- 1Programa de Pós-Graduação em Genética, Universidade Federal do Paraná, Curitiba, Brazil
- 2Department of Neurology, University of California, San Francisco, CA, United States
- 3Department of Structural Biology, Stanford University, Palo Alto, CA, United States
- 4Histocompatibility & Immunogenetics Laboratory, Stanford Blood Center, Palo Alto, CA, United States
- 5Division of Biomedical Informatics and Personalized Medicine, University of Colorado, Denver, CO, United States
The KIR (killer-cell immunoglobulin-like receptor) region is characterized by structural variation and high sequence similarity among genes, imposing technical difficulties for analysis. We undertook the most comprehensive study to date of KIR genetic diversity in a large population sample, applying next-generation sequencing in 2,130 United States European-descendant individuals. Data were analyzed using our custom bioinformatics pipeline specifically designed to address technical obstacles in determining KIR genotypes. Precise gene copy number determination allowed us to identify a set of uncommon gene-content KIR haplotypes accounting for 5.2% of structural variation. In this cohort, KIR2DL4 is the framework gene that most varies in copy number (6.5% of all individuals). We identified phased high-resolution alleles in large multi-locus insertions and also likely founder haplotypes from which they were deleted. Additionally, we observed 250 alleles at 5-digit resolution, of which 90 have frequencies ≥1%. We found sequence patterns that were consistent with the presence of novel alleles in 398 (18.7%) individuals and contextualized multiple orphan dbSNPs within the KIR complex. We also identified a novel KIR2DL1 variant, Pro151Arg, and demonstrated by molecular dynamics that this substitution is predicted to affect interaction with HLA-C. No previous studies have fully explored the full range of structural and sequence variation of KIR as we present here. We demonstrate that pairing high-throughput sequencing with state-of-art computational tools in a large cohort permits exploration of all aspects of KIR variation including determination of population-level haplotype diversity, improving understanding of the KIR system, and providing an important reference for future studies.
Introduction
A variety of membrane-bound receptors control the response of natural killer cells (NK) to infected or malignant cells (1, 2). The killer-cell immunoglobulin-like receptors (KIR) are the most polymorphic family of NK receptors and encoded by a gene family located at chromosomal region 19q13.4 (3, 4). The KIR genes exhibit extraordinary variation, both within populations and between them (5–7). Although KIR gene-content has been extensively studied in numerous populations worldwide (8–11), less is known about KIR allele diversity. The unusual structural variation of the KIR region, coupled with the numerous alleles for each KIR gene (12) and extensive sequence similarity within the KIR gene family are distinguishing characteristics of KIR variation. Further characterizing the KIR region are frequent duplications, large deletions, hybrid genes and recombinant alleles (13–17). Together, these obstacles have impeded high-resolution allelic characterization of all KIR genes in population studies, which has been accomplished in only a few studies (18–21).
Transduction of NK cell activating and inhibitory signals is achieved by a subset of human leukocyte antigen (HLA) class I molecules, which serve as KIR ligands (22–24). These two interacting molecule families evolve as a unique and integrated system (25–28), and combinations of KIR and HLA have been associated with numerous diseases (29–32), including autoimmune disorders (33–36), malignancies (37–39) and infections (40–43). Combinations of KIR and HLA class I also affect placentation and the success of reproduction (44–47). Therefore, high-resolution allelic analysis of KIR and HLA class I diversity across populations will be necessary to understand their evolution and lay a foundation for functional studies to determine disease mechanisms. To facilitate this progression, we have used our custom KIR genotyping and bioinformatics pipeline to interrogate KIR diversity in a sample of 2,130 US residents. These methods were explicitly designed to cope with the complexities of KIR alleles, gene and haplotypes. We describe the most comprehensive analysis to date of the KIR genes, exploring copy number variation, haplotype patterns, and novel variation not previously reported.
Material and Methods
Study Population
We analyzed a cohort of 2,130 unrelated healthy adult individuals previously described by Hollenbach et al., 2019 (48). All individuals self-identified as being of European descent and were resident of the United States.
KIR Genotyping
DNA samples were sequenced for all KIR genes, according to Norman and collaborators (49). After sequencing, raw fastq files were analyzed using our custom bioinformatics pipeline PING (Pushing Immunogenetics into the Next Generation) to obtain KIR gene content and allelic genotypes from next-generation sequencing (NGS) data (49). We applied an updated version of the pipeline that precisely determines the copy number of each locus through multiple alignment and filtration steps, also accurately identifying KIR genotypes. The updated pipeline increased the accuracy of KIR genotype determination and is publicly available (50).
Haplotype Estimation
Gene-content haplotypes were identified manually, based on the precise copy number determination, the known linkage disequilibrium among KIR genes, and allelic information. Uncommon haplotypes were identified based on previous observations (13, 51, 52). Candidates for novel gene-content haplotypes were identified when paired with common haplotypes and observed in two or more individuals. After identifying gene-content haplotypes, we inferred the haplotypes of their KIR alleles using the expectation-maximization (EM) algorithm and the R package haplo.stats (http://CRAN.R-project.org/package=haplo.stats).
Linkage Disequilibrium (LD) Analysis
Allelic genotyping data were transformed into an Arlequin entry file (.arp) using GenAlEx 6.5 (53). Gametic phase estimation using an EM algorithm and further pairwise linkage disequilibrium analysis were performed using Arlequin 3.5.2.2 (54).
Identification of Novel Alleles
We searched for the single nucleotide variants (SNV) in KIR2DL1 and KIR3DL1S1 that were identified by our software but not present in any allele listed at the Immuno Polymorphism Database (IPD)-KIR release 2.9.0 (12). For individuals carrying a candidate novel SNV in KIR2DL1 or KIR3DL1S1, the respective genes were re-sequenced using the Sanger method (55) using previously described primers (25, 56).
Simulations of Molecular Dynamics
In silico, the KIR2DL1 chain was isolated from the KIR-HLA complex (PDB ID: 1IM9) (57). To map the rs200879366 variation on the KIR2DL1 structure, the proline at position 151 was replaced by arginine using the Mutate plugin in Visual Molecular Dynamics (VMD) package (58). Both allotypes were solvated in separate simulation boxes using TIP3P solvent, and the ion concentration was adjusted to 150 KCl. Energy minimization was then performed on both systems for 150,000 steps. To mimic the anchorage of KIR2DL1 to the lipid membrane, the atom of residue 200 was fixed in space. Conformational transitions of KIR2DL1 allotypes were modeled using the NAMD software package (59) and the CHARMM36 forcefield (60) in NPT ensembles. Temperature and pressure were maintained at 310 K and 1 bar using the Langevin thermostat and Langevin piston Nose-Hoover, respectively. Periodic boundary condition in all directions and a timestep of 2fs were used. Simulations on both systems ran for 100ns. The angle between the D1 and D2 domains is the leading indicator of KIR2DL1 conformational transition. It was obtained by aligning the corresponding atom selections and calculating rotation and displacement at every timeframe using in-house tcl scripts. Structure visualizations were performed using VMD.
