- 1Division of Experimental Hematology and Cancer Biology, Cincinnati Children’s Medical Center, Cincinnati, OH, United States
- 2Department of Pediatrics, University of Cincinnati College of Medicine, Cincinnati, OH, United States
The shape and spatial organization -the anatomy- of a tissue profoundly influences its function. Knowledge of the anatomical relationships between parent and daughter cells is necessary to understand differentiation and how the crosstalk between the different cells in the tissue leads to physiological maintenance and pathological perturbations. Blood cell production takes place in the bone marrow through the progressive differentiation of stem cells and progenitors. These are maintained and regulated by a heterogeneous microenvironment composed of stromal and hematopoietic cells. While hematopoiesis has been studied in extraordinary detail through functional and multiomics approaches, much less is known about the spatial organization of blood production and how local cues from the microenvironment influence this anatomy. Here, we discuss some of the studies that revealed a complex anatomy of hematopoiesis where discrete local microenvironments spatially organize and regulate specific subsets of hematopoietic stem cells and/or progenitors. We focus on the open questions in the field and discuss how new tools and technological advances are poised to transform our understanding of the anatomy of hematopoiesis.
Introduction
The bone marrow tissue provides a unique microenvironment -composed of both hematopoietic and non-hematopoietic cells and extracellular matrix- that cooperate to accomplish several functions: promote stem cell and multipotent progenitor self-renewal, regulate the differentiation of each lineage, and provide structural support and spatial organization to the tissue. The microenvironment is defined by three large structures: the bone tissue that encloses the marrow; a vascular network, composed of arterioles that penetrate through the bone and give rise to a large sinusoidal network that drains through a central vein; and a network of reticular stromal cells that wraps around the different vessels. These structures cooperate with and regulate each other to maintain the tissue (1).
Many other cell types regulate -directly or indirectly- hematopoiesis and are thus considered part of the microenvironment. Non-hematopoietic cells include osteoblastic precursors, osteoblasts, osteocytes, adipocytes, Schwann cells, sympathetic and sensory nerves, and fibroblasts. Hematopoietic components include macrophages, megakaryocytes, myeloid cells, and dendritic cells. In-depth discussions on how these cells were recognized as components of the microenvironment and their precise role on hematopoiesis are available elsewhere (1–3). The components of the microenvironment are not evenly distributed through the bone marrow. As a result the microenvironment is spatially heterogeneous. Different regions of the bone marrow contain specialized microenvironments that organize hematopoiesis and regulate unique progenitors, cell types, and blood lineages. The next section discusses the evidence demonstrating that local microenvironments dictate the anatomy of hematopoiesis.
The Anatomy of Hematopoiesis Is Spatially Organized by Local Microenvironments
Spatial Organization of Hematopoietic Stem Cells and Their Niches
The discovery of the SLAM markers allowed imaging of HSC (defined as Lin-CD48-CD41-CD150+ cells) for the first time. This first study showed that most HSC were in perivascular location -in contrast to the established paradigm that stated that HSC were enriched in endosteal regions (4). It also paved the way for many other studies that used imaging to identify proximity between candidate niche cells and HSC and then a functional role for the niche cell was confirmed by genetic loss of function experiments (5–10). The composition, spatial organization, and function of HSC niches has been reviewed in detail elsewhere (1–3). Due to the sheer abundance of sinusoids and perivascular cells in the bone marrow virtually all (99%) hematopoietic cells –including HSC– localize within 30μm sinusoids or perivascular stromal cells (8, 11, 12). Both cell types are key regulators of HSC function (1, 3). Additionally, small subsets of HSC also localize near arterioles and/or the endosteum. Myeloid-biased HSC (detected using von Willebrand factor reporter mice) selectively localized near megakaryocytes -a key niche component that promotes HSC quiescence (9, 13, 14)- in the sinusoids. In contrast, lymphoid-biased HSC selectively localized near arterioles (15). Depletion of megakaryocytes led to expansion of myeloid biased HSC through loss of quiescence while lymphoid-biased HSC were unaffected. Similarly, depletion of Ng2+ periarteriolar stromal cells led to loss of the lymphoid-biased HSC (15). Other studies showed that the fraction of HSC with lowest levels of reactive oxygen species was enriched near arterioles (16); that increases in arteriole numbers also cause increases in HSC frequency (17); and that Ng2+ periarteriolar cells support HSC function (6). Together these results support the concept that sinusoids and megakaryocytes provide a niche for myeloid-biased LT-HSC whereas arterioles provide a niche for lymphoid-biased HSC. There is also evidence supporting the existence of an endosteal HSC niche that promotes regeneration. Imaging of fluorescently labeled HSC shortly after transplantation showed that the donor HSC are selectively enriched near the endosteal surface (18–20). Studies from the Li lab propose that CD49b- cells represent a small subset of HSC that selectively amplifies in the endosteum -supported by N-cadherin+ stromal cells- in response to chemotherapy (21). In agreement, live imaging analyses showed that a rare HSC subset (MFG HSC) localized and amplified near the endosteum after chemotherapy treatment (22).
It is important to note that although most studies agree with the overall distribution described above there are ongoing controversies regarding whether some HSC selectively localize – and are maintained- by arteriolar and endosteal niches (8, 23); whether HSC localization to different niche components is selective or random [and thus controlled by the relative abundance of each niche component (12)]; and about the motility of HSC in live imaging analyses (22, 24). These are likely because each group has used different cell surface markers, transgenic reporters, and statistical approaches to identify HSC and niche cells and to test for spatial relationships between these cells.
