- 1Department of Physiology, Faculty of Medicine, University of Malaya, Kuala Lumpur, Malaysia
- 2Neuroinflammation Group, Department of Biomedical Sciences, Faculty of Medicine and Health Sciences, Macquarie University, Sydney, NSW, Australia
- 3Psychoneuroimmunology Research Group, European Collaborative Project, Munich, Germany
- 4Department of Pre-Clinical Sciences, Faculty of Medicine and Health Sciences, Universiti Tunku Abdul Rahman, Bandar Sungai Long, Malaysia
- 5Department of Parasitology, Faculty of Medicine, University of Malaya, Kuala Lumpur, Malaysia
- 6Department of Paediatrics, Faculty of Medicine, University of Malaya, Kuala Lumpur, Malaysia
Recurrent abdominal pain (RAP) is a common medically unexplained symptom among children worldwide. However, the biological mechanisms behind the development of functional and behavioral symptoms and changes in blood markers have not been well explored. This study aimed to assess changes in the concentrations of inflammatory markers, including cytokines and tryptophan catabolites, in the serum of children with RAP compared to those with subclinical infections. Children with RAP but without organic diseases were included, and those with asymptomatic intestinal parasitic infections were used as a subclinical infection cohort. Blood samples were collected and used to measure the cytokine profile using Multiplex Immunoassay and tryptophan catabolites using high performance liquid chromatography. Children with RAP showed significantly higher concentrations of serum tumor necrotic factor-α, p<0.05, but lower concentrations of IL-10, p<0.001, IL-6, p<0.001 and brain-derived neurotrophic factors (BDNF) p<0.01. In addition, a significant increase in the metabolite of the kynurenine pathway, 3-hydroxyanthranilic acid (3-HAA) p<0.01, a significant decrease in the concentrations of anthranilic acid (AA) p<0.001, together with an increased ratio of serum 3-HAA to AA (3-HAA/AA) p<0.001, was found in this cohort. These findings indicate the significant activation of the immune system and presence of inflammation in children with RAP than those with subclinical parasitic infections. Moreover, children with RAP tested with the Strengths and Difficulties Questionnaire (SDQ), displayed high psychological problems though these SDQ scores were not statistically associated with measured cytokines and kynurenine metabolites. We however could hypothesize that the pro-inflammatory state together with concomitant low concentrations of BDNF in those children with RAP could play a role in psychological stress and experiencing medically unexplained symptoms.
Introduction
In paediatric primary care, children display symptoms that commonly include headache, fatigue and abdominal pain, but only about 10% of these symptoms have an identifiable infectious or metabolic aetiology (1). The term “medically unexplained symptoms (MUS)” is routinely used in paediatric literature to describe those cases (2). MUS or functional symptoms are defined as “somatic symptoms” where no clear infectious or metabolic cause can be identified after comprehensive medical assessment (3, 4). MUS are broad and most of the time, transient and self-limiting.
However, in most MUS cases, the underlying pathophysiology of abdominal pain cannot be identified and is found to be linked with a psychogenic origin. The term “recurrent abdominal pain (RAP)” was used by Apley to refer to the presence of 3 or more discrete episodes of pain over a period of at least 3 months, interfering with normal daily activity (5). RAP prevalence varies, ranging from 10.8% in British school children (5), 10-20% in school-going Singaporean children (1, 6) to 26.9% in Australian students (7). In Malaysia, the overall prevalence of RAP among school children aged from 11 to 16 years was 10.2% (8).
The pathophysiology of RAP and MUS in children is still not well understood. Evidence has shown that these symptoms can be triggered by recent stressful life events (9) and emotional stress (5). It is well established that chronic stress has a significant impact on the hypothalamo-pituitary adrenal (HPA) axis (10) and also on the immune system through inducing the secretion of pro-inflammatory mediators (11, 12). The evidence of stress- induced inflammation is well documented in young adults. Growing up in a socially tough environment (13), childhood abuse (14) and maltreatment (15), and also acute stressful life events (16) are all associated with an increased production of pro-inflammatory cytokines. A concomitant decreased sensitivity of immune cell to anti-inflammatory mediators has also been reported (17). Imbalance between the concentrations of pro-inflammatory- and anti-inflammatory cytokines is associated with the development of psychological illnesses (18–20). Moreover, neurotrophin, brain-derived neurotrophic factor (BDNF), appears to bridge the environmental challenges with enduring change in neuronal function through HPA axis and immune modulation, hence, failure of neuronal adaptive capability may also implicate in development of psychopathological and neurodegenerative diseases (21, 22). When combined, it is likely that a stress-induced immune activation could be associated with the development of symptoms in children with RAP. However, to our knowledge, there is no published literature that has looked at neurotrophin and cytokine responses in children with RAP.
The kynurenine pathway (KP) of the tryptophan (TRP) metabolism is considered to be a key cross-talk between immune and neuroendocrine systems (23–25). Stress through the increase in cortisol concentrations has a significant impact on the immune system imbalance diverting TRP metabolism away from serotonin and melatonin production towards production of the KP neuroactive metabolites (24, 26–31). The KP can be initiated by activation of indoleamine 2, 3-dioxygenase (IDO-1) in most organs and brain cells including astrocytes, microglia, microvascular endothelial cells and infiltrating macrophages (32) or tryptophan 2, 3-dioxygenase (TDO-2) in liver, kidney and brain (33). While TDO-2 is activated by cortisol, IDO-1 is induced by pro-inflammatory mediators especially interferon-γ (IFN-γ) (31, 34). The key branching point in the KP is the formation of the first stable intermediate kynurenine (KYN) (Figure 1). In the context of neuroinflammation, KYN can be converted in 3 ways: 1) by the kynurenine aminotransferases (KATs) into neuroprotective compound kynurenic acid (KYNA) (35, 36) or 2) to neurotoxic metabolites free-radical generator 3-hydroxykynurenine (3-HK), or 3) by kynureninase (KYNU) into anthranilic acid (AA) which can convert to the pro-oxidant and immunomodulatory metabolites 3-hydroxyanthranilic acid (3-HAA). 3-HAA is then metabolized to the excitotoxin quinolinic acid (QUIN) (37). Thus, the involvement of KP in pathophysiology of MUS is highly possible.
