- 1Department of Pathology, Stanford University School of Medicine, Stanford, CA, United States
- 2Stanford Blood Center, Palo Alto, CA, United States
- 3ATUM, Newark, CA, United States
- 4Sean N. Parker Center for Allergy and Asthma Research, Stanford, CA, United States
- 5Department of Medicine, Division of Infectious Diseases and Geographic Medicine, Stanford University, Stanford, CA, United States
- 6Chan Zuckerberg Biohub, San Francisco, CA, United States
- 7Department of Bioengineering, Stanford University, Stanford, CA, United States
- 8Department of Medicine, Division of Pulmonary, Allergy and Critical Care Medicine, Stanford University, Stanford, CA, United States
Background: Transfusion of COVID-19 convalescent plasma (CCP) containing high titers of anti-SARS-CoV-2 antibodies serves as therapy for COVID-19 patients. Transfusions early during disease course was found to be beneficial. Lessons from the SARS-CoV-2 pandemic could inform early responses to future pandemics and may continue to be relevant in lower resource settings. We sought to identify factors correlating to high antibody titers in convalescent plasma donors and understand the magnitude and pharmacokinetic time course of both transfused antibody titers and the endogenous antibody titers in transfused recipients.
Methods: Plasma samples were collected up to 174 days after convalescence from 93 CCP donors with mild disease, and from 16 COVID-19 patients before and after transfusion. Using ELISA, anti-SARS-CoV-2 Spike RBD, S1, and N-protein antibodies, as well as capacity of antibodies to block ACE2 from binding to RBD was measured in an in vitro assay. As an estimate for viral load, viral RNA and N-protein plasma levels were assessed in COVID-19 patients.
Results: Anti-SARS-CoV-2 antibody levels and RBD-ACE2 blocking capacity were highest within the first 60 days after symptom resolution and markedly decreased after 120 days. Highest antibody titers were found in CCP donors that experienced fever. Effect of transfused CCP was detectable in COVID-19 patients who received high-titer CCP and had not seroconverted at the time of transfusion. Decrease in viral RNA was seen in two of these patients.
Conclusion: Our results suggest that high titer CCP should be collected within 60 days after recovery from donors with past fever. The much lower titers conferred by transfused antibodies compared to endogenous production in the patient underscore the importance of providing CCP prior to endogenous seroconversion.
Highlights
High-titer convalescent plasma can be collected from low-severity outpatients with history of fever and typically within 60 days after symptom cessation. High-titer convalescent plasma should be adminstered to COVID-19 patients before endogenous seroconversion occurs.
Introduction
The COVID-19 pandemic is exacting a terrible toll on societies and health systems worldwide. Transfusion of COVID-19 convalescent plasma (CCP) containing anti-SARS-CoV-2 antibodies may have therapeutic benefit for COVID-19 patients until more efficacious therapeutics are widely available. CCP is also used as a source for purifying SARS-CoV-2-specific immunoglobulins for more standardized antibody treatment regimens (e.g. anti-coronavirus hyperimmune intravenous immunoglobulin). In the United States, vaccines and therapeutic monoclonal antibodies have been given emergency use authorization by the Food and Drug Administration (FDA), but logistical and financial limitations may limit the use of these interventions, especially in low- and middle-income countries, favoring the continued use of patient-derived antibody-based therapies such as CCP. It is therefore crucial to assess the magnitude and stability of serological responses in CCP donors and define an ideal timeframe for CCP donation. While some studies show SARS-CoV-2-specific B cells and detectable levels of SARS-CoV-2-specific antibodies for several months after infection (1–3), others have shown that antibody levels begin to decrease as early as one month after symptom onset, especially in less severely ill outpatients (4, 5). Although CCP efficacy in all COVID-19 patients is equivocal (6–8), recent studies suggest that high-titer CCP administered to patients early in disease course may be protective (9–12), a practice also recently recommended by the FDA (13). Since the majority of SARS-CoV-2-infected individuals, and hence also potential CCP donors, are mildly ill outpatients, we have sought to determine the patient characteristics associated with higher antibody titers in these individuals. Prior studies have lacked detailed time course data for analyzing the kinetics of antibodies derived from CCP in recipients, and for comparing the therapeutic antibody quantities to those derived from the patient’s own humoral immune response early during the disease course, to better understand the potential benefits of early transfusion.
Methods
Clinical Specimens
Venipuncture blood samples from 93 COVID-19 convalescent plasma (CCP) donors from the San Francisco Bay Area in California who donated CCP at Stanford Blood Center from 4/14/2020 to 8/25/2020, as well as from 16 COVID-19 patients admitted to Stanford Hospital were collected in sodium heparin- or K2EDTA-coated vacutainers and plasma was used for serology testing, N-antigenemia testing, and rRT-PCR detection of RNAemia. Plasma samples were stored at 4°C (short-term) or -80°C (long-term). For three transfused COVID-19 patients, the sampling timepoints were not ideal to assess whether the transfused CCP influenced the recipient’s plasma antibody levels (shown in Supplementary Figure 1). Retrospective chart review was performed on all COVID-19 patients admitted to Stanford Hospital. This study was approved by the Stanford University Institutional Review Board (Protocols IRB-48973, IRB-55689, and IRB-13952). All patients were transfused CCP as a part of the National Convalescent Plasma Expanded Access Protocol sponsored by the Mayo Clinic and approved by the Stanford University Institutional Review Board (Protocol IRB-56100).
