- 1Department of Neonatology, The Children’s Hospital, Zhejiang University School of Medicine, National Clinical Research Center for Child Health, Hangzhou, China
- 2Department of Orthopedic Surgery, The Second Affiliated Hospital, Zhejiang University School of Medicine, Hangzhou, China
- 3Orthopedics Research Institute of Zhejiang University, Hangzhou, China
- 4Key Laboratory of Motor System Disease Research and Precision Therapy of Zhejiang Province, Hangzhou, China
Bone metastasis is commonly seen in patients with breast cancer, prostate cancer and lung cancer. Tumor-intrinsic factors and the tumor microenvironment cooperate to affect the formation of bone metastatic niche. Within the bone microenvironment, immune cells have been regarded as a major contributor to metastatic progression. In this review, we describe the dynamic roles of immune cells in regulating metastatic homing, seeding, dormancy, and outgrowth in the bone. We also summarize the diverse functions of immune molecules including chemokines, cytokines, and exosomes in remodeling the bone metastatic niche. Furthermore, we discuss the therapeutic and prognostic potential of these cellular and molecular players in bone metastasis.
Introduction
Bone metastasis is a multi-stage process which involves escape from the primary site, survival in the circulation and metastatic colonization (1). These events are driven by both cancer cell-intrinsic traits and extrinsic factors in the tumor microenvironment (TME) (2). Emerging evidence has highlighted the importance of immune modulation in the bone metastatic niche formation (3–6). In this review, we aim to focus on the contribution of immune cellular components and molecular mediators to the homing, seeding, dormancy and subsequent outgrowth of metastatic cancer cells in the bone microenvironment (Figure 1).
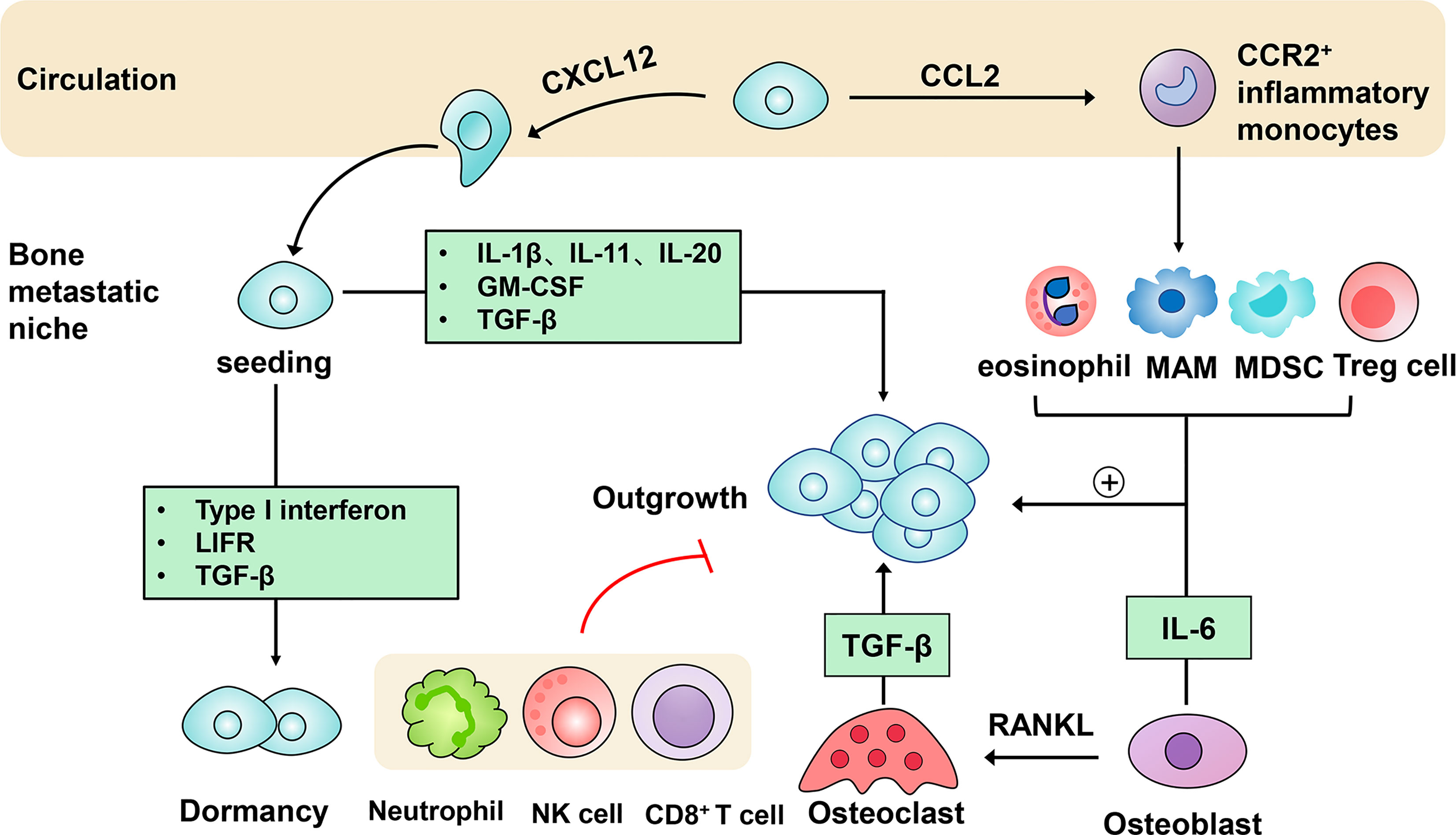
Figure 1 Immune mechanisms in the progression of bone metastasis. After being detached from the primary tumor, circulating tumor cells produce CXC-chemokine ligand 12 (CXCL12) to facilitate metastatic homing to the bone. They can also secrete CC-chemokine ligand 2 (CCL2) to recruit CC-chemokine receptor 2 (CCR2)+ inflammatory monocytes towards the bone metastatic niche, in which they differentiate into metastasis-associated macrophages (MAMs). Following metastatic seeding into the bone, disseminated tumor cells can remain in a dormant state driven by type I interferon, the receptor of leukemia inhibitory factor (LIFR), transforming growth factor-β (TGF-β). Induced by several immune molecules, dormant tumor cells can be reactivated and initiate metastatic outgrowth. Eosinophils, MAMs, myeloid-derived suppressor cells (MDSCs), regulatory T cells (Tregs) conspire to support the persistent growth of metastatic tumor cells. Furthermore, the tumor-stroma interactions are engaged in the acceleration of bone metastasis. Osteoblasts-derived receptor activator of nuclear factor-κB-ligand (RANKL) induces the differentiation of osteoclasts, which in turn stimulates bone resorption and tumor cell proliferation mediated by growth factors like transforming growth factor-β (TGF-β). In addition, immune cells including neutrophils, nature killer (NK) cells and CD8+ T cells act to restrict metastatic tumor cell growth.
