- 1Taizhou Hospital, Zhejiang University School of Medicine, Taizhou, China
- 2Guangdong Provincial People’s Hospital, Guangdong Academy of Medical Sciences, Guangzhou, China
- 3Medical Oncology Department, Affiliated Cancer Hospital & Institute of Guangzhou Medical University, Guangzhou, China
- 4State Key Laboratory of Oncology in Southern China, Collaborative Innovation Center for Cancer Medicine, Sun Yat-sen University Cancer Center, Guangzhou, China
Immune checkpoint blockade (ICB) has become a standard treatment for non-small cell lung cancer (NSCLC). However, most patients with NSCLC do not benefit from these treatments. Abnormal vasculature is a hallmark of solid tumors and is involved in tumor immune escape. These abnormalities stem from the increase in the expression of pro-angiogenic factors, which is involved in the regulation of the function and migration of immune cells. Anti-angiogenic agents can normalize blood vessels, and thus transforming the tumor microenvironment from immunosuppressive to immune-supportive by increasing the infiltration and activation of immune cells. Therefore, the combination of immunotherapy with anti-angiogenesis is a promising strategy for cancer treatment. Here, we outline the current understanding of the mechanisms of vascular endothelial growth factor/vascular endothelial growth factor receptor (VEGF/VEGFR) signaling in tumor immune escape and progression, and summarize the preclinical studies and current clinical data of the combination of ICB and anti-angiogenic drugs in the treatment of advanced NSCLC.
Introduction
Lung cancer is one of the most common cancer types with high mortality in the world (1). Adenocarcinoma, squamous cell carcinoma and large cell carcinoma are the three major kinds of NSCLC comprising 85% of all lung cancers (2). Because of the lack of early diagnosis indicators, more than 70% of cancer patients have experienced local invasion, lymph node and distant metastasis at the first diagnosis (3). These patients have extremely poor prognoses. The five-year survival rate of patients at this stage is only 4% (4).
In the past decade, immunotherapy has made significant progress for the treatment of NSCLC. Improving the therapeutic effect via combination strategy has become the main direction in the field. A number of clinical trials testing the combination of immunotherapy and anti-angiogenesis have shown promising results in different tumor types including NSCLC. However, due to the complicated regulatory mechanisms of these two kinds of therapies, how to collaboratively use them to obtain the maximal therapeutic effect remains to be answered. Understanding the potential mechanisms of combination might help to select appropriate patients and treat them at right timing with optimized dosages of drugs.
Immune Checkpoints and Inhibitors
Immune checkpoint inhibitors (ICIs) are widely used in the treatment of NSCLC. A series of receptor/ligand pairs such as CD28-CTLA4/B7 and programmed cell death-1/programmed death ligand 1 (PD-1/PD-L1) are involved in the antitumor immune response at different stages (5, 6). These costimulatory and coinhibitory receptor/ligand pairs are collectively referred to as immune checkpoints (7). PD-1 is expressed on a variety of immune cells, such as T cells, NK cells, B cells, and monocytes (8). The PD-1 pathway mediates inhibitory signaling triggered by the binding to PD-L1. PD-L1 expressed on cancer cells could suppress effector T cells and thus prevent T cell-mediated tumor destruction (9). Therefore, blocking the PD-1/PD-L1 inhibitory pathway can reactivate the immune attack on tumor cells, thereby treating cancer (10).
A number of PD-1, PD-L1 and CTLA-4 inhibitors, including Pembrolizumab (11), nivolumab (12), atezolizumab (13), durvalumab (14), avelumab (15) and ipilimumab (16), have been approved for the treatment of advanced NSCLC. Pembrolizumab and nivolumab have been approved by the U.S. Food and Drug Administration (FDA) for the treatment of non-small cell lung cancer with positive PD-L1 expression. The PACIFIC (17) Phase III clinical trial (NCT02125461) in Europe makes durvalumab the only phase III immunotherapy drug recommended by the current guidelines. Japan is also conducting trails of atezolizumab, such as J-TAIL (NCT03645330) (https://clinicaltrials.gov/ct2/show/NCT03645330), J-TAIL-2 (NCT04501497) (https://clinicaltrials.gov/ct2/show/NCT04501497), and durvalumab, AYAME (NCT03995875) (https://clinicaltrials.gov/ct2/show/NCT03995875). In China, according to the ORIENT-11 study (NCT03607539), sintilimab has been approved as the first-line treatment for non-squamous NSCLC combined with pemetrexed and platinum chemotherapy. The Phase III trial (NCT03134872) (18) of SHR-1210 combined with pemetrexed and carboplatin in the treatment of non-squamous non-small cell lung cancer is also ongoing. Nevertheless, due to the tumor heterogeneity and the complexity of the tumor microenvironment (TME), the overall response rates to ICI therapy keep at low levels (19). To increase the therapeutic efficacy, combination strategies have become the major focus of cancer immunotherapy (20). A large number of clinical trials are testing the combination of immunotherapy with traditional therapies such as surgery, chemotherapy, radiotherapy, targeted therapy and other treatment methods.
ICIs obtain therapeutic effect by inducing a durable antitumor immune response (21). However, high levels of immunosuppressive cells in the TME and insufficient infiltration of effector cells into tumor severely impair the antitumor immunity, and thus decreasing the efficacy of ICIs. Recent studies have shown that pro-angiogenic factors in tumor promote the development of immunosuppressive cells, and neovessels reduce the infiltration of effector cells (22). The combination with anti-angiogenic agents is thought to be a promising strategy to enhance the therapeutic efficacy of ICIs.
Tumor Angiogenesis and Inhibitors
Angiogenesis is a hallmark of cancer associated with occurrence, proliferation and metastasis of tumors (23). Targeting the angiogenesis pathway has been found to be effective in the treatment of a variety of cancers including NSCLC. The abnormal structure and function of tumor angiogenesis facilitate the development of a hostile tumor microenvironment characterized by increased interstitial pressure, hypoxia and acidosis (24). Hypoxia further induces the expression of genes involved in blood vessel formation and cell proliferation, and thus exacerbating the TME (25). VEGFs, a family of secreted glycoproteins, play an essential role in the angiogenesis of tumor, which include VEGF-A, VEGF-B, VEGF-C, VEGF-D, VEGF-E, VEGF-F, placental growth factor (PIGF) (26). There are three VEGF receptors, VEGFR-1, -2 and -3. The effect of VEGF in promoting angiogenesis is mainly mediated by VEGFR-2. Signaling pathways downstream VEGFR-2, such as phospholipase C gamma (PLCγ), Raf and phosphoinositide-3-kinase (PI3K) (22), promote angiogenesis and vascular permeability by regulating the differentiation, migration, proliferation and survival of microvascular endothelial cells (27). Both monoclonal antibodies blocking the interaction between VEGF and VEGFR or small molecules targeting downstream signaling could inhibit tumor angiogenesis (28). As listed in Figure 1, both monoclonal antibodies and small molecule inhibitors interfering angiogenesis have been approved for the treatment in various cancer types.