ELISA to Detect Anti-SARS-CoV-2 Antibodies in Plasma Samples
The ELISA protocol used in the present study was described by Röltgen et al. (4). In brief, ELISA plates were coated with SARS-CoV-2 spike RBD, S1, or N protein at a concentration of 0.1 μg per well (0.025 μg per well for the N protein IgG assay). Plasma samples from CCP donors and COVID-19 patients were incubated at a dilution of 1:100 for 1 hour. Anti-SARS-CoV-2 IgA, IgG, and IgM antibodies were detected using HRP (horseradish peroxidase) conjugated goat anti-human IgG (γ-chain specific, catalog no. 62-8420, Thermo Fisher, 1:5,000 dilution), IgM (μ-chain specific, catalog no. A6907, Sigma, 1:5,000 dilution), or IgA (α-chain specific, catalog no. P0216, Agilent, 1:2,000 dilution). Development was done using 3,3′,5,5′-Tetramethylbenzidine (TMB) substrate and optical density (OD) at 450 nm was measured with a microplate reader and blank values were subtracted from values obtained for plasma samples. Seroconversion for each isotype/protein assay was defined as values above mean ELISA ODs of 94 negative control samples from healthy blood donors collected before the pandemic plus three times their standard deviation (mean + 3 SD). All samples were tested twice in independent experiments.
Competition ELISA to Detect Antibodies That Block Binding of ACE2 to RBD
The protocol for the competition ELISA procedure used here was recently described by Röltgen et al. (4) In brief, plates were coated with SARS-CoV-2 spike RBD protein and then incubated with plasma samples at a dilution of 1:10 for 1 hour at room temperature. Then, recombinant ACE2 joined to a mouse IgG2a Fc (ACE2-mFc) at 0.5 µg/mL was added to the plasma sample for another 45 minutes. After washing, RBD-ACE2-mFc was detected using horseradish peroxidase conjugated anti-mouse IgG. ELISA plates were developed and measured as described above. A positive and a negative quality control (Access SARS-CoV-2 IgG QC, QC1-QC2, catalog no. C58964, Beckman Coulter) was included on each plate. OD values were converted to ‘% ACE2 blocking’ using the following formula: % ACE2 blocking = 100*(1-(sample OD - 0.2)/(QC1 OD – 0.2)), taking into account the background noise of the assay of 0.2 which was determined by testing negative control plasma samples that were collected before the pandemic. All samples were tested two times in independent experiments.
Real-Time PCR for Detection of SARS-CoV-2 RNA in Plasma Samples
The protocol for detection of SARS-CoV-2 RNA in plasma was performed based on a published rRT-PCR assay targeting the envelope (E) gene (14, 15). RNA was isolated from 400 μL of EDTA-anticoagulated plasma using Qiagen EZ1 Virus Mini Kit v2.0 (Qiagen German-town, MD). Ct values of positive tests with this assay normally range from Ct <20 to 45 cycles. Testing of plasma samples with a Ct value of 40 or higher were tested again to ensure reproducibility of the positive result. No viral culture was performed as part of this study, therefore, presence of SARS-CoV-2 in tested plasma was defined as RNAemia.
Antigen Detection
SARS-CoV-2 nucleocapsid antigen was quantified using S-PLEX Direct Detection Assay, S-PLEX SARS-CoV-2 N Kit (Catalog #K150ADHS, Meso Scale Discovery [MSD], Rockville, MD), according to manufacturer’s protocol. Raw signal was converted to a concentration based on linear regression to the 7-point calibration curve. Cut off for positivity was calculated as the mean value of 40 pre-pandemic plasma samples plus three times the standard deviation.
Statistics
GraphPad Prism version 8.4.1 software (GraphPad Software, San Diego, California, USA) was used to visualize data, analyze for differences in antibody responses and N-antigenemia levels between different timepoints to carry out linear regression of % RBD-ACE2 blocking and antibody titers. Ordinary one-way ANOVA test and Kruskal-Wallis test with Dunn’s multiple comparison test was used to compare more than two groups when samples either followed, or did not follow Gaussian distribution, respectively. Unpaired t-test was used to compare IgG levels and % RBD-ACE2 blocking in samples from symptom positive versus negative patients, while no correction for multiple comparison was performed. Goodness of fit for linear regression analyses was reported as the coefficient of determination (R2). Correlation between antibody OD450 values, RNAemia, and RBD-ACE2 blocking assay OD450 values were calculated as Spearman correlations with the R cor function. Two-sided tests with p<0.05 were considered as statistically significant.
Results
Time After Recovery and Symptoms Correlate to Humoral Immune Response in Mildly Ill COVID-19 Convalescent Plasma Donors
We studied the SARS-CoV-2-specific humoral immune response in 172 CCP samples collected from 93 non-hospitalized outpatients (Table 1). In contrast to earlier studies (4, 5), samples were collected up to 174 days after convalescence. We measured IgM, IgA, and IgG levels in the plasma of these donors against the SARS-CoV-2 Spike S1 region, receptor binding domain (RBD) and nucleocapsid antigen (N) using laboratory-developed ELISAs. Anti-RBD titers decreased with time after symptom cessation (Figure 1A). Antibody levels were highest in CCP donations collected within two months after symptom resolution and were markedly decreased after 120 days (Figure 1B). Similarly, antibody titers waned with time for anti-S1 (Supplementary Figure 2). Analysis of individual donors with four or more donation timepoints clearly revealed that antibody signals consistently decreased over time (Supplementary Figure 3).
