- Department of Nephrology, Zhongda Hospital, Southeast University School of Medicine, Nanjing, China
Kidney macrophages are central in kidney disease pathogenesis and have therapeutic potential in preventing tissue injury and fibrosis. Recent studies highlighted that kidney macrophages are notably heterogeneous immune cells that fulfill opposing functions such as clearing deposited pathogens, maintaining immune tolerance, initiating and regulating inflammatory responses, promoting kidney fibrosis, and degrading the extracellular matrix. Macrophage origins can partially explain macrophage heterogeneity in the kidneys. Circulating Ly6C+ monocytes are recruited to inflammatory sites by chemokines, while self-renewed kidney resident macrophages contribute to kidney repair and fibrosis. The proliferation of resident macrophages or infiltrating monocytes provides an alternative explanation of macrophage accumulation after kidney injury. In addition, dynamic Ly6C expression on infiltrating monocytes accompanies functional changes in handling kidney inflammation and fibrosis. Mechanisms underlying kidney macrophage heterogeneity, either by recruiting monocyte subpopulations, regulating macrophage polarization, or impacting distinctive macrophage functions, may help develop macrophage-targeted therapies for kidney diseases.
Introduction
Macrophage plays an important role in kidney disease pathogenesis and is a potential therapeutic target for kidney injury and fibrosis. Kidney macrophage subpopulations can either promote or prevent the extracellular matrix deposition in the kidney, drawing the possibility of reversing kidney fibrosis (1). However, the functionally opposing macrophage subpopulations rising ambivalence in understanding macrophage activities during kidney injury and fibrosis, disturbing the development of macrophage-targeted therapies (2). Studies have focused on macrophage functional diversities mechanisms and applied novel approaches in precisely identifying macrophage subpopulations. Therefore, intriguing questions have arisen, such as the macrophage origin (kidney-resident macrophages vs. circulating monocyte precursors), macrophage differentiation (oversimplified M1/M2 categorization vs. newly subsets defined by cell surface markers and single-cell RNA-sequence), and their effector functions in the pathogenesis of kidney diseases.
Macrophage Heterogeneity
Macrophage heterogeneity attracts attention since the discovery of macrophages. Following studies expand macrophage heterogeneity definition depending on the origin, cell surface markers, and cytokines secretion (3). Macrophages obtain distinct phenotypes under physiological conditions and differentiate into functional phenotypes in response to pathological stimulation. According to their cooperation with distinct T cell subsets, macrophages have generally been classified either into classical M1 or alternative M2 macrophages. M1 macrophages are characterized by pro-inflammatory effects and engage with T helper 1 (Th1) cells, whereas M2 macrophages exhibit immunoregulatory efforts and intimately cooperate with T helper 2 (Th2) cells (4). M1 macrophage differentiation is initiated by pathogen-associated molecular patterns (PAMPs), danger-associated molecular patterns (DAMPs), and pro-inflammatory cytokines, especially under acute deleterious conditions (5). Representative functions of M1 macrophages are host defense and secretion of pro-inflammatory cytokines, such as tumor necrosis factor-α (TNF-α), interleukine-1β (IL-1β), interleukine-6 (IL-6), and interleukine-12 (IL-12). M2 macrophage is typically polarized by interleukine-4 (IL-4) and interleukine-13 (IL-13), suppressing inflammation and promoting wound repair. Recent studies further classify M2 macrophages into different subsets depending on their differentiation stimuli, markers, and functions (6). While classic M2 macrophages are classified into M2a macrophages, M2b macrophages are induced by immune complexes, toll-like receptors and/or interleukine-1 receptors (IL-1R), contributing to immunoregulation and Th2 cells activation. M2c macrophages are induced by interleukine-10 (IL-10) and glucocorticoids, participating in immunosuppression, tissue repair, and matrix deposition. The simplified paradigm of M1/M2 macrophages facilitates the studies of macrophage phenotypes and functions. However, typical M1/M2 macrophages are induced in carefully regulated circumstances in vitro and not uniformly observed under the dynamic and complex environment in vivo. In fact, macrophages appear to express M1/M2 markers simultaneously during kidney injury, and their origins largely determine the functions of renal macrophages. Lineage tracing studies demonstrated that postnatal kidney macrophages predominately originate from the yolk sac EMP and hematopoietic stem cells, and bone marrow-derived monocytes infiltrate the kidney under inflammatory conditions (7, 8).
Renal Macrophage Distribution During Development, Health, and Disease
Macrophages contribute to morphogenesis during organ development. In cultured kidney explants, colony-stimulating factor-1 (CSF-1) application stimulates ureteric bud branching and nephron formation (9). By binding to the membrane receptor CSF1R, CSF-1 accelerates macrophage proliferation and differentiation (10, 11). Macrophages infiltrate the nephrogenic zone and facilitate nephron progenitor proliferation after the transient loss of nephron progenitor cells (12). Munro et al. (13) demonstrated that macrophages directly interacted with endothelium in developing cortical nephrogenic caliber vessels. These F4/80+CD206+ macrophages are perivascular and enriched for mRNAs associated with organogenesis. Moreover, the subpopulation of Gal3high myeloid cells are professional phagocytes and intermingle with pro-development F4/80+CD206+ macrophages in the developing mouse kidney. Therefore, fetal kidney macrophages possibly facilitate organogenesis by interacting with newly forming nephrogenic blood vessels. In addition, macrophages distribute around renal tubules during kidney development (9), but the underlying mechanisms are unclear. Experimental models of invertebrate species, such as Drosophila melanogaster (D. melanogaster), provide novel insights. In D. melanogaster, the Malpighian tubules are analogous to kidneys, while hemocytes are similar to macrophages. Hemocyte deposition around the developing Malpighian tubules is mediated by type IV collagen, necessary for the normal organogenesis of anterior Malpighian tubules (14). In mammalian kidneys, the renal tubular basement membrane is abundant in collagen IV and attracts macrophage recruitment (15, 16). However, it is difficult to extend these findings to mammalian kidneys due to the unique nephron structure.
In normal human kidneys, Marshall et al. (17) observed monocyte/macrophage distribution by immunoperoxidase staining of α-1-antitrypsin, muramidase, and serum 22. Most positive staining cells were scattered in glomerular capillaries and intertubular blood vessels. Macrophages rarely infiltrated into tubulointerstitium except in scarring tissues. However, the generality of these findings is limited by non-specific markers. Recently, Cao et al. (18) demonstrated that F4/80+CD11c− macrophages distribute throughout the renal cortex and medulla in healthy kidneys. In contrast, F4/80+CD11c+ mononuclear phagocytes are distributed in the renal cortex rather than the medulla under normal and injured conditions. These double-positive mononuclear phagocytes performed M1-like macrophage phenotype and aggravated kidney injury during Adriamycin nephropathy. However, a large percentage of CD11c+ dendritic cells co-express F4/80 marker in healthy kidneys, the preference to determine the nature of F4/80+CD11c+ cells should be circumspect.
