- 1Pediatric Clinic, University Hospital, Department of Medicine and Surgery, University of Parma, Parma, Italy
- 2Department of Pediatrics, University of British Columbia, Vancouver, BC, Canada
- 3Specialization Medical School of Hygiene, Department of Health Sciences, University of Florence, Florence, Italy
- 4Association Française de Pédiatrie Ambulatoire (AFPA), Paris, France
- 5Tasmanian Vaccine Trial Centre, Launceston General Hospital, Launceston, TAS, Australia
- 6School of Medicine, University of Tasmania, Launceston, TAS, Australia
- 7Department of Immunology and Pathology, Monash University, Melbourne, VIC, Australia
- 8School of Health and Biomedical Science, Royal Melbourne Institute of Technology (RMIT) University, Melbourne, VIC, Australia
- 9Translational Pediatrics and Infectious Diseases, Pediatrics Department, Hospital Clínico Universitario de Santiago de Compostela, Santiago de Compostela, Spain
- 10Genetics, Vaccines and Pediatrics Research Group, Instituto de Investigación Sanitaria de Santiago de Compostela, Universidad de Santiago, Santiago de Compostela, Spain
- 11Division of Infectious Diseases, Department of Pediatrics, Center for Vaccines and Immunity Nationwide Children’s Hospital-The Ohio State University College of Medicine, Columbus, OH, United States
- 12Department of Pharmacology and Pediatrics, Malaga Medical School, Malaga University, Malaga, Spain
- 13St. Mary’s Hospital, London, United Kingdom
- 14Department of Pediatrics, Santa Casa de Sao Paulo School of Medical Sciences, Sao Paulo, Brazil
- 15Klinik für Pädiatrische Onkologie und Hämatologie Universitätsklinikum des Saarlandes, Homburg, Germany
Routine childhood vaccinations are key for the protection of children from a variety of serious and potentially fatal diseases. Current pediatric vaccine schedules mainly cover active vaccines. Active vaccination in infants is a highly effective approach against several infectious diseases; however, thus far, for some important viral pathogens, including respiratory syncytial virus (RSV), vaccine development and license by healthcare authorities have not been accomplished. Nirsevimab is a human-derived, highly potent monoclonal antibody (mAb) with an extended half-life for RSV prophylaxis in all infants. In this manuscript, we consider the potential implications for the introduction of an anti-viral mAb, such as nirsevimab, into the routine pediatric vaccine schedule, as well as considerations for coadministration. Specifically, we present evidence on the general mechanism of action of anti-viral mAbs and experience with palivizumab, the only approved mAb for the prevention of RSV infection in preterm infants, infants with chronic lung disease of prematurity and certain infants with hemodynamically significant heart disease. Palivizumab has been used for over two decades in infants who also receive routine vaccinations without any alerts concerning the safety and efficacy of coadministration. Immunization guidelines (Advisory Committee on Immunization Practices, Joint Committee on Vaccination and Immunization, National Advisory Committee on Immunization, Centers for Disease Control and Prevention, American Academy of Pediatrics, The Association of the Scientific Medical Societies in Germany) support coadministration of palivizumab with routine pediatric vaccines, noting that immunobiologics, such as palivizumab, do not interfere with the immune response to licensed live or inactivated active vaccines. Based on the mechanism of action of the new generation of anti-viral mAbs, such as nirsevimab, which is highly specific targeting viral antigenic sites, it is unlikely that it could interfere with the immune response to other vaccines. Taken together, we anticipate that nirsevimab could be concomitantly administered to infants with routine pediatric vaccines during the same clinic visit.
Background
Vaccination is a highly effective strategy to reduce the morbidity and mortality of many infectious diseases. Currently, vaccination prevents 2–3 million deaths yearly from diseases such as diphtheria, tetanus, influenza, Hepatitis B, Haemophilus influenzae b (Hib), pertussis, Yellow fever, poliomyelitis and measles, and saves almost 97 million disability-adjusted life years (1, 2). Highlighting the importance of vaccination as a public health intervention, the Advisory Committee on Immunization Practices (ACIP) of the US Centres for Disease Control and Prevention recommends routine immunization against 17 vaccine-preventable diseases in infants, children, adolescents or adults (3). Children are particularly vulnerable to infections, and pediatric vaccines have dramatically reduced childhood mortality due to infectious diseases (4, 5). Since 1990, mortality in children 5–9 years of age has decreased by 61% due to a reduction in the incidence of infectious diseases (6). Over the last decade, more than 1 billion children have been vaccinated against infectious diseases and during 2019, around 85% of infants worldwide received three doses of the diphtheria-tetanus-pertussis (DTP) vaccine (2).
Although active infant vaccination is highly effective for a number of viral diseases (e.g., rotavirus, polio, measles, mumps, rubella and chickenpox), the development of effective vaccines against certain viral pathogens (e.g. human immunodeficiency virus [HIV], respiratory syncytial virus (RSV), hepatitis C, human cytomegalovirus) has not been successful so far (7). Passive immunization approaches with monoclonal antibodies (mAbs) could be considered for introduction into routine pediatric vaccination schedules (7), that currently include live and inactivated active vaccines. Several mAb-related clinical trials are being conducted in different countries around the world. However, only for few of them, data adequate to allow authorization by Food and Drug Administration and European Medicines Agency have been collected with well performed clinical trial. Most are in a very early stage and cannot be adequately evaluated (8). Although mAbs are one of the fastest-growing drug classes in last years, their precise mechanism of action is yet unknown. Any outcome with therapeutic mAb is related to several factors. Important factors include antigen cell-surface density, tissue distribution, specificity, avidity, and isotype (9). The reason for the slow speed in developing mAbs include unreasonable costing for research and development, especially when compared with small molecule drugs and vaccines (10). Additionally, the complexity and ambiguity of viruses as associated with their rapid mutation make it difficult for researchers to develop effective and long-lasting mAb therapy (11).
Typically, the introduction of a new active vaccine requires data on co-administration with passive vaccines with which it will be given, to ensure non-interference with immunity (12). There is no specific guidance regarding the introduction of mAbs for use with routine pediatric vaccines. To address this topic, consideration is given to the mechanism of action of antiviral mAbs and cumulated experience with palivizumab, the only marketed mAb used for prevention of serious lower respiratory tract disease caused by RSV in the pediatric population.
Respiratory Syncytial Virus: Disease Burden
RSV is the most common cause of acute lower respiratory infection (i.e. pneumonia and bronchiolitis) in infants and young children with most children experiencing at least one episode of RSV infection in the first 2 years of life (13). Consequently, RSV is a leading cause for infant hospitalization worldwide and is also responsible for a large number of outpatient and primary care visits contributing to substantial economic burden (14–17). In low- and middle-income countries, RSV is also a primary cause of infant mortality (18). It was estimated that globally in 2015, RSV lower respiratory tract infections were responsible for as many as 118200 deaths in children < 5 years of age (13). Apart from the acute morbidity, RSV can lead to long-term consequences; as it has been associated with subsequent development of wheezing or asthma in childhood (19–21), although it is still unclear if this association is causal (22). In line with this, there is a significant impact in the long-term healthcare resource utilization that last years following an RSV infection that occurred in the first year of life.
The burden imposed in the healthcare system post-RSV infection extends across all gestational ages and leads to a high economic burden through hospitalizations, physicians and emergency department visits (23). The majority of hospitalized infants due to RSV (67%–79%) are otherwise healthy with no underlying conditions, highlighting that severe RSV infection can occur in all infants (24–26). There is no available vaccines or definite antiviral treatment for RSV infection and clinical management is focused on symptomatic relief and supportive care (27). The only prophylactic agent currently approved against RSV is palivizumab, which is indicated only in specific groups of preterm infants or infants with comorbidities [i.e., chronic lung disease (CLD), hemodynamically significant congenital heart disease] (28). Therefore, there is a high unmet medical need for the prevention and management of RSV disease in all infants.