Spatial Organization of Hematopoietic Progenitors Downstream of HSC: Role of the Microenvironment
HSC give rise to several types of multipotent (MPP) and oligopotent progenitors (25–27). The localization of these cells in the microenvironment and whether they map near HSC and their niches is controversial. Early studies relied on short-term tracking of fluorescently-labeled MPP and HSC adoptively transplanted into non-myeloablated recipients. These revealed that the transplanted HSC and MPP did not overlap and that MPP localized further away from the endosteum than HSC (19). Much more recently, the Camargo lab generated Mds1GFP+ and Mds1GFP+Flt3-cre mice to differentially image subsets of multipotent progenitors and HSC. In the Mds1GFP+ mice GFP labels almost all HSC and subsets of MPP. In the Mds1GFP+Flt3-cre mice constitutive cre-mediated deletion of the floxed gfp allele restricts GFP expression to a small subset of HSC. They found that GFP+ cells in Mds1GFP+ mice were closer to transition zone vessels and farther away from the endosteum when compared to GFP+ cells in the Mds1GFP+Flt3-cre mice. This suggests that MPP and HSC reside in different microenvironments (22). The Pereira lab defined multipotent progenitors as Lin-CD41-CD48-cKIT-CD150-FLT3+ [which corresponds to the MPP4 subset (25)] and found a similar spatial distribution and interaction with perivascular stromal cells as HSC suggesting that they occupy the same niches (28). The differences between these studies are likely due to the different mouse reporters and methods used to image the multipotent progenitors.
It is likely that multipotent progenitors and lineage-committed progenitors do not overlap. In vivo imaging of adoptively transferred multipotent (Lineage-Sca1+c-kit+) or lineage-committed (Lineage-Sca1-c-kit+) progenitors into non-myeloablated recipients showed that both cells did not cluster and remained largely immobile while contacting the surrounding microenvironment (29). This suggested the existence of discrete niches for multipotent and lineage-committed progenitors. The existence of a distinct niche for erythropoiesis comes from classical electron microscopy studies that showed that rare macrophages, adjacent to sinusoids, provide a niche for islands of erythroblast maturation (30), and these have been the focus of many studies in the field (31). More recently, Comazzetto et al., demonstrated imaging of unipotent erythroid progenitors and showed that they selectively localize next to perivascular stromal cells that maintain them via SCF production (32). These indicate that erythropoiesis takes place in the sinusoids.
Herault et al., imaged Lineage-Sca1-CD150-c-kit+FcγR+ committed myeloid progenitors (33). These are a mixed population, containing granulocyte monocyte progenitors, and unipotent monocyte or neutrophil progenitors (34, 35). These myeloid progenitors were found as single cells evenly distributed through the bone marrow. In response to inflammation they formed large clusters that required signals provided by megakaryocytes to emerge (33). We recently developed strategies to image granulocyte progenitors, monocyte progenitors, monocyte dendritic cell progenitors (MDP) and most steps of terminal myeloid cell production (36). We found that myeloid progenitors do not colocalize with each other or HSC. Instead, they spatially segregate and attach to different sinusoids –away from arterioles and the endosteum– where they cluster with differentiated cells: granulocyte progenitors give rise and cluster with preneutrophils, monocyte progenitors cluster with Ly6Chi monocytes, and MDP cluster with dendritic cells and Ly6Clo non-classical monocytes. CSF1 is a key cytokine required for monocyte and dendritic cell production (37). When searching for microenvironmental signals that regulate this distribution we noticed that dendritic cells -which cluster with MDP- selectively localized to a rare subset of CSF1+ sinusoids (8% of all vessels). Conditional Csf1 deletion in the vasculature led to loss of MDP, dendritic cells, and non-classical monocytes. The surviving MDP no longer attached to sinusoids nor formed clusters with dendritic cell or monocytes. These demonstrated that myelopoiesis is spatially organized by signals produced by discrete sinusoids and that CSF1+ sinusoids provide a unique microenvironment for dendritic cell production (36).
Several studies indicate that B cell differentiation is spatially organized and regulated by the microenvironment [for a recent review see (38)]. Common lymphoid progenitors distribute between the endosteum and arterioles and are maintained by CXCL12 produced by osteoblastic cells (targeted using Col2.3-cre or Osx-cre mice) and stem cell factor produced by osteolectin+ periarteriolar stromal cells (39–41). Cordeiro-Gomes found that Ly6D+ common lymphoid progenitors were also in contact with -and maintained by- a subset of IL7-producing perivascular stromal cells but it is not clear whether these stromal cells are evenly distributed through the bone marrow or enriched in specific locations (28). Interestingly, subsets of stromal cells predicted to support lymphopoiesis selectively localize near the growth plate and trabecular regions (42). The Nagasawa lab showed that most Pre-pro-B cells are in contact with CXCL12-producing stromal reticular stromal cells but did not localize near IL7-producing reticular cells. In contrast most Pro-B cells did not contact CXCL12 producing cells but localized near IL7 producing cells (43). Mandal et al., showed that Pre-B cells and Immature B cells selectively localize near IL-7-CXCL12+ reticular cells and that CXCR4 (the ligand for CXCL12) was necessary for Pre-B cell differentiation (44). Yu et al., demonstrated that deletion of IFG1 in Osterix+ progenitors using Osx-cre mice did not affect common lymphoid progenitors but led to arrest of B-cell development at the Pro-B stage (45). Fistonich et al., found that approximately 50% of ProB cells were in contact with IL7+CXCL12+ reticular cells that simultaneously contacted with a PreB cell. This suggested an overlapping niche for these two populations (46). Interestingly, live imaging showed that while ProB cells are largely static and remain attached to the CXCL12+ reticular cells whereas PreB cells migrate between different reticular cells (46). Together these studies strongly suggest that -as B cell progenitors differentiate- they migrate between subsets of stromal cells producing different amounts of IL7, CXCL12, or IGF1. In contrast, the Mancini lab found that most reticular cells coexpress IL7, CXCL12 and LepR, that ProB cells localize near LepR+, and that ~15% of HSC colocalize with Pro-B cells –much higher than predicted from random distributions. They also identified Nidogen-1 as niche derived factor regulating lymphopoiesis (47). Since HSC map near IL7 producing cells (28) these suggest that HSC and B cell lymphopoiesis share overlapping niches.
