- 1GSK Vaccines Institute for Global Health (GVGH), Siena, Italy
- 2GSK, Research Centre, Siena, Italy
Nanoparticle systems are being explored for the display of carbohydrate antigens, characterized by multimeric presentation of glycan epitopes and special chemico-physical properties of nano-sized particles. Among them, outer membrane vesicles (OMVs) are receiving great attention, combining antigen presentation with the immunopotentiator effect of the Toll-like receptor agonists naturally present on these systems. In this context, we are testing Generalized Modules for Membrane Antigens (GMMA), OMVs naturally released from Gram-negative bacteria mutated to increase blebbing, as carrier for polysaccharides. Here, we investigated the impact of saccharide length, density, and attachment site on the immune response elicited by GMMA in animal models, using a variety of structurally diverse polysaccharides from different pathogens (i.e., Neisseria meningitidis serogroup A and C, Haemophilus influenzae type b, and streptococcus Group A Carbohydrate and Salmonella Typhi Vi). Anti-polysaccharide immune response was not affected by the number of saccharides per GMMA particle. However, lower saccharide loading can better preserve the immunogenicity of GMMA as antigen. In contrast, saccharide length needs to be optimized for each specific antigen. Interestingly, GMMA conjugates induced strong functional immune response even when the polysaccharides were linked to sugars on GMMA. We also verified that GMMA conjugates elicit a T-dependent humoral immune response to polysaccharides that is strictly dependent on the nature of the polysaccharide. The results obtained are important to design novel glycoconjugate vaccines using GMMA as carrier and support the development of multicomponent glycoconjugate vaccines where GMMA can play the dual role of carrier and antigen. In addition, this work provides significant insights into the mechanism of action of glycoconjugates.
Introduction
During the last years, nanoparticle systems have received increased interest for the display of carbohydrate antigens. Special physico-chemical properties of nano-sized particles and the presentation of multiple saccharide epitopes support the development of novel and more effective glycoconjugate vaccines (1–5). Among nanoparticles, outer membrane vesicles (OMVs) combine antigen presentation with intrinsic adjuvant properties (5–7). Traditionally, outer membrane protein complex (OMPC) from Neisseria meningitidis has been used as carrier for Haemophilus influenzae type b conjugate vaccine (8, 9). OMPC has been shown to possess TLR2-mediated adjuvant activity (10) and may contain TLR4 agonists such as lipopolysaccharides (LPS) since they derive from the outer membrane of Gram-negative bacteria.
More recently, Escherichia coli OMVs have been used as carriers for the display of heterologous polysaccharides (PS), resulting in glycoengineered OMVs (glyOMVs) (11). Streptococcus pneumoniae CPS14 capsule, for example, displayed on engineered E. coli OMVs induced IgG levels and efficacy in opsonophagocytic activity tests comparable with those induced by PCV13 (12).
Generalized Modules for Membrane Antigens (GMMA), OMVs naturally released from Gram-negative bacteria genetically manipulated to increase blebbing and modulate toxicity through modification of the lipid A portion of LPS (13, 14), have recently been proposed as delivery systems for O-antigen chains naturally present on their surface (15–19). O-antigens displayed on non-typhoidal Salmonella GMMA have been shown to induce high levels of anti-O-antigen-specific IgG antibodies, comparable with corresponding CRM197 conjugates formulated on alum (20). However, GMMA enhanced the IgG antibody isotype profile resulting in greater serum bactericidal activity than traditional protein conjugates. More recently, we have proposed GMMA as carrier for heterologous PS through chemical conjugation, and we have shown that GMMA glycoconjugates promote equal or enhanced saccharide immunogenicity as compared with more traditional glycoconjugates with CRM197 carrier protein (21).
It is well known that parameters such as saccharide length and density, conjugation chemistry, and attachment site can impact the immune response induced by glycoconjugate vaccines (22). Impact of such variables on the immune response elicited by OMV-based vaccines has not been greatly explored so far. Here, we have used different conjugation strategies to verify impact of saccharide length, density, and attachment site to proteins or LPS and lipooligosaccharide (LOS) molecules on GMMA surface on the immune response in animal models. Saccharide from different pathogens, having different structures, has been used as models and conjugated to GMMA from different pathogens.
Materials and Methods
Source of Generalized Modules for Membrane Antigens and Antigens
Salmonella Typhimurium GMMA (obtained from 1418 ΔtolR mutant strain) and meningococcal B (MenB) GMMA (produced from four knock-out ΔsynX, Δctra, Δgna33, and ΔlpxL1 N. meningitidis strains) were produced and characterized as previously described (15, 23). Meningococcal serogroup A (MenA), meningococcal serogroup C (MenC), and H. influenzae type b (Hib) oligosaccharides were provided by GSK. Vi and streptococcal Group A Carbohydrate (GAC) PS were purified as previously described (24–27). MenA and Vi PS of reduced length were generated as previously described (26, 28).
Synthesis and Characterization of the Generalized Modules for Membrane Antigens Conjugates
Conjugates were synthesized as described below. The main characteristics of all the conjugates tested in this study are reported in Table 1.
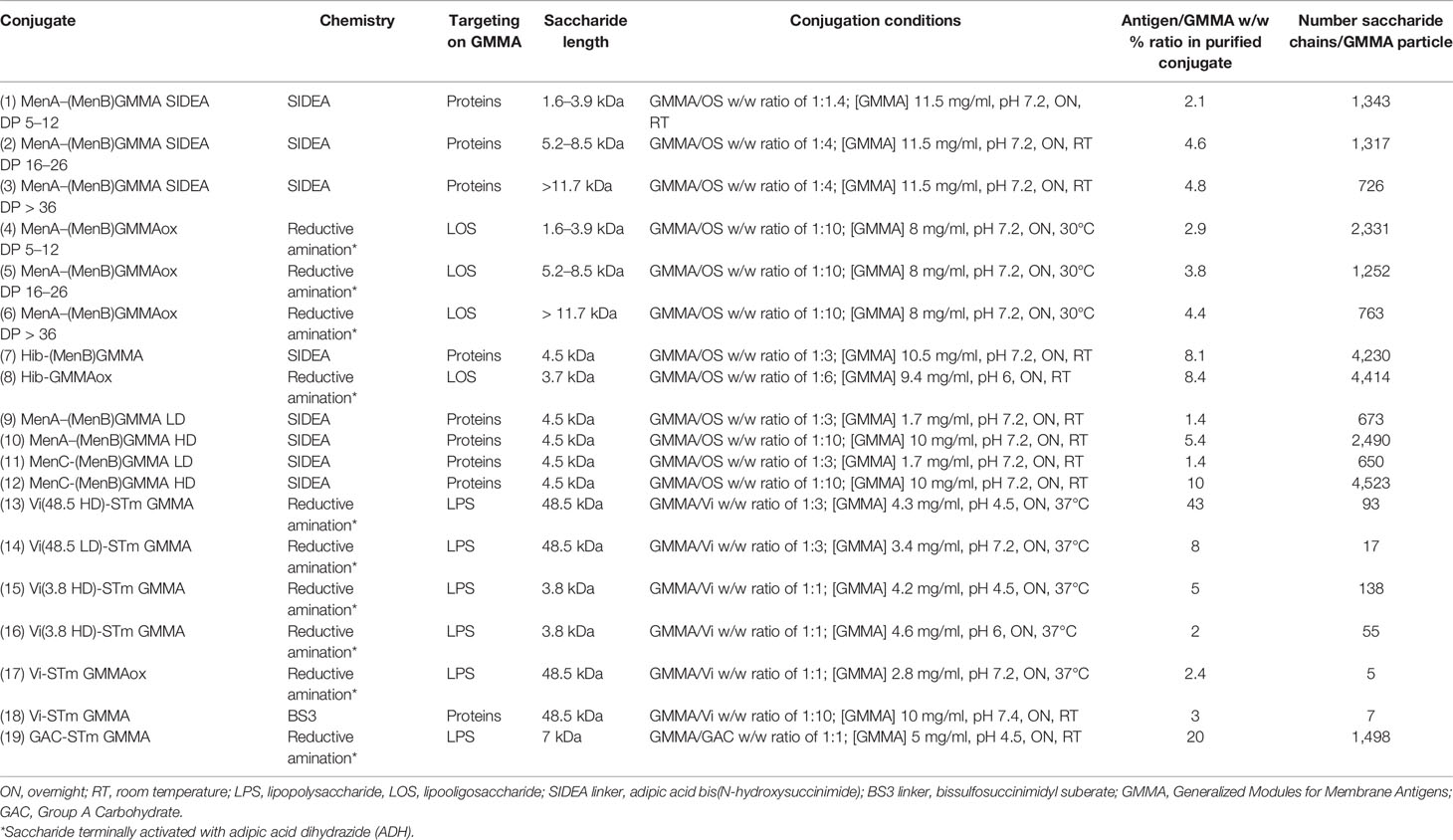
Table 1 Conjugation conditions used and main characteristics of the GMMA conjugates tested in this study.
Conjugation via Adipic Acid Bis(N-hydroxysuccinimide) Chemistry
MenA, MenC, or Hib oligosaccharides terminally activated with adipic acid bis(N-hydroxysuccinimide) (SIDEA) as previously described (29) were added to a suspension of GMMA in NaPi 50 mM pH 7.2. The mixture was stirred overnight at room temperature. Different conjugation conditions were used according to the PS linked and the GMMA used, as detailed in Table 1. Conjugates were purified by ultracentrifugation (110,000 rpm, 4°C, 1 h) and recovered in phosphate-buffered saline (PBS). Ultracentrifuge Thermo Scientific Sorvall MX 150+ Micro-Ultracentrifuge equipped with Thermo Scientific S110-AT rotor (K factor = 15) and 4-ml PC Thick Walled Tubes (Thermo Scientific Cat No. 45239) filled with 2 ml of solution were used.
