- 1Xiyuan Hospital, China Academy of Chinese Medical Sciences, Beijing, China
- 2Graduate School, Beijing University of Chinese Medicine, Beijing, China
- 3National Clinical Research Center for Chinese Medicine Cardiology, Xiyuan Hospital, China Academy of Chinese Medical Sciences, Beijing, China
Background: Pyroptosis is a new programmed cell death discovered in recent years. Pyroptosis plays an important role in various diseases. Nevertheless, there are few bibliometric analysis systematically studies this field. We aimed to visualize the research hotspots and trends of pyroptosis using a bibliometric analysis to help understand the future development of basic and clinical research.
Methods: The articles and reviews regarding pyroptosis were culled from Web of Science Core Collection. Countries, institutions, authors, references and keywords in this field were visually analyzed by using CtieSpace and VOSviewer software.
Results: A total of 2845 articles and reviews were included. The number of articles regarding pyroptosis significantly increased yearly. These publications mainly come from 70 countries led by China and the USA and 418 institutions. We identified 605 authors, among which Thirumaladevi Kanneganti had the most significant number of articles, and Shi JJ was co-cited most often. Frontiers in immunology was the journal with the most studies, and Nature was the most commonly cited journal. After analysis, the most common keywords are nod like receptor family pyrin domain containing 3 inflammasome, apoptosis, cell death, gasdermin D, mechanism, caspase-1, and others are current and developing areas of study.
Conclusion: Research on the pyroptosis is flourishing. Cooperation and exchanges between countries and institutions must be strengthened in the future. The related pathway mechanism of pyroptosis, the relationship between pyroptosis and other types of programmed cell deaths as well as the role of pyroptosis in various diseases have been the focus of current research and developmental trends in the future research.
Introduction
The term of “pyroptosis” was first proposed in 2001 by American scholars Cookson and Brennan (1), who discovered this pro-inflammatory programmed cell death (PCD) pattern dependent on caspase-1 in Salmonella-infected macrophages (2). Pyroptosis is characterized by the formation of plasma membrane pores and the release of inflammatory contents, accompanied by the occurrence of inflammatory responses (3). The assembly and activation of inflammasome plays a critical role in pyroptosis. It is currently believed that canonical inflammasome activation by caspase-1 as well as noncanonical inflammasome activation through caspase-4, 5, and 11 can both lead to pyroptosis. Importantly, a gasdermin family protein regarded as a executors of pyroptosis, gasdermin D (GSDMD) found in 2015, has been shown to be responsible for pore formation on the plasma membrane (4). Furthermore, when the integrity of the cell membrane is destroyed, a large amount of pro-inflammatory mediators, such as interleukin 1β (IL-1β), interleukin 18 (IL-18), are actively secreted out of the cell through the membrane pores. With the increase of intracellular osmotic pressure, water penetrates into the cell to cause cell swelling, lysis, and induce pyroptosis. After the release of pro-inflammatory mediators and the contents from the cells, the inflammatory cascade is initiated, which can amplify local and systemic inflammation (5).
Pyroptosis not only plays an important role in the body’s immune response and inflammatory response regulation, but also participates in the initiation and/or progression of various diseases including infectious diseases, nervous system diseases, and cardiovascular diseases (CVDs). As an important part of the innate immune system, pyroptosis controls inflammasome-dependent cytokine secretion and contributes to antimicrobial defense. Nevertheless, excessive or inappropriate pyroptosis can be harmful. Several clinical studies showed that inflammasome activation exacerbates immune dysregulation in coronavirus disease 2019 patients, eventually leading to severe diseases (6, 7). Inflammasome and pyroptosis are considered therapeutic targets for coronavirus disease 2019 (8). Pyroptosis is also thought to be involved in neurological diseases such as Parkinson’s disease (9), epilepsy (10) and ischemic stroke (11). A recent bibliometric analysis showed that the study of inflammasome/pyroptosis in the brain is a current and future research hotspot, and it was recommended to study the mechanism of mitochondrial molecules involved in the complex crosstalk of pyroptosis and regulated cell deaths in brain glial cells, which will promote the development of effective treatment strategies (12). In addition, CVD is a chronic condition associated with inflammation. The role of pyroptosis mediated by inflammasome activation in CVDs including atherosclerosis (13), ischemia-reperfusion injury (14), and myocardial infarction (15) has also been gradually confirmed. In general, in-depth study of pyroptosis will help to understand its role in the development and prognosis of related diseases, and provide new ideas for clinical prevention and treatment.
Bibliometrics was first proposed by American bibliographers in 1969. Bibliometrics refers to the discipline that applies mathematical and statistical methods to the study of books and other communication media (16). According to the characteristics of literature database and bibliometrics, bibliometrics can also conduct qualitative and quantitative evaluation of literature research trends. Bibliometrics can not only help scholars quickly grasp the research hotspots and development trends of a specific research field, but also evaluate the distribution of countries/regions, authors, and journals in the research field, laying a foundation for the direction and development of future research (17).
This study aims to explore the hotspots and development trends of pyroptosis in the past 20 years, and draw a map of scientific knowledge with CiteSpace and VOSviewer software, so as to provide new ideas for basic research and clinical prevention and treatment.
Materials And Methods
Data Collection
Data were extracted from Web of Science Core Collection and was downloaded within one day on June 14, 2021. The search formula was set to TS =(pyroptosis), and the date of the search were from June 14, 2001, to June 14, 2021. A total of 3,134 articles were retrieved, 289 irrelevant articles including meeting abstracts, editorial materials, corrections, letters, retractions and proceedings paper were excluded. A total of 2845 literatures were exported and the retrieved ones will be exported in the form of all records and references, saved as plain text files and stored in the format of download_txt (Figure 1).
Data Analysis
All valid data were collected in Web of Science Core Collection and imported to Microsoft Excel 2019, VOSviewer and CiteSpace for performing visual analysis.
VOSviewer is a program for building and viewing bibliometric maps. It can be used to build author or journal maps based on collaborative data, or to build keyword maps based on co-occurrence data. The project provides a viewer that allows for a comprehensive and detailed review of bibliometric maps. Different from the commonly used bibliometrics program, VOSviewer pays special attention to the graphic representation of bibliometrics, and it is particularly useful to display large bibliometrics in an easy-to-explain way. The main purpose of developing VOSviewer is to analyze the bibliometric network and build a visual network map, and finally realize a deep and comprehensive understanding of the structure and dynamic development of scientific research (18).
CiteSpace is a citation visualization analysis software that focuses on the analysis of the potential knowledge contained in the scientific literature and is gradually developed in the context of scientometrics and data visualization. The main goal of knowledge domain visualization is to detect and monitor the development of knowledge, which can present the organization, rule and distribution of scientific knowledge through visualization means (19). Knowledge map is a new field driven by information technology, which can intuitively understand the research hotspots and evolution process of various fields in the knowledge system, and predict the development trend of various fields. It is an effective method and means to analyze large-scale data.
We used Microsoft Office Excel 2019 to analyze the trend of the number of articles published in the year, and used CiteSpace and VOSviewer software to analyze the country/region and institutional distribution, author contributions, core journals, keywords and timeline viewer.
Results
The Trend of Publication Outputs
The number of articles published in each period reflects the development trend of research in this field. As is shown in Figure 2, the number of articles concerning pyroptosis increased year by year. From 2001 to 2012, publication outputs during this period are extremely low, and the research is still at a standstill. From 2012 to 2017, the amount of literatures have steadily increased, which demonstrates that the field of pyroptosis began to receive attention. From 2017 to 2020, the number of published articles has exploded, and publication outputs have reached 822 in 2020.
Distribution of Countries/Regions and Institutions
A total of 2845 articles were published from 70 different countries and 418 institutions. As can been seen from Table 1, the most significant number of publications came from China (1302, 45.76%) and USA (905, 31.81%), which are far more than five times higher than those of other countries. Research institutions with most number of publications are Chinese Acad Sci (67, 2.36%). Among the top 10 institutions, 70% of institutions belong to China. In addition, several countries and institutions, such as England (0.29), Sweden (1.10), Israel (0.40), Denmark (0.40) and Slovenia (0.39) demonstrated a high degree of centrality, as indicated by the purple circles in Figures 3 and 4. Each circle in the figure represents a country, and the size of the circle indicates the publication outputs by the country. The lines between the circles denote cooperation between countries, and the wider the lines, the closer the cooperation. A small number of countries and institutions showed active cooperations, such as England, Italy, Australia, France, Belgium and Switzerland, Israel, Portugal and Scotland, Chinese Acad Sci, Univ Massachusetts and Yale Univ. However, most countries and research institutions are scattered and lack stable and intensive cooperation and communication relations.
