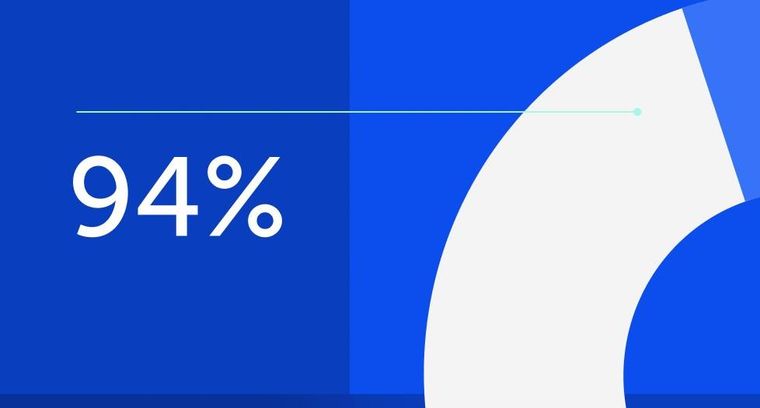
94% of researchers rate our articles as excellent or good
Learn more about the work of our research integrity team to safeguard the quality of each article we publish.
Find out more
ORIGINAL RESEARCH article
Front. Immunol., 28 October 2021
Sec. Alloimmunity and Transplantation
Volume 12 - 2021 | https://doi.org/10.3389/fimmu.2021.735564
This article is part of the Research TopicImmune Regulations in Reproductive Organs and Organ TransplantView all 34 articles
Pregnancy after renal transplantation is associated with an increased risk of complications. While a delicately balanced uterine immune system is essential for a successful pregnancy, little is known about the uterine immune environment of pregnant kidney transplant recipients. Moreover, children born to kidney transplant recipients are exposed in utero to immunosuppressive drugs, with possible consequences for neonatal outcomes. Here, we defined the effects of kidney transplantation on the immune cell composition during pregnancy with a cohort of kidney transplant recipients as well as healthy controls with uncomplicated pregnancies. Maternal immune cells from peripheral blood were collected during pregnancy as well as from decidua and cord blood obtained after delivery. Multiparameter flow cytometry was used to identify and characterize populations of cells. While systemic immune cell frequencies were altered in kidney transplant patients, immune cell dynamics over the course of pregnancy were largely similar to healthy women. In the decidua of women with a kidney transplant, we observed a decreased frequency of HLA-DR+ Treg, particularly in those treated with tacrolimus versus those that were treated with azathioprine next to tacrolimus, or with azathioprine alone. In addition, both the innate and adaptive neonatal immune system of children born to kidney transplant recipients was significantly altered compared to neonates born from uncomplicated pregnancies. Overall, our findings indicate a significant and distinct impact on the maternal systemic, uterine, and neonatal immune cell composition in pregnant kidney transplant recipients, which could have important consequences for the incidence of pregnancy complications, treatment decisions, and the offspring’s health.
Achieving successful pregnancy in women with advanced chronic kidney disease or end-stage renal disease is clinically challenging (1, 2). Renal transplantation is the treatment of choice for most of these patients, especially in women of childbearing age as renal transplantation greatly improves fertility and the ability to conceive (3–5). As a consequence, the number of pregnancies in patients with a kidney transplant is rising (6). Unfortunately, kidney transplant recipients have a higher risk of developing pregnancy complications (5). For instance, preeclampsia occurs in about one-third (21-38%) of the pregnant kidney transplant recipients, while the risk of preeclampsia in the general population is only 3-5% (3, 5–7). Low birth weight children (< 2500 g; 50%) and preterm delivery (< 37 weeks of gestation; 50%) is also more common in kidney transplant recipients compared to the general population (5, 6, 8). These adverse pregnancy outcomes may be a result of impaired (pre-pregnancy) graft function, pre-pregnancy hypertension, or the effect of immunosuppression (5, 9–11). Conversely, delicately balanced immune dynamics, both in the uterus and systemically, are essential for a pregnancy to be successful (12–16). Immune perturbations associated with pregnancy complications are well-documented and may offer an explanation to the higher incidence of complications observed in kidney transplant recipients (16–19).
In addition, the immunosuppressive drug tacrolimus accumulates in the placenta of women with a kidney transplant (20), while azathioprine, tacrolimus, and prednisone are able to cross the placenta and enter the fetal circulation (8). Although the use of kidney transplantation medication has not been directly linked to increased incidence of major congenital malformations (8, 21), limited evidence suggests that in utero drug exposure impacts the development of the neonatal immune system. For instance, infants born to kidney transplant recipients had reduced B cell numbers at birth and transplantation itself was associated with an increased risk for hospital admission in the first months of life (22).
In order to investigate the local uterine immune system and systemic immune signatures, we used multiparameter flow cytometry to phenotypically characterize maternal immune cells derived from the decidua after delivery as well as peripheral blood immune cells collected over the course of pregnancy from kidney transplant recipients and healthy individuals with uncomplicated pregnancies. In parallel, we analyzed immune cells in the cord blood of neonates in order to assess the development of the neonatal immune system.
Systemic maternal immune dynamics in kidney transplant recipients largely followed a similar dynamic profile over the course of pregnancy compared to pregnancy in healthy individuals. In addition, we show for the first time that the uterine HLA-DR+ regulatory T cell frequencies are affected in women with a kidney transplant, particularly in those treated with tacrolimus. This may suggest that the choice of immunosuppression could influence the risk for the development of complications differently. Compared to healthy controls, reduced regulatory T cell, B cell, NKT-like cell frequencies, and altered monocyte composition was observed at birth in the offspring of kidney transplant recipients, which could have consequences for the offspring’s health outcomes.
19 pregnant women having a healthy, uncomplicated pregnancy (HC) were recruited at the Radboud university medical center, and 14 pregnant women with a kidney transplant (KT) were enrolled at the Radboud university medical center and University Medical Center Utrecht in the Netherlands. 5 KT recipients developed pre-eclampsia during their pregnancy (Table 1). Pre-pregnancy kidney function, reported as the average of last 4 measurements before pregnancy, did not differ for those developing pre-eclampsia (Table 1). Tacrolimus trough levels did not differ between those receiving “Tacrolimus” or “Tacrolimus+Azathioprine” (data not shown) We collected cord blood (venipuncture of umbilical vein; EDTA tubes), maternal blood during pregnancy, and placentae after delivery. All KT recipients were required to be >1 year post-transplantation with stable graft function before pregnancy could be pursued.
First trimester material – as used in in vitro cultures – was obtained from discarded uterine tissue after elective pregnancy termination, upon written consent. No further clinical information was obtained from these donors.