Conjugation Through Reductive Amination Chemistry
GMMA oxidation. MenB GMMA at concentration of 8.0 mg/ml in NaPi 100 mM pH 6 were oxidized in the presence of NaIO4 5 mM for 30 min in the dark at the controlled temperature of 25°C. Excess of periodate was quenched with Na2SO3 10 mM for 15 min at room temperature before direct addition of the PS.
S. Typhimurium GMMA at 5 mg/ml in 100 mM of sodium acetate pH 5 was oxidized in the presence of 10 mM of NaIO4 for 2 h in the dark at the controlled temperature of 25°C. GMMA were purified by ultracentrifugation (110,000 rpm, 4°C, 30 min). Oxidized GMMA were resuspended in NaPi 100 mM pH 7.2. GMMA were characterized by micro BCA (>80% recovery), dynamic light scattering (confirming no aggregation), and high-performance anion-exchange chromatography with pulsed amperometric detection (HPAEC-PAD) (13% oxidation for S. Typhimurium GMMA and 41% for MenB GMMA).
Polysaccharide Derivatization With Adipic Acid Dihydrazide Linker
Vi (26), GAC PS, MenA, and Hib oligosaccharides were solubilized in 20 mM of sodium acetate buffer pH 4.5 at 30–40 mg/ml final concentration and added with ADH linker and NaBH3CN at a 1:1.2:1.2 w/w ratio. The solutions were mixed at 30°C for 5 days. The derivatized PS were purified by chromatography on two PD10 column equilibrated with 3 M of NaCl and then water. HPAEC-PAD was used for saccharide quantification, while TNBS colorimetric methods were used to check derivatization degree (100% for Vi, 56% for GAC, and >80% for MenA and Hib) (30). Free ADH was estimated by reversed-phase ultra-performance liquid chromatography (RP-UPLC) (<10% free NH2) (31).
Conjugations
Oxidized GMMA were added to the activated PS in the presence of NaBH3CN. Reaction conditions used for each conjugate are detailed in Table 1. The reaction was incubated overnight and purified by ultracentrifugation (110,000 rpm, 4°C, 1 h). The purified conjugate was resuspended in PBS.
Conjugation via Bissulfosuccinimidyl Suberate Chemistry
S. Typhimurium GMMA, at a protein concentration of 4.0 mg/ml in MES buffer pH 6, was added to BS3 linker at a final concentration of 50 mg/ml in the reaction mixture. The mixture was incubated at 25°C for 30 min; then activated GMMA were purified by ultracentrifugation (110,000 rpm, 16 min, 4°C). Resulting GMMA (70% recovery by micro BCA) had 43.8% of NH2 groups derivatized with the BS3 linker according to TNBS colorimetric method (30).
After GMMA-BS3 ultracentrifugation, Vi-ADH was immediately added. In the conjugation step, a 1:10 w/w ratio of GMMA to Vi-ADH was used, at Vi-ADH concentration of 100 mg/ml in NaPi 50 mM pH 7. After overnight incubation at room temperature (RT), the conjugate was purified by ultracentrifugation (110,000 rpm, 1 h, 4°) and recovered in PBS.
Conjugate Characterization
Conjugates were characterized by micro BCA for total protein recovery (21), while amount of saccharide antigen linked was determined by HPAEC-PAD after performing acid hydrolysis directly on GMMA as previously described (24, 32–36). It was verified that there was no interference from GMMA in the quantification of each saccharide. A known amount of the conjugated PS was physically mixed to GMMA, and it was verified that analysis by HPAEC-PAD gave results comparable with those obtained by testing the same amount of the PS alone. For MenA, MenC, Hib, and GAC saccharides, conjugate formation was also confirmed by sodium dodecyl sulfate–polyacrylamide gel electrophoresis (SDS-PAGE)/Western blotting as previously described (21). For MenA, MenC, and GAC conjugates, polyclonal sera internally generated in mice were used as primary antibodies, while for Hib conjugate, a commercial antibody (Bacto Hib DIFCO 2236-50-1) was used. NanoTracking Analysis (NTA) was used to count the number of GMMA particles in solution and estimate the number of PS chains per GMMA. NS300 Nanosight instrument (Malvern) equipped with a CMOS camera and a 488-nm monochromatic laser beam was used. Data acquisition and processing were performed using NTA software 3.2 build 3.2.16, and more details on the analysis can be found in De Benedetto et al. (23).
Percentage of free saccharide was calculated by solid-phase extraction (SPE) using a C4 cartridge (Vydac BioSelect) followed by HPAEC-PAD analysis for MenA, MenC, and Hib (33, 35), by high-performance liquid chromatography–size-exclusion chromatography (HPLC-SEC) (refractive index detection) for Vi and GAC conjugates.
Immunogenicity Studies in Animal Models
All animal sera used in this study were derived from mouse or rat immunization experiments performed at the GSK Animal Facility in Siena or at Toscana Life Sciences Animal Facility (Siena, Italy), in compliance with the relevant guidelines (Italian D. Lgs. n. 26/14 and European directive 2010/63/UE) and the institutional policies of GSK. The animal protocols were approved by the Animal Welfare Body of GSK, Siena, Italy, and by the Italian Ministry of Health (Approval number 804/2015-PR) and Animal Welfare Body of Toscana Life Sciences and by the Italian Ministry of Health (Approval number 479/2017-PR).
CD1 5-week-old female mice were immunized subcutaneously (s.c) or intramuscularly (i.m.) at days 0 and 28. CD1 5-week-old female nude mice (devoid of mature T cells) were immunized s.c. at days 0 and 28 (37). Crl : CD 8-week-old female rats were immunized i.m. at days 0 and 28. Mice and rats were bled from the retromandibular plexus and the tail vein, respectively. Rats were preheated for 5 min in a warming cage at 37°C before bleeding. Final bleed in rats was performed under general anesthesia (alfaxalone 20 mg/kg + medetomidine 0.05 mg/kg + fentanyl 0.1 mg/kg). Blood was kept at 37°C up to 2 h or at RT up to 3 h in the untreated collection tubes and then centrifuged for 10 min at 2,851 rcf, 4°C before serum collection. Animal models, immunization routes, and schemes were selected according to the PS antigens tested. Anti-antigen-specific IgG levels were measured at days −1, 27, and 42 (40 for the study in rats) by enzyme-linked immunosorbent assay (ELISA) (38). Purified O-antigen from S. Typhimurium and Streptococcal Group A Carbohydrate conjugated to human serum albumin (HSA) were used for ELISA plate coating at 5 and 1 µg/ml, respectively, in carbonate buffer pH 9.6; purified Vi at 1 µg/ml in phosphate buffer pH 7.0; purified MenA and MenC capsular PS were used at 5 µg/ml in PBS pH 8.2; and Hib PS conjugated to HSA was used at 2 µg/ml in PBS pH 7.4. ELISA units were expressed relative to a mouse antigen-specific antibody standard serum curve, with the best five-parameter fit determined by a modified Hill plot. One ELISA unit is defined as the reciprocal of the dilution of the standard serum that gives an absorbance value equal to 1 in this assay. Each mouse serum was run in triplicate.
Serum bactericidal antibody (SBA) against meningococcal (MenA, MenC, and MenB) strains was tested using baby rabbit complement as previously described (29, 39). F8238 MenA, C11 MenC, NZ98/254, M08-0240104, and M01-0240320 MenB strains were used. Pooled sera from each group were tested by SBA.
Statistical Analysis
Datasets were analyzed using two-tailed non-parametric Mann–Whitney test (for comparing the same time point for two different groups) or one-tailed non-parametric Wilcoxon matched-pairs signed rank test (for comparing different time points for a same group) with Prism (GraphPad Software). p-Values less than 0.05 were considered statistically significant.
Results
Chemical Linkage of Polysaccharides to Generalized Modules for Membrane Antigens
PS with different structural features and size were conjugated to GMMA from different pathogens. MenA, MenC, and Hib oligosaccharides were terminally activated with SIDEA linker (29) and randomly conjugated to lysines of GMMA surface proteins (Figure 1A). Alternatively, the oligosaccharides were terminally derivatized with ADH and linked to LOS on oxidized GMMA by reductive amination (Figure 1B).