Authors and Co-Cited Authors
A total of 605 authors were involved in the publication of literature on pyroptosis. As shown in Table 2, Thirumaladevi Kanneganti had the highest number of published papers (50, 1.76%). Among the top 10 authors, we can see that Feng Shao (0.18) and Petr Broz (0.13) have high centralities, which shows that these two authors have strong influence on each other’s work as well as works from other groups. Each circle represents an author, the lines between the circles mean the connections between authors, and the connection network of different colors shows the cooperation cluster between different authors. Figure 5 shows that there is a certain cooperation network between different authors, such as Thirumaladevi Kanneganti, Mohamed Lamkanfi, Rajendra Karki, Si Ming Man, Sannula Kesavardhana, R K Subbarao M Alireddi and David E Place, Timothy R Billiar, Jie Zhang, Ben Lu, Haichao Wang, Rui Kang and Yiting Tang. Co-cited authors are two or more authors who are cited by another or more papers at the same time, and these two or more authors constitute co-cited relationship. Among 1145 co-cited authors, 10 of them have been cited more than 500 times(Table 2). Shi JJ (1127) was the most frequently cited author, followed by Kayagaki N (895).
Journals and Co-Cited Academic Journals
We performed a visual analysis of published journals using the VOSviewer software. We found that 2845 articles related to pyroptosis were published in 829 academic journals. The journal Frontiers in immunology (90, 3.16%) had the highest number of outputs, followed by Cell death & disease (73, 2.57%). Among the top 10 academic journals, the highest impact factor (IF) is Cell death and differentiation (15.828). Furthermore, it can be seen from Table 3 that 60% of journals belong to Q1. The influence of journals depends on the number of times they are co-cited, which reflects whether the journal has significant influence in a particular research field. Among the top 10 co-cited academic journals, five journals have been cited more than 5000 times. As shown in Table 3, the journals with the highest number of citations is Nature (11443), followed by Proceedings of the national academy of sciences of the United States of America (6283). According to the 2020 Journal citation reports (JCR), except for Journal of immunology, almost all the co-cited journals in the top 10 journals were located in Q1 region.
The dual-map overlay of journals demonstrated relationship distribution among journals, with citing journals on the left and cited journals on the right, and the colored paths between them suggesting the cited relationships. An orange path in Figure 6 indicates that the documents published in Molecular/Biology/Genetics journals are often cited by Molecular/Biology/Immunology journals.
Co-Cited References and References Burst
As a research method to measure the degree of relationship between articles, co-citation refers to that two or more articles are cited by one or more papers at the same time, and the two articles are considered to be a co-citation relationship. Among the 1190 co-cited references retrieved, Table 4 shows the ten most frequently cited references, of which Cleavage of GSDMD by inflammatory caspases determines pyroptotic cell death (4) is the most frequently cited (856). According to references with the strongest citation bursts, Figure 7 shows that the first burst of co-cited reference began in 2005. The majority of the co-cited references have been frequently cited in recent 10 years, which implies that the research related to pyroptosis may continue to explode in the future.
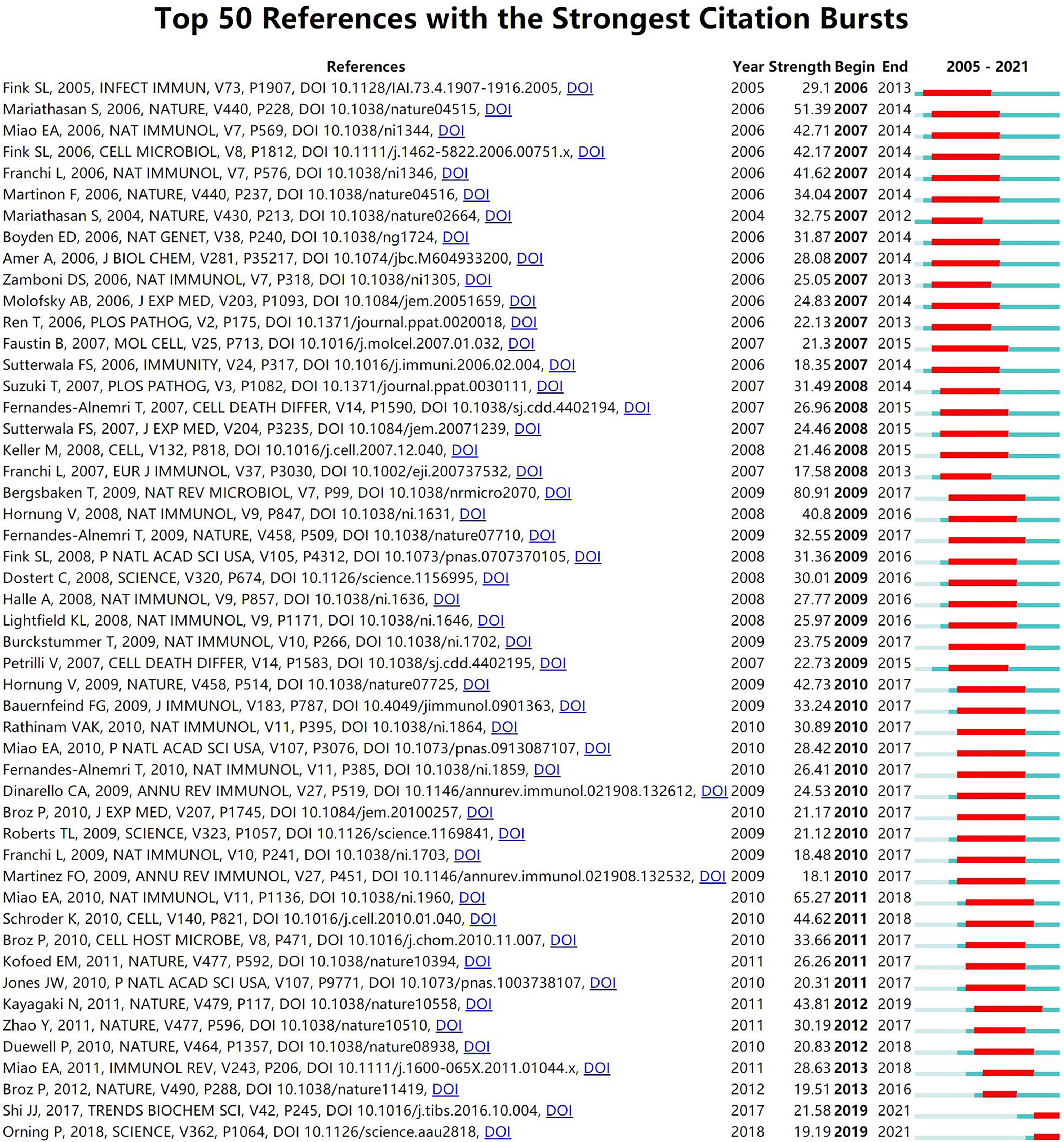
Figure 7 CiteSpace visualization map of top 50 references with the strongest citation bursts involved in pyroptosis.
The Analysis of Hotspots and Frontiers
Keywords are the core of a paper. By analyzing the keywords, we can summarize the study topics in a specific field and explore the hotspots and research directions. According to Table 5, excluding for pyroptosis (1459), the keywords appearing at high frequency in this study are nod like receptor family pyrin domain containing 3 (NLRP3) inflammasome (764), apoptosis (687), activation (662), cell death (608), and inflammasome (587). Among these keywords, NLRP3 inflammasome, apoptosis, activation, cell death and inflammasome appeared over 400 times, indicating that these fields were the hot topics in the study of pyroptosis.
Based on the analysis of keyword co-occurrence, the network map is clustered, which reflects the basic knowledge structure of related research fields. We use VOSviewer software to cluster keywords from literatures. Circles and labels form a unit, and units of different colors are made up for different clusters. As shown in Figure 8, we can see the clusters of red, green, blue and yellow, which respectively represent four different research directions. The main keywords of green cluster are pyroptosis, activation, mechanisms and pathway. The keywords of red cluster are cell death, inflammasome, caspase-1 and infection. While the keywords of blue cluster include GSDMD, cleavage and expression, and of yellow cluster mainly include apoptosis, autophagy, necrosis and ferroptosis.
Timeline viewer is based on the interaction and mutation relationship between keywords in a certain field, which is helpful for exploring the evolution track and stage characteristics of the research field. Figure 9 is the timeline viewer of focal death drawn based on CiteSpace software in this study, which visually shows the phased hotspots and development directions of the research on pyroptosis from the time dimension. From 2005 to 2011, the research mainly focuses on the functional analysis of inflammasomes and related mechanisms, and the main keywords are activation, cell death, ASC, NLRP3 inflammasome, innate immune response, toll like receptor, AIM2, tumor necrosis factor, inhibition and oxidative stress. From 2011 to 2021, the research is mainly based on the downstream pathway mechanism of pyroptosis, and the main keywords are caspase-11, GSDMD, human caspase-4, and mixed lineagekinase. It is worth noting that in the past 20 years, the study of pyroptosis has been closely related to various diseases (Figures 8 and 9), such as autoinflammatory diseases, atherosclerosis, ischemia-reperfusion injury, stroke, acute lung injury and brain injury.