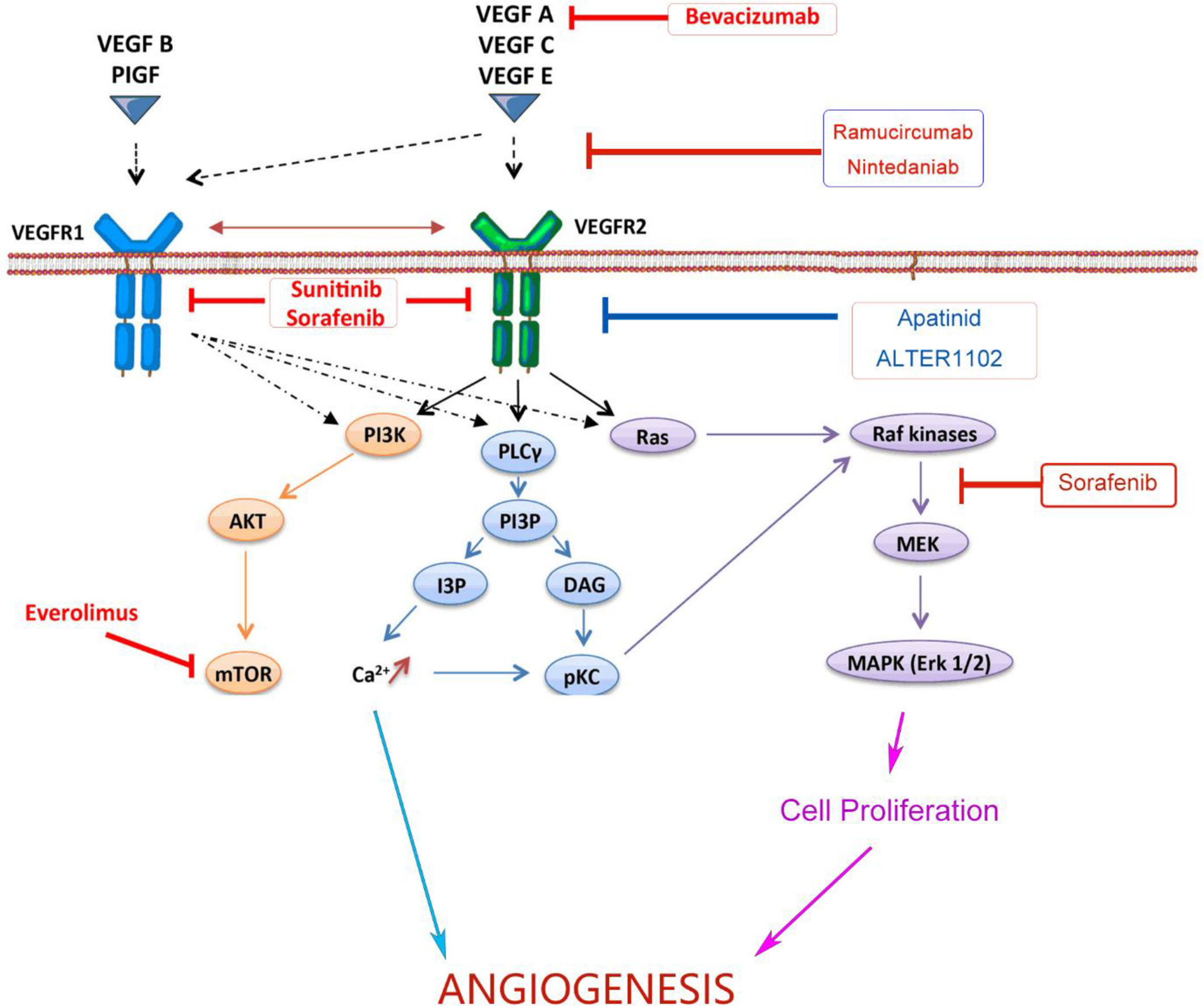
Figure 1 Monoclonal antibodies and small molecules targeting VEGF/VEGFR signaling in tumor angiogenesis. Monoclonal antibodies and small molecule TKIs targeting the VEGFA/VEGFR-2/PLCγ/Raf/PI3K signaling pathway could inhibit tumor angiogenesis and improve the efficiency of anticancer treatments. VEGF, Vascular Endothelial Growth Factor; VEGFR, Vascular Endothelial Growth Factor Receptor; TKI, Tyrosine Kinase Inhibitor; PI3K, Phosphoitide 3-Kinase; AKT, serine/threonine-specific protein kinase; mTOR, mammalian target of rapamycin; PLCγ, Phospholipase C γ; PI3P, Phosphatidylinositol 3-Phosphate; IP3, Inositol Triphosphate; DAG, Diacyl Glycerol; pKC, Protein Kinase C; MEK, Mitogen-activated protein kinase; MAPK, Mitogen Activated Protein Kinase.
Bevacizumab, or Avastin, is a humanized monoclonal antibody binding to VEGF-A. It has been approved for the treatment of advanced non-squamous NSCLC. Phase III clinical trials showed that bevacizumab combined with carboplatin and paclitaxel significantly improved the therapeutic efficacy (29). Ramucirumab is a recombinant human IgG1 monoclonal antibody targeting VEGFR2. According to the results of the REVEL study, the FDA and European Medicines Agency (EMA) have approved the combination of Ramucirumab and docetaxel for the treatment of metastatic NSCLC and progressed disease after the treatment of platinum (30).
Nintedanib is a small molecular inhibitor targeting three critical receptors signaling in angiogenesis, VEGFR, fibroblast growth factor receptor (FGFR) and platelet-derived growth factor receptor (PDGFR). The LUME-Lung 1 study showed that nintedanib in combination with pemetrexed significantly improved progress-free survival (PFS) of patients (31). It was approved by EMA as the second-line treatment for stage IV NSCLC. In addition, tyrosine kinase inhibitors (TKIs) including sorafenib, sunitinib and apatinib have also been clinically studied in advanced NSCLC, but no obvious overall survival (OS) benefit was observed. Anlotinib is another small molecular inhibitor targeting multiple receptor tyrosine kinases (RTKs), including VEGFR2 and VEGFR3. The results of the ALTER 0303 trial showed that anlotinib significantly prolonged the OS and PFS of patients with advanced NSCLC (32). It has been approved as the third-line treatment for advanced NSCLC.
Although a number of angiogenesis inhibitors have been tested in clinical trials, anti-angiogenesis alone showed limited therapeutic effect in cancer treatment (33). Most of the angiogenesis inhibitors were approved for the combination therapy with other drugs. Given that reduced vessels in tumor will result in decreased delivery of combinatory drugs as well, these results challenge the well-accepted mechanism of anti-angiogenesis in reducing vascular supply, and thus suppress tumor growth by starving tumor. This paradox is resolved by recent findings of vessel normalization, a process recovering the perfusion function and structure of vessels in tumor, which enhanced antitumor immune response by increasing immune cell infiltration and oxygen supply in tumor (33–36). Consistent with the mechanism of vessel normalization, low dose of anti-VEGFR2 antibody showed better effect on reprogramming the tumor microenvironment and displayed better therapeutic efficacy than the high-dose treatment (37). The vessel normalization theory provides novel perspectives in the combination of anti-angiogenesis with other drugs or therapies.
Rationale for Combination of ICI Inhibitors With Angiogenesis in NSCLC
Angiogenesis Fosters An Immunosuppressive Tumor Microenvironment by Modifying The Recruitment of Immune Cells
TME is a dynamic ecosystem composed of tumor cells, immune cells, fibroblasts, stroma cells, blood vessels and various soluble factors, which suppress antitumor immune response and promote resistance to immunotherapy (38). Excessive VEGF signaling drives aberrant angiogenesis in tumor. Compared to normal blood vessels in tissues, blood vessels in TME are leaky, tortuous, cystic dilation, interlaced and randomly connected. The tumor vascular endothelial cells have abnormal morphology, loose connections between pericytes and varied basement membrane thickness. These abnormalities of structure and function lead to the heterogeneity of tumor blood perfusion, and eventually form a microenvironment characterized by increased interstitial fluid pressure, hypoxia and acidosis (39). The hypoxic microenvironment induced by VEGF/VEGFR signaling suppresses the antitumor immune response through a variety of mechanisms (40, 41).
The TME is enriched with suppressive immune cells including regulatory T cells (Tregs), myeloid-derived suppressive cells (MDSCs), tumor associated macrophages (TAMs), and immature dendritic cells (imDC). Hypoxia facilitates the infiltration of these suppressive immune cells by inducing the expression of chemokines recruiting these immune cells. For example, C-C motif chemokine ligand 22 (CCL22) and C-C motif chemokine ligand 28 (CCL28) recruits Tregs into tumor (42); colony Stimulating factor 1 (CSF1), C-C motif chemokine ligand 2 (CCL2) and C-X-C motif chemokine ligand 12 (CXCL12) increases the recruitment of pro-inflammatory monocytes and TAMs, and convert TAMs from a pro-inflammatory M1-like type to a tumor-promoting M2-like type (43); Dendritic cells (DCs) are mainly recruited into tumor by C-C motif chemokine ligand 20 (CCL20), and granulocyte-macrophage colony stimulating factor (GM-CSF), Interleukin-6 (IL-6), Interleukin-10 (IL-10) prevent maturation of recruited DCs (44). Moreover, the hypoxic environment inhibits the infiltration of effector T cells. VEGF can reduce the expression of adhesion molecules critical for T cell infiltration, such as integrin ligand vascular cell adhesion protein 1 (VCAM1) and intercellular adhesion molecule 1 (ICAM1), on immune cells and endothelial cells (ECs) (45). VEGF-A, IL-10 and prostaglandin E2 (PGE2) induce the expression of Fas ligand on endothelial cells, which causes cell death of endothelial cells and CD8+ T cells through the Fas/FasL signaling pathway, and thus reduce T cell mobilization and infiltration (46). Consistently, blockade of the VEGF signaling reduced the recruitment of suppressive cells into tumor but increased the infiltration of effector T cells (37), indicating that anti-angiogenesis is a potential strategy to re-program the immunosuppressive TME, and thus improve the efficacy of immunotherapy.
Angiogenic Factors Directly Regulate Differentiation of Various Immune Cells
In addition to its effect on immune cell migration, the VEGF signaling directly regulates differentiation and proliferation of suppressive immune cells including Tregs, TAMs, MDSCs, and DCs (47, 48). VEGF (red stars) and angiopoietin-2 (ANG2) (green pentagons) are also produced by these immune cells, which foster both the paracrine and the autocrine VEGF (and/or ANG2) signaling in tumor (49). Immunosuppressive cytokines secreted by these suppressive immune cells, including IL-10, indoleamine 2,3-dioxygenase (IDO), and transforming growth factor beta (TGF-β) et al., further worsen the environment by inducing Tregs and inhibiting DC maturation, NK cell activation, T cell activation and proliferation (50). Therefore, angiogenesis inhibitors might normalize the aberrant vasculature in tumor, reduce the development of suppressive immune cells, enhance effector cell infiltration into tumor, and thus reprogram the immunosuppressive to immunosupportive (Figure 2).
