- 1Centre for Liver and Gastrointestinal Research and National Institute for Health Research (NIHR) Birmingham Biomedical Research Centre, Institute of Immunology and Immunotherapy, University of Birmingham, Birmingham, United Kingdom
- 2Liver Transplant and Hepatobiliary Unit, Queen Elizabeth Hospital, University Hospital of Birmingham NHS Foundation Trust, Birmingham, United Kingdom
- 3Centre for Rare Diseases, European Reference Network - Rare Liver Centre, Birmingham, United Kingdom
- 4Birmingham Advanced Cellular Therapy Facility, University of Birmingham, Birmingham, United Kingdom
- 5Queen Elizabeth Hospital, University Hospital of Birmingham National Health Service (NHS) Foundation Trust, Birmingham, United Kingdom
The tight relationship between the gut and liver on embryological, anatomical and physiological levels inspired the concept of a gut-liver axis as a central element in the pathogenesis of gut-liver axis diseases. This axis refers to the reciprocal regulation between these two organs causing an integrated system of immune homeostasis or tolerance breakdown guided by the microbiota, the diet, genetic background, and environmental factors. Continuous exposure of gut microbiome, various hormones, drugs and toxins, or metabolites from the diet through the portal vein adapt the liver to maintain its tolerogenic state. This is orchestrated by the combined effort of immune cells network: behaving as a sinusoidal and biliary firewall, along with a regulatory network of immune cells including, regulatory T cells and tolerogenic dendritic cells (DC). In addition, downregulation of costimulatory molecules on hepatic sinusoids, hepatocytes and biliary epithelial cells as well as regulating the bile acids chain also play a part in hepatic immune homeostasis. Recent evidence also demonstrated the link between changes in the gut microbiome and liver resident immune cells in the progression of cirrhosis and the tight correlation among primary sclerosing cholangitis (PSC) and also checkpoint induced liver and gut injury. In this review, we will summarize the most recent evidence of the bidirectional relationship among the gut and the liver and how it contributes to liver disease, focusing mainly on PSC and checkpoint induced hepatitis and colitis. We will also focus on completed therapeutic options and on potential targets for future treatment linking with immunology and describe the future direction of this research, taking advantage of modern technologies.
Introduction
The strategic anatomical localization and blood supply from the portal vein links the liver and the gut tightly both anatomically and functionally. A wide variety of commensal microorganisms, microbial and food antigens, or xenobiotics, reach the liver through the portal blood from the spleen and mesenteric veins, exposing the liver to continuous immunogenic stimuli. As a frontline organ facing the influx from gut mucosal barriers, the liver continuously maintains the balance between immunity and tolerance to maintain homeostatic state of human body.
On the other hand, molecules secreted by the liver in the bile reach the gut, to configure a bidirectional crosstalk the so-called gut-liver axis. Molecules that make up the bile salts can shape the gut immune system and regulate the gut microflora (1–3).
Gut Microbiota
The gut microbiome has recently been found to be an active part in the complex relationship between the liver and the gut (4). It is composed of a wide collection of microorganisms, and the dominant phyla are Firmicutes, Actinobacteria, Bacteroidetes, Proteobacteria, Fusobacteria, and Verrucomicrobia, with the Firmicutes and Bacteroidetes representing over 90% of the flora (5). The composition of the gut microflora changes during an individual’s lifespan (6, 7). After birth, the gut is colonized by vertical transmission from the mother to the infant and its maturation and composition is shaped by different factors, such as the method of feeding, the mother’s diet or the exposure to medications (e.g. antibiotics) (8–11). The microbiome composition becomes more stable after the first two years of life and is mostly characterized by Firmicutes and Bacteroides (7).
The gut microbiome has been extensively investigated over the last decade to dissect its physiological function and its association with gut and liver diseases. Although many aspects of the gut microbiome are yet to be clarified, it maintains stability of the gut barrier and the maturation of the mucosal associated immunology, and its primary function is the involvement of a large number of metabolic processes (12–15).
Role of Bile Acids in Gut-Liver Axis
One metabolic process is the metabolism of the biliary acids (BAs): the primary bile acids, namely the cholic acid and the chenodeoxycholic acid, are synthesized and secreted in the bile by the hepatocytes. The BAs are essential for the digestion of the lipids and the fat-soluble vitamins. The metabolism of the BAs is a paradigmatic example of the interaction between the gut and the liver. Primary BAs recirculate in a highly efficient enterohepatic circulation, and only around 10% of the secreted BAs are newly synthesized by the hepatocytes. In fact, a fraction of BAs are reabsorbed passively, whilst most of them are actively reabsorbed through the apical sodium dependent ileal BA transporter expressed by the enterocytes in the terminal ileum. The bile acids bind farnesoid X receptor (FXR) in the enterocyte, promoting the transcription the fibroblast growth factor (FGF 19) (16). FGF 19 binds FGF4 receptor and Klotho-beta, inhibiting the bile acid synthesis (Figure 1). A small proportion of the primary BAs, after reaching the colon, are deconjugated by the microflora into the secondary BAs, lithocholic acid and deoxycholic acid. The former is reabsorbed and after being conjugated in the liver is secreted in the bile (16). An abundance of the deoxycholic-acid affects the microbiota composition and has been related to obesity in non-alcoholic fatty liver disease (17). Thus, new treatment such as obeticholic acid has been shown to be hopeful for NAFLD therapy.
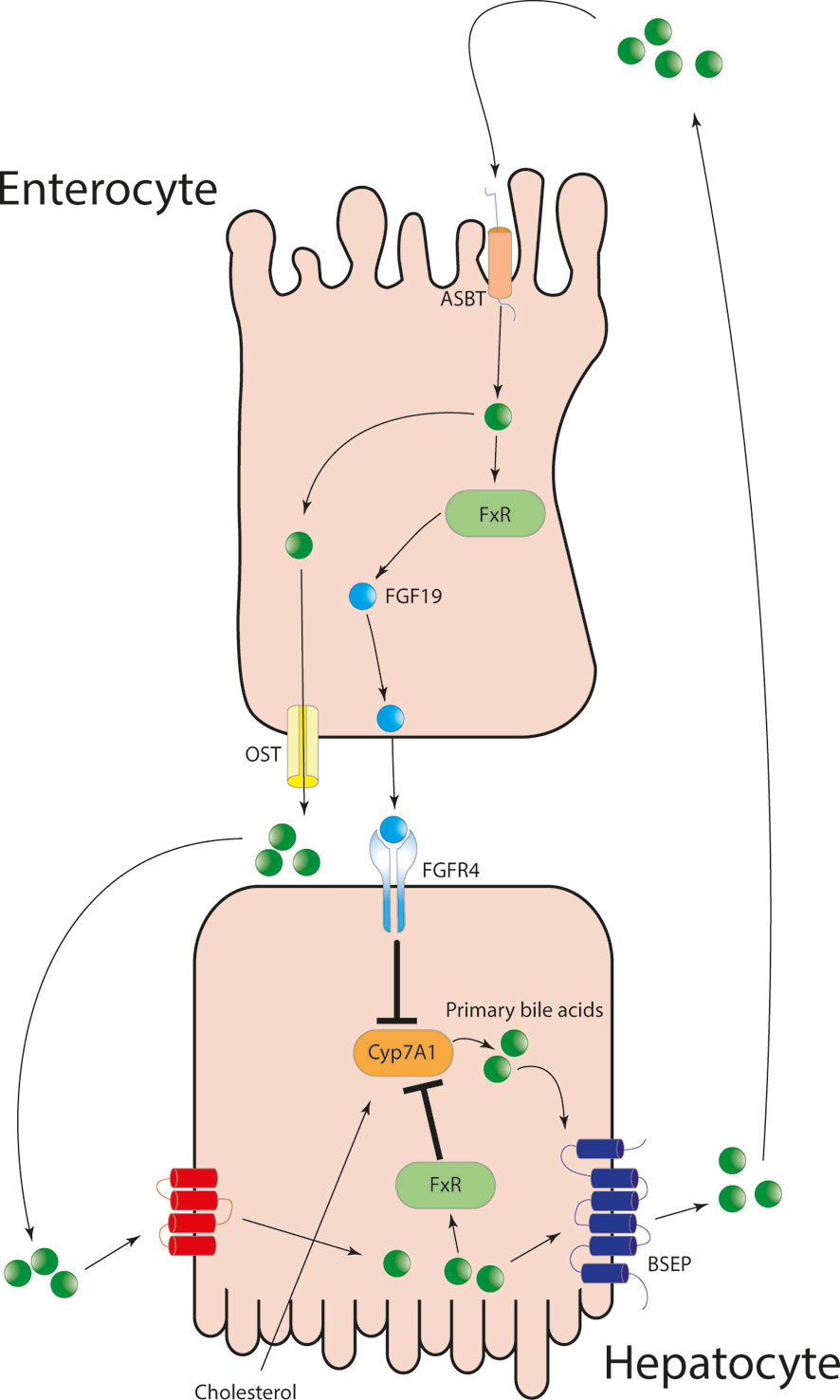
Figure 1 Primary bile acids after being secreted from the hepatocytes into the bile, are reabsorbed in the terminal ileum via the apical-sodium dependent bile acid transporter. The increased intracellular concentration of BAs is sensed via FXR and lead to the production of fibroblast growth factor 19 and to its secretion into the portal circulation. FGF19 binds FGF4 on hepatocytes surface and lead to the downregulation of Cyp7A1 and in turn inhibiting de-novo primary bile salt synthesis.
They exert the metabolic effect upon the activation of nuclear receptors (e.g. farnesoid X receptor, FXR) and G-protein coupled bile salt receptor, TGR5. The activation of FXR has been shown to improve glucose tolerance and insulin resistance in murine models (18, 19). Upon their action on FXR, bile acids can improve glucose metabolism after a meal. The stimulation of FXR and in turn the induction of FGF19, reduce the plasma glucose and induce glycogen synthesis (20). The FXR signaling also inhibits the glucose-induced transcription of several genes involved in glycolysis (21). The metabolic effects of bile acids extend to lipid synthesis. FXR-/- mice show increased triglycerides both in liver and serum, alongside cholesterol. FXR activation reduces hepatic lipogenesis, increases the synthesis of apolipoprtein CII and A5 and inhibits ApoA1 and ApoCIII, thereby promoting the reduction of serum triglycerides activating lipoprotein lipase in very low-density lipoprotein (VLDL) (22, 23). Finally, FXR stimulates fatty acid oxidation inducing human peroxisome proliferator-activated receptor α (PPARα) (24).
