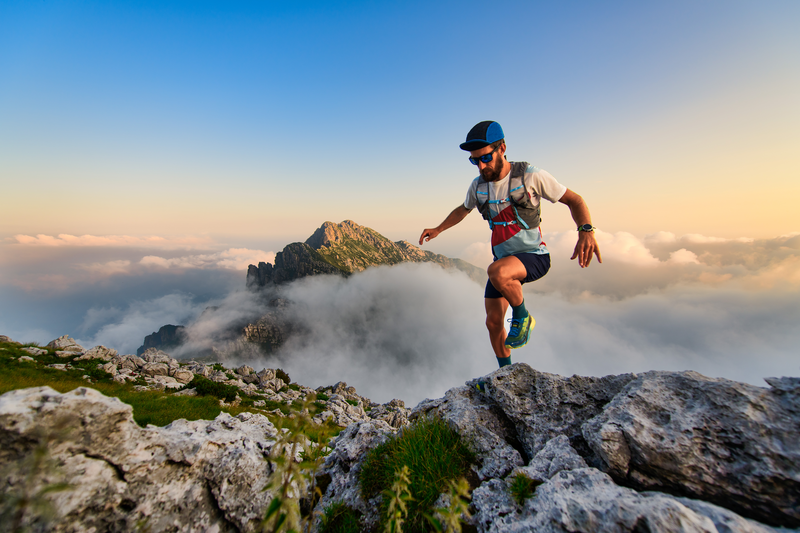
94% of researchers rate our articles as excellent or good
Learn more about the work of our research integrity team to safeguard the quality of each article we publish.
Find out more
REVIEW article
Front. Immunol. , 21 October 2021
Sec. Mucosal Immunity
Volume 12 - 2021 | https://doi.org/10.3389/fimmu.2021.735324
Intra-amniotic infection and inflammation (IAI) affect fetal development and are highly associated with preterm labor and premature rupture of membranes, which often lead to adverse neonatal outcomes. Human amniotic membrane (hAM), the inner part of the amnio-chorionic membrane, protects the embryo/fetus from environmental dangers, including microbial infection. However, weakened amnio-chorionic membrane may be breached or pathogens may enter through a different route, leading to IAI. The hAM and human amniotic fluid (hAF) respond by activation of all components of the innate immune system. This includes changes in 1) hAM structure, 2) presence of immune cells, 3) pattern recognition receptors, 4) cytokines, 5) antimicrobial peptides, 6) lipid derivatives, and 7) complement system. Herein we provide a comprehensive and integrative review of the current understanding of the innate immune response in the hAM and hAF, which will aid in design of novel studies that may lead to breakthroughs in how we perceive the IAI.
Amniotic membrane (AM) serves as a wall of an embryo/fetal annex, and is the innermost component of the fetal membrane (i.e. amnio-chorionic membrane) that envelops the amniotic fluid (AF) with the developing embryo/fetus (1). The evolutionary need for AM is thought to emerge concurrently with the transition of animals from water to land (2). The membrane of the so-called amniotic egg provided protection from dryer environment, while allowing the necessary gas exchange (3). These egg-laying animals are ancestors to today’s clade Amniotes, which comprises of reptiles, birds and mammals. Though there are significant differences between the AM and AF of these animals, its main functions remain the same: to provide nourishment, homeostatic environment and protection from physical, chemical and biological stress to the fetus (4–7).
Human AM (hAM), originates from the embryo and forms a multilayer structure that is between 0.02 and 0.5 mm thick (8–10). Human AF (hAF) is in direct contact with a monolayer of hAM epithelial cells (hAEC), which are attached to the basement membrane that borders on the compact layer of hAM. Next is the fibrous layer with hAM mesenchymal stromal cells (hAMSC), and finally the spongy layer of hAM, which adheres the human chorionic membrane (hCM) composed of reticular layer, basement membrane and chorion trophoblast cells (9, 10).
At term a subpopulation of hAEC expresses embryonic stem cell surface markers, such as tumor rejection antigens 1-60 (TRA1-60) and TRA1-81 (11–13), stage-specific embryonic antigens 3 and 4 (SSEA-3, SSEA-4) (11, 13, 14), and also some transcription factors characteristic for stem cells, such as OCT-4, NANOG, SOX-2 and SOX-3 (11, 12, 15, 16). Similarly, a subpopulation of hAMSC also expresses some of the pluripotency markers, namely TRA-1-61, TRA1-81 (17), OCT-3 (18), OCT-4 (17–19), SSEA-3, SSEA-4 (17, 20), SOX-2 and NANOG (19, 20). Moreover, hAEC and hAMSC are capable of differentiation into all three germ layers, namely ectoderm, mesoderm and endoderm (15, 17, 21).
Besides the nourishment and homeostatic functions, the cells of the hAM provide an extensive immune defense against the potential pathogens. hAM cells and cells involved in the immune response secrete a vast array of protective molecules including lipids, peptides, and proteins that comprise up to 25% of all identified proteins found in the hAF (22). Overall, a large number of identified molecules and complexes related to immune defense (22) appear redundant, but offer a possibly synergistic protection against various pathogens (22, 23).
At first, hAEC and hAMSC have been considered to be immune privileged, but it has been shown that they are able to elicit immune responses under certain conditions (24–26). The anti-inflammatory activity of hAM-derived cells has been well documented. hAM-derived cells and their conditioned medium block differentiation and maturation of monocytes into dendritic cells or inflammatory M1 macrophages (27–32). Moreover, when the M1 macrophages are cultured with hAMSC or their conditioned medium, their expression of co-stimulatory proteins CD80, CD86 and CD40, decreases. The hAMSC and their conditioned medium have been shown to shift differentiation of monocytes (under M1 differentiation conditions) towards the anti-inflammatory M2 phenotype and they also reduce the pro-inflammatory cytokine secretion interleukin (IL)-1α, IL-1β, IL-12, IL-8, tumor necrosis factor alpha (TNFα), macrophage inflammatory protein (MIP)1α, MIP1β, monokine induced by gamma interferon, regulated on activation, normal T expressed and secreted (RANTES), interferon-gamma inducible protein (IP)-10) by the M2 macrophages, while increasing the secretion of the anti-inflammatory cytokine IL-10 (32).
The hAEC inhibit neutrophil (33) and macrophage migration by secretion of the migration inhibitor factor (MIF) (34), while the hAMSC reduce neutrophil migration (35). The hAEC and hAMSC have also been shown to inhibit NK cell cytotoxicity and reduce IFN-gamma expression in a dose-dependent manner (36).
The versatile nature of hAM-derived cells is well demonstrated by their ability to induce immunosuppression as well as immunostimulation. Namely, when hAM-derived cells are co-cultured with unstimulated allogenic peripheral blood mononuclear cells (PBMCs) at low concentrations, they have been shown to stimulate PBMCs proliferation (25, 30, 37). Similarly, the co-culture of T lymphocytes with low concentrations of hAM-derived cells led to induction of proliferation in T lymphocytes (25, 31). Therefore, the hAM may function as a sensor and regulator of the inflammatory response to infections and/or inflammatory stimuli (38). Interestingly, there is a great shortage of studies (especially in the in vivo setting) that would investigate the immunomodulatory activity of hAM in the context of IAI. The studies investigating the expression and production of immunomodulatory molecules by hAM cells in IAI are described in the Chapter 3.
It is thought that at term, amnio-chorionic membrane weakening that occurs due to irreversible epithelial-to-mesenchymal transition (EMT), as well as the initiation of labor are inflammation dependent, and can be triggered by physical stretching of the membrane and an increased oxidative stress (39, 40). The resulting cellular stress produces Damage-Associated Molecular Patterns (DAMPs) and senescence-associated secretory phenotypes (SASPs), in the case of infection also Pathogen-Associated Molecular Patterns (PAMPs), which contribute to the necessary inflammation through downstream signalling cascades (40–42). Moreover, hAM was shown to be crucial in the production of prostaglandins, which induce cervical ripening and uterine contractions (43, 44).
Occasional infections of the amnio-chorionic membranes or hAF do occur and may harm the fetus or cause preterm labor and other complications (45, 46). Infections are present in 15-35% of all pregnancies that result in preterm birth (47, 48). As infection and inflammation are associated processes, it is sometimes difficult to distinguish between them, especially in cases when only limited analysis or sampling is available. Therefore, the term chorioamnionitis includes inflammation of intrauterine structures amnion and chorion, their infection, or both; in this paper we refer to this as intra-amniotic infection/inflammation (IAI) (49).
IAIs are most commonly caused by pathogenic bacteria and fungi, such as Mycoplasma sp., Ureaplasma sp., Bacteroides sp., group B Streptococcus sp., Escherichia coli, Enterococcus faecalis, Klebsiella pneumoniae, Fusobacterium nucleatum, Gardnerella vaginalis and Candida albicans (50, 51) and viruses, such as cytomegalovirus, herpes simplex virus types 1 and 2 and Zika virus (52, 53). SARS-Cov-2 that caused the current pandemic can in rare cases infect the fetus through a transplacental transmission (54, 55). Interestingly, IAI is often caused by multiple pathogens, since over 65% of all positive hAF cultures involve two or more microorganisms (56). While the identified pathogens are diverse, they are mainly typical genital pathogens, largely from the phylum Firmicutes, order Mycoplasmatales (57).
The question whether the placenta has its own microbiome, or if the presence of bacteria always indicates potential pathogens still remains open. A study performed by Aagaard et al. (58) demonstrated the presence of microbiome in human placenta, which was low in abundance but metabolically rich (58). Moreover, others have demonstrated the presence of microbiota in the human umbilical cord blood and hAF (59, 60). On the other hand, these findings were refuted by de Goffau et al. (61) who investigated the presence of bacterial DNA in the human placenta and demonstrated that the human placenta has no microbiome but can contain potential pathogens (61). Furthermore, not only was there no evidence for the presence of bacteria in the majority of samples, but all signs were related to the acquisition of bacteria during labor and delivery or to contamination of laboratory reagents with bacterial DNA (61–63). The contradicting observations of these studies reflect the difficulty of studying placental microbiome, since the placenta is a relatively inaccessible tissue during pregnancy, and after birth it is easily contaminated. Furthermore, the microbiome, if there is one, is limited in the amount of microbes, and therefore challenging to detect. This is especially true for less sensitive methods, such as classical cultivation techniques, whereas DNA amplification techniques are more sensitive but are also more prone to contamination and present an indirect evidence of microbes. In the future, further investigations will need to be done to answer the questions of presence and extent of placental microbiome.
Multiple routes of invasion have been proposed for IAI. Namely, pathogens can enter the amniotic cavity by 1) ascending migration from the lower genital tract through the cervix (most common route of infection), 2) haematogenous dissemination from distant sites (e.g. the intestine or oral cavity) through the placenta, 3) retrograde seeding from the peritoneal cavity through the fallopian tubes or 4) iatrogenic introduction during the invasive procedures (57, 64, 65). Interestingly, a study by Kim et al. (66) indicates that the microbial invasion of the amniotic cavity does not follow widespread infection of amnio-chorionic membrane, but precedes it. The authors proposed that the microbial invasion of the amniotic cavity starts by intra-amniotic bacterial invasion through a discrete region of the amnio-chorionic membrane, is followed by intra-amniotic proliferation and therefore, bacterial invasion of amnio-chorionic membrane primarily extends from the hAF (66). The pathogens are recognized by the pattern recognition receptors (PRRs), a component of the innate immune system, which activates the synthesis of cytokines, chemoattractant cytokines (chemokines), prostaglandins, proteases, antimicrobial peptides, and other mechanisms of the innate immune system, some of which are mediated by neutrophils, monocytes and lymphocytes (51, 67, 68). This immune cascade can lead to premature contractions, cervical changes and premature delivery. Furthermore, some bacteria are even capable of producing enzymes that degrade amnio-chorionic membrane or inducing synthesis and release of molecules that stimulate uterine contractions and lead to preterm labor (e.g. prostaglandins) (57, 69). Importantly, various pathogens and the inflammatory response that follows the infection, affect the fetal development (especially the developing fetal lung and brain), and may also impact the long-term health of the infant (70). IAI can affect different neurological developmental processes, inducing neuroinflammation, cerebral palsy, and periventricular white matter injury in the fetus, and even neuropsychiatric diseases have been linked to IAI (71–76). Chronically damaged lungs in the form of bronchopulmonary dysplasia are also highly associated with IAI (77–79).
The aim of this review is therefore to encompass the variability and importance of the innate immune response of the hAM and hAF implicated in the IAI. To provide a thorough overview of the literature, we collected an unbiased set of articles covering all parts of the hAM and hAF innate immune response related to IAI during pregnancy and in labor. The studies were screened and selected from PubMed database using key words “intraamniotic”, “infection”, “amnion”, and various components of the innate immune system. Studies, which were investigating immune response in tissues other than hAM or hAF were excluded. Several in vitro studies that used hAM or its derivatives are also considered, and an overview of animal models for IAI is presented in a recent paper by Cappelletti et al. (80).
The immune system of the fetus and (preterm) neonate is immature and requires stimulation to fully develop. Moreover, the fetal adaptive immune responses are downregulated, which may enhance their vulnerability to infection but at the same time this has a protective role against collateral inflammatory damage and is related to maintaining the intricate immune balance between the mother and the fetus (62, 81). Therefore, fetuses and neonates largely rely on their innate immune system and additional protection is provided by various components of the placenta, namely by the amnio-chorionic membrane and decidua and also by the hAF (62, 80, 82, 83). The studies that explored the involvement of the innate immune system in the hAM and hAF in relation to the aforementioned IAIs are summed up in the following subsections, and in Figure 1 and Table 1. The involvement of other important tissues in immune response in IAI is not covered in this review.
Figure 1 Innate immune system defense against pathogens in the hAF and hAM is comprehensive. hAM integrity prevents the entry of pathogens. Breaching of hAM causes upregulated expression and release of cytokines, including chemokines, which engage immune cells neutrophils, macrophages, monocytes and T lymphocytes. Immune cells regulate the inflammatory process and directly kill the microbes through phagocytosis, formation of neutrophil extracellular traps, and direct killing with granules. Using the pattern recognition receptors (PRRs), the hAEC recognize the pathogen-associated molecular patterns (PAMPs) (LPS, bacterial glycoprotein) and damage-associated molecular patterns (DAMPs) that are a result of the microbial infection. Most notably, these include toll-like receptors (TLRs) on cell membrane and the nucleotide-binding oligomerization domain (NOD)- Leucin Rich Repeats (LRR)-containing receptors (NLRs) in the cytosol, both of which induce various downstream signaling that culminates in the release and maturation of inflammatory cytokines, regulated cell death and secretion of antimicrobial peptides (AMPs). AMPs and activated complement system directly damage pathogens, mainly by targeting their cell wall and by causing lysis.
Table 1 Response of various components of the innate immune system to the intra-amniotic infection/inflammation.
