- Instituto de Biofísica Carlos Chagas Filho, Universidade Federal do Rio de Janeiro, Rio de Janeiro, Brazil
In the innate immunity to Leishmania infection tissue-resident macrophages and inflammatory monocytes accumulate host-cell, effector, and efferocytosis functions. In addition, neutrophils, as host, effector, and apoptotic cells, as well as tissue-resident and monocyte-derived dendritic cells (DCs) imprint innate and adaptive immunity to Leishmania parasites. Macrophages develop phenotypes ranging from antimicrobial M1 to parasite-permissive M2, depending on mouse strain, Leishmania species, and T-cell cytokines. The Th1 (IFN-γ) and Th2 (IL-4) cytokines, which induce classically-activated (M1) or alternatively-activated (M2) macrophages, underlie resistance versus susceptibility to leishmaniasis. While macrophage phenotypes have been well discussed, new developments addressed the monocyte functional phenotypes in Leishmania infection. Here, we will emphasize the role of inflammatory monocytes to access how potential host-directed therapies for leishmaniasis, such as all-trans-retinoic acid (ATRA) and the ligand of Receptor Activator of Nuclear Factor-Kappa B (RANKL) might modulate immunity to Leishmania infection, by directly targeting monocytes to develop M1 or M2 phenotypes.
Introduction
Vector-borne Leishmania spp. parasites cause leishmaniasis, characterized by localized lesions in the skin or mucosae, as well as disseminated and visceral diseases, which impact health across the globe, particularly in the most impoverished populations. Leishmaniasis affects 12 million people worldwide, while human vaccines are not available and current treatments induce resistance/side effects (1–4). Leishmania parasites first infect phagocytes, such as inflammatory neutrophils and tissue-resident macrophages, and then spread to inflammatory monocytes and monocyte-derived macrophages and dendritic cells at the infection site (5–9). The different routes of infection (intraperitoneal, subcutaneous, intradermic, the use of needles or insect bites) underly certain discrepancies in the timing and size of major inflammatory cell subsets (9, 10) and need to be considered when comparing different Leishmania experimental models. Undoubtedly, intradermic inoculation mimics better natural infection through sand-fly bites (9).
Environmental stimuli are key to determine macrophage activation and shape macrophage phenotype as permissive host versus effector cells during infection. The M1 and M2 terms, coined in reference to Th1 and Th2 responses, describe T-cell independent, strain-specific macrophage features related to the activation of L-arginine metabolism mediated by induced-NO synthase (iNOS) in M1 macrophages or arginase 1 (Arg1) in M2 macrophages (11). The M1 and M2 phenotypes correlate well with classically- and alternatively-activated macrophages, which are induced by IFN-γ (Th1) and IL-4 (Th2) T-cell derived cytokines, respectively (12–17). Most importantly, M1 and Th1 responses predominate in Leishmania-resistant strains, whereas M2 and Th2 responses underly susceptibility to infection in specific experimental models (18–23). It is assumed, however, that a range of intermediates from M1 to M2, rather than discrete extreme phenotypes, represents better macrophage plasticity in vivo (24, 25).
The role of tissue-resident macrophages and neutrophils in Leishmania infection has been addressed at the cutting-edge of knowledge (8, 9, 26, 27). Previous in vitro studies showed that neutrophils, either as apoptotic or effector cells, interact with elicited-peritoneal macrophages and affect immunity to Leishmania major in a mouse strain-dependent fashion. In resistant C57BL/6 (B6) mice, effector neutrophils helped macrophage activation and parasite killing, whereas in susceptible BALB/c mice, efferocytosis of neutrophils induced Leishmania-permissive macrophages (28, 29). Recent image data revealed that dermis-resident macrophages at the skin infection site express M2 phenotype hallmarks, such as the mannose receptor (MR), and are parasite-permissive hosts despite intense Th1 responses to non-healing isolates of L. major (8, 26). Remarkably, TAM-mediated efferocytosis of infected neutrophils transferred Leishmania parasites to tissue-resident macrophages and reduced innate immunity (9). Similarly, the efferocytosis of infected neutrophils by antigen (Ag)-presenting DCs downmodulated adaptive immunity to Leishmania antigens (La-Ag) (6, 30). Therefore, resistance or susceptibility to Leishmania infection might not correlate strictly with the Th1/Th2 paradigm.
Here we will discuss the role of inflammatory monocytes in Leishmania infection and how we can explore monocyte functional plasticity to shape M1 and M2 responses and promote immunity or restrain exacerbated inflammation.
Effector Versus Suppressor Monocytes in Leishmania Infection
Since early upon Leishmania infection, the CCL2-CCR2 axis mediates recruitment of monocytes to infection site where they act as major players in innate immunity to Leishmania parasites (31, 32). Mononuclear cells recruited to the skin infection site display either effector or host cell functions depending on resistant or susceptible mouse genotype (33–36). In addition, inflammatory monocytes might also play a suppressive role on anti-parasite T-cell responses (37, 38) in analogy to monocytic myeloid-derived suppressor cells (Mo-MDSCs) that compromise anti-tumoral adaptive immunity (39, 40).
The Mo-MDSC acronym highlights the role of monocytic (Ly6C+) cells on T-cell suppression, whereas PMN-MDSC refers to a morphologically and phenotypically (Ly6G+) distinct subset (39). Here, we will focus on inflammatory monocytes and Mo-MDSCs from B6 mice, which express equivalent CD11b+Gr1(Ly6C)+F4/80int phenotypes and morphological aspects, host Leishmania parasites, and function as effector cells to control parasite infection (31, 37, 38).
We previously reported that CD11b+Gr1+F4/80int Mo-MDSCs were present in large proportions in footpad lesions two weeks after L. major infection in B6 mice (37). To investigate the role of Mo-MDSCs in Leishmania infection, we first injected L. major in the peritoneum of B6 mice to generate and sort large numbers of CD11b+Gr1+F4/80int Mo-MDSCs for in vitro and in vivo analyses. Purified Mo-MDSCs expressed monocyte morphology and could be promptly infected in vitro with L. major promastigotes (37). Moreover, Mo-MDSCS controlled infection either spontaneously in a NO-dependent fashion or more efficiently after treatment with T-cell cytokines. Furthermore, co-injection of Mo-MDSCs and Leishmania promastigotes in footpads reduced lesions and parasite numbers in draining lymph nodes (LN), concomitant with reduced ex vivo T-cell proliferation to La-Ag (37). To directly address suppression of Ag-specific responses, Mo-MDSCs were cocultured with draining-LN T-cells collected two weeks after L. major infection. We found that Mo-MDSCs suppressed both polyclonal and Ag-specific T-cell proliferation in a NO-dependent manner (37). Therefore, Mo-MDSCs expressed a NO-producing effector phenotype that might paradoxically suppress subsequent adaptive immunity during L. major infection.
