- 1Department of Rheumatology and Clinical Immunology, Faculty of Medicine, School of Health Sciences, University of Thessaly, Larissa, Greece
- 2Department of Neurology, Faculty of Medicine, School of Health Sciences, University of Thessaly, Larissa, Greece
- 3Laboratory of Microbiology, Faculty of Medicine, Democritus University of Thrace, Alexandroupolis, Greece
- 4Medical School, University of Cyprus, Nicosia, Cyprus
Introduction: Limited data from clinical trials in multiple sclerosis (MS) reported that minocycline, a widely used antibiotic belonging to the family of tetracyclines (TCs), exerts a beneficial short-lived clinical effect A similar anti-inflammatory effect of minocycline attributed to a deviation from Th1 to Th2 immune response has been reported in experimental models of MS. Whether such an immunomodulatory mechanism is operated in the human disease remains largely unknown.
Aim: To assess the in vitro immunomodulatory effect of tetracyclines, and in particular minocycline and doxycycline, in naïve and treated patients with MS.
Material and Methods: Peripheral blood mononuclear cells from 45 individuals (35 MS patients, amongst which 15 naïve patients and 10 healthy controls, HCs) were cultured with minocycline or doxycycline and conventional stimulants (PMA/Ionomycin or IL-12/IL-18). IFN-γ and IL-17 producing T-, NK- and NKT cells were assessed by flow cytometry. The effect of TCs on cell viability and apoptosis was further assessed by flow cytometry with Annexin V staining.
Results: Both tetracyclines significantly decreased, in a dose dependent manner, IFN-γ production in NKT and CD4+ T lymphocytes from MS patients (naïve or treated) stimulated with IL-12/IL-18 but did not decrease IFN-γ producing CD8+ T cells from naive MS or treated RRMS patients. They also decreased IL-17+ T and NKT cells following PMA and Ionomycin-stimulation. Tetracyclines did not affect the viability of cell subsets.
Conclusion: Tetracyclines can in vitro suppress IFN-γ and IL-17- producing cells from MS patients, and this may explain their potential therapeutic effect in vivo.
Introduction
Multiple Sclerosis (MS) is a chronic autoimmune inflammatory demyelinating disease of the central nervous system (CNS) (1). Based on disease course, patients can be classified into three main categories, relapsing-remitting MS (RRMS), secondary progressive MS (SPMS) and primary progressive MS (PPMS) (2). The autoimmune pathogenesis of MS is well established by translational research in patients with MS and studies based on experimental autoimmune encephalomyelitis (EAE), a model of MS (3, 4).
The activation of myelin-specific T cells followed by dysregulation of Th1, Th2 and Th17 cytokines is believed to be a major event during the initiation phase of MS (5). CD4+ Th1 cells produce inflammatory mediators, such as interferon-γ (IFN-γ) which lead to autoantigen-specific inflammatory attack resulting in myelin degeneration (6, 7). IL-12 and IL-18, which can synergistically induce high levels of IFN-γ expression (8), are found elevated in MS and correlate with disease activity (9, 10). In addition to Th1 cells, it is now believed that pro-inflammatory Th17 and other IFN-γ and IL-17 producing cells, such as Th17, NK and NKT cells are also significantly involved in the initiation and perpetuation of the disease (11–13). Sharp reduction of CNS-penetrating IFN-γ and IL-17 producing cells is directly linked with disease prevention or disease remission in patients with MS (14, 15).
Current therapeutic agents in MS mainly affect pro-inflammatory cytokine production (15, 16), but they lack desirable efficacy (17–19).
In addition to their well-defined antimicrobial-bacteriostatic activity, tetracyclines (TCs) (20, 21), such as minocycline and doxycycline, demonstrate neuroprotective, anti-apoptotic, anti-inflammatory and immunomodulatory properties (22–25), and have been clinically tested with encouraging results in autoimmune diseases, such as rheumatoid arthritis and MS (26–29). For example, in a recent randomized, controlled clinical trial, conversion from a clinically isolated syndrome to MS at six months was significantly lower in the minocycline compared to placebo group, but this effect was short-lived (27). Other trials failed to report an efficacy of TCs in MS (30), contradicting data from animals whereby minocycline dramatically suppresses ongoing disease activity and remarkably limits EAE disease progression (31–33). Amongst the mechanisms involved in their immunomodulatory action are inhibition on IFN-γ and TNF-α production by T-lymphocytes or monocytes (34–37). An anti-inflammatory effect of minocycline has been reported in EAE when combined with other treatments such as IFN-β, steroids, glatiramer acetate, and atorvastatin (32, 38–40). Also, Popovic et al. reported a dramatic suppression of disease activity in EAE by minocycline and a deviation of MOG-specific T cell response towards a Th2-like response (31).
The precise cellular source and mechanism of cytokine modulation by TCs in MS patients, remains largely unknown and studies conducted so far limited their assessment in particular cell-subsets, selecting either TC but not both. The aim of our study was to comparatively investigate for the first time the in vitro effect of both minocycline and doxycycline on IFN-γ and IL-17 production by peripheral cell populations in patients with MS (newly-diagnosed naive and treated RRMS).
Patients and Methods
Patients and Healthy Controls
A total of 35 patients with MS diagnosed according to the McDonald criteria (41) were included in the study, including 15 naïve (12 females, mean age of 41.4 ± 13.4 years, range 19-59) and 20 RRMS (13 females, mean age of 40.9 ± 11.4 years, range 20-61) with a mean disease duration of (11.2 ± 8.8 years, range 1-33). All patients attended the Outpatient Clinics of the Neurology Department, of the University General Hospital of Larissa, Greece. RRMS patients (9 at relapse) were on standard treatment (glatiramer acetate, n=3; natalizumab, n=6; fingolimod, n=5; fampridine, n=1; and IFN-β n=5). Blood collection of all naive MS patients was performed before initiation of corticosteroid or other drug treatment. Ten age and sex-matched healthy individuals (7 females, mean age of 37.3 ± 10.4 years) were included as healthy controls (HCs). Patients and healthy HCs had not received TCs for at least three months before blood collection.
All experimental protocols were approved by the Local Institution’s Ethical Committee of the University General Hospital of Larissa, University of Thessaly while written informed consent has been obtained from all study participants according to the declaration of Helsinki.
PBMC Isolation and Cryopreservation
Peripheral blood samples (20-30mL) from MS patients and HCs were collected by venipuncture in preservative-free heparin tubes (50 U/mL) and aliquots were layered onto an equal volume of Ficoll-Hypaque (10ml Lymphoprep™) density gradient solution (Axis-Shield, Oslo, Norway). Peripheral blood mononuclear cells (PBMCs) were isolated by centrifugation at 300g, washed twice with RPMI-1640 (GIBCO™ -Thermo Fisher Scientific, Waltham, MA, USA), counted, and their viability, determined by trypan blue exclusion, routinely exceeded 95%. Cells were re-suspended in freezing medium containing 10% DMSO and 70% fetal calf serum (FCS), aliquoted into cryogenic vials (Corning™, Thermo Fisher Scientific), kept at −80°C for one day, and then stored in liquid nitrogen tanks until used.
Reagents
TCs (minocycline hydrochloride and doxycycline hyclate, ≥ 98% pure) were purchased from Cayman Chemical Company, Michigan USA. Both antibiotics were reconstituted in DMSO, further aliquoted at small volumes and stored at a final concentration of 5 mg/ml. TCs were supplemented simultaneously with cell stimuli (see below) at a final concentration of 50μg/ml unless otherwise stated and remained in culture for 5 hours (see Results section). DMSO concentration in TC-supplemented cultures was less than 0.1%. The same DMSO concentration (0.1%) was also added to control cultures in the absence of TCs, to exclude a direct cytotoxic effect of DMSO. Recombinant IL-12 and IL-18 were purchased from R and D Systems Inc (Abington, UK) and used at a final concentration of 20 ng/ml and 25 ng/ml, respectively (8). Phorbol 12-myristate 13-acetate (PMA) and Ionomycin were obtained from Sigma-Aldrich-Merck (Gillingham, UK) and were used at 20-50 ng/ml, and 1μg/ml, respectively in order to conventionally stimulate PBMCs in a non-specific manner (42).
Phenotypic Analysis of Peripheral Blood T and Innate Cells by Flow Cytometry
Phenotypic assessment and enumeration of peripheral blood sub-populations was performed using standard monoclonal antibodies (MoAbs) panels and protocols as described previously (43, 44). Briefly, upon thawing PBMCs were washed with serum-free RPMI-1640, counted to confirm more than 95% cell viability, pelleted, and re-suspended at 106 cells/mL in RPMI culture medium supplemented with L-glutamine and 10% heat-inactivated FCS (Biosera Europe, Nuaille, France). PBMCs were seeded in 24-well plates and allowed to rest at 37°C in a CO2 incubator for at least one hour before stimulation. In this study we used the following mouse anti-human MoAbs: (FITC)-conjugated anti-CD3 (clone UCHT-1), (PE)- and (PE-Cy7)-conjugated anti-CD56 (clones C5.9 and B159), (PE)- and (PE-Cy7)-conjugated anti-CD4 (clone RPA-T4) and PE conjugated anti-CD8 (clone SK1). All MoAbs were obtained from BD Biosciences (Mountain View, CA, USA) and Merck-Millipore (Burlington, MA, USA). Isolated PBMCs (0.5-1 x 106cells) were washed in phosphate-buffered saline (PBS) and re-suspended in staining buffer (PBS plus 1% FCS plus 0.09% sodium azide) and then incubated with labeled MoAbs specific for cell surface antigens for 30 minutes on ice and fixed with paraformaldehyde (2%). Background auto-fluorescence was monitored by equivalent 4-colour matched isotype control mouse anti-human MoAbs and formed the basis to set the cut-off for surface-positive cell discrimination. Flow cytometric analysis was performed in Guava® EasyCyte8 (Merck-Millipore, Burlington, MA, USA) benchtop flow cytometer using logarithmic amplification and a forward and side scatter-based gate for total lymphocyte populations. At least 3x105 events within the lymphocyte gate were collected for accurate measurement of infrequent cell subtypes.