This study was approved by the local review board (Commissie Mensgebonden onderzoek region Arnhem-Nijmegen; CMO nr. 2014-232 and CMO nr. 2017-3253). In accordance with the Dutch Medical Research Involving Human Subject Act (WMO), all participants provided written informed consent before material was donated and included in this study.
One ml of blood was lysed with 25 ml lysis buffer (NH4CL + KHCO3/Na4EDTA diluted in H2O) for 10 minutes and washed 3x times with PBS. Lysed blood samples were used when only surface staining was performed. For intracellular staining protocol, lymphocytes were isolated by density gradient centrifugation (Lymphoprep, Axis-Shield PoC AS). After centrifugation (801 x g, 15 minutes, no brake), the lymphocyte layer was collected and isolated cells were washed twice with PBS before further analysis.
Decidua parietalis was collected from the obtained term placentae as previously described (13). After removing the amnion, the decidua parietalis (i.e. maternal layer of the placental membranes surrounding the fetus) was carefully scraped from the chorionic trophoblast layer. First trimester decidual tissue was separated from villous tissue. The tissue was washed thoroughly with PBS, minced with scissors and washed again until the supernatant became clear. The tissue was incubated with 0.2% collagenase (Gibco Life Technologies) and 0.04% DNAse (Roche Diagnostics) in a water bath at 37°C while shaking. After 60 minutes, digested tissue was washed with supplemented RPMI (RPMI 1640 medium supplemented with 1 mM pyruvate, 2 mM glutamax, 100 U/ml penicillin, and 100 mg/ml streptomycin; Thermo Fischer) and passed through a 100 µm, 70 µm, and 40 µm cell strainer (Greiner). Lymphocytes were obtained after density gradient centrifugation (801 x g for 15 minutes, no brake) on a discontinuous Percoll gradient (1,050 g/ml, 1,056 g/ml and 1,084 g/ml; GE Healthcare). Lymphocytes were isolated from the 1,084-1,056 g/ml interface.
100.000 freshly isolated uterine lymphocytes were cultured with anti-CD3/CD28 microbead stimulation (1:2 bead-to-cell ratio) in the absence or presence of azathioprine (6-mercaptopurine; active metabolite of azathioprine) or tacrolimus (FK506), in 10% HPS culture media (RPMI 1640 medium supplemented with 1 mM pyruvate, 2 mM glutamax, 100 U/ml penicillin, 100 mg/ml streptomycin, and 10% Human Pooled Serum) in a 96-well U-bottom plate for 5 days at 37°C in a humidified 5% CO2 incubator. Azathioprine and tacrolimus concentrations were chosen based on in vivo serum levels. Serum levels for mercaptopurine (azathioprine) range 235-500 pmol per 8x108 red blood cells (communication with nephrologist) in adult renal transplant patients after oral administration, with a maximum serum concentration of 50 ng/ml after oral administration (23). Tacrolimus serum levels range 5-6 ng/ml (communication with nephrologist) in adult renal transplant patients, with serum concentration of 5-15 ng/ml reported during pregnancy (24). The influence of these immunosuppressive drugs on cytokine expression and regulatory T cell frequency was assessed by flow cytometry.
Supplemental Table 1 lists the fluorochrome-conjugated monoclonal antibodies that were used to phenotypically characterize immune cells in cord blood, peripheral blood, uterine samples, and in vitro assays. Samples were analyzed on a 10-color Navios™ flow cytometer (480 nm argon blue laser, 405 nm solid state violet laser, 636 nm solid state laser, Beckman Coulter). In brief, cells were washed twice with PBS-0.2%BSA (bovine serum albumin, Sigma-Aldrich) before staining with surface antibodies for 20 minutes at room temperature, protected from light. After permeabilization and fixation, intracellular staining was performed for 30 minutes at 4°C in the dark. For intracellular cytokine expression, cells were first stimulated for 4 hours with PMA (phorbol-12-myristate-13-acetate; 12.5 ng/ml), ionomycin (500 ng/ml), and brefeldin A (5 μg/ml) at 37°C in a humidified 5% CO2 incubator. IFN-γ and IL-17 were used as proxy cytokines for pro-inflammatory Th1 and Th17 cell subsets respectively. 28 immune cell subpopulations (Figure S1) were identified in uterine, peripheral blood, and cord blood samples by manual gating using Kaluza software v2.1 (Beckman Coulter). The gating strategy is illustrated in Figure S1.
GraphPad Prism was used to perform statistical analysis. For comparisons of 2 groups, non-parametric Mann-Whitney test was used to compare immune cell subsets in maternal blood, decidua, and blood of the control (HC) and transplanted group (KT). For comparison of multiple groups, non-parametric Kruskal-Wallis test was used, where p-values were calculated against the HC group with a post-hoc Dunn test. A simple linear regression was performed for data in Figures S3A, S8A to test whether the slope of the regression lines is significantly different. P-values < 0.05 are considered significant. Boxplots and percentages in text are depicted as median with [interquartile range].
To investigate the local uterine immune environment in women with a kidney transplant, we collected the decidua parietalis from placentae (after delivery) of kidney transplant recipients and healthy, uncomplicated pregnancies. The frequency of monocytes, NK cells, B cells/subsets, T cells/subsets [regulatory T cells (Treg), effector/memory T cells (CD45RA and CCR7 expression (13)], and cytokine expressing T cells (IFN-γ and IL-17) was examined by multiparameter flow cytometry (Figure S1A: gating strategy and assessed immune cell subpopulations).
We observed no difference in frequency for monocytes, NK cells, T cell subsets, and B cell subsets in the decidua of kidney transplant recipients compared to healthy individuals (Figure S2). In addition, no difference in percentage IFN-γ+ and IL-17+ CD4+ T cells in the decidua was observed, representing pro-inflammatory Th1 and Th17 cells respectively (Figure 1A). While we did not observe a difference in CD25+CD127lowFOXP3+ Treg frequency (Figure 1B), we did observe a significant decrease in the percentage of HLA-DR+ Treg within the total uterine Treg pool of kidney transplant recipients compared to healthy individuals (Figure 1C). Importantly, to exclude that this difference was due to a difference in gestational age at delivery (Table 1), HLA-DR+ Treg frequency was plotted against gestational age at delivery with a linear regression (Figure S3A). This showed that no significant difference was observed for the linear regression slopes, indicating that uterine HLA-DR+ Treg frequencies in kidney transplant recipients behave similarly over the course of pregnancy. This suggests that the decreased frequency is inherent to the kidney transplant environment, and likely not due to a difference in gestational age. Interestingly, the greatest decrease in percentage HLA-DR+ Treg could be observed for those kidney transplant recipients that used tacrolimus (without azathioprine); 44.2 [21.4]% versus 74.8 [8.9]% in controls (Figure 1C). Interestingly, this decrease in HLA-DR+ Treg was less when azathioprine was added to the drug regimen (63.3 [30.2]%), or when tacrolimus was not used at all (56.2 [34.8]%; azathioprine only). In addition, the bimodal distribution observed for NK, T, and B cell frequencies in Figure S2 is likely due to a difference in drug regimen as well (Figure S3B). This shows that different immunosuppressive drugs could have distinct effects on uterine immunity.
