- 1Laboratory of Immunobiology of Infections, Institute of Medical Biology, Polish Academy of Sciences, Łódź, Poland
- 2Department of Newborns’ Infectious Diseases, Poznań University of Medical Sciences, Poznań, Poland
- 3Department of Neonatology, Medical University of Gdańsk, Gdańsk, Poland
- 4Department of Perinatology, First Chair of Gynecology and Obstetrics, Medical University of Łódź, Łódź, Poland
- 5Department of Applied Biochemistry, Tokai University, Hiratsuka, Japan
- 6Scottish National Blood Transfusion Service, National Science Laboratory, Edinburgh, Scotland, United Kingdom
- 7Department of Immunology, Fukushima Medical University, Fukushima, Japan
Ficolin-2 is regarded as an important innate immunity factor endowed with both lectin (carbohydrate recognition) qualities and ability to induce complement activation. The aim of this study was to investigate the association of the FCN2 3’-untranslated region (3’UTR) polymorphisms with ficolin-2 expression and perinatal complications in preterm neonates. The sequencing analysis allowed us to identify six 3’UTR polymorphisms with minor allele frequency (MAF) >1%: rs4521835, rs73664188, rs11103564, rs11103565, rs6537958 and rs6537959. Except for rs4521835, all adhered to Hardy-Weinberg expectations. Moreover, rs6537958 and rs6537959 were shown to be in perfect linkage disequilibrium (LD) with nine other genetic polymorphisms: rs7040372, rs7046516, rs747422, rs7847431, rs6537957, rs6537960, rs6537962, rs11462298 and rs7860507 together stretched on a distance of 1242 bp and very high LD with rs11103565. The 3’UTR region was shown to bind nuclear extract proteins. The polymorphisms at rs4521835 and rs73664188 were found to influence serum ficolin-2 concentration significantly. All polymorphisms identified create (together with exon 8 polymorphism, rs7851696) two haplotype blocks. Among 49 diplotypes (D1-D49) created from rs7851696 (G>T), rs4521835 (T>G), rs73664188 (T>C), rs11103564 (T>C), rs11103565 (G>A) and rs6537959 (T>A), twenty two occurred with frequency >1%. Two diplotypes: D13 (GTTTGT/GGTCGT) and D10 (GTTTGT/GGTCGA), were significantly more frequent among preterm neonates with early onset of infection and pneumonia, compared with newborns with no infectious complications (OR 2.69 and 2.81, respectively; both p<0.05). The minor (C) allele at rs73664188 was associated with an increased risk of very low (≤1500 g) birthweight (OR=1.95, p=0.042) but was associated with the opposite effect at rs11103564 (OR=0.11, p=0.005).
Introduction
Ficolin-2 (L-ficolin) is a serum pattern-recognition molecule, which is able to accelerate clearance of microorganisms directly as an opsonin and indirectly by complement activation via the lectin pathway.
Ficolin-2 has been shown to recognise numerous clinically relevant isolates like enteroaggregative Escherichia coli, Aspergillus fumigatus, Mycobacterium tuberculosis, Streptococcus pneumoniae, Staphylococcus aureus and Trypanosma cruzi (1–5). Lipoteichoic acids, peptidoglycan, 1,3-β-glucans or mycobacterial Ag85 complex have been identified as ficolin-2 microbial targets (6–8). Moreover, ficolin-2 was shown to interact with endogenous factors like elastin, complement CR1 receptor, C-reactive protein (CRP) and long pentraxin 3 (9–12) and may be involved in the clearance of late apoptotic/necrotic cells (13). The CRP-ficolin-2 complex, formed under inflammatory conditions, enables a cross-talk between lectin and classical pathways of complement activation that results in enhancement of serum antimicrobial activity (11).
The FCN2 gene contains 8 exons and has been localised to chromosome 9 (9q34) (14). Exon 1 encodes the 5’UTR, the signal peptide (S) and the N-terminal fragment of the mature protein; exons 2 and 3 encode the collagen-like domain (C and C2), exon 4 encodes a linker peptide (L), exons 5-7 encode part of the fibrinogen like (FBG) domain (F1-F3), and exon 8 encodes the rest of the FBG and the 3’UTR (14). Three splicing variants have been identified: the first variant lacks the second exon sequence and has an additional 76 bp sequence extending from 3’UTR; the second variant is generated by the insertion of the fifth intron and also has an additional 3’UTR sequence of 1060 bp; and the third variant is generated by the insertion of both third and fifth introns (14).
The concentration of ficolin-2 in serum is known to depend on polymorphisms in both FCN2 promoter and structural regions. Hummelshoj et al. (15) and Kilpatrick et al. (16) demonstrated significant associations of ficolin-2 levels with promoter polymorphisms at positions -986 (rs3124952, A>G), -602 (rs3124953, G>A), -64 (rs7865453, A>C) and -4 (rs17514136, A>G). Two exon 8 single nucleotide polymorphisms (SNPs) (rs7851696 and rs17549193, at positions +6424 (G>T) and +6359 (C>T), respectively) were also shown to affect ficolin-2 concentration in serum/plasma, due to strong linkage disequilibria (LD) with rs7865453 (-64) and rs17514136 (-4), respectively (17, 18). Those two exon 8 SNPs influence the structure of the fibrinogen-like domain and the affinity of ficolin-2 to its ligands (12, 15). However, marked variations among individuals carrying the same polymorphic variants were reported and genotype-independent factors seem also to influence ficolin-2 serum concentration (16). For example, cord blood serum ficolin-2 level was shown to be associated with gestational age and birthweight (19). Furthermore, Troldborg et al. (20) found significantly lower serum ficolin-2 in female compared with male adult blood donors. In patients suffering from malaria, markedly higher serum ficolin-2 levels were also observed in the acute phase of disease than after treatment (21). Huge intra-genotype differences in ficolin-2 concentrations may lead to the difficulties in data interpretation and identification of ficolin-2 genotype/phenotype disease associations - especially in studies with a restricted number of samples. Therefore, genotyping alone cannot be used to predict serum concentration. However, an influence of other, still undefined polymorphisms on ficolin-2 serum level cannot be excluded.
93% of functional polymorphisms in the genome-wide association study (GWAS) catalogue are located in non-coding regions (22). Such polymorphisms can affect gene splicing, binding of transcription factors and the interaction between transcript and microRNA – posttranscriptional regulators of gene expression. Generally, 11% of human reference SNP are located in the 3’UTR and they are gaining growing attention (23). They were shown for example to have an impact on the susceptibility to bacterial or viral infections (24, 25), periodontitis (26), sepsis and risk of development of multiorgan dysfunction syndrome (MODS) in severe traumatic patients (27). Moreover, they may be associated with cancer (28–30) and pulmonary hypertension (31).
Previously, we have suggested that low ficolin-2 concentration in cord serum may contribute to the adverse consequences of prematurity (19). Since the marked intra-genotype variations in ficolin-2 levels cannot be fully explained with known promoter/exon 8 polymorphisms, we aimed to search for SNPs within FCN2 3’UTR and verify their biological significance using samples from neonates born preterm as a clinical model. In contrast to FCN2 gene 5’UTR and exon 8 SNPs, knowledge about 3’UTR variability and clinical associations is very limited. To our knowledge, this is the first report concerning the FCN2 3’UTR genotype-phenotype relationship.
Materials and Methods
Subjects
Cord blood samples from 504 Polish preterm neonates including 106 extremely/early preterm (born at gestational age <33 weeks, according to the classification of The World Health Organisation, WHO) and 398 moderate/late preterm (gestational age 33-37 weeks) (Table 1) were obtained from the Department of Newborns’ Infectious Diseases (University of Medical Sciences, Poznań, Poland), Department of Neonatology (Medical University of Gdańsk, Poland) and Department of Perinatology (Medical University of Łódź, Poland). 326 babies came from singleton pregnancies, 172 from 97 twin pregnancies (in 22, material from only one sibling was collected) and 6 from 2 triple pregnancies. The study was approved by the corresponding local ethics committees (Bioethics Committee of The Karol Marcinkowski Poznań University of Medical Sciences, Independent Bioethics Committee for Scientific Research at Medical University of Gdańsk, Bioethics Committee of The Medical University of Łódź). Written informed parental consent was obtained. This work conforms to the provisions of the Declaration of Helsinki. Isolated serum (blood taken into tubes with clot activator) was kept at -80°C. DNA (blood taken into tubes with sodium citrate) was isolated using GeneMATRIX Quick Blood Purifaction Kit (EURx Ltd. Gdańsk, Poland), according to the manufacturer’s protocol.
