- 1Division of Immunology, Department of Pathology, Faculty of Health Sciences, Institute of Infectious Diseases and Molecular Medicine (IDM), South African Medical Research Council (SAMRC) on Immunology of Infectious Diseases, University of Cape Town, Cape Town, South Africa
- 2International Centre for Genetic Engineering and Biotechnology, Cape Town Component, Cape Town, South Africa
- 3Faculty of Health Sciences, Wellcome Centre for Infectious Diseases Research in Africa (CIDRI), Institute of Infectious Diseases and Molecular Medicine (IDM), University of Cape Town, Cape Town, South Africa
- 4Centre for Biotechnology Research and Development, Kenya Medical Research Institute, Nairobi, Kenya
- 5Department of Molecular and Cell Biology, University of Cape Town, Cape Town, South Africa
- 6Institute of Virology and Immunobiology, University of Würzburg, Würzburg, Germany
IL-4 and IL-13 cytokines have been associated with a non-healing phenotype in murine leishmaniasis in L. mexicana -infected BALB/c mice as demonstrated in IL-4−/−, IL-13−/− and IL-4Rα-/- global knockout mouse studies. However, it is unclear from the studies which cell-type-specific IL-4/IL-13 signaling mediates protection to L. mexicana. Previous studies have ruled out a role for IL-4-mediated protection on CD4+ T cells during L. mexicana infections. A candidate for this role may be non-lymphocyte cells, particularly DCs, as was previously shown in L. major infections, where IL-4 production drives dendritic cell-IL-12 production thereby mediating a type 1 immune response. However, it is unclear if this IL-4-instruction of type 1 immunity also occurs in CL caused by L. mexicana, since the outcome of cutaneous leishmaniasis often depends on the infecting Leishmania species. Thus, BALB/c mice with cell-specific deletion of the IL-4Rα on CD11c+ DCs (CD11ccreIL-4Rα-/lox) were infected with L. mexicana promastigotes in the footpad and the clinical phenotype, humoral and cellular immune responses were investigated, compared to the littermate control. Our results show that CL disease progression in BALB/c mice is independent of IL-4Rα signaling on DCs as CD11ccreIL-4Rα-/lox mice had similar footpad lesion progression, parasite loads, humoral responses (IgE, IgG1, IgG 2a/b), and IFN-γ cytokine secretion in comparison to littermate controls. Despite this comparable phenotype, surprisingly, IL-4 production in CD11ccreIL-4Rα-/lox mice was significantly increased with an increasing trend of IL-13 when compared to littermate controls. Moreover, the absence of IL-4Rα signaling did not significantly alter the frequency of CD4 and CD8 lymphocytes nor their activation, or memory phenotype compared to littermate controls. However, these populations were significantly increased in CD11ccreIL-4Rα-/lox mice due to greater total cell infiltration into the lymph node. A similar trend was observed for B cells whereas the recruitment of myeloid populations (macrophages, DCs, neutrophils, and Mo-DCs) into LN was comparable to littermate IL-4Rα-/lox mice. Interestingly, IL-4Rα-deficient bone marrow-derived dendritic cells (BMDCs), stimulated with LPS or L. mexicana promastigotes in presence of IL-4, showed similar levels of IL-12p70 and IL-10 to littermate controls highlighting that IL-4-mediated DC instruction was not impaired in response to L. mexicana. Similarly, IL-4 stimulation did not affect the maturation or activation of IL-4Rα-deficient BMDCs during L. mexicana infection nor their effector functions in production of nitrite and arginine-derived metabolite (urea). Together, this study suggests that IL-4 Rα signaling on DCs is not key in the regulation of immune-mediated protection in mice against L. mexicana infection.
Introduction
Leishmaniases are classified as neglected tropical diseases (1, 2) that are caused by Leishmania spp; a vector-borne intracellular trypanosomatid protozoan parasite. This disease is characterized by a spectrum of clinical manifestations ranging from self-healing skin lesions of localized cutaneous leishmaniasis, mucocutaneous leishmaniasis, diffuse cutaneous leishmaniasis, and visceral leishmaniasis depending on Leishmania parasite species and host immune response (3, 4). Between 700,000 to 1 million new cases of leishmaniasis have been reported annually, with approximately 65,000 deaths (5). Additionally, the prevalence of leishmaniasis is estimated to be over 4.13 million globally and about 1.7 billion people are at risk of infection in more than 98 countries worldwide distributed in all continents except Antarctica (6–8). Cutaneous leishmaniasis (CL) mostly and widely occurs in tropical regions of Western and Central Asia, the Mediterranean basin, and America (5).
Several drugs currently in use for the treatment of various forms of leishmaniasis are known to cause side effects and require a long period of hospitalization for intravenous administration (9, 10). Despite sustained efforts, no effective human vaccine is yet available (11–14). A promising alternative is the use of chemoimmunotherapeutic approaches, which have the potential to increase efficacy and decrease the toxicity of antileishmanial drugs thereby extending their lifespan in clinical use (15). Central to this approach is the identification of correlates of immune protection in the host organism during active infection. Since the early ‘90s, the canonical T-helper (Th) cytokines, interleukin (IL)-4 and IL-13, have been infamously regarded as mediators of susceptibility to CL in mice. However, a paradigm change to this archetypal role was reported in 2001, where early administration of IL-4 during CL in BALB/c mice promoted Th1 immune response leading to the control of the disease (16). IL-4 and IL-13 share a common receptor, IL-4Rα, whose effects in CL have been widely studied in L. major (17, 18) infection and to some extent during L. mexicana infection (19). The phenotypic difference of IL-4-/- and IL-4Rα-/- from wildtype BALB/c mice after infection with L. mexicana indicated that gene knockout mice control the infection with characteristic smaller lesions and decreased parasite burden (19, 20) thereby suggesting a role for IL-4 and IL-13 in susceptibility to L. mexicana.
To better understand the specific cell subsets in the host that render IL-4Rα-/- mice resistant to CL, recent studies have shown that deficiency of IL-4Rα signaling on CD4+ T cells protects mice from L. major infections but the protection is sex-dependent in L. mexicana-infected BALB/c mice; with controlled infection in female mice, but persistent infection in male mice (21, 22). Further studies in our laboratory have shown that BALB/c mice lacking IL-4Rα signaling in dendritic cells (DCs) were hyper susceptible to cutaneous L. major infection in the footpad (17). These mice had upregulated Th2 responses and impaired macrophage killing capacity, pointing towards the critical role of IL-4Rα signaling on DCs for protection against CL caused by L. major. Mechanistically, this pointed to early IL-4 signaling instructing DC-derived IL-12 production early after infection, which contributes to the host to control infection. Given that IL-4Rα-/- mice are protected from L. mexicana infection, it could be possible that the absence of DC IL-4Rα signaling in IL-4Rα-/- mice infected with L. mexicana could be the primary contributor to protection. Thus, it is interesting to investigate the role of DCs in mice with IL-4Rα specific deletion in DCs following L. mexicana infection. Moreover, since the differences in the outcome of CL in BALB/c mice have been shown to depend on the infecting Leishmania species (23), we sought to investigate the importance of IL-4Rα signaling on DCs in the control of L. mexicana infection in BALB/c mice. To this end, we utilized BALB/c mice with a specific deletion of the IL-4Rα on DCs (17). Herein, we describe the clinical phenotype, humoral and cellular responses of BALB/c mice with IL-4Rα deficiency on DCs during experimental footpad infection with L. mexicana promastigotes. In summary, we show that CD11ccreIL-4Rα-/lox mice exhibited non-healing lesions similar to their littermate controls and unchanged parasite load in the footpad (FP) and lymph nodes (LN). Furthermore, we found no sex-related difference associated with L. mexicana infection in the absence of IL-4Rα expression on DCs, in contrast to that shown for CD4+ T cells (21). Importantly, similar type 2 and type 1 antibody production were observed between CD11ccreIL-4Rα-/lox and littermate IL-4Rα-/lox mice, conveying a sustained susceptible type 2 humoral responses compared to IL-4Rα-/lox mice. Surprisingly, although Th2 cytokine production was upregulated in CD11ccreIL-4Rα-/lox mice, this did not translate to heightened susceptibility. There was unchanged infiltration of myeloid cells (cDCs, macrophages, neutrophils, and monocyte-derived DCs) in the draining LN of CD11ccreIL-4Rα-/lox and littermate IL-4Rα-/lox mice. Interestingly, we found that IL-4-mediated DC instruction was unaltered in IL-4Rα-deficient bone marrow-derived dendritic cells (BMDCs) compared to IL-4Rα+ BMDCs. Accordingly, the loss of IL-4Rα signaling on CD11c+ cells did not alter the maturation or activation. nor the levels of IL-12p70, IL-10, nitrite and arginine-derived urea compared to IL-4Rα+ BMDCs. These findings, together, suggest that IL-4/IL-13-responsive DCs are dispensable in host resistance against L. mexicana infection. Moreover, IL-4-mediated instruction of DCs during infection appears to be pathogen and strain-specific in the context of L. major (24) and L. mexicana-induced cutaneous leishmaniasis in mice.
Materials and Methods
Mice
Six to eight week old, age and sex-matched IL-4Rα-/- (18), CD11ccreIL-4Rα-/lox and IL-4Ra-/lox (17) were used for L. mexicana challenge experiments. CD11ccreIL-4Rα−/lox mice were generated from CD11ccreC57/BL6 mice inter-crossed with IL4Rαlox/lox BALB/c mice (25) and homozygous IL-4Rα−/− BALB/c mice and backcrossed to BALB/c background for nine generations. CD11ccreIL4Rα−/lox mice have deficiency of IL-4Rα signaling on both DCs and alveolar macrophages (26). Transgene-bearing CD11ccreIL4Rα−/lox were identified by PCR genotyping as described previously (17). Hemizygous littermate (IL-4Rα-/lox) mice with functional one allele of IL-4Rα were used as controls in all experiments. All mice were bred and housed in a temperature/humidity-controlled room under a 12-hour light/12-hour dark cycle and maintained in cages with pressurized individually ventilated (PIV) cages under specific-pathogen-free conditions in the animal breeding facility of the University of Cape Town (UCT).
Ethical Statement
This study was performed according to the recommendations of the South African national guidelines and the University of Cape Town on practice for laboratory animal procedures. All experiments were done according to the Institutional Faculty of Health Sciences, Animal Research Ethics Committee (AEC) (AEC number: 019/001).
L. mexicana Parasite and Mice Infection
L. mexicana, LV4 parasites harvested from lesions in the FP of infected wild type (WT) BALB/c mice were cultured in M199 media (Invitrogen) supplemented with 10% Fetal calf serum (FCS) (Gibco) and 0.5% penicillin/streptomycin antibiotics (100 U/ml and 100 µg/ml, Gibco respectively). Parasites were left to grow at 27°C for 5-7 days until they fully differentiated into stationary-phase promastigotes (17).
Confluent parasites were washed with phosphate-buffered saline (PBS) by centrifugation, fixed in paraformaldehyde and counted in a Neubauer chamber. Mice were anesthetized with 12% ketamine (Anaket-V; Centaur Labs) and 8% xylazine (Rompun; Bayer). 2× 106 L. mexicana promastigotes in a final volume of 50 µl were humanely inoculated subcutaneously in the left hind footpad.
Measurement of Footpad Lesions
Mice were monitored daily, and lesion sizes of the left hind footpad were measured weekly using a Mitutoyo internal dial caliper gauge (Brütsch, Zu¨rich, Switzerland) and compared to a baseline of 2mm for 8 weeks. Weights of mice were also measured weekly to assess their health status.
Quantification of Parasite Using Limiting Dilution Assay
At 8 weeks post-infection, mice were euthanized and parasite quantification in the infected footpad and draining popliteal lymph nodes (pLNs) were performed using limiting dilution as previously described (27). Briefly, the homogenates of footpad tissues were prepared and resuspended in M199 media, whereas single lymph node cell suspensions were prepared by mechanical digestion of pLNs and passing through 40µm cell strainers. LN cells were washed through with DMEM supplemented with 10% FCS, 1M HEPES, 50mM β-mercaptoethanol, and penicillin/streptomycin (100 U/ml/100µg/ml, respectively, Gibco) and 200ul of the footpad, lymph node homogenates were added into respective neat wells for each mouse in a 96-well plate containing 100µl of complete DMEM. A serial 1:2 dilution was made for FP homogenates and LN cell suspensions and plates were incubated at 26°C for 7 days after which they were read microscopically to determine the lowest dilution at which parasites were observed (2n).
Analysis of Myeloid and Lymphoid Cells by Flow Cytometry
Single-cell suspension of 1×106 cells from the draining pLNs were stimulated with 200 µg/ml phorbol myristate acetate (PMA) and 1mg/ml ionomycin for 2 hours followed by 4 hours incubation with 2mM monensin. The cells were stained with 50 µl FACs buffer containing 1% rat serum, 1% FC-γ blocker (clone 2.4G2) (homemade), and a cocktail of fluorophore-conjugated antibodies for 30 minutes at 4°C in the dark. Antibodies used included surface extracellular staining markers: Ly6C-PerCPCy5.5 (clone AL-21), CD11b-V450 (clone M1/70), MHCII-AF700 (clone, M5/114), CD11c-APC (clone, HL3), F4/80- PE-Cy7 (clone, BM8), CD44-FITC (clone IM7), CD4-V500 (clone RM4-5), CD3-A700 (clone 500A2), CD62L-V450 (clone MEL-14), CD19-PerCPCy5.5 (clone 1D3), CD8-APC (clone 53-6.7) (BD Biosciences). For intracellular cytokine staining, single-cell suspensions were fixed in 4%paraformaldehyde and permeabilized with 0.5% saponin buffer and stained with IFN-γ-AF700 (clone XMGL2), IL-4-APC (clone 11B11), and IL-13-PE (clone eBio13A) (BD Biosciences). 100,000 events were acquired on a BD Fortessa (BD flow Biosciences). Data were obtained as a percentage of the total cells acquired. The data were analyzed using FlowJo software version 10 (TreeStar). Absolute cell numbers were calculated as the product of the cell percentage and the total number of cells counted by trypan blue exclusion method in the isolated pLN.