Due to a double set of arterioles and capillaries, the kidney owns a unique vascular supply and receives about 25% of the cardiac output. The renal cortex exposed continuously to large amounts of blood-derived antigens and antibodies, resulting in a high sensitivity to renal glomerular diseases (19). Glomerular macrophage accumulation is an important feature in human glomerulonephritis. Macrophage clearance decreased glomerular damage in experimental glomerulonephritis (20). Further studies targeting monocyte chemotactic molecule-1 (MCP-1) or leukocyte adhesion molecules (ICAM) successfully attenuated macrophage accumulation and kidney injury in experimental models (21, 22). Interestingly, the numbers of tubulointerstitial macrophages rather than glomerular macrophages predict renal dysfunction (23, 24). Our previous studies found that tubular epithelial exosomes contribute to macrophage infiltration and activation, providing a novel insight into tubulointerstitial macrophages (25–27). This review focus on macrophage heterogeneity in the kidney and excellent works have been done to card macrophage function and distribution during acute kidney injury (AKI) and chronic kidney disease (CKD) (1, 28).
Kidney Resident Macrophage Origin and Specificity
Kidney-resident macrophages are in situ self-renewed and characterized by their phagocytic activities, expression of pattern recognition receptors (PRRs), and immunological regulation capacity, thus, maintaining kidney homeostasis (29). Kidney-resident macrophages derive from 3 sources: (1) yolk sac erythro-myeloid progenitors (EMP)-derived macrophages, (2) fetal liver EMP-derived macrophages, and (3) hematopoietic stem cells (HSC)-derived macrophages. The relative proportion of each progenitor dramatically changes during the development, adulthood, and damaged kidney state.
At embryonic day 12.5, kidney macrophages are CD45+ CD11blo F4/80hi Ly6C− cells deriving from yolk sac EMP; in contrast, CD45+ CD11bhi F4/80lo Ly6C+ monocytes are undetectable at this stage (30). Using tamoxifen-inducible Runx1Cre/EYFP and Csf1rCre/EYFP mice, fate tracing studies demonstrated that the relative proportion of yolk sac EMP-derived macrophages in the kidney decrease dramatically after embryonic day 13.5 (30). Consistently, Csf1r-Cre positive yolk sac-derived macrophages represent a minimal proportion of kidney macrophages after postnatal, possibly due to their dilution by the later arrival of fetal liver EMP-derived and HSC-derived macrophages (7). Sheng et al. (8) provided evidence of HSC-derived kidney macrophages using tamoxifen-inducible c-KitCre/EYFP mice. They further concluded that HSC precursors rather than EMPs are the source of kidney resident macrophages (8). However, the non-specific expression of c-Kit-Cre makes this conclusion debatable (31, 32). As HSCs transiently expressing Flt3-cre (33), Epelman et al. (7) distinguished the origin of HSC-derived monocytes and EMP-derived monocytes using Flt3Cre/GFP mice and found their equal contribution to the pool of resident macrophages. In contrast, Hoeffel et al. (30) demonstrated that fetal liver EMP-derived c-Myb+ monocytes are the predominant source of kidney resident macrophages. Thus, further studies based on fate-mapping studies must concern the limitations of genetic models, and single-cell RNA-sequence classifies ability worth more attention. While the kidney exposes to circulating monocytes throughout the development and adulthood, the kidney resident macrophages are mainly EMP- and HSC-derived rather than bone marrow-derived (7, 8, 30, 32, 34), partially explained by the niche competition hypothesis (35). Recent studies found that under certain types of kidney disease, expanded macrophages derive from the subset of resident macrophages, especially yolk sac-derived macrophages (36, 37). Ide et al. (37) demonstrated that CX3CR1+ yolk sac-derived macrophages have a higher proliferating capacity and progressively expand in number in older mice kidneys. Kidney resident macrophage proliferation contributes to the proangiogenic and pro-inflammatory environment after ischemic AKI and is confirmed by staining with Ki67 or BrdU (36, 38).
Kidney resident macrophages monitor trans-endothelial transport of circulating immune complexes and regulate the infiltration of lymphocytes and neutrophils (39). Using an unbiased flow cytometry approach, Kawakami et al. (40) classified kidney resident mononuclear phagocytes into five distinct subpopulations according to their cell surface markers, including CD11bhi CD11chi, CD11bhi CD11clo, CD11bint CD11cint, CD11blo CD11chi, and CD11b− CD11cint. CD11bint CD11cint F4/80high monocytes perform anti-inflammation effects as endogenous defenders. Kidney resident macrophages are in situ self-renewal and minimally differentiated from circulating monocytes after ischemic AKI. However, bone marrow-derived monocytes can replenish the kidney resident macrophages when they are depleted using polyinosinic/polycytidylic acid (poly I:C), consistent with the niche competition hypothesis (38). Interestingly, kidney resident macrophages lack major histocompatibility complex class II (MHCII) expression in the repair phase after AKI, a phenotype occurring during the nephrogenesis, and enrich Wnt ligands production, such as Axin2, Tcf4, and Jun (38). In ischemic AKI, C-C chemokine receptor type 2 (CCR2) deficiency alleviates circulating Ly6C+ macrophage recruitment and kidney injury and augments interstitial accumulation of Ly6C- embryonic yolk sac-derived resident macrophages and kidney fibrosis in late phases (41). Clodronate Liposome-induced macrophage depletion attenuates kidney injury and fibrosis, which can be restored by adoptive transfer of Ly6C− macrophages from injured wide type kidneys. While Ly6C− macrophage-derived cytokines facilitate the fibroblast-myofibroblast differentiation in vivo and in vitro, direct evidence targeting the trans-differentiation from Ly6C− macrophages to myofibroblasts remains missing (41). Similarly, CX3CR1+ resident renal phagocytes amplify leukocyte infiltration in an NLRP3-dependent manner in contrast-induced acute kidney injury (42). Accumulation of infiltrating and resident macrophages augments in autosomal dominant polycystic kidneys. In unilateral nephrectomy accelerated Pkd1 mice, lrf5 expression in resident macrophages aggravates cystic disease severity by producing pro-inflammatory cytokines (43). In ischemia-reperfusion injury (IRI) accelerated cystic mice, the phenotype of kidney resident macrophages transfers from F4/80high CD11Clow to F4/80high CD11Chigh, and reappearance of juvenile-like resident macrophages correlated with the accelerated cyst formation (44).
However, kidney resident macrophages also perform protective effects during acute and chronic kidney disease. Park et al. (45) found renal repair after ischemic AKI in mice lacking kidney resident CD45+ Ly6G− F4/80high CD11bint macrophages but containing infiltrating CD45+ Ly6G− F4/80int CD11bhigh macrophages is delayed compared to the wide type mice. V-domain Ig suppressor of T cell activation (VISTA), an inhibitory immune checkpoint molecule, is mainly expressed by CD45+ Ly6G− F4/80high CD11bint kidney resident macrophages and has the biomarker potential in distinguishing the renal macrophages (45). CX3CR1 mediated phagocytes by kidney resident macrophages initiate within the first hours during the innate host defense against Candidiasis, confirmed by CX3CR1-M280 associated susceptibility to systemic candidiasis in humans (46). CD11bint F4/80bright kidney resident macrophages protect renal artery stenosis-induced kidney injury by promoting the proangiogenic environments (36). Thus, kidney resident macrophages perform diverse effects depending on the phase and injury types. Based on a better understanding of cell surface markers and Cre specificity, further strategies should be explored to maintain protective resident macrophage phenotype during kidney disease. To advance these studies toward clinic interventions in patients, we must overcome two shortages of kidney resident macrophages. Firstly, minimal information is known about the anatomy and functions of resident macrophages in human kidney. Secondly, the similarities and differences of resident macrophages between rodent models and human kidneys remain unclear.