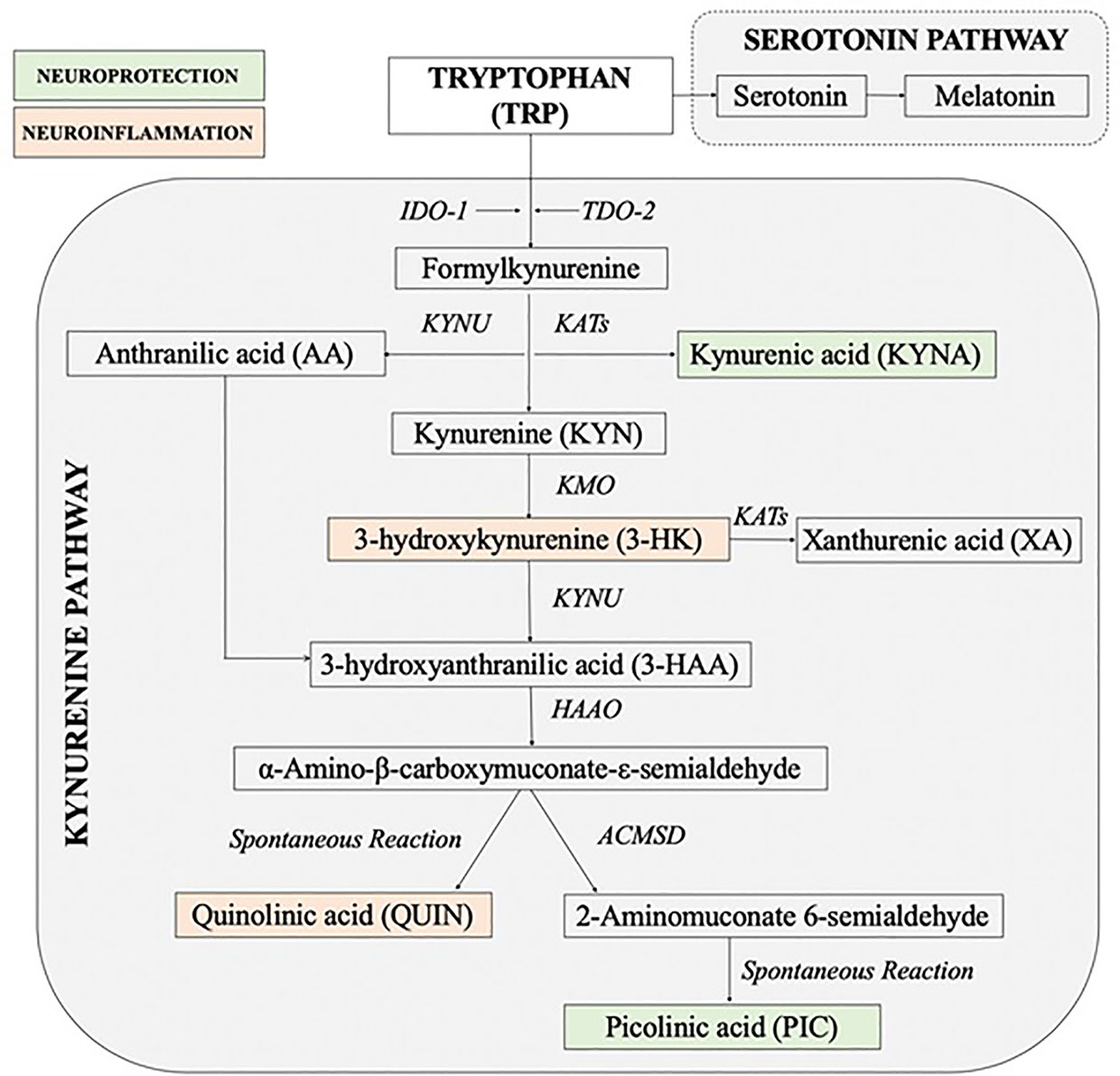
Figure 1 Simplified diagram of the kynurenine pathway [Adapted and modified from (26)]. 3-HAAO, 3-hydroxyanthranilic acid dioxygenase; ACMSD, aminocarboxymuconate semialdehyde decarboxylase; IDO, indoleamine 2, 3-dioxygenase; KATs, kynurenine aminotransferases; KMO, kynurenine 3-monooxygenase; KYNU, kynureninase; TDO, tryptophan 2, 3-dioxygenase.
The other problem associated with abdominal discomfort in paediatric primary care is intestinal helminth parasitic infection. A large proportion of children infected by parasites are relatively asymptomatic and they belong to the category of subclinical infections. A recent study showed that gastrointestinal nematodes, particularly infections with Ascaris, hookworm, and Trichuris species can interfere with immune regulation (38). A further study demonstrated that another type of parasitic infection with Toxoplasma gondii in animal model could alter the behavior of rodents and induce tryptophan degradation through the KP (39). Toxoplasmosis is associated with the incidence of specific neuropsychiatric conditions in humans (40). Understanding the biological mechanisms behind the complex interactions between parasites and the host immunoregulatory networks to maintain an asymptomatic status during enteric infections are of great interest to researchers and health professionals.
As mentioned above, the dysregulation of the KP through immune-neuroendocrine interactions is implicated in the development of psychological symptoms and neurodegenerative disorders. However, changes in the level of expression of this pathway during chronic stress and subclinical infections have remained unknown. Thus, the main aim of this study is to assess the possible changes in serum levels of neurotrophin, cytokines and KP metabolites in the children with RAP and those with parasitic infections.
Materials and Methods
Study Setting and Design
Ethical approval was obtained from the Ethics Committee of the University Malaya Medical Centre (UMMC) Malaysia (MEC Ref. No. 1017.24) prior to the commencement of the study.
Recruitment of Children With RAP
The children in this study have been attending the outpatient paediatrics clinics at the University of Malaya Medical Centre and University of Malaya Specialist Centre. Complying symptoms of abdominal pain and discomfort were closely monitored in male and female children between 7 to 12 years of age. The children met the RAP criteria set up by Apley (5), (i.e. presence of at least 3 discrete episodes of pain over a period of at least 3 months, interfering with normal daily activity). Patients with no abnormal findings on physical examination, routine blood, urine, and stool tests, abdominal ultrasonographic examination and/or no organic diseases as screened by pediatricians have been considered as children with RAP and included in the study. Strict exclusion criteria were applied for those with abnormal laboratory results, abnormal endoscopy results and any findings suggesting an organic disease. After obtaining written consent from the parent, 10 ml of blood (in a serum-separating tube) was collected from each child between 1630-1830 hr. Blood samples were then centrifuged for 20 min at 2000 rpm at 4°C. Serum was collected, aliquoted and stored at -80°C for quantification of BDNF, cytokines and tryptophan metabolites.
After confirming the absence of organic disease, the mental health status of this cohort of children was further assessed. Children were also requested to complete a validated 25-point Strength and Difficulty Questionnaire (SDQ) to assess their emotional and behavioral problems. This questionnaire is designed to assess the 5 subscales of psychological attributes: emotional symptoms, conduct problems, hyperactivity/inattention, peer relationship problems and prosocial behavior (41, 42). For the younger children, aged 4-10, a Parent Report Measures for Children and Adolescents SDQ (P) 04-10 (English/Malay Language) was used and parents were asked to complete the questionnaires (43). The older children, aged 11 years and above, were asked to complete the Self-Report Measures for Children and Adolescents SDQ (S) 11-17 (English/Indonesia Language) (44).