Results
KIR Gene Copy Number Analysis Identified Numerous Deletions and Duplications Involving KIR3DP1, KIR2DL4, and KIR3DL1S1
We determined KIR gene copy number for all 13 KIR genes (KIR2DL1, KIR2DL23, KIR2DL4, KIR2DL5A, KIR2DL5B, KIR2DS1, KIR2DS2, KIR2DS3, KIR2DS4, KIR2DS5, KIR3DL1S1, KIR3DL2, KIR3DL3) and the two pseudogenes (KIR2DP1, and KIR3DP1). Carrier frequencies and gene frequencies, based on the direct counting of all copies for each KIR, are given in Supplementary Table 1.
The KIR A haplotype is defined by the presence of only one gene encoding a short-tailed activating receptor (KIR2DS4) and a fixed number of genes encoding inhibitory KIR. In contrast, KIR B haplotypes encode various combinations of activating and inhibiting receptors (61). A total of 31.4% of individuals in the cohort of 2,130 Europeans are homozygous for the full-length KIR A haplotype (cA01~tA01/cA01~tA01, f = 0.58). The centromeric and telomeric portions of the KIR haplotype are flanked by the framework genes KIR3DL3-KIR3DP1 and KIR2DL4-KIR3DL2, respectively (62, 63). On analyzing these two regions separately, we observed that 94% of the centromeric diversity is explained by just three gene-content haplotypes (cA01, cB02, and cB01; Figure 1A), whereas tA01 and tB01 correspond to 93% of the telomeric haplotypes (Figure 1B). We also indentified the presence of two novel centromeric haplotypes, and two telomeric haplotypes not previoulsy described in Europeans. The novel cB06 haplotype differs from cB01 by lacking KIR2DP1, and cA03 differs from the more common cA01 by lacking KIR3DP1. Present in the telomeric region is tA02, which only differs from tA01 by lacking KIR2DS4. Of particular interest is a haplotype observed in two individuals that has only the KIR3DL2 framework gene in the telomeric region. The gene content and organization of 3.5% of the centromeric and 4.9% of the telomeric haplotypes could not be determined.
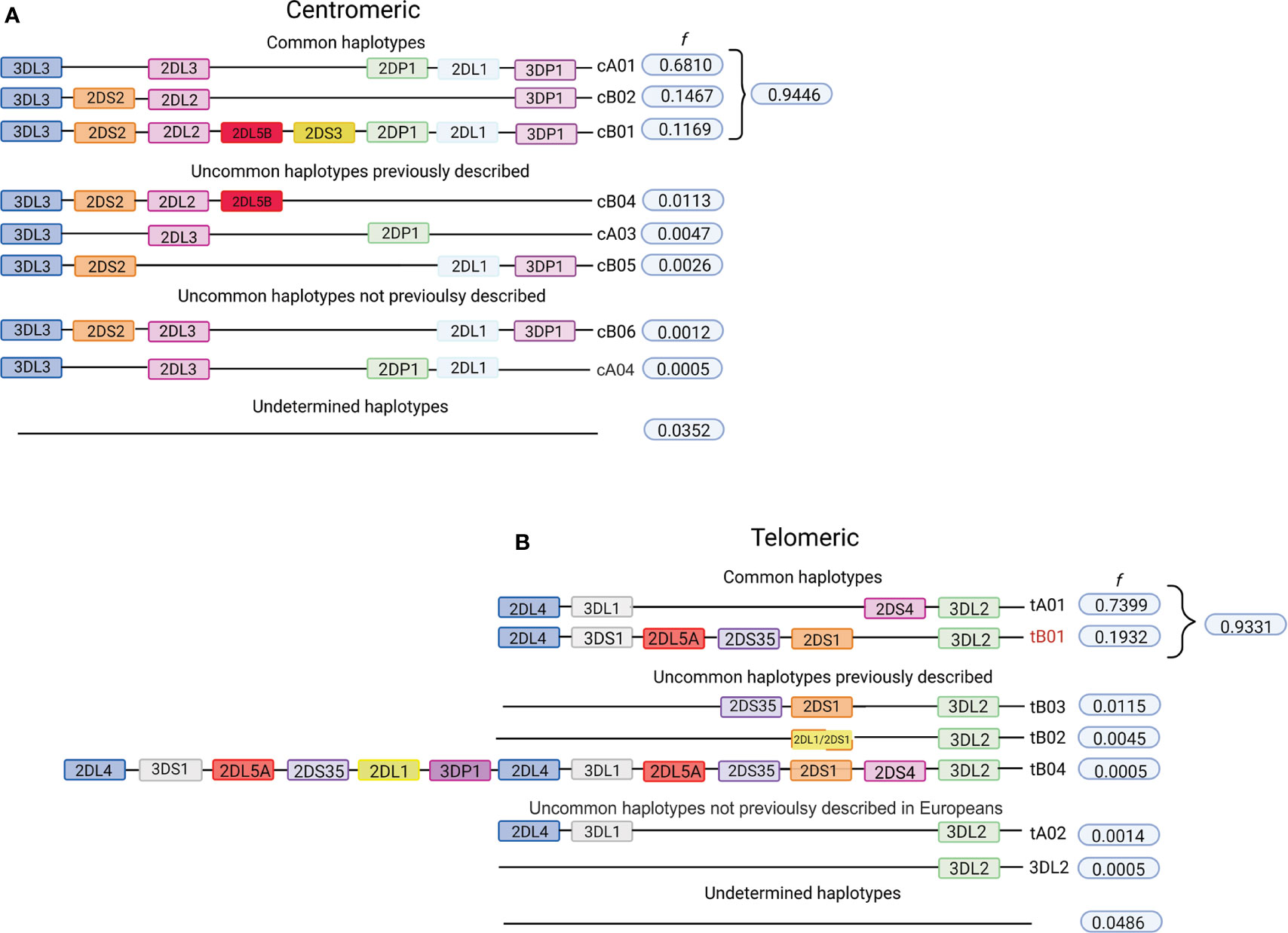
Figure 1 Telomeric and centromeric gene-content haplotypes in European-Americans. Although multiple variations of the KIR full-length haplotypes have been described, most are multiple variations of a few centromeric and telomeric haplotypes. The centromeric and telomeric regions of KIR haplotypes are flanked by the genes KIR3DL3-KIR3DP1 and KIR2DL4-KIR3DL2, respectively, which are referred to as framework genes (62, 63). (A) Frequencies of centromeric KIR gene-content haplotypes in the study population. (B) Frequencies of telomeric KIR gene-content haplotypes in the study population. All listed haplotypes that were not previously described, we have observed in multiple individuals and in combination with a high frequency haplotype, allowing their inference with confidence. All uncommon haplotypes for which the phase could not be determined are grouped as “undetermined”. Figure created with BioRender.com.