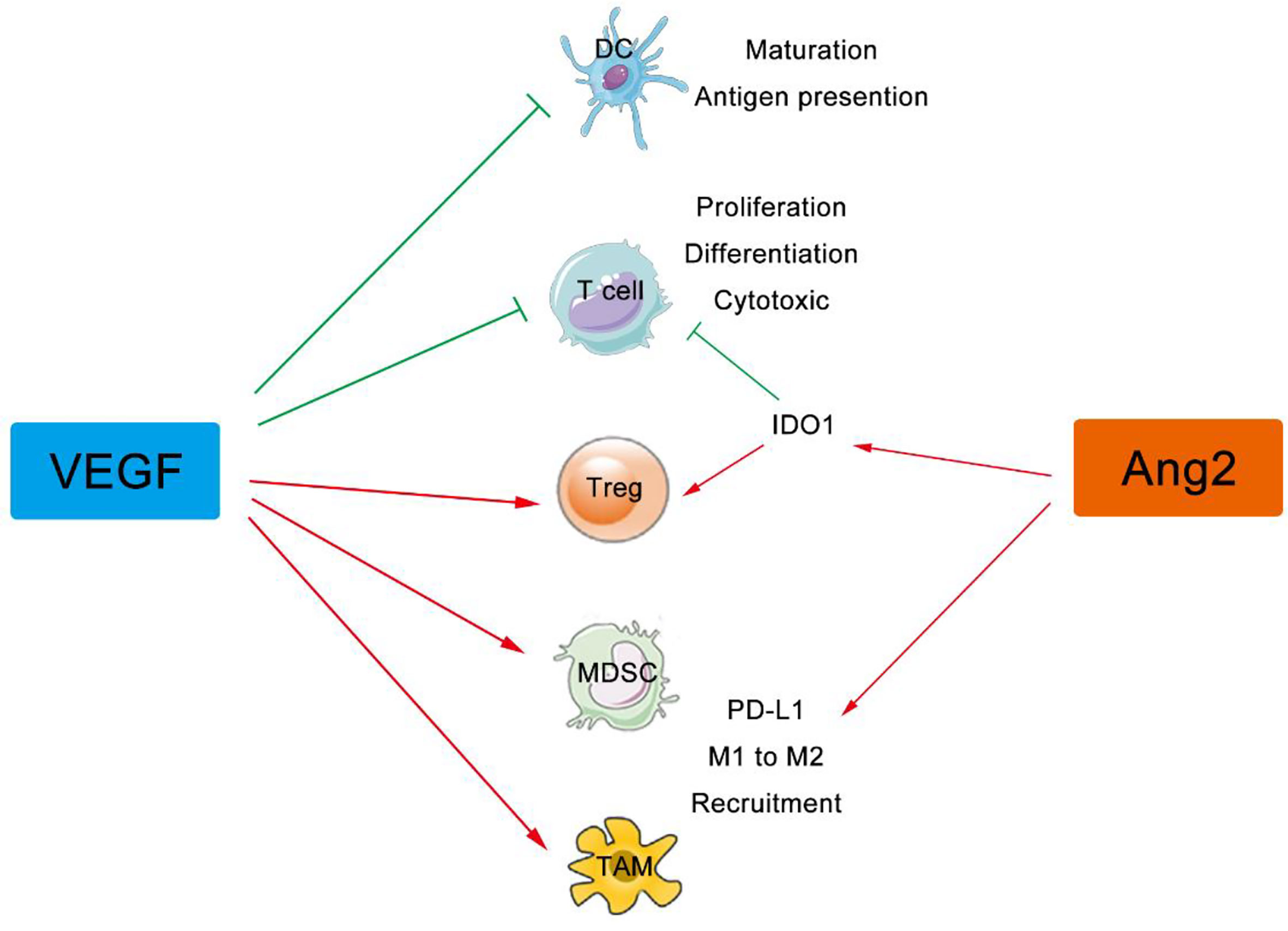
Figure 2 VEGF and ANG2 regulate immune cells in tumor. The VEGF family can suppress the maturation, differentiation, and antigen presentation of APCs, DCs, NKs, and T cells, while both VEGF and Ang2 can improve the suppressive effect of Tregs, TAMs, and MDSCs. VEGF, Vascular Endothelial Growth Factor; ANG2, Angiogenin 2; APCs, Antigen Presenting Cells, DCs, Dendritic Cells; Treg, Regulatory T cells; NKs, Natural Killer Cells; TAMs, Tumor Associated Macrophages; MDSCs, Myeloid Derived Suppressor Cells.
VEGF Inhibits the Maturation and Differentiation of DCs
DCs are the professional antigen-presenting cells (APCs) which play a critical role in the antitumor immune cycle. Following the exposure to tumor antigens, DCs migrate to lymph nodes and become mature during the migration. They initiate adaptive antitumor immune response by activating T cells recognizing tumor antigens (51). Plenty of evidence has shown that VEGF could inhibit differentiation and maturation of DCs (52, 53). It was found that elevated VEGF levels in mice hindered the development of DCs (48). Studies have showed that VEGF-A inhibited the differentiation of monocytes to DC, and VEGF-A inhibition using bevacizumab or sorafenib restored this process (54).
Due to the lack of costimulatory molecules, immature DCs promote tolerance instead of activation of T cells. It was reported that the binding of VEGF to VEGFR-2 on the surface of DC restrains its maturation by inhibiting the nuclear factor κB (NF-κB) signaling pathway (55). VEGF inhibition increases antigen uptake and migration of tumor-associated DCs in mouse tumor models (56). The VEGFR inhibitor Axitinib promotes maturation of monocyte-derived human DCs, featured with elevated levels of activation markers, major histocompatibility complex (MHC) molecules and co-stimulatory genes such as CD80, CD86, and CD83 (57).
VEGF Increases the Number of Tregs
It is known that Tregs in tumor suppress T cell response against cancer (58). Studies have shown that the VEGF signaling contributes to the induction, maintenance and activation of Tregs in tumors. The expression of VEGF was found to be positively associated with the levels of Tregs in tumor, which indicate poor prognosis in many cancer types (59). Consistent with this finding, higher expression of VEGFR2 was found in Tregs compared to other CD4+ T cells (59, 60), suggesting a preferential role of VEGF signaling in Tregs. Interestingly, neuropilin-1, an co-receptor increasing the binding affinity of VEGF for VEGFRs, is also highly expressed in Tregs (61), which mediates the activation of Tregs and thus enhances their suppressive function (62). VEGF can directly bind to Neuropilin 1 (Nrp-1) on Tregs and guide their migration into a tumor (63). Inhibition of VEGF signaling using sunitinib, bevacizumab or soluble VEGFR-1/-2 reduce Treg proportion in different mouse tumor models and in cancer patients (47, 64–66). Decreased proliferation of Tregs and reduced levels of peripheral Treg levels are also reported in some studies. Following the reduction of Tregs, enhanced antitumor immune response was detected in tumors.
VEGF Promotes the Expansion of MDSCs
MDSCs were initially defined as CD11b+Gr-1+ cells in tumors. There are two main major populations of MDSCs: monocytic MDSCs (M-MDSC) and polymorphonuclear MDSCs (PMN-MDSC). PMN-MDSCs are the dominant population of MDSCs in mouse tumor models, while M-MDSCs are mainly found in human tumors (67). MDSCs employ a number of mechanisms to suppress the antitumor immune response, for examples, consuming the nutrient of lymphocyte, reducing trafficking and viability of lymphocyte, generating oxidative stress, and inducing the differentiation of Tregs (67, 68).
The intratumoral level of MDSCs was found to be associated with the VEGF concentration in mouse tumor models. In addition, VEGF infusion significantly elevated levels of Gr1+ cells in normal mice without tumor (48), suggesting that VEGF signaling is involved the differentiation of myeloid cells. It was reported that VEGF-A-induced excessive activation of Janus kinase 2/Signal transducer and activator of transcription 3 (Jak2/STAT3) signaling contributes to the abnormal myeloid cell differentiation in cancer (69). Inhibition of VEGF signaling by sunitinib decreased the levels of MDSC in the spleen, bone marrow, and tumor in mouse models, and showed combinatory effect with HPV vaccine for the treatment of tumors expressing human papillomavirus (HPV) antigens (70). Mechanistically, sunitinib downregulates STAT3 signaling and leads to apoptosis in MDSCs (71). In addition to the reduction in MDSC quantity, VEGF inhibition impairs their suppressive function. Axitinib treatment decreases the suppressive capacity of MDSCs isolated from spleens or tumors in mouse models. Moreover, axitinib promotes the differentiation of MDSC toward a phenotype with enhanced capacity of antigen presentation (72). Reduction of MDSCs was also observed in cancer patient treated with sunitinib, which led to stronger T cell immune response against cancer (73). A recent study also showed that bevacizumab-containing regimens had low levels of the granulocytic MDSCs than regimens without bevacizumab in patient tumor samples of NSCLC (74).
VEGF Induces the Differentiation of Macrophages From M1 to M2
TAMs promote angiogenesis by expressing a high level of VEGF. The lacked expression of costimulatory molecules on TAMs induces T cell tolerance and apoptosis. TAMs also promote immunosuppression in tumor by secreting cytokines that can suppress T cell recruitment and activation, such as IL-10, TGFβ, and prostaglandins (75). In addition to the recruitment of TAMs into tumor, VEGF signaling is also involved in the conversion of TAMs from the M1 to M2 phenotype. High levels of TAMs were observed in tumors with increased expression of stromal-cell-derived factor 1 alpha (SDF-1α), CXCL12, C-X-C motif chemokine receptor 4 (CXCR4) and VEGF in mouse tumor models (76, 77). Teresa E Peterson et al. have shown that dual inhibition of VEGFRs and Ang-2 reduced macrophage recruitment and promoted the polarization of TAMs to a M1 antitumor phenotype (78). Deng et al. also found that VEGF blockade potentiated antitumor efficacy in glioblastoma by reducing TAM recruitment into tumor (79), The combination of VEGFR and CXCR4 inhibitors also showed therapeutic effect in glioblastoma multiforme (GBM) xenografts (80).