Amnio-chorionic membrane, including hAM, present a barrier for the potential intra-amniotic pathogens. Their loss of integrity, inflammation, and inflammation-related oxidative stress can increase the chance of preterm rupture of membranes (PROM) or directly permit the entry of pathogens to the hAF, which sequentially weakens the membrane further (212, 213). hAM integrity can be modified by changes in the extracellular matrix modified by a pro-inflammatory environment. For example, activin A, which is involved in connective tissue remodeling, is increased in IAI, and its stimulation by LPS or TNFα suppresses type I and type III collagen mRNA levels in hAMSC (84–86). Additionally, the amount of extracellular matrix glycoprotein tenascin X was changed with IAI (87) and an in vitro model hAMSC suppressed expression of collagen types I and III with activin A stimulation (85). Matrix metalloproteinases (MMPs) are important regulators of inflammation with their role extending beyond the degradation of extracellular matrix (214). The relationship between MMPs and fetal membrane weakening and preterm labor is reviewed by Vadillo-Ortega et al. (95). Studies investigating association of IAI and PROM with MMP concentration discovered the upregulation of MMP-7, MMP-8 and MMP-9, with inconsistent conclusions for MMP-2 (93, 96–103). The discrepancies for MMP-2 are the result of different experimental settings; the studies by Fortunato et al. (96) and Myntti et al. (102) have seen an increase in total levels of MMP-2 and in catalitically active levels of MMP-2 (tissue inhibitor of metalloproteinases (TIMP)-free MMP2) in the hAF samples, while Flores-Herrera et al. (99) used an in vitro model of infected amnio-chorionic membrane and did not observe an increase in the secretion of proMMP-2. MMP inhibitor TIMP1 was increased in preterm PROM and in IAI, while TIMP2 was decreased in preterm PROM (96, 99, 102). Similarly, a higher ratio of MMP : TIMP was observed also in the placenta and in maternal serum in cases of preterm birth (104, 105). Interestingly, the altered levels of MMPs and their inhibitors in the plasma of preterm infants appear to have a role in the development of bronchopulmonary dysplasia and intraventricular hemorrhage (106). Overall, there are still very few results concerning MMPs, therefore, definitive conclusions are still missing. Fetal membrane weakening can also be caused by prothrombin, which was shown to be upregulated in hAM when stimulated with Ureaplasma parvum in an in vitro study (108–110). Moreover, the disruption of tight junctions between AECs was observed and characterized by the loss of claudins 3 and 4 in mice, where IAI was induced with lipopolysaccharide (LPS) administration (88). In the same setting, apoptosis of the AECs was also observed. Studies of hAM samples, obtained at term, show high levels of endocytosis and autophagy, thought to be induced by lack of nutrients and possibly hormonal changes during the end of pregnancy (215). Autophagic death and apoptosis of hAECs are elevated at ruptured sites of the hAM, signifying their involvement in the weakening and rupture of hAM at term (216). Nevertheless, more studies are needed to decipher the role of different intrinsic cell deaths of hAECs in IAI. Infection of hAM cell line by Listeria monocytogenes, a rare but very dangerous pathogen in IAI, results in cell necrosis after bacteria have successfully multiplied, suggesting yet another way of hAM weakening (89).
Infection, inflammation, and related oxidative stress promote cell senescence of hAEC, and have been observed in hAM of premature PROM (90–92). Throughout pregnancy, EMT and the reverse mesenchymal-to-epithelial transition (MET) contribute to healing of small hAM ruptures and the maintenance of hAM integrity during the fetal growth (217). The process is partially regulated by oxidative stress and transforming growth factor β, which promote EMT, and by progesterone, which promotes MET (39, 218, 219). However, at term the homeostasis of hAM remodeling via cellular transitions is disrupted by irreversible EMT of hAECs, which is necessary for weakening of the membrane and parturition (92). Furthermore, in vitro studies with hAECs were shown to undergo EMT when stimulated with TNFα (93, 94). Therefore, an imbalance in EMT and MET may occur due to IAI and related oxidative stress, and may promote premature PROM. Altogether, the considerable changes to the extracellular matrix and hAM cells during IAI can weaken the hAM, promote preterm onset of labor, and allow further complications for the pregnancy (Figure 2).
Figure 2 Intra-amniotic infection and inflammation induce structural changes in the hAM, such as enzymatic degradation of extracellular matrix (ECM), EMT of hAEC, senescence, apoptosis and necrotic death of hAEC, and loss of tight junctions between hAEC, all of which weakens the hAM and can cause PROM. Change in levels of numerous molecules, as well as prostaglandin-prostamide ratio, can induce labor and lead to preterm labor.
In the hAF of women with IAI the total leukocyte count is up to 100-fold increased (68, 120, 140, 155, 220, 221). The leukocytes are also elevated in preterm PROM, especially in extremely early gestation (103). There is a general increase in T lymphocyte numbers associated with hAF inflammation, which is largely due to T helper cells, although NK and cytotoxic T lymphocytes have been implicated as well (68, 120, 221, 222). Importantly, the number of phagocytic monocytes/macrophages and neutrophils is drastically higher in the hAF of IAI cases (68, 114, 120, 221–223). Neutrophils found in the hAF during IAI, can mostly originate from the mother, or fetus, or both (112, 113), and have been shown to phagocytose bacteria and form neutrophil extracellular traps (NETs) (114, 115). NETs are actively formed by a process called NETosis, during which the chromatin and antimicrobial proteins are released into neutrophil vicinity, thereby entrapping, disarming, and killing the pathogens (224). Unsurprisingly, the immune cells are also present in amnio-chorionic membrane, and the presence (and abundance) of neutrophils or lymphocytes defines the stage of acute or chronic chorioamnionitis, respectively (116, 117). Neutrophils and monocytes/macrophages are most abundant in hAM in acute chorioamnionitis and natural killer cells can also be found, while CD3+ and CD8+ lymphocytes are present in moderate amounts in chronic chorioamnionitis with smaller number of CD4+ (helper T) cells (115–117, 141, 225–227). As is explained in the reviews by Cappelletti et al. (80) and Hoo et al. (227), during normal pregnancy, the decidual-placental interface is rich with immune cells, which become activated in IAI and migrate towards amnio-chorionic membrane (80). The immune cells involved in the inflammation are of maternal and fetal origin, and must maintain a delicate balance of eliminating an infection, while preventing the damage to the fetus. The role of both populations, maternal and fetal, should be further studied. A recent paper by Gomez-Lopez (228) suggests that the activation of fetal T lymphocytes in the hAF may be enough to cause preterm birth in some cases (228). The presence and activation of immune cells, however, is the result of a complex regulatory network that begins with the identification of pathogens or their components.
Pattern recognition receptors are proteins that have evolved to recognize conserved features of microbes PAMPs and molecules released by damaged cells DAMPs (229, 230). PRRs can be divided into four major groups, namely 1) Toll-like receptors (TLRs), 2) the nucleotide-binding oligomerization domain (NOD)- Leucin Rich Repeats (LRR)-containing receptors (NLRs), 3) the retinoic acid-inducible gene 1 (RIG-1)-like receptors (RLRs) and 4) the C-type lectin receptors (CLRs) (229, 231). The engagement of PRRs on the cells of the innate immune system induces co-stimulatory signals for the cells of the adaptive immune system (229, 232, 233). DAMPs and PAMPs, which trigger PRRs, can indirectly cause tissue remodeling and fetal membrane weakening, though the regulation of these processes in the context of IAI has not been elucidated yet (40). Furthermore, hAECs release exosomes packed with inflammatory mediators, such as DAMPs, PAMPs and SASP, which induce inflammation in uterine tissue that promotes (preterm) labor (213, 234–236).
TLRs1-10 are expressed in hAEC and hAMSC at transcriptional level under normal (non-IAI) conditions at term (122, 237, 238). Specifically, TLR1, TLR2, TLR4, TLR5, TLR7, TLR8, TLR9, and heterodimer TLR6/2 are involved in active sensing and regulation of IAI (122–127). For example, LPS simultaneously binds to myeloid differentiation factor 2 and TLR4 that relays signalling of NF-κB through a TLR mediator MyD88, thereby inducing cytokine release (123, 128, 129). Alternatively, TLR4 activation in hAEC can lead to apoptosis via Bax/Bcl-2 caspase-3 pathway, thereby triggering a different immune response (122). IL-1R-associated kinase 1 (IRAK1), which is a mediator in TLR/IL-1 pathway, is increased in the hAF in IAI, and showed strong response to intra-amniotic LPS stimulation in animal models (130). IRAK2 plays a similar role and is upregulated in women with preterm IAI (127).
Cytoplasmic PRRs, referred as NLRs, namely NLRP1, NLRP3, NLRC4 and NOD2 were increased at transcriptional level in the presence of IAI in women with preterm labor, although NOD2 was decreased at protein level (118). In a mouse model Faro et al. (132) demonstrated that LPS upregulated NLRP3 inflammasome and downstream caspase-1 and IL-1β at mRNA and protein level, ultimately leading to IAI-induced preterm birth (132). Moreover, several studies in humans have demonstrated increased levels of apoptosis-associated speck-like protein containing a CARD (ASC; adaptor protein involved in inflammasome assembly) in infection and inflammation (133, 220, 239). In addition, functional ASC activation observed by speck formation has been demonstrated in vitro in hAEC and hAMSC (240). Assembled and activated NLRP3 inflammasome cleaves pro-caspase-1, which in turn cleaves and activates IL-1β, IL-18 and gasdermin D (134, 135). Gasdermin D induces an inflammatory cell death pyroptosis, which releases cytokines IL-1β and IL-18 into the environment (136, 137). In line with this, several studies have indicated that IAI leads to an increase of caspase-1, gasdermin D and IL-1β and IL-18 in the hAM and hAF (118, 133, 138). The activation of PRR inflammatory pathways has multiple consequences, 1) it can cause cell death via various pathways, thereby damaging the integrity of the hAM; 2) PRR downstream signalling changes the expression profile of cells, synthesizing or activating the effector molecules; 3) living cells and cells undergoing inflammatory cell death release the cytokines in the environment, promoting inflammation. Altogether, the PRR activation has evolved to respond to the infection, but in the case of IAI, the inflammation itself is often equally damaging, as it can lead to preterm birth.
Numerous studies demonstrated an increase in pro-inflammatory IL-1β, IL18, TNFα, granulocyte-macrophage colony-stimulating factor, macrophage migration inhibitory factor, high-mobility group protein 1 (118, 121, 128, 129, 141–146, 201), anti-inflammatory IL10, nicotinamide phosphoribosyltransferase (128, 147, 201, 241),, and regulatory IL-6 (128, 129, 144, 146–151, 201, 241) cytokines in the hAF with IAI or preterm labor conditions. In fact, the IL-6 is considered as an important biomarker of IAI (241–243) and an increased presence of IL-6 (>2.6 ng/ml) in the hAF is used to define inflammation (244). Moreover, chemokines IL-16, IL-8, IP-10, monocyte chemoattractant protein-1, chemokine (C-X-C motif) ligands (CXCLs)-1, -3, -4, -5, -6, -11, and L-selectin have also been upregulated with IAI and related in vitro models (128, 146, 147, 154–159). Two comprehensive studies by Bhatti et al. (152) and Romero et al. (140) further reveal the plethora of IAI-induced cytokines in the hAF (140, 152). Of note, the type (e.g. Gram positive, Gram negative, or other) and amount of bacterial pathogens affect the quantity and diversity of cytokine upsurge in the hAF or hAM cells (124, 159, 245, 246). For example, the treatment of amnio-chorionic membrane with heat-inactivated Escherichia coli and Gardnerella vaginalis strongly induced secretion of IL-1β, while there were no statistically significant changes in IL-1β secretion when the amnio-chorionic membrane were treated with Group B streptococci, Mycoplasma hominis, Ureaplasma urealyticum or Ureaplasma parvum. Similarly, there was a strong increase in TNF-α secretion when the amnio-chorionic membrane were treated with E. coli, Porphyromonas gingivalis and G. vaginalis, while the treatment with M. hominis, U. urealyticum and U. parvum only moderately increased the secretion of TNF-α and there was no change in TNF-α secretion in case of treatment with Group B streptococci (245). In another study the amnio-chorionic membrane were treated with heat-inactivated E. coli, G. vaginalis, Group B streptococci and U. parvum, which led to the increase in IL-6 and IL-10 secretion. On the other hand, there was a statistically significant increase in IFNγ secretion only when the amnio-chorionic membrane were treated with E. coli (247). These studies underline the variety of the immune response depending on the bacterial species. Moreover, this together with technical reasons in study design (small sample size, different measurement techniques), as well as natural endogenous variability of the immune response explains why some cytokines were not always consistently increased upon IAI (120, 144, 248).
Antimicrobial peptides (AMPs) are typically small proteins with a positive charge that directs them to negatively charged bacterial surface. Most commonly they have a broad-spectrum activities, can work synergistically and can disrupt bacterial membrane, causing cell lysis (AMPs are nicely reviewed in (160, 161). AMPs are expressed by the hAM cells (162–165) and are present in the hAF (23, 162, 166–173). They play an important role in the amniotic defence against pathogens and can be constitutively expressed or upregulated in IAI. Most notable are human beta defensins (HBDs)1-3, elafin and secretory leukocyte protease inhibitor (SLPI), which are expressed by hAM cells (162, 174, 175). Specifically, HBD3 was shown to be upregulated in LPS and peptidoglycan presence, both of which are components of bacterial cell wall (176). The amounts of HBD1-3, elafin and SLPI are also increased in the case of IAI (23, 102, 162, 172, 173), though there is some evidence that SLPI and elafin are decreased in preterm PROM (174, 249). Human neutrophil (or alpha) defensins (HNPs)1-3, lysozyme and lactoferrin were also found in the hAF in women with non-complicated pregnancies (170, 182). Moreover, HNP1-3, calgranulin/calprotectin, bactericidal permeability-increasing protein, lactoferrin, lipocalin 2 and cathelicidin family members are increased in the hAF of IAI or preterm labor (100, 101, 150, 152, 166, 168, 169, 171, 177, 178, 183, 179, 180) Overall, the presence and activation of numerous variable AMPs in normal pregnancy and in IAI imply an important role of AMPs in prevention and resolution of infections.
Bioactive lipids, such as prostaglandins, are crucial in the onset of labor, specifically in the induction of uterine contractions, and are in fact used to artificially stimulate labor (250–252). Given that they are also involved in inflammation, they might be involved with induction of preterm labor in the case of IAI (184). Even though there are a few conflicting results, most studies show that hAF of IAI patients has increased prostaglandin-prostamide ratio due to an increase of prostaglandins PGE2, PGF2 and PGF2α and decrease of their respective prostamides (155, 185–194). Similar conclusions were obtained in in vitro models, in which LPS, IL-1β, TNFα, or whole bacteria stimulation was used to mimic infection and inflammation, though 13,14-dihydro-15-keto-PGF2α levels were decreased in one study (139, 195, 196). Additionally, Gillaux et al. (122) demonstrated an upregulation of prostaglandin-endoperoxide synthase 2, a key enzyme in the synthesis of prostaglandins, through TLR5 and TLR6/2 stimulation in hAECs (122). Interestingly, Maddipati et al. (191) detected a decrease in lipids with anti-inflammatory/proresolution properties in the case of IAI (191). Perhaps most striking is an observation that women with a PGF2α concentration in the hAF above the 95th percentile had a higher rate of IAI, shorter amniocentesis-to-delivery time, and significantly more preterm deliveries (190, 253). Additionally, proinflammatory lipid mediators belonging to the 5-lipoxygenase pathway (5-hydroxyeicosatetraenoic acid and leukotriene B4) are increased in IAI in comparison to sterile inflammation (254).
The complement system is composed of over 40 proteins (serine proteases, receptors and regulators), and it becomes activated through one of the three pathways depending on the trigger – classical, alternative or lectin pathway. After the initial trigger molecule binding, the convertase enzymes cleave proteins C3 and C5, leading to the assembly of membrane attack complex on the microbe membrane that causes the lysis of the pathogen (255, 256). Besides direct destruction of pathogens, the complement system is also a mediator for several innate and adaptive inflammatory processes (256, 257). Hammerschmidt et al. (258) demonstrated that hAF is capable of activating complement, with higher activation in hAF from women with distressed pregnancies, meaning that the women suffered from amnionitis, nonimmune hydrops fetalis, class T diabetes, or severe preeclampsia (258). Another study showed that elevated hAF levels of C3a, but not C5a, were associated with IAI and preterm delivery in women with cervical insufficiency or a short cervix (199). Moreover, the concentration of catalytical subunit of complement factor B (Bb) in hAF was higher in the case of IAI among women with preterm labor or preterm PROM, indicating the activation by alternative complement pathway (200). In addition, regulation of complement activation could be mediated by a membrane-bound complement inhibitor CD59, which was shown to be expressed by hAEC and hAMSC (259). The existing studies show the involvement of complement system in IAI, however, more research is needed to determine the extent of activation, triggered pathways, and the significance of complement activation in this setting.
Numerous studies that were mentioned in this review have proven that all parts of the innate immune response are active in the hAM and hAF in the presence of IAI. The components of this response are summed up in Table 1, along with their respective function and response to IAI. Other pathways and molecules with less-obvious connection to the immune system are also listed in Table 1 and their importance as biomarkers is discussed in Chapter 4.3. Given the complexity of the immune response and the consequences, it has become clear that even though the role of the immune response is to prevent or eradicate the pathogens, severe inflammation itself might cause additional undesired complications for the fetus.
