- 1Cell Therapy Center, The University of Jordan, Amman, Jordan
- 2Department of Clinical Laboratory Science, The University of Jordan, School of Science, Amman, Jordan
- 3Department of Anatomy & Histology, The University of Jordan, School of Medicine, Amman, Jordan
- 4Department of Hematology & Oncology, The University of Jordan, School of Medicine, Amman, Jordan
The unique immunomodulation and immunosuppressive potential of Wharton’s jelly-derived mesenchymal stromal cells (WJ-MSCs) make them a promising therapeutic approach for autoimmune diseases including type 1 diabetes (T1D). The immunomodulatory effect of MSCs is exerted either by cell-cell contact or by secretome secretion. Cell-cell contact is a critical mechanism by which MSCs regulate immune-responses and generate immune regulatory cells such as tolerogenic dendritic cells (tolDCs) and regulatory T cell (Tregs). In this study, we primed WJ-MSCs with TNF-α and IFN-γ and investigated the immunomodulatory properties of primed WJ-MSCs on mature dendritic cells (mDCs) and activated T cells differentiated from mononuclear cells (MNCs) of T1D patient’s. Our findings revealed that primed WJ-MSCs impaired the antigen-mediated immunity, upregulated immune-tolerance genes and downregulated immune-response genes. We also found an increase in the production of anti-inflammatory cytokines and suppression of the production of pro-inflammatory cytokines. Significant upregulation of FOXP3, IL10 and TGFB1 augmented an immunosuppressive effect on adaptive T cell immunity which represented a strong evidence in support of the formation of Tregs. Furthermore, upregulation of many critical genes involved in the immune-tolerance mechanism (IDO1 and PTGES2/PTGS) was detected. Interestingly, upregulation of ENTPD1/NT5E genes express a strong evidence to switch immunostimulatory response toward immunoregulatory response. We conclude that WJ-MSCs primed by TNF-α and IFN-γ may represent a promising tool to treat the autoimmune disorders and can provide a new evidence to consider MSCs- based therapeutic approach for the treatment of TID.
Introduction
Type 1 diabetes (T1D) is a T-cell mediated autoimmune disorder in which insulin-secreting β-cells of the pancreas are selectively destroyed. The mechanisms involved in the β-cell destruction are still not understood. Currently, tyrosine kinase, islet-specific glucose-6-phosphatase catalytic subunit-related protein (IGRP), insulinoma-associated protein 2 (IA-2), zinc transporter (ZnT), and glutamic acid decarboxylase 65 (GAD65) are all considered to be involved in the β-cell specific autoimmune process (1, 2). However, GAD65 is identified as the major antigen targeted by T-cell autoantibodies in T1D (3).
Mature dendritic cells (mDCs) and T cells are implicated in the pathogenesis of T1D. Mature dendritic cells play a key role in all stages of β-cells destruction due to their immunostimulatory effect on naïve T cells (4). As a result, mDCs trigger two important functions in controlling T-cell immunity; T-cell activation by the expression of T-cell antigen and secretion of specific cytokines which determine the nature of T-cell responses (5).
To date, the most reliable approach for the management of T1D remains the islet or pancreas transplantation but there are many obstacles in their use that include; allo-immune graft rejection, recurrence of autoimmunity, as well as inadequate supply of donor tissues (6–8). Hence, there is a need for an efficacious alternative approach to control T1D.
In the past few decades, human mesenchymal stromal cells (MSCs) presented a promising strategy for treating various immune-mediated diseases including T1D due to their ability to interact with many types of immune cells. Furthermore, MSCs exert immunomodulatory and anti-inflammatory effects on the adaptive and innate immune system both in vivo and in vitro (9, 10), through their secretion of chemokines, cytokines, growth factors, and biological active substances (11, 12). Unfortunately, many limitations have threatened the use of MSCs-based therapies such as, cell senescence, loss of function after cryopreservation, and unpredictability of MSCs behavior in vivo (13, 14). On the other hand, various studies demonstrated that under normal conditions, MSCs express low or insufficient levels of immunomodulatory and immunosuppressive factors (15–17). For these reasons, many ongoing researches proposed the requirement of a practical approach to improve MSCs survival, differentiation potential, therapeutic efficacy, and immunomodulatory functions (18–20). Recently, cell priming or cell activation is considered one of the most interesting functional approaches (21–23). “Human mesenchymal stromal cells Priming” or “MSCs licensing” is defined as the exposure of MSCs to pro-inflammatory cytokines, such as IFN-γ, TNF-α, IL-1α and IL-1β, to increase their anti-inflammatory and immunomodulatory effects on the innate and adaptive immunity (24, 25).
Human mesenchymal stromal cells can strongly interact with many types of immune cells either by cell-cell contact or by their secretome (26). Cell-cell contact is a critical immunosuppressive mechanism of MSCs due to their ability to express a wide range of chemokine receptors and surface adhesion molecules, such as CXCR3, CCR5, CXCL9, CXCL10, CXCL11, VCAM, ICAM-1 and ICAM-2, which enhance their affinity to bind to immune cells and exert their immunomodulatory functions (27–31).
We hypothesized that preconditioning Wharton’s jelly-derived mesenchymal stromal cells (WJ-MSCs) with IFN-γ and TNF-α may lead to robust immunomodulatory effects on immune cells leading to the induction of immunoregulatory cells such as tolDCs and Tregs. Wharton’s jelly-derived mesenchymal stromal cells are optimal candidates for cellular therapies in allogeneic transplantation due to their low immunogenicity and their immunomodulatory properties in addition to their ability to release large amounts of tolerogenic factors by direct and indirect contact with immune cells. Therefore, this study aimed to investigate the effect of primed WJ-MSCs on the profile and functions of mDCs and activated T cells that differentiated from T1D patients.
Materials and Methods
Human Platelet Lysate Preparation
According to Awidi et al. (32), human platelet lysate (PL) was obtained from different platelet apheresis collections at blood banking unit in the Jordan University Hospital, Jordan. The count of platelets was performed using automated hematology analyzer. Pooled samples were subjected to three repeated temperature cycles, frozen at -80°C, then heated at 37°C, then frozen and stored at - 20°C until experimental use.
Isolation and Culture of WJ-MSCs
Human WJ-MSCs were obtained and processed immediately after cesarean section (n=5). All donors signed a consent form before delivery. The protocol was approved by Institutional Review Board (IRB) committee of the Cell Therapy Center, Jordan. (IRB NO. 07-11-2019). Briefly, MSCs were isolated from the Wharton’s Jelly region of the Umbilical Cord (UC). The cords were washed twice with PBS supplemented with 100u/ml Penicillin-Streptomycin, cut into 2 mm2 pieces and placed into 148 cm2 tissue culture plates containing α-MEM supplemented with 10% PL, 100u/ml penicillin/streptomycin and 2mM/ml L-Glutamine. Plates were incubated in a 5% CO2 incubator at 37°c and 95% relative humidity for 7 to 9 days. The medium was changed every 2–3 days. After that, the pieces were removed and the cells were harvested and seeded into T-175 flask for 4 passages. For all experiments, MSCs were used at passages 3-4.
To induce primed cells, WJ-MSCs were cultured in 6- well plates (1x10^5/well) and stimulated with recombinant human 50ng/ml TNF- α and 50ng/ml IFN-γ (R&D Systems, USA) for 48 hours. then cells were washed and stromal cell markers were characterized using hMSCs Analysis kit (BD, UK). The differentiation potential was determined by using StemPro® Differentiation Kit (GIBCO, USA). In parallel experiment, WJ-MSCs were cultured in 6- well plates (1x10^5/well) for 48 hours, then the morphological features of unprimed and primed WJ-MSCs were assessed using Evos Cell Imaging System AMEX1200 (Life Technologies, USA) and the morphological differences were analyzed by Imagej software. The concentration of stimulants was chosen depending on WJ-MSCs morphological changes and based on the expression of immunomodulatory factors.
Generation and Culture of Human mDCs
All patients signed a consent form before blood samples collection. The data of patients groupes were documented before samples collection (Table 1). Fresh heparinized peripheral blood was obtained from five newly onset T1D patients recruited from the Jordan University Hospital, Jordan. In short, mononuclear cells (MNCs) were separated by a Ficoll-Paque gradient centrifugation (specific gravity, 1.077g/ml- Sigma-Aldrich, UK) and cultured in 6-well plates with RPMI 1640 supplemented with 10% PL, 100u/ml penicillin/streptomycin and 2Mm/ml L-Glutamine for two hours in a 5% CO2 incubator at 37°c and 95% relative humidity. Non-adherent cells, T lymphocytes, were collected and tested for CD3+ expression (86.9 ± 5.9) by Flow cytometry using CD3-APC (Biolegend, USA). Adherent cells, monocytes, were washed twice with PBS and were then evaluated for CD14+ expression (92.3± 2) by flow cytometry using CD14-FITC (BD Bioscience, USA). Monocytes were differentiated into immature DCs (iDCs) using 100 ng/ml granulocyte macrophage-colony stimulating factor (GM-CSF) and 50 ng/ml IL-4 (R&D Systems, USA) and incubated for 5 days. Immature DCs were pulsed at a concentration of 1x106 cells/ml with 10µg/ml GAD65 (Abcam, UK), and cultured with 50 ng/ml IL-1β and 50 ng/ml TNF- α (R&D Systems, USA) for 48 hours to generate mDCs. The concentrations were chosen according to Favaro et al. (33). All cells were cultured in 6-well plates with RPMI 1640 supplemented with 10% PL, 100u/ml penicillin/streptomycin and 2Mm/ml L-Glutamine.