VEGF Inhibits the Development and Activation of T Cells
T cells play an essential role in the antitumor immune response by directly killing tumor cells. Boosting the T cell immune response against cancer has become the primary goal of most immunotherapies. Low expression of VEGF was detected in T cells from tumor (81), suggesting that T cells might also promote angiogenesis. Ohm et al. found that the infusion of VEGF-A to tumor-bearing mice led to severe thymic atrophy resulted from a dramatic reduction in CD4+/CD8+ thymocytes (82). The inhibition of thymocyte maturation is mediated by the VEGFR2. These findings indicate that the VEGF signaling could directly inhibit T cell development. In addition, studies have shown that VEGF-A produced in the tumor microenvironment promotes T cell exhaustion by inducing the expression of co-inhibitory molecules in CD8+ T cell, and targeting VEGF-A/VEGFR signaling could reduce the expression of these suppressive genes (83).
VEGF-induced recruitment and expansion of suppressive immune cells in tumor inhibit the activation of tumor antigen-specific T cells. A lot of clinical and preclinical studies support that blockade of the VEGF/VEGFR signaling can enhance T cell response in tumor. Bevacizumab (Avastin) administration increased cytotoxic T cell levels in colorectal cancer and NSCLC patients (84, 85). Sunitinib treatment increase the levels of CD4+ and CD8+ T cell in mouse cancer models. Stronger cytotoxic activity and elevated expression of Th1 cytokine (Interferon-gamma, IFN-γ) were observed in these T cells from sunitinib-treated tumors (71). Similarly, Schmittnaegel et al. found that dual targeting of ANG2 and VEGFA increased the levels of effector CD8+ T cells in tumors (86). Furthermore, IFN-γ secreted by activated T cells has strong anti-angiogenic activity, suggesting that immunotherapy can also be antiangiogenic. The IFN-γR signaling could directly modulate the function and phenotype of vascular endothelial cells, and thereby normalize tumor blood vessels and promote effector T cell infiltration (87).
Lenvatinib is a RTK that specifically inhibits the kinase activities of VEGF receptors 1-3. Studies have shown that Lenvatinib reduced TAMs and increased the levels of effector CD8+ T cells. Combined with PD-1 blockade can further elevate the levels of activated CD8+ T cells, and thereby enhance antitumor immunity via the IFN signaling pathway (88).
Synergism of Anti-Angiogenesis Inhibitors and ICB
Taken together, the VEGF signaling plays a pivotal role in the immunosuppressive TME which severely inhibits antitumor immune response. VEGF/VEGFR inhibition could reprogram the TME from immunosuppressive into immunostimulating by modulate the recruitment and function of immune suppressive cells and T cells. Therefore, anti-VEGF/VEGFR therapy not only has anti-angiogenic effects but also promotes immune response against cancer.
On the other hand, hypoxia-inducible factor 1-alpha (HIF-1α) up-regulates the expression of immune checkpoint molecules in tumor (83). VEGF-A directly increases the expression of PD-1 on activated CD8+ T cells and Tregs through VEGFR2 (83). Besides, elevated levels of IFN-γ in tumor resulted from VEGF signaling inhibition could induce the expression of PD-L1 on tumor cells. These mechanisms provide a theoretical basis for the combined treatment of advanced NSCLC with ICB and anti-angiogenic agents.
Immunotherapy and Antiangiogenic Agents: Preclinical Study
Plenty of preclinical evidence also indicates that combining immunotherapy with anti-angiogenic inhibitors can improve the therapeutic efficacy in advanced NSCLC. It was reported that endostatin could improve the therapeutic effect of adoptive transfer of cytokine-induced killer cells (CIKs) for the treatment of lung carcinomas (89). Another preclinical study also showed that the VEGF inhibitor bevacizumab improved the effect of CIKs therapy in treating NSCLC (90). These findings provide evidence for the combination of anti-angiogenesis therapy and immunotherapy to treat lung cancer. In addition, the effects of different doses of antiangiogenic inhibitors on the combination with immunotherapy are also studied. A small dose of apatinib was enough to increase T cells infiltration, reduce hypoxia, and decrease the recruitment of TAMs into tumor (37, 91). Consistently, the combination of low-dose apatinib and PD-L1 antibody can significantly inhibit tumor growth and increase the survival time in mouse models (91).
Immunotherapy and Antiangiogenic Agents: Clinical Data
Given that both the potential molecular mechanism and preclinical evidence support the combination of immunotherapy with anti-angiogenesis therapy, a number of clinical trials are underway to evaluate the safety and efficacy of this new therapy in NSCLC (Table 1). Preliminary data indicate that immunotherapy combined with anti-vascular therapy is a promising approach for the treatment of NSCLC.
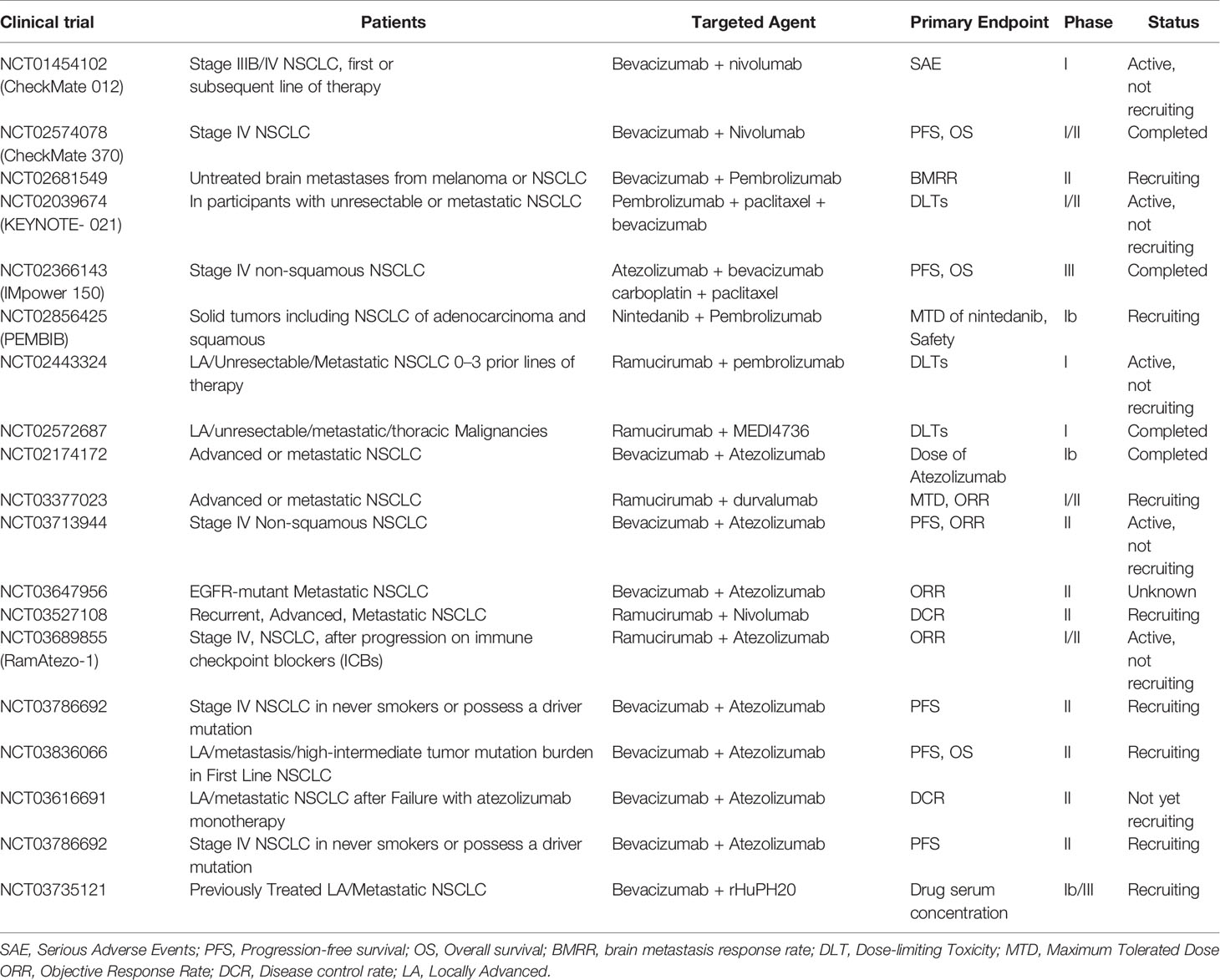
Table 1 Clinical trials of the combination of anti-angiogenic inhibitors with immune checkpoint blockade in NSCLC.