Schmid et al. (38) further addressed the role of Mo-MDSC in L. major infection by comparing resistant B6 with susceptible BALB/c mice. They showed that BM from B6 mouse yielded increased proportions of Mo-MDSCs compared with BALB/c BM. In addition, in GM-CSF treated cultures, B6 BM produced increased frequencies of Mo-MDSCs, whereas BM from BALB/c mice preferentially generated PMN-MDSCs. Moreover, increased numbers of Mo-MDSCs accumulated in foot pad lesions 10 days after infection in B6 compared with BALB/c mice (38). Similar to peritoneal Mo-MDSCs, Mo-MDSCs from B6 BM suppressed CD4 T-cell proliferation to L. major Ag in a NO-dependent mechanism (37, 38). By contrast, Mo-MDSCs from BALB/c BM expressed reduced NO production and are less likely to suppress T-cell responses (38). Therefore, Mo-MDSCs/monocytes play a role as NO-producing effector and suppressor cells in a strain-specific fashion. The observations of increased effector cell responses in inflammatory monocytes from B6 mice might explain, at least in part, innate control of resistance versus susceptibility to Leishmania infection in experimental murine models.
Effector Monocytes and Innate Immunity to Leishmania Infection
To investigate the correlation of monocyte functional phenotypes and effective innate immunity, we took advantage of distinct susceptibility versus resistance of BALB/c mice in L. major versus Leishmania braziliensis models (41). Early studies suggested that resident peritoneal macrophages infected with L. major, but not those infected with L. braziliensis, required activation by T-cell cytokines to fight parasite infection (42). Then, we analysed the functional phenotypes of peritoneal exudate cell (PECs) recruited after Leishmania infection, by conducting kinetic analyses 1-3 dpi, before the establishment of adaptive immunity (36). We observed that CD11b+Ly6C+F4/80int monocytes replaced peritoneal-resident (F4/80hi) macrophages 1-2 days post infection with either L. major or L. braziliensis, both in BALB/c and B6 mice. In BALB/c mice, CD11b+ PECs developed increased frequencies of M1 hallmarks (IL-12p35+ and iNOS+ cells), as well as reduced Arg1 expression in response to L. braziliensis compared with L. major infection. Moreover, restimulation with L. braziliensis parasites induced production of proinflammatory cytokines in vitro, whereas IL-10 predominated in L. major-infected cultures (36).
To address the role of mouse strain, we compared B6 versus BALB/c mice infected with L. braziliensis and observed that CD11b+ PECs from B6 mice expressed increased frequencies of IL-12p35+ and iNOS+ (M1) cells, but reduced proportions of Arg1+ (M2) cells, as well as increased NO production in infected cultures (36). Altogether, monocytes developed M1 responses in a Leishmania spp. (L. braziliensis > L. major)- and mouse genotype (B6>BALB)-dependent fashion. Therefore, the shaping of monocyte phenotypes might be useful to improve M1 responses in therapeutic vaccination of susceptible hosts, based on the benefits of IL-12 administration (43). By contrast, host-directed therapies (2) designed to restrict exacerbated M1 responses to L. braziliensis infection might help the control of inflammation (41, 44, 45) in mucocutaneous leishmaniasis.
Functional Monocyte Responses to CD4 T-Cell Cytokines
When adaptive immunity to Leishmania infection ensues, T-cell cytokines IFN-γ and IL-4 play a major role, by inducing classically and alternatively-activated macrophages. Nevertheless, whereas Th1 and Th2 cytokines correlate well with resistance and susceptibility to infection in certain experimental Leishmania models, some observations do not fit well with this paradigm and the role of IL-4 is particularly controversial (29). Only recently, Carneiro et al. (46) fully addressed how IFN-γ and IL-4 signalling pathways coordinate inflammatory monocyte responses in Leishmania amazonensis infection. Interestingly, although IFN-γ mediated monocyte recruitment to the infection site and induced parasite control by infected monocytes, many other monocytes harboured parasites and sustained infection (46), adding evidence to the notion that monocytes can be parasite-permissive host cells (7, 47). Paradoxically, by downmodulating IFN-γ-mediated recruitment of parasite-permissive monocytes, IL-4 signalling helped resistance rather than susceptibility, particularly in those models where strong Th1 and Th2 responses coexist (46).
In this regard, we previously observed increased Th1 and Th2 responses to L. major in vFLIP (caspase-8 inhibitor) transgenic mice, most likely owing to inhibited Fas-mediated apoptosis of CD4 T cells. Moreover, infected B6.vFLIP mice were more resistant to infection than WT mice in an IL-4-dependent fashion (48). Another non-excluding hypothesis to explain IL-4-mediated resistance to L. major is the synergism between IL-4 and IFN-γ on macrophage activation for parasite killing (49). Likewise, monocytes from L. major-infected B6 mice responded in vitro to recombinant IL-4 and IFN-γ versus IFN-γ alone, by increasing NO-dependent L. major killing (37).
Overall, these studies indicate that monocytes play a key role in innate immunity either as effector cells or permissive hosts for Leishmania parasites and that monocyte phenotypes can be modulated by cytokines in adaptive immunity. Therefore, monocytes express functional plasticity and stand as preferential targets for immunomodulation in infectious and inflammatory diseases.
Discrete M1 (IFN-γ/LPS) and M2 (IL-4)-induced macrophages show polarized responses regarding to L-Arginine metabolism (iNOS versus Arg1), NO-dependent microbial killing versus tissue repair functions, inflammatory cytokines versus certain chemokine and surface marker expression (11–14, 17). To address how mouse strain genotype affects monocyte plasticity, we generated BM-derived macrophages (BMDMs) from B6 and BALB/c in the presence of M-CSF. F4/80int BMDMs were then treated with IFN-γ, IL-4, and LPS to provide altogether the environmental correlates of both M1 and M2 conditions. We showed that BMDMs from B6 and BALB/c mice expressed equivalent M1 responses, such as TNF-α and CXCL9 proinflammatory cytokines, as well as NO production in response to mixed M1/M2 conditions (36). Therefore, BMDMs from BALB/c and B6 mice express similar plasticity to respond to Th1 and Th2 cytokines in the infection environment, mimicked by LPS stimulation. It is likely that these experimental settings (M-CSF followed by mixed cytokines and LPS) compensated the BALB/c deficit in the production of BM-derived monocytes (38). We suggest that stronger proinflammatory stimuli, such as LPS plus IFN-γ or L. braziliensis versus L. major parasites are less likely to unveil functional differences between BALB/c and B6 monocytes.