Recruitment of Children With Subclinical Infection
Children with asymptomatic parasitic infections were considered as “apparently healthy children with subclinical infections”. As intestinal parasitic infections are highly prevalent among children in the peninsular Malaysian Orang Asli population (45–47), we used samples collected from this community as a part of a cross-sectional study. The original study aimed to determine the prevalence of intestinal helminth infections. The demographic data was obtained and fecal and serum samples collected.
The fecal samples were screened for the presence of ova, cysts, oocysts and parasites. Samples of children 7 to 12 years old that were microscopically positive for either one or more of Trichuris, Ascaris, hookworm, Entamoeba, Giardia and Hymenolepis nana infection, and also revealed no history of abdominal pain on screening by questionnaire, were used in this study. Their serum samples were tested for BDNF, cytokines and KP metabolites. Any positive samples from children with a history of gastrointestinal symptoms (abdominal discomfort, abdominal pain, diarrhea, etc.) were excluded from the study.
Determination of Serum Samples
The concentrations of cytokines, interleukin (IL)-6, IL-10 and tumor necrosis factor-alpha (TNF-α) were quantified using ProcartaPlex multiplex immunoassay (Affymetrix, eBioscience) at the i-DNA laboratory in Kuala Lumpur, Malaysia. The assay was performed according to the manufacturer’s guide, using recommended buffers, diluents and substrates. The endogenous concentration of each individual cytokine analyte was determined based on the respective sample absorbance using a four-parameter logistics curve. The intra-assay coefficient of variation (CV) (calculated as the mean of the CVs for each sample’s duplicate measurements) ranged from 8.36 to 11.42% for all cytokines with limit of detection (LOD) (pg/ml) of 0.34 for BDNF, 0.14 for IL-10, 1.64 for IL-6 and 0.24 for TNF-α.
Serum TRP, KYN, AA, 3-HK and 3-HAA levels were analyzed using ultra high performance liquid chromatography (UHPLC), at the Faculty of Medicine and Health Sciences, Macquarie University, Australia. Concurrent quantification of TRP, KYN and its metabolites were performed based on the method previously described by Guillemin et al. (48) with slight modification (49). An Agilent 1290 infinity UHPLC system, coupled with variable thermostatted volume auto-sampler, the diode-array detector with optofluidic wave guides, fluorescence detector and an Agilent ZORBAX Eclipse Plus C18 reverse-phase column, was used to perform the analytical liquid chromatography. A 0.1 M sodium acetate buffer (sodium acetate 8.2 g in 1 L of ultrapure MilliQ water) with adjusted pH 4.60 (by addition with hydrochloric acid) was used for mobile phase. The concentrations of KYN and 3-HK were quantified spectrophotometrically, at UV absorbance 365 nm with retention time 3.1 and 1.2 minutes, respectively. The TRP, 3-HAA and AA were measured fluorimetrically at specific excitation/emission (λex/λem) wavelength and retention time (RT); TRP (Ex280 nm/Em438 nm, RT 7.4 min), 3-HAA (Ex320 nm/Em438 nm, RT 3.3 min) and AA (Ex320 nm/Em438 nm, RT 9.8 min). The intra-assay CVs were within the acceptable range of 4-8% for all metabolites. Each measurement was performed in duplicate using deproteinized serum samples. Ten percent trichloroacetic acid (2 g of trichloroacetic acid in 20 mL of ultrapure water) was used to remove the proteins in the samples by precipitation. The targeted different amino acid standards were prepared from respective highest purity (≥98.0%) stock standards (Sigma–Aldrich, Germany). The LOD/limit of quantification (LOQ) were TRP (0.07 uM/0.22 uM), KYN (0.13 uM/0.39 uM), 3-HK (17.79 nM/53.91 nM), 3-HAA (7.06 nM/21.41 nM) and AA (2.48 nM/7.5 nM).
Statistical Analysis
The SDQ total scores and each subscale score were analyzed according to method used by Goodman (43). In all five subscales of SDQ; emotional problems; conduct disorders; hyperactivity disorders; peer problems and pro-social behaviors; mental health status was predicted as being “probable” (high risk), “possible” (borderline) or “unlikely” (normal).
For the serum parameters, the results were screened for the normality of data by the Shapiro–Wilk test. For normally distributed data, unpaired t test with Welch’s correction was used to compare the differences between two independent groups and the results are expressed as mean ± SEM. The Mann-Whitney U test (Wilcoxon two-sample test) was used for those data that were not normally distributed and results are expressed as median and interquartile range (IQR). Correlation between the parameters was analyzed using the Spearman correlation matrix. A p-value < 0.05 was considered significant. All statistical analyses were performed using a GraphPad Prism 9.
Results
Analysis of SDQ Scores for Children With RAP
Risk of mental health problems as screened by SDQ total scores and each subscale scores are presented in Table 1. Twenty and 40% of children are at high and possible risk of mental health problems, respectively.
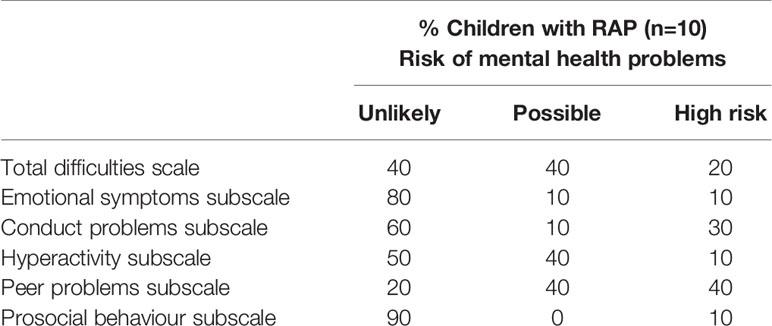
Table 1 Risk of mental health problems as screened by SDQ total scores and each subscale scores in children with RAP.
Cytokine Profiles in Children With RAP and Children With Subclinical Parasitic Infections
The concentrations of BDNF and cytokines in the serum samples from the 2 cohorts of children are shown in Tables 2a–b. The BDNF concentrations were significantly lower (p<0.01) in children with RAP compared to children with subclinical parasitic infections. Higher concentrations of pro-inflammatory cytokines, TNF-α (p<0.05) and lower concentrations of anti-inflammatory cytokines, IL-10 (p<0.001) were also observed in children with RAP. Meanwhile, the concentrations of IL-6 (p<0.001) were higher in children with parasitic infections.