Immune Cells Modulating Metastatic Niche Formation in the Bone
Innate Immune Cells
Macrophages participate in all steps of metastasis. They can be polarized into either proinflammatory M1-like macrophages or anti-inflammatory M2-like macrophages in response to environmental stimuli (7). In the primary site, tumor-associated macrophages (TAMs) are involved in angiogenesis, migration and intravasation (8). At the distant organs, metastasis-associated macrophages (MAMs), a distinct population of TAMs, were first described in mouse models of breast cancer lung metastasis (9). They were reported to promote tumor cell extravasation, seeding and metastatic outgrowth (10, 11). Similar to lung MAMs, bone MAMs also play metastasis-promoting roles. Our recent study reveals that MAMs are enriched in mouse models of breast cancer bone metastasis and patient samples. Macrophage depletion with clodronate liposomes and colony-stimulating factor 1 receptor (CSF1R) reduces breast cancer cell growth in the bone. Using lineage tracing methods and gene expression analysis, we are the first to demonstrate bone MAMs originate from circulating inflammatory monocytes and bear specific cell surface markers CD204 and interleukin 4 receptor (IL4R). IL4R acts as promoters of metastatic outgrowth. The absence of IL4R in bone MAMs results in a reduction of metastatic tumor growth in the bone, which holds therapeutic promise for bone metastatic patients (12).
Neutrophils, a key player in the innate immunity, have gained attention in the metastatic process (13–15). The neutrophil-to-lymphocyte ratio in the blood has been reported as a prognostic indicator of survival in patients with bone metastasis (16). Moreover, neutrophil accumulation in the TME showed resistance to immune checkpoint blockade (ICB) relative to other immune compositions (17). Given the highly heterogeneity and plasticity of neutrophils, they display opposing behaviors during the metastatic dissemination and colonization. On one hand, neutrophils elicit cytotoxic responses by directly killing tumor cell to suppress metastatic growth. In the bone metastatic prostate cancer, Ly6G+ neutrophils are recruited to the bone lesions and induce apoptosis of prostate cancer cells through inhibition of signal transducer and activator of transcription (STAT) 5. Notably, the cytotoxic property of neutrophils can be gradually lost during tumor progression, which is consistent with previously reported immunosuppressive activity of neutrophils in the late stages of cancer (18, 19). On the other hand, increasing evidence indicates the relevance of neutrophils in supporting metastatic establishment in other distal organs. For example, neutrophils are shown to facilitate extravasation of tumor cells and lung colonization through secretion of inflammatory cytokines and suppression of natural killer (NK) cells (20). Overall, these studies uncover the context-dependent roles of neutrophils in metastasis.
NK cells are innate cytotoxic lymphocytes that mediate cancer cell killing in the tumor progression (21). In the experimental metastasis models of breast cancer, metastatic cancer cells have been found to evade the NK cell immune attack and remain dormancy by downregulating levels of ligands for NK-cell activating receptors (22). The functions of NK cell can also be impaired by certain signaling pathways and inflammatory cytokines in bone metastasis. A recent study indicated that inhibition of the JAK/STAT pathway dramatically decreased NK cell activity and enhanced the metastatic burden, which could be restored by interleukin-15 (IL-15) stimulation (23).
While initially regarded as an anti-tumor immune component (24), eosinophils in the context of inflammation also contribute to bone metastasis. Mechanistically, they secrete CC-chemokine ligand 6 (CCL6) to attract tumor cells to the bone metastatic niche through CC-chemokine receptor 1 (CCR1). Inhibition of the CCL6-CCR1 signaling significantly attenuates the tumor cell migration and bone metastasis formation, which suggests the close connection between inflammation and metastasis and provides new paradigms for cancer prevention (25). Furthermore, plasmacytoid dentritic cells have been described in mouse models of breast cancer bone metastasis. Their metastasis-supporting effects are driven by induction of immunosuppressive cells and osteolytic cytokine receptor activator of nuclear factor-κB-ligand (RANKL) (26).