Due to its structural and physiological differences, pathogen species is an important factor in IAI immune response (124, 125, 159, 202, 245, 246). Moreover, there are several environmental and endogenous factors that influence the immune response, not only its strength, but also which of its pathways are triggered. For example, it was observed decades ago that black and African American women have a higher prevalence of poor perinatal outcomes, and later studies show significant differences in hAF immune system response between different races (245, 260–264). For instance, it has been shown that in comparison to African Americans, Caucasians have higher hAF levels of soluble TNF receptor in response to IAI, meaning that TNF-α activity in African Americans is more pronounced (265). Recent advances have shown that these differences are partly a result of genetic predispositions, and multiple single nucleotide polymorphisms were linked to an increased risk for IAI and spontaneous preterm birth (262, 263, 266–269). Nevertheless, environmental factors, such as psychosocial and socioeconomic factors, maternal stress, and epigenetic changes, contribute to the disparities between demographic groups (270–274). For example, psychological stress and depression likely promote preterm birth through inflammation, as is evidenced by increased proinflammatory cytokines IL-6 and TNF-α in the maternal serum (275, 276). Additionally, lifestyle determinants, in particular inadequate diet and nutrition, higher body mass index or obesity, smoking and alcohol consumption, were shown to be relevant risk factors for preterm birth (277–283). For instance, obese patients (class II and III) had increased hAF concentrations of IL-1β and IL-6, and MMP-1, MMP-6, and MMP-13 (284). Finally, the maternal infection with SARS-Cov-2 has been associated with higher incidence of premature delivery, PROM, and neonatal intensive care unit admissions, and the effect of SARS-Cov-2 on pregnancy is currently under intense investigation (54, 55, 285).
In this review we have focused on the innate immune system components present in the hAM and hAF. However, other molecules and pathways have been monitored in relation to IAI, preterm labor and preterm birth in order to find specific and reliable biomarkers to evaluate the risk of pregnancy complications. For instance, a study by Hong et al. (178) identified several proteins and pathways with altered protein levels in the hAF, such as vascular endothelial growth factor receptor 1 and Fc fragment of IgG binding protein, and pathways of glycolysis, gluconeogenesis, and iron homeostasis (178). In line with this, glucose concentration in the hAF was lower in case of IAI, while hepcidin – a regulator of iron release – was increased (203, 204). Understandably, neutrophil products myeloperoxidase and neutrophil elastase have also been increased in the presence of microbes in the hAF (102, 174, 205), as have been signaling molecules nitric oxide metabolites (197, 202, 206). To name a few more, neurotrophin 3, brain-derived neurotrophic factor, triggering receptor expressed on myeloid cells 1, resistin, soluble human leukocyte antigen-G, angiopoietin-2, cell-free DNA, and histones H3, H4, H2B have all been increased in hAF of women with IAI (100, 201, 207–211). Currently, the samples for determination of IAI are obtained by amniocentesis, which is an invasive approach, while ideally, biomarkers for pregnancy complications should be measured from harmlessly obtained sources. So far, maternal blood, urine, cervicovaginal fluid, and even saliva have been proposed (286–292). Lamont et al. (293) outlined the potential, feasibility and problems of the existing biomarkers, including their retrieval, and hopefully, increasing endeavors and knowledge will bring reliable and safely obtained biomarkers to the clinic in the near future (293).
It is important to note that other tissues, such as placenta and maternal blood, are also involved in the immune response that occurs in the hAF. For example, maternal and fetal neutrophils are recruited to the hAF during IAI (113). Specifically, decidua acts as an important immune cell reservoir that is heavily involved in the immune response in IAI acting from maternal side, and two recent reviews have covered decidual role in IAI (80, 227). Due to the high complexity and extent of the immune system related to the IAI, we have not covered the contributions of other tissues and adaptive immune response in this paper, but they should be considered as well. Understanding the engagement of other relevant tissues in IAI immune response may also prove beneficial in detecting the IAI.
In conclusion, the innate immune response of the hAF and hAM is a complex and highly regulated process, signified by the involvement of immune cells, mediators and effectors discussed in this paper. However, because of the complexity, an infection might not always be resolved, or the inflammation might become uncontrolled, both of which present a danger of pregnancy complications that may harm the fetus. Thus, it is vital that we understand the pathogenesis of different microorganisms and the variable involvement of the innate immune system of the hAF and hAM. While most of the studies covered in this article were designed to detect specific parts of the immune response, such as immune cells, cytokines, or AMPs, there is a lack of comprehensive and mechanistic studies. Such studies, covering all parts of the immune response in a specific setting, or studies that would reveal detailed regulation of the immune signalling network depending on the pathogen and endogenous factors, would give us a more holistic view on the interplay between a pathogen and the immune response in the hAM and hAF. This understanding is critical in early detection of IAI, its categorization, and sequential treatment options, which will altogether lower the incidence of pregnancy complications and related risks for the fetus.
Conceptualization, TŠ, TŽR, MSE, and MEK. Visualization, TŠ, Writing – original draft preparation, TŠ and TŽR. Writing – review and editing, TŠ, TŽ̌R, MSE, and MEK. All authors contributed to the article andapproved the submitted version.
This research was funded by the Slovenian Research Agency (project J7-2594, and research core funding no. P3-0108, P1-0198) and the MRIC UL IP-0510 Infrastructure program.
The authors declare that the research was conducted in the absence of any commercial or financial relationships that could be construed as a potential conflict of interest.
All claims expressed in this article are solely those of the authors and do not necessarily represent those of their affiliated organizations, or those of the publisher, the editors and the reviewers. Any product that may be evaluated in this article, or claim that may be made by its manufacturer, is not guaranteed or endorsed by the publisher.
This work contributes to the COST Action CA17116 International Network for Translating Research on Perinatal Derivatives into Therapeutic Approaches (SPRINT), supported by COST (European Cooperation in Science and Technology).
1. Silini AR, Di Pietro R, Lang-Olip I, Alviano F, Banerjee A, Basile M, et al. Perinatal Derivatives: Where Do We Stand? A Roadmap of the Human Placenta and Consensus for Tissue and Cell Nomenclature. Front Bioeng Biotechnol (2020) 8:610544. doi: 10.3389/fbioe.2020.610544
2. Stewart JR. “Chapter 9 - Morphology and Evolution of the Egg of Oviparous Amniotes”. In: Sumida SS, Martin KLM, editors. Amniote Origins. San Diego: Academic Press (1997). p. 291–326.
3. Sues HD. Amniotes, Diversification of. In: Kliman RM, editor. Encyclopedia of Evolutionary Biology. Oxford: Academic Press (2016). p. 56–62.
4. Underwood MA, Gilbert WM, Sherman MP. Amniotic Fluid: Not Just Fetal Urine Anymore. J Perinatol (2005) 25(5):341–8. doi: 10.1038/sj.jp.7211290
5. Underwood MA, Sherman MP. Nutritional Characteristics of Amniotic Fluid. NeoReviews (2006) 7(6):e310. doi: 10.1542/neo.7-6-e310
6. Mamede AC, Carvalho MJ, Abrantes AM, Laranjo M, Maia CJ, Botelho MF. Amniotic Membrane: From Structure and Functions to Clinical Applications. Cell Tissue Res (2012) 349(2):447–58. doi: 10.1007/s00441-012-1424-6
7. Da Silva M, Dombre C, Brionne A, Monget P, Chessé M, De Pauw M, et al. The Unique Features of Proteins Depicting the Chicken Amniotic Fluid. Mol Cell Proteomics (2019) 18(Suppl 1):S174–S90. doi: 10.1074/mcp.RA117.000459
8. Danforth D, Hull RW. The Microscopic Anatomy of the Fetal Membranes With Particular Reference to the Detailed Structure of the Amnion. Am J Obstet Gynecol (1958) 75(3):536–47; discussion 48-50. doi: 10.1016/0002-9378(58)90610-0
9. Bourne GL. The Microscopic Anatomy of the Human Amnion and Chorion. Am J Obstet Gynecol (1960) 79:1070–3. doi: 10.1016/0002-9378(60)90512-3
10. Benirschke K, Driscoll SG. The Pathology of the Human Placenta. In: Strauss F, Benirschke K, Driscoll SG, editors. Placenta. Berlin, Heidelberg: Springer Berlin Heidelberg (1967). p. 97–571.
11. Miki T, Lehmann T, Cai H, Stolz DB, Strom SC. Stem Cell Characteristics of Amniotic Epithelial Cells. Stem Cells (2005) 23(10):1549–59. doi: 10.1634/stemcells.2004-0357
12. García-Castro IL, García-López G, Ávila-González D, Flores-Herrera H, Molina-Hernández A, Portillo W, et al. Markers of Pluripotency in Human Amniotic Epithelial Cells and Their Differentiation to Progenitor of Cortical Neurons. PLoS One (2015) 10(12):e0146082. doi: 10.1371/journal.pone.0146082
13. Evron A, Goldman S, Shalev E. Human Amniotic Epithelial Cells Cultured in Substitute Serum Medium Maintain Their Stem Cell Characteristics for Up to Four Passages. Int J Stem Cells (2011) 4(2):123–32. doi: 10.15283/ijsc.2011.4.2.123
14. Bryzek A, Czekaj P, Plewka D, Komarska H, Tomsia M, Lesiak M, et al. Expression and Co-Expression of Surface Markers of Pluripotency on Human Amniotic Cells Cultured in Different Growth Media. Ginekol Pol (2013) 84(12):1012–24. doi: 10.17772/gp/1673
15. Ilancheran S, Michalska A, Peh G, Wallace EM, Pera M, Manuelpillai U. Stem Cells Derived From Human Fetal Membranes Display Multilineage Differentiation Potential. Biol Reprod (2007) 77(3):577–88. doi: 10.1095/biolreprod.106.055244
16. Zhou K, Koike C, Yoshida T, Okabe M, Fathy M, Kyo S, et al. Establishment and Characterization of Immortalized Human Amniotic Epithelial Cells. Cell Reprogram (2013) 15(1):55–67. doi: 10.1089/cell.2012.0021
17. Parolini O, Alviano F, Bagnara GP, Bilic G, Buhring HJ, Evangelista M, et al. Concise Review: Isolation and Characterization of Cells From Human Term Placenta: Outcome of the First International Workshop on Placenta Derived Stem Cells. Stem Cells (2008) 26(2):300–11. doi: 10.1634/stemcells.2007-0594
18. Bačenková D, Rosocha J, Tóthová T, Rosocha L, Šarisský M. Isolation and Basic Characterization of Human Term Amnion and Chorion Mesenchymal Stromal Cells. Cytotherapy (2011) 13(9):1047–56. doi: 10.3109/14653249.2011.592522
19. Ding C, Li H, Wang Y, Wang F, Wu H, Chen R, et al. Different Therapeutic Effects of Cells Derived From Human Amniotic Membrane on Premature Ovarian Aging Depend on Distinct Cellular Biological Characteristics. Stem Cell Res Ther (2017) 8(1):173. doi: 10.1186/s13287-017-0613-3
20. Weidinger A, Poženel L, Wolbank S, Banerjee A. Sub-Regional Differences of the Human Amniotic Membrane and Their Potential Impact on Tissue Regeneration Application. Front Bioeng Biotechnol (2020) 8:613804. doi: 10.3389/fbioe.2020.613804
21. Alviano F, Fossati V, Marchionni C, Arpinati M, Bonsi L, Franchina M, et al. Term Amniotic Membrane Is a High Throughput Source for Multipotent Mesenchymal Stem Cells With the Ability to Differentiate Into Endothelial Cells In Vitro. BMC Dev Biol (2007) 7:11. doi: 10.1186/1471-213X-7-11
22. Michaels JE, Dasari S, Pereira L, Reddy AP, Lapidus JA, Lu X, et al. Comprehensive Proteomic Analysis of the Human Amniotic Fluid Proteome: Gestational Age-Dependent Changes. J Proteome Res (2007) 6(4):1277–85. doi: 10.1021/pr060543t
23. Soto E, Espinoza J, Nien JK, Kusanovic JP, Erez O, Richani K, et al. Human Beta-Defensin-2: A Natural Antimicrobial Peptide Present in Amniotic Fluid Participates in the Host Response to Microbial Invasion of the Amniotic Cavity. J Matern Fetal Neonatal Med (2007) 20(1):15–22. doi: 10.1080/14767050601036212
24. Kubo M, Sonoda Y, Muramatsu R, Usui M. Immunogenicity of Human Amniotic Membrane in Experimental Xenotransplantation. Invest Ophthalmol Vis Sci (2001) 42(7):1539–46.
25. Magatti M, Vertua E, Cargnoni A, Silini A, Parolini O. The Immunomodulatory Properties of Amniotic Cells: The Two Sides of the Coin. Cell Transplant (2018) 27(1):31–44. doi: 10.1177/0963689717742819
26. Wassmer CH, Berishvili E. Immunomodulatory Properties of Amniotic Membrane Derivatives and Their Potential in Regenerative Medicine. Curr Diabetes Rep (2020) 20(8):31. doi: 10.1007/s11892-020-01316-w
27. Pianta S, Magatti M, Vertua E, Bonassi Signoroni P, Muradore I, Nuzzo AM, et al. Amniotic Mesenchymal Cells From Pre-Eclamptic Placentae Maintain Immunomodulatory Features as Healthy Controls. J Cell Mol Med (2016) 20(1):157–69. doi: 10.1111/jcmm.12715
28. Banas R, Miller C, Guzik L, Zeevi A. Amnion-Derived Multipotent Progenitor Cells Inhibit Blood Monocyte Differentiation Into Mature Dendritic Cells. Cell Transplant (2014) 23(9):1111–25. doi: 10.3727/096368913X670165
29. Magatti M, De Munari S, Vertua E, Nassauto C, Albertini A, Wengler GS, et al. Amniotic Mesenchymal Tissue Cells Inhibit Dendritic Cell Differentiation of Peripheral Blood and Amnion Resident Monocytes. Cell Transplant (2009) 18(8):899–914. doi: 10.3727/096368909X471314
30. Kronsteiner B, Peterbauer-Scherb A, Grillari-Voglauer R, Redl H, Gabriel C, van Griensven M, et al. Human Mesenchymal Stem Cells and Renal Tubular Epithelial Cells Differentially Influence Monocyte-Derived Dendritic Cell Differentiation and Maturation. Cell Immunol (2011) 267(1):30–8. doi: 10.1016/j.cellimm.2010.11.001
31. Magatti M, De Munari S, Vertua E, Gibelli L, Wengler GS, Parolini O. Human Amnion Mesenchyme Harbors Cells With Allogeneic T-Cell Suppression and Stimulation Capabilities. Stem Cells (2008) 26(1):182–92. doi: 10.1634/stemcells.2007-0491
32. Magatti M, Vertua E, De Munari S, Caro M, Caruso M, Silini A, et al. Human Amnion Favours Tissue Repair by Inducing the M1-To-M2 Switch and Enhancing M2 Macrophage Features. J Tissue Eng Regener Med (2017) 11(10):2895–911. doi: 10.1002/term.2193
33. Li H, Niederkorn JY, Neelam S, Mayhew E, Word RA, McCulley JP, et al. Immunosuppressive Factors Secreted by Human Amniotic Epithelial Cells. Invest Ophthalmol Vis Sci (2005) 46(3):900–7. doi: 10.1167/iovs.04-0495
34. Karlsson H, Erkers T, Nava S, Ruhm S, Westgren M, Ringdén O. Stromal Cells From Term Fetal Membrane Are Highly Suppressive in Allogeneic Settings In Vitro. Clin Exp Immunol (2012) 167(3):543–55. doi: 10.1111/j.1365-2249.2011.04540.x
35. Anam K, Lazdun Y, Davis PM, Banas RA, Elster EA, Davis TA. Amnion-Derived Multipotent Progenitor Cells Support Allograft Tolerance Induction. Am J Transplant (2013) 13(6):1416–28. doi: 10.1111/ajt.12252
36. Li J, Koike-Soko C, Sugimoto J, Yoshida T, Okabe M, Nikaido T. Human Amnion-Derived Stem Cells Have Immunosuppressive Properties on NK Cells and Monocytes. Cell Transplant (2015) 24(10):2065–76. doi: 10.3727/096368914X685230
37. Wolbank S, Peterbauer A, Fahrner M, Hennerbichler S, van Griensven M, Stadler G, et al. Dose-Dependent Immunomodulatory Effect of Human Stem Cells From Amniotic Membrane: A Comparison With Human Mesenchymal Stem Cells From Adipose Tissue. Tissue Eng (2007) 13(6):1173–83. doi: 10.1089/ten.2006.0313
38. Kallapur SG, Presicce P, Rueda CM, Jobe AH, Chougnet CA. Fetal Immune Response to Chorioamnionitis. Semin Reprod Med (2014) 32(1):56–67. doi: 10.1055/s-0033-1361823
39. Richardson LS, Taylor RN, Menon R. Reversible EMT and MET Mediate Amnion Remodeling During Pregnancy and Labor. Sci Signal (2020) 13(618):eaay1486. doi: 10.1126/scisignal.aay1486
40. Padron JG, Saito Reis CA, Kendal-Wright CE. The Role of Danger Associated Molecular Patterns in Human Fetal Membrane Weakening. Front Physiol (2020) 11:602. doi: 10.3389/fphys.2020.00602
41. Behnia F, Taylor BD, Woodson M, Kacerovsky M, Hawkins H, Fortunato SJ, et al. Chorioamniotic Membrane Senescence: A Signal for Parturition? Am J Obstet Gynecol (2015) 213(3):359.e1–16. doi: 10.1016/j.ajog.2015.05.041
42. Menon R, Behnia F, Polettini J, Richardson LS. Novel Pathways of Inflammation in Human Fetal Membranes Associated With Preterm Birth and Preterm Pre-Labor Rupture of the Membranes. Semin Immunopathol (2020) 42(4):431–50. doi: 10.1007/s00281-020-00808-x
43. Kinoshita K, Satoh K, Sakamoto S. Human Amniotic Membrane and Prostaglandin Biosynthesis. Biol Res Pregnancy Perinatol (1984) 5(2):61–7.
