- 1Division of Endocrinology, ASST Fatebenefratelli-Sacco, Milan, Italy
- 2International Center for T1D, Pediatric Clinical Research Center Romeo ed Enrica Invernizzi, DIBIC, Università di Milano, Milan, Italy
- 3Nephrology Division, Boston Children’s Hospital and Transplantation Research Center, Brigham and Women’s Hospital, Harvard Medical School, Boston, MA, United States
- 4Department of Pediatrics, Buzzi Children’s Hospital, Milan, Italy
Despite the increasing knowledge of pathophysiological mechanisms underlying the onset of type 1 diabetes (T1D), the quest for therapeutic options capable of delaying/reverting the diseases is still ongoing. Among all strategies currently tested in T1D, the use of hematopoietic stem cell (HSC)-based approaches and of teplizumab, showed the most encouraging results. Few clinical trials have already demonstrated the beneficial effects of HSCs in T1D, while the durability of the effect is yet to be established. Investigators are also trying to understand whether the use of selected and better-characterized HSCs subsets may provide more benefits with less risks. Interestingly, ex vivo manipulated HSCs showed promising results in murine models and the recent introduction of the humanized mouse models accelerated the translational potentials of such studies and their final road to clinic. Indeed, immunomodulatory as well as trafficking abilities can be enhanced in genetically modulated HSCs and genetically engineered HSCs may be viewed as a novel “biologic” therapy, to be further tested and explored in T1D and in other autoimmune/immune-related disorders.
Introduction
Hematopoietic stem cells (HSCs) have been extensively used as an effective therapeutic approach in hematological malignancies and have demonstrated to be safe in human subjects (1). Over the last 10 years, several studies documented the extraordinary immunoregulatory properties of HSCs, which render them a potential useful tool in the fight for immune-mediated diseases (2). Despite being in limited number in the circulating blood of healthy individuals, HSCs are extremely potent and able to suppress the immune system response, as several in vitro and in vivo studies have shown (2). Based on these premises, the use of HSCs has been tested in numerous autoimmune diseases such as type 1 diabetes (T1D), multiple sclerosis (MS), systemic sclerosis, systemic lupus erythematosus and Chron’s disease, with relevant benefits (3–6). Indeed, HSCs may reset the immune response, thus reshaping the chronic derangement of the immune system to a more self-tolerant state (7, 8). Interestingly, it has been also demonstrated that the bone marrow-derived and blood HSCs are altered in some autoimmune conditions such as T1D and MS, with HSCs being scanty in the circulation and often unable to exploit their immunoregulatory function (9–11). Here we are presenting major advances in the preclinical and clinical studies of HSCs in T1D. We report recent insights coming from novel T1D in vivo research and provide an update on the most relevant clinical studies that have been performed by using HSCs in human subjects with T1D. In this perspective, we envision to consider HCSs as a novel “biologic”, which can be personalized and modeled, as a novel relevant therapeutic option in T1D.
HSCs in Type 1 Diabetes: The Murine Scenario
The rationale behind the use of HSCs in autoimmune disease such as T1D has been extensively studied in the last decade by taking advantage of the NOD mouse model. This mouse spontaneously develops autoimmune diabetes at the age of 12–15 weeks, with severe hyperglycemia (12, 13). However, signs of activation of the immune system against pancreatic islets are already visible at 8–10 weeks of age when the NOD mouse shows insulitis with an abundant T cell infiltrate (12). Over the last two decades, two major HSCs-based strategies have been pursued to prevent the onset of experimental autoimmune diabetes in murine models: (i) HSCs have been infused to induce mixed chimerism and to re-establish the peripheral deletion of autoreactive T cells, (ii) HSCs have been genetically engineered to reshape the immune reservoir and facilitate tolerance towards auto-antigens. The use of HSCs infusion was extremely successful in preventing diabetes onset in NOD mice through the induction of a mixed chimerism. Indeed, a deletion of autoreactive T cells generated at the thymus level (14) as well as the re-establishment of immune tolerance in the periphery were obtained. Furthermore, in the presence of a tolerogenic network between donor Regulatory T cells (Tregs) and host-donor dendritic cells (DCs), costimulatory pathways, particularly PDL-1, play a major role (15). However, the HSC-mediated chimerism, despite effective in reshaping the autoimmune response, requires the use of myeloablative agents/approaches, which may further limit translational applications (16, 17). Given that common polymorphisms exist in MHC class II in T1D patients and in NOD mice, which confer a higher risk of developing T1D, genetically engineering of single HSCs to express the proper and protective MHC class II, held great promises in the new therapies in T1D (18). Indeed, the introduction of new protective MHC class II through lentiviral delivery in HSCs of NOD mice was able to prevent the onset of T1D, mainly through the deletion of autoreactive T cells which did not engage in the MHC class II-mediated response (19, 20). While this approach was again limited by the need of immune ablation for the HSCs infusion, which is feasible in NOD mice but at high risk in humans, it paved the way for exploring genetic engineering of HSCs to better exploit their