Monocyte as Precursors of Kidney Macrophages
While circulating monocytes minimally contribute to the renal macrophage pool under homeostasis, toxic or infectious damages result in augmented recruitment of monocyte-derived macrophages into the kidney. When renal macrophage niches are ablated, peripheral monocytes rapidly differentiate and replenish kidney macrophages (Munro et al. Nature Communications 11(1):2280 DOI:10.1038/s41467-020-16158-z). Bone marrow-derived monocyte precursors can reconstitute ischemic kidney macrophages in niches when kidney resident macrophages are depleted (38). Colony-stimulating factor-1 (CSF-1) stimulates macrophage proliferation at various time phases and tissues (47). Genetic deficiency or pharmacological blockade targeting CSF-1 inhibits macrophage proliferation, therefore prolonging the tissue repair phase after AKI (48). Rodent models revealed that Ly6Chigh pro-inflammatory monocytes infiltrate early in damaged kidneys (49, 50), depending on chemokines such as CCL2, CCL5, and CX3CR1 (26, 51–53). Initial infiltrating bone marrow-derived macrophages are characterized as Ly6C high iNOS+ cells by flow cytometry in rodent kidneys, whereas late Ly6Clow macrophages perform profibrotic M2-like effects (49, 50). CD11b diphtheria toxin receptor (DTR)-mediated depletion of monocyte/macrophages (50) or pharmacological blockade targeting chemokine pathways (54) attenuates kidney fibrosis, suggesting a profibrotic role of infiltrating macrophages in renal fibrogenesis. In severe IRI-induced AKI-CKD models, adoptive transplantation of F4/80int B7-H4high (M2c) macrophages rather than F4/80high B7-H4 int (M1) macrophages restore renal interstitial fibrosis in liposome clodronate-induced macrophage abrogated mice (55). Similarly, adoptive transfer of F4/80+ CD301+ (M2) macrophages rescue renal fibrosis in obstructed kidneys after macrophage depletion (56). Monocyte-derived kidney macrophages aggravate fibroblast activation and renal fibrosis by secreting cytokines (1, 57). Despite the direct and indirect profibrotic effects, bone marrow-derived macrophages can transdifferentiate into collagen-producing myofibroblasts via macrophage-myofibroblast transition (MMT) (58). Using Lyz2-Cre/Rosa26-Tomato mice, lineage tracing studies demonstrated that approximal 50% of the αSMA+ Collagen+ myofibroblasts derive from F4/80+ Tomato+ myeloid cells (59, 60). In contrast, a 2018 study challenges the MMT hypothesis as bone marrow-derived myofibroblasts make a limited contribution to the myofibroblasts in the obstructed kidney (61). The conflicting results come from the identification of myeloid cells by CD45+ and myofibroblasts by PDGFRβ+, as unspecific markers amplify the miscalculation. Another limitation is the deficiency of markers to distinguish bone marrow-derived fibroblasts from macrophage-derived myofibroblasts.
Moreover, macrophages perform diversified roles in renal fibrogenesis via secreting matrix metalloproteinases. Matrix metalloproteinases, especially macrophage-derived matrix metalloproteinase-9 (MMP-9), promote kidney fibrosis through stimulating extracellular matrix deposition (62, 63). In contrast, Twist1 in infiltrating macrophages promotes extracellular matrix degradation by stimulating CD11b+ Ly6Clow-derived matrix metalloproteinase-13 (MMP-13) production (64). As CD11b+ Ly6Chigh monocytes freshly infiltrate after kidney injury and represent the onset of renal inflammation, CD11b+ Ly6Cint and CD11b+ Ly6Clow populations expand in the phases of repair and fibrosis (41). The evidence that terminally differentiated macrophages rather than freshly infiltrating monocyte progenitors are the major players in kidney fibrogenesis suggests that kidney macrophages function varies due to disease types and time phases.
The Ly6Clow subpopulation of circulating monocytes is characterized by monitoring and phagocytosing circulating immune complexes (65). These Ly6Clow monocytes present antigens and activate effector CD4 + T lymphocytes in the glomerular capillaries (66). Circulating myeloid-derived suppressor cells (MDSCs) are immune suppressive populations and initially investigated in cancer (67). MDSCs were firstly reported to maintain cardiac transplant tolerance in rodent models (46), whereas renal MDSCs accumulation positively correlates with graft survival and kidney transplant recipients (68, 69). The effects of MDSCs further expand to immune-regulation and fibrogenesis during septic and metabolic kidney disease (70, 71), suggesting the therapeutic potential of MDSCs in acute and chronic kidney disease.
Heterogeneity of Human Kidney Macrophages and Clinical Transformation
Human monocyte/macrophages are classified into three distinct populations, including classical CD14++ CD16− subset, non-classical CD14+ CD16+ subset, and intermediate CD14++ CD16+ subset (72). In a 35 months cohort with 94 dialysis patients, the number of classical CD14++ CD16− monocytes can independently predict cardiovascular events and death (73). Rogacev et al. (74) demonstrated CD14++ CD16– monocytes numbers can also predict cardiovascular events in CKD patients. Non-classical CD14+ CD16+ monocytes from CKD patients express high levels of chemokines, facilitating their adhesion to vascular walls (75). Pro-inflammatory CD14+ CD16+ monocytes correlate with blood vessel stiffness in predialysis CKD patients, suggesting that non-classical CD14+ CD16+ subset damage endothelial cells (76). However, most clinical studies lack direct evidence of macrophage populations inside the kidney, and the predictive ability of human macrophage populations in kidney injury and fibrosis requires further investigations.
To expand experimental knowledge of macrophages to clinical applications, we still have to overcome several obstacles. Firstly, available data of human kidney macrophages is significantly insufficient, especially the dynamic changes of macrophage function and subpopulations. Secondly, CD14++CD16− and CD14++CD16+ monocytes resemble mouse Ly6C+ inflammatory monocytes, whereas CD14+CD16+ monocytes share phenotypic features with Ly6C- anti-inflammatory monocytes and adhering vascular endothelium (77). As classical CD14++ CD16– subset is known for the phagocytic capability, the overlapping and sometimes conflicting features of human and murine monocytes require further investigation. Thirdly, the functionality of kidney macrophages is dynamically variable and affected by the nature of kidney diseases. Thus, clinic translation must address the characteristics of kidney macrophages in different types and phases of the disease (Table 1).
Nevertheless, characterized macrophage recruitment and maturation pathways are also appliable in patients with kidney diseases. Renoprotective effects of interventions targeting CCL2/CCR2 have been confirmed in rodent models of kidney inflammation and fibrosis (26, 41). Consistently, CCR2 inhibitor CCX140-B further attenuates albuminuria levels in patients with type 2 diabetes in addition to the standard renin-angiotensin system (RAS) blocking therapies (84). Although the definite effects of CSF1R in macrophage recruitment and proliferation, the role of CSF1R inhibitors and neutralizing antibodies have not been tested in clinical trials to treat kidney diseases. The JAK-STAT pathway regulates macrophage activation and renal function decline in patients with type 2 diabetic nephropathy (85). JAK1 and JAK2 inhibition by Bariticinib decreases albuminuria levels in patients with diabetic kidney diseases (DKD) (86). Our recent studies found that macrophage-derived extracellular vesicles are kidney-targeted drug carriers and worth further expansion into clinical trials (87).