Flow Cytometric Analysis of Apoptotic Cells by Annexin V
IL-12 and IL-18 activated PBMCs supplemented with 50μg/ml minocycline or doxycycline were collected and stained with FITC-labeled Annexin V (BioLegend, San Diego, CA, USA), which is used to specifically target and identify apoptotic cells (45), in the presence of Annexin V binding buffer according to manufacturer’s instructions. Experiments in the absence of IL-12 and IL-18 were also performed as controls. Lymphocyte subsets were identified using fluorescent labeled mAbs directed against lymphocyte surface markers (section 2.4) and subjected to conventional FACS analysis.
Intracellular IFN-γ Production by Peripheral Blood Cell Subsets
In order to measure intracellular IFN-γ protein production by peripheral T cells and innate NK and NKT cells, PBMCs were left untreated, or cultured in 10% RPMI supplemented with 20 ng/ml PMA plus 1μg/ml ionomycin for 5 hours in the presence of Brefeldin A (GolgiPlug™, BD Biosciences). Cells were surface stained, fixed and subsequently permeabilized using commercially available Perm/Wash buffers (BD Biosciences). Intracellular IFN-γ protein was detected using APC-conjugated MoAbs (clone 4S.B3) obtained from BD Biosciences.
Intracellular IL-17 Production by Th17 Cells
In order to measure intracellular IL-17 protein production by peripheral Th17 cells, PBMCs were left untreated or cultured in 10% RPMI supplemented with 50 ng/ml PMA plus 1μg/ml ionomycin for 5 hours in the presence of Brefeldin A. Cells were surface stained, fixed and subsequently permeabilized using commercially available Perm/Wash buffers (BD Biosciences). Intracellular IL-17 was detected using FITC- and PE-conjugated MoAbs clones (BL-168) all obtained from BD Biosciences and Merck-Millipore.
Statistical Analysis
Percentages of cells expressing cell surface markers and mean fluorescence intensities (MFI) were described as median of the individuals in each group. Variation in each patient group was defined by standard deviation (SD). Differences between healthy controls and patients and between patient groups one-way analysis of variance (ANOVA) and the nonparametric Mann-Whitney test. P-values smaller than 0.05 were considered significant. All graphs and statistical calculations were performed with Graph Pad Prism 9 software.
Results
Tetracyclines Decreased IFN-γ Producing Cells in a Dose-Dependent Manner
To assess the in vitro effect of either minocycline or doxycycline on IFN-γ production, antibiotics in isolation were supplemented in PBMC cell cultures at different concentrations ranging from of 0.1μg - 50μg/ml, similarly to previous reports in whole blood cultures and THP-1 human monocytes (46, 47). These concentrations are within the in vivo pharmacological plasma concentration levels noted following oral tetracycline administration; in human subjects who have taken oral doxycycline (200mg), doxycycline plasma concentrations (Cmax) of 1.5 to 7.0 μg/ml were usually reached within 3 h, and the drug had a half-life of 14 to 24 h (48, 49). Also, such TCs concentrations were administered in accordance with in vivo studies of experimental endotoxemia where doxycycline and other TCs are efficacious in downregulating inflammatory cytokines and preventing shock when the drug was injected immediately following the LPS challenge (50).
IFN-γ production was measured following stimulation with IL-12 plus IL-18 or PMA plus ionomycin for 5 hours on the basis of TCs optimal Cmax and limited half-life in vivo, as well as our previous published data, where both stimuli induce kinase (p38 MAPK) activation and robust IFN-γ production within 2-6hrs post stimulation in innate and adaptive cells (8).
Supplementary Figures 1, 2 show cumulative data from TC dose-response experiments in MS patients (n=3) and HCs (n=3). Minocycline or doxycycline decreased IFN-γ production by innate and adaptive T cells in a dose-dependent manner when IL-12 plus IL-18 was used for cell stimulation. Both drugs clearly inhibited cytokine expression in CD4+ T cells and NKT cells at pharmacological concentrations (1μg/ml-10μg/ml). However, the maximal inhibitory effect was noted when IL-12 plus IL-18 and 50μg/ml minocycline or doxycycline were used (see below). In all tested concentrations there was no detrimental effect of TCs on cell viability, as assessed by microscopic evaluation and trypan blue exclusion in line with previously published observations (47). The effect of TCs on cell viability was further assessed using Annexin V staining. As shown in Supplementary Figures 3, 4 neither antibiotic exerted a toxicity effect influencing the viability, apoptosis and percentages of any cell subset at the maximal concentration (50μg/ml). Neither minocycline nor doxycycline exerted an inhibitory effect on IFN-γ producing CD3+ and non CD3+ cell subsets from naive MS and RRMS patients, after stimulation with PMA and Ionomycin (Supplementary Figures 2, 6). There was a marginal statistically significant decrease in IFN-γ+ NKT (CD3+CD56+) cells following treatment with doxycycline (p = 0.04, n = 9) (Supplementary Figure 7). Thus, all subsequent experiments were performed using IL-12 plus IL-18 and minocycline or doxycycline at 50μg/ml.
Tetracyclines Decreased IFN-γ Producing CD4+ T Cells in MS
The flow cytometric gating strategy followed for phenotypic analysis of different cell subsets, including CD4+ T cells, is shown at Supplementary Figures 3, 5. In general, we observed a statistically significant reduction in IFN-γ producing CD4+ T cells from both MS patients and HCs in the presence of minocycline or doxycycline. Figure 1 illustrates representative cases and cumulative data. The percentage of IFN-γ+ CD4+ T lymphocytes following IL-12 plus IL-18 stimulation in naïve MS patients (n=15) decreased from 1.1 ± 0.41% to 0.53 ± 0.21% in the presence of minocycline (p = 0.004) and to 0.42 ± 0.20% in the presence of doxycycline (p = 0.001). The percentage of IFN-γ+ CD4+ T cells in RRMS patients (n=20) decreased from 0.89 ± 0.43% to 0.53 ± 0.27% in the presence of minocycline (p = 0.009) and to 0.44 ± 0.22% in the presence of doxycycline (p = 0.002). In HCs (n=10), the percentage of IFN-γ+ CD4+ T lymphocytes was reduced from 1.87 ± 0.79% to 0.80 ± 0.51% in the presence of minocycline (p = 0.0015) and to 0.71 ± 0.45% in the presence of doxycycline (p = 0.001) (Figure 1).
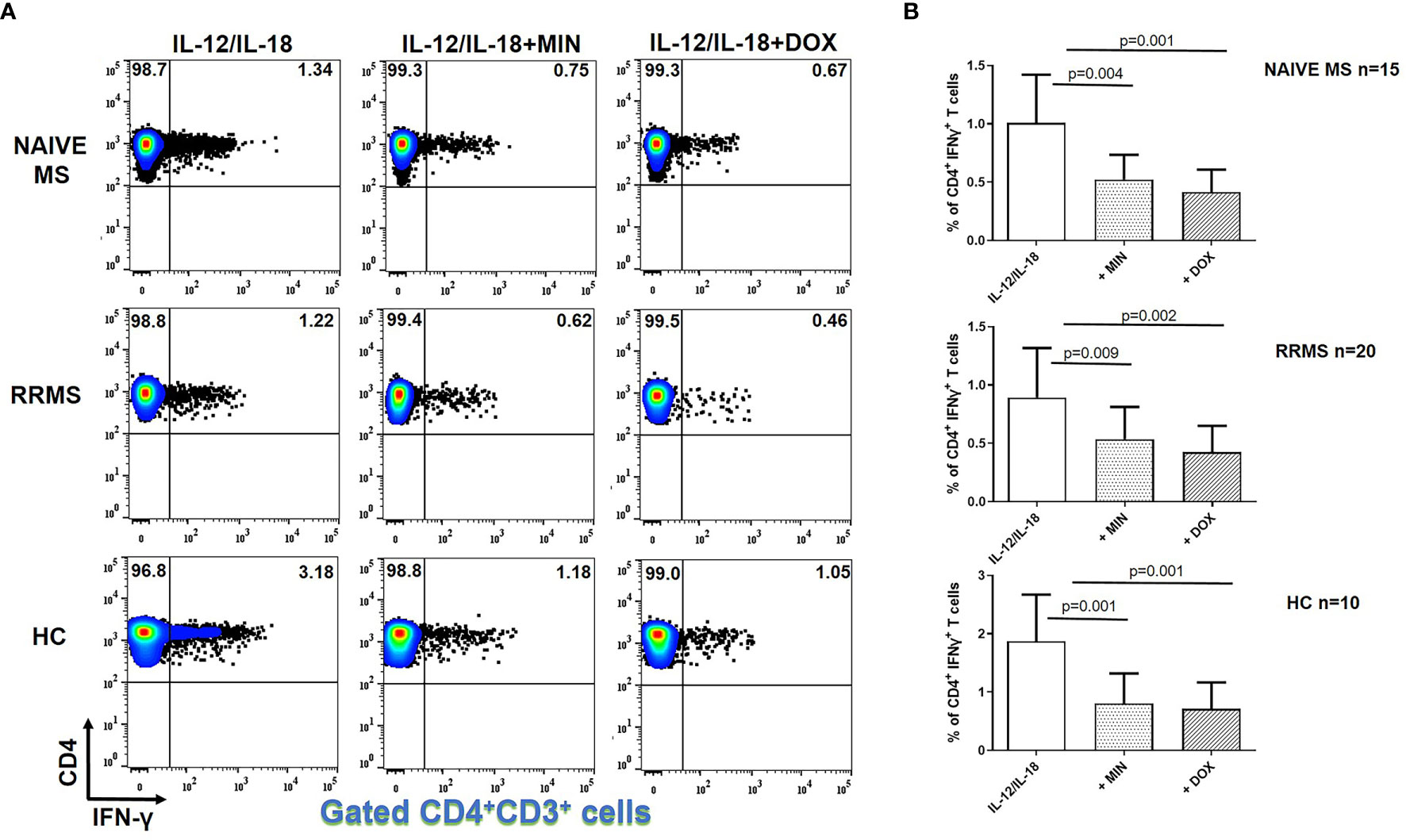
Figure 1 Tetracycline-mediated inhibition of CD4+ IFNγ+ T cells. PBMCs from naïve MS patients (n=15), RRMS patients (n=20) and HCs (n=10) were seeded in cell culture plates (1 x 106 per well) and stimulated with IL-12 plus IL-18 (IL-12/IL-18), IL-12/IL-18 plus minocycline (MIN) and IL-12/IL-18 plus doxycycline (DOX) for 5h. Cells were collected, washed, surface stained with appropriate monoclonal antibodies an analyzed for intracellular IFN-γ production by flow cytometry (see also methods section). Individual cell subsets were sub-gated according to expression of CD3 and CD4 surface epitopes. (A) Flow cytometry dot-plots showing the frequency of CD4+IFNγ+ T cells in IL-12/IL-18, IL-12/IL-18/MIN and IL-12/IL-18/DOX treated cells from representative MS cases and HCs. (B) Box and whiskers graphical representation showing significant reduction in the percentages of IFN-γ-producing CD4+ T cells in the presence of tetracyclines in naïve MS, RRMS and HCs.