DNA Sequencing of the FCN2 3’UTR Region
The FCN2 3’UTR region was generally amplified in three separate PCRs: reaction A (amplified region on chromosome 9: 134 887 339-134 888 460), reaction B (amplified region on chromosome 9: 134 888 251-134 890 157) and reaction C (amplified region on chromosome 9: 134 889 426-134 890 521). Alternatively, in the case of problems with sequencing of the product of reaction B, the additional reaction D (amplified region on chromosome 9: 134 887 339-134 890 506) was performed. PCR conditions, as well as sequences of primers and expected PCR products lengths are listed in Table 2. The PCR mixtures (in a total volume of 20 µl) consisted of 50 ng of genomic DNA, 1× DreamTaq polymerase reaction buffer (includes 20 mM MgCl2) (Life Technologies, Carlsbad, CA, USA), 1 U DreamTaq polymerase (Life Technologies, USA), 1 mM of each deoxynucleotide, and 0.5 µM of each primer. Reactions were performed using a Veriti™ 96-Well Thermal Cycler (Thermo Fisher Scientific, Waltham, MA, USA). PCR amplicons were analyzed using horizontal 2% agarose gel electrophoresis in a 1 × TAE buffer. The 100 bp Plus DNA size marker (Thermo Fisher Scientific) was used to normalize the size of each PCR product. The PCR products were purified using the EPPiC Fast mixture (A&A Biotechnology, Gdańsk, Poland), according to the manufacturer’s protocol. For sequencing of PCR products, the BrilliantDye™ Terminator (v.3.1) Cycle Sequencing kit (Life Technologies) was used, according to the manufacturer’s protocol. For both A and C reactions – primers A_ F, A_R, C_F and C_R, were used (Table 2), respectively whereas for sequencing of the PCR products of B and D reactions, the forward primer GCCTGACCAGGCTTTTAGAG or reverse primer AGGTGCACACACACACACAC were employed. For the analysis of following SNP: rs4521835, rs73664188 and rs11103564 SNP, the reaction E (amplified region on chromosome 9: 134 887 339-134 888 155) was performed. The sequencing was successful in 97% at rs11103564 and 99% at rs4521835 and rs73664188. PCR products were purified with the use of BigDye XTerminator™ Purification Kit (Thermo Fisher Scientific), according to the manufacturer’s protocol and then sequenced using the 3500xl Genetic Analyzer (Applied Biosystems, San Diego, CA, USA). Sequencing results were analyzed using Chromas software version 2.4.1 (Technelysium, Brisbane, Australia).
Allelic Discrimination Using TaqMan Probes
Three SNPs were analyzed using TaqMan® Assays purchased from Thermo Fisher Scientific: rs6537958 (Assay ID: C_29220561_10), rs6537959 (Assay ID: C_29220562_20) and rs11103565 (Assay ID: C_31552275_10). For all three SNPs, the PCR mixture consisted of 10 µl of 2 × TaqMan™ Genotyping Master Mix (Thermo Fisher Scientific), 1 µl of appropriate 20X TaqMan® Assay, and 10 ng of genomic DNA. The PCR mixture was filled up with a distilled, DNase and RNase free water (Gibco, Waltham, MA, USA) to the final volume of 20 µl. The real-time PCR was performed using the 7900HT Fast Real-Time PCR System (Applied Biosystems) with 96-Well Block Module using standard mode thermal cycling setting under the following conditions: an initial denaturation step at 95°C for 10 min, followed by 40 cycles of denaturation (95°C, 15 s), single annealing and extension step (60°C for 1 minute). Fluorescence signal detection for both dyes was performed after each cycle. For each plate, a no template control (NTC) was included. Allelic discrimination analysis was performed using a SDS 2.3 software (Applied Biosystems).
Determination of the FCN2 Gene Promoter and Exon 8 Polymorphisms
Promoter polymorphisms at positions -986 (rs3124952, A>G) and -602 (rs3124953, G>A) were investigated according to the procedures described by Metzger et al. (32) while those at positions -64 (rs7865453, A>C) and -4 (rs17514136, A>G) as well as exon 8 SNPs (+6359, rs17549193, C>T; +6424, rs7851696, G>T) were determined as described by Szala et al. (33), with minor modifications.
Determination of Ficolin-2 Concentration in Cord Sera
Ficolin-2 in 395 cord blood serum samples was determined in TRIFMA as described by Świerzko et al. (34). Briefly, anti-ficolin-2 mAb (ABS 005-16, BioPorto Diagnostics, Hellerup, Denmark) were employed for 384 HB Optiplate coating, whereas biotinylated mAb (GN4, Hycult Biotech, Uden, The Netherlands) and Eu3+-labelled streptavidin (Perkin Elmer, Boston, MA, USA) were used for protein detection. The inter-assay and intra-assay coefficients of variation (%CV) were 18% and 13% for serum with ficolin-2 concentration of 3500 ng/ml, respectively whereas for serum with ficolin-2 concentration of 4900 ng/ml they equalled 10% and 7%, respectively.
Electrophoretic Mobility Shift Assay
Electrophoretic Mobility Shift Assay was performed using LightShift™ Chemiluminescent EMSA Kit (Thermo Fisher Scientific), according to the manufacturer’s protocol. A 1325 bp DNA PCR product was obtained using F_F and F_R primers (Table 2). The PCR mixtures (in a total volume of 50 µl) consisted of 20 ng of genomic DNA, 1× DreamTaq polymerase reaction buffer (includes 20 mM MgCl2) (Life Technologies), 1 U DreamTaq polymerase (Life Technologies), 800 µM of each deoxynucleotide, and 0.4 µM of each primer. PCR product was labelled with biotin at 3’ end of the probe using the Pierce™ Biotin 3’ End DNA Labeling Kit (Thermo Fisher Scientific), according to the manufacturer’s protocol. Nuclear extract was prepared from human hepatocyte HepG2 cell line using a Nuclear Extract kit (Thermo Fisher Scientific). Four fmol of biotinylated PCR product was incubated (20 min, RT) with or without 3 µl of nuclear extract in the binding buffer supplemented with glycerol (2.5%), MgCl2 (5 mM), Poly (dI·dC) 50 ng/µl, NP-40 (0.05%), 50 mM KCl and 10 mM EDTA. For inhibition experiments, four fmol of biotinylated PCR product was incubated (20 min, RT) with or without 3 µl of nuclear extract, as well as with or without inhibitor (0.4 pmol of unlabelled PCR product). After electrophoresis in native 6% polyacrylamide gel in TBE buffer, DNA was transferred to Amersham Hybond-N+ nylon membrane (Cytiva, Marlborough, MA, USA) and crosslinked at 120 mJ/cm2 using a commercial UV-light crosslinking instrument (auto-crosslinking function). The biotin-labelled DNA was detected by chemiluminescence by placing a membrane in a film cassette and exposure membrane to X-ray for 1 minute.
Statistics
The Hardy-Weinberg equilibrium (HWE) exact test was used for the analysis of the genotype distribution consistency for all polymorphisms. Linkage disequilibrium (LD) and haplotype block analysis were performed by Haploview 4.2 software (http://www.broad.mit.edu/mpg/haploview/). LD analysis was performed for each pair of polymorphisms using D’ and r2, indicating the amount of LD between two genetic loci. A value of 1 suggests no random association of alleles at different loci. Haplotype block identification was performed based on Four Gamete Rule. The PHASE software (http://stephenslab.uchicago.edu/phase/download.html, version 2.1.1.) was used for diplotype reconstruction from genotype data. In silico analysis was performed with SNPinfo (FuncPred) (https://snpinfo.niehs.nih.gov) and RegulomeDB (https://www.regulomedb.org) databases. The statistical significance of the genetic impact on serum levels was tested with Mann-Whitney U test, Kruskal-Wallis ANOVA test and Spearman Rank correlation. The frequencies of genotypes were compared by two-sided Fischer’s exact test (or χ2 when appropriate). The GraphPad Prism 6 software (https://www.graphpad.com) was used for those calculations. P values <0.05 were considered statistically significant. Odds ratio was calculated using online MedCalc software (https://www.medcalc.org).
Results
Identification of Polymorphisms in 3’UTR Region of FCN2 Gene and Their Frequencies in Preterm Neonates
To identify the polymorphic sites in FCN2 3’UTR region, in the preliminary experiment the 3183 bp fragment in 75 consecutive DNA samples was analyzed via Sanger sequencing. Initial screening allowed us to identify fifteen polymorphic sites with MAF>0.1 (Table 3).
Next, the frequency of those genetic variants was determined in the remaining samples of preterm neonates (Table 4). Rs4521835, rs73664188 and rs111103564 were investigated using Sanger sequencing, whereas rs11103565 was investigated by using the TaqMan Allelic Discrimination assay. The agreement between sequencing analysis and TaqMan probes for 89 samples was 91%. Disagreement was observed in three cases, whereas in five cases the SDS 2.3 software was not able to determine the genotype. Eleven polymorphic sites located between 134 888 378 and 134 889 620 bp analyzed in the 75 samples mentioned were shown to be in complete LD. Using the SNPinfo algorithm for the Caucasian population two tag SNPs: rs6537958 (correlated with rs6537957, rs6537960, rs7040372 and rs7847431) and rs6537959 (correlated with rs7046516, rs6537962a and rs7860507) were indicated. The frequencies of rs4521835, rs73664188, rs111103564 as well as rs6537958 and rs6537959 (representing eleven polymorphic sites) in preterm neonates are presented in Table 4. They did not differ from those reported for the Central European (CEU) population. Except for rs4521835, all FCN2 polymorphisms tested adhered to the Hardy-Weinberg expectations (p>0.05) (Table 4).