Open Questions and Future Developments
The studies above demonstrate that the bone marrow is a complex organ with a unique spatial architecture where specific lineages are supported by discrete regions of the bone marrow (Figure 1). The studies also lead to new questions and reveal major gaps in our understanding of how spatial relationships regulate hematopoiesis.
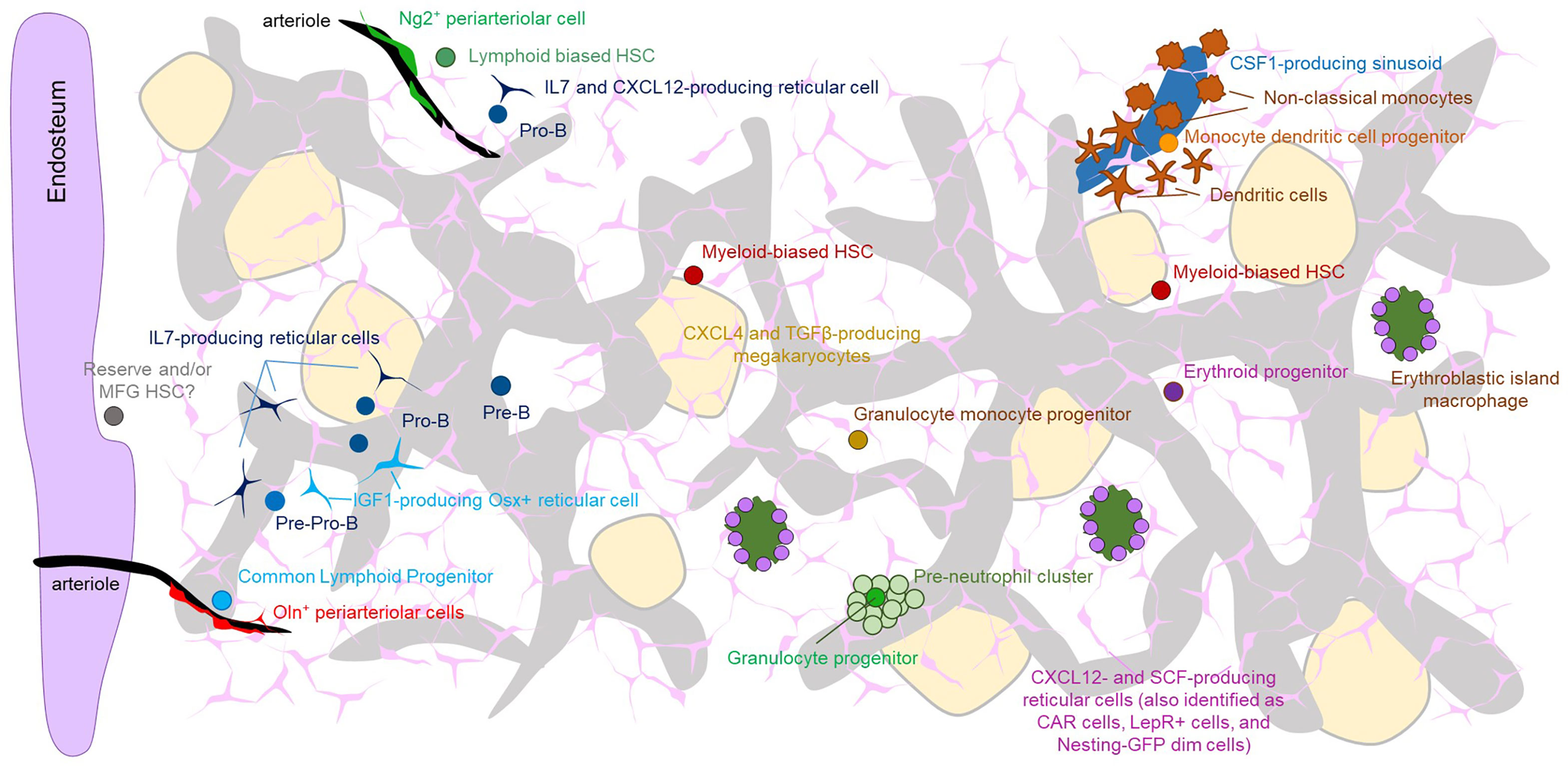
Figure 1 The Figure shows the overall architecture of the microenvironment in the bone marrow as well and the localization of the indicated progenitors with specific microenvironment. Note that because of the abundance of sinusoids and CXCL12- and SCF-producing perivascular cells virtually all cells are proximal to both of these structures. Also note that most types of stem cells and progenitors have been imaged with a limited number of partner cells. Therefore, it is likely that some of the structures depicted overlap (e.g. both erythroid progenitors and HSC have been shown to localize to SCF-producing perivascular cells). The precise location of most multi and oligopotent progenitors remains unknown.