Discussion
General Information
The annual number and trend of literatures can reflect the development speed and research progress of this study, and can also indicate the concentration of research in this field. It can be seen from Figure 2 that the overall number of publications is on the rise. Specifically, from 2001 to 2012, the relatively small amount of literature in this period indicates that the study of pyroptosis is in its infancy. From 2012 to 2017, the amount of literature showed a steady growth. During this period, the protein GSDMD, which is considered as the executor of pyroptosis, was proposed (4). GSDMD is a common substrate of inflammatory caspases, which not only clearly illustrates the molecular basis of pyroptosis, but also redefines pyroptosis as gasdermin-mediated programmed cell necrosis, opening up a new path for subsequent studies on programmed cell necrosis and innate immunity. From 2017 to 2020, research related to pyroptosis has exploded and the number of publications has reached 822 in 2020. It can be seen that the research related to pyroptosis is in the hot study direction in recent years, and has an excellent development trend in the future.
According to the distribution of countries/regions and institutions in Table 1, we can see that the country with the highest number of publications is China (1302, 45.76%), followed by the USA (905, 31.81%), which together account for 77.57% of the total. This indicates that China and the USA are the leading countries on pyroptosis research. Centrality is an index to measure the importance of a node in a network. It is mainly used to measure the value of the bridge function of the node in the entire network structure. Generally, nodes greater than 0.1 are considered as relatively important nodes. Among the top 10 countries in Table 1, England has the highest centrality (0.29), which means it plays a key role as a bridge in the worldwide network of state cooperation. Among the top ten research institutions, seven are from China and two are from the USA, with the Chinese Academy of Sciences (0.20) having the highest impact. Nevertheless, from Figures 3 and 4, the distribution of individual countries and institutions are scattered, and the main research subjects, such as China and USA, the Chinese Academy of Sciences and St Jude Children’s Research Hospital do not form a network, indicating a lack of academic exchange between countries as well as research institutions. This situation hinders the development of this research field. Therefore, it is strongly suggested that the US and China and research institutions from other countries should remove academic barriers, cooperate, and communicate to promote the research and development of pyroptosis.
From the perspective of authors and co-cited authors, Thirumaladevi Kanneganti (50, 1.76%) is the author with the highest number of publications, followed by Mohamed Lamkanfi (23, 0.81%), Petr Broz (22, 0.77%), Feng Shao (22, 0.77%), and Hao Wu (22, 0.77%). It is noteworthy that Feng Shao (0.18) exerts the significant greatest publication impact and have made the most outstanding contributions in the field of pyroptosis. Feng Shao, a biochemist, was elected as an academician of Chinese Academy of Sciences in 2015, mainly engaged in the molecular mechanism of pathogenic bacteria infection and innate immune defense of hosts. In 2015, Feng Shao and his colleagues identified that GSDMD is a common substrate for caspase-1 and caspase-4/5/11, and is a key effector molecule for mediating inflammatory cell death (4). This discovery not only reveals the mechanism and function of GSDMD, which may provide a new way for the treatment of inflammation-caspase-related autoimmune state and septic shock, but also redefines the concept that pyroptosis is programmed necrosis mediated by gasdermin. Recently, Feng Shao and Zhibo Liu revealed the anti-tumor immune function of pyroptosis by constructing biorthogonal system, and found that gasdermin agonist could improve the efficacy of cancer immunotherapy. The effect on immune-dependent anticancer chemotherapy drugs may be due to the activation of gasdermin (27). This indicates that the research on the relationship between pyroptosis and diseases has gradually become a hot topic. As for co-cited authors, the articles of 8 authors were cited more than 500 times, with Shi JJ (1127) having the highest number of citations, followed by Kayagaki N (895), and Miao Ea (717).
According to the journals and co-cited journals in Table 3, the journals with the most articles about pyroptosis were Frontiers in immunology (90, 3.16%), followed by Cell death & disease (73, 2.57%), Journal of immunology (59, 2.07%). It can be seen that the research on the mechanism of pyroptosis in immunity and diseases is a hot topic at present and also a development trend in the future. As for co-cited journals, Nature (11443) is a journal with highest citations, followed by Proceedings of the national academy of sciences of the United States of America (6283), Journal of immunology (6274), Cell (5503), and Journal of biological chemistry (5062). As is shown in Table 3, five journals cited more than 5000 times. Among the top ten journals, nine belong to Q1. The analysis of the distribution of literature sources is helpful to find the core journals published in the research regarding pyroptosis. It can be seen that the cited literatures are all from high-impact journals, indicating that research on pyroptosis is highly valued in the global academic field.
Among top 10 co-cited references related to pyroptosis, the top five co-cited references are all about gasdermin proteins, especially GSDMD. According to top 50 references with the strongest citation bursts, it can be seen that the most cited reference in recent years is Pyroptosis: Gasdermin-Mediated Programmed Necrotic Cell Death, which indicates that the gasdermin family suggests a new area of research on pyroptosis function in immunity and disease. Nevertheless, the function of many gasdermins and their mechanism of activation remain to be proved.
The Hotspots and Frontiers
Keywords are the research themes and core contents of the literature. Based on keyword co-occurrence analysis, it is possible to understand the distribution and development of different research hotspots in a certain field. It can be seen from Table 5 that the keywords with high occurrence frequency are pyroptosis (1459), NLRP3 inflammasome (746), apoptosis (687), activation (662), cell death (608), inflammasome (587), and GSDMD (455). Cluster analysis was conducted on the basis of keywords, and finally four colors clusters were formed. Then, according to the timeline viewer analysis of clustering, we determine the research hotspots and development frontiers in the field of pyroptosis. The main contents are as follows:
The Pathway Mechanism of Pyroptosis
Pyroptosis is mainly mediated by inflammasomes to activate various caspases, including caspase-1. Inflammatory caspases can directly shear and activate GSDMD, mediate the formation of plasma membrane pores, and cause cell death. Meanwhile, with the release of a large number of pro-inflammatory factors such as IL-1β and IL-18, inflammatory cascade waterfalls are triggered (28, 29).
Inflammasomes, first proposed by the Tschopp team in 2002, play an important role in innate immunity (30). Inflammasomes are polyprotein complexes that consist of pattern recognition receptors(PRRs), the adapter protein apoptosis-associated speck-like protein containing caspase recruitment domain(ASC), and the effector molecule pro-caspase (31). PRRs can detect pathogen-associated molecular patterns(PAMPs) of unique microbial structures such as bacteria, or host-derived risk/damage associated molecular patterns(DAMPs) including high mobility group protein 1 (HMGB1), heat shock protein (HSP), S100 protein, ATP, and uric acid crystals to recruit and activate inflammatory caspases (32). To date, the inflammasomes associated with pyroptosis that have been studied extensively include NLRP1, NLRP3, NLRC4 (also known as IPAF) inflammasomes in the NLR family and AIM2 inflammasomes, of which NLRP3 is the inflammasome that has been studied most concretely and its mechanism is relatively well understood. NLRP3 protein has three basic domains, including the C-terminal leucine-rich repeat(LRR) domain, which has the function of ligand recognition; the nucleotide-binding and oligomerization domain(NACHT) in the central region, which is important for the oligomerization and activation of the receptor itself; and the N-terminal pyrin domain(PYD), which binds to ASC through PYD-PYD. ASC has the PYD and caspase recruitment domain (CARD), which connects with the upstream NLRP3 protein through PYD and the downstream pro-caspase-1 through CARD (33–35). Other subtypes in the NLR family have similar domains. A variety of pathogens and intracellular and extracellular stimuli are recognized by LRR. The NLRP3 protein structure changes, exposing the NATCH domain, and then polymerizes to form a highly ordered NLRP3 protein oligomer, and recruits ASC through the PYD-PYD structure. ASC recruits pro-caspase-1 through the Card-Card domain to complete the assembly of inflammasomes. The assembled NLRP3 inflammasomes had the function of self-activation, leading to the hydrolysis of pro-caspase-1 to generate active caspase-1, and further promoting the maturation of IL-1β and IL-18 (36–41). However, there are also inflammasomes such as (NLRP1 and NLRC4) which do not need the adaptor protein ASC and whose receptor themself have CARDs that can be directly connected to pro-caspase-1 (42, 43). Additionally, AIM2 inflammasome is composed of AIM2, connective protein ASC and effector protein caspase-1. The AIM2 protein has a C-terminal HIN200 domain and an N-terminal PYD. After assembly, the AIM2 inflammasome can promote caspase-1 activation and the maturation of IL-1β and IL-18 (44, 45). According to our analysis, the mechanism of the relationship between other types of inflammasomes such as NLRP1 and NLRC4 and pyroptosis has also gradually attracted attention.
