- 1Department of Laboratory Medicine, Affiliated Hospital of Jiangsu University, Zhenjiang, China
- 2Department of Immunology, Jiangsu Key Laboratory of Laboratory Medicine, School of Medicine, Jiangsu University, Zhenjiang, China
Recent years, the immunosuppressive properties of mesenchymal stem cells (MSCs) have been demonstrated in preclinical studies and trials of inflammatory and autoimmune diseases. Emerging evidence indicates that the immunomodulatory effect of MSCs is primarily attributed to the paracrine pathway. As one of the key paracrine effectors, mesenchymal stem cell-derived exosomes (MSC-EXOs) are small vesicles 30-200 nm in diameter that play an important role in cell-to-cell communication by carrying bioactive substances from parental cells. Recent studies support the finding that MSC-EXOs have an obvious inhibitory effect toward different effector cells involved in the innate and adaptive immune response. Moreover, substantial progress has been made in the treatment of autoimmune diseases, including multiple sclerosis (MS), systemic lupus erythematosus (SLE), type-1 diabetes (T1DM), uveitis, rheumatoid arthritis (RA), and inflammatory bowel disease (IBD). MSC-EXOs are capable of reproducing MSC function and overcoming the limitations of traditional cell therapy. Therefore, using MSC-EXOs instead of MSCs to treat autoimmune diseases appears to be a promising cell-free treatment strategy. In this review, we review the current understanding of MSC-EXOs and discuss the regulatory role of MSC-EXOs on immune cells and its potential application in autoimmune diseases.
1 Introduction
Mesenchymal stem cells (MSCs) are pluripotent stem cells with the capacity for self-renewal and multidirectional differentiation into osteoblasts, chondrocytes, adipocytes, and other types of cells (1, 2). MSCs are widely distributed in the body and have been isolated from a variety of tissues, among which the bone marrow and subcutaneous fat are common cellular sources (3). The International Committee established the recognition characteristics of human MSCs, including under standard culture conditions to maintain adhesion appearance, expression of CD105, CD73, and CD90 molecules, no expression of CD45, CD34, CD14, CD45, CD11b, CD79a, CD19, and HLA-DR, with the ability to differentiate into osteoblasts, adipocytes, chondrocytes in vitro (4). In addition to its strong differentiation capacity, MSCs also have immunomodulatory potential to modulate innate and adaptive immune cells (5). Abundant evidence indicates that MSCs can act on natural killer (NK) cells, dendritic cells (DCs), macrophages, B lymphocytes, and T lymphocytes via inhibiting the activation, proliferation and differentiation into effector cells (6–9). Following stimulation with inflammatory factors, MSCs exhibit the properties of reducing the inflammatory response, improving tissue repair, and avoiding infection by secreting various immune regulatory factors (10). At present, substantial evidence suggests that MSCs exert their immunomodulatory function through paracrine pathway, especially via exosomes (10, 11).
Extracellular vesicles(EVs) are a family of particles/vesicles found in blood and body fluids. They are composed of phospholipid bilayer and carry a variety of molecules that serve as mediators for intercellular communication (12). In 1946, Chargaffaf et al. suspected the existence of EVs and published the first study of EVs (13). It was not until 1967 that Wolf et al. confirmed the existence of EVs with electron microscopy (EM) (14). The term exosome (“ exo ”= external,“ some ”= body) was introduced in the 1970s, and it wasn’t until 1981 that the term“ exosome ”was first used by Trams to refer to Evs (15). Shortly after Johnstone et al. described multivesicular bodies (MVBs) and their 40-80nm exosomes in 1985 (16), differential centrifugation and ultracentrifugation above 100,000 g were used to separate and distinguish the smallest vesicles (17). With the increase in EVs (especially exosomes) publications, most studies do not clearly distinguish exosomes from other vesicles. To address this problem, in 2013, the International Extracellular Vesicle Society (ISEV) proposed a set of criteria for EV Science (18). In order to ensure normative research, the Minimal Information of Studies of Extracellular Vesicles (MISEV) was revised in 2018 to update knowledge in the field (19).
Exosomes are spherical vesicles composed of lipid bilayer membranes with a diameter of 40-200 nm, which contain complex and abundant active substances such as proteins and nucleic acids. Expression of exosome markers, proteins, nucleic acids, and other bioactive molecules is related to the cell of origin (20). There is increasing evidence that MSC-derived exosomes (MSC-EXOs) play an important role in immune regulation. MSC-EXOs are spherical vesicles secreted by MSCs that contain many anti-inflammatory compounds and modulate the immune response by interacting with immune effector cells (12). In the treatment of autoimmune diseases, MSC-EXOs as a carrier of cell-free therapy have attracted extensive attention, because they not only carry most of the therapeutic effects of MSC itself, but also reduce the concerns about the safety of injecting live cells. MSC-EXOs has significant advantages over MSCs in clinical treatment and may completely replace MSC therapy in the future.
2 Characteristics of Mesenchymal Stem Cell-Derived Exosomes
Both eukaryotic and prokaryotic cells release EVs, which are regarded as a part of their normal physiology and acquired abnormalities (20). EVs represent an important substance for intercellular communication. They participate in normal physiological processes, as well as play a critical role in disease occurrence and progression. Numerous experimental and clinical studies have shown that the immunomodulatory effects of MSCs can be primarily attributed to MSC-derived extracellular vesicles (MSC-EVs) (21). Although the classification of EVs is constantly evolving, it is generally accepted that EVs are classified into three main categories according to their size and biogenesis: apoptotic bodies (ABs), microvesicles, and exosomes (22–24). ABs (greater than 1,000 nm in diameter) are comprised of relatively large fragments of cells containing organelles derived from the cells undergoing apoptosis, which are transferred to phagocytes (25, 26). Microvesicles (100–1,000 nm in diameter) and exosomes (40–200 nm in diameter) belong to EVs at the nano level. Microvesicles, also known as ectosomes or microparticles, are released into the extracellular environment after directly budding or shedding from the plasma membrane (27). Exosomes originate from the endosomal pathway. Extracellular material fuses with early endosomes (ESEs) through membrane invagination and endocytosis, and gradually matures and develops into late endosomes (LSEs). The invagination of LSEs leads to the formation of intraluminal vesicles (ILVs), and multiple vesicles assemble to form MVBs, which fuse with the cell membrane and are subsequently released (Figure 1) (20). With regards to the delivery of exosome contents, exosomes can be bound by target cells through multiple pathways, of which the main mechanisms include endocytosis, ligand-receptor binding, or direct binding (Figure 1) (28–31). Exosomes are formed by budding through the endosome pathway and are wrapped in a lipid bilayer, contents of which can be protected by the external environment to maintain exosome integrity.
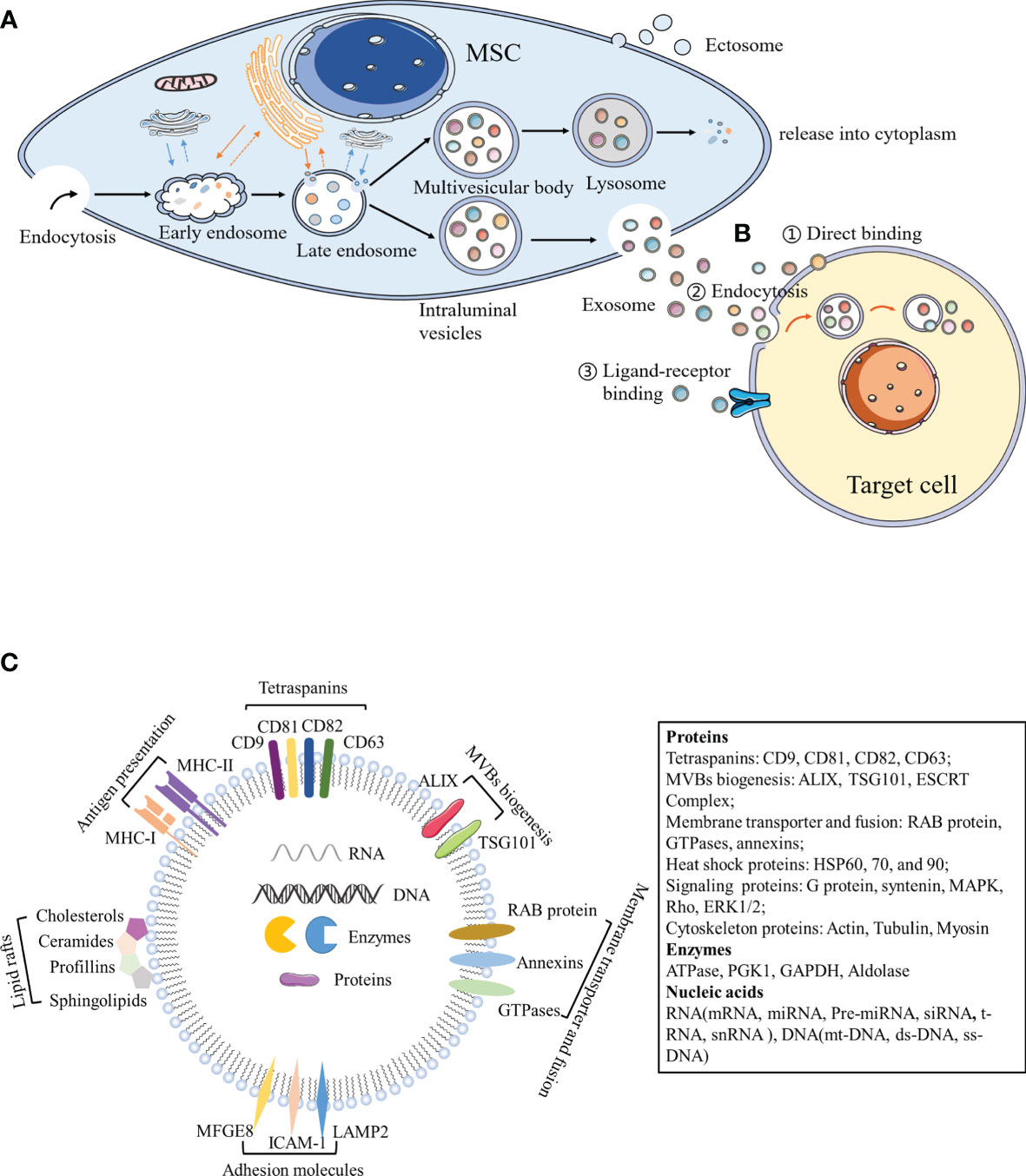
Figure 1 Biogenesis and components of exosomes. (A) Exosomes originate from the endosomal pathway. Extracellular material enters the cytoplasm through plasma membrane depression and endocytosis, and fuses with early endosomes, endoplasmic reticulum and preformed Golgi bodies, to develop into late endosomes, which are interlinked with the cell membrane network structure to form ILVs containing a vesicle structure. Different concentrations and sizes of ILVs constitute MVBs. On the one hand, MVBs fuse with lysosomes, degrade the contents, and release them into the cytoplasm. On the other hand, MVBs are transferred to the cytoplasmic membrane through the membrane system and vesicles are released outside the cell, which are termed exosomes. (B) Exosomes can act by binding to receptors present on the surface of target cells, by binding to endocytosis, or by the direct binding to recipient cells. (C) Exosome components. MFGE8, milk fat globule-EGF factor 8 protein; ICAM-1, intercellular adhesion molecule 1; MHC I and II, major histocompatibility complex I and II; LAMP2, lysosomal-associated membrane protein 2; MAPK, mitogen-activated protein kinase; ERK, extracellular signal-regulated kinase; PGK1, phosphoglycerate kinase 1; GAPDH, glyceraldehyde 3-phosphate dehydrogenase.