Passive Immunization
Passive immunization with mAbs is promising strategy for protecting infants from infections. mAbs can be manufactured in vitro in large quantities with high specificity and consistency, and minimal biohazard potential (29, 30). In recent years, the development of highly potent human mAbs provide new opportunities for prophylaxis. These antibodies have primarily been isolated from suitable donors via large-scale screening and single B cell-based methods (7, 31). Furthermore, the antibodies may be engineered to increase their neutralization potency, extend their half-life, and alter their effector function, to provide a fine-tuned antibody designed to neutralize a specific pathogen both effectively and safely. In particular, the combination of increased potency and extended half-life mAbs affects dosing; enhanced potency means that less antibody is required to neutralize the antigen and reach the effective concentration, and extended half-life maintains that effective concentration for a longer duration. This may result in a lower dose (in terms of antibody concentration and fluid volume), allowing for easier administration (i.e., IM injection rather than IV infusion) (32), and less frequent dosing (half-life extension may require antibody administration only every ~3–6 months to maintain antibody effectiveness) (7).
Immunization in pregnancy is an alternative strategy to achieve passive immunization in infants and has proven to protect some vaccine-preventable diseases in infancy (33–35). Protection to the infant is provided via the active transplacental transfer of antigen-specific maternal IgG (34), which leads to passive immunization for the first months of the infant’s life (36). Immunization in pregnancy has proven to be an effective strategy for protecting infants against influenza, tetanus and pertussis (34), in the first few months of life when infants are susceptible to infections, severe disease and death (37). Hence, inactivated influenza and tetanus toxoid and acellular pertussis vaccines, which offer protection in infancy (34), are recommended during pregnancy in the United Kingdom, the United States and other countries (38, 39).
Although there is increasing evidence to support vaccination in pregnancy due to its demonstrated benefits on prevention of influenza and pertussis in infants in the first 6 months of life (40), maternal immunization offers transient protection (36), that can be variable as it depends on the levels of antibodies transferred to the infant, which in turn depends on the levels of antibodies produced by the mother (34, 40). Maternal immunization could interfere with vaccine responses in infants. A recent study showed that maternal immunization with tetanus-diphtheria-acellular-pertussis was associated with reduced antibody responses to diphtheria and pertussis, and also pneumococcus vaccine antigens that were administered as routine immunizations in the first year of life, although the clinical significance of these findings are uncertain (41). For diseases with a seasonal pattern (e.g. RSV), appropriate timing of immune protection is key and needs to be adapted to the seasonality of the virus (7). Importantly, a recent large randomized controlled clinical trial, in which pregnant women received an RSV nanoparticle-based vaccine, failed to meet the pre-specified criterion of reduction of medically significant RSV infection in infants during the first 90 days of life, although the suggestion of a possible benefit with respect to other end-point events involving RSV-associated respiratory disease in infants (42–44).
Palivizumab
The only approved mAb for prophylactic protection against RSV in preterm infants and infants with comorbidities is palivizumab (Astra Zeneca/Medimmune). Palivizumab is a humanized mAb, which targets antigenic site II located in the pre and post fusion (F) conformations of the RSV F protein and has a half-life of 19–27 days (45). It requires monthly injections to maintain protection during the typical 5-month RSV season, at a dose of 15 mg/kg of body weight (28, 46, 47). It has shown efficacy against RSV disease by reducing the risk of hospitalization by 39–78% in groups of infants who are susceptible to severe RSV disease (48, 49). In the European Union (EU) and US, palivizumab was approved for use in preterm infants (≤35 weeks gestational age) who are aged <6 months, children with chronic lung disease (CLD) <2 years, and children with hemodynamically significant congenital heart disease (CHD) aged <2 years (46, 47). The American Academy of Pediatrics (AAP) has subsequently restricted the use of palivizumab to preterm infants with a gestational age of < 29 weeks (50), infants < 32 weeks with CLD, and those with CHD, limiting RSV prophylaxis to <2% of the annual US birth cohort (51). Recommendations for prophylaxis with palivizumab in preterm infants without comorbidities, which take into account the role of environmental risk factors, exist in several European countries (e.g. Italy, Spain, Germany) (52–54).
Nirsevimab
Nirsevimab is a mAb that has been developed for the prevention of RSV disease, which is currently in Phase 3 trials. It is a highly potent, recombinant human mAb that specifically targets site Ø in the prefusion conformation of the F protein. Nirsevimab was functionally optimized from its parental antibody D25, originally derived from a human B cell (55, 56). It neutralizes a diverse panel of both RSV A and B strains in vitro with a >50-fold higher potency than palivizumab. Furthermore, it contains a three amino acid YTE substitution in the Fc region, resulting in an extended half-life in comparison with palivizumab (45), which could translate into durable protection throughout 150 days instead of 19-27 days (55).
In a Phase 1b/2a dose-escalation study, target serum concentrations remained above the 90% effective concentration level of 6.8 μg/mL in 87% of infants who received a 50 mg dose of nirsevimab and 90% of healthy preterm infants receiving this dose showed a ≥4-fold rise from baseline in serum RSV-neutralizing antibody levels. In addition, nirsevimab was well tolerated at all study doses tested (55). In a randomized, placebo-controlled Phase 2b study, nirsevimab reduced the incidence of medically attended RSV-associated lower respiratory tract infections (LRTI) by 70.1% [95% confidence intervals (CI): 52.3, 81.2], as well as the incidence of hospitalization for RSV-associated LRTI by 78.4% (95% CI: 51.9, 90.3) compared with placebo in healthy preterm infants (2.6% vs 9.5%, p<0.0001, and 0.8% vs 4.1%, p<0.0001, respectively). These differences were consistently observed throughout the 150-day period following nirsevimab administration across different geographical locations and for both RSV A and B subtypes (57). The incidence of non-RSV LRTI was similar between the nirsevimab- and placebo arms, suggesting that nirsevimab did not modify the prevalence of infections caused by other respiratory pathogens. Furthermore, the incidence of antidrug antibodies (ADA) against RSV following administration of nirsevimab was low (5.6% vs 3.8% for placebo) with no difference in safety results between groups when analyzed by positive or negative ADA status (57). Nirsevimab is currently in Phase 3 trials for RSV prevention in healthy full-term and late-preterm infants >35 weeks’ gestation, as well as in preterm infants ≤35 weeks’ gestation who are eligible for palivizumab administration (Phase 2/3) (58, 59).
Prophylaxis with RSV-specific mAbs has shown to be protective in specific risk groups with palivizumab, and the results from the nirsevimab study support its continued development for the wider prevention of RSV infection in healthy infants. If proven safe and efficacious also in healthy newborns, nirsevimab might be incorporated into the routine immunization program. Nirsevimab implementation might be based on a different strategy for infants born in the RSV season compared with those born out of season. For infants born during the RSV season, nirsevimab could be given as soon as possible after birth in advance of most routine vaccines. For infants born outside the RSV season, nirsevimab might need to be administered concomitantly with other recommended pediatric vaccines during routine vaccination visits, as this approach would minimize clinic visits and increase compliance (60).
Vaccination Schedule: Examples From the US and Europe
Optimal response to a vaccine depends on various factors, including vaccine type, age and immune status of the host. Recommendation on vaccine administration depends on age-specific risks for the development of the disease, complications and responses to vaccination, as well as potential interference with the immune response by passively-transferred maternal antibodies. In general, vaccination is recommended for the age group which is the youngest at risk of experiencing the disease for which the vaccine has shown efficacy and safety (3).