In addition to the prototype Th1 and Th2 cytokines, we investigated the role of RANKL, which confers resistance to L. major infection in CD40L-defective B6 mice (50). In the B6 model, we cocultured splenic CD4 T cells (from 5 weeks-infected mice) with monocytes (recruited 24 h post i.p. infection), which were then reinfected with L. major parasites. We observed that monocytes secreted IL-12 in response to endogenous RANKL and IFN-γ produced during the crosstalk between CD4 T-cells and infected monocytes. In turn, RANKL-stimulated monocytes increased Th1 responses (51). In the next section, we will discuss how RANKL and IFN-γ affect the phenotype of inflammatory monocytes to promote immunity to L. major infection (51).
Targeting Inflammatory Monocytes: RANKL Helps a M2-Like to M1 Phenotype Shift
RANKL (or TRANCE) binds to the Receptor Activator of Nuclear Factor-Kappa B (RANK) and activates osteoclasts, macrophages, and DCs (52–54). To address the potential of RANKL to modulate monocyte phenotype, we employed inflammatory peritoneal cells induced upon thioglycolate injection, which expressed RANK. In addition, inflammatory monocytes from B6 mice expressed F4/80int and a M2-like phenotype, characterized by heterogeneous expression of M2 markers such as MGL (CD301), mannose receptor (CD206), IL-4Rα and IL-10 (51). Strikingly, treatment with either RANKL or IFN-γ induced monocytes to express F4/80hi and M1 features, such as IL-12p35 and iNOS expression, IL-12 and TNF-α production, coupled with reduced M2 MGL marker (51). We also observed an intermediate M1-M2 subset, which expressed both MGL and iNOS after treatment with RANKL (51). Likewise, monocytes can co-express iNOS and Arg1, where Arg1 outcompetes iNOS for the L-arginine substrate and promotes L. major infection (55). These observations indicate that M2 to M1-phenotypical transition can occur through intermediate phenotypes and it is not an all-or-nothing process.
More importantly, RANKL and suboptimal IFN-γ doses cooperated for the induction of fully-effector M1-macrophages, which showed higher iNOS/NO expression and killed L. major parasites in a NO and ROS-dependent fashion (51). Likewise, RANKL and IFN-γ synergized to increase NO production by BALB/c inflammatory monocytes (51). Overall, these findings extend previous observations (50) and indicate a protective role of RANKL in Leishmania infection, by inducing M1-mediated immunity (51). Nonetheless, the outcomes of treatment with RANKL in vivo depend on the immune context to induce M1 (56) or M2 (57) macrophages. In addition, the systemic therapeutic use of RANKL might affect bone homeostasis, by promoting osteoclast differentiation and activation (54). By contrast, the local uses of RANKL in immunization (58, 59) and in therapeutic vaccines stand as a potential means to improve the activation of DCs and shape macrophage phenotypes in Leishmania infection. Interestingly, both RANKL and its inhibitor osteoprotegerin were detected in cutaneous lesions after human infection with Leishmania tropica (60).
ATRA Affects Monocyte-Mediated Immunity to Leishmania by Skewing M1 Into M2-Phenotype
All-trans-retinoic acid (ATRA), a vitamin A active metabolite that binds to intracellular receptors (61), has been proposed as a potential tool to enhance anti-tumoral immunity, by reducing MDSC-mediated suppression of T cell responses (39, 62). We tested this concept in B6 mice, which were treated with ATRA in the footpads at the time of L. major infection. After 17 days, ATRA improved polyclonal T-cell responses concomitant with reduced spontaneous NO production in draining LNs (37). However, treatment with ATRA also increased development of lesions and parasite loads. Moreover, these deleterious effects were recapitulated in peritoneal exudate cells (PECs) collected 24 h after L. major infection and then reinfected in vitro (37). We observed that treatment with ATRA: (i) increased monocyte differentiation into macrophage; (ii) reduced NO-responses to T cell cytokines; (iii) increased parasite burden in PECs and sorted MDSCs (37). Therefore, ATRA induced monocytes to differentiate into macrophages that were unable to control L. major infection, by uncoupling maturation and development of effector functions in monocyte-derived macrophages.
Because treatment with ATRA during infection could have other effects, such as induction of regulatory T cells (61), we further addressed how ATRA affects monocytes, by injecting ATRA i.p. 24 h after L. major infection. Increased proportions of F4/80+ PECs harboured parasites in the following day after treatment with ATRA, concomitant with reduced detection of inflammatory cytokines in peritoneal exudates from both B6 and BALB/c mice (36). Likewise, reinfection in vitro resulted in increased parasite burden in PECs from mice treated with ATRA (36). We concluded that ATRA induced parasite-permissive macrophages regardless of the B6 or BALB/c background. Similarly, vitamin A supplement increased parasite infection in experimental visceral leishmaniasis (63). Conversely, vitamin A might play a protective role in immunity to extracellular parasites (64, 65). Moreover, vitamin A metabolism is part of the alternative macrophage program (65, 66) and contributes to tissue repair (67).
To formally address whether ATRA interferes with the development of M1 and M2 phenotypes, we used the mixed M1/M2 conditions, as described in the previous section (36). Strikingly, BMDMS from both BALB/c and B6 mice developed reduced M1 responses, such as production of CXCL9 and NO, but increased production of M2 chemokines after treatment with ATRA. Moreover, ATRA reduced iNOS expression in F4/80+ cells both in vitro and in vivo (36). Therefore, irrespective of experimental settings and genetic background, treatment with ATRA skewed M1 into M2 or M2-like phenotype, which might be more permissive to intracellular L. major parasites.