Single-Cell RNA-Sequencing Revolution
After the qualitative leap from the oversimplified M1/M2 paradigm to individual subpopulations identified by cell surface markers, single-cell RNA-sequencing (scRNA-seq) categorizes macrophages by their function and phenotype, revealing the continuum and complexity of macrophages during the development and pathogenesis of kidney disease (88, 89). Zimmerman et al. (80) identified C1q expression as a novel marker of resident macrophage clusters in mouse kidneys, and C1q expressing clusters in other species were verified by the expression of Cd74, Cd81, and Apoe. In a rodent model of rhabdomyolysis-induced AKI, F4/80low CD11bhigh Ly6bhigh CD206low pro-inflammatory macrophages infiltrate early after rhabdomyolysis, while F4/80high CD11b+ Ly6blow CD206high reparative macrophages are dominant at late phase. However, the scRNA-seq analysis demonstrated that signal pathways do not precisely match macrophage phenotypes and the existence of individual subpopulations simultaneously expressing heterogeneous markers (81). At the late phase after ischemic AKI, macrophages expressing Ccl2 and Ccr2 infiltrate around the Vcam1+ damaged tubules (79). By evaluating the typical C1qa, Cd74, and Adgre1 expression, macrophages are defined as the predominant immune cells in diabetic glomeruli and mainly M1-like macrophages (90). The scRNA-seq analysis revealed that both extent and levels of Axl expression increased in F4/80+ macrophages from rejecting allografts compared to tolerized kidneys; and Axl promotes the differentiation of intra-graft myeloid cells towards pro-inflammatory phenotypes after transplantation (82). Moreover, the combination of scRNA-seq and lineage tracing technique attracts particular attention. Lineage tracing can elucidate the clonal relationships during development and differentiation, enable lifecycle monitoring. In contrast, scRNA-seq can identify exact cell types but unable to determine the lineage relationships. Thus, integrating scRNA-seq and lineage tracing will provide extra information about cell types, development, and differentiation in a longstanding pattern.
Stewart et al. (78) demonstrated that human kidney monocyte phagocytes (MNPs) expressing ITGAX and HLA-DRA are categorized into four distinct clusters (MNPa to MNPd). MNPa subpopulation expressing CD14 in mature kidney analogous classical monocytes, whereas MNPb expressing CD16 is transcriptionally similar to non-classical monocytes. Consistently, CD14+ CD68+ monocyte/macrophages are the most abundant immune cells in urine and account for one-third of urinary clusters. These monocyte/macrophages are enriched in genes related to antigen presentation and macrophage activation and further classified into CD16+ and CD16- subpopulations (83).
Conclusions and Perspectives
Macrophages are recruited by chemokines and contribute to the pathogenesis of kidney injury, repair, and fibrosis. Despite the wide application of the oversimplified pro-inflammatory M1 and anti-inflammatory M2 macrophage paradigm, macrophage complexity in origin, phenotype, and function has attracted attention. Macrophage subpopulations were distinguished by combining cell surface markers and using novel single-cell RNA sequence technology to explore macrophage contribution in tissue injury, regeneration, and fibrosis. Our data and others have confirmed the therapeutic potential of macrophage pathways in acute and chronic kidney diseases; however, the functionally opposing macrophage subpopulations require incisive and tissue-specific strategies (Figure 1). Moreover, an in-depth understanding of the specialty and commonality in scRNA-seq defined macrophage clusters requires further investigation.
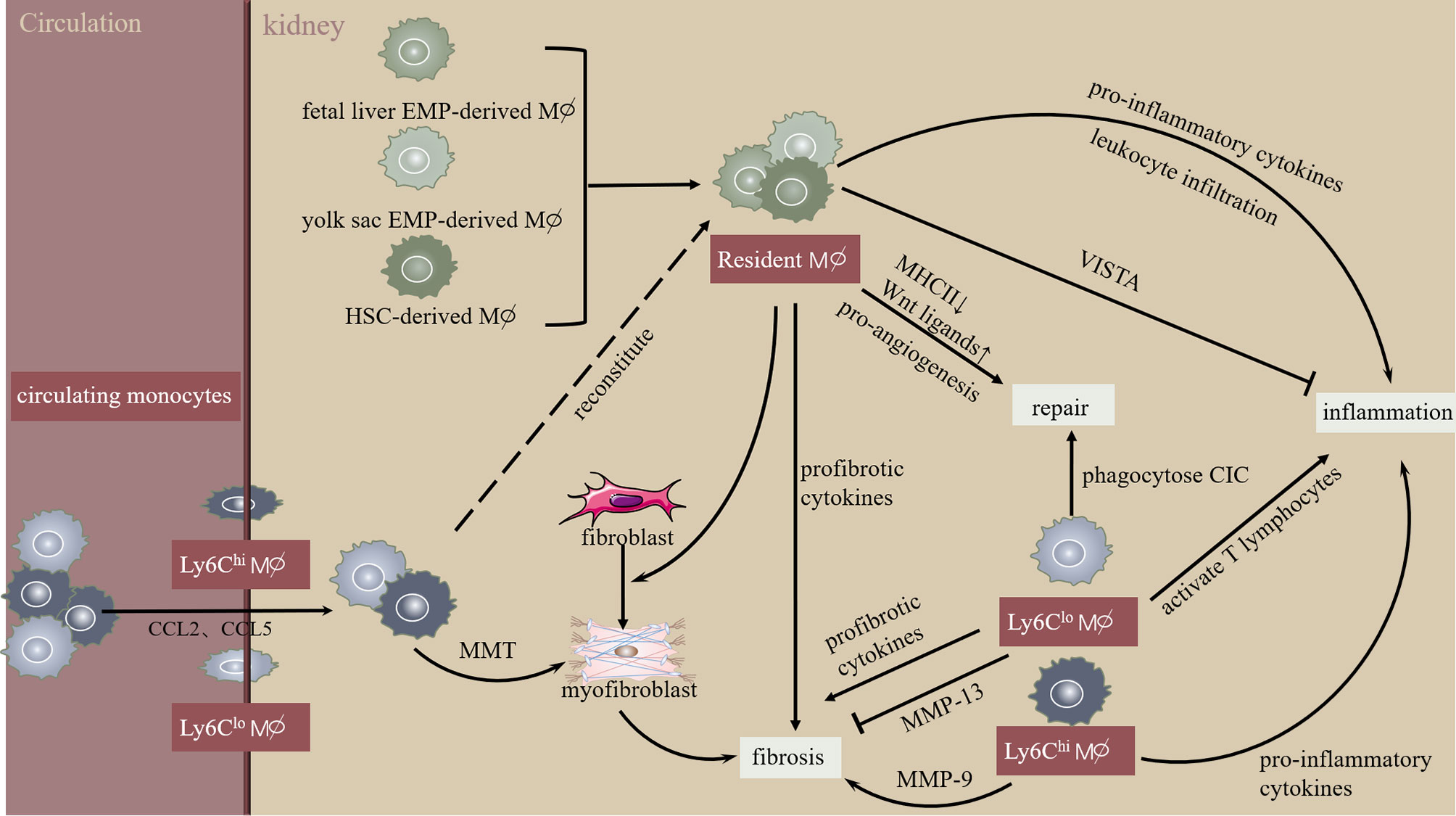
Figure 1 Macrophage heterogeneity during initiation and progression of kidney injury and fibrosis. Kidney resident macrophages derive from multi-sources and monitor trans-endothelial transport of circulating immune complexes. In the initial phase of kidney injury, resident macrophages stimulate leukocyte infiltration and cytokine secretion. Interestingly, kidney resident macrophages express V-domain Ig suppressor of T cell activation (VISTA), an inhibitory immune checkpoint molecule. Increased chemokines (CCL2, CCL5) promote circulating monocyte chemotaxis into the kidney, developing into infiltrating Ly-6Chi macrophages exhibiting pro-inflammatory phenotype or macrophage-myofibroblast transition (MMT). Similarly, infiltrating Ly6Clow macrophages promote kidney inflammation and fibrosis via activating T lymphocytes or pro-inflammatory cytokines. However, Ly6Chi macrophages inhibit kidney fibrosis by producing MMP-13. Overall, these mechanisms lead to extracellular matrix dynamic homeostasis during the resolution of kidney injury and fibrosis.