Of the framework genes, KIR2DL4 has the most copy number variation in our study. We observed 72 individuals (3.4%) carrying one copy of KIR2DL4 and 67 (3.1%) carrying three copies (Figure 2A). In this cohort, deletion of KIR2DL4 is invariably accompanied by the deletion of KIR3DL1S1. KIR3DP1 is deleted in 63 of the 72 (87.5%) haplotypes having the KIR2DL4~KIR3DL1S1 deletion. KIR3DL1S1 and KIR3DP1 are duplicated in 63 out of 68 individuals carrying duplications of KIR2DL4. Insertion of KIR3DP1~KIR2DL4~KIR3DS1 into a tA01 haplotype created a novel haplotype, carried by 38 individuals (f = 0.009; Figure 2B). Insertion of the segment KIR2DS3*00103~KIR2DP1~KIR2DL1*00401~KIR3DP1~ KIR2DL4*00501~KIR3DS1*01301~KIR2DL5A*00501 also into tA01 gave another novel haplotype observed in 19 individuals (f = 0.004; Figure 2C). In addition, we always observed the centromeric cB04 haplotype to be in the same gametic phase as tB03, and cA03 always with tB02. Duplication of KIR2DL2 present in cB02 was observed in 7 individuals (f = 0.002)
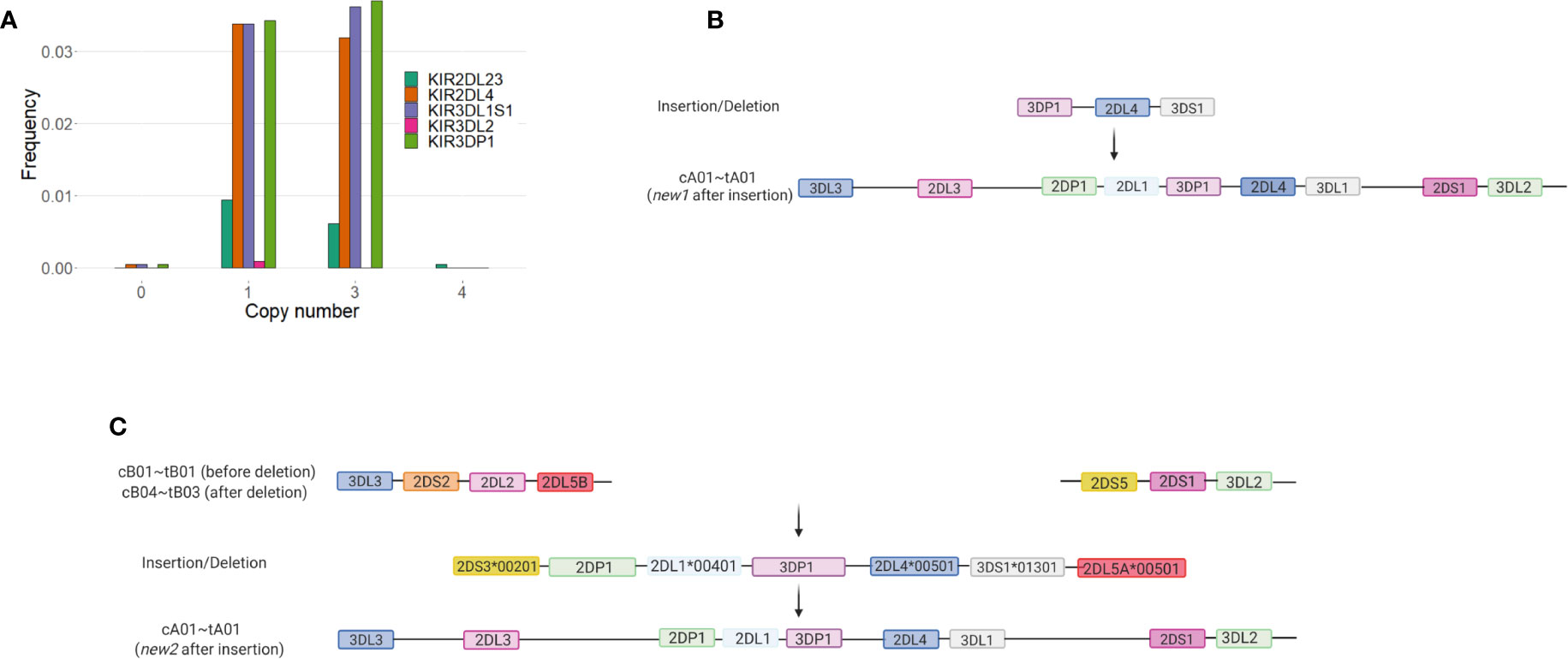
Figure 2 Deletions and duplications involving KIR2DL4, KIR3DL1S1, and KIR3DP1 were observed in more than 6% of KIR haplotypes Together with KIR2DL23 and KIR3DL1S1, framework genes (KIR3DL3, KIR3DP1, KIR2DL4, and KIR3DL2) were initially considered to be present in all KIR haplotypes, with only rare exceptions. Here, we show that deletions and duplications in these genes are relatively frequent in the study population. (A) Frequencies of gene copy number for individual genes. (B) Suggested origin of the novel haplotype containing a duplication of KIR3DP1~KIR2DL4~KIR3DS1 and observed in 38 individuals (f = 0.009). Created with BioRender.com. (C) Suggested origin of the novel haplotype containing a duplication of KIR2DS3~KIR2DP1~KIR2DL1~KIR3DP1~KIR2DL4~KIR3DS1~KIR2DL5A, observed in 19 individuals (f = 0.004). Figure created with BioRender.com.