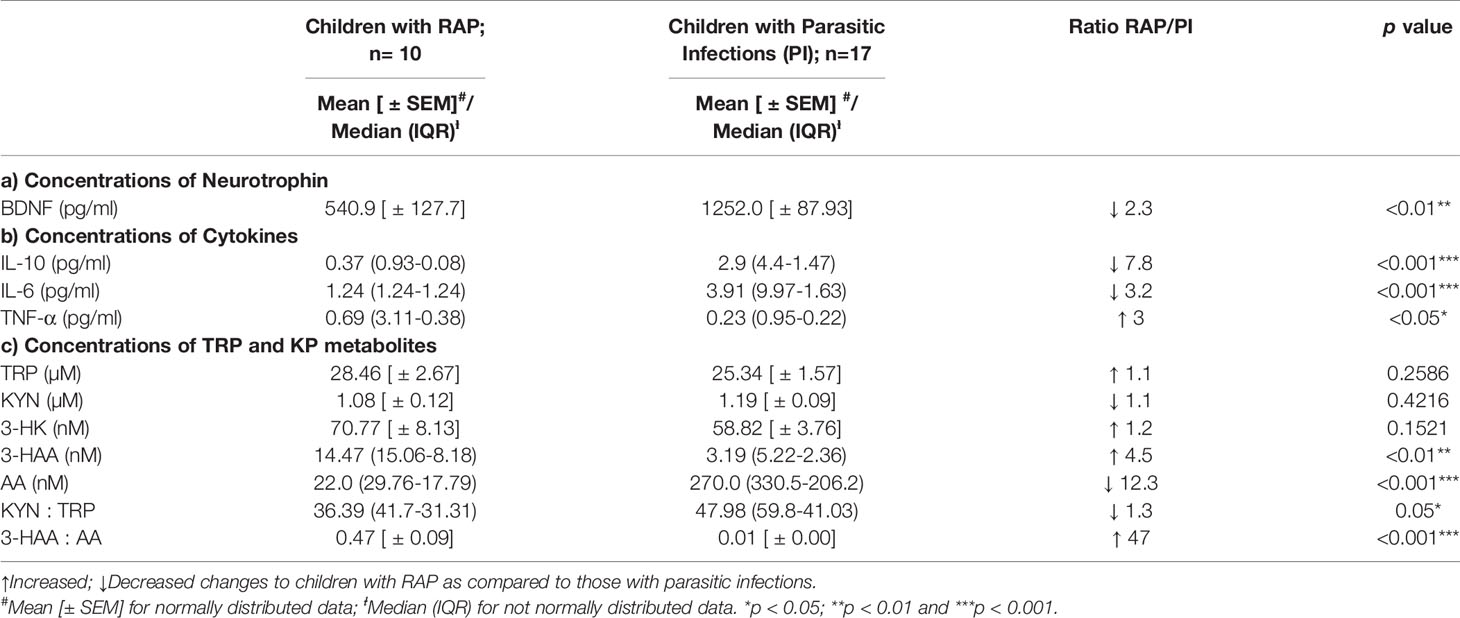
Table 2 The BDNF, cytokines and kynurenine profiles in children with RAP and children with subclinical parasitic infections.
KP Profiles in Children With RAP and Children With Subclinical Parasitic Infections
The results of the serum concentrations of the KP metabolites for two groups of children are shown in Table 2c. The concentrations of TRP and 3-HK increased in children with RAP but did not significantly change between the groups. However, in children with RAP, we found significantly high concentrations of 3-HAA (p<0.01), high 3-HAA : AA ratio (p<0.001) and a low AA concentrations (p<0.001) and low KYN : TRP ratio (p<0.05).
Correlation Analysis
Correlation analysis showed no significant associations between the concentrations BDNF, cytokines: IL-10, IL-6, TNF-α and KP metabolites in both cohorts of children. In addition, there was no association between SDQ scores and tested biochemical parameters in children with RAP.
Discussion
The medically unexplained or functional somatic symptoms constitute a major clinical problem in paediatric primary care (3, 4), however, the mechanisms behind the occurrence of these symptoms have not yet been addressed. Thus, this study is aimed to access the changes in serum levels of neurotrophins, cytokines and KP metabolites in the children with RAP and those with parasitic infections. As evidenced by previous reports (9, 13, 14, 16), psychological stress appeared to be a potential candidate accounting for occurrence of unexplained or functional somatic symptoms, thus the cohort of children with RAP were assessed by SDQ to assess their emotional and behavioral status. As compared to normative SDQ data on total difficulty scores (43), 60% of children with RAP were found to be at risk of getting mental health problems. SDQ-symptoms scores were also interpreted and “caseness” from symptoms scores were further defined according to normative data provided in the manual (43). Thirty-and 40% of children were at high risk of conduct and peer problems respectively while 10% of children showed abnormal emotional, hyperreactivity and prosocial behavior problems. As SDQ is used clinically and appears the best-suited test to identify the psychological problems in both children and adolescents (41, 42), we confirmed that children with RAP strongly imply the presence of severe psychological problems.
These borderline and abnormal total difficulties as well as symptoms scores are likely to be associated with significantly lower concentrations of BDNF in children with RAP. The BDNF is an essential mediator of neuronal plasticity and is expressed throughout the brain, with higher abundance in areas controlling cognition, mood and emotion such as the hippocampus and cerebral cortex (50). Although the peripheral sources of BDNF remain unclear, this neurotrophin is found in large amounts in platelets (51). This finding on BDNF is in accordance with previously published studies that showed stress was associated with down regulation of BDNF production (52, 53) and high activity of glucocorticoids receptors (54). Patients with depressive disorders have significantly lower concentrations of BDNF in their blood (22, 55) and interestingly, after antidepressant treatments, BDNF concentrations were back to physiological concentrations (52, 55). We hypothesize that the low concentrations of BDNF in the children with RAP could trigger brain biochemical dysfunction and ultimately, lead to the development of psychopathological symptoms.
The results of cytokine profiles showed interesting findings. The concentrations of TNF-α, proinflammatory cytokines, are expected to be increased in infection; however, its concentrations were significantly increased in children with RAP than that with parasitic infection. This may be explained by repeated and chronic psychological stress-induced pro-inflammatory cytokines production (11, 15, 56, 57). The inflammatory markers, IL-1β and TNF-α are the most consistently reported cytokines responsible for psychological stress response regardless of stressors and species (11, 58). The lower concentrations of TNF-α in this study cohort of children with parasitic infections may be due to their significantly higher concentrations of IL-10. IL-10, a cytokine with broad immunoregulatory function, is known to inhibit the production of iNOS, IFN-γ, IL-12, TNF-α production and suppresses parasite killing in a variety of protozoan and helminth infections (59, 60). In addition, these cohort of children also showed increased concentrations of a pleiotropic cytokine, IL-6. This may be due to its immunosuppressive effect as well as regenerative effect as IL-6-dependent mucosal protection was demonstrated in enteric bacterial pathogen (61). Together, the impact of IL-10 and IL-6 modulated immunosuppression is found to be beneficial and could contribute the apparently healthy status in them (60).
No significant changes were observed in TRP and KYN concentrations between the 2 groups. However, lower (downstream) KP metabolites showed significant changes. We found higher concentrations of 3-HK (1.2 folds - not statistically significant), and significantly elevated 3-HAA concentrations (4.5 folds) in RAP children. In contrast, the concentrations of AA were significantly decreased (12.3 folds) in RAP children. This can be explained by a higher activity of the KMO enzyme directing KYN catabolism towards the production of 3-HK instead of AA. 3-HK is then catabolized by KYNU to produce 3-HAA. This shows that the KP is moving towards its inflammatory/neurotoxic branch which is highlighted by the high increase in the 3-HAA/AA ration (47 folds).