T Cells Activation and Proliferation Assay
T lymphocytes were stained with carboxyfluorescein succinimidyl ester (CFSE). The concentration of CFSE fluorescent dye is reduced by cell division. During cell division, CFSE is distributed among daughter cells. Hence, proliferation is assessed by dilution of the CFSE dye (34). Briefly, 1x106 lymphocytes were incubated with CFSE (10mM; Abcam, UK) for 10 minutes in a 5% CO2 incubator at 37°c and 95% relative humidity. The reaction was stopped with RPMI supplemented with 10%PL. T Lymphocytes were co-cultured with GAD65 pulsed mDCs (mDCs: T cell ratio, 1:10) in 6-well plates with RPMI 1640 supplemented with 10% PL, 100u/ml penicillin/streptomycin, 2Mm/ml L-Glutamine, and pulsed at concentration of 1x106 cells/ml with 10µg/ml GAD65 (Abcam, UK) for 4 days (referred to as activated T cells). After that, activated T cells (CD4+ and CD8+) were tested using flow cytometry. In a parallel experiment, T lymphocytes were cultured alone under the same conditions without activation (referred to as inactivated T cells).
WJ-MSCs Co-Cultured With GAD65 Pulsed mDCs and T Cells
Primed WJ-MSCs were cultured overnight in 6-well plates (1x10^5 cells/well) with α-MEM media (Gibco, UK) supplemented with 10% platelet lysate (PL), 100u/ml penicillin/streptomycin and 2Mm/ml L-Glutamine. The medium was replaced by RPMI 1640 supplemented with 10% PL, 100u/ml penicillin/streptomycin and 2Mm/ml L-Glutamine. After that, GAD65-pulsed mDCs were co-cultured with primed WJ-MSCs monolayer (mDCs: WJ-MSCs, ratio 1:1) for 48hours. The ratio was chosen according to Favaro et al. (33). In parallel experiment, primed WJ-MSCs were cultured overnight in 6-well plates (1x10^5 cells/well) with α-MEM media (Gibco, UK) supplemented with 10% platelet lysate (PL), 100u/ml penicillin/streptomycin and 2Mm/ml L-Glutamine. The medium was replaced with RPMI 1640 supplemented with 10% PL, 100u/ml penicillin/streptomycin and 2Mm/ml L-Glutamine. Then, activated T cells were co-cultured with primed WJ-MSCs monolayer (WJ-MSCs: T cells, ratio 1:5) for 48hours. The ratio was chosen according to Liu et al. (35).
Flow Cytometry Analysis of DCs and T Cells
The expression of co-stimulatory molecules and maturation surface markers protein of iDCs, mDCs and mDCs co-cultured with primed WJ-MSCs were analysed by flow cytometer using the following antibodies: CD14- PE-CY7, CD83- PE-CY5, CD80-FITC, CD86- BV421, CD1a-PE, CD40-BV510, CD209-APC and HLA-DR- Percp-CY5.5, all antibodies are purchased from (BD Bioscience, USA). Cell proliferation and cell-surface marker expression of activated T cells (CD4+ and CD8+) were detected using the following antibodies: CD4-APC and CD8- PE-CY7 (Biolegend, USA). Activation of T cells was detected using CD69-PE (Biolegend, USA).
Enzyme- Linked Immunosorbent Spot (ELISPOT)
T lymphocytes (2x 10^5) were pulsed with GAD65 and were then cultured under the following conditions: inactivated T cells, activated T cells cultured with or without primed WJ-MSCs. IFN-γ ELISPOT analysis was performed as previously described (36, 37) and according to the manufacturer’s instructions (Abcam, UK). All patients were positive for GAD65 antigen.
Cytokine Quantification by ELISA
Levels of secreted IL-10, IL-6, and TGF-β in mDCs were measured in cell-free culture supernatant under the following conditions: primed WJ-MSCs alone, mDCs alone, and primed WJ-MSCs co-cultured with mDCs. Regarding T cells cytokines, levels of secreted IL-10, IL-6, IFN-γ, IL-17 and TGF-β were measured in cell-free culture supernatant under the following conditions: inactivated T cells alone, activated T cells alone and primed WJ-MSCs co-cultured with activated T cells. All cytokine kits were purchased from Abcam, UK.
qPCR for Quantification of Gene Expression
To determine the expression of the target genes at the mRNA level, qPCR was performed. Shortly, mDCs and activated T cells were collected from culture by gentle pipetting followed by centrifugation, then the cell pellets were lysed by Trizol-hybrid method for RNA extraction using RNeasy micro kit (Qiagen, USA). The extracted RNA was quantified by a Nanodrop (Thermofisher, USA). To synthesize cDNA, 0.5 μg of total RNA was reverse transcribed by using the PrimeScript RT Master Mix (Takara, China) using T100™ Thermal cycler PCR instrument (BioRad, USA). Primers were designed using Primer-BLAST (RRID : SCR- 003095) and obtained from IDT (USA) (Table 2). The qPCR reaction mix was prepared by mixing 25 ng of cDNA with and 200 nM of gene-specific forward and reverse primers (IDT), 7.2 μL of free nuclease water, and10 μL of SYBR Premix Ex Taq II (Tli RNaseH Plus) (Takara, China). The amplification was performed on CFX96 C1000 Touch thermal cycler (BioRad, USA) with the following temperature setting: (i) 95°C for 3 minutes, (ii) 40 cycles 95°C for 5 seconds and 61°C for 30 seconds. 18s rRNA was used as a reference gene. Each sample was performed in triplicate, and a mean value was calculated. Data were analyzed according to 2−ΔΔCT method using CFX Maestro™ Software - Bio-Rad.
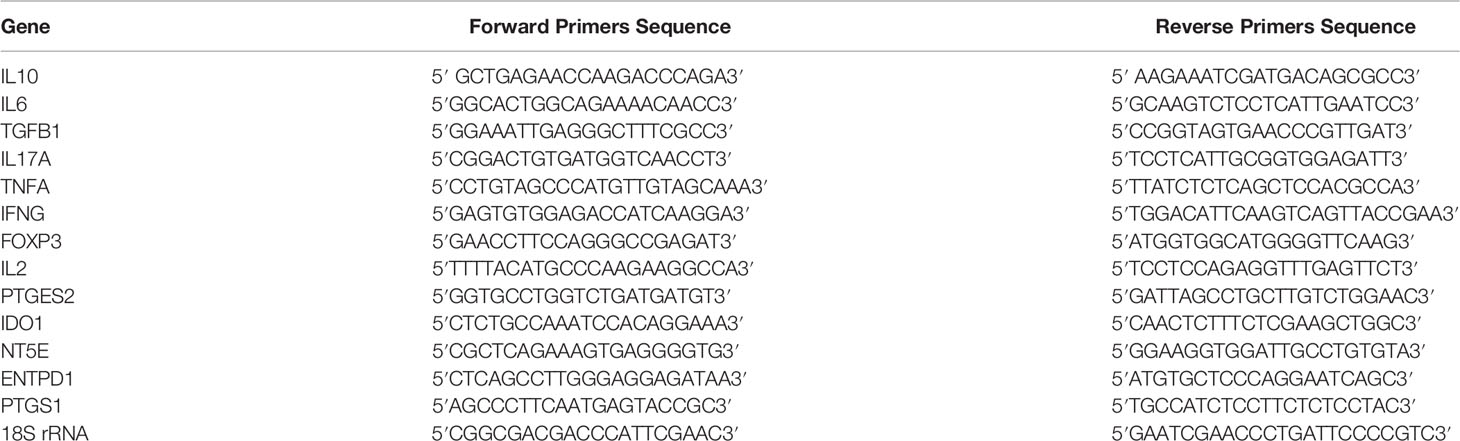
Table 2 qPCR primer sequences of immunostimulatory and immunomodulatory genes that are involved in the immunoregulatory effect of primed WJ-MSCs.
Statistical Analysis
Kolmogorov-Smirnov test was used to verified the normal distribution of data. Graph Pad Prism 6 was used for statistical analysis. Data were presented as Mean ± Standard Deviation (SD). One-way ANOVA, unpaired t-test, and two-way ANOVA with Tukey’s post hoc analysis were performed to analyze the differences between experimental points. qPCR data were analyzed according to 2−ΔΔCT method using CFX Maestro™ Software - Bio-Rad. In all analyses P values <0.05 were considered significant. In qPCR Data Analyses P values <0.01 were considered significant. The used test was described in the figure legends.
Results
Characterization and Differentiation of Primed WJ-MSCs
Morphology and surface marker expression of WJ-MSCs were evaluated at passage 3. Primed WJ-MSCs expressed typical MSCs surface markers CD90 (100% ± 0.0%), CD73 (99.7% ± 0.2%), CD44 (100% ± 0.0%), and CD105 (90.1% ± 4%), and did not express hematopoietic markers; CD34, CD11b, CD19, CD45 and HLA-DR (0.1% ± 0.05%) (Figure 1A). Primed WJ-MSCs exhibited unique morphological features after their priming with TNF- α and IFN-γ compared with unprimed WJ-MSCs (Figures 1B, C and Table 3). The change of MSCs morphology upon TNF- α and IFN-γ stimulation strongly correlated with a previous study that investigated the morphological features of bone marrow derived MSCs (BM-MSCs after being primed with IFN-γ (38).