Several KIR Haplotypes Are Marked by Specific KIR Alleles
All 13 KIR genes were genotyped to five-digit allele resolution in the study sample. We identified 250 KIR alleles, of which 90 (37.6%) have frequencies equal or greater than 0.01, and 40 (17%) have frequencies equal or greater than 0.05 (Figure 3A). KIR3DL3 has the highest variety of alleles (n = 83), followed by KIR3DL2 (n = 48) and KIR3DL1S1 (n = 39) (Figure 3B). Figure 4 summarizes KIR allele diversity of the cohort and complete allele frequencies are given in Supplementary Table 2. Among the 20 most common centromeric haplotypes, 16 are cA01 (Figure 5A). Similarly, 17 of the 20 most common telomeric haplotypes were tA01 (Figure 5B).
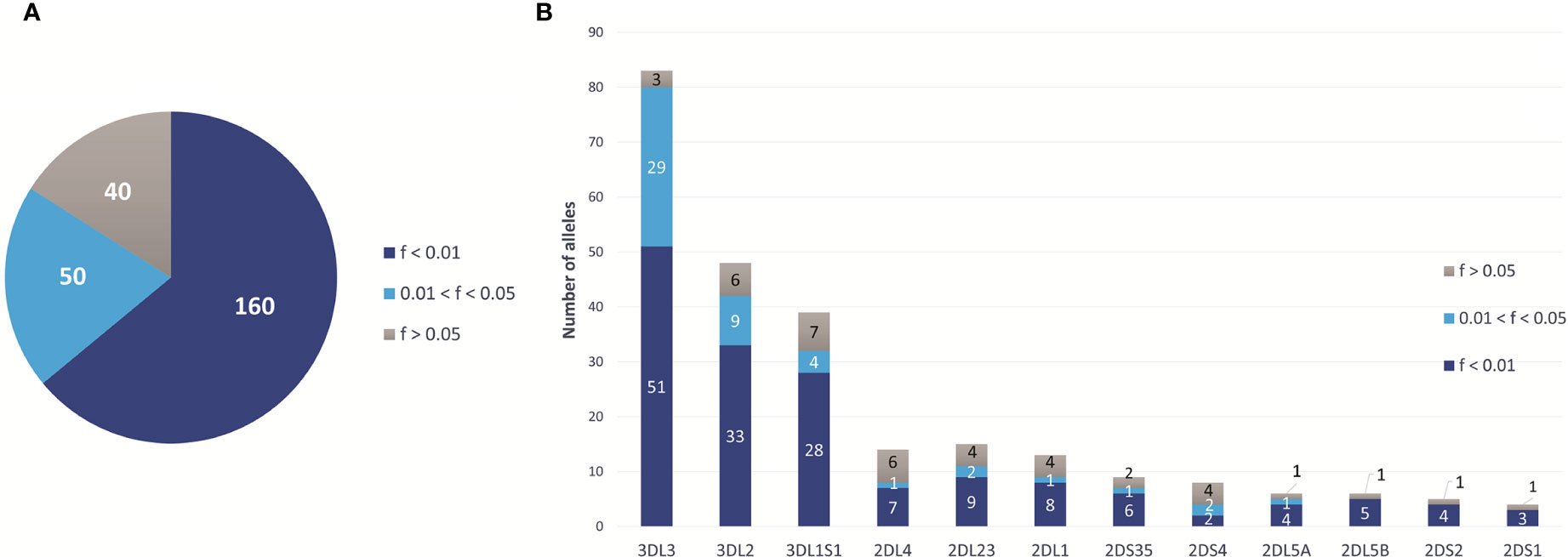
Figure 3 Overview of the KIR allelic diversity in European-Americans. (A) Total number of KIR alleles at 5-digit resolution observed in 2,130 European Americans and stratified by frequency. (B) Number of alleles observed for each gene (5-digit resolution), stratified by frequency.
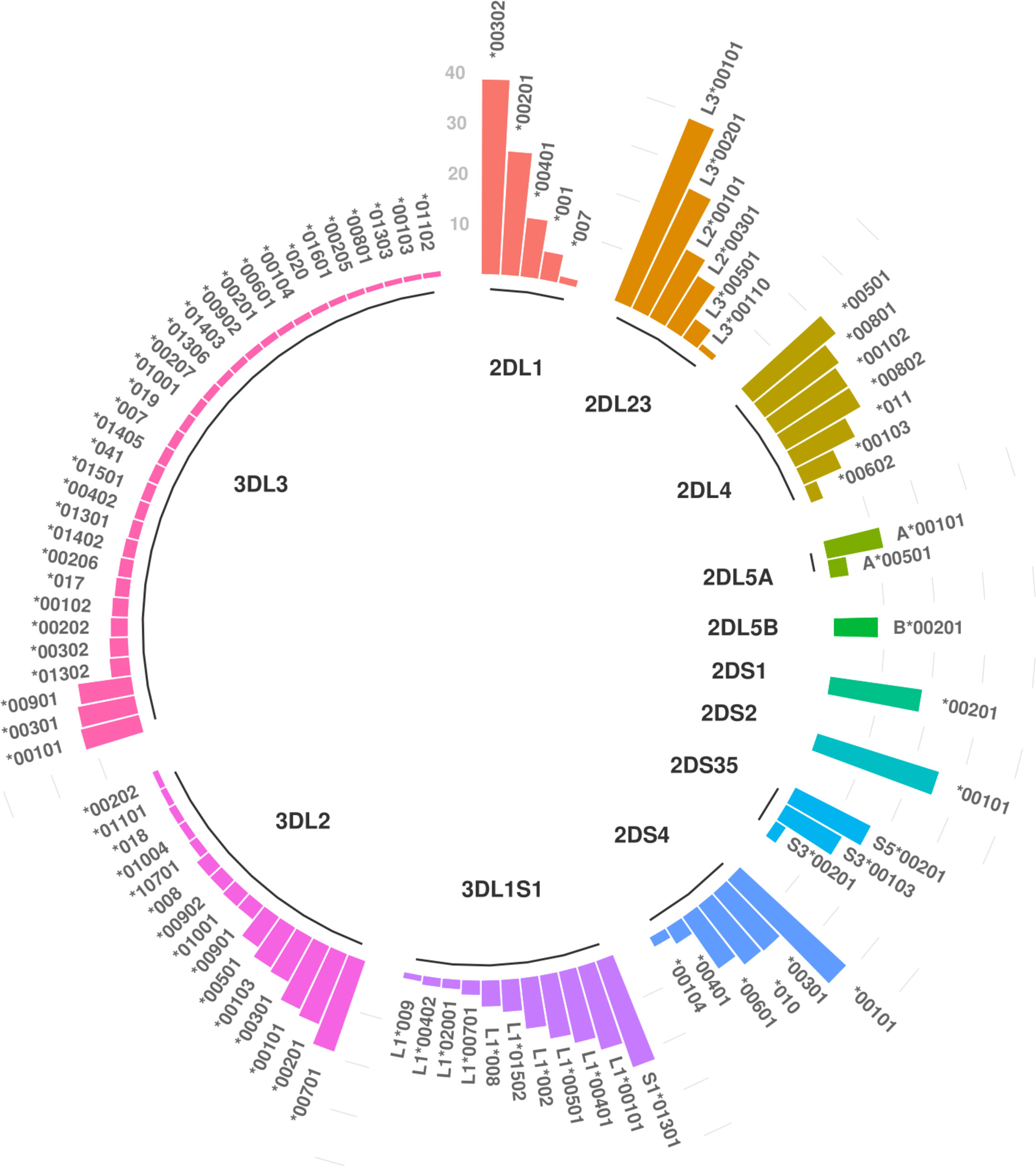
Figure 4 Overview of the most common alleles in European-Americans. Only alleles with frequencies greater than 1% are shown. For full list of allelic frequencies, see Supplementary Table 2.