Adaptive Immune Cells
CD8+ T cells exert cytotoxic effects in the bone metastatic niche. They act as negative regulators of tumor growth in the bone. Adoptive transfer of cytotoxic CD8+ T cells into PLCγ2-/- mice prevents the growth of B16 melanoma cells in the bone (27). Their anti-tumor responses in the bone are further enhanced by the transcription factor estrogen-related receptor alpha (ERRα). On one hand, overexpression of ERRα increased the production of the chemokines CCL17 and CCL20 to attract more cytotoxic T cells the bone. On the other hand, ERRα decreased the tumor cell secretion of anti-inflammatory cytokine transforming growth factor-β (TGF-β) (28). In contrast to CD8+ T cells, cancer-primed CD4+ T cells are reported to create the premetastatic niche via the release of RANKL before bone colonization in breast cancer models (29). As a subtype of CD4+ T cells, the immunosuppressive regulatory T cells (Tregs) enrichment has been detected in the bone marrow of patients with prostate cancer. These cells are found to inhibit the differentiation of osteoclasts (OCs), which might elucidate the potential mechanisms of osteoblastic lesions of prostate cancer (30).
Other Non-Immune Cells
Myeloid-derived suppressor cells (MDSCs) represent a heterogenous population of immature myeloid cells with immunosuppressive features (31). An abundance of MDSCs is observed in both preclinical mouse models and patients with bone metastasis (32, 33). Several factors are required for the accumulation and function of MDSCs in the bone microenvironment. Interferon regulatory factor 7 (IRF7) was reported to suppress pro-metastatic activity of MDSCs in the 4T1 murine metastatic model. Overexpression of IRF7 reduced metastatic burden and prolonged survival time by counteracting the action of MDSCs and restoring CD8+ T cell and NK cell activity (34). More recently, DKK1, an inhibitor of the Wnt pathway, has been directly targets β-catenin in MDSCs from mouse models and patients. Neutralization of DKK1 leads to reduced tumor growth and MDSC expansion (35). Additionally, since MDSCs are progenitors of macrophages, they also have the capacity to differentiate into bone-resorbing OCs in a nitric oxide-dependent manner, thereby enhancing bone destruction and tumor growth (36).
Immune Mediators Remodeling the Bone Metastatic Niche
Chemokines
Increasing evidence reveals the involvement of chemokines in the bone metastatic progression. In breast cancer, CCL2 induces the recruitment of circulating inflammatory monocytes to the bone through the interaction with CCR2 (12). Similarly, prostate cancer-derived CCL2 also facilitates tumor growth in the bone, which results from increased OC differentiation and angiogenesis (37). Treatment with anti-CCL2 antibody attenuated metastatic outgrowth and prolonged survival in mouse models of breast cancer metastasis (12). However, application of anti-CCL2 agents alone should be cautioned because discontinuation of CCL2 inhibition has rebound effects that augment metastasis in mice (38). Furthermore, inhibition of CCR2 with humanized monoclonal antibodies, MLN1202, has shown beneficial effects in patients with bone metastases, as evidenced by reduced levels of bone-resorbing factors urinary N-telopeptide of type I collagen (uNTX) (ClinicalTrials. gov ID: NCT01015560). CCL4 binding to CCR5 on bone marrow fibroblasts stimulates their release of connective tissue growth factor/CCN2, which supports breast cancer cell growth in bone (39). High expressions of CCL5 are associated with high Gleason grade and poor prognosis in prostate cancer patients (40). In response to prostate cancer cell growth-induced pressure, osteocytes produce CCL5, which promotes tumor proliferation in the bone (41). CXC-chemokine ligand 5 (CXCL5) are also reported to deliver growth signals to malignant cells. Exposure to TME elicits CXCL5 secretion from bone marrow cells, which allows breast cancer cells to exit from dormancy and colonize the bone microenvironment (42). The CXCL12/CXC-chemokine receptor 4 (CXCR4) axis plays an important role in regulating DTC homing and survival. In triple negative breast cancer (TNBC), CXCL12 from cancer-associated fibroblasts is found to select cancer cells with high Src activity homing to the bone (43). Moreover, the CXCL12/CXCR4 pathway modulates tumor growth in the bone. In prostate cancer, pyruvate kinase M2 (PKM2) released by primary cancer-derived exosomes initiates the downstream release of hypoxia inducible factor-1α (HIF-1α) and CXCL12, which promotes metastatic seeding and outgrowth. Genetic inhibition and pharmacological blockade of CXCR4 diminish the number of bone metastases and total metastatic burden (44). This study suggests that the growth of tumor cells in the distant organs can be conditioned by metabolic alterations at the primary site.
Cytokines
Interferons (IFN), comprised of type I IFNs and type II IFNs (IFNγ), are critical cytokines during the anti-metastatic immune response (45). The importance of type I IFNs in the bone metastatic niche was shown in three non-bone metastatic breast cancer models in which loss of host type I IFN signaling accelerated metastatic spreading to the bone in part through impairing the ability of NK cells to eliminate cancer cells (46). As observed in breast cancer, tumor-inherent type I IFN was also downregulated in proliferative prostate cancer cells from bone, which disrupted dormancy status and promoted metastatic outgrowth. This effect could be reversed by the use of histone deacetylase inhibitors (HDACi), suggesting the therapeutic potential of epigenetic modifications in the regulation of IFN signaling (47). Importantly, the presence of IRF9 is a positive prognostic biomarker for the chemotherapeutic response in TNBC patients (48). In addition, IFN-γ can directly target OCs to inhibit bone loss and bone metastasis (49).