Linkage Disequilibrium and Haploblock Analysis
Based on four FCN2 promoter, two exon 8 and five 3’UTR polymorphisms, linkage disequilibrium analysis was performed in 504 samples (Figure 1). According to the r2 and D’ parameters, perfect linkage (r2 = 1, D’=1) was detected between two 3’UTR SNPs (rs6537958 and rs6537959). As a result of sequencing analysis of 75 DNA samples, a perfect LD with them was also detected for other nine 3’UTR polymorphisms (rs7040372, rs7046516, rs7847422, rs7847431, rs6537957, rs6537960, rs6537962, rs114662298 and rs7860507) and confirmed in the ensembl database (https://www.ensembl.org/Homo_sapiens/Info/Index) for the CEU population. Very high r2 and D’ values (0.669 and 0.98, respectively) were observed also between rs11103565 and rs6537958 as well as rs11103565 and rs6537959. Low r2 but high D’ values (>0.8) were observed between 3’UTR rs73664188 and promoter rs3124953 (-602), rs7865453 (-64), rs17514136 (-4), exon 8 rs7851696 (+6424) as well as 3’UTR polymorphisms (rs11103564, rs11103565, rs6537958, rs6537959 and presumably nine others mentioned above).
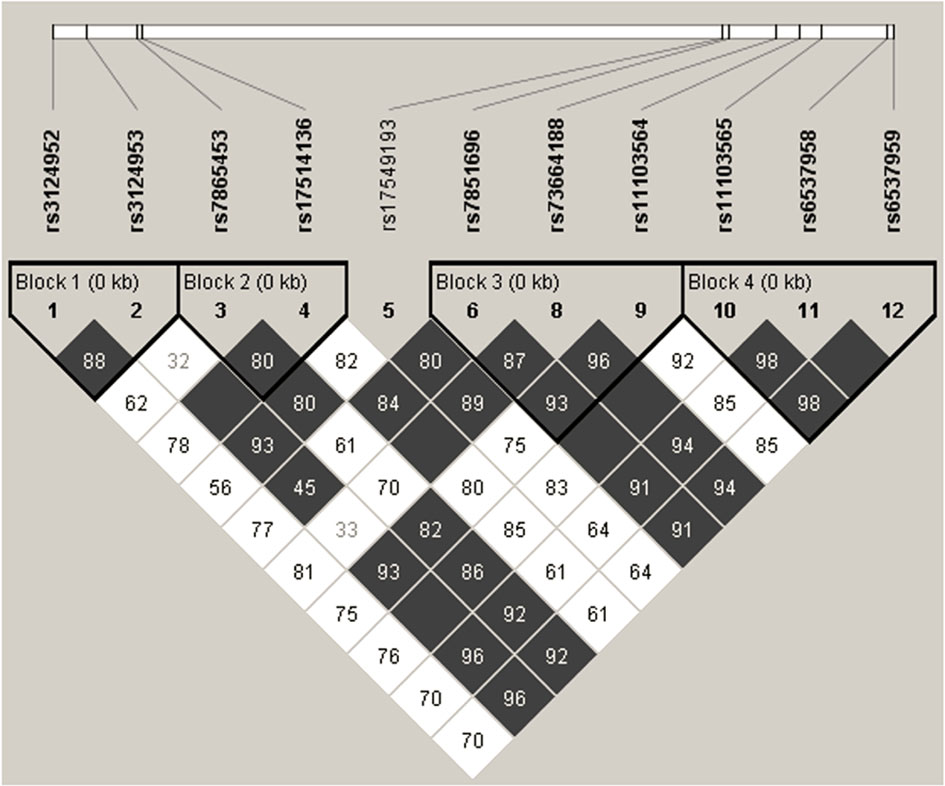
Figure 1 Linkage disequilibrium analysis of promoter [rs3124952 (-986); rs3124953 (-602); rs7865453 (-64); rs17514136 (-4)], exon 8 [rs17549193 (+6359); rs7851696 (+6424)] SNP and polymorphisms identified in this study (rs73664188; rs11103564; rs11103565; rs6537958; rs6537959). The numbers in the grid refer to D’ parameter (presented as percent) of the given pairs of SNPs. Empty grey squares represent perfect LD (D’=1). SNP identifiers are indicated on the abscissas. Bolded triangles shows haplotype blocks identified using Four Gamete Rule test.
Since rs4521835 did not comply with Hardy-Weinberg equilibrium, it was not included in the analysis. However, in the subgroup of early preterm neonates, no deviation from HWE was found for this SNP and Haploview analysis revealed a high LD with rs73664188, rs11103564, rs11103565, rs6537958 and rs6537959. The LD plots for separated extremely/early and moderate/preterm neonates are presented in Supplementary Materials (Figure S1).
Using the Four Gamete Rule approach, four haplotype blocks were identified: block 1 and block 2 - each created by two promoter SNPs [rs3124952 (-986) and rs3124953 (-602) or rs7865453 (-64) and rs17514136 (-4), respectively], block 3 [involving three SNPs: exon 8 rs7851696 (+6424), rs73664188 and rs11103564, both 3’UTR] and block 4 [created by 3’UTR rs11103565, rs6537958, rs6537959 and presumably nine others variants] (Figure 2). As mentioned above, rs4521835 was excluded from analysis. Four haplotypes corresponding to block 3 were identified. Among them GTT (the reference genotype) and GTC had frequency >1%. Within the block 4, three haplotypes were found, including GTA (the reference genotype) and ATA with frequency >1%. The D’ between 3 and 4 blocks was 0.85. The frequency of haploblocks identified in extremely/early and moderate/late preterm neonates is presented in Supplementary Materials (Figure S2). In the case of neonates born before 33 week of gestation, data analysis gave five haploblocks, whereas for those having gestational age ≥33 weeks at birth, only three.
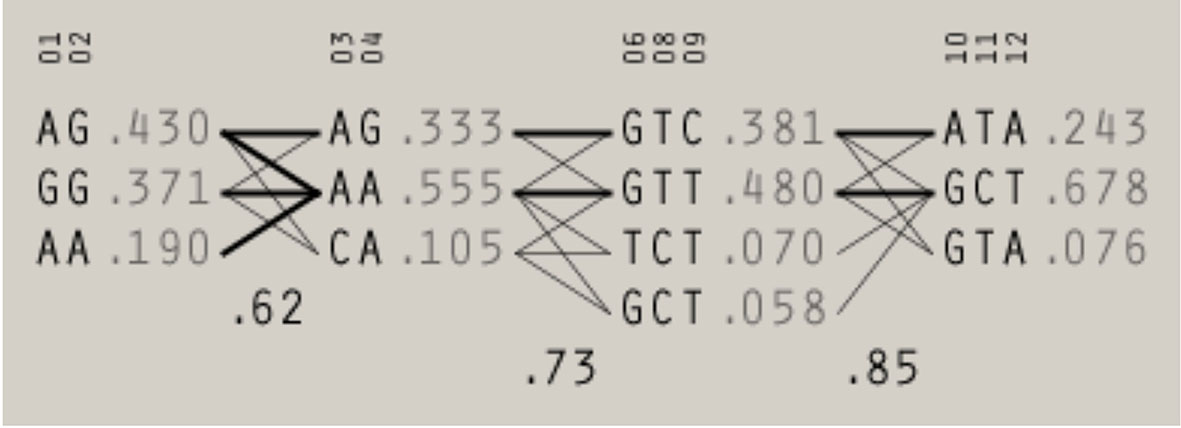
Figure 2 The haplotype blocks identified in preterm neonates using Four Gamete Rule test. The frequency and the level of recombination between blocks are given. 01 - rs3124952 (-986); 02 - rs3124953 (-602); 03 - rs7865453 (-64); 04 - rs17514136 (-4), 06 - rs7851696 (+6424), 08 -rs73664188; 09 - rs11103564; 10 - rs11103565; 11 - rs6537958; 12 - rs6537959. A multiallelic D’ statistic, which indicates the level of recombination between two blocks, is shown in the crossing area.
The Diplotype-Phenotype Association
PHASE software allowed us to identify 49 diplotypes (D1-D49), corresponding to block 3 and block 4 genetic variants: exon 8 rs7851696 (+6424, G>T) and five 3’UTR SNPs: rs4521835 (T>G), rs73664188 (T>C), rs11103564 (T>C), rs11103565 (G>A) and rs6537959 (T>A). Twenty of them, with frequency ≥1% are listed in the Table 5, whereas all identified diplotypes are presented in the Supplementary Materials (Table S1). GTTTGT/GTTTGT (D15) represents the reference diplotype. Diplotypes differing only in SNPs with no greater influence on ficolin-2 level, were grouped into five subgroups (I-V). The sixth subgroup was associated with relatively low ficolin-2 concentration (Table 6 and Figure 3). There were no significant differences in gestational age or birthweight between subgroups. Two diplotypes: D28, (GTTTGT/GGTTGA) and D35 (GGTCGT/GGTCGA) with frequency of 1% were not included in any subgroup.
