- Department of Neurosurgery, Renmin Hospital of Wuhan University, Wuhan, China
Gliomas are the most common primary malignant tumor in adults’ central nervous system. While current research on glioma treatment is advancing rapidly, there is still no breakthrough in long-term treatment. Abnormalities in the immune regulatory mechanism in the tumor microenvironment are essential to tumor cell survival. The alteration of amino acid metabolism is considered a sign of tumor cells, significantly impacting tumor cells and immune regulation mechanisms in the tumor microenvironment. Despite the fact that the metabolism of tryptophan in tumors is currently discussed in the literature, we herein focused on reviewing the immune regulation of tryptophan metabolism in the tumor microenvironment of gliomas and analyzed possible immune targets. The objective is to identify potential targets for the treatment of glioma and improve the efficiency of immunotherapy.
Introduction
Gliomas are the most common primary malignant tumor among brain and other CNS tumors (24.1% of all tumors). Additionally, glioblastoma accounts for 60% of gliomas. Although standard treatment, including surgical resection, targeted radiation therapy, chemotherapy treatment, has significantly progressed (1), the median survival for glioblastoma for all patients (regardless of treatment) remained 14-15 months (2). Immunotherapy and tumor-treating fields (TTFields) (3, 4) have swiftly developed in recent years, but there is yet to be any breakthrough. Meanwhile, more and more investigations have revealed novel biomolecular insights that focus on the metabolism of cells to explore the malignant phenotype of gliomas (5, 6). Indeed, the tumor microenvironment of glioma is emerging as a critical regulator of cancer progression in the immune-suppressive aspect (7). These highly and complex netting metabolic pathways, which exist in both the tumor cell and the microenvironment, are surfacing throughout onco-metabolomics in gliomas.
Amino acid metabolism emerges as an essential role in the metabolic reprogramming of cancer cells. A previous study has constructed an amino acid-related risk signature for gliomas, which could predict patients’ survival and clinical features (8). Amino acids and their derivatives can not only regulate cancer cells but also modulate the surrounding microenvironment, which enhances malignancy and immunosuppression (9). For instance, Kynurenine, the catabolic product of tryptophan, induces the invasion of cancer cells and immunosuppression of the tumor microenvironment (10, 11) by binding to transcription factor aryl hydrocarbon receptor (12–14). Moreover, the activation of AHR hampers the performance of macrophages and T cells, which play an antitumoral role (10, 14). Thus, the metabolism of amino acids is diverse in tumors and has a crucial role not only in the biological process of tumor cells but also in the tumor microenvironment, particularly in the modulation of the immune system. These indicate that a better comprehension of amino acid metabolism will provide potentially efficient targets for glioma treatment (15, 16).
Herein, we focused on reviewing how the immune mechanism in the glioma tumor microenvironment is affected by tryptophan metabolism. The aim is to provide potential targets for the treatment of glioma and improve the efficacy of immunotherapy.
The Immune Microenvironment of Glioma
The brain tumor microenvironment is composed of tumor populations, interstitial cells and immune cells. The majority of immune cells in gliomas, including GBM, comprises a vast diversity of myeloid cells, which include bone marrow-derived macrophages (BMDMs), microglia, myeloid-derived suppressor cells (MDSCs), dendritic cells and neutrophils (17). Glioma-associated macrophages (GAMs) are the primary immune component of brain gliomas, which consisting of a mixture of bone marrow-derived macrophages (BMDMs) and resident macrophages called microglia (MG) (18). These cells are recruited into the glioma environment and can release a large number of growth factors and cytokines to influence tumor proliferation, migration and so on.
Recruitment of GAMs into the microenvironment is driven by multiple chemokines secreted by gliomas, including monocyte chemotactic protein (MCP)-1 (19), MCP-3 (20), TME motif chemokine 12(CXCL12) (21), colony-stimulating factor (CSF-1) (22), glial cell-derived neurotrophic factor (GDNF) and granulocyte-macrophage colony-stimulating factors (23). These factors are expressed differently in different glioma types and may guide the variations in immune infiltration. Studies have found that the degree of GAMs invasion is correlated with tumor grade (24, 25), and differences in GAMs were found in the microenvironment of gliomas with different isocitrate dehydrogenase 1/2 (IDH1/2) somatic mutation types (26). IDH1/2 mutation-induced methylation suppresses the recruitment of TAM and induces an immune response in gliomas. Compared to IDH wild type, patients with IDH mutations have lower immune infiltration and better prognosis. Glioblastoma can be classified into classical (CL), mesenchymal (MES), neural (N), and neurogenic (PN) tumors based on unique transcriptome characteristics (27). Compared with non-MES tumors, several cell types were increased in MES-GBM, including CD4+T cells, type 2 polarized macrophages, and neutrophils (28). MES-GBM was associated with vascular remodeling and immune cell aggregation, while CL/PN-GBM was linked to reduced immune cell infiltration and better prognosis (29).
Besides GAMs, several other immune cells have been identified in the glioma parenchyma, although the proportion is very low. In fact, T cells account for the majority of lymphocytes in glioma. CD8+ cytotoxic T cells are cellular immune effectors that are essential for killing tumor cells, but they are only sparsely distributed in the GBM parenchyma (30). These cells may not exert significant effector responses, and their function is impaired by immunosuppressive factors derived from myeloid cells (such as GAMs) (31). We speculate that a higher level of GAMs may hinder the infiltration of effector T cells. Moreover, regulatory T cells (Tregs) are also present in the glioma parenchyma. These cells actually possess immunosuppressive functions and are thought to inhibit anti-tumor immunity in various solid tumors such as ovarian, breast, and pancreatic cancers (32).
Glioma cells escape the immune system by inhibiting immune mechanisms in the microenvironment. Furthermore, glioma releases immunosuppressive and tumor-support factors to the microenvironment, resulting in proliferation, invasion, and immune escape (33).
Tryptophan Metabolism
Tryptophan (Trp) is an essential amino acid for animals and humans, obtained mainly from the diet. Protein in food is digested in the small intestine to release Trp, which is subsequently absorbed into the bloodstream through the intestine (34, 35). The most significant function of free Trp is its contribution to host protein synthesis (36). Besides, Trp and its metabolites perform a crucial roles in different physiological processes, as a protein- building molecule to maintain cell growth and, simultaneously coordinating the body’s response to the environment, as a neurotransmitter and signal molecule (37).
The activity of Trp metabolic pathways determines the level of free Trp. There are three pathways of tryptophan catabolism including (1): tryptamine is formed after decarboxylation by aromatic l-amino acid decarboxylase (AADC) (2); formation of 5-hydroxytryptamine (5-HT) by tryptophan hydroxylase (TPH) (3); kynurenine (Kyn) pathway (KP) is the most critical way of Trp degradation; more than 95% of free Trp is a substrate of the KP for Trp degradation (36, 38, 39). Interestingly, indoleamine-2,3-dioxygenase 1/2 (IDO1/IDO2) and tryptophan-2,3-dioxygenase (TDO) are major rate-limiting enzymes, which catalyze the oxidative cleavage of the indole ring of Trp in the KP (40). At the same time, kynurenase (KYNU), kynurenine aminotransferase (KATI KATIII), Kynurenine monooxygenase (KMO), and other enzymes participate in the KP and produce a variety of metabolites, including neuroactive and neurotoxic substances (anthranilic acid (AA) and quinolinic acid (QA)), which affects the growth and function of human cells (41) (42) (43) (Figure 1).