What Is the Anatomy of Stepwise Hematopoiesis in the Steady-State?
Hematopoiesis occurs via stepwise differentiation of progenitors. However, it has not been possible to map the location of many progenitor populations – including different subsets of multipotent progenitors, common myeloid progenitors, and megakaryocyte erythroid progenitors. Additionally, for most progenitors, it has not been possible to simultaneously image multiple types of progenitors. Therefore, it is not known whether different types of progenitors share the same niche (and are likely regulated by the same cells and structures) or different niches (which will suggest differential regulation). The main reasons limiting studies to answer these questions are technological. For example, progenitor populations can be routinely defined using complex multicolor flow cytometry panels (25–27). However, most confocal microscopes can only resolve a much more limited number of fluorescent channels. Additionally, scRNAseq studies demonstrated that many of the different flow gates used to prospectively isolate the different progenitors contain heterogeneous populations [e.g., heterogeneity of myeloid progenitors (34)]. Precise mapping of the different steps of blood maturation will require developing approaches to define each type of progenitor by using fewer fluorescence channels as done recently for stepwise mapping of myelopoiesis (36). Alternatively, it might be possible to adapt iterative imaging methods. In these, the samples are stained and imaged with a set of fluorescent probes followed by removal of the fluorescence and staining and imaging with new fluorescent probes. Two of these methods, CODEX and IBEX, are able to resolve dozens of parameters using confocal microscopy (48, 49).
Precise mapping of differentiation will also require clonal fate-mapping to determine developmental relationships between progenitors and adjacent cells. Different studies have used confetti mice [in which cre recombination leads to expression of one out of four fluorescent proteins (50) to examine clonal relationships between cells of interest in the marrow (22, 51)]. However, the confetti model only allows simultaneous detection of a very limited number of fluorescent tags in discrete progenitor populations. A possible way of overcoming this limitation is single-cell spatial transcriptomics, which is developing at a breakneck pace. It might soon be possible to obtain transcriptomic data, track thousands of barcodes for clonal analyses, and obtain spatial information for single cells in the bone marrow (52).
What Are the Cells and Extracellular Matrix Structures Forming These Specialized Microenvironments and How Do They Function?
Answering these might require microdissection of the region of interest followed by transcriptomics analyses to interrogate the identity of the local cells. This technology is already available as shown by a study from the Van Galen lab demonstrating heterogeneity of growth factor production in different regions of the bone marrow (53).
After identification of the components of each local microenvironment the next step will be defining how they function in regulating the proximal progenitors. This has been accomplished by conditional Cre-mediated deletion of one cytokine or growth factor in the candidate cells. However, scRNAseq revealed extraordinary complexity of stromal cell types (53–55) whereas common Cre drivers available to the field target broad, heterogeneous, populations of stromal cells (55, 56). Development of new CreERT2 mouse models, specific for cells in local microenvironments -as done recently with Oln-creERT mice to target the periarteriolar stromal cells that maintain common lymphoid progenitors (41)- will greatly facilitate answering these questions.
If Local Microenvironments Regulate Unique Stem/Progenitors What Regulates Progenitor Localization to These Structures?
One possibility is that the specialized microenvironment produces one or more chemotactic cues that selectively attract the desired progenitor. Alternatively, this process might be stochastic with progenitors migrating through the bone marrow transiently interacting with stromal components. This type of transient interactions was shown recently for HSC (24). Eventually, one of these interactions will be of sufficient strength and specificity to retain the progenitor in a specific microenvironment. In this case the relative abundance of each local microenvironment will profoundly influence the likelihood of successful interactions. A third possibility is that the stem/progenitors themselves remodel local cells into a supportive microenvironment. This type of remodeling has been shown to occur in the zebrafish HSC niche (57). Distinguishing between these possibilities will require live imaging of specific subsets of progenitors. The major technical constrain will likely be the development of fluorescent reporter strains to allow visualization of unique progenitor subsets. Live bone marrow imaging has been done in the mouse calvarium [where the bone is thin enough to allow imaging with sufficient resolution (19, 22)] or by carving a “window” in leg bones to image the marrow within (24, 29, 58). Importantly, recent studies have shown differences in the frequencies of erythroid and lymphoid progenitors across different bones (42, 59). These would have to be considered when deciding which bones to study via live imaging.
What Is the Anatomy of Hematopoiesis and Local Microenvironment in Response to Insults?
Hematopoiesis is highly plastic and capable of sensing different insults and respond by quickly adjusting blood cell production to demand. Examples of this plasticity include hemorrhage which triggers emergency red blood cell production and infection which -depending on the infectious agent- can trigger emergency neutrophil, monocyte, and/or dendritic cell production. The bone marrow microenvironment plays critical roles in both sensing and orchestrating the progenitor response to infection [reviewed in (60)]. Importantly, inflammation and infection also profoundly remodel the sinusoidal network and perivascular stromal cells that maintain hematopoiesis and perturb stem cell localization within the marrow (60). Key open questions are a) whether hematopoietic stress responses use the same anatomical structures as normal hematopoiesis or instead depend on stress-specific anatomical cues and b) to what extend remodeling of sinusoids perturbs the anatomical structures that maintain the different progenitors. The most dramatic example of acute insult to the bone marrow is myeloablation. This eliminates not only hematopoietic cells but also the sinusoids and associated perivascular cells whereas endosteal regions and arterioles are more protected (6, 21, 61, 62). In this case the key open questions are: a) how are the local microenvironments restored? and b) what are the anatomical structures that support regenerative hematopoiesis? Identification of these will likely lead to novel therapies to promote restoration of blood cell production after myeloablation.