More research is needed to address the potential of ATRA for restricting exacerbated macrophage responses to L. braziliensis infection as it has been suggested for other inflammatory diseases (68). ATRA reduced inflammatory responses in vivo, by limiting NF-κB-activation in macrophages (68). Otherwise, induction of NF-κB signalling pathway underlies increased M1 responses to RANKL plus IFN-γ in inflammatory macrophages (51). Further investigation might formally address the common mechanisms involved in reciprocal M1-M2 phenotype changes induced by ATRA, RANKL (Figure 1), and other potential pharmacological tools.
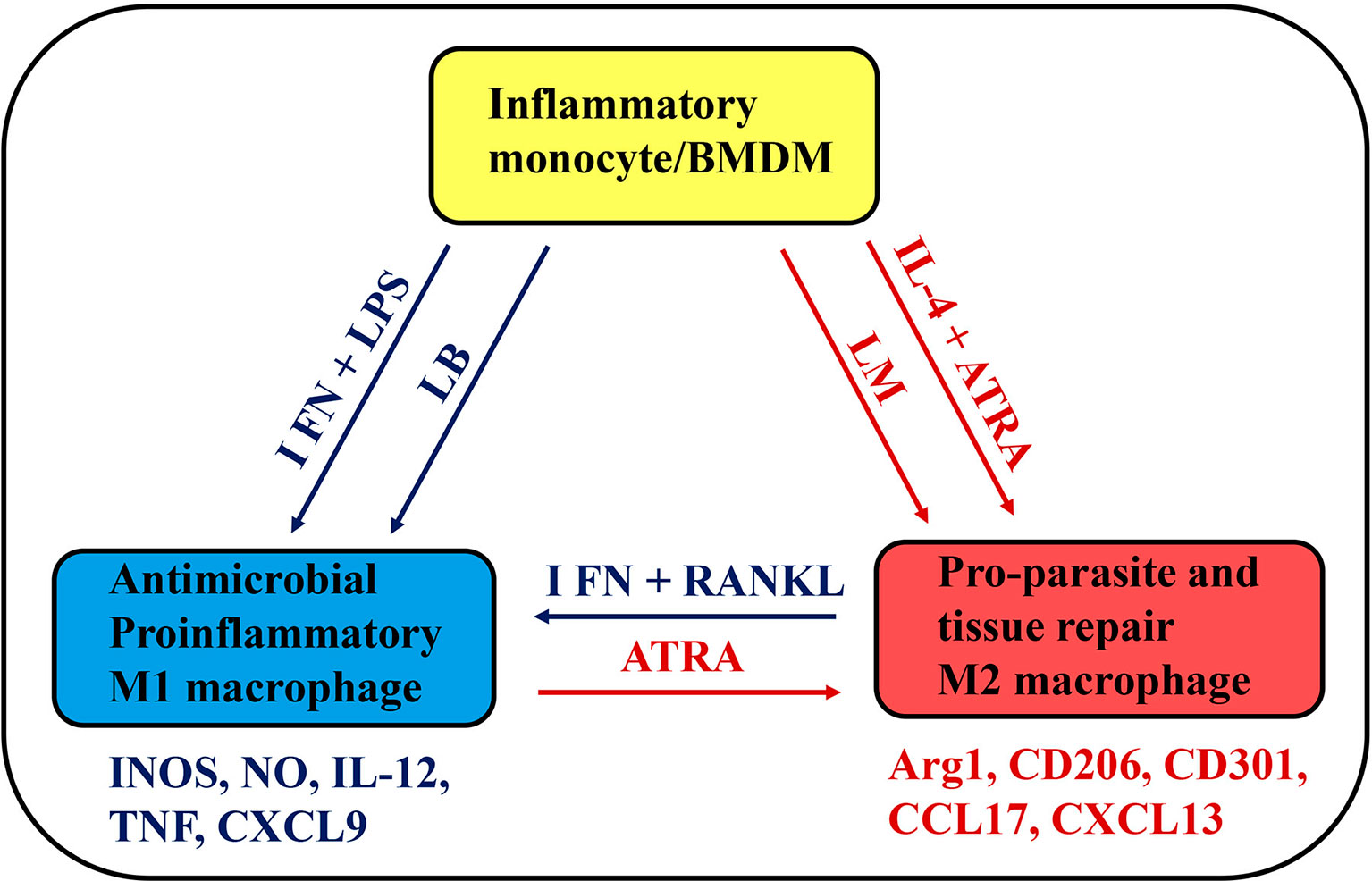
Figure 1 A simplified schematic view of monocyte plasticity as a therapeutic target in Leishmania infection. BMDMS and inflammatory monocytes develop into M1, intermediate (not represented) or M2 macrophages depending on environmental stimuli, such as cytokines (IFN-γ or IL-4) and microbial (LPS) or parasite products. L. braziliensis (LB) and L. major (LM) parasites might differ in their ability to provide stimulus for monocyte activation and differentiation into M1 or M2 macrophages. Potential therapeutic tools, such as RANKL and ATRA have opposing effects on functional phenotypes, by inducing M1 or M2 macrophages, which express a distinct set of skills and play a key role in infection, inflammatory disease, and tissue repair.
Conclusion
The targeting of M1-M2 phenotypes includes a range of cell metabolism, signalling, epigenetics, transcriptional and post-transcriptional (i.e. miRNA) (69) targets, which might be able to guide the generation of therapeutic tools able to modulate exacerbated or defective immune responses in inflammation, autoimmunity, cancer, metabolic, and infectious diseases (16, 27, 66, 70–73).
Additionally, the clearance of apoptotic cells changes monocyte phenotype to promote parasite infection (74, 75) and stands as a potential therapeutic target for other diseases as well (76, 77). In a Leishmania model, efferocytosis contributes to parasite transfer from apoptotic neutrophils to tissue-resident macrophages and inhibits M1 phenotype to favour infection (9). Accordingly, mouse defective in certain efferocytosis receptors had reduced parasite burden coupled with increased pathology (9). This example highlights the need of accessing the possible outcomes of intervening with the multiple effector and tissue repair macrophage functions to advise caution and prevent potential side effects.
Finally, the use of currently-identified therapeutic tools to modulate monocyte phenotypes demands further research to safely translate preclinical findings into clinical trials and reliable therapies.
Author Contributions
NV and TR co-wrote the manuscript and contributed equally to this work. ML analysed the literature and wrote the manuscript. All authors contributed to the article and approved the submitted version.
