- 1School of Pharmacy, Section of Experimental Medicine, University of Camerino, Camerino, Italy
- 2School of Biosciences and Veterinary Medicine, University of Camerino, Camerino, Italy
- 3Macerata Hospital, Oncology Unit, Macerata, Italy
- 4Department of Molecular Medicine, Sapienza University, Rome, Italy
- 5IRCCS Neuromed, Pozzilli, Italy
Natural killer (NK) cells are a main subset of innate lymphocytes that contribute to host immune protection against viruses and tumors by mediating target cell killing and secreting a wide array of cytokines. Their functions are finely regulated by a balance between activating and inhibitory receptors and involve also adhesive interactions. Mechanotransduction is the process in which physical forces sensed by mechanosensors are translated into chemical signaling. Herein, we report findings on the involvement of this mechanism that is mainly mediated by actin cytoskeleton, in the regulation of NK cell adhesion, migration, tissue infiltration and functions. Actin represents the structural basis for NK cell immunological synapse (NKIS) and polarization of secretory apparatus. NK-target cell interaction involves the formation of both uropods and membrane nanotubes that allow target cell interaction over long distances. Actin retrograde flow (ARF) regulates NK cell signaling and controls the equilibrium between activation versus inhibition. Activating NKIS is associated with rapid lamellipodial ARF, whereas lower centripetal actin flow is present during inhibitory NKIS where β actin can associate with the tyrosine phosphatase SHP-1. Overall, a better knowledge of mechanotransduction might represent a future challenge: Realization of nanomaterials tailored for NK cells, would be important to translate in vitro studies in in vivo new immunotherapeutic approaches.
Natural Killer (NK) Cell Target Recognition and Functions
Natural Killer (NK) cells represent the prototype of innate lymphoid cells that act as first line of defense against microbial infections, and tumor cell transformation, growth and metastatic spreading (1–3). NK cells prompt the response mainly on their ability to release lytic mediators, such as perforin and granzymes, or to express ligands triggering death receptors on target cells; moreover, they can secrete a wide array of cytokines and chemokines to recruit and educate other immune cell types (4, 5). NK cell activation depends on a delicate balance between activating and inhibitory signals, being the latter mainly transduced by killer-cell immunoglobulin-like receptors (KIRs), cluster of differentiation 94 (CD94)/natural-killer group 2, member A (NKG2A) receptors for class I MHC. Recognition of abnormal self on tumor or viral infected cells triggers a number of non MHC I-restricted activating receptors such as NKG2D, the receptor for the human MHC I-related sequence A and B (MICA/B) and UL16 binding proteins (ULBPs), DNAX accessory molecule-1 (DNAM-1) that recognizes nectin-2 (Nec2, CD112) and nectin-15 (Necl5, PVR, CD155), and the natural citotoxicity receptors (NCR) receptors (1). In addition, NK cells express receptors belonging to the β1 and β2 integrin family with lymphocyte function-associated antigen 1 (LFA-1) playing a central role in priming NK cells for cytotoxicity. The dialog of the β1 and β2 integrins with a number of chemokine receptors is also crucial for the homing and migration of NK cells in lymphoid and non-lymphoid organs.
Moreover, it is becoming clear that although they act in cooperative manner, different activating receptors can independently trigger discrete steps of NK cytolytic process (i.e. target cell contact and adhesion, granule polarization, degranulation and target lysis) by initiating diverse signaling cascades (6). In this regard, signaling components controlling cytoskeleton rearrangement, are emerging as critical events for NK cell cytotoxicity and migration.
NK/target cell interaction is regulated by adhesion molecules such as β1 and β2 integrins that play either a receptor or co-receptor role (7). High-avidity/affinity of integrins occurs in response of different activating receptors and depends on the activation of different signaling pathways including tyrosine kinases (PTK) belonging to the proto-oncogene tyrosine-protein kinase (Src) family, phosphoinositide 3-kinase (PI3K), small G proteins, and cytoskeletal integrity (inside-outside signaling). β2 integrins have been also shown to be critical in the interaction between NK and target cells by controlling the formation of the immunological synapse, which is an assembly of membrane receptors and signal transduction molecules largely driven by actin cytoskeleton. Development of NK cell cytotoxic functions requires the activation of a complex cascade of signaling pathways, including the activation of spleen tyrosine kinase (Syk) family PTKs, phospholipase C gamma (PLC-γ) and D (PLD), PI3K, Vav/Rac pathway, ERK, p38 and MAPKs (6). Some of these events are shared by different activating receptors, but distinct signals are also transduced depending on the type of receptor or the sensitive target triggering the cytotoxicity. In this regard, it has been reported that the activation of focal adhesion PTK Pyk2, that has been shown to be activated by β1 and β2 integrins, is a discriminating event between natural and antibody-mediated cytotoxicity. Moreover, ligation of integrins on human NK cells transduces intracellular signals leading to tyrosine phosphorylation of paxillin, intracellular calcium elevation (8), and co-stimulation of NK cell cytotoxic functions.