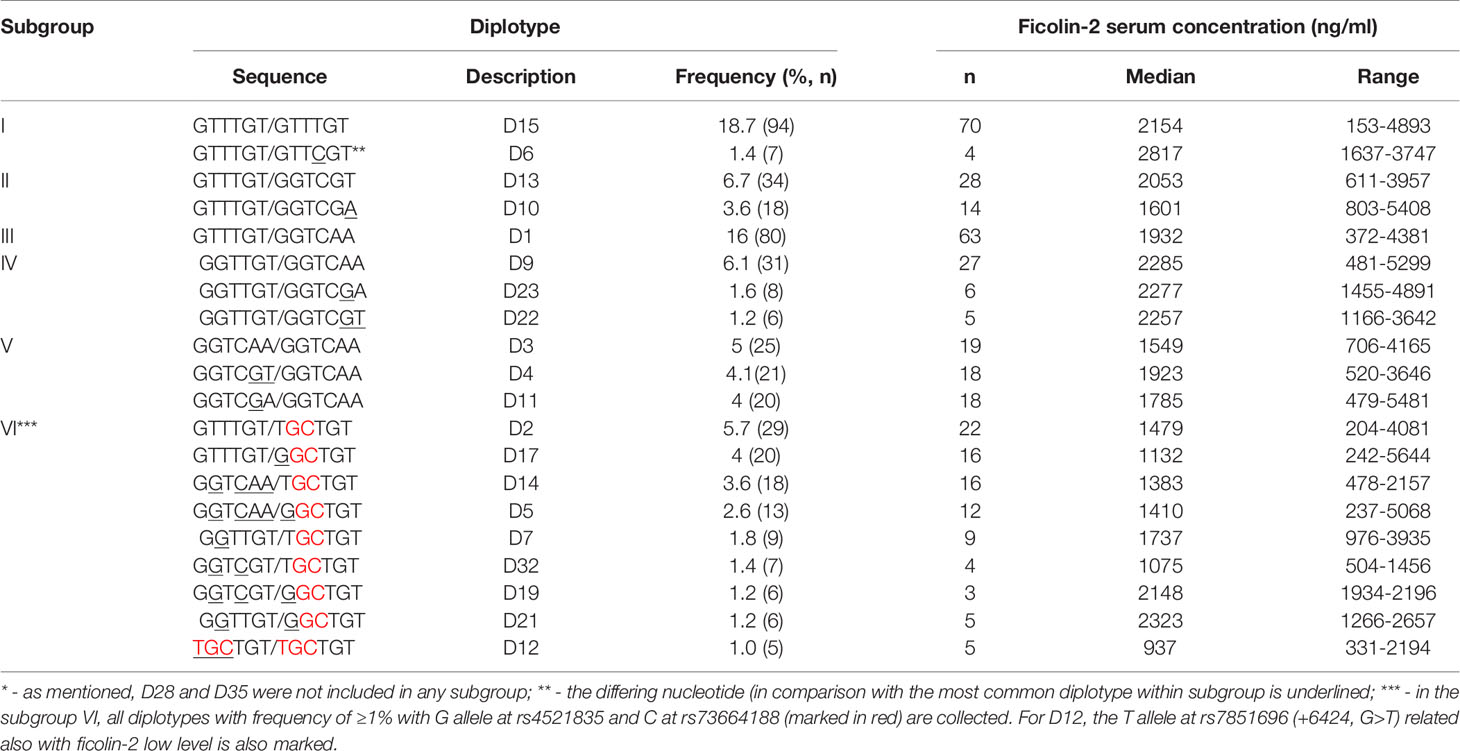
Table 5 The subgroups including identified diplotypes (with the frequency ≥1%*) in preterm neonates.
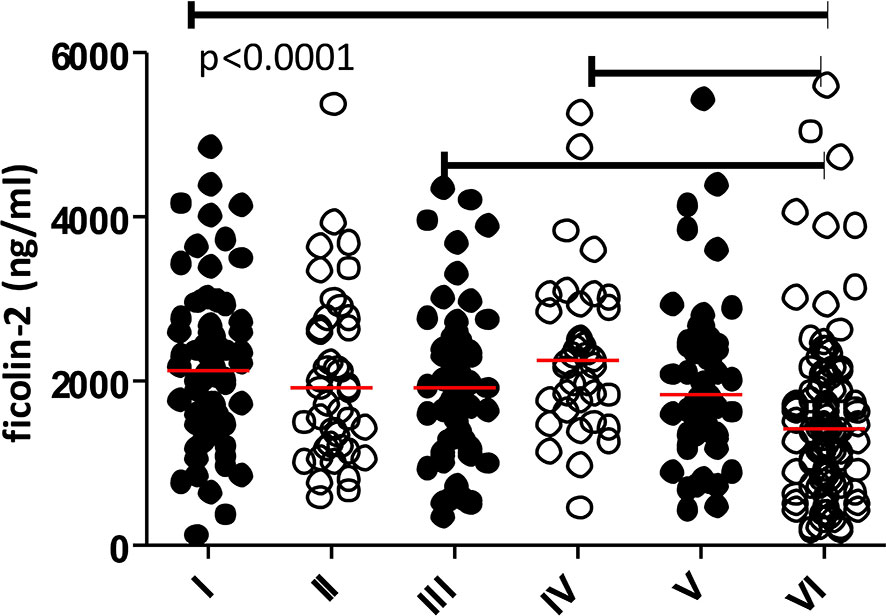
Figure 3 Individual concentrations of ficolin-2 in cord serum samples from preterm neonates in diplotype subgroups. Medians are shown as red bars. Median for the VIth subgroup differs significantly from those for the Ist, IIIrd and IVth ones.
The diplotype association with ficolin-2 concentration in cord serum was found to be markedly influenced by SNPs at rs4521835 and rs73664188 (Figures 4A, B). Genetic variant GC (present in D2, D5, D7, D12, D14, D17, D19, D21 and D32 diplotypes, included in subgroup VI; Table 5) was associated with low ficolin-2 serum concentration. The lowest median was associated with D12, where both haplotypes carry GC variants. Additionally, D12 represents the T allele at rs7851696 (+6424), also associated with low ficolin-2 level in cord serum (data not shown). The trend towards lower protein concentrations was found for the A variant of rs11103565 as well as TA variants at rs6537958 and rs6537959, being in perfect LD (Figure 4F). No association was observed for rs11103564 (Figure 4D). Interestingly, T/G heterozygosity at rs4521835 seems to be related to a higher protein concentration (Figure 4A).
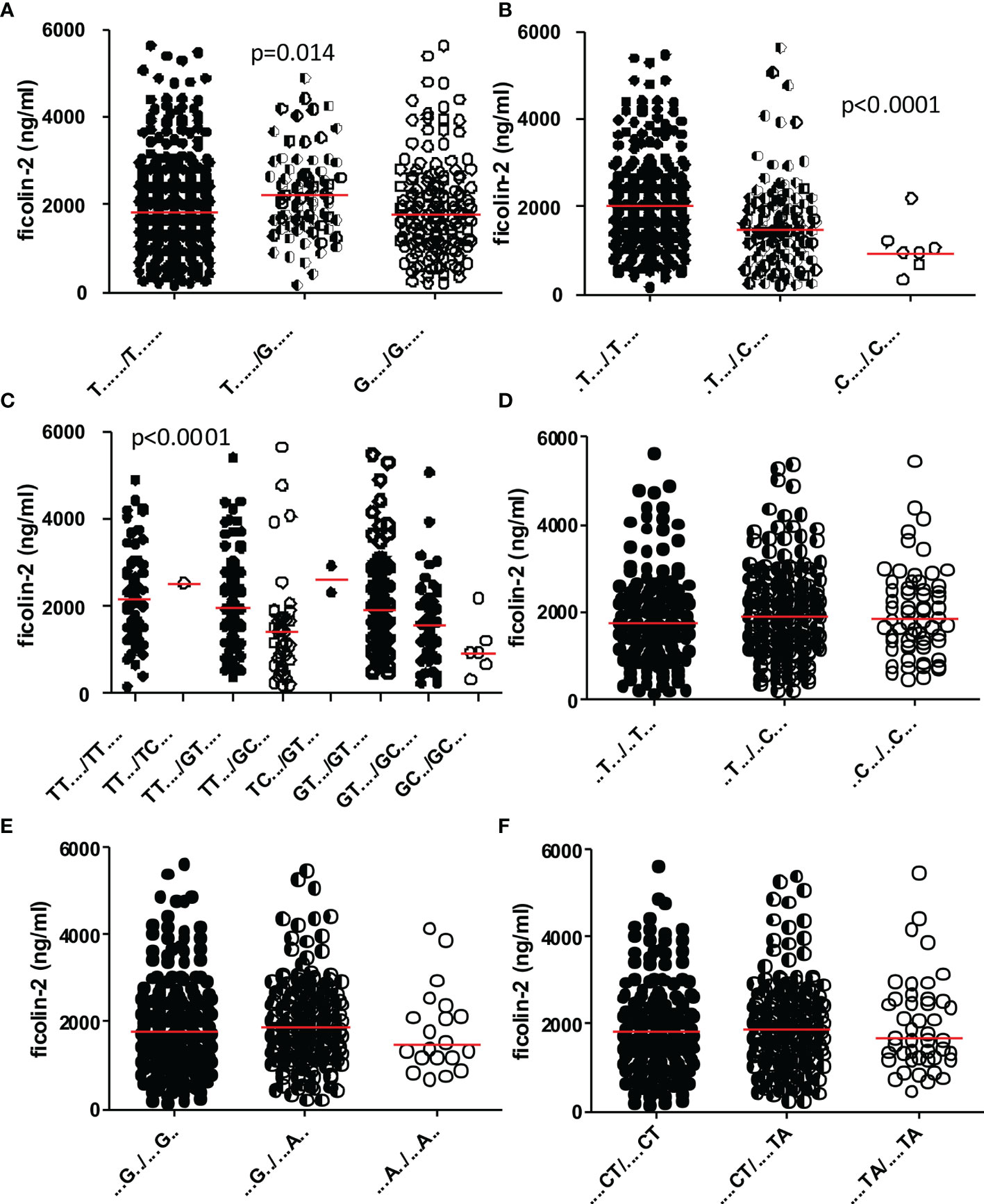
Figure 4 The influence of selected single nucleotide polymorphisms on ficolin-2 cord blood serum concentration in preterm neonates. Genetic variants: rs4521835 (A), rs73664188 (B), rs4521835 and rs73664188 (C), rs11103564 (D), rs11103565 (E), rs6537958 and rs6537959 (F). Medians are shown as red bars. Ficolin-2 concentrations among carriers of various genotypes were compared using Kruskal-Wallis ANOVA.