Chronic insults also lead to progressive remodeling of the microenvironment diminishing its capacity to support normal progenitors and -in some cases- hijacking it to promote pathogenesis. Examples of this pathogenic remodeling occur during physiological aging, leukemia, and other proliferative diseases (63, 64). Most studies have focused on determining how this remodeling perturbs HSC function. Little is known about how the different pathologies affect the structures that support more mature cells and whether protecting these structures can maintain normal hematopoiesis during disease.
Conclusion
Hematopoiesis in the bone marrow is spatially and regionally organized by specialized local microenvironments that support different types of stem cells and progenitors. The challenges in imaging the bone marrow tissue have limited progress (65). However, the future is bright. Adapting technological advances validated in other tissues -including live imaging, multiparameter microscopy, new reporter strains, and spatial transcriptomics- will allow systematic examination of blood production in situ to define how local cues from the microenvironment control normal and pathological hematopoiesis.
Author Contributions
DL conceived the manuscript who was written by all the authors. All authors contributed to the article and approved the submitted version.
Funding
JZ is supported by an EvansMDS Young Investigator Award. DL is supported by R01 HL136529, R01 HL153229, R01 HL158616, and U54 DK126108.
Conflict of Interest
The authors declare that the research was conducted in the absence of any commercial or financial relationships that could be construed as a potential conflict of interest.
Publisher’s Note
All claims expressed in this article are solely those of the authors and do not necessarily represent those of their affiliated organizations, or those of the publisher, the editors and the reviewers. Any product that may be evaluated in this article, or claim that may be made by its manufacturer, is not guaranteed or endorsed by the publisher.
References
1. Wei Q, Frenette PS. Niches for Hematopoietic Stem Cells and Their Progeny. Immunity (2018) 48:632–48. doi: 10.1016/j.immuni.2018.03.024
2. May M, Slaughter A, Lucas D. Dynamic Regulation of Hematopoietic Stem Cells by Bone Marrow Niches. Curr Stem Cell Rep (2018) 4:201–8. doi: 10.1007/s40778-018-0132-x
3. Comazzetto S, Shen B, Morrison SJ. Niches That Regulate Stem Cells and Hematopoiesis in Adult Bone Marrow. Dev Cell (2021) 56:1848–60. doi: 10.1016/j.devcel.2021.05.018
4. Kiel MJ, Yilmaz OH, Iwashita T, Yilmaz OH, Terhorst C, Morrison SJ. SLAM Family Receptors Distinguish Hematopoietic Stem and Progenitor Cells and Reveal Endothelial Niches for Stem Cells. Cell (2005) 121:1109–21. doi: 10.1016/j.cell.2005.05.026
5. Mendez-Ferrer S, Michurina TV, Ferraro F, Mazloom AR, Macarthur BD, Lira SA, et al. Mesenchymal and Haematopoietic Stem Cells Form a Unique Bone Marrow Niche. Nature (2010) 466:829–34. doi: 10.1038/nature09262
6. Kunisaki Y, Bruns I, Scheiermann C, Ahmed J, Pinho S, Zhang D, et al. Arteriolar Niches Maintain Haematopoietic Stem Cell Quiescence. Nature (2013) 502:637–43. doi: 10.1038/nature12612
7. Ding L, Saunders TL, Enikolopov G, Morrison SJ. Endothelial and Perivascular Cells Maintain Haematopoietic Stem Cells. Nature (2012) 481:457–62. doi: 10.1038/nature10783
8. Acar M, Kocherlakota KS, Murphy MM, Peyer JG, Oguro H, Inra CN, et al. Deep Imaging of Bone Marrow Shows Non-Dividing Stem Cells Are Mainly Perisinusoidal. Nature (2015) 526:126–30. doi: 10.1038/nature15250
9. Bruns I, Lucas D, Pinho S, Ahmed J, Lambert MP, Kunisaki Y, et al. Megakaryocytes Regulate Hematopoietic Stem Cell Quiescence Through CXCL4 Secretion. Nat Med (2014) 20:1315–20. doi: 10.1038/nm.3707
10. Yamazaki S, Ema H, Karlsson G, Yamaguchi T, Miyoshi H, Shioda S, et al. Nonmyelinating Schwann Cells Maintain Hematopoietic Stem Cell Hibernation in the Bone Marrow Niche. Cell (2011) 147:1146–58. doi: 10.1016/j.cell.2011.09.053
11. Gomariz A, Helbling PM, Isringhausen S, Suessbier U, Becker A, Boss A, et al. Quantitative Spatial Analysis of Haematopoiesis-Regulating Stromal Cells in the Bone Marrow Microenvironment by 3D Microscopy. Nat Commun (2018) 9:2532. doi: 10.1038/s41467-018-04770-z