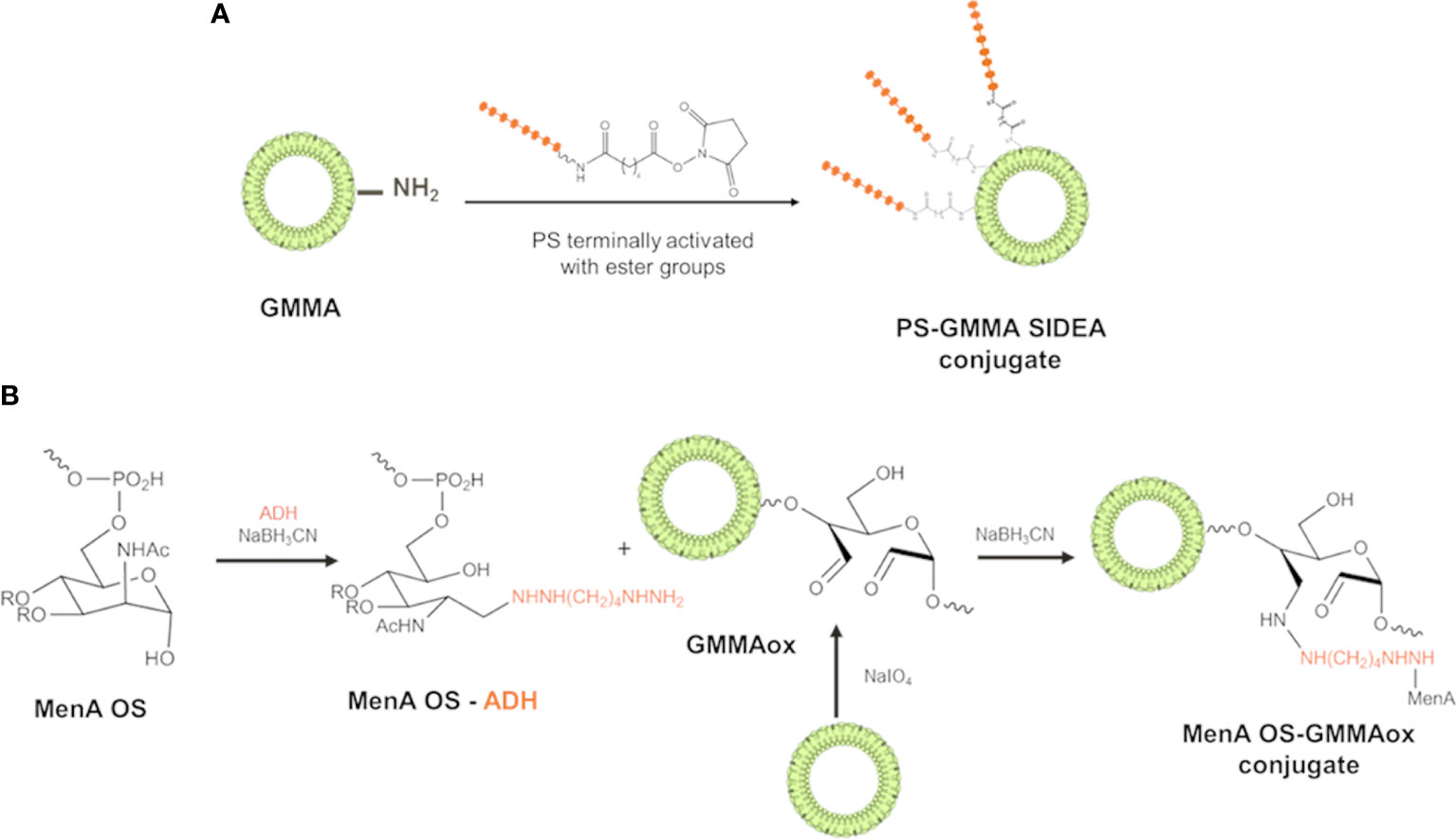
Figure 1 Conjugation schemes used for linkage of PS to GMMA. (A) PS were terminally activated with adipic acid bis(N-hydroxysuccinimide) (SIDEA) linker and randomly conjugated to lysines of GMMA surface proteins. (B) PS (MenA structure reported as example) were terminally derivatized with adipic acid dihydrazide (ADH) and linked to LPS/LOS on oxidized GMMA by reductive amination. PS, polysaccharides; GMMA, Generalized Modules for Membrane Antigens; ADH, adipic acid dihydrazide; LPS, lipopolysaccharides; LOS, lipooligosaccharides.
A similar approach was used for linkage of streptococcal GAC and S. Typhi Vi PS to GMMA. By playing with conjugation conditions, in particular by using different saccharide-to-protein molar ratios (as for meningococcal oligosaccharides) or different buffer pH (as for Vi), it was easy to modulate the number of sugar chains per GMMA particle (Table 1). Formation of saccharide–GMMA conjugates was verified by Western blotting analysis (Figure 2); and the amount of total saccharide and total protein were quantified by HPAEC-PAD and micro BCA, respectively. The saccharide-to-protein w/w ratio, coupled with an estimate of the number of GMMA particles per ml measured by NTA, allowed us to count the average number of saccharide chains per GMMA particle (Table 1).
Impact of Saccharide Length, Density, and Attachment Site on the Immune Response Induced by Generalized Modules for Membrane Antigens Conjugates
The impact that sugar length and linkage site on GMMA could have on the immune response induced by the conjugates was initially studied with MenA oligosaccharides linked to MenB GMMA. MenA oligosaccharides of different and non-overlapping length (polymerization degree (DP) equal to 5–12, 16–26, and >36) were conjugated to proteins or LOS on MenB GMMA (Table 1, constructs 1–6) and tested in mice. GMMA alone or physically mixed to MenA oligosaccharides were used as negative controls, while MenA–CRM197 conjugate was the positive control. Conjugation to proteins or LOS on GMMA resulted in induction of a strong anti-MenA IgG response at a level comparable with that of MenA–CRM197. We found that the sugar length did not influence MenA-specific serum IgG response, because no difference in antibody production was observed after immunization with the different MenA–GMMA conjugates (Figure 3A), regardless of whether conjugation was directed to LOS or proteins. Interestingly, the conjugates generated from saccharides attached to proteins invariably elicited a higher MenA-specific IgG response 2 weeks after second immunization compared with MenA oligosaccharides linked to LOS (Figure 3A). However, all GMMA conjugates, independently from the attachment site of meningococcal oligosaccharides to GMMA, induced antibodies with bactericidal activity against a homologous MenA strain (Figure 3B).
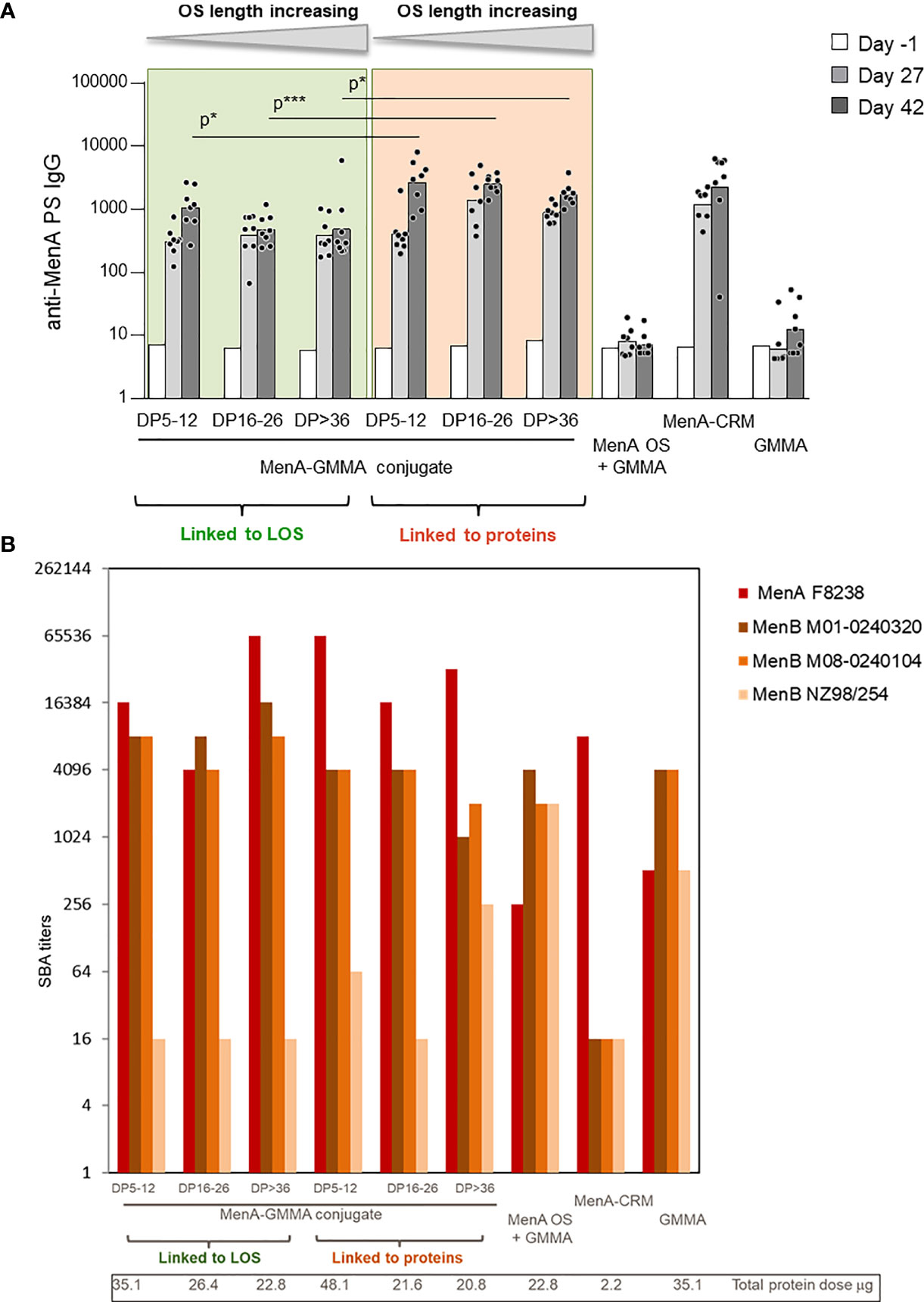
Figure 3 Impact of saccharide length and attachment site on the immune response induced by MenA–MenB GMMA conjugates. Eight CD1 mice per group were immunized i.m. at days 0 and 28, with 1 μg MenA/dose in the presence of Alhydrogel. Sera were collected at days −1, 27, and 42. (A) Summary graphs of anti-MenA PS IgG geometric mean units (bars) and individual antibody levels (dots) are reported (A). SBA titers of pooled sera collected 2 weeks after second injection against MenA and MenB strains are reported (B). GMMA, Generalized Modules for Membrane Antigens; PS, polysaccharides; SBA, serum bactericidal antibody. * 0.01 < p < 0.5; *** 0.0001 < p < 0.001.