Besides the relationship of 3’UTR diplotypes with ficolin-2 cord serum concentration described above, the association of genotype with selected complications like early prematurity, low birthweight, susceptibility to perinatal infection, pPROM and RDS was analyzed (Table 6).
It revealed that the probability of carrying one of subgroup II diplotypes, [heterozygous at rs4521835: GTTTGT/GGTCGT (D13) and GTTTGT/GGTCGA (D10)] is significantly higher among neonates with early onset of infection or pneumonia (OR=2.69, p=0.008 and OR=2.81, p=0.026, respectively) than in the group of neonates without such complications (Table 6). Diplotypes representing subgroup V (homozygous for C allele at rs11103564) were less frequent among babies born with birthweight ≤1500 g (OR=0.11 p=0.005) than among neonates born with a higher body mass. In contrast the over-representation of subgroup VI diplotypes with GC variants at rs4521835 and rs73664188 was found among newborns with the same birthweight range (OR=1.95, p=0.042). Moreover, that association seems to be independent of multiple pregnancies, since after excluding twins and triplets, the OR was even higher (2.98, p=0.003). No association of diplotypes with other prematurity-related complications was found.
In Silico Functional Analysis
SNPinfo (FuncPred) software was used for in silico determination of the interactions of genetic variants with microRNA (Table 7). Only rs4521835 was predicted to be a target for microRNA (miR-150, miR-484, miR-618 miR-1226 and miR-1229). The regulomeDB database [designed to assess the probability of particular variant that affects binding of transcription factor (35)] helped to determine the regulatory function of SNPs investigated, with lower score indication of more evidence of functionality (36). Rs11103564 with score of 1f was predicted to be linked to expression of a gene target and to be an eQTL (expression quantitative trait locus) for OLFM1 (olfactomedin 1), whereas rs4521835, rs7847431 and rs11462298 with rank of 3a are less likely to be associated within the functional region. The remaining genetic variants with score 4-5 have minimal evidence of binding. Variants of rs4521835, rs11103565, rs7040372, rs7046516, rs7847431 and rs11462298 may alter significantly transcription factor (TF) binding motifs.
The Impact of the FCN2 Gene 3’UTR Genetic Variants on Protein Binding
Sanger sequencing and LD analysis revealed a perfect linkage among eleven SNPs (rs7040372, rs7046516, rs7847422, rs7847431, rs6537957, rs6537958, rs6537959, rs6537960, rs6537962, rs11462298 and rs7860507) and a very high LD with rs11103565. In order to investigate the biological significance of non-random perfect linkage of twelve SNPs, their impact on nuclear protein binding was tested. Biotin-labelled 1325 bp PCR products of DNA samples from homozygotes for reference/variant alleles as well as from heterozygotes were incubated with nuclear extract. A significant shift in electrophoretic mobility was observed in all cases (Figure 5A). Moreover, the interaction between biotinylated wild-type DNA and nuclear extract proteins was inhibited not only by corresponding unlabelled PCR product but also by DNA of homozygote for variant alleles as well as by unrelated DNA PCR product (Figure 5B).
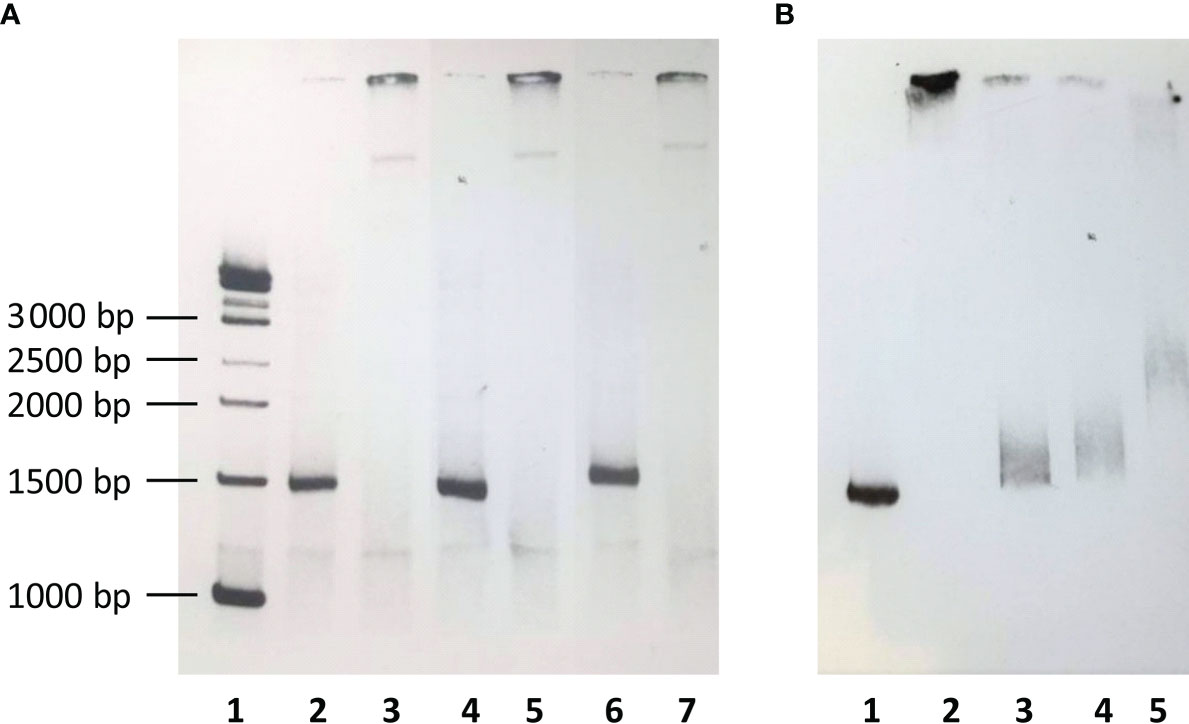
Figure 5 (A) The electrophoretic mobility of biotin-labelled DNA fragment including polymorphic sites at rs11103565, rs7040372, rs7046516, rs7847422, rs7847431, rs6537957, rs6537958, rs6537959, rs6537960, rs6537962, rs11462298 and rs7860507. 1 – biotinylated molecular weight marker; 2, 3 - major allele homozygote; 4, 5 - variant allele homozygote; 6, 7 – heterozygote; lanes 2, 4, 6 – with no nuclear extract; lanes 3, 5, 7 – preincubated with nuclear extract. (B) Inhibition of binding of nuclear extract to aforementioned biotin-labelled DNA fragment from major allele homozygote. 1 – no nuclear extract, no inhibitor; 2 – nuclear extract, no inhibitor; 3 – nuclear extract, unlabelled DNA from major alelle homozygote as inhibitor; 4 - nuclear extract, unlabelled DNA from minor alelle homozygote as inhibitor; 5 - nuclear extract, unlabelled, unrelated DNA as inhibitor (1122 bp PCR product, corresponding to the 134 887 339-134 888 460 region of FCN2 gene.
Discussion
Ficolin-2 has been associated with a variety of disorders (3, 32, 34, 37–42) and proposed as a potential biomarker of certain clinical conditions. Previous studies have demonstrated that ficolin-2 serum concentration/activity is significantly affected by polymorphisms located in both the promoter region and exon 8 (15, 16, 18). However, marked intra-genotype variations in ficolin-2 levels can complicate disease association studies.