Caspase is a family of proteases with many members, and pyroptosis is mainly mediated by inflammatory caspase-1 (46–48). Recent studies have also shown that pyroptosis can also be mediated by human caspase-4 (49), caspase-5 (50) or mouse caspase-11 (51). Pro-caspase-1 was hydrolyzed by activated inflammasome to form active P10/P20 tetramer. Caspase-1, also known as interleukin-1β converting enzyme, can promote the cleavage and maturation of pro-IL-1β and pro-IL-18 to produce active IL-1β and IL-18, which are important pro-inflammatory cytokines involved in multitude of human diseases (52). In addition, caspase-1 can also directly shear and activate GSDMD protein, mediate the formation of plasma membrane pores and induce pyroptosis. This is the classic way of pyroptosis. While non-classical pathways are mainly mediated by human homologous genes caspase-4, caspase-5 or mouse caspase-11, which can be activated by various Gram-negative bacterial infections. Lipopolysaccharide(LPS) is the PAMP that promotes Caspase-11 activation. LPS in the cytoplasm can directly bind to and activate caspase-11 independently of toll-like receptor 4(TLR4). Caspase-11 can also trigger the activation of classical pathways and mature activation of pro-IL-1β through pyroptosis, which leads to the death of infected cells (20, 24, 53–55). Although there is no caspase-11 in human body, its homologous caspase-4 and caspase-5 have the same function and activation method as caspase-11. To date, non-classical pathways of pyroptosis have attracted more attention and become hot research directions (56–58).
In 2015, Feng Shao et al. identified GSDMD by genome-wide clustered regularly interspaced palindromic repeat (CRISPR)-Cas9 nuclease screens of caspase-11- and caspase-1-mediated pyroptosis in mouse bone marrow macrophages, and proposed that GSDMD is a central mediator of caspase-1 and caspase-4/5/11 downstream causing pyroptosis and is essential for IL-1β secretion (4). GSDMD is a member of the gasdermin protein family that also includes gasdermin A, B, C, D, E (also known as DFNA5) and DFNB59 (59, 61). GSDMD is generally found in the cytoplasm and is in an auto-inhibited state at steady state. When activated inflammatory caspases cleave the GSDMD protein, an N-terminal P30 fragment (GSDMD-NT) and a hydrophilic C-terminal P20 fragment (GSDMD-C) are formed. GSDMD-NT can specifically bind to the lipid bilayer of the cell membrane, forming small pores of 10-15nm in diameter, also known as gasdermin pores, which disrupt the cell membrane integrity, a large number of pro-inflammatory mediators IL-1β and IL-18 are actively secreted outside the cell, water enters the cell, the cell swells, lyses, and induces pyroptosis (21, 62). GSDMD-C also has an important role in inhibiting the cytotoxicity of GSDMD-N, promoting the water solubility of GSDMD-NT, and exerting a structural self-inhibitory effect when it binds to GSDMD-NT (49). Elucidating the structure of GSDMD pores, pore-forming mechanism and the means of inflammatory factor secretion have always been difficult points in the study of pyroptosis. Recently, Hao Wu’s team demonstrated the high-resolution structure of GSDMD pores, proposed the pore-forming mechanism of GSDMD, and revealed the molecular mechanism of IL -1 release through structural analysis and functional experiments. GSDMD contains 31 to 34 subunits, with the highest pore resolution of 33 subunits. Its transmembrane domain is a large barreled structure surrounded by 132 β strands, called the β-barrel. Next to the β-barrel there was a layer of globular domain on the cytosolic side. In addition, they also showed GSDMD prepore, which is globular and lacked β-barrel, and its conformation was quite different from that of GSDMD pores. The biological significance of GSDMD prepore is not clear, but the authors suggest that it may be an intermediate state during the pore assembly of GSDMD. In addition to the charge interaction between the α1 helix of GSDMD and lipid molecules on the cell membrane, GSDMD also has two charge-rich regions that are important for GSDMD to bind to the cell membrane. Surprisingly, the closest region of GSDMD prepore to the cell membrane is a hydrophobic loop structure called β1-β2 loop, which affects the pore formation efficiency of GSDMD. There was a large accumulation of negative charges in the pores of GSDMD, especially in the area near the cytoplasm. Interestingly, the IL-1 precursor surface charge was negative, while the mature IL-1 surface charge was positive. Based on these analyses, the authors proposed and validated the importance of charge for IL-1 release, which is another major progress related to pyroptosis (63). Petr Broz et al. found that when the cells execute pyroptosis, the calcium ion influx through the GSDMD hole could be used as a signal to start the membrane repair, and recruited the endosomal sorting complexes required for transport (ESCRT) machinery to the damaged membrane area. After activation of typical or atypical inflammasomes, inhibition of ESCRT-III can significantly increase the rate of pyroptosis. This study found a remedy mechanism in the process of endogenous pyroptosis, which provides a new idea for the treatment of inflammatory diseases (64). Recently, Wandel and colleagues found that guanylate-binding proteins(GBPs) assembled on the surfaces of Salmonella typhimurium and Shigella flexneri to form the multivalent signaling platform required for caspase-4 activation in interferon-gamma-stimulated cells. The assembly of platform, the recruitment of caspase-4 and the activation of caspase-4 are controlled by specific GBPs. In response to invading cytoplasmic bacteria, activation of caspase-4 via the GBP platform is critical for inducing GSDMD-dependent pyroptosis and IL-18 processing, thereby destroying intracellular bacterial replication sites and alerting adjacent cells (65). This achievement has been an important finding in recent years, providing new ideas for the various activation mechanisms of the gasdermin family. Additionally, studies reported that other types of gasdermin proteins can also induce pyroptosis through other pathways. For example, Hu et al. have found that tumor necrosis factor α+ cycloheximide and navitoclax-induced cancer cell pyroptosis through a BAK/BAX-caspase-3-GSDME signaling pathway. GSDME knockdown inhibited the pyroptosis, indicating the essential role of GSDME in this process (66). Our study also suggests that other types of gasdermin proteins will receive increasing attention in the future.
Comparison and Crosstalk of Pyroptosis With Other Types of Cell Death
Cell death can be divided into two major categories: PCD and non-programmed cell death. PCD includes apoptosis (58–60), autophagy (67), pyroptosis, necroptosis (68), and ferroptosis (69), while non-programmed cell death includes necrosis.
PCD plays a crucial role in biological development, normal physiological activities and various diseases. Different types of PCD do not play a single role, and there are several evidences have shown that different PCD pathways are interconnected at multiple levels. A study has demonstrated that pyroptosis stimuli can activate apoptosis through a variety of mechanisms. On the contrary, apoptotic stimuli can avoid or even inactivate pyroptosis. Taabazuing and colleagues found that small-molecule inhibitors of serine peptidases DPP8 and DPP9 (DPP8/9) selectively induced caspase-1-dependent pyroptosis in monocytes and macrophages. However, in the absence of GSDMD, the active caspase-1 induced apoptosis by activating caspase-3/7, confirming that GSDMD was the only caspase-1 substrate that induced pyroptosis in cells. In addition, they also confirmed that caspase-3/7 and inflammatory caspase have different cleavage sites for GSDMD, and caspase3/7 can cleave to inactivate GSDMD, specifically blocking pyroptosis. Thus revealing the complex interactions between the pyroptosis and apoptosis pathways in innate immune cells (70). Another study also supports the existence of crosstalk between pyroptosis and apoptosis. Orning et al. identified that caspase-8-mediated cell death triggered by pathogens is involved in the inflammatory pathway of GSDMD. It was confirmed that caspase-8 activated GSDMD by hydrolysis after Yersinia infected macrophages, which triggered the process of pyroptosis (71). Sarhan et al. also supported that in the process of transforming growth factor beta-activated kinase 1 inhibition, the activation of caspase-8 led to the cleavage of GSDMD in mouse macrophages, leading to pyroptosis. When the absence of GSDMD delayed membrane rupture, the cell death morphology turned to apoptosis (72). Another recent study suggested that the activity of caspase-8 was the key switch between apoptosis, necroptosis and pyroptosis, and determined the fate of cells by inducing crosstalk between them (73). Enzyme-inactivated caspase-8 could not induce apoptosis, but it mainly induced lysis and death through necroptosis mechanism. Nevertheless, when necroptosis was blocked, inactive caspase-8 induced pyroptosis, leading to severe inflammatory reaction (74). These studies further demonstrated that there is no strict boundary between apoptotic caspase and inflammatory caspase in the regulation of downstream molecules. The pathogen and its secretion system components affect different signaling proteins simultaneously and may involve multiple pathways of cell death pathway.