The ability of MenB GMMA to induce an immune response after attachment of MenA oligosaccharides was verified by testing the bactericidal activity of antibodies induced against three different MenB strains (Figure 3B). While bactericidal activity against UK320 and UK104 strains was not impaired by conjugation, the one against the New Zealand strain was negatively impacted. As bactericidal activity against this strain is mainly mediated by the PorA antigen on MenB GMMA (40, 41), we could speculate that the random conjugation of MenA oligosaccharides to proteins on GMMA could impact on PorA structure and conformation. However, the same was true for the glycoconjugates obtained by linkage of oligosaccharides to LOS on MenB GMMA, indicating that probably the saccharide chains masked some protein components shifting the immune response toward themselves.
Next, Hib oligosaccharides were conjugated to MenB GMMA by targeting proteins or LOS (constructs 7–8, Table 1), and the Hib-specific serum IgG response was measured in rats, in comparison with Hib oligosaccharides mixed to GMMA and Hib-CRM197 conjugate (Figure 4). As observed for MenA conjugates, also in this case, the conjugate obtained by linkage of Hib to proteins induced a stronger anti-Hib IgG response than the conjugate produced by linking oligosaccharides to LOS. Both GMMA conjugates elicited anti-Hib PS IgG titers significantly higher than Hib simply mixed to GMMA and comparable with Hib-CRM197.
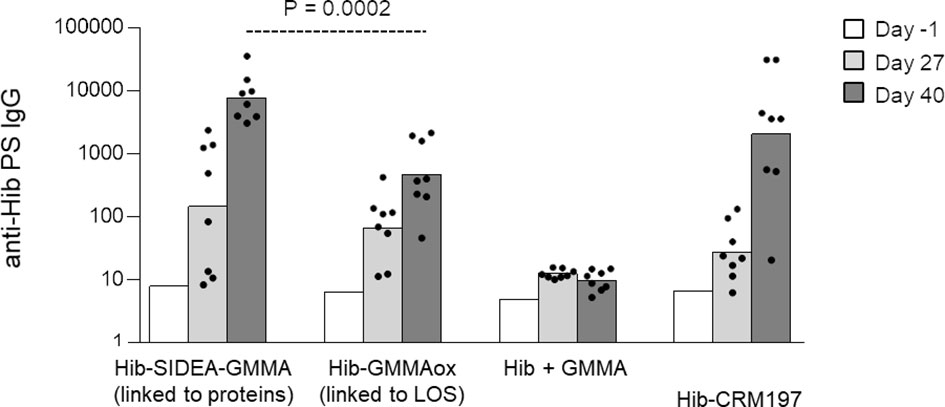
Figure 4 Hib oligosaccharides were conjugated to proteins (Hib-SIDEA-GMMA) or LOS (Hib-GMMAox) of GMMA. Resulting conjugates were compared in rats together with Hib physically mixed to GMMA and Hib-CRM197 with Alhydrogel. Eight adult rats per group were i.m. immunized at days 0 and 28 (0.5 μg Hib/dose). Sera were collected at days −1, 27, and 40 and analyzed for anti-Hib PS IgG response. Summary graphs of anti-PS IgG geometric mean units (bars) and individual antibody levels (dots) are reported. LOS, lipooligosaccharides; GMMA, Generalized Modules for Membrane Antigens.
After having investigated the impact of saccharide length and attachment via proteins or LOS on GMMA, we interrogated the effect of the density of saccharide conjugated to GMMA particles, which is another important feature for glycoconjugate vaccines. We produced conjugates differing for the average number of MenA or MenC oligosaccharides linked per GMMA particle (constructs 9–12, Table 1). No major impact of oligosaccharide density on anti-PS IgG response (Figure 5A) and functionality of the sera induced in mice was found (Figure 5B). However, control of glycosylation density could be useful to fully preserve the immune response induced by GMMA per se. In fact, by testing the bactericidal activity of the sera against a panel of different MenB strains, we assessed that the largest was the number of meningococcal oligosaccharide chains conjugated per GMMA particle, and the highest was the impact on the functionality of the elicited sera (this was particularly evident for MenB NZ98/254 strain). Therefore, linkage of fewer sugar chains per GMMA particle seems preferable.
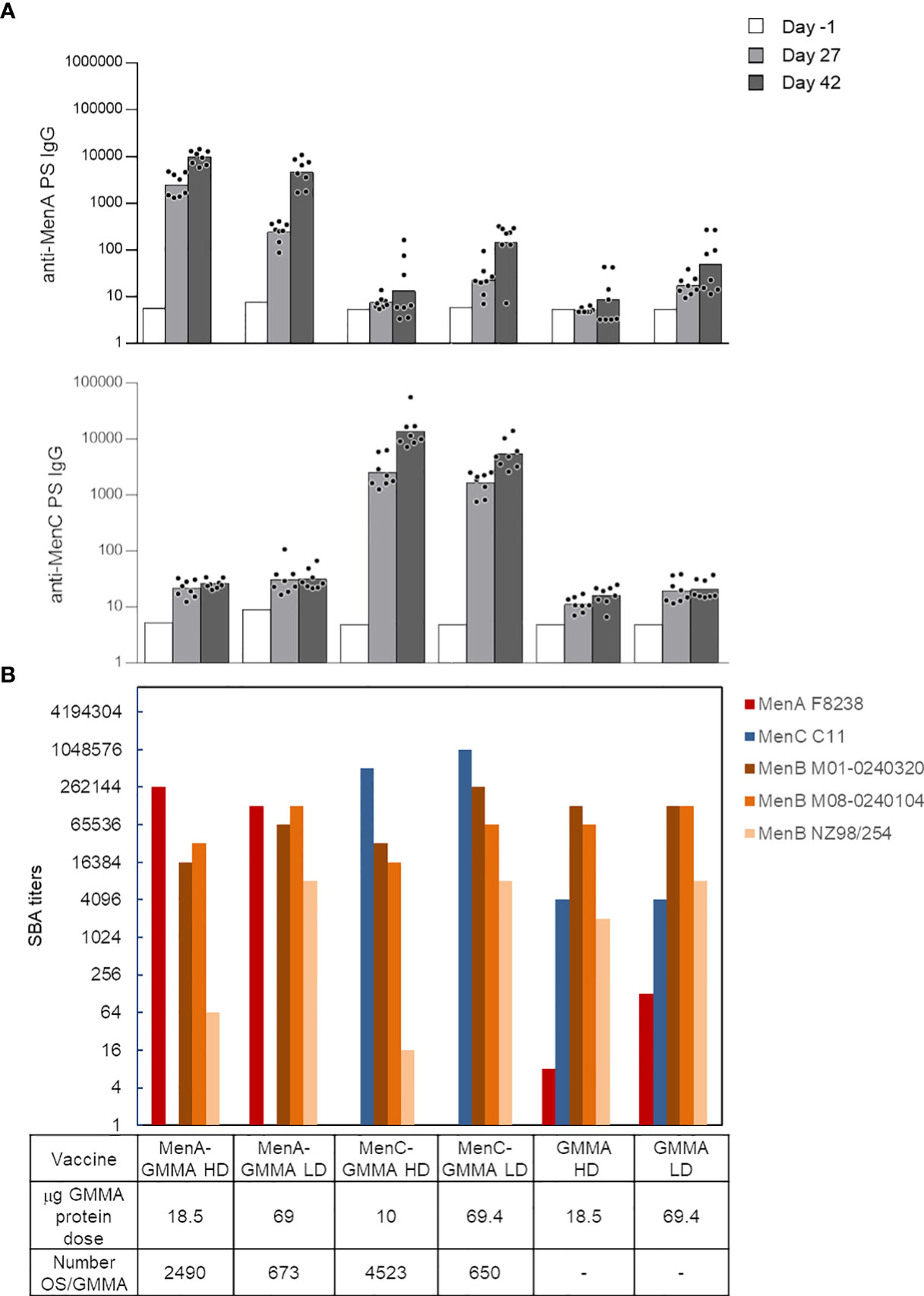
Figure 5 Impact of saccharide density on the immune response induced by MenA/MenC–MenB GMMA conjugates. Eight CD1 mice per group were immunized i.m. at days 0 and 28, with 1 μg MenA/MenC/dose in the presence of Alhydrogel. Sera were collected at days −1, 27, and 42. Anti-MenA and MenC IgG response (A) and SBA titers of pooled sera collected 2 weeks after second injection against MenA, MenC, and MenB strains (B) are reported. HD, high density; LD, low density. Summary graphs of IgG geometric mean units (bars) and individual antibody levels (dots) are reported. In (B), absent bars for MenA and MenC strains represent measures not done. GMMA alone were used as control. GMMA, Generalized Modules for Membrane Antigens; SBA, serum bactericidal antibody.