Interleukin-1β(IL-1β) is highly expressed in tumor cells and bone marrow cells during progression of bone metastasis. IL-1β in tumor cells induces epithelial to mesenchymal transition (EMT) and correlates with recurrence and bone relapse in patients with stage II and III breast cancer. Bone marrow-derived IL-1β can activate intracellular nuclear factor Kappa B (NF-κB) and the Wnt signaling to promote metastatic expansion to form overt metastases. Targeting IL-1β with anti-IL-1β antibody or the IL-1 receptor antagonist, Anakinra, prevents metastatic seeding and colony formation (50, 51). IL-6 is a critical promoter of tumor growth in osteolytic bone metastasis of breast cancer. In this process, tumor cell-derived Jagged1 simulates Notch signaling in osteoblasts, which increases the IL-6 release and OC differentiation (52). IL-11, a member of the IL-6 family, has a similar effect in osteolytic lesions dependent on the STAT3 signaling (53). By contrast, the receptor of leukemia inhibitory factor (LIFR), whose ligand LIF belongs to the IL-6 family of cytokines, shows an anti-tumor effect through induction of dormancy in the bone (54). IL-20 is a tumor-promoting cytokine partly due to their capacity to induce proliferation and migration of tumor cells. Mice treated with anti-IL-20 antibody 7E displayed a reduction in bone colonization and osteolysis (55). Mesenchymal stem cell-derived IL-28 confers apoptotic resistance properties on prostate cancer cells disseminated to bone lesions via STAT3 activation (56). Although initially identified as an inhibitor of osteoclastogenesis (57), granulocyte macrophage-colony stimulating factor (GM-CSF), produced by breast cancer cells, has been recently found to increase the number of OCs and bone metastatic potential (58). Another cytokine TGF-β is involved in bone metastasis. TGF-β released by the bone matrix is a well-established driver of tumor growth and bone degradation (59). However, osteoblast-derived TGF-β induces dormancy of metastatic prostate cancer cells in the bone mediated by TGFβR-III-p38MAPK-pS249/T252RB pathway (60). This demonstrates the temporal differences of TGF-β signaling during different stages of bone metastasis. Beyond stromal cells, cancer cells can also express TGF-β to suppress the Wnt signaling through dishevelled binding antagonist of β-catenin 1(DACT1)- dependent biochemical condensates formation, which in turn promotes bone metastatic outgrowth (61). Finally, in the mouse model of prostate cancer bone metastasis, high levels of TGF-β in the TME were associated with poor response to ICB. This immunosuppressive effect of TGF-β is achieved by restraining type 1 T helper cell development. The efficacy of ICB was enhanced by TGF-β inhibition, suggesting the potential value of targeting TGF-β to improve ICB response (62).
Exosomes
Exosomes are nano-sized extracellular vesicles secreted by multiple cell types. They deliver signaling molecules such as proteins, lipids, microRNAs (miRNAs) to specific target cells, affecting the bone metastatic formation (63, 64). Breast cancer-derived exosomes containing CCL3, CCL27 and other molecules are found to remodel the bone microenvironment, characterized by stimulation of osteoclastogenesis and angiogenesis (65). Similarly, exosomes from melanoma cells can reprogram the phenotype of bone marrow progenitor cells and support tumor metastasis to the bone (66). In addition, exosomes from stromal cells contribute to the dormancy of breast cancer cells via reducing CXCL12 levels (67). Stromal-derived exosomal miR-148a-3p was reported to target the ERK1/2 signaling to suppress the proliferation of bone metastatic cancer cells (68). Furthermore, plasma exosomes act as noninvasive biomarkers to predict response to Radium-223 treatment. In bone metastatic prostate cancer, exosomal programmed death-ligand 1 (PD-L1) was upregulated in patients with unfavorable response to radium-223 treatment (69).
Perspectives
Immune cells exhibit either pro-metastatic or anti-metastatic activity in the bone microenvironment. Their reciprocal interactions with tumor cells and other bone-resident cells are essential for the bone metastatic progression. Technological advances have allowed us to identify specific immune cell subtypes and define their molecular characteristics. Blocking the pro-tumoral phenotypes of immune cells and exploiting their anti-tumor activities represent a novel therapeutic strategy to prevent the formation and recurrence of bone metastasis. In addition, immune mediators including chemokines, cytokines, and exosomes have shown great therapeutic and prognostic value in the bone metastases. Furthermore, the bone microenvironment can also influence the metastatic seeding into other distant organs triggered by epigenetic reprogramming (70). Despite deep insights into the immune landscape of bone metastasis, future studies are still needed to identify novel determinants and elucidate their immunological mechanisms, which will pave the way for immune-based therapy and improve clinical outcomes.
Author Contributions
XC wrote the manuscript. ZW revised the manuscript. All authors contributed to the article and approved the submitted version.
Funding
This work was supported by the China Postdoctoral Science Foundation (2021M692792) and National Natural Science Foundation of China (82103499).
Conflict of Interest
The authors declare that the research was conducted in the absence of any commercial or financial relationships that could be construed as a potential conflict of interest.
Publisher’s Note
All claims expressed in this article are solely those of the authors and do not necessarily represent those of their affiliated organizations, or those of the publisher, the editors and the reviewers. Any product that may be evaluated in this article, or claim that may be made by its manufacturer, is not guaranteed or endorsed by the publisher.