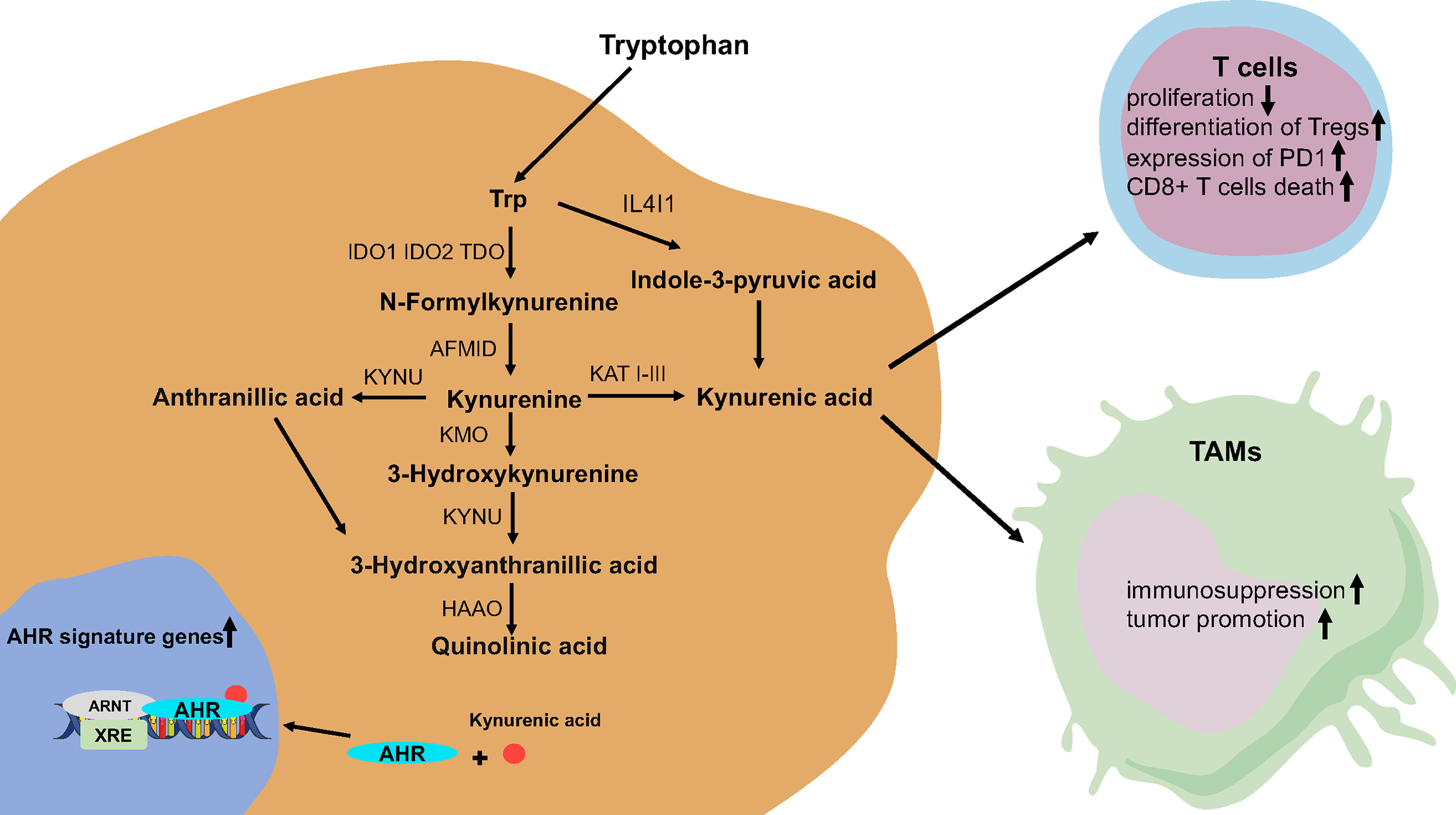
Figure 1 The tryptophan catabolic pathway in glioma. AFMID, kynurenine formamidase; AHR, aryl hydrocarbon receptor; HAAO, 3-hydroxyanthranilate 3,4-dioxygenase; IDO, indoleamine-2,3-dioxygenase; KATs, kynurenine amino transferases I–III; KMO, kynurenine 3-monooxygenase; KYNU, kynureninase; TDO, tryptophan-2,3-dioxygenase.
The Reprogramming of Tryptophan Metabolism in Glioma
Generally, the expression level of KP enzyme and metabolites in different cells and tissues is strictly regulated, but the abnormality of Trp metabolism and the accumulation of metabolites to varying degrees are involved in a wide range of disease processes, including tumors, neurodegenerative diseases, and self-immunity and mental illness, etc. Moreover, dysfunction of KP enzymes can trigger or facilitate diseases of CNS (41). For example, tryptophan tryptophan metabolites were found to accumulate in large amounts in the cerebrospinal fluid of glioma patients (44). Conversely, the enhanced activity of enzymes such as IDO, which are highly expressed in glioma cells, leads to the decrease of tryptophan and the accumulation of metabolites in the cells and microenvironment. Finally, these two promote the growth and invasion of gliomas, and meanwhile inhibit the anti-tumor immune response in the microenvironment (45).
Studies have revealed that changes in tryptophan metabolism can promote tumor development by enhancing the malignant characteristics of tumor cells and the immune suppression in the tumor microenvironment (46, 47). IDO1 is widely expressed in gliomas and is predictive of a poor prognosis in glioma patients. Likewise, it has also been established that TDO can promote tumor progression (47, 48). IDO1 and TDO, which are both positively related with the glioma grade, could promote the migration and invasion of glioma cells via the Kyn/AHR/APQ4 signaling pathway (49).The IDO1 expression level is elevated in glioma stem cells compared to GBM cells, and IDO1 leads to therapeutic resistance through the promotion of immunosuppression (50). More recently, interleukin-4-induced-1 (IL4I1) has been demonstrated to be more closely associated with AHR activity than IDO1 or TDO2 and is defined as the main Trp-catabolic enzymes in GBM (51). Furthermore, IL4I1 catabolizes tryptophan into indole-3-pyruvate (I3P) to inhibit ferroptosis by expressing an anti-oxidative gene expression program (52). Accumulation of the neurotoxic substance QA has also been found in gliomas. However, some studies revealed that in the glioma tumor microenvironment, QA is mainly produced in microglia rather than tumor cells (6, 53). The relationship between QA and the pathophysiological process of glioma warrants further studies. More importantly, the role of tryptophan and its metabolites in the immune microenvironment of glioma cannot be overlooked, and new immunotherapy targets may be discovered regarding its regulatory mechanisms (Figure 2).