Notably, crosstalk between different types of PCD has crucial implications for clinical applications as well. A recent study showed that GSDME can be cleaved by caspase-3, and the cleavage site and result are extremely similar to caspase-1 cleavage of GSDMD, and it forms and releases an N-terminal domain with pore-forming activity to induce cell pyroptosis. Additionally, caspase-3 also plays an important role in apoptosis. Due to epigenetic silencing mediated by DNA methylation, GSDME is not expressed in cancer cells but is highly expressed in many normal tissues. Therefore, after caspase-3 is activated by treatment with chemotherapy drugs, these normal cells will undergo GSDME activation and die through pyroptosis. Since cancer cells do not express GSDME, caspase-3 activation induces cancer cells to die in an apoptotic manner. However, GSDME-/- mice can be protected from a variety of side effects such as tissue damage and body weight loss caused by chemotherapy drugs, which indicates that traditional chemotherapy drugs have great toxic and side effects, which may be due to the pyroptosis of normal tissues caused by the drugs (26). In general, understanding the crosstalk between different types of PCDs will be helpful for clinical diagnosis and treatment.
Role of Pyroptosis in Diseases
When stimulated by exogenous pathogens or host-derived hazard signals, pyroptosis is responsible for cell lysis and the release of various pro-inflammatory cytokines, such as IL-1β and IL-18. Normally, moderate pyroptosis is conducive to the timely removal of infected cells. Nevertheless, excessive activation of pyroptosis leads to a large number of cell deaths and inflammatory cascade reactions, seriously damaging tissues and organs, and even leading to organ failure, which is closely related to the pathogenesis of certain diseases (75–79). A large number of studies have shown that pyroptosis is widely involved in the occurrence and progression of infectious diseases (80), metabolic diseases (81), tumors (82), nervous system diseases (83) and cardiovascular diseases (84). To date, pyroptosis is a highly regulated cell death process, and its regulation by drugs or other treatments may have a protective effect in various diseases (Table 6).
Pyroptosis is a double-edged sword. This contradiction is particularly significant in cancer and its treatment. Tumors can use a variety of strategies to avoid or limit the pathways of cell death. On the other hand, tumors can be killed in different ways (81, 82). This complex mechanism poses a challenge to clinical diagnosis and treatment. To date, a large number of studies have begun to focus on targeted therapy strategies centered on molecules in various links of the pyroptosis, such as NLRP3 inflammasome, caspase-1, and GSDMD. Although the role of the pyroptosis effector protein GSDMD has gradually emerged in recent years, many researchers have begun to look for inhibitors of GSDMD, hoping to block the process of pyroptosis (94, 95). In 2018, Rathkey et al. found necrosulfonamide, a chemical blocker of GSDMD, in a model of sepsis, likely through alkylating a key cysteine residue (Cys191in human or Cys192 in mouse), which destroyed the function of GSDMD-NT domain and pore formation (96). A recent study also showed that dithiamine, an FDA-approved drug for the treatment of alcohol addiction, inhibited the formation of membrane pores by covalently modifying Cys191/Cys192 in GSDMD (97). According to relevant studies (98, 99), Cys191/Cys 192 has been found to be the most effective site for the inhibition of GSDMD, followed by the inhibition of unknown sites of GSDMD-NT, and regulation of the cleavage between caspase and GSDMD. Additionally, several NLRP3 inflammasome inhibitors such as colchicine, OLT1177 and MCC950 have also been identified to inhibit the activity of ATPase and prevent the assembly and activation of NLRP3 inflammasome. Caspase-1 inhibitors, including VX-765, are also used in the treatment of CVDs and infectious diseases (100). Although VX-765 has a high tolerance for effectively reducing inflammatory reaction, its clinical effect is not optimistic, and clinical data show that its efficacy is lower than expected. In general, although a great deal of evidence shows the effectiveness of pyroptosis inhibitors in animal experiments, there is still a lack of large-scale clinical data to prove the safe dose and clinical efficacy of pyroptosis inhibitors.
Limitation
CiteSpace and VOSviewer software can not completely replace system retrieval, and there are still some limitations to be solved. First of all, the literature we obtained was from 2001 to 2021. However, with the continuous updating of the literature in WoSCC, the retrieval results of this study are somewhat different from the actual number of included literatures. Secondly, this study includes both articles and reviews, and the uneven quality of the collected literatures may reduce the credibility of the map analysis. Finally, several core keywords in the paper were not included entirely in the analysis, and the results may be affected by incomplete keyword extraction. However, the visualized analysis based on literature undoubtedly lays a foundation for scholars to quickly understand the research subjects, research hotspots and development trends in the field of pyroptosis.
Conclusion
The research on the mechanism of pyroptosis, the crosstalk between different types of PCDs, and the role of pyroptosis in diseases has important research value and broad application prospect. Using CiteSpace and VOSviewer software for visual analysis, the research on pyroptosis is generally on the rise yearly. Globally, China and the USA are the leading countries in this research. Among the research institutions, Chinese Academy of Sciences is the institution with the highest influence on achievements. Different countries and institutions need to strengthen cooperation and exchanges. Feng Shao is an outstanding contributor to the field of pyroptosis. Most of the articles concerning pyroptosis are cited from internationally influential journals, indicating that pyroptosis has received much attention. At present, the research on pyroptosis mainly focuses on its mechanism molecules, the crosstalk of different types of PCD and its role in diseases, which will also be the focus of future research.
Data Availability Statement
The original contributions presented in the study are included in the article/supplementary material. Further inquiries can be directed to the corresponding authors.
Author Contributions
DM and SL conceived the study. BY, BG, and LS collected the data. LZ, TW, and ZZ re-examined the data. QL and YF analyzed the data. DM wrote the manuscript. ZG, SL, and HX reviewed and revised the manuscript. All authors contributed to the article and approved the submitted version.
Funding
This study was supported by “National natural science foundation of China” (No.82004192) and “the Fundamental Research Funds for the Central public welfare research institutes” (ZZ14-YQ-004). National natural science foundation of China (No. 82074215); CACMS Innovation fund (CI2021A00917); National Clinical Research Center for Chinese Medicine Cardiology, Xiyuan Hospital, China Academy of Chinese Medical Sciences (CI2021A00920).
Conflict of Interest
The authors declare that the research was conducted in the absence of any commercial or financial relationships that could be construed as a potential conflict of interest.
Publisher’s Note
All claims expressed in this article are solely those of the authors and do not necessarily represent those of their affiliated organizations, or those of the publisher, the editors and the reviewers. Any product that may be evaluated in this article, or claim that may be made by its manufacturer, is not guaranteed or endorsed by the publisher.
Acknowledgments
The authors thank Xiyuan Hospital, China Academy of Chinese Medical Sciences, for their support of this work and the reviewers for allowing us to make improvements to the manuscript.
