.1183/09031936.00120908
21. Reichler MR, Khan A, Sterling TR, Zhao H, Moran J, McAuley J, et al. Risk and Timing of Tuberculosis Among Close Contacts of Persons With Infectious Tuberculosis. J Infect Dis (2018) 218:1000–8. doi: 10.1093/infdis/jiy265
22. Doherty TM, Demissie A, Olobo J, Wolday D, Britton S, Eguale T, et al. Immune Responses to the Mycobacterium Tuberculosis-Specific Antigen ESAT-6 Signal Subclinical Infection Among Contacts of Tuberculosis Patients. J Clin Microbiol (2002) 40:704–6. doi: 10.1128/JCM.40.2.704-706.2002
23. North RJ, Jung Y-J. Immunity to Tuberculosis. Annu Rev Immunol (2004) 22:599–623. doi: 10.1146/annurev.immunol.22.012703.104635
24. Jenkins AO, Michel A, Rutten V. Original Mycobacterial Sin, A Consequence of Highly Homologous Antigens? Vet Microbiol (2017) 203:286–93. doi: 10.1016/j.vetmic.2017.03.028
25. Maher J, Kelly P, Hughes P, Clancy L. Skin Anergy and Tuberculosis. Respir Med (1992) 86:481–4. doi: 10.1016/S0954-6111(96)80006-0
26. Cobat A, Poirier C, Hoal E, Boland-Auge A, de la Rocque F, Corrard F, et al. Tuberculin Skin Test Negativity Is Under Tight Genetic Control of Chromosomal Region 11p14-15 in Settings With Different Tuberculosis Endemicities. J Infect Dis (2015) 211:317–21. doi: 10.1093/infdis/jiu446
27. Bourgarit A, Carcelain G, Martinez V, Lascoux C, Delcey V, Gicquel B, et al. Explosion of Tuberculin-Specific Th1-Responses Induces Immune Restoration Syndrome in Tuberculosis and HIV Co-Infected Patients. Aids (2006) 20:F1–7. doi: 10.1097/01.aids.0000202648.18526.bf
28. Xue M, Xie R, Pang Y, Yan S, Du Y, Guan C, et al. Prevalence and Risk Factors of Paradoxical Tuberculosis Associated Immune Reconstitution Inflammatory Syndrome Among HIV-Infected Patients in Beijing, China. BMC Infect Dis (2020) 20:554. doi: 10.1186/s12879-020-05225-x
29. Barber DL, Mayer-Barber KD, Antonelli LR, Wilson MS, White S, Caspar P, et al. Th1-Driven Immune Reconstitution Disease in Mycobacterium Avium–Infected Mice. Blood (2010) 116:3485–93. doi: 10.1182/blood-2010-05-286336
30. Jin HT, Ahmed R, Okazaki T. Role of PD-1 in Regulating T-Cell Immunity. Curr Topics Microbiol Immunol (2011) 350:17–37. doi: 10.1007/82_2010_116
31. Lázár-Molnár E, Chen B, Sweeney KA, Wang EJ, Liu W, Lin J, et al. Programmed Death-1 (PD-1)–Deficient Mice Are Extraordinarily Sensitive to Tuberculosis. Proc Natl Acad Sci (2010) 107:13402–7. doi: 10.1073/pnas.1007394107
32. Sakai S, Kauffman KD, Sallin MA, Sharpe AH, Young HA, Ganusov VV, et al. CD4 T Cell-Derived IFN-γ Plays a Minimal Role in Control of Pulmonary Mycobacterium Tuberculosis Infection and Must be Actively Repressed by PD-1 to Prevent Lethal Disease. PloS Pathog (2016) 12:e1005667. doi: 10.1371/journal.ppat.1005667
33. Barber DL, Sakai S, Kudchadkar RR, Fling SP, Day TA, Vergara JA, et al. Tuberculosis Following PD-1 Blockade for Cancer Immunotherapy. Sci Trans Med (2019) 11(475):eaat2702. doi: 10.1126/scitranslmed.aat2702
34. Verver S, Warren RM, Beyers N, Richardson M, van der Spuy GD, Borgdorff MW, et al. Rate of Reinfection Tuberculosis After Successful Treatment Is Higher Than Rate of New Tuberculosis. Am J Respir Crit Care Med (2005) 171:1430–5. doi: 10.1164/rccm.200409-1200OC
35. Tapaninen P, Korhonen A, Pusa L, Seppälä I, Tuuminen T. Effector Memory T-Cells Dominate Immune Responses in Tuberculosis Treatment: Antigen or Bacteria Persistence? Int J Tuberc Lung Dis (2010) 14:347–55.