TGR5 activation increases basal metabolism and in turn promotes energy expenditure. Secondary bile acids activate TGR5 in brown adipose tissue in mice or in muscle in human, increasing the basal energy consumption (25, 26). This metabolic change prevents obesity and reduce insulin resistance in mice (27). The effect on the glucose metabolism includes the induction of the glucagon like peptide 1 secreted from enteroendocrine L cells, an incretin secreted after the meal in order to regulate insulin secretion (28).
Ultimately, the bile acids binding the farnesoid X receptor, in the enterocyte promote the gut vascular barrier integrity, preventing the translocation of pathogens in the portal circulation (29). Agonist of both these receptors have been proposed as treatment in liver diseases. Obeticholic acid an FXR agonist, has been approved in 2016 as an add on treatment for ursodeoxycholic acid non-responders in primary biliary cholangitis. This medication has been shown to be effective in reducing liver fibrosis (NASH fibrosis) in non-cirrhotic patients (30).
Gut Barrier and Mucosal Immune Response
The gut barrier is a functional unit which prevents bacterial adhesion and controls paracellular trafficking. It is composed, starting from the outer layer, by the gut microbiota, the mucus layer which contains antimicrobial products (such as defensin) and the secretory IgA, the epithelium which is both a physical and immunological barrier and the gut associated lymphoid tissue. A new entity has been recently discovered on the gut defense line, the gut-vascular barrier, known as 4KDa large which prevents the translocation of the bacteria from the gut to the bloodstream (31). Its structure resembles the blood-brain barrier, formed by the endothelial cells linked by tight junctions in close contact with pericytes and fibroblast.
The disruption of the gut barrier alongside an overgrowth of Gram negative bacteria has been linked to multiple liver diseases, as NASH and alcoholic liver disease. Inflammatory mediators such as interferon-gamma or toxic molecules like ethanol can affect the functional integrity of the gut-barrier, causing a “leaky gut” (32, 33). This setting would allow endotoxin, Gram negative bacteria and lipopolysaccharide (LPS) to translocate to the portal system and these inflammatory triggers are postulated to be involved in the pathogenesis of chronic liver diseases.
Hepatic Immunological Response to Gut Permeability
The gut-barrier prevents the translocation of microbial antigen into the portal circulation. Nonetheless, the small part of microbial associated molecular patterns (MAMPs) (e.g., LPS, peptidoglycan or lipoteichoic acid) which eludes the barrier and reaches the liver parenchyma is detected and destroyed before reaching the systemic circulation (34). This is carried out by Kupffer cells which play a major role in the initial immune response creating a sinusoidal firewall against circulating pathogenetic material and bacterial derivatives from the gastrointestinal tract (35). They are anchored to the sinusoidal surface and their protoplasmic processes can insinuate in the endothelial fenestrae to reach the Disse space.
This strategic position of the liver exposes them to a large number of antigens coming from the portal bloodstream. In physiological settings they promote tolerance, reducing the recruitment and activation of T cells including regulatory T cells (Tregs) which control effector T cells via suppression of effector cells proliferation and their cytokine secretions (36, 37). A protective barrier has been shown also on the biliary side and it is represented by the Mucosal Associated Invariant T (MAIT) cells (38). These unconventional T cells express a conserved TCR alpha chain Vα7.2-Jα33 and recognize vitamin B metabolites of microbial origins via the MHC-related molecules 1 (39). Intrahepatic MAIT cells have been localized in the portal area around the bile tract. They have been found to interact with the biliary epithelial cells to protect the biliary mucosa (40). Liver retained MAIT cells express chemokine receptors CCR6 and CXCR6 homing them close to the biliary epithelial cells (BECs) which express and secrete CCL20 and CXCL16. Thus, when exposed to bacterial products, MAITs degranulates, releasing IFN-gamma upon MR1 stimulation to protect the biliary epithelium (40–42).
The protective function of the gut and liver barriers and the tolerogenic response of the liver are vital for a harmless cooperation between the liver and the gut. The failure of the gut barrier in preventing the translocation of microbe associated molecular patterns (MAMPs) exposes the liver to an increased volume of immune stimuli. Hepatic Kupffer cells, hepatic sinusoidal endothelial cells (HSECs) and biliary epithelial cells (BECs), recognize MAMPs via pattern recognition receptors (PRRs) or nucleotide-binding oligomerization domain like receptors (NLR) and in turn shape the immune liver milieu toward an inflammatory status causing a liver injury and eventually progression to fibrosis (43–45).
A “leaky gut”, alongside a reduction in LPS tolerance has been theorized as a central player in chronic inflammation in liver diseases (46). A typical example of this paradigm is the primary sclerosing cholangitis (PSC). PSC is characterized by chronic inflammation and fibrotic destruction of the biliary system. It is frequently associated with inflammatory bowel disease, particularly with ulcerative colitis (47). The extent of the gut inflammation is associated with the grade of the intestine permeability and, in turn, with the bacterial-derived endotoxin in the portal circulation (48). We, hereby describe the immunological cross-talk between the gut and the liver, focusing on PSC compared with PBC and checkpoint induced hepatitis and colitis with particular attention on completed therapeutic attempts.
Checkpoint Inhibitors in Gut-Liver Axis
Checkpoint inhibitors (CPI) are currently used as standard of care treatment for melanoma, non-small cell lung cancer, urothelial malignancy, and prostate cancer. Therapies that target coinhibitory receptors or immune checkpoints inhibitors, CTLA-4, PD1, PDL-1, TIGIT, LAG-3 and TIM3 are currently at the forefront of treatment strategies for cancer. Immune checkpoint inhibitor (ICI) therapy is rapidly developing and is positioned as a crucial treatment within the field of oncology. Although these therapies have improved survival rates of patients carrying various tumors (49, 50), ICIs are also associated with immune-related adverse events and reflect their unique mechanisms of action. These adverse events are known to cause severe colitis and liver injury.
Immune checkpoint blockade with mono-clonal antibodies (mAbs) targeting programmed cell death protein 1 (PD-1) provides long-term clinical benefits to nearly 40% of patients with advanced melanoma (51–54). It is now recognized that ICIs are frequently associated with luminal gastrointestinal side effects such as diarrhea and enterocolitis and hepatic complications such as hepatitis.
Prototype Disease of Gut-Liver Axis: Primary Sclerosing Cholangitis
PSC, a chronic, progressive biliary disease associated with inflammatory bowel disease (IBD), is one of the best examples of immune mediated liver disease related to gut-liver axis. Currently there is neither curative therapy (55) or treatment to slow the progression of PSC to advanced liver disease (56). Genetic studies have shown that HLA class II alleles associated with ulcerative colitis (UC) are different from those found in patients with PSC and patients with PSC and inflammatory bowel diseases (IBD) (57).
While the pathogenesis of PSC has not been fully elucidated, one hypothesis involves the migration of gut mucosal memory lymphocytes to the liver which then causes focal inflammation and fibrosis within the large and/or small bile ducts (58, 59). Current available standard medical therapy only includes ursodeoxycholic acid (UDCA) which does not benefit the overall survival. Liver transplantation remains the only curative therapy in PSC (60, 61). 60-80% of PSC patients have coexistent IBD (62). The phenotype of IBD in PSC has particular characteristics, such as involvement of the entire colon with right-sided dominance, ileal inflammation, and relative rectal sparing. It has been suggested that the IBD associated with PSC is its own distinct entity separate from Ulcerative colitis (UC) or Crohn’s disease (CD) alone (63).
Sufficient epithelial barrier function as well as innate and adaptive immune regulation is required for a lifelong balanced response to dietary antigens as well as for commensal and pathogenic organisms which can infect the small and large intestine. If the immune regulation fails because of changes in environment and lifestyle, the accumulation of common genetic susceptibility variants, chronic intestinal inflammation can arise. Inflammatory bowel disease (IBD) encompasses Crohn’s disease (CD) and ulcerative colitis (UC). IBD is characterized by chronic relapsing disease activity of acute flares and intervals of remission (64, 65).
One hypothesis to explain the co-occurrence of IBD and PSC due to the interaction between the gut and the liver is known as ‘the aberrant gut homing lymphocyte hypothesis’ (58). This hypothesis describes an aberrant expression of gut homing molecules such as CCL25 and MadCAM-1 in PSC liver, leading to homing of CCR9 and α4β7 expressing gut-primed CD8 memory T-cells into the liver. In patients with PSC-IBD, there is a recurrence of PSC following transplantation if small bowel or colon is still intact (66). This would explain the mechanism for T cell trafficking between the gut towards the liver supporting the important mechanism of liver-gut immune axis in these diseases (67). Current standard of care treatment for IBD not only includes immunosuppressive medication and biologic therapies such as anti-TNF therapies (infliximab), α4β7 blockade (Vedolizumab) is now routinely used for IBD patients to prevent mucosal T cell homing (68). Sequencing of the TCRβ repertoire data demonstrated that memory T cells of common clonal origin were detected in paired gut and liver samples of patients with PSC-IBD. This suggests that memory T cells driven by shared antigens are present in the gut and liver of PSC-IBD patients (69). Therefore, therapeutical targeting memory T cell recruitment in PSC-IBD with Vedolizumab is a beneficial therapy.
‘PSC microbiota’ or the ‘leaky gut’ theory implies that the pathogenic gut microbiota crosses through the inflamed gut wall into the portal circulation, and then into the biliary tree, leading to PSC (70). We currently have fecal microbiome treatment for IBD patients and this will be extended to PSC patients in the near future.
Primary Biliary Cholangitis: Is There a Gut-Liver Link?
Primary biliary cholangitis (PBC) is a chronic autoimmune cholestatic liver disease. The immune damage targets the small and medium intrahepatic biliary ducts, causing a progressive damage which eventually leads to fibrosis and cirrhosis (71). The PBC pathogenesis is still enigmatic and the current hypothesis is that environmental factors (e.g. infections) can start the process in individuals with a genetic predisposition (72).
A damage in the gut barrier in PBC has been hypothesized as a pathogenetic factor. The higher permeability of the small intestine shown in PBC (73, 74), might be at the basis of the increased LPS levels observed in PBC patients compared with healthy controls (75). Intriguingly, long-standing exposure to bacterial antigens causes the development of PBC-like histological features in BALB/c mice (76). Altogether these evidences suggest a failure in gut barrier in PBC patients.
The interest around this pathogenic angle has recently increased as well as the effort to investigate the microbiome in PBC (77–79). Microbiome alpha diversity is reduced in PBC and it shows a distinct beta diversity compared with healthy controls due to the increased of the genera Streptococcus, Lactobacillus, and Bifidobacterium (80). The interplay between bile acids metabolism and microbiome in PBC has been nicely elucidated by Chen and colleagues. They showed how the BA composition in serum and feces significantly differ in UDCA-naïve PBC patients compared with healthy controls. PBC BA pool was associated with a decreased conversion of conjugated and unconjugated, and primary to secondary BAs. These results suggest an impaired microbial BA metabolism. This effect appears to be related with the severity of the disease, as the BA abnormality correlates positively with the disease stage (77). The first line of treatment in PBC is ursodeoxicholic acid (UDCA) which seems, to have a role in restoring the gut microbiome in PBC patients (80).