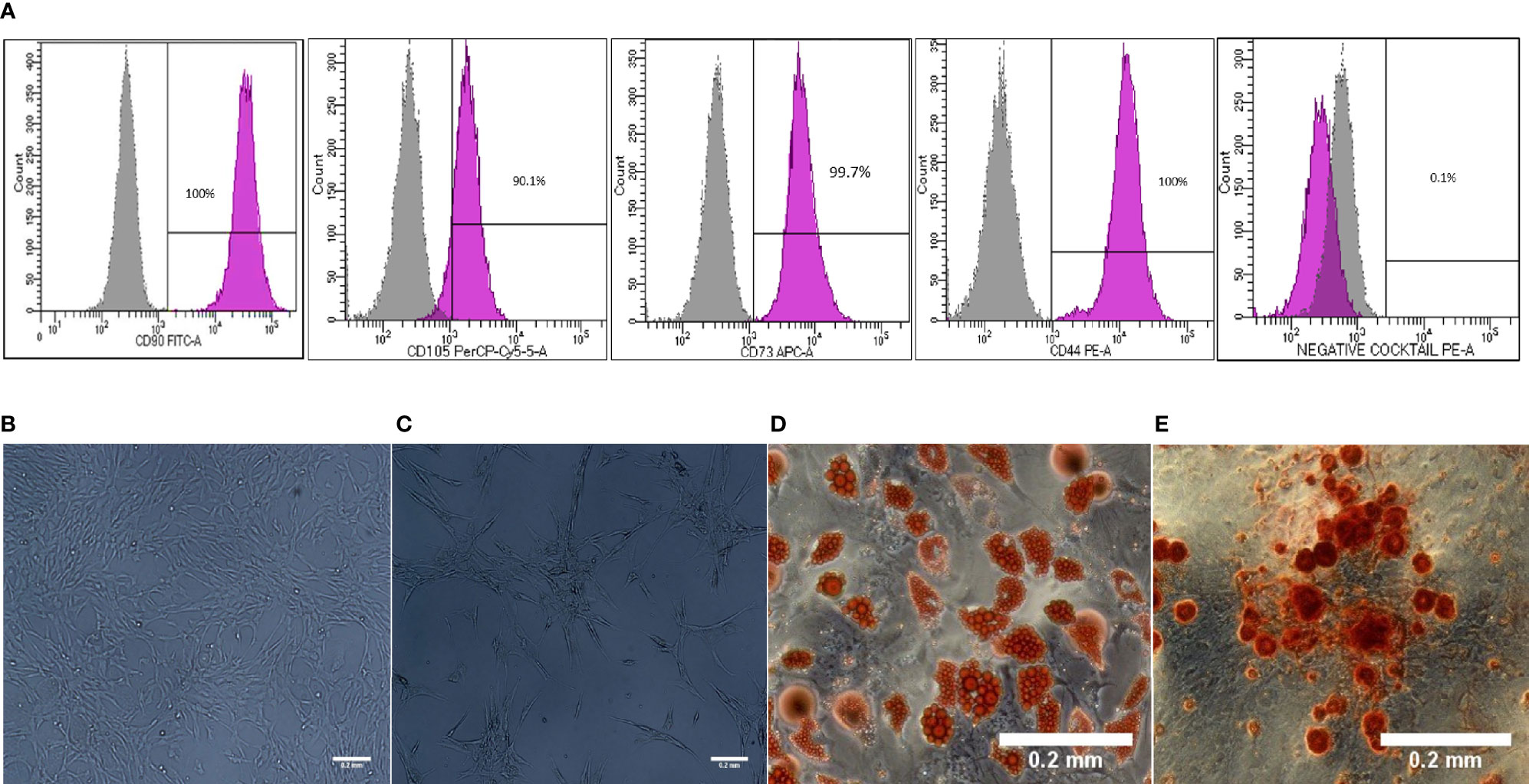
Figure 1 Characterization and differentiation potential of WJ-MSCs priming with IFN-γ and TNF-α (A) Representative flow cytometry histograms showing high expression of CD90, CD105, CD73 and CD44 and lack the expression of negative MSCs cocktail (CD34, CD11b, CD19, CD45 and HLA-DR). Gray peak corresponds with isotype control and the violet peak corresponds with the antibodies. (B) Unprimed WJ-MSCs. (C) Primed WJ-MSCs. (D) Oil red staining of primed WJ-MSCs after culture in adipogenic differentiation media. (E) Alizarin red staining of primed WJ-MSCs after culture with Osteogenic differentiation media. Data were calculated from five samples. Experiments performed in triplicate for each sample. Data were analyzed using FACS canto II. Magnification = 100x.
Primed WJ-MSCs were grown in adipogenic and osteogenic induction media for 14-21 days. All primed WJ-MSCs demonstrated the multilineage differentiation potential (Figures 1D, E).
Effect of Primed WJ-MSCs on GAD65 Pulsing mDCs Profile and Function
The phenotypic analysis of monocyte-generated mDCs cultured in the presence of primed WJ- MSCs showed skewing of mDCs toward an immature state with decreased expression of all co-stimulatory molecules and maturation markers (CD80, CD84, CD86, CD40, CD1a, CD209, and HLA-DR) (Figure 2).
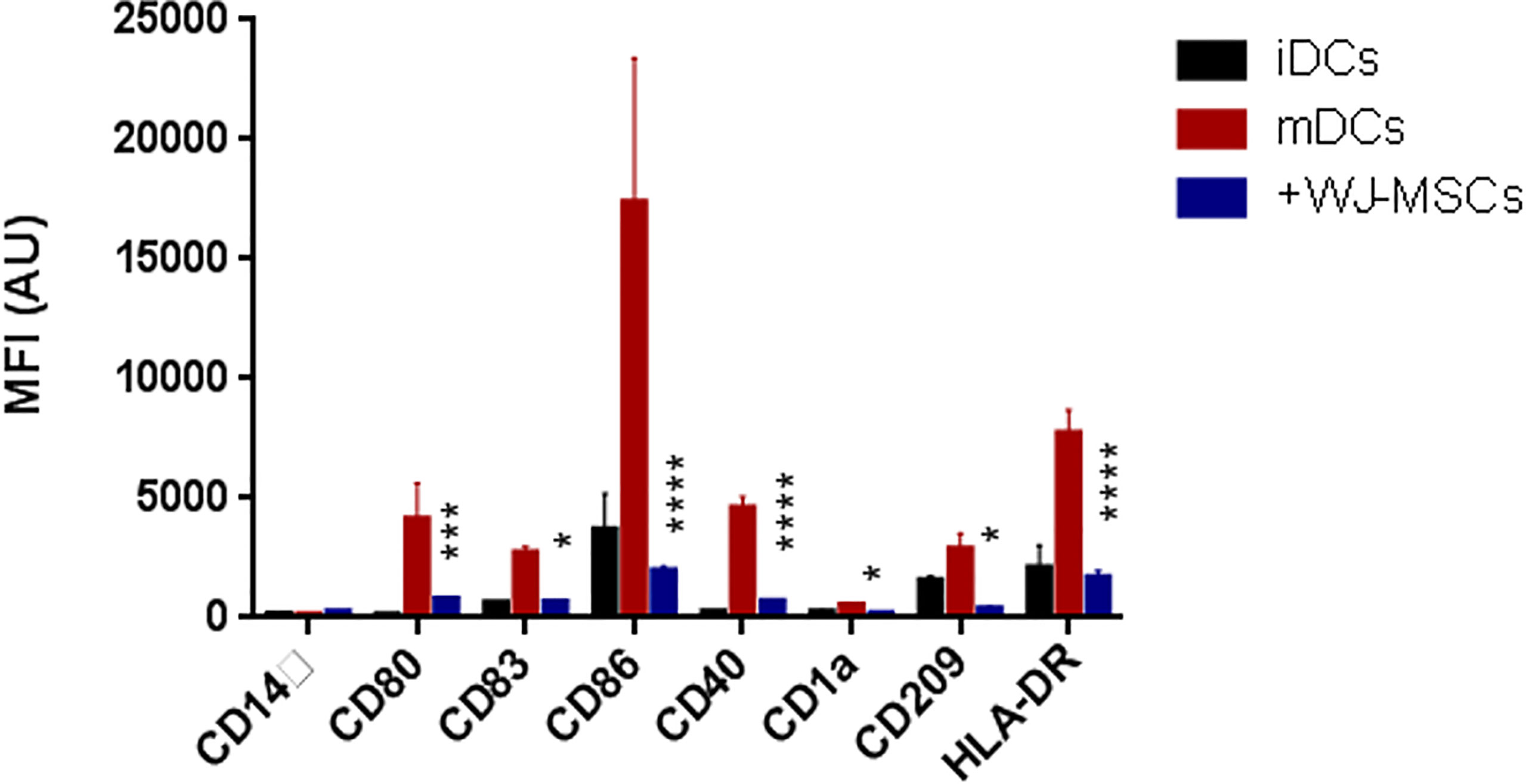
Figure 2 Phenotype of DCs. Mean fluorescence intensity (MFI) ± SD for the surface intensity of co-stimulatory molecules and maturation markers on iDCs (black), GAD65 pulsed mDCs (red), and GAD65 pulsed mDCs co-cultured with primed WJ-MSCs (blue). Data were calculated from three different experiments for each patient. Experiments performed in triplicate for each patient. DCs from five patients were used. Statistical significance was tested using a two-way ANOVA with Tukey’s post hoc for multiple comparisons. In all analyses *p < 0.05, ***p < 0.001 and ****p < 0.0001.
Effect of Primed WJ-MSCs on Activated T Cells Profile and Function
No proliferation was observed in quiescent or inactivated T cells while activated T cells showed high proliferation rate which decreased significantly when co-cultured with primed WJ-MSCs (Figures 3A, B). Activated T cells also exhibited a significant decrease in activated CD69+ T cells. Furthermore, the percentage of CD4+ and CD8+ T cells was also significantly decreased from 21% to 5% of CD4+ and from 17% to 8% of CD8+ (Figure 3C). Moreover, ELISPOT analysis showed significant inhibition of positive IFN-γ response to GAD65 when activated T cells were co-cultured with primed WJ-MSCs (Figure 3D).