In the US, the vaccination schedule in children and adolescents (<18 years of age) includes administration of vaccines against hepatitis B; rotavirus; diphtheria, tetanus and pertussis; Hib; pneumococcal disease; poliovirus; influenza; hepatitis A; measles, mumps and rubella (MMR); varicella; HPV and meningococcal disease as recommended by the CDC (Table 1). A catch-up immunization schedule for children aged 4 months to 18 years who start late or are >1 month behind exists providing minimal intervals between doses for children based on age who have experienced delay in vaccination. Specific recommendations exist for certain high-risk groups (e.g. children undergoing chemotherapy or radiation treatment, children with HIV) (61). In Latin America, beyond all these vaccines, immunization programmes in most countries also include BCG vaccine in the neonatal period as well as yellow fever vaccine in countries where the disease is endemic (62). In Europe, the vaccination schedules between different countries are similar, but there are differences in terms of the dosing, the exact type of vaccine and whether a vaccine is administered alone or in combination with other vaccines. Childhood vaccination schedules in all EU/European Economic Area (EEA) countries include vaccination against MMR, DTP, poliomyelitis, Hib and human papillomavirus (adolescent, pre-adolescent girls) (63).
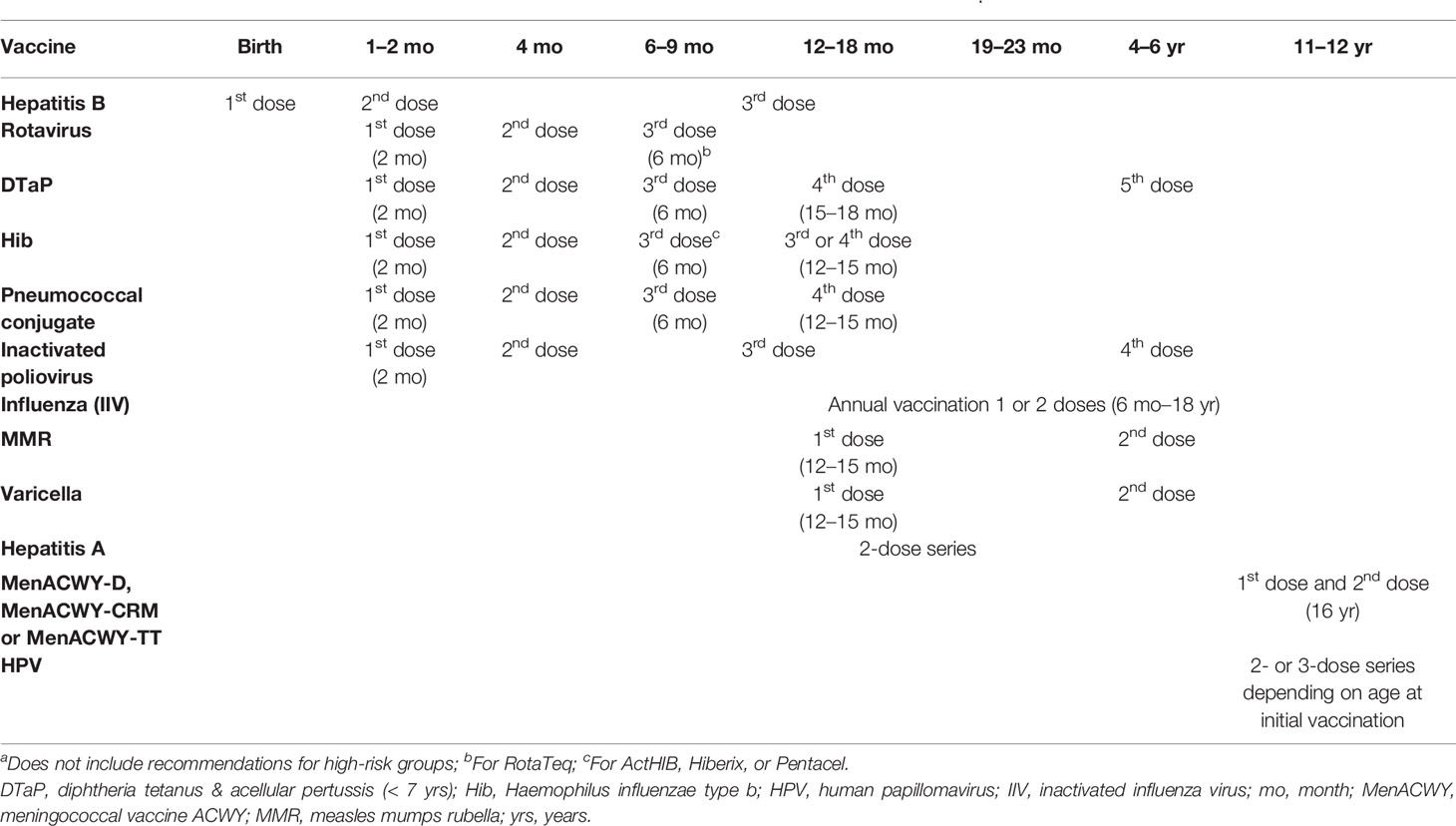
Table 1 Main vaccines included in the US vaccination schedule for children aged <18 years (CDC, 2020) (56)a.
Although the World Health Organization (WHO) recommends vaccination against hepatitis B (64), some EU/EEA countries offer this vaccine only in high-risk groups (63). In some EU/EEA countries, the vaccination schedule includes immunization against hepatitis A, influenza, invasive disease caused by Neisseria meningitidis or Streptococcus pneumoniae, rotavirus, tuberculosis and varicella (63).
Coadministration of Pediatric Vaccines
Coadministration or simultaneous administration of vaccines is defined as administering more than one vaccine on the same clinic day, at different anatomic sites, not combined in the same syringe (3). Coadministration of all vaccines that a child is eligible for at the time of a clinic visit is key for ensuring timely vaccination and also for bringing children up to date (62). The value of pediatric vaccine coadministration, to avoid missing recommended vaccinations, is highlighted by a study investigating a measles outbreak among unvaccinated children aged <5 years in the US. The study found that 38% of these children had received vaccinations against DTP and pertussis and/or oral poliovirus at a time when they could also have simultaneously received measles vaccination (65).
Several studies have investigated the efficacy/immunogenicity and safety of pediatric vaccines administered simultaneously and support current recommendations (ACIP, AAP and WHO) on coadministration of vaccines in children (3, 66, 67). Studies addressing the coadministration of different vaccines, such as MMR with DTP and oral poliovirus, Hib with either MMR or DTP and oral poliovirus, and hepatitis B with DT and oral poliovirus, have demonstrated that simultaneous administration of commonly used live and inactivated vaccines results in seroconversion rates and rates of adverse events that are similar to those following single administration (3, 68, 69). Coadministration of routine pediatric vaccines is recommended for children with no contraindications at the time of vaccination (3). Examples of coadministered vaccines showing no evidence of interference with the immunologic response to any of the antigens include MMR and varicella vaccines; live attenuated influenza and MMR or varicella vaccines; PPSV and inactivated influenza vaccines (IIV); hepatitis B and yellow fever vaccines (3, 70). Although no data are available on the immunogenicity of the Ty21a typhoid vaccine when administered simultaneously or within 30 days of live-virus vaccination, if typhoid vaccination is recommended, it should not be delayed due to recent receipt of live-virus vaccines (3). Furthermore, the ACIP still recommends that IIV and PCV13 are administered simultaneously following a benefit–risk assessment based on the 2010–2011 influenza season data (3, 71, 72).
There are few exceptions to the recommendation of coadministration of pediatric vaccines. In patients with anatomic or functional asplenia and/or HIV, the quadrivalent meningococcal conjugate vaccine MCV4-D (MenACWY-D) and the pneumococcal conjugate vaccine PCV13 should not be administered concomitantly (3). This contraindication is based on data from immunocompetent children showing reduced antibody concentrations (anti-pneumococcal IgG) for vaccine serotypes following coadministration of MenACWY-D and PCV7 compared with PCV7 alone (3). Therefore, due to their baseline high risk of invasive pneumococcal disease, children with anatomic or functional asplenia and/or HIV should not receive vaccination against MenACWY-D concomitantly with PCV-13 before 2 years of age. If these children are older than 2 years and have not yet received all recommended doses of PCV, they should receive all the doses separately from MenACWY-D by at least 4 weeks (3, 73, 74).