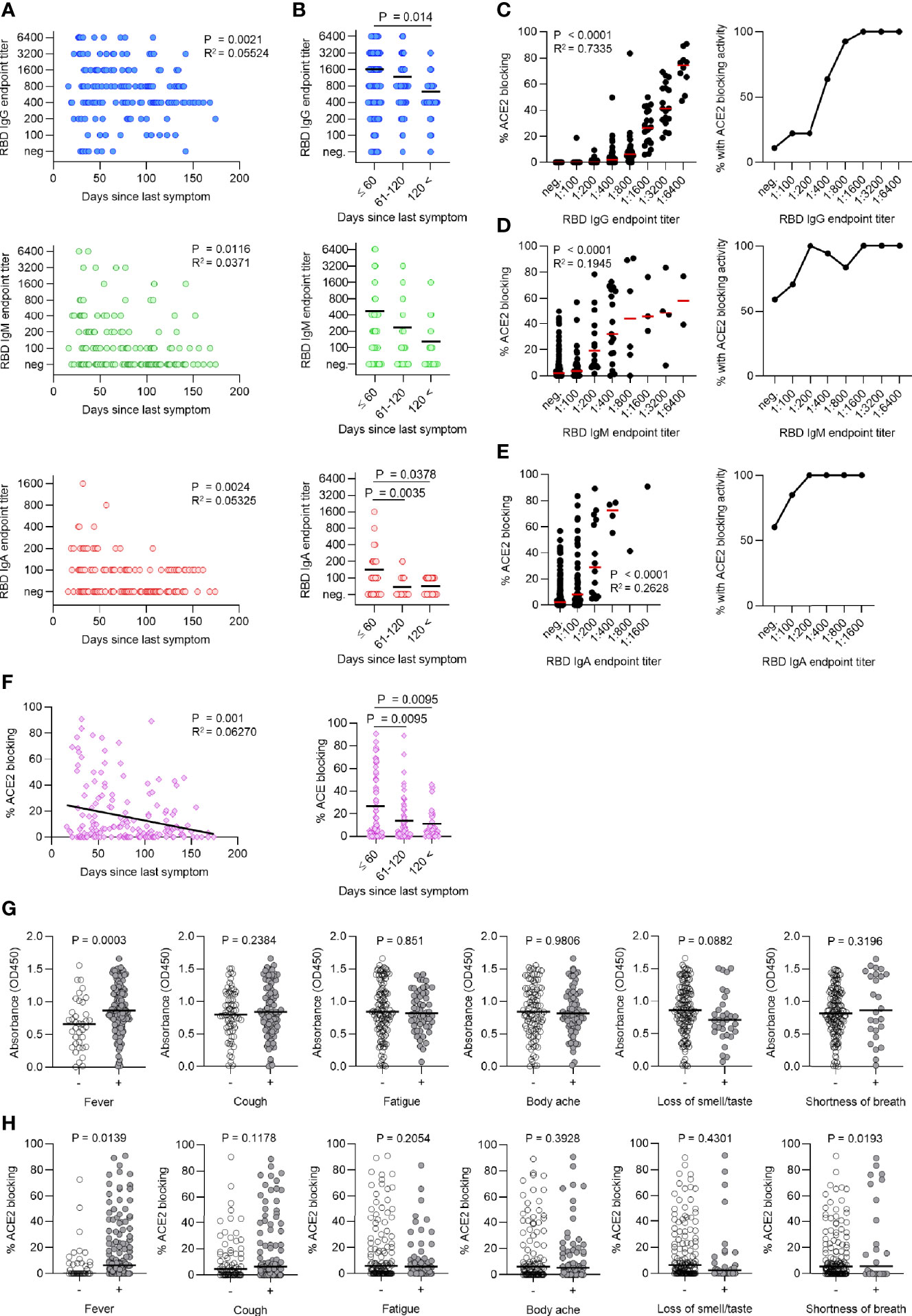
Figure 1 Time after recovery and symptoms correlate to humoral immune response in mildly ill COVID-19 convalescent plasma donors. (A) Titers of SARS-CoV-2 RBD-specific IgG, IgM, and IgA in the plasma of 93 COVID-convalescent plasma (CCP) donors (172 individual samples) decrease over time after symptom cessation. (B) Antibody titers begin to decrease after 120 days. (C–E) Titers of IgG (C), IgM (D), and IgA (E) were correlated to RBD-ACE2 blocking activity (in %, left panel). The percentage of cases with any detectable RBD-ACE2 blocking activity is shown for each titer (right panel). (F) RBD-ACE2 blocking capacity (in %) over time shown for all cases (left panel) and separated in bins of 60 days (right panel). (G, H) Comparison of RBD-specific IgG titers (G) (Absorbance, OD450) and RBD-ACE2 blocking activity (H) in symptom positive versus negative cases for most common reported symptoms.
Viral spike RBD interaction with human angiotensin-converting enzyme 2 (ACE2) initiates SARS-CoV-2 entry into host cells. We performed an RBD-ACE2 blocking ELISA to measure the functional activity of plasma antibodies to block RBD-ACE2 interaction. CCP donor anti-RBD IgG levels were positively correlated to RBD-ACE2 blocking capacity and all samples with titers of at least 1:1600 exhibited RBD-ACE2 blocking activity, while only a subset of samples with lower titers showed RBD-ACE2 blocking activity (Figure 1C). We also measured anti-RBD IgM and IgA titers, which showed weaker correlations to RBD-ACE2 blocking (Figures 1D, E). Similarly, RBD-ACE2 blocking capacity was significantly higher in CCP samples collected within 60 days post symptom (Figure 1F). Together, this further emphasized the importance of CCP donations early after recovery.