References
1. Cookson BT, Brennan MA. Pro-Inflammatory Programmed Cell Death. Trends Microbiol (2001) 9:113–4. doi: 10.1016/S0966-842X(00)01936-3
2. Brennan MA, Cookson BT. Salmonella Induces Macrophage Death by Caspase-1-Dependent Necrosis. Mol Microbiol (2000) 38:31–40. doi: 10.1046/j.1365-2958.2000.02103.x
3. Liu X, Zhang Z, Ruan J, Pan Y, Magupalli VG, Wu H, et al. Inflammasome-Activated Gasdermin D Causes Pyroptosis by Forming Membrane Pores. Nature (2016) 535:153–8. doi: 10.1038/nature18629
4. Shi J, Zhao Y, Wang K, Shi X, Wang Y, Huang H, et al. Cleavage of GSDMD by Inflammatory Caspases Determines Pyroptotic Cell Death. Nature (2015) 526:660–5. doi: 10.1038/nature15514
5. He W, Wan H, Hu L, Chen P, Wang X, Huang Z, et al. Gasdermin D Is an Executor of Pyroptosis and Required for Interleukin-1β Secretion. Cell Res (2015) 25:1285–98. doi: 10.1038/cr.2015.139
6. Freeman TL, Swartz TH. Targeting the NLRP3 Inflammasome in Severe COVID-19. Front Immunol (2020) 11:1518. doi: 10.3389/fimmu.2020.01518
7. Kroemer A, Khan K, Plassmeyer M, Alpan O, Haseeb MA, Gupta R, et al. Inflammasome Activation and Pyroptosis in Lymphopenic Liver Patients With COVID-19. J Hepatol (2020) 73:1258–62. doi: 10.1016/j.jhep.2020.06.034
8. Yap JKY, Moriyama M, Iwasaki A. Inflammasomes and Pyroptosis as Therapeutic Targets for COVID-19. J Immunol (2020) 205:307–12. doi: 10.4049/jimmunol.2000513
9. Zhang M, He Q, Chen G, Li PA. Suppression of NLRP3 Inflammasome, Pyroptosis, and Cell Death by NIM811 in Rotenone-Exposed Cells as an In Vitro Model of Parkinson’s Disease. Neurodegener Dis (2021) 20:73–83. doi: 10.1159/000511207
10. Xia S, Yang P, Li F, Yu Q, Kuang W, Zhu Y, et al. Chaihu-Longgu-Muli Decoction Exerts an Antiepileptic Effect in Rats by Improving Pyroptosis in Hippocampal Neurons. J Ethnopharmacol (2021) 270:113794. doi: 10.1016/j.jep.2021.113794
11. Hou K, Li G, Yu J, Xu K, Wu W. Receptors, Channel Proteins, and Enzymes Involved in Microglia-Mediated Neuroinflammation and Treatments by Targeting Microglia in Ischemic Stroke. Neuroscience (2021) 460:167–80. doi: 10.1016/j.neuroscience.2021.02.018
12. Chen Y, Li Y, Guo L, Hong J, Zhao W, Hu X, et al. Bibliometric Analysis of the Inflammasome and Pyroptosis in Brain. Front Pharmacol (2020) 11:626502. doi: 10.3389/fphar.2020.626502
13. Wu P, Chen J, Chen J, Tao J, Wu S, Xu G, et al. Trimethylamine N-Oxide Promotes apoE-/- Mice Atherosclerosis by Inducing Vascular Endothelial Cell Py Roptosis via the SDHB/ROS Pathway. J Cell Physiol (2020) 235:6582–91. doi: 10.1002/jcp.29518
14. Shen S, He F, Cheng C, Xu B, Sheng J. Uric Acid Aggravates Myocardial Ischemia-Reperfusion Injury via ROS/NLRP3 Pyroptosis Pathway. BioMed \& Pharmacother (2021) 133:110990. doi: 10.1016/j.biopha.2020.110990
15. Lei Q, Yi T, Chen C. NF-Kappa B-Gasdermin D (GSDMD) Axis Couples Oxidative Stress and NACHT, LRR and PYD Domains-Containing Protein 3 (NLRP3) Inflammasome-Mediated Cardiomyocyte Pyroptosis Following Myocardial Infarction. Med Sci Monit (2018) 24:6044–52. doi: 10.12659/MSM.908529
16. Smith DR. Bibliometrics, Dermatology and Contact Dermatitis. Contact Dermatitis (2008) 59:133–6. doi: 10.1111/j.1600-0536.2008.01405.x
17. Ma C, Su H, Li H. Global Research Trends on Prostate Diseases and Erectile Dysfunction: A Bibliometric and Visualized Study. Front Oncol (2021) 10:627891. doi: 10.3389/fonc.2020.627891
18. van Eck NJ, Waltman L. Software Survey: VOSviewer, a Computer Program for Bibliometric Mapping. Scientometrics (2010) 84:523–38. doi: 10.1007/s11192-009-0146-3
19. Chen C. Searching for Intellectual Turning Points: Progressive Knowledge Domain Visualization. Proc Natl Acad Sci USA (2004) 101:5303–10. doi: 10.1073/pnas.0307513100
20. Kayagaki N, Stowe IB, Lee BL, O’Rourke K, Anderson K, Warming S, et al. Caspase-11 Cleaves Gasdermin D for non-Canonical Inflammasome Signalling. Nature (2015) 526:666–71. doi: 10.1038/nature15541
21. Ding J, Wang K, Liu W, She Y, Sun Q, Shi J, et al. Pore-Forming Activity and Structural Autoinhibition of the Gasdermin Family. Nature (2016) 535:111–6. doi: 10.1038/nature18590
22. Shi J, Zhao Y, Wang Y, Gao W, Ding J, Li P, et al. Inflammatory Caspases Are Innate Immune Receptors for Intracellular LPS. Nature (2014) 514:187–92. doi: 10.1038/nature13683
23. Shi J, Gao W, Shao F. Pyroptosis: Gasdermin-Mediated Programmed Necrotic Cell Death. Trends Biochem Sci (2017) 42:245–54. doi: 10.1016/j.tibs.2016.10.004
24. Kayagaki N, Warming S, Lamkanfi M, Vande WL, Louie S, Dong J. Non-Canonical Inflammasome Activation Targets Caspase-11. Nature (2011) 479:117–21. doi: 10.1038/nature10558
25. Lamkanfi M, Dixit VM. Mechanisms and Functions of Inflammasomes. Cell (2014) 157:1013–22. doi: 10.1016/j.cell.2014.04.007
26. Wang Y, Gao W, Shi X, Ding J, Liu W, He H, et al. Chemotherapy Drugs Induce Pyroptosis Through Caspase-3 Cleavage of a Gasdermin. Nature (2017) 547:99–103. doi: 10.1038/nature22393
27. Wang Q, Wang Y, Ding J, Wang C, Zhou X, Gao W, et al. A Bioorthogonal System Reveals Antitumour Immune Function of Pyroptosis. Nature (2020) 579:421–6. doi: 10.1038/s41586-020-2079-1
28. Aglietti RA, Dueber EC. Recent Insights Into the Molecular Mechanisms Underlying Pyroptosis and Gasdermin Family Functions. Trends Immunol (2017) 38:261–71. doi: 10.1016/j.it.2017.01.003
29. Kepp O, Galluzzi L, Zitvogel L, Kroemer G. Pyroptosis - a Cell Death Modality of its Kind? Eur J Immunol (2010) 40:627–30. doi: 10.1002/eji.200940160
30. Martinon F, Burns K, Boveresses C, Epalinges C. The Inflammasome: A Molecular Platform Triggering Activation of Inflammatory Caspases and Processing of proIL-β. Mol Cell (2002) 10:417–26. doi: 10.1016/s1097-2765(02)00599-3
31. Yao X, Zhang C, Xing Y, Xue G, Zhang Q, Pan F, et al. Remodelling of the Gut Microbiota by Hyperactive NLRP3 Induces Regulatory T Cells to Maintain Homeostasis. Nat Commun (2017) 8:1896. doi: 10.1038/s41467-017-01917-2
32. Takeuchi O, Akira S. Pattern Recognition Receptors and Inflammation. Cell (2010) 140:805–20. doi: 10.1016/j.cell.2010.01.022
33. Sharma BR, Kanneganti T-D. NLRP3 Inflammasome in Cancer and Metabolic Diseases. Nat Immunol (2021) 22:550–9. doi: 10.1038/s41590-021-00886-5
34. Swanson KV, Deng M, Ting JP-Y. The NLRP3 Inflammasome: Molecular Activation and Regulation to Therapeutics. Nat Rev Immunol (2019) 19:477–89. doi: 10.1038/s41577-019-0165-0.The
35. Mangan MSJ, Olhava EJ, Roush WR, Seidel HM, Glick GD, Latz E. Targeting the NLRP3 Inflammasome in Inflammatory Diseases. Nat Rev Drug Discovery (2018) 17:588–606. doi: 10.1038/nrd.2018.97
36. Duncan JA, Bergstralh DT, Wang Y, Willingham SB, Ye Z, Zimmermann AG, et al. Cryopyrin/NALP3 Binds ATP/dATP, Is an ATPase, and Requires ATP Binding to Mediate Inflammatory Signaling. Proc Natl Acad Sci USA (2007) 104:8041–6. doi: 10.1073/pnas.0611496104
37. He Y, Hara H, Núñez G. Mechanism and Regulation of NLRP3 Inflammasome Activation. Trends Biochem Sci (2016) 41:1012–21. doi: 10.1016/j.tibs.2016.09.002