multiple properties in autoimmunity. Ex vivo genetic manipulation of NOD HSCs, to encode proinsulin and transgenically target MHC class II, successfully prevented T1D onset (21, 22). Also, HSCs can be engineered for tolerogenic purposes such as those aimed at inducing tolerance to autoantigens or at replacing genetic alleles associated with increased disease susceptibility (23). In view of this, some studies explored whether HSCs in diabetic NOD mice are altered and might be fixed through genetic engineering or pharmacological modulation. Elevated levels of CXCL12 (SDF-1) in bone marrow-HSCs of NOD mice have been suggested to alter trafficking of HSCs and Tregs in the periphery, thus favoring the onset of T1D (24). The use of ADAM3100, which antagonizes the CXCL12 receptor SDF-1, was associated with increased mobilization of HSCs and T cells, and delayed onset of experimental autoimmune diabetes in NOD mice (24). Recently, a defect in PDL-1 expression has been demonstrated in HSCs of NOD mice, which was associated with a reduced immunomodulatory function (9, 25). Genetic and pharmacological modulation of PDL-1 on HSCs restored the HSCs immunomodulatory properties, reset the immune balance and prevented the onset of T1D. In summary, all the aforementioned studies support the use of ex vivo manipulation of HSCs in the NOD mouse model as a successful tool to delay the onset of autoimmune diabetes. Genetic engineering of HSCs has been recently employed in a humanized mouse model in which ex vivo manipulated human HSCs successfully restored the development of functional Tregs and rescued the autoimmune IPEX syndrome (26). Recently, the introduction of the NOD-Rag1null IL2rγnull Ins2Akita (NRG-Akita) mouse, a humanized mouse model available in diabetes research which develops spontaneous hyperglycemia, fostered studies in the field (27, 28). This model, in which human immune cells can be infused without being rejected, may be extremely useful in testing the potency of newly genetically engineered human HSCs in the diabetes prevention.
HSCs in Type 1 Diabetes: The Human Landscape
In the last 20 years, autologous hematopoietic stem cells transplantation (AHSCT) has been used in several clinical trials to treat refractory autoimmune disease such as multiple sclerosis (MS), systemic sclerosis (SSc), systemic lupus erythematosus (SLE), Crohn’s disease (CD), type 1 diabetes (T1D) and a range of other immune-mediated disorders (29). With regard to patients with T1D, the use of AHSCT obtained significant insulin independence and a well-preserved glycometabolic control in the short and mid-term follow-up (Figure 1A and Table 1) (17, 31, 34, 35). Also, an increase in C-peptide levels and C-peptide area under the curve (AUC) measurement were detectable in AHSCT-treated T1D patients as compared to baseline, and only minor adverse events were registered in the mid-term (25, 36). A reduction of the T-helper-1 and T-helper-17 subsets was also observed in the short-term (37). Interestingly, a cost-effectiveness analysis conducted in patients with T1D undergoing AHSCT as compared to patients with T1D remaining on insulin therapy demonstrated that AHSCT provides some benefits over time depending on the duration of preserved glycated hemoglobin levels achieved with AHSCT, but overall being cost-effective for treatment of T1D if the AHSCT lasts from 3 to 8 years (38). Long-term follow-up analyses for AHSCT-treated T1D patients were only reported for a few studies, due to the high number of patients lost at follow-up and the worldwide spreading of the studies, which also accounted for a small sample size, missed randomization process, lack in standardized procedures and enrolment of a heterogenous patients’ population (39). Despite all these limitations, a minor percentage of relapse in the autoimmune disease was evident between 4 and 6 years of follow-up after the AHSCT, which varied among centers and lead few patients to resume insulin treatment (40, 41). Moreover, a subgroup of AHSCT-treated patients exhibited a prolonged remission and remained insulin independent for more than 4 years, thus leading to hypothesize that the response to the AHSCT treatment may differ in patients with T1D (17, 35, 42). Indeed, Malmegrim and Colleagues demonstrated that a different immune profile exists between patients experiencing short and prolonged remission, with the latter exhibiting lower frequencies of effector-memory CD4 T cells and islet-specific autoreactive CD8 T cells, paralleled by a detectable expansion of immunoregulatory T cells (35). Moreover, the favorable outcome of AHSCT in T1D was also associated with a less islet-specific autoreactive immune profile at baseline, thus delineating a subgroup of patients with T1D who may benefit the most from an AHSCT-based strategy (43, 44). This also emphasized the importance of the conditioning regimen, which may need to be employed in association with AHSCT in patients with a high level of autoimmune response. In summary, results of the use of HSC-based approach, primarily the AHSCT, in patients with T1D (Table 1), suggest two major observations: (i) AHSCT treatment has to be limited to a subgroup of T1D patients and it requires high-level immunosuppression to obtain long-term effect, and (ii) the immune profile of T1D patients plays a central role in the achievement of long-term insulin-independence when using HSC-based strategies. Therefore, the infusion of a subset of HSCs, rather than the whole HSCs pool, such as in AHSCT, endowed with immunoregulatory properties may provide additional benefits in terms of balancing autoimmunity and achieving the proper clinical and metabolic outcomes.