Inflammatory Bowel Disease and PSC
Chemokines and chemokine receptors crosstalk are essential in gut-liver immune traffic. Lymphocyte recruitment to the gut from the circulation involves a range of chemokines and adhesion molecules. Chemokine CCL25 aids in the trans-endothelial migration of gut-homing T-cells, so enhanced expression will promote infiltration of T cells. Expression of CCL25 is normally confined to the epithelium and mucosal vessels in the small intestine (81), where it interacts with gut-homing B and T cells expressing its receptor, CCR9 (Figure 2). In the inflamed liver there have been reporting’s of strong expression of CCL25 on the hepatic vessels (47). Although CCL25 is largely absent from the non-inflamed human colon, expression is markedly upregulated in colitis and correlates with inflammatory activity (84). CCL25 expression in the colon is associated with high frequencies of CCR9+ tissue-infiltrating effector T-cells in patients with colitis. Thus, CCR9 and CCL25 interactions drive the recruitment of mucosal effector cells in the gut as well as the liver in patients with ulcerative colitis (84). Once an antigen is recognized by gut dendritic cells, they imprint naïve T cells with gut specific chemokine receptor CCR9 and integrin, α4β7 (85). These primed T cells subsequently migrate back to liver (47, 83, 86). Deamination of methylamine by vascular adhesion protein-1 (VAP-1) - semicarbazide sensitive amine oxidase found in the human liver – induces the expression of function MAsCAM-1 in hepatic endothelial cells and intact human liver tissue ex-vivo. This is associated with increased adhesion of lymphocytes from patients with PSC to hepatic vessels (87). It has been reported that there is an aberrant expression of the gut-specific molecules MAdCAM-1 and CCL25 in human PSC livers (47, 59, 88). This correlates with α4β7+CCR9+effector memory T-cells which constitute approximately 20% of the liver-infiltrating lymphocytes in PSC patients (47). This would explain the recurrence of PSC after liver transplantation in patients with intact inflamed colon.
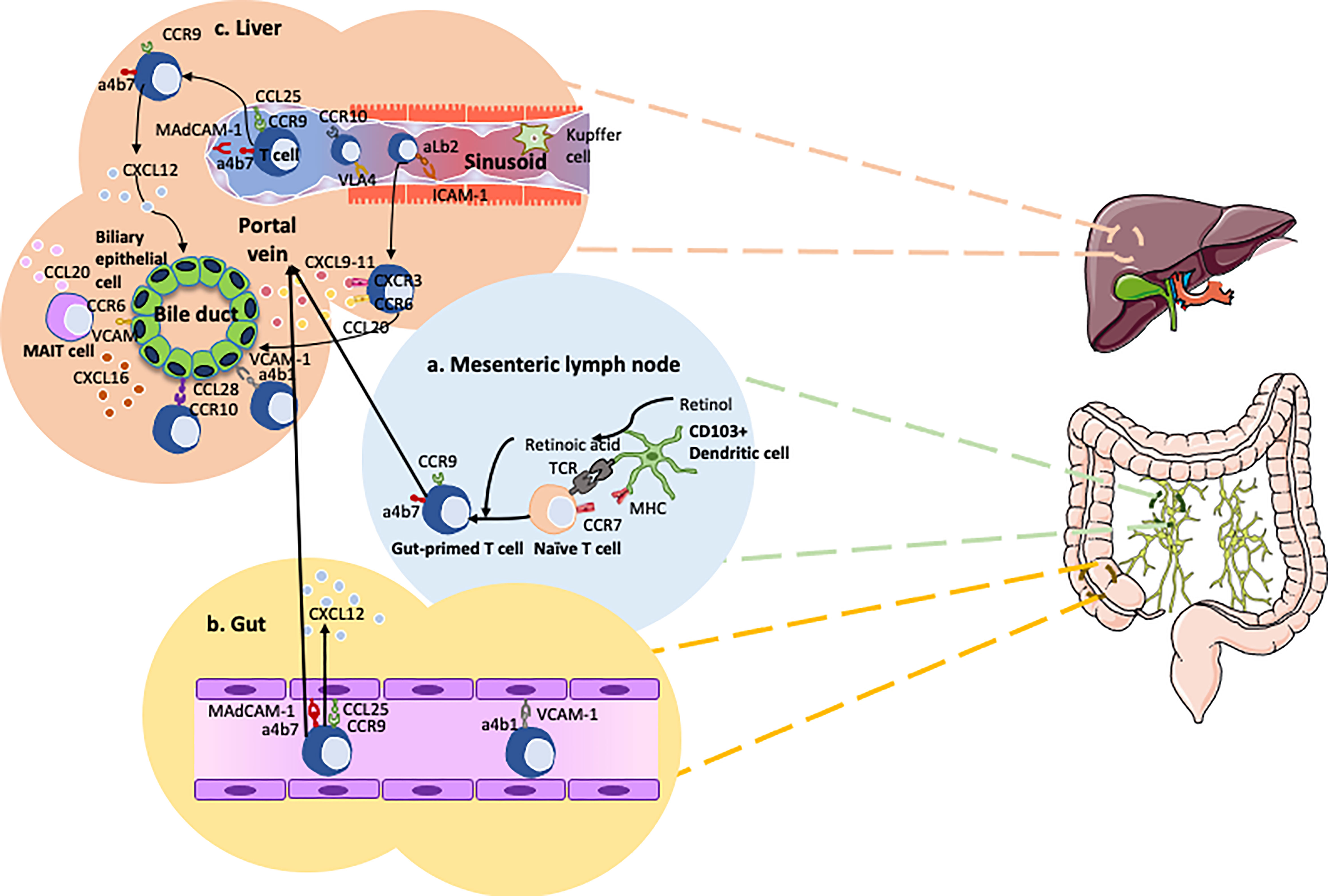
Figure 2 Overview of the gut-liver axis homing of cells in primary sclerosing cholangitis (PSC). (A) Dendritic cells recognize pathogens penetrating the mucosal barrier and subsequently migrate to the mesenteric lymph node to present their antigens to naïve T cells (82). These naive T cells are imprinted with the gut-homing integrin α4β7 and chemokine receptor CCR9 via the transformation of retinol into retinoic acid. (B) The gut-primed T cells recirculate into the gut bind onto the endothelium of the blood vessels via interactions between MAdCAM-1 and α4β7, CCL25 and CCR9. (C) CCR10 expressing Tregs and gut-primed memory T cells expressing α4β7 and CCR9 migrate from the gut to the liver via the portal vein. These concepts are applied in PSC to develop new therapies such as Vedolizumab. Chemokine CXCL12 is thought to play a role in maintaining CCR9+ lymphocytes around the bile ducts (83).
Due to MAdCAM-1 expression by portal endothelium coupled with the presence of chemokine CCL28 and integrin α1β7 around the bile ducts, the inflammatory trigger in PSC originates in the portal area e.g. in the bile ducts, thereby provoking cholangitis and not hepatitis (89). Krijger et al. demonstrated the expression of CCL28 in PSC liver (89). CCL28 is also upregulated in the epithelium of the colon during inflammation, subsequently attracting T and B cells expressing CCR10 (90). Studies using flow-based adhesion assays demonstrate CCL28 is able to trigger α4β7-dependent lymphocyte arrest (83). In addition, VCAM-1 expression by cholangiocytes promotes the survival of intrahepatic α4β7 expressing effector T lymphocytes subsequently contributing to the persistent inflammation (91).
To translate our knowledge of discovery science to clinics, several compounds have been developed to tackle and block the migration of gut-homing mucosal lymphocytes to treat patients with IBD and PSC. These compounds block α4β7 (vedolizumab, abrilumab), integrin β7 (etrolizumab), and MAdCAM-1 (ontamalimab). Although studies have focused predominantly on the treatment of IBD with these compounds, their potential to block trafficking to the liver suggests they could also be a future treatment option for PSC-IBD and also for inflamed bowel even after liver transplantation (92, 93).
Translating Immunology to Therapy: Vedolizumab in PSC and IBD
Vedolizumab is a humanized monoclonal antibody that binds specifically to the α4β7 integrin, a mediator of gastrointestinal inflammation (94, 95). Blocking α4β7 on memory T-helper lymphocytes by vedolizumab inhibits the adhesion of cells to MAdCAM-1 which is expressed on inflamed portal vein endothelium in patients with IBD and PSC. The inhibition of this interaction prevents the transmigration of gut-homing memory T cells across the vascular endothelium leading to reduction in inflammation (96, 97). Safety and efficacy of vedolizumab has been demonstrated in large-scale GEMINI clinical trials in both Crohn’s disease (CD) and ulcerative colitis (UC). Clinical remission rates of 39% and 42% was achieved with vedolizumab in both CD and UK respectively, and this effect was maintained for a year (98, 99). This led to the approval of vedolizumab by the UK Food and Drug Administration (FDA) and European Medical Agency (EMA) in 2014 to treat CD and UC. Studies since its approval have proven its efficacy [e.g., the VERSIFY study (100)] and the efficacy of early versus late use in CD [LOVE-CD trial (101)]. Cohort studies have also demonstrated improved disease control, corticosteroid sparing and a decline in concomitant drugs, with a significant proportion of patients remaining on vedolizumab by the end of the observation period (102).
Several studies have suggested that the gut-homing pathways that vedolizumab targets is implicated in the pathophysiology of PSC (47, 59, 103). Therefore, vedolizumab may play a role in reducing lymphocyte infiltration in PSC and thereby in reducing hepatic and biliary inflammation. However, there have been varied findings on the efficacy of vedolizumab in PSC. Researchers have shown that vedolizumab therapy did not significantly improve markers of biliary inflammation (104, 105), however, vedolizumab can induce clinical remission in rheumatologic extraintestinal manifestations of IBD (106). Patients with more aggressive disease, such as the presence of cirrhosis and potentially those with a raised ALP at baseline, were more likely to respond (107).
Manipulating Gut Microbiome With Vancomycin in PSC
The microbiota plays an important role in the pathogenesis and the progression of PSC. This concept was applied recently to treat patients with PSC. Antibiotics, such as vancomycin, was utilized in clinical trials to modify intestinal microbiota flora. Trial data demonstrated that vancomycin could improve liver biochemistry in PSC patients (108). This evidence suggested that disease progression in PSC can be modified by antibiotic therapies.