Lymph Node Cell Stimulation and Cytokine Detection
1 × 106 cells resuspended in DMEM supplemented with 10% FCS and penicillin/streptomycin (100 U/ml/100 µg/ml, respectively) were cultured in 48 well plates coated with 20 µg/ml αCD3 or stimulated with 8x106 heat killed L. mexicana promastigotes at 37°C with 5% CO2 for 72 hrs. Concentrations of IFN-γ, IL-4 (BD Biosciences), IL-13 (R&D Systems) were detected in the supernatants using ELISA as previously described (17).
Detection of Leishmania mexicana Specific -IgG1, IgG2a, IgG2b, and Total IgE
Leishmania mexicana specific-IgG1, IgG2a, and IgG2b were detected in the serum of infected mice using 10 µg/ml coating of L. mexicana soluble Leishmania antigens (SLA) in coating buffer and detected using AP-conjugated specific antibodies (Southern Biotech) by ELISA, as previously described (28). Total IgE antibody ELISA was detected in the serum of infected mice by capture ELISA using 1 µg/ml of coating IgE mAb and biotinylated rat anti-mouse IgE (Southern Biotech) as previously described (17).
Bone Marrow Derived DCs
Generation of BMDCs was performed as described by Lutz et al. (29) with slight modifications. Briefly, 2×106 of bone marrow cells, harvested from femurs and tibia of CD11ccreIL-4Rα-/lox BALB/c mice and their littermate controls, were differentiated into BMDCs in presence of complete RPMI1640 media (10% FCS, 0.5% of 100 U/ml penicillin/100µg/ml streptomycin antibiotics) supplemented with 50% GM-CSF supernatant (R10) and incubated at 37°C in 5% CO2 for 10 days. 5 ml of fresh R10 media were added to the cells at day 3 and 6 of incubation. On day 10, non-adherent cells were gently collected and counted by trypan blue exclusion method (30).
Subsequently, 5×105 cells were seeded into 48 well plates and stimulated with 1 µg/ml of LPS or L. mexicana promastigotes at ratio of 10:1 (i.e 5×106 parasite to 5×105 cells) in the presence or absence of [1000 U/ml] recombinant mouse IL-4 (BD Biosciences) at 37°C, 5% CO2 for 48 hrs. The samples were gently pipetted to dissociate DC clusters and to promote contact between parasites and DCs. After 48 hrs, culture supernatant was collected for nitrite assay and pelleted cells were used for determination of urea as described by previously (17). Levels of IL-12 and IL-10 were determined, maturation and activation were assessed by flow cytometry using fluorochrome conjugated antibodies.
Statistical Analysis
Statistical analysis was performed using a one-way ANOVA test with Bonferroni post-test for multiple comparisons, and results are presented as means± standard errors of the means (SEM). Each experimental group was compared to IL-4Rα-/lox littermate controls. Statistical p values of *p < 0.05, **p < 0.01 and ***p< 0.001 were considered significant.
Results
Susceptibility to L. mexicana in BALB/c Mice Is Independent of IL-4Rα Signaling on CD11c+ Cells
To determine the effects of the absence of IL-4Rα on CD11c+ cells in the host response following L. mexicana infection, CD11ccreIL-4Rα-/lox, global IL-4Rα-/- and IL-4Rα-/lox (littermate control) BALB/c mice were infected with 2×106 promastigotes in the hind footpad, and footpad swelling was measured weekly for 8 weeks using a vernier caliper.
Similar to previous findings (18, 19) our data show that global IL-4Rα-/- female mice-controlled footpad (FP) swelling in response to L. mexicana (Figure 1A). In contrast, female CD11ccreIL-4Rα-/lox showed fulminant cutaneous leishmaniasis characterized by increasing footpad swelling similar to IL-4Rα-/lox littermate control BALB/c mice during the 8week period (Figure 1A). Enumeration of parasites in the footpad and lymph node was performed using a limiting dilution assay (17). Female BALB/c mice deficient for IL-4Rα on CD11c+ DCs had similar numbers of parasites in their lesions and pLNs compared to their littermate controls at week 8 post-infection (p.i) (Figure 1B). In contrast, IL-4Rα-/- mice had consistently lower parasite burdens in female mice in the FP and dLN compared to littermate control and CD11ccreIL-4Rα-/lox mice (Figure 1B). Similarly, male CD11ccreIL-4Rα-/lox mice displayed non-healing footpad swelling with characteristic systemic parasite dissemination into pLN that was comparable to littermate IL-4Rα-/lox controls (Figures 1C, D). Notably, there was no significant difference in kinetics of FP swelling between male and female mice, but a slightly enhanced FP swelling was observed in female mice (Figure 1E).
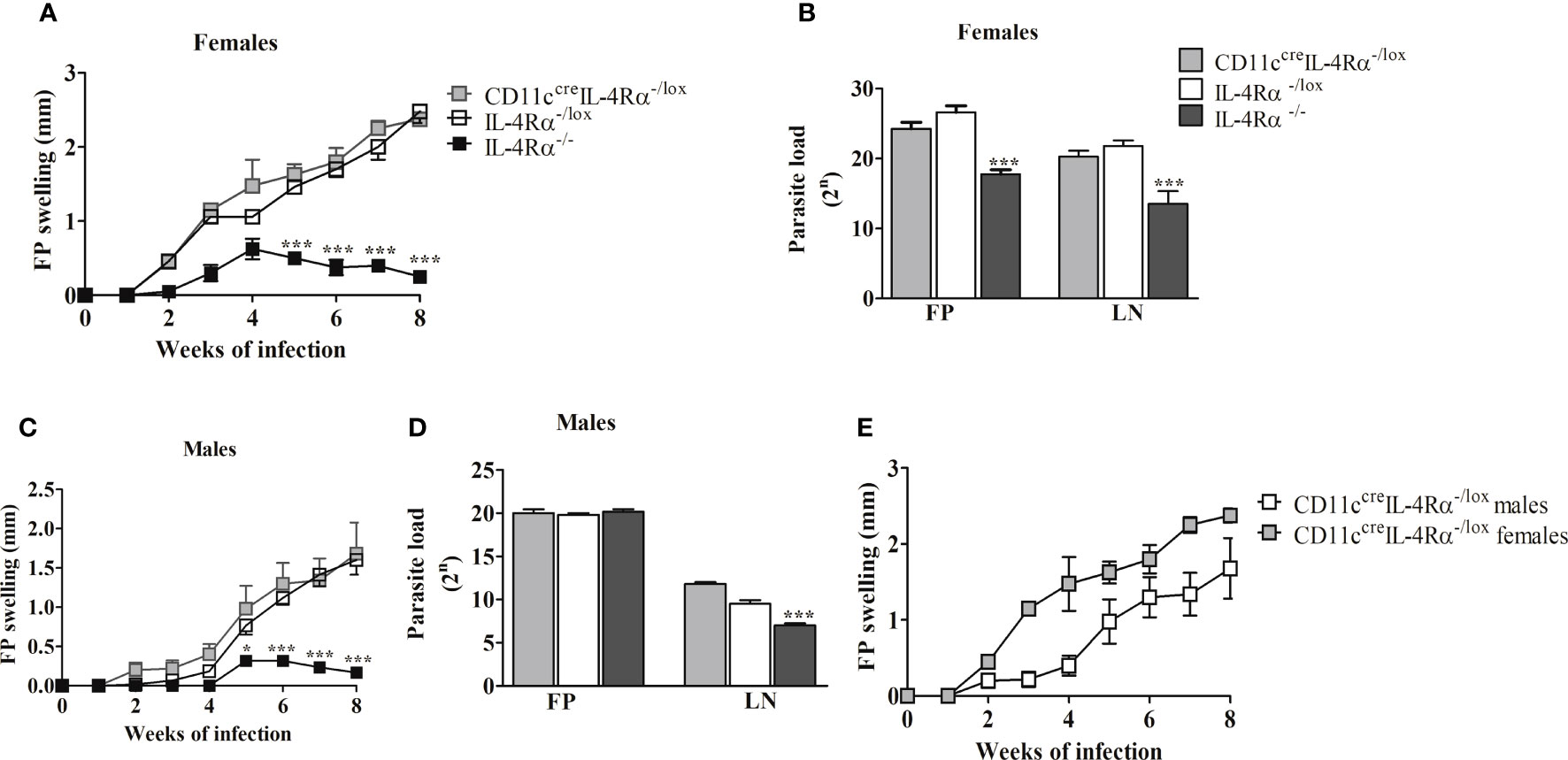
Figure 1 │The Footpad (FP) swelling and parasite burden of CD11CcreIL-4Rα-/lox, IL-4Rα-/lox, and IL-4Rα-/- mice subcutaneously infected with L. mexicana in the hind footpad. Fp swelling of CD11ccreIL-4Rα-/lox and IL-4Rα-/- BALB/c mice were compared to IL-4Rα-/lox control using one-way ANOVA (Bonferroni post-test). FP swelling in female (A) and male (C), comparison of Fp swelling between male and female mice (E), and parasite burden in both FP and lymph node (LN) of female (B) and male mice (D). Data are presented as the mean ± SEM of 4-6 mice, and P values of ≤ 0.05 were considered significant (*P ≤ 0.05; ***P ≤ 0.001). Data from two experiments are shown.
Overall, these findings suggest that the expression of IL-4Rα on CD11c+ DCs is dispensable in conferring susceptibility to male and female BALB/c mice infected with L. mexicana parasites in the footpad. Given this susceptibility was independent of sex, we focused on the analysis of humoral and cellular responses in female CD11ccreIL-4Rα-/lox mice bearing in mind that in previous studies, cell-specific deficiency of IL-4Rα (on CD4+ T cells) was particularly relevant in female mice (21).
The Absence of IL-4Rα Signaling on CD11c+ DCs Does Not Alter Humoral Immune Responses in BALB/c Mice Following L. mexicana Infection
Since CD11ccreIL-4Rα-/lox and IL-4Rα-/lox mice displayed non-healing lesion progression (Figure 1A), we determined whether the infected mice had a skewed type 2 immune response by first evaluating the levels of type 2-associated IgE and IgG1 (31) in contrast to type 1-associated IgG2a/IgG2b in sera (32). ELISA analysis demonstrated no difference in either total IgE (Figure 2A), antigen-specific IgG1 (Figure 2B), antigen-specific IgG2b (Figure 2C) or IgG2a (Figure 2D) production between L. mexicana-infected CD11ccreIL-4Rα-/lox BALB/c mice and littermate controls. In contrast, IL-4Rα-/- mice demonstrated a skewed type 1 humoral response evidenced by reduced IgE and IgG1 (Figures 2A, B) and concomitantly increased IgG2a and IgG2b (Figures 2C, D). These data highlight the correlation between systemic type 2 antibody responses and susceptibility to CL caused by L. mexicana. Furthermore, the development of type 2 antibody responses in non-healing cutaneous leishmaniasis occurs independently of IL-4Rα signaling on DCs. An increase in absolute numbers of B cells in both CD11ccreIL-4Rα-/lox and IL-4Rα-/lox compared to IL-4Rα-/- mice, thus suggesting that B cells play a role in enhancing the pathogenesis during L. mexicana infection (Figures 2E, F).