To further explore the effect of glycan length and density with a larger-size PS, conjugates formed by S. Typhi Vi PS attached to S. Typhimurium GMMA were generated (constructs 13–16, Table 1). Linkage of Vi to LPS on GMMA allowed to introduce a different number of PS chains to GMMA, while conjugation to proteins resulted in few Vi chains per GMMA particle only. As previously verified for MenA and MenC (Figure 5), we did not find impact of antigen density on Vi-specific serum IgG response. However, the saccharide length in this case generated a significant effect, because longer Vi PS (48.5 kDa) were able to induce significantly higher Vi-specific serum IgG titers than the shorter Vi (3.8 kDa) (Figure 6A). All conjugates induced anti-S. Typhimurium O-antigen IgG response, preserving immunogenicity of GMMA per se (Figure 6B).
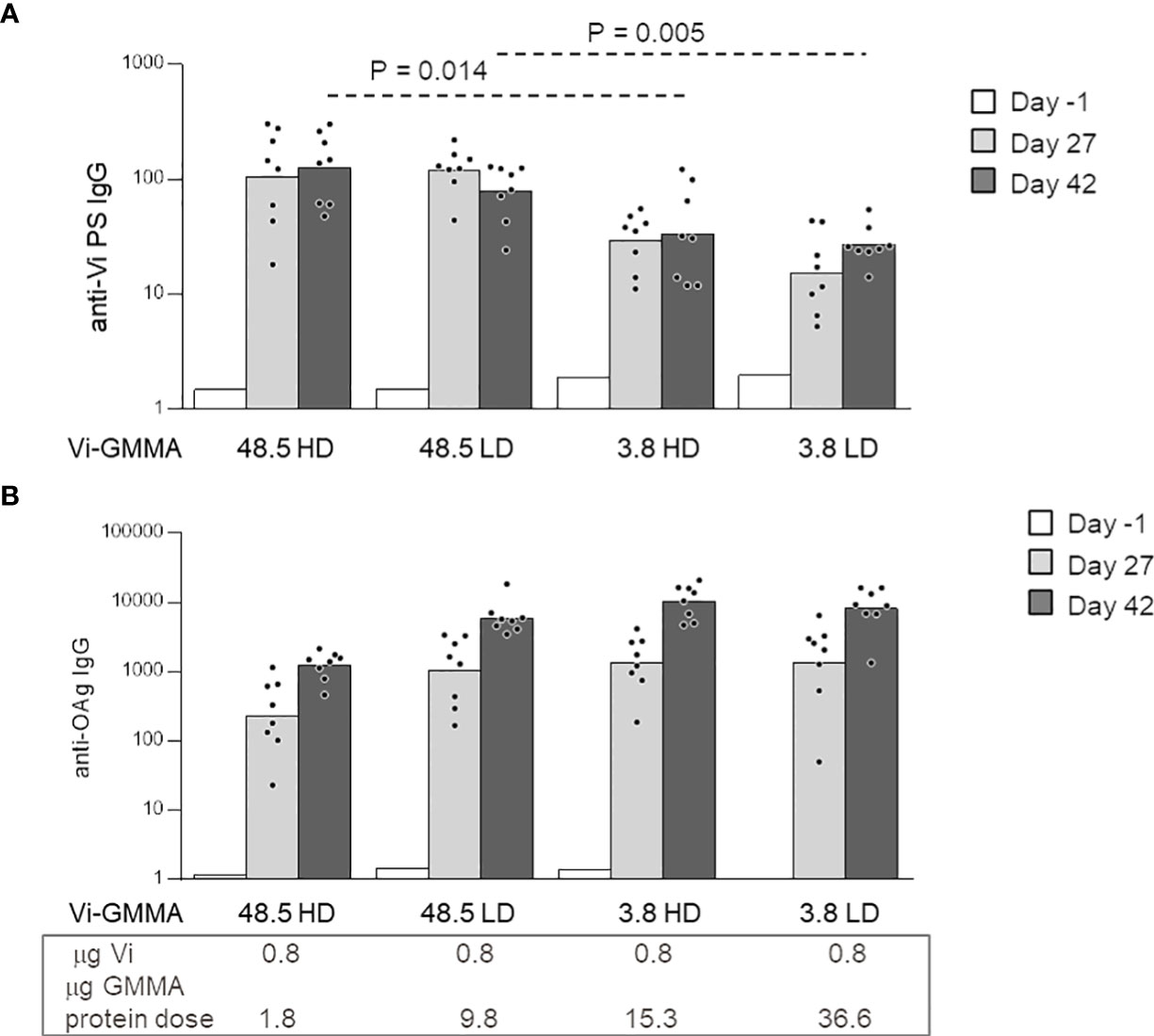
Figure 6 Impact of saccharide length and density on the immune response induced by Vi-Salmonella Typhimurium GMMA conjugates. Eight CD1 mice per group were immunized s.c. at days 0 and 28, with 0.8 μg Vi/dose with no Alhydrogel. Sera were collected at days −1, 27, and 42 and analyzed for anti-Vi (A) and anti-S. Typhimurium O-antigen (OAg) (B) IgG response. Summary graphs of IgG geometric mean units (bars) and individual antibody levels (dots) are reported. HD, high density; LD, low density; GMMA, Generalized Modules for Membrane Antigens.
Glycoconjugation to Generalized Modules for Membrane Antigens Promotes a Shift Toward a T-Independent Humoral Immune Response Based on the Type of Conjugated Saccharide
The display of PS on GMMA, especially if in high-density modality, generates repetitive epitope moieties on GMMA surface that can facilitate cognate B-cell receptor cross-linking, which could lead to B-cell activation in the absence of T-cell help. This shift toward a strong and fast T-independent B-cell stimulation would not promote germinal center formation and the consequent generation of long-lived plasma cells secreting high-affinity antibodies and memory B cells. Therefore, the T-independent B-cell response can have a negative impact on the efficacy of the humoral immune response, especially in infants or young children, and a detrimental effect on immunological memory and persistence of the antibody response. To investigate any potential T-independent nature of the humoral immune response induced by PS conjugated on GMMA, we evaluated different PS–GMMA conjugates immunizing wild-type and nude mice, devoid of mature T cells. We used MenC, Vi, and GAC PS conjugated to S. Typhimurium GMMA, so as to test PS with different structural features (constructs 12 and 17–19, Table 1).
MenC–GMMA conjugate was unable to induce a significant MenC-specific antibody response in nude mice compared with wild-type animals, clearly confirming the need of the T-cell help to promote a humoral response against MenC oligosaccharide (Figure 7A). On the contrary, Vi-GMMA induced a strong anti-Vi-specific serum IgG response in wild-type as well as nude mice, revealing that this PS promoted a T-independent humoral immune response (Figure 7B). Antibody levels induced in wild-type mice were high after the first dose, with no booster after re-injection. The same was verified by linking Vi PS to LPS or proteins on GMMA. Using GAC–GMMA conjugate, we observed an intermediate situation, since nude mice immunized with GAC–GMMA conjugate generated a GAC-specific serum IgG response, which was significantly lower than that induced in wild-type mice (Figure 7C). Thus, GMMA-conjugated GAC PS elicit a weak T-independent saccharide response.
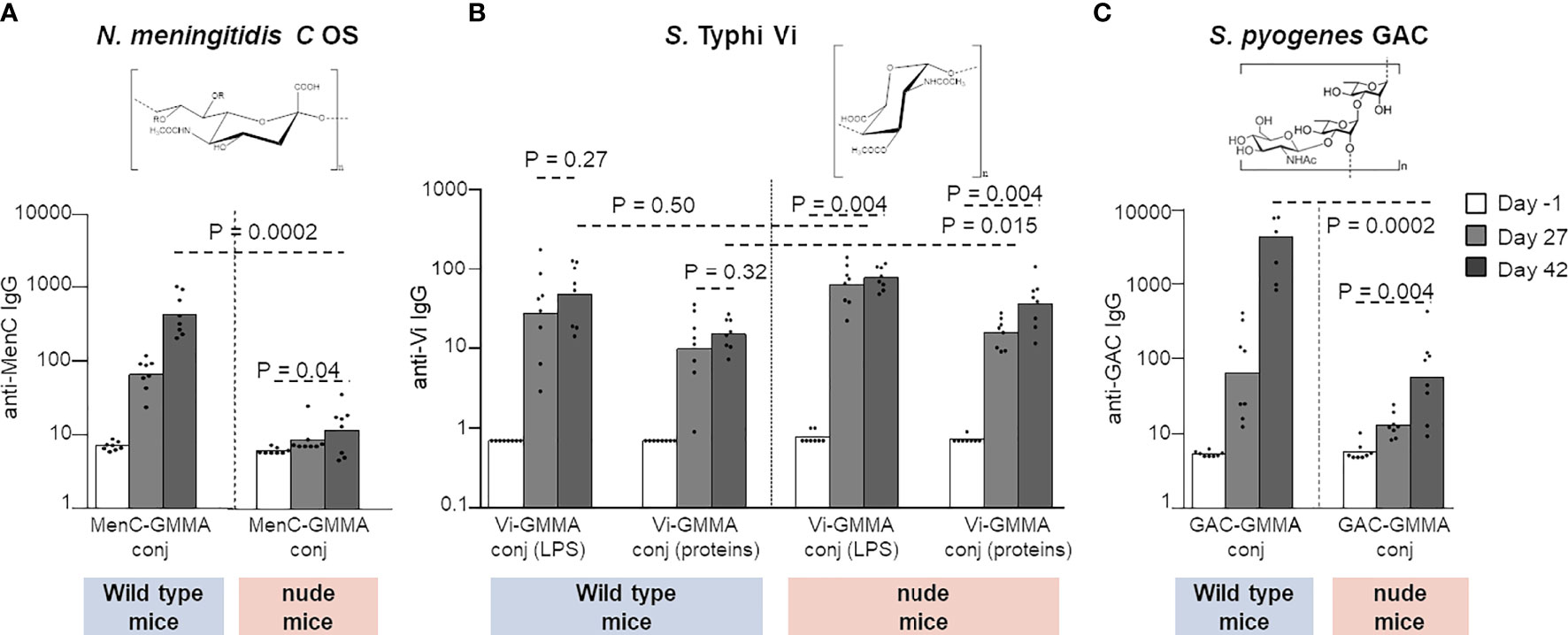
Figure 7 Different PS were linked to GMMA and tested in wild-type or CD1 nude mice. Eight mice per group were s.c. immunized at days 0 and 28 with 1 μg MenC oligosaccharide (A), 0.8 μg Vi PS (B), or 1 μg GAC (C). All formulations were tested in the absence of Alhydrogel. Sera were collected at days −1, 27, and 42 and analyzed for anti-PS-specific IgG response. Summary graphs of IgG geometric mean units (bars) and individual antibody levels (dots) are reported. PS, polysaccharides; GMMA, Generalized Modules for Membrane Antigens; GAC, Group A Carbohydrate.