38. Place DE, Kanneganti T-D. Recent Advances in Inflammasome Biology. Curr Opin Immunol (2018) 50:32–8. doi: 10.1016/j.coi.2017.10.011
39. Groslambert M, Py BF. Spotlight on the NLRP3 Inflammasome Pathway. J Inflammation Res (2018) 11:359–74. doi: 10.2147/JIR.S141220
40. Cai X, Chen J, Xu H, Liu S, Jiang QX, Halfmann R, et al. Prion-Like Polymerization Underlies Signal Transduction in Antiviral Immune Defense and Inflammasome Activation. Cell (2014) 156:1207–22. doi: 10.1016/j.cell.2014.01.063
41. Ratajczak MZ, Adamiak M, Thapa A, Bujko K, Brzezniakiewicz-Janus K, Lenkiewicz AM. NLRP3 Inflammasome Couples Purinergic Signaling With Activation of the Complement Cascade for the Optimal Release of Cells From Bone Marrow. Leukemia (2019) 33:815–25. doi: 10.1038/s41375-019-0436-6
42. Mitchell PS, Sandstrom A, Vance RE. The NLRP1 Inflammasome: New Mechanistic Insights and Unresolved Mysteries. Curr Opin Immunol (2019) 60:37–45. doi: 10.1016/j.coi.2019.04.015
43. Duncan JA, Canna SW. The NLRC4 Inflammasome. Immunol Rev (2018) 281:115–23. doi: 10.1111/imr.12607
44. Wang B, Bhattacharya M, Roy S, Tian Y, Yin Q. Immunobiology and Structural Biology of AIM2 Inflammasome. Mol Aspects Med (2020) 76:100869. doi: 10.1016/j.mam.2020.100869
45. Wang B, Yin Q. AIM2 Inflammasome Activation and Regulation: A Structural Perspective. J Struct Biol (2017) 200:279–82. doi: 10.1016/j.jsb.2017.08.001
46. Winkler S, Rösen-Wolff A. Caspase-1: An Integral Regulator of Innate Immunity. Semin Immunopathol (2015) 37:419–27. doi: 10.1007/s00281-015-0494-4
47. Gan J, Huang M, Lan G, Liu L, Xu F. High Glucose Induces the Loss of Retinal Pericytes Partly via NLRP3-Caspase-1-GSDMD-Mediated Pyroptosis. BioMed Res Int (2020) 37:419–27. doi: 10.1155/2020/4510628
48. Jin Y, Li H, Xie G, Chen S, Wu S, Fang X. Sevoflurane Combined With ATP Activates Caspase-1 and Triggers Caspase-1-Dependent Pyroptosis in Murine J774 Macrophages. Inflammation (2013) 36:330–6. doi: 10.1007/s10753-012-9550-6
49. Qiao L, Wu X, Zhang J, Liu L, Sui X, Zhang R, et al. Alpha-NETA Induces Pyroptosis of Epithelial Ovarian Cancer Cells Through the GSDMD/Caspase-4 Pathway. FASEB J (2019) 33:12760–7. doi: 10.1096/fj.201900483RR
50. Baker PJ, Boucher D, Bierschenk D, Tebartz C, Whitney PG, D’Silva DB, et al. NLRP3 Inflammasome Activation Downstream of Cytoplasmic LPS Recognition by Both Caspase-4 and Caspase-5. Eur J Immunol (2015) 45:2918–26. doi: 10.1002/eji.201545655
51. Aglietti RA, Estevez A, Gupta A, Ramirez MG, Liu PS, Kayagaki N, et al. GsdmD P30 Elicited by Caspase-11 During Pyroptosis Forms Pores in Membranes. Proc Natl Acad Sci USA (2016) 113:7858–63. doi: 10.1073/pnas.1607769113
52. Cerretti DP, Kozlosky CJ, Mosley B, Nelson N, Van Ness K, Greenstreet TA, et al. Molecular Cloning of the Interleukin-1β Converting Enzyme. Science (1992) 256:97–100. doi: 10.1126/science.1373520
53. Ding J, Shao F. SnapShot: The Noncanonical Inflammasome. Cell (2017) 168:544–544.e1. doi: 10.1016/j.cell.2017.01.008
54. Yi Y-S. Caspase-11 non-Canonical Inflammasome: A Critical Sensor of Intracellular Lipopolysaccharide in Macro Phage-Mediated Inflammatory Responses. Immunology (2017) 152:207–17. doi: 10.1111/imm.12787
55. Qiu S, Liu J, Xing F. “Hints” in the Killer Protein Gasdermin D: Unveiling the Secrets of Gasdermins Driving Cell Death. Cell Death Differ (2017) 24:588–96. doi: 10.1038/cdd.2017.24
56. Yang J, Zhao Y, Shao F. Non-Canonical Activation of Inflammatory Caspases by Cytosolic LPS in Innate Immunity. Curr Opin Immunol (2015) 32:78–83. doi: 10.1016/j.coi.2015.01.007
57. Chen Q, Shi P, Wang Y, Zou D, Wu X, Wang D, et al. GSDMB Promotes non-Canonical Pyroptosis by Enhancing Caspase-4 Activity. J Mol Cell Biol (2019) 11:496–508. doi: 10.1093/jmcb/mjy056
58. Chen S, Jin P, Chen H, Wu D, Li S, Zhang Y, et al. Dual Function of a Turbot Inflammatory Caspase in Mediating Both Canonical and non-Canonical Inflammasome Activation. Dev Comp Immunol (2021) 121:104078. doi: 10.1016/j.dci.2021.104078
59. Katoh M, Katoh M. Identification and Characterization of Human DFNA5L, Mouse Dfna5l, and Rat Dfna5l Genes In Silico. Int J Oncol (2004) 25:765–70. doi: 10.3892/ijo.25.3.765
60. Kerr JFR, Wyllie AH, Currie AR. Apoptosis: A Basic Biological Phenomenon With Wide-Ranging Implications in Tissue Kinetics. Br J Cancer (1972) 26:239–57. doi: 10.1038/bjc.1972.33
61. Tamura M, Tanaka S, Fujii T, Aoki A, Komiyama H, Ezawa K, et al. Members of a Novel Gene Family, Gsdm, Are Expressed Exclusively in the Epithelium of the Skin and Gastrointestinal Tract in a Highly Tissue-Specific Manner. Genomics (2007) 89:618–29. doi: 10.1016/j.ygeno.2007.01.003
62. Broz P, Pelegrín P, Shao F. The Gasdermins, a Protein Family Executing Cell Death and Inflammation. Nat Rev Immunol (2020) 20:143–57. doi: 10.1038/s41577-019-0228-2
63. Xia S, Zhang Z, Magupalli VG, Pablo JL, Dong Y, Vora SM, et al. Gasdermin D Pore Structure Reveals Preferential Release of Mature Interleukin-1. Nature (2021) 593:607–11. doi: 10.1038/s41586-021-03478-3
64. Ruhl S, Shkarina K, Demarco B, Heilig R, Santos JC, Broz P. ESCRT-Dependent Membrane Repair Negatively Regulates Pyroptosis Downstream of GSDMD Activation. Science (2018) 362:956–60. doi: 10.1126/science.aar7607
65. Wandel MP, Kim B-H, Park E-S, Boyle KB, Nayak K, Lagrange B, et al. Guanylate-Binding Proteins Convert Cytosolic Bacteria Into Caspase-4 Signaling Platforms. Nat Immunol (2020) 21:880–91. doi: 10.1038/s41590-020-0697-2
66. Hu L, Chen M, Chen X, Zhao C, Fang Z, Wang H, et al. Chemotherapy-Induced Pyroptosis Is Mediated by BAK/BAX-Caspase-3-GSDME Pathway and Inhibited by 2-Bromopalmitate. Cell Death Dis (2020) 11:281. doi: 10.1038/s41419-020-2476-2
67. Mizushima N, Levine B. Autophagy in Human Diseases. N Engl J Med (2020) 383:1564–76. doi: 10.1056/nejmra2022774
68. Xia X, Lei L, Wang S, Hu J, Zhang G. Necroptosis and its Role in Infectious Diseases. Apoptosis (2020) 25:169–78. doi: 10.1007/s10495-019-01589-x
69. Jiang X, Stockwell BR, Conrad M. Ferroptosis: Mechanisms, Biology and Role in Disease. Nat Rev Mol Cell Biol (2021) 22:266–82. doi: 10.1038/s41580-020-00324-8
70. Taabazuing CY, Okondo MC, Bachovchin DA. Pyroptosis and Apoptosis Pathways Engage in Bidirectional Crosstalk in Monocytes and Macrophages. Cell Chem Biol (2017) 24:507–514.e4. doi: 10.1016/j.chembiol.2017.03.009
71. Orning P, Weng D, Starheim K, Ratner D, Best Z, Lee B, et al. Pathogen Blockade of TAK1 Triggers Caspase-8-Dependent Cleavage of Gasdermin D and Cell Death. Science (2018) 362:1064–9. doi: 10.1126/science.aau2818
72. Sarhan J, Liu BC, Muendlein HI, Li P, Nilson R, Tang AY, et al. Caspase-8 Induces Cleavage of Gasdermin D to Elicit Pyroptosis During Yersinia Infection. Proc Natl Acad Sci USA (2018) 115:E10888–97. doi: 10.1073/pnas.1809548115
73. Newton K, Wickliffe KE, Maltzman A, Dugger DL, Reja R, Zhang Y, et al. Activity of Caspase-8 Determines Plasticity Between Cell Death Pathways. Nature (2019) 575:679–82. doi: 10.1038/s41586-019-1752-8