Minocycline and doxycycline did not decrease IFN-γ producing CD8+ T cells from naive MS (n=15) or RRMS patients (n=20) (Figure 2). However, in HCs (n=10), the percentage of IFN-γ+CD8+ T lymphocytes was reduced from 3.43 ± 0.45% to 2.18 ± 0.78% in the presence of minocycline (p = 0.001) and to 2.10 ± 0.75% following doxycycline supplementation (p = 0.001) (Figure 2).
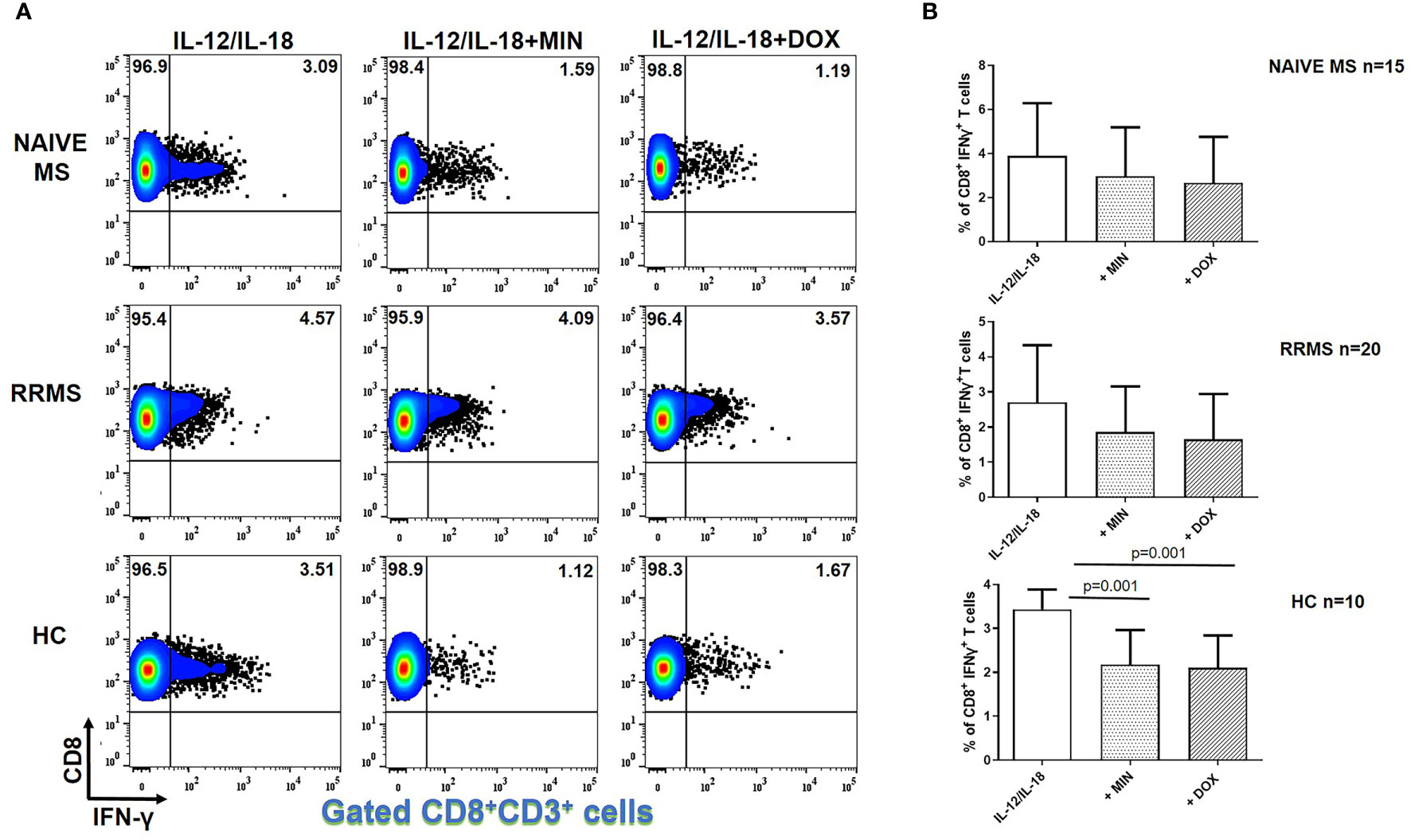
Figure 2 Tetracycline-mediated inhibition of CD8+ IFNγ+ T cells. PBMCs from naïve MS patients (n=15), RRMS patients (n=20) and HCs (n=10) were seeded in cell culture plates (1 x 106 per well) and stimulated with IL-12 plus IL-18 (IL-12/IL-18), IL-12/IL-18 plus minocycline (MIN) and IL-12/IL-18 plus doxycycline (DOX) for 5h. Cells were collected, washed, surface stained with appropriate monoclonal antibodies an analyzed for intracellular IFN-γ production by flow cytometry (see also methods section). Individual cell subsets were sub-gated according to expression of CD3 and CD8 surface epitopes. (A) Flow cytometry dot-plots showing the frequency of CD8+IFNγ+ T cells in IL-12/IL-18, IL-12/IL-18/MIN and IL-12/IL-18/DOX treated cells from representative MS cases and HCs. (B) Box and whiskers graphical representation showing significant reduction in the percentages of IFNγ-producing CD8+ T cells in the presence of tetracyclines in HCs but not naïve MS and RRMS patients.
Tetracyclines Decreased IFN-γ Producing NKT Cells in MS
IFN-γ+ NKT lymphocytes following IL-12 plus IL-18 stimulation in naïve MS (n=15) patients decreased from 6.49 ± 4.10% to 3.61 ± 2.39% in the presence of minocycline (p = 0.02) and to 2.52 ± 1.87% in the presence of doxycycline (p = 0.003) (Figure 3). The percentage of IFN-γ+ NKT cells in RRMS patients (n=20) decreased from 7.34 ± 4.40% to 3.92 ± 2.51% in the presence of minocycline (p = 0.01) and to 2.40 ± 1.89% in the presence of doxycycline (p = 0.001). In HCs (n=10), the percentage of IFN-γ+ NKT lymphocytes was reduced from 14.73 ± 7.53% to 7.35 ± 4.27% following minocycline supplementation (p = 0.03) and to 5.41 ± 3.07% following doxycycline supplementation (p = 0.005).