Identifying CCP donor factors associated with high antibody titers would contribute to more efficient donor recruitment strategies. We therefore explored whether certain symptoms reported in our cohort of mildly ill outpatients (Table 1) correlated with anti-RBD IgG levels (Figure 1G) and RBD-ACE2 blocking capacity (Figure 1H). Interestingly, fever was the only symptom that distinguished CCP donors with higher levels of anti-RBD IgG and RBD-ACE2 blocking activity (Figures 1G, H). Similarly, increased anti-S1 and anti-N IgG antibodies were found in patients with fever (data not shown).
SARS-CoV-2-Specific Antibody Levels, Viral N-Antigenemia and RNAemia in COVID-19 Patients Before and After Convalescent Plasma Therapy
Several reports showed the benefits of CCP transfusions at early times during the disease course for patients infected with SARS-CoV-2 (9–12, 16), likely because CCP was transfused before these patients seroconverted. We therefore aimed to understand the patients’ immune response at the time of transfusion, analyze potential immediate biological effect of CCP transfusions, and compare these to the endogenous response. To address this, we measured anti-SARS-CoV-2 antibodies, RBD-ACE2 blocking functional antibody levels, viral RNAemia and N-antigenemia in a group of 16 COVID-19 inpatients prior to CCP transfusion and daily for up to one week thereafter (Patient information, Table 2). Increases in antibody levels one day after CCP transfusion were observed in four COVID-19 patients who had not yet seroconverted and who received CCP units with high levels of specific IgG antibodies (Figure 2A). Anti-RBD IgG antibody titer increased immediately after the transfusion, followed by a plateau or slight decrease; we attribute this serological response to the CCP transfusion.
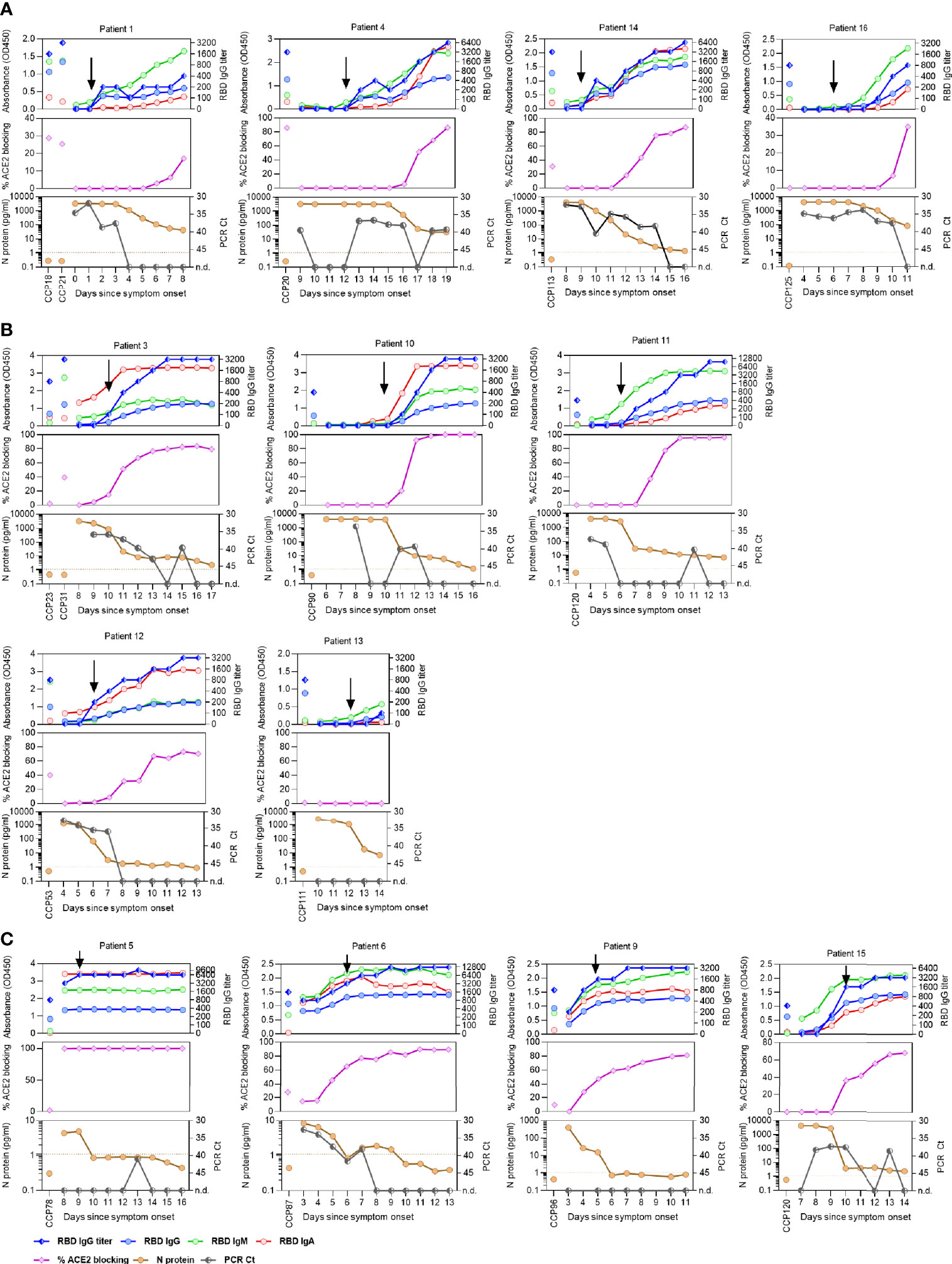
Figure 2 SARS-CoV-2-specific antibody titers, viral N-antigenemia and RNAemia in COVID-19 patients before and after convalescent plasma therapy. Absorbance level of SARS-CoV-2 RBD-specific IgG, IgM, and IgA (1:100 diluted plasma samples), titer of RBD-specific IgG, ACE2 blocking activity (in %), as well as levels of N-antigenemia, and RNAemia are shown for patients that received COVID-19 convalescent plasma (CCP) before (A), during (B), or after seroconversion (C). Timepoints of CCP transfusion are indicated by black arrows.