Vancomycin is a glycopeptide antibiotic with bactericidal activity against Gram positive bacteria (109) which includes various Clostridium spp. known to be primarily involved with the dihydroxylation of primary bile acids, into the secondary bile acids present in the distal small intestine and colon (110, 111). Secondary bile acids are highly hydrophobic and toxic, and increased concentrations in the liver have been linked to inflammation, cholestasis and carcinogenesis (112). Therefore, vancomycin could influence bile acid metabolism. A meta-analysis study by Shah et al. exploring the effects of antibiotics vancomycin and metronidazole in PSC patients demonstrated that overall antibiotic therapy significantly improved primary outcome measure alkaline phosphatase enzyme level. The use of vancomycin in patients with PSC have not only biochemical benefits but also cholangiography and histological improvements to PSC and decreased intestinal inflammation of the IBD on colonic biopsies (113). Multiple studies have reported similar results in both adults and children with PSC (108, 114–116) supporting that modification of intestinal microbiota has beneficial effects by changing gut-liver immune dynamics.
Potential Role of Regulatory T Cell Therapy in PSC
Tregs are crucial to maintain control of the magnitude of immune responses to self-antigens and to limit tissue damage caused by immune activation in response to these antigens (117). The immune-regulatory function is impaired in autoimmune or inflammatory diseases. Thus, Treg therapy in PSC is worth exploring as a potentially curative therapy. IL-2 infusion therapy to expand Tregs in vivo has been investigated in mouse models of cholangitis reporting a 5-fold increase in hepatic Tregs, and cells are localized around the inflamed portal tracts. The increase in Tregs subsequently resulted in reduction of pro-inflammatory IL-17 cytokines and increase in anti-inflammatory IL-10 production by hepatic lymphocytes. Low dose IL-2 infusion therapies have been carried out in human autoimmune diseases (118) and a majority of findings resulted in a decrease in disease severity. Although this has not yet been trialed in autoimmune liver diseases, the current mouse and human data suggest IL-2 infusion alone may not be enough to decrease the disease severity but should be considered in combination with Treg infusion therapies.
Thus, vendolizumab, vanomycin and Treg therapy are the current in-trial strategies with described benefits in aspects of the gut-liver injury related to PSC and IBD. However, further trials are needed to prove their beneficial effects in a high percentage of patients. However, not all patients respond to a particular treatment and therefore it is important that we have a variety of treatments to have the best possible chance to help every patient. Therefore, it is also important that we continue to explore new therapies as well as progressing those that are currently looking promising in the trail phases.
Immune Mediated Gut-Liver Injury: Checkpoint Inhibitor Induced Colitis and Hepatitis
Checkpoint Inhibitors Induced Hepatitis
It is not uncommon that checkpoint inhibitors lead to untoward immune mediated injury including hepatitis and colitis. In a study looking at 414 patients undergoing treatment with CPI, 28 (6.8%) were diagnosed with immune related hepatitis (119). The rate of severe acute hepatitis was similar in patients treated with anti-CTLA-4 and patients treated with anti-PD1/PD-L1. Immune related hepatitis is one of the highest occurring adverse event associated with immunotherapy, especially when anti-CTLA-4 and anti-PD1/PD-L1 are prescribed as a combination therapy (120, 121). Immunological changes resulting from CPI drugs may contribute to the immune related hepatitis. Peripheral CD8 T cells in CPI induced hepatitis showed elevated activation/cytotoxicity and is associated with peripheral monocyte activation (122). CD8 T cells/macrophage were also shown to aggregate in CPI induced hepatitis patients shown using immunohistochemistry staining.
Check Point Inhibitors Induced Colitis
Check point inhibitors can lead to colitis as an adverse effect. Recent summary work on colitis suggested that most cancer patients were treated with CTLA-4 inhibitors, followed by PD-1 inhibitors, or combination therapy. A study on a total of 226 patients with CPI-induced colitis suggested that these patients were treated with steroids (oral or intravenous). Because CPI induced injury is an immune mediated event, it responds to immunosuppressive therapy and biologic treatment which targets the immune pathway. Of these, 61% responded to steroids alone whilst 47% required further treatment with anti-TNF, infliximab. 94% of patients treated with infliximab had resolution of colitis. 8 patients were treated with vedolizumab after steroid failure and all of these patients had resolution (123). Thus, global immunosuppression with steroid, manipulating TNF and blocking α4β7 on memory T-helper lymphocytes are current approach of treatment is these cases. Excitingly, new research focuses on understanding the role of modifying microbiome in the gut and also to enhance the regulatory arm of the immune system to treat CPI induced colitis and hepatitis (Figure 3).
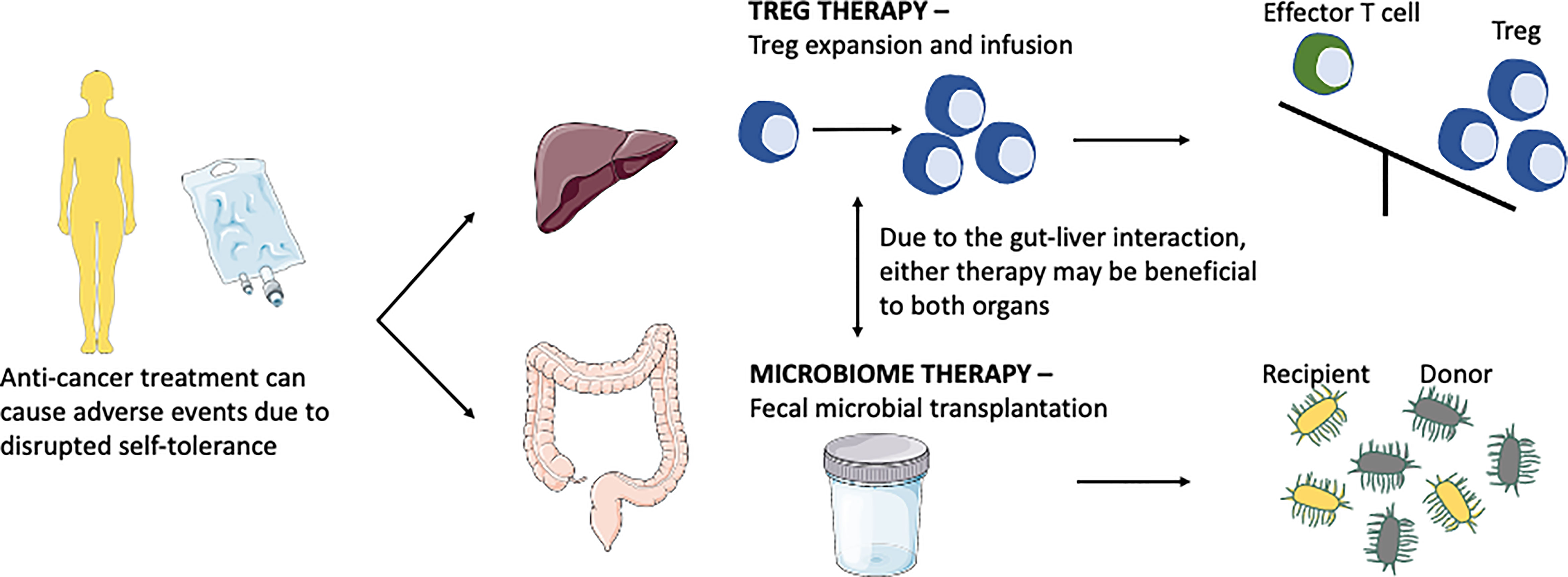
Figure 3 Anti-cancer treatment disrupts self-tolerance leading to colitis and hepatitis, current research is focusing on microbiome therapy and Treg therapy to combat these diseases. Treg therapy aims to switch the balance from the effector cells arm to the Treg arm. Microbiome therapy via fecal microbial transplantation aims to modulate the microbiome by direct interaction or competition leading to host immunity modulation.
Potential of Fecal Microbiome Therapy CPI-Induced Colitis and Hepatitis
Microbiome-targeted therapies manipulate the gut micro flora and can be considered in several categories, namely probiotics, synbiotic, antibiotics and fecal microbiome therapy (FMT). Probiotics are defined as a culture of living microorganisms which could have health benefits for the human if consumed in adequate amounts (124). Synbiotics are a combination of probiotics and prebiotics, in which prebiotics are composed of fermentable dietary fibers that stimulate the growth and survival of probiotics (124). Vancomycin is a typical example of antibiotic which change gut flora.
FMT is a procedure in which stool is collected from a healthy donor and transferred to a patient, via a range of delivery routes including colonoscopy, nasogastric tube, and enema (125). The gut microbiome is a major regulator of responses to anti-PD-1 (126–128). In mice, composition of the gut microbiome modulates therapeutic activity and anti-PD-1 and anti-programmed death-ligand 1(PD-L1), and administration of FMT promotes anti-PD-1 efficacy in melanoma bearing mice (129–131). A single FMT administered colonoscopically together with PD-1 blockade successfully colonized the gut of patients and reprogrammed the tumor microenvironment to overcome primary resistance to anti-PD-1 in a subset of patients with advanced melanoma (132).
The STOP-COLITIS trial (lead by University of Birmingham) is currently ongoing for FMT therapy in non-CPI colitis and the data is encouraging. A recent study applying FMT to treat 2 cases of CPI-colitis demonstrated that it is effective in a subset of patients. Although both patients received stool from the same donor, they experienced contrasting treatment responses. One patient had a high level of activation (as measured by co-expression of HLA-DR and CD38) on CD4, CD8 and MAIT cell subsets as well as high Ki-67 and low expression of Bcl-2 before FMT treatment. Post FMT, there was reduced expression of HLA-DR and CD38 and lower expression of Ki-67 with high/homeostatic levels of Bcl-2 and an increased proportion of Tregs (133). Thus, actual mechanism and predictor of FMT treatment still requires further investigations.
In the context of liver disease and FMT application, the gut microbiota has been implicated as a central factor in the pathophysiology of PSC. Commensal microbiota and its metabolites protect against biliary injury (134). PSC patients not only display a characteristic microbial signature but also have inflammatory bowel disease, thereby suggesting that manipulation of the gut microbiota could potentially influence the disease process in PSC. Thus, FMT therapy to treat gut-liver disease such as PSC is a new and upcoming therapeutic option. One pilot study conducted by Allegretti et al. demonstrated the safety of FMT in 10 patients with PSC, they also suggested that the subsequent increases in bacterial diversity and engraftment may correlate with an improvement in Alkaline phosphatase enzyme among patients with PSC (135).