Funding
This work was supported by the Brazilian National Research Council (Conselho Nacional de Desenvolvimento Científico e Tecnológico, CNPq) and the Rio de Janeiro State Science Foundation (Fundação Carlos Chagas Filho de Amparo à Pesquisa do Estado do Rio de Janeiro, FAPERJ). ML is a research fellow at CNPq, Brazil. We also received fellowships from CNPq (TR), FAPERJ (NV) and the American Association of Immunologists (NV and ML).
Conflict of Interest
The authors declare that the research was conducted in the absence of any commercial or financial relationships that could be construed as a potential conflict of interest.
Acknowledgments
We thank George Alexandre DosReis (in memoriam) for his contributions to this work.
References
1. Okwor I, Uzonna J. Social and Economic Burden of Human Leishmaniasis. Am J Trop Med Hyg (2016) 94(3):489–93. doi: 10.4269/ajtmh.15-0408
2. Novais FO, Amorim CF, Scott P. Host-Directed Therapies for Cutaneous Leishmaniasis. Front Immunol (2021) 12:660183. doi: 10.3389/fimmu.2021.660183
3. Kip AE, Balasegaram M, Beijnen JH, Schellens JH, de Vries PJ, Dorlo TP. Systematic Review of Biomarkers to Monitor Therapeutic Response in Leishmaniasis. Antimicrob Agents Chemother (2015) 59(1):1–14. doi: 10.1128/AAC.04298-14
4. Dorlo TP, Balasegaram M, Beijnen JH, de Vries PJ. Miltefosine: A Review of its Pharmacology and Therapeutic Efficacy in the Treatment of Leishmaniasis. J Antimicrob Chemother (2012) 67(11):2576–97. doi: 10.1093/jac/dks275
5. Peters NC, Egen JG, Secundino N, Debrabant A, Kimblin N, Kamhawi S, et al. In Vivo Imaging Reveals an Essential Role for Neutrophils in Leishmaniasis Transmitted by Sand Flies. Science (2008) 321(5891):970–4. doi: 10.1126/science.1159194
6. Ribeiro-Gomes FL, Peters NC, Debrabant A, Sacks DL. Efficient Capture of Infected Neutrophils by Dendritic Cells in the Skin Inhibits the Early Anti-Leishmania Response. PLoS Pathog (2012) 8(2):e1002536. doi: 10.1371/journal.ppat.1002536
7. Romano A, Carneiro MBH, Doria NA, Roma EH, Ribeiro-Gomes FL, Inbar E, et al. Divergent Roles for Ly6C+CCR2+CX3CR1+ Inflammatory Monocytes During Primary or Secondary Infection of the Skin With the Intra-Phagosomal Pathogen Leishmania major. PLoS Pathog (2017) 13(6):e1006479. doi: 10.1371/journal.ppat.1006479
8. Lee SH, Charmoy M, Romano A, Paun A, Chaves MM, Cope FO, et al. Mannose Receptor High, M2 Dermal Macrophages Mediate Nonhealing Leishmania Major Infection in a Th1 Immune Environment. J Exp Med (2018) 215(1):357–75. doi: 10.1084/jem.20171389
9. Chaves MM, Lee SH, Kamenyeva O, Ghosh K, Peters NC, Sacks D. The Role of Dermis Resident Macrophages and Their Interaction With Neutrophils in the Early Establishment of Leishmania major infection transmitted by sand fly bite. PLoS Pathog (2020) 16(11):e1008674. doi: 10.1371/journal.ppat.1008674
10. Ribeiro-Gomes FL, Roma EH, Carneiro MB, Doria NA, Sacks DL, Peters NC. Site-Dependent Recruitment of Inflammatory Cells Determines the Effective Dose of Leishmania major. Infect Immun (2014) 82(7):2713–27. doi: 10.1128/IAI.01600-13
11. Mills CD, Kincaid K, Alt JM, Heilman MJ, Hill AM. M-1/M-2 Macrophages and the Th1/Th2 Paradigm. J Immunol (2000) 164(12):6166–73. doi: 10.4049/jimmunol.164.12.6166
12. Mantovani A, Sozzani S, Locati M, Allavena P, Sica A. Macrophage Polarization: Tumor-Associated Macrophages as a Paradigm for Polarized M2 Mononuclear Phagocytes. Trends Immunol (2002) 23(11):549–55. doi: 10.1016/s1471-4906(02)02302-5
13. Murray PJ, Wynn TA. Protective and Pathogenic Functions of Macrophage Subsets. Nat Rev Immunol (2011) 11(11):723–37. doi: 10.1038/nri3073
14. Murray PJ, Allen JE, Biswas SK, Fisher EA, Gilroy DW, Goerdt S, et al. Macrophage Activation and Polarization: Nomenclature and Experimental Guidelines. Immunity (2014) 41(1):14–20. doi: 10.1016/j.immuni.2014.06.008
15. Italiani P, Boraschi D. From Monocytes to M1/M2 Macrophages: Phenotypical vs. Functional Differentiation. Front Immunol (2014) 5:514. doi: 10.3389/fimmu.2014.00514
16. Locati M, Curtale G, Mantovani A. Diversity, Mechanisms, and Significance of Macrophage Plasticity. Annu Rev Pathol (2019) 15:123–47. doi: 10.1146/annurev-pathmechdis-012418-012718
17. Martinez FO, Helming L, Gordon S. Alternative Activation of Macrophages: An Immunologic Functional Perspective. Annu Rev Immunol (2009) 27:451–83. doi: 10.1146/annurev.immunol.021908.132532
18. Sacks D, Noben-Trauth N. The Immunology of Susceptibility and Resistance to Leishmania major in Mice. Nat Rev Immunol (2002) 2(11):845–58. doi: 10.1038/nri933
19. McMahon-Pratt D, Alexander J. Does the Leishmania major Paradigm of Pathogenesis and Protection Hold for New World Cutaneous Leishmaniases or the Visceral Disease? Immunol Rev (2004) 201:206–24. doi: 10.1111/j.0105-2896.2004.00190.x
20. Alexander J, Bryson K. T Helper (H)1/Th2 and Leishmania: Paradox Rather Than Paradigm. Immunol Lett (2005) 99(1):17–23. doi: 10.1016/j.imlet.2005.01.009