In contrast, CCP was transfused in nine patients either near the timepoint of anti-RBD IgG seroconversion (Figure 2B), or who already developed high antibody titers and RBD-ACE2 blocking activity (Figure 2C). Here, it is difficult to separate the serological effect of the transfused CCP from the patients’ own response. For three patients, the sampling timepoints were not suitable to assess whether the transfused CCP influenced the recipient’s plasma antibody levels (Supplementary Figure 1). Similar results were found when we measured titers of antibodies specific for Spike S1 region and N-antigen in these patients (Supplementary Figure 4). With the plasma dilution used in our experiment, two patients reached maximal RBD-ACE2 blocking activity between one and two weeks after symptom onset, as a result of their own serological response.
With the aim to assess an effect of the transfused plasma, N-antigen and viral RNA levels in the blood were measured to estimate viral load. N-antigenemia was found in 93.75% (15/16) and RNAemia in 75% (12/16) of patients (Figure 2, Supplementary Figure 1). Inversely correlating with their serological responses, N-antigenemia and RNAemia were reduced in all patients over the course of their illness (Figure 2, Supplementary Figure 1). Interestingly, two patients (1 and 14) who received CCP before seroconversion showed reduced RNAemia immediately following CCP transfusion (Figure 2A), potentially supporting efficacy of early CCP administration. Over the course of the study period, N-antigenemia was becoming undetectable for 40% (6/15) of the patients while RNAemia resolved in 75% (9/12) of previously positive patients. The persistence of low levels of declining viral RNA and protein in the blood of seroconverted patients could be due to the antibodies not yet having achieved the concentrations needed to fully bind and opsonize the remaining viral proteins in the body. Antibody titers negatively correlated to N-antigen levels in these patients (Supplementary Figure 5A) and level of N-antigen at timepoint of transfusion distinguished hospitalized patients where CCP was given before seroconversion (Supplementary Figure 5B).
Discussion
Here, we studied the immune response to SARS-CoV-2 infection in mildly ill outpatients that donated COVID-19 convalescent plasma (CCP). High titer plasma important for transfusion was mainly found within 60 days after symptom cessation and in patients that had fever. Furthermore, we analyzed whether transfused CCP can be detected and has a direct effect on viral antigens and viral RNA levels in 16 hospitalized COVID-19 patients. An effect was found only in those individuals that did not seroconvert yet.
Logistical and financial limitations may still limit the use of vaccines and therapeutic monoclonal antibodies, especially in low- and middle-income countries, favoring the continued use of patient-derived antibody-based therapies such as CCP. Here, we aimed to identify donor factors associated with high antibody titers to improve CCP donor recruitment strategies. For this, we first assessed titers of SARS-CoV-2 Spike-RBD, -S1, and N-specific antibodies in relation to symptom cessation.
Antibody titers continuously waned after symptoms ended with most marked decrease after 120 days. Our data indicated that CCP collected within 60 days after symptom resolution is most likely to maximize antibody levels for transfusion. While we studied mildly ill outpatient which make up the majority of SARS-CoV-2 infected individuals and hence potential blood donors, a similar timeframe for collecting high titer plasma was also suggested for more severely ill patients (17). In addition, we also measured the functional activity of antibodies to block RBD-ACE2 interaction. Results from the here used RBD-ACE2 blocking assay closely correlate with a SARS-CoV-2 pseudotyped virus neutralization assay (4). RBD-ACE2 blocking activity was found in all plasma units with an anti-RBD IgG titer of at least 1:1600. This is concordant with a recent study where similar IgG titers were associated with efficient virus neutralization (5). Since anti-RBD antibodies of IgM and IgA isotypes showed weaker correlation to RBD-ACE2 blocking activity, we concluded that anti-RBD IgG titers were the best correlate for virus-neutralizing activity.
In our donor cohort consisting of mildly ill outpatients, we found that fever was the only symptom correlating to higher antibody levels. While dyspnea was relatively rare among our studied cohort (19.4%), a recent study including CCP donors that were more severely ill found increased antibody levels among patients with dyspnea (5).
Several trials have studied the benefit of high titer CCP transfusions on COVID-19 outcome with median duration of symptoms at day of transfusion ranging from 3 to 30 days (10, 18–20). The importance of transfusions at early times during the disease course has been noted for patients infected with SARS-CoV-2 (9–12, 16), and also for SARS-CoV (21). The benefits from CCP transfusion are likely to be greatest for patients who have not yet seroconverted, if the patient’s endogenous neutralizing antibody response is greater in magnitude than the transfused antibody quantity. It is therefore critically important to understand the patients’ immune response at the time of transfusion, analyze the immediate biological effect of CCP transfusions, and compare these to the endogenous response. Sampling COVID-19 patients before and daily up to one week after the CCP transfusion allowed to detect transfused antibodies in four of the studied COVID-19 patients that did not seroconvert yet. At later times afterwards, a rapid increase in antibody levels was seen, very likely reflecting the patients’ own endogenous antibody production and seroconversion. At the same time when we observed the endogenous antibody increase, N-antigenemia and RNAemia resolved in most patients. While the four patients who received CCP before seroconversion recovered from COVID-19, our analysis was not designed to evaluate the efficacy of CCP transfusions. These findings, however, are in line with previous studies showing reduced fatal disease outcomes when CCP was administered early after symptom onset and before seroconversion (10, 11, 16, 21). In contrast, little clinical effect was seen when CCP was transfused more than 14 days after symptom onset (12, 18, 22, 23), likely because at this timepoint the patients already seroconverted with high antibody levels (4). The data are consistent with a role for early CCP administration as a bridging therapy until the patient mounts their own humoral immune response. Standardized serological testing, as opposed to temporal assessment of symptomatology, would be a more mechanistically supported approach to determine patient eligibility for CCP administration.