Author Contributions
YW and H-RY contributed equally to the writing of the manuscript. BW contributed to the figure of the manuscript. B-CL conceived the concept and contributed to the writing of the manuscript. All authors contributed to the article and approved the submitted version.
Funding
This work was supported by China National Natural Science Foundation (8203000544, 81720108007) and the key research project of the Ministry of China Science and Technology (2018YFC1314000) to B-CL as PI, and National Natural Science Foundation of China (81900623), and the Natural Science Foundation of Jiangsu Province (BK20190349) to YW as PI.
Conflict of Interest
The authors declare that the research was conducted in the absence of any commercial or financial relationships that could be construed as a potential conflict of interest.
References
1. Tang PM, Nikolic-Paterson DJ, Lan HY. Macrophages: Versatile Players in Renal Inflammation and Fibrosis. Nat Rev Nephrol (2019) 15(3):144–58. doi: 10.1038/s41581-019-0110-2
2. Wen Y, Crowley SD. The Varying Roles of Macrophages in Kidney Injury and Repair. Curr Opin Nephrol Hypertens (2020) 29(3):286–92. doi: 10.1097/MNH.0000000000000595
3. Cao Q, Wang Y, Harris DC. Macrophage Heterogeneity, Phenotypes, and Roles in Renal Fibrosis. Kidney Int Suppl (2011) (2014) 4(1):16–9. doi: 10.1038/kisup.2014.4
4. Lee S, Huen S, Nishio H, Nishio S, Lee HK, Choi BS, et al. Distinct Macrophage Phenotypes Contribute to Kidney Injury and Repair. J Am Soc Nephrol (2011) 22(2):317–26. doi: 10.1681/ASN.2009060615
5. Lv LL, Tang PM, Li CJ, You YK, Li J, Huang XR, et al. The Pattern Recognition Receptor, Mincle, is Essential for Maintaining the M1 Macrophage Phenotype in Acute Renal Inflammation. Kidney Int (2017) 91(3):587–602. doi: 10.1016/j.kint.2016.10.020
6. Chen T, Cao Q, Wang Y, Harris DCH. M2 Macrophages in Kidney Disease: Biology, Therapies, and Perspectives. Kidney Int (2019) 95(4):760–73. doi: 10.1016/j.kint.2018.10.041
7. Epelman S, Lavine KJ, Beaudin AE, Sojka DK, Carrero JA, Calderon B, et al. Embryonic and Adult-Derived Resident Cardiac Macrophages are Maintained Through Distinct Mechanisms at Steady State and During Inflammation. Immunity (2014) 40(1):91–104. doi: 10.1016/j.immuni.2013.11.019
8. Sheng J, Ruedl C, Karjalainen K. Most Tissue-Resident Macrophages Except Microglia Are Derived From Fetal Hematopoietic Stem Cells. Immunity (2015) 43(2):382–93. doi: 10.1016/j.immuni.2015.07.016
9. Rae F, Woods K, Sasmono T, Campanale N, Taylor D, Ovchinnikov DA, et al. Characterisation and Trophic Functions of Murine Embryonic Macrophages Based Upon the Use of a Csf1r-EGFP Transgene Reporter. Dev Biol (2007) 308(1):232–46. doi: 10.1016/j.ydbio.2007.05.027
10. Dai XM, Ryan GR, Hapel AJ, Dominguez MG, Russell RG, Kapp S, et al. Targeted Disruption of the Mouse Colony-Stimulating Factor 1 Receptor Gene Results in Osteopetrosis, Mononuclear Phagocyte Deficiency, Increased Primitive Progenitor Cell Frequencies, and Reproductive Defects. Blood (2002) 99(1):111–20. doi: 10.1182/blood.v99.1.111
11. Mouchemore KA, Pixley FJ. CSF-1 Signaling in Macrophages: Pleiotrophy Through Phosphotyrosine-Based Signaling Pathways. Crit Rev Clin Lab Sci (2012) 49(2):49–61. doi: 10.3109/10408363.2012.666845
12. Muthukrishnan SD, Ryzhov S, Karolak M, Mukherjee E, Sims-Lucas S, Oxburgh L. A Macrophage-Based Regenerative Response to Fetal Kidney Damage. Mech Dev (2017) 145:S50–0. doi: 10.1016/j.mod.2017.04.094
13. Munro DAD, Wineberg Y, Tarnick J, Vink CS, Li Z, Pridans C, et al. Macrophages Restrict the Nephrogenic Field and Promote Endothelial Connections During Kidney Development. Elife (2019) 8:e43271. doi: 10.7554/eLife.43271
14. Bunt S, Hooley C, Hu N, Scahill C, Weavers H, Skaer H. Hemocyte-Secreted Type IV Collagen Enhances BMP Signaling to Guide Renal Tubule Morphogenesis in Drosophila. Dev Cell (2010) 19(2):296–306. doi: 10.1016/j.devcel.2010.07.019
15. Schnoor M, Cullen P, Lorkowski J, Stolle K, Robenek H, Troyer D, et al. Production of Type VI Collagen by Human Macrophages: A New Dimension in Macrophage Functional Heterogeneity. J Immunol (2008) 180(8):5707–19. doi: 10.4049/jimmunol.180.8.5707
16. Abrahamson DR, Leardkamolkarn V. Development of Kidney Tubular Basement Membranes. Kidney Int (1991) 39(3):382–93. doi: 10.1038/ki.1991.50
17. Marshall RJ, MacIver AG. The Monocyte/Macrophage Population of the Normal Human Kidney. J Pathol (1984) 143(4):275–80. doi: 10.1002/path.1711430407
18. Cao Q, Wang Y, Wang XM, Lu J, Lee VW, Ye Q, et al. Renal F4/80+ CD11c+ Mononuclear Phagocytes Display Phenotypic and Functional Characteristics of Macrophages in Health and in Adriamycin Nephropathy. J Am Soc Nephrol (2015) 26(2):349–63. doi: 10.1681/ASN.2013121336
19. Couser WG. Basic and Translational Concepts of Immune-Mediated Glomerular Diseases. J Am Soc Nephrol (2012) 23(3):381–99. doi: 10.1681/ASN.2011030304
20. Duffield JS, Tipping PG, Kipari T, Cailhier JF, Clay S, Lang R, et al. Conditional Ablation of Macrophages Halts Progression of Crescentic Glomerulonephritis. Am J Pathol (2005) 167(5):1207–19. doi: 10.1016/S0002-9440(10)61209-6
21. Tesch GH, Schwarting A, Kinoshita K, Lan HY, Rollins BJ, Kelley VR. Monocyte Chemoattractant Protein-1 Promotes Macrophage-Mediated Tubular Injury, But Not Glomerular Injury, in Nephrotoxic Serum Nephritis. J Clin Invest (1999) 103(1):73–80. doi: 10.1172/JCI4876
22. Chow FY, Nikolic-Paterson DJ, Ozols E, Atkins RC, Tesch GH. Intercellular Adhesion Molecule-1 Deficiency is Protective Against Nephropathy in Type 2 Diabetic db/db Mice. J Am Soc Nephrol (2005) 16(6):1711–22. doi: 10.1681/ASN.2004070612