Mechanosensing in the NK Cell-Mediated Immune Responses
Mechanotransduction is the process in which physical forces sensed by mechanosensors are translated into chemical signaling pathways. This mechanism mediated by the cytoskeleton that serves as a global mechanosensor apparatus, permits cells to sense their extracellular environment and rapidly respond to different stimuli. NK cell mechanosensors include a large range of activating receptors as well as β1 and β2 integrins and CD62L selectin (9, 10).The actomyosin network plays an important role in mechanotransduction, in that actin polymerization generates a “pushing” force, whereas the myosin produces a “pulling” force, and together are translated into several signaling cascades (11, 12). Thus, the actin cytoskeleton provides the mechanical forces necessary for adhesion, migration and tissue infiltration of NK cells as well as for their cytotoxic function. The actin interactome represents the structural basis for the formation of a stable NKIS, integration of molecular complexes and signaling components, and the secretion of cytolytic granules and mediators (e.g., perforin) leading to target cell killing. The mature activating NKIS contains a central and peripheral supramolecular activation cluster (SMAC). The β2 integrins, namely αLβ2 (LFA-1) and αMβ2 (Mac-1) and F-actin accumulate in the peripheral SMAC (pSMAC), whereas perforin is present in the central SMAC. The accumulation of F-actin and β2 integrins is rapid, it depends on Wiskott–Aldrich syndrome protein (WASp)-driven actin polymerization, and is not affected by microtubule depolymerization. Conversely, the polarization of perforin is slower and requires intact actin, WASp protein, and microtubule function (13–15).
Uropods and Nanotubes Mediate Mechanotaxis in NK Cells
Cell guiding is involved in a number of biological processes, but however, its mechanisms remain still partially elucidated. Immune cells are able to migrate directionally thanks to chemical guiding or chemotaxis in response to chemo-attractants, to haptotaxis in response to surface-bound chemicals, and to mechanical guiding or mechanotaxis in response to mechanical stimuli such as substrate stiffness, cell deformation or osmotic stress (16).
A large body of evidences mainly regard chemotaxis, whereas mechanotaxis has been considered only recently. Although a role in the activation of leukocytes has been reported (17), the basic remain largely elusive. Immune cell trafficking is not only supported by chemical signals, but also involves mechanical signals like hydrodynamic shear stress (18–21). The external forces are perceived by leukocytes mainly through the integrin receptors, which undergo conformational changes by inside-out (22) and outside-in (23) signaling, and initiate an intracellular signaling cascade in response to mechanical forces. Thus, integrins are key players both in the tissue recruitment of leukocytes from blood flow, and in leukocyte mechanotaxis under flow.
Two types of orientation mechanisms by flow have been suggested to mediate leukocyte integrin-mediated adhesion. Shear stress involves integrin-mediated outside-in signaling at anchoring sites (24–26). Such mechanisms are considered “active”, in that a specific intracellular signaling pathway is initiated in response to flow, and relies on mechanotransduction. Alternatively, a “passive” model that does not require signaling by the external cue, has been also proposed for upstream crawling lymphocytes (27). In this model, flow direction is detected by the passive orientation uropod, which is not adherent and freely rotates. Reorientation of the whole cell against flow, follows tail orientation via the cell realignment by front-rear polarization. At molecular level, the cross-talk between LFA-1 and integrin very late antigen-4 (VLA-4, α4β1) and the opposite polarization of LFA-1 and VLA-4 integrins sustain a differential adhesion of leukocytes either by their leading or trailing edge.
NK cells circulate in the blood against fluid flow, as shown by the orientation of the non-adherent cell rear, the uropod. Uropods sense and transmit flow directions into cell steering through the polarity maintenance (27). In addition, NK cell migration involves the convergence of signaling events triggered by engagement of both β2 and β1 integrins, and a number of chemokine receptors including C–X–C motif chemokine receptor (CXCR) 4, C–C chemokine receptor type (CCR) 2, CCR5 and CXCR3 (28, 29).
Cell polarization is also a crucial event for the formation of NK/target cell conjugates. NK cells contact target cells exploiting the region with high concentration of CCR2 and CCR5, whereas the ligands of β2 integrin intercellular adhesion molecule (ICAM)-1 and ICAM-3, are concentrated at the distal pole in the uropods. Blocking cell polarization and adhesion receptor redistribution, inhibits NK cell cytotoxic activity as result of impaired effector–target cell conjugate formation. Thus, cell polarization regulates different steps of NK cell functions: the leading edge where the CCR are concentrated, is involved in the adhesion to target cells, polarization of the secretory apparatus and release of lytic granules during the NK cell killing (30, 31); remarkably, different types of lytic granules undergo polarized secretion to the site of membrane contact between NK and target cells (32). The uropods by accumulating ICAM molecules, are involved in the recruitment of NK cells.
Cell polarization and cytotoxic activity, can be blocked by adenosine diphosphate (ADP) ribosylation of the guanosine-5’-triphosphate (GTP) binding protein Ras homolog family member A (RhoA), indicating the important role of this signaling pathway triggered by LFA-1 as well as by CCR in these events (33, 34).
Notably, formation of NK/target cell conjugates also stimulates chemokine release, which can further promote NK cell binding to target cells by inducing integrin inside-outside signaling and support NK cell migration.
Beside the involvement of uropods in the regulation of the intimate NK cell contact with target cells, NK cells can also generate membrane nanotubes that allow these cells to functionally interact with targets over long distances. Target cells that relocate along the nanotube path, are polarized with their uropods facing the direction of movement, are then lysed. Removing the nanotubes by a micromanipulator reduces target cell lysis (35). The frequency of nanotube formation depends on the number of interactions between activating receptor and the respective ligand(s), and increases upon the NK cell activation. Imaging studies have demonstrated that proteins, such as the signaling adaptor that associates with NKG2D, DAP10, the G protein exchange factor Vav-1, and the NKG2D ligand MICA accumulate at nanotube synapses in a such high concentration to stimulate cell activation (35).