Although current experience supports coadministration of vaccines, the introduction of a new vaccine is typically supported by data demonstrating that the vaccine does not interfere with the immune response to other vaccines that are likely to be administered at the same visit (75). While the European Medicines Agency (EMA) does not generally require vaccine coadministration studies to be conducted prior to licensure (75), vaccine use may be limited until data are available on coadministration with types of vaccines to be given at the same time (76). The Food and Drug Administration (FDA) recommends including an assessment of coadministration with relevant vaccines in Phase 3 trials to support vaccine licensure and appropriate label claim (12).
Coadministration of Anti-Viral Monoclonal Antibodies With Pediatric Vaccines
There is limited published evidence on the coadministration of mAbs with pediatric vaccines. The mechanism of action of mAbs might provide insights into the potential for an anti-viral mAb to interfere with the immune response to a vaccine. As described above, most mAbs are human antibodies which exhibit high potency and expected specificity against viral pathogens and are often engineered to further enhance their in vivo functions (7, 31). Anti-viral effects of antibodies can be mediated via several mechanisms. The mechanism that targets free viral particles and is associated with protection in vivo is neutralization, which is measured in vitro as the ability of an antibody to prevent viral entry into target cells. Effector functions mostly mediated via the Fc portion of antibodies include antibody-dependent cellular cytotoxicity (ADCC), complement-dependent cytotoxicity, antibody-dependent cellular phagocytosis (ADCP), antibody-dependent neutrophil phagocytosis and it is believed that these mechanisms may also play a key role in mediating protection against the pathogen. As neutralization often correlates with the ability to bind to native structures on the viral surface, it can give some indication of the ability of antibodies to mediate effector activities such as ADCC and ADCP (7).
Based on the mechanism of action of nirsevimab, which specifically neutralizes RSV preF to provide protection in vivo, its effects are not expected to interfere with the immune response to other vaccines (77). However, mAb–vaccine interaction studies are generally not required by regulatory authorities to support licensure indicating the lack of necessity or clinical relevance of such evaluations. This is reflected in regulatory guidelines. Neither the FDA nor EMA guidelines on clinical evaluation of products for RSV prophylaxis recommend trials to assess coadministration with other vaccines for mAbs. The recent guidance issued by the EMA and FDA covering the clinical development of RSV mAbs for prophylaxis neither require or suggest conducting coadministration studies with other vaccines, although the EMA guidance does include such recommendations for development of RSV vaccines (75, 76). FDA guidance on assessing potential drug interactions for therapeutic proteins advises to consider the potential mechanism for drug-drug interactions taking into consideration the pharmacology of the drug and potential coadministered drugs in the patient population (78). For an anti-viral mAb there is no yet potential mechanism for interference with the immune response to vaccines targeting other viruses or bacteria (e.g. bezlotoxumab) (79). To date, the conduct of mAb–vaccine interference studies has been limited to mAbs directed against immune mediators to assess if the mAb would alter the function of the immune system and affect the response to vaccinations in the patient population (e.g. secukinumab, dupilumab) (46, 80, 81). However, there aren’t large enough studies to determine if mAbs influence immune functions. WHO recommendations (draft version) regarding the preferred product characteristics of mAbs for passive immunization against RSV, state that there is “no significant negative impact on immune responses to co-delivered vaccines” (82).
There are limited country-specific guidelines focusing on the coadministration of antibodies and vaccines. Polyclonal intravenous Ig (IVIG) might interfere with the immune response to many live vaccine viruses, as antibodies may prevent replication of the vaccine virus. Therefore, live viral vaccines should be administered at least three weeks before or 3 to 11 months after an injection of polyclonal IVIG depending on the dose, with exceptions to these recommendations being vaccines against yellow fever, rubella and BCG (83). However, these recommendations are not based on the use of highly specific mAbs. In France, coadministration of pediatric vaccines is possible for DTP, Hib, hepatitis B and pneumococcus at 2 months and 4 months of age, meningococcus C and MMR at 12 months of age, as well as Tdap and HPV at 11–13 years of age; however, further details on coadministration of vaccines or vaccines and antibodies are not provided (84). In general, the administration of live viral vaccines within 3–6 months of polyclonal IVIG is discouraged, as the IVIG product may contain IgG antibodies, that could neutralize the live virus in the vaccine and impair vaccine uptake (85). However, this is less relevant in the case of mAbs specific for a particular pathogen, unless that same specific pathogen is included in the live virus vaccine (86, 87).
There is limited published evidence on the coadministration of palivizumab with pediatric vaccines; only one observational study from Japan has investigated the effects of coadministration in infants and confirmed that there are no safety concerns (88). Guideline recommendations on palivizumab use are consistent and support its coadministration with pediatric vaccines. The ACIP states the following: “A humanized mouse monoclonal antibody product (palivizumab) is available as prophylaxis for serious lower respiratory tract disease from RSV among infants and young children. This product contains only antibody to RSV and does not interfere with the immune response to licensed live or inactivated vaccines” (3). Recommendations by the Joint Committee on Vaccination and Immunization (JCVI) in the UK state that palivizumab “can be given at the same time as vaccines administered as part of the routine childhood immunization programme” (83). According to the National Advisory Committee on Immunization (NACI) in Canada: “Palivizumab is a passive immunizing agent with a highly specific antigen target (the F glycoprotein of RSV). It therefore does not interfere with the immune response to vaccines and can be administered at the same time at a separate site” (88). Similarly, based on the CDC Pink Book: “While certain antibody products like immune globulins interfere with live-virus vaccines, monoclonal antibody products specific to one, non-vaccine microbe do not interfere with live vaccines. Since palivizumab does not contain any other antibody except RSV antibody, it will not interfere with the response to a live virus vaccine” (89). In addition, according to the AAP: “Palivizumab does not interfere with the immune response to live or inactivated vaccines. The childhood immunization schedule should be followed for all children, regardless of palivizumab use” (90). The Association of the Scientific Medical Societies (AWMF) in Germany states that “Prophylaxis with palivizumab can be given at the same time as active vaccinations” (54).
No formal drug–drug or drug–vaccine interaction studies were conducted for palivizumab (47). During clinical development, palivizumab safety was assessed by vaccination status. Based on the very specific mechanism of action of RSV mAbs, safety assessments by vaccination status in clinical development are adequate to support licensure and co-administration with pediatric vaccines. In line with guideline recommendations, it should be noted that in clinical trials using palivizumab, the proportion of children in the placebo and palivizumab groups who received routine childhood vaccines or influenza vaccine were similar, with no increase in adverse reactions between the groups (47, 49). Furthermore, palivizumab has been used for over 22 years in preterm infants and infants with CHD or CLD who also receive routine pediatric vaccinations (FDA and EMA approval in 1998 and 1999, respectively).
There have been limited safety concerns due to palivizumab concomitant administration with other vaccines based on real-world evidence. Notably, the Phase 2b trial of nirsevimab allowed routine pediatric vaccinations to take place as planned and therefore, the efficacy and safety of nirsevimab looking at RSV and non-RSV infections was demonstrated in the context of infants receiving other pediatric vaccines (57). The ongoing nirsevimab Phase 3 trial will also allow administration of routine pediatric vaccines (58, 59). However, some attention should be given to immune enhancement, as exemplified by flavivisus (91). Palivizumab in infancy in otherwise healthy preterm infants has been associated with persistent effects on the abundance of specific, potentially pathogenic, microbial taxa in the respiratory tract, although the meaning of this effect is unclear (92–94).