The 3’-untranslated region may be involved in regulation of gene expression by controlling mRNA nuclear export, cytoplasmic localization and stability. It may be a target for transcriptional factors and microRNAs. 3’UTR polymorphic variants may create or abolish their binding sites, or modulate enhancer/silencer effects. The influence of 3’UTR variants on concentration of corresponding proteins in the circulation has been demonstrated previously. For example, Ning and Zhang (43) described the impact of two PTX3 gene 3’UTR SNPs on pentraxin-3 level in the Chinese Han population. Doi et al. (44) described the association of two VEGF gene 3’UTR polymorphisms with plasma VEGF levels in Japanese. Furthermore, Zanetti et al. (28) and Świerzko et al. (29) found MBL2 gene 3’UTR polymorphisms to influence mannose-binding lectin (MBL) serum concentration. The polymorphisms in the FCN2 gene 3’UTR were still unexplored and to our knowledge, this is the first report to investigate this issue in depth. Previously, there was only one report describing a trend towards a higher frequency of the G allele at rs4521835 in Polish kidney allograft recipients with delayed graft function (45).
We report here 15 genetic variants with MAF >1% in the FCN2 3’UTR in a cohort of 504 Polish preterm neonates. Their frequencies were similar to the frequencies reported for Caucasian/Central European populations (Table 4). RegulomeDB predicted four SNPs (rs11103564, rs4521835, rs7847431and rs11462298) to lie in the functional location and likely to result in functional consequences (Table 7).
Eleven SNPs (rs7040372, rs7046516, rs747422, rs7847431, rs6537957, rs6537958, s6537959, rs6537960, rs6537962, rs11462298 and rs7860507) were shown to be in complete linkage disequilibrium and together with rs73664188, rs11103564 and rs11103565 as well as with exon 8 rs7851696 (+6424) formed two related haplotype blocks. Among 49 diplotypes created from rs7851696 (G>T), rs4521835 (T>G), rs73664188 (T>C), rs11103564 (T>C), rs11103565 (G>A) and rs6537959 (T>A), twenty two occurred with frequency ≥1% (Table 5). Rs4521835 - due to deviation from HWE - was excluded from haplotype creation by Haploview software, but it was included in diplotypes reconstructed by PHASE software. Interestingly, deviation from HWE for rs4521835 was observed in neonates with gestational age ≥33 weeks only, but not in the neonates born before 33rd week of pregnancy.
Two polymorphisms (rs4521835 and rs73664188) were shown to significantly influence ficolin-2 concentration in cord serum, with the lowest levels observed in the GC haplotype carriers (Table 5 and Figures 3, 4). When the diplotype-serum concentration relationship was analyzed, the lowest ficolin-2 levels were shown in individuals carrying the C allele at rs73664188, the G allele at rs4521835 and the T allele at exon 8 rs7851696 (+6424) at least on one strand (Table 5). Interestingly, the SNPinfo database indicated possible involvement of rs4521835 genetic variants in creating or abolishing miRNA targets sites, which may suggest epigenetic regulation of ficolin-2 synthesis. Moreover, the rs73664188 SNP seems to modify an impact of promoter polymorphisms on ficolin-2 concentration in cord serum (Figure S3).
There have been some reports of significant differences in FCN2 genotypes (involving promoter and/or structural regions) between patients and healthy controls in several clinical contexts (34, 39, 46–49). The results presented here demonstrate an association of subgroup II diplotypes D13 (GTTTGT/GGTCGT) and D10 (GTTTGT/GGTCGA) among preterm neonates with early onset of infection and/or pneumonia (OR in range 2.69-2.81, p<0.05, in comparison with newborns with no infections till hospital discharge). Moreover, in the group of neonates with birthweight ≤1500 g the lower frequency of subgroup V genotypes (both C allele at rs11103564, OR=0.11, p=0.005) but higher probability of subgroup VI diplotypes [associated with low ficolin-2 level resulting from GC variants at rs4521835 and rs73664188 (OR=1.95, p=0.042)] were observed. The assumption that carrying C allele at rs73664188 is associated with low birthweight is supported by an increased odds ratio when only data from singleton pregnancies were analyzed (OR=2.98, p=0.003). Therefore, this variant seems to be a risk factor for one of the adverse effects of prematurity.
Eleven SNPs stretched on the distance of 1242 bp analyzed here were shown to be in perfect LD and with very high LD with rs11103565. They had no greater impact on ficolin-2 concentration in cord sera, however some of them were predicted by RegulomeDB to be associated with transcription factor binding (rs7040372, rs7046516, rs7847431 and rs11462298). We assumed that absolute LD between eleven SNP may be associated with nuclear protein binding. Polymorphism of DNA and RNA 3’ untranslated regions may modulate such interactions. For example, the 3’UTR T variant of the XRCC2 gene at rs3218550 was shown to enhance nuclear protein binding (50). Using a gel shift assay, we tested whether 1325 bp PCR product including twelve SNPs may be involved in protein binding and whether such interaction may be influenced by their genetic variants. Our results indicate that three tested genetic variants were able to bind nuclear extract factors. The results are not easy to interpret since the protein-DNA interaction can be either DNA sequence-nonspecific (protein-sugar phosphate DNA backbone interactions) or DNA sequence-specific (protein-base pair interactions) (51). The inhibition of nuclear extract induced electrophoretic mobility of biotinylated wild type DNA with variant alleles homozygote DNA may suggest non-allele specific binding, however partial inhibition by an unrelated DNA PCR product may indicate partially specific interaction between wild type DNA and nuclear protein(s).
In addition to RegulomeDB, in silico analysis using PROMO software, 31 different transcription factors (TF) were predicted to be able to recognise putative binding sites including 12 SNPs. Five of them were able to bind to major allele variants only, and seven to minor variants only, whereas fourteen were predicted to be reactive independently of genotype. For example p53 was shown to possibly interact with both variants at rs7046516, rs7847422 and rs6537957. In contrast to other TFs, p53 DNA targets are not defined by a particular consensus sequence, but it is able to bind various DNA sequences and DNA targets defined by their secondary structures and the majority of p53 target structures are out with the promoter region [reviewed by Brazda and Fojta (52)]. Moreover, p53 was shown to interact with other TFs, like YY-1, which was predicted to interact at rs7040372, rs7847422 and rs6537962. This, among other things, makes the elucidation of the molecular basis of the interactions of the nuclear extract factors with the FCN2 3’UTR region more difficult.
To summarize, sequencing of the 3’UTR of the FCN2 gene in a relatively large cohort of preterm babies and a multiway in silico analysis allowed us to find potential associations of certain polymorphic variants of that region with common adverse effects of prematurity. Furthermore, some polymorphisms were demonstrated to affect ficolin-2 concentration in cord serum, although the associations mentioned may reflect linkage disequilibrium with promoter/coding region SNP. The 3’UTR polymorphism can be also involved in nuclear protein binding.
Data Availability Statement
The raw data supporting the conclusions of this article will be made available by the authors, without undue reservation.
Ethics Statement
The studies involving human participants were reviewed and approved by Bioethics Committee of The Karol Marcinkowski Poznań University of Medical Sciences; Independent Bioethics Committee for Scientific Research at Medical University of Gdańsk; Bioethics Committee of The Medical University of Łódź. Written informed consent to participate in this study was provided by the participants’ legal guardian/next of kin.
Author Contributions
AŚ coordinated project realisation, designed the study, planned and supervised experimental work, determined ficolin-2 concentrations, sequenced FCN2 gene 3’UTR and determined SNP at -986/-602, analyzed experimental data. DJ and MMi designed the procedure for FCN2 gene 3’UTR sequencing and produced corresponding data. DJ determined SNP using TaqMan probes; analyzed haplotypes and preformed EMSA experiments. GG determined SNP at -64/-4/+6359/+6424 and performed EMSA experiments. AS-P analyzed diplotypes. KC, PK, MK-B, and KS were responsible for patients’ qualification, taking and collecting samples as well as collecting clinical data. JM, ID-P, and JK supervised qualification and recruitment of patients and analysis of clinical data. MMa and HS provided anti-ficolin-2 mAbs for initial experiments, contributed to modification of the procedure and discussed corresponding data. DK contributed to the study design and data analysis. MC contributed to the study design, supervision of experimental work, analyzed haplotypes and performed statistical analysis. Draft manuscript was written by AŚ, DJ, and MC, and revised by DK and MC. All authors contributed to the article and approved the submitted version.
Funding
This work was funded by National Science Centre, Poland, grant 2015/17/B/NZ6/04250.
Conflict of Interest
The authors declare that the research was conducted in the absence of any commercial or financial relationships that could be construed as a potential conflict of interest.
Publisher’s Note
All claims expressed in this article are solely those of the authors and do not necessarily represent those of their affiliated organizations, or those of the publisher, the editors and the reviewers. Any product that may be evaluated in this article, or claim that may be made by its manufacturer, is not guaranteed or endorsed by the publisher.