3-HAA is a free-radical generator, producing hydrogen peroxide and superoxide promoting oxidative protein damage (62, 63) and inducing apoptosis (64). 3-HAA can also have excitotoxic effects (65). More recently, its immune modulatory functions have been demonstrated (66). 3-HAA is also the main precursor for biosynthesis of the excitotoxin QUIN (67). It is likely that, in children with RAP, the KP is shifted to the neurotoxic branch leading to increased QUIN production. Existing literature has documented the involvement of 3-HAA in the initiation, development and amplification of neurodegenerative processes (27, 68–70). Unfortunately, the concentrations of PIC and QUIN (67, 71) have not been measured and represent the limitations of this study. These parameters could have tightened the association with the KP activation and psychopathological symptoms (72). As we previously published, QUIN has been identified as a key player in depression (73) and suicide (72).
In addition, the ratio of serum 3-HAA to AA (3-HAA/AA) significantly increased in children with RAP. This indicates the lack of a neuroprotective response, likely due to the lack of KYNA, since a decreased 3-HAA/AA ratio is found in many neuroinflammatory conditions and associated with a loss of anti-inflammatory response (74). It is well documented that the activation of KP is controlled by inflammatory mediators especially cytokines. The initial step in the KP, conversion of TRP to KYN, is regulated by enzyme IDO-1, which is induced by pro-inflammatory cytokines such as IFN-γ, IL-1β and also IL-6 (23, 75, 76). This is not surprising as they are not the cytokines known to be involved in KP activation except for IL-6. However, the interesting results were the significant higher concentrations of pro-inflammatory TNF-α- and lower concentrations of anti-inflammatory IL-10- cytokine in the RAP children without organic disease compared to children with parasitic infections. It should be emphasized that stress can trigger immune activation, and, even more importantly, contribute to the induction of pro-inflammatory cytokine, TNF-α. However, an increased kynurenine/tryptophan (KYN/TRP) ratio in children with parasitic infection may indicate the possibility of other cytokine induced IDO-1 activation, especially IFN-γ, which has not been quantified in this study. The fact that there are no changes in TRP, KYN, a decreased KYN/TRP ratio, high concentrations of 3-HAA and low concentrations of AA clearly implies that the enzyme kynurenine monooxygenase (KMO) is strongly activated and could represent a relevant therapeutic target for children with RAP.
There are some limitations in the present study. In this study, we did not find any correlation between the concentrations of the inflammatory cytokines and KP metabolites. We neither found statistical association between SDQ scores and biochemical data. This indicates the needs of further study with larger sample size to find out the associations between SDQ data and inflammatory markers and KP metabolites. As the study population is small, the results obtained cannot be extrapolated to the general population. In addition, it would be of interest in the future to determine the KP profile in normal healthy children although it is hard to get the samples due to ethical concerns. Furthermore, as stated above, the quantification of the late KP metabolites of QUIN and PIC, are lacking in this study.
To conclude, this study is the first study to examine the involvement of the KP, inflammatory cytokines and BDNF in children with RAP. Our results strongly suggest that the decrease in BDNF concentrations and concomitantly, the production of the neuroactive KP metabolites, 3-HAA, and, to a lesser extent, 3-HK, may lead to the alteration of physiological processes and possibly explain the emergence of psychological symptoms in these children. A future longitudinal study with a larger cohort would be critical to validate our pilot study to better understand the complex interplay between chronic stress, cytokine networks, and KP dynamics in psychological processes in RAP children. The wider significance of this study lies in the fact that it provides evidence to shed light on the possible mechanisms for mind-body interactions that are increasingly observed and recognized in clinical practice.
Data Availability Statement
The raw data supporting the conclusions of this article will be made available by the authors upon request.
Ethics Statement
The studies involving human participants were reviewed and approved by University Malaya Medical Centre (UMMC) Malaysia (MEC Ref. No. 1017.24). Written informed consent to participate in this study was provided by the participants’ legal guardian/next of kin.
Author Contributions
AM, SH, SL, and KM conceptualized the study. YL and CB contributed in sample collection. GG designed the experiments. KM and KJ performed the assays and analysis. KM wrote the manuscript. SH, YL, CB, SL, and GG edited the manuscript. All authors contributed to the article and approved the submitted version.
Funding
The work of this study is fully funded by University of Malaya Research Grant, UMRG 489/12 HTM. GG is supported by the National Health and Medical Research Council (NHMRC), the Australian Research Council (ARC) and Macquarie University.
Conflict of Interest
The authors declare that the research was conducted in the absence of any commercial or financial relationships that could be construed as a potential conflict of interest.
Publisher’s Note
All claims expressed in this article are solely those of the authors and do not necessarily represent those of their affiliated organizations, or those of the publisher, the editors and the reviewers. Any product that may be evaluated in this article, or claim that may be made by its manufacturer, is not guaranteed or endorsed by the publisher.
Acknowledgments
The authors thank all the children who participated in the study. This manuscript was professionally edited by Red Fern Communication.