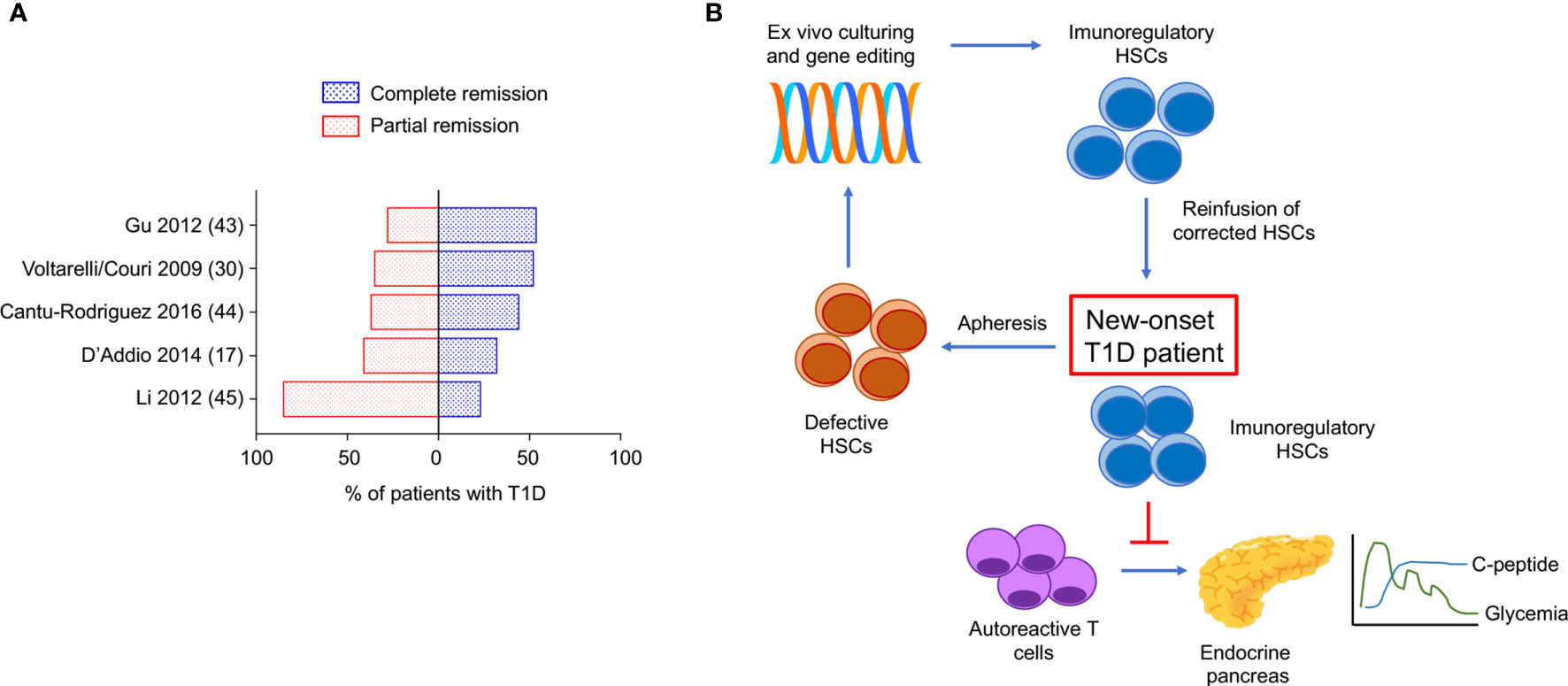
Figure 1 Complete/partial remission of type 1 diabetes obtained with AHSCT in the long-term. Proposed genetic engineered HSC-based approach to target type 1 diabetes. (A) Proportion of patients with T1D undergoing AHSCT who achieved complete remission (insulin independence) and partial remission (low dose exogenous insulin requirement) at the latest timepoint analyzed within each clinical study registered in ClinicalTrials.gov available as publication. (B) Use of genetically engineered HSCs to target T1D: proposed approach. T1D, type 1 diabetes; AHSCT, autologous hematopoietic stem cell transplantation.