74. Fritsch M, Günther SD, Schwarzer R, Albert M-C, Schorn F, Werthenbach JP, et al. Caspase-8 Is the Molecular Switch for Apoptosis, Necroptosis and Pyroptosis. Nature (2019) 575:683–7. doi: 10.1038/s41586-019-1770-6
75. Wang M, Chen X, Zhang Y. Biological Functions of Gasdermins in Cancer: From Molecular Mechanisms to Therapeutic Potential. Front Cell Dev Biol (2021) 9:638710. doi: 10.3389/fcell.2021.638710
76. Aluganti Narasimhulu C, Singla DK. Amelioration of Diabetes-Induced Inflammation Mediated Pyroptosis, Sarcopenia, and Adverse Muscle Rem Odelling by Bone Morphogenetic Protein-7. J Cachexia Sarcopenia Muscle (2021) 12:403–20. doi: 10.1002/jcsm.12662
77. Al Mamun A, Wu Y, Monalisa I, Jia C, Zhou K, Munir F, et al. Role of Pyroptosis in Spinal Cord Injury and its Therapeutic Implications. J Adv Res (2021) 28:97–109. doi: 10.1016/j.jare.2020.08.004
78. Yue R, Zheng Z, Luo Y, Wang X, Lv M, Qin D, et al. NLRP3-Mediated Pyroptosis Aggravates Pressure Overload-Induced Cardiac Hypertrophy, Fibrosis, and Dysfunction in Mice: Cardioprotective Role of Irisin. Cell Death Discovery (2021) 7:50. doi: 10.1038/s41420-021-00434-y
79. Sun Z, Chai Q, Zhang Z, Lu D, Meng Z, Wu W. Inhibition of SGLT1 Protects Against Glycemic Variability-Induced Cardiac Damage and Pyroptosis of Cardiomyocytes in Diabetic Mice. Life Sci (2021) 271:119116. doi: 10.1016/j.lfs.2021.119116
80. Place DE, Lee S, Kanneganti T-D. PANoptosis in Microbial Infection. Curr Opin Microbiol (2021) 59:42–9. doi: 10.1016/j.mib.2020.07.012
81. Anand PK. Lipids, Inflammasomes, Metabolism, and Disease. Immunol Rev (2020) 297:108–22. doi: 10.1111/imr.12891
82. Yu S, Zhao N, He M, Zhang K, Bi X. MiRNA-214 Promotes the Pyroptosis and Inhibits the Proliferation of Cervical Cancer Cells via Regulating the Expression of NLRP3. Cell Mol Biol (2020) 66:59–64. doi: 10.14715/cmb/2020.66.6.11
83. An J, Yang H, Yang E, Chung S, Kim D-Y, Jou I, et al. Dying Neurons Conduct Repair Processes in the Injured Brain Through Osteopontin Expression in Cooperation With Infiltrated Blood Monocytes. Glia (2021) 69:1037–52. doi: 10.1002/glia.23947
84. Wang Y, Liu X, Shi H, Yu Y, Yu Y, Li M, et al. NLRP3 Inflammasome, an Immune-Inflammatory Target in Pathogenesis and Treatment of Cardiovascular Diseases. Clin Transl Med (2020) 10:91–106. doi: 10.1002/ctm2.13
85. Zhang Y, Liu X, Bai X, Lin Y, Li Z, Fu J, et al. Melatonin Prevents Endothelial Cell Pyroptosis via Regulation of Long Noncoding RNA MEG3/miR-223/NLRP3 Axis. J Pineal Res (2018) 64:e12449. doi: 10.1111/jpi.12449. undefined.
86. Hage C, Hoves S, Strauss L, Bissinger S, Prinz Y, Poeschinger T, et al. Sorafenib Induces Pyroptosis in Macrophages and Triggers Natural Killer Cell-Mediated Cytotoxicity Against Hepatocellular Carcinoma. Hepatology (2019) 70:1280–97. doi: 10.1002/hep.30666
87. Heo MJ, Kim TH, You JS, Blaya D, Sancho-Bru P, Kim SG. Alcohol Dysregulates miR-148a in Hepatocytes Through FoxO1, Facilitating Pyroptosis via TXNIP Overexp Ression. Gut (2019) 68:708–20. doi: 10.1136/gutjnl-2017-315123
88. Chen A, Chen Z, Xia Y, Lu D, Yang X, Sun A, et al. Liraglutide Attenuates NLRP3 Inflammasome-Dependent Pyroptosis via Regulating SIRT1/ NOX4/ ROS Pathway in H9c2 Cells. Biochem Biophys Res Commun (2018) 499:267–72. doi: 10.1016/j.bbrc.2018.03.142
89. Li P, Zhong X, Li J, Liu H, Ma X, He R, et al. MicroRNA-30c-5p Inhibits NLRP3 Inflammasome-Mediated Endothelial Cell Pyroptosis Through FOX03 Down-Regulation in Atherosclerosis. Biochem Biophys Res Commun (2018) 503:2833–40. doi: 10.1016/j.bbrc.2018.08.049
90. Dubois H, Sorgeloos F, Sarvestani ST, Martens L, Saeys Y, Mackenzie JM, et al. Nlrp3 Inflammasome Activation and Gasdermin D-Driven Pyroptosis Are Immunopathogenic Upon Gastrointes Tinal Norovirus Infection. PloS Pathog (2019) 15:e1007709. doi: 10.1371/journal.ppat.1007709
91. Li Y, Guo X, Hu C, Du Y, Guo C, Zhao W, et al. Type I IFN Operates Pyroptosis and Necroptosis During Multidrug-Resistant A. Baumannii Infection. Cell Death Differ (2018) 25:1304–18. doi: 10.1038/s41418-017-0041-z
92. Rebe C, Derangere V, Ghiringhelli F. Induction of Pyroptosis in Colon Cancer Cells by LXR Beta. Mol Cell Oncol (2015) 2:e970094. doi: 10.4161/23723548.2014.970094
93. Li X, Zeng L, Cao C, Lu C, Lian W, Han J, et al. Long Noncoding RNA MALAT1 Regulates Renal Tubular Epithelial Pyroptosis by Modulated miR-23c Targeting of ELAVL1 in Diabetic Nephropathy. Exp Cell Res (2017) 350:327–35. doi: 10.1016/j.yexcr.2016.12.006
94. Yang J, Liu Z, Wang C, Yang R, Rathkey JK, Pinkard OW, et al. Mechanism of Gasdermin D Recognition by Inflammatory Caspases and Their Inhibition by a Gasdermin D-Derived Peptide Inhibitor. Proc Natl Acad Sci USA (2018) 115:6792–7. doi: 10.1073/pnas.1800562115
95. Pandeya A, Li L, Li Z, Wei Y. Gasdermin D (GSDMD) as a New Target for the Treatment of Infection. Medchemcomm (2019) 10:660–7. doi: 10.1039/c9md00059c
96. Rathkey JK, Zhao J, Liu Z, Chen Y, Yang J, Kondolf HC, et al. Chemical Disruption of the Pyroptotic Pore-Forming Protein Gasdermin D Inhibits Inflammatory Cell Death and Sepsis. Sci Immunol (2018) 3:eaat2738. doi: 10.1126/sciimmunol.aat2738
97. Hu JJ, Liu X, Xia S, Zhang Z, Zhang Y, Zhao J, et al. FDA-Approved Disulfiram Inhibits Pyroptosis by Blocking Gasdermin D Pore Formation. Nat Immunol (2020) 21:736–45. doi: 10.1038/s41590-020-0669-6
98. Cerella C, Teiten MH, Radogna F, Dicato M, Diederich M. From Nature to Bedside: Pro-Survival and Cell Death Mechanisms as Therapeutic Targets in Cancer Treatment. Biotechnol Adv (2014) 32:1111–22. doi: 10.1016/j.biotechadv.2014.03.006
99. Yu P, Zhang X, Liu N, Tang L, Peng C, Chen X. Pyroptosis: Mechanisms and Diseases. Signal Transduct Target Ther (2021) 6:128. doi: 10.1038/s41392-021-00507-5
Keywords: pyroptosis, CiteSpace, VOSviewer, programmed cell death, NLRP3, GSDMD, caspase
Citation: Ma D, Yang B, Guan B, Song L, Liu Q, Fan Y, Zhao L, Wang T, Zhang Z, Gao Z, Li S and Xu H (2021) A Bibliometric Analysis of Pyroptosis From 2001 to 2021. Front. Immunol. 12:731933. doi: 10.3389/fimmu.2021.731933
Received: 28 June 2021; Accepted: 03 August 2021;
Published: 18 August 2021.
Edited by:
Bart Tummers, St. Jude Children’s Research Hospital, United StatesReviewed by:
Alberto Baroja-Mazo, Biomedical Research Institute of Murcia (IMIB), SpainBeiyun Liu, St. Jude Children’s Research Hospital, United States
Copyright © 2021 Ma, Yang, Guan, Song, Liu, Fan, Zhao, Wang, Zhang, Gao, Li and Xu. This is an open-access article distributed under the terms of the Creative Commons Attribution License (CC BY). The use, distribution or reproduction in other forums is permitted, provided the original author(s) and the copyright owner(s) are credited and that the original publication in this journal is cited, in accordance with accepted academic practice. No use, distribution or reproduction is permitted which does not comply with these terms.
*Correspondence: Zhuye Gao, emh1eWVnYW9AMTI2LmNvbQ==; Siming Li, bWluZ3NpbGk5OUAxNjMuY29t; Hao Xu, eHVoYW90Y21AaG90bWFpbC5jb20=