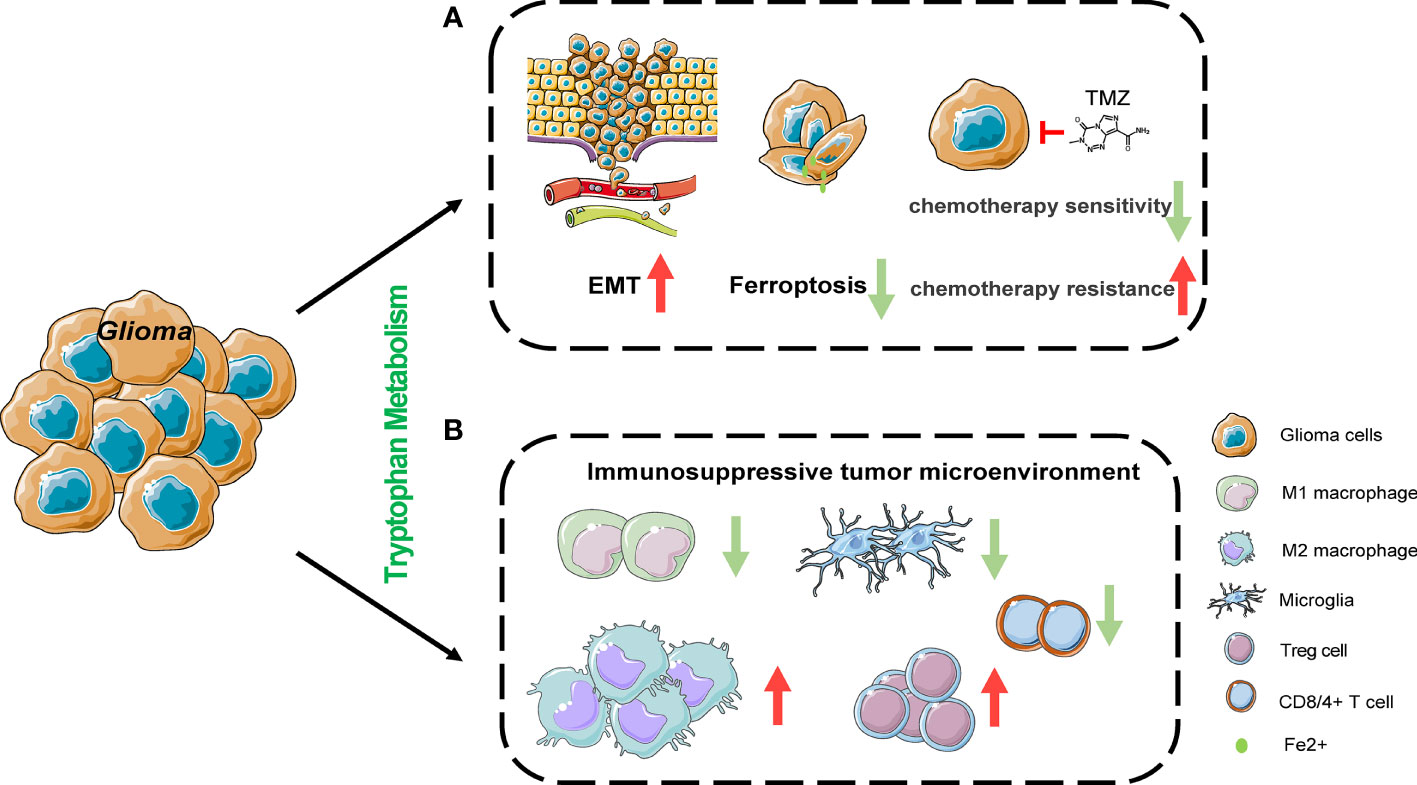
Figure 2 The effects of tryptophan metabolism in cancer cell and its microenvironment. (A) Tryptophan metabolism mediated by IDO1 and TDO could promote the migration and invasion of glioma cells via Kyn/AHR signaling pathway. IL4I1 catabolizes tryptophan into indole-3-pyruvate (I3P) to inhibit ferroptosis by expressing anti-oxidative gene expression program in Hela cells. IDO1 is induced as an undesired effect of chemotherapy in non-small-cell lung cancer and breast cancer. (B) Tryptophan metabolism suppresses T cell proliferation and function by inducing the differentiation of regulatory T cells (Tregs), the expression of programmed cell death protein 1 (PD1) on CD8+ T cells, cell death of CD8+ T cells and the recruitment of immunosuppressive tumor-associated macrophages. EMT, epithelial-mesenchymal transition.
The Effect of Tryptophan Metabolism on the Immune Microenvironment of Glioma
The Distribution of GAMs
Present-day research has found that GAMs control the onset, progression, metastasis, and response to treatment of glioma (54, 55). Enhancing the immunosuppression of the tumor microenvironment is considered a potential mechanism of GAMs to promote cancer. The phenotype M2-like macrophages of GAMs is associated with progression and immunosuppression in glioma (56). M1-type macrophages mainly utilize aerobic glycolysis during activation, which is associated with increased glucose uptake and the conversion of pyruvate to lactic acid. Nevertheless, the energy of M2-type macrophages is derived from fatty acid oxidative metabolism (57). The blockage of lipid metabolism may not only block M2 polarization, but also drive macrophages to return to the M1 state (58). The tryptophan metabolite Kyn produced by glioma cells participates in its function by activating the AHR in GAMs (59). The AHR-dependent transcription program regulates the recruitment and activation of GAMs in GBM. AHR can upregulate the expression of chemokine (C-C motif) receptor 2 (CCR2) of GAMs, and further drive GAM recruitment and aggregation (28). A study has found, in gliomas, AHR participates in the key pathways that regulate the polarization of macrophages in glioma by inhibiting NF-κB signaling and promotes the anti-inflammatory response driven by KLF4 (60).
At the same time, there may be multiple mechanisms regulating GAMs activation and infiltration in GBM. It was found that CSF1R signaling can alter the phenotype of GAMs (61). Moreover, the inactivation of NF1 in MES-GBM promotes the infiltration of GAMs. MES-GBM showed higher infiltration of GAMs than other subtypes, consistent with the high expression of CCL2 and CCR2 (62). Since the expression of AHR in MES-GBM is not higher than that in other subtypes, these findings indicate that AHR is one of several mechanisms in GBM that control GAMs recruitment and functions through mediators such as CCL2 and CCR2.
In conclusion, AHR activated during tryptophan metabolism participates in the mechanism of GBM controlling GAMs.