Figure 1 Frequency of HLA-DR+ regulatory T cells is affected in decidua of kidney transplant recipients (KT). (A) Percentage IFN-γ+ and IL-17+ CD4+ T cells, (B) percentage CD25+CD127lowFOXP3+ regulatory T cells (Treg) and (C) percentage HLA-DR+ Treg in decidua from KT and healthy individuals (HC) are shown. Percentage HLA-DR+ Treg is separated based on which combination of tacrolimus (Tacro) and azathioprine (Aza) is used.
Pregnancy complications such as preeclampsia are considered a consequence of defective placentation during the first weeks of pregnancy (25). Due to ethical constraints, it is not feasible to assess the in vivo effect of different immunosuppressive drugs on the first trimester uterine immune environment. Moreover, studies conducted with peripheral cells cannot be extrapolated completely to the uterine environment due to the clear difference in immune cell composition and function (13, 15). Therefore, we cultured uterine immune cells isolated from first trimester decidual tissue in the presence of tacrolimus or azathioprine, at concentrations that resemble in vivo serum levels (see Methods). While azathioprine did not influence T cell cytokine expression, diminished cytokine expression could be observed in decidual T cells cultured in the presence of tacrolimus (Figure S4A). Frequency of FOXP3+CD127lowCD4+ Treg was diminished by both azathioprine and tacrolimus after in vitro culture (Figure S4B).
Overall, we showed that kidney transplant recipients have lower frequencies of uterine HLA-DR+ Treg upon delivery, predominantly when tacrolimus is used, and that in vitro exposure of uterine immune cells to immunosuppressive drugs affected Treg frequency and T cell cytokine expression.
Systemic immune signatures can be observed over the course of pregnancy (12, 26) and changing signatures are associated with pregnancy complications (27). In kidney transplant recipients, it is unclear how systemic maternal immune dynamics change during pregnancy. Immune cell frequencies were assessed by multiparameter flow cytometry (Figure S1B: gating strategy and assessed immune cell subpopulations) in peripheral blood samples collected over the course of pregnancy. To assess dynamic changes in systemic immunity over the course of pregnancy, the relationship between cell frequency and gestational age at time of sample collection was plotted with a LOESS regression.
Monocytes, NK cells, several T cell subset frequencies, and IFN-γ+ and IL-17+ CD4+ T cells in peripheral blood of pregnant women with a kidney transplant followed similar immune dynamic changes over the course of pregnancy as compared to healthy individuals (Figure S5), while slightly different maternal immune dynamics could be observed for CD4 and CD8 (central memory) T cells, and DC-like cells (Figure 2). In addition, combining frequencies from all gestational ages into a discrete variable showed that the overall frequency of CD25+CD127lowFOXP3+ Treg was consistently lower in kidney transplant recipients compared to controls (Figure 2C), in accordance with numerous observations in peripheral blood of kidney transplant patients receiving immunosuppression, especially when receiving calcineurin inhibitors (28–31). The decreased frequency of HLA-DR+ Treg observed in decidua was not reflected in the mother’s peripheral blood (Figure 2C). In addition, the observation in the decidua that tacrolimus use showed the greatest decrease in percentage HLA-DR+ Treg was not reflected in maternal blood either (Figure S6). Tacrolimus (without azathioprine) did however showed the greatest decrease in percentage of Treg (Figure S6). While B cell subset immune dynamics did not differ significantly over the course of pregnancy, a decreased percentage of naïve B cells and increased percentage of plasmablast and switched memory B cells could be observed overall in peripheral blood of pregnant kidney transplant recipients compared to controls (Figure 3), suggesting a switch in B cell phenotype from naïve to a more memory phenotype in pregnant women with a kidney transplant.
Figure 2 Maternal systemic immunity is affected in pregnant kidney transplant recipients (KT). (A) Percentage DC-like cells (CD45+CD19-CD3-CD56- CD16-CD14low HLA-DR+), (B) percentage CD4+ T cell, CD8+ T cell, and central memory (CM; CD45RA−CCR7+) CD4 and CD8 T cells, and (C) percentage regulatory T cells and HLA-DR+ Treg in peripheral blood from KT and healthy individuals (HC) are shown. Frequencies of peripheral blood immune cells are depicted both in boxplots (median + interquartile range) and as regression (LOESS) with gestational age (GA) at time of sample collection.
Figure 3 Systemic B cell frequencies are affected in pregnant kidney transplant recipients (KT). Percentage B cells, naïve B cells (CD27-IgD+), switched memory B cells (CD27+IgD-), and plasmablasts (CD24+IgD-CD38+) in peripheral blood from KT and healthy individuals (HC) are shown. Frequencies of peripheral blood immune cells are depicted both in boxplots (median + interquartile range) and as regression (LOESS) with gestational age (GA) at time of sample collection.
Overall, results showed that while the systemic immune system of pregnant kidney transplant recipients is different compared to uncomplicated and healthy pregnancies, the immune cell changes associated with pregnancy progression largely followed the same dynamic profile.
In kidney transplant recipients, neonatal immune development occurs under immunosuppressive drug exposure (8, 32–34). To assess whether being born to a mother with a kidney transplant influences the development of the neonatal immune system, we collected cord blood (umbilical vein) of neonates born to kidney transplant recipients and to healthy, uncomplicated pregnancies. Similar to the maternal blood and decidual phenotyping, the neonatal immune cell composition was characterized using multiparameter flow cytometry (Figure S1C).
In comparison to newborns of mothers with a healthy and uncomplicated pregnancy, cord blood of neonates born to kidney transplant recipients showed decreased B cell, Treg, and HLA-DR+ Treg frequencies with no difference in percentage IFN-γ+ and IL-17+ CD4+ T cells (Figure 4 and Figure S7), complementing prior reports (22, 35–39). This decreased percentage of Treg in the neonates born to kidney transplant recipients – who are largely born prematurely (Table 1) – is in contrast to literature where neonates born earlier show increased Treg frequencies that decrease with advancing gestational age (40, 41). When plotting Treg frequencies according to gestational age, we indeed observed decreasing Treg frequencies with advancing gestational age in both group (Figure S8A). This suggests that the in utero environment might have had an influence on the development of Treg. In addition, similarly to our results in the decidua, the strongest decrease in HLA-DR+ Treg percentages in cord blood was observed when tacrolimus, with or without azathioprine, was used during pregnancy (Figure S8B).