36. Wu-Hsieh BA, Chen C-K, Chang J-H, Lai S-Y, Wu CH, Cheng W-C, et al. Long-Lived Immune Response to Early Secretory Antigenic Target 6 in Individuals Who had Recovered From Tuberculosis. Clin Infect Dis (2001) 33:1336–40. doi: 10.1086/323044
37. Seo KW, Ahn J-J, Ra SW, Kwon W-J, Jegal Y. Persistently Retained Interferon-Gamma Responsiveness in Individuals With a History of Pulmonary Tuberculosis. Tohoku J Exp Med (2014) 233:123–8. doi: 10.1620/tjem.233.123
38. Marchant A, Goetghebuer T, Ota MO, Wolfe I, Ceesay SJ, De Groote D, et al. Newborns Develop a Th1-Type Immune Response to Mycobacterium Bovis Bacillus Calmette-Guerin Vaccination. J Immunol (Baltimore Md: 1950) (1999) 163:2249–55.
39. Vekemans J, Amedei A, Ota MO, D’Elios MM, Goetghebuer T, Ismaili J, et al. Neonatal Bacillus Calmette-Guerin Vaccination Induces Adult-Like IFN-Gamma Production by CD4+ T Lymphocytes. Eur J Immunol (2001) 31:1531–5. doi: 10.1002/1521-4141(200105)31:5<1531::AID-IMMU1531>3.0.CO;2-1
40. Hussey GD, Watkins ML, Goddard EA, Gottschalk S, Hughes EJ, Iloni K, et al. Neonatal Mycobacterial Specific Cytotoxic T-Lymphocyte and Cytokine Profiles in Response to Distinct BCG Vaccination Strategies. Immunology (2002) 105:314–24. doi: 10.1046/j.1365-2567.2002.01366.x
41. Ota MO, Vekemans J, Schlegel-Haueter SE, Fielding K, Sanneh M, Kidd M, et al. Influence of Mycobacterium Bovis Bacillus Calmette-Guérin on Antibody and Cytokine Responses to Human Neonatal Vaccination. J Immunol (Baltimore Md: 1950) (2002) 168:919–25. doi: 10.4049/jimmunol.168.2.919
42. Murray RA, Mansoor N, Harbacheuski R, Soler J, Davids V, Soares A, et al. Bacillus Calmette Guerin Vaccination of Human Newborns Induces a Specific, Functional CD8+ T Cell Response. J Immunol (2006) 177:5647–51. doi: 10.4049/jimmunol.177.8.5647
43. Soares AP, Scriba TJ, Joseph S, Harbacheuski R, Murray RA, Gelderbloem SJ, et al. Bacillus Calmette-Guérin Vaccination of Human Newborns Induces T Cells With Complex Cytokine and Phenotypic Profiles. J Immunol (Baltimore Md: 1950) (2008) 180:3569–77. doi: 10.4049/jimmunol.180.5.3569
44. Zufferey C, Germano S, Dutta B, Ritz N, Curtis N. The Contribution of non-Conventional T Cells and NK Cells in the Mycobacterial-Specific Ifnγ Response in Bacille Calmette-Guérin (BCG)-Immunized Infants. PloS One (2013) 8:e77334. doi: 10.1371/journal.pone.0077334
45. Meraviglia S, El Daker S, Dieli F, Martini F, Martino A. γδ T Cells Cross-Link Innate and Adaptive Immunity in Mycobacterium Tuberculosis Infection. Clin Dev Immunol (2011) 2011:587315. doi: 10.1155/2011/587315
46. Soares AP, Kwong Chung CK, Choice T, Hughes EJ, Jacobs G, van Rensburg EJ, et al. Longitudinal Changes in CD4(+) T-Cell Memory Responses Induced by BCG Vaccination of Newborns. J Infect Dis (2013) 207:1084–94. doi: 10.1093/infdis/jis941
47. Whittaker E, Nicol MP, Zar HJ, Tena-Coki NG, Kampmann B. Age-Related Waning of Immune Responses to BCG in Healthy Children Supports the Need for a Booster Dose of BCG in TB Endemic Countries. Sci Rep (2018) 8:15309. doi: 10.1038/s41598-018-33499-4
48. Kagina BM, Abel B, Bowmaker M, Scriba TJ, Gelderbloem S, Smit E, et al. Delaying BCG Vaccination From Birth to 10 Weeks of Age May Result in an Enhanced Memory CD4 T Cell Response. Vaccine (2009) 27:5488–95. doi: 10.1016/j.vaccine.2009.06.103
49. Zammarchi L, Bartalesi F, Bartoloni A. Tuberculosis in Tropical Areas and Immigrants. Mediterr J Hematol Infect Dis (2014) 6:e2014043. doi: 10.4084/mjhid.2014.043
51. Trial TP. Trial of BCG Vaccines in South India for Tuberculosis Prevention: First Report. Bull World Health Organ (1979) 57:819–27.