References
1. Garber J, Zeman J, Walker LS. Recurrent Abdominal Pain in Children: Psychiatric Diagnoses and Parental Psychopathology. J Am Acad Child Adolesc Psychiatry (1990) 29(4):648–56. doi: 10.1097/00004583-199007000-00021
2. Eminson DM. Medically Unexplained Symptoms in Children and Adolescents. Clin Psychol Rev (2007) 27(7):855–71. doi: 10.1016/j.cpr.2007.07.007
3. Olde Hartman TC, Woutersen-Koch H, van der Horst HE. Medically Unexplained Symptoms: Evidence, Guidelines, and Beyond. Br J Gen Pract (2013) 63(617):625–6. doi: 10.3399/bjgp13X675241
4. Heijmans M, Olde Hartman TC, van Weel-Baumgarten E, Dowrick C, Lucassen PL, van Weel C. Experts’ Opinions on the Management of Medically Unexplained Symptoms in Primary Care. A Qualitative Analysis of Narrative Reviews and Scientific Editorials. Fam Pract (2011) 28(4):444–55. doi: 10.1093/fampra/cmr004
5. Apley J, Naish N. Recurrent Abdominal Pains: A Field Survey of 1,000 School Children. Arch Dis Child (1958) 33(168):165–70. doi: 10.1136/adc.33.168.165
6. Quek SH. Recurrent Abdominal Pain in Children: A Clinical Approach. Singapore Med J (2015) 56(3):125–8; quiz 32. doi: 10.11622/smedj.2015038
7. Faull C, Nicol AR. Abdominal Pain in Six-Year-Olds: An Epidemiological Study in a New Town. J Child Psychol Psychiatry (1986) 27(2):251–60. doi: 10.1111/j.1469-7610.1986.tb02287.x
8. Boey C, Yap S, Goh KL. The Prevalence of Recurrent Abdominal Pain in 11- to 16-Year-Old Malaysian Schoolchildren. J Paediatrics Child Health (2000) 36(2):114–6. doi: 10.1046/j.1440-1754.2000.00465.x
9. Boey CC, Goh KL. Stressful Life Events and Recurrent Abdominal Pain in Children in a Rural District in Malaysia. Eur J Gastroenterol Hepatol (2001) 13(4):401–4. doi: 10.1097/00042737-200104000-00017
10. Herman JP, McKlveen JM, Ghosal S, Kopp B, Wulsin A, Makinson R, et al. Regulation of the Hypothalamic-Pituitary-Adrenocortical Stress Response. Compr Physiol (2016) 6(2):603–21. doi: 10.1002/cphy.c150015
11. Clark SM, Song C, Li X, Keegan AD, Tonelli LH. CD8(+) T Cells Promote Cytokine Responses to Stress. Cytokine (2019) 113:256–64. doi: 10.1016/j.cyto.2018.07.015
12. Carlsson E, Frostell A, Ludvigsson J, Faresjo M. Psychological Stress in Children may Alter the Immune Response. J Immunol (2014) 192(5):2071–81. doi: 10.4049/jimmunol.1301713
13. Cho HJ, Bower JE, Kiefe CI, Seeman TE, Irwin MR. Early Life Stress and Inflammatory Mechanisms of Fatigue in the Coronary Artery Risk Development in Young Adults (CARDIA) Study. Brain Behav Immun (2012) 26(6):859–65. doi: 10.1016/j.bbi.2012.04.005
14. Gouin JP, Glaser R, Malarkey WB, Beversdorf D, Kiecolt-Glaser JK. Childhood Abuse and Inflammatory Responses to Daily Stressors. Ann Behav Med (2012) 44(2):287–92. doi: 10.1007/s12160-012-9386-1
15. Muller N, Krause D, Barth R, Myint AM, Weidinger E, Stettinger W, et al. Childhood Adversity and Current Stress Are Related to Pro- and Anti-Inflammatory Cytokines in Major Depression. J Affect Disord (2019) 253:270–6. doi: 10.1016/j.jad.2019.04.088
16. Slopen N, Kubzansky LD, McLaughlin KA, Koenen KC. Childhood Adversity and Inflammatory Processes in Youth: A Prospective Study. Psychoneuroendocrinology (2013) 38(2):188–200. doi: 10.1016/j.psyneuen.2012.05.013
17. Miller GE, Chen E, Parker KJ. Psychological Stress in Childhood and Susceptibility to the Chronic Diseases of Aging: Moving Toward a Model of Behavioral and Biological Mechanisms. Psychol Bull (2011) 137(6):959–97. doi: 10.1037/a0024768
18. Maes M. Depression Is an Inflammatory Disease, But Cell-Mediated Immune Activation Is the Key Component of Depression. Prog Neuropsychopharmacol Biol Psychiatry (2011) 35(3):664–75. doi: 10.1016/j.pnpbp.2010.06.014
19. Muller N, Myint AM, Schwarz MJ. Inflammatory Biomarkers and Depression. Neurotox Res (2011) 19(2):308–18. doi: 10.1007/s12640-010-9210-2
20. Myint AM, Leonard BE, Steinbusch HW, Kim YK. Th1, Th2, and Th3 Cytokine Alterations in Major Depression. J Affect Disord (2005) 88(2):167–73. doi: 10.1016/j.jad.2005.07.008
21. Calabrese F, Rossetti AC, Racagni G, Gass P, Riva MA, Molteni R. Brain-Derived Neurotrophic Factor: A Bridge Between Inflammation and Neuroplasticity. Front Cell Neurosci (2014) 8:430. doi: 10.3389/fncel.2014.00430
22. Phillips C. Brain-Derived Neurotrophic Factor, Depression, and Physical Activity: Making the Neuroplastic Connection. Neural Plast (2017) 2017:7260130. doi: 10.1155/2017/7260130
23. Myint AM, Kim YK. Cytokine-Serotonin Interaction Through IDO: A Neurodegeneration Hypothesis of Depression. Med Hypotheses (2003) 61(5-6):519–25. doi: 10.1016/S0306-9877(03)00207-X
24. Oxenkrug GF. Tryptophan Kynurenine Metabolism as a Common Mediator of Genetic and Environmental Impacts in Major Depressive Disorder: The Serotonin Hypothesis Revisited 40 Years Later. Israel J Psychiatry Related Sci (2010) 47(1):56–63.
25. Maes M, Leonard BE, Myint AM, Kubera M, Verkerk R. The New ‘5-Ht’ Hypothesis of Depression: Cell-Mediated Immune Activation Induces Indoleamine 2,3-Dioxygenase, Which Leads to Lower Plasma Tryptophan and an Increased Synthesis of Detrimental Tryptophan Catabolites (TRYCATs), Both of Which Contribute to the Onset of Depression. Prog Neuropsychopharmacol Biol Psychiatry (2011) 35(3):702–21. doi: 10.1016/j.pnpbp.2010.12.017
26. O’Farrell K, Harkin A. Stress-Related Regulation of the Kynurenine Pathway: Relevance to Neuropsychiatric and Degenerative Disorders. Neuropharmacology (2017) 112(Pt B):307–23. doi: 10.1016/j.neuropharm.2015.12.004
27. Chen Y, Guillemin GJ. Kynurenine Pathway Metabolites in Humans: Disease and Healthy States. Int J Tryptophan Res (2009) 2:1–19. doi: 10.4137/IJTR.S2097
28. Lovelace MD, Varney B, Sundaram G, Lennon MJ, Lim CK, Jacobs K, et al. Recent Evidence for an Expanded Role of the Kynurenine Pathway of Tryptophan Metabolism in Neurological Diseases. Neuropharmacology (2017) 112(Pt B):373–88. doi: 10.1016/j.neuropharm.2016.03.024