Limitations of this study include the relatively low number of CCP-treated COVID-19 patients and non-seroconverted patients at time of transfusion. Because most patients seroconvert during infection, the small volume of a unit of CCP (200-300 ml) compared to total plasma volume of patients make it difficult to detect increases in specific antibodies after transfusion in seroconverted patients. We note that we studied outpatient CCP donors, who may have had lower antibody levels compared to inpatients (4).
In this study, we demonstrated that anti-SARS-CoV-2 antibody levels and RBD-ACE2 blocking ability in plasma from outpatient donors were highest within the first two months after symptom resolution, strongly favoring CCP collection early after donor recovery. Donors who had fever during infection had elevated anti-SARS-CoV-2 antibody levels; this criterion may help CCP donor outreach strategies to identify donors with high antibody levels. We showed that increased antibody levels after CCP transfusion were only detected in patients who had not seroconverted at the time of administration, providing a mechanistic basis that could explain why the clinical benefit of CCP therapy appears to be greatest in recipients who are treated soon after symptom onset. In our view, transfusion prior to the patient’s own seroconversion should be considered the relevant clinical goal, informed by rapid serological testing in evaluating the potential benefit of convalescent plasma transfusion in individual patients. This study was performed before the widespread occurrence of viral variants. As new variants continue to emerge, the inter- and intra-strain effectiveness of CCP transfusion should be assessed. Further efforts should be directed at studying the efficacy of CCP administration in COVID-19 patients who have already seroconverted but are still early in the disease course. Use of CCP in immunocompromised patients warrants further study, as this group may stand to benefit the most from the treatment (24, 25).
Data Availability Statement
The original contributions presented in the study are included in the article/Supplementary Material. Further inquiries can be directed to the corresponding authors.
Ethics Statement
The studies involving human participants were reviewed and approved by Stanford University Institutional Review Board. Written informed consent for participation was not required for this study in accordance with the national legislation and the institutional requirements.
Author Contributions
OW, TP, and SB conceptualized and designed the study. OW, KR, MS, MV, and KN performed the experiments. OW, KR, BS, SP, MS, LT, MV, KN, TS, and AS collected data and/or contributed samples/reagents or EHR processing methods. CB, JC, JZ, KN, and BP provided intellectual contributions throughout the study. OW and TP performed statistical analyses. OW, TP, and SB analyzed the data. OW, KR, TP, and SB wrote the manuscript. All authors contributed to the article and approved the submitted version.
Funding
This work was supported by NIH/NIAID R01AI127877 (SB), NIH/NIAID R01AI130398 (SB), NIH 1U54CA260517 (SB), an endowment to S.D.B. from the Crown Family Foundation, an Early Postdoc. Mobility Fellowship Stipend to OW from the Swiss National Science Foundation (SNSF), and a Coulter COVID-19 Rapid Response Award to JC and SB. This work was also supported by MesoScale Diagnostics (MSD), who provided the S-PLEX SARS-CoV-2 N Kits, BioTek 405 Select automated 96-well plate washer, and MESO® SECTOR S 600 Reader used in this study. MSD had no role in study design, data collection and analysis, decision to publish, or preparation of the manuscript.
Conflict of Interest
SB has consulted for Regeneron, Sanofi, and Novartis on topics unrelated to this study, and owns stock in AbCellera. KN reports grants from National Institute of Allergy and Infectious Diseases (NIAID), Food Allergy Research & Education (FARE), End Allergies Together (EAT); National Heart, Lung, and Blood Institute (NHLBI), and National Institute of Environmental Health Sciences (NIEHS). KN is Director of the FARE and World Allergy Organization (WAO) Center of Excellence at Stanford University; Advisor at Cour Pharmaceuticals; Cofounder of Before Brands, Alladapt, Latitude, and IgGenix; National Scientific Committee member for the Immune Tolerance Network (ITN) of NIAID; recipient of a Research Sponsorship from Nestle; Consultant and Advisory Board Member at Before Brands, Alladapt, IgGenix, NHLBI, and ProBio; and Data and Safety Monitoring Board member at NHLBI. CB is on the board of Catamaran Bio. Author MH was employed by company ATUM.
The remaining authors declare that the research was conducted in the absence of any commercial or financial relationships that could be construed as a potential conflict of interest.
Publisher’s Note
All claims expressed in this article are solely those of the authors and do not necessarily represent those of their affiliated organizations, or those of the publisher, the editors and the reviewers. Any product that may be evaluated in this article, or claim that may be made by its manufacturer, is not guaranteed or endorsed by the publisher.
Acknowledgments
The authors thank Dana Anderson for helpful advice about protein production, and the ATUM Bio team for optimization, production and providing of antigens.