The Differentiation of Regulatory T Cells (Treg)
Treg is defined as a functional subset of suppressor T cells, mainly produced by the differentiation of CD4+ T cells induced by cytokines in vivo. Immunosuppression and immune incompetence are two crucial functional characteristics of Treg cells. The Trp-Kyn-AHR pathway is known to play a significant role in the differentiation process of Treg cells. The increased activity of rate-limiting enzymes such as IDO leads to the continuous consumption of tryptophan in the microenvironment. This relatively hungry environment causes the surrounding T cell cycle to stagnate, facilitating Treg production (63). Some studies also believe that the activation of the KP leads to the accumulation of Kyn in the microenvironment. Kyn activates AHR on CD4+ T cells through classic response genes such as Cyp1a1 and Cyp1b1, thereby inducing CD4+ T cells to differentiate into Tregs (44). Thus, Treg cells inhibit the function of effector T cells and regulate the body’s immunity by secreting inhibitory cytokines or interacting with antigen-presenting cells. The differentiation of IL-10 producing Tr1 cells can be promoted by AHR (64, 65) contribute to tumor-associated immunosuppression together with Foxp3+ Tregs (32).
A previous study noted that M2-type polarized microglia could induce Tregs production in vitro, highlighting the regulatory role of microglia in adaptive immune response and demonstrating the indirect regulatory role of AHR on Tregs (66).
T Cell Anergy or Death
Due to the increased expression and activity of rate-limiting enzymes such as IDO and TDO, and the activation of KP, the loss of tryptophan in the tumor microenvironment increases, which activates the amino acid starvation stress response, such as the expression of protein kinase GCN2 in surrounding cells (67). Protein kinase GCN2 is a vital regulator of the integrated stress response (ISR), regulating protein synthesis when amino acids are scarce. Studies have found that T cells with high GCN2 expression appear anergic and induce cell death (68). After the knockout of the GCN2 gene by antigen-presenting cells (APCs), autophagy decreases and amount of reactive oxygen species increase, which in turn activates the production of inflammasomes and IL-1β, leading to increased inflammation and helper T lymphocytes 17 (Th17) response. Therefore, local depletion of tryptophan is considered to be the principal “starvation and death” mechanism of immunosuppression. Other than the depletion of tryptophan, the activation of KP leads to the accumulation of tryptophan metabolites in the tumor microenvironment. These metabolites may individually or synergistically cause T cell anergy or death (14). The Trp-Kyn-AHR pathway executes an essential role in the regulation of T cell immunity in cancer. After a high level of Kyn is released into the tumor microenvironment, it is transferred to adjacent CD8+ T cells through transporters such as SLC7A8 and PAT4 (69). Besides, AHR is activated and increased by Kyn in T cells, thereby upregulating the expression level of PD-1, inhibiting T cell activity, and promoting immune tolerance. The expression of CD39 is promoted by AHR in GAMs, which promotes CD8+ T cell dysfunction by cooperating with CD73 to produce adenosine in the immune microenvironment of glioma (70). Apart from IDO and TDO, IL4I1, a secreted L-phenylalanine oxidase, has recently been defined as a novel immune checkpoint, playing a paramount role in Trp catabolism (71). IL4I1 promotes glioma cell migration via the Kyn/AHR pathway, and suppresses T cell proliferation in glioblastoma, which is associated with poor survival of glioma patients (51). Kyn can induce selective apoptosis of mouse thymocytes and Th1-cells, but cannot induce apoptosis of Th2-cells (72). GBM cells overexpress TDO2 to suppress T cell proliferation under standard oxygen conditions, however, the proliferation of the T cells could recover because of the reduction in kynurenine levels produced by the GBM cells (73). The immunomodulatory effect of Kyn on different lymphocyte subsets may be necessary for maintaining the homeostasis of peripheral lymphocytes and the accumulation of autologous lymphocytes.
GBM produces an angiogenic and inflammatory microenvironment that results in increased expression of adhesion molecules on endothelial cells and decreased tight junctions, leading to the formation of a highly permeable blood-brain barrier (BBB).These changes will enable more peripheral lymphocytes to participate in the immune microenvironment of the tumor. Nevertheless, GAMs remain the most essential part of the immune regulation of glioma.
The Regulation of Antigen-Presenting Cells (APCs) and Myeloid-Derived Suppressor Cells (MDSCs)
Studies have found that after activation of kyn, AHR can participate in regulating the function of dendritic cells (DC) and promote the differentiation of effector T cells and regulatory T cells (74). Moreover, AHR can induce DCs to produce kyn and retinoic acid (RA), and promote the differentiation of Tregs (75). Furthermore, AHR also inhibits the activation of NF-kB in DCs through a mechanism mediated by SOCS2 (76), thereby interfering with the production of cytokines that promote the differentiation of effector T cells.
The loss of the BBB integrity allows MDSCs to infiltration in GBM, and these cells actively inhibit immune responses through PDL1 and CTLA4 (77). MDSCs have been considered as a prime mediator in the tumor microenvironment, delivering a powerful immunosuppressive effect on T cells (78). IDO overexpressed in tumors recruits and activates MDSCs through a Treg-dependent mechanism to exert its immunosuppressive effect. At the same time, studies have found that IDO is highly induced in tumor-invasive MDSCs (78, 79). These results indicate that IDO has functional diversity in immune evasion related to MDSCs.
In addition, AHR can participate in the regulation of the activities of astrocytes and microglia, which play a substantial role in the immune activities of the tumor microenvironment (80). Therefore, the signaling pathways induced by KYN-AHR participate in the regulation of GAMs and other immune cells. Identifying of common cell-specific mechanisms, including tumor metabolites and other molecules in the tumor microenvironment, can guide therapies for GAMs and other immune cells.
Therapeutic Strategies for Immune Checkpoints
Recent researches demonstrate a series of therapy which set aberrant tryptophan metabolism as a target to improve the effects of glioma therapy. The Kyn/AHR pathway is considered as a significant factor in radiotherapy-induced immune checkpoint reactivation. Radiotherapy response can be enhanced by GDC-0919, known as IDO1 inhibitor, with the ability to pass through BBB and reduces radiotherapy-induced immunosuppression (81). 1-MT, an IDO1 inhibitor, added with two-fraction radiotherapy significantly reduced tumor size and increased survival in bears with GBM compared to untreated controls (82). These findings provide novel insights to enhance the effect of radiotherapy in glioblastoma. In chemotherapy, PCC0208009, a highly effective IDO inhibitor, combined with TMZ, enhanced the chemotherapy by promoting the immune response in vivo, including the percentages of CD3+, CD4+ and CD8+ up-regulated T cells. This result indicates that the combination of IDO inhibitor-based immunotherapy with chemotherapy is a potential strategy for brain tumor treatment (83). Lately, inhibitors that specifically target tryptophan metabolism have been involved in the clinical study of glioma. PF-06840003 is a highly effective IDO1 inhibitor with antitumor effects. Up to 500mg BID was generally well tolerated. Long-term clinical benefits can be gained by a subset of patients with recurrent malignant glioma (84). All these findings support the hypothesis that targeting Trp metabolism can consolidate immune therapy and provide a novel insight for antitumor treatment. At present, there are various researches on targeted drugs in the metabolism of tryptophan in gliomas, as shown in Table 1.