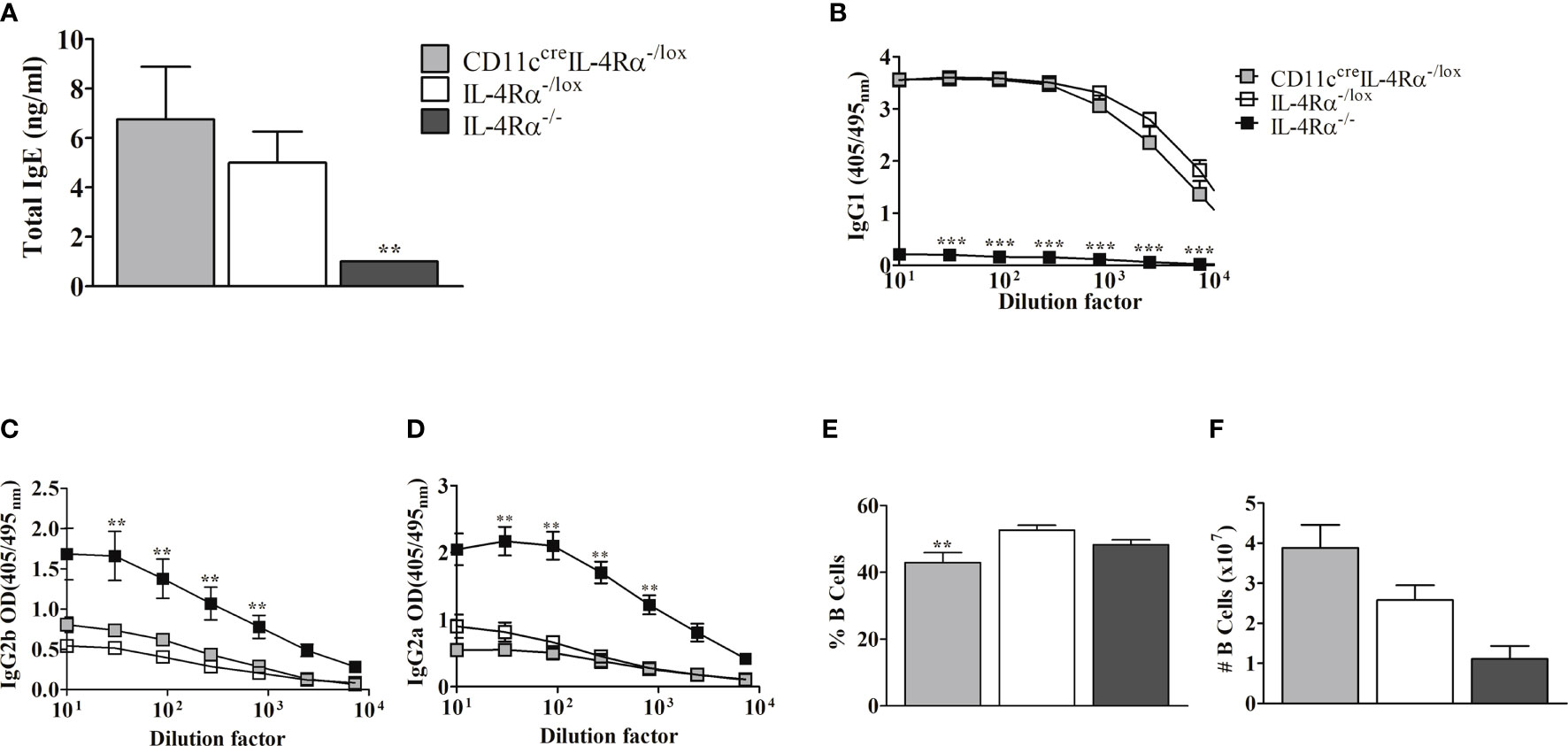
Figure 2 Serum levels of antibody responses and B cell population following infection of CD11ccreIL-4Rα-/lox, IL-4Rα-/lox, and IL-4Rα-/- BALB/c female mice with L. mexicana. Total IgE (A) antibodies levels in serum were compared between CD11ccreIL-4Rα-/lox, IL-4Rα-/lox, and IL-4Rα-/- BALB/c mice. Leishmania Ag-specific IgG1 (B), IgG2b (C), and IgG2a (D) were measured. The frequency and absolute cell numbers of B lymphocytes were also enumerated from FACs analysis (E, F respectively). Data represent means plus SEM values from one experiment representative of two performed is shown. And P values of ≤.01 were considered significant (**P ≤ 0.01; ***P≤ 0.001). Error bars represent the mean ± SE of 4-6 mice per group.
Type 2 Cytokine Responses Are Increased in CD11ccreIL-4Rα-/lox but Did Not Confer Hyper-Susceptibility Over Littermate IL-4Rα-/lox Mice
Susceptibility and resistance to CL in mice are dependent on Th1/Th2 immune responses (33). Therefore, we investigated the role of IL-4Rα signaling on DCs in the cellular response to L. mexicana infection by evaluating the cytokine profile (IFN-γ, IL-4, and IL-13) in infected mice by ELISA.
At week 8 p.i the amount of IFN-γ secreted by pLN cells of female mice was similar between CD11ccreIL-4Rα-/lox and IL-4Rα-/lox mice but lower compared to IL-4Rα-/- mice (Figure 3A). The higher levels of IFN-γ in IL-4Rα-/- mice correlated with the lower FP swelling seen in female mice (Figure 1A). Additionally, we investigated whether type 2 cytokines in CD11ccreIL-4Rα-/lox and control mice were responsible for susceptibility. On analysis of cytokine from LN cells stimulated with αCD3, we surprisingly found that IL-4 secretion by lymph node cells was significantly increased in CD11ccreIL-4Rα-/lox as compared to IL-4Rα-/lox and IL-4Rα-/- mice (Figure 3B). Notably, there were also increased IL-13 levels in CD11ccreIL-4Rα-/lox as compared to IL-4Rα-/lox and IL-4Rα-/- mice although no statistically significant differences were observed (Figure 3C). To supplement the αCD3 stimulated cytokine secretion by LN cells, we restimulated lymph node cells with 8x106 heat killed L. mexicana promastigotes in order to investigate the antigen-specific cytokine responses. The data show similar levels of IFN-γ but an increasing trend of IL-4 in CD11ccreIL-4Rα-/lox mice compared to their littermate controls (Figures 3D, E). In addition, IL-13 levels were significantly increased in CD11ccreIL-4Rα-/lox compared to their littermate controls (Figure 3F). These findings suggest that IL-4/IL-13 signaling through IL-4Rα on DCs does not influence the production of host-protective cytokines (IFN-γ) during L. mexicana infection, however, IL-4Rα deficiency on DCs does enhance IL-4 and IL-13 secretion. Interestingly, Hurdayal et al. (17) reported comparable findings with regard to IL-4 and IL-13 levels, which translated to hypersusceptibility to L. major yet surprisingly, in the study herein, this does not translate to hypersusceptibility to L. mexicana suggesting that CL caused by different Leishmania species leads to different phenotypic outcomes (17).
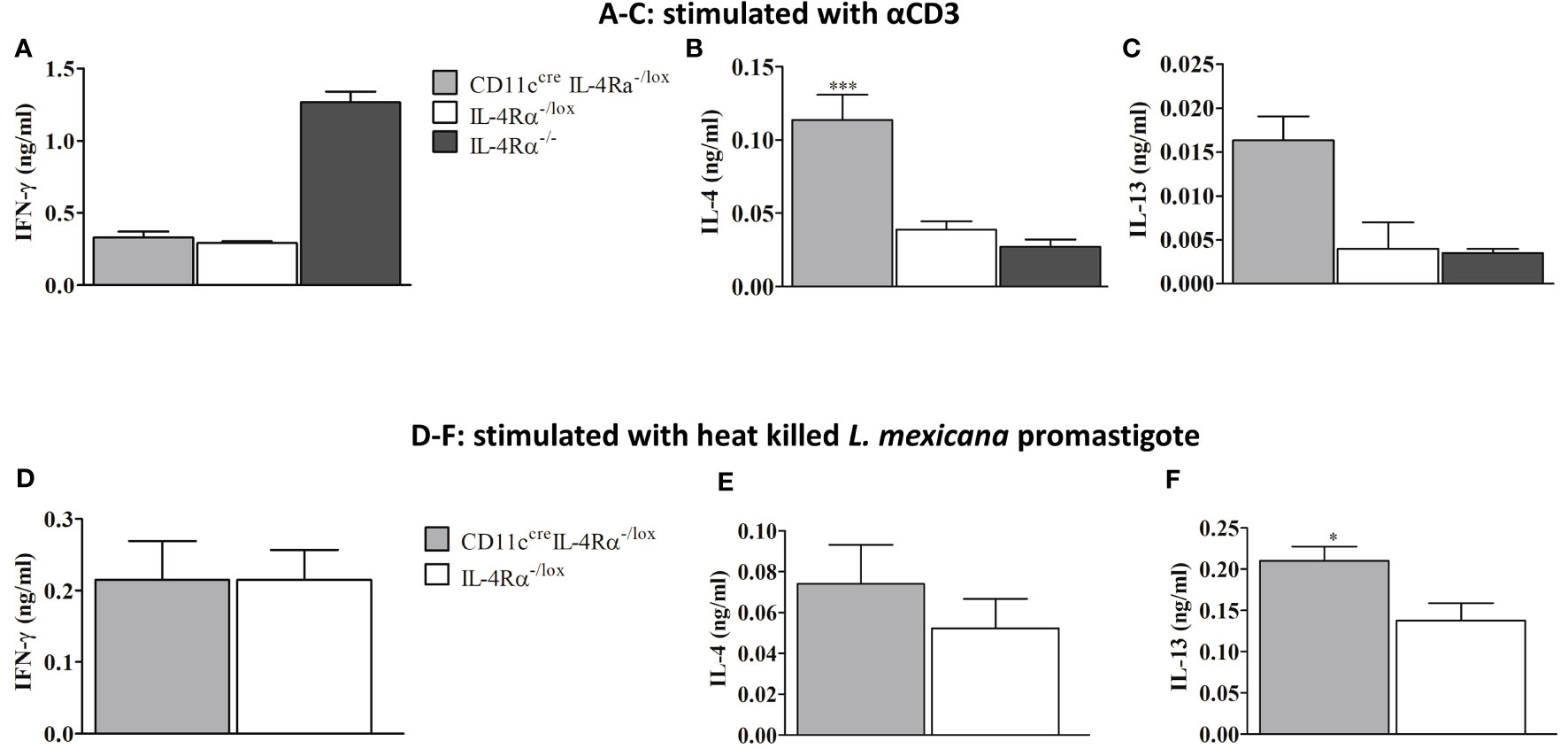
Figure 3 Cytokine response in L. mexicana-infected CD11ccreIL-4Rα-/lox, IL-4Rα-/lox, and IL-4Rα-/- BALB/c mice. Cells from draining Lymph nodes (dLNs) of experimental female mice injected subcutaneously with L. mexicana promastigotes at week 8 p.i were stimulated with α-CD3 and heat killed L. mexicana promastigotes. IFN-γ, IL-4, and IL-13 cytokines were measured using ELISA. The levels of IFN-γ (A), IL-4 (B), and IL-13 (C) secretion by dLN cells stimulated with α-CD3 were shown. Levels of IFN-γ (D), IL-4 (E) and IL-13 (F) cytokines secretion by cells stimulated with heat killed L. mexicana promastigotes was also shown. Data represent means and SEM of values of one experiment representative of two performed is shown. Statistical analysis was performed using one-way ANOVA comparing the differences to IL-4Rα-/lox BALB/c mice as significant (*P ≤ 0.05; ***p = 0.001).
Loss of IL-4Rα Signaling on DCs Does Not Change the Expansion and Activation of CD4+ and CD8+ T Cells During L. mexicana Infection
Next, we sought to determine if the absence of IL-4Rα+ DCs in CD11ccreIL-4Rα-/lox mice altered the abundance and involvement of CD4+ and CD8+ T lymphocytes in the draining pLN following L. mexicana infection compared to those of IL-4Rα-/lox mice by flow cytometry analysis (Supplementary Figure 1). Overall, lymph node cells comprised a similar percentage of both CD4+ and CD8+ T cells in CD11ccreIL-4Rα-/lox and IL-4Rα-/lox female mice (Figures 4A, B). In contrast, we found that the percentage of CD4+ T cells in LN of IL-4Rα-/- mice was significantly increased (Figure 4A) whereas the CD8+ T cell percentage was significantly decreased (Figure 4B). In terms of effector T cell function, there were no significant differences in CD4+ T cell activation (TA), effector memory (TEM), and central memory (TCM) subsets between CD11ccreIL-4Rα-/lox mice and their littermate controls (Figure 4A). In contrast, activated CD4+ T cells and EM in IL-4Rα-/- were showing an increasing trend in CD11ccreIL-4Rα-/lox mice whilst TCM levels were similar to IL-4Rα-/lox mice (Figure 4A). With regards to CD8+ T cells, no differences in frequency of both activated, central, and effector memory CD8+ T cells were found in CD11ccreIL-4Rα-/lox female mice compared to their littermate controls (Figure 4B). Contrary to this, IL-4Rα-/- mice had a significantly decreased percentage of activated, central, and effector memory CD8+ T cells in comparison to CD11ccreIL-4Rα-/lox and littermate controls (Figure 4B). Notably, the percentage of naïve CD4+ and CD8+ T cells in all three groups was not different in LN during L. mexicana infection (Figures 4A, B) highlighting that loss of IL-4Rα signaling on DCs does not alter the expansion of T cell with a naïve phenotype in the LN during L. mexicana infection.