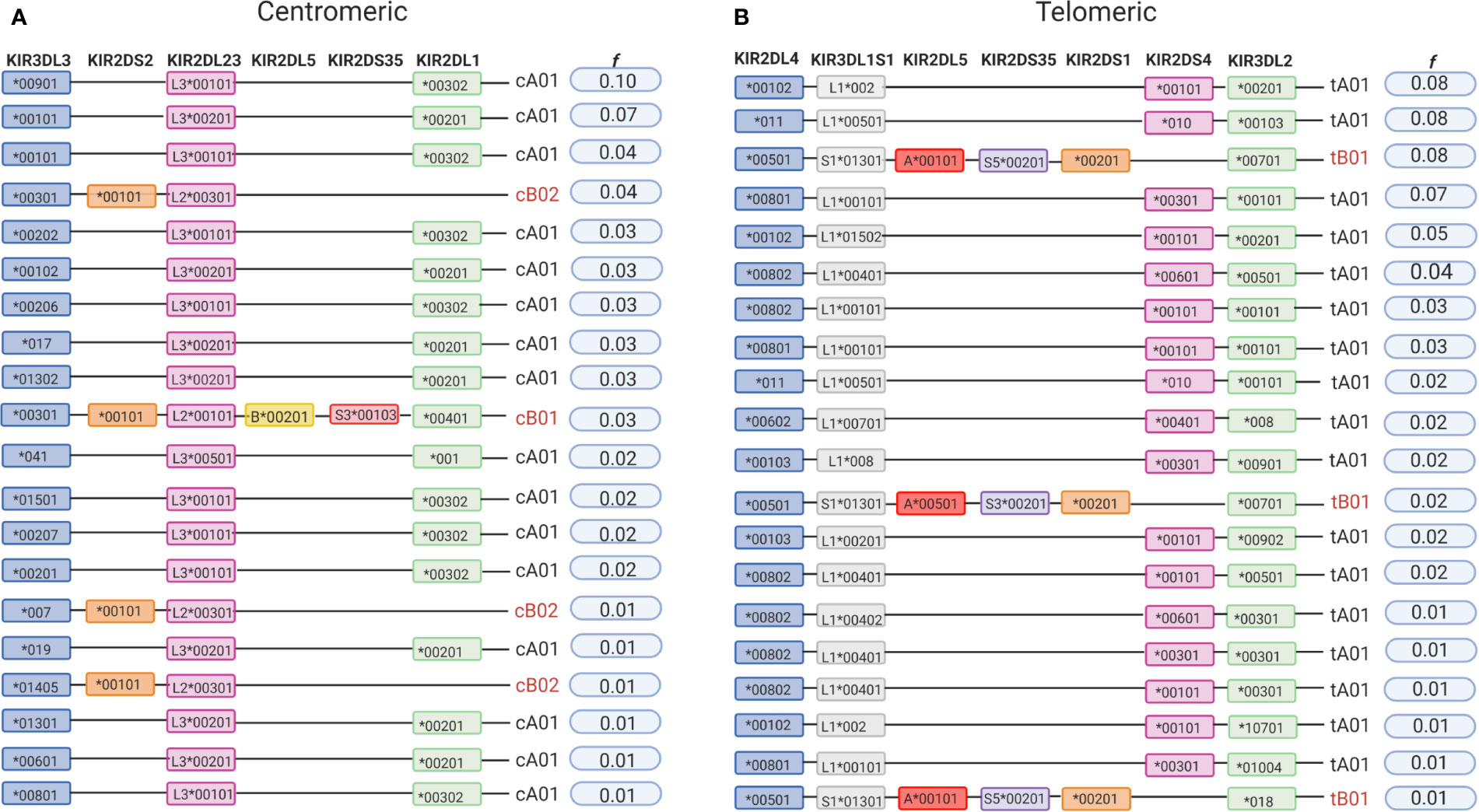
Figure 5 High-resolution allelic haplotypes in European-Americans. (A) The 20 most common centromeric KIR haplotypes. (B) The 20 most common telomeric KIR haplotypes observed in European Americans (n = 2,130). Created with BioRender.com.
This is the first study to describe high-resolution (5-digit) KIR haplotypes for all functional KIR genes in a large population sample. Analyzing this large number of individuals gave us sufficient power to fully explore the patterns of LD and identify alleles that are exclusively or predominately associated with specific haplotypes (Figure 6). For example, the alleles KIR3DL2*00701 and *018 were observed solely in tB01 haplotypes, whereas KIR3DL2*00103, *00201, *00501, *00901, *00101, and *008 were observed only in tA01. Similarly, KIR3DL3*00301 and *00402 are characteristic of CenB, whereas *00901 and *00101 are exclusive to CenA haplotypes. With few exceptions, 2DL4*00501 is the only KIR2DL4 allele found in tB01 haplotypes (99.4%), being in complete LD with KIR3DS1*013. Additionally, a few low-frequency alleles are associated with specific, uncommon haplotypes; for example, KIR3DL2*034 (f = 0.002) is present only in tB03, and KIR2DL5B*00801 (f = 0.004), is present only in cB04 (f = 0.01). Furthermore, for the first time we describe multiple high-resolution allelic configurations of the full-length cB04~tB03 haplotype (Supplementary Table 3).