NK Immunological Synapse (NKIS): Role of the Actin Cytoskeleton and Retrograde Flow in Controlling NK Cell Responses
As above mentioned, NK cell cytotoxicity is a tightly regulated multistep process. It moves through the initial contact between NK cells with target cells which is mediated by tethering receptors such as CD2 and the selectin CD62L, adhesive integrin receptors (LFA1 and Mac1) that interact with ICAM1, and activating receptors such as NCRs, NKG2D and DNAM1 (36). Both activating and integrin signaling initiate the formation of NKIS. In particular, engagement of β2 integrins, but not of CD-16 activating receptor on CD16.NK-92 cells, was found to affect the size and the dynamics of signaling microclusters in a Pyk2-dependent manner (37).
Signaling is required for cytoskeleton remodeling, formation of activating microclusters and adhesion ring junction, polarization of effector cells and cytolytic granule releases (34). Accumulation of F-actin at the NKIS is the pre-requisite for the clustering of activating receptors which stimulate the polarization of cytolytic granules to the immunological synapse (IS). Lytic granules navigate to actin meshwork at the IS and reach the plasma membrane through gaps sized to accommodate lytic granule movements. The movement of lytic granules is dependent to myosin IIa that generates force and movements along actin filaments. In NK cells, myosin IIa mediates the interaction between lytic granules and F-actin UNC45-dependent manner at the NKIS, and facilitates the movement of lytic cargo along actin filaments (38). The movement of lytic granules toward the IS, initially depends on dynein that mediates the movement of secretory vesicles to the MTOC (39), and then on the granule-associated small GTPase Rab27a that recruits the Slp3–kinesin-1 complex, thus enabling the polarized cytotoxic granules to reach the membrane and release their contents at the IS (9, 40, 41). Activating receptor-triggered secretion of lytic granules requires binding of Rab27a to Munc13-4, with formation of a complex that co-localizes with lytic granules (42). Lytic granules docked and tethered to NKIS, are primed through interaction between Munc 13-4 and STX11 to form a trans-soluble NSF attachment protein receptor (SNARE) complex comprising the syntaxin STX11 and synaptosomal SNAP23 protein together with the SNARE proteins vesicle associated membrane protein (VAMP) 4 and VAMP7, likely mediated by syntaxin-binding protein 2 (STXBP2) (43). After the fusion of lytic granules at the synaptic cleft, the lytic granule membrane proteins are endocytosed to replenish lytic granules for further killing.
F-actin is a major driver of the IS formation between NK and target cells, and the polarization of the secretory apparatus (44–46). Actin retrograde flow (ARF) regulates NK cell signaling and controls the equilibrium between NK cell activation versus inhibition. The actomyosin dynamics is responsible for the “mechanotransduction” (11, 12, 47). The F-actin turnover and myosin IIa force at the NKIS are the most important forces involved in the guide of the F-actin centripetal flow, with actin polymerization pushing at the external part of the spreading cell, and the contractile myosin force pulling the F-actin away from the cell membrane (48). It has been well accepted that the actin flow plays a pivotal role in the centralization of receptors and signaling proteins. Activating NKIS is associated with a rapid lamellipodial ARF, whereas a lower centripetal actin flow is present during inhibitory NKIS (49). It has been recently demonstrated that tyrosine protein phosphatase (SHP)-1 and β-actin specifically associate at the inhibitory NKIS where ARF is slower. In addition, SHP-1 is maintained in a closed status and catalytically inactive form, if actin flow is inhibited by using jasplakinolide or cytochalasin D pharmacological inhibitors, or by growing cells on rigid surfaces. Thus, SHP-1 inhibition is the result of actin flow reduction that leads to increased Vav-1 and PLCγ-1/2 tyrosine phosphorylation and to NK cell activation. SHP-1 does not interact with the actin machinery when ARF velocity is rapid and activating NK cell interactions occur; in this case it has a closed catalytically inactive conformation, allowing Vav-1 and PLC-γ1/2 tyrosine phosphorylation (Figure 1) (47, 50). Thus, stimulation of NK cell activating receptors induces a fast actin flow that inhibits the binding of SHP-1 with the actin network. This NK activating status favors SHP-1 closed inactive conformation promoting target cell killing. Engagement of KIR inhibitory receptors results in slow actin flow, enabling the formation of the SHP-1/β-actin complex. SHP-1 inhibits NK cell activation by dephosphorylating important signaling molecules. Given that the actin flow dynamics rapidly change, it may enable a rapid switching from an inhibitory to activating NK cell response (47).
NK cell inhibition is also mediated by SHP-2 recruitment to KIR receptors (51, 52), and in non-immune cells SHP-2 is associated with the actin cytoskeleton (53). Given that SHP-1 and SHP-2 display a high level of structural homology, it can be suggested that a similar regulation of SHP-2 occurs also in NK cells via dynamic actin movement.