52. Roelsgaard E, Iversen E, Blocher C. Tuberculosis in Tropical Africa. An Epidemiological Study. Bull World Health Organ (1964) 30:459–518.
53. Capewell S, Leitch AG. Tuberculin Reactivity in a Chest Clinic: The Effects of Age and Prior BCG Vaccination. Br J Dis Chest (1986) 80:37–44. doi: 10.1016/0007-0971(86)90007-0
54. Nyboe J. The Efficacy of the Tuberculin Test: An Analysis Based on Results From 33 Countries. Bull World Health Organ (1960) 22:5–37.
55. Edwards LB, Acquaviva FA, Livesay VT, Cross FW, Palmer CE. An Atlas of Sensitivity to Tuberculin, PPD-B, and Histoplasmin in the United States. Am Rev Respir Dis (1969) 99:Suppl:1–132.
56. Paramasivan CN, Govindan D, Prabhakar R, Somasundaram PR, Subbammal S, Tripathy SP. Species Level Identification of Non-Tuberculous Mycobacteria From South Indian BCG Trial Area During 1981. Tubercle (1985) 66:9–15. doi: 10.1016/0041-3879(85)90048-0
57. Doocy SC, Todd CS, Llainez YB, Ahmadzai A, Burnham GM. Population-Based Tuberculin Skin Testing and Prevalence of Tuberculosis Infection in Afghanistan. World Health Popul (2008) 10:44–53. doi: 10.12927/whp.2008.19785
58. Comstock GW, Livesay VT, Woolpert SF. Evaluation of BCG Vaccination Among Puerto Rican Children. Am J Public Health (1974) 64:283–91. doi: 10.2105/AJPH.64.3.283
59. Pönnighaus JM, Fine PE, Sterne JA, Wilson RJ, Msosa E, Gruer PJ, et al. Efficacy of BCG Vaccine Against Leprosy and Tuberculosis in Northern Malawi. Lancet (London England) (1992) 339:636–9. doi: 10.1016/0140-6736(92)90794-4
Keywords: tuberculosis, BCG, vaccine efficacy, immune response, environmental mycobacteria, heterogeneity, geographical latitude
Citation: Kumar P (2021) A Perspective on the Success and Failure of BCG. Front. Immunol. 12:778028. doi: 10.3389/fimmu.2021.778028
Received: 16 September 2021; Accepted: 18 November 2021;
Published: 14 December 2021.
Edited by:
Juraj Ivanyi, King’s College London, United KingdomReviewed by:
Warwick Britton, The University of Sydney, AustraliaCopyright © 2021 Kumar. This is an open-access article distributed under the terms of the Creative Commons Attribution License (CC BY). The use, distribution or reproduction in other forums is permitted, provided the original author(s) and the copyright owner(s) are credited and that the original publication in this journal is cited, in accordance with accepted academic practice. No use, distribution or reproduction is permitted which does not comply with these terms.
*Correspondence: Pawan Kumar, cGtzYmlvMzBAZ21haWwuY29t
Disclaimer: All claims expressed in this article are solely those of the authors and do not necessarily represent those of their affiliated organizations, or those of the publisher, the editors and the reviewers. Any product that may be evaluated in this article or claim that may be made by its manufacturer is not guaranteed or endorsed by the publisher.
Research integrity at Frontiers
Learn more about the work of our research integrity team to safeguard the quality of each article we publish.