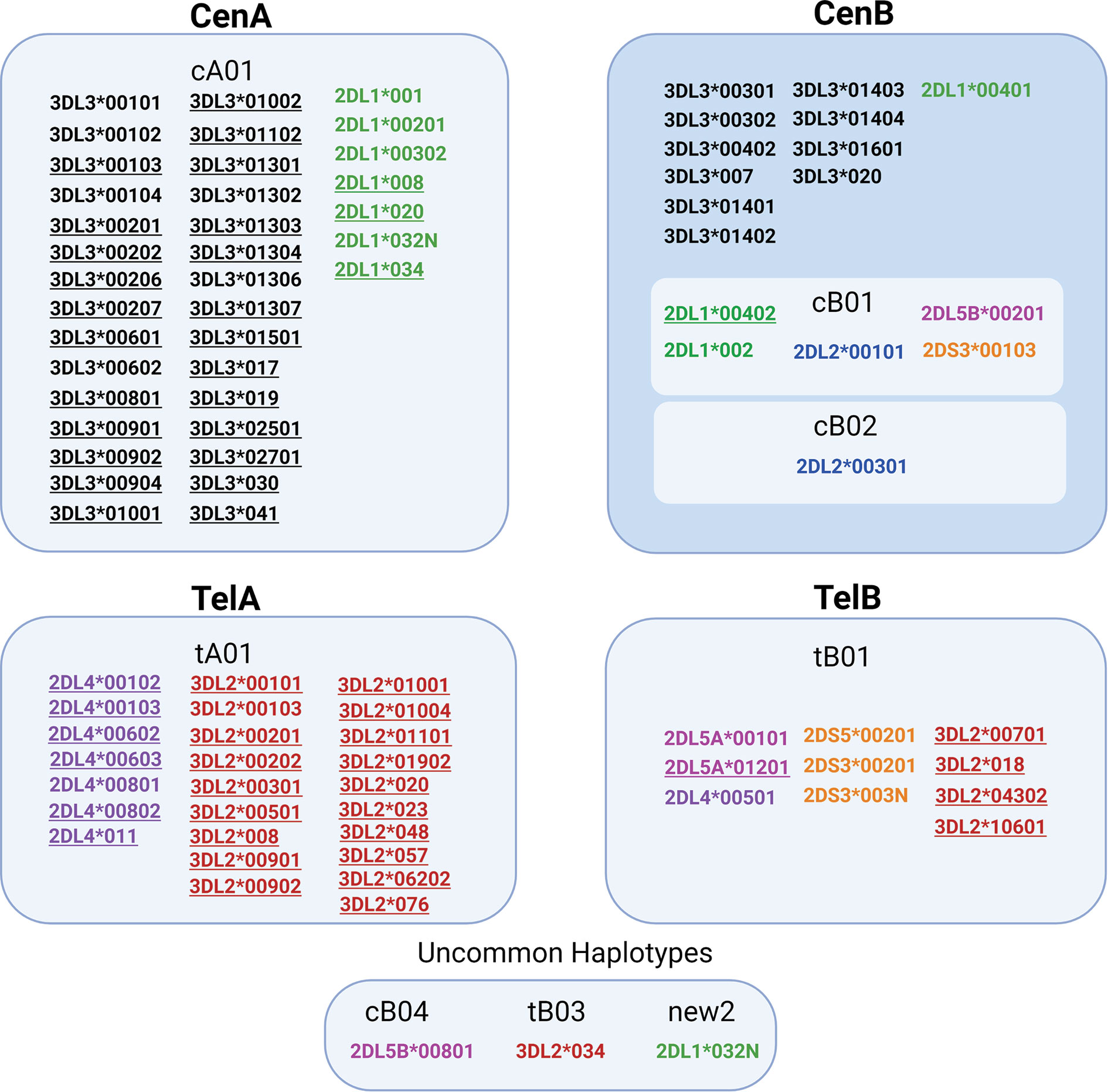
Figure 6 Some alleles are associated with specific KIR haplotypes. Alleles associated with specific haplotypes are listed inside each box. Underline marks alleles exclusively present in the specific haplotype. The other alleles were differentially associated with particular haplotypes, but not exclusively (>95% of the observations). For haplotype new2, please see Figure 2.
As well as the haplotypic associations, we observed many instances of strong LD among specific sets of KIR alleles (Figure 7). In summary, KIR2DL4*00501, KIR3DS1*01301, KIR2DS1*00201, KIR2DL5A*00101, KIR2DS5*00201, and KIR3DL2*00701 are frequently observed together. Many other KIR2DL4 alleles are in strong LD with specific KIR3DL1 alleles. Specific examples are KIR2DL4*00801 with KIR3DL1*00101 (D’ = 0.99, r2 = 0.85); KIR2DL4*011 with KIR3DL1*00501 (D’ = 0.95, r2 = 0.85); KIR2DL4*00802 with KIR3DL1*00401 (D’= 0.95, r2 = 0.73); KIR2DL4*00602 with KIR3DL1*00701 (D’ = 0.91, r2 = 0.68); and KIR2DL4*00103 with KIR3DL1*008 (D’ = 1, r2 = 0.6). In the centromeric region, KIR2DL5B*00201 and KIR2DS3*00103 are always observed together. KIR2DL1*00401 is in strong LD with KIR2DS3*00103 (D’ = 0.98 and r2 = 0.83) and KIR2DL5B*00201 (D’ = 0.95 and r2 = 0.82), whereas KIR2DL3*00201 is associated with KIR2DL1*00201 (D’ = 0.97, r2 = 0.89). A full list of LD values for pairs of KIR alleles is given in Supplementary Table 4.