21. Kaye P, Scott P. Leishmaniasis: Complexity at the Host-Pathogen Interface. Nat Rev Microbiol (2011) 9(8):604–15. doi: 10.1038/nrmicro2608
22. Scott P, Novais FO. Cutaneous Leishmaniasis: Immune Responses in Protection and Pathogenesis. Nat Rev Immunol (2016) 16(9):581–92. doi: 10.1038/nri.2016.72
23. Tomiotto-Pellissier F, Bortoleti B, Assolini JP, Goncalves MD, Carloto ACM, Miranda-Sapla MM, et al. Macrophage Polarization in Leishmaniasis: Broadening Horizons. Front Immunol (2018) 9:2529. doi: 10.3389/fimmu.2018.02529
24. Mosser DM, Edwards JP. Exploring the Full Spectrum of Macrophage Activation. Nat Rev Immunol (2008) 8(12):958–69. doi: 10.1038/nri2448
25. Sica A, Mantovani A. Macrophage Plasticity and Polarization: In Vivo veritas. J Clin Invest (2012) 122(3):787–95. doi: 10.1172/JCI59643
26. Lee SH, Chaves MM, Kamenyeva O, Gazzinelli-Guimaraes PH, Kang B, Pessenda G, et al. M2-Like, Dermal Macrophages Are Maintained Via IL-4/CCL24-Mediated Cooperative Interaction With Eosinophils in Cutaneous Leishmaniasis. Sci Immunol (2020) 5(46):eaaz4415. doi: 10.1126/sciimmunol.aaz4415
27. Bogdan C. Macrophages as Host, Effector and Immunoregulatory Cells in Leishmaniasis: Impact of Tissue Micro-Environment and Metabolism. Cytokine X (2020) 2(4):100041. doi: 10.1016/j.cytox.2020.100041
28. Ribeiro-Gomes FL, Otero AC, Gomes NA, Moniz-De-Souza MC, Cysne-Finkelstein L, Arnholdt AC, et al. Macrophage Interactions With Neutrophils Regulate Leishmania major infection. J Immunol (2004) 172(7):4454–62. doi: 10.4049/jimmunol.172.7.4454
29. Lopes MF, Costa-da-Silva AC, DosReis GA. Innate Immunity to Leishmania Infection: Within Phagocytes. Mediators Inflamm (2014) 2014:754965. doi: 10.1155/2014/754965
30. Ribeiro-Gomes FL, Romano A, Lee S, Roffe E, Peters NC, Debrabant A, et al. Apoptotic Cell Clearance of Leishmania major-Infected Neutrophils by Dendritic Cells Inhibits CD8(+) T-Cell Priming In Vitro by Mer Tyrosine Kinase-Dependent Signaling. Cell Death Dis (2015) 6:e2018. doi: 10.1038/cddis.2015.351
31. Goncalves R, Zhang X, Cohen H, Debrabant A, Mosser DM. Platelet Activation Attracts a Subpopulation of Effector Monocytes to Sites of Leishmania major Infection. J Exp Med (2011) 208(6):1253–65. doi: 10.1084/jem.20101751
32. Costa DL, Lima-Junior DS, Nascimento MS, Sacramento LA, Almeida RP, Carregaro V, et al. CCR2 Signaling Contributes to the Differentiation of Protective Inflammatory Dendritic Cells in Leishmania braziliensis Infection. J Leukocyte Biol (2016) 100(2):423–32. doi: 10.1189/jlb.4A0715-288R
33. Beil WJ, Meinardus-Hager G, Neugebauer DC, Sorg C. Differences in the Onset of the Inflammatory Response to Cutaneous Leishmaniasis in Resistant and Susceptible Mice. J Leukocyte Biol (1992) 52(2):135–42. doi: 10.1002/jlb.52.2.135
34. Sunderkotter C, Kunz M, Steinbrink K, Meinardus-Hager G, Goebeler M, Bildau H, et al. Resistance of Mice to Experimental Leishmaniasis is Associated With More Rapid Appearance of Mature Macrophages In Vitro and In Vivo. J Immunol (1993) 151(9):4891–901.
35. Steinbrink K, Schonlau F, Rescher U, Henseleit U, Vogel T, Sorg C, et al. Ineffective Elimination of Leishmania major by Inflammatory (MRP14-Positive) Subtype of Monocytic Cells. Immunobiology (2000) 202(5):442–59. doi: 10.1016/s0171-2985(00)80103-5
36. Vellozo NS, Pereira-Marques ST, Cabral-Piccin MP, Filardy AA, Ribeiro-Gomes FL, Rigoni TS, et al. All-Trans Retinoic Acid Promotes an M1- to M2-Phenotype Shift and Inhibits Macrophage-Mediated Immunity to Leishmania major. Front Immunol (2017) 8:1560. doi: 10.3389/fimmu.2017.01560
37. Pereira WF, Ribeiro-Gomes FL, Guillermo LV, Vellozo NS, Montalvao F, Dosreis GA, et al. Myeloid-Derived Suppressor Cells Help Protective Immunity to Leishmania major Infection Despite Suppressed T Cell Responses. J Leukocyte Biol (2011) 90(6):1191–7. doi: 10.1189/jlb.1110608
38. Schmid M, Zimara N, Wege AK, Ritter U. Myeloid-Derived Suppressor Cell Functionality and Interaction With Leishmania major Parasites Differ in C57BL/6 and BALB/c Mice. Eur J Immunol (2014) 44(11):3295–306. doi: 10.1002/eji.201344335
39. Gabrilovich DI, Nagaraj S. Myeloid-Derived Suppressor Cells as Regulators of the Immune System. Nat Rev Immunol (2009) 9(3):162–74. doi: 10.1038/nri2506
40. Gallina G, Dolcetti L, Serafini P, De Santo C, Marigo I, Colombo MP, et al. Tumors Induce a Subset of Inflammatory Monocytes With Immunosuppressive Activity on CD8+ T Cells. J Clin Invest (2006) 116(10):2777–90. doi: 10.1172/JCI28828
41. Rocha FJ, Schleicher U, Mattner J, Alber G, Bogdan C. Cytokines, Signaling Pathways, and Effector Molecules Required for the Control of Leishmania (Viannia) braziliensis in Mice. Infect Immun (2007) 75(8):3823–32. doi: 10.1128/IAI.01335-06
42. Scott P, Sher A. A Spectrum in the Susceptibility of Leishmanial Strains to Intracellular Killing by Murine Macrophages. J Immunol (1986) 136(4):1461–6.