44. O'Brien WF. The Role of Prostaglandins in Labor and Delivery. Clin Perinatol (1995) 22(4):973–84. doi: 10.1016/S0095-5108(18)30265-3
45. Romero R, Gómez R, Chaiworapongsa T, Conoscenti G, Kim JC, Kim YM. The Role of Infection in Preterm Labour and Delivery. Paediatr Perinat Epidemiol (2001) 15(Suppl 2):41–56. doi: 10.1046/j.1365-3016.2001.00007.x
46. Romero R, Espinoza J, Gonçalves LF, Kusanovic JP, Friel LA, Nien JK. Inflammation in Preterm and Term Labour and Delivery. Semin Fetal Neonatal Med (2006) 11(5):317–26. doi: 10.1016/j.siny.2006.05.001
47. Combs CA, Garite TJ, Lapidus JA, Lapointe JP, Gravett M, Rael J, et al. Detection of Microbial Invasion of the Amniotic Cavity by Analysis of Cervicovaginal Proteins in Women With Preterm Labor and Intact Membranes. Am J Obstet Gynecol (2015) 212(4):482.e1–.e12. doi: 10.1016/j.ajog.2015.02.007
48. Cobo T, Vives I, Rodríguez-Trujillo A, Murillo C, Ángeles MA, Bosch J, et al. Impact of Microbial Invasion of Amniotic Cavity and the Type of Microorganisms on Short-Term Neonatal Outcome in Women With Preterm Labor and Intact Membranes. Acta Obstet Gynecol Scand (2017) 96(5):570–9. doi: 10.1111/aogs.13095
49. Kachikis A, Eckert LO, Walker C, Bardají A, Varricchio F, Lipkind HS, et al. Chorioamnionitis: Case Definition & Guidelines for Data Collection, Analysis, and Presentation of Immunization Safety Data. Vaccine (2019) 37(52):7610–22. doi: 10.1016/j.vaccine.2019.05.030
50. Seliga-Siwecka JP, Kornacka MK. Neonatal Outcome of Preterm Infants Born to Mothers With Abnormal Genital Tract Colonisation and Chorioamnionitis: A Cohort Study. Early Hum Dev (2013) 89(5):271–5. doi: 10.1016/j.earlhumdev.2012.10.003
51. Helmo FR, Alves EAR, Moreira RAA, Severino VO, Rocha LP, Monteiro MLGD, et al. Intrauterine Infection, Immune System and Premature Birth. J Matern Fetal Neonatal Med (2018) 31(9):1227–33. doi: 10.1080/14767058.2017.1311318
52. McDonagh S, Maidji E, Ma W, Chang HT, Fisher S, Pereira L. Viral and Bacterial Pathogens at the Maternal-Fetal Interface. J Infect Dis (2004) 190(4):826–34. doi: 10.1086/422330
53. Oliveira Melo AS, Malinger G, Ximenes R, Szejnfeld PO, Alves Sampaio S, Bispo de Filippis AM. Zika Virus Intrauterine Infection Causes Fetal Brain Abnormality and Microcephaly: Tip of the Iceberg? Ultrasound Obstet Gynecol (2016) 47(1):6–7. doi: 10.1002/uog.15831
54. Sisman J, Jaleel MA, Moreno W, Rajaram V, Collins RRJ, Savani RC, et al. Intrauterine Transmission of SARS-COV-2 Infection in a Preterm Infant. Pediatr Infect Dis J (2020) 39(9):e265–7. doi: 10.1097/INF.0000000000002815
55. Vivanti AJ, Vauloup-Fellous C, Prevot S, Zupan V, Suffee C, Do Cao J, et al. Transplacental Transmission of SARS-CoV-2 Infection. Nat Commun (2020) 11(1):3572. doi: 10.1038/s41467-020-17436-6
56. Tita AT, Andrews WW. Diagnosis and Management of Clinical Chorioamnionitis. Clin Perinatol (2010) 37(2):339–54. doi: 10.1016/j.clp.2010.02.003
57. Mendz GL, Kaakoush NO, Quinlivan JA. Bacterial Aetiological Agents of Intra-Amniotic Infections and Preterm Birth in Pregnant Women. Front Cell Infect Microbiol (2013) 3:58. doi: 10.3389/fcimb.2013.00058
58. Aagaard K, Ma J, Antony KM, Ganu R, Petrosino J, Versalovic J. The Placenta Harbors a Unique Microbiome. Sci Transl Med (2014) 6(237):237ra65. doi: 10.1126/scitranslmed.3008599
59. Bearfield C, Davenport ES, Sivapathasundaram V, Allaker RP. Possible Association Between Amniotic Fluid Micro-Organism Infection and Microflora in the Mouth. BJOG (2002) 109(5):527–33. doi: 10.1111/j.1471-0528.2002.01349.x
60. Jiménez E, Fernández L, Marín ML, Martín R, Odriozola JM, Nueno-Palop C, et al. Isolation of Commensal Bacteria From Umbilical Cord Blood of Healthy Neonates Born by Cesarean Section. Curr Microbiol (2005) 51(4):270–4. doi: 10.1007/s00284-005-0020-3
61. de Goffau MC, Lager S, Sovio U, Gaccioli F, Cook E, Peacock SJ, et al. Human Placenta has No Microbiome But can Contain Potential Pathogens. Nature (2019) 572(7769):329–34. doi: 10.1038/s41586-019-1451-5
62. van Well GTJ, Daalderop LA, Wolfs T, Kramer BW. Human Perinatal Immunity in Physiological Conditions and During Infection. Mol Cell Pediatr (2017) 4(1):4. doi: 10.1186/s40348-017-0070-1
63. Mei C, Yang W, Wei X, Wu K, Huang D. The Unique Microbiome and Innate Immunity During Pregnancy. Front Immunol (2019) 10:2886. doi: 10.3389/fimmu.2019.02886
64. Goldenberg RL, Hauth JC, Andrews WW. Intrauterine Infection and Preterm Delivery. N Engl J Med (2000) 342(20):1500–7. doi: 10.1056/NEJM200005183422007
65. Romero R, Gomez-Lopez N, Winters AD, Jung E, Shaman M, Bieda J, et al. Evidence That Intra-Amniotic Infections Are Often the Result of an Ascending Invasion - a Molecular Microbiological Study. J Perinat Med (2019) 47(9):915–31. doi: 10.1515/jpm-2019-0297
66. Kim MJ, Romero R, Gervasi MT, Kim JS, Yoo W, Lee DC, et al. Widespread Microbial Invasion of the Chorioamniotic Membranes Is a Consequence and Not a Cause of Intra-Amniotic Infection. Lab Invest (2009) 89(8):924–36. doi: 10.1038/labinvest.2009.49
67. Witkin SS, Linhares IM, Bongiovanni AM, Herway C, Skupski D. Unique Alterations in Infection-Induced Immune Activation During Pregnancy. BJOG (2011) 118(2):145–53. doi: 10.1111/j.1471-0528.2010.02773.x
68. Gomez-Lopez N, Romero R, Xu Y, Miller D, Leng Y, Panaitescu B, et al. The Immunophenotype of Amniotic Fluid Leukocytes in Normal and Complicated Pregnancies. Am J Reprod Immunol (2018) 79(4):e12827. doi: 10.1111/aji.12827
69. Keelan JA, Blumenstein M, Helliwell RJ, Sato TA, Marvin KW, Mitchell MD. Cytokines, Prostaglandins and Parturition–a Review. Placenta (2003) 24(Suppl A):S33–46. doi: 10.1053/plac.2002.0948
70. Adams Waldorf KM, McAdams RM. Influence of Infection During Pregnancy on Fetal Development. Reproduction (2013) 146(5):R151–62. doi: 10.1530/REP-13-0232
71. Gussenhoven R, Westerlaken RJJ, Ophelders DRMG, Jobe AH, Kemp MW, Kallapur SG, et al. Chorioamnionitis, Neuroinflammation, and Injury: Timing Is Key in the Preterm Ovine Fetus. J Neuroinflamm (2018) 15(1):113. doi: 10.1186/s12974-018-1149-x
72. Hester MS, Tulina N, Brown A, Barila G, Elovitz MA. Intrauterine Inflammation Reduces Postnatal Neurogenesis in the Hippocampal Subgranular Zone and Leads to Accumulation of Hilar Ectopic Granule Cells. Brain Res (2018) 1685:51–9. doi: 10.1016/j.brainres.2018.02.005
73. Lawrence SM, Wynn JL. Chorioamnionitis, IL-17A, and Fetal Origins of Neurologic Disease. Am J Reprod Immunol (2018) 79(5):e12803. doi: 10.1111/aji.12803
74. Ying YQ, Yan XQ, Jin SJ, Liang Y, Hou L, Niu WT, et al. Inhibitory Effect of LPS on the Proliferation of Oligodendrocyte Precursor Cells Through the Notch Signaling Pathway in Intrauterine Infection-Induced Rats. Curr Med Sci (2018) 38(5):840–6. doi: 10.1007/s11596-018-1951-9
75. Briana DD, Malamitsi-Puchner A. Chorioamnionitis In Utero, Schizophrenia in Adulthood: Limited Current Evidence-Future Research Focus? J Matern Fetal Neonatal Med (2021) 1–6. doi: 10.1080/14767058.2020.1863370
76. Amgalan A, Andescavage N, Limperopoulos C. Prenatal Origins of Neuropsychiatric Diseases. Acta Paediatr (2021) 110(6):1741–9. doi: 10.1111/apa.15766
77. Schelonka RL, Katz B, Waites KB, Benjamin DK. Critical Appraisal of the Role of Ureaplasma in the Development of Bronchopulmonary Dysplasia With Metaanalytic Techniques. Pediatr Infect Dis J (2005) 24(12):1033–9. doi: 10.1097/01.inf.0000190632.31565.83
78. Thomas W, Speer CP. Chorioamnionitis Is Essential in the Evolution of Bronchopulmonary Dysplasia–The Case in Favour. Paediatr Respir Rev (2014) 15(1):49–52. doi: 10.1016/j.prrv.2013.09.004
79. Pan J, Zhan C, Yuan T, Wang W, Shen Y, Sun Y, et al. Effects and Molecular Mechanisms of Intrauterine Infection/Inflammation on Lung Development. Respir Res (2018) 19(1):93. doi: 10.1186/s12931-018-0787-y
80. Cappelletti M, Presicce P, Kallapur SG. Immunobiology of Acute Chorioamnionitis. Front Immunol (2020) 11:649. doi: 10.3389/fimmu.2020.00649
81. Rugeles MT, Shearer GM. Alloantigen Recognition In Utero: Dual Advantage for the Fetus? Trends Immunol (2004) 25(7):348–52. doi: 10.1016/j.it.2004.04.007
82. Holt PG, Jones CA. The Development of the Immune System During Pregnancy and Early Life. Allergy (2000) 55(8):688–97. doi: 10.1034/j.1398-9995.2000.00118.x
83. Adkins B, Leclerc C, Marshall-Clarke S. Neonatal Adaptive Immunity Comes of Age. Nat Rev Immunol (2004) 4(7):553–64. doi: 10.1038/nri1394
84. Rosenberg VA, Buhimschi IA, Dulay AT, Abdel-Razeq SS, Oliver EA, Duzyj CM, et al. Modulation of Amniotic Fluid Activin-a and Inhibin-a in Women With Preterm Premature Rupture of the Membranes and Infection-Induced Preterm Birth. Am J Reprod Immunol (2012) 67(2):122–31. doi: 10.1111/j.1600-0897.2011.01074.x
85. Abe Y, Komatsubara M, Saito M, Toda M, Shinozaki H, Tamura T, et al. Activin A Is Stimulated by Tumor Necrosis Factor-Alpha and Modulates Collagen Gene Expression in Human Amniotic Cells. J Endocrinol Invest (2013) 36(7):515–20. doi: 10.3275/8816
86. Hardy JT, Buhimschi IA, McCarthy ME, Zhao G, Laky CA, Shook LL, et al. Imbalance of Amniotic Fluid Activin-A and Follistatin in Intraamniotic Infection, Inflammation, and Preterm Birth. J Clin Endocrinol Metab (2016) 101(7):2785–93. doi: 10.1210/jc.2015-4147
87. Rood KM, Buhimschi CS, Zhao G, Oliver EA, Summerfield T, Bahtiyar MO, et al. Tenascin-X in Amniotic Fluid and Reproductive Tissues of Pregnancies Complicated by Infection and Preterm Prelabor Rupture of Membranes†. Biol Reprod (2019) 100(3):773–82. doi: 10.1093/biolre/ioy216
88. Kobayashi K, Miwa H, Yasui M. Inflammatory Mediators Weaken the Amniotic Membrane Barrier Through Disruption of Tight Junctions. J Physiol (2010) 588(Pt 24):4859–69. doi: 10.1113/jphysiol.2010.197764
89. Ammendolia MG, Superti F, Bertuccini L, Seganti L, Cipriani D, Longhi C. Necrotic Cell Death in Human Amniotic Cells Infected by Listeria Monocytogenes. Int J Immunopathol Pharmacol (2009) 22(1):153–62. doi: 10.1177/039463200902200117
90. Dutta EH, Behnia F, Boldogh I, Saade GR, Taylor BD, Kacerovský M, et al. Oxidative Stress Damage-Associated Molecular Signaling Pathways Differentiate Spontaneous Preterm Birth and Preterm Premature Rupture of the Membranes. Mol Hum Reprod (2016) 22(2):143–57. doi: 10.1093/molehr/gav074
91. Dixon CL, Richardson L, Sheller-Miller S, Saade G, Menon R. A Distinct Mechanism of Senescence Activation in Amnion Epithelial Cells by Infection, Inflammation, and Oxidative Stress. Am J Reprod Immunol (2018) 79(3):10.1111/aji.12790. doi: 10.1111/aji.12790
92. Richardson LS, Radnaa E, Urrabaz-Garza R, Lavu N, Menon R. Stretch, Scratch, and Stress: Suppressors and Supporters of Senescence in Human Fetal Membranes. Placenta (2020) 99:27–34. doi: 10.1016/j.placenta.2020.07.013
93. de Castro Silva M, Richardson LS, Kechichian T, Urrabaz-Garza R, da Silva MG, Menon R. Inflammation, But Not Infection, Induces EMT in Human Amnion Epithelial Cells. Reproduction (2020) 160(4):627–38. doi: 10.1530/REP-20-0283
94. Janzen C, Sen S, Lei MY, Gagliardi de Assumpcao M, Challis J, Chaudhuri G. The Role of Epithelial to Mesenchymal Transition in Human Amniotic Membrane Rupture. J Clin Endocrinol Metab (2017) 102(4):1261–9. doi: 10.1210/jc.2016-3150
95. Vadillo-Ortega F, Estrada-Gutiérrez G. Role of Matrix Metalloproteinases in Preterm Labour. BJOG (2005) 112 Suppl 1:19–22. doi: 10.1111/j.1471-0528.2005.00579.x
96. Fortunato SJ, Menon R, Lombardi SJ. MMP/TIMP Imbalance in Amniotic Fluid During PROM: An Indirect Support for Endogenous Pathway to Membrane Rupture. J Perinat Med (1999) 27(5):362–8. doi: 10.1515/JPM.1999.049
97. Maymon E, Romero R, Pacora P, Gomez R, Athayde N, Edwin S, et al. Human Neutrophil Collagenase (Matrix Metalloproteinase 8) in Parturition, Premature Rupture of the Membranes, and Intrauterine Infection. Am J Obstet Gynecol (2000) 183(1):94–9. doi: 10.1016/S0002-9378(00)99072-0
98. Maymon E, Romero R, Chaiworapongsa T, Berman S, Conoscenti G, Gomez R, et al. Amniotic Fluid Matrix Metalloproteinase-8 in Preterm Labor With Intact Membranes. Am J Obstet Gynecol (2001) 185(5):1149–55. doi: 10.1067/mob.2001.118165
99. Flores-Herrera H, García-López G, Díaz NF, Molina-Hernández A, Osorio-Caballero M, Soriano-Becerril D, et al. An Experimental Mixed Bacterial Infection Induced Differential Secretion of Proinflammatory Cytokines (IL-1β, Tnfα) and proMMP-9 in Human Fetal Membranes. Placenta (2012) 33(4):271–7. doi: 10.1016/j.placenta.2012.01.007
100. Tambor V, Kacerovsky M, Lenco J, Bhat G, Menon R. Proteomics and Bioinformatics Analysis Reveal Underlying Pathways of Infection Associated Histologic Chorioamnionitis in pPROM. Placenta (2013) 34(2):155–61. doi: 10.1016/j.placenta.2012.11.028