Supplementary Material
The Supplementary Material for this article can be found online at: https://www.frontiersin.org/articles/10.3389/fimmu.2021.739037/full#supplementary-material
Supplementary Figure 1 | Measurements in three additional COVID-19 patients. Titers of SARS-CoV-2 RBD-specific IgG, IgM, and IgA, RBD-specific IgG titers, RBD-ACE2 blocking activity (in %), as well as levels of N-antigenemia, and RNAemia are shown for three patients for whom available sample timepoints were not suitable to assess whether the patients had seroconverted before CCP transfusion. Timepoint(s) of CCP transfusion are indicated by black arrows.
Supplementary Figure 2 | Titers of SARS-CoV-2 RBD-specific, S1-specific and N-specific IgG, IgM, and IgA antibodies. Titers of SARS-CoV-2 RBD-specific, S1-specific and N-specific IgG, IgM, and IgA antibodies (Absorbance at OD450) plotted versus timepoint post symptom cessation.
Supplementary Figure 3 | Titers of SARS-CoV-2 RBD-specific, S1-specific and N-specific IgG, IgM, and IgA antibodies for individual donors with multiple timepoints. Titers of SARS-CoV-2 RBD-specific, S1-specific and N-specific IgG, IgM, and IgA antibodies (Absorbance at OD450) plotted versus timepoint post symptom cessation for a selection of donors with > 3 samples.
Supplementary Figure 4 | SARS-CoV-2 S1/N-specific antibody titers in COVID-19 patients who received convalescent plasma. Levels of SARS-CoV-2 S1/N-specific IgG, IgM, and IgA antibodies (Absorbance at OD450) are shown for patients who received COVID-convalescent plasma (CCP) before (A), during (B), or after seroconversion (C). (D) Shows three patients for whom available sample timepoints were not suitable to assess if patients already seroconverted before CCP transfusion. Timepoint(s) of CCP transfusion indicated by black arrow.
Supplementary Figure 5 | N-antigenemia levels negatively correlate with developing immune response. (A) Viral N-antigenemia levels for all samples from COVID-19 patients correlated with (from left to right) RBD-specific IgG titers, RBD-specific IgG Absorbance OD450, N-specific IgG Absorbance OD450, and RBD-ACE2 blocking capacity. Reduced N-antigenemia in samples correlated with developing immune response. (B) Levels of N-antigenemia in collected plasma from COVID-19 patients distinguished patients that received CCP before, during or after seroconversion.
References
1. Wajnberg A, Amanat F, Firpo A, Altman DR, Bailey MJ, Mansour M, et al. Robust Neutralizing Antibodies to SARS-CoV-2 Infection Persist for Months. Science (2020) 370(6521):1227–30. doi: 10.1126/science.abd7728
2. Dan JM, Mateus J, Kato Y, Hastie KM, Yu ED, Faliti CE, et al. Immunological Memory to SARS-CoV-2 Assessed for Up to 8 Months After Infection. Science (2021) 371(6529):eabf4063. doi: 10.1126/science.abf4063
3. Ogega CO, Skinner NE, Blair PW, Park HS, Littlefield K, Ganesan A, et al. Durable SARS-CoV-2 B Cell Immunity After Mild or Severe Disease. J Clin Invest (2021) 131(7):e145516. doi: 10.1172/JCI145516
4. Röltgen K, Powell AE, Wirz OF, Stevens BA, Hogan CA, Najeeb J, et al. Defining the Features and Duration of Antibody Responses to SARS-CoV-2 Infection Associated With Disease Severity and Outcome. Sci Immunol (2020) 5:eabe0240. doi: 10.1126/sciimmunol.abe0240
5. Salazar E, Kuchipudi SV, Christensen PA, Eagar T, Yi X, Zhao P, et al. Convalescent Plasma Anti-SARS-CoV-2 Spike Protein Ectodomain and Receptor-Binding Domain Igg Correlate With Virus Neutralization. J Clin Invest (2020) 130:6728–38. doi: 10.1172/JCI141206
6. Casadevall A, Joyner MJ, Pirofski L-A. A Randomized Trial of Convalescent Plasma for COVID-19 - Potentially Hopeful Signals. JAMA (2020) 324:455–7. doi: 10.1001/jama.2020.10218
7. Correction to Data in Trial of Convalescent Plasma Treatment for COVID-19. Jama (2020) 324:519. doi: 10.1001/jama.2020.13216
8. Gharbharan A, Jordans CCE, Geurtsvankessel C, den Hollander JG, Karim F, Mollema FPN, et al. Effects of potent neutralizing antibodies from convalescent plasma in patients hospitalized for severe SARS-CoV-2 infection. Nat Commun. (2021) 12(1):3189. doi: 10.1038/s41467-021-23469-2
9. Salazar E, Christensen PA, Graviss EA, Nguyen DT, Castillo B, Chen J, et al. Significantly Decreased Mortality in a Large Cohort of Coronavirus Disease 2019 (COVID-19) Patients Transfused Early With Convalescent Plasma Containing High-Titer Anti-Severe Acute Respiratory Syndrome Coronavirus 2 (SARS-CoV-2) Spike Protein Igg. Am J Pathol (2021) 191:90–107. doi: 10.1016/j.ajpath.2020.10.008
10. Libster R, Pérez Marc G, Wappner D, Coviello S, Bianchi A, Braem V, et al. Early High-Titer Plasma Therapy to Prevent Severe Covid-19 in Older Adults. N Engl J Med (2021) 384(7):610–8. doi: 10.1056/NEJMoa2033700
11. Joyner MJ, Senefeld JW, Klassen SA, Mills JR, Johnson PW, Theel ES, et al. Effect of Convalescent Plasma on Mortality Among Hospitalized Patients With COVID-19: Initial Three-Month Experience. medRxiv (2020). 2020.08.12.20169359. doi: 10.1101/2020.08.12.20169359
12. Xia X, Li K, Wu L, Wang Z, Zhu M, Huang B, et al. Improved Clinical Symptoms and Mortality Among Patients With Severe or Critical COVID-19 After Convalescent Plasma Transfusion. Blood (2020) 136:755–9. doi: 10.1182/blood.2020007079
13. The U.S. Food and Drug Administration (FDA). EUA Fact Sheet for Health Care Providers COVID-19 Convalescent Plasma (2021). Available at: https://www.fda.gov/news-events/fda-brief/fda-brief-fda-updates-emergency-use-authorization-covid-19-convalescent-plasma-reflect-new-data (Accessed 18 February 2021).