Future Directions
Understanding the immunological link between the gut and the liver is essential for the success of curative therapies to treat related diseases. Current treatment for IBD patients is hampered by side effects such as myelotoxicity, sepsis, or reactivation of opportunistic infections. To date, there is no treatment in PSC. In addition, only global immune suppression with steroids is generally applied for checkpoint induced hepatitis and colitis in Oncology arena. Thus, dissecting immune pathway is crucial in targeted and organ-specific personalized therapy based on pathogenic mechanisms. New technology including single cell genomic, proteomic, metabolomic, microbiome (Multi-OMICs) profile of patients will guide us to apply treatment as a stratified medicine approach with reduced side effects. FMT therapy currently seems to be the most promising but future studies with substaintial patient numbers are needed to confirm that this is a beneficial treatment. For this to be an effective and curative therapy, exploring the tissue resident cell gene, protein and functional signatures and understanding their microenvironment is an absolute requisite. Application of modern techniques such as single cell RNA sequencing, adaptive cells clonotype and spatial gene signature expression of gut-liver immune mediated diseases would guide us to achieve a personalized medicine approach in future.
Author Contributions
AB conceptualized, collected the data, wrote, and edited the manuscript as well as creating the illustrations. VR conceptualized wrote, and edited the manuscript. DO-B contributed to the writing of the manuscript. YO conceptualized and edited the manuscript and supervised the work. All authors contributed to the article and approved the submitted version.
Funding
Transbioline Innovative Medicine Initiative, Sir Jules Thorn Biomedical Research Award, Medical Research Foundation, European Association of Study of Liver Disease Juan Rodes Fellowship, NIHR BRC Birmingham, GSK, Queen Elizabeth Hospital Birmingham Charity.
Conflict of Interest
The authors declare that the research was conducted in the absence of any commercial or financial relationships that could be construed as a potential conflict of interest.
Publisher’s Note
All claims expressed in this article are solely those of the authors and do not necessarily represent those of their affiliated organizations, or those of the publisher, the editors and the reviewers. Any product that may be evaluated in this article, or claim that may be made by its manufacturer, is not guaranteed or endorsed by the publisher.
Acknowledgments
All figures were created using ServierMedical Art templates, which are licensed under a Creative Commons Attribution 3.0 Unported License; https://smart.servier.com.
References
1. Schaap FG, van der Gaag NA, Gouma DJ, Jansen PLM. High Expression of the Bile Salt-Homeostatic Hormone Fibroblast Growth Factor 19 in the Liver of Patients With Extrahepatic Cholestasis. Hepatology (2009) 49:1228–35. doi: 10.1002/hep.22771
2. Hang S, Paik D, Sloan Devlin A, Jamma T, Lu J, Ha S, et al. Bile Acid Metabolites Control Th17 and Treg Cell Differentiation. bioRxiv (2019) 576:143–8. doi: 10.1101/465344
3. Hang S, Paik D, Yao L, Kim E, Jamma T, Lu J, et al. Bile Acid Metabolites Control Th17 and Treg Cell Differentiation. Nature (2020) 576:143–8. doi: 10.1038/s41586-019-1785-z.Bile
4. Wang R, Tang R, Li B, Ma X, Schnabl B, Tilg H. Gut Microbiome, Liver Immunology, and Liver Diseases. Cell Mol Immunol (2021) 18:4–17. doi: 10.1038/s41423-020-00592-6
5. Arumugam M, Raes J, Pelletier E, Le Paslier D, Yamada T, Mende DR, et al. Enterotypes of the Human Gut Microbiome. Nature (2011) 473:174–80. doi: 10.1038/nature09944
6. Bäckhed F, Roswall J, Peng Y, Feng Q, Jia H, Kovatcheva-Datchary P, et al. Dynamics and Stabilization of the Human Gut Microbiome During the First Year of Life. Cell Host Microbe (2015) 17:690–703. doi: 10.1016/j.chom.2015.04.004
7. Yatsunenko T, Rey FE, Manary MJ, Trehan I, Dominguez-Bello MG, Contreras M, et al. Human Gut Microbiome Viewed Across Age and Geography. Nature (2012) 486:222–7. doi: 10.1038/nature11053
8. Pannaraj PS, Li F, Cerini C, Bender JM, Yang S, Rollie A, et al. Association Between Breast Milk Bacterial Communities and Establishment and Development of the Infant Gut Microbiome. JAMA Pediatr (2017) 171:647–54. doi: 10.1001/jamapediatrics.2017.0378
9. Cho I, Yamanishi S, Cox L, Methé BA, Zavadil J, Li K, et al. Antibiotics in Early Life Alter the Murine Colonic Microbiome and Adiposity. Nature (2012) 488:621–6. doi: 10.1038/nature11400
10. Dawson-Hahn EE, Rhee KE. The Association Between Antibiotics in the First Year of Life and Child Growth Trajectory. BMC Pediatr (2019) 19:1–9. doi: 10.1186/s12887-018-1363-9
11. Saari A, Virta LJ, Sankilampi U, Dunkel L, Saxen H. Antibiotic Exposure in Infancy and Risk of Being Overweight in the First 24 Months of Life. Pediatrics (2015) 135:617–26. doi: 10.1542/peds.2014-3407
12. Kau AL, Ahern PP, Griffin NW, Goodman AL, Gordon JI. Human Nutrition, the Gut Microbiome and the Immune System. Nature (2011) 474:327–36. doi: 10.1038/nature10213
13. Kabouridis PS, Lasrado R, McCallum S, Chng SH, Snippert HJ, Clevers H, et al. The Gut Microbiota Keeps Enteric Glial Cells on the Move; Prospective Roles of the Gut Epithelium and Immune System. Gut Microbes (2015) 6:398–403. doi: 10.1080/19490976.2015.1109767
14. Wells JM, Brummer RJ, Derrien M, MacDonald TT, Troost F, Cani PD, et al. Homeostasis of the Gut Barrier and Potential Biomarkers. Am J Physiol - Gastrointest Liver Physiol (2017) 312:G171–93. doi: 10.1152/ajpgi.00048.2015
15. Carmody RN, Turnbaugh PJ. Host-Microbial Interactions in the Metabolism of Therapeutic and Diet-Derived Xenobiotics. J Clin Invest (2014) 124:4173–81. doi: 10.1172/JCI72335
16. Di Ciaula A, Garruti G, Baccetto RL, Molina-Molina E, Bonfrate L, Wang DQH, et al. Bile Acid Physiology. Ann Hepatol (2017) 16:s4–14. doi: 10.5604/01.3001.0010.5493
17. Janssen AWF, Houben T, Katiraei S, Dijk W, Boutens L, van der Bolt N, et al. Modulation of the Gut Microbiota Impacts Nonalcoholic Fatty Liver Disease: A Potential Role for Bile Acids. J Lipid Res (2017) 58:1399–416. doi: 10.1194/jlr.M075713
18. Prawitt J, Abdelkarim M, Stroeve JHM, Popescu I, Duez H, Velagapudi VR, et al. Farnesoid X Receptor Deficiency Improves Glucose Homeostasis in Mouse Models of Obesity. Diabetes (2011) 60:1861–71. doi: 10.2337/db11-0030
19. Trauner M. Bile Acids as Regulators of Hepatic Lipid and Glucose Metabolism. liver Metab Syndr (2009) 28:220–4. doi: 10.1159/000282091
20. Kir S, Beddow SA, Samuel VT, Miller P, Previs SF, Suino-Powell K, et al. FGF19 as a Postprandial, Insulin-Independent Activator of Hepatic Protein and Glycogen Synthesis. Sci (80-) (2011) 331:1621–4. doi: 10.1126/science.1198363
21. Caron S, Huaman Samanez C, Dehondt H, Ploton M, Briand O, Lien F, et al. Farnesoid X Receptor Inhibits the Transcriptional Activity of Carbohydrate Response Element Binding Protein in Human Hepatocytes. Mol Cell Biol (2013) 33:2202–11. doi: 10.1128/mcb.01004-12
22. Clarke SF, Murphy EF, Nilaweera K, Ross PR, Shanahan F, O’Toole PW. The Gut Microbiota and its Relationship to Diet and Obesity: New Insights. Gut Microbes (2012) 3:186–202. doi: 10.4161/gmic.20168
23. Sinal CJ, Tohkin M, Miyata M, Ward JM, Lambert G, Gonzalez FJ. Targeted Disruption of the Nuclear Receptor FXR/BAR Impairs Bile Acid and Lipid Homeostasis. Cell (2000) 102:731–44. doi: 10.1016/S0092-8674(00)00062-3
24. Torra P, Claudel T, Duval C, Kosykh V, Fruchart J, Staels B. Bile Acids Induce the Expression of the Human Peroxisome Proliferator-Activated Receptor {Alpha} Gene via Activation of the Farnesoid X Receptor. Mol Endocrinol (2003) 17:259–72. doi: 10.1210/me.2002-0120
25. Fiorucci S, Mencarelli A, Palladino G, Cipriani S. Bile-Acid-Activated Receptors: Targeting TGR5 and Farnesoid-X-Receptor in Lipid and Glucose Disorders. Trends Pharmacol Sci (2009) 30:570–80. doi: 10.1016/j.tips.2009.08.001
26. Pols TWH, Noriega LG, Nomura M, Auwerx J, Schoonjans K. The Bile Acid Membrane Receptor TGR5 as an Emerging Target in Metabolism and Inflammation. J Hepatol (2011) 54:1263–72. doi: 10.1016/j.jhep.2010.12.004