101. Rood KM, Buhimschi IA, Rodewald Millen K, Bahtiyar MO, Thung S, Summerfield T, et al. Evidence for Participation of Neutrophil Gelatinase-Associated Lipocalin/Matrix Metalloproteinase-9 (NGAL•MMP-9) Complex in the Inflammatory Response to Infection in Pregnancies Complicated by Preterm Birth. Am J Reprod Immunol (2016) 76(2):108–17. doi: 10.1111/aji.12523
102. Myntti T, Rahkonen L, Nupponen I, Pätäri-Sampo A, Tikkanen M, Sorsa T, et al. Amniotic Fluid Infection in Preterm Pregnancies With Intact Membranes. Dis Markers (2017) 2017:8167276. doi: 10.1155/2017/8167276
103. Oh KJ, Romero R, Park JY, Hong JS, Yoon BH. The Earlier the Gestational Age, the Greater the Intensity of the Intra-Amniotic Inflammatory Response in Women With Preterm Premature Rupture of Membranes and Amniotic Fluid Infection by Ureaplasma Species. J Perinat Med (2019) 47(5):516–27. doi: 10.1515/jpm-2019-0003
104. Sundrani D, Narang A, Mehendale S, Joshi S, Chavan-Gautam P. Investigating the Expression of MMPs and TIMPs in Preterm Placenta and Role of CpG Methylation in Regulating MMP-9 Expression. IUBMB Life (2017) 69(12):985–93. doi: 10.1002/iub.1687
105. Tency I, Verstraelen H, Kroes I, Holtappels G, Verhasselt B, Vaneechoutte M, et al. Imbalances Between Matrix Metalloproteinases (MMPs) and Tissue Inhibitor of Metalloproteinases (TIMPs) in Maternal Serum During Preterm Labor. PLoS One (2012) 7(11):e49042. doi: 10.1371/journal.pone.0049042
106. Schulz CG, Sawicki G, Lemke RP, Roeten BM, Schulz R, Cheung PY. MMP-2 and MMP-9 and Their Tissue Inhibitors in the Plasma of Preterm and Term Neonates. Pediatr Res (2004) 55(5):794–801. doi: 10.1203/01.PDR.0000120683.68630.FB
107. McGregor JA, Lawellin D, Franco-Buff A, Todd JK, Makowski EL. Protease Production by Microorganisms Associated With Reproductive Tract Infection. Am J Obstet Gynecol (1986) 154(1):109–14. doi: 10.1016/0002-9378(86)90404-7
108. Kumar D, Schatz F, Moore RM, Mercer BM, Rangaswamy N, Mansour JM, et al. The Effects of Thrombin and Cytokines Upon the Biomechanics and Remodeling of Isolated Amnion Membrane, In Vitro. Placenta (2011) 32(3):206–13. doi: 10.1016/j.placenta.2011.01.006
109. Kumar D, Moore RM, Mercer BM, Mansour JM, Redline RW, Moore JJ. The Physiology of Fetal Membrane Weakening and Rupture: Insights Gained From the Determination of Physical Properties Revisited. Placenta (2016) 42:59–73. doi: 10.1016/j.placenta.2016.03.015
110. Feng L, Allen TK, Marinello WP, Murtha AP. Infection-Induced Thrombin Production: A Potential Novel Mechanism for Preterm Premature Rupture of Membranes (PPROM). Am J Obstet Gynecol (2018) 219(1):101.e1–.e12. doi: 10.1016/j.ajog.2018.04.014
111. Strauss JF. Extracellular Matrix Dynamics and Fetal Membrane Rupture. Reprod Sci (2013) 20(2):140–53. doi: 10.1177/1933719111424454
112. Sampson JE, Theve RP, Blatman RN, Shipp TD, Bianchi DW, Ward BE, et al. Fetal Origin of Amniotic Fluid Polymorphonuclear Leukocytes. Am J Obstet Gynecol (1997) 176(1 Pt 1):77–81. doi: 10.1016/S0002-9378(97)80015-4
113. Gomez-Lopez N, Romero R, Xu Y, Leng Y, Garcia-Flores V, Miller D, et al. Are Amniotic Fluid Neutrophils in Women With Intraamniotic Infection and/or Inflammation of Fetal or Maternal Origin? Am J Obstet Gynecol (2017) 217(6):693.e1–.e16. doi: 10.1016/j.ajog.2017.09.013
114. Gomez-Lopez N, Romero R, Garcia-Flores V, Xu Y, Leng Y, Alhousseini A, et al. Amniotic Fluid Neutrophils can Phagocytize Bacteria: A Mechanism for Microbial Killing in the Amniotic Cavity. Am J Reprod Immunol (2017) 78(4):10.1111/aji.12723. doi: 10.1111/aji.12723
115. Gomez-Lopez N, Romero R, Leng Y, Garcia-Flores V, Xu Y, Miller D, et al. Neutrophil Extracellular Traps in Acute Chorioamnionitis: A Mechanism of Host Defense. Am J Reprod Immunol (2017) 77(3):10.1111/aji.12617. doi: 10.1111/aji.12617
116. Jacques SM, Qureshi F. Chronic Chorioamnionitis: A Clinicopathologic and Immunohistochemical Study. Hum Pathol (1998) 29(12):1457–61. doi: 10.1016/S0046-8177(98)90016-8
117. Kim CJ, Romero R, Chaemsaithong P, Chaiyasit N, Yoon BH, Kim YM. Acute Chorioamnionitis and Funisitis: Definition, Pathologic Features, and Clinical Significance. Am J Obstet Gynecol (2015) 213(4 Suppl):S29–52. doi: 10.1016/j.ajog.2015.08.040
118. Gomez-Lopez N, Romero R, Xu Y, Plazyo O, Unkel R, Leng Y, et al. A Role for the Inflammasome in Spontaneous Preterm Labor With Acute Histologic Chorioamnionitis. Reprod Sci (2017) 24(10):1382–401. doi: 10.1177/1933719116687656
119. Gomez-Lopez N, Romero R, Leng Y, Xu Y, Slutsky R, Levenson D, et al. The Origin of Amniotic Fluid Monocytes/Macrophages in Women With Intra-Amniotic Inflammation or Infection. J Perinat Med (2019) 47(8):822–40. doi: 10.1515/jpm-2019-0262
120. Gomez-Lopez N, Romero R, Galaz J, Xu Y, Panaitescu B, Slutsky R, et al. Cellular Immune Responses in Amniotic Fluid of Women With Preterm Labor and Intra-Amniotic Infection or Intra-Amniotic Inflammation. Am J Reprod Immunol (2019) 82(5):e13171. doi: 10.1111/aji.13171
121. Gomez-Lopez N, Romero R, Varrey A, Leng Y, Miller D, Done B, et al. RNA Sequencing Reveals Diverse Functions of Amniotic Fluid Neutrophils and Monocytes/Macrophages in Intra-Amniotic Infection. J Innate Immun (2020) 13:63–82. doi: 10.1159/000509718
122. Gillaux C, Méhats C, Vaiman D, Cabrol D, Breuiller-Fouché M. Functional Screening of TLRs in Human Amniotic Epithelial Cells. J Immunol (2011) 187(5):2766–74. doi: 10.4049/jimmunol.1100217
123. Kim YM, Romero R, Chaiworapongsa T, Kim GJ, Kim MR, Kuivaniemi H, et al. Toll-Like Receptor-2 and -4 in the Chorioamniotic Membranes in Spontaneous Labor at Term and in Preterm Parturition That Are Associated With Chorioamnionitis. Am J Obstet Gynecol (2004) 191(4):1346–55. doi: 10.1016/j.ajog.2004.07.009
124. Dulay AT, Buhimschi CS, Zhao G, Oliver EA, Mbele A, Jing S, et al. Soluble TLR2 Is Present in Human Amniotic Fluid and Modulates the Intraamniotic Inflammatory Response to Infection. J Immunol (2009) 182(11):7244–53. doi: 10.4049/jimmunol.0803517
125. Abrahams VM, Potter JA, Bhat G, Peltier MR, Saade G, Menon R. Bacterial Modulation of Human Fetal Membrane Toll-Like Receptor Expression. Am J Reprod Immunol (2013) 69(1):33–40. doi: 10.1111/aji.12016
126. Triantafilou M, De Glanville B, Aboklaish AF, Spiller OB, Kotecha S, Triantafilou K. Synergic Activation of Toll-Like Receptor (TLR) 2/6 and 9 in Response to Ureaplasma Parvum & Urealyticum in Human Amniotic Epithelial Cells. PLoS One (2013) 8(4):e61199. doi: 10.1371/journal.pone.0061199
127. Waring GJ, Robson SC, Bulmer JN, Tyson-Capper AJ. Inflammatory Signalling in Fetal Membranes: Increased Expression Levels of TLR 1 in the Presence of Preterm Histological Chorioamnionitis. PLoS One (2015) 10(5):e0124298. doi: 10.1371/journal.pone.0124298
128. Flores-Espinosa P, Pineda-Torres M, Vega-Sánchez R, Estrada-Gutiérrez G, Espejel-Nuñez A, Flores-Pliego A, et al. Progesterone Elicits an Inhibitory Effect Upon LPS-Induced Innate Immune Response in Pre-Labor Human Amniotic Epithelium. Am J Reprod Immunol (2014) 71(1):61–72. doi: 10.1111/aji.12163
129. Dulay AT, Buhimschi CS, Zhao G, Oliver EA, Abdel-Razeq SS, Shook LL, et al. Amniotic Fluid Soluble Myeloid Differentiation-2 (sMD-2) as Regulator of Intra-Amniotic Inflammation in Infection-Induced Preterm Birth. Am J Reprod Immunol (2015) 73(6):507–21. doi: 10.1111/aji.12362
130. Jain VG, Kong F, Kallapur SG, Presicce P, Senthamaraikannnan P, Cappelletti M, et al. IRAK1 Is a Critical Mediator of Inflammation-Induced Preterm Birth. J Immunol (2020) 204(10):2651–60. doi: 10.4049/jimmunol.1901368
131. Amirchaghmaghi E, Taghavi SA, Shapouri F, Saeidi S, Rezaei A, Aflatoonian R. The Role of Toll Like Receptors in Pregnancy. Int J Fertil Steril (2013) 7(3):147–54.
132. Faro J, Romero R, Schwenkel G, Garcia-Flores V, Arenas-Hernandez M, Leng Y, et al. Intra-Amniotic Inflammation Induces Preterm Birth by Activating the NLRP3 Inflammasome†. Biol Reprod (2019) 100(5):1290–305. doi: 10.1093/biolre/ioy261
133. Gomez-Lopez N, Romero R, Panaitescu B, Leng Y, Xu Y, Tarca AL, et al. Inflammasome Activation During Spontaneous Preterm Labor With Intra-Amniotic Infection or Sterile Intra-Amniotic Inflammation. Am J Reprod Immunol (2018) 80(5):e13049. doi: 10.1111/aji.13049
134. Martinon F, Burns K, Tschopp J. The Inflammasome: A Molecular Platform Triggering Activation of Inflammatory Caspases and Processing of proIL-Beta. Mol Cell (2002) 10(2):417–26. doi: 10.1016/S1097-2765(02)00599-3
135. Wang L, Manji GA, Grenier JM, Al-Garawi A, Merriam S, Lora JM, et al. PYPAF7, a Novel PYRIN-Containing Apaf1-Like Protein That Regulates Activation of NF-Kappa B and Caspase-1-Dependent Cytokine Processing. J Biol Chem (2002) 277(33):29874–80. doi: 10.1074/jbc.M203915200
136. Shi J, Zhao Y, Wang K, Shi X, Wang Y, Huang H, et al. Cleavage of GSDMD by Inflammatory Caspases Determines Pyroptotic Cell Death. Nature (2015) 526(7575):660–5. doi: 10.1038/nature15514
137. He WT, Wan H, Hu L, Chen P, Wang X, Huang Z, et al. Gasdermin D Is an Executor of Pyroptosis and Required for Interleukin-1β Secretion. Cell Res (2015) 25(12):1285–98. doi: 10.1038/cr.2015.139
138. Gomez-Lopez N, Romero R, Tarca AL, Miller D, Panaitescu B, Schwenkel G, et al. Gasdermin D: Evidence of Pyroptosis in Spontaneous Preterm Labor With Sterile Intra-Amniotic Inflammation or Intra-Amniotic Infection. Am J Reprod Immunol (2019) 82(6):e13184. doi: 10.1111/aji.13184
139. Motedayyen H, Fathi F, Fasihi-Ramandi M, Sabzghabaee AM, Taheri RA. Toll-Like Receptor 4 Activation on Human Amniotic Epithelial Cells Is a Risk Factor for Pregnancy Loss. J Res Med Sci (2019) 24:1. doi: 10.4103/jrms.JRMS_463_18
140. Romero R, Chaemsaithong P, Korzeniewski SJ, Tarca AL, Bhatti G, Xu Z, et al. Clinical Chorioamnionitis at Term II: The Intra-Amniotic Inflammatory Response. J Perinat Med (2016) 44(1):5–22. doi: 10.1515/jpm-2015-0045
141. Romero R, Chaiworapongsa T, Alpay Savasan Z, Xu Y, Hussein Y, Dong Z, et al. Damage-Associated Molecular Patterns (DAMPs) in Preterm Labor With Intact Membranes and Preterm PROM: A Study of the Alarmin HMGB1. J Matern Fetal Neonatal Med (2011) 24(12):1444–55. doi: 10.3109/14767058.2011.591460
142. Romero R, Mazor M, Sepulveda W, Avila C, Copeland D, Williams J. Tumor Necrosis Factor in Preterm and Term Labor. Am J Obstet Gynecol (1992) 166(5):1576–87. doi: 10.1016/0002-9378(92)91636-O
143. Daskalakis G, Thomakos N, Papapanagiotou A, Papantoniou N, Mesogitis S, Antsaklis A. Amniotic Fluid Interleukin-18 at Mid-Trimester Genetic Amniocentesis: Relationship to Intraamniotic Microbial Invasion and Preterm Delivery. BJOG (2009) 116(13):1743–8. doi: 10.1111/j.1471-0528.2009.02364.x
144. Marconi C, de Andrade Ramos BR, Peraçoli JC, Donders GG, da Silva MG. Amniotic Fluid Interleukin-1 Beta and Interleukin-6, But Not Interleukin-8 Correlate With Microbial Invasion of the Amniotic Cavity in Preterm Labor. Am J Reprod Immunol (2011) 65(6):549–56. doi: 10.1111/j.1600-0897.2010.00940.x
145. Hsu C-D, Hong S. Amniotic Fluid Levels of Interleukin-18 and Thrombomodulin in Intraamniotic Infection. Am J Obstetr Gynecol (2006) 195(6):S59. doi: 10.1016/j.ajog.2006.10.179
146. Yin F, Zhu Y, Wang H, Wang Y, Li D, Qin J. Microengineered hiPSC-Derived 3d Amnion Tissue Model to Probe Amniotic Inflammatory Responses Under Bacterial Exposure. ACS Biomater Sci Eng (2020) 6(8):4644–52. doi: 10.1021/acsbiomaterials.0c00592
147. Kim SA, Park KH, Lee SM, Kim YM, Hong S. Inflammatory Proteins in the Amniotic Fluid, Plasma, and Cervicovaginal Fluid for the Prediction of Intra-Amniotic Infection/Inflammation and Imminent Preterm Birth in Preterm Labor. Am J Perinatol (2020). doi: 10.1055/s-0040-1718575
148. Combs CA, Gravett M, Garite TJ, Hickok DE, Lapidus J, Porreco R, et al. Amniotic Fluid Infection, Inflammation, and Colonization in Preterm Labor With Intact Membranes. Am J Obstet Gynecol (2014) 210(2):125.e1–.e15. doi: 10.1016/j.ajog.2013.11.032
149. Romero R, Avila C, Santhanam U, Sehgal PB. Amniotic Fluid Interleukin 6 in Preterm Labor. Association With Infection. J Clin Invest (1990) 85(5):1392–400. doi: 10.1172/JCI114583
150. Otsuki K, Yoda A, Saito H, Mitsuhashi Y, Toma Y, Shimizu Y, et al. Amniotic Fluid Lactoferrin in Intrauterine Infection. Placenta (1999) 20(2-3):175–9. doi: 10.1053/plac.1998.0368
151. Mitsuhashi Y, Otsuki K, Yoda A, Shimizu Y, Saito H, Yanaihara T. Effect of Lactoferrin on Lipopolysaccharide (LPS) Induced Preterm Delivery in Mice. Acta Obstet Gynecol Scand (2000) 79(5):355–8.