Discussion
Conjugation to appropriate carrier proteins, providing T-cell help, is an established way for improving immunogenicity of PS antigens giving rise to immunological memory, isotype switching, affinity maturation, persistence of antibody response, and ability to induce adequate protection in infants and children under 2 years of age (42–46). Few carrier proteins have been used so far for licensed glycoconjugates (1), highlighting in certain cases reduced immunogenicity against the PS hapten due to preexisting immunity toward the protein (the so-called “carrier epitope suppression”) (47). Recent years have seen efforts to identify alternative carrier proteins, particularly with a dual role of carrier and antigen (3).
Recently, we have proposed GMMA as carrier for heterologous PS, showing the ability to enhance the antigen-specific humoral immune response compared with the antigen alone or physically mixed with GMMA (21).
Compared with traditional carrier proteins, GMMA are nanoparticle systems, with optimal size for immune stimulation and presenting multiple copies of the PS favoring B-cell activation. They possess immunostimulatory molecules, such as LPS, lipoproteins, or peptidoglycans, that can stimulate innate immunity and consequently enhance adaptive immunity (5, 7). Importantly, GMMA can be produced using a simple manufacturing process (15, 48). Here, we developed conjugation chemistries to easily and efficiently link PS differing in structure and size to GMMA surface, targeting both LPS and proteins on GMMA. Also, conjugation to GMMA was modulated not to impact on the immune response induced by GMMA as antigen. This supports the use of GMMA with a dual role of carrier and antigen for the development of multicomponent vaccines covering various diseases at the same time. In particular, Salmonella and meningococcal diseases, here used as models, are both common in several countries of sub-Saharan Africa (49–51); Hib and MenB are two critical etiological agents of meningitis, and a unique pan-meningococcal vaccine could offer a unique opportunity to combat the meningococcal meningitis worldwide; finally, S. Typhi and non-typhoidal Salmonella are leading causes of disease and mortality in Africa (52).
Antigen length and density are parameters that can play a role on the immune response elicited by glycoconjugate vaccines (22). Here, by playing with these features on GMMA, we observed that PS density seems not to play major role on the anti-saccharide-specific immune response induced in mice. Also, a limited number of oligosaccharide chains linked to GMMA is sufficient for inducing a strong immune response. On the other hand, saccharide length can play a role depending on the specific PS used. This confirms that, similar to traditional glycan–protein conjugates, saccharide length needs to be investigated and optimized specifically for each antigen of interest.
Another relevant aspect of this work is the observation that the carrier effect of GMMA for PS is observed irrespectively of whether the antigen is linked on GMMA proteins or LPS/LOS and can be dependent or not on T-cell help, based on the nature of the PS. Recently, it has been shown that glycan–protein conjugates induce a T-cell-dependent response through generation in B cells of peptides or glycopeptides (depending on the nature of the conjugated sugar) that are presented to the helper T cells (53, 54). Our finding suggests that the immunological mechanisms of the “carrier” effect of GMMA for PS could be the result of different coexisting mechanisms, which would be also depending on the nature of the PS linked.
Interestingly, even when GAC was linked to LPS on GMMA, the immune response was strongly mediated by T-cell activation as verified by the much lower response induced by the conjugate in nude mice. This supports the finding that direct linkage of PS to proteins is not needed, although co-presentation seems crucial. It is important that the interaction between protein and PS moieties is strong enough to allow internalization in the same B cell to assure T-cell engagement (3). Finally, our data show that linkage of certain PS (e.g., MenA and Hib) to proteins on GMMA can result in higher anti-PS-specific IgG response and seems preferable to conjugation to the LPS. Recently, E. coli glycoengineered OMVs have been proposed for the expression of heterologous PS that are anchored to lipid A-core as acceptor (11, 12, 55). Our findings can be informative also for this approach. Compared with the chemical conjugation proposed here, glycoengineering holds the potential for simplified and lower-cost vaccine production. However, chemical conjugation can be more easily applied to OMVs/GMMA from different pathogens and to PS with different structures and can represent a fast tool to investigate how parameters such as those investigated here impact the immune response elicited by these novel glycoconjugates, including glycoengineered OMVs.
Also additional nanoparticle systems, such as Qβ (56, 57) and hepatitis B core antigen virus-like particles (58), are being proposed as novel carrier systems to provide a strong anti-PS immune response. It will be interesting to compare GMMA and OMVs with these other systems for their ability to induce strong response after one only injection, persistency of the response, memory, and ultimately efficacy in infants and to see if they will behave similarly mainly due to their particulate nature and display of multiple antigens or if there will be specific features for a difference.
In conclusion, we found that optimization of parameters such as sugar length and density is crucial to fully exploit the potential of GMMA as platform for multicomponent vaccines, where GMMA can act as T-cell helper and antigen. The action of GMMA as carrier seems independent of the direct linkage of the sugar to the protein and present some specificity that deserves to be further investigated. Additional studies, including evaluation of IgG subclasses, IgM, antibody affinity, and cellular response, will be needed to further characterize the quality of the immune response elicited by GMMA conjugates and to better understand the mechanism of action elicited by these novel carrier systems. Unraveling these immunological mechanisms could guide the design of even more effective GMMA-based vaccines and would be informative for other nanoparticle based conjugates under development.
Data Availability Statement
The raw data supporting the conclusions of this article will be made available by the authors, without undue reservation.
Ethics Statement
The animal study was reviewed and approved by Animal Welfare Body of GSK, Siena, Italy, and by the Italian Ministry of Health (Approval number 804/2015-PR) and Animal Welfare Body of Toscana Life Sciences and by the Italian Ministry of Health (Approval number 479/2017-PR).
Author Contributions
FMi, RAd, and DP designed the study. FMi wrote the manuscript. FMi, RAl, RD, FS, FM, MC, DO, OP, CB, NB, GG, and BB performed the experiments and analyzed the data. FMi, FN, CB, BB, DP, and RAd supervised research and reviewed the data. All authors contributed to the article and approved the submitted version.
Funding
The authors declare that this study received funding from GlaxoSmithKline Biologicals SA. The funder was not involved in the study design, collection, analysis, interpretation of data, the writing of this article or the decision to submit it for publication.
Conflict of Interest
All the authors were employees of the GSK group of companies when the study was performed. FMi, RAl, and RD are listed as inventors on patents related to this work owned by the GSK group of companies. GSK Vaccines Institute for Global Health Srl is an affiliate of GlaxoSmithKline Biologicals SA.
Publisher’s Note
All claims expressed in this article are solely those of the authors and do not necessarily represent those of their affiliated organizations, or those of the publisher, the editors and the reviewers. Any product that may be evaluated in this article, or claim that may be made by its manufacturer, is not guaranteed or endorsed by the publisher.
Acknowledgments
We thank M. R. Romano and her team, S. Savino and her team (GSK), and T.D. in GVGH for providing starting materials for this study. Meningococcal strain NZ98/254 was kindly provided by Diana Martin (Institute of Environmental Science and Research, Porirua, New Zealand), while M01-0240320 and M08-0240104 strains were kindly provided by Ray Borrow (Health Protection Agency, Manchester, United Kingdom, now Public Health England, Manchester, United Kingdom). We thank Allan Saul for useful discussions during the execution of this work. This manuscript is dedicated to the memory of Paolo Costantino for the exceptional contribution provided.