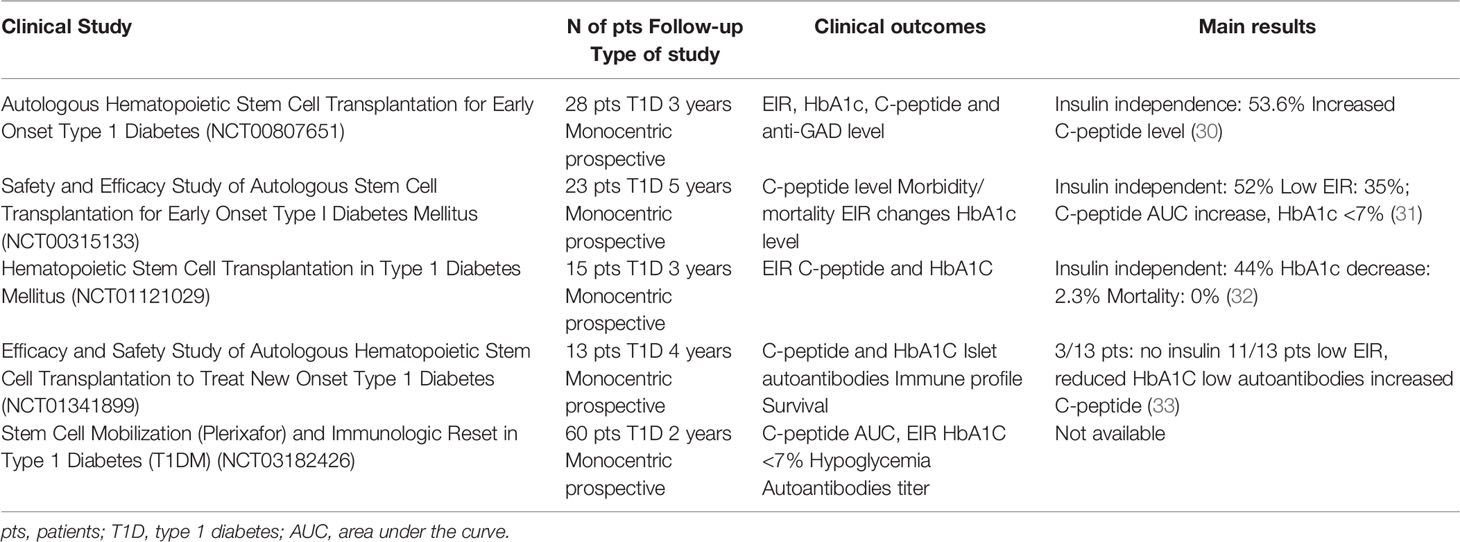
Table 1 Summary of main characteristics, clinical outcomes and results obtained in the clinical studies conducted in T1D and registered in ClinicalTrials.gov.