Exosomes contain a large number of proteins, lipids, transcription factors, as well as DNA, mRNA, and miRNA (32, 33). Exosome membranes contain lipid raft structures composed of cholesterol, spingomyosin, and ceramide and the tetraspanin protein family (CD63, CD81, and CD9) as an exosome marker (34). Exosomes also contain other common proteins, including MVB biogenesis proteins (Alix, TSG101, and ESCRT Complex), membrane transporter and fusion proteins (RAB protein, GTPases, and annexins), heat shock proteins (HSP60, 70, and 90), lipid related proteins, and phospholipases (35). Notably, MSC-EXOs express not only common surface markers such as CD81 and CD9, but also mesenchymal stem cell surface markers (CD44, CD73, and CD90) by flow cytometry (34). Proteomic analysis of exosomes isolated from human bone marrow-derived mesenchymal stem cells (hBM-MSC)provided evidence of 730 functional proteins associated with MSC proliferation, adhesion, migration, and morphogenesis (36). Surprisingly, protein packaging in exosomes is not random, because human primed MSCs secrete exosomes (pMEX), compared to human primed MSCs (pMSC), has a high concentration of specific subcategories of proteins, including secretory proteins and extracellular matrix (ECM) associated proteins, which may provide the molecular basis for its unique functional properties (37). In addition to proteins, MSC-EXOs also contain numerous RNA. Interestingly, RNA is specifically incorporated into exosomes, where it accumulates and then enters the recipient cell to do its job (38). Interestingly, enrichment of MSC-EXOs with network-informed miRNA further enhanced the intrinsic ability of MSC-EXOs to prevent apoptosis, promote angiogenesis and induce myocardial cell proliferation (39). MSC-EXOs change the activity and function of target cells primarily through the horizontal transfer of these substances (Figure 1). Due to the lack of specific markers, MSC-EXOs are currently prepared according to size or density. Most laboratories separate exosomes from conditioned media via hypercentrifugation, which cannot differentiate exosomes from other EVs or biological macromolecules (40). The MISEV2018recommends using the generic term “small/medium/large EVs” based on its size or density, rather than the classic terms “exosome”, “vesicle”, and “apoptotic body” (19). However, most of the current articles continue to use classic terms. The sizes of small/medium/large EVs are partially overlapped and cannot be strictly distinguished. Therefore, this review takes a cautious attitude towards the absolute definition of different types of vesicles, and focuses on the effects of nanoscale EVs (e.g., exosomes on immune cells) and various autoimmune diseases.
Numerous studies have shown that MSC-EXOs exhibit similar functions to that of MSCs (e.g., repairing damaged tissues, regulating immune responses, and playing anti-inflammatory effects) (10, 41). Although MSC therapy is widely regarded as an effective therapy for several immunological diseases, the direct therapeutic effect of MSCs remains limited. Due to the relatively large size of MSCs, intravascular administration may lead to vascular obstruction, which can result in pulmonary embolism and death in severe cases (42). In addition, allogenic immune rejection or abnormal chromosomal differentiation may occur during in vivo transplantation, and even malignant tumors may form (43). Also, MSCs cells age rapidly and are expensive to have a large-scale production (44). Using MSC-EXOs in humans has several potential advantages over MSCs. First, nano-scale exosomes are free to pass through various biological barriers without blocking microvascular circulation. Second, their application effectively prevents the metastasis of DNA-mutated cells and hinders the tumor development. Third, the number of MSCs decreases rapidly following transplantation, whereas the delivery of MSC-EXOs can continue to function in the body (10). In addition, MSC-EXOs are considered to be non-immunogenic and can be produced in a large-scale production for clinic application (44). However, toxicological studies of nanoparticles in vivo still need to be fully evaluated, particularly following long-term exposure (45). Moreover, the discovery of a broad therapeutic effect of exosome-mediated MSCs eliminates many of the challenges associated with the use of living replicative cells, as it fundamentally shifts living-cell-based MSCs therapy to a “cell-free” treatment, reducing the risk of living cell therapy. Therefore, MSC-EXOs, as cell-secreted natural EVs, has the advantage of being an ideal nanoscale drug carrier.
3 Immunomodulatory Function of Mesenchymal Stem Cell-Derived Exosomes
3.1 Innate Immunity
3.1.1 Macrophages
As an important aspect of the innate immune system, macrophages originate from either the yolk sac during embryonic development or bone marrow-derived monocytes (46). Under microenvironmental activation, macrophages may evolve into an M1 phenotype of pro-inflammatory macrophages or an M2 phenotype of anti-inflammatory macrophages. In general, M1 macrophages secrete pro-inflammatory molecules, including TNF-α and IL-1β, whereas M2 macrophages secrete immune regulatory factors (e.g., IL-10) (47). Recent data support the findings that the anti-inflammatory effect of MSC-EVs is inseparably related to macrophage polarization. MSC-EVs inhibit pro-inflammatory M1 macrophage activation and promote their polarization to M2 macrophages, which are consistent with a decrease in the levels of VEGF-A, IFN-γ, IL-12, and TNF-α, and an upregulation of IL-10 (48, 49). Previous studies have reported that macrophages are the main target cells for MSC-EVs to alleviate colon inflammation. In dextran sodium sulfate (DSS)-induced colitis, MSC-EVs effectively alleviate colitis by inducing an immunosuppressive M2 phenotype exhibited by colonic macrophage polarization. Compared with the control group, MSC-EV-treated mice produced a greater number of IL-10-producing M2 macrophages (49). Similarly, in a study of sepsis conducted by Song et al., the authors demonstrated that MSC-EVs promoted M2 macrophage polarization. Human umbilical cord-derived MSCs(hUC-MSCs) pretreated with IL-1β effectively induce macrophage polarization into an anti-inflammatory M2 phenotype via exosomal miR-146a, which ultimately resulted in prolonging the survival of sepsis mice (50). Moreover, the results showed that MSC-EVs down-regulated the production of IL-23 and IL-22, enhanced the anti-inflammatory phenotype of mature human regulatory macrophages (Mregs), which led to a weakened Th17 response (51). Zhao et al. reported that adipose-derived MSC-EXOs(AD-MSC-EXOs) promoted an M2 macrophage polarization by activating STAT3 transcription, up-regulating the expression of IL-10 and Arg-1 in macrophages, thereby inhibiting the inflammatory response of macrophages (52). In addition, in the rat model of experimental autoimmune uveitis (EAU), Bai et al. demonstrated that MSC-EXOs treatment downregulated the proportion of CD68+ macrophages in the retina and demonstrated the down-regulation of MSC-EXOs on macrophage migration to the retina (Figure 2) (53). Interestingly, MSC-EXOs also enhanced the immunosuppressive function of macrophage precursor, which is called myeloid-derived suppressor cells (MDSCs), a heterogeneous population of immature Myeloid derived cells. IL-6 secreted by olfactory ecto-mesenchymal stem cell-derived exosomes (OE-MSC-EXOs) activates the JAK2/STAT3 pathway in MDSCs, and enhances the inhibitory function of MDSCs by upregulating the levels of arginase, ROS and NO (54). In addition, abundant S100A4 in OE-MSC-EXOs mediated endogenous IL-6 production of MDSCs through TLR4 signaling, and along with exosomal-derived IL-6 promotes the immunosuppressive function of MDSC (54).
3.1.2 Natural Killer Cells
NK cells are important effector cells in the innate immune response and play an important role in the host pathogen defense. When pathogens invade a host, they kill target cells by releasing cytotoxic particles containing perforin and granzyme (55). Although autoimmune diseases are primarily caused by T and B lymphocytes, NK cells possess excessive activation and inhibitory receptors, which can play a role in regulating autologous cell reactivity (56). In addition, NK cells may regulate the activity of other immune cells by secreting cytokines and influence the development of the adaptive immune response through these pathways (57). MSC-EVs primarily induce an immunosuppressive effect on NK cells, including the proliferation, activation, and cytotoxicity of NK cells. In a rat model of EAU, an injection of MSC-EXOs around the eye restored EAU damage by downregulating the transport of CD161+ NK cells in the lesion (53). Recent studies have shown that human fetal liver (FL) MSC-derived exosomes(hFL-MSC-EXOs) mediated downstream TGF-β/Smad signal transduction through the surface expression of TGF-β to inhibit the proliferation and activation of NK cells (58). In human graft-versus host disease (GVHD) experiments, researchers noted that MSC-EXOs suppressed the release of IFN-γ and TNF-α by activated NK cells, which reduced the cytotoxic effect of NK cells, and lessened the inflammatory response (Figure 2) (59).