In the existing immune cell therapy, activating CD4+ and CD8+ T cells would increase the risk of autoimmune diseases if all Tregs are eliminated. Therefore, only part of Treg cells can be eliminated before the T cells are returned to the patient. The use of Treg cell-specific antibodies to eliminate the effect of Treg on immunosuppression is worthy of consideration.
Conclusions
The changes in tryptophan metabolism in glioma lead to a series of alterations in tumor cells and the surrounding tumor microenvironment. These metabolic variations may enable glioma to evade the immune system by affecting tumor cells and immune microenvironment and further promote tumor progression. Consequently, research on new metabolic, immune checkpoints can strengthen the effect of immunotherapy in the future. This will open up a new era of comprehensive treatment of glioma.
Author Contributions
YX and HZ wrote the article. QS and RG drew the figures and table. FY polished the language. BL and QC supervised the article. All authors contributed to the article and approved the submitted version.
Funding
This work was supported by the National Natural Science Foundation of China (No.82072764).
Conflict of Interest
The authors declare that the research was conducted in the absence of any commercial or financial relationships that could be construed as a potential conflict of interest.
Publisher’s Note
All claims expressed in this article are solely those of the authors and do not necessarily represent those of their affiliated organizations, or those of the publisher, the editors and the reviewers. Any product that may be evaluated in this article, or claim that may be made by its manufacturer, is not guaranteed or endorsed by the publisher.
Abbreviation
AADC, amino acid decarboxylase; DC, dendritic cell; IDO-1, Indoleamine 2,3-dioxygenase 1; IDO2, indoleamine 2,3-dioxygenase 2; TDO, tryptophan 2,3-dioxygenase; Trp, L-tryptophan; Kyn, L-kynurenine; QA, quinolinic acid; AHR, aryl hydrocarbon receptor; 5-HT, 5-hydroxytryptamine; GCN2, general control nonderepressible 2; GAM, Glioma-associated macrophage; GSCs, Glioma stem cells; LAT, large amino acid transport; GSH, glutathione; GS, glutamine synthetase; APC, antigen-presenting cell; MDSC, myeloid-derived suppressor cell.
References
1. Aldape K, Brindle KM, Chesler L, Chopra R, Gajjar A, Gilbert MR, et al. Challenges to Curing Primary Brain Tumours. Nat Rev Clin Oncol (2019) 16:509–20. doi: 10.1038/s41571-019-0177-5
2. Ostrom QT, Patil N, Cioffi G, Waite K, Kruchko C, Barnholtz-Sloan JS. CBTRUS Statistical Report: Primary Brain and Other Central Nervous System Tumors Diagnosed in the United States in 2013-2017. Neuro Oncol (2020) 22:iv1–iv96. doi: 10.1093/neuonc/noaa200
3. Stupp R, Taillibert S, Kanner A, Read W, Steinberg D, Lhermitte B, et al. Effect of Tumor-Treating Fields Plus Maintenance Temozolomide vs Maintenance Temozolomide Alone on Survival in Patients With Glioblastoma: A Randomized Clinical Trial. JAMA (2017) 318:2306–16. doi: 10.1001/jama.2017.18718
4. Sampson JH, Gunn MD, Fecci PE, Ashley DM. Brain Immunology and Immunotherapy in Brain Tumours. Nat Rev Cancer (2020) 20:12–25. doi: 10.1038/s41568-019-0224-7
5. Griffin JL, Kauppinen RA. A Metabolomics Perspective of Human Brain Tumours. FEBS J (2007) 274:1132–9. doi: 10.1111/j.1742-4658.2007.05676.x
6. Ahmed KA, Chinnaiyan P. Applying Metabolomics to Understand the Aggressive Phenotype and Identify Novel Therapeutic Targets in Glioblastoma. Metabolites (2014) 4:740–50. doi: 10.3390/metabo4030740
7. Quail DF, Joyce JA. The Microenvironmental Landscape of Brain Tumors. Cancer Cell (2017) 31:326–41. doi: 10.1016/j.ccell.2017.02.009
8. Liu Y-Q, Chai R-C, Wang Y-Z, Wang Z, Liu X, Wu F, et al. Amino Acid Metabolism-Related Gene Expression-Based Risk Signature can Better Predict Overall Survival for Glioma. Cancer Sci (2019) 110:321–33. doi: 10.1111/cas.13878
9. Sivanand S, Vander Heiden MG. Emerging Roles for Branched-Chain Amino Acid Metabolism in Cancer. Cancer Cell (2020) 37(2):147–56. doi: 10.1016/j.ccell.2019.12.011
10. Fallarino F, Grohmann U, Hwang KW, Orabona C, Vacca C, Bianchi R, et al. Modulation of Tryptophan Catabolism by Regulatory T Cells. Nat Immunol (2003) 4:1206–12. doi: 10.1038/ni1003
11. Greene LI, Bruno TC, Christenson JL, D’Alessandro A, Culp-Hill R, Torkko K, et al. A Role for Tryptophan-2,3-Dioxygenase in CD8 T-Cell Suppression and Evidence of Tryptophan Catabolism in Breast Cancer Patient Plasma. Mol Cancer Res (2019) 17:131–9. doi: 10.1158/1541-7786.MCR-18-0362
12. DiNatale BC, Murray IA, Schroeder JC, Flaveny CA, Lahoti TS, Laurenzana EM, et al. Kynurenic Acid Is a Potent Endogenous Aryl Hydrocarbon Receptor Ligand That Synergistically Induces Interleukin-6 in the Presence of Inflammatory Signaling. Toxicol Sci (2010) 115:89–97. doi: 10.1093/toxsci/kfq024
13. Nguyen NT, Kimura A, Nakahama T, Chinen I, Masuda K, Nohara K, et al. Aryl Hydrocarbon Receptor Negatively Regulates Dendritic Cell Immunogenicity via a Kynurenine-Dependent Mechanism. Proc Natl Acad Sci U.S.A. (2010) 107:19961–6. doi: 10.1073/pnas.1014465107
14. Opitz CA, Litzenburger UM, Sahm F, Ott M, Tritschler I, Trump S, et al. An Endogenous Tumour-Promoting Ligand of the Human Aryl Hydrocarbon Receptor. Nature (2011) 478:197–203. doi: 10.1038/nature10491