References
1. Coleman RE, Croucher PI, Padhani AR, Clezardin P, Chow E, Fallon M, et al. Bone Metastases. Nat Rev Dis Primers (2020) 6:83. doi: 10.1038/s41572-020-00216-3
2. Hofbauer LC, Bozec A, Rauner M, Jakob F, Perner S, Pantel K. Novel Approaches to Target the Microenvironment of Bone Metastasis. Nat Rev Clin Oncol (2021) 18:488–505. doi: 10.1038/s41571-021-00499-9
3. Esposito M, Ganesan S, Kang Y. Emerging Strategies for Treating Metastasis. Nat Cancer (2021) 2:258–70. doi: 10.1038/s43018-021-00181-0
4. Kitamura T, Qian BZ, Pollard JW. Immune Cell Promotion of Metastasis. Nat Rev Immunol (2015) 15:73–86. doi: 10.1038/nri3789
5. Doak GR, Schwertfeger KL, Wood DK. Distant Relations: Macrophage Functions in the Metastatic Niche. Trends Cancer (2018) 4:445–59. doi: 10.1016/j.trecan.2018.03.011
6. Chen F, Han Y, Kang Y. Bone Marrow Niches in the Regulation of Bone Metastasis. Br J Cancer (2021) 124:1912–20. doi: 10.1038/s41416-021-01329-6
7. Guc E, Pollard JW. Redefining Macrophage and Neutrophil Biology in the Metastatic Cascade. Immunity (2021) 54:885–902. doi: 10.1016/j.immuni.2021.03.022
8. Noy R, Pollard JW. Tumor-Associated Macrophages: From Mechanisms to Therapy. Immunity (2014) 41:49–61. doi: 10.1016/j.immuni.2014.06.010
9. Qian B, Deng Y, Im JH, Muschel RJ, Zou Y, Li J, et al. A Distinct Macrophage Population Mediates Metastatic Breast Cancer Cell Extravasation, Establishment and Growth. PLoS One (2009) 4:e6562. doi: 10.1371/journal.pone.0006562
10. Qian BZ, Li J, Zhang H, Kitamura T, Zhang J, Campion LR, et al. CCL2 Recruits Inflammatory Monocytes to Facilitate Breast-Tumour Metastasis. Nature (2011) 475:222–5. doi: 10.1038/nature10138
11. Qian BZ, Zhang H, Li J, He T, Yeo EJ, Soong DY, et al. FLT1 Signaling in Metastasis-Associated Macrophages Activates an Inflammatory Signature That Promotes Breast Cancer Metastasis. J Exp Med (2015) 212:1433–48. doi: 10.1084/jem.20141555
12. Ma RY, Zhang H, Li XF, Zhang CB, Selli C, Tagliavini G, et al. Monocyte-Derived Macrophages Promote Breast Cancer Bone Metastasis Outgrowth. J Exp Med (2020) 217:e20191820. doi: 10.1084/jem.20191820
13. Saini M, Szczerba BM, Aceto N. Circulating Tumor Cell-Neutrophil Tango Along the Metastatic Process. Cancer Res (2019) 79:6067–73. doi: 10.1158/0008-5472.CAN-19-1972
14. Jaillon S, Ponzetta A, Di Mitri D, Santoni A, Bonecchi R, Mantovani A. Neutrophil Diversity and Plasticity in Tumour Progression and Therapy. Nat Rev Cancer (2020) 20:485–503. doi: 10.1038/s41568-020-0281-y
15. Hedrick CC, Malanchi I. Neutrophils in Cancer: Heterogeneous and Multifaceted. Nat Rev Immunol (2021). doi: 10.1038/s41577-021-00571-6
16. Thio Q, Goudriaan WA, Janssen SJ, Paulino Pereira NR, Sciubba DM, Rosovksy RP, et al. Prognostic Role of Neutrophil-to-Lymphocyte Ratio and Platelet-to-Lymphocyte Ratio in Patients With Bone Metastases. Br J Cancer (2018) 119:737–43. doi: 10.1038/s41416-018-0231-6
17. Kim IS, Gao Y, Welte T, Wang H, Liu J, Janghorban M, et al. Immuno-Subtyping of Breast Cancer Reveals Distinct Myeloid Cell Profiles and Immunotherapy Resistance Mechanisms. Nat Cell Biol (2019) 21:1113–26. doi: 10.1038/s41556-019-0373-7
18. Costanzo-Garvey DL, Keeley T, Case AJ, Watson GF, Alsamraae M, Yu Y, et al. Neutrophils Are Mediators of Metastatic Prostate Cancer Progression in Bone. Cancer Immunol Immunother (2020) 69:1113–30. doi: 10.1007/s00262-020-02527-6
19. Patel S, Fu S, Mastio J, Dominguez GA, Purohit A, Kossenkov A, et al. Unique Pattern of Neutrophil Migration and Function During Tumor Progression. Nat Immunol (2018) 19:1236–47. doi: 10.1038/s41590-018-0229-5
20. Spiegel A, Brooks MW, Houshyar S, Reinhardt F, Ardolino M, Fessler E, et al. Neutrophils Suppress Intraluminal NK Cell-Mediated Tumor Cell Clearance and Enhance Extravasation of Disseminated Carcinoma Cells. Cancer Discov (2016) 6:630–49. doi: 10.1158/2159-8290.CD-15-1157
21. Huntington ND, Cursons J, Rautela J. The Cancer-Natural Killer Cell Immunity Cycle. Nat Rev Cancer (2020) 20:437–54. doi: 10.1038/s41568-020-0272-z