27. Svensson PA, Olsson M, Andersson-Assarsson JC, Taube M, Pereira MJ, Froguel P, et al. The TGR5 Gene is Expressed in Human Subcutaneous Adipose Tissue and is Associated With Obesity, Weight Loss and Resting Metabolic Rate. Biochem Biophys Res Commun (2013) 433:563–6. doi: 10.1016/j.bbrc.2013.03.031
29. Sorribas M, Jakob MO, Yilmaz B, Li H, Stutz D, Noser Y, et al. FXR Modulates the Gut-Vascular Barrier by Regulating the Entry Sites for Bacterial Translocation in Experimental Cirrhosis. J Hepatol (2019) 71:1126–40. doi: 10.1016/j.jhep.2019.06.017
30. Jevan NTBALRSA, Amhameed NMLAMFC. Farnesoid X Nuclear Receptor Ligand Obeticholic Acid for non-Cirrhotic, non-Alcoholic Steatohepatitis (Flint): A Multicentre, Randomised, Placebo-Controlled Trial. Lancet (2015) 385:956–65. doi: 10.1016/S0140-6736(14)61933-4
31. Spadoni I, Zagato E, Bertocchi A, Paolinelli R, Hot E, Di Sabatino A, et al. A Gut-Vascular Barrier Controls the Systemic Dissemination of Bacteria. Sci (80-) (2015) 350:830–4. doi: 10.1126/science.aad0135
32. Mouries J, Brescia P, Silvestri A, Spadoni I, Sorribas M, Wiest R, et al. Microbiota-Driven Gut Vascular Barrier Disruption is a Prerequisite for non-Alcoholic Steatohepatitis Development. J Hepatol (2019) 71:1216–28. doi: 10.1016/j.jhep.2019.08.005
33. Miyake Y, Yamamoto K. Role of Gut Microbiota in Liver Diseases. Hepatol Res (2013) 43:139–46. doi: 10.1111/j.1872-034X.2012.01088.x
34. Di Ciaula A, Baj J, Garruti G, Celano G, De Angelis M, Wang HH, et al. Liver Steatosis, Gut-Liver Axis, Microbiome and Environmental Factors. A Never-Ending Bidirectional Cross-Talk. J Clin Med (2020) 9:2648. doi: 10.3390/jcm9082648
35. Balmer ML, Slack E, De Gottardi A, Lawson MAE, Hapfelmeier S, Miele L, et al. The Liver may Act as a Firewall Mediating Mutualism Between the Host and its Gut Commensal Microbiota. Sci Transl Med (2014) 6:1–11. doi: 10.1126/scitranslmed.3008618
36. You Q, Cheng L, Kedl RM, Ju C. Mechanism of T Cell Tolerance Induction by Murine Hepatic Kupffer Cells. Hepatology (2008) 48:978–90. doi: 10.1002/hep.22395
37. Breous E, Somanathan S, Vandenberghe LH, Wilson JM. Hepatic Regulatory T Cells and Kupffer Cells are Crucial Mediators of Systemic T Cell Tolerance to Antigens Targeting Murine Liver. Hepatology (2009) 50:612–21. doi: 10.1002/hep.23043
38. Provine NM, Klenerman P. MAIT Cells in Health and Disease. Annu Rev Immunol (2020) 38:203–28. doi: 10.1146/annurev-immunol-080719-015428
39. Kjer-Nielsen L, Patel O, Corbett AJ, Le Nours J, Meehan B, Liu L, et al. MR1 Presents Microbial Vitamin B Metabolites to MAIT Cells. Nature (2012) 491:717–23. doi: 10.1038/nature11605
40. Jeffery HC, Hunter S, Humphreys EH, Bhogal R, Wawman RE, Birtwistle J, et al. Bidirectional Cross-Talk Between Biliary Epithelium and Th17 Cells Promotes Local Th17 Expansion and Bile Duct Proliferation in Biliary Liver Diseases. J Immunol (2019) 203:1151–9. doi: 10.4049/jimmunol.1800455
41. Jeffery HC, Van Wilgenburg B, Kurioka A, Parekh K, Stirling K, Roberts S, et al. Biliary Epithelium and Liver B Cells Exposed to Bacteria Activate Intrahepatic MAIT Cells Through MR1. J Hepatol (2016) 64:1118–27. doi: 10.1016/j.jhep.2015.12.017
42. Walker LJ, Kang YH, Smith MO, Tharmalingham H, Ramamurthy N, Fleming VM, et al. Human MAIT and CD8αα Cells Develop From a Pool of Type-17 Precommitted CD8 + T Cells. Blood (2012) 119:422–33. doi: 10.1182/blood-2011-05-353789
43. Seki E, Schnabl B. Role of Innate Immunity and the Microbiota in Liver Fibrosis: Crosstalk Between the Liver and Gut. J Physiol (2012) 590:447–58. doi: 10.1113/jphysiol.2011.219691
44. Deng M, Scott MJ, Loughran P, Gibson G, Sodhi C, Watkins S, et al. Lipopolysaccharide Clearance, Bacterial Clearance, and Systemic Inflammatory Responses Are Regulated by Cell Type–Specific Functions of TLR4 During Sepsis. J Immunol (2013) 190:5152–60. doi: 10.4049/jimmunol.1300496
45. Hoque R, Vodovotz Y, Mehal W. Therapeutic Strategies in Inflammasome Mediated Diseases of the Liver. J Hepatol (2013) 58:1047–52. doi: 10.1016/j.jhep.2012.12.017
46. Knolle PA, Wohlleber D. Immunological Functions of Liver Sinusoidal Endothelial Cells. Cell Mol Immunol (2016) 13:347–53. doi: 10.1038/cmi.2016.5
47. Eksteen B, Grant AJ, Miles A, Curbishley SM, Lalor PF, Hübscher SG, et al. Hepatic Endothelial CCL25 Mediates the Recruitment of CCR9+ Gut-Homing Lymphocytes to the Liver in Primary Sclerosing Cholangitis. J Exp Med (2004) 200:1511–7. doi: 10.1084/jem.20041035
48. Rojo ÓP, San Román AL, Arbizu EA, Martínez ADLH, Sevillano ER, Martínez AA. Serum Lipopolysaccharide-Binding Protein in Endotoxemic Patients With Inflammatory Bowel Disease. Inflamm Bowel Dis (2007) 13:269–77. doi: 10.1002/ibd.20019
49. Bell LN, Chalasani N. Epidemiology of Idiosyncratic Drug-Induced Liver Injury. Semin Liver Dis (2009) 29:337–47. doi: 10.1055/s-0029-1240002
50. Bjornsson ES. Epidemiology and Risk Factors for Idiosyncratic Drug-Induced Liver Injury. Semin Liver Dis (2009) 29:337–47. doi: 10.1055/s-0029-1240004
51. Larkin J, Lao CD, Urba WJ, McDermott DF, Horak C, Jiang J, et al. Efficacy and Safety of Nivolumab in Patients With BRAF V600 Mutant and BRAF Wild-Type Advanced Melanoma: A Pooled Analysis of 4 Clinical Trials. JAMA Oncol (2015) 3:801–9. doi: 10.1001/jamaoncol.2015.1184
52. Ribas A, Hamid O, Daud A, Hodi FS, Wolchok JD, Kefford R, et al. Association of Pembrolizumab With Tumor Response and Survival Among Patients With Advanced Melanoma. JAMA - J Am Med Assoc (2016) 315:1600–9. doi: 10.1001/jama.2016.4059
53. Robert C, Ribas A, Schachter J, Arance A, Grob JJ, Mortier L, et al. Pembrolizumab Versus Ipilimumab in Advanced Melanoma (KEYNOTE-006): Post-Hoc 5-Year Results From an Open-Label, Multicentre, Randomised, Controlled, Phase 3 Study. Lancet Oncol (2019) 20:1239–51. doi: 10.1016/S1470-2045(19)30388-2
54. Robert C, Schachter J, Long GV, Arance A, Grob JJ, Mortier L, et al. Pembrolizumab Versus Ipilimumab in Advanced Melanoma. N Engl J Med (2015) 372:2521–32. doi: 10.1056/nejmoa1503093
55. Lee Y-M, Kaplan MM. Primary Sclerosing Cholangitis. N Engl J Med (1995) 332:924–33. doi: 10.1016/S1058-2746(09)80121-3
56. Williamson KD, Chapman RW. New Therapeutic Strategies for Primary Sclerosing Cholangitis. Semin Liver Dis (2016) 36:5–14. doi: 10.1055/s-0035-1571274
57. Karlsen TH, Hampe J, Wiencke K, Schrumpf E, Thorsby E, Lie BA, et al. Genetic Polymorphisms Associated With Inflammatory Bowel Disease do Not Confer Risk for Primary Sclerosing Cholangitis. Am J Gastroenterol (2007) 102:115–21. doi: 10.1111/j.1572-0241.2006.00928.x
58. Adams DH, Eksteen B. Aberrant Homing of Mucosal T Cells and Extra-Intestinal Manifestations of Inflammatory Bowel Disease. Nat Rev Immunol (2006) 6:244–51. doi: 10.1038/nri1784
59. Grant AJ, Lalor PF, Hübscher SG, Briskin M, Adams DH. MAdCAM-1 Expressed in Chronic Inflammatory Liver Disease Supports Mucosal Lymphocyte Adhesion to Hepatic Endothelium (MAdCAM-1 in Chronic Inflammatory Liver Disease). Hepatology (2001) 33:1065–72. doi: 10.1053/jhep.2001.24231
60. Silveira MG, Lindor KD. Clinical Features and Management of Primary Sclerosing Cholangitis. World J Gastroenterol (2008) 14:3338. doi: 10.3748/wjg.14.3338
61. L KN, L NF. Primary Sclerosing Cholangitis. N Engl J Med (2016) 375:1161–70. doi: 10.1056/NEJMra1506330
62. Hirschfield GM, Karlsen TH, Lindor KD, Adams DH. Primary Sclerosing Cholangitis. Lancet (2013) 382:1587–99. doi: 10.1016/S0140-6736(13)60096-3
63. Loftus EV, Harewood GC, Loftus CG, Tremaine WJ, Harmsen WS, Zinsmeister AR, et al. PSC-IBD: A Unique Form of Inflammatory Bowel Disease Associated With Primary Sclerosing Cholangitis. Gut (2005) 54:91–6. doi: 10.1136/gut.2004.046615
64. Peloquin JM, Goel G, Villablanca EJ, Xavier RJ. Mechanisms of Pediatric Inflammatory Bowel Disease. Annu Rev Immunol (2016) 21:1419–27. doi: 10.1146/annurev-immunol-032414-112151
65. Khor B, Gardet A, Xavier RJ. Genetics and Pathogenesis of Inflammatory Bowel Disease. Nature (2011) 474:307–17. doi: 10.1038/nature10209
66. Trivedi PJ, Reece J, Laing RW, Slaney E, Cooney R, Gunson BK, et al. The Impact of Ileal Pouch-Anal Anastomosis on Graft Survival Following Liver Transplantation for Primary Sclerosing Cholangitis. Aliment Pharmacol Ther (2018) 48:322–32. doi: 10.1111/apt.14828
67. Eksteen B. The Gut-Liver Axis in Primary Sclerosing Cholangitis. Clin Liver Dis (2016) 20:1–14. doi: 10.1016/j.cld.2015.08.012
68. Neurath MF. Current and Emerging Therapeutic Targets for IBD. Nat Rev Gastroenterol Hepatol (2017) 14:269–78. doi: 10.1038/nrgastro.2016.208