Conclusions and Future Directions
The use of HSCs has hold great promises in the treatment of autoimmune diabetes, however, in the last decade. The results obtained in clinical trials with the use of AHSCT in T1D suggest a potential novel approach to treat autoimmune diseases, despite all the aforementioned limitations. The use of a selected subset of HSCs endowed with immunoregulatory properties, without the need of additional immunosuppressive agents remains unexplored so far and deserves more investigation and testing from the scientific community. Patients with T1D who may benefit the most from this therapeutic approach need to be carefully identified, probably based on disease stages, degree of cellular and humoral autoimmune response, presence or not of diabetic ketoacidosis (17). The recent findings on the use of teplizumab in patients at risk for T1D (45) confirmed that immune ablation aimed at preventing T1D onset is a hot topic. HSCs, endowed with immunomodulatory properties, may offer a potent immunoregulatory effect without inducing T lymphocytes depletion, which is commonly observed with teplizumab. Indeed, several studies demonstrated that in absence of “healthy” HSCs central tolerance may be difficulty obtained. Autoimmune disorders, particularly type 1 diabetes, are associated with altered HSCs, which fail in exerting their immunomodulatory properties. Strategies aimed at targeting this defect successfully delayed diabetes onset in murine models. Feasibility and effectiveness in of the ex vivo manipulation and genetic engineering of HSCs are well-established in mouse models, while studies on safety for translational purposes are still required. In view of this, the use of humanized mouse model may accelerate the translation from murine experiments to human studies. The outstanding results collected in the past and ongoing clinical trials are encouraging in pursuing the research around the use of genetic engineered-HSCS in type 1 diabetes. Therefore, in our opinion, genetic modulation to reset HSCs physiological function, may find an interesting field of application not only in type 1 diabetes (Figure 1B) but in other autoimmune conditions too. Finally, in the era of the development of biologic therapy to treat immune-mediated diseases, we envision genetically engineered HSCs as a novel “biologic” agent and a “natural immunosuppressant” to be considered in the portfolio of alternative therapeutic options in type 1 diabetes and autoimmune diseases.
Author Contributions
IP and EA wrote the paper. AB, MB, AM, CL, VU, AS, and AA, collected clinical and preclinical data. GZ edited the paper. FD’A and PF conceived the idea, wrote and edited the paper. All authors contributed to the article and approved the submitted version.
Funding
FD is supported by SID Lombardia Grant and by EFSD/JDRF/Lilly Programme on Type 1 Diabetes Research 2019. PF is supported by the Italian Ministry of Health grant RF-2016-02362512 and by the Linea-2 2019 funding from Università di Milano. We thank the “Fondazione Romeo e Enrica Invernizzi” for extraordinary support.
Conflict of Interest
PF and MB hold a patent of modulated HSCs and founded Altheia Science.
The remaining authors declare that the research was conducted in the absence of any commercial or financial relationships that could be construed as a potential conflict of interest.
References
1. Copelan EA. Hematopoietic Stem-Cell Transplantation. N Engl J Med (2006) 354(17):1813–26. doi: 10.1056/NEJMra052638
2. Fiorina P, Voltarelli J, Zavazava N. Immunological Applications of Stem Cells in Type 1 Diabetes. Endocr Rev (2011) 32(6):725–54. doi: 10.1210/er.2011-0008
3. Alchi B, Jayne D, Labopin M, Demin A, Sergeevicheva V, Alexander T, et al. Autologous Haematopoietic Stem Cell Transplantation for Systemic Lupus Erythematosus: Data From the European Group for Blood and Marrow Transplantation Registry. Lupus (2013) 22:245–53. doi: 10.1177/0961203312470729