29. Myint AM, Kim YK. Network Beyond IDO in Psychiatric Disorders: Revisiting Neurodegeneration Hypothesis. Prog Neuropsychopharmacol Biol Psychiatry (2014) 48:304–13. doi: 10.1016/j.pnpbp.2013.08.008
30. Ruddick JP, Evans AK, Nutt DJ, Lightman SL, Rook GA, Lowry CA. Tryptophan Metabolism in the Central Nervous System: Medical Implications. Expert Rev Mol Med (2006) 8(20):1–27. doi: 10.1017/S1462399406000068
31. Cervenka I, Agudelo LZ, Ruas JL. Kynurenines: Tryptophan’s Metabolites in Exercise, Inflammation, and Mental Health. Science (2017) 357(6349):eaaf9794. doi: 10.1126/science.aaf9794
32. Guillemin GJ, Smythe G, Takikawa O, Brew BJ. Expression of Indoleamine 2,3-Dioxygenase and Production of Quinolinic Acid by Human Microglia, Astrocytes, and Neurons. Glia (2005) 49(1):15–23. doi: 10.1002/glia.20090
33. Watanabe Y, Fujiwara M, Yoshida R, Hayaishi O. Stereospecificity of Hepatic L-Tryptophan 2,3-Dioxygenase. Biochem J (1980) 189(3):393–405. doi: 10.1042/bj1890393
34. Fatokun AA, Hunt NH, Ball HJ. Indoleamine 2,3-Dioxygenase 2 (IDO2) and the Kynurenine Pathway: Characteristics and Potential Roles in Health and Disease. Amino Acids (2013) 45(6):1319–29. doi: 10.1007/s00726-013-1602-1
35. Parrott JM, O’Connor JC. Kynurenine 3-Monooxygenase: An Influential Mediator of Neuropathology. Front Psychiatry (2015) 6:116. doi: 10.3389/fpsyt.2015.00116
36. Smith JR, Jamie JF, Guillemin GJ. Kynurenine-3-Monooxygenase: A Review of Structure, Mechanism, and Inhibitors. Drug Discovery Today (2016) 21(2):315–24. doi: 10.1016/j.drudis.2015.11.001
37. Guillemin GJ. Quinolinic Acid: Neurotoxicity. FEBS J (2012) 279(8):1355. doi: 10.1111/j.1742-4658.2012.08493.x
38. McSorley HJ, Maizels RM. Helminth Infections and Host Immune Regulation. Clin Microbiol Rev (2012) 25(4):585–608. doi: 10.1128/CMR.05040-11
39. Notarangelo FM, Wilson EH, Horning KJ, Thomas MA, Harris TH, Fang Q, et al. Evaluation of Kynurenine Pathway Metabolism in Toxoplasma Gondii-Infected Mice: Implications for Schizophrenia. Schizophr Res (2014) 152(1):261–7. doi: 10.1016/j.schres.2013.11.011
40. Tyebji S, Seizova S, Hannan AJ, Tonkin CJ. Toxoplasmosis: A Pathway to Neuropsychiatric Disorders. Neurosci Biobehav Rev (2018) 96:72–92. doi: 10.1016/j.neubiorev.2018.11.012
41. Algorta GP, Dodd AL, Stringaris A, Youngstrom EA. Diagnostic Efficiency of the SDQ for Parents to Identify ADHD in the UK: A ROC Analysis. Eur Child Adolesc Psychiatry (2016) 25(9):949–57. doi: 10.1007/s00787-015-0815-0
42. Stone LL, Otten R, Engels RC, Vermulst AA, Janssens JM. Psychometric Properties of the Parent and Teacher Versions of the Strengths and Difficulties Questionnaire for 4- to 12-Year-Olds: A Review. Clin Child Fam Psychol Rev (2010) 13(3):254–74. doi: 10.1007/s10567-010-0071-2
43. Goodman R. The Strengths and Difficulties Questionnaire: A Research Note. J Child Psychol Psychiatry (1997) 38(5):581–6. doi: 10.1111/j.1469-7610.1997.tb01545.x
44. Goodman R, Meltzer H, Bailey V. The Strengths and Difficulties Questionnaire: A Pilot Study on the Validity of the Self-Report Version. Eur Child Adolesc Psychiatry (1998) 7(3):125–30. doi: 10.1007/s007870050057
45. Al-Delaimy AK, Al-Mekhlafi HM, Nasr NA, Sady H, Atroosh WM, Nashiry M, et al. Epidemiology of Intestinal Polyparasitism Among Orang Asli School Children in Rural Malaysia. PloS Negl Trop Dis (2014) 8(8):e3074. doi: 10.1371/journal.pntd.0003074
46. Al-Harazi T, Ghani MK, Othman H. Prevalence of Intestinal Protozoan Infections Among Orang Asli Schoolchildren in Pos Senderut, Pahang, Malaysia. J Egypt Soc Parasitol (2013) 43(3):561–8. doi: 10.12816/0006413
47. Anuar TS, Al-Mekhlafi HM, Ghani MK, Osman E, Yasin AM, Nordin A, et al. Giardiasis Among Different Tribes of Orang Asli in Malaysia: Highlighting the Presence of Other Family Members Infected With Giardia Intestinalis as a Main Risk Factor. Int J Parasitol (2012) 42(9):871–80. doi: 10.1016/j.ijpara.2012.07.003
48. Sundaram G, Brew BJ, Jones SP, Adams S, Lim CK, Guillemin GJ. Quinolinic Acid Toxicity on Oligodendroglial Cells: Relevance for Multiple Sclerosis and Therapeutic Strategies. J Neuroinflamm (2014) 11:204. doi: 10.1186/s12974-014-0204-5
49. Jones SP, Franco NF, Varney B, Sundaram G, Brown DA, de Bie J, et al. Expression of the Kynurenine Pathway in Human Peripheral Blood Mononuclear Cells: Implications for Inflammatory and Neurodegenerative Disease. PloS One (2015) 10(6):e0131389. doi: 10.1371/journal.pone.0131389
50. Wetmore C, Ernfors P, Persson H, Olson L. Localization of Brain-Derived Neurotrophic Factor mRNA to Neurons in the Brain by In Situ Hybridization. Exp Neurol (1990) 109(2):141–52. doi: 10.1016/0014-4886(90)90068-4
51. Fujimura H, Altar CA, Chen R, Nakamura T, Nakahashi T, Kambayashi J, et al. Brain-Derived Neurotrophic Factor Is Stored in Human Platelets and Released by Agonist Stimulation. Thromb Haemost (2002) 87(4):728–34. doi: 10.1055/s-0037-1613072
52. Duman RS, Monteggia LM. A Neurotrophic Model for Stress-Related Mood Disorders. Biol Psychiatry (2006) 59(12):1116–27. doi: 10.1016/j.biopsych.2006.02.013
53. Myint K, Jacobs K, Myint AM, Lam SK, Henden L, Hoe SZ, et al. Effects of Stress Associated With Academic Examination on the Kynurenine Pathway Profile in Healthy Students. PloS One (2021) 16(6):e0252668. doi: 10.1371/journal.pone.0252668
54. Numakawa T, Odaka H, Adachi N. Actions of Brain-Derived Neurotrophic Factor and Glucocorticoid Stress in Neurogenesis. Int J Mol Sci (2017) 18(11):2312. doi: 10.3390/ijms18112312
55. Sen S, Duman R, Sanacora G. Serum Brain-Derived Neurotrophic Factor, Depression, and Antidepressant Medications: Meta-Analyses and Implications. Biol Psychiatry (2008) 64(6):527–32. doi: 10.1016/j.biopsych.2008.05.005