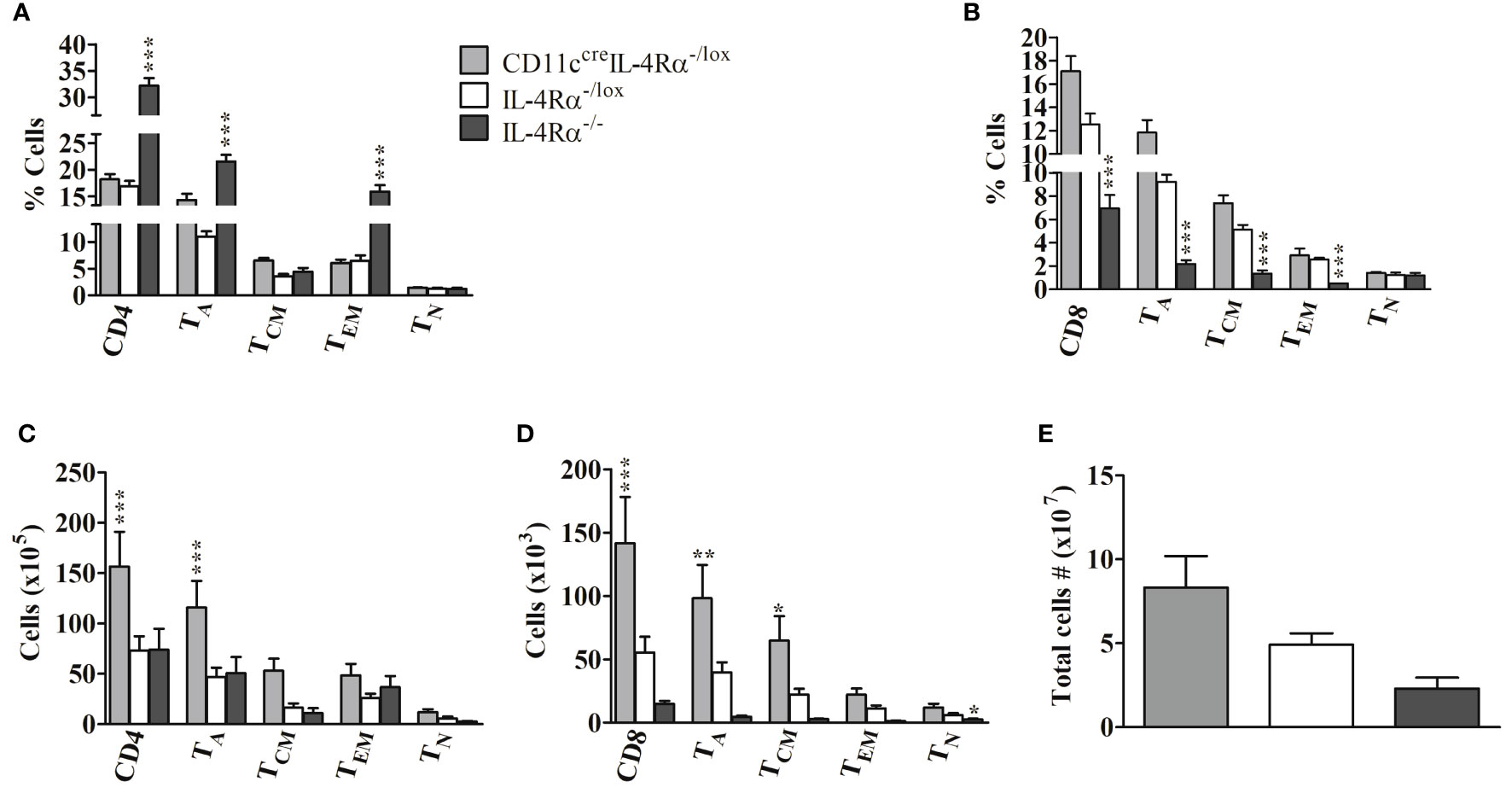
Figure 4 Frequency of lymphocytes during L. mexicana infection in CD11ccreIL-4Rα-/lox, IL-4Rα-/lox, and IL-4Rα-/- mice. Mice were infected subcutaneously with 2×106 L. mexicana promastigotes into the hind footpad. At week 8 after infection, frequency of CD4+ (A) and CD8+ (B) T cells with their activated (TA), central memory (TCM), effector memory (TEM), and Naïve T cells (TN) T cells were enumerated in the LNs by flow cytometry. Absolute numbers of CD4+ (C) and CD8+ (D) T cells were calculated and presented. Cells were differentiated based on following markers; CD4+ T cells (CD4+CD3+), CD8+ T cells (CD8+CD3+), TA (CD4+CD8+CD44+), TCM (CD4+/CD8+CD44+CD62L+), TEM T cells (CD4+/CD8+CD44+CD62L-) and TN (CD4+/CD8+CD44-CD62L+). Total cell numbers in the LN were also evaluated (E). Data from one experiment representative of two performed are shown. Statistical analysis was performed using one-way ANOVA comparing differences to IL-4Rα-/lox BALB/c mice as significant (*P ≤ 0.05; **p ≤0.01; ***p ≤ 0.001).
Considering absolute cell numbers in the LNs, CD11ccreIL-4Rα-/lox mice generally demonstrated an increase in CD4+ and CD8+ T cells, activation, and central memory phenotype as compared to littermate controls and IL-4Rα-/- mice (Figures 4C, D). The absolute cell number is a product of percentages (obtained from flow cytometry) and total cell numbers infiltrating the draining pLN (enumerated by trypan blue exclusion method). Total cell numbers showed an increased trend in the LN of CD11ccreIL-4Rα-/lox mice compared to their littermate controls (Figure 4E), hence this could be the reason why absolute numbers of CD4+ and CD8+ T cells were increased in pLN of CD11ccreIL-4Rα-/lox mice. Interestingly, absolute numbers of activated CD8+ and memory phenotypes were significantly lower in IL-4Rα-/- compared to CD11ccreIL-4Rα-/lox and littermate controls (Figures 4C, D). No differences were shown in absolute numbers of CD4+ and CD8+ T cells with naïve phenotype in all three groups but naïve CD4+ and CD8+ T cells were consistently reduced in IL-4Rα-/- mice (Figures 4C, D). Primarily, no statistical differences in total LN cell numbers were shown between the three groups, however, there was a trend in the increased number total cells infiltrating the popliteal dLN in CD11ccreIL-4Rα-/lox compared to IL-4Rα-/lox and IL-4Rα-/- mice (Figure 4E).
Mice Without IL-4Rα Signaling on DCs Display Heightened Levels of Th2-Derived IL-4 and IL-13 Cytokines in Response to L. mexicana
Despite the absence of IL-4 signaling on DCs and increased IL-4 and IL-13 secreting potential of pLN cells (Figures 3B, C), CD11ccreIL-4Rα-/lox mice did not show augmented CL as previously reported during L. major infection (17). In that study, hypersusceptibility to L. major in CD11ccreIL-4Rα-/lox mice was linked particularly to increased IL-4 and IL-13 secretion by CD4+ T cells because of impaired IL-4-mediated DC instruction (16, 17). Herein, we, therefore, investigated IL-4 and IL-13 production by CD4+ and CD8+ T cells in CD11ccreIL-4Rα-/lox mice, in an attempt to corroborate increased IL-4/IL-13 in LN cytokine data and explain comparable disease progression observed between CD11ccreIL-4Rα-/lox mice and their littermate controls.
In terms of frequency of cytokine-producing cells, our study showed no significant changes in the percentage of CD4+ and CD8+ T cells producing IFN-γ, IL-4, and IL-13 in both CD11ccreIL-4Rα-/lox and IL-4Rα-/lox female mice in contrast to increased IFN-γ, and decreased IL-4 and IL-13 observed in IL-4Rα-/- mice (Figures 5A, B). The latter explains the ability of IL-4Rα-/- mice to control infection induced by L. mexicana as previously reported (21). Notably, there were increased absolute numbers of CD4+ and CD8+ T cells secreting IL-4 and IL-13 in CD11ccreIL-4Rα-/lox compared to IL-4Rα-/lox (Figures 5C, D). We also found that absolute numbers of CD4+ T cells producing IFN-γ were comparable amongst the three mouse strains (Figure 5C), however, we show significantly decreased CD8+ T cells producing IFN-γ in IL-4Rα-/- mice compared to CD11ccreIL-4Rα-/lox and IL-4Rα-/lox mice (Figure 5D), perhaps due to increased total cell numbers in the pLNs of these mice (Figure 4E). Despite the increase in type 2 cytokines by absolute numbers of CD4+ and CD8+ T cells, the CD11ccreIL-4Rα-/lox mice did not show hypersusceptibility as documented previously (17). Potentially, the increased IFN-γ produced by CD4+ T cells in CD11ccreIL-4Rα-/lox may have been counteracted by the elevated IL-4 and IL-13 (Figures 5C, D). Altogether, our findings provide supporting evidence that the absence of IL-4-mediated DC instruction does induce higher levels of IL-4 and IL-13 by CD4+ and CD8+ T cells and that infection control in this L. mexicana setting is dependent on IFN-γ production by T cells which counteracts IL-4 and IL-13 for the survival of the parasites, as observed in the IL-4Rα-/- mice.
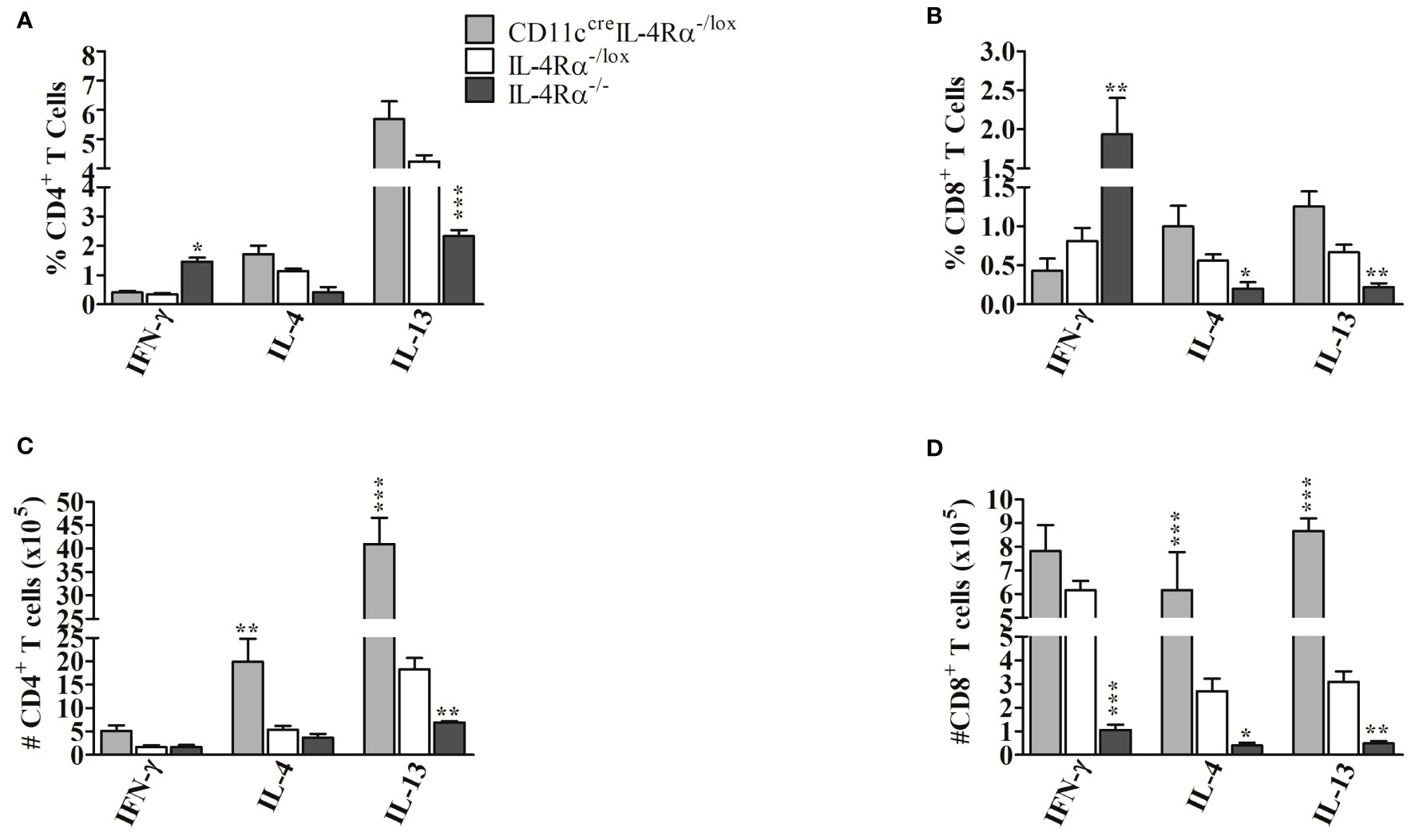
Figure 5 Cytokine producing CD4+ and CD8+ T cells from CD11ccreIL-4Rα-/lox, IL-4Rα-/lox and IL-4Rα-/- female mice infected with L. mexicana. Female mice were injected subcutaneously in the footpad with 2×106 L. mexicana for 8 weeks. 1×106 cells were isolated from popliteal lymph node cells and stimulated with PMA, ionomycin for 2 hours followed by 4hrs incubation with monensin. Percentage CD4+ (A), CD8+ (B), total CD4+ (C), total CD8+ (D) cytokine-producing T cells were determined by flow cytometry. Statistical analysis was performed using one-way ANOVA comparing the differences to IL-4Rα-/lox BALB/c mice as significant (*p ≤ 0.05; **p ≤ 0.01; ***p ≤ 0.001). Results are from one experiment, a representative of two performed is shown (n=4-6 mice).
The Deficiency of IL-4Rα Signaling on DCs Does Not Interfere With the Infiltration of Myeloid Cells Into LN During L. mexicana Infection
Since myeloid cells are important for replication and clearance of Leishmania, we investigated whether IL-4Rα deficiency on DCs may have modulated myeloid cell recruitment into the LN (24). Infiltration of myeloid cells into LN therefore evaluated for both percentage and total cell numbers using flow cytometry analysis. The cells were identified by gating as shown in Supplementary Figure 2. F4/80+/CD11c+CD11b+ can be a typical gate for monocyte-derived DCs (Mo-DCs) with an inflammatory phenotype and monocyte origin, shown to promote CL disease in cell specific DC-IL-4Rα deficient mice (24, 34, 35). CD11ccreIL-4Rα-/lox mice had similar percentages of macrophages, cDCs, neutrophils, and Mo-DCs within the LN compared to littermate controls (Figures 6A–D) suggesting that the deletion of IL-4Rα in CD11c+ DCs did not affect infiltration of these cells in pLN. However, the percentage of macrophages, DCs, neutrophils and monocyte-derived DCs (Mo-DCs) were significantly reduced in global IL-4Rα-/- compared to CD11ccreIL-4Rα-/lox mice and their littermate controls (Figures 6A–D). Considering the absolute cell numbers, CD11ccreIL-4Rα-/lox female mice exhibited an increase in DCs in comparison to their littermate controls whereas macrophages and neutrophil numbers were similar (Figures 6E–H). Again, akin to percentages, there was a considerably significant reduction in absolute numbers of macrophages, cDCs, and neutrophils in IL-4Rα-/- mice (Figures 6E–H). Altogether, these data indicate that IL-4Rα+ expression on CD11c+ DCs does not alter the trafficking and expansion of macrophages, cDCs, neutrophils, and Mo-DCs in the draining pLN during L. mexicana infection.