3.1.3 Dendritic Cells
DCs play an immunological role as antigen presenting cells (APCs), which can ingest, process, and present antigens to T and B lymphocytes (60). Most DCs in the body are immature DCs (iDCs) under normal circumstances and express low levels of class II major histocompatibility complex (MHC II) and T cell costimulatory molecules (CD80, CD86, and CD40). In addition, iDCs up-regulate the surface expression of MHC II and costimulatory molecules after ingesting antigens or cytokine stimulation, and are converted into mature DC (mDCs) (61). Previous studies indicate that the immunosuppressive effect of MSC-EVs on DCs is primarily realized by inhibiting DC maturation and activation. In the presence of MSC-EVs, iDCs are impaired in their ability to receive antigens and differentiate mDCs, which results in the decreased expression of mature and activation markers (e.g., CD83, CD38 and CD80), and correspondingly decreased the secretion of pro-inflammatory cytokines (i.e., IL-6 and IL-12p70) (62). At the same time, MSC-EVs can also enhance the release of TGF-β and IL-10 in CD11c+ DCs, thereby inhibiting lymphocyte proliferation (63). Researchers suggest that MSC-EVs treatment inhibits cell surface expression of MHC II and costimulatory molecules on CD11c+ cells in a dose-dependent manner. The results showed that DCs exhibited a hypoactive phenotype and thereby suppressed the subsequent T cell activation and proliferation (64). Moreover, the co-culture of MSC-EV-treated DCs with T cells reduced Th17 cell counts and IL-17 levels, and increased Foxp3+ regulatory T cells. This finding is consistent with the previous conclusion (65). In brief, there was a weakened ability of iDCs co-cultured with MSC-EXOs to differentiate into mDCs. This led to an inability to perform antigen presentation and effectively stimulate T lymphocyte proliferation. In contrast, it promoted an increase in Treg cells, which indirectly reflected that MSC-EXOs can enhance host immune tolerance (Figure 2).
3.2 Adaptive Immunity
3.2.1 B Cells
B cells are important adaptive immune cells, whose main functions are to mediate humoral immunity and secrete antibodies. The regulation of MSC-EVs on B lymphocytes was investigated in vitro. In the CpG-stimulated PBMC co-culture system, MSC-EVs can completely replicate the inhibitory effect of MSCs on immunoglobulin secretion, B cell proliferation and differentiation in a dose-dependent manner (66, 67). Budoni et al. also claimed that MSC-EVs were internalized by activated CD19+/CD86+ B cells to inhibit B cell proliferation, differentiation, and antibody production, as well as inhibit memory B cell maturation (68). There are functional B cell subsets, known as regulatory B cells (Bregs). The key function of Bregs is to release IL-10, which inhibits the production of proinflammatory cytokines and supports regulatory T cell differentiation (69). MSC-EVs exert dose-dependent anti-inflammatory effects by inhibiting B cell maturation and inducing Bregs in lymph nodes in a murine model of collagen-induced arthritis (CIA) and delayed-type hypersensitivity (DTH). Moreover, MSC-EVs also regulate cellular function through the differential expression of mRNA in related genes. Researchers found that MSC-EVs induced B cells to down-regulate the PI3K/Akt signaling pathway through miR-155-5p, inhibited B cell proliferation and reduced the activation capacity of B lymphocytes (70). Real-time PCR analysis confirmed that following exosome treatment from MSC sources, the expression of genes that played an important role in B cell immune regulation (e.g., CXCL8 and MZB1) were upregulated (67). These studies confirmed that MSC-EXOs can play an immunomodulatory role by acting on B cells (Figure 2).
3.2.2 T Cell
T cell proliferation and activation contribute to the occurrence and development of many autoimmune diseases. MSC-EVs play a negative role in T cell proliferation and activation. MSC-EVs have been shown to carry a variety of active molecules, including TGF-β (68, 71), IDO protein (72), and miR-125a-3p (73). These molecules give MSC-EVs the ability to inhibit T cell proliferation and activation. Studies have revealed that adenosine is associated with a robust immunosuppressive effect, and MSC-EVs inhibits T cell proliferation in vitro through adenosine signal transduction (74). MSC-EVs can also adjust the balance of T helper type 1 (Th1) and T helper type 2 (Th2) cells and reconstruct the stable state of Th1 and Th2. Chen et al. co-cultured bone marrow mesenchymal stem cell (BM-MSC)-derived exosomes (BM-MSC-EXOs) and peripheral blood mononuclear cells. The authors subsequently found that it could promote the Th1 to Th2 conversion of helper T cells, significantly reduced the levels of pro-inflammatory factors, IL-1β and TNF-a, and improved the levels of anti-inflammatory TGF-β (66). In a similar study, human adipose tissue MSC-EXOs inhibited the differentiation and activation of T cells and the release of pro-inflammatory factors (e.g., IFN-γ) (75). In addition, MSC-EXOs can facilitate the differentiation of Tregs. Treg cells are immune cells with negative feedback regulation. Similarly, inflammatory IL-1β-primed MSC-EVs upregulated PD-L1 and TGF-β expression, which induced the apoptosis of activated T cells, and increases the proportion of Treg cells in a mouse model of autoimmune encephalomyelitis (76). Previous studies in our laboratory have also found that OE-MSC-EXOs had a potent inhibitory effect on the proliferation of CD4+ T cells in experimental colitis mice. At the same time, the secretion of IL-17 and IFN-γ by T cells was reduced, whereas the secretion of TGF-β and IL-10 was enhanced, which could significantly slow disease progression (77). Following treatment with OE-MSC-EXOs, the Th1/Th17 subsets were significantly reduced, while Treg cells were increased (77). It was found that transgene-free human induced pluripotent stem cells (iPSCs) derived EVs could prevent the progression of Sjögren’s syndrome (SS) by inhibiting the differentiation of follicular helper T (Tfh) and Th17 cells (78). In addition, other studies have reported that MSC-EXOs can promote the differentiation of monocytes into an M2 macrophage phenotype, thereby activating the differentiation of CD4+ T cells into Treg cells and delaying the occurrence of immune rejection (Figure 2) (79).
4 Applications of Mesenchymal Stem Cell-Derived Exosomes in Autoimmune Diseases
To develop EVs as a cell-free therapy and elucidate its potential role in stem cell effects in vivo, the following contents can assess the role of exosomes released by MSCs in the treatment of autoimmune diseases, including Multiple sclerosis (MS), Systemic lupus erythematosus (SLE), Type-1 diabetes (T1DM), uveitis, Rheumatoid arthritis (RA), and Inflammatory bowel disease (IBD) (Table 1).
4.1 Multiple Sclerosis
MS is the most common inflammatory disease of the central nervous system (CNS), which is characterized by demyelination, neuronal damage and loss, and ultimately neurological dysfunction (80). Experimental autoimmune encephalomyelitis (EAE) is the commonly used animal model of MS (81). As the primary immune cells of the central nervous system, microglia can maintain tissue homeostasis and contribute to central nervous system development under normal physiological conditions (82). Following pathogen invasion, activated microglia with an M1 phenotype as the first line of defense secrete pro-inflammatory cytokines to eliminate invading pathogens. However, when tissue homeostasis is restored, microglia exhibiting an M2 phenotype need to be activated, otherwise the excessive release of pro-inflammatory cytokines can lead to neuronal damage (83). Microglia have both neurodestructive and neuroprotective functions. An imbalance of the M1/M2 phenotype inhibits the nerve protection function and promotes the occurrence of MS (84). Therefore, M1 to M2 polarization of microglia may be beneficial to the neuroprotective function of microglia, thereby preventing disease progression. The study by Zijian et al. demonstrated that BM-MSC-EXOs therapy inhibits microglia from developing into an M1 phenotype, promotes M2 phenotype polarization, and secretes anti-inflammatory cytokines (e.g., TNF-α, IL-10, and TGF-β). Moreover, BM-MSC-EXOs treatment significantly improved the neurobehavioral symptoms of EAE rats and relieved the inflammation and demyelination of CNS (85). Human periodontal ligament stem cells (hPDLSCs)-EVs from MS patients has been shown to inhibit NALP3 inflammasome activation in an EAE model (86). Although substantial progress has been made in the treatment of MS, the appropriate treatment for MS remains controversial. The currently available drugs may have potentially harmful side effects and cannot meet future needs (87). MSC-EXOs is a natural non-toxic vesicle that can deliver mRNA, miRNA, and proteins, as well as alleviate the condition of EAE by regulating microglia polarization (88). As the carrier of MSC-specific tolerance molecules (e.g., PD-L1, Galecin-1 [GAL-1], and TGF-β), MSC-EVs can effectively inhibit the activation and proliferation of self-responding lymphocytes and promote the secretion of anti-inflammatory cytokines derived from lymphocytes, thereby alleviating EAE disease progression (76). In another study, it was also shown that the infiltration of microglia in spinal cord sections treated with MSC-EXOs was significantly reduced, and MSC-EXOs induced the formation of Tregs and alleviated the development of EAE (89). Therefore, MSC-EXOs may hold great potential for the treatment of MS.
4.2 Systemic Lupus Erythematosus
SLE is a chronic autoimmune disease characterized by immune inflammation and multiple organ damage. In addition, SLE pathogenesis is extremely complex, primarily due to the comprehensive influence of genetic, environmental, hormonal, epigenetic, and other factors (90). With the help of Tfh cells, anti-nuclear antibodies (ANA) are produced, leading to the deposition of immune complexes in vital organs. The immune complex triggers an influx of large inflammatory cells by activating the complement cascade, causing tissue inflammatory damage (e.g., nephritis) (91). Nephritis represents the leading cause of morbidity and mortality in SLE and occurs in approximately half of all patients (92). In previous studies, researchers found that the infusion of hBM-MSCs into mouse models of lupus nephritis reduced the level of autoantibodies, improved the survival rate in mice, and alleviated the clinical symptoms of glomerulonephritis by inhibiting the development of Tfh (91). Moreover, the loss of BM-MSC/osteoblast function in an SLE mouse model results in an impairment of the osteoblastic niche and an imbalance of immune homeostasis. Allogeneic bone marrow mesenchymal stem cell transplantation (MSCT) plays a positive role in reconstructing the osteoblast niche and restoring immune homeostasis; thus, effectively reversing multiple organ dysfunction (93). The above findings confirm a positive therapeutic effect of MSCs on SLE; however, cellular therapy continues to face technical, cost, and regulatory challenges in clinical trials. Recent studies have demonstrated that the immunoregulatory activity of MSCs was mainly mediated by paracrine factors (e.g., MSC-EVs) (66). In a novel porcine model of coexisting metabolic syndrome (MetS) and renal artery stenosis (RAS), MSC-EVs isolated from adipose tissue were capable of improving renal structuring and function, and reducing renal injury and dysfunction by up-regulating the level of IL-10 expression (94). Although MSC-EVs have been observed to inhibit autoimmune diseases in vitro, there have been no studies on the use of MSC-EVs for the treatment of SLE in mice or humans. In the future, MSCs are expected to provide a novel and safe treatment for SLE patients.