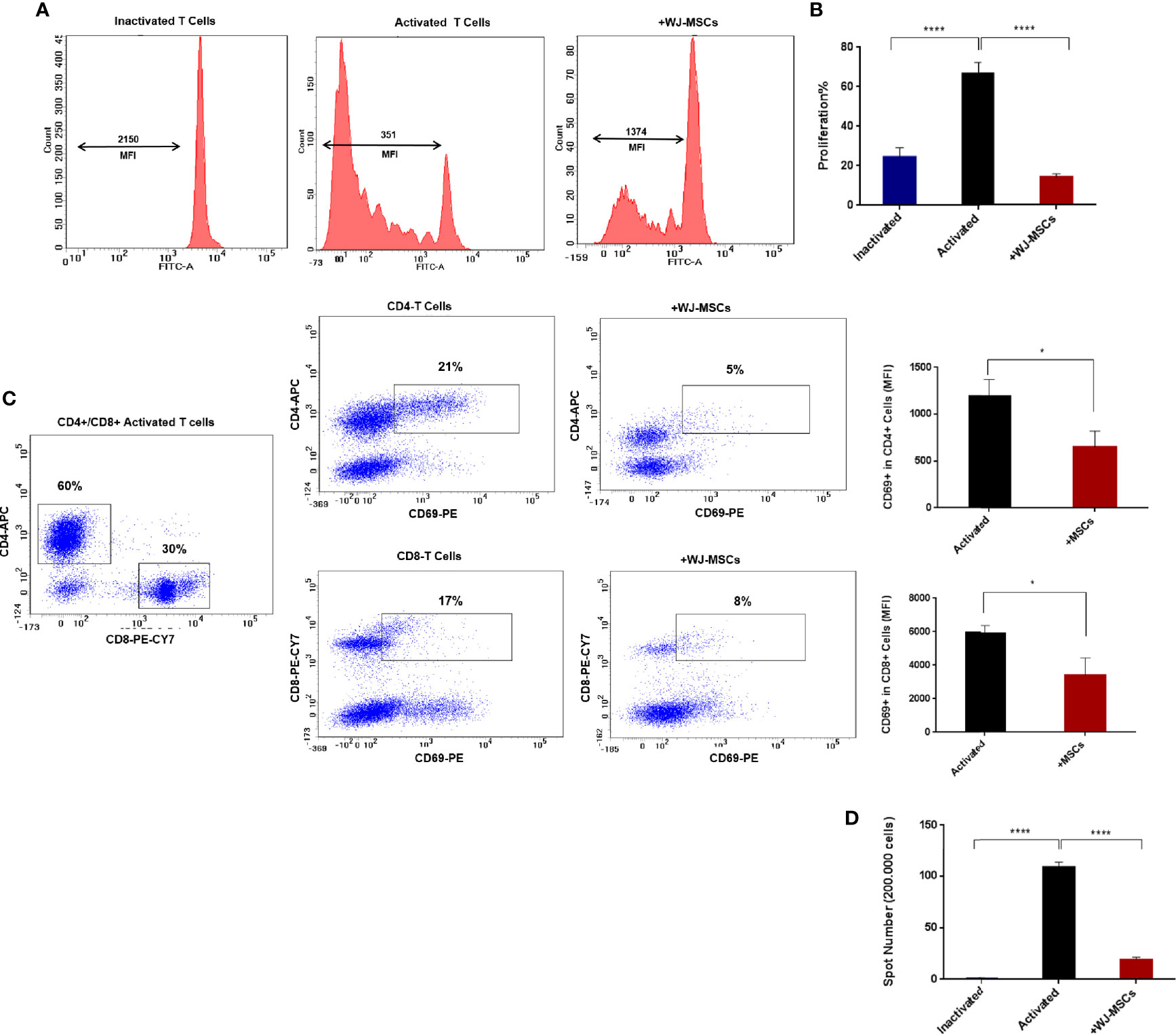
Figure 3 T cells proliferation and activation and IFN-γ ELISPOT analysis (A) Representative flow cytometry analysis and mean fluorescent intensity (MFI) the dilution of CFSE fluorescent dye (B) Percentage of T cell proliferation under the following conditions: inactivated T cells (blue), activated T cells cultured without (black) and with primed WJ-MSCs (red). Statistical significance was tested using a one-way ANOVA with Tukey’s post hoc for multiple comparisons (C) Representative flow cytometry analysis of CD4+/ CD8+ activated T cells and activated CD69+ T cells cultured with and without primed WJ-MSCs and MFI ± SD of CD69+ T cell in CD4+ and CD8+ activated T cells under the following conditions: activated T cells alone (black) and activated T cells co-cultured with primed WJ-MSCs (red). An unpaired t-test was performed to test statistical significance (D). Mean ± SD of IFN-γ spots per well (200.000 cells) under the following conditions: inactivated T cells (blue), activated T cells cultured without (black) and with primed WJ-MSCs (red). Statistical significance was tested using a one-way ANOVA with Tukey’s post hoc for multiple comparisons. Data were calculated from three different experiments for each patient. Experiments performed in triplicate for each patient. T cells from five patients were used. For all analyses *p < 0.05, and ****p < 0.0001.
Effect of Primed WJ-MSCs on the Secretion Level of Pro-Inflammatory and Anti-Inflammatory Cytokines
The secretion levels of anti-inflammatory cytokines (IL-6, IL-10 and TGF-β) were significantly increased when mDCs were co-cultured with primed WJ-MSCs (Figure 4A). Furthermore, the secretion levels of anti-inflammatory cytokines (IL-6, IL-10 and TGF-β1) were significantly increased when activated T cells were co-cultured with primed WJ-MSCs. Activated T cells secreted larger amounts of IFN-γ and IL-17 than quiescent or inactivated T cells. The levels of IFN-γ and IL-17 were significantly decreased when activated T cells were co-cultured with primed WJ-MSCs (Figure 4B).
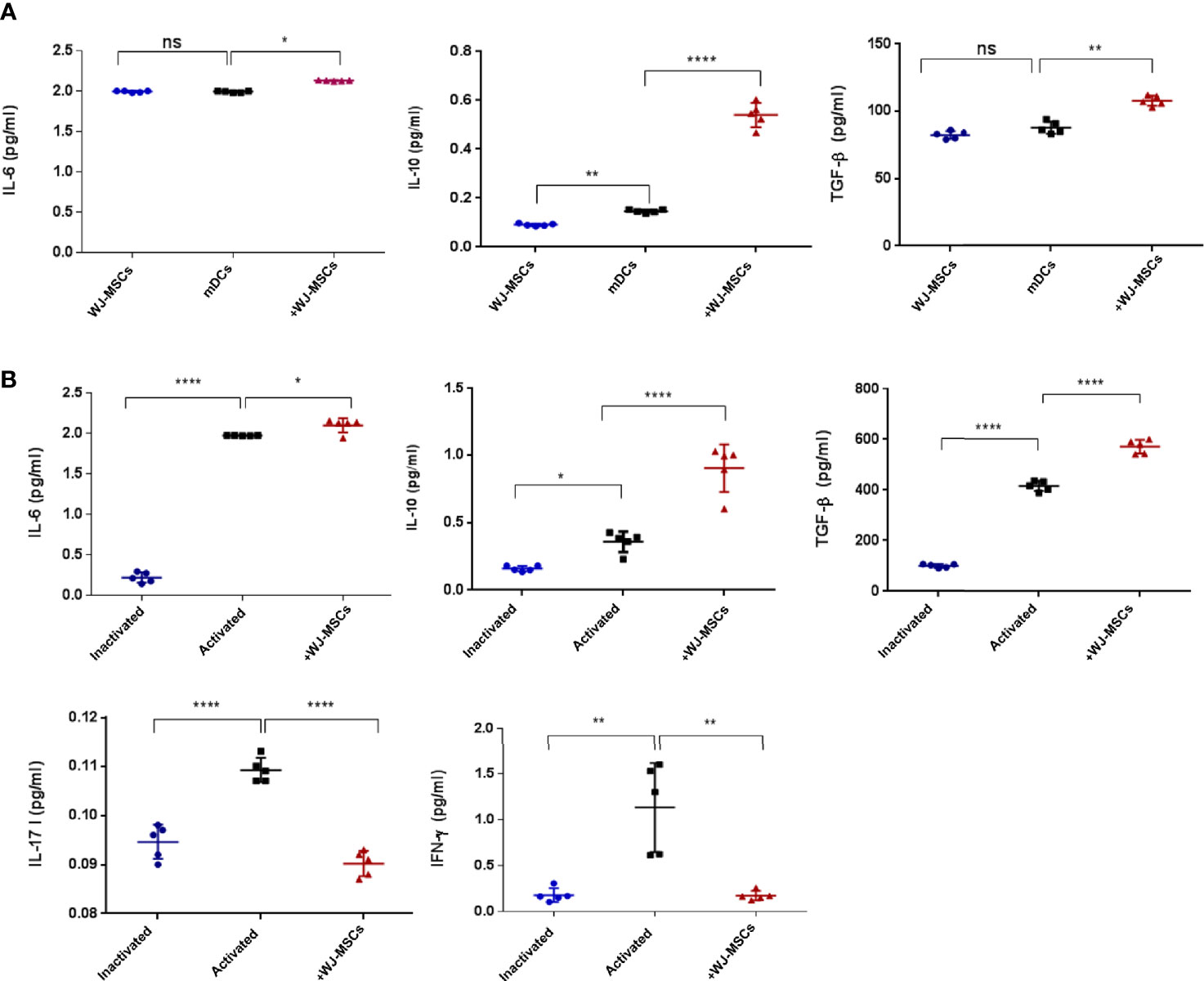
Figure 4 Cytokines secretion level. (A) Mean ± SD levels of secreted IL-6, IL-10, and TGF-β in cell free culture supernatant under the following culture conditions: primed WJ-MSCs alone (blue), GAD65- pulsed mDCs co-cultured without (black) and with primed WJ-MSCs (red). (B) Mean ± SD levels of secreted IL-6, IL-10, TGF-β, IL-17 and IFN-γ in cell free culture supernatant under the following culture conditions: inactivated T cells (blue), activated T cells without (black) or with primed WJ-MSCs (red). Data were calculated from five patients. Experiments performed in duplicate for each patient. In all analyses *p < 0.05, **p < 0.01 and ****p < 0.0001. ns, not significant.