Figure 4 Innate immunity is affected in neonates born to pregnant kidney transplant recipients (KT). (A) Percentage of total B cells and B cell subsets. B cell subsets: naïve (CD27-IgD+), plasmablast (CD24+IgD-CD38+), non-switched memory (CD27+IgD+, switched memory (CD27+IgD-), and CD24hiCD38hi in cord blood of neonates born to KT and HC are shown as a percentage of B cells. (B) Percentage classical (CD14++CD16-), intermediate (CD14++CD16+), non-classical (CD14+CD16+) monocytes, and NKT-like cells in cord blood of neonates born to KT and healthy individuals (HC) are shown. (C) Percentage of regulatory T cells (Treg) and HLA-DR+ Treg in cord blood of neonates born to KT and HC are shown.
While B cell frequencies were decreased, in-depth B cell phenotyping performed here showed that the composition of the B cell population did not differ between our two groups of infants; i.e. no difference in naive, non-switched, switched, plasmablast, and CD24hiCD38hi B cell frequencies were observed at birth (Figure 4A), suggesting there is an overall B cell decrease rather than a decrease of a specific subset.
In addition, NKT-like cells and classical monocyte (CD14++CD16-) percentages were significantly decreased and non-classical monocytes (CD14+CD16+) and intermediate monocytes (CD14++CD16+) monocytes increased in cord blood of children born to kidney transplant recipients compared to infants born to women with healthy and uncomplicated pregnancies (Figure 4B). This shows that next to the adaptive immune system, also the innate system is affected at birth in neonates born to kidney transplant recipients.
Renal transplantation greatly restores fertility in women with chronic kidney or end-stage renal disease (1–5). However, a higher risk of pregnancy complications can be observed in women with a kidney transplant (3, 5–8). Here, we provide an overview of the immune cell characteristics of the maternal systemic and uterine immune environment of women with a kidney transplant and healthy individuals with uncomplicated pregnancies, and the neonatal immune system of their offspring. We showed decreased frequencies of HLA-DR+ Treg in the decidua of women with a kidney transplant, particularly in those treated with tacrolimus. While systemic immune cell frequencies were altered in kidney transplant patients, immune cell dynamics over the course of pregnancy were largely similar to healthy women. In addition, we report that the neonatal immune system at birth is affected in the offspring of kidney transplant recipients.
To mediate suppression against fetus-specific antigens, Treg with a suppressive phenotype are enriched in the decidua during healthy pregnancy (13, 42–46). HLA-DR+ Treg have been shown to be highly suppressive (47, 48) and here, we found decreased frequencies of HLA-DR+ Treg in decidual tissue after delivery of women with a kidney transplant. This decreased frequency is likely not due to a difference in gestational age at delivery. Systemically, reduced Treg frequencies could also be observed during pregnancy in kidney transplant recipients. Pregnancy complications such as preterm labor, are associated with altered Treg populations and disturbed Treg tolerance (46, 49, 50). This may suggest there is a loss of fetal tolerance at the maternal-fetal interface in women with a kidney transplant, which could explain the observed incidence of pregnancy complications such as preterm birth in these patients (5, 6, 8). Moreover, the strongest decrease in uterine HLA-DR+ Treg and maternal blood Treg was observed in those women who were prescribed tacrolimus, in comparison to those who used azathioprine (only azathioprine or azathioprine in combination with tacrolimus). This suggests that azathioprine may have a less detrimental impact on the uterine and systemic immune system than tacrolimus and perhaps could be more favorable to use during pregnancy. A large retrospective study conducted in the Netherlands indeed showed a trend towards lower birthweight (< 2500 grams) with a high rate of preterm delivery in pregnant kidney transplant recipients with a calcineurin inhibitor-based regimen (e.g. tacrolimus) (51) (unpublished manuscript). In our in vitro culture of first trimester uterine lymphocytes, both drugs reduced the frequency of Treg but only tacrolimus diminished cytokine expression by T cells, again potentially suggesting that azathioprine might have a less detrimental impact on the uterine system.
Dynamic changes in immune signatures can be observed in maternal peripheral blood over the course of pregnancy (12, 26). Here, we observed that these dynamics largely progressed in the same manner for women with a kidney transplant compared to controls. Only CD4+ and CD8+ (central memory) T cells, and DC-like cells frequencies progressed differently. This suggests that the majority of normal pregnancy associated frequency changes are not affected in kidney transplant recipients. However, it would be interesting to assess whether changes in signaling and functional responses could be affected. In addition, we did observe clear differences in the overall frequency of peripheral immune cells such as a change in B cell phenotype from naïve to a more memory phenotype (plasmablast and switched memory B cell) in pregnant women with a kidney transplant compared to women with uncomplicated pregnancies. Although calcineurin inhibitors such as tacrolimus can inhibit B cell differentiation in vitro (52), kidney transplant recipients with a B cell phenotype enriched for plasmablasts have a better transplant prognosis, i.e. better renal function and lower acute rejection incidence (53). In contrast, increased frequencies of B cells with a memory phenotype (CD27+CD38+/-) could be observed in the circulation of preeclamptic women (54, 55), suggesting that while the observed phenotypes could be beneficial for transplant survival they could be associated with the increased risk of complications in pregnant kidney transplant recipients.
Immunosuppressive drugs are able to cross the placenta and enter the fetal circulation (8, 32), thereby potentially influencing fetal immune development during pregnancy (33, 34) and influencing the offspring’s health in later life (56–58). We indeed observed that the neonatal immune system is affected at birth in offspring born to women with a kidney transplant. Paralleling our uterine and maternal blood results, Treg frequencies are decreased in cord blood of children born to kidney transplant recipients (22, 35–37, 39). Impaired Treg numbers and function at birth (cord blood) have been associated with an increased risk to develop sensitization to food allergens and atopic dermatitis in the first year of life (59, 60). We also observed decreased B cell frequencies in cord blood of neonates born to kidney transplant mothers, corresponding with prior reports (22, 35–38). Reduced B cell numbers at birth are associated with an increased hospitalization risk in the first year of life for children born from kidney transplant recipients (22, 61), and could potentially interfere with vaccination responses (62, 63). Next to the adaptive immune system, also the innate system is affected at birth in neonates born to kidney transplant recipients. Significantly decreased NKT-like cell and classical monocyte, and increased non-classical monocyte and intermediate monocyte percentages were observed in cord blood of infants born to kidney transplant recipients. A similar monocyte composition and NKT cell decrease can be found in inflammatory disorders such as systemic lupus erythematosus (SLE) and rheumatoid arthritis (64–67). As such, this pro-inflammatory monocyte state and altered NKT-like cell frequencies might predispose children of women with a transplanted kidney to develop inflammatory or autoimmune disorders. Indeed, SLE development is more common in children born to kidney transplant patients compared to controls (61). The longitudinal assessment of neonatal immunity in children born from women with a kidney transplant is an important consideration in order to fully understand long term effects of in utero exposure. Unfortunately, only few studies exist that indicate that immune changes can persist up to 1 year after birth (22, 62, 63, 68). Overall, the impaired neonatal innate and adaptive immune system at birth observed here underscores that children born to kidney transplant recipients could be at an increased risk for developing health complications early and later in life.