Overall, these data indicate that actin mechanotransduction is essential to increase the NK cell capability to rapidly respond to local environmental changes. While NKIS formation requires minutes/hours and is a slow process, alterations of the actin flow dynamics and the following SHP-1 status are fast events (seconds/minutes) that permit a rapid on/off control of inhibitory signaling (47). Thus, ARF, by controlling actin dynamics, represents a novel mechanism to regulate the functional outcome of NK cells.
Mechanical Properties of Surrounding Matrix and Targets Drive NK Cell Responsiveness
During their lifetime, NK cells infiltrate a variety of normal, inflamed, or neoplastic tissues with different mechanical properties, exposing the NK cells to big fluctuations in matrix stiffness with potential impact on their responsiveness (54). Moreover, during the development, bone-marrow NK cells are exposed to substrate stiffness ranging from 25 to 100 kPa (55). Both NK cell degranulation and cytokine production are modified by the rigidity of the target substrate. In the study by Malaton and colleagues, the effect of substrate stiffness on efficiency of NK cell degranulation was evaluated by using a cell-sized beads coated with sodium alginate at defined soft (9 kPa), medium (34 kPa) or stiff (254 kPa) alone, or in the presence of antibodies directed against LFA-1 or the NCR receptor NKp30. Increased substrate stiffness stimulated NK cell degranulation, that was further enhanced by NKp30 and LFA1 triggering. The lower degranulating capacity of NK cells contacting soft targets was suggested to depend on an impaired recruitment of phosphorylated zinc-finger antiviral protein (Zap) 70 PTK to the NKIS (47). Similarly, a recent report demonstrated that target rigidity impacts on perforin granule polarization and degranulation, being secretion of granzymes A and B, granulysin and FAS ligand improved with increased substrate stiffness (56). Soft target stiffness was found to reduce F-actin accumulation and talin polarization and recruitment at the NKIS, leading to the formation of an instable asymmetrical synapse and decreased proportion of NK cells in the conjugates with targets. In addition, interaction with softer targets resulted in impaired microtubule organizing center (MTOC) and lytic granule polarization (Figure 2) (56).
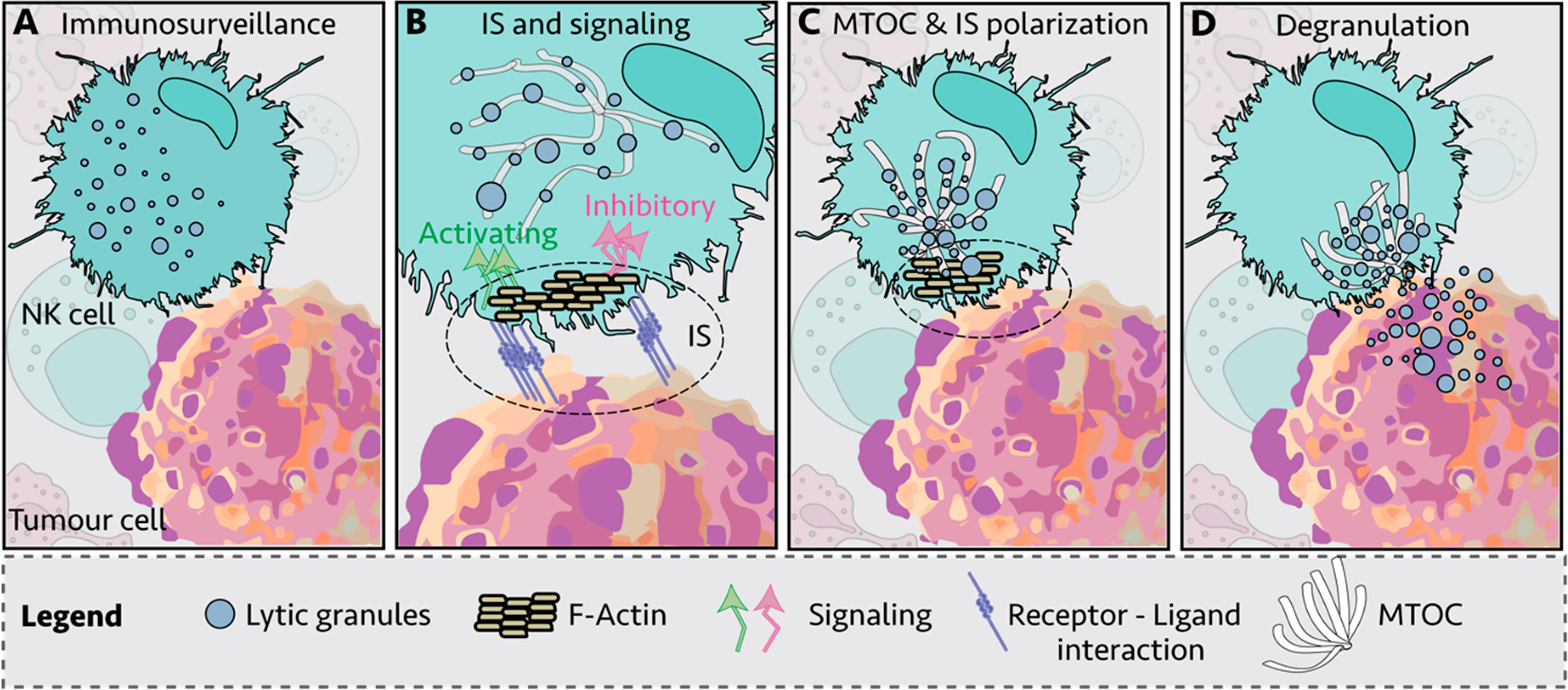
Figure 2 Immunological synapse (IS) of Natural Killer (NK) cell and target cell. (A) NK cells engage other cells to create an immunological synapse (IS); (B) First, filamentous actin (F-actin) is recruited to the IS; (C) NK lytic granules move along microtubules by dynein–dynactin motor proteins toward the microtubule-organizing center (MTOC); (D) the polarized lytic granules and MTOC dock at the IS, and degranulate (57).