22. Malladi S, Macalinao DG, Jin X, He L, Basnet H, Zou Y, et al. Metastatic Latency and Immune Evasion Through Autocrine Inhibition of WNT. Cell (2016) 165:45–60. doi: 10.1016/j.cell.2016.02.025
23. Bottos A, Gotthardt D, Gill JW, Gattelli A, Frei A, Tzankov A, et al. Decreased NK-Cell Tumour Immunosurveillance Consequent to JAK Inhibition Enhances Metastasis in Breast Cancer Models. Nat Commun (2016) 7:12258. doi: 10.1038/ncomms12258
24. Rosenberg HF, Dyer KD, Foster PS. Eosinophils: Changing Perspectives in Health and Disease. Nat Rev Immunol (2013) 13:9–22. doi: 10.1038/nri3341
25. Li F, Du X, Lan F, Li N, Zhang C, Zhu C, et al. Eosinophilic Inflammation Promotes CCL6-Dependent Metastatic Tumor Growth. Sci Adv (2021) 7:eabb5943. doi: 10.1126/sciadv.abb5943
26. Sawant A, Hensel JA, Chanda D, Harris BA, Siegal GP, Maheshwari A, et al. Depletion of Plasmacytoid Dendritic Cells Inhibits Tumor Growth and Prevents Bone Metastasis of Breast Cancer Cells. J Immunol (2012) 189:4258–65. doi: 10.4049/jimmunol.1101855
27. Zhang K, Kim S, Cremasco V, Hirbe AC, Collins L, Piwnica-Worms D, et al. CD8+ T Cells Regulate Bone Tumor Burden Independent of Osteoclast Resorption. Cancer Res (2011) 71:4799–808. doi: 10.1158/0008-5472.CAN-10-3922
28. Bouchet M, Laine A, Boyault C, Proponnet-Guerault M, Meugnier E, Bouazza L, et al. ERRalpha Expression in Bone Metastases Leads to an Exacerbated Antitumor Immune Response. Cancer Res (2020) 80:2914–26. doi: 10.1158/0008-5472.CAN-19-3584
29. Monteiro AC, Leal AC, Goncalves-Silva T, Mercadante AC, Kestelman F, Chaves SB, et al. T Cells Induce Pre-Metastatic Osteolytic Disease and Help Bone Metastases Establishment in a Mouse Model of Metastatic Breast Cancer. PLoS One (2013) 8:e68171. doi: 10.1371/journal.pone.0068171
30. Zhao E, Wang L, Dai J, Kryczek I, Wei S, Vatan L, et al. Regulatory T Cells in the Bone Marrow Microenvironment in Patients With Prostate Cancer. Oncoimmunology (2012) 1:152–61. doi: 10.4161/onci.1.2.18480
31. Marvel D, Gabrilovich DI. Myeloid-Derived Suppressor Cells in the Tumor Microenvironment: Expect the Unexpected. J Clin Invest (2015) 125:3356–64. doi: 10.1172/JCI80005
32. Danilin S, Merkel AR, Johnson JR, Johnson RW, Edwards JR, Sterling JA. Myeloid-Derived Suppressor Cells Expand During Breast Cancer Progression and Promote Tumor-Induced Bone Destruction. Oncoimmunology (2012) 1:1484–94. doi: 10.4161/onci.21990
33. Wen J, Huang G, Liu S, Wan J, Wang X, Zhu Y, et al. Polymorphonuclear MDSCs Are Enriched in the Stroma and Expanded in Metastases of Prostate Cancer. J Pathol Clin Res (2020) 6:171–7. doi: 10.1002/cjp2.160
34. Bidwell BN, Slaney CY, Withana NP, Forster S, Cao Y, Loi S, et al. Silencing of Irf7 Pathways in Breast Cancer Cells Promotes Bone Metastasis Through Immune Escape. Nat Med (2012) 18:1224–31. doi: 10.1038/nm.2830
35. D'Amico L, Mahajan S, Capietto AH, Yang Z, Zamani A, Ricci B, et al. Dickkopf-Related Protein 1 (Dkk1) Regulates the Accumulation and Function of Myeloid Derived Suppressor Cells in Cancer. J Exp Med (2016) 213:827–40. doi: 10.1084/jem.20150950
36. Sawant A, Deshane J, Jules J, Lee CM, Harris BA, Feng X, et al. Myeloid-Derived Suppressor Cells Function as Novel Osteoclast Progenitors Enhancing Bone Loss in Breast Cancer. Cancer Res (2013) 73:672–82. doi: 10.1158/0008-5472.CAN-12-2202
37. Li X, Loberg R, Liao J, Ying C, Snyder LA, Pienta KJ, et al. A Destructive Cascade Mediated by CCL2 Facilitates Prostate Cancer Growth in Bone. Cancer Res (2009) 69:1685–92. doi: 10.1158/0008-5472.CAN-08-2164
38. Bonapace L, Coissieux MM, Wyckoff J, Mertz KD, Varga Z, Junt T, et al. Cessation of CCL2 Inhibition Accelerates Breast Cancer Metastasis by Promoting Angiogenesis. Nature (2014) 515:130–3. doi: 10.1038/nature13862
39. Sasaki S, Baba T, Nishimura T, Hayakawa Y, Hashimoto S, Gotoh N, et al. Essential Roles of the Interaction Between Cancer Cell-Derived Chemokine, CCL4, and Intra-Bone CCR5-Expressing Fibroblasts in Breast Cancer Bone Metastasis. Cancer Lett (2016) 378:23–32. doi: 10.1016/j.canlet.2016.05.005