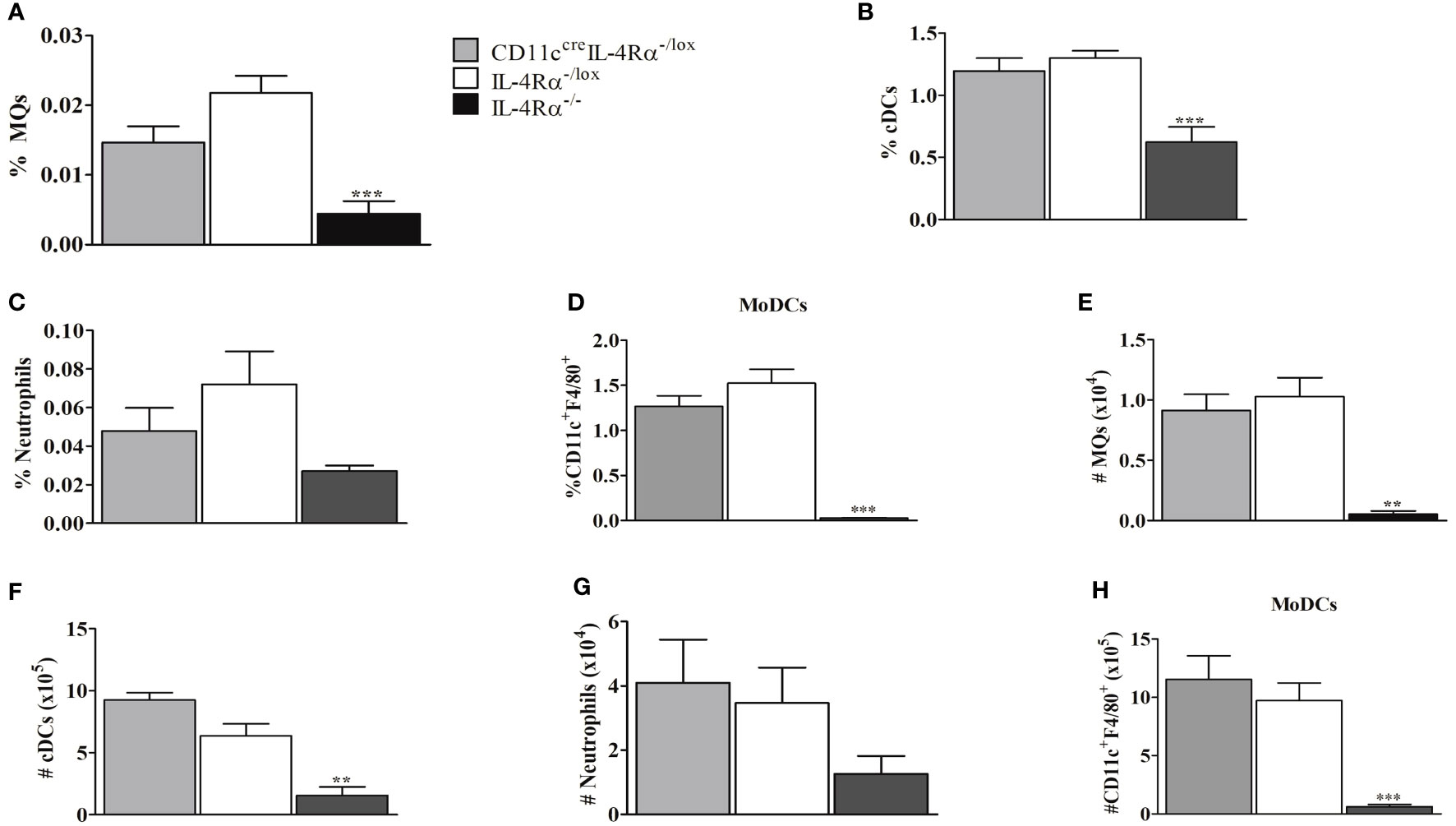
Figure 6 Myeloid cell populations in LNs from CD11ccreIL-4Rα-/lox, IL-4Rα-/lox, and IL-4Rα-/- mice infected with L. mexicana. Mice were injected into the footpad with 2×106 L. mexicana metacyclic promastigotes and at 8 weeks post-infection the cells were isolated from LNs and characterized by flow cytometry. The frequency of macrophage (A), conventional DCs (cDC) (B), neutrophils (C) and monocyte-derived DCs (Mo-DCs) (D) infiltration into LNs were determined. Absolute cell numbers were calculated by multiplying the percentages and total cell numbers in LNs as shown in (E–H). Activated macrophages (MQs) were identified as (CD11b+CD11c-F4/80+MHCII+), cDCs (CD11b+CD11c+MHCII+), Neutrophils (Neu) (CD11b+L6G+), and Mo-DCs (CD11b+CD11c+F4/80+). Statistical analysis was performed using one-way ANOVA comparing the differences to IL-4Rα-/lox BALB/c mice as significant (**P ≤ 0.01; ***p ≤0.001). Results are representative of one of the two experiments.
IL-4 Mediated DC Instruction Is Unaltered in CD11ccreIL-4Rα-/lox Mice Compared to Their Littermate Controls During L. mexicana Infection
We have shown that CD11ccreIL-4Rα-/lox mice had increased IL-4 and IL-13 compared to their littermate controls. IL-4 is known to modulate DC instruction by the production of IL-12 via IL-4Rα signaling or impairment of IL-10 (16, 36). To investigate DC instruction in L. mexicana infection, we generated BMDCs from CD11ccreIL-4Rα-/lox and IL-4Rα-/lox mice. BMDCs were infected and treated with either IL-4 or lipopolysaccharide (LPS) for 48 hours, IL-12p70 and IL-10 cytokine levels were measured in the supernatants. IL-4 stimulation did not alter the maturation and activation of BMDCs as shown by unchanged proportions of MHCII and CD80 respectively, between the two groups (Figures 7A–D). Moreover, IL-4Rα -deficient and IL-4Rα sufficient BMDCs produced similar levels of IL-12p70 and IL-10 during infection (Figures 7E, F) hence suggesting that IL-4-mediated DC instruction was not impaired in CD11ccreIL-4Rα-/lox.
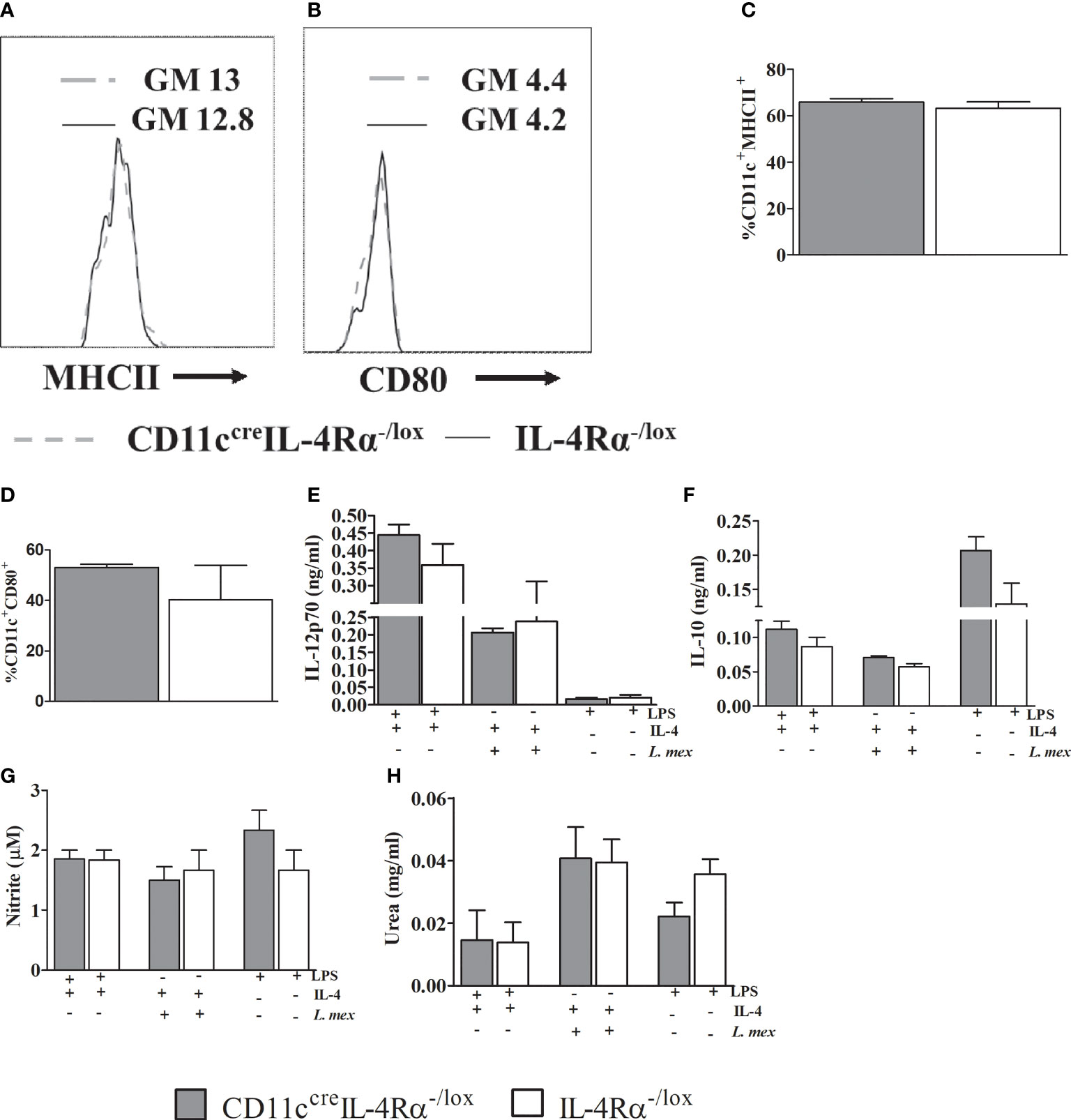
Figure 7 Abrogation of IL-4Rα expression on BMDCs does not either affect instruction or the DC phenotype in vitro. Bone marrow cells harvested from the mice were differentiated in RPMI 1640 containing 50% GMCSF supernatant incubated at 37°C, 5 % CO2 for 10 days. 5x105 cells were treated with IL-4 in presence of 1 μg/ml LPS or L. mexicana parasites (MOI 10:1). Cells stimulated with LPS only were used as controls. After 48hrs of incubation cells, supernatants were collected for analysis of MHCII (A, C), CD80 (B, D). Histogram showing geometric mean (GM) and % expression of MHCII and CD80 on CD11C+ cells is shown. The GM of IL-4Rα-/lox is shown by solid line and CD11CcreIL-4Rα-/lox by dashed grey line. IL-12p70 (E) and IL-10 (F) were measured using ELISA. Supernatants were also used for nitrite assay (G) and pelleted cells were used for arginase activity by measuring quantities of urea (H). Data was analysed using one way ANOVA and unpaired student t-test defining differences to IL-4Rα-/lox BMDCs as significant (p ≤ 0.05).
Nitric oxide (NO) and arginase-1 can be synthesized and secreted by activated DCs during Leishmania infection subsequently leading protection or disease severity (17, 37, 38). Since we had found similar numbers of mature DCs in pLN, we wanted to investigate if the mature DCs exhibited any alteration in effector function in their ability to secrete nitrite and urea by virtue of intrinsic iNOS and arginase I activity (39, 40). CD11ccreIL-4Rα-/lox BMDCs infected with L. mexicana promastigotes and stimulated with IL-4 produced similar levels of nitrite and urea when compared to their littermate controls (Figures 7G, H). This unchanged effector function of BMDCs may suggest the similar progressive non-healing lesions and parasite proliferation and dissemination between two strains.
Discussion
The IL-4Rα pathway, a common receptor for IL-4 and IL-13 signaling, is essential in generating anti-inflammatory responses and mediating susceptibility to infection by Leishmania parasites causing CL in mice. In global abrogation of IL-4Rα, the progression of cutaneous leishmaniasis (caused by L. major and L. mexicana) is severely impaired resulting in protection from infection and eventually a healing outcome (18, 19). Hence there is need for studies on the abrogation of IL-4Rα on specific cells to investigate the cell responsible for resistance to CL infection. IL-4 and IL-13 signaling has been implicated in the maturation and activation of myeloid cells leading to the production of Th1 and Th2 cytokines important for eliminating some parasites (17, 41, 42). In addition, exogenous IL-4 has been shown to instruct DCs to produce IL-12 hence driving healing Th1 immune responses to L. major infection in BALB/c mice (16). This was further exemplified in vivo, where the abrogation of IL-4Rα on DCs (CD11ccreIL-4Rα-/lox) led to the host’s susceptibility to L. major infection, hence indicating a protective role for IL-4Rα signaling on DCs in BALB/c mice (17). Following this study, we aimed to investigate whether IL-4Rα signaling on CD11c+ DCs was the main signaling cascade mediating host protection to L. mexicana using CD11ccreIL-4Rα-/lox mice and controls. To achieve this, we infected these mice with L. mexicana promastigotes in the footpad, and at 8 weeks post infection, we evaluated disease progression, humoral and cellular immune responses.