14. Hogan CA, Sahoo MK, Pinsky BA. Sample Pooling as a Strategy to Detect Community Transmission of SARS-CoV-2. JAMA (2020) 323:1967–9. doi: 10.1001/jama.2020.5445
15. Corman VM, Landt O, Kaiser M, Molenkamp R, Meijer A, Chu DK, et al. Detection of 2019 Novel Coronavirus (2019-nCoV) by Real-Time RT-PCR. Euro Surveill (2020) 25:2000045. doi: 10.2807/1560-7917.ES.2020.25.3.2000045
16. Hegerova L, Gooley TA, Sweerus KA, Maree C, Bailey N, Bailey M, et al. Use of Convalescent Plasma in Hospitalized Patients With COVID-19: Case Series. Blood (2020) 136:759–62. doi: 10.1182/blood.2020006964
17. Gontu A, Srinivasan S, Salazar E, Nair MS, Nissly RH, Greenawalt D, et al. Limited Window for Donation of Convalescent Plasma With High Live-Virus Neutralizing Antibody Titers for COVID-19 Immunotherapy. Commun Biol (2021) 4:267. doi: 10.1038/s42003-021-01813-y
18. Li L, Zhang W, Hu Y, Tong X, Zheng S, Yang J, et al. Effect of Convalescent Plasma Therapy on Time to Clinical Improvement in Patients With Severe and Life-Threatening COVID-19: A Randomized Clinical Trial. JAMA (2020) 324:1–11. doi: 10.1001/jama.2020.10044
19. Agarwal A, Mukherjee A, Kumar G, Chatterjee P, Bhatnagar T, Malhotra P. Convalescent Plasma in the Management of Moderate Covid-19 in Adults in India: Open Label Phase II Multicentre Randomised Controlled Trial (PLACID Trial). BMJ (2020) 371:m3939. doi: 10.1136/bmj.m3939
20. Simonovich VA, Burgos Pratx LD, Scibona P, Beruto MV, Vallone MG, Vázquez C, et al. A Randomized Trial of Convalescent Plasma in Covid-19 Severe Pneumonia. N Engl J Med (2021) 384:619–29. doi: 10.1056/NEJMoa2031304
21. Cheng Y, Wong R, Soo YO, Wong WS, Lee CK, Ng MH, et al. Use of Convalescent Plasma Therapy in SARS Patients in Hong Kong. Eur J Clin Microbiol Infect Dis (2005) 24:44–6. doi: 10.1007/s10096-004-1271-9
22. Duan K, Liu B, Li C, Zhang H, Yu T, Qu J, et al. Effectiveness of Convalescent Plasma Therapy in Severe COVID-19 Patients. Proc Natl Acad Sci USA (2020) 117:9490–6. doi: 10.1073/pnas.2004168117
23. Zeng QL, Yu ZJ, Gou JJ, Li GM, Ma SH, Zhang GF, et al. Effect of Convalescent Plasma Therapy on Viral Shedding and Survival in Patients With Coronavirus Disease 2019. J Infect Dis (2020) 222:38–43. doi: 10.1093/infdis/jiaa228
24. Fung M, Nambiar A, Pandey S, Aldrich JM, Teraoka J, Freise C, et al. Treatment of Immunocompromised COVID-19 Patients With Convalescent Plasma. Transpl Infect Dis (2020) 23:e13477. doi: 10.1111/tid.13477
Keywords: SARS-CoV-2, COVID-19, convalescent plasma for COVID-19 therapy, humoral immune response, antiviral antibodies
Citation: Wirz OF, Röltgen K, Stevens BA, Pandey S, Sahoo MK, Tolentino L, Verghese M, Nguyen K, Hunter M, Snow TT, Singh AR, Blish CA, Cochran JR, Zehnder JL, Nadeau KC, Pinsky BA, Pham TD and Boyd SD (2021) Use of Outpatient-Derived COVID-19 Convalescent Plasma in COVID-19 Patients Before Seroconversion. Front. Immunol. 12:739037. doi: 10.3389/fimmu.2021.739037
Received: 09 July 2021; Accepted: 24 August 2021;
Published: 14 September 2021.
Edited by:
Raymund Razonable, Mayo Clinic, United StatesCopyright © 2021 Wirz, Röltgen, Stevens, Pandey, Sahoo, Tolentino, Verghese, Nguyen, Hunter, Snow, Singh, Blish, Cochran, Zehnder, Nadeau, Pinsky, Pham and Boyd. This is an open-access article distributed under the terms of the Creative Commons Attribution License (CC BY). The use, distribution or reproduction in other forums is permitted, provided the original author(s) and the copyright owner(s) are credited and that the original publication in this journal is cited, in accordance with accepted academic practice. No use, distribution or reproduction is permitted which does not comply with these terms.
*Correspondence: Scott D. Boyd, sboyd1@stanford.edu; Tho D. Pham, thopham@stanford.edu
†These authors have contributed equally to this work