12. Kokkaliaris KD, Kunz L, Cabezas-Wallscheid N, Christodoulou C, Renders S, Camargo F, et al. Adult Blood Stem Cell Localization Reflects the Abundance of Reported Bone Marrow Niche Cell Types and Their Combinations. Blood (2020) 136:2296–307. doi: 10.1182/blood.2020006574
13. Zhao M, Perry JM, Marshall H, Venkatraman A, Qian P, He XC, et al. Megakaryocytes Maintain Homeostatic Quiescence and Promote Post-Injury Regeneration of Hematopoietic Stem Cells. Nat Med (2014) 20:1321–6. doi: 10.1038/nm.3706
14. Nakamura-Ishizu A, Takubo K, Fujioka M, Suda T. Megakaryocytes Are Essential for HSC Quiescence Through the Production of Thrombopoietin. Biochem Biophys Res Commun (2014) 454:353–7. doi: 10.1016/j.bbrc.2014.10.095
15. Pinho S, Marchand T, Yang E, Wei Q, Nerlov C, Frenette PS. Lineage-Biased Hematopoietic Stem Cells Are Regulated by Distinct Niches. Dev Cell (2018) 44:634–641 e4. doi: 10.1016/j.devcel.2018.01.016
16. Itkin T, Gur-Cohen S, Spencer JA, Schajnovitz A, Ramasamy SK, Kusumbe AP, et al. Distinct Bone Marrow Blood Vessels Differentially Regulate Haematopoiesis. Nature (2016) 532:323–8. doi: 10.1038/nature17624
17. Kusumbe AP, Ramasamy SK, Itkin T, Mae MA, Langen UH, Betsholtz C, et al. Age-Dependent Modulation of Vascular Niches for Haematopoietic Stem Cells. Nature (2016) 532:380–4. doi: 10.1038/nature17638
18. Nilsson SK, Johnston HM, Coverdale JA. Spatial Localization of Transplanted Hemopoietic Stem Cells: Inferences for the Localization of Stem Cell Niches. Blood (2001) 97:2293–9. doi: 10.1182/blood.V97.8.2293
19. Lo Celso C, Fleming HE, Wu JW, Zhao CX, Miake-Lye S, Fujisaki J, et al. Live-Animal Tracking of Individual Haematopoietic Stem/Progenitor Cells in Their Niche. Nature (2009) 457:92–6. doi: 10.1038/nature07434
20. Silberstein L, Goncalves KA, Kharchenko PV, Turcotte R, Kfoury Y, Mercier F, et al. Proximity-Based Differential Single-Cell Analysis of the Niche to Identify Stem/Progenitor Cell Regulators. Cell Stem Cell (2016) 19:530–43. doi: 10.1016/j.stem.2016.07.004
21. Zhao M, Tao F, Venkatraman A, Li Z, Smith SE, Unruh J, et al. N-Cadherin-Expressing Bone and Marrow Stromal Progenitor Cells Maintain Reserve Hematopoietic Stem Cells. Cell Rep (2019) 26:652–669 e6. doi: 10.1016/j.celrep.2018.12.093
22. Christodoulou C, Spencer JA, Yeh SA, Turcotte R, Kokkaliaris KD, Panero R, et al. Live-Animal Imaging of Native Haematopoietic Stem and Progenitor Cells. Nature (2020) 578:278–83. doi: 10.1038/s41586-020-1971-z
23. Kiel MJ, Radice GL, Morrison SJ. Lack of Evidence That Hematopoietic Stem Cells Depend on N-Cadherin-Mediated Adhesion to Osteoblasts for Their Maintenance. Cell Stem Cell (2007) 1:204–17. doi: 10.1016/j.stem.2007.06.001
24. Upadhaya S, Krichevsky O, Akhmetzyanova I, Sawai CM, Fooksman DR, Reizis B. Intravital Imaging Reveals Motility of Adult Hematopoietic Stem Cells in the Bone Marrow Niche. Cell Stem Cell (2020) 27:336–345 e4. doi: 10.1016/j.stem.2020.06.003
25. Pietras EM, Reynaud D, Kang YA, Carlin D, Calero-Nieto FJ, Leavitt AD, et al. Functionally Distinct Subsets of Lineage-Biased Multipotent Progenitors Control Blood Production in Normal and Regenerative Conditions. Cell Stem Cell (2015) 17:35–46. doi: 10.1016/j.stem.2015.05.003
26. Pronk CJ, Rossi DJ, Mansson R, Attema JL, Norddahl GL, Chan CK, et al. Elucidation of the Phenotypic, Functional, and Molecular Topography of a Myeloerythroid Progenitor Cell Hierarchy. Cell Stem Cell (2007) 1:428–42. doi: 10.1016/j.stem.2007.07.005
27. Wilson A, Laurenti E, Oser G, van der Wath RC, Blanco-Bose W, Jaworski M, et al. Hematopoietic Stem Cells Reversibly Switch From Dormancy to Self-Renewal During Homeostasis and Repair. Cell (2008) 135:1118–29. doi: 10.1016/j.cell.2008.10.048
28. Cordeiro Gomes A, Hara T, Lim VY, Herndler-Brandstetter D, Nevius E, Sugiyama T, et al. Hematopoietic Stem Cell Niches Produce Lineage-Instructive Signals to Control Multipotent Progenitor Differentiation. Immunity (2016) 45:1219–31. doi: 10.1016/j.immuni.2016.11.004
29. Kohler A, Schmithorst V, Filippi MD, Ryan MA, Daria D, Gunzer M, et al. Altered Cellular Dynamics and Endosteal Location of Aged Early Hematopoietic Progenitor Cells Revealed by Time-Lapse Intravital Imaging in Long Bones. Blood (2009) 114:290–8. doi: 10.1182/blood-2008-12-195644
30. Mohandas N, Prenant M. Three-Dimensional Model of Bone Marrow. Blood (1978) 51:633–43. doi: 10.1182/blood.V51.4.633.633