CD11ccreIL-4Rα-/lox mice exhibited non-healing footpad swelling and parasite loads, similar to the littermate IL-4Rα-/lox, suggesting that IL-4Rα responsive DCs do not play a role in modulating host-protection to CL disease caused by L. mexicana. Sex hormones have been shown to impact the immune responses against leishmaniasis (43). Since, opposite sexes have been found to differ in the intensity, prevalence, and pathogenesis in various infections including CL (44, 45) and in relation to IL-4Rα deletion (21), we compared male and female mice deficient for the IL-4Rα on DCs. By and large, we found no sex-related difference associated with L. mexicana infection in the absence of IL-4Rα expression on DCs. It is by this basis we narrowed our investigation to evaluate the humoral and cellular responses in female mice infected with L. mexicana parasites bearing in mind that in previous studies, female mice deficient for IL-4Rα on T cells developed a healing phenotype whilst males were unchanged to littermate controls (21).
We next investigated the humoral immune response to explain unaltered disease progression in CD11ccreIL-4Rα-/lox and controls. We measured the levels of total IgE and Leishmania specific IgG1, IgG2a/b antibodies. The high levels of IgG1 in CD11ccreIL-4Rα-/lox and littermate controls compared to IL-4Rα-/- mice may be the causative reason why CD11ccreIL-4Rα-/lox mice and their littermate controls were unable to control lesion development. In support, higher titres of IgG1 are present in patients with active CL disease (46). Previous studies built on this evidence and reported that during L. mexicana infection, IgG1 preferentially binds to FcγRIII on macrophages thereby suppressing protective immunity, likely through macrophage IL-10 production (47). In addition, other studies have shown that the absence of IgG1 in L. major infected mice results in smaller lesions with controlled parasite proliferation (48). Antibody production is dependent on the differentiation of B cells into plasma cells (49). It is therefore not surprising that B cells in totality also do contribute to the host’s susceptibility and are therefore used as correlates of host susceptibility (50). For instance, we show that L. mexicana resistant mice (IL-4Rα-/-) have decreased population of B cells whilst susceptible CD11ccreIL-4Rα-/lox mice and controls have higher numbers. We hypothesize that majority of plasma B cells in CD11ccreIL-4Rα-/lox and their littermate controls may be tailored towards IgG1 and IgE antibody production and not protective IgG2a/b antibodies. In summary, our data demonstrate that IL-4Rα signaling on DCs does not lead to events that alter the production of IgG1 and IgE antibodies associated with susceptibility.
IL-4 and IL-13 bind to type I/II receptor that is constitutively expressed on B cells in both mice and humans (51) to induce IgE and IgG1 class switching (52). In our study, higher levels of IL-4 and IL-13 by LN cells stimulated with α-CD3 and heat killed L. mexicana promastigotes in CD11ccreIL-4Rα-/lox mice did not correlate with heightened levels of IgG1 and IgE compared to littermate controls. Surprisingly, however, the increased Th2 cytokines in CD11ccreIL-4Rα-/lox mice did not tilt IgG1 production over littermate controls. The same applies to total IgE, which was significantly lower in IL-4Rα-/- mice but increased in CD11ccreIL-4Rα-/lox mice highlighting its systemic role in susceptibility in L. mexicana infected mice. Regarding the latter, a clinical study conducted on patients from Brazil and Iraq revealed that high levels of IgE antibodies are associated with disease activity in CL (53, 54). The low levels of type 1 antibodies, namely, IgG2a and IgG2b, exhibited in CD11ccreIL-4Rα-/lox and IL-4Rα-/lox mice also further demonstrate the systemic inability of these mice to control the infection as type 1 antibodies have been associated with parasite clearance (17, 32). In this context, a previous study showed that interaction of mouse IgG1-amastigote immune complexes with FcγRIII on macrophages is detrimental in L. mexicana infections, but not with interactions involving IgG2a/c (28). Interestingly, IgG2a facilitates the killing of L. amazonensis in macrophages (32). Due to the central role of follicular DCs within the germinal center (GC), we speculate that loss of IL-4Rα signaling on DCs did not alter isotype class-switching during GC formation hence no differences in B cell differentiation to plasma cells that could lead to changes in antibody titres (55–57).
The presence of CD4+ T cells is important in the control of CL as shown in L. braziliensis and L. major infection (37). We demonstrated that the loss of IL-4Rα signaling on DCs leads to heightened absolute numbers of CD4+ and CD8+ T cells. Surprisingly, a negative feedback loop appeared to exist in the activation of T cells and IL-4Rα signaling on CD11c+ DCs, with higher levels observed in the absence of IL-4Rα+ DCs. Notably, the loss of IL-4Rα signaling on CD4+ T cells has been shown to protect BALB/c female mice from L. mexicana infection (21).
Central memory T cells (TCM), are long-lived cells that can survive without the presence of the parasite and can transform into effector memory cells upon secondary infection (58). Our data reveal an increased absolute TCM cell number in CD11ccreIL-4Rα-/lox mice suggesting that these mice have long-term memory hence, they are likely to respond effectively in case of secondary infection with L. mexicana. Deletion of IL-4Rα on DCs had no consequences on the infiltration of T cells with naïve phenotype into LNs cells with no prior encounter with L. mexicana. Naïve T-cells can differentiate into effector memory T cells (TEM) and TCM to facilitate their function in immune responses against infections (59). We also suspect that the interaction between CD4+ and CD8+ T cells is important in disease control vs susceptibility (60). In support, bystander CD8+ T cells can also contribute to a harmful role in CL disease progression (61). On the other hand, in L. major infections, CD8+ T cells play a vital role in protection (62). In this regard, our results clearly show an increased population of CD8+ T cells in LNs of CD11ccreIL-4Rα-/lox and IL-4Rα-/lox female mice compared to resistant IL-4Rα-/- mice, suggesting that CD8+ T cells perhaps plays a role in the immunopathogenesis of CL caused by L. mexicana infection. In an alternate hypothesis, expansion of CD8+ T cells in CD11ccreIL-4Rα-/lox mice, and their subsequent activation, could lead to degranulation and release of perforin and cytotoxic granzyme effectors, thereby promoting disease severity and mediating immunopathology, as shown in L. braziliensis-infected mice (63–65). In parallel, it may be possible that perforin-mediated attack of L. mexicana-infected cells prevented hypersusceptibility, contrary to that seen in L. major infection (17). Alternatively, this may be either through cell lysis, leading to parasite dissemination, or onset of apoptosis and when apoptotic cells are not cleared by phagocytes, this could lead to an increase in the inflammatory response (66). CD8+ T cell effectors generated during L. mexicana infection may have tilted to cytotoxic T lymphocytes (CTLs) exhibiting a cytolytic activity and/or lysis of infected cells without killing the parasites hence promoting disease severity, perhaps through granzyme B production, perforins, and IL-1β/inflammasome activation (63, 67). This finding suggests that CD8+ T cells may play a minimal role in the protection of the host from L. mexicana infections indicating that their presence may result in increased pathology (63). Therefore, an understanding of the mechanisms that drive CD8+ T cells to become pathogenic or protective depending on Leishmania species, will provide interesting insights.
IFN-γ is unambiguously a host-protector in CL (33). Indeed, studies have shown evidence that IFN-γ secretion by macrophages and DCs fuel a rapid escalation of nitric oxide (NO) production that induces parasite killing (68, 69). Our data show that loss of IL-4Rα signaling on CD11c+ DCs does not lead to altered IFN-γ production by CD8+ T cells but slight increases in CD4+ T cells were observed in the pLN. This may provide an explanation as to why no differences were observed in the footpad lesion progression between CD11ccreIL-4Rα-/lox and IL-4Rα-/lox mice even though levels of IL-4 and IL-13 were heightened. Our data, therefore, suggests that the effector functions of IFN-γ on macrophages and DCs remained unchanged by the presence or absence of IL-4Rα signaling on CD11c+ DCs. Indeed, it has been documented that the modulation of Type 2 immune responses in murine models of leishmaniasis is more important in the balance of resistance and susceptibility, rather than the Type 1 immune responses. However, the data herein throws caution to this dogma as the heightened IL-4 and IL-13 in CD11ccreIL-4Rα-/lox did not equate to hyper-susceptibility as previously reported (17).
Innate cells such as macrophages, neutrophils, and DCs are the first line of defense before adaptive immunity comes into action. Surprisingly, these cells may favor parasite survival hence enhancing disease severity (70, 71). The absence of IL-4Rα signaling on DCs did not affect the infiltration of these innate cells into LN. However, the increased levels in CD11ccreIL-4Rα-/lox mice, as compared to resistant IL-4Rα-/-, mice suggest that these cells may play a role in susceptibility to L. mexicana, perhaps as host cells that favor replication of parasites (71), and thus investigation of their effector responses could be important in controlling CL. The effect of IL-4 in neutrophil counts and migration has been highlighted during bacterial infections (72). This study particularly demonstrated that mice treated with IL-4 have reduced neutrophil migration possibly by affecting CXCR2-CXCR4 chemokine signaling. In our study, we have demonstrated that the absence of IL-4Rα signaling on DCs leads to increased IL-4 cytokines following α-CD3 stimulation, but this did not reduce the infiltration of neutrophils into LN from circulation during L. mexicana infection. We thus suspect that the effects of IL-4 on neutrophil migration into the LN may have been counteracted by the presence of the parasite. Transient expression of IL-13Rα2 on DCs may have inhibited IL-4 mediated signaling in littermate controls hence similar myeloid infiltration consequently leading to similar disease phenotype to CD11ccreIL-4Rα-/lox (73). It would therefore be interesting to investigate IL-13Rα2 levels in these animals in future studies. Neutrophils may have facilitated the silent entry of parasites into macrophages in a “Trojan Horse” model hence enhancing the infection (74). Considering absolute cell numbers, increased DC population in CD11ccreIL-4Rα-/lox mice appears not to reflect in disease control but susceptibility, which suggests that either the loss of IL-4Rα signaling on CD11c+ DCs may lead to events that do not cause polarization of these cells to produce pro-inflammatory mediators or signaling in other myeloid cells may be vital in protection against L. mexicana infection. More importantly, the loss of IL-4Rα signaling on CD11c+ DCs did not affect the production of nitrite and urea (in ex vivo infection of BMDCs), which are known substrate for iNOS and arginine-derived metabolite respectively. Specifically, L. mexicana infected DCs appear to impair cellular and immunological mechanisms hence leading to the inability to resolve cutaneous disease (39).
Conventional DCs (cDCs) are specialized in uptake of Leishmania parasites, process, and present parasite-derived antigens to T cells. More importantly, the cDC1 identified by their CD8α expression as opposed to cDC2, cross-present antigens to CD8+ T cells (75, 76). In addition, cDC1 are IL-12p70 producers hence they are less permissive to Leishmania infection unlike cDC2 cells that skew CD4 T cell differentiation to Th2 cells (77, 78). Therefore, in our study it is more likely that the cDCs may have majorly been of cDC2 subset, given the increase in IL-4 and IL-13 production by CD4+ T cells in the dLN of CD11ccreIL-4Rα-/lox mice during L. mexicana infection.
Inflammatory DCs, also known as monocyte-derived DCs (mo-DCs) expand during L. major infections and been shown to harbour parasites in the lymph node of DC-IL-4Rα deficient mice (24). Analysis on the Mo-DCs revealed unchanged frequencies regardless of the presence or absence of IL-4Rα signaling on DCs. But the IL-4Rα-/- mice that controlled L. mexicana infection had decreased frequencies of these cells, demonstrating the vital role they may play in susceptibility to L. mexicana infections. In addition, Mo-DCs may have enhanced the differentiation of memory CD8+ T cells in both CD11ccreIL-4Rα-/lox and their littermate controls compared to global IL-4Rα-/- mice (79).
We also sought to investigate whether the absence of IL-4 signaling in CD11ccreIL-4Rα-/lox mice affected IL-4-mediated DC instruction in terms of IL-12p70 and IL-10 cytokine production (16, 36). Surprisingly, the amount of IL-12p70 and IL-10 production by BMDCs was unchanged between the two groups. This observation corroborated with a similar expression of maturation and activation markers such as MHCII and CD80 on CD11c+ cells respectively when stimulated with IL-4 (76). Together, we conclude that the loss of IL-4Rα signaling on DCs does not affect their maturation and activation, in addition to unaltered IL-4-DC instruction in IL-12 and IL-10 production. These findings may suggest why we observed similar disease outcome between CD11ccreIL-4Rα-/lox mice and their littermates during L. mexicana infection.