This study has certain limitations. Adverse pregnancy outcomes may be a result of several risk factors (5, 9–11, 16–19). Although the incidence of pre-eclampsia amongst our kidney transplant recipients was 35.7% (5 out of 14), corroborating reported incidence (3, 5–7), our sample size is unfortunately not sufficiently powered to allow for extensive covariate analysis. Delineating the effect of transplantation, immunosuppression, pre-pregnancy graft function, and so on, on the pregnancy-specific and neonatal immune system and correlating this with the development of adverse pregnancy outcomes, would require a larger and more diverse cohort. In addition, it would also be interesting to compare the pregnancy-specific and neonatal immune changes after other solid organ transplantations to find common or transplant-specific risk factors. Secondly, only changes in immune cell frequencies were reported here, as white blood cell counts were not available. However, frequency changes in immune cell subsets is likely to affect the immunological balance which in turn could affect pregnancy outcome.
In conclusion, the maternal peripheral, uterine, and neonatal immune system development is dysregulated in kidney transplant recipients, with distinct effects of the immunosuppressive drug regimen on frequency of HLA-DR+ Treg. This could have important consequences for the risk of pregnancy complications and health outcomes in the offspring. Moreover, it will be crucial to carefully select any immune intervention during pregnancy for its intended effect, placental accumulation, and possible side-effect on the neonatal immune system. Placental explants (69), uterine organoids (70, 71), and/or ex vivo placental perfusion experiments (72) will be important models to use during this decision-making process. Future studies that follow offspring’s health outcomes longitudinally, including vaccination responses and immunological evaluation at later time points, should be aimed at elucidating the effect of different drug regimens and dosages on pregnancy outcomes and the neonatal immune environment.
The raw data supporting the conclusions of this article will be made available by the authors, without undue reservation.
The studies involving human participants were reviewed and approved by Commissie Mensgebonden Onderzoek region Arnhem-Nijmegen. The patients/participants provided their written informed consent to participate in this study.
Conceptualization: HH, OH, RM, IJ, and DF. Data curation: DF, LRM, RM, IJ, HH, and OH. Formal Analysis: DF and JG. Investigation/Data acquisition: DF, BC, TL, HH, OH, LM, MB, and GC. Methodology: DF, JG, and BC. Project administration: DF, OH, HH, RM, and IJ. Supervision: RM and IJ. Visualization: DF and JG. Writing – original draft: DF. Writing – review and editing: DF, JG, IJ, RM, and all authors. All authors contributed to the article and approved the submitted version.
The authors declare that the research was conducted in the absence of any commercial or financial relationships that could be construed as a potential conflict of interest.
All claims expressed in this article are solely those of the authors and do not necessarily represent those of their affiliated organizations, or those of the publisher, the editors and the reviewers. Any product that may be evaluated in this article, or claim that may be made by its manufacturer, is not guaranteed or endorsed by the publisher.
The authors would like to thank all pregnant women who participated in this study, the research nurses and residents from the Radboud University Medical Center and University Medical Center Utrecht for their help in patient recruitment and sample collection. The authors would also like to thank Tadeja Kuret, Esther Fasse, Esther van Rijsen, and Marilen Benner for their help with sample processing.
The Supplementary Material for this article can be found online at: https://www.frontiersin.org/articles/10.3389/fimmu.2021.735564/full#supplementary-material
1. Nevis IF, Reitsma A, Dominic A, McDonald S, Thabane L, Akl EA, et al. Pregnancy Outcomes in Women With Chronic Kidney Disease: A Systematic Review. Clin J Am Soc Nephrol (2011) 6:2587–98. doi: 10.2215/CJN.10841210
2. Jesudason S, Grace BS, McDonald SP. Pregnancy Outcomes According to Dialysis Commencing Before or After Conception in Women With ESRD. Clin J Am Soc Nephrol (2014) 9:143–9. doi: 10.2215/CJN.03560413
3. Chittka D, Hutchinson JA. Pregnancy After Renal Transplantation. Transplantation (2017) 101:675–8. doi: 10.1097/TP.0000000000001649
4. Piccoli GB, Cabiddu G, Daidone G, Guzzo G, Maxia S, Ciniglio I, et al. The Children of Dialysis: Live-Born Babies From on-Dialysis Mothers in Italy–an Epidemiological Perspective Comparing Dialysis, Kidney Transplantation and the Overall Population. Nephrology Dialysis Transplant Off Publ Eur Dialysis Transplant Assoc - Eur Renal Assoc (2014) 29:1578–86. doi: 10.1093/ndt/gfu092