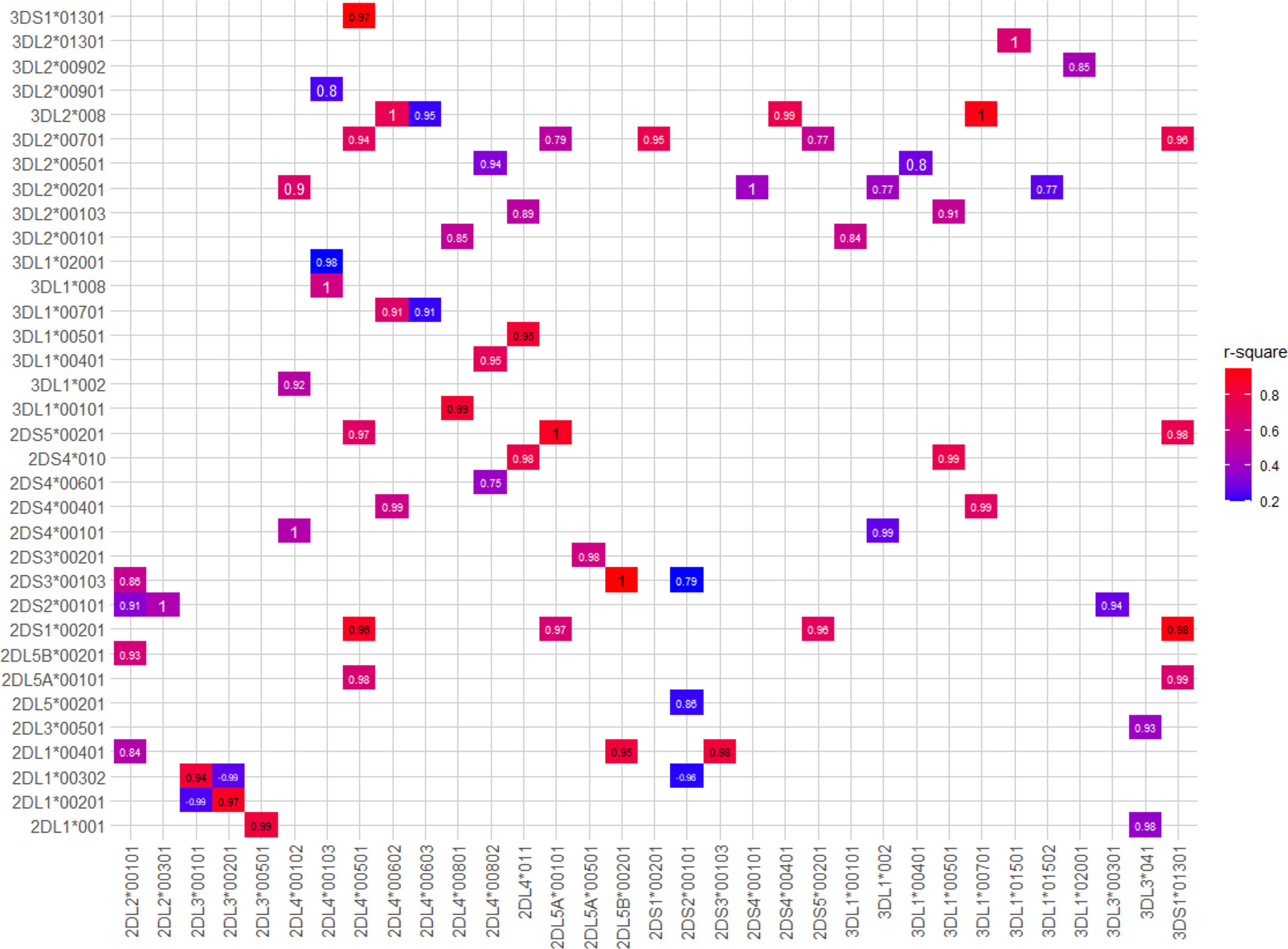
Figure 7 Strong linkage disequilibrium (LD) between KIR alleles. Boxes represent the pairs of KIR alleles with the strongest LD in the present study. D’ values are written inside of each box and color scale represent r2 values. Only pairs with r2 > 0.2, D’ > 0.7 were shown. The p-value of all pairs was < 10-5.
Numerous Novel KIR Variants Are Present in the Cohort of European Americans
In this analysis of 2,130 individuals, we identified 398 individuals (18.7%) carrying at least one KIR recombinant allele that do not correspond to any sequences deposited in the KIR database. We define as recombinant allele those that are characterized by different phasing combinations of previously known variable sites. These observations are likely to represent the presence of new alleles that were not present or not detected in previous studies of KIR variation. KIR3DL1S1 accounts for 33% of the observations of candidate new alleles, corresponding to a total allelic frequency of 0.04 at this locus. A large proportion of individuals carrying possible novel alleles were also observed for other KIR2D and KIR3D genes (Table 1).
In addition to these candidate novel KIR recombinant alleles, we also used our software to identify possible novel SNVs. In some cases, these SNVs may have been reported in the dbSNP database (64), but they were not associated with any KIR allele sequence deposited in the IPD-KIR database release 2.9.0 (12). Therefore, in the context of KIR, these SNVs would be contributing to novel alleles that each differ from a known KIR allele by a single nucleotide substitution. To confirm the sequences of novel variants, we used the Sanger method to re-sequence individuals carrying any possible novel SNV in KIR2DL1 and KIR3DL1S1. While confirmation of all novel variants was out of scope for the current project, we selected these loci as exemplars for this work due to substantial previous work examining their structure and function (25, 56, 61, 65–71), including the availability of crystal for molecular modeling structures (57, 72–76). In future work we will continue to explore novel variants that were detected at other loci during this study.
For KIR2DL1, 8 of 30 variants were confirmed by Sanger sequencing, while 10 of 32 variants were similarly confirmed for KIR3DL1S1. Most of the SNVs in KIR3DL1S1 were observed in only a single individual, except for two synonymous variants, rs754894112 and rs1462310393 (Table 2). In contrast, the majority of confirmed novel variants in KIR2DL1 were observed in several individuals (Table 3). Interestingly, 14 out of the 18 confirmed variants in these two genes were non-synonymous substitutions, with functional effects ranging from conservative to radical according to the Grantham scale of physicochemical distances between amino acids (77).