Stiffness of the activating substrates not only impacts on NK cell cytotoxic function, but secretion of cytokines, namely interferon-gamma (IFN-γ), is also modulated, with increased release observed upon NK cell interaction with the stiffer substrates.
The stiffness properties of a cell undergoes changes during the neoplastic process, with primary tumor cells being stiffer than healthy cells, and conversely metastatic cells showing profound reduction in stiffness (58); in addition, also viral infection increases stiffness by inducing cortical actin rearrangement (59). Thus, we would like to envisage that stiffening or softening would be able to profoundly affect the responsiveness of NK cells infiltrating neoplastic or infected tissues. Because no evidences are presently addressing this issue, we would like to suggest that investigation of mechanotransduction mechanisms in tissue resident NK cells would represent a research area of increasing interest.
Nanomaterials in the Regulation of NK Cell Functions
The use of nanowires or nanodots functionalized or not with ligands, permits now to explore more in depth the mechanosensitivity of NK cells, and in particular the mechanical features of NKIS. Shaping NK cell activity by nanomaterials to functionally upregulate their activating receptors, may represent an emerging strategy for NK-cell based adaptive immunotherapy. Enhanced NK cell activation on antigen-functionalized and mechanically stimulating nanowires and nanodots, and the isolation of activated NK cell subpopulations, foreruns novel nanoengineered platforms for cell expansion toward therapeutic purposes, with improved efficiency and control of cytotoxic activity (60).
Finally, in the recent years with the development of chimeric antigen receptor (CARs) of T and NK lineages, researchers are closer to achieving high specificity and low off-side effects, with clinical trials achieving remission rates. In this regard, a role of mechanosensing in the antigen/target discrimination is a key to engineered high specific CARs. Further work would be required to completely address the mechanosensing properties of NK cells in vivo. These studies can provide important fundamental insights on cytotoxic functions of NK cells and allow rational design of future immunotherapies.
Concluding Remarks
Cells sense their environment by transducing mechanical stimuli into biochemical signals. Conventional tools for study cell mechanosensing provide limited spatial and force resolution. Recent advances in biomaterials and device engineering have facilitated the generation of numerous artificial cellular microenvironments, which produce synthetic signals mimicking those delivered by the physiological environment. Several advanced materials and devices have been recently produced for the study of mechanical activity in NK cells. NK cells exposed to nanowires functionalized with MICA ligands exhibit higher expression of CD107 degranulation marker, suggesting that the combination of a physical stimulus with the chemical stimulus of MICA, enhances NK cell activation (60, 61). This model may mimic the in vivo NK cell/dendritic cell interaction, where the branched projections of DC are similar in size and shape to nanowires (62).
Author Contributions
GS and AS drafted the manuscript. GS conceived and designed the study. MM, CA, FM, and MS critically revised the manuscript. All authors contributed to the article and approved the submitted version.
Funding
PRIN-2017 Italian Ministry of Education Ministry of University and Research.
Conflict of Interest
The authors declare that the research was conducted in the absence of any commercial or financial relationships that could be construed as a potential conflict of interest.
References
1. Trinchieri G. Biology of Natural Killer Cells. Adv Immunol (1989) 47:187–376. doi: 10.1016/s0065-2776(08)60664-1
2. Lanier LL. NK Cell Recognition. Annu Rev Immunol (2005) 23:225–74. doi: 10.1146/annurev.immunol.23.021704.115526
3. Spits H, Di Santo JP. The Expanding Family of Innate Lymphoid Cells: Regulators and Effectors of Immunity and Tissue Remodeling. Nat Immunol (2011) 12:21–7. doi: 10.1038/ni.1962
4. Moretta L, Bottino C, Pende D, Castriconi R, Mingari MC, Moretta A. Surface NK Receptors and Their Ligands on Tumor Cells. Semin Immunol (2006) 18:151–8. doi: 10.1016/j.smim.2006.03.002