69. Henriksen EKK, Jørgensen KK, Kaveh F, Holm K, Hamm D, Olweus J, et al. Gut and Liver T-Cells of Common Clonal Origin in Primary Sclerosing Cholangitis-Inflammatory Bowel Disease. J Hepatol (2017) 53:762–8. doi: 10.1016/j.jhep.2016.09.002
70. Tabibian JH, Varghese C, Larusso NF, O’Hara SP. The Enteric Microbiome in Hepatobiliary Health and Disease. Liver Int (2016) 46:480–7. doi: 10.1111/liv.13009
71. Lleo A, Wang GQ, Gershwin ME, Hirschfield GM. Primary Biliary Cholangitis. Lancet (2020) 396:1915–26. doi: 10.1016/S0140-6736(20)31607-X
72. Ronca V, Carbone M, Bernuzzi F, Malinverno F, Mouse HS, Gershwin ME, et al. From Pathogenesis to Novel Therapies in the Treatment of Primary Biliary Cholangitis. Expert Rev Clin Immunol (2017) 13:1121–31. doi: 10.1080/1744666X.2017.1391093
73. Fukui H. Increased Intestinal Permeability and Decreased Barrier Function: Does It Really Influence the Risk of Inflammation? Inflamm Intest Dis (2016) 1:135–45. doi: 10.1159/000447252
74. Di Leo V, Venturi C, Baragiotta A, Martines D, Floreani A. Gastroduodenal and Intestinal Permeability in Primary Biliary Cirrhosis. Eur J Gastroenterol Hepatol (2003) 15:967–73. doi: 10.1097/00042737-200309000-00005
75. Zhao J, Zhao S, Zhou G, Liang L, Guo X, Mao P, et al. Altered Biliary Epithelial Cell and Monocyte Responses to Lipopolysaccharide as a TLR Ligand in Patients With Primary Biliary Cirrhosis. Scand J Gastroenterol (2011) 46:485–94. doi: 10.3109/00365521.2010.539624
76. Haruta I, Kikuchi K, Hashimoto E, Nakamura M, Miyakawa H, Hirota K, et al. Long-Term Bacterial Exposure can Trigger Nonsuppurative Destructive Cholangitis Associated With Multifocal Epithelial Inflammation. Lab Investig (2010) 90:577–88. doi: 10.1038/labinvest.2010.40
77. Chen W, Wei Y, Xiong A, Li Y, Guan H, Wang Q, et al. Comprehensive Analysis of Serum and Fecal Bile Acid Profiles and Interaction With Gut Microbiota in Primary Biliary Cholangitis. Clin Rev Allergy Immunol (2020) 58:25–38. doi: 10.1007/s12016-019-08731-2
78. Furukawa M, Moriya K, Nakayama J, Inoue T, Momoda R, Kawaratani H, et al. Gut Dysbiosis Associated With Clinical Prognosis of Patients With Primary Biliary Cholangitis. Hepatol Res (2020) 50:840–52. doi: 10.1111/hepr.13509
79. Abe K, Takahashi A, Fujita M, Imaizumi H, Hayashi M, Okai K, et al. Dysbiosis of Oral Microbiota and its Association With Salivary Immunological Biomarkers in Autoimmune Liver Disease. PloS One (2018) 13:e0198757. doi: 10.1371/journal.pone.0198757
80. Tang R, Wei Y, Li Y, Chen W, Chen H, Wang Q, et al. Gut Microbial Profile is Altered in Primary Biliary Cholangitis and Partially Restored After UDCA Therapy. Gut (2018) 67:534–71. doi: 10.1136/gutjnl-2016-313332
81. Kunkel EJ, Campbell JJ, Haraldsen G, Pan J, Boisvert J, Roberts AI, et al. Lymphocyte CC Chemokine Receptor 9 and Epithelial Thymus-Expressed Chemokine (TECK) Expression Distinguish the Small Intestinal Immune Compartment: Epithelial Expression of Tissue-Specific Chemokines as an Organizing Principle in Regional Immunity. J Exp Med (2000) 192:761–8. doi: 10.1084/jem.192.5.761
82. Mora JR, Bono MR, Manjunath N, Weninger W, Cavanagh LL, Rosemblatt M, et al. Selective Imprinting of Gut-Homing T Cells by Peyer’s Patch Dendritic Cells. Nature (2003) 424:88–93. doi: 10.1038/nature01726
83. Miles A, Liaskou E, Eksteen B, Lalor PF, Adams DH. CCL25 and CCL28 Promote α4β7-Integrin- Dependent Adhesion of Lymphocytes to MAdCAM-1 Under Shear Flow. Am J Physiol - Gastrointest Liver Physiol (2008) 21:527–38. doi: 10.1152/ajpgi.00266.2007
84. Trivedi PJ, Bruns T, Ward S, Mai M, Schmidt C, Hirschfield GM, et al. Intestinal CCL25 Expression is Increased in Colitis and Correlates With Inflammatory Activity. J Autoimmun (2016) 151:97. doi: 10.1016/j.jaut.2016.01.001
85. Iwata M, Hirakiyama A, Eshima Y, Kagechika H, Kato C, Song SY. Retinoic Acid Imprints Gut-Homing Specificity on T Cells. Immunity (2004) 294:G1257–67. doi: 10.1016/j.immuni.2004.08.011
86. Briskin M, Winsor-Hines D, Shyjan A, Cochran N, Bloom S, Wilson J, et al. Human Mucosal Addressin Cell Adhesion Molecule-1 is Preferentially Expressed in Intestinal Tract and Associated Lymphoid Tissue. Am J Pathol (1997) 53:661. doi: 10.1002/hep.24085
87. Liaskou E, Karikoski M, Reynolds GM, Lalor PF, Weston CJ, Pullen N, et al. Regulation of MAdCAM-1 Expression in Human and Mice By VAP-1 Amine Oxidase Activity. Hepatology (2011) 53:661–72. doi: 10.1002/hep.24085.Regulation
88. Hillan KJ, Hagler KE, MacSween RNM, Ryan AM, Renz ME, Chiu HH, et al. Expression of the Mucosal Vascular Addressin, MAdCAM-1, in Inflammatory Liver Disease. Liver (1999) 3:100054. doi: 10.1111/j.1478-3231.1999.tb00084.x
89. de Krijger M, Visseren T, Wildenberg ME, Hooijer GKJ, Verstegen MMA, van der Laan LJW, et al. Characterization of Gut-Homing Molecules in non-Endstage Livers of Patients With Primary Sclerosing Cholangitis and Inflammatory Bowel Disease. J Transl Autoimmun (2020) 287:G1062–9. doi: 10.1016/j.jtauto.2020.100054
90. Ogawa H, Iimura M, Eckmann L, Kagnoff MF. Regulated Production of the Chemokine CCL28 in Human Colon Epithelium. Am J Physiol - Gastrointest Liver Physiol (2004) 59:1932–43. doi: 10.1152/ajpgi.00162.2004
91. Afford SC, Humphreys EH, Reid DT, Russell CL, Banz VM, Oo Y, et al. Vascular Cell Adhesion Molecule 1 Expression by Biliary Epithelium Promotes Persistence of Inflammation by Inhibiting Effector T-Cell Apoptosis. Hepatology (2014) 59:1932–43. doi: 10.1002/hep.26965
92. Mumtaz S, Goh J, Hirschfield GM, Ferguson J, Cooper SC. Evolving Strategies to Reduce Colectomy Rates in Primary Sclerosing Cholangitis-Inflammatory Bowel Disease: Clinical Remission of Corticosteroid Refractory Colitis Post-Liver Transplant With Vedolizumab. Frontline Gastroenterol (2016) 7:271–4. doi: 10.1136/flgastro-2016-100711
93. Martín RVO, Trillo VA, Grande RG, Pérez MJ. Efficacy and Safety of Vedolizumab as a Treatment Option for Moderate to Severe Refractory Ulcerative Colitis in Two Patients After Liver Transplant Due to Primary Sclerosing Cholangitis. Rev Esp Enfermadades Dig (2017) 109:659–63. doi: 10.2147/DDDT.S50348
94. Krupka N, Baumgart DC. Designing Biologic Selectivity for Inflammatory Bowel Disease – Role of Vedolizumab. Drug Des Devel Ther (2014) 9:147–54. doi: 10.2147/DDDT.S50348
95. Cherry LN, Vaughan D, Yunker NS, Lambert ER, Lowe DK. Vedolizumab: An α4β7 Integrin Antagonist for Ulcerative Colitis and Crohn’s Disease. Ther Adv Chronic Dis (2015) 6:224–33. doi: 10.1177/2040622315586970
96. Petkau JMV, Eksteen B. Selective Biologics for Ulcerative Colitis and Crohn’s Disease - Clinical Utility of Vedolizumab. Biol Targets Ther (2016) 10:33–52. doi: 10.2147/BTT.S71679
97. Lobaton T, Vermeire S, Van Assche G, Rutgeerts P. Review Article: Anti-Adhesion Therapies for Inflammatory Bowel Disease. Aliment Pharmacol Ther (2014) 39:579–94. doi: 10.1111/apt.12639
98. Sandborn WJ, Feagan BG, Rutgeerts P, Hanauer S, Colombel J-F, Sands BE, et al. Vedolizumab as Induction and Maintenance Therapy for Crohn’s Disease: Commentary. N Engl J Med (2013) 269:711–21. doi: 10.1056/NEJMoa1215739
99. Feagan BG, Rutgeerts P, Sands BE, Hanauer S, Colombel J-F, Sandborn WJ, et al. Vedolizumab as Induction and Maintenance Therapy for Ulcerative Colitis. N Engl J Med (2013) 369:699–710. doi: 10.1056/nejmoa1215734
100. Danese S, Sandborn WJ, Colombel JF, Vermeire S, Glover SC, Rimola J, et al. Endoscopic, Radiologic, and Histologic Healing With Vedolizumab in Patients With Active Crohn’s Disease. Gastroenterology (2019) 157:1007–18.e7. doi: 10.1053/j.gastro.2019.06.038
101. Berends SE, Lowenberg M, Baert FJ, Mathot R, Clasquin E, van der Woude CJ, et al. Higher Serum Concentrations of Vedolizumab are Associated With Superior Endoscopic Outcomes in Crohn’s Disease: Data From the LOVE-CD Trial. Gastroenterology (2018) 154:S822–3. doi: 10.1016/S0016-5085(18)32809-9
102. White JR, Din S, Ingram RJM, Foley S, Alam MA, Robinson R, et al. Experiences of Using Vedolizumab in the Treatment of Inflammatory Bowel Disease in the East Midlands UK–a Retrospective Observational Study. Scand J Gastroenterol (2020) 55:1–10. doi: 10.1080/00365521.2020.1790647
103. Ponsioen CY, Kuiper H, Ten Kate FJ, Van Milligen de Wit M, Van Deventer SJ, Tytgat G. Immunohistochemical Analysis of Inflammation in Primary Sclerosing Cholangitis. Eur J Gastroenterol Hepatol (1999) 11:769–74. doi: 10.1097/00042737-199907000-00015