Nivolumab Combined With Bevacizumab
The combination between PD-1 blockade and bevacizumab was tested in the Checkmate012 phase I clinical trial (NCT01454102). Advanced NSCLC patients who failed in the first-line chemotherapy of platinum were divided into two groups, and treated with nivolumab or the combination of nivolumab with bevacizumab. The median PFS in the combination group was 37.1 weeks, while the nivolumab monotherapy group was 16 weeks in patients with squamous cancers and 21.4 weeks in patients with non-squamous cancers. Lower incidence of severe adverse events (AEs) (grade 3 and above) was observed in the combination. However, the objective response rates (ORR) are similar in these two groups. Follow-up studies are ongoing (12).
Pembrolizumab Combined With Ramucirumab
The combination between ramucirumab and pembrolizumab has been studying by a multicenter phase I study (NCT02443324) in different types of cancers. 27 patients were recruited in this study. The objective reactions in these NSCLC patients were 30%. The median treatment time is 6.8 months or longer, and the median response time is 1.45 months. The most common serious AEs related to treatment in NSCLC patients were fatigue and myocardial infarction (7%) (92). The team has also expanded a multi-center, open-label Phase 1a/b trial to study ramoxiimab plus pembrolizumab in the treatment of advanced newly-treated NSCLC (N=26) (11). The results showed that 22 (84.6%) patients had any grade of treatment-related AEs, and hypertension is the most common side-effect (n = 4, 15.4%). The ORR of the treatment group was 42.3%. The ORR in patients with high PD-L1 expression levels (tumor proportion score (TPS)≥50%) and low levels (TPS 1%-49%) were 56.3% and 22.2%, respectively. The median PFS was 9.3 months in the treated group, and the patients with PD-L1 TPS 1%-49% were 4.2 months. The patients with PD-L1 TPS≥50% did not reach the median PFS. The median OS was not reached in the treated population.
Atezolizumab Combined With Bevacizumab
The combination of bevacizumab with atezolizumab and chemotherapy was studied by IMpower150, which is a phase III randomized controlled clinical trial (NCT02366143). 1202 non-squamous NSCLC patients with stage IV or recurrent metastatic diseases who have not treated with chemotherapy were included. Patients were randomized 1:1:1 to receive atezolizumab combined with carboplatin + paclitaxel (ACP) (n = 402), atezolizumab combined with carboplatin + paclitaxel + bevacizumab (ABCP) (n = 400), carboplatin + Paclitaxel + Bevacizumab (BCP) (n = 400), after 4-6 courses of treatment, receive atezolizumab or bevacizumab or both for maintenance treatment until the disease progresses or no clinical benefit. The results of the study show that immunotherapy on the basis of the combination of bevacizumab and chemotherapy can prolong patient survival. The median PFS of the ABCP was 8.3 months, and the BCP was 6.8 months (HR: 0.59, P<0.0001). The median OS was 19.2 months for the ABCP group, and 14.7 months for the BCP group (HR: 0.78, P=0.02). The incidence of treatment-related serious AEs was 25.4% for ABCP group and 19.3% for BCP group. However, 77.4% of ABCP patients had grade 1-2 AEs. This study shows that, regardless of the PD-L1 expression, VEGFR or anaplastic lymphoma kinase mutation status, the use of ABCP can significantly improve PFS and OS in patients with metastatic non-squamous NSCLC (93). According to this study, the FDA approved the combination therapy of ABCP as the first-line treatment for metastatic non-squamous NSCLC in December 2018. This combination is currently being tested in hepatocellular carcinoma (HCC) as well. At the 2019 (ESMO) annual meeting, it was reported that atilizumab combined with bevacizumab and bisorafenib had better OS and PFS in patients with unresectable hepatocellular carcinoma (94).
Apatinib Combined With SHR-1210
A single-arm phase II trial studying the combination of Apatinib with SHR-1210 was reported at the ASCO meeting in 2019. 96 patients were recruited in this study. Apatinib is a small TKI that primarily act on VEGFR-2, and SHR-1210 is another PD-1 antibody. These two drugs are developed in China. Patients failed at least one previous line of chemotherapy received intravenous infusion of SHR-1210 200 mg q2w combined with oral Apatinib 250 mg qd. The ORR of all evaluable patients was 30.8%. DCR was 82.4%. Median PFS was 5.9 months. The OS endpoint was not reached. Among the patients with bTMB 1.54 mutations/Mb, the ORR was 52.6%, and the DCR was 81.6%, suggesting that apatinib combined with SHR-1210 might have better therapeutic effect in patients with high tumor mutation burden (TMB) (95).
Overall, the combination of ICI and anti-angiogenic agents has shown encouraging results in treating advanced NSCLC. To achieve maximal therapeutic effect, a number of questions need to be addressed in future trails, including the effect of different anti-angiogenic inhibitors, the drug dose, the timing and schedule of the two type of drugs in the treatment etc.
Conclusion
In this paper, we overviewed the updated knowledge of ICB, anti-angiogenesis, and the combination of these two kinds of therapies. A lot of preclinical studies have revealed the potential mechanisms of abnormal angiogenesis in the regulation of antitumor immunity in mouse tumor models, and support the application of combining immunotherapy and anti-angiogenesis for cancer treatment. The combination of immunotherapy and anti-angiogenesis is expected to enhance the efficacy of immunotherapy by converting the immunosuppressive TME to immunosupportive. Results of the ongoing clinical trials also support that the combination of ICB and anti-angiogenesis is a promising approach for the treatment of NSCLC. Translational studies and innovative clinical trials are needed in the future to address important questions not resolved in current studies, including the identification of biomarkers precisely the response to the combination therapy, optimizing the drug dose, administration schedule and the timing of the treatment.
Author Contributions
JS and PZ conceptualized the idea for the review. SR and XX performed the literature search, analyzed cited references and wrote the first draft of the manuscript. HY, JS, and PZ wrote sections of the manuscript. All authors contributed to the article and approved the submitted version.
Funding
This study is supported by grants from the National Key Research and Development Program of China (2016YFA0500304), the National Nature Science Foundation in China (NSFC) (81802853, 81773052, 81572806, 82002400), the Natural Science Foundation of Zhejiang Provincial (Y19H160116, Q18H160119), the Postdoctoral Science Foundation in China (2018M633237), the Guangzhou Science Technology and Innovation Commission (201607020038), the Science and technology projects of Guangdong Province (2016A020215086), the Guangdong Innovative and Entrepreneurial Research Team Program (2016ZT06S638), and the leading talents of Guangdong province program.
Conflict of Interest
The authors declare that the research was conducted in the absence of any commercial or financial relationships that could be construed as a potential conflict of interest.
Glossary
References
1. Bray F, Ferlay J, Soerjomataram I, Siegel RL, Torre LA, Jemal A. Global Cancer Statistics 2018: GLOBOCAN Estimates of Incidence and Mortality Worldwide for 36 Cancers in 185 Countries. CA Cancer J Clin (2018) 68:394–424. doi: 10.3322/caac.21492