31. May A, Forrester LM. The Erythroblastic Island Niche: Modeling in Health, Stress, and Disease. Exp Hematol (2020) 91:10–21. doi: 10.1016/j.exphem.2020.09.185
32. Comazzetto S, Murphy MM, Berto S, Jeffery E, Zhao Z, Morrison SJ. Restricted Hematopoietic Progenitors and Erythropoiesis Require SCF From Leptin Receptor+ Niche Cells in the Bone Marrow. Cell Stem Cell (2019) 24:477–86 e6. doi: 10.1016/j.stem.2018.11.022
33. Herault A, Binnewies M, Leong S, Calero-Nieto FJ, Zhang SY, Kang YA, et al. Myeloid Progenitor Cluster Formation Drives Emergency and Leukaemic Myelopoiesis. Nature (2017) 544:53–8. doi: 10.1038/nature21693
34. Olsson A, Venkatasubramanian M, Chaudhri VK, Aronow BJ, Salomonis N, Singh H, et al. Single-Cell Analysis of Mixed-Lineage States Leading to a Binary Cell Fate Choice. Nature (2016) 537:698–702. doi: 10.1038/nature19348
35. Yanez A, Coetzee SG, Olsson A, Muench DE, Berman BP, Hazelett DJ, et al. Granulocyte-Monocyte Progenitors and Monocyte-Dendritic Cell Progenitors Independently Produce Functionally Distinct Monocytes. Immunity (2017) 47:890–902.e4. doi: 10.1016/j.immuni.2017.10.021
36. Zhang J, Wu Q, Johnson CB, Pham G, Kinder JM, Olsson A, et al. In Situ Mapping Identifies Distinct Vascular Niches for Myelopoiesis. Nature (2021) 590:457–62. doi: 10.1038/s41586-021-03201-2
37. Stanley ER, Chitu V. CSF-1 Receptor Signaling in Myeloid Cells. Cold Spring Harb Perspect Biol 6 (2014) 6(6):a021857. doi: 10.1101/cshperspect.a021857
38. Zehentmeier S, Pereira JP. Cell Circuits and Niches Controlling B Cell Development. Immunol Rev (2019) 289:142–57. doi: 10.1111/imr.12749
39. Ding L, Morrison SJ. Haematopoietic Stem Cells and Early Lymphoid Progenitors Occupy Distinct Bone Marrow Niches. Nature (2013) 495:231–5. doi: 10.1038/nature11885
40. Greenbaum A, Hsu YM, Day RB, Schuettpelz LG, Christopher MJ, Borgerding JN, et al. CXCL12 in Early Mesenchymal Progenitors Is Required for Haematopoietic Stem-Cell Maintenance. Nature (2013) 495:227–30. doi: 10.1038/nature11926
41. Shen B, Tasdogan A, Ubellacker JM, Zhang J, Nosyreva ED, Du L, et al. A Mechanosensitive Peri-Arteriolar Niche for Osteogenesis and Lymphopoiesis. Nature (2021) 591:438–44. doi: 10.1038/s41586-021-03298-5
42. Green AC, Tjin G, Lee SC, Chalk AM, Straszkowski L, Kwang D, et al. The Characterization of Distinct Populations of Murine Skeletal Cells That Have Different Roles in B Lymphopoiesis. Blood (2021) 138(4):304–17. doi: 10.1182/blood.2020005865
43. Tokoyoda K, Egawa T, Sugiyama T, Choi BI, Nagasawa T. Cellular Niches Controlling B Lymphocyte Behavior Within Bone Marrow During Development. Immunity (2004) 20:707–18. doi: 10.1016/j.immuni.2004.05.001
44. Mandal M, Okoreeh MK, Kennedy DE, Maienschein-Cline M, Ai J, McLean KC, et al. CXCR4 Signaling Directs Igk Recombination and the Molecular Mechanisms of Late B Lymphopoiesis. Nat Immunol (2019) 20:1393–403. doi: 10.1038/s41590-019-0468-0
45. Yu VW, Lymperi S, Oki T, Jones A, Swiatek P, Vasic R, et al. Distinctive Mesenchymal-Parenchymal Cell Pairings Govern B Cell Differentiation in the Bone Marrow. Stem Cell Rep (2016) 7:220–35. doi: 10.1016/j.stemcr.2016.06.009
46. Fistonich C, Zehentmeier S, Bednarski JJ, Miao R, Schjerven H, Sleckman BP, et al. Cell Circuits Between B Cell Progenitors and IL-7(+) Mesenchymal Progenitor Cells Control B Cell Development. J Exp Med (2018) 215:2586–99. doi: 10.1084/jem.20180778
47. Balzano M, De Grandis M, Vu Manh TP, Chasson L, Bardin F, Farina A, et al. Nidogen-1 Contributes to the Interaction Network Involved in Pro-B Cell Retention in the Peri-Sinusoidal Hematopoietic Stem Cell Niche. Cell Rep (2019) 26:3257–71.e8. doi: 10.1016/j.celrep.2019.02.065
48. Radtke AJ, Kandov E, Lowekamp B, Speranza E, Chu CJ, Gola A, et al. IBEX: A Versatile Multiplex Optical Imaging Approach for Deep Phenotyping and Spatial Analysis of Cells in Complex Tissues. Proc Natl Acad Sci USA (2020) 117:33455–65. doi: 10.1073/pnas.2018488117
49. Goltsev Y, Samusik N, Kennedy-Darling J, Bhate S, Hale M, Vazquez G, et al. Deep Profiling of Mouse Splenic Architecture With CODEX Multiplexed Imaging. Cell (2018) 174:968–81.e15. doi: 10.1016/j.cell.2018.07.010