152. Bhatti G, Romero R, Rice GE, Fitzgerald W, Pacora P, Gomez-Lopez N, et al. Compartmentalized Profiling of Amniotic Fluid Cytokines in Women With Preterm Labor. PLoS One (2020) 15(1):e0227881. doi: 10.1371/journal.pone.0227881
153. Musilova I, Kolackova M, Andrys C, Drahosova M, Baranová I, Chmelarova M, et al. Nicotinamide Phosphoribosyltransferase and Intra-Amniotic Inflammation in Preterm Prelabor Rupture of Membranes. J Matern Fetal Neonatal Med (2021) 34(5):736–46. doi: 10.1080/14767058.2019.1615049
154. Lee SM, Park KH, Jung EY, Kook SY, Park H, Jeon SJ. Inflammatory Proteins in Maternal Plasma, Cervicovaginal and Amniotic Fluids as Predictors of Intra-Amniotic Infection in Preterm Premature Rupture of Membranes. PLoS One (2018) 13(7):e0200311. doi: 10.1371/journal.pone.0200311
155. Hsu CD, Meaddough E, Aversa K, Copel JA. The Role of Amniotic Fluid L-Selectin, GRO-Alpha, and Interleukin-8 in the Pathogenesis of Intraamniotic Infection. Am J Obstet Gynecol (1998) 178(3):428–32. doi: 10.1016/S0002-9378(98)70414-4
156. Athayde N, Romero R, Maymon E, Gomez R, Pacora P, Yoon BH, et al. Interleukin 16 in Pregnancy, Parturition, Rupture of Fetal Membranes, and Microbial Invasion of the Amniotic Cavity. Am J Obstet Gynecol (2000) 182(1 Pt 1):135–41. doi: 10.1016/S0002-9378(00)70502-3
157. Keelan JA, Yang J, Romero RJ, Chaiworapongsa T, Marvin KW, Sato TA, et al. Epithelial Cell-Derived Neutrophil-Activating Peptide-78 Is Present in Fetal Membranes and Amniotic Fluid at Increased Concentrations With Intra-Amniotic Infection and Preterm Delivery. Biol Reprod (2004) 70(1):253–9. doi: 10.1095/biolreprod.103.016204
158. Mittal P, Romero R, Kusanovic JP, Edwin SS, Gotsch F, Mazaki-Tovi S, et al. CXCL6 (Granulocyte Chemotactic Protein-2): A Novel Chemokine Involved in the Innate Immune Response of the Amniotic Cavity. Am J Reprod Immunol (2008) 60(3):246–57. doi: 10.1111/j.1600-0897.2008.00620.x
159. Kasper DC, Mechtler TP, Reischer GH, Witt A, Langgartner M, Pollak A, et al. The Bacterial Load of Ureaplasma Parvum in Amniotic Fluid Is Correlated With an Increased Intrauterine Inflammatory Response. Diagn Microbiol Infect Dis (2010) 67(2):117–21. doi: 10.1016/j.diagmicrobio.2009.12.023
160. Lazzaro BP, Zasloff M, Rolff J. Antimicrobial Peptides: Application Informed by Evolution. Science (2020) 368(6490):eaau5480. doi: 10.1126/science.aau5480
161. Zasloff M. Antimicrobial Peptides of Multicellular Organisms. Nature (2002) 415(6870):389–95. doi: 10.1038/415389a
162. Zhang Q, Shimoya K, Moriyama A, Yamanaka K, Nakajima A, Nobunaga T, et al. Production of Secretory Leukocyte Protease Inhibitor by Human Amniotic Membranes and Regulation of Its Concentration in Amniotic Fluid. Mol Hum Reprod (2001) 7(6):573–9. doi: 10.1093/molehr/7.6.573
163. King AE, Paltoo A, Kelly RW, Sallenave JM, Bocking AD, Challis JR. Expression of Natural Antimicrobials by Human Placenta and Fetal Membranes. Placenta (2007) 28(2-3):161–9. doi: 10.1016/j.placenta.2006.01.006
164. King AE, Kelly RW, Sallenave JM, Bocking AD, Challis JR. Innate Immune Defences in the Human Uterus During Pregnancy. Placenta (2007) 28(11-12):1099–106. doi: 10.1016/j.placenta.2007.06.002
165. Szukiewicz D, Alkhalayla H, Pyzlak M, Watroba M, Szewczyk G, Wejman J. Human Beta-Defensin 1, 2 and 3 Production by Amniotic Epithelial Cells With Respect to Human Papillomavirus (HPV) Infection, HPV Oncogenic Potential and the Mode of Delivery. Microb Pathog (2016) 97:154–65. doi: 10.1016/j.micpath.2016.06.010
166. Heine RP, Wiesenfeld H, Mortimer L, Greig PC. Amniotic Fluid Defensins: Potential Markers of Subclinical Intrauterine Infection. Clin Infect Dis (1998) 27(3):513–8. doi: 10.1086/514691
167. Denison FC, Kelly RW, Calder AA, Riley SC. Secretory Leukocyte Protease Inhibitor Concentration Increases in Amniotic Fluid With the Onset of Labour in Women: Characterization of Sites of Release Within the Uterus. J Endocrinol (1999) 161(2):299–306. doi: 10.1677/joe.0.1610299
168. Espinoza J, Chaiworapongsa T, Romero R, Edwin S, Rathnasabapathy C, Gomez R, et al. Antimicrobial Peptides in Amniotic Fluid: Defensins, Calprotectin and Bacterial/Permeability-Increasing Protein in Patients With Microbial Invasion of the Amniotic Cavity, Intra-Amniotic Inflammation, Preterm Labor and Premature Rupture of Membranes. J Matern Fetal Neonatal Med (2003) 13(1):2–21. doi: 10.1080/jmf.13.1.2.21
169. Yoshio H, Tollin M, Gudmundsson GH, Lagercrantz H, Jornvall H, Marchini G, et al. Antimicrobial Polypeptides of Human Vernix Caseosa and Amniotic Fluid: Implications for Newborn Innate Defense. Pediatr Res (2003) 53(2):211–6. doi: 10.1203/00006450-200302000-00003
170. Akinbi HT, Narendran V, Pass AK, Markart P, Hoath SB. Host Defense Proteins in Vernix Caseosa and Amniotic Fluid. Am J Obstet Gynecol (2004) 191(6):2090–6. doi: 10.1016/j.ajog.2004.05.002
171. Tambor V, Kacerovsky M, Andrys C, Musilova I, Hornychova H, Pliskova L, et al. Amniotic Fluid Cathelicidin in PPROM Pregnancies: From Proteomic Discovery to Assessing Its Potential in Inflammatory Complications Diagnosis. PLoS One (2012) 7(7):e41164. doi: 10.1371/journal.pone.0041164
172. Varrey A, Romero R, Panaitescu B, Miller D, Chaiworapongsa T, Patwardhan M, et al. Human β-Defensin-1: A Natural Antimicrobial Peptide Present in Amniotic Fluid That Is Increased in Spontaneous Preterm Labor With Intra-Amniotic Infection. Am J Reprod Immunol (2018) 80(4):e13031. doi: 10.1111/aji.13031
173. Para R, Romero R, Miller D, Panaitescu B, Varrey A, Chaiworapongsa T, et al. Human β-Defensin-3 Participates in Intra-Amniotic Host Defense in Women With Labor at Term, Spontaneous Preterm Labor and Intact Membranes, and Preterm Prelabor Rupture of Membranes. J Matern Fetal Neonatal Med (2020) 33(24):4117–32. doi: 10.1080/14767058.2019.1597047
174. Helmig BR, Romero R, Espinoza J, Chaiworapongsa T, Bujold E, Gomez R, et al. Neutrophil Elastase and Secretory Leukocyte Protease Inhibitor in Prelabor Rupture of Membranes, Parturition and Intra-Amniotic Infection. J Matern Fetal Neonatal Med (2002) 12(4):237–46. doi: 10.1080/jmf.12.4.237.246
175. Stock SJ, Kelly RW, Riley SC, Calder AA. Natural Antimicrobial Production by the Amnion. Am J Obstet Gynecol (2007) 196(3):255.e1–6. doi: 10.1016/j.ajog.2006.10.908
176. Buhimschi IA, Jabr M, Buhimschi CS, Petkova AP, Weiner CP, Saed GM. The Novel Antimicrobial Peptide Beta3-Defensin Is Produced by the Amnion: A Possible Role of the Fetal Membranes in Innate Immunity of the Amniotic Cavity. Am J Obstet Gynecol (2004) 191(5):1678–87. doi: 10.1016/j.ajog.2004.03.081
177. Vajrychova M, Kacerovsky M, Tambor V, Hornychova H, Lenco J. Microbial Invasion and Histological Chorioamnionitis Upregulate Neutrophil-Gelatinase Associated Lipocalin in Preterm Prelabor Rupture of Membranes. J Matern Fetal Neonatal Med (2016) 29(1):12–21. doi: 10.3109/14767058.2014.991305
178. Hong S, Lee JE, Kim YM, Park Y, Choi JW, Park KH. Identifying Potential Biomarkers Related to Pre-Term Delivery by Proteomic Analysis of Amniotic Fluid. Sci Rep (2020) 10(1):19648. doi: 10.1038/s41598-020-76748-1
179. Buhimschi IA, Christner R, Buhimschi CS. Proteomic Biomarker Analysis of Amniotic Fluid for Identification of Intra-Amniotic Inflammation. Bjog-an Int J Obstetr Gynaecol (2005) 112(2):173–81. doi: 10.1111/j.1471-0528.2004.00340.x
180. Yoshio H, Yamada M, Yoshida M, Takeuchi A, Fujii S, Kunii Y, et al. Expression of a Human Cathelicidin Antimicrobial Peptide, LL-37, in Amniotic Fluid With Neonatal or Maternal Infection. Pediatr Res (2005) 58(2):427–. doi: 10.1203/00006450-200508000-00455
181. Tromp G, Kuivaniemi H, Romero R, Chaiworapongsa T, Kim YM, Kim MR, et al. Genome-Wide Expression Profiling of Fetal Membranes Reveals a Deficient Expression of Proteinase Inhibitor 3 in Premature Rupture of Membranes. Am J Obstetr Gynecol (2004) 191(4):1331–8. doi: 10.1016/j.ajog.2004.07.010
182. Niemelä A, Kulomaa M, Vija P, Tuohimaa P, Saarikoski S. Lactoferrin in Human Amniotic Fluid. Hum Reprod (1989) 4(1):99–101. doi: 10.1093/oxfordjournals.humrep.a136854
183. Pacora P, Maymon E, Gervasi MT, Gomez R, Edwin SS, Yoon BH, et al. Lactoferrin in Intrauterine Infection, Human Parturition, and Rupture of Fetal Membranes. Am J Obstet Gynecol (2000) 183(4):904–10. doi: 10.1067/mob.2000.108882
184. Harris SG, Padilla J, Koumas L, Ray D, Phipps RP. Prostaglandins as Modulators of Immunity. Trends Immunol (2002) 23(3):144–50. doi: 10.1016/S1471-4906(01)02154-8
185. Romero R, Emamian M, Quintero R, Wan M, Hobbins JC, Mitchell MD. Amniotic Fluid Prostaglandin Levels and Intra-Amniotic Infections. Lancet (1986) 1(8494):1380. doi: 10.1016/S0140-6736(86)91685-5
186. Romero R, Emamian M, Wan M, Quintero R, Hobbins JC, Mitchell MD. Prostaglandin Concentrations in Amniotic Fluid of Women With Intra-Amniotic Infection and Preterm Labor. Am J Obstet Gynecol (1987) 157(6):1461–7. doi: 10.1016/S0002-9378(87)80245-4
187. Romero R, Wu YK, Mazor M, Hobbins JC, Mitchell MD. Amniotic Fluid Prostaglandin E2 in Preterm Labor. Prostaglandins Leukot Essent Fatty Acids (1988) 34(3):141–5. doi: 10.1016/0952-3278(88)90137-8
188. Romero R, Wu YK, Sirtori M, Oyarzun E, Mazor M, Hobbins JC, et al. Amniotic Fluid Concentrations of Prostaglandin F2 Alpha, 13,14-Dihydro-15-Keto-Prostaglandin F2 Alpha (PGFM) and 11-Deoxy-13,14-Dihydro-15-Keto-11, 16-Cyclo-Prostaglandin E2 (PGEM-LL) in Preterm Labor. Prostaglandins (1989) 37(1):149–61. doi: 10.1016/0090-6980(89)90038-5
189. Mazor M, Wiznitzer A, Maymon E, Leiberman JR, Cohen A. Changes in Amniotic Fluid Concentrations of Prostaglandins E2 and F2 Alpha in Women With Preterm Labor. Isr J Med Sci (1990) 26(8):425–8.