5. Moretta L, Ferlazzo G, Bottino C, Vitale M, Pende D, Mingari MC, et al. Effector and Regulatory Events During Natural Killer-Dendritic Cell Interactions. Immunol Rev (2006) 214:219–28. doi: 10.1111/j.1600-065X.2006.00450.x
6. Bryceson YT, March ME, Ljunggren H-G, Long EO. Activation, Coactivation, and Costimulation of Resting Human Natural Killer Cells. Immunol Rev (2006) 214:73–91. doi: 10.1111/j.1600-065X.2006.00457.x
7. Helander TS, Timonen T. Adhesion in NK Cell Function. Curr Top Microbiol Immunol (1998) 230:89–99. doi: 10.1007/978-3-642-46859-9_7
8. Long EO, Kim HS, Liu D, Peterson ME, Rajagopalan S. Controlling Natural Killer Cell Responses: Integration of Signals for Activation and Inhibition. Annu Rev Immunol (2013) 31:227–58. doi: 10.1146/annurev-immunol-020711-075005
9. Phatarpekar PV, Billadeau DD. Molecular Regulation of the Plasma Membrane-Proximal Cellular Steps Involved in NK Cell Cytolytic Function. J Cell Sci (2020) 133(5):jcs240424. doi: 10.1242/jcs.240424
10. Zhang X, Kim T-H, Thauland TJ, Li H, Majedi FS, Ly C, et al. Unraveling the Mechanobiology of Immune Cells. Curr Opin Biotechnol (2020) 66:236–45. doi: 10.1016/j.copbio.2020.09.004
11. Vogel V, Sheetz M. Local Force and Geometry Sensing Regulate Cell Functions. Nat Rev Mol Cell Biol (2006) 7:265–75. doi: 10.1038/nrm1890
12. Jaalouk DE, Lammerding J. Mechanotransduction Gone Awry. Nat Rev Mol Cell Biol (2009) 10:63–73. doi: 10.1038/nrm2597
13. Orange JS, Harris KE, Andzelm MM, Valter MM, Geha RS, Strominger JL. The Mature Activating Natural Killer Cell Immunologic Synapse Is Formed in Distinct Stages. Proc Natl Acad Sci USA (2003) 100:14151–6. doi: 10.1073/pnas.1835830100
14. Roda-Navarro P. Assembly and Function of the Natural Killer Cell Immune Synapse. Front Biosci (Landmark Ed) (2009) 14:621–33. doi: 10.2741/3268
15. Stabile H, Carlino C, Mazza C, Giliani S, Morrone S, Notarangelo LD, et al. Impaired NK-Cell Migration in WAS/XLT Patients: Role of Cdc42/WASp Pathway in the Control of Chemokine-Induced β2 Integrin High-Affinity State. Blood (2010) 115:2818–26. doi: 10.1182/blood-2009-07-235804
16. Swaney KF, Huang C-H, Devreotes PN. Eukaryotic Chemotaxis: A Network of Signaling Pathways Controls Motility, Directional Sensing, and Polarity. Annu Rev Biophys (2010) 39:265–89. doi: 10.1146/annurev.biophys.093008.131228
17. Feng Y, Brazin KN, Kobayashi E, Mallis RJ, Reinherz EL, Lang MJ. Mechanosensing Drives Acuity of αβ T-Cell Recognition. Proc Natl Acad Sci (2017) 114:E8204–13. doi: 10.1073/pnas.1703559114
18. Smith LA, Aranda-Espinoza H, Haun JB, Hammer DA. Interplay Between Shear Stress and Adhesion on Neutrophil Locomotion. Biophys J (2007) 92:632–40. doi: 10.1529/biophysj.105.079418
19. Dominguez GA, Anderson NR, Hammer DA. The Direction of Migration of T-lymphocytes Under Flow Depends Upon Which Adhesion Receptors are Engaged. Integr Biol (Camb) (2015) 7:345–55. doi: 10.1039/c4ib00201f
20. Sumagin R, Prizant H, Lomakina E, Waugh RE, Sarelius IH. LFA-1 and Mac-1 Define Characteristically Different Intralumenal Crawling and Emigration Patterns for Monocytes and Neutrophils In Situ. J Immunol (2010) 185:7057–66. doi: 10.4049/jimmunol.1001638
21. Valignat M-P, Theodoly O, Gucciardi A, Hogg N, Lellouch AC. T Lymphocytes Orient Against the Direction of Fluid Flow During LFA-1-Mediated Migration. Biophys J (2013) 104:322–31. doi: 10.1016/j.bpj.2012.12.007
22. Grönholm M, Jahan F, Bryushkova EA, Madhavan S, Aglialoro F, Soto Hinojosa L, et al. LFA-1 Integrin Antibodies Inhibit Leukocyte α4β1-Mediated Adhesion by Intracellular Signaling. Blood (2016) 128:1270–81. doi: 10.1182/blood-2016-03-705160
23. Schürpf T, Springer TA. Regulation of Integrin Affinity on Cell Surfaces. EMBO J (2011) 30:4712–27. doi: 10.1038/emboj.2011.333
24. Hoffman BD, Grashoff C, Schwartz MA. Dynamic Molecular Processes Mediate Cellular Mechanotransduction. Nature (2011) 475:316–23. doi: 10.1038/nature10316
25. del Rio A, Perez-Jimenez R, Liu R, Roca-Cusachs P, Fernandez JM, Sheetz MP. Stretching Single Talin Rod Molecules Activates Vinculin Binding. Science (2009) 323:638–41. doi: 10.1126/science.1162912
26. Sawada Y, Tamada M, Dubin-Thaler BJ, Cherniavskaya O, Sakai R, Tanaka S, et al. Force Sensing by Mechanical Extension of the Src Family Kinase Substrate p130Cas. Cell (2006) 127:1015–26. doi: 10.1016/j.cell.2006.09.044
27. Valignat M-P, Nègre P, Cadra S, Lellouch AC, Gallet F, Hénon S, et al. Lymphocytes Can Self-Steer Passively With Wind Vane Uropods. Nat Commun (2014) 5:5213. doi: 10.1038/ncomms6213