TNF-α and IFN-γ Priming WJ-MSCs Enhances the Expression of Immunomodulatory Genes and Impair the Expression of Immunostimulatory Genes in mDCs and Activated T Cells
As shown in (Figure 5), gene expression profile was evaluated in mDCs. The results showed significant increase in the expression of immunoregulatory genes (IL10, IDO1, NT5E/ENTPD1, FOXP3, IL6, and PTGES2/PTGS1). In addition, they showed insignificant increase in the expression of TGFB1. Furthermore, mDCs showed significant increase in the expression of TNFA. Fold regulation results confirmed the upregulation and downregulation of mDCs target genes. Also, no expression of IFNG, IL-17A and, IL-2 was detected in mDCs (Table 4).
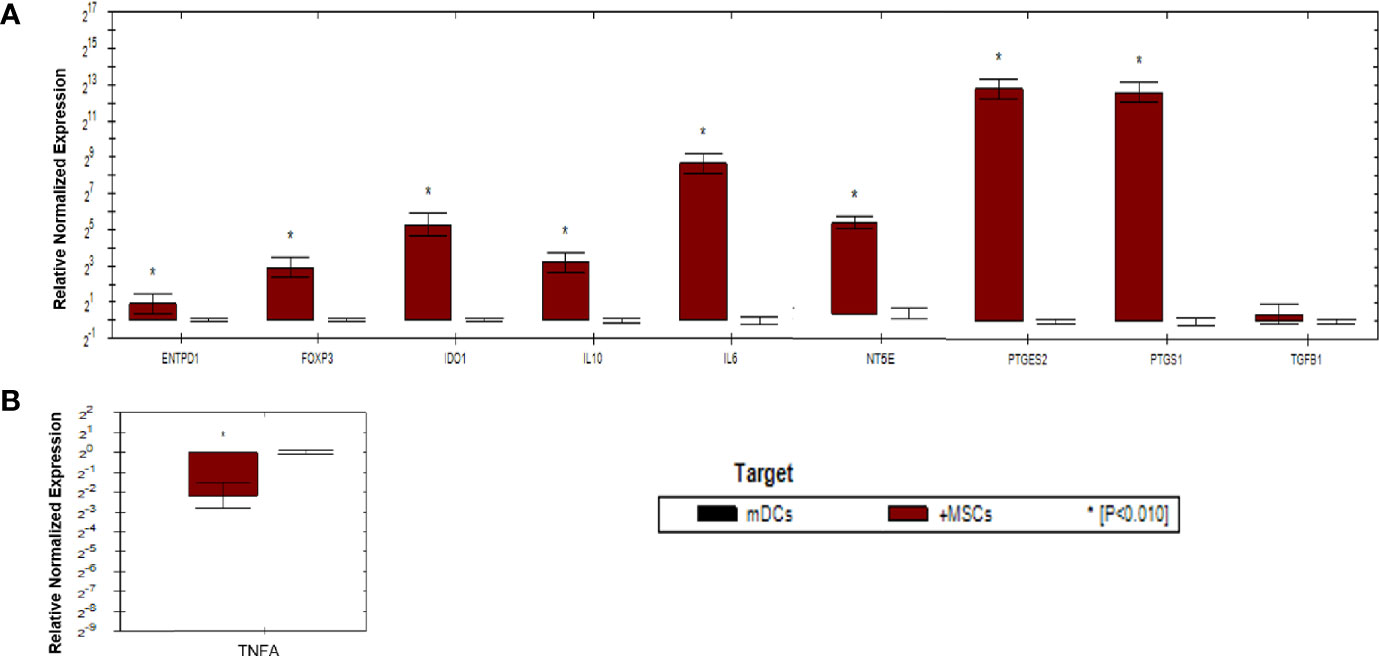
Figure 5 Relative normalized expression of immunomodulatory and immunostimulatory genes of mDCs after co-cultured with primed WJ-MSCs. (A) upregulated genes. (B) downregulated gene. Mature dendritic cells from four patients were used. Results were normalized to 18S rRNA Each sample was performed in triplicate, and a mean value was calculated. Data were analyzed according to 2−ΔΔCT method using CFX Maestro™ Software - Bio-Rad. *p ≤ 0.01 and fold change ≥ 1.5.
As shown in (Figure 6), activated T cells showed significant increase in the expression of immunoregulatory genes (IL10, IDO1, NT5E/ENTPD1, FOXP3, IL6, and PTGES2/PTGS1). Significant increase in the expression of TGFB1 was detected in activated T cells compared to mDCs. Moreover, significant downregulation of immunostimulatory genes (TNFA, IFNG, IL-17A, and IL-2) was also detected. Fold regulation results confirmed the upregulation and downregulation of activated T cells target genes. However, T cells exhibited more upregulation and downregulation in gene expression than mDCs (Table 5).
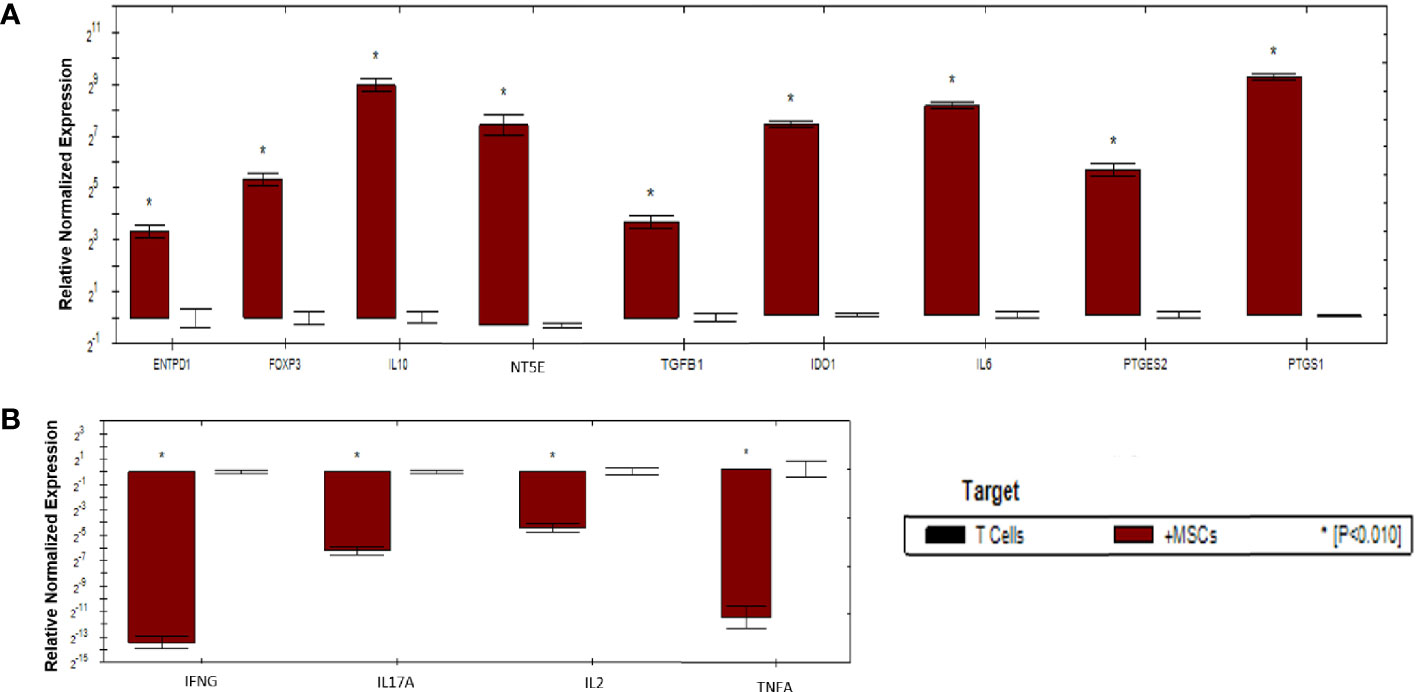
Figure 6 Relative normalized expression of immunomodulatory and immunostimulatory genes of activated T cells after co-cultured with primed WJ-MSCs. (A) upregulated genes. (B) downregulated genes. T cells from four patients were used. Results were normalized to 18S rRNA Each sample was performed in triplicate, and a mean value was calculated. Data were analyzed according to 2−ΔΔCT method using CFX Maestro™ Software - Bio-Rad. *p ≤ 0.01 and fold change ≥ 1.5.