4.3 Type-1 Diabetes
T1DM is considered a chronic autoimmune disease that is influenced by genetic, immune, and environmental factors (95). T1DM is primarily caused by the autoimmune destruction of beta-cells in the islets of Langerhans, leading to insufficient insulin secretion (96). Non-obese diabetic (NOD) mice are the preferred spontaneous disease model of T1DM. NOD mice exhibited the same clinical symptoms of diabetes as human beings-hyperglycemia, polyuria, and polydipsia (95). Although insulin therapy remains the main treatment method in the short term, MSCs have recently attracted wide attention as a promising method for the treatment of diabetes (96). MSCs display immunomodulatory properties in inflammatory diseases, and their immunomodulatory effects have been studied in T1DM (97, 98). Human MSCs have the ability to delay the onset of autoimmune diabetes by inhibiting the development of Th1 cells, which may improve the efficacy of islet transplantation in patients with T1DM (99). Currently, it is generally believed that a paracrine mechanism of action is more direct in MSCs in vivo, particularly MSC-EXOs (10). In previous studies, the effect of MSC-EXOs on diabetes has been investigated. Previous studies have shown that exosomes released by BM-MSCs had similar functions to those of BM-MSCs, and they had the ability to improve cognitive impairment in diabetic mice by repairing damaged neurons and astrocytes (100). In another study, AD-MSC-EXOs were found to have a significant mitigatory effect on T1MD by increasing the expression of anti-inflammatory factors (e.g., IL-10), and the population of Tregs that are equipped to suppress the immune response, preventing immune overactivation and autoimmune damage (101). In addition, the results of the study by Shigemoto-kuroda et al. also confirmed that MSC-EVs could inhibit islet inflammation, significantly increasing the plasma insulin levels, and effectively delaying the occurrence of T1DM in mice (64). These results suggested that MSC-EVs have great potential as a cellular therapy for the prevention of T1DM.
4.4 Uveitis
Uveitis is the leading cause of visual disability worldwide. Uveitis can be divided into three categories according to etiology: 1) infectious uveitis; 2) non-infectious uveitis; and 3) masquerade uveitis, of which non-infectious uveitis is believed to be caused by autoimmunity, and EAU is used as a mouse model (102). Traditional immunosuppressive drugs (e.g., corticosteroids) and novel biological agents have been shown to be effective for the treatment of uveitis; however, side effects and unknown long-term safety often limit the use of these drugs (103). A large number of experimental results have suggested that MSC-EXOs have a positive effect on inflammatory eye disease. EAU mice that do not MSC-EXOs therapy have severely damaged retinal photoreceptors and the infiltration of inflammatory cells, whereas EAU mice with exosomes injected through tail vein displayed eyes similar to that of normal mouse retinas, with almost no structural damage and inflammatory infiltration (64). Compared with EAU mice treated with PBS, mice treated with MSC-EXOs exhibited a significant decrease in CD3+ T cells infiltrating the retina and a decrease in the number of macrophages migrating to the retina (53). In addition, Th1 and Th17 cells represent important pathogenic factors in the development of EAU disease. Flow cytometry results of the cervical draining lymph nodes (CLNs) showed that the number of IFN-γ+CD4+ cells (Th1) and IL-17+CD4+ cells (Th17) in EAU mice treated with MSC-EXOs was significantly lower than that in the PBS-treated mice (64). The above results indicate that MSC-EXOs can inhibit the development of EAU by inhibiting Th1 and Th17 cells. Part of the immunomodulatory function of MSCs on EAU is to induce the transformation of CD4+ T cells into antigen-specific CD4+CD25+Foxp3+ Tregs by secreting TGF-β in a paracrine manner (104). In another study, MSC-EVs alleviated EAU by directly inhibiting the development of Th1 and Th17 cells rather than inducing Tregs to inhibit T cell proliferation (64). Although there may be some differences between MSC-EVs and MSCs in various immunomodulatory pathways, the treatment of EAU using MSC-EVs also represents a potential new method.
4.5 Rheumatoid Arthritis
RA is a chronic inflammatory disease characterized by synovial hyperplasia and immune cell infiltration, leading to joint destruction (105, 106). The microvesicles derived from BM-MSCs carry the regulatory molecules that exist in the mother cell, including PD-L1, GAL-1, and TGF-β1 (76). As a PD-1 receptor, PD-L1 plays a key role in regulating the development of inducible T regulatory cells (iTregs) (107). In addition, GAL-1 is an endogenous lectin that has been shown to induce growth arrest and the apoptosis of activated T cells, as well as the immunoregulation mediated by regulatory T cells (108, 109). Researchers found that the injection of co-gene DBA/1 fibroblasts secreting GAL-1 inhibited the progression of arthritis through T cell apoptosis in collagen-induced arthritis (110).
Moreover, exosomal miRNA also plays an important role in alleviating the development of RA (111). For example, MSC-derived miRNA-150-5p-expressing exosomes decreased the migration and invasion in RA fibroblast-like synoviocytes (FLS) and downregulated tube formation in HUVECs by targeting MMP14 and VEGF (112). In 2020, researchers found that BM-MSCs-secreted exosomal miRNA-320a and miRNA-192-5p also acted on FLS, reducing inflammatory response and alleviating the progression of RA (113, 114). Despite the pathogenic role of B cells in RA, recent studies have demonstrated the therapeutic effect of MSC-EXOs via expanding Bregs (48, 115). BM-MSC-EXOs-treated CIA mice exhibited a lower disease incidence and deceased clinical score, accompanied by reduced levels of serum auto-antibodies (115). Furthermore, such phenomena was associated with decreased plasmablast differentiation and the generation of Bregs (48). Interestingly, similar therapeutic effects have been revealed in osteoarthritis (OA). The lncRNA KLF3-AS1 was significantly enriched in MSC-EXOs, which promoted cartilage repair and chondrocyte proliferation in OA rat models (116). In addition, in a model of porcine synovitis induced by bovine serum albumin, the intraarticular injection of BM-MSC-EVs into pigs had an anti-inflammatory effect, with a reduced number of synovial lymphocytes and down-regulated level of TNF-α transcription (117). These results provide evidence for a role of MSC-EVs for the treatment of inflammatory diseases (e.g., arthritis). MSC-EVs provide novel insight into the treatment of RA, which may lead to new therapeutic opportunities and strategies for RA.
4.6 Inflammatory Bowel Disease
IBD is a chronic, nonspecific, relapsing inflammatory gastrointestinal disease associated with mucosal immune system disorders and gastrointestinal injury. IBD, which primarily includes ulcerative colitis (UC) and Crohn’s disease (CD), has become a global disease with an increasing incidence (118). Studies of this disease have mainly used DSS and 2,4,6-trinitrobenzenesulfonic acid (TNBS) to induce IBD in mouse models (119). Existing drugs to treat IBD are still very limited, Therefore, there is an urgent need to develop safe and effective treatments for IBD (120). At present, several studies have shown that MSC-EXOs/exosome components have potential functions in the development of IBD and can serve as potential targets for the diagnosis and treatment of IBD. In a model of IBD induced by DSS, hUC-MSC-EXOs treatment decreased the infiltration of macrophages in colon tissue and inhibited the expression of IL-7 (121). hUC-MSC-EXOs also inhibited neddylation and alleviated IBD by miR-326 (122). Another study also showed that exosomal miRNA of MSCs, such us miR-146a, downregulated TNF receptor-associated factor 6 (TRAF6) and IL-1 receptor-associated kinase 1 (IRAK1) expression, inhibited pro-inflammatory cytokine and enhanced the expression of IL-10 (123).Moreover, tumor necrosis factor-α stimulated gene 6 (TSG-6), as detected by hUC-MSC-EXOs, regulated the immune response of Th2 and Th17 cells in mesenteric lymph nodes (MLN), down-regulated the levels of pro-inflammatory cytokines in colon tissue, and up-regulated the levels of anti-inflammatory cytokines to protect the intestinal barrier (119). Other tissue-derived MSC-EXOs showed similar efficacy to that of hUC-MSC-EXOs in the treatment of DSS induced IBD. OE-MSC-EXOs significantly improved the severity of experimental colitis in mice primarily by modulating the immune response of Th-cells, including a significant reduction in Th1/Th17 subsets and an increase in Treg cells (77). Neda Heidari et al. reported that AD-MSC-EXOs therapy could restore the percentage of Treg in the spleen to a baseline level similar to that in normal mice and improved inflammation in DSS induced acute colitis (124). Furthermore, metallothionein-2 in hBM-MSC-EXOs inhibited inflammatory responses, polarized M2b macrophages, and maintained intestinal barrier integrity (125). With the further research on exosomes, exosomal-structure design of novel drugs may provide new insights for IBD.
5 Conclusion and Prospects
The treatment of autoimmune diseases is challenging and there is currently no effective cure. In this review, we discussed the regulatory role of MSC-EXOs on immune cells and the new progress of MSC-EXOs in the treatment of autoimmune diseases, suggesting that MSC-EXOs may be a new cell-free drug for the treatment of autoimmune diseases.