Supplementary Material
The Supplementary Material for this article can be found online at: https://www.frontiersin.org/articles/10.3389/fimmu.2021.741140/full#supplementary-material
References
1. Sorensen CA, Rosbjerg A, Jensen HB, Krogfelt KA, Garred P. The Lectin Complement Pathway is Involved in Protection Against Enteroaggregative Escherichia Coli Infection. Front Immunol (2018) 9:1153. doi: 10.3389/fimmu.2018.01153
2. Bidula S, Sexton DW, Abdolrasouli A, Shah A, Reed A, Armstrong JD, et al. The Serum Opsonin L-Ficolin is Detected in Lungs of Human Transplant Recipients Following Fungal Infections and Modulates Inflammation and Killing of Aspergillus Fumigatus. J Infect Dis (2015) 212:234–46. doi: 10.1093/infdis/jiv027
3. Luo F, Sun X, Wang Y, Wang Q, Wu Y, Pan Q, et al. Ficolin-2 Defends Against Virulent Mycobacteria Tuberculosis Infection In Vivo, and Its Insufficiency is Associated With Infection in Humans. PloS One (2013) 8(9):e73859. doi: 10.1371/journal.pone.0073859
4. Sosoniuk E, Vallejos G, Kenawy H, Gaboriaud C, Thielens N, Fujita T, et al. Trypanosoma Cruzi Calreticulin Inhibits the Complement Lectin Pathway Activation by Direct Interaction With L-Ficolin. Mol Immunol (2014) 60:80–5. doi: 10.1016/j.molimm.2014.03.014
5. Krarup A, Sorensen UB, Matsushita M, Jensenius JC, Thiel S. Effect of Capsulation of Opportunistic Pathogenic Bacteria on Binding of the Pattern Recognition Molecules Mannan-Binding Lectin, L-Ficolin, and H-Ficolin. Infect Immun (2005) 73:1052–60. doi: 10.1128/IAI.73.2.1052-1060.2005
6. Ma YG, Cho MY, Zhao M, Park JW, Matsushita M, Fujita T, et al. Human Mannose-Binding Lectin and L-Ficolin Function as Specific Pattern Recognition Proteins in the Lectin Activation Pathway of Complement. J Biol Chem (2004) 279:25307–12. doi: 10.1074/jbc.M400701200
7. Lynch NJ, Roscher S, Hartung T, Morath S, Matsushita M, Maennel DN, et al. L-Ficolin Specifically Binds to Lipoteichoic Acid, a Cell Wall Constituent of Gram-Positive Bacteria, and Activates the Lectin Pathway of Complement. J Immunol (2004) 72:1198–202. doi: 10.4049/jimmunol.172.2.1198
8. Świerzko AS, Bartłomiejczyk MA, Brzostek A, Łukasiewicz J, Michalski M, Dziadek J, et al. Mycobacterial Antigen 85 Complex (Ag85) as a Target for Ficolins and Mannose-Binding Lectin. Int J Med Microbiol (2016) 306:212–21. doi: 10.1016/j.ijmm.2016.04.004
9. Harumiya S, Takeda K, Suquira T, Fukumoto Y, Tachikawa H, Miyazono K, et al. Characterization of Ficolins as Novel Elastin-Binding Proteins and Molecular Cloning of Human Ficolin-1. J Biochem (1996) 120:745–51. doi: 10.1093/oxfordjournals.jbchem.a021474
10. Jacquet M, Lacroix M, Ancelet S, Gout E, Gaboriaud Ch, Thielens NM, et al. Deciphering Complement Receptor Type 1 Interactions With Recognition Proteins of the Lectin Pathway. J Immunol (2013) 190:3721–31. doi: 10.4049/jimmunol.1202451
11. Zhang J, Kh J, Lu J, Thiel S, Leong BSH, Sethi S, et al. Local Inflammation Induces Complement Crosstalk Which Amplifies the Antimicrobial Response. PloS Pathog (2009) 5:e1000282. doi: 10.1371/journal.ppat.1000282
12. Ma YJ, Doni A, Hummeshoj T, Honore C, Bastone A, Mantovani A, et al. Synergy Between Ficolin-2 and Pentraxin 3 Boots Innate Immune Recognition and Complement Deposition. J Biol Chem (2009) 284:28263–75. doi: 10.1074/jbc.M109.009225
13. Jensen ML, Honore C, Hummeshoj T, Hansen BE, Madsen HO, Garred P. Ficolin-2 Recognizes DNA and Participates in the Clearance If Dying Host Cells. Mol Immunol (2007) 44:856–65. doi: 10.1016/j.molimm.2006.04.002
14. Endo Y, Sato Y, Matsushita M, Fujita T. Cloning and Characterization of the Human Lectin P35 Gene and Its Related Gene. Genomics (1996) 36:515–21. doi: 10.1006/geno.1996.0497
15. Hummelshoj T, Munthe-Fog L, Madsen HO, Fujita T, Matsushita M, Garred P. Polymorphisms in the FCN2 Gene Determine Serum Variation and Function of Ficolin-2. Hum Mol Genet (2005) 14:1651–8. doi: 10.1093/hmg/ddi173
16. Kilpatrick DC, Świerzko A, Matsushita M, Domżalska-Popadiuk I, Borkowska-Kłos M, Szczapa J, et al. The Relationship Between FCN2 Geneotypes and Serum Ficolin-2 (L-Ficolin) Protein Concentrations From a Large Cohort of Neonates. Hum Immunol (2013) 74:867–71. doi: 10.1016/j.humimm.2013.04.011
17. Munthe-Fog L, Hummelshoj T, Hansen BE, Koch C, Madsen HO, Skjodt K, et al. The Impact of FCN2 Polymorphisms and Haplotypes on the Ficolin-2 Serum Levels. Scand J Immunol (2007) 65:383–92. doi: 10.1111/j.1365-3083.2007.01915.x
18. Cedzynski M, Nuytinck L, Atkinson AP, Swierzko A, Zeman K, Szemraj J, et al. Extremes of L-Ficolin Concentration in Children With Recurrent Infections Are Associated With Single Nucleotide Polymorphisms in the FCN2 Gene. Clin Exp Immunol (2007) 50:99–104. doi: 10.1111/j.1365-2249.2007.03471.x
19. Świerzko A, Atkinson APM, Cedzyński M, MacDonald SL, Szala A, Domżalska-Popadiuk I, et al. Two Factors of the Lectin Pathway of Complement, L-Ficolin and Mannan-Binding Lectin, and Their Associations With Prematurity, Low Birthweight and Infections in a Large Cohort of Polish Neonates. Mol Immunol (2009) 46:551–8. doi: 10.1016/j.molimm.2008.07.025
20. Troldborg A, Hansen A, Hansen SKW, Jensenius JC, Stengaard-Pedersen K, Thiel S. Lectin Complement Pathway Proteins in Healthy Individuals. Clin Exp Immunol (2017) 188:138–47. doi: 10.1111/cei.12909
21. Faik I, Segun I, Oyedeji A, Zulkarnain I, de Messias-Reason IJ, Lell B, et al. Ficolin-2 Levels and Genetic Polymorphisms of FCN2 in Malaria. Hum Immunol (2011) 72:74–9. doi: 10.1016/j.humimm.2010.10.003
22. Tak YG, Farnham PJ. Making Sense of GWAS: Using Epigenomics and Genome Engineering to Understand the Functional Relevance of SNPs in Noncoding Regions of the Human Genome. Epigenet Chromatin (2015) 8:57. doi: 10.1186/s13072-015-0050-4
23. Moszyńska A, Gebert M, Collawn JF, Bartoszewski R. SNP in microRNA Target Sites and Their Potential Role in Human Disease. Open Biol (2017) 7:170019. doi: 10.1098/rsob.170019
24. Liu Y, Zhao E, Zhu L, Zhang D, Wang Z. 3’UTR Polymorphisms in NRAMP1 Are Associated With the Susceptibility to Pulmonary Tuberculosis: A MOOSE-Compliant Meta-Analysis. Med (Baltimore) (2019) 98:e15955. doi: 10.1097/MD.0000000000015955
25. Sy BT, Hoan NX, Tong HV, Meyer CG, Toan NL, Song LH, et al. Genetic Variants of Interferon Regulatory Factor 5 Associated With Chronic Hepatitis B Infection. World J Gastoenterol (2018) 24:248–56. doi: 10.3748/wjg.v24.i2.248
26. Fukusaki T, Ohara N, Hara Y, Yoshimura A, Yoshiura K. Evidence for Association Between a Toll-Like Receptor 4 Gene Polymorphism and Moderate/Severe Periodontitis in the Japanese Population. J Periodontal Res (2007) 42:541–5. doi: 10.1111/j.1600-0765.2007.00979.x
27. Gan L, Hu C, Deng Z, Lu H, Sun J, Peng G, et al. Rs1982809 is a Functional Biomarker for the Prognosis of Severe Post-Traumatic Sepsis and MODs. Exp Biol Med (Maywood) (2019) 244:1438–45. doi: 10.1177/1535370219880490
28. Zanetti KA, Haznadar M, Welsh JA, Robles AI, Ryan BM, McClary AC, et al. 3’-UTR and Functional Secretor Haplotypes in Mannose-Binding Lectin-2 Are Associated With Increased Colon Cancer Risk in African Americans. Cancer Res (2012) 72:1467–77. doi: 10.1158/0008-5472.CAN-11-3073