190. Lee SE, Park IS, Romero R, Yoon BH. Amniotic Fluid Prostaglandin F2 Increases Even in Sterile Amniotic Fluid and Is an Independent Predictor of Impending Delivery in Preterm Premature Rupture of Membranes. J Matern Fetal Neonatal Med (2009) 22(10):880–6. doi: 10.1080/14767050902994648
191. Maddipati KR, Romero R, Chaiworapongsa T, Chaemsaithong P, Zhou SL, Xu Z, et al. Clinical Chorioamnionitis at Term: The Amniotic Fluid Fatty Acyl Lipidome. J Lipid Res (2016) 57(10):1906–16. doi: 10.1194/jlr.P069096
192. Peiris HN, Romero R, Vaswani K, Reed S, Gomez-Lopez N, Tarca AL, et al. Preterm Labor Is Characterized by a High Abundance of Amniotic Fluid Prostaglandins in Patients With Intra-Amniotic Infection or Sterile Intra-Amniotic Inflammation. J Matern Fetal Neonatal Med (2019) 1–16. doi: 10.1080/14767058.2019.1702953
193. Peiris HN, Romero R, Vaswani K, Gomez-Lopez N, Tarca AL, Gudicha DW, et al. Prostaglandin and Prostamide Concentrations in Amniotic Fluid of Women With Spontaneous Labor at Term With and Without Clinical Chorioamnionitis. Prostaglandins Leukot Essent Fatty Acids (2020) 163:102195. doi: 10.1016/j.plefa.2020.102195
194. Menon R, Fortunato SJ, Milne GL, Brou L, Carnevale C, Sanchez SC, et al. Amniotic Fluid Eicosanoids in Preterm and Term Births: Effects of Risk Factors for Spontaneous Preterm Labor. Obstet Gynecol (2011) 118(1):121–34. doi: 10.1097/AOG.0b013e3182204eaa
195. Feng L, Ransom CE, Nazzal MK, Allen TK, Li YJ, Truong T, et al. The Role of Progesterone and a Novel Progesterone Receptor, Progesterone Receptor Membrane Component 1, in the Inflammatory Response of Fetal Membranes to Ureaplasma Parvum Infection. PLoS One (2016) 11(12):e0168102. doi: 10.1371/journal.pone.0168102
196. Peiris HN, Vaswani K, Holland O, Koh YQ, Almughlliq FB, Reed S, et al. Altered Productions of Prostaglandins and Prostamides by Human Amnion in Response to Infectious and Inflammatory Stimuli Identified by Mutliplex Mass Spectrometry. Prostaglandins Leukotrienes Essential Fatty Acids (2020) 154:102059. doi: 10.1016/j.plefa.2020.102059
197. Hsu CD, Meaddough E, Aversa K, Hong SF, Lee IS, Bahodo-Singh RO, et al. Dual Roles of Amniotic Fluid Nitric Oxide and Prostaglandin E2 in Preterm Labor With Intra-Amniotic Infection. Am J Perinatol (1998) 15(12):683–7. doi: 10.1055/s-2007-999302
198. Maddipati KR, Romero R, Chaiworapongsa T, Chaemsaithong P, Zhou S-L, Xu Z, et al. Lipidomic Analysis of Patients With Microbial Invasion of the Amniotic Cavity Reveals Up-Regulation of Leukotriene B4. FASEB J (2016) 30(10):3296–307. doi: 10.1096/fj.201600583R
199. Kim YM, Park KH, Park H, Yoo HN, Kook SY, Jeon SJ. Complement C3a, But Not C5a, Levels in Amniotic Fluid Are Associated With Intra-Amniotic Infection and/or Inflammation and Preterm Delivery in Women With Cervical Insufficiency or an Asymptomatic Short Cervix (≤ 25 Mm). J Korean Med Sci (2018) 33(35):e220. doi: 10.3346/jkms.2018.33.e220
200. Vaisbuch E, Romero R, Erez O, Mazaki-Tovi S, Kusanovic JP, Soto E, et al. Fragment Bb in Amniotic Fluid: Evidence for Complement Activation by the Alternative Pathway in Women With Intra-Amniotic Infection/Inflammation. J Matern Fetal Neonatal Med (2009) 22(10):905–16. doi: 10.1080/14767050902994663
201. Kacerovsky M, Celec P, Vlkova B, Skogstrand K, Hougaard DM, Cobo T, et al. Amniotic Fluid Protein Profiles of Intraamniotic Inflammatory Response to Ureaplasma Spp. and other bacteria. PLoS One (2013) 8(3):e60399. doi: 10.1371/journal.pone.0060399
202. Hsu CD, Aversa KR, Lu LC, Meaddough E, Jones D, Bahado-Singh RO, et al. Nitric Oxide: A Clinically Important Amniotic Fluid Marker to Distinguish Between Intra-Amniotic Mycoplasma and Non-Mycoplasma Infections. Am J Perinatol (1999) 16(4):161–6. doi: 10.1055/s-2007-993851
203. Fisher AL, Sangkhae V, Presicce P, Chougnet CA, Jobe AH, Kallapur SG, et al. Fetal and Amniotic Fluid Iron Homeostasis in Healthy and Complicated Murine, Macaque, and Human Pregnancy. JCI Insight (2020) 5(4):e135321. doi: 10.1172/jci.insight.135321
204. Kacerovsky M, Holeckova M, Stepan M, Gregor M, Vescicik P, Lesko D, et al. Amniotic Fluid Glucose Level in PPROM Pregnancies: A Glance at the Old Friend. J Matern Fetal Neonatal Med (2020) 1–13. doi: 10.1080/14767058.2020.1783232
205. Kacerovsky M, Tambor V, Vajrychová M, Lenco J, Hornychova H, Musilova I, et al. Amniotic Fluid Myeloperoxidase in Pregnancies Complicated by Preterm Prelabor Rupture of Membranes. J Matern Fetal Neonatal Med (2013) 26(5):463–8. doi: 10.3109/14767058.2012.735997
206. Hsu CD, Meaddough E, Hong SF, Aversa K, Lu LC, Copel JA. Elevated Amniotic Fluid Nitric Oxide Metabolites and Interleukin-6 in Intra-Amniotic Infection. J Soc Gynecol Investig (1998) 5(1):21–4. doi: 10.1016/S1071-5576(97)00099-3
207. Pacora P, Romero R, Chaiworapongsa T, Kusanovic JP, Erez O, Vaisbuch E, et al. Amniotic Fluid Angiopoietin-2 in Term and Preterm Parturition, and Intra-Amniotic Infection/Inflammation. J Perinat Med (2009) 37(5):503–11. doi: 10.1515/JPM.2009.093
208. Kusanovic JP, Romero R, Jodicke C, Mazaki-Tovi S, Vaisbuch E, Erez O, et al. Amniotic Fluid Soluble Human Leukocyte Antigen-G in Term and Preterm Parturition, and Intra-Amniotic Infection/Inflammation. J Matern Fetal Neonatal Med (2009) 22(12):1151–66. doi: 10.3109/14767050903019684
209. Kusanovic JP, Romero R, Chaiworapongsa T, Mittal P, Mazaki-Tovi S, Vaisbuch E, et al. Amniotic Fluid sTREM-1 in Normal Pregnancy, Spontaneous Parturition at Term and Preterm, and Intra-Amniotic Infection/Inflammation. J Matern Fetal Neonatal Med (2010) 23(1):34–47. doi: 10.3109/14767050903009248
210. Kusanovic JP, Romero R, Mazaki-Tovi S, Chaiworapongsa T, Mittal P, Gotsch F, et al. Resistin in Amniotic Fluid and Its Association With Intra-Amniotic Infection and Inflammation. J Matern Fetal Neonatal Med (2008) 21(12):902–16. doi: 10.1080/14767050802320357
211. Kacerovsky M, Vlkova B, Musilova I, Andrys C, Pliskova L, Zemlickova H, et al. Amniotic Fluid Cell-Free DNA in Preterm Prelabor Rupture of Membranes. Prenat Diagn (2018) 38(13):1086–95. doi: 10.1002/pd.5366
212. Parry S, Strauss JF. Premature Rupture of the Fetal Membranes. N Engl J Med (1998) 338(10):663–70. doi: 10.1056/NEJM199803053381006
213. Menon R, Richardson LS. Preterm Prelabor Rupture of the Membranes: A Disease of the Fetal Membranes. Semin Perinatol (2017) 41(7):409–19. doi: 10.1053/j.semperi.2017.07.012
214. Fingleton B. Matrix Metalloproteinases as Regulators of Inflammatory Processes. Biochim Biophys Acta Mol Cell Res (2017) 1864(11 Pt A):2036–42. doi: 10.1016/j.bbamcr.2017.05.010
215. Shen ZY, Xu LY, Li EM, Zhuang BR, Lu XF, Shen J, et al. Autophagy and Endocytosis in the Amnion. J Struct Biol (2008) 162(2):197–204. doi: 10.1016/j.jsb.2006.10.010
216. Shen ZY, Li EM, Lu SQ, Shen J, Cai YM, Wu YE, et al. Autophagic and Apoptotic Cell Death in Amniotic Epithelial Cells. Placenta (2008) 29(11):956–61. doi: 10.1016/j.placenta.2008.09.001
217. Mogami H, Hari Kishore A, Akgul Y, Word RA. Healing of Preterm Ruptured Fetal Membranes. Sci Rep (2017) 7(1):13139. doi: 10.1038/s41598-017-13296-1
218. Canciello A, Russo V, Berardinelli P, Bernabò N, Muttini A, Mattioli M, et al. Progesterone Prevents Epithelial-Mesenchymal Transition of Ovine Amniotic Epithelial Cells and Enhances Their Immunomodulatory Properties. Sci Rep (2017) 7(1):3761. doi: 10.1038/s41598-017-03908-1
219. Richardson L, Dixon CL, Aguilera-Aguirre L, Menon R. Oxidative Stress-Induced TGF-Beta/TAB1-Mediated P38mapk Activation in Human Amnion Epithelial Cells. Biol Reprod (2018) 99(5):1100–12. doi: 10.1093/biolre/ioy135
220. Gomez-Lopez N, Romero R, Maymon E, Kusanovic JP, Panaitescu B, Miller D, et al. Clinical Chorioamnionitis at Term IX: In Vivo Evidence of Intra-Amniotic Inflammasome Activation. J Perinat Med (2019) 47(3):276–87. doi: 10.1515/jpm-2018-0271
221. Galaz J, Romero R, Slutsky R, Xu Y, Motomura K, Para R, et al. Cellular Immune Responses in Amniotic Fluid of Women With Preterm Prelabor Rupture of Membranes. J Perinat Med (2020) 48(3):222–33. doi: 10.1515/jpm-2019-0395
222. Galaz J, Romero R, Xu Y, Miller D, Slutsky R, Levenson D, et al. Cellular Immune Responses in Amniotic Fluid of Women With Preterm Clinical Chorioamnionitis. Inflammation Res (2020) 69(2):203–16. doi: 10.1007/s00011-019-01308-x
223. Martinez-Varea A, Romero R, Xu Y, Miller D, Ahmed AI, Chaemsaithong P, et al. Clinical Chorioamnionitis at Term VII: The Amniotic Fluid Cellular Immune Response. J Perinat Med (2017) 45(5):523–38. doi: 10.1515/jpm-2016-0225
224. Papayannopoulos V. Neutrophil Extracellular Traps in Immunity and Disease. Nat Rev Immunol (2018) 18(2):134–47. doi: 10.1038/nri.2017.105
225. Steel JH, Malatos S, Kennea N, Edwards AD, Miles L, Duggan P, et al. Bacteria and Inflammatory Cells in Fetal Membranes do Not Always Cause Preterm Labor. Pediatr Res (2005) 57(3):404–11. doi: 10.1203/01.PDR.0000153869.96337.90
226. Bae GE, Hong JS, Kim JS, Park HY, Jang JY, Kim YS, et al. Differential Immunophenotype of Macrophages in Acute and Chronic Chorioamnionitis. J Perinat Med (2017) 45(4):483–91. doi: 10.1515/jpm-2015-0353
227. Hoo R, Nakimuli A, Vento-Tormo R. Innate Immune Mechanisms to Protect Against Infection at the Human Decidual-Placental Interface. Front Immunol (2020) 11:2070. doi: 10.3389/fimmu.2020.02070
228. Gomez-Lopez N, Romero R, Xu Y, Miller D, Arenas-Hernandez M, Garcia-Flores V, et al. Fetal T Cell Activation in the Amniotic Cavity During Preterm Labor: A Potential Mechanism for a Subset of Idiopathic Preterm Birth. J Immunol (2019) 203(7):1793–807. doi: 10.4049/jimmunol.1900621
229. Amarante-Mendes GP, Adjemian S, Branco LM, Zanetti LC, Weinlich R, Bortoluci KR. Pattern Recognition Receptors and the Host Cell Death Molecular Machinery. Front Immunol (2018) 9:2379. doi: 10.3389/fimmu.2018.02379
230. Takeuchi O, Akira S. Pattern Recognition Receptors and Inflammation. Cell (2010) 140(6):805–20. doi: 10.1016/j.cell.2010.01.022
231. Walsh D, McCarthy J, O'Driscoll C, Melgar S. Pattern Recognition Receptors–Molecular Orchestrators of Inflammation in Inflammatory Bowel Disease. Cytokine Growth Factor Rev (2013) 24(2):91–104. doi: 10.1016/j.cytogfr.2012.09.003
232. Bortoluci KR, Medzhitov R. Control of Infection by Pyroptosis and Autophagy: Role of TLR and NLR. Cell Mol Life Sci (2010) 67(10):1643–51. doi: 10.1007/s00018-010-0335-5
233. Medzhitov R, Janeway CA. Innate Immunity: Impact on the Adaptive Immune Response. Curr Opin Immunol (1997) 9(1):4–9. doi: 10.1016/S0952-7915(97)80152-5
234. Hadley EE, Sheller-Miller S, Saade G, Salomon C, Mesiano S, Taylor RN, et al. Amnion Epithelial Cell-Derived Exosomes Induce Inflammatory Changes in Uterine Cells. Am J Obstet Gynecol (2018) 219(5):478.e1–.e21. doi: 10.1016/j.ajog.2018.08.021
235. Menon R, Mesiano S, Taylor RN. Programmed Fetal Membrane Senescence and Exosome-Mediated Signaling: A Mechanism Associated With Timing of Human Parturition. Front Endocrinol (Lausanne) (2017) 8:196. doi: 10.3389/fendo.2017.00196
236. Sheller-Miller S, Trivedi J, Yellon SM, Menon R. Exosomes Cause Preterm Birth in Mice: Evidence for Paracrine Signaling in Pregnancy. Sci Rep (2019) 9(1):608. doi: 10.1038/s41598-018-37002-x
237. Sato BL, Collier ES, Vermudez SA, Junker AD, Kendal-Wright CE. Human Amnion Mesenchymal Cells Are Pro-Inflammatory When Activated by the Toll-Like Receptor 2/6 Ligand, Macrophage-Activating Lipoprotein-2. Placenta (2016) 44:69–79. doi: 10.1016/j.placenta.2016.06.005
238. Bryant AH, Menzies GE, Scott LM, Spencer-Harty S, Davies LB, Smith RA, et al. Human Gestation-Associated Tissues Express Functional Cytosolic Nucleic Acid Sensing Pattern Recognition Receptors. Clin Exp Immunol (2017) 189(1):36–46. doi: 10.1111/cei.12960
239. Theis KR, Romero R, Motomura K, Galaz J, Winters AD, Pacora P, et al. Microbial Burden and Inflammasome Activation in Amniotic Fluid of Patients With Preterm Prelabor Rupture of Membranes. J Perinat Med (2020) 48(2):115–31. doi: 10.1515/jpm-2019-0398
240. Panaitescu B, Romero R, Gomez-Lopez N, Xu Y, Leng YZ, Maymon E, et al. In Vivo Evidence of Inflammasome Activation During Spontaneous Labor at Term. J Maternal-Fetal Neonatal Med (2019) 32(12):1978–91. doi: 10.1080/14767058.2017.1422714
241. Musilova I, Andrys C, Drahosova M, Soucek O, Pliskova L, Jacobsson B, et al. Cervical Fluid Interleukin 6 and Intra-Amniotic Complications of Preterm Prelabor Rupture of Membranes. J Matern Fetal Neonatal Med (2018) 31(7):827–36. doi: 10.1080/14767058.2017.1297792
242. Leaños-Miranda A, Nolasco-Leaños AG, Carrillo-Juárez RI, Molina-Pérez CJ, Isordia-Salas I, Ramírez-Valenzuela KL. Interleukin-6 in Amniotic Fluid: A Reliable Marker for Adverse Outcomes in Women in Preterm Labor and Intact Membranes. Fetal Diagn Ther (2021) 48(4):313–20. doi: 10.1159/000514898
243. Hadzi Lega M, Daneva Markova A, Stefanovic M, Tanturovski M. Interleukin 6 and Fetal Fibronectin as a Predictors of Preterm Delivery in Symptomatic Patients. Bosn J Basic Med Sci (2015) 15(1):51–6. doi: 10.17305/bjbms.2015.1.93
244. Chaemsaithong P, Romero R, Korzeniewski SJ, Dong Z, Yeo L, Hassan SS, et al. A Point of Care Test for the Determination of Amniotic Fluid Interleukin-6 and the Chemokine CXCL-10/IP-10. J Maternal-fetal Neonatal Med (2015) 28(13):1510–9. doi: 10.3109/14767058.2014.961417
245. Peltier MR, Drobek CO, Bhat G, Saade G, Fortunato SJ, Menon R. Amniotic Fluid and Maternal Race Influence Responsiveness of Fetal Membranes to Bacteria. J Reprod Immunol (2012) 96(1-2):68–78. doi: 10.1016/j.jri.2012.07.006
246. Chaiworapongsa T, Romero R, Espinoza J, Kim YM, Edwin S, Bujold E, et al. Macrophage Migration Inhibitory Factor in Patients With Preterm Parturition and Microbial Invasion of the Amniotic Cavity. J Matern Fetal Neonatal Med (2005) 18(6):405–16. doi: 10.1080/14767050500361703
247. Menon R, Peltier MR, Eckardt J, Fortunato SJ. Diversity in Cytokine Response to Bacteria Associated With Preterm Birth by Fetal Membranes. Am J Obstet Gynecol (2009) 201(3):306.e1–6. doi: 10.1016/j.ajog.2009.06.027
248. Dudley DJ, Collmer D, Mitchell MD, Trautman MS. Inflammatory Cytokine mRNA in Human Gestational Tissues: Implications for Term and Preterm Labor. J Soc Gynecologic Invest (1996) 3(6):328–35. doi: 10.1016/S1071-5576(96)00042-1
249. Tromp G, Kuivaniemi H, Romero R, Chaiworapongsa T, Kim YM, Kim MR, et al. Genome-Wide Expression Profiling of Fetal Membranes Reveals a Deficient Expression of Proteinase Inhibitor 3 in Premature Rupture of Membranes. Am J Obstet Gynecol (2004) 191(4):1331–8. doi: 10.1016/j.ajog.2004.07.010
250. Romero R, Baumann P, Gonzalez R, Gomez R, Rittenhouse L, Behnke E, et al. Amniotic Fluid Prostanoid Concentrations Increase Early During the Course of Spontaneous Labor at Term. Am J Obstet Gynecol (1994) 171(6):1613–20. doi: 10.1016/0002-9378(94)90412-X
251. Romero R, Gonzalez R, Baumann P, Behnke E, Rittenhouse L, Barberio D, et al. Topographic Differences in Amniotic Fluid Concentrations of Prostanoids in Women in Spontaneous Labor at Term. Prostaglandins Leukot Essent Fatty Acids (1994) 50(2):97–104. doi: 10.1016/0952-3278(94)90154-6
252. Romero R, Munoz H, Gomez R, Parra M, Polanco M, Valverde V, et al. Increase in Prostaglandin Bioavailability Precedes the Onset of Human Parturition. Prostaglandins Leukot Essent Fatty Acids (1996) 54(3):187–91. doi: 10.1016/S0952-3278(96)90015-0
253. Park JY, Romero R, Lee J, Chaemsaithong P, Chaiyasit N, Yoon BH. An Elevated Amniotic Fluid Prostaglandin F2α Concentration Is Associated With Intra-Amniotic Inflammation/Infection, and Clinical and Histologic Chorioamnionitis, as Well as Impending Preterm Delivery in Patients With Preterm Labor and Intact Membranes. J Matern Fetal Neonatal Med (2016) 29(16):2563–72. doi: 10.3109/14767058.2015.1094794
254. Maddipati KR, Romero R, Chaiworapongsa T, Chaemsaithong P, Zhou SL, Xu Z, et al. Lipidomic Analysis of Patients With Microbial Invasion of the Amniotic Cavity Reveals Up-Regulation of Leukotriene B4. FASEB J (2016) 30(10):3296–307. doi: 10.1096/fj.201600583R
255. Conigliaro P, Triggianese P, Ballanti E, Perricone C, Perricone R, Chimenti MS. Complement, Infection, and Autoimmunity. Curr Opin Rheumatol (2019) 31(5):532–41. doi: 10.1097/BOR.0000000000000633
256. Lo MW, Woodruff TM. Complement: Bridging the Innate and Adaptive Immune Systems in Sterile Inflammation. J Leukoc Biol (2020) 108(1):339–51. doi: 10.1002/JLB.3MIR0220-270R
257. Rus H, Cudrici C, Niculescu F. The Role of the Complement System in Innate Immunity. Immunol Res (2005) 33(2):103–12. doi: 10.1385/IR:33:2:103
258. Hammerschmidt DE, Ogburn PL, Williams JE. Amniotic Fluid Activates Complement. A Role in Amniotic Fluid Embolism Syndrome? J Lab Clin Med (1984) 104(6):901–7.