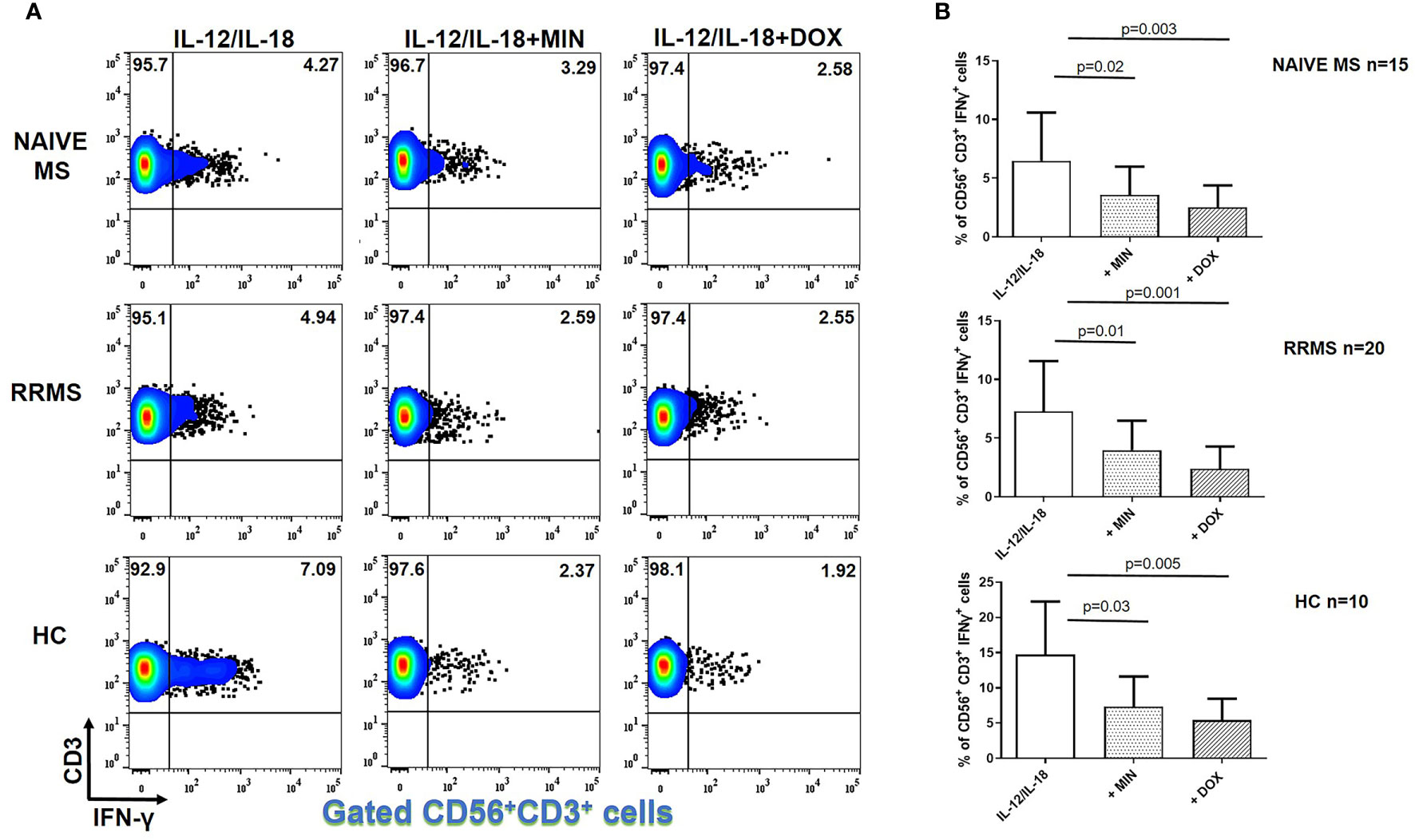
Figure 3 Tetracycline-mediated inhibition of CD56+CD3+IFN-γ+ T cells. PBMCs from naïve MS patients (n=15), RRMS patients (n=20) and HCs (n=10) were seeded in cell culture plates (1 x 106 per well) and stimulated with IL-12 plus IL-18 (IL-12/IL-18), IL-12/IL-18 plus minocycline (MIN) and IL-12/IL-18 plus doxycycline (DOX) for 5h. Cells were collected, washed, surface stained with appropriate monoclonal antibodies an analyzed for intracellular IFN-γ production by flow cytometry (see also methods section). Individual cell subsets were sub-gated according to expression of CD3 and CD56 surface epitopes. (A) Flow cytometry dot-plots showing the frequency of IFN-γ+ NKT cells (gated CD56+CD3+ cells two-dimensionally depicted in CD3 vs IFN-γ plots) in IL-12/IL-18, IL-12/IL-18/MIN and IL-12/IL-18/DOX treated cells from representative MS cases. (B) Box and whiskers graphical representation showing significant reduction in the percentages of IFN-γ-producing NKT cells in the presence of tetracyclines in naïve MS, RRMS and HCs.
Tetracyclines Did Not Affect IFN-γ Producing NK Cells in MS
In IL-12 and IL-18- stimulated NK cells, there was no difference in IFN-γ+ cells in the presence of minocycline or doxycycline in naïve MS (n=15), RRMS (n=20) or HCs (n=10) (Figure 4).
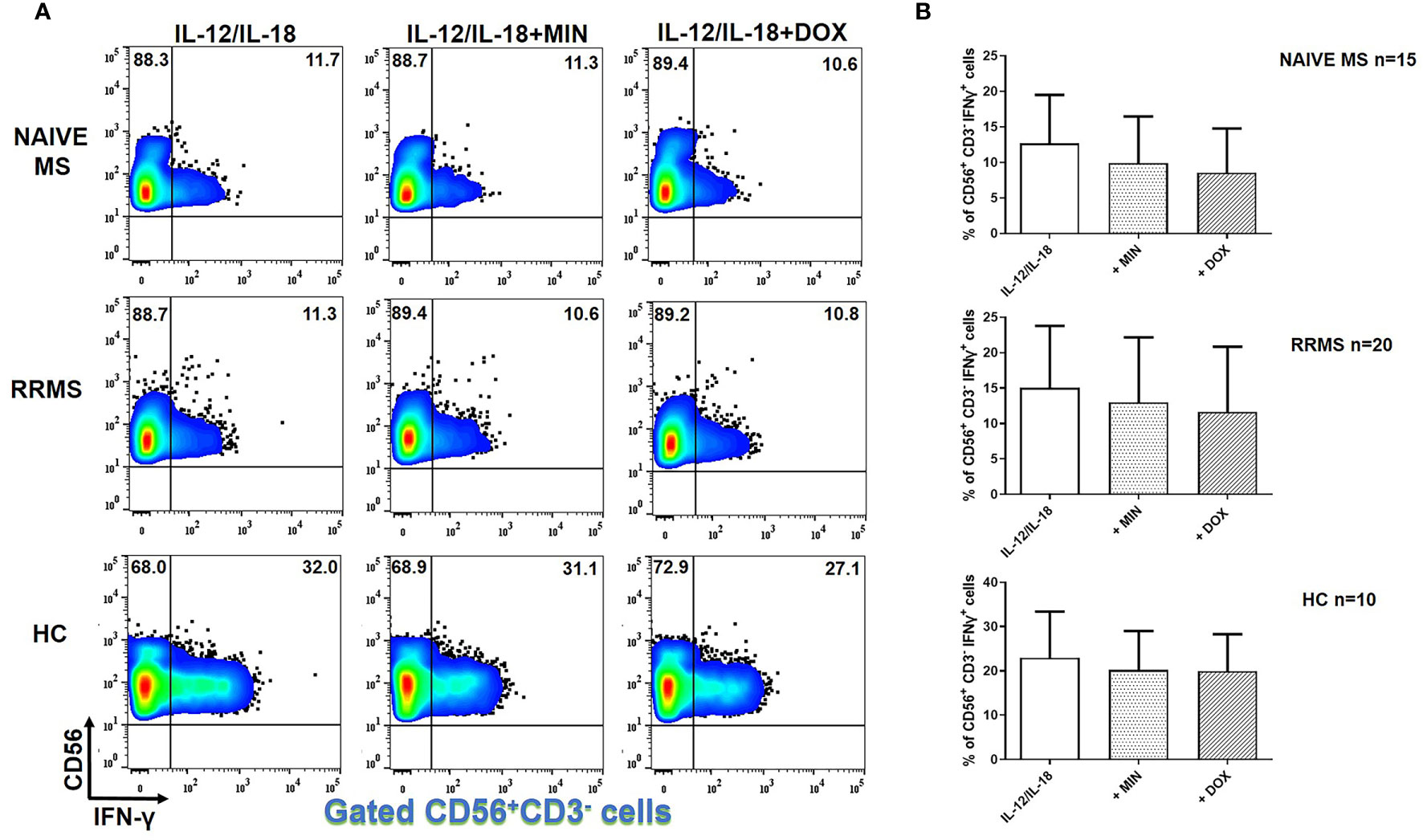
Figure 4 Tetracyclines have no significant effect on IFN-γ+ NK cells. PBMCs from naïve MS patients (n=15), RRMS patients (n=20) and HCs (n=10) were seeded in cell culture plates (1 x 106 per well) and stimulated with IL-12 plus IL-18 (IL-12/IL-18), IL-12/IL-18 plus minocycline (MIN) and IL-12/IL-18 plus doxycycline (DOX) for 5h. Cells were collected, washed, surface stained with appropriate monoclonal antibodies an analyzed for intracellular IFN-γ production by flow cytometry (see also methods section). Individual cell subsets were sub-gated according to expression of CD3 and CD56 surface epitopes. (A) Flow cytometry dot-plots showing the frequency of CD56+CD3-IFNγ+ NK cells (gated CD56+CD3- cells two-dimensionally depicted in CD56 vs IFN-γ plots) in IL-12/IL-18, IL-12/IL-18/MIN and IL-12/IL-18/DOX treated cells from representative MS cases. (B) Box and whiskers graphical representation show no significant reduction in the percentages of IFN-γ-producing NK cells in the presence of tetracyclines in either HCs or MS patients.