References
1. Micoli F, Adamo R, Costantino P. Protein Carriers for Glycoconjugate Vaccines: History, Selection Criteria, Characterization and New Trends. Molecules (2018) 23(6). doi: 10.3390/molecules23061451
2. Micoli F, Del Bino L, Alfini R, Carboni F, Romano MR, Adamo R. Glycoconjugate Vaccines: Current Approaches Towards Faster Vaccine Design. Expert Rev Vaccines (2019) 1–15. doi: 10.1080/14760584.2019.1657012
3. Berti F, Micoli F. Improving Efficacy of Glycoconjugate Vaccines: From Chemical Conjugates to Next Generation Constructs. Curr Opin Immunol (2020) 65:42–9. doi: 10.1016/j.coi.2020.03.015
4. Irvine DJ, Read BJ. Shaping Humoral Immunity to Vaccines Through Antigen-Displaying Nanoparticles. Curr Opin Immunol (2020) 65:1–6. doi: 10.1016/j.coi.2020.01.007
5. Micoli F, MacLennan CA. Outer Membrane Vesicle Vaccines. Semin Immunol (2020) 50:101433. doi: 10.1016/j.smim.2020.101433
6. Gerritzen MJH, Martens DE, Wijffels RH, van der Pol L, Stork M. Bioengineering Bacterial Outer Membrane Vesicles as Vaccine Platform. Biotechnol Adv (2017) 35(5):565–74. doi: 10.1016/j.biotechadv.2017.05.003
7. Mancini F, Rossi O, Necchi F, Micoli F. OMV Vaccines and the Role of TLR Agonists in Immune Response. Int J Mol Sci (2020) 21(12):4416. doi: 10.3390/ijms21124416
8. Donnelly JJ, Deck RR, Liu MA. Immunogenicity of a Haemophilus Influenzae Polysaccharide-Neisseria Meningitidis Outer Membrane Protein Complex Conjugate Vaccine. J Immunol (1990) 145(9):3071–9.
9. Granoff DM, Holmes SJ. Comparative Immunogenicity of Haemophilus Influenzae Type B Polysaccharide-Protein Conjugate Vaccines. Vaccine (1991) 9(Suppl S30–34):discussion S42-33. doi: 10.1016/0264-410x(91)90178-9
10. Latz E, Franko J, Golenbock DT, Schreiber JR. Haemophilus Influenzae Type B-Outer Membrane Protein Complex Glycoconjugate Vaccine Induces Cytokine Production by Engaging Human Toll-Like Receptor 2 (TLR2) and Requires the Presence of TLR2 for Optimal Immunogenicity. J Immunol (2004) 172(4):2431–8. doi: 10.4049/jimmunol.172.4.2431%J
11. Valguarnera E, Feldman MF. Glycoengineered Outer Membrane Vesicles as a Platform for Vaccine Development. Methods Enzymol (2017) 597:285–310. doi: 10.1016/bs.mie.2017.06.032
12. Price NL, Goyette-Desjardins G, Nothaft H, Valguarnera E, Szymanski CM, Segura M, et al. Glycoengineered Outer Membrane Vesicles: A Novel Platform for Bacterial Vaccines. Sci Rep (2016) 6(1):24931. doi: 10.1038/srep24931
13. Rossi O, Pesce I, Giannelli C, Aprea S, Caboni M, Citiulo F, et al. Modulation of Endotoxicity of Shigella Generalized Modules for Membrane Antigens (GMMA) by Genetic Lipid A Modifications: Relative Activation of TLR4 and TLR2 Pathways in Different Mutants. J Biol Chem (2014) 289(36):24922–35. doi: 10.1074/jbc.M114.566570
14. Rossi O, Caboni M, Negrea A, Necchi F, Alfini R, Micoli F, et al. Toll-Like Receptor Activation by Generalized Modules for Membrane Antigens From Lipid A Mutants of Salmonella Enterica Serovars Typhimurium and Enteritidis. Clin Vaccine Immunol (2016) 23(4):304–14. doi: 10.1128/CVI.00023-16
15. Gerke C, Colucci AM, Giannelli C, Sanzone S, Vitali CG, Sollai L, et al. Production of a Shigella Sonnei Vaccine Based on Generalized Modules for Membrane Antigens (GMMA), 1790gahb. PloS One (2015) 10(8):e0134478. doi: 10.1371/journal.pone.0134478
16. De Benedetto G, Alfini R, Cescutti P, Caboni M, Lanzilao L, Necchi F, et al. Characterization of O-Antigen Delivered by Generalized Modules for Membrane Antigens (GMMA) Vaccine Candidates Against Nontyphoidal Salmonella. Vaccine (2017a) 35(3):419–26. doi: 10.1016/j.vaccine.2016.11.089
17. Schager AE, Dominguez-Medina CC, Necchi F, Micoli F, Goh YS, Goodall M, et al. IgG Responses to Porins and Lipopolysaccharide Within an Outer Membrane-Based Vaccine Against Nontyphoidal Salmonella Develop at Discordant Rates. MBio (2018) 9(2):e02379–17. doi: 10.1128/mBio.02379-17
18. Raso MM, Gasperini G, Alfini R, Schiavo F, Aruta MG, Carducci M, et al. GMMA and Glycoconjugate Approaches Compared in Mice for the Development of a Vaccine Against Shigella Flexneri Serotype 6. Vaccines (Basel) (2020) 8(2):160. doi: 10.3390/vaccines8020160
19. Gasperini G, Raso MM, Arato V, Aruta MG, Cescutti P, Necchi F, et al. Effect of O-Antigen Chain Length Regulation on the Immunogenicity of Shigella and Salmonella Generalized Modules for Membrane Antigens (GMMA). Int J Mol Sci (2021) 22(3):1309. doi: 10.3390/ijms22031309
20. Micoli F, Rondini S, Alfini R, Lanzilao L, Necchi F, Negrea A, et al. Comparative Immunogenicity and Efficacy of Equivalent Outer Membrane Vesicle and Glycoconjugate Vaccines Against Nontyphoidal Salmonella. Proc Natl Acad Sci USA (2018) 41(115):10428–33. doi: 10.1073/pnas.1807655115
21. Micoli F, Alfini R, Di Benedetto R, Necchi F, Schiavo F, Mancini F, et al. GMMA Is a Versatile Platform to Design Effective Multivalent Combination Vaccines. Vaccines (Basel) (2020) 8(3). doi: 10.3390/vaccines8030540
22. Costantino P, Rappuoli R, Berti F. The Design of Semi-Synthetic and Synthetic Glycoconjugate Vaccines. Expert Opin Drug Discov (2011) 6(10):1045–66. doi: 10.1517/17460441.2011.609554
23. De Benedetto G, Cescutti P, Giannelli C, Rizzo R, Micoli F. Multiple Techniques for Size Determination of Generalized Modules for Membrane Antigens From Salmonella Typhimurium and Salmonella Enteritidis. ACS Omega (2017) 2(11):8282–9. doi: 10.1021/acsomega.7b01173
24. Kabanova A, Margarit I, Berti F, Romano MR, Grandi G, Bensi G, et al. Evaluation of a Group A Streptococcus Synthetic Oligosaccharide as Vaccine Candidate. Vaccine (2010) 29(1):104–14. doi: 10.1016/j.vaccine.2010.09.018
25. Micoli F, Rondini S, Pisoni I, Giannelli C, Di Cioccio V, Costantino P, et al. Production of a Conjugate Vaccine for Salmonella Enterica Serovar Typhi From Citrobacter Vi. Vaccine (2012) 30(5):853–61. doi: 10.1016/j.vaccine.2011.11.108
26. Arcuri M, Di Benedetto R, Cunningham AF, Saul A, MacLennan CA, Micoli F. The Influence of Conjugation Variables on the Design and Immunogenicity of a Glycoconjugate Vaccine Against Salmonella Typhi. PloS One (2017) 12(12):e0189100. doi: 10.1371/journal.pone.0189100
27. Di Benedetto R, Mancini F, Carducci M, Gasperini G, Moriel DG, Saul A, et al. Rational Design of a Glycoconjugate Vaccine Against Group A Streptococcus. Int J Mol Sci (2020) 21(22):8558. doi: 10.3390/ijms21228558
28. Henriques P, Dello Iacono L, Gimeno A, Biolchi A, Romano MR, Arda A, et al. Structure of a Protective Epitope Reveals the Importance of Acetylation of Neisseria Meningitidis Serogroup A Capsular Polysaccharide. Proc Natl Acad Sci USA (2020) 117(47):29795–802. doi: 10.1073/pnas.2011385117
29. Costantino P, Viti S, Podda A, Velmonte MA, Nencioni L, Rappuoli R. Development and Phase 1 Clinical Testing of a Conjugate Vaccine Against Meningococcus A and C. Vaccine (1992) 10(10):691–8. doi: 10.1016/0264-410x(92)90091-w
30. Satake K, Okuyama T, Ohashi M, Shinoda T. The Spectrophotometric Determination of Amine, Amino Acid and Peptide With 2,4,6- Trinitrobenzene 1-Sulfonic Acid*. J Biochem (1960) 47:654. doi: 10.1093/oxfordjournals.jbchem.a127107
31. Micoli F, Rondini S, Gavini M, Lanzilao L, Medaglini D, Saul A, et al. O:2-CRM(197) Conjugates Against Salmonella Paratyphi A. PloS One (2012a) 7(11):e47039. doi: 10.1371/journal.pone.0047039
32. Bardotti AA, Ravenscroft NN, Ricci SS, D’Ascenzi SS, Guarnieri VV, Averani GG, et al. Quantitative Determination of Saccharide in Haemophilus Influenzae Type B Glycoconjugate Vaccines, Alone and in Combination With DPT, by Use of High-Performance Anion-Exchange Chromatography With Pulsed Amperometric Detection. Vaccine (2000) 18(19):1982–93. doi: 10.1016/s0264-410x(99)00535-6