2. Siegel RL, Miller KD, Jemal A. Cancer Statistics, 2019. CA Cancer J Clin (2019) 69:7–34. doi: 10.3322/caac.21551
3. DeSantis CE, Lin CC, Mariotto AB, Siegel RL, Stein KD, Kramer JL, et al. Cancer Treatment and Survivorship Statistics, 2014. CA Cancer J Clin (2014) 64:252–71. doi: 10.3322/caac.21235
4. Siegel RL, Miller KD, Jemal A. Cancer Statistics, 2016. CA Cancer J Clin (2016) 66:7–30. doi: 10.3322/caac.21332
5. Corthay A. Does the Immune System Naturally Protect Against Cancer? Front Immunol (2014) 5:197. doi: 10.3389/fimmu.2014.00197
6. Callahan MK, Wolchok JD. At the Bedside: CTLA-4- and PD-1-Bocking Antibodies in Cancer Immunotherapy. J Leukoc Biol (2013) 94:41–53. doi: 10.1189/jlb.1212631
7. Li X, Shao C, Shi Y, Han W. Lessons Learned From the Blockade of Immune Checkpoints in Cancer Immunotherapy. J Hematol Oncol (2018) 11:31. doi: 10.1186/s13045-018-0578-4
8. Dermani FK, Samadi P, Rahmani G, Kohlan AK, Najafi R. PD-1/PD-L1 Immune Checkpoint: Potential Target for Cancer Therapy. J Cell Physiol (2019) 234:1313–25. doi: 10.1002/jcp.27172
9. Topalian SL, Drake CG, Pardoll DM. Targeting the PD-1/B7-H1(PD-L1) Pathway to Activate Anti-Tumor Immunity. Curr Opin Immunol (2012) 24:207–12. doi: 10.1016/j.coi.2011.12.009
10. Domling A, Holak TA. Programmed Death-1: Therapeutic Success After More Than 100 Years of Cancer Immunotherapy. Angew Chem Int Ed Engl (2014) 53:2286–8. doi: 10.1002/anie.201307906
11. Herbst RS, Arkenau HT, Bendell J, Arrowsmith E, Wermke M, Soriano A, et al. Phase 1 Expansion Cohort of Ramucirumab Plus Pembrolizumab in Advanced Treatment-Naive Non-Small Cell Lung Cancer. J Thorac Oncol (2020) 16:289–98. doi: 10.1016/j.jtho.2020.10.004
12. Borghaei H, Paz-Ares L, Horn L, Spigel DR, Steins M, Ready NE, et al. Nivolumab Versus Docetaxel in Advanced Nonsquamous Non-Small-Cell Lung Cancer. N Engl J Med (2015) 373:1627–39. doi: 10.1056/NEJMoa1507643
13. Rittmeyer A, Barlesi F, Waterkamp D, Park K, Ciardiello F, von Pawel J, et al. Atezolizumab Versus Docetaxel in Patients With Previously Treated non-Small-Cell Lung Cancer (OAK): A Phase 3, Open-Label, Multicentre Randomised Controlled Trial. Lancet (2017) 389:255–65. doi: 10.1016/s0140-6736(16)32517-x
14. Shibata Y, Murakami S. Safety Evaluation of Durvalumab for the Treatment of non-Small-Cell Lung Cancer. Expert Opin Drug Saf (2020) 19:653–9. doi: 10.1080/14740338.2020.1764936
15. Barlesi F, Vansteenkiste J, Spigel D, Ishii H, Garassino M, de Marinis F, et al. Avelumab Versus Docetaxel in Patients With Platinum-Treated Advanced Non-Small-Cell Lung Cancer (JAVELIN Lung 200): An Open-Label, Randomised, Phase 3 Study. Lancet Oncol (2018) 19:1468–79. doi: 10.1016/s1470-2045(18)30673-9
16. Pinto JA, Raez LE, Oliveres H, Rolfo CC. Current Knowledge of Ipilimumab and its Use in Treating non-Small Cell Lung Cancer. Expert Opin Biol Ther (2019) 19:509–15. doi: 10.1080/14712598.2019.1610380
17. Antonia SJ, Villegas A, Daniel D, Vicente D, Murakami S, Hui R, et al. Durvalumab After Chemoradiotherapy in Stage III Non-Small-Cell Lung Cancer. N Engl J Med (2017) 377:1919–29. doi: 10.1056/NEJMoa1709937
18. Zhou C, Chen G, Huang Y, Zhou J, Lin L, Feng J, et al. Camrelizumab Plus Carboplatin and Pemetrexed Versus Chemotherapy Alone in Chemotherapy-Naive Patients With Advanced Non-Squamous Non-Small-Cell Lung Cancer (CameL): A Randomised, Open-Label, Multicentre, Phase 3 Trial. Lancet Respir Med (2021) 9:305–14. doi: 10.1016/S2213-2600(20)30365-9
19. Taube JM, Klein A, Brahmer JR, Xu H, Pan X, Kim JH, et al. Association of PD-1, PD-1 Ligands, and Other Features of the Tumor Immune Microenvironment With Response to Anti-PD-1 Therapy. Clin Cancer Res (2014) 20:5064–74. doi: 10.1158/1078-0432.CCR-13-3271
20. Guo H, Bai R, Cui J. Advances in Combination Therapy of Immune Checkpoint Inhibitors for Lung Cancer. Zhongguo Fei Ai Za Zhi (2020) 23:101–10. doi: 10.3779/j.issn.1009-3419.2020.02.05
21. Nguyen LT, Ohashi PS. Clinical Blockade of PD1 and LAG3–potential Mechanisms of Action. Nat Rev Immunol (2015) 15:45–56. doi: 10.1038/nri3790
22. Voron T, Marcheteau E, Pernot S, Colussi O, Tartour E, Taieb J, et al. Control of the Immune Response by Pro-Angiogenic Factors. Front Oncol (2014) 4:70. doi: 10.3389/fonc.2014.00070
23. Charu Aggarwal NS, George R. Simon, Antiangiogenic Agents in the Management of non-Small Cell Lung Cancer Where do We Stand Now and Where are We Headed? Landes Bioscience (2012) 13:5. doi: 10.4161/cbt.13.5.19594
24. Jain RK. Normalizing Tumor Microenvironment to Treat Cancer: Bench to Bedside to Biomarkers. J Clin Oncol (2013) 31:2205–18. doi: 10.1200/JCO.2012.46.3653
25. Tredan O, Galmarini CM, Patel K, Tannock IF. Drug Resistance and the Solid Tumor Microenvironment. J Natl Cancer Inst (2007) 99:1441–54. doi: 10.1093/jnci/djm135
26. Saharinen P, Eklund L, Pulkki K, Bono P, Alitalo K. VEGF and Angiopoietin Signaling in Tumor Angiogenesis and Metastasis. Trends Mol Med (2011) 17:347–62. doi: 10.1016/j.molmed.2011.01.015
27. Hicklin DJ, Ellis LM. Role of the Vascular Endothelial Growth Factor Pathway in Tumor Growth and Angiogenesis. J Clin Oncol (2005) 23:1011–27. doi: 10.1200/JCO.2005.06.081
28. Janning M, Loges S. Anti-Angiogenics: Their Value in Lung Cancer Therapy. Oncol Res Treat (2018) 41:172–80. doi: 10.1159/000488119
29. Sandler A, Gray R, Perry MC, Brahmer J, Schiller JH, Dowlati A, et al. Paclitaxel-Carboplatin Alone or With Bevacizumab for non-Small-Cell Lung Cancer. N Engl J Med (2006) 355:2542–50. doi: 10.1056/NEJMoa061884
30. Garon EB, Ciuleanu TE, Arrieta O, Prabhash K, Syrigos KN, Goksel T, et al. Ramucirumab Plus Docetaxel Versus Placebo Plus Docetaxel for Second-Line Treatment of Stage IV Non-Small-Cell Lung Cancer After Disease Progression on Platinum-Based Therapy (REVEL): A Multicentre, Double-Blind, Randomised Phase 3 Trial. Lancet (London England) (2014) 384:665–73. doi: 10.1016/s0140-6736(14)60845-x
31. Hanna NH, Kaiser R, Sullivan RN, Aren OR, Ahn MJ, Tiangco B, et al. Nintedanib Plus Pemetrexed Versus Placebo Plus Pemetrexed in Patients With Relapsed or Refractory, Advanced Non-Small Cell Lung Cancer (LUME-Lung 2): A Randomized, Double-Blind, Phase III Trial. Lung Cancer (Amsterdam Netherlands) (2016) 102:65–73. doi: 10.1016/j.lungcan.2016.10.011
32. Han B, Li K, Wang Q, Zhang L, Shi J, Wang Z, et al. Effect of Anlotinib as a Third-Line or Further Treatment on Overall Survival of Patients With Advanced non-Small Cell Lung Cancer: The ALTER 0303 Phase 3 Randomized Clinical Trial. JAMA Oncol (2018) 4:1569–75. doi: 10.1001/jamaoncol.2018.3039
33. Yi M, Jiao D, Qin S, Chu Q, Wu K, Li A. Synergistic Effect of Immune Checkpoint Blockade and Anti-Angiogenesis in Cancer Treatment. Mol Cancer (2019) 18:60. doi: 10.1186/s12943-019-0974-6
34. Winkler F, Kozin SV, Tong RT, Chae SS, Booth MF, Garkavtsev I, et al. Kinetics of Vascular Normalization by VEGFR2 Blockade Governs Brain Tumor Response to Radiation: Role of Oxygenation, Angiopoietin-1, and Matrix Metalloproteinases. Cancer Cell (2004) 6:553–63. doi: 10.1016/j.ccr.2004.10.011
35. Mazzone M, Dettori D, de Oliveira RL, Loges S, Schmidt T, Jonckx B, et al. Heterozygous Deficiency of PHD2 Restores Tumor Oxygenation and Inhibits Metastasis Via Endothelial Normalization. Cell (2009) 136:839–51. doi: 10.1016/j.cell.2009.01.020
36. Stockmann C, Doedens A, Weidemann A, Zhang N, Takeda N, Greenberg JI, et al. Deletion of Vascular Endothelial Growth Factor in Myeloid Cells Accelerates Tumorigenesis. Nature (2008) 456:814–8. doi: 10.1038/nature07445