29. Świerzko AS, Michalski M, Sokołowska A, Nowicki M, Eppa Ł, Szala-Poździej A, et al. The Role of Complement Activating Collectins and Associated Serine Proteases in Patients With Hematological Malignancies, Receiving High-Dose Chemotherapy, and Autologous Hematopoietic Stem Cell Transplantations (Auto-HSCT). Front Immunol (2018) 9:2153. doi: 10.3389/fimmu.2018.02153
30. Yan S, Sun R, Wu S, Jin T, Zhang S, Niu F, et al. Single Nucleotide Polymorphism in the 3’untranslated Region of LPP Is a Risk Factor for Lung Cancer: A Case-Control Study. BMC Cancer (2019) 19:35. doi: 10.1186/s12885-018-5241-5
31. Li D, Sun Y, Kong X, Luan C, Yu Y, Chen F, et al. Association Between a Single Nucleotide Polymorphism in the 3’UTR of ARGHEF18 and the Risk of Nonidiopathic Pulmonary Arterial Hyportension in Chinese Population. Dis Markers (2018) 2018:2461845. doi: 10.1155/2018/2461845
32. Metzger ML, Michelfelder I, Goldacker S, Mekaoui K, Liztman J, Guzman D, et al. Low Ficolin-2 Levels in Common Variable Immunodeficiency Patients With Bronchiectasis. Clin Exp Immunol (2015) 179:256–64. doi: 10.1111/cei.12459
33. Szala A, Świerzko A, Cedzyński M. Cost-Effective Procedures for Genotyping of Human FCN2 Gene Single Nucleotide Polymorphisms. Immunogenetics (2013) 65:439–46. doi: 10.1007/s00251-013-0696-7
34. Świerzko AS, Michalski M, Sokołowska A, Nowicki M, Szala-Poździej A, Eppa Ł, et al. Associations of Ficolins With Haematological Malignancies in Patients Receiving High-Dose Chemotherapy and Autologous Hematopoietic Stem Cell Transplantations. Front Immunol (2020) 10:3097. doi: 10.3389/fimmu.2019.03097
35. Maqbool SN, Nazeer HS, Rafiq M, Javed A, Hanif R. Bridging the Gap by Discrerning SNPs in Linkage Disequilibrium and Their Role in Breast Cancer. Gene (2018) 679:44–56. doi: 10.1016/j.gene.2018.06.102
36. Boyle AP, Hong EL, Hariharan M, Cheng Y, Schaub MA, Kasowski M, et al. Annotation of Functional Variation in Personal Genomes Using RegulomeDB. Genome Res (2012) 22:1790–7. doi: 10.1101/gr.137323.112
37. Cedzynski M, Atkinson AP, Swierzko A, MacDonald SL, Szala A, Zeman K, et al. L-Ficolin (Ficolin-2) Insufficiency Is Associated With Combined Allergic and Infectious Respiratory Disease in Children. Mol Immunol (2009) 47:415–9. doi: 10.1016/j.molimm.2009.08.028
38. Kilpatrick DC, Chalmers JD, MacDonald SL, Murray M, Mohammed A, Hart SP, et al. Stable Bronchiectasis Is Associated With Low Serum L-Ficolin Concentrations. Clin Respir J (2009) 3:29–33. doi: 10.1111/j.1752-699X.2008.00105.x
39. de Messias-Reason I, Kremsner PG, Kun JFJ. Functional Haplotypes That Produce Normal Ficolin-2 Levels Protect Against Clinical Leprosy. J Infect Dis (2009) 199:801–4. doi: 10.1086/597070
40. Szala A, Sawicki S, Świerzko AS, Szemraj J, Śniadecki M, Michalski M, et al. Ficolin-2 and Ficolin-3 in Woman With Malignant and Benign Ovarian Tumours. Cancer Immunol Immunother (2013) 62:1411–9. doi: 10.1007/s00262-013-1445-3
41. Kasperkiewicz K, Eppa Ł, Świerzko AS, Bartłomiejczyk MA, Żuber ZM, Siniewicz-Luzeńczyk K, et al. Lectin Pathway Factors in Patients Suffering From Juvenile Idiopathic Arthritis. Immunol Cell Biol (2017) 95:666–75. doi: 10.1038/icb.2017.31
42. Sokołowska A, Świerzko AS, Gajek G, Gołos A, Michalski M, Nowicki M, et al. Associations of Ficolins and Mannose-Binding Lectin With Acute Myeloid Leukemia in Adults. Sci Rep (2020) 10:10561. doi: 10.1038/s41598-020-67516-2
43. Ning X, Zhang W. PTX3 Gene 3’UTR Polymorphism and its Interaction With Environmental Factors With the Risk of Preeclampsia in a Chinese Han Population. Med (Baltimore) (2020) 99:e18740. doi: 10.1097/MD.0000000000018740
44. Doi K, Noiri E, Nakao A, Fujita T, Kobayashi S, Tokunaga K. Functional Polymorphisms in the Vascular Endothelial Growth Factor Gene are Associated With Development of End-Stage Renal Disease in Males. Am Soc Nephrol (2006) 17:823–30. doi: 10.1681/ASN.2005010094
45. Dabrowska-Zamojcin E, Czerewaty M, Malinowski D, Tarnowski M, Sluczanowska-Glabowska S, Domanski L, et al. Ficolin-2 Gene Rs785196 Polymorphism Is Associated With Delayed Graft Function and Acute Rejection in Kidney Allograft Recipients. Arch Immunol Ther Exp (2018) 66:65–72. doi: 10.1007/s00005-017-0475-5
46. Giang NT, Tong HV, Nghia TH, Hung HV, Anh DT, Nam LV, et al. Association of FCN2 Polymorphisms and Ficolin-2 Levels With Dengue Fever in Vietnamese Patients. Int J Infect Dis (2020) 95:253–61. doi: 10.1016/j.ijid.2020.02.029
47. Van Kempen H, Meijvis S, Endeman H, Vlaminckx B, Meek B, de Jong B, et al. Mannose-Binding Lectin and L-Ficolin Polymorphisms in Patients With Community-Acquired Pneumonia Caused by Intracellular Pathogens. Immunology (2017) 151:81–8. doi: 10.1111/imm.12705
48. Assaf A, Van Hoang T, Faik I, Aebischer T, Kremsner PG, Kun JFJ, et al. Genetic Evidence of Functional Ficolin-2 Haplotypes as Susceptibility Factor in Cutaneous Leishmaniasis. PloS One (2012) 7(3):e34113. doi: 10.1371/journal.pone.0034113
49. Bronkhorst MWGA, Lomax MAZ, Vossen RHAM, Bakker J, Patka P, van Lieshout EMM. Risk of Infection and Sepsis in Severely Injured Patients Related to Single Nucleotide Polymorphisms in the Lectin Pathway. Br J Surg (2013) 100:1818–26. doi: 10.1002/bjs.9319
50. Sirisena ND, Samaranayake N, Dissanayake VHW. Electrophoretic Mobility Shift Assays Implicate XRCC2: Rs3218559c>T as a Potential Low-Penetrant Susceptibility Allele for Sporadic Breast Cancer. BMC Res Notes (2019) 12:476. doi: 10.1186/s13104-019-4512-9
51. Batabyal S, Choudhury S, Sao D, Mondol T, Pal SK. Dynamical Perspective of Protein-DNA Interaction. Biomol Concepts (2014) 5:21–43. doi: 10.1515/bmc-2013-0037
Keywords: 3’UTR, ficolin-2, FCN2, newborn, prematurity
Citation: Świerzko AS, Jarych D, Gajek G, Chojnacka K, Kobiela P, Kufelnicka-Babout M, Michalski M, Sobczuk K, Szala-Poździej A, Matsushita M, Mazela J, Domżalska-Popadiuk I, Kilpatrick DC, Kalinka J, Sekine H and Cedzyński M (2021) Polymorphisms of the FCN2 Gene 3’UTR Region and Their Clinical Associations in Preterm Newborns. Front. Immunol. 12:741140. doi: 10.3389/fimmu.2021.741140
Received: 27 July 2021; Accepted: 12 October 2021;
Published: 28 October 2021.
Edited by:
Attila Mócsai, Semmelweis University, HungaryReviewed by:
Jiayi Qu, University of California, Davis, United StatesYing Jie Ma, Copenhagen University Hospital, Denmark
Copyright © 2021 Świerzko, Jarych, Gajek, Chojnacka, Kobiela, Kufelnicka-Babout, Michalski, Sobczuk, Szala-Poździej, Matsushita, Mazela, Domżalska-Popadiuk, Kilpatrick, Kalinka, Sekine and Cedzyński. This is an open-access article distributed under the terms of the Creative Commons Attribution License (CC BY). The use, distribution or reproduction in other forums is permitted, provided the original author(s) and the copyright owner(s) are credited and that the original publication in this journal is cited, in accordance with accepted academic practice. No use, distribution or reproduction is permitted which does not comply with these terms.
*Correspondence: Maciej Cedzyński, bWNlZHp5bnNraUBjYm0ucGFuLnBs
†These authors have contributed equally to this work and share first authorship