23. Silva GE, Costa RS, Ravinal RC, Ramalho LN, Reis MA, Moyses-Neto M, et al. Renal Macrophage Infiltration is Associated With a Poor Outcome in IgA Nephropathy. Clinics (Sao Paulo) (2012) 67(7):697–703. doi: 10.6061/clinics/2012(07)01
24. Liu D, Xu M, Ding LH, Lv LL, Liu H, Ma KL, et al. Activation of the Nlrp3 Inflammasome by Mitochondrial Reactive Oxygen Species: A Novel Mechanism of Albumin-Induced Tubulointerstitial Inflammation. Int J Biochem Cell Biol (2014) 57:7–19. doi: 10.1016/j.biocel.2014.09.018
25. Li ZL, Lv LL, Tang TT, Wang B, Feng Y, Zhou LT, et al. HIF-1alpha Inducing Exosomal microRNA-23a Expression Mediates the Cross-Talk Between Tubular Epithelial Cells and Macrophages in Tubulointerstitial Inflammation. Kidney Int (2019) 95(2):388–404. doi: 10.1016/j.kint.2018.09.013
26. Lv LL, Feng Y, Wen Y, Wu WJ, Ni HF, Li ZL, et al. Exosomal CCL2 From Tubular Epithelial Cells is Critical for Albumin-Induced Tubulointerstitial Inflammation. J Am Soc Nephrol (2018) 29(3):919–35. doi: 10.1681/ASN.2017050523
27. Lv LL, Feng Y, Wu M, Wang B, Li ZL, Zhong X, et al. Exosomal miRNA-19b-3p of Tubular Epithelial Cells Promotes M1 Macrophage Activation in Kidney Injury. Cell Death Differ (2020) 27(1):210–26. doi: 10.1038/s41418-019-0349-y
28. Cao Q, Harris DC, Wang Y. Macrophages in Kidney Injury, Inflammation, and Fibrosis. Physiol (Bethesda) (2015) 30(3):183–94. doi: 10.1152/physiol.00046.2014
29. Munro DAD, Hughes J. The Origins and Functions of Tissue-Resident Macrophages in Kidney Development. Front Physiol (2017) 8:837. doi: 10.3389/fphys.2017.00837
30. Hoeffel G, Chen J, Lavin Y, Low D, Almeida FF, See P, et al. C-Myb(+) Erythro-Myeloid Progenitor-Derived Fetal Monocytes Give Rise to Adult Tissue-Resident Macrophages. Immunity (2015) 42(4):665–78. doi: 10.1016/j.immuni.2015.03.011
31. Gomez Perdiguero E, Klapproth K, Schulz C, Busch K, Azzoni E, Crozet L, et al. Tissue-Resident Macrophages Originate From Yolk-Sac-Derived Erythro-Myeloid Progenitors. Nature (2015) 518(7540):547–51. doi: 10.1038/nature13989
32. Hoeffel G, Ginhoux F. Ontogeny of Tissue-Resident Macrophages. Front Immunol (2015) 6:486. doi: 10.3389/fimmu.2015.00486
33. Boyer SW, Schroeder AV, Smith-Berdan S, Forsberg EC. All Hematopoietic Cells Develop From Hematopoietic Stem Cells Through Flk2/Flt3-positive Progenitor Cells. Cell Stem Cell (2011) 9(1):64–73. doi: 10.1016/j.stem.2011.04.021
34. Epelman S, Lavine KJ, Randolph GJ. Origin and Functions of Tissue Macrophages. Immunity (2014) 41(1):21–35. doi: 10.1016/j.immuni.2014.06.013
35. Guilliams M, Scott CL. Does Niche Competition Determine the Origin of Tissue-Resident Macrophages? Nat Rev Immunol (2017) 17(7):451–60. doi: 10.1038/nri.2017.42
36. Puranik AS, Leaf IA, Jensen MA, Hedayat AF, Saad A, Kim KW, et al. Kidney-Resident Macrophages Promote a Proangiogenic Environment in the Normal and Chronically Ischemic Mouse Kidney. Sci Rep (2018) 8(1):13948. doi: 10.1038/s41598-018-31887-4
37. Ide S, Yahara Y, Kobayashi Y, Strausser SA, Ide K, Watwe A, et al. Yolk-Sac-Derived Macrophages Progressively Expand in the Mouse Kidney With Age. Elife (2020) 9:e51756. doi: 10.7554/eLife.51756
38. Lever JM, Hull TD, Boddu R, Pepin ME, Black LM, Adedoyin OO, et al. Resident Macrophages Reprogram Toward a Developmental State After Acute Kidney Injury. JCI Insight (2019) 4(2):e125503. doi: 10.1172/jci.insight.125503
39. Stamatiades EG, Tremblay ME, Bohm M, Crozet L, Bisht K, Kao D, et al. Immune Monitoring of Trans-endothelial Transport by Kidney-Resident Macrophages. Cell (2016) 166(4):991–1003. doi: 10.1016/j.cell.2016.06.058
40. Kawakami T, Lichtnekert J, Thompson LJ, Karna P, Bouabe H, Hohl TM, et al. Resident Renal Mononuclear Phagocytes Comprise Five Discrete Populations With Distinct Phenotypes and Functions. J Immunol (2013) 191(6):3358–72. doi: 10.4049/jimmunol.1300342
41. Yang Q, Wang Y, Pei G, Deng X, Jiang H, Wu J, et al. Bone Marrow-Derived Ly6C(-) Macrophages Promote Ischemia-Induced Chronic Kidney Disease. Cell Death Dis (2019) 10(4):291. doi: 10.1038/s41419-019-1531-3
42. Lau A, Chung H, Komada T, Platnich JM, Sandall CF, Choudhury SR, et al. Renal Immune Surveillance and Dipeptidase-1 Contribute to Contrast-Induced Acute Kidney Injury. J Clin Invest (2018) 128(7):2894–913. doi: 10.1172/JCI96640
43. Zimmerman KA, Huang J, He L, Revell DZ, Li Z, Hsu JS, et al. Interferon Regulatory Factor-5 in Resident Macrophage Promotes Polycystic Kidney Disease. Kidney360 (2020) 1(3):179–90. doi: 10.34067/KID.0001052019
44. Zimmerman KA, Song CJ, Li Z, Lever JM, Crossman DK, Rains A, et al. Tissue-Resident Macrophages Promote Renal Cystic Disease. J Am Soc Nephrol (2019) 30(10):1841–56. doi: 10.1681/ASN.2018080810
45. Park JG, Lee CR, Kim MG, Kim G, Shin HM, Jeon YH, et al. Kidney Residency of VISTA-positive Macrophages Accelerates Repair From Ischemic Injury. Kidney Int (2020) 97(5):980–94. doi: 10.1016/j.kint.2019.11.025
46. Lionakis MS, Swamydas M, Fischer BG, Plantinga TS, Johnson MD, Jaeger M, et al. CX3CR1-Dependent Renal Macrophage Survival Promotes Candida Control and Host Survival. J Clin Invest (2013) 123(12):5035–51. doi: 10.1172/JCI71307
47. Menke J, Rabacal WA, Byrne KT, Iwata Y, Schwartz MM, Stanley ER, et al. Circulating CSF-1 Promotes Monocyte and Macrophage Phenotypes That Enhance Lupus Nephritis. J Am Soc Nephrol (2009) 20(12):2581–92. doi: 10.1681/ASN.2009050499