33. Ho MM, Bolgiano B, Corbel MJ. Assessment of the Stability and Immunogenicity of Meningococcal Oligosaccharide C-CRM197 Conjugate Vaccines. Vaccine (2000) 19(7-8):716–25. doi: 10.1016/s0264-410x(00)00261-9
34. Ho MM, Mawas F, Bolgiano B, Lemercinier X, Crane DT, Huskisson R, et al. Physico-Chemical and Immunological Examination of the Thermal Stability of Tetanus Toxoid Conjugate Vaccines. Vaccine (2002) 20(29-30):3509–22. doi: 10.1016/s0264-410x(02)00342-0
35. Ispasanie E, Micoli F, Lamelas A, Keller D, Berti F, De Riccio R, et al. Spontaneous Point Mutations in the Capsule Synthesis Locus Leading to Structural and Functional Changes of the Capsule in Serogroup A Meningococcal Populations. Virulence (2018) 9(1):1138–49. doi: 10.1080/21505594.2018.1467710
36. Giannelli C, Raso MM, Palmieri E, De Felice A, Pippi F, Micoli F. Development of a Specific and Sensitive HPAEC-PAD Method for Quantification of Vi Polysaccharide Applicable to Other Polysaccharides Containing Amino Uronic Acids. Anal Chem (2020) 92(9):6304–11. doi: 10.1021/acs.analchem.9b05107
37. Micoli F, Bjarnarson SP, Arcuri M, Aradottir Pind AA, Magnusdottir GJ, Necchi F, et al. Short Vi-Polysaccharide Abrogates T-Independent Immune Response and Hyporesponsiveness Elicited by Long Vi-CRM(197) Conjugate Vaccine. Proc Natl Acad Sci USA (2020) 117(39):24443–9. doi: 10.1073/pnas.2005857117
38. Rondini S, Micoli F, Lanzilao L, Hale C, Saul AJ, Martin LB. Evaluation of the Immunogenicity and Biological Activity of the Citrobacter Freundii Vi-CRM197 Conjugate as a Vaccine for Salmonella Enterica Serovar Typhi. Clin Vaccine Immunol (2011) 18(3):460–8. doi: 10.1128/cvi.00387-10
39. Giuliani MM, Santini L, Brunelli B, Biolchi A, Arico B, Di Marcello F, et al. The Region Comprising Amino Acids 100 to 255 of Neisseria Meningitidis Lipoprotein GNA 1870 Elicits Bactericidal Antibodies. Infect Immun (2005) 73(2):1151–60. doi: 10.1128/iai.73.2.1151-1160.2005
40. Holst J, Martin D, Arnold R, Huergo CC, Oster P, O’Hallahan J, et al. Properties and Clinical Performance of Vaccines Containing Outer Membrane Vesicles From Neisseria Meningitidis. Vaccine (2009) 27(Suppl 2):B3–12. doi: 10.1016/j.vaccine.2009.04.071
41. Pizza M, Rappuoli R. Neisseria Meningitidis: Pathogenesis and Immunity. Curr Opin Microbiol (2015) 23:68–72. doi: 10.1016/j.mib.2014.11.006
42. Schneerson R, Barrera O, Sutton A, Robbins JB. Preparation, Characterization, and Immunogenicity of Haemophilus Influenzae Type B Polysaccharide-Protein Conjugates. J Exp Med (1980) 152:361–76. doi: 10.1084/jem.152.2.361
43. Kelly DF, Pollard AJ, Moxon ER. Immunological Memory: The Role of B Cells in Long-Term Protection Against Invasive Bacterial Pathogens. JAMA (2005) 294(23):3019–23. doi: 10.1001/jama.294.23.3019
44. Pollard AJ, Perrett KP, Beverley PC. Maintaining Protection Against Invasive Bacteria With Protein-Polysaccharide Conjugate Vaccines. Nat Rev Immunol (2009) 9(3):213–20. doi: 10.1038/nri2494
45. Rappuoli R. Glycoconjugate Vaccines: Principles and Mechanisms. Sci Transl Med (2018) 10(456):eaat4615. doi: 10.1126/scitranslmed.aat4615
46. Rappuoli R, De Gregorio E, Costantino P. On the Mechanisms of Conjugate Vaccines. Proc Natl Acad Sci USA (2019) 116(1):14–6. doi: 10.1073/pnas.1819612116
47. Dagan R, Poolman J, Siegrist CA. Glycoconjugate Vaccines and Immune Interference: A Review. Vaccine (2010) 28(34):5513–23. doi: 10.1016/j.vaccine.2010.06.026
48. Kis Z, Shattock R, Shah N, Kontoravdi C. Emerging Technologies for Low-Cost, Rapid Vaccine Manufacture. Biotechnol J (2019) 14(1):e1800376. doi: 10.1002/biot.201800376
49. Koeberling O, Ispasanie E, Hauser J, Rossi O, Pluschke G, Caugant DA, et al. A Broadly-Protective Vaccine Against Meningococcal Disease in Sub-Saharan Africa Based on Generalized Modules for Membrane Antigens (GMMA). Vaccine (2014) 32(23):2688–95. doi: 10.1016/j.vaccine.2014.03.068
50. Tennant SM, MacLennan CA, Simon R, Martin LB, Khan MI. Nontyphoidal Salmonella Disease: Current Status of Vaccine Research and Development. Vaccine (2016) 34(26):2907–10. doi: 10.1016/j.vaccine.2016.03.072
51. Haselbeck AH, Panzner U, Im J, Baker S, Meyer CG, Marks F. Current Perspectives on Invasive Nontyphoidal Salmonella Disease. Curr Opin Infect Dis (2017) 30(5):498–503. doi: 10.1097/qco.0000000000000398
52. MacLennan CA, Martin LB, Micoli F. Vaccines Against Invasive Salmonella Disease: Current Status and Future Directions. Hum Vaccin Immunother (2014) 10(6):1478–93. doi: 10.4161/hv.29054
53. Avci FY, Li X, Tsuji M, Kasper DL. A Mechanism for Glycoconjugate Vaccine Activation of the Adaptive Immune System and Its Implications for Vaccine Design. Nat Med (2011) 17(12):1602–9. doi: 10.1038/nm.2535
54. Sun X, Stefanetti G, Berti F, Kasper DL. Polysaccharide Structure Dictates Mechanism of Adaptive Immune Response to Glycoconjugate Vaccines. Proc Natl Acad Sci USA (2019) 116(1):193–8. doi: 10.1073/pnas.1816401115
55. Chen L, Valentine JL, Huang C-J, Endicott CE, Moeller TD, Rasmussen JA, et al. Outer Membrane Vesicles Displaying Engineered Glycotopes Elicit Protective Antibodies. Proceed Natl Acad Sci USA (2016) E3609–18. doi: 10.1073/pnas.1518311113
56. Polonskaya Z, Deng S, Sarkar A, Kain L, Comellas-Aragones M, McKay CS, et al. T Cells Control the Generation of Nanomolar-Affinity Anti-Glycan Antibodies. J Clin Invest (2017) 127(4):1491–504. doi: 10.1172/jci91192
57. Dhara D, Baliban SM, Huo CX, Rashidijahanabad Z, Sears KT, Nick ST, et al. Syntheses of Salmonella Paratyphi A Associated Oligosaccharide Antigens and Development Towards Anti-Paratyphoid Fever Vaccines. Chemistry (2020) 26(68):15953–68. doi: 10.1002/chem.202002401
58. Xu L, Li Z, Su Z, Yang Y, Ma G, Yu R, et al. Development of Meningococcal Polysaccharide Conjugate Vaccine That can Elicit Long-Lasting and Strong Cellular Immune Response With Hepatitis B Core Antigen Virus-Like Particles as a Novel Carrier Protein. Vaccine (2019) 37(7):956–64. doi: 10.1016/j.vaccine.2018.12.073
Keywords: glycoconjugate, GMMA, carrier protein, polysaccharide, vaccine
Citation: Micoli F, Alfini R, Di Benedetto R, Necchi F, Schiavo F, Mancini F, Carducci M, Oldrini D, Pitirollo O, Gasperini G, Balocchi C, Bechi N, Brunelli B, Piccioli D and Adamo R (2021) Generalized Modules for Membrane Antigens as Carrier for Polysaccharides: Impact of Sugar Length, Density, and Attachment Site on the Immune Response Elicited in Animal Models. Front. Immunol. 12:719315. doi: 10.3389/fimmu.2021.719315
Received: 02 June 2021; Accepted: 16 August 2021;
Published: 14 September 2021.
Edited by:
Bertrand Kaeffer, Institut National de recherche pour l’agriculture, l’alimentation et l’environnement (INRAE), FranceReviewed by:
Grégoire Mignot, INRA UMREA4644 Endocrinologie Cellulaire et Moléculaire Immuno, FranceBernd Lepenies, University of Veterinary Medicine Hannover, Germany
Copyright © 2021 Micoli, Alfini, Di Benedetto, Necchi, Schiavo, Mancini, Carducci, Oldrini, Pitirollo, Gasperini, Balocchi, Bechi, Brunelli, Piccioli and Adamo. This is an open-access article distributed under the terms of the Creative Commons Attribution License (CC BY). The use, distribution or reproduction in other forums is permitted, provided the original author(s) and the copyright owner(s) are credited and that the original publication in this journal is cited, in accordance with accepted academic practice. No use, distribution or reproduction is permitted which does not comply with these terms.
*Correspondence: Francesca Micoli, francesca.x.micoli@gsk.com