In summary, our data suggest that IL-4Rα signaling on CD11c+ DCs does not have an essential role in the control of CL caused by L. mexicana, irrespective of sex and with regard to lack of differences in both humoral and Th1 immune responses. Interestingly, the route or site of infection has been shown to significantly influence the outcome of CL (80, 81), thus it will be interesting to decipher the phenotype exhibited following an intradermal infection with L. mexicana. Surprisingly, Type 2 immune responses, in the absence of IL-4Rα signaling on DCs, do increase substantially, yet disease outcome remains similar compared to littermate control. This is paradoxical to some extent because, in the same mouse strain, disease severity is enhanced during L. major infection, with a characteristic increase in IL-4 (17). Altogether, this may suggest that the outcome of IL-4-mediated instruction of DCs in vivo may be species-specific with respect to CL-causing species.
Data Availability Statement
The original contributions presented in the study are included in the article/Supplementary Material. Further inquiries can be directed to the corresponding authors.
Ethics Statement
The animal study was reviewed and approved by Faculty of Health Science, Animal Research Ethics Committee, University of Cape Town.
Author Contributions
Conceptualization: RH and FB. Methodology and investigation: BOO, RA, RH, ZC, RM, MO, and MBL. Writing, Review, and Editing: BOO, MBL, RH, and FB. Funding Acquisition: RH and FB. Supervision: RH and FB. GM-CSF cell line: MBL. Cell-specific gene-deficient mice: FB. All authors contributed to the article and approved the submitted version.
Funding
This research was supported by funds from the National Research Foundation (grant numbers: 113446 and 120407), University of Cape Town, Research, and Innovation: 2019 Enabling Grant Seeker Excellence Award and Poliomyelitis Research Foundation (grant number: 19/12), under RH. Also, financial support from the National Research Foundation (NRF) of South Africa, Department of Science and Technology (DST), South African Research Chair Initiative, International Center for Genetic Engineering and Biotechnology, and Wellcome CIDRI-Africa (grant number: 203135/Z/16/Z) under FB.
Conflict of Interest
The authors declare that the research was conducted in the absence of any commercial or financial relationships that could be construed as a potential conflict of interest.
Publisher’s Note
All claims expressed in this article are solely those of the authors and do not necessarily represent those of their affiliated organizations, or those of the publisher, the editors and the reviewers. Any product that may be evaluated in this article, or claim that may be made by its manufacturer, is not guaranteed or endorsed by the publisher.
Acknowledgments
The authors thank Munadia Ansarie for genotyping of mice, Zarinah Sonday and Wendy Green for technical and administrative assistance, the animal unit of the Faculty of Health Sciences, University of Cape Town, for breeding and maintenance of the mice during all the experiments. BOO thanks International Center for Genetic Engineering and Biotechnology (ICGEB) for Arturo Falaschi PhD scholarship.
Supplementary Material
The Supplementary Material for this article can be found online at: https://www.frontiersin.org/articles/10.3389/fimmu.2021.759021/full#supplementary-material
References
1. Burza S, Croft SL, Boelaert M. Leishmaniasis. Lancet (2018) 392:951–70. doi: 10.1016/S0140-6736(18)31204-2
2. Molyneux DH, Savioli L, Engels D. Neglected Tropical Diseases: Progress Towards Addressing the Chronic Pandemic. Lancet (2017) 389:312–25. doi: 10.1016/S0140-6736(16)30171-4
3. Hartley MA, Drexler S, Ronet C, Beverley SM, Fasel N. The Immunological, Environmental, and Phylogenetic Perpetrators of Metastatic Leishmaniasis. Trends Parasitol (2014) 30:412–22. doi: 10.1016/j.pt.2014.05.006
5. WHO. Leishmaniasis (2021). Available at: https://www.who.int/news-room/fact-sheets/detail/leishmaniasis.
6. Oualha R, Barhoumi M, Marzouki S, Harigua-Souiai E, Ben Ahmed M, Guizani I. Infection of Human Neutrophils With Leishmania Infantum or Leishmania Major Strains Triggers Activation and Differential Cytokines Release. Front Cell Infect Microbiol (2019) 9:153. doi: 10.3389/fcimb.2019.00153
7. James SL, Abate D, Abate KH, Abay SM, Abbafati C, Abbasi N, et al. Global, Regional, and National Incidence, Prevalence, and Years Lived With Disability for 354 Diseases and Injuries for 195 Countries and Territories 1990—2017: A Systematic Analysis for the Global Burden of Disease Study 2017. Lancet (2018) 392:1789–858. doi: 10.1016/S0140-6736(18)32279-7
8. Pigott DM, Bhatt S, Golding N, Duda KA, Battle KE, Brady OJ, et al. Global Distribution Maps of the Leishmaniases. Elife (2014) 3:e02851. doi: 10.7554/eLife.02851
9. Ghorbani M, Farhoudi R. Leishmaniasis in Humans: Drug or Vaccine Therapy? Drug Des Dev Ther (2018) 12:25. doi: 10.2147/DDDT.S146521
10. McGwire BSA, S AR. Leishmaniasis: Clinical Syndromes and Treatment. QJM (2014) 107:7–14. doi: 10.1093/qjmed/hct116
11. Didwania N, Shadab M, Sabur A, Ali N. Alternative to Chemotherapy-The Unmet Demand Against Leishmaniasis. Front Immunol (2017) 8:1779. doi: 10.3389/fimmu.2017.01779
12. Hefnawy A, Berg M, Dujardin JC, De Muylder G. Exploiting Knowledge on Leishmania Drug Resistance to Support the Quest for New Drugs. Trends Parasitol (2017) 33:162–74. doi: 10.1016/j.pt.2016.11.003
13. Kumar R, Engwerda C. Vaccines to Prevent Leishmaniasis. Clin Transl Immunol (2014) 3:e13. doi: 10.1038/cti.2014.4
14. Seyed N, Peters NC, Rafati S. Translating Observations From Leishmanization Into Non-Living Vaccines: The Potential of Dendritic Cell-Based Vaccination Strategies Against Leishmania. Front Immunol (2018) 9:1227. doi: 10.3389/fimmu.2018.01227
15. Aruleba RT, Carter KC, Brombacher F, Hurdayal R. Can We Harness Immune Responses to Improve Drug Treatment in Leishmaniasis? Microorganisms (2020) 8(7):1069. doi: 10.3390/microorganisms8071069
16. Biedermann T, Zimmermann S, Himmelrich H, Gumy A, Egeter O, Sakrauski AK, et al. IL-4 Instructs Th 1 Responses and Resistance to Leishmania Major in Susceptible BALB/c Mice. Nat Immunol (2001) 2:1054–60. doi: 10.1038/ni725
17. Hurdayal R, Nieuwenhuizen NE, Revaz-Breton M, Smith L, Hoving JC, Parihar SP, et al. Deletion of IL-4 Receptor Alpha on Dendritic Cells Renders BALB/c Mice Hypersusceptible to Leishmania Major Infection. PloS Pathog (2013) 9:e1003699. doi: 10.1371/journal.ppat.1003699
18. Mohrs M, Ledermann B, Köhler G, Dorfmüller A, Gessner A, Brombacher F. Differences Between IL-4-and IL-4 Receptor α-Deficient Mice in Chronic Leishmaniasis Reveal a Protective Role for IL-13 Receptor Signaling. J Immunol (1999) 162:7302–8.
19. Alexander J, Brombacher F, McGachy HA, McKenzie AN, Walker W, Carter KC. An Essential Role for IL-13 in Maintaining a Non-Healing Response Following Leishmania Mexicana Infection. Eur J Immunol (2002) 32:2923–33. doi: 10.1002/1521-4141(2002010)32:10<2923::AID-IMMU2923>3.0.CO;2-E
20. Satoskar A, Bluethmann H, Alexander J. Disruption of the Murine Interleukin-4 Gene Inhibits Disease Progression During Leishmania Mexicana Infection But Does Not Increase Control of Leishmania Donovani Infection. Infect Immun (1995) 63:4894–9. doi: 10.1128/iai.63.12.4894-4899.1995
21. Bryson KJ, Millington OR, Mokgethi T, McGachy HA, Brombacher F, Alexander J. BALB/c Mice Deficient in CD4 T Cell IL-4Ralpha Expression Control Leishmania Mexicana Load Although Female But Not Male Mice Develop a Healer Phenotype. PloS Negl Trop Dis (2011) 5:e930. doi: 10.1371/journal.pntd.0000930
22. Radwanska M, Cutler AJ, Hoving JC, Magez S, Holscher C, Bohms A, et al. Deletion of IL-4Ralpha on CD4 T Cells Renders BALB/c Mice Resistant to Leishmania Major Infection. PloS Pathog (2007) 3:e68. doi: 10.1371/journal.ppat.0030068
23. Spotin A, Parvizi P. Comparative Study of Viscerotropic Pathogenicity of Leishmania Major Amastigotes and Promastigotes Based on Identification of Mitochondrial and Nucleus Sequences. Parasitol Res (2016) 115:1221–8. doi: 10.1007/s00436-015-4858-4
24. Hurdayal R, Nieuwenhuizen NE, Khutlang R, Brombacher F. Inflammatory Dendritic Cells, Regulated by IL-4 Receptor Alpha Signaling, Control Replication, and Dissemination of Leishmania Major in Mice. Front Cell Infect Microbiol (2020) 9:479. doi: 10.3389/fcimb.2019.00479
25. Herbert DR, Holscher C, Mohrs M, Arendse B, Schwegmann A, Radwanska M, et al. Alternative Macrophage Activation is Essential for Survival During Schistosomiasis and Downmodulates T Helper 1 Responses and Immunopathology. Immunity (2004) 20:623–35. doi: 10.1016/s1074-7613(04)00107-4
26. Nieuwenhuizen NE, Kirstein F, Hoving JC, Brombacher F. House Dust Mite Induced Allergic Airway Disease Is Attenuated in CD11c Cre IL-4rα–/L° X Mice. Sci Rep (2018) 8:1–10. doi: 10.1038/s41598-017-19060-9
27. Ghotloo S, Mollahoseini MH, Najafi A, Yeganeh F. Comparison of Parasite Burden Using Real-Time Polymerase Chain Reaction Assay and Limiting Dilution Assay in Leishmania Major Infected Mouse. Iranian J Parasitol (2015) 10:571.