28. Robertson MJ. Role of Chemokines in the Biology of Natural Killer Cells. J Leukoc Biol (2002) 71:173–83.
29. Bernardini G, Antonangeli F, Bonanni V, Santoni A. Dysregulation of Chemokine/Chemokine Receptor Axes and NK Cell Tissue Localization During Diseases. Front Immunol (2016) 7:402. doi: 10.3389/fimmu.2016.00402
30. Lang P, Guizani L, Vitté-Mony I, Stancou R, Dorseuil O, Gacon G, et al. ADP-Ribosylation of the Ras-Related, GTP-Binding Protein RhoA Inhibits Lymphocyte-Mediated Cytotoxicity. J Biol Chem (1992) 267:11677–80. doi: 10.1016/S0021-9258(19)49747-0
31. Billadeau DD, Brumbaugh KM, Dick CJ, Schoon RA, Bustelo XR, Leibson PJ. The Vav–Rac1 Pathway in Cytotoxic Lymphocytes Regulates the Generation of Cell-mediated Killing. J Exp Med (1998) 188:549–59. doi: 10.1084/jem.188.3.549
32. Griffiths GM, Argon Y. Structure and Biogenesis of Lytic Granules. Curr Top Microbiol Immunol (1995) 198:39–58. doi: 10.1007/978-3-642-79414-8_3
33. Lang P, Gesbert F, Delespine-Carmagnat M, Stancou R, Pouchelet M, Bertoglio J. Protein Kinase A Phosphorylation of RhoA Mediates the Morphological and Functional Effects of Cyclic AMP in Cytotoxic Lymphocytes. EMBO J (1996) 15:510–9. doi: 10.1002/j.1460-2075.1996.tb00383.x
34. Ben-Shmuel A, Sabag B, Biber G, Barda-Saad M. The Role of the Cytoskeleton in Regulating the Natural Killer Cell Immune Response in Health and Disease: From Signaling Dynamics to Function. Front Cell Dev Biol (2021) 9:609532. doi: 10.3389/fcell.2021.609532
35. Chauveau A, Aucher A, Eissmann P, Vivier E, Davis DM. Membrane Nanotubes Facilitate Long-Distance Interactions Between Natural Killer Cells and Target Cells. Proc Natl Acad Sci (2010) 107:5545–50. doi: 10.1073/pnas.0910074107
36. Orange JS. Formation and Function of the Lytic NK-cell Immunological Synapse. Nat Rev Immunol (2008) 8:713–25. doi: 10.1038/nri2381
37. Steblyanko M, Anikeeva N, Campbell KS, Keen JH, Sykulev Y. Integrins Influence the Size and Dynamics of Signaling Microclusters in a Pyk2-Dependent Manner. J Biol Chem (2015) 290:11833–42. doi: 10.1074/jbc.M114.614719
38. Iizuka Y, Cichocki F, Sieben A, Sforza F, Karim R, Coughlin K, et al. Unc-45a Is a Nonmuscle Myosin IIA Chaperone Required for NK Cell Cytotoxicity Via Control of Lytic Granule Secretion. J Immunol (2015) 195:4760–70. doi: 10.4049/jimmunol.1500979
39. Mentlik AN, Sanborn KB, Holzbaur EL, Orange JS. Rapid Lytic Granule Convergence to the MTOC in Natural Killer Cells Is Dependent on Dynein But Not Cytolytic Commitment. Mol Biol Cell (2010) 21:2241–56. doi: 10.1091/mbc.e09-11-0930
40. Wood SM, Meeths M, Chiang SCC, Bechensteen AG, Boelens JJ, Heilmann C, et al. Different NK Cell–Activating Receptors Preferentially Recruit Rab27a or Munc13-4 to Perforin-Containing Granules for Cytotoxicity. Blood (2009) 114:4117–27. doi: 10.1182/blood-2009-06-225359
41. Kurowska M, Goudin N, Nehme NT, Court M, Garin J, Fischer A, et al. Terminal Transport of Lytic Granules to the Immune Synapse Is Mediated by the kinesin-1/Slp3/Rab27a Complex. Blood (2012) 119:3879–89. doi: 10.1182/blood-2011-09-382556
42. Elstak ED, Neeft M, Nehme NT, Voortman J, Cheung M, Goodarzifard M, et al. The munc13-4-rab27 Complex Is Specifically Required for Tethering Secretory Lysosomes at the Plasma Membrane. Blood (2011) 118:1570–8. doi: 10.1182/blood-2011-02-339523
43. Capuano C, Paolini R, Molfetta R, Frati L, Santoni A, Galandrini R. PIP2-Dependent Regulation of Munc13-4 Endocytic Recycling: Impact on the Cytolytic Secretory Pathway. Blood (2012) 119:2252–62. doi: 10.1182/blood-2010-12-324160
44. Fassett MS, Davis DM, Valter MM, Cohen GB, Strominger JL. Signaling at the Inhibitory Natural Killer Cell Immune Synapse Regulates Lipid Raft Polarization But Not Class I MHC Clustering. Proc Natl Acad Sci USA (2001) 98:14547–52. doi: 10.1073/pnas.211563598
45. Poggi A, Panzeri MC, Moretta L, Zocchi MR. CD31-Triggered Rearrangement of the Actin Cytoskeleton in Human Natural Killer Cells. Eur J Immunol (1996) 26:817–24. doi: 10.1002/eji.1830260414
46. Radošsević K, van Leeuwen AMT, Segers-Nolten IMJ, Figdor CG, de Grooth BG, Greve J. Changes in Actin Organization During the Cytotoxic Process. Cytometry (1994) 15:320–6. doi: 10.1002/cyto.990150407