40. Huang R, Wang S, Wang N, Zheng Y, Zhou J, Yang B, et al. CCL5 Derived From Tumor-Associated Macrophages Promotes Prostate Cancer Stem Cells and Metastasis via Activating Beta-Catenin/STAT3 Signaling. Cell Death Dis (2020) 11:234. doi: 10.1038/s41419-020-2435-y
41. Sottnik JL, Dai J, Zhang H, Campbell B, Keller ET. Tumor-Induced Pressure in the Bone Microenvironment Causes Osteocytes to Promote the Growth of Prostate Cancer Bone Metastases. Cancer Res (2015) 75:2151–8. doi: 10.1158/0008-5472.CAN-14-2493
42. Romero-Moreno R, Curtis KJ, Coughlin TR, Miranda-Vergara MC, Dutta S, Natarajan A, et al. The CXCL5/CXCR2 Axis Is Sufficient to Promote Breast Cancer Colonization During Bone Metastasis. Nat Commun (2019) 10:4404. doi: 10.1038/s41467-019-12108-6
43. Zhang XH, Jin X, Malladi S, Zou Y, Wen YH, Brogi E, et al. Selection of Bone Metastasis Seeds by Mesenchymal Signals in the Primary Tumor Stroma. Cell (2013) 154:1060–73. doi: 10.1016/j.cell.2013.07.036
44. Dai J, Escara-Wilke J, Keller JM, Jung Y, Taichman RS, Pienta KJ, et al. Primary Prostate Cancer Educates Bone Stroma Through Exosomal Pyruvate Kinase M2 to Promote Bone Metastasis. J Exp Med (2019) 216:2883–99. doi: 10.1084/jem.20190158
45. Parker BS, Rautela J, Hertzog PJ. Antitumour Actions of Interferons: Implications for Cancer Therapy. Nat Rev Cancer (2016) 16:131–44. doi: 10.1038/nrc.2016.14
46. Rautela J, Baschuk N, Slaney CY, Jayatilleke KM, Xiao K, Bidwell BN, et al. Loss of Host Type-I IFN Signaling Accelerates Metastasis and Impairs NK-Cell Antitumor Function in Multiple Models of Breast Cancer. Cancer Immunol Res (2015) 3:1207–17. doi: 10.1158/2326-6066.CIR-15-0065
47. Owen KL, Gearing LJ, Zanker DJ, Brockwell NK, Khoo WH, Roden DL, et al. Prostate Cancer Cell-Intrinsic Interferon Signaling Regulates Dormancy and Metastatic Outgrowth in Bone. EMBO Rep (2020) 21:e50162. doi: 10.15252/embr.202050162
48. Brockwell NK, Rautela J, Owen KL, Gearing LJ, Deb S, Harvey K, et al. Tumor Inherent Interferon Regulators as Biomarkers of Long-Term Chemotherapeutic Response in TNBC. NPJ Precis Oncol (2019) 3:21. doi: 10.1038/s41698-019-0093-2
49. Xu Z, Hurchla MA, Deng H, Uluckan O, Bu F, Berdy A, et al. Interferon-Gamma Targets Cancer Cells and Osteoclasts to Prevent Tumor-Associated Bone Loss and Bone Metastases. J Biol Chem (2009) 284:4658–66. doi: 10.1074/jbc.M804812200
50. Tulotta C, Lefley DV, Freeman K, Gregory WM, Hanby AM, Heath PR, et al. Endogenous Production of IL1B by Breast Cancer Cells Drives Metastasis and Colonization of the Bone Microenvironment. Clin Cancer Res (2019) 25:2769–82. doi: 10.1158/1078-0432.CCR-18-2202
51. Eyre R, Alferez DG, Santiago-Gomez A, Spence K, McConnell JC, Hart C, et al. Microenvironmental IL1beta Promotes Breast Cancer Metastatic Colonisation in the Bone via Activation of Wnt Signalling. Nat Commun (2019) 10:5016. doi: 10.1038/s41467-019-12807-0
52. Sethi N, Dai X, Winter CG, Kang Y. Tumor-Derived JAGGED1 Promotes Osteolytic Bone Metastasis of Breast Cancer by Engaging Notch Signaling in Bone Cells. Cancer Cell (2011) 19:192–205. doi: 10.1016/j.ccr.2010.12.022
53. Liang M, Ma Q, Ding N, Luo F, Bai Y, Kang F, et al. IL-11 is Essential in Promoting Osteolysis in Breast Cancer Bone Metastasis via RANKL-Independent Activation of Osteoclastogenesis. Cell Death Dis (2019) 10:353. doi: 10.1038/s41419-019-1594-1
54. Johnson RW, Finger EC, Olcina MM, Vilalta M, Aguilera T, Miao Y, et al. Induction of LIFR Confers a Dormancy Phenotype in Breast Cancer Cells Disseminated to the Bone Marrow. Nat Cell Biol (2016) 18:1078–89. doi: 10.1038/ncb3408
55. Hsu YH, Hsing CH, Li CF, Chan CH, Chang MC, Yan JJ, et al. Anti-IL-20 Monoclonal Antibody Suppresses Breast Cancer Progression and Bone Osteolysis in Murine Models. J Immunol (2012) 188:1981–91. doi: 10.4049/jimmunol.1102843
56. McGuire JJ, Frieling JS, Lo CH, Li T, Muhammad A, Lawrence HR, et al. Mesenchymal Stem Cell-Derived Interleukin-28 Drives the Selection of Apoptosis Resistant Bone Metastatic Prostate Cancer. Nat Commun (2021) 12:723. doi: 10.1038/s41467-021-20962-6