In view of the therapeutic potential of MSC-EXOs in preclinical studies, there are currently 19 clinical trials looking at its application in a variety of diseases (Available online: http://www.clinicaltrials.gov/). Although encouraging results have been achieved with MSC-EXOs, several uncharted territories remain to be explored before MSC-EXOs can be used as drug vectors for clinical use. First, although it has been reported that clinical-grade exosomes can be produced using good manufacturing techniques and standard operating procedures, large-scale production of exosomes for clinical use remains to be explored (126). Second, support materials can be used to maximize the therapeutic power of MSC-EXOs. In recent years, hydrogel has attracted much attention among biocompatible auxiliary materials. In the experimental model of ischemia, the retention time of MSC-EXOs with an injectable hydrogel was prolonged, which enhanced the therapeutic effect of exosomes (127). In the experiment of preventing hyperplastic scar in rabbit ear model, adipose-derived stem cell conditioned Medium (ADSC-CM) was combined with polysaccharide hydrogel to prolong the therapeutic effect of cytokines (128). Third, in order to ensure the safety of patients treated with MSC-EXOs, the route and dose of exosomes must be explored. At present, the main route of administration in clinical studies is intravenous infusion. However, aerosol inhalation of MSC-EXOs was used in clinical trials to explore the efficacy of MSC-EXOs in severe pulmonary diseases (Clinical Trials. Gov Identifier: NCT04313647). In addition, the clinical trial assessing the safety and efficacy of MSC-EXOs in Patients with Alzheimer’s disease was conducted with nasal drip (ClinicalTrials.gov Identifier: NCT04388982). Therefore, the route of exosome administration needs to be determined according to the actual situation of the disease. In addition, clinical trials need to monitor patients treated with MSC-EXOs in real time to ensure that the smallest dose is most effective. Therefore, future work should focus on the combination of basic research on MSC-EXOs with emerging technologies to bring new breakthroughs for the treatment of autoimmune diseases.
Author Contributions
ZS, WH, and JL drafted and revised the manuscript. JT and SW discussed and revised the manuscript. KR conceived the topic and revised the manuscript. All authors contributed to the article and approved the submitted version.
Funding
This work was supported by the National Natural Science Foundation of China (Grant Nos. 81971542, 82171771), Summit of the Six Top Talents Program of Jiangsu Province (Grant No. 2017-YY-006).
Conflict of Interest
The authors declare that the research was conducted in the absence of any commercial or financial relationships that could be construed as a potential conflict of interest.
Publisher’s Note
All claims expressed in this article are solely those of the authors and do not necessarily represent those of their affiliated organizations, or those of the publisher, the editors and the reviewers. Any product that may be evaluated in this article, or claim that may be made by its manufacturer, is not guaranteed or endorsed by the publisher.
References
1. Ding DC, Shyu WC, Lin SZ. Mesenchymal Stem Cells. Cell Transplant (2011) 20(1):5–14. doi: 10.3727/096368910X
2. Pittenger MF, Mackay AM, Beck SC, Jaiswal RK, Douglas R, Mosca JD, et al. Multilineage Potential of Adult Human Mesenchymal Stem Cells. Science (1999) 284(5411):143–7. doi: 10.1126/science.284.5411.143
3. Crisan M, Yap S, Casteilla L, Chen CW, Corselli M, Park TS, et al. A Perivascular Origin for Mesenchymal Stem Cells in Multiple Human Organs. Cell Stem Cell (2008) 3(3):301–13. doi: 10.1016/j.stem.2008.07.003
4. Dominici M, Le Blanc K, Mueller I, Slaper-Cortenbach I, Marini F, Krause D, et al. Minimal Criteria for Defining Multipotent Mesenchymal Stromal Cells. The International Society for Cellular Therapy Position Statement. Cytotherapy (2006) 8(4):315–7. doi: 10.1080/14653240600855905
5. Nauta AJ, Fibbe WE. Immunomodulatory Properties of Mesenchymal Stromal Cells. Blood (2007) 110(10):3499–506. doi: 10.1182/blood-2007-02-069716
6. Castro-Manrreza ME, Montesinos JJ. Immunoregulation by Mesenchymal Stem Cells: Biological Aspects and Clinical Applications. J Immunol Res (2015) 2015:394917. doi: 10.1155/2015/394917
7. de Castro LL, Lopes-Pacheco M, Weiss DJ, Cruz FF, Rocco PRM. Current Understanding of the Immunosuppressive Properties of Mesenchymal Stromal Cells. J Mol Med (Berlin Germany) (2019) 97(5):605–18. doi: 10.1007/s00109-019-01776-y
8. Asari S, Itakura S, Ferreri K, Liu CP, Kuroda Y, Kandeel F, et al. Mesenchymal Stem Cells Suppress B-Cell Terminal Differentiation. Exp Hematol (2009) 37(5):604–15. doi: 10.1016/j.exphem.2009.01.005
9. Shokri MR, Bozorgmehr M, Ghanavatinejad A, Falak R, Aleahmad M, Kazemnejad S, et al. Human Menstrual Blood-Derived Stromal/Stem Cells Modulate Functional Features of Natural Killer Cells. Sci Rep (2019) 9(1):10007. doi: 10.1038/s41598-019-46316-3
10. Phinney DG, Pittenger MF. Concise Review: MSC-Derived Exosomes for Cell-Free Therapy. Stem Cells (2017) 35(4):851–8. doi: 10.1002/stem.2575
11. Liang X, Ding Y, Zhang Y, Tse HF, Lian Q. Paracrine Mechanisms of Mesenchymal Stem Cell-Based Therapy: Current Status and Perspectives. Cell Transplant (2014) 23(9):1045–59. doi: 10.3727/096368913x667709
12. Bazzoni R, Takam Kamga P, Tanasi I, Krampera M. Extracellular Vesicle-Dependent Communication Between Mesenchymal Stromal Cells and Immune Effector Cells. Front Cell Dev Biol (2020) 8:596079. doi: 10.3389/fcell.2020.596079
13. Chargaff E, West R. The Biological Significance of the Thromboplastic Protein of Blood. J Biol Chem (1946) 166(1):189–97. doi: 10.1016/S0021-9258(17)34997-9
14. Wolf P. The Nature and Significance of Platelet Products in Human Plasma. Br J haematol (1967) 13(3):269–88. doi: 10.1111/j.1365-2141.1967.tb08741.x
15. Trams EG, Lauter CJ, Salem N Jr., Heine U. Exfoliation of Membrane Ecto-Enzymes in the Form of Micro-Vesicles. Biochim Biophys Acta (1981) 645(1):63–70. doi: 10.1016/0005-2736(81)90512-5
16. Pan BT, Teng K, Wu C, Adam M, Johnstone RM. Electron Microscopic Evidence for Externalization of the Transferrin Receptor in Vesicular Form in Sheep Reticulocytes. J Cell Biol (1985) 101(3):942–8. doi: 10.1083/jcb.101.3.942
17. Johnstone RM, Bianchini A, Teng K. Reticulocyte Maturation and Exosome Release: Transferrin Receptor Containing Exosomes Shows Multiple Plasma Membrane Functions. Blood (1989) 74(5):1844–51. doi: 10.1182/blood.V74.5.1844.1844
18. Lötvall J, Hill AF, Hochberg F, Buzás EI, Di Vizio D, Gardiner C, et al. Minimal Experimental Requirements for Definition of Extracellular Vesicles and Their Functions: A Position Statement From the International Society for Extracellular Vesicles. J Extracell Vesicles (2014) 3:26913. doi: 10.3402/jev.v3.26913
19. Théry C, Witwer KW, Aikawa E, Alcaraz MJ, Anderson JD, Andriantsitohaina R, et al. Minimal Information for Studies of Extracellular Vesicles 2018 (MISEV2018): A Position Statement of the International Society for Extracellular Vesicles and Update of the MISEV2014 Guidelines. J Extracellular Vesicles (2018) 7(1):1535750. doi: 10.1080/20013078.2018.1535750
20. Kalluri R, LeBleu VS. The Biology, Function, and Biomedical Applications of Exosomes. Sci (New York NY) (2020) 367(6478):640–+. doi: 10.1126/science.aau6977
21. Harrell CR, Jankovic MG, Fellabaum C, Volarevic A, Djonov V, Arsenijevic A, et al. Molecular Mechanisms Responsible for Anti-Inflammatory and Immunosuppressive Effects of Mesenchymal Stem Cell-Derived Factors. Adv Exp Med Biol (2019) 1084:187–206. doi: 10.1007/5584_2018_306
22. Lai P, Weng J, Guo L, Chen X, Du X. Novel Insights Into MSC-EVs Therapy for Immune Diseases. Biomark Res (2019) 7(1):6. doi: 10.1186/s40364-019-0156-0
23. Yáñez-Mó M, Siljander PR, Andreu Z, Zavec AB, Borràs FE, Buzas EI, et al. Biological Properties of Extracellular Vesicles and Their Physiological Functions. J Extracellular Vesicles (2015) 4:27066. doi: 10.3402/jev.v4.27066