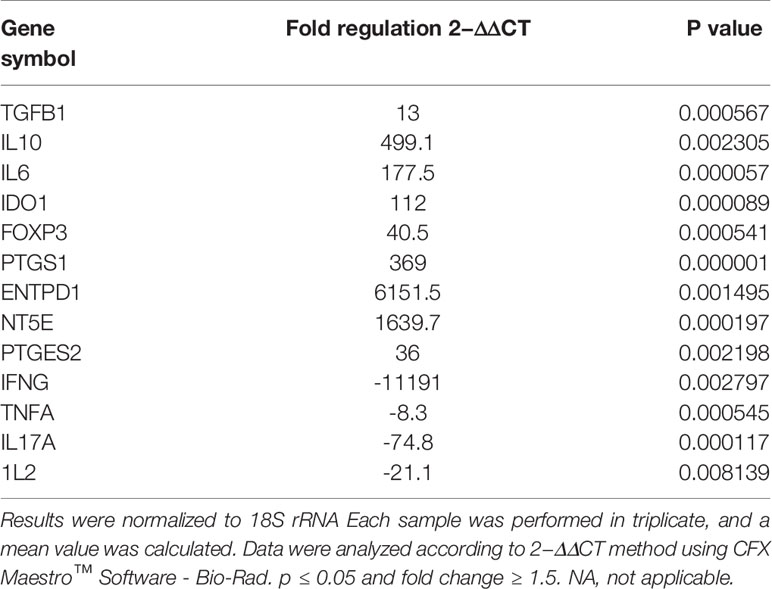
Table 5 Upregulated and downregulated genes upon priming WJ-MSCs with TNF-α and IFN-γ and cultured with activated T cells.
Discussion
Type 1 diabetes is a well-known autoimmune disease characterized by specific adaptive immunity against β-cell antigens. Type 1 diabetes occurs when the balance between the regulatory and inflammatory T-cells is lost. To date, there is no effective therapeutic approach for the management of T1D but recently, MSCs have been reported as a promising immunosuppressant in various autoimmune diseases including T1D (39, 40). However, successful MSCs-based therapy still faces obstacles due to the high sensitivity of MSCs to the environment of immune-mediated diseases, the differences of culturing protocols, and the cell senescence that results from overexpansion of cells. Therefore, a current concern is how to enhance the immunomodulatory effects of MSCs.
In this study, the immunomodulatory effects of primed WJ-MSCs were investigated. The results showed that high differentiation potential and unique morphological features were obtained when WJ-MSCs were primed with both IFN-γ and TNF-α. Accordingly, our findings provide an additional evidence that the morphological appearance can be used to predict the function of MSCs in vitro when pre-conditioned with both TNF-α and IFN-γ.
Primed WJ-MSCs exerted an immunomodulatory effect on mDCs by skewing toward tolerogenic or immature phenotype. Tolerogenic DCs express low amounts of co-stimulatory molecules on their surfaces and display increased production of anti-inflammatory cytokines including IL-6, IL-10, and TGF-β1. Furthermore, tolDCs are capable of driving T cells to differentiate into Tregs. This increase is a result of the overexpression of IL6, IL10, and FOXP3.
Primed WJ-MSCs exhibited immunomodulatory effect on CD4+ and CD8+ T cells by producing tolDCs which inhibit antigen-specific T cell responses through induction T cell anergy.
The levels of IFN-γ were significantly decreased when activated T cells were co-cultured with primed WJ-MSCs. This led us to conclude that primed WJ-MSCs suppress T cells-mediated autoimmunity. It is well known that IFN-γ are produced extensively by activated T cells and it is a key moderator of T cells-mediated immunity (41, 42).
As for the production of immunomodulatory factors by mDCs and by activated T cells after co-culturing with primed WJ-MSCs, significant upregulation of IDO1 expression in both mDCs and activated T cells was detected. This provides a potent evidence which confirms that priming WJ-MSC with TNF-α and IFN-γ promotes the immunosuppressive potential of these cells. This can be considered a critical finding because quiescent MSCs are unable to express IDO1 (43). However, IDO1 is considered one of the key modulators of the immune tolerance mechanism (43, 44), and is involved in the tryptophan catabolites or depletion (45–47) which is responsible for the inhibition of T cell activation and proliferation via the induction of T cell anergy (48, 49).
Both TGF-β1 and IL-10 are critical anti-inflammatory cytokines for Treg formation (50, 51). Moreover, they are capable of inhibiting T cells proliferation and activation as well as suppressing of Th17 generation. These results are consequences of the increase in the production of TGF-β1, IL-10, IFN-γ, and IL-17 after activated T cells are co-cultured with primed WJ-MSCs. Furthermore, TGF-β1 is a key anti-inflammatory cytokine which is responsible for the formation of Tregs due to its ability to upregulate FOXP3.
The downregulation of IFNG, IL2 and IL17A expression and the significant reduction of IFN-γ and IL-17 cytokines after activated T cells were co-cultured with primed WJ-MSCs represent a strong evidence of the suppression of T cells proliferation and activation. In addition, the downregulation of IL2 represents a strong evidence of the inhibition of T cells-mediated autoimmunity because IL2 are produced only by CD4+ and CD8+ T cells. Moreover, IL2 plays a pivotal role in T cells- receptor signaling pathway (52).
Significant expression of PTGS1 and PTGES2 was observed when primed WJ-MSCs were co-cultured with mDCs and activated T cells. PTGES2 and PTGS1 genes are responsible for the production of prostaglandin E2 (PGE2) which is involved in the immune-suppressive mechanism of MSCs and it increases the expression of anti-inflammatory factors (53–55). The upregulation of IL10, and TGFB1 as well as the significant production of TGF-β1 and IL-10 might be explained by the overexpression of PTGS1 and PTGES2.
Interleukine-6 (IL-6) is a key factor in the formation of Tregs and in the suppression of pro-inflammatory responses (56, 57). The upregulation of IL6 gene and the increase of IL-6 cytokine may explain the inhibition of pro-inflammatory responses of mDCs and activated T cells which is caused by primed WJ-MSCs. Moreover, a previous study illustrated that immunosuppressive properties of amnion-derived MSCs are not constitutive, but require a supportive signal to produce PGE2, IDO1, and a high level of IL-6 (51). Our findings may support the immunomodulatory effect of primed WJ-MSCs on mDCs and activated Tcells.
Destructed β cells play a key role in the progression of T1D through the release of dangerous extracellular ATP signal which acts as a potent immune-stimulator to enhance inflammatory responses. So, we investigated the effect of primed WJ-MSCs on the expression of ENTPD1/NT5E genes which play a pivotal role in the production of extracellular adenosine through ATP hydrolysis. However, extracellular adenosine act as a potent immune-regulator signal that modulate innate and adaptive immunity. Moreover, adenosine can prevent the activation, proliferation and cytokine production in CD4+ and CD8+ T cells (58–60). ENTPD1/NT5E are considered the most important genes in the immunosuppressive mechanisms that attribute to Treg formation and T cell anergy (61, 62). Furthermore, they maintain the balance between ATP/adenosine to increase immune hemostasis (63). Significant increase in the expression of ENTPD1/NT5E may be considered a robust finding because of their role in maintaining balance between anti-inflammatory and pro-inflammatory factors.
Although the direct contact with MSCs is critical for the immunomodulatory effects, the paracrine effects of MSCs cannot be ignored. The Increase in the production of anti-inflammatory cytokines (IL-10, IL-6 and TGF-β1) and the suppression in the production of pro-inflammatory cytokines (IL-17 and IFN-γ) in conditioned media suggest that the immunomodulatory effects of primed WJ-MSCs after being co-cultured with mDC and T cells seems to be a consequence of a synergic effect mediated by both the direct contact and secretome of primed WJ-MSCs.
Collectively, this study confirmed that WJ-MSCs primed by IFN-γ and TNF-α modulated mDCs-mediated antigen presentation through the induction of tolDCs in addition to the modulation of antigen-specific-T cell responses through the induction of T cell anergy. More importantly, this study paves the road to utilizing primed WJ-MSCs-based transplantation therapies. Further study of primed WJ-MSCs cellular therapies in an animal model of T1D is recommended before attempting in humans. Successful treatment in humans would involve establishing safety first, then optimizing administration in respect to disease stage. Premature of primed WJ-MSCs therapies in human would not only risk safety and efficacy, but also provide false hope to patients.
Data Availability Statement
The raw data supporting the conclusions of this article will be made available by the authors, without undue reservation.
Ethics Statement
The protocol was approved by Institutional Review Board (IRB) committee of the Cell Therapy Center, The University of Jordan, Jordan (IRB NO. 07-11-2019). Written informed consent to participate in this study was provided by the participants’ legal guardian/next of kin.
Author Contributions
MA-M designed and conducted the study. MA-M and SM analyzed and interpreted data. MA-M wrote the original manuscript. NA revised the manuscript. HJ and AA were the administrators of the project. AA provided the advices for study. All authors contributed to the article and approved the submitted version.
Funding
This work was financed by The University of Jordan, Dean of Scientific Research, and Cell Therapy Center/The University of Jordan.
Conflict of Interest
The authors declare that the research was conducted in the absence of any commercial or financial relationships that could be construed as a potential conflict of interest.
Publisher’s Note
All claims expressed in this article are solely those of the authors and do not necessarily represent those of their affiliated organizations, or those of the publisher, the editors and the reviewers. Any product that may be evaluated in this article, or claim that may be made by its manufacturer, is not guaranteed or endorsed by the publisher.
Acknowledgments
We are grateful for blood sample donors to contribution in this study. All the participants at Cell Therapy Center are thanked for their distinguish help.