43. Fernandes AP, Carvalho FA, Tavares CA, Santiago HC, Castro GA, Tafuri WL, et al. Combined interleukin-12 and Topical Chemotherapy for Established Leishmaniasis Drastically Reduces Tissue Parasitism and Relapses in Susceptible Mice. J Infect Dis (2001) 183(11):1646–52. doi: 10.1086/320699
44. Giudice A, Vendrame C, Bezerra C, Carvalho LP, Delavechia T, Carvalho EM, et al. Macrophages Participate in Host Protection and the Disease Pathology Associated With Leishmania braziliensis Infection. BMC Infect Dis (2012) 12:75. doi: 10.1186/1471-2334-12-75
45. Reverte M, Eren RO, Jha B, Desponds C, Snaka T, Prevel F, et al. The Antioxidant Response Favors Leishmania Parasites Survival, Limits Inflammation and Reprograms the Host Cell Metabolism. PLoS Pathog (2021) 17(3):e1009422. doi: 10.1371/journal.ppat.1009422
46. Carneiro MB, Lopes ME, Hohman LS, Romano A, David BA, Kratofil R, et al. Th1-Th2 Cross-Regulation Controls Early Leishmania Infection in the Skin by Modulating the Size of the Permissive Monocytic Host Cell Reservoir. Cell Host Microbe (2020) 27(5):752–68.e7. doi: 10.1016/j.chom.2020.03.011
47. Heyde S, Philipsen L, Formaglio P, Fu Y, Baars I, Hobbel G, et al. CD11c-Expressing Ly6C+CCR2+ Monocytes Constitute a Reservoir for Efficient Leishmania Proliferation and Cell-to-Cell Transmission. PLoS Pathog (2018) 14(10):e1007374. doi: 10.1371/journal.ppat.1007374
48. Pereira-Manfro WF, Ribeiro-Gomes FL, Filardy AA, Vellozo NS, Guillermo LV, Silva EM, et al. Inhibition of Caspase-8 Activity Promotes Protective Th1- and Th2-mediated Immunity to Leishmania major Infection. J Leukoc Biol (2014) 95(2):347–55. doi: 10.1189/jlb.0912463
49. Bogdan C, Stenger S, Rollinghoff M, Solbach W. Cytokine Interactions in Experimental Cutaneous Leishmaniasis. Interleukin 4 Synergizes With Interferon-Gamma to Activate Murine Macrophages for Killing of Leishmania major Amastigotes. Eur J Immunol (1991) 21(2):327–33. doi: 10.1002/eji.1830210213
50. Padigel UM, Kim N, Choi Y, Farrell JP. Trance-RANK Costimulation is Required for IL-12 Production and the Initiation of a Th1-Type Response to Leishmania major Infection in CD40L-deficient Mice. J Immunol (2003) 171(10):5437–41. doi: 10.4049/jimmunol.171.10.5437
51. Rigoni TS, Vellozo NS, Cabral-Piccin M, Fabiano-Coelho L, Lopes UG, Filardy AA, et al. Rank Ligand Helps Immunity to Leishmania major by Skewing M2-Like Into M1 Macrophages. Front Immunol (2020) 11:886. doi: 10.3389/fimmu.2020.00886
52. Anderson DM, Maraskovsky E, Billingsley WL, Dougall WC, Tometsko ME, Roux ER, et al. A Homologue of the TNF Receptor and its Ligand Enhance T-Cell Growth and Dendritic-Cell Function. Nature (1997) 390(6656):175–9. doi: 10.1038/36593
53. Wong BR, Josien R, Lee SY, Sauter B, Li HL, Steinman RM, et al. TRANCE (Tumor Necrosis Factor [TNF]-Related Activation-Induced Cytokine), a New TNF Family Member Predominantly Expressed in T Cells, Is a Dendritic Cell-Specific Survival Factor. J Exp Med (1997) 186(12):2075–80. doi: 10.1084/jem.186.12.2075
54. Lacey DL, Timms E, Tan HL, Kelley MJ, Dunstan CR, Burgess T, et al. Osteoprotegerin Ligand Is a Cytokine That Regulates Osteoclast Differentiation and Activation. Cell (1998) 93(2):165–76. doi: 10.1016/s0092-8674(00)81569-x
55. Schleicher U, Paduch K, Debus A, Obermeyer S, Konig T, Kling JC, et al. Tnf-Mediated Restriction of Arginase 1 Expression in Myeloid Cells Triggers Type 2 NO Synthase Activity at the Site of Infection. Cell Rep (2016) 15(5):1062–75. doi: 10.1016/j.celrep.2016.04.001
56. Huang R, Wang X, Zhou Y, Xiao Y. RANKL-Induced M1 Macrophages are Involved in Bone Formation. Bone Res (2017) 5:17019. doi: 10.1038/boneres.2017.19
57. Meng YH, Zhou WJ, Jin LP, Liu LB, Chang KK, Mei J, et al. RANKL-Mediated Harmonious Dialogue Between Fetus and Mother Guarantees Smooth Gestation by Inducing Decidual M2 Macrophage Polarization. Cell Death Dis (2017) 8(10):e3105. doi: 10.1038/cddis.2017.505
58. Josien R, Li HL, Ingulli E, Sarma S, Wong BR, Vologodskaia M, et al. TRANCE, A Tumor Necrosis Factor Family Member, Enhances the Longevity and Adjuvant Properties of Dendritic Cells In Vivo. J Exp Med (2000) 191(3):495–502. doi: 10.1084/jem.191.3.495
59. Miyahira Y, Akiba H, Katae M, Kubota K, Kobayashi S, Takeuchi T, et al. Cutting Edge: A Potent Adjuvant Effect of Ligand to Receptor Activator of NF-Kappa B Gene for Inducing Antigen-Specific CD8+ T Cell Response by DNA and Viral Vector Vaccination. J Immunol (2003) 171(12):6344–8. doi: 10.4049/jimmunol.171.12.6344
60. Taslimi Y, Agbajogu C, Brynjolfsson SF, Masoudzadeh N, Mashayekhi V, Gharibzadeh S, et al. Profiling Inflammatory Response in Lesions of Cutaneous Leishmaniasis Patients Using a Non-Invasive Sampling Method Combined With a High-Throughput Protein Detection Assay. Cytokine (2020) 130:155056. doi: 10.1016/j.cyto.2020.155056
61. Hall JA, Grainger JR, Spencer SP, Belkaid Y. The Role of Retinoic Acid in Tolerance and Immunity. Immunity (2011) 35(1):13–22. doi: 10.1016/j.immuni.2011.07.002