4. Atkins HL, Bowman M, Allan D, Anstee G, Arnold DL, Bar-Or A, et al. Immunoablation and Autologous Haemopoietic Stem-Cell Transplantation for Aggressive Multiple Sclerosis: A Multicentre Single-Group Phase 2 Trial. Lancet (2016) 388(10044):576–85. doi: 10.1016/S0140-6736(16)30169-6
5. Lindsay JO, Allez M, Clark M, Labopin M, Ricart E, Rogler G, et al. Autologous Stem-Cell Transplantation in Treatment-Refractory Crohn’s Disease: An Analysis of Pooled Data From the ASTIC Trial. Lancet Gastroenterol Hepatol (2017) 2(6):399–406. doi: 10.1016/S2468-1253(17)30056-0
6. van Laar JM, Farge D, Sont JK, Naraghi K, Marjanovic Z, Larghero J, et al. Autologous Hematopoietic Stem Cell Transplantation vs Intravenous Pulse Cyclophosphamide in Diffuse Cutaneous Systemic Sclerosis: A Randomized Clinical Trial. JAMA (2014) 311(24):2490–8. doi: 10.1001/jama.2014.6368
7. Alexander T, Farge D, Badoglio M, Lindsay JO, Muraro PA, Snowden JA, et al. Hematopoietic Stem Cell Therapy for Autoimmune Diseases - Clinical Experience and Mechanisms. J Autoimmun (2018) 92:35–46. doi: 10.1016/j.jaut.2018.06.002
8. Lutter L, Spierings J, van Rhijn-Brouwer FCC, van Laar JM, van Wijk F. Resetting the T Cell Compartment in Autoimmune Diseases With Autologous Hematopoietic Stem Cell Transplantation: An Update. Front Immunol (2018) 9:767. doi: 10.3389/fimmu.2018.00767
9. Ben Nasr M, Tezza S, D'Addio F, Mameli C, Usuelli V, Maestroni A, et al. PD-L1 Genetic Overexpression or Pharmacological Restoration in Hematopoietic Stem and Progenitor Cells Reverses Autoimmune Diabetes. Sci Transl Med (2017) 9(416). doi: 10.1126/scitranslmed.aam7543
10. de Oliveira GL, de Lima KW, Colombini AM, Pinheiro DG, Panepucci RA, Palma PV, et al. Bone Marrow Mesenchymal Stromal Cells Isolated From Multiple Sclerosis Patients Have Distinct Gene Expression Profile and Decreased Suppressive Function Compared With Healthy Counterparts. Cell Transplant (2015) 24(2):151–65. doi: 10.3727/096368913X675142
11. Fadini GP, Ciciliot S, Albiero M. Concise Review: Perspectives and Clinical Implications of Bone Marrow and Circulating Stem Cell Defects in Diabetes. Stem Cells (2017) 35(1):106–16. doi: 10.1002/stem.2445
12. Anderson MS, Bluestone JA. The NOD Mouse: A Model of Immune Dysregulation. Annu Rev Immunol (2005) 23:447–85. doi: 10.1146/annurev.immunol.23.021704.115643
13. Delovitch TL, Singh B. The Nonobese Diabetic Mouse as a Model of Autoimmune Diabetes: Immune Dysregulation Gets the NOD. Immunity (1997) 7(6):727–38. doi: 10.1016/S1074-7613(00)80392-1
14. Racine J, Wang M, Zhang C, Lin CL, Liu H, Todorov I, et al. Induction of Mixed Chimerism With MHC-Mismatched But Not Matched Bone Marrow Transplants Results in Thymic Deletion of Host-Type Autoreactive T-Cells in NOD Mice. Diabetes (2011) 60(2):555–64. doi: 10.2337/db10-0827
15. Zhang M, Racine JJ, Lin Q, Liu Y, Tang S, Qin Q, et al. MHC-Mismatched Mixed Chimerism Restores Peripheral Tolerance of Noncross-Reactive Autoreactive T Cells in NOD Mice. Proc Natl Acad Sci USA (2018) 115(10):E2329–37. doi: 10.1073/pnas.1720169115
16. Atkinson MA, von Herrath M, Powers AC, Clare-Salzler M. Current Concepts on the Pathogenesis of Type 1 Diabetes–Considerations for Attempts to Prevent and Reverse the Disease. Diabetes Care (2015) 38(6):979–88. doi: 10.2337/dc15-0144
17. D’Addio F, Valderrama Vasquez A, Ben Nasr M, Franek E, Zhu D, Li L, et al. Autologous Nonmyeloablative Hematopoietic Stem Cell Transplantation in New-Onset Type 1 Diabetes: A Multicenter Analysis. Diabetes (2014) 63:3041–6. doi: 10.2337/db14-0295
18. Tian C, Bagley J, Cretin N, Seth N, Wucherpfennig KW, Iacomini J, et al. Prevention of Type 1 Diabetes by Gene Therapy. J Clin Invest (2004) 114:969–78. doi: 10.1172/JCI22103
19. Bagley J, Tian C, Iacomini J. Prevention of Type 1 Diabetes in NOD Mice by Genetic Engineering of Hematopoietic Stem Cells. Methods Mol Biol (2008) 433:277–85. doi: 10.1007/978-1-59745-237-3_17