24. Riazifar M, Pone EJ, Lötvall J, Zhao W. Stem Cell Extracellular Vesicles: Extended Messages of Regeneration. Annu Rev Pharmacol Toxicol (2017) 57:125–54. doi: 10.1146/annurev-pharmtox-061616-030146
25. Xie M, Xiong W, She Z, Wen Z, Abdirahman AS, Wan W, et al. Immunoregulatory Effects of Stem Cell-Derived Extracellular Vesicles on Immune Cells. Front Immunol (2020) 11:13. doi: 10.3389/fimmu.2020.00013
26. Battistelli M, Falcieri E. Apoptotic Bodies: Particular Extracellular Vesicles Involved in Intercellular Communication. Biology (2020) 9(1):21. doi: 10.3390/biology9010021
27. Harrell CR, Fellabaum C, Jovicic N, Djonov V, Arsenijevic N, Volarevic V. Molecular Mechanisms Responsible for Therapeutic Potential of Mesenchymal Stem Cell-Derived Secretome. Cells (2019) 8(5):467. doi: 10.3390/cells8050467
28. Costa Verdera H, Gitz-Francois JJ, Schiffelers RM, Vader P. Cellular Uptake of Extracellular Vesicles is Mediated by Clathrin-Independent Endocytosis and Macropinocytosis. J Controlled Release Off J Controlled Release Soc (2017) 266:100–8. doi: 10.1016/j.jconrel.2017.09.019
29. Rai AK, Johnson PJ. Trichomonas Vaginalis Extracellular Vesicles are Internalized by Host Cells Using Proteoglycans and Caveolin-Dependent Endocytosis. Proc Natl Acad Sci USA (2019) 116(43):21354–60. doi: 10.1073/pnas.1912356116
30. Svensson KJ, Christianson HC, Wittrup A, Bourseau-Guilmain E, Lindqvist E, Svensson LM, et al. Exosome Uptake Depends on ERK1/2-Heat Shock Protein 27 Signaling and Lipid Raft-Mediated Endocytosis Negatively Regulated by Caveolin-1. J Biol Chem (2013) 288(24):17713–24. doi: 10.1074/jbc.M112.445403
31. Di Trapani M, Bassi G, Midolo M, Gatti A, Kamga PT, Cassaro A, et al. Differential and Transferable Modulatory Effects of Mesenchymal Stromal Cell-Derived Extracellular Vesicles on T, B and NK Cell Functions. Sci Rep (2016) 6:24120. doi: 10.1038/srep24120
32. Hunter MP, Ismail N, Zhang X, Aguda BD, Lee EJ, Yu L, et al. Detection of microRNA Expression in Human Peripheral Blood Microvesicles. PloS One (2008) 3(11):e3694. doi: 10.1371/journal.pone.0003694
33. Robbins PD, Morelli AE. Regulation of Immune Responses by Extracellular Vesicles. Nat Rev Immunol (2014) 14(3):195–208. doi: 10.1038/nri3622
34. LR T, Sánchez-Abarca LI, Muntión S, Preciado S, Puig N, López-Ruano G, et al. MSC Surface Markers (CD44, CD73, and CD90) can Identify Human MSC-Derived Extracellular Vesicles by Conventional Flow Cytometry. Cell Commun Signaling CCS (2016) 14:2. doi: 10.1186/s12964-015-0124-8
35. Joo HS, Suh JH, Lee HJ, Bang ES, Lee JM. Current Knowledge and Future Perspectives on Mesenchymal Stem Cell-Derived Exosomes as a New Therapeutic Agent. Int J Mol Sci (2020) 21(3):727. doi: 10.3390/ijms21030727
36. Kim HS, Choi DY, Yun SJ, Choi SM, Kang JW, Jung JW, et al. Proteomic Analysis of Microvesicles Derived From Human Mesenchymal Stem Cells. J Proteome Res (2012) 11(2):839–49. doi: 10.1021/pr200682z
37. Yuan O, Lin C, Wagner J, Archard JA, Deng P, Halmai J, et al. Exosomes Derived From Human Primed Mesenchymal Stem Cells Induce Mitosis and Potentiate Growth Factor Secretion. Stem Cells Dev (2019) 28(6):398–409. doi: 10.1089/scd.2018.0200
38. Bolukbasi MF, Mizrak A, Ozdener GB, Madlener S, Ströbel T, Erkan EP, et al. miR-1289 and "Zipcode"-Like Sequence Enrich mRNAs in Microvesicles. Mol Ther Nucleic Acids (2012) 1(2):e10. doi: 10.1038/mtna.2011.2
39. Ferguson SW, Wang J, Lee CJ, Liu M, Neelamegham S, Canty JM, et al. The microRNA Regulatory Landscape of MSC-Derived Exosomes: A Systems View. Sci Rep (2018) 8(1):1419. doi: 10.1038/s41598-018-19581-x
40. Kim DK, Nishida H, An SY, Shetty AK, Bartosh TJ, Prockop DJ. Chromatographically Isolated CD63+CD81+ Extracellular Vesicles From Mesenchymal Stromal Cells Rescue Cognitive Impairments After TBI. Proc Natl Acad Sci USA (2016) 113(1):170–5. doi: 10.1073/pnas.1522297113
41. Yu B, Zhang X, Li X. Exosomes Derived From Mesenchymal Stem Cells. Int J Mol Sci (2014) 15(3):4142–57. doi: 10.3390/ijms15034142
42. Furlani D, Ugurlucan M, Ong L, Bieback K, Pittermann E, Westien I, et al. Is the Intravascular Administration of Mesenchymal Stem Cells Safe? Mesenchymal Stem Cells and Intravital Microscopy. Microvasc Res (2009) 77(3):370–6. doi: 10.1016/j.mvr.2009.02.001
43. Jeong JO, Han JW, Kim JM, Cho HJ, Park C, Lee N, et al. Malignant Tumor Formation After Transplantation of Short-Term Cultured Bone Marrow Mesenchymal Stem Cells in Experimental Myocardial Infarction and Diabetic Neuropathy. Circ Res (2011) 108(11):1340–7. doi: 10.1161/circresaha.110.239848
44. Xunian Z, Kalluri R. Biology and Therapeutic Potential of Mesenchymal Stem Cell-Derived Exosomes. Cancer Sci (2020) 111(9):3100–10. doi: 10.1111/cas.14563
45. Malam Y, Loizidou M, Seifalian AM. Liposomes and Nanoparticles: Nanosized Vehicles for Drug Delivery in Cancer. Trends Pharmacol Sci (2009) 30(11):592–9. doi: 10.1016/j.tips.2009.08.004
46. Epelman S, Lavine KJ, Randolph GJ. Origin and Functions of Tissue Macrophages. Immunity (2014) 41(1):21–35. doi: 10.1016/j.immuni.2014.06.013
47. Murray PJ, Allen JE, Biswas SK, Fisher EA, Gilroy DW, Goerdt S, et al. Macrophage Activation and Polarization: Nomenclature and Experimental Guidelines. Immunity (2014) 41(1):14–20. doi: 10.1016/j.immuni.2014.06.008
48. Cosenza S, Ruiz M, Toupet K, Jorgensen C, Noël D. Mesenchymal Stem Cells Derived Exosomes and Microparticles Protect Cartilage and Bone From Degradation in Osteoarthritis. Sci Rep (2017) 7(1):16214. doi: 10.1038/s41598-017-15376-8
49. Cao L, Xu H, Wang G, Liu M, Tian D, Yuan Z. Extracellular Vesicles Derived From Bone Marrow Mesenchymal Stem Cells Attenuate Dextran Sodium Sulfate-Induced Ulcerative Colitis by Promoting M2 Macrophage Polarization. Int Immunopharmacol (2019) 72:264–74. doi: 10.1016/j.intimp.2019.04.020
50. Song Y, Dou H, Li X, Zhao X, Li Y, Liu D, et al. Exosomal miR-146a Contributes to the Enhanced Therapeutic Efficacy of Interleukin-1β-Primed Mesenchymal Stem Cells Against Sepsis. Stem Cells (2017) 35(5):1208–21. doi: 10.1002/stem.2564
51. Hyvärinen K, Holopainen M, Skirdenko V, Ruhanen H, Lehenkari P, Korhonen M, et al. Mesenchymal Stromal Cells and Their Extracellular Vesicles Enhance the Anti-Inflammatory Phenotype of Regulatory Macrophages by Downregulating the Production of Interleukin (IL)-23 and IL-22. Front Immunol (2018) 9:771. doi: 10.3389/fimmu.2018.00771
52. Zhao H, Shang Q, Pan Z, Bai Y, Li Z, Zhang H, et al. Exosomes From Adipose-Derived Stem Cells Attenuate Adipose Inflammation and Obesity Through Polarizing M2 Macrophages and Beiging in White Adipose Tissue. Diabetes (2018) 67(2):235–47. doi: 10.2337/db17-0356
53. Bai L, Shao H, Wang H, Zhang Z, Su C, Dong L, et al. Effects of Mesenchymal Stem Cell-Derived Exosomes on Experimental Autoimmune Uveitis. Sci Rep (2017) 7(1):4323. doi: 10.1038/s41598-017-04559-y
54. Rui K, Hong Y, Zhu Q, Shi X, Xiao F, Fu H, et al. Olfactory Ecto-Mesenchymal Stem Cell-Derived Exosomes Ameliorate Murine Sjögren’s Syndrome by Modulating the Function of Myeloid-Derived Suppressor Cells. Cell Mol Immunol (2021) 18(2):440–51. doi: 10.1038/s41423-020-00587-3
55. Zitti B, Bryceson YT. Natural Killer Cells in Inflammation and Autoimmunity. Cytokine Growth Factor Rev (2018) 42:37–46. doi: 10.1016/j.cytogfr.2018.08.001
56. Poggi A, Zocchi MR. NK Cell Autoreactivity and Autoimmune Diseases. Front Immunol (2014) 5:27. doi: 10.3389/fimmu.2014.00027
57. Gianchecchi E, Delfino DV, Fierabracci A. NK Cells in Autoimmune Diseases: Linking Innate and Adaptive Immune Responses. Autoimmun Rev (2018) 17(2):142–54. doi: 10.1016/j.autrev.2017.11.018
58. Fan Y, Herr F, Vernochet A, Mennesson B, Oberlin E, Durrbach A. Human Fetal Liver Mesenchymal Stem Cell-Derived Exosomes Impair Natural Killer Cell Function. Stem Cells Dev (2019) 28(1):44–55. doi: 10.1089/scd.2018.0015
59. Kordelas L, Rebmann V, Ludwig AK, Radtke S, Ruesing J, Doeppner TR, et al. MSC-Derived Exosomes: A Novel Tool to Treat Therapy-Refractory Graft-Versus-Host Disease. Leukemia (2014) 28(4):970–3. doi: 10.1038/leu.2014.41
60. Sozzani S, Del Prete A, Bosisio D. Dendritic Cell Recruitment and Activation in Autoimmunity. J Autoimmun (2017) 85:126–40. doi: 10.1016/j.jaut.2017.07.012
61. Théry C, Amigorena S. The Cell Biology of Antigen Presentation in Dendritic Cells. Curr Opin Immunol (2001) 13(1):45–51. doi: 10.1016/s0952-7915(00)00180-1