Conclusions
While specific studies investigating the coadministration of anti-viral mAbs with vaccines have not been performed, the mechanism of action of mAbs, which in the case of viruses is based on targeting highly specific viral antigenic sites, does not raise any concerns regarding coadministration with vaccines. In line with this, palivizumab, the only approved mAb for prophylactic use in preterm infants and infants with comorbidities to protect against severe RSV infection, has been used for more than two decades in infants who also receive routine vaccinations. To date, concerns related to vaccine efficacy or safety have not been reported. In addition, various guidelines (ACIP, JCVI, NACI, CDC, AAP, AWMF) support coadministration of palivizumab with routine pediatric vaccines, as it is highly unlikely that palivizumab interferes with the immune response to other vaccines. Based on the evidence discussed in this white paper and the mechanism of action of nirsevimab, it is not anticipated that coadministration of nirsevimab with routine pediatric vaccines will interfere with the immune response to the vaccine antigens. Therefore, although future studies on efficacy and safety of coadministration of nirsevimab with routine pediatric vaccines are important as well as there is the need to call for research on the administration of mAbs on vaccination and immunity, nirsevimab could be given to infants concomitantly with routine vaccinations during the same clinic visit unless evidence suggests otherwise.
Author Contributions
SE proposed the project, coordinated the study group, and wrote the first draft of the manuscript. BA-R, PB, FC-S, KF, FM, AM, SN, MS, and AS revised the initial draft of the manuscript, provided comments, and substantially contributed to the content of the manuscript. All authors contributed to the article and approved the submitted version.
Funding
The World Association of Infectious Diseases and Immunological Disorders received an unrestricted grant from Sanofi Pasteur for research and medical education activities on RSV monoclonal antibodies and co-administration with pediatric vaccines (Waidid_2021_03). The funder was not involved in the study design, collection, analysis, interpretation of data, the writing of this article or the decision to submit it for publication.
Conflict of Interest
SE: Research support from GSK, Sanofi, and Vifor. Speaker’s fees from GSK, Janssen, Pfizer, Novartis, Sanofi Pasteur, and MSD in the past 3 years. BA is supported by the Canadian Health and Research Institute Vanier Canada scholarship. PB received grants for epidemiological and HTA research projects from GlaxoSmithKline, MSD, Sanofi Pasteur, Pfizer, Seqirus and Astra Zeneca, and fees for taking part in advisory boards on different vaccines from the same companies plus Janssen. FM has received honoraria from GSK, Pfizer, Sanofi Pasteur, MSD, Seqirus, and Janssen for taking part in advisory boards and expert meetings, and for acting as speaker in congresses outside the scope of the submitted work. FM has also acted as principal investigator in RCTs of the above-mentioned companies as well as Ablynx, Regeneron, Roche, Abbot, Novavax, and Medimmune, with honoraria paid to his institution. FM research activities received support from the Instituto de Salud Carlos III (Proyecto de Investigación en Salud, Acción Estratégica en Salud): project ReSVinext ISCIII/PI16/01569/Cofinanciado FEDER and project Enterogen (ISCIII/PI19/01090). KF is a member of the Australian Technical Advisory Group on Immunisation (ATAGI) but the views in this manuscript are her own and not necessarily those of ATAGI. KF has received honoraria as a member of the vaccine advisory boards for Seqiris and Sanofi Pasteur in the last 5 years. AM has received research grants from NIH, Janssen and Merck AND fees for participation in advisory boards from Janssen, Sanofi-Pasteur, Merck and Roche. MS reports research grants and personal fees for advisory boards from GSK, Pfizer, Sanofi-Pasteur, Janssen and Seqirus.
The remaining authors declare that the research was conducted in the absence of any commercial or financial relationships that could be construed as a potential conflict of interest.
Publisher’s Note
All claims expressed in this article are solely those of the authors and do not necessarily represent those of their affiliated organizations, or those of the publisher, the editors and the reviewers. Any product that may be evaluated in this article, or claim that may be made by its manufacturer, is not guaranteed or endorsed by the publisher.
References
1. Ehreth J. The Global Value of Vaccination. Vaccine (2003) 21(7-8):596–600. doi: 10.1016/S0264-410X(02)00623-0
2. World Health Organization. Immunization Coverage. Available at: https://www.who.int/news-room/fact-sheets/detail/immunization-coverage (Accessed December 2020).
3. Advisory Committee on Immunization Practices. General Best Practice Guidelines for Immunization. Available at: https://www.cdc.gov/vaccines/hcp/acip-recs/general-recs/downloads/general-recs.pdf (Accessed December 2020).
4. Public Health England. Green Book. Chapter 1: Immunity and How Vaccines Work. Available at: https://assets.publishing.service.gov.uk/government/uploads/system/uploads/attachment_data/file/815159/Greenbook_chapter_1_Immunity_and_how_vaccines_work.pdf (Accessed December 2020).
5. World Health Organization. Vaccination Greatly Reduces Disease, Disability, Death and Inequity Worldwide. Available at: https://www.who.int/bulletin/volumes/86/2/07-040089/en/ (Accessed December 2020).
7. Walker LM, Burton DR. Passive Immunotherapy of Viral Infections: ’Super-Antibodies’ Enter the Fray. Nat Rev Immunol (2018) 18(5):297–308. doi: 10.1038/nri.2017.148
8. Taylor PC, Adams AC, Hufford MM, de la Torre I, Winthrop K, Gottlieb RL. Neutralizing Monoclonal Antibodies for Treatment of COVID-19. Nat Rev Immunol (2021) 21:382–93. doi: 10.1038/s41577-021-00542-x
9. Shanmugaraj B, Siriwattananon K, Wangkanont K, Phoolcharoen W. Perspectives on Monoclonal Antibody Therapy as Potential Therapeutic Intervention for Coronavirus Disease-19 (COVID-19). Asian Pac J Allergy Immunol (2020) 38:10–8. doi: 10.12932/ap-200220-0773
10. Deb P, Molla MMA, Saif-Ur-Rahman KM. An Update to Monoclonal Antibody as Therapeutic Option Against COVID-19. Biosaf Health (2021) 3:87–91. doi: 10.1016/j.bsheal.2021.02.001
11. Cohen MS, Wohl DA, Fischer WA, Smith DJ, Eron JJ. Outpatient Treatment of SARS-CoV-2 Infection to Prevent COVID-19 Progression. Clin Infect Dis (2021), ciab494. doi: 10.1093/cid/ciab494
12. Food and Drug Administration. Guidance for Industry: General Principles for the Development of Vaccines to Protect Against Global Infectious Diseases. Available at: https://www.fda.gov/media/82306/download (Accessed December 2020).