37. Huang Y, Yuan J, Righi E, Kamoun WS, Ancukiewicz M, Nezivar J, et al. Vascular Normalizing Doses of Antiangiogenic Treatment Reprogram the Immunosuppressive Tumor Microenvironment and Enhance Immunotherapy. Proc Natl Acad Sci USA (2012) 109:17561–6. doi: 10.1073/pnas.1215397109
38. Cheng HS, Lee JXT, Wahli W, Tan NS. Exploiting Vulnerabilities of Cancer by Targeting Nuclear Receptors of Stromal Cells in Tumor Microenvironment. Mol Cancer (2019) 18:51. doi: 10.1186/s12943-019-0971-9
39. Jain RK. Antiangiogenesis Strategies Revisited: From Starving Tumors to Alleviating Hypoxia. Cancer Cell (2014) 26:605–22. doi: 10.1016/j.ccell.2014.10.006
40. Huang Y, Kim BYS, Chan CK, Hahn SM, Weissman IL, Jiang W. Improving Immune-Vascular Crosstalk for Cancer Immunotherapy. Nat Rev Immunol (2018) 18:195–203. doi: 10.1038/nri.2017.145
41. Huang Y, Goel S, Duda DG, Fukumura D, Jain RK. Vascular Normalization as an Emerging Strategy to Enhance Cancer Immunotherapy. Cancer Res (2013) 73:2943–8. doi: 10.1158/0008-5472.CAN-12-4354
42. Facciabene A, Peng X, Hagemann IS, Balint K, Barchetti A, Wang LP, et al. Tumour Hypoxia Promotes Tolerance and Angiogenesis Via CCL28 and T(reg) Cells. Nature (2011) 475:226–30. doi: 10.1038/nature10169
43. Movahedi K, Laoui D, Gysemans C, Baeten M, Stange G, Van den Bossche J, et al. Different Tumor Microenvironments Contain Functionally Distinct Subsets of Macrophages Derived From Ly6C(high) Monocytes. Cancer Res (2010) 70:5728–39. doi: 10.1158/0008-5472.CAN-09-4672
44. Bell D, Chomarat P, Broyles D, Netto G, Harb GM, Lebecque S, et al. In Breast Carcinoma Tissue, Immature Dendritic Cells Reside Within the Tumor, Whereas Mature Dendritic Cells are Located in Peritumoral Areas. J Exp Med (1999) 190:1417–26. doi: 10.1084/jem.190.10.1417
45. Robert GCK, Melder J, Witwer BP. During Angiogenesis, Vascular Endothelial Growth Factor and Basic Fibroblast Growth Factor Regulate Natural Killer Cell Adhesion to Tumor Endothelium. Nat Publishing Group (1996) 2:992–7. doi: 10.1038/nm0996-992
46. Motz GT, Santoro SP, Wang LP, Garrabrant T, Lastra RR, Hagemann IS, et al. Tumor Endothelium FasL Establishes a Selective Immune Barrier Promoting Tolerance in Tumors. Nat Med (2014) 20:607–15. doi: 10.1038/nm.3541
47. Terme M, Pernot S, Marcheteau E, Sandoval F, Benhamouda N, Colussi O, et al. VEGFA-VEGFR Pathway Blockade Inhibits Tumor-Induced Regulatory T-cell Proliferation in Colorectal Cancer. Cancer Res (2013) 73:539–49. doi: 10.1158/0008-5472.CAN-12-2325
48. Gabrilovich D, Ishida T, Oyama T, Ran S, Kravtsov V, Nadaf S, et al. Vascular Endothelial Growth Factor Inhibits the Development of Dendritic Cells and Dramatically Affects the Differentiation of Multiple Hematopoietic Lineages In Vivo. Blood (1998) 92:4150–66. doi: 10.1182/blood.V92.11.4150
49. Fukumura D, Kloepper J, Amoozgar Z, Duda DG, Jain RK. Enhancing Cancer Immunotherapy Using Antiangiogenics: Opportunities and Challenges. Nat Rev Clin Oncol (2018) 15:325–40. doi: 10.1038/nrclinonc.2018.29
50. Kudo M. Scientific Rationale for Combined Immunotherapy With PD-1/PD-L1 Antibodies and VEGF Inhibitors in Advanced Hepatocellular Carcinoma. Cancers (Basel) (2020) 12:1089. doi: 10.3390/cancers12051089
51. Kantoff PW, Higano CS, Shore ND, Berger ER, Small EJ, Penson DF, et al. Sipuleucel-T Immunotherapy for Castration-Resistant Prostate Cancer. N Engl J Med (2010) 363:411–22. doi: 10.1056/NEJMoa1001294
52. Dmitry HLC, Gabrilovich I, Girgis KR, Cunningham HT. Production of Vascular Endothelial Growth Factor by Human Tumors Inhibits the Functional Maturation of Dendritic Cells. Nat Publishing Group (1996) 2:1267. doi: 10.1038/nm1096-1096
53. Huang Y, Chen X, Dikov MM, Novitskiy SV, Mosse CA, Yang L, et al. Distinct Roles of VEGFR-1 and VEGFR-2 in the Aberrant Hematopoiesis Associated With Elevated Levels of VEGF. Blood (2007) 110:624–31. doi: 10.1182/blood-2007-01-065714
54. Alfaro C, Suarez N, Gonzalez A, Solano S, Erro L, Dubrot J, et al. Influence of Bevacizumab, Sunitinib and Sorafenib as Single Agents or in Combination on the Inhibitory Effects of VEGF on Human Dendritic Cell Differentiation From Monocytes. Br J Cancer (2009) 100:1111–9. doi: 10.1038/sj.bjc.6604965
55. Tsunehiro Oyama SR, Ishida T, Nadaf S. Vascular Endothelial Growth Factor Affects Dendritic Cell Maturation Through the Inhibition of Nuclear Factor- κB Activation in Hemopoietic Progenitor Cells. J Immunol (1996) 160:1224–32.
56. Gallucci S, Lolkema M, Matzinger P. Natural Adjuvants: Endogenous Activators of Dendritic Cells. Nat Med (1999) 5:1249–55. doi: 10.1038/15200
57. Heine A, Held SA, Daecke SN, Riethausen K, Kotthoff P, Flores C, et al. The VEGF-Receptor Inhibitor Axitinib Impairs Dendritic Cell Phenotype and Function. PloS One (2015) 10:e0128897. doi: 10.1371/journal.pone.0128897
58. Curiel TJ. Tregs and Rethinking Cancer Immunotherapy. J Clin Invest (2007) 117:1167–74. doi: 10.1172/JCI31202
59. Wada J, Suzuki H, Fuchino R, Yamasaki A, Nagai S, Yanai K, et al. The Contribution of Vascular Endothelial Growth Factor to the Induction of Regulatory T-cells in Malignant Effusions. Anticancer Res (2009) 29:881–8.
60. Suzuki H, Onishi H, Wada J, Yamasaki A, Tanaka H, Nakano K, et al. VEGFR2 is Selectively Expressed by FOXP3high CD4+ Treg. Eur J Immunol (2010) 40:197–203. doi: 10.1002/eji.200939887
61. Seifi-Alan M, Shams R, Bandehpour M, Mirfakhraie R, Ghafouri-Fard S. Neuropilin-1 Expression is Associated With Lymph Node Metastasis in Breast Cancer Tissues. Cancer Manag Res (2018) 10:1969–74. doi: 10.2147/CMAR.S169533
62. Kandalaft LE, Motz GT, Busch J, Coukos G. Angiogenesis and the Tumor Vasculature as Antitumor Immune Modulators: The Role of Vascular Endothelial Growth Factor and Endothelin. Curr Top Microbiol Immunol (2011) 344:129–48. doi: 10.1007/82_2010_95
63. Hansen W, Hutzler M, Abel S, Alter C, Stockmann C, Kliche S, et al. Neuropilin 1 Deficiency on CD4+Foxp3+ Regulatory T Cells Impairs Mouse Melanoma Growth. J Exp Med (2012) 209:2001–16. doi: 10.1084/jem.20111497
64. Roland CL, Lynn KD, Toombs JE, Dineen SP, Udugamasooriya DG, Brekken RA. Cytokine Levels Correlate With Immune Cell Infiltration After anti-VEGF Therapy in Preclinical Mouse Models of Breast Cancer. PloS One (2009) 4:e7669. doi: 10.1371/journal.pone.0007669
65. Li B, Lalani AS, Harding TC, Luan B, Koprivnikar K, Huan Tu G, et al. Vascular Endothelial Growth Factor Blockade Reduces Intratumoral Regulatory T Cells and Enhances the Efficacy of a GM-CSF-secreting Cancer Immunotherapy. Clin Cancer Res (2006) 12:6808–16. doi: 10.1158/1078-0432.CCR-06-1558
66. Finke JH, Rini B, Ireland J, Rayman P, Richmond A, Golshayan A, et al. Sunitinib Reverses Type-1 Immune Suppression and Decreases T-regulatory Cells in Renal Cell Carcinoma Patients. Clin Cancer Res (2008) 14:6674–82. doi: 10.1158/1078-0432.CCR-07-5212
67. Gabrilovich DI, Ostrand-Rosenberg S, Bronte V. Coordinated Regulation of Myeloid Cells by Tumours. Nat Rev Immunol (2012) 12:253–68. doi: 10.1038/nri3175
68. Huang B, Pan PY, Li Q, Sato AI, Levy DE, Bromberg J, et al. Gr-1+CD115+ Immature Myeloid Suppressor Cells Mediate the Development of Tumor-Induced T Regulatory Cells and T-cell Anergy in Tumor-Bearing Host. Cancer Res (2006) 66:1123–31. doi: 10.1158/0008-5472.CAN-05-1299
69. Nefedova Y, Huang M, Kusmartsev S, Bhattacharya R, Cheng P, Salup R, et al. Hyperactivation of STAT3 is Involved in Abnormal Differentiation of Dendritic Cells in Cancer. J Immunol (2004) 172:464–74. doi: 10.4049/jimmunol.172.1.464
70. Draghiciu O, Nijman HW, Hoogeboom BN, Meijerhof T, Daemen T. Sunitinib Depletes Myeloid-Derived Suppressor Cells and Synergizes With a Cancer Vaccine to Enhance Antigen-Specific Immune Responses and Tumor Eradication. Oncoimmunology (2015) 4:e989764. doi: 10.4161/2162402X.2014.989764