Tetracyclines Decreased IL-17A Producing CD4+ T Cell and NKT Cells in MS
We also investigated the effect of TCs on IL-17A production in PMA plus ionomycin activated PBMCs from patients with naïve MS (n=9), RRMS patients (n=9) and HCs (n=9). As shown in Figure 5, TCs decreased IL-17 production from CD4-expressing cells. These consisted of CD3+CD4+ T cells and CD56+CD4+ NKT cells. In patients with naïve MS the percentage of CD4+IL-17A+ T cells decreased from 1.77 ± 1.33% to 0.74 ± 0.43% in the presence of minocycline (p = 0.02) and to 0.55 ± 0.44% in the presence of doxycycline (p = 0.01) (Figure 5). In RRMS patients the percentage of CD4+IL-17A+ T cells decreased from 2.14 ± 1.68% to 1.2 ± 0.9% in the presence of minocycline (p = 0.04) and to 0.76 ± 0.71% in the presence of doxycycline (p = 0.02). In HCs the percentage of CD4+IL-17A+ T cells decreased from 1.35 ± 0.51% to 0.64 ± 0.35% in the presence of minocycline (p = 0.01) and to 0.43 ± 0.29% in the presence of doxycycline (p = 0.02).
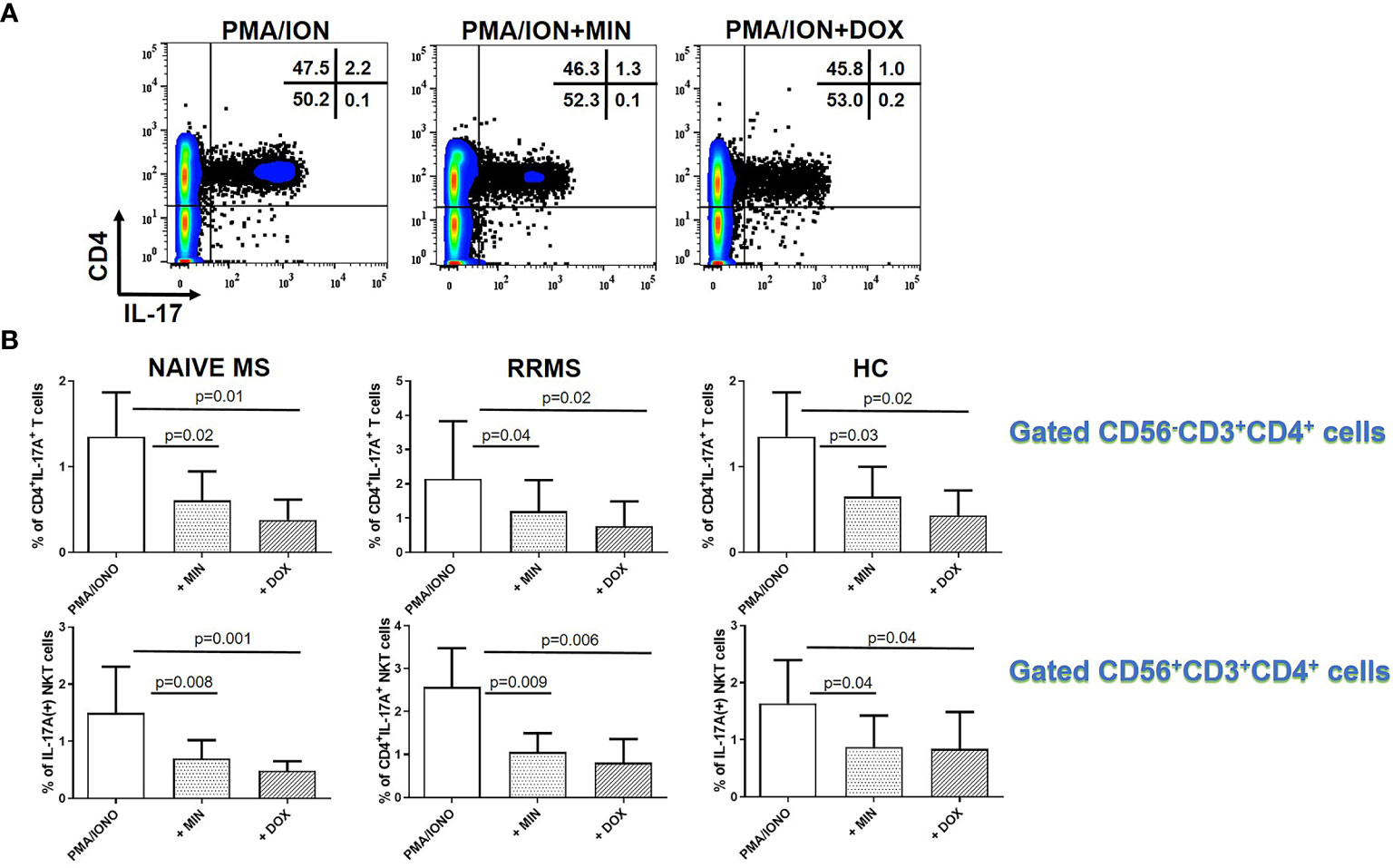
Figure 5 Tetracycline-mediated inhibition of CD4+CD3+IL-17A+ T cells and CD56+CD4+IL-17A+ T (NKT) cells. PBMCs from naïve MS patients (n=9), RRMS patients (n=9) and HCs (n=9) were seeded in cell culture plates (1 x 106 per well) and stimulated with PMA plus ionomycin (PMA/ION), PMA/ION plus minocycline (MIN) and PMA/ION plus doxycycline (DOX) for 5h. Cells were collected, washed, surface stained with appropriate monoclonal antibodies an analyzed for intracellular IL-17A production by flow cytometry (see also methods section). (A) Flow cytometry dot-plots showing the frequency of total CD4+IL-17A+ cells in PMA/IONO, PMA/IONO/MIN and PMA/IONO/DOX treated cells from a representative naïve MS case. (B) CD4+IL-17A+ cells were sub-divided according to expression of CD3 or CD56 surface epitopes. Box and whiskers graphical representation showing significant reduction in the percentages of IL-17A-producing T and NKT cells in the presence of tetracyclines in naïve MS patients, RRMS patients and HCs.
The percentage of CD4+IL-17A+ NKT lymphocytes in naïve MS patients (n=9) decreased from 1.49 ± 0.80% to 0.69 ± 0.33% in the presence of minocycline (p = 0.008) and to 0.48 ± 0.17% in the presence of doxycycline (p = 0.001). The percentage of CD4+IL-17A+ NKT lymphocytes in RRMS patients (n=9) decreased from 2.59 ± 0.90% to 1.09 ± 0.43% in the presence of minocycline (p = 0.009) and to 0.8 ± 0.55% in the presence of doxycycline (p = 0.006). The percentage of CD4+IL-17A+ NKT lymphocytes in HCs (n=9) decreased from 1.63 ± 0.75% to 0.87 ± 0.54% in the presence of minocycline (p = 0.04) and to 0.84 ± 0.64% in the presence of doxycycline (p = 0.04).
Discussion
The major finding of our study is that of an inhibitory effect of minocycline and doxycycline on IFN-γ and IL-17 producing cells in a cell subset-related, dose-dependent manner in patients with MS, with the most prominent effect being noted in CD4+ T and NKT lymphocytes.
Our results are of importance for studies conducted in humans, as previous studies in MS have only been limited to EAE, with their findings emphasizing an anti-inflammatory role of antibiotics and in particular minocycline, in synergy with prednisone (51), IFN-β1 (52) glatiramer acetate (38) or atorvastatin (39). While studies in EAE showed that minocycline attenuates the disease by reducing T cell infiltration into the spinal cord without affecting the cytokine production profile (33), our human data clearly demonstrate significant reduction of IFN-γ (and/or IL-17) by both T cells and NK/NKT cells, the inhibition varying amongst different cell types. No clear data on the effect of doxycycline on pro-inflammatory cytokine production in EAE currently exist, though our human data support the notion that this antibiotic also diminishes the ability of adaptive and innate immunity cells to produce IFN-γ and IL-17. In support, doxycycline decreased inflammatory infiltration of T-cell, B-cell and macrophage infiltration, sharply diminished IL-17 production and attenuated demyelination in sciatic nerves of rats with experimental autoimmune neuritis, a model of human inflammatory demyelinating polyneuropathies (53).
In our cohort of patients with MS, a comparable TC-mediated reduction of cytokine production was noted between naïve and treated RRMS patients. Subsequent stratified analysis amongst patients receiving different therapies revealed no statistically significant differences regarding the levels of TC-mediated cytokine inhibition within CD4+ T and NKT cells. It remains to be seen whether the in vitro effect of TC could be augmented following supplementation of MS-related immunomodulatory agents and whether this effect could be seen in vivo.
Clinical trials using minocycline, alone or in combination with conventional therapeutic agents, have produced inconclusive results, some demonstrating beneficial effect on the risk of conversion from a clinically isolated syndrome to MS (27), while others failed to show a superiority of minocycline when added to IFN β-1a (30).
Those studies by no means can de-emphasize the importance of our in vitro study. In rheumatoid arthritis, a typical autoimmune disease, both experimental and human data reveal a beneficial effect of TCs in combination with methotrexate over methotrexate alone, especially in early disease state, suggesting that timing of initiation of TCs may be of importance (54, 55). This effect is clearly attributed to the dose-dependent inhibition of T cell proliferation and reduction of IFN-γ and other pro-inflammatory cytokines (56), a finding that perfectly fits with those obtained in our study.