62. Reis M, Mavin E, Nicholson L, Green K, Dickinson AM, Wang XN. Mesenchymal Stromal Cell-Derived Extracellular Vesicles Attenuate Dendritic Cell Maturation and Function. Front Immunol (2018) 9:2538. doi: 10.3389/fimmu.2018.02538
63. Shahir M, Mahmoud Hashemi S, Asadirad A, Varahram M, Kazempour-Dizaji M, Folkerts G, et al. Effect of Mesenchymal Stem Cell-Derived Exosomes on the Induction of Mouse Tolerogenic Dendritic Cells. J Cell Physiol (2020) 235(10):7043–55. doi: 10.1002/jcp.29601
64. Shigemoto-Kuroda T, Oh JY, Kim DK, Jeong HJ, Park SY, Lee HJ, et al. MSC-Derived Extracellular Vesicles Attenuate Immune Responses in Two Autoimmune Murine Models: Type 1 Diabetes and Uveoretinitis. Stem Cell Rep (2017) 8(5):1214–25. doi: 10.1016/j.stemcr.2017.04.008
65. Favaro E, Carpanetto A, Caorsi C, Giovarelli M, Angelini C, Cavallo-Perin P, et al. Human Mesenchymal Stem Cells and Derived Extracellular Vesicles Induce Regulatory Dendritic Cells in Type 1 Diabetic Patients. Diabetologia (2016) 59(2):325–33. doi: 10.1007/s00125-015-3808-0
66. Chen W, Huang Y, Han J, Yu L, Li Y, Lu Z, et al. Immunomodulatory Effects of Mesenchymal Stromal Cells-Derived Exosome. Immunol Res (2016) 64(4):831–40. doi: 10.1007/s12026-016-8798-6
67. Khare D, Or R, Resnick I, Barkatz C, Almogi-Hazan O, Avni B. Mesenchymal Stromal Cell-Derived Exosomes Affect mRNA Expression and Function of B-Lymphocytes. Front Immunol (2018) 9:3053. doi: 10.3389/fimmu.2018.03053
68. Budoni M, Fierabracci A, Luciano R, Petrini S, Di Ciommo V, Muraca M. The Immunosuppressive Effect of Mesenchymal Stromal Cells on B Lymphocytes Is Mediated by Membrane Vesicles. Cell Transplant (2013) 22(2):369–79. doi: 10.3727/096368911X582769
69. Mauri C, Bosma A. Immune Regulatory Function of B Cells. Annu Rev Immunol (2012) 30:221–41. doi: 10.1146/annurev-immunol-020711-074934
70. Adamo A, Brandi J, Caligola S, Delfino P, Bazzoni R, Carusone R, et al. Extracellular Vesicles Mediate Mesenchymal Stromal Cell-Dependent Regulation of B Cell PI3K-AKT Signaling Pathway and Actin Cytoskeleton. Front Immunol (2019) 10:446. doi: 10.3389/fimmu.2019.00446
71. Cerwenka A, Swain SL. TGF-Beta1: Immunosuppressant and Viability Factor for T Lymphocytes. Microbes Infect (1999) 1(15):1291–6. doi: 10.1016/s1286-4579(99)00255-5
72. Romani R, Pirisinu I, Calvitti M, Pallotta MT, Gargaro M, Bistoni G, et al. Stem Cells From Human Amniotic Fluid Exert Immunoregulatory Function via Secreted Indoleamine 2,3-Dioxygenase1. J Cell Mol Med (2015) 19(7):1593–605. doi: 10.1111/jcmm.12534
73. Fujii S, Miura Y, Fujishiro A, Shindo T, Shimazu Y, Hirai H, et al. Graft-Versus-Host Disease Amelioration by Human Bone Marrow Mesenchymal Stromal/Stem Cell-Derived Extracellular Vesicles Is Associated With Peripheral Preservation of Naive T Cell Populations. Stem Cells (2018) 36(3):434–45. doi: 10.1002/stem.2759
74. Kerkelä E, Laitinen A, Räbinä J, Valkonen S, Takatalo M, Larjo A, et al. Adenosinergic Immunosuppression by Human Mesenchymal Stromal Cells Requires Co-Operation With T Cells. Stem Cells (2016) 34(3):781–90. doi: 10.1002/stem.2280
75. Blazquez R, Sanchez-Margallo FM, de la Rosa O, Dalemans W, Alvarez V, Tarazona R, et al. Immunomodulatory Potential of Human Adipose Mesenchymal Stem Cells Derived Exosomes on In Vitro Stimulated T Cells. Front Immunol (2014) 5:556. doi: 10.3389/fimmu.2014.00556
76. Mokarizadeh A, Delirezh N, Morshedi A, Mosayebi G, Farshid AA, Mardani K. Microvesicles Derived From Mesenchymal Stem Cells: Potent Organelles for Induction of Tolerogenic Signaling. Immunol Lett (2012) 147(1-2):47–54. doi: 10.1016/j.imlet.2012.06.001
77. Tian J, Zhu Q, Zhang Y, Bian Q, Hong Y, Shen Z, et al. Olfactory Ecto-Mesenchymal Stem Cell-Derived Exosomes Ameliorate Experimental Colitis via Modulating Th1/Th17 and Treg Cell Responses. Front Immunol (2020) 11:598322. doi: 10.3389/fimmu.2020.598322
78. Hai B, Shigemoto-Kuroda T, Zhao Q, Lee RH, Liu F. Inhibitory Effects of iPSC-MSCs and Their Extracellular Vesicles on the Onset of Sialadenitis in a Mouse Model of Sjögren’s Syndrome. Stem Cells Int (2018) 2018:2092315. doi: 10.1155/2018/2092315
79. Zhang B, Yin Y, Lai RC, Tan SS, Choo AB, Lim SK. Mesenchymal Stem Cells Secrete Immunologically Active Exosomes. Stem Cells Dev (2014) 23(11):1233–44. doi: 10.1089/scd.2013.0479
80. Dendrou CA, Fugger L, Friese MA. Immunopathology of Multiple Sclerosis. Nat Rev Immunol (2015) 15(9):545–58. doi: 10.1038/nri3871
81. Martin R. Immunological Aspects of Experimental Allergic Encephalomyelitis and Multiple Sclerosis and Their Application for New Therapeutic Strategies. J Neural Transm Supplementum (1997) 49:53–67. doi: 10.1007/978-3-7091-6844-8_6
82. Xiong XY, Liu L, Yang QW. Functions and Mechanisms of Microglia/Macrophages in Neuroinflammation and Neurogenesis After Stroke. Prog Neurobiol (2016) 142:23–44. doi: 10.1016/j.pneurobio.2016.05.001
83. Subhramanyam CS, Wang C, Hu Q, Dheen ST. Microglia-Mediated Neuroinflammation in Neurodegenerative Diseases. Semin Cell Dev Biol (2019) 94:112–20. doi: 10.1016/j.semcdb.2019.05.004
84. Chu F, Shi M, Zheng C, Shen D, Zhu J, Zheng X, et al. The Roles of Macrophages and Microglia in Multiple Sclerosis and Experimental Autoimmune Encephalomyelitis. J Neuroimmunol (2018) 318:1–7. doi: 10.1016/j.jneuroim.2018.02.015
85. Li Z, Liu F, He X, Yang X, Shan F, Feng J. Exosomes Derived From Mesenchymal Stem Cells Attenuate Inflammation and Demyelination of the Central Nervous System in EAE Rats by Regulating the Polarization of Microglia. Int Immunopharmacol (2019) 67:268–80. doi: 10.1016/j.intimp.2018.12.001
86. Soundara Rajan T, Giacoppo S, Diomede F, Bramanti P, Trubiani O, Mazzon E. Human Periodontal Ligament Stem Cells Secretome From Multiple Sclerosis Patients Suppresses NALP3 Inflammasome Activation in Experimental Autoimmune Encephalomyelitis. Int J Immunopathol Pharmacol (2017) 30(3):238–52. doi: 10.1177/0394632017722332
87. Gholamzad M, Ebtekar M, Ardestani MS, Azimi M, Mahmodi Z, Mousavi MJ, et al. A Comprehensive Review on the Treatment Approaches of Multiple Sclerosis: Currently and in the Future. Inflamm Res (2019) 68(1):25–38. doi: 10.1007/s00011-018-1185-0
88. Pusic AD, Pusic KM, Kraig RP. What are Exosomes and How can They be Used in Multiple Sclerosis Therapy? Expert Rev Neurother (2014) 14(4):353–5. doi: 10.1586/14737175.2014.890893
89. Riazifar M, Mohammadi MR, Pone EJ, Yeri A, Lässer C, Segaliny AI, et al. Stem Cell-Derived Exosomes as Nanotherapeutics for Autoimmune and Neurodegenerative Disorders. ACS Nano (2019) 13(6):6670–88. doi: 10.1021/acsnano.9b01004
90. Tsokos GC. Systemic Lupus Erythematosus. N Engl J Med (2011) 365(22):2110–21. doi: 10.1056/NEJMra1100359
91. Jang E, Jeong M, Kim S, Jang K, Kang BK, Lee DY, et al. Infusion of Human Bone Marrow-Derived Mesenchymal Stem Cells Alleviates Autoimmune Nephritis in a Lupus Model by Suppressing Follicular Helper T-Cell Development. Cell Transplant (2016) 25(1):1–15. doi: 10.3727/096368915x688173
92. Nowling TK, Gilkeson GS. Mechanisms of Tissue Injury in Lupus Nephritis. Arthritis Res Ther (2011) 13(6):250. doi: 10.1186/ar3528
93. Sun L, Akiyama K, Zhang H, Yamaza T, Hou Y, Zhao S, et al. Mesenchymal Stem Cell Transplantation Reverses Multiorgan Dysfunction in Systemic Lupus Erythematosus Mice and Humans. Stem Cells (2009) 27(6):1421–32. doi: 10.1002/stem.68
94. Eirin A, Zhu XY, Puranik AS, Tang H, McGurren KA, van Wijnen AJ, et al. Mesenchymal Stem Cell-Derived Extracellular Vesicles Attenuate Kidney Inflammation. Kidney Int (2017) 92(1):114–24. doi: 10.1016/j.kint.2016.12.023
95. Acharjee S, Ghosh B, Al-Dhubiab BE, Nair AB. Understanding Type 1 Diabetes: Etiology and Models. Can J Diabetes (2013) 37(4):269–76. doi: 10.1016/j.jcjd.2013.05.001
96. van Belle TL, Coppieters KT, von Herrath MG. Type 1 Diabetes: Etiology, Immunology, and Therapeutic Strategies. Physiol Rev (2011) 91(1):79–118. doi: 10.1152/physrev.00003.2010
97. Shi Y, Wang Y, Li Q, Liu K, Hou J, Shao C, et al. Immunoregulatory Mechanisms of Mesenchymal Stem and Stromal Cells in Inflammatory Diseases. Nat Rev Nephrol (2018) 14(8):493–507. doi: 10.1038/s41581-018-0023-5