48. Zhang MZ, Yao B, Yang S, Jiang L, Wang S, Fan X, et al. CSF-1 Signaling Mediates Recovery From Acute Kidney Injury. J Clin Invest (2012) 122(12):4519–32. doi: 10.1172/JCI60363
49. Menezes S, Melandri D, Anselmi G, Perchet T, Loschko J, Dubrot J, et al. The Heterogeneity of Ly6C(hi) Monocytes Controls Their Differentiation Into iNOS(+) Macrophages or Monocyte-Derived Dendritic Cells. Immunity (2016) 45(6):1205–18. doi: 10.1016/j.immuni.2016.12.001
50. Lin SL, Castano AP, Nowlin BT, Lupher ML Jr., Duffield JS. Bone Marrow Ly6Chigh Monocytes are Selectively Recruited to Injured Kidney and Differentiate Into Functionally Distinct Populations. J Immunol (2009) 183(10):6733–43. doi: 10.4049/jimmunol.0901473
51. Rudemiller NP, Patel MB, Zhang JD, Jeffs AD, Karlovich NS, Griffiths R, et al. C-C Motif Chemokine 5 Attenuates Angiotensin II-Dependent Kidney Injury by Limiting Renal Macrophage Infiltration. Am J Pathol (2016) 186(11):2846–56. doi: 10.1016/j.ajpath.2016.07.015
52. Cassini MF, Kakade VR, Kurtz E, Sulkowski P, Glazer P, Torres R, et al. Mcp1 Promotes Macrophage-Dependent Cyst Expansion in Autosomal Dominant Polycystic Kidney Disease. J Am Soc Nephrol (2018) 29(10):2471–81. doi: 10.1681/ASN.2018050518
53. Chousterman BG, Boissonnas A, Poupel L, Baudesson de Chanville C, Adam J, Tabibzadeh N, et al. Ly6Chigh Monocytes Protect Against Kidney Damage During Sepsis Via a CX3CR1-Dependent Adhesion Mechanism. J Am Soc Nephrol (2016) 27(3):792–803. doi: 10.1681/ASN.2015010009
54. McRae JL, Vikstrom IB, Bongoni AK, Salvaris EJ, Fisicaro N, Ng M, et al. Blockade of the G-CSF Receptor Is Protective in a Mouse Model of Renal Ischemia-Reperfusion Injury. J Immunol (2020) 205(5):1433–40. doi: 10.4049/jimmunol.2000390
55. Kim MG, Kim SC, Ko YS, Lee HY, Jo SK, Cho W. The Role of M2 Macrophages in the Progression of Chronic Kidney Disease Following Acute Kidney Injury. PloS One (2015) 10(12):e0143961. doi: 10.1371/journal.pone.0143961
56. Shen B, Liu X, Fan Y, Qiu J. Macrophages Regulate Renal Fibrosis Through Modulating TGFbeta Superfamily Signaling. Inflammation (2014) 37(6):2076–84. doi: 10.1007/s10753-014-9941-y
57. Wen Y, Lu X, Ren J, Privratsky JR, Yang B, Rudemiller NP, et al. KLF4 in Macrophages Attenuates TNFalpha-Mediated Kidney Injury and Fibrosis. J Am Soc Nephrol (2019) 30(10):1925–38. doi: 10.1681/ASN.2019020111
58. Phua YL, Martel N, Pennisi DJ, Little MH, Wilkinson L. Distinct Sites of Renal Fibrosis in Crim1 Mutant Mice Arise From Multiple Cellular Origins. J Pathol (2013) 229(5):685–96. doi: 10.1002/path.4155
59. Wang YY, Jiang H, Pan J, Huang XR, Wang YC, Huang HF, et al. Macrophage-to-Myofibroblast Transition Contributes to Interstitial Fibrosis in Chronic Renal Allograft Injury. J Am Soc Nephrol (2017) 28(7):2053–67. doi: 10.1681/ASN.2016050573
60. Meng XM, Wang S, Huang XR, Yang C, Xiao J, Zhang Y, et al. Inflammatory Macrophages can Transdifferentiate Into Myofibroblasts During Renal Fibrosis. Cell Death Dis (2016) 7(12):e2495. doi: 10.1038/cddis.2016.402
61. Kramann R, Machado F, Wu H, Kusaba T, Hoeft K, Schneider RK, et al. Parabiosis and Single-Cell RNA Sequencing Reveal a Limited Contribution of Monocytes to Myofibroblasts in Kidney Fibrosis. JCI Insight (2018) 3(9):e99561. doi: 10.1172/jci.insight.99561
62. Tan TK, Zheng G, Hsu TT, Lee SR, Zhang J, Zhao Y, et al. Matrix Metalloproteinase-9 of Tubular and Macrophage Origin Contributes to the Pathogenesis of Renal Fibrosis Via Macrophage Recruitment Through Osteopontin Cleavage. Lab Invest (2013) 93(4):434–49. doi: 10.1038/labinvest.2013.3
63. Wang X, Zhou Y, Tan R, Xiong M, He W, Fang L, et al. Mice Lacking the Matrix Metalloproteinase-9 Gene Reduce Renal Interstitial Fibrosis in Obstructive Nephropathy. Am J Physiol Renal Physiol (2010) 299(5):F973–982. doi: 10.1152/ajprenal.00216.2010
64. Ren J, Zhang J, Rudemiller NP, Griffiths R, Wen Y, Lu X, et al. Twist1 in Infiltrating Macrophages Attenuates Kidney Fibrosis Via Matrix Metallopeptidase 13-Mediated Matrix Degradation. J Am Soc Nephrol (2019) 30(9):1674–85. doi: 10.1681/ASN.2018121253
65. Carlin LM, Stamatiades EG, Auffray C, Hanna RN, Glover L, Vizcay-Barrena G, et al. Nr4a1-Dependent Ly6C(low) Monocytes Monitor Endothelial Cells and Orchestrate Their Disposal. Cell (2013) 153(2):362–75. doi: 10.1016/j.cell.2013.03.010
66. Westhorpe CLV, Norman MU, Hall P, Snelgrove SL, Finsterbusch M, Li A, et al. Effector CD4(+) T Cells Recognize Intravascular Antigen Presented by Patrolling Monocytes. Nat Commun (2018) 9(1):747. doi: 10.1038/s41467-018-03181-4
67. Iglesias-Escudero M, Sansegundo-Arribas D, Riquelme P, Merino-Fernandez D, Guiral-Foz S, Perez C, et al. Myeloid-Derived Suppressor Cells in Kidney Transplant Recipients and the Effect of Maintenance Immunotherapy. Front Immunol (2020) 11:643. doi: 10.3389/fimmu.2020.00643
68. Pengam S, Durand J, Usal C, Gauttier V, Dilek N, Martinet B, et al. SIRPalpha/CD47 Axis Controls the Maintenance of Transplant Tolerance Sustained by Myeloid-Derived Suppressor Cells. Am J Transplant (2019) 19(12):3263–75. doi: 10.1111/ajt.15497
69. Du XX, Guo YL, Zhao YP, Yang M, Chang S, Liu B, et al. Accumulation of High Levels of Monocytic Myeloid-Derived Suppressor Cells Enhances Graft Survival in Almost-Tolerant Kidney Transplant Recipients. Transplant Proc (2018) 50(10):3314–20. doi: 10.1016/j.transproceed.2018.04.043
70. Venet F, Monneret G. Advances in the Understanding and Treatment of Sepsis-Induced Immunosuppression. Nat Rev Nephrol (2018) 14(2):121–37. doi: 10.1038/nrneph.2017.165