The inhibitory effect of antibiotics on IFN-γ was noted after the physiological stimulation of PBMC with IL-12 and IL-18 but not with PMA and ionomycin, a phenomenon, which requires further discussion. Previous work has shown that the effect of minocycline on cytokine production by T-cells and monocytes is stimulus specific, as T cells stimulated by a Ca2+-independent manner exhibited a decrease in TNF-alpha mRNA in the presence of minocycline, whereas the TNF-alpha mRNA level remained unaffected by minocycline when cells were stimulated in a Ca2+-dependent manner, like in the case of PMA/ionomycin stimulation (36, 56). The limitation of the in vitro studies, even if those are conducted in patients with MS and not in animal models of MS, cannot be ignored. While no in vivo studies have looked at the effect of TCs in IFN-γ or IL-17 producing T and NK cell-subsets in patients with MS, a study in EAE has provided data of interest. An early report by Popovic et al. (31) found that minocycline administration suppressed migration of inflammatory cells into CNS and further activation by a direct effect on the cytokine milieu in EAE. Treatment with minocycline shifted the balance from Th-1 to Th-2 and resulted in enhanced IL-10, reduced TNF-α and a minimal effect on IFN-γ production, as measured by ELISA in cell-culture supernatants (31). The investigation of cell-subset T cell specific cytokine production was not included in the aims of that study. Those latter data further emphasize the need for a vigorous attempt to assess the in vivo the effect of TCs on T-cell activation and cell-subset specific pro- and anti-inflammatory cytokine production in patients with MS in well-designed clinical trials.
Conclusion
In conclusion, this is the first in vitro study to show that minocycline and doxycycline have a significant inhibitory effect in CD4+ T and NKT cell producing IFN-γ and/or IL-17 in patients with MS (naïve or under treatment). If this is proved in vivo in prospectively collected biological material from MS patients under treatment with these tetracyclines, it may have potential clinical relevance. In vivo inhibition of IFN-γ (and possibly IL-17)-producing NKT cells, for example, is a favorable pre-requisite for successful remission in patients with MS (57–59), and the effect of tetracyclines towards achieving this goal may provide an additional therapeutic tool, most likely in combination with standard treatment regimen in stratified cohorts of patients.
Data Availability Statement
The raw data supporting the conclusions of this article will be made available by the authors, without undue reservation.
Ethics Statement
The studies involving human participants were reviewed and approved by Ethics and Scientific Committee, University General Hospital of Larissa, University of Thessaly, Faculty of Medicine, School of Health Sciences. The patients/participants provided their written informed consent to participate in this study.
Author Contributions
DF, AM, and ED contributed equally. LS, GH, and DB had the original idea. AM, ED, LS, GH and DB scripted considerable part of original draft. DF, AM, ED, CK, LS, and DB had performed extensive literature search and scripted relevant parts of the manuscript. DF, ED, VT, AA, VS, CT, and GH collected clinical and biological material and clinical data. DF, AM and CL analyzed the data. DF and DB prepared the artwork. DB, DF, and AM had edited the final version of the manuscript. DB and ED provided overall supervision. All authors performed extensive literature search and approved the final version of the manuscript. All authors contributed to the article and approved the submitted version.
Funding
This research was funded in part by the Special Account for Research Grants University of Thessaly, grant number 6357, 5158 and 5847.
Conflict of Interest
Author ED: Allergan, Novartis, Genesis, ELPEN, Bayer, Teva, Merck- Serono, Genzyme-Sanofi, Roche, UCB - speaker or chairman horonaria, advisory or travel grants, and clinical research-educational support grants. Author DB: AbbVie, Novartis, Genesis, ELPEN, Pfizer, Aenorasis, Menarini, Kopper, ITF Hellas, Roche, MSD, GSK, Hospital Line - speaker or chairman horonaria or paid investigator or clinical research and educational support grants.
The remaining authors declare that the research was conducted in the absence of any commercial or financial relationships that could be construed as a potential conflict of interest.
Publisher’s Note
All claims expressed in this article are solely those of the authors and do not necessarily represent those of their affiliated organizations, or those of the publisher, the editors and the reviewers. Any product that may be evaluated in this article, or claim that may be made by its manufacturer, is not guaranteed or endorsed by the publisher.
Acknowledgments
We would like to thank Dr Zisis Tsouris for his contribution in clinical data collection, and Prof Eirini Rigopoulou for critical comments regarding data presentation and interpretation.
Supplementary Material
The Supplementary Material for this article can be found online at: https://www.frontiersin.org/articles/10.3389/fimmu.2021.739186/full#supplementary-material
Supplementary Figure 1 | IFN-γ producing PBMC subsets following stimulation with IL-12 plus IL-18 in the presence of different doses of tetracyclines. The in vitro effect of either minocycline or doxycycline supplemented at different concentrations ranging from of 0.1μg - 50μg/ml on IFN-γ production was assessed in PBMC cell cultures of MS patients (n=3) and HCs (n=3) following stimulation with IL-12 plus IL-18 for 5 hours (Box and whiskers graphical representation of cumulative data with standard deviation errors bars are shown). *P < 0.05 **P < 0.005 ***P < 0.0005.
Supplementary Figure 2 | IFN-γ producing PBMC subsets following stimulation with PMA plus ionomycin in the presence of different doses of tetracyclines. The in vitro effect of either minocycline or doxycycline supplemented at different concentrations ranging from of 0.1μg - 50μg/ml on IFN-γ production was assessed in PBMC cell cultures of MS patients (n=3) and HCs (n=3) following stimulation with PMA plus ionomycin for 5 hours. (Box and whiskers graphical representation of cumulative data with standard deviation errors bars are shown). Data show a dose-depending inhibition only in IFN-γ producing NKT cells *P < 0.05 (see also Supplementary Figure 7).
Supplementary Figure 3 | Tetracyclines do not affect PBMC flow cytometric forward/side scatter characteristics and proportions of sub-gated cell subsets. Representative flow cytometric assessment (MS case) of the in vitro effect of either minocycline or doxycycline supplemented at the highest concentration (50μg/ml) on PBMC cell viability and on cytometric analysis characteristics based on size/granularity (FSC/SSC) and phenotypic discrimination of the proportions of individual cell subset.
Supplementary Figure 4 | Tetracyclines do not induce apoptosis of cell subsets. Representative flow cytometric illustration (MS case) of the in vitro effect of either minocycline or doxycycline supplemented at the highest concentration (50μg/ml) on apoptosis of sub-gated cell subpopulations of CD4 and CD8 T cells assessed by annexin V staining. Data are suggestive of lack of an in vitro effect of tetracyclines in inducting apoptosis and affecting viability.
Supplementary Figure 5 | Representative flow cytometric gating strategy of tetracycline treated IL-12 plus IL-18 stimulated PBMC subsets. Individual cell subsets from a representative MS case were sub-gated according to expression of CD3, CD4, CD8 and CD56 surface markers. All surface epitopes were sufficiently maintained and detected following cell activation with IL-12 plus IL-18 in the presence of minocycline and doxycycline.
Supplementary Figure 6 | Flow cytometric illustration of tetracycline-mediated effects on IFNγ production by PMA/ionomycin and IL-12 plus IL-18 stimulated CD3+ and non-CD3+ cell subsets from MS patients. Data of the effect of tetracyclines using different stimuli in a representative naïve MS case are shown in A and B and in a representative RRMS are shown in C and D. PBMCs were analyzed for IFN-γ production by flow cytometry following minocycline (MIN) or doxycycline (DOX) supplementation (50μg/ml) and simultaneous treatment with PMA plus ionomycin (A, C) or IL-12 plus IL-18 stimulation (B, D). CD3+ and non-CD3+ cell subsets were sub-gated according to expression of CD3 surface epitope.
Supplementary Figure 7 | Doxycycline-mediated inhibition of CD56+CD3+IFNγ+ T cells subsets following stimulation with PMA plus ionomycin. PBMCs from naïve MS patients (n=9) were analyzed for IFN-γ production by flow cytometry following minocycline (MIN) or doxycycline (DOX) supplementation and simultaneous PMA plus ionomycin stimulation (see also methods section). NKT cells were sub-gated according to expression of CD3 and CD56 surface markers. (A) CD3 versus IFN-γ Flow cytometry dot-plots in sub-gated NKT cells showing the frequency of IFN-γ+ NKTs following PMA/IONO, PMA/IONO/MIN and PMA/IONO/DOX treatment. (B) Box and whiskers graphical representation showing significant reduction in the percentages of IFN-γ-producing NKT cells in the presence of doxycycline in naïve MS.
Supplementary Figure 8 | CD4+IFN-γ+ cells represent T helper 1 (TH1) cells and not other CD4+ subsets like macrophage or DC subsets. PBMCs from MS patients were analyzed for IFN-γ production by flow cytometry following minocycline (MIN) or doxycycline (DOX) supplementation and IL-12 plus IL-18 stimulation (see also methods section).Th1 cells were assessed by staining against CD3, CD4 and intracellular IFN-γ simultaneously. A representative case of n=5 is illustrated where the effect of minocycline and doxycycline on IFN-γ production is clearly seen on CD3+ and CD4+ ie Th1 cells.
Abbreviations
CNS, Central Nervous System; EAE, Experimental Autoimmune encephalomyelitis; FCS, Fetal Calf Serum; MoAb, Monoclonal Antibody; MS, Multiple Sclerosis; PMA, Phorbol Myristate Acetate; TC, Tetracycline.