259. Füst A, Pállinger E, Stündl A, Kovács E, Imre L, Tóth S, et al. Both Freshly Prepared and Frozen-Stored Amniotic Membrane Cells Express the Complement Inhibitor CD59. ScientificWorldJournal (2012) 2012:815615. doi: 10.1100/2012/815615
260. Fiscella K. Race, Perinatal Outcome, and Amniotic Infection. Obstet Gynecol Surv (1996) 51(1):60–6. doi: 10.1097/00006254-199601000-00022
261. Shen JJ, Tymkow C, MacMullen N. Disparities in Maternal Outcomes Among Four Ethnic Populations. Ethn Dis (2005) 15(3):492–7.
262. Menon R, Fortunato SJ, Edwards DR, Williams SM. Association of Genetic Variants, Ethnicity and Preterm Birth With Amniotic Fluid Cytokine Concentrations. Ann Hum Genet (2010) 74(2):165–83. doi: 10.1111/j.1469-1809.2010.00562.x
263. Menon R, Velez DR, Morgan N, Lombardi SJ, Fortunato SJ, Williams SM. Genetic Regulation of Amniotic Fluid TNF-Alpha and Soluble TNF Receptor Concentrations Affected by Race and Preterm Birth. Hum Genet (2008) 124(3):243–53. doi: 10.1007/s00439-008-0547-z
264. Menon R, Dunlop AL, Kramer MR, Fortunato SJ, Hogue CJ. An Overview of Racial Disparities in Preterm Birth Rates: Caused by Infection or Inflammatory Response? Acta Obstet Gynecol Scand (2011) 90(12):1325–31. doi: 10.1111/j.1600-0412.2011.01135.x
265. Menon R, Thorsen P, Vogel I, Jacobsson B, Morgan N, Jiang L, et al. Racial Disparity in Amniotic Fluid Concentrations of Tumor Necrosis Factor (TNF)-Alpha and Soluble TNF Receptors in Spontaneous Preterm Birth. Am J Obstet Gynecol (2008) 198(5):533.e1–10. doi: 10.1016/j.ajog.2007.11.025
266. Menon R, Velez DR, Thorsen P, Vogel I, Jacobsson B, Williams SM, et al. Ethnic Differences in Key Candidate Genes for Spontaneous Preterm Birth: TNF-Alpha and Its Receptors. Hum Hered (2006) 62(2):107–18. doi: 10.1159/000096301
268. Sheikh ES, Fetterolf DE. Use of Dehydrated Human Amniotic Membrane Allografts to Promote Healing in Patients With Refractory Non Healing Wounds. Int Wound J (2014) 11(6):711–7. doi: 10.1111/iwj.12035
269. Strauss JF, Romero R, Gomez-Lopez N, Haymond-Thornburg H, Modi BP, Teves ME, et al. Spontaneous Preterm Birth: Advances Toward the Discovery of Genetic Predisposition. Am J Obstet Gynecol (2018) 218(3):294–314.e2. doi: 10.1016/j.ajog.2017.12.009
270. Braveman PA, Heck K, Egerter S, Marchi KS, Dominguez TP, Cubbin C, et al. The Role of Socioeconomic Factors in Black-White Disparities in Preterm Birth. Am J Public Health (2015) 105(4):694–702. doi: 10.2105/AJPH.2014.302008
271. Braveman P, Heck K, Egerter S, Dominguez TP, Rinki C, Marchi KS, et al. Worry About Racial Discrimination: A Missing Piece of the Puzzle of Black-White Disparities in Preterm Birth? PLoS One (2017) 12(10):e0186151. doi: 10.1371/journal.pone.0186151
272. Giurgescu C, Misra DP. Psychosocial Factors and Preterm Birth Among Black Mothers and Fathers. MCN Am J Matern Child Nurs (2018) 43(5):245–51. doi: 10.1097/NMC.0000000000000458
273. Das J, Maitra A. Maternal DNA Methylation During Pregnancy: A Review. Reprod Sci (2021). doi: 10.1007/s43032-020-00456-4
274. Hong X, Bartell TR, Wang X. Gaining a Deeper Understanding of Social Determinants of Preterm Birth by Integrating Multi-Omics Data. Pediatr Res (2021) 89(2):336–43. doi: 10.1038/s41390-020-01266-9
275. Christian LM, Franco A, Glaser R, Iams JD. Depressive Symptoms Are Associated With Elevated Serum Proinflammatory Cytokines Among Pregnant Women. Brain Behav Immun (2009) 23(6):750–4. doi: 10.1016/j.bbi.2009.02.012
276. Coussons-Read ME, Okun ML, Schmitt MP, Giese S. Prenatal Stress Alters Cytokine Levels in a Manner That may Endanger Human Pregnancy. Psychosom Med (2005) 67(4):625–31. doi: 10.1097/01.psy.0000170331.74960.ad
277. Kyrklund-Blomberg NB, Cnattingius S. Preterm Birth and Maternal Smoking: Risks Related to Gestational Age and Onset of Delivery. Am J Obstet Gynecol (1998) 179(4):1051–5. doi: 10.1016/S0002-9378(98)70214-5
278. Romero R, Chaiworapongsa T, Espinoza J. Micronutrients and Intrauterine Infection, Preterm Birth and the Fetal Inflammatory Response Syndrome. J Nutr (2003) 133(5 Suppl 2):1668S–73S. doi: 10.1093/jn/133.5.1668S
279. Savitz DA, Harmon Q, Siega-Riz AM, Herring AH, Dole N, Thorp JM. Behavioral Influences on Preterm Birth: Integrated Analysis of the Pregnancy, Infection, and Nutrition Study. Matern Child Health J (2012) 16(6):1151–63. doi: 10.1007/s10995-011-0895-5
280. Shaw GM, Wise PH, Mayo J, Carmichael SL, Ley C, Lyell DJ, et al. Maternal Prepregnancy Body Mass Index and Risk of Spontaneous Preterm Birth. Paediatric Perinatal Epidemiol (2014) 28(4):302–11. doi: 10.1111/ppe.12125
281. Vinturache A, McKeating A, Daly N, Sheehan S, Turner M. Maternal Body Mass Index and the Prevalence of Spontaneous and Elective Preterm Deliveries in an Irish Obstetric Population: A Retrospective Cohort Study. BMJ Open (2017) 7(10):e015258. doi: 10.1136/bmjopen-2016-015258
282. Ikehara S, Kimura T, Kakigano A, Sato T, Iso H. The Japan Environment Children's Study G. Association Between Maternal Alcohol Consumption During Pregnancy and Risk of Preterm Delivery: The Japan Environment and Children's Study. BJOG (2019) 126(12):1448–54. doi: 10.1111/1471-0528.15899
283. Borkowski K, Newman JW, Aghaeepour N, Mayo JA, Blazenović I, Fiehn O, et al. Mid-Gestation Serum Lipidomic Profile Associations With Spontaneous Preterm Birth Are Influenced by Body Mass Index. PLoS One (2020) 15(11):e0239115. doi: 10.1371/journal.pone.0239115
284. Melekoglu R, Ciftci O, Eraslan S, Basak N, Celik E. The Effects of Body Mass Index on Second-Trimester Amniotic Fluid Cytokine and Matrix Metalloproteinase Levels. Gynecol Obstet Invest (2018) 83(1):70–5. doi: 10.1159/000455192
285. Martinez-Perez O, Prats Rodriguez P, Muner Hernandez M, Encinas Pardilla MB, Perez Perez N, Vila Hernandez MR, et al. The Association Between SARS-CoV-2 Infection and Preterm Delivery: A Prospective Study With a Multivariable Analysis. BMC Pregnancy Childbirth (2021) 21(1):273. doi: 10.1186/s12884-021-03742-4
286. Chan RL. Biochemical Markers of Spontaneous Preterm Birth in Asymptomatic Women. BioMed Res Int (2014) 2014:164081. doi: 10.1155/2014/164081
287. Park H, Park KH, Kim YM, Kook SY, Jeon SJ, Yoo HN. Plasma Inflammatory and Immune Proteins as Predictors of Intra-Amniotic Infection and Spontaneous Preterm Delivery in Women With Preterm Labor: A Retrospective Study. BMC Pregnancy Childbirth (2018) 18(1):146. doi: 10.1186/s12884-018-1780-7
288. Aung MT, Yu Y, Ferguson KK, Cantonwine DE, Zeng L, McElrath TF, et al. Prediction and Associations of Preterm Birth and Its Subtypes With Eicosanoid Enzymatic Pathways and Inflammatory Markers. Sci Rep (2019) 9(1):17049. doi: 10.1038/s41598-019-53448-z
289. Qiu L, Pan M, Zhang R, Ren K. Maternal Peripheral Blood Platelet-to-White Blood Cell Ratio and Platelet Count as Potential Diagnostic Markers of Histological Chorioamnionitis-Related Spontaneous Preterm Birth. J Clin Lab Anal (2019) 33(4):e22840. doi: 10.1002/jcla.22840
290. Ansari A, Lee H, You YA, Jung Y, Park S, Kim SM, et al. Identification of Potential Biomarkers in the Cervicovaginal Fluid by Metabolic Profiling for Preterm Birth. Metabolites (2020) 10(9):349. doi: 10.3390/metabo10090349
291. Ma M, Zhu M, Zhuo B, Li L, Chen H, Xu L, et al. Use of Complete Blood Count for Predicting Preterm Birth in Asymptomatic Pregnant Women: A Propensity Score-Matched Analysis. J Clin Lab Anal (2020) 34(8):e23313. doi: 10.1002/jcla.23313
292. Oskovi Kaplan ZA, Ozgu-Erdinc AS. Prediction of Preterm Birth: Maternal Characteristics, Ultrasound Markers, and Biomarkers: An Updated Overview. J Pregnancy (2018) 2018:8367571–. doi: 10.1155/2018/8367571
Keywords: human amniotic membrane, placenta, intrauterine infection, innate immune system, antimicrobial activity, bacteria, preterm birth
Citation: Šket T, Ramuta TŽ, Starčič Erjavec M and Kreft ME (2021) The Role of Innate Immune System in the Human Amniotic Membrane and Human Amniotic Fluid in Protection Against Intra-Amniotic Infections and Inflammation. Front. Immunol. 12:735324. doi: 10.3389/fimmu.2021.735324
Received: 02 July 2021; Accepted: 23 September 2021;
Published: 21 October 2021.
Edited by:
Harry D. Dawson, Agricultural Research Service (USDA), United StatesReviewed by:
Pietro Presicce, UCLA Department of Pediatrics, United StatesCopyright © 2021 Šket, Ramuta, Starčič Erjavec and Kreft. This is an open-access article distributed under the terms of the Creative Commons Attribution License (CC BY). The use, distribution or reproduction in other forums is permitted, provided the original author(s) and the copyright owner(s) are credited and that the original publication in this journal is cited, in accordance with accepted academic practice. No use, distribution or reproduction is permitted which does not comply with these terms.
*Correspondence: Mateja Erdani Kreft, bWF0ZWphLmVyZGFuaUBtZi51bmktbGouc2k=; Marjanca Starčič Erjavec, bWFyamFuY2Euc3RhcmNpYy5lcmphdmVjQGJmLnVuaS1sai5zaQ==
Disclaimer: All claims expressed in this article are solely those of the authors and do not necessarily represent those of their affiliated organizations, or those of the publisher, the editors and the reviewers. Any product that may be evaluated in this article or claim that may be made by its manufacturer is not guaranteed or endorsed by the publisher.
Research integrity at Frontiers
Learn more about the work of our research integrity team to safeguard the quality of each article we publish.