98. Wu H, Mahato RI. Mesenchymal Stem Cell-Based Therapy for Type 1 Diabetes. Discov Med (2014) 17(93):139–43.
99. Kota DJ, Wiggins LL, Yoon N, Lee RH. TSG-6 Produced by hMSCs Delays the Onset of Autoimmune Diabetes by Suppressing Th1 Development and Enhancing Tolerogenicity. Diabetes (2013) 62(6):2048–58. doi: 10.2337/db12-0931
100. Nakano M, Nagaishi K, Konari N, Saito Y, Chikenji T, Mizue Y, et al. Bone Marrow-Derived Mesenchymal Stem Cells Improve Diabetes-Induced Cognitive Impairment by Exosome Transfer Into Damaged Neurons and Astrocytes. Sci Rep (2016) 6:24805. doi: 10.1038/srep24805
101. Nojehdehi S, Soudi S, Hesampour A, Rasouli S, Soleimani M, Hashemi SM. Immunomodulatory Effects of Mesenchymal Stem Cell-Derived Exosomes on Experimental Type-1 Autoimmune Diabetes. J Cell Biochem (2018) 119(11):9433–43. doi: 10.1002/jcb.27260
102. Krishna U, Ajanaku D, Denniston AK, Gkika T. Uveitis: A Sight-Threatening Disease Which can Impact All Systems. Postgraduate Med J (2017) 93(1106):766–73. doi: 10.1136/postgradmedj-2017-134891
103. Yeh S, Nussenblatt RB, Levy-Clarke GA. Emerging Biologics in the Treatment of Uveitis. Expert Rev Clin Immunol (2007) 3(5):781–96. doi: 10.1586/1744666x.3.5.781
104. Tasso R, Ilengo C, Quarto R, Cancedda R, Caspi RR, Pennesi G. Mesenchymal Stem Cells Induce Functionally Active T-Regulatory Lymphocytes in a Paracrine Fashion and Ameliorate Experimental Autoimmune Uveitis. Invest Ophthalmol Visual Sci (2012) 53(2):786–93. doi: 10.1167/iovs.11-8211
105. Noack M, Miossec P. Selected Cytokine Pathways in Rheumatoid Arthritis. Semin Immunopathol (2017) 39(4):365–83. doi: 10.1007/s00281-017-0619-z
106. Firestein GS. Evolving Concepts of Rheumatoid Arthritis. Nature (2003) 423(6937):356–61. doi: 10.1038/nature01661
107. Francisco LM, Salinas VH, Brown KE, Vanguri VK, Freeman GJ, Kuchroo VK, et al. PD-L1 Regulates the Development, Maintenance, and Function of Induced Regulatory T Cells. J Exp Med (2009) 206(13):3015–29. doi: 10.1084/jem.20090847
108. Garín MI, Chu CC, Golshayan D, Cernuda-Morollón E, Wait R, Lechler RI. Galectin-1: A Key Effector of Regulation Mediated by CD4+CD25+ T Cells. Blood (2007) 109(5):2058–65. doi: 10.1182/blood-2006-04-016451
109. Bianco GA, Toscano MA, Ilarregui JM, Rabinovich GA. Impact of Protein-Glycan Interactions in the Regulation of Autoimmunity and Chronic Inflammation. Autoimmun Rev (2006) 5(5):349–56. doi: 10.1016/j.autrev.2006.02.003
110. Rabinovich GA, Daly G, Dreja H, Tailor H, Riera CM, Hirabayashi J, et al. Recombinant Galectin-1 and its Genetic Delivery Suppress Collagen-Induced Arthritis via T Cell Apoptosis. J Exp Med (1999) 190(3):385–98. doi: 10.1084/jem.190.3.385
111. Liu H, Chen Y, Yin G, Xie Q. Therapeutic Prospects of MicroRNAs Carried by Mesenchymal Stem Cells-Derived Extracellular Vesicles in Autoimmune Diseases. Life Sci (2021) 277:119458. doi: 10.1016/j.lfs.2021.119458
112. Chen Z, Wang H, Xia Y, Yan F, Lu Y. Therapeutic Potential of Mesenchymal Cell-Derived miRNA-150-5p-Expressing Exosomes in Rheumatoid Arthritis Mediated by the Modulation of MMP14 and VEGF. J Immunol (Baltimore Md 1950) (2018) 201(8):2472–82. doi: 10.4049/jimmunol.1800304
113. Meng Q, Qiu B. Exosomal MicroRNA-320a Derived From Mesenchymal Stem Cells Regulates Rheumatoid Arthritis Fibroblast-Like Synoviocyte Activation by Suppressing CXCL9 Expression. Front Physiol (2020) 11:441. doi: 10.3389/fphys.2020.00441
114. Zheng J, Zhu L, Iok In I, Chen Y, Jia N, Zhu W. Bone Marrow-Derived Mesenchymal Stem Cells-Secreted Exosomal microRNA-192-5p Delays Inflammatory Response in Rheumatoid Arthritis. Int Immunopharmacol (2020) 78:105985. doi: 10.1016/j.intimp.2019.105985
115. Cosenza S, Toupet K, Maumus M, Luz-Crawford P, Blanc-Brude O, Jorgensen C, et al. Mesenchymal Stem Cells-Derived Exosomes are More Immunosuppressive Than Microparticles in Inflammatory Arthritis. Theranostics (2018) 8(5):1399–410. doi: 10.7150/thno.21072
116. Liu Y, Zou R, Wang Z, Wen C, Zhang F, Lin F. Exosomal KLF3-AS1 From hMSCs Promoted Cartilage Repair and Chondrocyte Proliferation in Osteoarthritis. Biochem J (2018) 475(22):3629–38. doi: 10.1042/bcj20180675
117. Casado JG, Blázquez R, Vela FJ, Álvarez V, Tarazona R, Sánchez-Margallo FM. Mesenchymal Stem Cell-Derived Exosomes: Immunomodulatory Evaluation in an Antigen-Induced Synovitis Porcine Model. Front vet Sci (2017) 4:39. doi: 10.3389/fvets.2017.00039
118. Ng SC, Shi HY, Hamidi N, Underwood FE, Tang W, Benchimol EI, et al. Worldwide Incidence and Prevalence of Inflammatory Bowel Disease in the 21st Century: A Systematic Review of Population-Based Studies. Lancet (London England) (2017) 390(10114):2769–78. doi: 10.1016/s0140-6736(17)32448-0
119. Yang S, Liang X, Song J, Li C, Liu A, Luo Y, et al. A Novel Therapeutic Approach for Inflammatory Bowel Disease by Exosomes Derived From Human Umbilical Cord Mesenchymal Stem Cells to Repair Intestinal Barrier via TSG-6. Stem Cell Res Ther (2021) 12(1):315. doi: 10.1186/s13287-021-02404-8
120. Lamb CA, Kennedy NA, Raine T, Hendy PA, Smith PJ, Limdi JK, et al. British Society of Gastroenterology Consensus Guidelines on the Management of Inflammatory Bowel Disease in Adults. Gut (2019) 68(Suppl 3):s1–106. doi: 10.1136/gutjnl-2019-318484
121. Mao F, Wu Y, Tang X, Kang J, Zhang B, Yan Y, et al. Exosomes Derived From Human Umbilical Cord Mesenchymal Stem Cells Relieve Inflammatory Bowel Disease in Mice. BioMed Res Int (2017) 2017:5356760. doi: 10.1155/2017/5356760
122. Wang G, Yuan J, Cai X, Xu Z, Wang J, Ocansey DKW, et al. HucMSC-Exosomes Carrying miR-326 Inhibit Neddylation to Relieve Inflammatory Bowel Disease in Mice. Clin Trans Med (2020) 10(2):e113. doi: 10.1002/ctm2.113
123. Wu H, Fan H, Shou Z, Xu M, Chen Q, Ai C, et al. Extracellular Vesicles Containing miR-146a Attenuate Experimental Colitis by Targeting TRAF6 and IRAK1. Int Immunopharmacol (2019) 68:204–12. doi: 10.1016/j.intimp.2018.12.043
124. Heidari N, Abbasi-Kenarsari H, Namaki S, Baghaei K, Zali MR, Ghaffari Khaligh S, et al. Adipose-Derived Mesenchymal Stem Cell-Secreted Exosome Alleviates Dextran Sulfate Sodium-Induced Acute Colitis by Treg Cell Induction and Inflammatory Cytokine Reduction. J Cell Physiol (2021) 236(8):5906–20. doi: 10.1002/jcp.30275
125. Liu H, Liang Z, Wang F, Zhou C, Zheng X, Hu T, et al. Exosomes From Mesenchymal Stromal Cells Reduce Murine Colonic Inflammation via a Macrophage-Dependent Mechanism. JCI Insight (2019) 4(24):e131273. doi: 10.1172/jci.insight.131273
126. Mendt M, Kamerkar S, Sugimoto H, McAndrews KM, Wu CC, Gagea M, et al. Generation and Testing of Clinical-Grade Exosomes for Pancreatic Cancer. JCI Insight (2018) 3(8):e99263. doi: 10.1172/jci.insight.99263
127. Zhang K, Zhao X, Chen X, Wei Y, Du W, Wang Y, et al. Enhanced Therapeutic Effects of Mesenchymal Stem Cell-Derived Exosomes With an Injectable Hydrogel for Hindlimb Ischemia Treatment. ACS Appl Mater Interfaces (2018) 10(36):30081–91. doi: 10.1021/acsami.8b08449
Keywords: mesenchymal stem cells, exosomes, immunoregulation, therapy, autoimmune diseases
Citation: Shen Z, Huang W, Liu J, Tian J, Wang S and Rui K (2021) Effects of Mesenchymal Stem Cell-Derived Exosomes on Autoimmune Diseases. Front. Immunol. 12:749192. doi: 10.3389/fimmu.2021.749192
Received: 29 July 2021; Accepted: 13 September 2021;
Published: 27 September 2021.
Edited by:
Yann Lamarre, University of São Paulo, BrazilReviewed by:
Elizabeth Shpall, University of Texas MD Anderson Cancer Center, United StatesSeok-Goo Cho, The Catholic University of Korea, South Korea
Copyright © 2021 Shen, Huang, Liu, Tian, Wang and Rui. This is an open-access article distributed under the terms of the Creative Commons Attribution License (CC BY). The use, distribution or reproduction in other forums is permitted, provided the original author(s) and the copyright owner(s) are credited and that the original publication in this journal is cited, in accordance with accepted academic practice. No use, distribution or reproduction is permitted which does not comply with these terms.
*Correspondence: Ke Rui, ajgyNzg2NDk4OEAxNjMuY29t