28. Chu N, Thomas BN, Patel SR, Buxbaum LU. IgG1 Is Pathogenic in Leishmania Mexicana Infection. J Immunol (2010) 185:6939–46. doi: 10.4049/jimmunol.1002484
29. Lutz MB, Kukutsch N, Ogilvie AL, Rössner S, Koch F, Romani N, et al. An Advanced Culture Method for Generating Large Quantities of Highly Pure Dendritic Cells From Mouse Bone Marrow. J Immunol Methods (1999) 223(1):77–92. doi: 10.1016/s0022-1759(98)00204-x
30. Prina E, Abdi SZ, Lebastard M, Perret E, Winter N, Antoine JC. Dendritic Cells as Host Cells for the Promastigote and Amastigote Stages of Leishmania Amazonensis: The Role of Opsonins in Parasite Uptake and Dendritic Cell Maturation. J Cell Sci (2004) 117:315–25. doi: 10.1242/jcs.00860
31. Hurdayal R, Ndlovu HH, Revaz-Breton M, Parihar SP, Nono JK, Govender M, et al. IL-4–Producing B Cells Regulate T Helper Cell Dichotomy in Type 1-and Type 2-Controlled Diseases. Proc Natl Acad Sci (2017) 114:E8430–9. doi: 10.1073/pnas.1708125114
32. Gibson-Corley KN, Bockenstedt MM, Li H, Boggiatto PM, Phanse Y, Petersen CA, et al. An In Vitro Model of Antibody-Enhanced Killing of the Intracellular Parasite Leishmania Amazonensis. PloS One (2014) 9:e106426. doi: 10.1371/journal.pone.0106426
33. Osero BO, Aruleba RT, Brombacher F, Hurdayal R. Unravelling the Unsolved Paradoxes of Cytokine Families in Host Resistance and Susceptibility to Leishmania Infection. Cytokine X (2020) 2:100043. doi: 10.1016/j.cytox.2020.100043
34. Sheng J, Chen Q, Soncin I, Ng SL, Karjalainen K, Ruedl C. A Discrete Subset of Monocyte-Derived Cells among Typical Conventional Type 2 Dendritic Cells Can Efficiently Cross-Present. Cell Rep (2017) 21(5):1203–14. doi: 10.1016/j.celrep.2017.10.024
35. Cao Q, Wang Y, Wang XM, Lu J, Lee VW, Ye Q, et al. Renal F4/80+ CD11c+ Mononuclear Phagocytes Display Phenotypic and Functional Characteristics of Macrophages in Health and in Adriamycin Nephropathy. J Am Soc Nephrol (2015) 26(2):349–63. doi: 10.1681/ASN.2013121336
36. Yao Y, Li W, Kaplan MH, Chang CH. Interleukin (IL)-4 Inhibits IL-10 to Promote IL-12 Production by Dendritic Cells. J Exp Med (2005) 201:1899–903. doi: 10.1084/jem.20050324
37. da Silva Santos C, Boaventura V, Cardoso CR, Tavares N, Lordelo MJ, Noronha A, et al. CD8+ Granzyme B+–mediated Tissue Injury vs. CD4+ Ifnγ+–Mediated Parasite Killing in Human Cutaneous Leishmaniasis. J Invest Dermatol (2013) 133:1533–40. doi: 10.1038/jid.2013.4
38. Schleicher U, Paduch K, Debus A, Obermeyer S, Konig T, Kling JC, et al. TNF-Mediated Restriction of Arginase 1 Expression in Myeloid Cells Triggers Type 2 NO Synthase Activity at the Site of Infection. Cell Rep (2016) 15:1062–75. doi: 10.1016/j.celrep.2016.04.001
39. Contreras I, Estrada JA, Guak H, Martel C, Borjian A, Ralph B, et al. Impact of Leishmania Mexicana Infection on Dendritic Cell Signaling and Functions. PloS Negl Trop Dis (2014) 8:e3202. doi: 10.1371/journal.pntd.0003202
40. Rivera-Fernandez I, Argueta-Donohue J, Wilkins-Rodriguez AA, Gutierrez-Kobeh L. Effect of Two Different Isolates of Leishmania Mexicana in the Production of Cytokines and Phagocytosis by Murine Dendritic Cells. J Parasitol (2019) 105:359–70. doi: 10.1645/17-158
41. Bosurgi L, Cao YG, Cabeza-Cabrerizo M, Tucci A, Hughes LD, Kong Y, et al. Macrophage Function in Tissue Repair and Remodeling Requires IL-4 or IL-13 With Apoptotic Cells. Science (2017) 356:1072–6. doi: 10.1126/science.aai8132
42. Heeb LEM, Egholm C, Boyman O. Evolution and Function of Interleukin-4 Receptor Signaling in Adaptive Immunity and Neutrophils. Genes Immun (2020) 21:143–9. doi: 10.1038/s41435-020-0095-7
43. Snider H, Lezama-Davila C, Alexander J, Satoskar AR. Sex Hormones and Modulation of Immunity Against Leishmaniasis. Neuroimmunomodulation (2009) 16:106–13. doi: 10.1159/000180265
44. Fathi A, Addo MM, Dahlke C. Sex Differences in Immunity: Implications for the Development of Novel Vaccines Against Emerging Pathogens. Front Immunol (2020) 11:601170. doi: 10.3389/fimmu.2020.601170
45. vom Steeg LG, Klein SL. SeXX Matters in Infectious Disease Pathogenesis. PloS Pathog (2016) 12:e1005374. doi: 10.1371/journal.ppat.1005374
46. Silva G, Goncalves RV, Nepomuceno M, de Souza M, Favoreto S, Oliveira Neto M, et al. Decrease in Anti-Leishmania IgG3 and IgG1 After Cutaneous Leishmaniasis Lesion Healing is Correlated With the Time of Clinical Cure. Parasit Immunol (2012) 34(10):486–91. doi: 10.1111/j.1365-3024.2012.01379.x
47. Thomas BN, B LU. FcgammaRIII Mediates Immunoglobulin G-Induced Interleukin-10 and is Required for Chronic Leishmania Mexicana Lesions. Infect Immun (2008) 76:623–31. doi: 10.1128/IAI.00316-07
48. Miles SA, Conrad SM, Alves RG, Jeronimo SM, Mosser DM. A Role for IgG Immune Complexes During Infection With the Intracellular Pathogen Leishmania. J Exp Med (2005) 201:747–54. doi: 10.1084/jem.20041470
49. Nothelfer K, Sansonetti PJ, Phalipon A. Pathogen Manipulation of B Cells: The Best Defence is a Good Offence. Nat Rev Microbiol (2015) 13:173–84. doi: 10.1038/nrmicro3415
50. De Lima CMF, Magalhães AS, Costa R, Barreto CC, Machado PR, Carvalho EM, et al. High Anti-Leishmania IgG Antibody Levels Are Associated With Severity of Mucosal Leishmaniasis. Front Cell Infect Microbiol (2021) 11:258. doi: 10.3389/fcimb.2021.652956
51. Graber P, Gretener D, Herren S, Aubry JP, Elson G, Poudrier J, et al. The Distribution of IL-13 Receptor >1 Expression on B Cells, T Cells and Monocytes and its Regulation by IL-13 and IL-4. Eur J Immunol (1998) 28:4286–98. doi: 10.1002/(SICI)1521-4141(199812)28:12<4286::AID-IMMU4286>3.0.CO;2-H
52. Junttila IS. Tuning the Cytokine Responses: An Update on Interleukin (IL)-4 and IL-13 Receptor Complexes. Front Immunol (2018) 9:888. doi: 10.3389/fimmu.2018.00888
53. Al-Qadhi BN, Musa IS, Hummadi YMKA-M. Comparative Immune Study on Cutaneous Leishmaniasis Patients With Single and Multiple Sores. J Parasitic Dis (2015) 39:361–70. doi: 10.1007/s12639-013-0368-4
54. Sousa-Atta ML, Salamé GS, D’Oliveira J, Almeida RP, Atta AM, Carvalho EM. Immunoglobulin E Antileishmanial Antibody Response in Cutaneous Leishmaniasis. Clin Vaccine Immunol (2002) 9:101–4. doi: 10.1128/CDLI.9.1.101-104.2002
55. van der Poel CE, Bajic G, Macaulay CW, van den Broek T, Ellson CD, Bouma G, et al. Follicular Dendritic Cells Modulate Germinal Center B Cell Diversity Through Fcγriib. Cell Rep (2019) 29:2745–55.e2744. doi: 10.1016/j.celrep.2019.10.086
56. Smulski CR, Eibel H. BAFF and BAFF-Receptor in B Cell Selection and Survival. Front Immunol (2018) 9:2285. doi: 10.3389/fimmu.2018.02285
57. Wang X, Cho B, Suzuki K, Xu Y, Green JA, An J, et al. Follicular Dendritic Cells Help Establish Follicle Identity and Promote B Cell Retention in Germinal Centers. J Exp Med (2011) 208:2497–510. doi: 10.1084/jem.20111449
58. Zaph C, Uzonna J, Beverley SM, Scott P. Central Memory T Cells Mediate Long-Term Immunity to Leishmania Major in the Absence of Persistent Parasites. Nat Med (2004) 10:1104–10. doi: 10.1038/nm1108
59. O'Shea JJ, Paul WE. Mechanisms Underlying Lineage Commitment and Plasticity of Helper CD4+ T Cells. Science (2010) 327:1098–102. doi: 10.1126/science.1178334
60. Herath S, Kropf P, Muller I. Cross-Talk Between CD8(+) and CD4(+) T Cells in Experimental Cutaneous Leishmaniasis: CD8(+) T Cells are Required for Optimal IFN-Gamma Production by CD4(+) T Cells. Parasite Immunol (2003) 25:559–67. doi: 10.1111/j.0141-9838.2004.00668.x
61. Crosby EJ, Goldschmidt MH, Wherry EJ, Scott P. Engagement of NKG2D on Bystander Memory CD8 T Cells Promotes Increased Immunopathology Following Leishmania Major Infection. PloS Pathog (2014) 10:e1003970. doi: 10.1371/journal.ppat.1003970
62. Kronenberg K, Brosch S, Butsch F, Tada Y, Shibagaki N, Udey MC, et al. Vaccination With TAT-Antigen Fusion Protein Induces Protective, CD8(+) T Cell-Mediated Immunity Against Leishmania Major. J Invest Dermatol (2010) 130:2602–10. doi: 10.1038/jid.2010.171
63. Novais FO, Carvalho AM, Clark ML, Carvalho LP, Beiting DP, Brodsky IE, et al. CD8+ T Cell Cytotoxicity Mediates Pathology in the Skin by Inflammasome Activation and IL-1β Production. PloS Pathog (2017) 13:e1006196. doi: 10.1371/journal.ppat.1006196
64. Novais FO, Carvalho LP, Graff JW, Beiting DP, Ruthel G, Roos DS, et al. Cytotoxic T Cells Mediate Pathology and Metastasis in Cutaneous Leishmaniasis. PloS Pathog (2013) 9:e1003504. doi: 10.1371/journal.ppat.1003504
65. Novais FO, Carvalho LP, Passos S, Roos DS, Carvalho EM, Scott P, et al. Genomic Profiling of Human Leishmania Braziliensis Lesions Identifies Transcriptional Modules Associated With Cutaneous Immunopathology. J Invest Dermatol (2015) 135:94–101. doi: 10.1038/jid.2014.305
66. Kono H, Rock KL. How Dying Cells Alert the Immune System to Danger. Nat Rev Immunol (2008) 8:279–89. doi: 10.1038/nri2215
67. Campos TM, Novais FO, Saldanha M, Costa R, Lordelo M, Celestino D, et al. Granzyme B Produced by Natural Killer Cells Enhances Inflammatory Response and Contributes to the Immunopathology of Cutaneous Leishmaniasis. J Infect Dis (2020) 221:973–82. doi: 10.1093/infdis/jiz538
68. Sacks D, N-T N. The Immunology of Susceptibility and Resistance to Leishmania Major in Mice. Nat Rev Immunol (2002) 2:845–58. doi: 10.1038/nri933
69. De Trez C, Magez S, Akira S, Ryffel B, Carlier Y, Muraille E. iNOS-Producing Inflammatory Dendritic Cells Constitute the Major Infected Cell Type During the Chronic Leishmania Major Infection Phase of C57BL/6 Resistant Mice. PloS Pathog (2009) 5:e1000494. doi: 10.1371/journal.ppat.1000494
70. Bogdan C. Macrophages as Host, Effector and Immunoregulatory Cells in Leishmaniasis: Impact of Tissue Micro-Environment and Metabolism. Cytokine X (2020) 2:100041. doi: 10.1016/j.cytox.2020.100041
71. Rossi M, Fasel N. How to Master the Host Immune System? Leishmania Parasites Have the Solutions! Int Immunol (2018) 30:103–11. doi: 10.1093/intimm/dxx075
72. Woytschak J, Keller N, Krieg C, Impellizzieri D, Thompson RW, Wynn TA, et al. Type 2 Interleukin-4 Receptor Signaling in Neutrophils Antagonizes Their Expansion and Migration During Infection and Inflammation. Immunity (2016) 45:172–84. doi: 10.1016/j.immuni.2016.06.025
73. Rahaman SO, Sharma P, Harbor PC, Aman MJ, Vogelbaum MA, Haque SJ. IL-13R(Alpha)2, a Decoy Receptor for IL-13 Acts as an Inhibitor of IL-4-Dependent Signal Transduction in Glioblastoma Cells. Cancer Res (2002) 62:1103–9.
74. Laskay T, van Zandbergen G, Solbach W. Neutrophil Granulocytes as Host Cells and Transport Vehicles for Intracellular Pathogens: Apoptosis as Infection-Promoting Factor. Immunobiology (2008) 213:183–91. doi: 10.1016/j.imbio.2007.11.010
75. den Haan JM, Lehar SM, Bevan MJ. CD8(+) But Not CD8(-) Dendritic Cells Cross-Prime Cytotoxic T Cells In Vivo. J Exp Med (2000) 192:1685–96. doi: 10.1084/jem.192.12.1685
76. Feijo D, Tiburcio R, Ampuero M, Brodskyn C, Tavares N. Dendritic Cells and Leishmania Infection: Adding Layers of Complexity to a Complex Disease. J Immunol Res (2016) 2016:3967436. doi: 10.1155/2016/3967436
77. Henri S, Curtis J, Hochrein H, Vremec D, Shortman K, Handman E. Hierarchy of Susceptibility of Dendritic Cell Subsets to Infection by Leishmania Major: Inverse Relationship to Interleukin-12 Production. Infect Immun (2002) 70:3874–80. doi: 10.1128/IAI.70.7.3874-3880.2002
78. Martinez-Lopez M, Iborra S, Conde-Garrosa R, Sancho D. Batf3-Dependent CD103+ Dendritic Cells are Major Producers of IL-12 That Drive Local Th1 Immunity Against Leishmania Major Infection in Mice. Eur J Immunol (2015) 45:119–29. doi: 10.1002/eji.201444651
79. Shin KS, Jeon I, Kim BS, Kim IK, Park YJ, Koh CH, et al. Monocyte-Derived Dendritic Cells Dictate the Memory Differentiation of CD8(+) T Cells During Acute Infection. Front Immunol (2019) 10:1887. doi: 10.3389/fimmu.2019.01887
80. Baldwin TM, Elso C, Curtis J, Buckingham L, Handman E. The Site of Leishmania Major Infection Determines Disease Severity and Immune Responses. Infect Immun (2003) 71:6830–4. doi: 10.1128/IAI.71.12.6830-6834.2003
Keywords: Dendritic cells, IL-4Rα, IL-4, Leishmania mexicana, mice
Citation: Osero BO, Cele Z, Aruleba RT, Maine RA, Ozturk M, Lutz MB, Brombacher F and Hurdayal R (2022) Interleukin-4 Responsive Dendritic Cells Are Dispensable to Host Resistance Against Leishmania mexicana Infection. Front. Immunol. 12:759021. doi: 10.3389/fimmu.2021.759021
Received: 15 August 2021; Accepted: 29 December 2021;
Published: 28 January 2022.
Edited by:
Tamás Laskay, University of Lübeck, GermanyReviewed by:
Maria Agallou, Pasteur Hellenic Institute, GreeceDiana Moreira, Trinity College Dublin, Ireland
Copyright © 2022 Osero, Cele, Aruleba, Maine, Ozturk, Lutz, Brombacher and Hurdayal. This is an open-access article distributed under the terms of the Creative Commons Attribution License (CC BY). The use, distribution or reproduction in other forums is permitted, provided the original author(s) and the copyright owner(s) are credited and that the original publication in this journal is cited, in accordance with accepted academic practice. No use, distribution or reproduction is permitted which does not comply with these terms.
*Correspondence: Frank Brombacher, brombacherfrank@gmail.com; Ramona Hurdayal, ramona.hurdayal@uct.ac.za