104. Tse CS, Loftus EV, Raffals LE, Gossard AA, Lightner AL. Effects of Vedolizumab, Adalimumab and Infliximab on Biliary Inflammation in Individuals With Primary Sclerosing Cholangitis and Inflammatory Bowel Disease. Aliment Pharmacol Ther (2018) 48:190–5. doi: 10.1111/apt.14829
105. Christensen B, Micic D, Gibson PR, Yarur A, Bellaguarda E, Corsello P, et al. Vedolizumab in Patients With Concurrent Primary Sclerosing Cholangitis and Inflammatory Bowel Disease Does Not Improve Liver Biochemistry But is Safe and Effective for the Bowel Disease. Aliment Pharmacol Ther (2018) 47:753–62. doi: 10.1111/apt.14525
106. Tadbiri S, Peyrin-Biroulet L, Serrero M, Filippi J, Pariente B, Roblin X, et al. Impact of Vedolizumab Therapy on Extra-Intestinal Manifestations in Patients With Inflammatory Bowel Disease: A Multicentre Cohort Study Nested in the OBSERV-IBD Cohort. Aliment Pharmacol Ther (2018) 47:485–93. doi: 10.1111/apt.14419
107. Lynch KD, Chapman RW, Keshav S, Montano-Loza AJ, Mason AL, Kremer AE, et al. Effects of Vedolizumab in Patients With Primary Sclerosing Cholangitis and Inflammatory Bowel Diseases. Clin Gastroenterol Hepatol (2020) 18:179–87.e6. doi: 10.1016/j.cgh.2019.05.013
108. Tabibian JH, Weeding E, Jorgensen RA, Petz JL, Keach JC, Talwalkar JA, et al. Randomised Clinical Trial: Vancomycin or Metronidazole in Patients With Primary Sclerosing Cholangitis - A Pilot Study. Aliment Pharmacol Ther (2013) 60:824–31. doi: 10.1111/apt.12232
109. Vrieze A, Out C, Fuentes S, Jonker L, Reuling I, Kootte RS, et al. Impact of Oral Vancomycin on Gut Microbiota, Bile Acid Metabolism, and Insulin Sensitivity. J Hepatol (2014) 72:1729–38. doi: 10.1016/j.jhep.2013.11.034
110. Begley M, Hill C, Gahan CGM. Bile Salt Hydrolase Activity in Probiotics. Appl Environ Microbiol (2006) 48:367–75. doi: 10.1128/AEM.72.3.1729-1738.2006
111. Kitahara M, Sakata S, Sakamoto M, Benno Y. Comparison Among Fecal Secondary Bile Acid Levels, Fecal Microbiota and Clostridium Scindens Cell Numbers in Japanese. Microbiol Immunol (2004) 12:1–5. doi: 10.1111/j.1348-0421.2004.tb03526.x
112. Ajouz H, Mukherji D, Shamseddine A. Secondary Bile Acids: An Underrecognized Cause of Colon Cancer. World J Surg Oncol (2014) 32:397–406. doi: 10.1186/1477-7819-12-164
113. Abarbanel DN, Seki SM, Davies Y, Marlen N, Benavides JA, Cox K, et al. Immunomodulatory Effect of Vancomycin on Treg in Pediatric Inflammatory Bowel Disease and Primary Sclerosing Cholangitis. J Clin Immunol (2013) 25:457–64. doi: 10.1007/s10875-012-9801-1
114. Rahimpour S, Nasiri-Toosi M, Khalili H, Daryani NE, Taromlou MKN, Azizi Z. A Triple Blinded, Randomized, Placebo-Controlled Clinical Trial to Evaluate the Efficacy and Safety of Oral Vancomycin in Primary Sclerosing Cholangitis: A Pilot Study. J Gastrointest Liver Dis (2016) 2017:bcr-2017-221165. doi: 10.15403/jgld.2014.1121.254.rah
115. Hey P, Lokan J, Johnson P, Gow P. Efficacy of Oral Vancomycin in Recurrent Primary Sclerosing Cholangitis Following Liver Transplantation. BMJ Case Rep (2017) 10:1247–52. doi: 10.1136/bcr-2017-221165
116. De Chambrun GP, Nachury M, Funakoshi N, Gerard R, Bismuth M, Valats JC, et al. Oral Vancomycin Induces Sustained Deep Remission in Adult Patients With Ulcerative Colitis and Primary Sclerosing Cholangitis. Eur J Gastroenterol Hepatol (2018) 7:334. doi: 10.1097/MEG.0000000000001223
117. Jeffery HC, Braitch MK, Brown S, Oo YH. Clinical Potential of Regulatory T Cell Therapy in Liver Diseases: An Overview and Current Perspectives. Front Immunol (2016) 7:334:2749–55. doi: 10.3389/fimmu.2016.00334
118. Tahvildari M, Dana R. Low-Dose IL-2 Therapy in Transplantation, Autoimmunity, and Inflammatory Diseases. J Immunol (2019) 203:2749–55. doi: 10.4049/jimmunol.1900733
119. Riveiro-Barciela M, Barreira-Díaz A, Vidal-González J, Muñoz-Couselo E, Martínez-Valle F, Viladomiu L, et al. Immune-Related Hepatitis Related to Checkpoint Inhibitors: Clinical and Prognostic Factors. Liver Int (2020) 40:1906–16. doi: 10.1111/liv.14489
120. Postow MA, Sidlow R, Hellmann MD. Immune-Related Adverse Events Associated With Immune Checkpoint Blockade. N Engl J Med (2018) 378:158–68. doi: 10.1056/nejmra1703481
121. Suzman DL, Pelosof L, Rosenberg A, Avigan MI. Hepatotoxicity of Immune Checkpoint Inhibitors: An Evolving Picture of Risk Associated With a Vital Class of Immunotherapy Agents. Liver Int (2018) 38:976–87. doi: 10.1111/liv.13746
122. Gudd CLC, Au L, Triantafyllou E, Shum B, Liu T, Nathwani R, et al. Activation and Transcriptional Profile of Monocytes and CD8+ T Cells are Altered in Checkpoint Inhibitor-Related Hepatitis. J Hepatol (2021) 75:177–89. doi: 10.1016/j.jhep.2021.02.008
123. Swaminathan M, Olsson-Brown A, Sreedhar S, Pritchard M. PTU-064 Immune Checkpoint Inhibitor Colitis- a Review of Current Management Trends. N Engl J Med (2018) 375:2369–79. doi: 10.1056/NEJMra1600266
124. Lynch SV, Pedersen O. The Human Intestinal Microbiome in Health and Disease. N Engl J Med (2016) 149:223–37. doi: 10.1056/nejmra1600266
125. Kelly CR, Kahn S, Kashyap P, Laine L, Rubin D, Atreja A, et al. Update on Fecal Microbiota Transplantation 2015: Indications, Methodologies, Mechanisms, and Outlook. Gastroenterology (2015) 20:522–8. doi: 10.1053/j.gastro.2015.05.008
126. Finlay BB, Goldszmid R, Honda K, Trinchieri G, Wargo J, Zitvogel L. Can We Harness the Microbiota to Enhance the Efficacy of Cancer Immunotherapy? Nat Rev Immunol (2020) 3:103–9. doi: 10.1038/s41577-020-0374-6
127. Goldszmid RS, Dzutsev A, Viaud S, Zitvogel L, Restifo NP, Trinchieri G. Microbiota Modulation of Myeloid Cells in Cancer Therapy. Cancer Immunol Res (2015) 22:1856–64. doi: 10.1158/2326-6066.CIR-14-0225
128. Zarour HM. Reversing T-Cell Dysfunction and Exhaustion in Cancer. Clin Cancer Res (2016) 359:91–7. doi: 10.1158/1078-0432.CCR-15-1849
129. Gopalakrishnan V, Spencer CN, Nezi L, Reuben A, Andrews MC, Karpinets TV, et al. Gut Microbiome Modulates Response to Anti-PD-1 Immunotherapy in Melanoma Patients. Sci (80-) (2018) 359:97–166. doi: 10.1126/science.aan4236
130. Routy B, Le Chatelier E, Derosa L, Duong CPM, Alou MT, Daillère R, et al. Gut Microbiome Influences Efficacy of PD-1-Based Immunotherapy Against Epithelial Tumors. Sci (80-) (2018) 359:104–8. doi: 10.1126/science.aan3706
131. Matson V, Fessler J, Bao R, Chongsuwat T, Zha Y, Alegre ML, et al. The Commensal Microbiome is Associated With Anti-PD-1 Efficacy in Metastatic Melanoma Patients. Sci (80-) (2018) 371:595–602. doi: 10.1126/science.aao3290
132. Davar D, Dzutsev AK, Mcculloch JA, Rodrigues RR, Pagliano O, Zidi B, et al. Fecal Microbiota Transplant Overcomes Resistance to Anti – PD-1 Therapy in Melanoma Patients. Gut (2021) 602:595–602. doi: 10.1136/gutjnl-2020-bsgcampus.168
133. Cheung V, Sasson S, Misheva M, Slevin S, Gupta T, Webb G, et al. P93 Faecal Microbiota Transplant for Refractory Checkpoint Inhibitor Immunotherapy-Related Colitis. Hepatology (2021) 70:A89.2–90. doi: 10.1136/gutjnl-2020-bsgcampus.169
134. Tabibian JH, O’Hara SP, Trussoni CE, Tietz PS, Splinter PL, Mounajjed T, et al. Absence of the Intestinal Microbiota Exacerbates Hepatobiliary Disease in a Murine Model of Primary Sclerosing Cholangitis. Hepatology (2016) 114:1071–9. doi: 10.1002/hep.27927
Keywords: plasticity, therapy, liver disease, Gut microbiota, Metabolites, PSC, PBC, IBD
Citation: Bozward AG, Ronca V, Osei-Bordom D and Oo YH (2021) Gut-Liver Immune Traffic: Deciphering Immune-Pathogenesis to Underpin Translational Therapy. Front. Immunol. 12:711217. doi: 10.3389/fimmu.2021.711217
Received: 18 May 2021; Accepted: 09 August 2021;
Published: 25 August 2021.
Edited by:
Andras Perl, Upstate Medical University, United StatesReviewed by:
Gyorgy Baffy, Harvard Medical School, United StatesYu-Jih Su, Kaohsiung Chang Gung Memorial Hospital, Taiwan
Copyright © 2021 Bozward, Ronca, Osei-Bordom and Oo. This is an open-access article distributed under the terms of the Creative Commons Attribution License (CC BY). The use, distribution or reproduction in other forums is permitted, provided the original author(s) and the copyright owner(s) are credited and that the original publication in this journal is cited, in accordance with accepted academic practice. No use, distribution or reproduction is permitted which does not comply with these terms.
*Correspondence: Amber G. Bozward, QS5HLkJvendhcmRAYmhhbS5hYy51aw==; Ye Htun Oo, eS5oLm9vQGJoYW0uYWMudWs=