13. Shi T, McAllister DA, O’Brien KL, Simoes EAF, Madhi SA, Gessner BD, et al. Global, Regional, and National Disease Burden Estimates of Acute Lower Respiratory Infections Due to Respiratory Syncytial Virus in Young Children in 2015: A Systematic Review and Modelling Study. Lancet (2017) 390(10098):946–58. doi: 10.1016/S0140-6736(17)30938-8
14. Leader S, Kohlhase K. Respiratory Syncytial Virus-Coded Pediatric Hospitalizations, 1997 to 1999. Pediatr Infect Dis J (2002) 21(7):629–32. doi: 10.1097/00006454-200207000-00005
15. McLaurin KK, Farr AM, Wade SW, Diakun DR, Stewart DL. Respiratory Syncytial Virus Hospitalization Outcomes and Costs of Full-Term and Preterm Infants. J Perinatol (2016) 36(11):990–6. doi: 10.1038/jp.2016.113
16. Nair H, Nokes DJ, Gessner BD, Dherani M, Madhi SA, Singleton RJ, et al. Global Burden of Acute Lower Respiratory Infections Due to Respiratory Syncytial Virus in Young Children: A Systematic Review and Meta-Analysis. Lancet (2010) 375(9725):1545–55. doi: 10.1016/S0140-6736(10)60206-1
17. Palmer L, Hall CB, Katkin JP, Shi N, Masaquel AS, McLaurin KK, et al. Healthcare Costs Within a Year of Respiratory Syncytial Virus Among Medicaid Infants. Pediatr Pulmonol (2010) 45(8):772–81. doi: 10.1002/ppul.21244
18. Lozano R, Naghavi M, Foreman K, Lim S, Shibuya K, Aboyans V, et al. Global and Regional Mortality From 235 Causes of Death for 20 Age Groups in 1990 and 2010: A Systematic Analysis for the Global Burden of Disease Study 2010. Lancet (2012) 380(9859):2095–128. doi: 10.1016/S0140-6736(12)61728-0
19. Backman K, Ollikainen H, Piippo-Savolainen E, Nuolivirta K, Korppi M. Asthma and Lung Function in Adulthood After a Viral Wheezing Episode in Early Childhood. Clin Exp Allergy (2018) 48(2):138–46. doi: 10.1111/cea.13062
20. Homaira N, Briggs N, Pardy C, Hanly M, Oei JL, Hilder L, et al. Association Between Respiratory Syncytial Viral Disease and the Subsequent Risk of the First Episode of Severe Asthma in Different Subgroups of High-Risk Australian Children: A Whole-of-Population-Based Cohort Study. BMJ Open (2017) 7(11):e017936. doi: 10.1136/bmjopen-2017-017936
21. Piedimonte G, Perez MK. Respiratory Syncytial Virus Infection and Bronchiolitis. Pediatr Rev (2014) 35(12):519–30. doi: 10.1542/pir.35-12-519
22. Driscoll AJ, Arshad SH, Bont L, Brunwasser SM, Cherian T, Englund J, et al. Does Respiratory Syncytial Virus Lower Respiratory Illness in Early Life Cause Recurrent Wheeze of Early Childhood and Asthma? Critical Review of the Evidence and Guidance for Future Studies From a World Health Organization-Sponsored Meeting. Vaccine (2020) 38(11):2435–48. doi: 10.1016/j.vaccine.2020.01.020
23. Simões EAF, Chirikov V, Botteman M, Kwon Y, Kuznik A. Long-Term Assessment of Healthcare Utilization 5 Years After Respiratory Syncytial Virus Infection in US Infants. J Infect Dis (2020) 221(8):1256–70. doi: 10.1093/infdis/jiz278
24. Arriola CS, Kim L, Langley G, Anderson EJ, Openo K, Martin AM, et al. Estimated Burden of Community-Onset Respiratory Syncytial Virus-Associated Hospitalizations Among Children Aged <2 Years in the United States, 2014-15. J Pediatr Infect Dis Soc (2020) 9(5):587–95. doi: 10.1093/jpids/piz087
25. Hall CB, Weinberg GA, Blumkin AK, Edwards KM, Staat MA, Schultz AF, et al. Respiratory Syncytial Virus-Associated Hospitalizations Among Children Less Than 24 Months of Age. Pediatrics (2013) 132(2):e341–8. doi: 10.1542/peds.2013-0303
26. Rha B, Curns AT, Lively JY, Campbell AP, Englund JA, Boom JA, et al. Respiratory Syncytial Virus–Associated Hospitalizations Among Young Children: 2015–2016. Pediatrics (2020) 146(1):e20193611. doi: 10.1542/peds.2019-3611
27. Centers for Disease Control and Prevention. RSV Symptoms and Care. Available at: https://www.cdc.gov/rsv/about/symptoms.html (Accessed December 2020).
28. Drysdale SB, Green CA, Sande CJ. Best Practice in the Prevention and Management of Paediatric Respiratory Syncytial Virus Infection. Ther Adv Infect Dis (2016) 3(2):63–71. doi: 10.1177/2049936116630243
29. Laupland KB. Polyclonal Intravenous Immunoglobulin for the Prophylaxis and Treatment of Infection in Critically Ill Adults. Can J Infect Dis (2002) 13(2):100–6. doi: 10.1155/2002/127953
30. Slifka MK, Amanna IJ. Passive Immunization. Plotkin’s Vaccines (2018) 2018:84–95.e10. doi: 10.1016/B978-0-323-35761-6.00008-0
31. Salazar G, Zhang N, Fu T-M, An Z. Antibody Therapies for the Prevention and Treatment of Viral Infections. NPJ Vaccines (2017) 2(1):19. doi: 10.1038/s41541-017-0019-3
32. Griffin MP, Khan AA, Esser MT, Jensen K, Takas T, Kankam MK, et al. Safety, Tolerability, and Pharmacokinetics of MEDI8897, the Respiratory Syncytial Virus Prefusion F-Targeting Monoclonal Antibody With an Extended Half-Life, in Healthy Adults. Antimicrob Agents Chemother (2017) 61(3):e01714-16. doi: 10.1128/AAC.01714-16
33. Boonyaratanakornkit J, Chu HY. Why Should We Advocate Maternal Immunization? Pediatr Infect Dis J (2019) 38(6S Suppl 1):S28–32. doi: 10.1097/INF.0000000000002312
34. Abu-Raya B, Maertens K, Edwards KM, Omer SB, Englund JA, Flanagan KL, et al. Global Perspectives on Immunization During Pregnancy and Priorities for Future Research and Development: An International Consensus Statement. Front Immunol (2020) 11:1282. doi: 10.3389/fimmu.2020.01282
35. Englund J, Glezen WP, Piedra PA. Maternal Immunization Against Viral Disease. Vaccine (1998) 16(14-15):1456–63. doi: 10.1016/S0264-410X(98)00108-X
36. Waaijenborg S, Hahné SJ, Mollema L, Smits GP, Berbers GA, van der Klis FR, et al. Waning of Maternal Antibodies Against Measles, Mumps, Rubella, and Varicella in Communities With Contrasting Vaccination Coverage. J Infect Dis (2013) 208(1):10–6. doi: 10.1093/infdis/jit143
37. Maródi L. Neonatal Innate Immunity to Infectious Agents. Infect Immun (2006) 74(4):1999–2006. doi: 10.1128/IAI.74.4.1999-2006.2006
38. Advisory Committee on Immunization Practices. Guidelines for Vaccinating Pregnant Women. Available at: https://www.cdc.gov/vaccines/pregnancy/hcp-toolkit/guidelines.html (Accessed December 2020).
39. Public Health England. Green Book. Chapter 11: The UK Immunization Schedule. Available at: https://assets.publishing.service.gov.uk/government/uploads/system/uploads/attachment_data/file/855727/Greenbook_chapter_11_UK_Immunisation_schedule.pdf (Accessed December 2020).
40. Abu-Raya B, Maertens K, Edwards KM, Omer SB, Englund JA, Flanagan KL, et al. Global Perspectives on Immunization During Pregnancy and Priorities for Future Research and Development: An International Consensus Statement. Front Immunol (2020) 11:1282. doi: 10.3389/fimmu.2020.01282
41. Abu Raya B, Srugo I, Kessel A, Peterman M, Bader D, Gonen R, et al. The Effect of Timing of Maternal Tetanus, Diphtheria, and Acellular Pertussis (Tdap) Immunization During Pregnancy on Newborn Pertussis Antibody Levels - A Prospective Study. Vaccine (2014) 32:5787–93. doi: 10.1016/j.vaccine.2014.08.038
42. Zimmermann P, Perrett KP, Messina NL, Donath S, Ritz N, van der Klis FRM, et al. The Effect of Maternal Immunisation During Pregnancy on Infant Vaccine Responses. EClinicalMedicine (2019) 13:21–30. doi: 10.1016/j.eclinm.2019.06.010
43. Madhi SA, Polack FP, Piedra PA, Munoz FM, Trenholme AA, Simões EAF, et al. Prepare Study Group. Respiratory Syncytial Virus Vaccination During Pregnancy and Effects in Infants. N Engl J Med (2020) 383:426–34. doi: 10.1056/NEJMoa1908380
44. Obando-Pacheco P, Justicia-Grande AJ, Rivero-Calle I, Rodríguez-Tenreiro C, Sly P, Ramilo O, et al. Respiratory Syncytial Virus Seasonality: A Global Overview. J Infect Dis (2018) 217(9):1356–64. doi: 10.1093/infdis/jiy056
45. Robbie GJ, Zhao L, Mondick J, Losonsky G, Roskos LK. Population Pharmacokinetics of Palivizumab, a Humanized Anti-Respiratory Syncytial Virus Monoclonal Antibody, in Adults and Children. Antimicrob Agents Chemother (2012) 56(9):4927–36. doi: 10.1128/AAC.06446-11
46. AbbVie. Synagis SmPC. Available at: https://www.ema.europa.eu/en/documents/product-information/synagis-epar-product-information_en.pdf (Accessed December 2020).