15. Commisso C, Davidson SM, Soydaner-Azeloglu RG, Parker SJ, Kamphorst JJ, Hackett S, et al. Macropinocytosis of Protein Is an Amino Acid Supply Route in Ras-Transformed Cells. Nature (2013) 497:633–7. doi: 10.1038/nature12138
16. Mayers JR, Torrence ME, Danai LV, Papagiannakopoulos T, Davidson SM, Bauer MR, et al. Tissue of Origin Dictates Branched-Chain Amino Acid Metabolism in Mutant Kras-Driven Cancers. Science (2016) 353:1161–5. doi: 10.1126/science.aaf5171
17. De Leo A, Ugolini A, Veglia F. Myeloid Cells in Glioblastoma Microenvironment. Cells (2020) 10:E18. doi: 10.3390/cells10010018
18. Roesch S, Rapp C, Dettling S, Herold-Mende C. When Immune Cells Turn Bad-Tumor-Associated Microglia/Macrophages in Glioma. Int J Mol Sci (2018) 19:E436. doi: 10.3390/ijms19020436
19. Platten M, Kretz A, Naumann U, Aulwurm S, Egashira K, Isenmann S, et al. Monocyte Chemoattractant Protein-1 Increases Microglial Infiltration and Aggressiveness of Gliomas. Ann Neurol (2003) 54:388–92. doi: 10.1002/ana.10679
20. Okada M, Saio M, Kito Y, Ohe N, Yano H, Yoshimura S, et al. Tumor-Associated Macrophage/Microglia Infiltration in Human Gliomas Is Correlated With MCP-3, But Not MCP-1. Int J Oncol (2009) 34:1621–7. doi: 10.3892/ijo_00000292
21. Feng X, Szulzewsky F, Yerevanian A, Chen Z, Heinzmann D, Rasmussen RD, et al. Loss of CX3CR1 Increases Accumulation of Inflammatory Monocytes and Promotes Gliomagenesis. Oncotarget (2015) 6:15077–94. doi: 10.18632/oncotarget.3730
22. Coniglio SJ, Eugenin E, Dobrenis K, Stanley ER, West BL, Symons MH, et al. Microglial Stimulation of Glioblastoma Invasion Involves Epidermal Growth Factor Receptor (EGFR) and Colony Stimulating Factor 1 Receptor (CSF-1R) Signaling. Mol Med (2012) 18:519–27. doi: 10.2119/molmed.2011.00217
23. Ku M-C, Wolf SA, Respondek D, Matyash V, Pohlmann A, Waiczies S, et al. GDNF Mediates Glioblastoma-Induced Microglia Attraction But Not Astrogliosis. Acta Neuropathol (2013) 125:609–20. doi: 10.1007/s00401-013-1079-8
24. Lu-Emerson C, Snuderl M, Kirkpatrick ND, Goveia J, Davidson C, Huang Y, et al. Increase in Tumor-Associated Macrophages After Antiangiogenic Therapy Is Associated With Poor Survival Among Patients With Recurrent Glioblastoma. Neuro Oncol (2013) 15:1079–87. doi: 10.1093/neuonc/not082
25. Chen X, Zhang L, Zhang IY, Liang J, Wang H, Ouyang M, et al. RAGE Expression in Tumor-Associated Macrophages Promotes Angiogenesis in Glioma. Cancer Res (2014) 74:7285–97. doi: 10.1158/0008-5472.CAN-14-1240
26. Huang Y, Kim BYS, Chan CK, Hahn SM, Weissman IL, Jiang W. Improving Immune-Vascular Crosstalk for Cancer Immunotherapy. Nat Rev Immunol (2018) 18:195–203. doi: 10.1038/nri.2017.145
27. Verhaak RGW, Hoadley KA, Purdom E, Wang V, Qi Y, Wilkerson MD, et al. Integrated Genomic Analysis Identifies Clinically Relevant Subtypes of Glioblastoma Characterized by Abnormalities in PDGFRA, IDH1, EGFR, and NF1. Cancer Cell (2010) 17:98–110. doi: 10.1016/j.ccr.2009.12.020
28. Wang Q, Hu B, Hu X, Kim H, Squatrito M, Scarpace L, et al. Tumor Evolution of Glioma-Intrinsic Gene Expression Subtypes Associates With Immunological Changes in the Microenvironment. Cancer Cell (2017) 32:42–56.e6. doi: 10.1016/j.ccell.2017.06.003
29. Martinez-Lage M, Lynch TM, Bi Y, Cocito C, Way GP, Pal S, et al. Immune Landscapes Associated With Different Glioblastoma Molecular Subtypes. Acta Neuropathol Commun (2019) 7:203. doi: 10.1186/s40478-019-0803-6
30. Han S, Ma E, Wang X, Yu C, Dong T, Zhan W, et al. Rescuing Defective Tumor-Infiltrating T-Cell Proliferation in Glioblastoma Patients. Oncol Lett (2016) 12:2924–9. doi: 10.3892/ol.2016.4944
31. Chongsathidkiet P, Jackson C, Koyama S, Loebel F, Cui X, Farber SH, et al. Sequestration of T Cells in Bone Marrow in the Setting of Glioblastoma and Other Intracranial Tumors. Nat Med (2018) 24:1459–68. doi: 10.1038/s41591-018-0135-2
32. Zou W. Regulatory T Cells, Tumour Immunity and Immunotherapy. Nat Rev Immunol (2006) 6:295–307. doi: 10.1038/nri1806
33. Al-Kharboosh R, ReFaey K, Lara-Velazquez M, Grewal SS, Imitola J, Quiñones-Hinojosa A. Inflammatory Mediators in Glioma Microenvironment Play a Dual Role in Gliomagenesis and Mesenchymal Stem Cell Homing: Implication for Cellular Therapy. Mayo Clin Proc Innov Qual Outcomes (2020) 4:443–59. doi: 10.1016/j.mayocpiqo.2020.04.006
34. Yu M, Mu C, Yang Y, Zhang C, Su Y, Huang Z, et al. Increases in Circulating Amino Acids With in-Feed Antibiotics Correlated With Gene Expression of Intestinal Amino Acid Transporters in Piglets. Amino Acids (2017) 49:1587–99. doi: 10.1007/s00726-017-2451-0
35. Mu C, Yang Y, Yu K, Yu M, Zhang C, Su Y, et al. Alteration of Metabolomic Markers of Amino-Acid Metabolism in Piglets With in-Feed Antibiotics. Amino Acids (2017) 49:771–81. doi: 10.1007/s00726-017-2379-4
36. Le Floc’h N, Otten W, Merlot E. Tryptophan Metabolism, From Nutrition to Potential Therapeutic Applications. Amino Acids (2011) 41:1195–205. doi: 10.1007/s00726-010-0752-7
37. Agus A, Planchais J, Sokol H. Gut Microbiota Regulation of Tryptophan Metabolism in Health and Disease. Cell Host Microbe (2018) 23:716–24. doi: 10.1016/j.chom.2018.05.003
38. Stone TW, Stoy N, Darlington LG. An Expanding Range of Targets for Kynurenine Metabolites of Tryptophan. Trends Pharmacol Sci (2013) 34:136–43. doi: 10.1016/j.tips.2012.09.006
39. van der Goot AT, Nollen EAA. Tryptophan Metabolism: Entering the Field of Aging and Age-Related Pathologies. Trends Mol Med (2013) 19:336–44. doi: 10.1016/j.molmed.2013.02.007