20. Tian C, Ansari MJ, Paez-Cortez J, Bagley J, Godwin J, Donnarumma M, et al. Induction of Robust Diabetes Resistance and Prevention of Recurrent Type 1 Diabetes Following Islet Transplantation by Gene Therapy. J Immunol (2007) 179(10):6762–9. doi: 10.4049/jimmunol.179.10.6762
21. Chan J, Clements W, Field J, Nasa Z, Lock P, Yap F, et al. Transplantation of Bone Marrow Genetically Engineered to Express Proinsulin II Protects Against Autoimmune Insulitis in NOD Mice. J Gene Med (2006) 8(11):1281–90. doi: 10.1002/jgm.968
22. Steptoe RJ, Ritchie JM, Harrison LC. Transfer of Hematopoietic Stem Cells Encoding Autoantigen Prevents Autoimmune Diabetes. J Clin Invest (2003) 111(9):1357–63. doi: 10.1172/JCI15995
23. Coleman MA, Steptoe RJ. Induction of Antigen-Specific Tolerance Through Hematopoietic Stem Cell-Mediated Gene Therapy: The Future for Therapy of Autoimmune Disease? Autoimmun Rev (2012) 12(2):195–203. doi: 10.1016/j.autrev.2011.08.012
24. Leng Q, Nie Y, Zou Y, Chen J. Elevated CXCL12 Expression in the Bone Marrow of NOD Mice Is Associated With Altered T Cell and Stem Cell Trafficking and Diabetes Development. BMC Immunol (2008) 9:51. doi: 10.1186/1471-2172-9-51
25. Ben Nasr M, D'Addio F, Malvandi AM, Faravelli S, Castillo-Leon E, Usuelli V, et al. Prostaglandin E2 Stimulates the Expansion of Regulatory Hematopoietic Stem and Progenitor Cells in Type 1 Diabetes. Front Immunol (2018) 9:1387. doi: 10.3389/fimmu.2018.01387
26. Masiuk KE, Laborada J, Roncarolo MG, Hollis RP, Kohn DB. Lentiviral Gene Therapy in HSCs Restores Lineage-Specific Foxp3 Expression and Suppresses Autoimmunity in a Mouse Model of IPEX Syndrome. Cell Stem Cell (2019) 24(2):309–17.e7. doi: 10.1016/j.stem.2018.12.003
27. Greiner DL, Brehm MA, Hosur V, Harlan DM, Powers AC, Shultz LD, et al. Humanized Mice for the Study of Type 1 and Type 2 Diabetes. Ann N Y Acad Sci (2011) 1245:55–8. doi: 10.1111/j.1749-6632.2011.06318.x
28. Shultz LD, Brehm MA, Garcia-Martinez JV, Greiner DL. Humanized Mice for Immune System Investigation: Progress, Promise and Challenges. Nat Rev Immunol (2012) 12(11):786–98. doi: 10.1038/nri3311
29. Delemarre EM, van den Broek T, Mijnheer G, Meerding J, Wehrens EJ, Olek S, et al. Autologous Stem Cell Transplantation Aids Autoimmune Patients by Functional Renewal and TCR Diversification of Regulatory T Cells. Blood (2016) 127(1):91–101. doi: 10.1182/blood-2015-06-649145
30. Gu W, Hu J, Wang W, Li L, Tang W, Sun S, et al. Diabetic Ketoacidosis at Diagnosis Influences Complete Remission After Treatment With Hematopoietic Stem Cell Transplantation in Adolescents With Type 1 Diabetes. Diabetes Care (2012) 35(7):1413–9. doi: 10.2337/dc11-2161
31. Couri CE, Oliveira MC, Stracieri AB, Moraes DA, Pieroni F, Barros GM, et al. C-Peptide Levels and Insulin Independence Following Autologous Nonmyeloablative Hematopoietic Stem Cell Transplantation in Newly Diagnosed Type 1 Diabetes Mellitus. JAMA (2009) 301(15):1573–9. doi: 10.1001/jama.2009.470
32. Cantu-Rodriguez OG, Lavalle-Gonzalez F, Herrera-Rojas MA, Jaime-Perez JC, Hawing-Zarate JA, Gutierrez-Aguirre CH, et al. Long-Term Insulin Independence in Type 1 Diabetes Mellitus Using a Simplified Autologous Stem Cell Transplant. J Clin Endocrinol Metab (2016) 101(5):2141–8. doi: 10.1210/jc.2015-2776
33. Li L, Shen S, Ouyang J, Hu Y, Hu L, Cui W, et al. Autologous Hematopoietic Stem Cell Transplantation Modulates Immunocompetent Cells and Improves Beta-Cell Function in Chinese Patients With New Onset of Type 1 Diabetes. J Clin Endocrinol Metab (2012) 97(5):1729–36. doi: 10.1210/jc.2011-2188
34. Snarski E, Milczarczyk A, Torosian T, Paluszewska M, Urbanowska E, Krol M, et al. Independence of Exogenous Insulin Following Immunoablation and Stem Cell Reconstitution in Newly Diagnosed Diabetes Type I. Bone Marrow Transplant (2011) 46(4):562–6. doi: 10.1038/bmt.2010.147