50. Snippert HJ, van der Flier LG, Sato T, van Es JH, van den Born M, Kroon-Veenboer C, et al. Intestinal Crypt Homeostasis Results From Neutral Competition Between Symmetrically Dividing Lgr5 Stem Cells. Cell (2010) 143:134–44. doi: 10.1016/j.cell.2010.09.016
51. Zhang J, Wu Q, Johnson G, Pham JM, Kinder JM, Olsson A, et al. In Situ Mapping Identifies Distinct Vascular Niches for Myelopoiesis. Nature (2021) 590(7846):457–62. doi: 10.1038/s41586-021-03201-2
52. Method of the Year 2020: Spatially Resolved Transcriptomics. Nat Methods (2021) 18:1. doi: 10.1038/s41592-020-01042-x
53. Baccin C, Al-Sabah J, Velten L, Helbling PM, Grunschlager F, Hernandez-Malmierca P, et al. Combined Single-Cell and Spatial Transcriptomics Reveals the Molecular, Cellular and Spatial Bone Marrow Niche Organization. Nat Cell Biol (2020) 22(1):38–48. doi: 10.1101/718395
54. Baryawno N, Przybylski D, Kowalczyk MS, Kfoury Y, Severe N, Gustafsson K, et al. A Cellular Taxonomy of the Bone Marrow Stroma in Homeostasis and Leukemia. Cell (2019) 177:1915–32.e16. doi: 10.1016/j.cell.2019.04.040
55. Tikhonova AN, Dolgalev I, Hu H, Sivaraj KK, Hoxha E, Cuesta-Dominguez A, et al. The Bone Marrow Microenvironment at Single-Cell Resolution. Nature (2019) 569:222–8. doi: 10.1038/s41586-019-1104-8
56. Zhang J, Link DC. Targeting of Mesenchymal Stromal Cells by Cre-Recombinase Transgenes Commonly Used to Target Osteoblast Lineage Cells. J Bone Miner Res (2016) 31:2001–7. doi: 10.1002/jbmr.2877
57. Tamplin OJ, Durand EM, Carr LA, Childs SJ, Hagedorn EJ, Li P, et al. Hematopoietic Stem Cell Arrival Triggers Dynamic Remodeling of the Perivascular Niche. Cell (2015) 160:241–52. doi: 10.1016/j.cell.2014.12.032
58. Reismann D, Stefanowski J, Gunther R, Rakhymzhan A, Matthys R, Nutzi R, et al. Longitudinal Intravital Imaging of the Femoral Bone Marrow Reveals Plasticity Within Marrow Vasculature. Nat Commun (2017) 8:2153. doi: 10.1038/s41467-017-01538-9
59. Myneni VD, Szalayova I, Mezey E. Differences in Steady-State Erythropoiesis in Different Mouse Bones and Postnatal Spleen. Front Cell Dev Biol (2021) 9:646646. doi: 10.3389/fcell.2021.646646
60. Johnson CB, Zhang J, Lucas D. The Role of the Bone Marrow Microenvironment in the Response to Infection. Front Immunol (2020) 11:585402. doi: 10.3389/fimmu.2020.585402
61. Olson TS, Caselli A, Otsuru S, Hofmann TJ, Williams R, Paolucci P, et al. Megakaryocytes Promote Murine Osteoblastic HSC Niche Expansion and Stem Cell Engraftment After Radioablative Conditioning. Blood (2013) 121:5238–49. doi: 10.1182/blood-2012-10-463414
62. Hooper AT, Butler JM, Nolan DJ, Kranz A, Iida K, Kobayashi M, et al. Engraftment and Reconstitution of Hematopoiesis is Dependent on VEGFR2-Mediated Regeneration of Sinusoidal Endothelial Cells. Cell Stem Cell (2009) 4:263–74. doi: 10.1016/j.stem.2009.01.006
63. Ho YH, Mendez-Ferrer S. Microenvironmental Contributions to Hematopoietic Stem Cell Aging. Haematologica (2020) 105:38–46. doi: 10.3324/haematol.2018.211334
64. Mendez-Ferrer S, Bonnet D, Steensma DP, Hasserjian RP, Ghobrial IM, Gribben JG, et al. Bone Marrow Niches in Haematological Malignancies. Nat Rev Cancer (2020) 20:285–98. doi: 10.1038/s41568-020-0245-2
Keywords: anatomy of the bone marrow, spatial organization of hematopoiesis, microenvironment, niches, dynamics of hematopoiesis
Citation: Wu Q, Zhang J and Lucas D (2021) Anatomy of Hematopoiesis and Local Microenvironments in the Bone Marrow. Where to? Front. Immunol. 12:768439. doi: 10.3389/fimmu.2021.768439
Received: 31 August 2021; Accepted: 13 October 2021;
Published: 11 November 2021.
Edited by:
Stéphane JC Mancini, INSERM UMR1236 Microenvironnement, Différenciation cellulaire, Immunologie et Cancer (INSERM), FranceReviewed by:
Marion Espeli, Institut National de la Santé et de la Recherche Médicale (INSERM), FranceNoriaki Ono, University of Texas Health Science Center at Houston, United States
Copyright © 2021 Wu, Zhang and Lucas. This is an open-access article distributed under the terms of the Creative Commons Attribution License (CC BY). The use, distribution or reproduction in other forums is permitted, provided the original author(s) and the copyright owner(s) are credited and that the original publication in this journal is cited, in accordance with accepted academic practice. No use, distribution or reproduction is permitted which does not comply with these terms.
*Correspondence: Daniel Lucas, ZGFuaWVsLmx1Y2FzQGNjaG1jLm9yZw==