40. Cervenka I, Agudelo LZ, Ruas JL. Kynurenines: Tryptophan’s Metabolites in Exercise, Inflammation, and Mental Health. Science (2017) 357(6349):eaaf9794. doi: 10.1126/science.aaf9794
41. Schwarcz R, Stone TW. The Kynurenine Pathway and the Brain: Challenges, Controversies and Promises. Neuropharmacology (2017) 112:237–47. doi: 10.1016/j.neuropharm.2016.08.003
42. Guillemin GJ, Smythe G, Takikawa O, Brew BJ. Expression of Indoleamine 2,3-Dioxygenase and Production of Quinolinic Acid by Human Microglia, Astrocytes, and Neurons. Glia (2005) 49:15–23. doi: 10.1002/glia.20090
43. Munn DH, Mellor AL. IDO in the Tumor Microenvironment: Inflammation, Counter-Regulation, and Tolerance. Trends Immunol (2016) 37:193–207. doi: 10.1016/j.it.2016.01.002
44. Mezrich JD, Fechner JH, Zhang X, Johnson BP, Burlingham WJ, Bradfield CA. An Interaction Between Kynurenine and the Aryl Hydrocarbon Receptor can Generate Regulatory T Cells. J Immunol (2010) 185:3190–8. doi: 10.4049/jimmunol.0903670
45. Platten M, Weller M, Wick W. Shaping the Glioma Immune Microenvironment Through Tryptophan Metabolism. CNS Oncol (2012) 1:99–106. doi: 10.2217/cns.12.6
46. Muller AJ, DuHadaway JB, Donover PS, Sutanto-Ward E, Prendergast GC. Inhibition of Indoleamine 2,3-Dioxygenase, an Immunoregulatory Target of the Cancer Suppression Gene Bin1, Potentiates Cancer Chemotherapy. Nat Med (2005) 11:312–9. doi: 10.1038/nm1196
47. Pilotte L, Larrieu P, Stroobant V, Colau D, Dolusic E, Frédérick R, et al. Reversal of Tumoral Immune Resistance by Inhibition of Tryptophan 2,3-Dioxygenase. Proc Natl Acad Sci U.S.A. (2012) 109:2497–502. doi: 10.1073/pnas.1113873109
48. Théate I, van Baren N, Pilotte L, Moulin P, Larrieu P, Renauld J-C, et al. Extensive Profiling of the Expression of the Indoleamine 2,3-Dioxygenase 1 Protein in Normal and Tumoral Human Tissues. Cancer Immunol Res (2015) 3:161–72. doi: 10.1158/2326-6066.CIR-14-0137
49. Du L, Xing Z, Tao B, Li T, Yang D, Li W, et al. Both IDO1 and TDO Contribute to the Malignancy of Gliomas via the Kyn-AhR-AQP4 Signaling Pathway. Signal Transduct Target Ther (2020) 5:10. doi: 10.1038/s41392-019-0103-4
50. Ozawa Y, Yamamuro S, Sano E, Tatsuoka J, Hanashima Y, Yoshimura S, et al. Indoleamine 2,3-Dioxygenase 1 Is Highly Expressed in Glioma Stem Cells. Biochem Biophys Res Commun (2020) 524:723–9. doi: 10.1016/j.bbrc.2020.01.148
51. Sadik A, Somarribas Patterson LF, Öztürk S, Mohapatra SR, Panitz V, Secker PF, et al. IL4I1 Is a Metabolic Immune Checkpoint That Activates the AHR and Promotes Tumor Progression. Cell (2020) 182:1252–70.e34. doi: 10.1016/j.cell.2020.07.038
52. Zeitler L, Fiore A, Meyer C, Russier M, Zanella G, Suppmann S, et al. Anti-Ferroptotic Mechanism of IL4i1-Mediated Amino Acid Metabolism. Elife (2021) 10:e64806. doi: 10.7554/eLife.64806
53. Quintana FJ, Murugaiyan G, Farez MF, Mitsdoerffer M, Tukpah A-M, Burns EJ, et al. An Endogenous Aryl Hydrocarbon Receptor Ligand Acts on Dendritic Cells and T Cells to Suppress Experimental Autoimmune Encephalomyelitis. Proc Natl Acad Sci U.S.A. (2010) 107:20768–73. doi: 10.1073/pnas.1009201107
54. Junttila MR, de Sauvage FJ. Influence of Tumour Micro-Environment Heterogeneity on Therapeutic Response. Nature (2013) 501:346–54. doi: 10.1038/nature12626
55. Franklin RA, Liao W, Sarkar A, Kim MV, Bivona MR, Liu K, et al. The Cellular and Molecular Origin of Tumor-Associated Macrophages. Science (2014) 344:921–5. doi: 10.1126/science.1252510
56. Hambardzumyan D, Gutmann DH, Kettenmann H. The Role of Microglia and Macrophages in Glioma Maintenance and Progression. Nat Neurosci (2016) 19:20–7. doi: 10.1038/nn.4185
57. Rodríguez-Prados J-C, Través PG, Cuenca J, Rico D, Aragonés J, Martín-Sanz P, et al. Substrate Fate in Activated Macrophages: A Comparison Between Innate, Classic, and Alternative Activation. J Immunol (2010) 185:605–14. doi: 10.4049/jimmunol.0901698
58. Vats D, Mukundan L, Odegaard JI, Zhang L, Smith KL, Morel CR, et al. Oxidative Metabolism and PGC-1beta Attenuate Macrophage-Mediated Inflammation. Cell Metab (2006) 4:13–24. doi: 10.1016/j.cmet.2006.05.011
59. Gabriely G, Wheeler MA, Takenaka MC, Quintana FJ. Role of AHR and HIF-1α in Glioblastoma Metabolism. Trends Endocrinol Metab (2017) 28:428–36. doi: 10.1016/j.tem.2017.02.009
60. Nirschl CJ, Suárez-Fariñas M, Izar B, Prakadan S, Dannenfelser R, Tirosh I, et al. Ifnγ-Dependent Tissue-Immune Homeostasis Is Co-Opted in the Tumor Microenvironment. Cell (2017) 170:127–41.e15. doi: 10.1016/j.cell.2017.06.016
61. Pyonteck SM, Akkari L, Schuhmacher AJ, Bowman RL, Sevenich L, Quail DF, et al. CSF-1R Inhibition Alters Macrophage Polarization and Blocks Glioma Progression. Nat Med (2013) 19:1264–72. doi: 10.1038/nm.3337
62. Wang Q, Hu B, Hu X, Kim H, Squatrito M, Scarpace L, et al. Tumor Evolution of Glioma-Intrinsic Gene Expression Subtypes Associates With Immunological Changes in the Microenvironment. Cancer Cell (2018) 33:152. doi: 10.1016/j.ccell.2017.12.012
63. Fallarino F, Grohmann U, You S, McGrath BC, Cavener DR, Vacca C, et al. The Combined Effects of Tryptophan Starvation and Tryptophan Catabolites Down-Regulate T Cell Receptor Zeta-Chain and Induce a Regulatory Phenotype in Naive T Cells. J Immunol (2006) 176:6752–61. doi: 10.4049/jimmunol.176.11.6752
64. Apetoh L, Quintana FJ, Pot C, Joller N, Xiao S, Kumar D, et al. The Aryl Hydrocarbon Receptor Interacts With C-Maf to Promote the Differentiation of Type 1 Regulatory T Cells Induced by IL-27. Nat Immunol (2010) 11:854–61. doi: 10.1038/ni.1912