47. Matalon O, Ben-Shmuel A, Kivelevitz J, Sabag B, Fried S, Joseph N, et al. Actin Retrograde Flow Controls Natural Killer Cell Response by Regulating the Conformation State of SHP-1. EMBO J (2018) 37(5):e96264. doi: 10.15252/embj.201696264
48. Hammer JA, Burkhardt JK. Controversy and Consensus Regarding Myosin II Function at the Immunological Synapse. Curr Opin Immunol (2013) 25:300–6. doi: 10.1016/j.coi.2013.03.010
49. Jankowska KI, Williamson EK, Roy NH, Blumenthal D, Chandra V, Baumgart T, et al. Integrins Modulate T Cell Receptor Signaling by Constraining Actin Flow at the Immunological Synapse. Front Immunol (2018) 9:25. doi: 10.3389/fimmu.2018.00025
50. Davis DM. Mechanisms and Functions for the Duration of Intercellular Contacts Made by Lymphocytes. Nat Rev Immunol (2009) 9:543–55. doi: 10.1038/nri2602
51. Yusa S, Campbell KS. Src Homology Region 2-Containing Protein Tyrosine Phosphatase-2 (Shp-2) Can Play a Direct Role in the Inhibitory Function of Killer Cell Ig-Like Receptors in Human Nk Cells. J Immunol (2003) 170:4539–47. doi: 10.4049/jimmunol.170.9.4539
52. Purdy AK, Campbell KS. Natural Killer Cells and Cancer: Regulation by the Killer Cell Ig-like Receptors (KIR). Cancer Biol Ther (2009) 8:2209–18. doi: 10.4161/cbt.8.23.10455
53. Xu F, Zhao R, Peng Y, Guerrah A, Zhao ZJ. Association of Tyrosine Phosphatase SHP-2 With F-Actin at Low Cell Densities. J Biol Chem (2001) 276:29479–84. doi: 10.1074/jbc.M104428200
54. Mace EM, Orange JS. Multiple Distinct NK-Cell Synapses. Blood (2011) 118:6475–6. doi: 10.1182/blood-2011-10-381392
55. Wells RG. Tissue Mechanics and Fibrosis. Biochim Biophys Acta - Mol Basis Dis (2013) 1832:884–90. doi: 10.1016/j.bbadis.2013.02.007
56. Friedman D, Simmonds P, Hale A, Bere L, Hodson NW, White MRH, et al. Natural Killer Cell Immune Synapse Formation and Cytotoxicity Are Controlled by Tension of the Target Interface. J Cell Sci (2021) 134:jcs.258570. doi: 10.1242/jcs.258570
57. Meza Guzman LG, Keating N, Nicholson SE. Natural Killer Cells: Tumor Surveillance and Signaling.. Cancers (Basel) (2020) 12(4):952. doi: 10.3390/cancers12040952
58. Zoeller RT, Vandenberg LN. Assessing Dose-Response Relationships for Endocrine Disrupting Chemicals (Edcs): A Focus on Non-Monotonicity. Environ Health (2015) 14:42. doi: 10.1186/s12940-015-0029-4
59. Kräter M, Sapudom J, Bilz N, Pompe T, Guck J, Claus C. Alterations in Cell Mechanics by Actin Cytoskeletal Changes Correlate With Strain-Specific Rubella Virus Phenotypes for Cell Migration and Induction of Apoptosis. Cells (2018) 7:136. doi: 10.3390/cells7090136
60. Le Saux G, Schvartzman M. Advanced Materials and Devices for the Regulation and Study of NK Cells. Int J Mol Sci (2019) 20:646. doi: 10.3390/ijms20030646
61. Le Saux G, Bar-Hanin N, Edri A, Hadad U, Porgador A, Schvartzman M. Nanoscale Mechanosensing of Natural Killer Cells is Revealed by Antigen-Functionalized Nanowires. Adv Mater (2019) 31:1805954. doi: 10.1002/adma.201805954
Keywords: mechanosensation, mechanotransduction, natural killer (NK) cells, immunological synapse, cytotoxicity
Citation: Santoni G, Amantini C, Santoni M, Maggi F, Morelli MB and Santoni A (2021) Mechanosensation and Mechanotransduction in Natural Killer Cells. Front. Immunol. 12:688918. doi: 10.3389/fimmu.2021.688918
Received: 31 March 2021; Accepted: 28 June 2021;
Published: 15 July 2021.
Edited by:
Shaik O. Rahaman, University of Maryland, College Park, United StatesReviewed by:
Yuri Sykulev, Thomas Jefferson University, United StatesMarit Inngjerdingen, University of Oslo, Norway
Copyright © 2021 Santoni, Amantini, Santoni, Maggi, Morelli and Santoni. This is an open-access article distributed under the terms of the Creative Commons Attribution License (CC BY). The use, distribution or reproduction in other forums is permitted, provided the original author(s) and the copyright owner(s) are credited and that the original publication in this journal is cited, in accordance with accepted academic practice. No use, distribution or reproduction is permitted which does not comply with these terms.
*Correspondence: Giorgio Santoni, giorgio.santoni@unicam.it