5. Deshpande NA, James NT, Kucirka LM, Boyarsky BJ, Garonzik-Wang JM, Montgomery RA, et al. Pregnancy Outcomes in Kidney Transplant Recipients: A Systematic Review and Meta-Analysis. Am J Transplant (2011) 11:2388–404. doi: 10.1111/j.1600-6143.2011.03656.x
6. McKay DB, Josephson MA. Pregnancy in Recipients of Solid Organs–Effects on Mother and Child. N Engl J Med (2006) 354:1281–93. doi: 10.1056/NEJMra050431
7. Mol BWJ, Roberts CT, Thangaratinam S, Magee LA, de Groot CJM, Hofmeyr GJ. Pre-Eclampsia. Lancet (2016) 387:999–1011. doi: 10.1016/S0140-6736(15)00070-7
8. McKay DB, Josephson MA. Pregnancy After Kidney Transplantation. Clin J Am Soc Nephrol (2008) 3(Suppl 2):S117–25. doi: 10.2215/CJN.02980707
9. Davison JM, Bailey DJ. Pregnancy Following Renal Transplantation. J Obstet Gynaecol Res (2003) 29:227–33. doi: 10.1046/j.1341-8076.2003.00106.x
10. You JY, Kim MK, Choi SJ, Oh SY, Kim SJ, Kim JH, et al. Predictive Factors for Adverse Pregnancy Outcomes After Renal Transplantation. Clin Transplant (2014) 28:699–706. doi: 10.1111/ctr.12367
11. Shah S, Verma P. Overview of Pregnancy in Renal Transplant Patients. Int J Nephrol (2016) 2016:4539342. doi: 10.1155/2016/4539342
12. Aghaeepour N, Ganio EA, McIlwain D, Tsai AS, Tingle M, Van Gassen S, et al. An Immune Clock of Human Pregnancy. Sci Immunol (2017) 2. doi: 10.1126/sciimmunol.aan2946
13. Feyaerts D, Benner M, van Cranenbroek B, van der Heijden OWH, Joosten I, van der Molen RG. Human Uterine Lymphocytes Acquire a More Experienced and Tolerogenic Phenotype During Pregnancy. Sci Rep (2017) 7:2884. doi: 10.1038/s41598-017-03191-0
14. Arck PC, Hecher K. Fetomaternal Immune Cross-Talk and Its Consequences for Maternal and Offspring’s Health. Nat Med (2013) 19:548–56. doi: 10.1038/nm.3160
15. Benner M, Feyaerts D, Garcia CC, Inci N, Lopez SC, Fasse E, et al. Clusters of Tolerogenic B Cells Feature in the Dynamic Immunological Landscape of the Pregnant Uterus. Cell Rep (2020) 32:108204. doi: 10.1016/j.celrep.2020.108204
16. Mor G, Aldo P, Alvero AB. The Unique Immunological and Microbial Aspects of Pregnancy. Nat Rev Immunol (2017) 17:469–82. doi: 10.1038/nri.2017.64
17. Saito S, Shiozaki A, Nakashima A, Sakai M, Sasaki Y. The Role of the Immune System in Preeclampsia. Mol Aspects Med (2007) 28:192–209. doi: 10.1016/j.mam.2007.02.006
18. Darmochwal-Kolarz D, Kludka-Sternik M, Tabarkiewicz J, Kolarz B, Rolinski J, Leszczynska-Gorzelak B, et al. The Predominance of Th17 Lymphocytes and Decreased Number and Function of Treg Cells in Preeclampsia. J Reprod Immunol (2012) 93:75–81. doi: 10.1016/j.jri.2012.01.006
19. Gomez-Lopez N, StLouis D, Lehr MA, Sanchez-Rodriguez EN, Arenas-Hernandez M. Immune Cells in Term and Preterm Labor. Cell Mol Immunol (2014) 11:571–81. doi: 10.1038/cmi.2014.46
20. Freriksen JJM, Feyaerts D, van den Broek PHH, van der Heijden OWH, van Drongelen J, van Hamersvelt HW, et al. Placental Disposition of the Immunosuppressive Drug Tacrolimus in Renal Transplant Recipients and in Ex Vivo Perfused Placental Tissue. Eur J Pharm Sci (2018) 119:244–8. doi: 10.1016/j.ejps.2018.04.017
21. Ponticelli C, Moroni G. Fetal Toxicity of Immunosuppressive Drugs in Pregnancy. J Clin Med (2018) 7:552. doi: 10.3390/jcm7120552
22. Ono E, Dos Santos AM, Viana PO, Dinelli MI, Sass N, De Oliveira L, et al. Immunophenotypic Profile and Increased Risk of Hospital Admission for Infection in Infants Born to Female Kidney Transplant Recipients. Am J Transplant (2015) 15:1654–65. doi: 10.1111/ajt.13143
23. Hutson JR, Lubetsky A, Walfisch A, Ballios BG, Garcia-Bournissen F, Koren G, et al. The Transfer of 6-Mercaptopurine in the Dually Perfused Human Placenta. Reprod Toxicol (2011) 32:349–53. doi: 10.1016/j.reprotox.2011.08.008
24. Zheng S, Easterling TR, Umans JG, Miodovnik M, Calamia JC, Thummel KE, et al. Pharmacokinetics of Tacrolimus During Pregnancy. Ther Drug Monit (2012) 34:660–70. doi: 10.1097/FTD.0b013e3182708edf
25. Burton GJ, Redman CW, Roberts JM, Moffett A. Pre-Eclampsia: Pathophysiology and Clinical Implications. BMJ (2019) 366:l2381. doi: 10.1136/bmj.l2381
26. Stelzer IA, Ghaemi MS, Han X, Ando K, Hedou JJ, Feyaerts D, et al. Integrated Trajectories of the Maternal Metabolome, Proteome, and Immunome Predict Labor Onset. Sci Transl Med (2021) 13. doi: 10.1126/scitranslmed.abd9898
27. Han X, Ghaemi M, Ando K, Peterson L, Ganio E, Tsai A, et al. Differential Dynamics of the Maternal Immune System in Healthy Pregnancy and Preeclampsia. Front Immunol (2019) 10:1305. doi: 10.3389/fimmu.2019.01305
28. Noris M, Casiraghi F, Todeschini M, Cravedi P, Cugini D, Monteferrante G, et al. Regulatory T Cells and T Cell Depletion: Role of Immunosuppressive Drugs. J Am Soc Nephrol (2007) 18:1007–18. doi: 10.1681/ASN.2006101143
29. Korczak-Kowalska G, Wierzbicki P, Bocian K, Klosowska D, Niemczyk M, Wyzgal J, et al. The Influence of Immuosuppressive Therapy on the Development of CD4+CD25+ T Cells After Renal Transplantation. Transplant Proc (2007) 39:2721–3. doi: 10.1016/j.transproceed.2007.09.015
30. Vallotton L, Hadaya K, Venetz JP, Buehler LH, Ciuffreda D, Nseir G, et al. Monitoring of CD4+CD25highIL-7Ralphahigh Activated T Cells in Kidney Transplant Recipients. Clin J Am Soc Nephrol (2011) 6:2025–33. doi: 10.2215/CJN.09611010