65. Mascanfroni ID, Takenaka MC, Yeste A, Patel B, Wu Y, Kenison JE, et al. Metabolic Control of Type 1 Regulatory T Cell Differentiation by AHR and HIF1-α. Nat Med (2015) 21:638–46. doi: 10.1038/nm.3868
66. Ebner F, Brandt C, Thiele P, Richter D, Schliesser U, Siffrin V, et al. Microglial Activation Milieu Controls Regulatory T Cell Responses. J Immunol (2013) 191:5594–602. doi: 10.4049/jimmunol.1203331
67. Dong J, Qiu H, Garcia-Barrio M, Anderson J, Hinnebusch AG. Uncharged tRNA Activates GCN2 by Displacing the Protein Kinase Moiety From a Bipartite tRNA-Binding Domain. Mol Cell (2000) 6:269–79. doi: 10.1016/s1097-2765(00)00028-9
68. Rashidi A, Miska J, Lee-Chang C, Kanojia D, Panek WK, Lopez-Rosas A, et al. GCN2 Is Essential for CD8+ T Cell Survival and Function in Murine Models of Malignant Glioma. Cancer Immunol Immunother (2020) 69:81–94. doi: 10.1007/s00262-019-02441-6
69. Liu Y, Liang X, Dong W, Fang Y, Lv J, Zhang T, et al. Tumor-Repopulating Cells Induce PD-1 Expression in CD8+ T Cells by Transferring Kynurenine and AhR Activation. Cancer Cell (2018) 33:480–94.e7. doi: 10.1016/j.ccell.2018.02.005
70. Takenaka MC, Gabriely G, Rothhammer V, Mascanfroni ID, Wheeler MA, Chao C-C, et al. Control of Tumor-Associated Macrophages and T Cells in Glioblastoma via AHR and CD39. Nat Neurosci (2019) 22:729–40. doi: 10.1038/s41593-019-0370-y
71. Molinier-Frenkel V, Prévost-Blondel A, Castellano F. The IL4I1 Enzyme: A New Player in the Immunosuppressive Tumor Microenvironment. Cells (2019) 8(7):757. doi: 10.3390/cells8070757
72. Fallarino F, Grohmann U, Vacca C, Bianchi R, Orabona C, Spreca A, et al. T Cell Apoptosis by Tryptophan Catabolism. Cell Death Differ (2002) 9:1069–77. doi: 10.1038/sj.cdd.4401073
73. Mohapatra SR, Sadik A, Tykocinski L-O, Dietze J, Poschet G, Heiland I, et al. Hypoxia Inducible Factor 1α Inhibits the Expression of Immunosuppressive Tryptophan-2,3-Dioxygenase in Glioblastoma. Front Immunol (2019) 10:2762. doi: 10.3389/fimmu.2019.02762
74. Quintana FJ, Sherr DH. Aryl Hydrocarbon Receptor Control of Adaptive Immunity. Pharmacol Rev (2013) 65:1148–61. doi: 10.1124/pr.113.007823
75. Yeste A, Takenaka MC, Mascanfroni ID, Nadeau M, Kenison JE, Patel B, et al. Tolerogenic Nanoparticles Inhibit T Cell-Mediated Autoimmunity Through SOCS2. Sci Signal (2016) 9:ra61. doi: 10.1126/scisignal.aad0612
76. Quintana FJ, Yeste A, Mascanfroni ID. Role and Therapeutic Value of Dendritic Cells in Central Nervous System Autoimmunity. Cell Death Differ (2015) 22:215–24. doi: 10.1038/cdd.2014.125
77. Pinton L, Masetto E, Vettore M, Solito S, Magri S, D’Andolfi M, et al. The Immune Suppressive Microenvironment of Human Gliomas Depends on the Accumulation of Bone Marrow-Derived Macrophages in the Center of the Lesion. J Immunother Cancer (2019) 7:58. doi: 10.1186/s40425-019-0536-x
78. Solito S, Pinton L, Damuzzo V, Mandruzzato S. Highlights on Molecular Mechanisms of MDSC-Mediated Immune Suppression: Paving the Way for New Working Hypotheses. Immunol Invest (2012) 41:722–37. doi: 10.3109/08820139.2012.678023
79. Yu J, Du W, Yan F, Wang Y, Li H, Cao S, et al. Myeloid-Derived Suppressor Cells Suppress Antitumor Immune Responses Through IDO Expression and Correlate With Lymph Node Metastasis in Patients With Breast Cancer. J Immunol (2013) 190:3783–97. doi: 10.4049/jimmunol.1201449
80. Schreiber RD, Old LJ, Smyth MJ. Cancer Immunoediting: Integrating Immunity’s Roles in Cancer Suppression and Promotion. Science (2011) 331:1565–70. doi: 10.1126/science.1203486
81. Kesarwani P, Prabhu A, Kant S, Kumar P, Graham SF, Buelow KL, et al. Tryptophan Metabolism Contributes to Radiation-Induced Immune Checkpoint Reactivation in Glioblastoma. Clin Cancer Res (2018) 24:3632–43. doi: 10.1158/1078-0432.CCR-18-0041
82. Ahlstedt J, Konradsson E, Ceberg C, Redebrandt HN. Increased Effect of Two-Fraction Radiotherapy in Conjunction With IDO1 Inhibition in Experimental Glioblastoma. PloS One (2020) 15:e0233617. doi: 10.1371/journal.pone.0233617
83. Sun S, Du G, Xue J, Ma J, Ge M, Wang H, et al. PCC0208009 Enhances the Anti-Tumor Effects of Temozolomide Through Direct Inhibition and Transcriptional Regulation of Indoleamine 2,3-Dioxygenase in Glioma Models. Int J Immunopathol Pharmacol (2018) 32:2058738418787991. doi: 10.1177/2058738418787991
Keywords: glioma, tryptophan, metabolism, immunomodulation, tumor microenvironment
Citation: Xu Y, Zhang H, Sun Q, Geng R, Yuan F, Liu B and Chen Q (2021) Immunomodulatory Effects of Tryptophan Metabolism in the Glioma Tumor Microenvironment. Front. Immunol. 12:730289. doi: 10.3389/fimmu.2021.730289
Received: 26 June 2021; Accepted: 20 September 2021;
Published: 01 October 2021.
Edited by:
Jinquan Cai, Harbin Medical University, ChinaCopyright © 2021 Xu, Zhang, Sun, Geng, Yuan, Liu and Chen. This is an open-access article distributed under the terms of the Creative Commons Attribution License (CC BY). The use, distribution or reproduction in other forums is permitted, provided the original author(s) and the copyright owner(s) are credited and that the original publication in this journal is cited, in accordance with accepted academic practice. No use, distribution or reproduction is permitted which does not comply with these terms.
*Correspondence: Qianxue Chen, Y2hlbnF4NjY2QHdodS5lZHUuY24=; Baohui Liu, YmxpdTY2NkB3aHUuZWR1LmNu
†These authors share first authorship