35. Malmegrim KC, de Azevedo JT, Arruda LC, Abreu JR, Couri CE, de Oliveira GL, et al. Immunological Balance Is Associated With Clinical Outcome After Autologous Hematopoietic Stem Cell Transplantation in Type 1 Diabetes. Front Immunol (2017) 8:167. doi: 10.1007/s00592-015-0724-1
36. Couri CE, de Oliveira MC, Simoes BP. Risks, Benefits, and Therapeutic Potential of Hematopoietic Stem Cell Transplantation for Autoimmune Diabetes. Curr Diabetes Rep (2012) 12(5):604–11. doi: 10.1007/s11892-012-0309-0
37. Ye L, Li L, Wan B, Yang M, Hong J, Gu W, et al. Immune Response After Autologous Hematopoietic Stem Cell Transplantation in Type 1 Diabetes Mellitus. Stem Cell Res Ther (2017) 8(1):90. doi: 10.1186/s13287-017-0542-1
38. Snarski E, Szmurlo D, Halaburda K, Krol M, Urbanowska E, Milczarczyk A, et al. An Economic Analysis of Autologous Hematopoietic Stem Cell Transplantation (AHSCT) in the Treatment of New Onset Type 1 Diabetes. Acta Diabetol (2015) 52(5):881–8. doi: 10.1007/s00592-015-0724-1
39. Sun SY, Gao Y, Liu GJ, Li YK, Gao W, Ran XW, et al. Efficacy and Safety of Stem Cell Therapy for T1DM: An Updated Systematic Review and Meta-Analysis. J Diabetes Res (2020) 2020:5740923. doi: 10.1155/2020/5740923
40. Gu B, Miao H, Zhang J, Hu J, Zhou W, Gu W, et al. Clinical Benefits of Autologous Haematopoietic Stem Cell Transplantation in Type 1 Diabetes Patients. Diabetes Metab (2018) 44(4):341–5. doi: 10.1016/j.diabet.2017.12.006
41. Walicka M, Milczarczyk A, Snarski E, Jedynasty K, Halaburda K, Torosian T, et al. Lack of Persistent Remission Following Initial Recovery in Patients With Type 1 Diabetes Treated With Autologous Peripheral Blood Stem Cell Transplantation. Diabetes Res Clin Pract (2018) 143:357–63. doi: 10.1016/j.diabres.2018.07.020
42. Snarski E, Milczarczyk A, Halaburda K, Torosian T, Paluszewska M, Urbanowska E, et al. Immunoablation and Autologous Hematopoietic Stem Cell Transplantation in the Treatment of New-Onset Type 1 Diabetes Mellitus: Long-Term Observations. Bone Marrow Transplant (2016) 51(3):398–402. doi: 10.1038/bmt.2015.294
43. Couri CEB, Malmegrim KCR, Oliveira MC. New Horizons in the Treatment of Type 1 Diabetes: More Intense Immunosuppression and Beta Cell Replacement. Front Immunol (2018) 9:1086. doi: 10.3389/fimmu.2018.01086
44. van Megen KM, van ‘T Wout ET, Forman SJ, Roep BO. A Future for Autologous Hematopoietic Stem Cell Transplantation in Type 1 Diabetes. Front Immunol (2018) 9:690. doi: 10.3389/fimmu.2018.00690
Keywords: type 1 diabetes, hematopoietic stem cells, autoimmune response, NOD mouse model, genetic modulation
Citation: Pastore I, Assi E, Ben Nasr M, Bolla AM, Maestroni A, Usuelli V, Loretelli C, Seelam AJ, Abdelsalam A, Zuccotti GV, D’Addio F and Fiorina P (2021) Hematopoietic Stem Cells in Type 1 Diabetes. Front. Immunol. 12:694118. doi: 10.3389/fimmu.2021.694118
Received: 12 April 2021; Accepted: 24 May 2021;
Published: 09 July 2021.
Edited by:
Alexander Steinkasserer, University Hospital Erlangen, GermanyReviewed by:
Kelen Cristina Ribeiro Malmegrim, University of São Paulo, BrazilFabio Grassi, Institute for Research in Biomedicine (IRB), Switzerland
Copyright © 2021 Pastore, Assi, Ben Nasr, Bolla, Maestroni, Usuelli, Loretelli, Seelam, Abdelsalam, Zuccotti, D’Addio and Fiorina. This is an open-access article distributed under the terms of the Creative Commons Attribution License (CC BY). The use, distribution or reproduction in other forums is permitted, provided the original author(s) and the copyright owner(s) are credited and that the original publication in this journal is cited, in accordance with accepted academic practice. No use, distribution or reproduction is permitted which does not comply with these terms.
*Correspondence: Paolo Fiorina, paolo.fiorina@childrens.harvard.edu; Francesca D’Addio, francesca.daddio@unimi.it
†These authors share first authorship