31. Fourtounas C, Dousdampanis P, Sakellaraki P, Rodi M, Georgakopoulos T, Vlachojannis JG, et al. Different Immunosuppressive Combinations on T-Cell Regulation in Renal Transplant Recipients. Am J Nephrol (2010) 32:1–9. doi: 10.1159/000313940
32. Prevot A, Martini S, Guignard JP. In Utero Exposure to Immunosuppressive Drugs. Biol Neonate (2002) 81:73–81. doi: 10.1159/000047187
33. Holt PG, Jones CA. The Development of the Immune System During Pregnancy and Early Life. Allergy (2000) 55:688–97. doi: 10.1034/j.1398-9995.2000.00118.x
34. Zhang X, Zhivaki D, Lo-Man R. Unique Aspects of the Perinatal Immune System. Nat Rev Immunol (2017) 17:495–507. doi: 10.1038/nri.2017.54
35. Dinelli MI, Ono E, Viana PO, Spina FG, Weckx LY, Dos Santos AM, et al. Response to Immunization in Children Born to Renal Transplant Recipients Using Immunosuppressive Drugs During Gestation. Vaccine (2016) 34:404–7. doi: 10.1016/j.vaccine.2015.12.017
36. Di Paolo S, Schena A, Morrone LF, Manfredi G, Stallone G, Derosa C, et al. Immunologic Evaluation During the First Year of Life of Infants Born to Cyclosporine-Treated Female Kidney Transplant Recipients: Analysis of Lymphocyte Subpopulations and Immunoglobulin Serum Levels. Transplantation (2000) 69:2049–54. doi: 10.1097/00007890-200005270-00013
37. Schena FP, Stallone G, Schena A, Manfredi G, Derosa C, Procino A, et al. Pregnancy in Renal Transplantation: Immunologic Evaluation of Neonates From Mothers With Transplanted Kidney. Transplant Immunol (2002) 9:161–4. doi: 10.1016/S0966-3274(02)00028-X
38. Takahashi N, Nishida H, Hoshi J. Severe B Cell Depletion in Newborns From Renal Transplant Mothers Taking Immunosuppressive Agents. Transplantation (1994) 57:1617–21. doi: 10.1097/00007890-199457110-00014
39. Pilarski LM, Yacyshyn BR, Lazarovits AI. Analysis of Peripheral Blood Lymphocyte Populations and Immune Function From Children Exposed to Cyclosporine or to Azathioprine In Utero. Transplantation (1994) 57:133–44. doi: 10.1097/00007890-199401000-00021
40. Peterson LS, Hedou J, Ganio EA, Stelzer IA, Feyaerts D, Harbert E, et al. Single-Cell Analysis of the Neonatal Immune System Across the Gestational Age Continuum. Front Immunol (2021) 12:714090. doi: 10.3389/fimmu.2021.714090
41. Takahata Y, Nomura A, Takada H, Ohga S, Furuno K, Hikino S, et al. CD25+CD4+ T Cells in Human Cord Blood: An Immunoregulatory Subset With Naive Phenotype and Specific Expression of Forkhead Box P3 (Foxp3) Gene. Exp Hematol (2004) 32:622–9. doi: 10.1016/j.exphem.2004.03.012
42. Mjosberg J, Berg G, Jenmalm MC, Ernerudh J. FOXP3+ Regulatory T Cells and T Helper 1, T Helper 2, and T Helper 17 Cells in Human Early Pregnancy Decidua. Biol Reprod (2010) 82:698–705. doi: 10.1095/biolreprod.109.081208
43. Loewendorf AI, Nguyen TA, Yesayan MN, Kahn DA. Normal Human Pregnancy Results in Maternal Immune Activation in the Periphery and at the Uteroplacental Interface. PloS One (2014) 9:e96723. doi: 10.1371/journal.pone.0096723
44. Tilburgs T, Roelen DL, van der Mast BJ, van Schip JJ, Kleijburg C, de Groot-Swings GM, et al. Differential Distribution of CD4(+)CD25(bright) and CD8(+)CD28(-) T-Cells in Decidua and Maternal Blood During Human Pregnancy. Placenta (2006) 27(Suppl A):S47–53. doi: 10.1016/j.placenta.2005.11.008
45. Tilburgs T, Roelen DL, van der Mast BJ, de Groot-Swings GM, Kleijburg C, Scherjon SA, et al. Evidence for a Selective Migration of Fetus-Specific CD4+CD25bright Regulatory T Cells From the Peripheral Blood to the Decidua in Human Pregnancy. J Immunol (2008) 180:5737–45. doi: 10.4049/jimmunol.180.8.5737
46. Tsuda S, Nakashima A, Shima T, Saito S. New Paradigm in the Role of Regulatory T Cells During Pregnancy. Front Immunol (2019) 10:573. doi: 10.3389/fimmu.2019.00573
47. Baecher-Allan C, Wolf E, Hafler DA. MHC Class II Expression Identifies Functionally Distinct Human Regulatory T Cells. J Immunol (2006) 176:4622–31. doi: 10.4049/jimmunol.176.8.4622
48. Schaier M, Seissler N, Schmitt E, Meuer S, Hug F, Zeier M, et al. DR(high+)CD45RA(-)-Tregs Potentially Affect the Suppressive Activity of the Total Treg Pool in Renal Transplant Patients. PloS One (2012) 7:e34208. doi: 10.1371/journal.pone.0034208
49. Kisielewicz A, Schaier M, Schmitt E, Hug F, Haensch GM, Meuer S, et al. A Distinct Subset of HLA-DR+-Regulatory T Cells Is Involved in the Induction of Preterm Labor During Pregnancy and in the Induction of Organ Rejection After Transplantation. Clin Immunol (2010) 137:209–20. doi: 10.1016/j.clim.2010.07.008
50. Ruocco MG, Chaouat G, Florez L, Bensussan A, Klatzmann D. Regulatory T-Cells in Pregnancy: Historical Perspective, State of the Art, and Burning Questions. Front Immunol (2014) 5:389. doi: 10.3389/fimmu.2014.00389
51. Koenjer LM, Meinderts JR, van der Heijden OWH, Lely T, de Jong M, van der Molen RG, et al. Calcineurin Inhibitor-Based Immunosuppression Has Negligible Negative Effects on Pregnancy Outcomes After Renal Transplantation in the Netherlands. Kidney Week 2020 (2020) Abstract PO2541.