References
1. Di Lorenzo TP, Peakman M, Roep BO. Translational Mini-Review Series on Type 1 Diabetes: Systematic Analysis of T Cell Epitopes in Autoimmune Diabetes. Clin Exp Immunol (2007) 148(1):1–16. doi: 10.1111/j.1365-2249.2006.03244.x
2. Scotto M, Afonso G, Larger E, Raverdy C, Lemonnier FA, Carel J, et al. Zinc Transporter (ZnT) 8 186–194 Is an Immunodominant CD8+ T Cell Epitope in HLA-A2+ Type 1 Diabetic Patients. Diabetologia (2012) 55(7):2026–31. doi: 10.1007/s00125-012-2543-z
3. Ludvigsson J, Krisky D, Casas R, Battelino T, Castaño L, Greening J, et al. GAD65 Antigen Therapy in Recently Diagnosed Type 1 Diabetes Mellitus. New Engl J Med (2012) 366(5):433–42. doi: 10.1056/NEJMoa1107096
4. Calderon B, Unanue ER. Antigen Presentation Events in Autoimmune Diabetes. Curr Opin Immunol (2012) 24(1):119–28. doi: 10.1016/j.coi.2011.11.005
5. Wållberg M, Cooke A. Immune Mechanisms in Type 1 Diabetes. Trends Immunol (2013) 34(12):583–91. doi: 10.1016/j.it.2013.08.005
6. Ilgun H, Kim JW, Luo L. Adult Stem Cells and Diabetes Therapy. J Stem Cell Res Transplant (2015) 2(2):1020.
7. Lilly MA, Davis MF, Fabie JE, Terhune EB, Gallicano GI. Current Stem Cell Based Therapies in Diabetes. Am J Stem Cells (2016) 5(3):87.
8. Chen S, Du K, Zou C. Current Progress in Stem Cell Therapy for Type 1 Diabetes Mellitus. Stem Cell Res Ther (2020) 11(1):1–13. doi: 10.1186/s13287-020-01793-6
9. Wang M, Yuan Q, Xie L. Mesenchymal Stem Cell-Based Immunomodulation: Properties and Clinical Application. Stem Cells Int (2018) 2018:3057624. doi: 10.1155/2018/3057624
10. Cipriani P, Carubbi F, Liakouli V, Marrelli A, Perricone C, Perricone R, et al. Stem Cells in Autoimmune Diseases: Implications for Pathogenesis and Future Trends in Therapy. Autoimmun Rev (2013) 12(7):709–16. doi: 10.1016/j.autrev.2012.10.004
11. Siegel G, Schäfer R, Dazzi F. The Immunosuppressive Properties of Mesenchymal Stem Cells. Transplantation (2009) 87(9S):S45–9. doi: 10.1097/TP-0b013e3181a285b0
12. Ren G, Zhang L, Zhao X, Xu G, Zhang Y, Roberts AI, et al. Mesenchymal Stem Cell-Mediated Immunosuppression Occurs via Concerted Action of Chemokines and Nitric Oxide. Cell Stem Cell (2008) 2(2):141–50. doi: 10.1016/j.stem.2007.11.014
13. Cesarz Z, Tamama K. Spheroid Culture of Mesenchymal Stem Cells. Stem Cells Int (2016) 2016:9176357. doi: 10.1155/2016/9176357
14. Chinnadurai R, Copland IB, Garcia MA, Petersen CT, Lewis CN, Waller EK, et al. Cryopreserved Mesenchymal Stromal Cells Are Susceptible to T-Cell Mediated Apoptosis Which Is Partly Rescued by Ifnγ Licensing. Stem Cells (2016) 34(9):2429–42. doi: 10.1002/stem.2415
15. Li W, Ren G, Huang Y, Su J, Han Y, Li J, et al. Mesenchymal Stem Cells: A Double-Edged Sword in Regulating Immune Responses. Cell Death Differ (2012) 19(9):1505–13. doi: 10.1038/cdd.2012.26
16. Gebler A, Zabel O, Seliger B. The Immunomodulatory Capacity of Mesenchymal Stem Cells. Trends Mol Med (2012) 18(2):128–34. doi: 10.1016/j.molmed.2011.10.004
17. de Cássia Noronha N, Mizukami A, Caliári-Oliveira C, Cominal JG, Rocha JLM, Covas DT, et al. Priming Approaches to Improve the Efficacy of Mesenchymal Stromal Cell-Based Therapies. Stem Cell Res Ther (2019) 10(1):1–21. doi: 10.1186/s13287-019-1224-y
18. Zhou Y, Tsai TL, Li WJ. Strategies to Retain Properties of Bone Marrow–Derived Mesenchymal Stem Cells Ex Vivo. Ann New York Acad Sci (2017) 1409(1):3. doi: 10.1111/nyas.13451
19. Nava MM, Raimondi MT, Pietrabissa R. Controlling Self-Renewal and Differentiation of Stem Cells via Mechanical Cues. J Biomedicine Biotechnol (2012) 2012:797410. doi: 10.1155/2012/797410
20. Huang C, Dai J, Zhang XA. Environmental Physical Cues Determine the Lineage Specification of Mesenchymal Stem Cells. Biochim Biophys Acta (BBA) General Subj (2015) 1850(6):1261–6. doi: 10.1016/j.bbagen-2015.02.011
21. Najar M, Krayem M, Merimi M, Burny A, Meuleman N, Bron D, et al. Insights Into Inflammatory Priming of Mesenchymal Stromal Cells: Functional Biological Impacts. Inflammation Res (2018) 67(6):467–77. doi: 10.1007/s00011-018-1131-1
22. Hu C, Li L. Preconditioning Influences Mesenchymal Stem Cell Properties In Vitro and In Vivo. J Cell Mol Med (2018) 22(3):1428–42. doi: 10.1111/jcmm.13492
23. Ji ST, Kim H, Yun J, Chung JS, Kwon SM. Promising Therapeutic Strategies for Mesenchymal Stem Cell-Based Cardiovascular Regeneration: From Cell Priming to Tissue Engineering. Stem Cells Int (2017) 2017:3945403. doi: 10.1155/2017/3945403
24. Chen G, Ye Y, Cheng M, Tao Y, Zhang K, Huang Q, et al. Quercetin Combined With Human Umbilical Cord Mesenchymal Stem Cells Regulated Tumour Necrosis Factor-A/Interferon-G-Stimulated Peripheral Blood Mononuclear Cells via Activation of Toll-Like Receptor 3 Signalling. Front Pharmacol (2020) 11:org/10.3389/fphar.2020.00499. doi: 10.3389/fphar.2020.00499
25. Tu Z, Li Q, Bu H, Lin F. Mesenchymal Stem Cells Inhibit Complement Activation by Secreting Factor H. Stem Cells Dev (2010) 19(11):1803–9. doi: 10.1089/scd.2009.0418
26. Najar M, Raicevic G, Fayyad-Kazan H, Bron D, Toungouz M, Lagneaux L. Mesenchymal Stromal Cells and Immunomodulation: A Gathering of Regulatory Immune Cells. Cytotherapy (2016) 18(2):160–71. doi: 10.1016/j.jcyt-2015.10.011
27. Haddad R, Saldanha-Araujo F. Mechanisms of T-Cell Immunosuppression by Mesenchymal Stromal Cells: What Do We Know So Far? BioMed Res Int (2014) 2014:216806. doi: 10.1155/2014/216806
28. Ghannam S, Pène J, Torcy-Moquet G, Jorgensen C, Yssel H. Mesenchymal Stem Cells Inhibit Human Th17 Cell Differentiation and Function and Induce a T Regulatory Cell Phenotype. J Immunol (2010) 185(1):302–12. doi: 10.4049/jimmunol.0902007
29. Galipeau J, Krampera M, Barrett J, Dazzi F, Deans RJ, DeBruijn J, et al. International Society for Cellular Therapy Perspective on Immune Functional Assays for Mesenchymal Stromal Cells as Potency Release Criterion for Advanced Phase Clinical Trials. Cytotherapy (2016) 18(2):151–9. doi: 10.1016/j.jcyt.2015.11.008
30. Carrero R, Cerrada I, Lledó E, Dopazo J, García-García F, Rubio MP, et al. Il1β Induces Mesenchymal Stem Cells Migration and Leucocyte Chemotaxis Through NF-κB. Stem Cell Rev Rep (2012) 8(3):905–16. doi: 10.1007/s12015-012-9364-9
31. Kim DS, Jang IK, Lee MW, Ko YJ, Lee DH, Lee JW, et al. Enhanced Immunosuppressive Properties of Human Mesenchymal Stem Cells Primed by Interferon-γ. EBioMedicine (2018) 28:261–73. doi: 10.1016/j.ebiom-2018.01.002
32. Awidi A, Ababneh N, Alkilani H, Salah B, Nazzal S, Zoghool M, et al. Evaluation of the Growth and Osteogenic Differentiation of ASCs Cultured With PL and Seeded on PLGA Scaffolds. J Materials Sci: Materials Med (2015) 26(2):84. doi: 10.1007/s10856-015-5404-8
33. Favaro E, Carpanetto A, Caorsi C, Giovarelli M, Angelini C, Cavallo-Perin P, et al. Human Mesenchymal Stem Cells and Derived Extracellular Vesicles Induce Regulatory Dendritic Cells in Type 1 Diabetic Patients. Diabetologia (2016) 59(2):325–33. doi: 10.1007/s00125-015-3808-0
34. Hross S, Hasenauer J. Analysis of CFSE Time-Series Data Using Division-, Age-and Label-Structured Population Models. Bioinformatics (2016) 32(15):2321–9. doi: 10.1093/bioinformatics/btw131
35. Liu Q, Zheng H, Chen X, Peng Y, Huang W, Li X, et al. Human Mesenchymal Stromal Cells Enhance the Immunomodulatory Function of CD8+ CD28– Regulatory T Cells. Cell Mol Immunol (2015) 12(6):708–18. doi: 10.1038/cmi.2014.118