56. Elenkov IJ. Glucocorticoids and the Th1/Th2 Balance. Ann N Y Acad Sci (2004) 1024:138–46. doi: 10.1196/annals.1321.010
57. Elenkov IJ, Chrousos GP. Stress System–Organization, Physiology and Immunoregulation. Neuroimmunomodulation (2006) 13(5-6):257–67. doi: 10.1159/000104853
58. Steptoe A, Hamer M, Chida Y. The Effects of Acute Psychological Stress on Circulating Inflammatory Factors in Humans: A Review and Meta-Analysis. Brain Behav Immun (2007) 21(7):901–12. doi: 10.1016/j.bbi.2007.03.011
59. Redpath SA, Fonseca NM, Perona-Wright G. Protection and Pathology During Parasite Infection: IL-10 Strikes the Balance. Parasite Immunol (2014) 36(6):233–52. doi: 10.1111/pim.12113
60. Samant M, Sahu U, Pandey SC, Khare P. Role of Cytokines in Experimental and Human Visceral Leishmaniasis. Front Cell Infect Microbiol (2021) 11:624009. doi: 10.3389/fcimb.2021.624009
61. Dann SM, Spehlmann ME, Hammond DC, Iimura M, Hase K, Choi LJ, et al. IL-6-Dependent Mucosal Protection Prevents Establishment of a Microbial Niche for Attaching/Effacing Lesion-Forming Enteric Bacterial Pathogens. J Immunol (2008) 180(10):6816–26. doi: 10.4049/jimmunol.180.10.6816
62. Goldstein LE, Leopold MC, Huang X, Atwood CS, Saunders AJ, Hartshorn M, et al. 3-Hydroxykynurenine and 3-Hydroxyanthranilic Acid Generate Hydrogen Peroxide and Promote Alpha-Crystallin Cross-Linking by Metal Ion Reduction. Biochemistry (2000) 39(24):7266–75. doi: 10.1021/bi992997s
63. Forrest CM, Mackay GM, Stoy N, Egerton M, Christofides J, Stone TW, et al. Tryptophan Loading Induces Oxidative Stress. Free Radic Res (2004) 38(11):1167–71. doi: 10.1080/10715760400011437
64. Morita T, Saito K, Takemura M, Maekawa N, Fujigaki S, Fujii H, et al. 3-Hydroxyanthranilic Acid, an L-Tryptophan Metabolite, Induces Apoptosis in Monocyte-Derived Cells Stimulated by Interferon-Gamma. Ann Clin Biochem (2001) 38(Pt 3):242–51. doi: 10.1258/0004563011900461
65. Jhamandas K, Boegman RJ, Beninger RJ, Bialik M. Quinolinate-Induced Cortical Cholinergic Damage: Modulation by Tryptophan Metabolites. Brain Res (1990) 529(1-2):185–91. doi: 10.1016/0006-8993(90)90826-W
66. Lopez AS, Alegre E, Diaz-Lagares A, Garcia-Giron C, Coma MJ, Gonzalez A. Effect of 3-Hydroxyanthranilic Acid in the Immunosuppressive Molecules Indoleamine Dioxygenase and HLA-G in Macrophages. Immunol Lett (2008) 117(1):91–5. doi: 10.1016/j.imlet.2008.01.001
67. Speciale C, Schwarcz R. On the Production and Disposition of Quinolinic Acid in Rat Brain and Liver Slices. J Neurochem (1993) 60(1):212–8. doi: 10.1111/j.1471-4159.1993.tb05840.x
68. Schwarcz R, Bruno JP, Muchowski PJ, Wu HQ. Kynurenines in the Mammalian Brain: When Physiology Meets Pathology. Nat Rev Neurosci (2012) 13(7):465–77. doi: 10.1038/nrn3257
69. Lim CK, Fernandez-Gomez FJ, Braidy N, Estrada C, Costa C, Costa S, et al. Involvement of the Kynurenine Pathway in the Pathogenesis of Parkinson’s Disease. Prog Neurobiol (2017) 155:76–95. doi: 10.1016/j.pneurobio.2015.12.009
70. Guillemin GJ, Smith DG, Smythe GA, Armati PJ, Brew BJ. Expression of the Kynurenine Pathway Enzymes in Human Microglia and Macrophages. Adv Exp Med Biol (2003) 527:105–12. doi: 10.1007/978-1-4615-0135-0_12
71. Lugo-Huitron R, Ugalde Muniz P, Pineda B, Pedraza-Chaverri J, Rios C, Perez-de la Cruz V. Quinolinic Acid: An Endogenous Neurotoxin With Multiple Targets. Oxid Med Cell Longev (2013) 2013:104024. doi: 10.1155/2013/104024
72. Erhardt S, Lim CK, Linderholm KR, Janelidze S, Lindqvist D, Samuelsson M, et al. Connecting Inflammation With Glutamate Agonism in Suicidality. Neuropsychopharmacology (2013) 38(5):743–52. doi: 10.1038/npp.2012.248
73. Steiner J, Walter M, Gos T, Guillemin GJ, Bernstein H-G, Sarnyai Z, et al. Severe Depression Is Associated With Increased Microglial Quinolinic Acid in Subregions of the Anterior Cingulate Gyrus: Evidence for an Immune-Modulated Glutamatergic Neurotransmission? J Neuroinflamm (2011) 8(1):94. doi: 10.1186/1742-2094-8-94
74. Darlington LG, Forrest CM, Mackay GM, Smith RA, Smith AJ, Stoy N, et al. On the Biological Importance of the 3-Hydroxyanthranilic Acid: Anthranilic Acid Ratio. Int J Tryptophan Res (2010) 3:51–9. doi: 10.4137/IJTR.S4282
75. Wichers MC, Koek GH, Robaeys G, Verkerk R, Scharpe S, Maes M. IDO and Interferon-Alpha-Induced Depressive Symptoms: A Shift in Hypothesis From Tryptophan Depletion to Neurotoxicity. Mol Psychiatry (2005) 10(6):538–44. doi: 10.1038/sj.mp.4001600
Keywords: recurrent abdominal pain, stress, neurotrophin, immune mediators, kynurenine pathway
Citation: Myint K, Jacobs K, Myint A-M, Lam SK, Lim YA-L, Boey CC-M, Hoe SZ and Guillemin GJ (2021) Psychological Stresses in Children Trigger Cytokine- and Kynurenine Metabolite-Mediated Abdominal Pain and Proinflammatory Changes. Front. Immunol. 12:702301. doi: 10.3389/fimmu.2021.702301
Received: 29 April 2021; Accepted: 09 August 2021;
Published: 01 September 2021.
Edited by:
Jean-Pierre Routy, McGill University, CanadaReviewed by:
Ido Kema, University Medical Center Groningen, NetherlandsChutima Roomruangwong, Chulalongkorn University, Thailand
Copyright © 2021 Myint, Jacobs, Myint, Lam, Lim, Boey, Hoe and Guillemin. This is an open-access article distributed under the terms of the Creative Commons Attribution License (CC BY). The use, distribution or reproduction in other forums is permitted, provided the original author(s) and the copyright owner(s) are credited and that the original publication in this journal is cited, in accordance with accepted academic practice. No use, distribution or reproduction is permitted which does not comply with these terms.
*Correspondence: See Ziau Hoe, hoesz@ummc.edu.my; Gilles J. Guillemin, gilles.guillemin@mq.edu.au