52. De Bruyne R, Bogaert D, De Ruyck N, Lambrecht BN, Van Winckel M, Gevaert P, et al. Calcineurin Inhibitors Dampen Humoral Immunity by Acting Directly on Naive B Cells. Clin Exp Immunol (2015) 180:542–50. doi: 10.1111/cei.12604
53. Alfaro R, Legaz I, Gonzalez-Martinez G, Jimenez-Coll V, Martinez-Banaclocha H, Galian JA, et al. Monitoring of B Cell in Kidney Transplantation: Development of a Novel Clusters Analysis and Role of Transitional B Cells in Transplant Outcome. Diagnostics (Basel) (2021) 11. doi: 10.3390/diagnostics11040641
54. Liao AH, Liu LP, Ding WP, Zhang L. Functional Changes of Human Peripheral B-Lymphocytes in Pre-Eclampsia. Am J Reprod Immunol (2009) 61:313–21. doi: 10.1111/j.1600-0897.2009.00697.x
55. Zeng B, Kwak-Kim J, Liu Y, Liao AH. Treg Cells are Negatively Correlated With Increased Memory B Cells in Pre-Eclampsia While Maintaining Suppressive Function on Autologous B-Cell Proliferation. Am J Reprod Immunol (2013) 70:454–63. doi: 10.1111/aji.12154
56. Hsu P, Nanan R. Foetal Immune Programming: Hormones, Cytokines, Microbes and Regulatory T Cells. J Reprod Immunol (2014) 104-105:2–7. doi: 10.1016/j.jri.2014.02.005
57. Santner-Nanan B, Straubinger K, Hsu P, Parnell G, Tang B, Xu B, et al. Fetal-Maternal Alignment of Regulatory T Cells Correlates With IL-10 and Bcl-2 Upregulation in Pregnancy. J Immunol (2013) 191:145–53. doi: 10.4049/jimmunol.1203165
58. Stojanovska V, Scherjon SA, Plosch T. Preeclampsia as Modulator of Offspring Health. Biol Reprod (2016) 94:53. doi: 10.1095/biolreprod.115.135780
59. Hinz D, Bauer M, Roder S, Olek S, Huehn J, Sack U, et al. Cord Blood Tregs With Stable FOXP3 Expression Are Influenced by Prenatal Environment and Associated With Atopic Dermatitis at the Age of One Year. Allergy (2012) 67:380–9. doi: 10.1111/j.1398-9995.2011.02767.x
60. Smith M, Tourigny MR, Noakes P, Thornton CA, Tulic MK, Prescott SL. Children With Egg Allergy Have Evidence of Reduced Neonatal CD4(+)CD25(+)CD127(lo/-) Regulatory T Cell Function. J Allergy Clin Immunol (2008) 121:1460–1466, 1466 e1461-1467. doi: 10.1016/j.jaci.2008.03.025
61. Norrman E, Bergh C, Wennerholm UB. Pregnancy Outcome and Long-Term Follow-Up After In Vitro Fertilization in Women With Renal Transplantation. Hum Reprod (2015) 30:205–13. doi: 10.1093/humrep/deu293
62. Cimaz R, Meregalli E, Biggioggero M, Airo P, Danieli E, Antonioli CM, et al. Response to Tetanus Vaccination in Infants Exposed In Utero to Immunosuppressants for Maternal Autoimmune Disorders. Lupus (2007) 16:129–32. doi: 10.1177/0961203306075738
63. Szczawinska-Poplonyk A, Breborowicz A, Samara H, Ossowska L, Dworacki G. Impaired Antigen-Specific Immune Response to Vaccines in Children With Antibody Production Defects. Clin Vaccine Immunol (2015) 22:875–82. doi: 10.1128/CVI.00148-15
64. Mukherjee R, Kanti Barman P, Kumar Thatoi P, Tripathy R, Kumar Das B, Ravindran B. Non-Classical Monocytes Display Inflammatory Features: Validation in Sepsis and Systemic Lupus Erythematous. Sci Rep (2015) 5:13886. doi: 10.1038/srep13886
65. Wong KL, Yeap WH, Tai JJ, Ong SM, Dang TM, Wong SC. The Three Human Monocyte Subsets: Implications for Health and Disease. Immunol Res (2012) 53:41–57. doi: 10.1007/s12026-012-8297-3
66. Chen J, Wu M, Wang J, Li X. Immunoregulation of NKT Cells in Systemic Lupus Erythematosus. J Immunol Res (2015) 2015:206731. doi: 10.1155/2015/206731
67. Zahran AM, Abdel-Rahim MH, Elsayh KI, Hassanien MM, Mahran SA, Hetta HF. Natural Killer and Natural Killer T Cells in Juvenile Systemic Lupus Erythematosus: Relation to Disease Activity and Progression. Arch Immunol Ther Exp (Warsz) (2019) 67:161–9. doi: 10.1007/s00005-019-00537-6
68. Boulay H, Mazaud-Guittot S, Supervielle J, Chemouny JM, Dardier V, Lacroix A. Maternal, Foetal and Child Consequences of Immunosuppressive Drugs During Pregnancy in Women With Organ Transplant: A Review. Clin Kidney J (2021) 14:1871–8. doi: 10.1093/ckj/sfab049
69. Gohner C, Svensson-Arvelund J, Pfarrer C, Hager JD, Faas M, Ernerudh J, et al. The Placenta in Toxicology. Part IV: Battery of Toxicological Test Systems Based on Human Placenta. Toxicol Pathol (2014) 42:345–51. doi: 10.1177/0192623313482206
70. Turco MY, Gardner L, Hughes J, Cindrova-Davies T, Gomez MJ, Farrell L, et al. Long-Term, Hormone-Responsive Organoid Cultures of Human Endometrium in a Chemically Defined Medium. Nat Cell Biol (2017) 19:568–77. doi: 10.1038/ncb3516
71. Turco MY, Gardner L, Kay RG, Hamilton RS, Prater M, Hollinshead MS, et al. Trophoblast Organoids as a Model for Maternal-Fetal Interactions During Human Placentation. Nature (2018) 564:263–7. doi: 10.1038/s41586-018-0753-3
Keywords: renal transplantation, neonatal immunity, decidua, uterine immunity, pregnancy, kidney transplantation
Citation: Feyaerts D, Gillard J, van Cranenbroek B, Rigodanzo Marins L, Baghdady MMS, Comitini G, Lely AT, van Hamersvelt HW, van der Heijden OWH, Joosten I and van der Molen RG (2021) Maternal, Decidual, and Neonatal Lymphocyte Composition Is Affected in Pregnant Kidney Transplant Recipients. Front. Immunol. 12:735564. doi: 10.3389/fimmu.2021.735564
Received: 02 July 2021; Accepted: 04 October 2021;
Published: 28 October 2021.
Edited by:
Andrew L. Mellor, Newcastle University, United KingdomReviewed by:
Sanda Stankovic, The Alfred Hospital, AustraliaCopyright © 2021 Feyaerts, Gillard, van Cranenbroek, Rigodanzo Marins, Baghdady, Comitini, Lely, van Hamersvelt, van der Heijden, Joosten and van der Molen. This is an open-access article distributed under the terms of the Creative Commons Attribution License (CC BY). The use, distribution or reproduction in other forums is permitted, provided the original author(s) and the copyright owner(s) are credited and that the original publication in this journal is cited, in accordance with accepted academic practice. No use, distribution or reproduction is permitted which does not comply with these terms.
*Correspondence: Renate G. van der Molen, UmVuYXRlLnZhbmRlck1vbGVuQHJhZGJvdWR1bWMubmw=
†Present address: Dorien Feyaerts, Department of Anesthesiology, Perioperative and Pain Medicine, Stanford University School of Medicine, Stanford, CA, United States
Disclaimer: All claims expressed in this article are solely those of the authors and do not necessarily represent those of their affiliated organizations, or those of the publisher, the editors and the reviewers. Any product that may be evaluated in this article or claim that may be made by its manufacturer is not guaranteed or endorsed by the publisher.
Research integrity at Frontiers
Learn more about the work of our research integrity team to safeguard the quality of each article we publish.