References
1. Ghasemi N, Razavi S, Nikzad E. Multiple Sclerosis: Pathogenesis, Symptoms, Diagnoses and Cell-Based Therapy. Cell J (2017) 19(1):1–10. doi: 10.22074/cellj.2016.4867
2. Lublin FD, Reingold SC, Cohen JA, Cutter GR, Sorensen PS, Thompson AJ, et al. Defining the Clinical Course of Multiple Sclerosis: The 2013 Revisions. Neurology (2014) 83(3):278–86. doi: 10.1212/WNL.0000000000000560
3. Dargahi N, Katsara M, Tselios T, Androutsou ME, de Courten M, Matsoukas J, et al. Multiple Sclerosis: Immunopathology and Treatment Update. Brain Sci (2017) 7(7):78. doi: 10.3390/brainsci7070078
4. Mastorodemos V, Ioannou M, Verginis P. Cell-Based Modulation of Autoimmune Responses in Multiple Sclerosis and Experimental Autoimmmune Encephalomyelitis: Therapeutic Implications. Neuroimmunomodulation (2015) 22(3):181–95. doi: 10.1159/000362370
5. Rostami A, Ciric B. Role of Th17 Cells in the Pathogenesis of CNS Inflammatory Demyelination. J Neurol Sci (2013) 333(1-2):76–87. doi: 10.1016/j.jns.2013.03.002
6. Dettke M, Scheidt P, Prange H, Kirchner H. Correlation Between Interferon Production and Clinical Disease Activity in Patients With Multiple Sclerosis. J Clin Immunol (1997) 17(4):293–300. doi: 10.1023/A:1027374615106
7. Ottum PA, Arellano G, Reyes LI, Iruretagoyena M, Naves R. Opposing Roles of Interferon-Gamma on Cells of the Central Nervous System in Autoimmune Neuroinflammation. Front Immunol (2015) 6:539. doi: 10.3389/fimmu.2015.00539
8. Mavropoulos A, Sully G, Cope AP, Clark AR. Stabilization of IFN-Gamma mRNA by MAPK P38 in IL-12- and IL-18-Stimulated Human NK Cells. Blood (2005) 105(1):282–8. doi: 10.1182/blood-2004-07-2782
9. Comabella M, Balashov K, Issazadeh S, Smith D, Weiner HL, Khoury SJ. Elevated Interleukin-12 in Progressive Multiple Sclerosis Correlates With Disease Activity and Is Normalized by Pulse Cyclophosphamide Therapy. J Clin Invest (1998) 102(4):671–8. doi: 10.1172/JCI3125
10. Fassbender K, Mielke O, Bertsch T, Muehlhauser F, Hennerici M, Kurimoto M, et al. Interferon-Gamma-Inducing Factor (IL-18) and Interferon-Gamma in Inflammatory CNS Diseases. Neurology (1999) 53(5):1104–6. doi: 10.1212/WNL.53.5.1104
11. McGinley AM, Edwards SC, Raverdeau M, Mills KHG. Th17cells, Gammadelta T Cells and Their Interplay in EAE and Multiple Sclerosis. J Autoimmun (2018) S0896-8411(18)30007-6. doi: 10.1016/j.jaut.2018.01.001
12. El-Behi M, Ciric B, Dai H, Yan Y, Cullimore M, Safavi F, et al. The Encephalitogenicity of T(H)17 Cells Is Dependent on IL-1- and IL-23-Induced Production of the Cytokine GM-CSF. Nat Immunol (2011) 12(6):568–75. doi: 10.1038/ni.2031
13. Van Kaer L, Postoak JL, Wang C, Yang G, Wu L. Innate, Innate-Like and Adaptive Lymphocytes in the Pathogenesis of MS and EAE. Cell Mol Immunol (2019) 16(6):531–9. doi: 10.1038/s41423-019-0221-5
14. da Costa DS, Hygino J, Ferreira TB, Kasahara TM, Barros PO, Monteiro C, et al. Vitamin D Modulates Different IL-17-Secreting T Cell Subsets in Multiple Sclerosis Patients. J Neuroimmunol (2016) 299:8–18. doi: 10.1016/j.jneuroim.2016.08.005
15. Wing AC, Hygino J, Ferreira TB, Kasahara TM, Barros PO, Sacramento PM, et al. Interleukin-17- and Interleukin-22-Secreting Myelin-Specific CD4(+) T Cells Resistant to Corticoids Are Related With Active Brain Lesions in Multiple Sclerosis Patients. Immunology (2016) 147(2):212–20. doi: 10.1111/imm.12552
16. Petek-Balci B, Coban A, Shugaiv E, Turkoglu R, Ulusoy C, Icoz S, et al. Predictive Value of Early Serum Cytokine Changes on Long-Term Interferon Beta-1A Efficacy in Multiple Sclerosis. Int J Neurosci (2015) 125(5):352–6. doi: 10.3109/00207454.2014.939747
17. Longbrake EE, Racke MK. Why did IL-12/IL-23 Antibody Therapy Fail in Multiple Sclerosis? Expert Rev Neurother (2009) 9(3):319–21. doi: 10.1586/14737175.9.3.319
18. Dumitrescu L, Constantinescu CS, Tanasescu R. Recent Developments in Interferon-Based Therapies for Multiple Sclerosis. Expert Opin Biol Ther (2018) 18(6):665–80. doi: 10.1080/14712598.2018.1462793
19. Avasarala J. It’s Time for Combination Therapies: In Multiple Sclerosis. Innov Clin Neurosci (2017) 14(5-6):28–30.
20. Chopra I, Hawkey PM, Hinton M. Tetracyclines, Molecular and Clinical Aspects. J Antimicrob Chemother (1992) 29(3):245–77. doi: 10.1093/jac/29.3.245
21. Saivin S, Houin G. Clinical Pharmacokinetics of Doxycycline and Minocycline. Clin Pharmacokinet (1988) 15(6):355–66. doi: 10.2165/00003088-198815060-00001
22. Sapadin AN, Fleischmajer R. Tetracyclines: Nonantibiotic Properties and Their Clinical Implications. J Am Acad Dermatol (2006) 54(2):258–65. doi: 10.1016/j.jaad.2005.10.004
23. Garrido-Mesa J, Rodriguez-Nogales A, Algieri F, Vezza T, Hidalgo-Garcia L, Garrido-Barros M, et al. Immunomodulatory Tetracyclines Shape the Intestinal Inflammatory Response Inducing Mucosal Healing and Resolution. Br J Pharmacol (2018) 175(23):4353–70. doi: 10.1111/bph.14494
24. Chen X, Ma X, Jiang Y, Pi R, Liu Y, Ma L. The Prospects of Minocycline in Multiple Sclerosis. J Neuroimmunol (2011) 235(1-2):1–8. doi: 10.1016/j.jneuroim.2011.04.006
25. Maier K, Merkler D, Gerber J, Taheri N, Kuhnert AV, Williams SK, et al. Multiple Neuroprotective Mechanisms of Minocycline in Autoimmune CNS Inflammation. Neurobiol Dis (2007) 25(3):514–25. doi: 10.1016/j.nbd.2006.10.022
26. Kloppenburg M, Breedveld FC, Terwiel JP, Mallee C, Dijkmans BA. Minocycline in Active Rheumatoid Arthritis. A Double-Blind, Placebo-Controlled Trial. Arthritis Rheum (1994) 37(5):629–36. doi: 10.1002/art.1780370505
27. Metz LM, Li DKB, Traboulsee AL, Duquette P, Eliasziw M, Cerchiaro G, et al. Trial of Minocycline in a Clinically Isolated Syndrome of Multiple Sclerosis. N Engl J Med (2017) 376(22):2122–33. doi: 10.1056/NEJMoa1608889
28. Minagar A, Alexander JS, Schwendimann RN, Kelley RE, Gonzalez-Toledo E, Jimenez JJ, et al. Combination Therapy With Interferon Beta-1a and Doxycycline in Multiple Sclerosis: An Open-Label Trial. Arch Neurol (2008) 65(2):199–204. doi: 10.1001/archneurol.2007.41
29. Zabad RK, Metz LM, Todoruk TR, Zhang Y, Mitchell JR, Yeung M, et al. The Clinical Response to Minocycline in Multiple Sclerosis Is Accompanied by Beneficial Immune Changes: A Pilot Study. Mult Scler (2007) 13(4):517–26. doi: 10.1177/1352458506070319
30. Sorensen PS, Sellebjerg F, Lycke J, Farkkila M, Creange A, Lund CG, et al. Minocycline Added to Subcutaneous Interferon Beta-1a in Multiple Sclerosis: Randomized RECYCLINE Study. Eur J Neurol (2016) 23(5):861–70. doi: 10.1111/ene.12953
31. Popovic N, Schubart A, Goetz BD, Zhang SC, Linington C, Duncan ID. Inhibition of Autoimmune Encephalomyelitis by a Tetracycline. Ann Neurol (2002) 51(2):215–23. doi: 10.1002/ana.10092
32. Brundula V, Rewcastle NB, Metz LM, Bernard CC, Yong VW. Targeting Leukocyte Mmps and Transmigration: Minocycline as a Potential Therapy for Multiple Sclerosis. Brain (2002) 125(Pt 6):1297–308. doi: 10.1093/brain/awf133
33. Nikodemova M, Lee J, Fabry Z, Duncan ID. Minocycline Attenuates Experimental Autoimmune Encephalomyelitis in Rats by Reducing T Cell Infiltration Into the Spinal Cord. J Neuroimmunol (2010) 219(1-2):33–7. doi: 10.1016/j.jneuroim.2009.11.009
34. Garrido-Mesa N, Zarzuelo A, Galvez J. Minocycline: Far Beyond an Antibiotic. Br J Pharmacol (2013) 169(2):337–52. doi: 10.1111/bph.12139