47. MedImmune. Synagis Prescribing Information. Available at: https://synagis.com/synagis.pdf (Accessed December 2020).
48. Feltes TF, Cabalka AK, Meissner HC, Piazza FM, Carlin DA, Top FH Jr, et al. Palivizumab Prophylaxis Reduces Hospitalization Due to Respiratory Syncytial Virus in Young Children With Hemodynamically Significant Congenital Heart Disease. J Pediatr (2003) 143(4):532–40. doi: 10.1067/S0022-3476(03)00454-2
49. Group I-RS. Palivizumab, a Humanized Respiratory Syncytial Virus Monoclonal Antibody, Reduces Hospitalization From Respiratory Syncytial Virus Infection in High-Risk Infants. The IMpact-RSV Study Group. Pediatrics (1998) 102(3 Pt 1):531–7. doi: 10.1542/peds.102.3.531
50. Ralston SL, Lieberthal AS, Meissner HC, Alverson BK, Baley JE, Gadomski AM, et al. Clinical Practice Guideline: The Diagnosis, Management, and Prevention of Bronchiolitis. Pediatrics (2014) 134(5):e1474–502. doi: 10.1542/peds.2015-2862
51. Martin JA, Hamilton BE, Osterman MJK, Driscoll AK, Drake P. Births: Final Data for 2016. Natl Vital Stat Rep (2018) 67(1):1–55.
52. Sánchez Luna M, Pérez Muñuzuri A, Leante Castellanos JL, Ruiz Campillo CW, Sanz López E, Benavente Fernández I, et al. [An Update of the Recommendations of the Spanish Neonatology Society for the Use of Palivizumab as Prophylaxis for Severe Infections Due to Syncytial Respiratory Virus in High Risk Infants]. An Pediatr (Barc) (2019) 91(5):348–50. doi: 10.1016/j.anpede.2019.08.003
53. Cutrera R, Wolfler A, Picone S, Rossi GA, Gualberti G, Merolla R, et al. Impact of the 2014 American Academy of Pediatrics Recommendation and of the Resulting Limited Financial Coverage by the Italian Medicines Agency for Palivizumab Prophylaxis on the RSV-Associated Hospitalizations in Preterm Infants During the 2016-2017 Epidemic Season: A Systematic Review of Seven Italian Reports. Ital J Pediatr (2019) 45(1):139. doi: 10.1186/s13052-019-0736-5
54. The Association of the Scientific Medical Societies in Germany (AWMF). Guideline for the Prophylaxis of Serious Diseases Caused by Respiratory Syncytial Virus (RSV) in Children at Risk: Update 2017/2018 (2018). Available at: https://www.awmf.org/uploads/tx_szleitlinien/048-012l_S2k_Prophylaxe-von-schweren_RSV-Erkrankungen-Risikokindern-Palivizumab_2018-11.pdf (Accessed December 2020).
55. Domachowske JB, Khan AA, Esser MT, Jensen K, Takas T, Villafana T, et al. Safety, Tolerability and Pharmacokinetics of MEDI8897, an Extended Half-Life Single-Dose Respiratory Syncytial Virus Prefusion F-Targeting Monoclonal Antibody Administered as a Single Dose to Healthy Preterm Infants. Pediatr Infect Dis J (2018) 37(9):886–92. doi: 10.1097/INF.0000000000001916
56. Zhu Q, McLellan JS, Kallewaard NL, Ulbrandt ND, Palaszynski S, Zhang J, et al. A Highly Potent Extended Half-Life Antibody as a Potential RSV Vaccine Surrogate for All Infants. Sci Trans Med (2017) 9(388):eaaj1928. doi: 10.1126/scitranslmed.aaj1928
57. Griffin MP, Yuan Y, Takas T, Domachowske JB, Madhi SA, Manzoni P, et al. Single-Dose Nirsevimab for Prevention of RSV in Preterm Infants. N Engl J Med (2020) 383(5):415–25. doi: 10.1056/NEJMoa1913556
58. ClinicalTrials.gov. A Study to Evaluate the Safety and Efficacy of MEDI8897 for the Prevention of Medically Attended RSV LRTI in Healthy Late Preterm and Term Infants (MELODY). Available at: https://clinicaltrials.gov/ct2/show/NCT03979313?term=MEDI8897&draw=2&rank=3 (Accessed December 2020).
59. ClinicalTrials.gov. A Study to Evaluate the Safety of MEDI8897 for the Prevention of Medically Attended Respiratory Syncytial Virus(RSV) Lower Respiratory Track Infection (LRTI) in High-Risk Children. Available at: https://clinicaltrials.gov/ct2/show/NCT03959488?term=MEDI8897&draw=2&rank=1 (Accessed December 2020).
60. King GE, Hadler SC. Simultaneous Administration of Childhood Vaccines: An Important Public Health Policy That Is Safe and Efficacious. Pediatr Infect Dis J (1994) 13(5):394–407. doi: 10.1097/00006454-199405000-00012
61. Centers for Disease Control and Prevention. Immunization Schedules. Available at: https://www.cdc.gov/vaccines/schedules/hcp/imz/child-adolescent.html (Accessed December 2020).
62. Guzman-Holst A, DeAntonio R, Prado-Cohrs D, Juliao P. Barriers to Vaccination in Latin America: A Systematic Literature Review. Vaccine (2020) 38(3):470–81. doi: 10.1016/j.vaccine.2019.10.088
63. European Vaccination Information Portal. Vaccination Schedules in the EU/EEA. Available at: https://vaccination-info.eu/en/vaccination/when-vaccinate/vaccination-schedules-eueea (Accessed December 2020).
64. World Health Organization. Policy Brief: Guidelines for the Prevention, Care and Treatment of Persons With Chronic Hepatitis B Infection. Available at: https://apps.who.int/iris/bitstream/handle/10665/155081/WHO_HIV_2015.5_eng.pdf;jsessionid=56B10A636F86685D5199F0DA4CF750A9?sequence=1 (Accessed December 2020).
65. Hutchins SS, Escolan J, Markowitz LE, Hawkins C, Kimbler A, Morgan A, et al. Measles Outbreak Among Unvaccinated Preschool-Aged Children: Opportunities Missed by Health Care Providers to Administer Measles Vaccine. Pediatrics (1989) 83(3):369–74.
66. American Academy of Pediatrics. Red Book: Simultaneous Administration of Multiple Vaccines. Available at: https://redbook.solutions.aap.org/chapter.aspx?sectionid=88186975&bookid=1484 (Accessed December 2020).
67. World Health Organization. Recommendations for Routine Immunization. Available at: https://www.who.int/immunization/policy/Immunization_routine_table1.pdf?ua.
68. Dashefsky B, Wald E, Guerra N, Byers C. Safety, Tolerability, and Immunogenicity of Concurrent Administration of Haemophilus Influenzae Type B Conjugate Vaccine (Meningococcal Protein Conjugate) With Either Measles-Mumps-Rubella Vaccine or Diphtheria-Tetanus-Pertussis and Oral Poliovirus Vaccines in 14- to 23-Month-Old Infants. Pediatrics (1990) 85(4 Pt 2):682–9.
69. Deforest A, Long SS, Lischner HW, Girone JA, Clark JL, Srinivasan R, et al. Simultaneous Administration of Measles-Mumps-Rubella Vaccine With Booster Doses of Diphtheria-Tetanus-Pertussis and Poliovirus Vaccines. Pediatrics (1988) 81(2):237–46.