62. Nefedova Y, Fishman M, Sherman S, Wang X, Beg AA, Gabrilovich DI. Mechanism of All-Trans Retinoic Acid Effect on Tumor-Associated Myeloid-Derived Suppressor Cells. Cancer Res (2007) 67(22):11021–8. doi: 10.1158/0008-5472.CAN-07-2593
63. Garg R, Singh N, Dube A. Intake of Nutrient Supplements Affects Multiplication of Leishmania donovani in Hamsters. Parasitology (2004) 129(Pt 6):685–91. doi: 10.1017/s0031182004006055
64. Gundra UM, Girgis NM, Gonzalez MA, San Tang M, van der Zande HJP, Lin JD, et al. Vitamin A Mediates Conversion of Monocyte-Derived Macrophages Into Tissue-Resident Macrophages During Alternative Activation. Nat Immunol (2017) 18(6):642–53. doi: 10.1038/ni.3734
65. Broadhurst MJ, Leung JM, Lim KC, Girgis NM, Gundra UM, Fallon PG, et al. Upregulation of Retinal Dehydrogenase 2 in Alternatively Activated Macrophages During Retinoid-Dependent Type-2 Immunity to Helminth Infection in Mice. PLoS Pathog (2012) 8(8):e1002883. doi: 10.1371/journal.ppat.1002883
66. Ouimet M, Ediriweera HN, Gundra UM, Sheedy FJ, Ramkhelawon B, Hutchison SB, et al. MicroRNA-33-Dependent Regulation of Macrophage Metabolism Directs Immune Cell Polarization in Atherosclerosis. J Clin Invest (2015) 125(12):4334–48. doi: 10.1172/JCI81676
67. Chiba T, Skrypnyk NI, Skvarca LB, Penchev R, Zhang KX, Rochon ER, et al. Retinoic Acid Signaling Coordinates Macrophage-Dependent Injury and Repair After AKI. J Am Soc Nephrol (2016) 27(2):495–508. doi: 10.1681/ASN.2014111108
68. Hong K, Zhang Y, Guo Y, Xie J, Wang J, He X, et al. All-Trans Retinoic Acid Attenuates Experimental Colitis Through Inhibition of NF-kappaB Signaling. Immunol Lett (2014) 162(1 Pt A):34–40. doi: 10.1016/j.imlet.2014.06.011
69. Das S, Mukherjee S, Ali N. Super Enhancer-Mediated Transcription of miR146a-5p Drives M2 Polarization During Leishmania donovani infection. PLoS Pathog (2021) 17(2):e1009343. doi: 10.1371/journal.ppat.1009343
70. Lee B, Wu CY, Lin YW, Park SW, Wei LN. Synergistic Activation of Arg1 Gene by Retinoic Acid and IL-4 Involves Chromatin Remodeling for Transcription Initiation and Elongation Coupling. Nucleic Acids Res (2016) 44(16):7568–79. doi: 10.1093/nar/gkw392
71. Cabanel M, Brand C, Oliveira-Nunes MC, Cabral-Piccin MP, Lopes MF, Brito JM, et al. Epigenetic Control of Macrophage Shape Transition Towards an Atypical Elongated Phenotype by Histone Deacetylase Activity. PLoS One (2015) 10(7):e0132984. doi: 10.1371/journal.pone.0132984
72. Climaco-Arvizu S, Dominguez-Acosta O, Cabanas-Cortes MA, Rodriguez-Sosa M, Gonzalez FJ, Vega L, et al. Aryl Hydrocarbon Receptor Influences Nitric Oxide and Arginine Production and Alters M1/M2 Macrophage Polarization. Life Sci (2016) 155:76–84. doi: 10.1016/j.lfs.2016.05.001
73. Allman WR, Dey R, Liu L, Siddiqui S, Coleman AS, Bhattacharya P, et al. TACI Deficiency Leads to Alternatively Activated Macrophage Phenotype and Susceptibility to Leishmania Infection. Proc Natl Acad Sci U S A (2015) 112(30):E4094–103. doi: 10.1073/pnas.1421580112
74. Freire-de-Lima CG, Nascimento DO, Soares MB, Bozza PT, Castro-Faria-Neto HC, de Mello FG, et al. Uptake of Apoptotic Cells Drives the Growth of a Pathogenic Trypanosome in Macrophages. Nature (2000) 403(6766):199–203. doi: 10.1038/35003208
75. Cabral-Piccin MP, Guillermo LV, Vellozo NS, Filardy AA, Pereira-Marques ST, Rigoni TS, et al. Apoptotic CD8 T-Lymphocytes Disable Macrophage-Mediated Immunity to Trypanosoma Cruzi Infection. Cell Death Dis (2016) 7:e2232. doi: 10.1038/cddis.2016.135
76. Bosurgi L, Cao YG, Cabeza-Cabrerizo M, Tucci A, Hughes LD, Kong Y, et al. Macrophage Function in Tissue Repair and Remodeling Requires IL-4 or IL-13 With Apoptotic Cells. Science (2017) 356(6342):1072–6. doi: 10.1126/science.aai8132
Keywords: ATRA, Leishmania major, M1 and M2 macrophages, monocytes, nitric oxide, RANKL
Citation: Vellozo NS, Rigoni TS and Lopes MF (2021) New Therapeutic Tools to Shape Monocyte Functional Phenotypes in Leishmaniasis. Front. Immunol. 12:704429. doi: 10.3389/fimmu.2021.704429
Received: 03 May 2021; Accepted: 14 June 2021;
Published: 25 June 2021.
Edited by:
Tamás Laskay, University of Lübeck, GermanyReviewed by:
Sandra Marcia Muxel, University of São Paulo, BrazilWendy W. J. Unger, Erasmus MC-Sophia Children’s Hospital, Netherlands
Copyright © 2021 Vellozo, Rigoni and Lopes. This is an open-access article distributed under the terms of the Creative Commons Attribution License (CC BY). The use, distribution or reproduction in other forums is permitted, provided the original author(s) and the copyright owner(s) are credited and that the original publication in this journal is cited, in accordance with accepted academic practice. No use, distribution or reproduction is permitted which does not comply with these terms.
*Correspondence: Marcela F. Lopes, bWFyY2VsYWxAYmlvZi51ZnJqLmJy
†These authors share first authorship