71. Islam J, Lee HJ, Yang SH, Kim DK, Joo KW, Kim YS, et al. Expansion of Myeloid-Derived Suppressor Cells Correlates With Renal Progression in Type 2 Diabetic Nephropathy. Immune Netw (2020) 20(2):e18. doi: 10.4110/in.2020.20.e18
72. Kapellos TS, Bonaguro L, Gemund I, Reusch N, Saglam A, Hinkley ER, et al. Human Monocyte Subsets and Phenotypes in Major Chronic Inflammatory Diseases. Front Immunol (2019) 10:2035. doi: 10.3389/fimmu.2019.02035
73. Heine GH, Ulrich C, Seibert E, Seiler S, Marell J, Reichart B, et al. CD14(++)CD16+ Monocytes But Not Total Monocyte Numbers Predict Cardiovascular Events in Dialysis Patients. Kidney Int (2008) 73(5):622–9. doi: 10.1038/sj.ki.5002744
74. Rogacev KS, Seiler S, Zawada AM, Reichart B, Herath E, Roth D, et al. CD14++CD16+ Monocytes and Cardiovascular Outcome in Patients With Chronic Kidney Disease. Eur Heart J (2011) 32(1):84–92. doi: 10.1093/eurheartj/ehq371
75. Ramirez R, Carracedo J, Merino A, Soriano S, Ojeda R, Alvarez-Lara MA, et al. CD14+CD16+ Monocytes From Chronic Kidney Disease Patients Exhibit Increased Adhesion Ability to Endothelial Cells. Contrib Nephrol (2011) 171:57–61. doi: 10.1159/000327134
76. Lee JW, Cho E, Kim MG, Jo SK, Cho WY, Kim HK. Proinflammatory CD14(+)CD16(+) Monocytes are Associated With Vascular Stiffness in Predialysis Patients With Chronic Kidney Disease. Kidney Res Clin Pract (2013) 32(4):147–52. doi: 10.1016/j.krcp.2013.08.001
77. Geissmann F, Jung S, Littman DR. Blood Monocytes Consist of Two Principal Subsets With Distinct Migratory Properties. Immunity (2003) 19(1):71–82. doi: 10.1016/s1074-7613(03)00174-2
78. Stewart BJ, Ferdinand JR, Young MD, Mitchell TJ, Loudon KW, Riding AM, et al. Spatiotemporal Immune Zonation of the Human Kidney. Science (2019) 365(6460):1461–6. doi: 10.1126/science.aat5031
79. Kirita Y, Wu H, Uchimura K, Wilson PC, Humphreys BD. Cell Profiling of Mouse Acute Kidney Injury Reveals Conserved Cellular Responses to Injury. Proc Natl Acad Sci USA (2020) 117(27):15874–83. doi: 10.1073/pnas.2005477117
80. Zimmerman KA, Bentley MR, Lever JM, Li Z, Crossman DK, Song CJ, et al. Single-Cell RNA Sequencing Identifies Candidate Renal Resident Macrophage Gene Expression Signatures Across Species. J Am Soc Nephrol (2019) 30(5):767–81. doi: 10.1681/ASN.2018090931
81. Belliere J, Casemayou A, Ducasse L, Zakaroff-Girard A, Martins F, Iacovoni JS, et al. Specific Macrophage Subtypes Influence the Progression of Rhabdomyolysis-Induced Kidney Injury. J Am Soc Nephrol (2015) 26(6):1363–77. doi: 10.1681/ASN.2014040320
82. Dangi A, Natesh NR, Husain I, Ji Z, Barisoni L, Kwun J, et al. Single Cell Transcriptomics of Mouse Kidney Transplants Reveals a Myeloid Cell Pathway for Transplant Rejection. JCI Insight (2020) 5(20):e141321. doi: 10.1172/jci.insight.141321
83. Wang Y, Zhao Y, Zhao Z, Li D, Nie H, Sun Y, et al. Single-Cell RNA-Seq Analysis Identified Kidney Progenitor Cells From Human Urine. Protein Cell (2021) 12(4):305–12. doi: 10.1007/s13238-020-00816-5
84. de Zeeuw D, Bekker P, Henkel E, Hasslacher C, Gouni-Berthold I, Mehling H, et al. The Effect of CCR2 Inhibitor CCX140-B on Residual Albuminuria in Patients With Type 2 Diabetes and Nephropathy: A Randomised Trial. Lancet Diabetes Endocrinol (2015) 3(9):687–96. doi: 10.1016/S2213-8587(15)00261-2
85. Berthier CC, Zhang H, Schin M, Henger A, Nelson RG, Yee B, et al. Enhanced Expression of Janus Kinase-Signal Transducer and Activator of Transcription Pathway Members in Human Diabetic Nephropathy. Diabetes (2008) 58(2):469–77. doi: 10.2337/db08-1328
86. Tuttle KR, Brosius FC 3rd, Adler SG, Kretzler M, Mehta RL, Tumlin JA, et al. JAK1/JAK2 Inhibition by Baricitinib in Diabetic Kidney Disease: Results From a Phase 2 Randomized Controlled Clinical Trial. Nephrol Dial Transplant (2018) 33(11):1950–9. doi: 10.1093/ndt/gfx377
87. Tang TT, Lv LL, Wang B, Cao JY, Feng Y, Li ZL, et al. Employing Macrophage-Derived Microvesicle for Kidney-Targeted Delivery of Dexamethasone: An Efficient Therapeutic Strategy Against Renal Inflammation and Fibrosis. Theranostics (2019) 9(16):4740–55. doi: 10.7150/thno.33520
88. Potter SS. Single-Cell RNA Sequencing for the Study of Development, Physiology and Disease. Nat Rev Nephrol (2018) 14(8):479–92. doi: 10.1038/s41581-018-0021-7
89. Clatworthy MR. How to Find a Resident Kidney Macrophage: The Single-Cell Sequencing Solution. J Am Soc Nephrol (2019) 30(5):715–6. doi: 10.1681/ASN.2019030245
Keywords: macrophage heterogeneity, resident macrophage, kidney, inflammation, fibrosis
Citation: Wen Y, Yan H-R, Wang B and Liu B-C (2021) Macrophage Heterogeneity in Kidney Injury and Fibrosis. Front. Immunol. 12:681748. doi: 10.3389/fimmu.2021.681748
Received: 17 March 2021; Accepted: 07 May 2021;
Published: 20 May 2021.
Edited by:
Cheng Yang, Fudan University, ChinaReviewed by:
Guillaume Hoeffel, U1104 Centre d’immunologie de Marseille-Luminy (CIML) (INSERM), FranceYinghong Hu, Emory University, United States
Copyright © 2021 Wen, Yan, Wang and Liu. This is an open-access article distributed under the terms of the Creative Commons Attribution License (CC BY). The use, distribution or reproduction in other forums is permitted, provided the original author(s) and the copyright owner(s) are credited and that the original publication in this journal is cited, in accordance with accepted academic practice. No use, distribution or reproduction is permitted which does not comply with these terms.
*Correspondence: Bi-Cheng Liu, liubc64@163.com
†These authors have contributed equally to this work