71. Ozao-Choy J, Ma G, Kao J, Wang GX, Meseck M, Sung M, et al. The Novel Role of Tyrosine Kinase Inhibitor in the Reversal of Immune Suppression and Modulation of Tumor Microenvironment for Immune-Based Cancer Therapies. Cancer Res (2009) 69:2514–22. doi: 10.1158/0008-5472.CAN-08-4709
72. Du Four S, Maenhout SK, De Pierre K, Renmans D, Niclou SP, Thielemans K, et al. Axitinib Increases the Infiltration of Immune Cells and Reduces the Suppressive Capacity of Monocytic MDSCs in an Intracranial Mouse Melanoma Model. Oncoimmunology (2015) 4:e998107. doi: 10.1080/2162402X.2014.998107
73. Ko JS, Zea AH, Rini BI, Ireland JL, Elson P, Cohen P, et al. Sunitinib Mediates Reversal of Myeloid-Derived Suppressor Cell Accumulation in Renal Cell Carcinoma Patients. Clin Cancer Res (2009) 15:2148–57. doi: 10.1158/1078-0432.CCR-08-1332
74. Koinis F, Vetsika EK, Aggouraki D, Skalidaki E, Koutoulaki A, Gkioulmpasani M, et al. Effect of First-Line Treatment on Myeloid-Derived Suppressor Cells’ Subpopulations in the Peripheral Blood of Patients With Non-Small Cell Lung Cancer. J Thorac Oncol (2016) 11:1263–72. doi: 10.1016/j.jtho.2016.04.026
75. Lapeyre-Prost A, Terme M, Pernot S, Pointet AL, Voron T, Tartour E, et al. Immunomodulatory Activity of VEGF in Cancer. Int Rev Cell Mol Biol (2017) 330:295–342. doi: 10.1016/bs.ircmb.2016.09.007
76. Gabrusiewicz K, Liu D, Cortes-Santiago N, Hossain MB, Conrad CA, Aldape KD, et al. Anti-Vascular Endothelial Growth Factor Therapy-Induced Glioma Invasion is Associated With Accumulation of Tie2-expressing Monocytes. Oncotarget (2014) 5:2208–20. doi: 10.18632/oncotarget.1893
77. Dalton HJ, Pradeep S, McGuire M, Hailemichael Y, Ma S, Lyons Y, et al. Macrophages Facilitate Resistance to Anti-VEGF Therapy by Altered VEGFR Expression. Clin Cancer Res (2017) 23:7034–46. doi: 10.1158/1078-0432.CCR-17-0647
78. Peterson TE, Kirkpatrick ND, Huang Y, Farrar CT, Marijt KA, Kloepper J, et al. Dual Inhibition of Ang-2 and VEGF Receptors Normalizes Tumor Vasculature and Prolongs Survival in Glioblastoma by Altering Macrophages. Proc Natl Acad Sci USA (2016) 113:4470–5. doi: 10.1073/pnas.1525349113
79. Deng L, Stafford JH, Liu SC, Chernikova SB, Merchant M, Recht L, et al. SDF-1 Blockade Enhances Anti-VEGF Therapy of Glioblastoma and Can be Monitored by MRI. Neoplasia (2017) 19:1–7. doi: 10.1016/j.neo.2016.11.010
80. Pham K, Luo D, Siemann DW, Law BK, Reynolds BA, Hothi P, et al. VEGFR Inhibitors Upregulate CXCR4 in VEGF Receptor-Expressing Glioblastoma in a TGFbetaR Signaling-Dependent Manner. Cancer Lett (2015) 360:60–7. doi: 10.1016/j.canlet.2015.02.005
81. Zhang L, Conejo-Garcia JR, Katsaros D, Gimotty PA, Massobrio M, Regnani G, et al. Intratumoral T Cells, Recurrence, and Survival in Epithelial Ovarian Cancer. N Engl J Med (2003) 348:203–13. doi: 10.1056/NEJMoa020177
82. Ohm JE, Gabrilovich DI, Sempowski GD, Kisseleva E, Parman KS, Nadaf S, et al. VEGF Inhibits T-cell Development and may Contribute to Tumor-Induced Immune Suppression. Blood (2003) 101:4878–86. doi: 10.1182/blood-2002-07-1956
83. Voron T, Colussi O, Marcheteau E, Pernot S, Nizard M, Pointet AL, et al. VEGF-a Modulates Expression of Inhibitory Checkpoints on CD8+ T Cells in Tumors. J Exp Med (2015) 212:139–48. doi: 10.1084/jem.20140559
84. Manzoni M, Rovati B, Ronzoni M, Loupakis F, Mariucci S, Ricci V, et al. Immunological Effects of Bevacizumab-Based Treatment in Metastatic Colorectal Cancer. Oncology (2010) 79:187–96. doi: 10.1159/000320609
85. Martino EC, Misso G, Pastina P, Costantini S, Vanni F, Gandolfo C, et al. Immune-Modulating Effects of Bevacizumab in Metastatic Non-Small-Cell Lung Cancer Patients. Cell Death Discovery (2016) 2:16025. doi: 10.1038/cddiscovery.2016.25
86. Martina Schmittnaegel NR, Kadioglu E. Dual Angiopoietin-2 and VEGFA Inhibition Elicits Antitumor Immunity That Is Enhanced by PD-1 Checkpoint Blockade. Sci Transl Med (2017) 9:eaak9670. doi: 10.1126/scitranslmed.aak9670
87. Beatty G, Paterson Y. IFN-Gamma-Dependent Inhibition of Tumor Angiogenesis by Tumor-Infiltrating CD4+ T Cells Requires Tumor Responsiveness to IFN-Gamma. J Immunol (2001) 166:2276–82. doi: 10.4049/jimmunol.166.4.2276
88. Kato Y, Tabata K, Kimura T, Yachie-Kinoshita A, Ozawa Y, Yamada K, et al. Lenvatinib Plus anti-PD-1 Antibody Combination Treatment Activates CD8+ T Cells Through Reduction of Tumor-Associated Macrophage and Activation of the Interferon Pathway. PloS One (2019) 14:e0212513. doi: 10.1371/journal.pone.0212513
89. Shi S, Wang R, Chen Y, Song H, Chen L, Huang G. Combining Antiangiogenic Therapy With Adoptive Cell Immunotherapy Exerts Better Antitumor Effects in non-Small Cell Lung Cancer Models. PloS One (2013) 8:e65757. doi: 10.1371/journal.pone.0065757
90. Tao L, Huang G, Shi S, Chen L. Bevacizumab Improves the Antitumor Efficacy of Adoptive Cytokine-Induced Killer Cells Therapy in non-Small Cell Lung Cancer Models. Med Oncol (2014) 31:777. doi: 10.1007/s12032-013-0777-3
91. Zhao S, Ren S, Jiang T, Zhu B, Li X, Zhao C, et al. Low-Dose Apatinib Optimizes Tumor Microenvironment and Potentiates Antitumor Effect of PD-1/PD-L1 Blockade in Lung Cancer. Cancer Immunol Res (2019) 7:630–43. doi: 10.1158/2326-6066.Cir-17-0640
92. Roy S Herbst H-TA, Santana-Davila R, Calvo E, Paz-Ares L, Cassier PA, Bendell J, et al. Ramucirumab Plus Pembrolizumab in Patients With Previously Treated Advanced Non-Small-Cell Lung Cancer, Gastro-Oesophageal Cancer, or Urothelial Carcinomas (JVDF):a Multicohort, Non-Randomised, Open-Label, Phase 1A/B Trial. Lancet Oncol (2019) 20:1109–23. doi: 10.1016/S1470-2045(19)30458-9
93. Reck M, Mok TSK, Nishio M, Jotte RM, Cappuzzo F, Orlandi F, et al. Atezolizumab Plus Bevacizumab and Chemotherapy in Non-Small-Cell Lung Cancer (Impower150): Key Subgroup Analyses of Patients With EGFR Mutations or Baseline Liver Metastases in a Randomised, Open-Label Phase 3 Trial. Lancet Respir Med (2019) 7:387–401. doi: 10.1016/s2213-2600(19)30084-0
94. Finn RS, Qin S, Ikeda M, Galle PR, Ducreux M, Kim TY, et al. Atezolizumab Plus Bevacizumab in Unresectable Hepatocellular Carcinoma. N Engl J Med (2020) 382:1894–905. doi: 10.1056/NEJMoa1915745
Keywords: NSCLC, immunotherapy, immune checkpoint blockade, angiogenesis inhibitors, combination therapy, tumor microenvironment
Citation: Ren S, Xiong X, You H, Shen J and Zhou P (2021) The Combination of Immune Checkpoint Blockade and Angiogenesis Inhibitors in the Treatment of Advanced Non-Small Cell Lung Cancer. Front. Immunol. 12:689132. doi: 10.3389/fimmu.2021.689132
Received: 31 March 2021; Accepted: 18 May 2021;
Published: 02 June 2021.
Edited by:
Xi Wang, Capital Medical University, ChinaReviewed by:
Ming Yi, Huazhong University of Science and Technology, ChinaXing Chang, Westlake University, China
Yuhui Huang, Soochow University, China
Copyright © 2021 Ren, Xiong, You, Shen and Zhou. This is an open-access article distributed under the terms of the Creative Commons Attribution License (CC BY). The use, distribution or reproduction in other forums is permitted, provided the original author(s) and the copyright owner(s) are credited and that the original publication in this journal is cited, in accordance with accepted academic practice. No use, distribution or reproduction is permitted which does not comply with these terms.
*Correspondence: Jianfei Shen, amlhbmZlaTA1MUAxNjMuY29t; Penghui Zhou, emhvdXBoQHN5c3VjYy5vcmcuY24=
†These authors have contributed equally to this work