57. Udagawa N, Horwood NJ, Elliott J, Mackay A, Owens J, Okamura H, et al. Interleukin-18 (Interferon-Gamma-Inducing Factor) Is Produced by Osteoblasts and Acts via Granulocyte/Macrophage Colony-Stimulating Factor and Not via Interferon-Gamma to Inhibit Osteoclast Formation. J Exp Med (1997) 185:1005–12. doi: 10.1084/jem.185.6.1005
58. Park BK, Zhang H, Zeng Q, Dai J, Keller ET, Giordano T, et al. NF-kappaB in Breast Cancer Cells Promotes Osteolytic Bone Metastasis by Inducing Osteoclastogenesis via GM-CSF. Nat Med (2007) 13:62–9. doi: 10.1038/nm1519
59. Weilbaecher KN, Guise TA, McCauley LK. Cancer to Bone: A Fatal Attraction. Nat Rev Cancer (2011) 11:411–25. doi: 10.1038/nrc3055
60. Yu-Lee LY, Yu G, Lee YC, Lin SC, Pan J, Pan T, et al. Osteoblast-Secreted Factors Mediate Dormancy of Metastatic Prostate Cancer in the Bone via Activation of the TGFbetaRIII-P38mapk-Ps249/T252RB Pathway. Cancer Res (2018) 78:2911–24. doi: 10.1158/0008-5472.CAN-17-1051
61. Esposito M, Fang C, Cook KC, Park N, Wei Y, Spadazzi C, et al. TGF-Beta-Induced DACT1 Biomolecular Condensates Repress Wnt Signalling to Promote Bone Metastasis. Nat Cell Biol (2021) 23:257–67. doi: 10.1038/s41556-021-00641-w
62. Jiao S, Subudhi SK, Aparicio A, Ge Z, Guan B, Miura Y, et al. Differences in Tumor Microenvironment Dictate T Helper Lineage Polarization and Response to Immune Checkpoint Therapy. Cell (2019) 179:1177–90.e13. doi: 10.1016/j.cell.2019.10.029
63. Wortzel I, Dror S, Kenific CM, Lyden D. Exosome-Mediated Metastasis: Communication From a Distance. Dev Cell (2019) 49:347–60. doi: 10.1016/j.devcel.2019.04.011
64. Giannandrea D, Citro V, Lesma E, Bignotto M, Platonova N, Chiaramonte R. Restoring Tissue Homeostasis at Metastatic Sites: A Focus on Extracellular Vesicles in Bone Metastasis. Front Oncol (2021) 11:644109. doi: 10.3389/fonc.2021.644109
65. Loftus A, Cappariello A, George C, Ucci A, Shefferd K, Green A, et al. Extracellular Vesicles From Osteotropic Breast Cancer Cells Affect Bone Resident Cells. J Bone Miner Res (2020) 35:396–412. doi: 10.1002/jbmr.3891
66. Peinado H, Aleckovic M, Lavotshkin S, Matei I, Costa-Silva B, Moreno-Bueno G, et al. Melanoma Exosomes Educate Bone Marrow Progenitor Cells Toward a Pro-Metastatic Phenotype Through MET. Nat Med (2012) 18:883–91. doi: 10.1038/nm.2753
67. Lim PK, Bliss SA, Patel SA, Taborga M, Dave MA, Gregory LA, et al. Gap Junction-Mediated Import of microRNA From Bone Marrow Stromal Cells can Elicit Cell Cycle Quiescence in Breast Cancer Cells. Cancer Res (2011) 71:1550–60. doi: 10.1158/0008-5472.CAN-10-2372
68. Shupp AB, Neupane M, Agostini LC, Ning G, Brody JR, Bussard KM. Stromal-Derived Extracellular Vesicles Suppress Proliferation of Bone Metastatic Cancer Cells Mediated By Erk2. Mol Cancer Res (2021) 19:1763–77. doi: 10.1158/1541-7786.MCR-20-0981
69. Vardaki I, Corn P, Gentile E, Song JH, Madan N, Hoang A, et al. Radium-223 Treatment Increases Immune Checkpoint Expression in Extracellular Vesicles From the Metastatic Prostate Cancer Bone Microenvironment. Clin Cancer Res (2021) 27:3253–64. doi: 10.1158/1078-0432.CCR-20-4790
Keywords: bone metastasis, disseminated tumor cells, immune cells, chemokines, cytokines
Citation: Cheng X and Wang Z (2021) Immune Modulation of Metastatic Niche Formation in the Bone. Front. Immunol. 12:765994. doi: 10.3389/fimmu.2021.765994
Received: 28 August 2021; Accepted: 04 October 2021;
Published: 20 October 2021.
Edited by:
Fabrizio Mattei, National Institute of Health (ISS), ItalyReviewed by:
Eun Jeong Park, Mie University, JapanAndrea Alyssa McAlester, Baylor College of Medicine, United States
Copyright © 2021 Cheng and Wang. This is an open-access article distributed under the terms of the Creative Commons Attribution License (CC BY). The use, distribution or reproduction in other forums is permitted, provided the original author(s) and the copyright owner(s) are credited and that the original publication in this journal is cited, in accordance with accepted academic practice. No use, distribution or reproduction is permitted which does not comply with these terms.
*Correspondence: Zhan Wang, d2FuZ3poYW5oekB6anUuZWR1LmNu