70. Grohskopf LA, Sokolow LZ, Broder KR, Olsen SJ, Karron RA, Jernigan DB, et al. Recommendations of the Advisory Committee on Immunization Practices — United States, 2016–17 Influenza Season. Recomm Rep (2016) 65(5):1–54. doi: 10.15585/mmwr.rr6505a1
71. Leroy Z, Broder K, Menschik D, Shimabukuro T, Martin D. Febrile Seizures After 2010-2011 Influenza Vaccine in Young Children, United States: A Vaccine Safety Signal From the Vaccine Adverse Event Reporting System. Vaccine (2012) 30(11):2020–3. doi: 10.1016/j.vaccine.2011.12.042
72. Tse A, Tseng HF, Greene SK, Vellozzi C, Lee GM. Signal Identification and Evaluation for Risk of Febrile Seizures in Children Following Trivalent Inactivated Influenza Vaccine in the Vaccine Safety Datalink Project, 2010-2011. Vaccine (2012) 30(11):2024–31. doi: 10.1016/j.vaccine.2012.01.027
73. Centers for Disease Control and Prevention. Recommendation of the Advisory Committee on Immunization Practices (ACIP) for Use of Quadrivalent Meningococcal Conjugate Vaccine (MenACWY-D) Among Children Aged 9 Through 23 Months at Increased Risk for Invasive Meningococcal Disease. MMWR Morb Mortal Wkly Rep (2011) 60(40):1391–2.
74. Tomczyk S, Bennett NM, Stoecker C, Gierke R, Moore MR, Whitney CG, et al. Use of 13-Valent Pneumococcal Conjugate Vaccine and 23-Valent Pneumococcal Polysaccharide Vaccine Among Adults Aged ≥65 Years: Recommendations of the Advisory Committee on Immunization Practices (ACIP). MMWR Morb Mortal Wkly Rep (2014) 63(37):822–5.
75. European Medicines Agency. Guideline on Clinical Evaluation of Vaccines (2018). Available at: https://www.ema.europa.eu/en/documents/scientific-guideline/draft-guideline-clinical-evaluation-vaccines-revision-1_en.pdf (Accessed December 2020).
76. European Medicines Agency. Guideline on the Clinical Evaluation of Medicinal Products Indicated for the Prophylaxis or Treatment of Respiratory Syncytial Virus (RSV) Disease (2018). Available at: https://www.ema.europa.eu/en/documents/scientific-guideline/guideline-clinical-evaluation-medicinal-products-indicated-prophylaxis-treatment-respiratory_en.pdf (Accessed December 2020).
77. Rao DS. Overview and Compartmentalization of the Immune System Hematology: Basic Principles and Practice. 7th Edition. New York (NY), USA: Elsevier (2018) p. 199–209.
78. Food and Drug Administration. Respiratory Syncytial Virus Infection: Developing Antiviral Drugs for Prophylaxis and Treatment (Guidance for Industry) (2017). Available at: https://www.fda.gov/media/108437/download (Accessed December 2020).
79. Food and Drug Administration. Guidance Document: Drug-Drug Interaction Assessment for Therapeutic Proteins (Guidance for Industry) (2020). Available at: https://www.fda.gov/media/140909/download (Accessed December 2020).
80. Dohme MS. Zinplava SMPC. Available at: https://www.medicines.org.uk/emc/product/2669#gref (Accessed December 2020).
81. Novartis. Cosentyx SmPC. Available at: https://www.medicines.org.uk/emc/product/3669/smpc#gref (Accessed December 2020).
82. World Health Organization. Preferred Product Characteristics of Monoclonal Antibodies for Passive Immunization Against RSV (2021). Available at: https://apps.who.int/iris/handle/10665/341635 (Accessed July 2021).
83. Public Health England. Green Book. Chapter 6: Contraindications and Special Considerations. Available at: https://assets.publishing.service.gov.uk/government/uploads/system/uploads/attachment_data/file/655225/Greenbook_chapter_6.pdf (Accessed December 2020).
84. Ministère des Solidarités et de la Santé. Calendrier Des Vaccinations Et Recommandations Vaccinales (2020). Available at: https://solidarites-sante.gouv.fr/IMG/pdf/calendrier_vaccinal_29juin20.pdf (Accessed December 2020).
85. Advisory Committee on Immunization Practices. Timing and Spacing of Immunobiologics. Available at: https://www.cdc.gov/vaccines/hcp/acip-recs/general-recs/timing.html (Accessed December 2020).
86. Toishi S EA. Safety Study on Simultaneous Administration of Vaccines and Palivizumab in Children. J-Global (2017) 121(6):1063–6.
87. Public Health England. Green Book. Chapter 27a: Respiratory Syncytial Virus. Available at: https://assets.publishing.service.gov.uk/government/uploads/system/uploads/attachment_data/file/458469/Green_Book_Chapter_27a_v2_0W.PDF (Accessed December 2020).
88. Immunization NACo. Statement on the Recommended Use of Monoclonal Anti-RSV Antibody (Palivizumab). Available at: http://publications.gc.ca/collections/Collection/H12-21-2-29-7.pdf (Accessed December 2020).
89. Centers for Disease Control and Prevention. The Pink Book: Principles of Vaccination. Available at: https://www.cdc.gov/vaccines/pubs/pinkbook/downloads/prinvac.pdf?fbclid=IwAR1Z-PI4jhTBPYQsdbbtaS9O8M3_TfkAGiwTX4l931FImN4gTt0Kmm-GMkA (Accessed December 2020).
90. American Academy of Pediatrics Committee on Infectious Diseases. Updated Guidance for Palivizumab Prophylaxis Among Infants and Young Children at Increased Risk of Hospitalization for Respiratory Syncytial Virus Infection. Pediatrics (2014) 134(2):e620–38. doi: 10.1542/peds.2014-1666
91. Wessel AW, Kose N, Bombardi RG, Roy V, Chantima W, Mongkolsapaya J, et al. Antibodies Targeting Epitopes on the Cell-Surface Form of NS1 Protect Against Zika Virus Infection During Pregnancy. Nat Commun (2020) 11(1):5278. doi: 10.1038/s41467-020-19096-y
92. Man WH, Scheltema NM, Clerc M, van Houten MA, Nibbelke EF, Achten NB, et al. Infant Respiratory Syncytial Virus Prophylaxis and Nasopharyngeal Microbiota Until 6 Years of Life: A Subanalysis of the MAKI Randomised Controlled Trial. Lancet Respir Med (2020) 8(10):1022–31. doi: 10.1016/S2213-2600(19)30470-9
93. Achten NB, Wu P, Bont L, Blanken MO, Gebretsadik T, Chappell JD, et al. Interference Between Respiratory Syncytial Virus and Human Rhinovirus Infection in Infancy. J Infect Dis (2017) 215(7):1102–6. doi: 10.1093/infdis/jix031
Keywords: monoclonal antibodies, nirsevimab, palivizumab, vaccine, RSV
Citation: Esposito S, Abu-Raya B, Bonanni P, Cahn-Sellem F, Flanagan KL, Martinon Torres F, Mejias A, Nadel S, Safadi MAP and Simon A (2021) Coadministration of Anti-Viral Monoclonal Antibodies With Routine Pediatric Vaccines and Implications for Nirsevimab Use: A White Paper. Front. Immunol. 12:708939. doi: 10.3389/fimmu.2021.708939
Received: 12 May 2021; Accepted: 15 July 2021;
Published: 11 August 2021.
Edited by:
Gene S. Tan, J. Craig Venter Institute (La Jolla), United StatesReviewed by:
Wayne Robert Thomas, University of Western Australia, AustraliaGabriel M. Gutierrez, Leidos, United States
Copyright © 2021 Esposito, Abu-Raya, Bonanni, Cahn-Sellem, Flanagan, Martinon Torres, Mejias, Nadel, Safadi and Simon. This is an open-access article distributed under the terms of the Creative Commons Attribution License (CC BY). The use, distribution or reproduction in other forums is permitted, provided the original author(s) and the copyright owner(s) are credited and that the original publication in this journal is cited, in accordance with accepted academic practice. No use, distribution or reproduction is permitted which does not comply with these terms.
*Correspondence: Susanna Esposito, c3VzYW5uYS5lc3Bvc2l0b0B1bmltaS5pdA==