35. Giuliani F, Hader W, Yong VW. Minocycline Attenuates T Cell and Microglia Activity to Impair Cytokine Production in T Cell-Microglia Interaction. J Leukoc Biol (2005) 78(1):135–43. doi: 10.1189/jlb.0804477
36. Kloppenburg M, Verweij CL, Miltenburg AM, Verhoeven AJ, Daha MR, Dijkmans BA, et al. The Influence of Tetracyclines on T Cell Activation. Clin Exp Immunol (1995) 102(3):635–41. doi: 10.1111/j.1365-2249.1995.tb03864.x
37. Krakauer T, Buckley M. Doxycycline Is Anti-Inflammatory and Inhibits Staphylococcal Exotoxin-Induced Cytokines and Chemokines. Antimicrob Agents Chemother (2003) 47(11):3630–3. doi: 10.1128/AAC.47.11.3630-3633.2003
38. Giuliani F, Metz LM, Wilson T, Fan Y, Bar-Or A, Yong VW. Additive Effect of the Combination of Glatiramer Acetate and Minocycline in a Model of MS. J Neuroimmunol (2005) 158(1-2):213–21. doi: 10.1016/j.jneuroim.2004.09.006
39. Luccarini I, Ballerini C, Biagioli T, Biamonte F, Bellucci A, Rosi MC, et al. Combined Treatment With Atorvastatin and Minocycline Suppresses Severity of EAE. Exp Neurol (2008) 211(1):214–26. doi: 10.1016/j.expneurol.2008.01.022
40. Nikodemova M, Watters JJ, Jackson SJ, Yang SK, Duncan ID. Minocycline Down-Regulates MHC II Expression in Microglia and Macrophages Through Inhibition of IRF-1 and Protein Kinase C (PKC)Alpha/Betaii. J Biol Chem (2007) 282(20):15208–16. doi: 10.1074/jbc.M611907200
41. Thompson AJ, Banwell BL, Barkhof F, Carroll WM, Coetzee T, Comi G, et al. Diagnosis of Multiple Sclerosis: 2017 Revisions of the Mcdonald Criteria. Lancet Neurol (2018) 17(2):162–73. doi: 10.1016/S1474-4422(17)30470-2
42. Skyvalidas D, Mavropoulos A, Tsiogkas S, Dardiotis E, Liaskos C, Mamuris Z, et al. Curcumin Mediates Attenuation of Pro-Inflammatory Interferon Gamma and Interleukin 17 Cytokine Responses in Psoriatic Disease, Strengthening Its Role as a Dietary Immunosuppressant. Nutr Res (2020) 75:95–108. doi: 10.1016/j.nutres.2020.01.005
43. Mavropoulos A, Simopoulou T, Varna A, Liaskos C, Katsiari CG, Bogdanos DP, et al. Breg Cells Are Numerically Decreased and Functionally Impaired in Patients With Systemic Sclerosis. Arthritis Rheumatol (2016) 68(2):494–504. doi: 10.1002/art.39437
44. Mavropoulos A, Zafiriou E, Simopoulou T, Brotis AG, Liaskos C, Roussaki-Schulze A, et al. Apremilast Increases IL-10-Producing Regulatory B Cells and Decreases Proinflammatory T Cells and Innate Cells in Psoriatic Arthritis and Psoriasis. Rheumatol (Oxford) (2019) 58(12):2240–50. doi: 10.1093/rheumatology/kez204
45. Domae E, Hirai Y, Ikeo T, Goda S, Shimizu Y. Cytokine-Mediated Activation of Human Ex Vivo-Expanded Vgamma9Vdelta2 T Cells. Oncotarget (2017) 8(28):45928–42. doi: 10.18632/oncotarget.17498
46. Cazalis J, Tanabe S, Gagnon G, Sorsa T, Grenier D. Tetracyclines and Chemically Modified Tetracycline-3 (CMT-3) Modulate Cytokine Secretion by Lipopolysaccharide-Stimulated Whole Blood. Inflammation (2009) 32(2):130–7. doi: 10.1007/s10753-009-9111-9
47. Tai K, Iwasaki H, Ikegaya S, Ueda T. Minocycline Modulates Cytokine and Chemokine Production in Lipopolysaccharide-Stimulated THP-1 Monocytic Cells by Inhibiting Ikappab Kinase Alpha/Beta Phosphorylation. Transl Res (2013) 161(2):99–109. doi: 10.1016/j.trsl.2012.10.001
48. Newton PN, Chaulet JF, Brockman A, Chierakul W, Dondorp A, Ruangveerayuth R, et al. Pharmacokinetics of Oral Doxycycline During Combination Treatment of Severe Falciparum Malaria. Antimicrob Agents Chemother (2005) 49(4):1622–5. doi: 10.1128/AAC.49.4.1622-1625.2005
49. Welling PG, Koch PA, Lau CC, Craig WA. Bioavailability of Tetracycline and Doxycycline in Fasted and Nonfasted Subjects. Antimicrob Agents Chemother (1977) 11(3):462–9. doi: 10.1128/AAC.11.3.462
50. Milano S, Arcoleo F, D’Agostino P, Cillari E. Intraperitoneal Injection of Tetracyclines Protects Mice From Lethal Endotoxemia Downregulating Inducible Nitric Oxide Synthase in Various Organs and Cytokine and Nitrate Secretion in Blood. Antimicrob Agents Chemother (1997) 41(1):117–21. doi: 10.1128/AAC.41.1.117
51. Chen X, Pi R, Liu M, Ma X, Jiang Y, Liu Y, et al. Combination of Methylprednisolone and Minocycline Synergistically Improves Experimental Autoimmune Encephalomyelitis in C57 BL/6 Mice. J Neuroimmunol (2010) 226(1-2):104–9. doi: 10.1016/j.jneuroim.2010.05.039
52. Giuliani F, Fu SA, Metz LM, Yong VW. Effective Combination of Minocycline and Interferon-Beta in a Model of Multiple Sclerosis. J Neuroimmunol (2005) 165(1-2):83–91. doi: 10.1016/j.jneuroim.2005.04.020
53. Yi C, Zhang Z, Wang W, Zug C, Schluesener HJ, Zhang Z. Doxycycline Attenuates Peripheral Inflammation in Rat Experimental Autoimmune Neuritis. Neurochem Res (2011) 36(11):1984–90. doi: 10.1007/s11064-011-0522-2
54. Kloppenburg M, Mattie H, Douwes N, Dijkmans BA, Breedveld FC. Minocycline in the Treatment of Rheumatoid Arthritis: Relationship of Serum Concentrations to Efficacy. J Rheumatol (1995) 22(4):611–6.
55. Kloppenburg M, Terwiel JP, Mallee C, Breedveld FC, Dijkmans BA. Minocycline in Active Rheumatoid Arthritis. A Placebo-Controlled Trial. Ann N Y Acad Sci (1994) 732:422–3. doi: 10.1111/j.1749-6632.1994.tb24773.x
56. Kloppenburg M, Brinkman BM, de Rooij-Dijk HH, Miltenburg AM, Daha MR, Breedveld FC, et al. The Tetracycline Derivative Minocycline Differentially Affects Cytokine Production by Monocytes and T Lymphocytes. Antimicrob Agents Chemother (1996) 40(4):934–40. doi: 10.1128/AAC.40.4.934
57. Zaguia F, Saikali P, Ludwin S, Newcombe J, Beauseigle D, McCrea E, et al. Cytotoxic NKG2C+ CD4 T Cells Target Oligodendrocytes in Multiple Sclerosis. J Immunol (2013) 190(6):2510–8. doi: 10.4049/jimmunol.1202725
58. Fujii C, Kondo T, Ochi H, Okada Y, Hashi Y, Adachi T, et al. Altered T Cell Phenotypes Associated With Clinical Relapse of Multiple Sclerosis Patients Receiving Fingolimod Therapy. Sci Rep (2016) 6:35314. doi: 10.1038/srep35314
Keywords: multiple sclerosis, doxycycline, minocycline, proinflammatory, interferon-γ, interleukin-17, NKT cells
Citation: Florou DT, Mavropoulos A, Dardiotis E, Tsimourtou V, Siokas V, Aloizou A-M, Liaskos C, Tsigalou C, Katsiari C, Sakkas LI, Hadjigeorgiou G and Bogdanos DP (2021) Tetracyclines Diminish In Vitro IFN-γ and IL-17-Producing Adaptive and Innate Immune Cells in Multiple Sclerosis. Front. Immunol. 12:739186. doi: 10.3389/fimmu.2021.739186
Received: 10 July 2021; Accepted: 16 September 2021;
Published: 26 November 2021.
Edited by:
Narendra Prasad Singh, University of South Carolina, United StatesReviewed by:
Nilesh C. Sharma, Western Kentucky University, United StatesHasan Alghetaa, University of Baghdad, Iraq
Copyright © 2021 Florou, Mavropoulos, Dardiotis, Tsimourtou, Siokas, Aloizou, Liaskos, Tsigalou, Katsiari, Sakkas, Hadjigeorgiou and Bogdanos. This is an open-access article distributed under the terms of the Creative Commons Attribution License (CC BY). The use, distribution or reproduction in other forums is permitted, provided the original author(s) and the copyright owner(s) are credited and that the original publication in this journal is cited, in accordance with accepted academic practice. No use, distribution or reproduction is permitted which does not comply with these terms.
*Correspondence: Dimitrios P. Bogdanos, Ym9nZGFub3NAbWVkLnV0aC5ncg==
†These authors have contributed equally to this work