36. Zanone MM, Favaro E, Miceli I, Grassi G, Camussi E, Caorsi C, et al. Human Mesenchymal Stem Cells Modulate Cellular Immune Response to Islet Antigen Glutamic Acid Decarboxylase in Type 1 Diabetes. J Clin Endocrinol Metab (2010) 95(8):3788–97. doi: 10.1210/jc.2009-2350
37. Favaro E, Carpanetto A, Lamorte S, Fusco A, Caorsi C, Deregibus MC, et al. Human Mesenchymal Stem Cell-Derived Microvesicles Modulate T Cell Response to Islet Antigen Glutamic Acid Decarboxylase in Patients With Type 1 Diabetes. Diabetologia (2014) 57(8):1664–73. doi: 10.1007/s00125-014-3262-4
38. Klinker MW, Marklein RA, Surdo JLL, Wei CH, Bauer SR. Morphological Features of IFN-γ–Stimulated Mesenchymal Stromal Cells Predict Overall Immunosuppressive Capacity. Proc Natl Acad Sci (2017) 114(13):E2598–607. doi: 10.1073/pnas.1617933114
39. Yang C, Wu M, You M, Chen Y, Luo M, Chen Q. The Therapeutic Applications of Mesenchymal Stromal Cells From Human Perinatal Tissues in Autoimmune Diseases. Stem Cell Res Ther (2021) 12(1):1–15. doi: 10.1186/s13287-021-02158-3
40. Figliuzzi M, Bonandrini B, Silvani S, Remuzzi A. Mesenchymal Stem Cells Help Pancreatic Islet Transplantation to Control Type 1 Diabetes. World J Stem Cells (2014) 6(2):163. doi: 10.4252/wjsc.v6.i2.163
41. Bernardo ME, Fibbe WE. Mesenchymal Stromal Cells: Sensors and Switchers of Inflammation. Cell Stem Cell (2013) 13(4):392–402. doi: 10.1016/j.stem-2013.09.006
42. Yu PF, Huang Y, Han YY, Lin LY, Sun WH, Rabson AB, et al. Tnfα-Activated Mesenchymal Stromal Cells Promote Breast Cancer Metastasis by Recruiting CXCR2+ Neutrophils. Oncogene (2017) 36(4):482–90. doi: 10.1038/onc.2016.217
43. Mattar P, Bieback K. Comparing the Immunomodulatory Properties of Bone Marrow, Adipose Tissue, and Birth-Associated Tissue Mesenchymal Stromal Cells. Front Immunol (2015) 6:2015.00560. doi: 10.3389/fimmu.2015.00560
44. Opitz CA, Litzenburger UM, Lutz C, Lanz TV, Tritschler I, Köppel A, et al. Toll-Like Receptor Engagement Enhances the Immunosuppressive Properties of Human Bone Marrow-Derived Mesenchymal Stem Cells by Inducing Indoleamine-2, 3-Dioxygenase-1 via Interferon-β and Protein Kinase R. Stem Cells (2009) 27(4):909–19. doi: 10.1002/stem.7
45. Munn DH, Mellor AL. Indoleamine 2, 3 Dioxygenase and Metabolic Control of Immune Responses. Trends Immunol (2013) 34(3):137–43. doi: 10.1016/j.it.2012.10.001
46. Munn DH, Shafizadeh E, Attwood JT, Bondarev I, Pashine A, Mellor AL. Inhibition of T Cell Proliferation by Macrophage Tryptophan Catabolism. J Exp Med (1999) 189(9):1363–72. doi: 10.1084/jem.189.9.1363
47. Nguyen NT, Kimura A, Nakahama T, Chinen I, Masuda K, Nohara K, et al. Aryl Hydrocarbon Receptor Negatively Regulates Dendritic Cell Immunogenicity via a Kynurenine-Dependent Mechanism. Proc Natl Acad Sci (2010) 107(46):19961–6. doi: 10.1073/pnas.1014465107
48. Soleymaninejadian E, Pramanik K, Samadian E. Immunomodulatory Properties of Mesenchymal Stem Cells: Cytokines and Factors. Am J Reprod Immunol (2012) 67(1):1–8. doi: 10.1111/j.1600-0897.2011.01069.x
49. Bulati M, Miceli V, Gallo A, Amico G, Carcione C, Pampalone M, et al. The Immunomodulatory Properties of the Human Amnion-Derived Mesenchymal Stromal/Stem Cells Are Induced by INF-γ Produced by Activated Lymphomonocytes and Are Mediated by Cell-to-Cell Contact and Soluble Factors. Front Immunol (2020) 11:2020.00054. doi: 10.3389/fimmu.2020.00054
50. Fan MY, Turka LA. Immunometabolism and PI (3) K Signaling as a Link Between IL-2, Foxp3 Expression, and Suppressor Function in Regulatory T Cells. Front Immunol (2018) 9:2018.00069. doi: 10.3389/fimmu.2018.00069
51. Park CW, Kim KS, Bae S, Son HK, Myung PK, Hong HJ, et al. Cytokine Secretion Profiling of Human Mesenchymal Stem Cells by Antibody Array. Int J Stem Cells (2009) 2(1):59. doi: 10.15283/ijsc.2009.2.1.59
52. Opal SM, Depalo VA. Anti-Inflammatory Cytokines. Chest (2000) 117(4):1162–72. doi: 10.1378/chest.117.4.1162
53. Kyurkchiev D, Bochev I, Ivanova-Todorova E, Mourdjeva M, Oreshkova T, Belemezova K, et al. Secretion of Immunoregulatory Cytokines by Mesenchymal Stem Cells. World J Stem Cells (2014) 6(5):552. doi: 10.4252/wjsc.v6.i5.552
54. Prasanna SJ, Gopalakrishnan D, Shankar SR, Vasandan AB. Pro-Inflammatory Cytokines, Ifnγ and Tnfα, Influence Immune Properties of Human Bone Marrow and Wharton Jelly Mesenchymal Stem Cells Differentially. PloS One (2010) 5(2):e9016. doi: 10.1371/journal.pone.0009016
55. DelaRosa O, Dalemans W, Lombardo E. Mesenchymal Stem Cells as Therapeutic Agents of Inflammatory and Autoimmune Diseases. Curr Opin Biotechnol (2012) 23(6):978–83. doi: 10.1016/j.copbio.2012.05.005
56. Liu X, Ren S, Qu X, Ge C, Cheng K, Zhao RCH. Mesenchymal Stem Cells Inhibit Th17 Cells Differentiation via IFN-γ-Mediated SOCS3 Activation. Immunol Res (2015) 61(3):219–29. doi: 10.1007/s12026-014-8612-2
57. Laurence A, Tato CM, Davidson TS, Kanno Y, Chen Z, Yao Z, et al. Interleukin-2 Signaling via STAT5 Constrains T Helper 17 Cell Generation. Immunity (2007) 26(3):371–81. doi: 10.1016/j.immuni.2007.02.009
58. Rafei M, Campeau PM, Aguilar-Mahecha A, Buchanan M, Williams P, Birman E, et al. Mesenchymal Stromal Cells Ameliorate Experimental Autoimmune Encephalomyelitis by Inhibiting CD4 Th17 T Cells in a CC Chemokine Ligand 2-Dependent Manner. J Immunol (2009) 182(10):5994–6002. doi: 10.4049/jimmunol.0803962
59. Andreeva E, Bobyleva P, Gornostaeva A, Buravkova L. Interaction of Multipotent Mesenchymal Stromal and Immune Cells: Bidirectional Effects. Cytotherapy (2017) 19(10):1152–66. doi: 10.1016/j.jcyt.2017.07.001
60. Steensberg A, Fischer CP, Keller C, Møller K, Pedersen BK. IL-6 Enhances Plasma IL-1ra, IL-10, and Cortisol in Humans. Am J Physiol Endocrinol Metab (2003) 285(2):E433–7. doi: 10.1152/ajpendo.00074.2003
61. García-González P, Ubilla-Olguín G, Catalán D, Schinnerling K, Aguillón JC. Tolerogenic Dendritic Cells for Reprogramming of Lymphocyte Responses in Autoimmune Diseases. Autoimmun Rev (2016) 15(11):1071–80. doi: 10.1016/j.autrev.2016.07.032
62. Jorgovanovic D, Song M, Wang L, Zhang Y. Roles of IFN-γ in Tumor Progression and Regression: A Review. Biomark Res (2020) 8(1):1–16. doi: 10.1186/s40364-020-00228-x
Keywords: type 1 diabetes, Immunomodulation, priming, Wharton’s jelly-derived mesenchymal stromal cells, regulatory T cells, tolerogenic dendritic cells
Citation: Mrahleh MA-, Matar S, Jafar H, Wehaibi S, Aslam N and Awidi A (2021) Human Wharton’s Jelly-Derived Mesenchymal Stromal Cells Primed by Tumor Necrosis Factor-α and Interferon-γ Modulate the Innate and Adaptive Immune Cells of Type 1 Diabetic Patients. Front. Immunol. 12:732549. doi: 10.3389/fimmu.2021.732549
Received: 29 June 2021; Accepted: 06 September 2021;
Published: 28 September 2021.
Edited by:
David Markusic, Purdue University Indianapolis, United StatesReviewed by:
Christian Behm, University Dental Clinic Vienna, AustriaMohamed Mostafa Kamal, The British University in Egypt, Egypt
Jennifer Michelle Cassano, Veterinary Medical Teaching Hospital, United States
Copyright © 2021 Mrahleh, Matar, Jafar, Wehaibi, Aslam and Awidi. This is an open-access article distributed under the terms of the Creative Commons Attribution License (CC BY). The use, distribution or reproduction in other forums is permitted, provided the original author(s) and the copyright owner(s) are credited and that the original publication in this journal is cited, in accordance with accepted academic practice. No use, distribution or reproduction is permitted which does not comply with these terms.
*Correspondence: Abdalla Awidi, aabbadi@ju.edu.jo