Simulation of Molecular Dynamics Predicts That Dimorphism in Codon 151 of KIR2DL1 Affects Binding to HLA Class I
To explore the functional differences of KIR2DL1 alleles that differ by a single nucleotide, we simulated and compared the molecular dynamics simulations of KIR2DL1 allotypes that differ by the non-conservative substitution of proline to arginine at position 151. Underlying this difference is rs200879366*C>G. Different conformations were sampled during the simulation trajectory, so that each time step features an individual conformer. The angle change mediated the transition between free and HLA-bound states of KIR2DL1 between the Ig domains (D1 and D2) that eventually affected the HLA binding region (Figure 8A). Within 100ns of simulation, the angle between the D1 and D2 domains was decreased by 10° in the wildtype (Pro) but was not perturbed in the mutant (Arg) (Figure 8B). The conformational transition appears to be mediated by a network of interactions spanning from Met44 to Arg 151. In the wildtype, Met44 is released from Pro185, allowing the angle between D1 and D2 to decrease (Figure 8C). By contrast, the angle is increased in the mutant. This enables Arg151 to form a salt bridge with Asp135, leading to an interaction between Met136 and Pro185 (Figure 8D) that allows Pro185 to more strongly associate with Met44. This set of localized rearrangements in KIR2DL1 is likely essential for its stable binding to HLA class I.
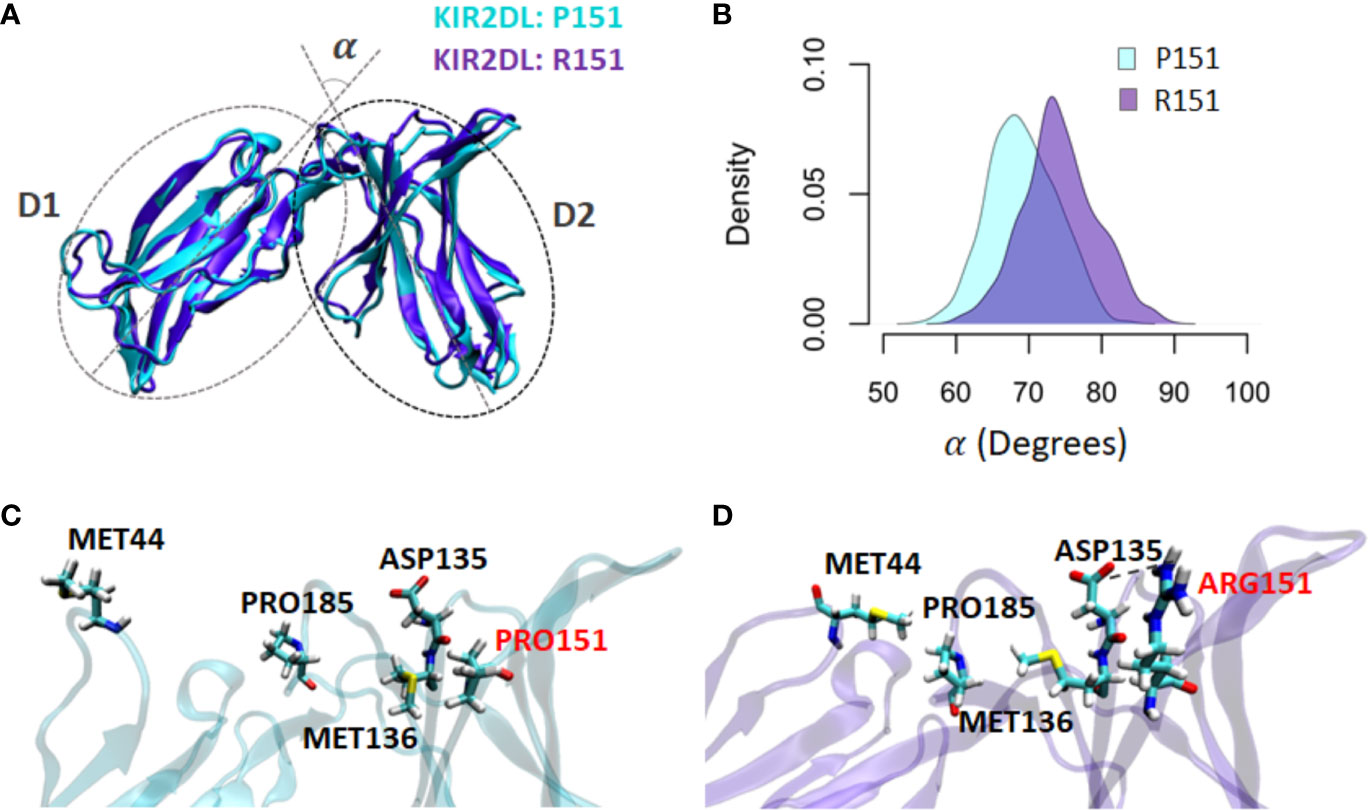
Figure 8 Pro151Arg substitution in KIR2DL1 is predicted to affect the stability of its binding to HLA-C. The impact of the Pro151Arg mutation on the KIR2DL1 conformation and its HLA-C binding site was studied by simulating both the mutant and wildtype structures. (A). The angle between D1 and D2 domains (alpha) of KIR2DL1 was computed in both allotypes as an indicator of conformational transition from the HLA-bound to a free state. (B) The alpha angle in the mutant is closer to the HLA-bound state, whereas the transition to the unbound state occurs smoothly in the wildtype. (C) The interaction between Met44 and Pro185 was disrupted upon angle change between D1 and D2 in the wildtype. (D) Arg151 forms an interaction with Asp135, which also leads to new interactions between Met136, Pro185, and Met44, maintaining the open conformation of the mutant.
Discussion
The general configuration of KIR gene-content haplotypes was first described two decades ago, when it was observed that four framework genes separate two distinct sub-clusters of genes (7, 24, 62, 78, 79). KIR3DL3 and KIR3DP1 were seen to delimit the centromeric region, while KIR2DL4 and KIR3DL2 delimit the telomeric region of the KIR gene family. The other two genes that are present in most KIR haplotypes are KIR2DL23 and KIR3DL1S1 (6). Although deletions and duplications of these genes have been previously reported (15–17, 80–82), technical limitations have precluded direct copy number determination of all KIR in large-scale population studies. We show here that large structural deletions and duplications involving the framework genes are relatively frequent in European-descendant individuals. For instance, more than 6% of individuals carry a deletion or duplication of KIR2DL4. Similar to haplotype variants described in the literature (13, 51, 52), all gene-content KIR haplotypes lacking KIR2DL4 also lacked KIR3DL1S1 (tB02 and tB03). These haplotypes have been described for other European-Americans (52), while an extensive study of Europeans from Germany did not seek to analyze novel gene-content haplotypes (83). Observation of KIR2DL4~KIR3DL1S1 deletions at high-frequencies in Africans (18, 25) raises the possibility that these variant KIR haplotypes originated prior to the modern human migration out of Africa, and that they might also be present in most worldwide populations.
Interestingly, haplotypes carrying KIR2DL4 duplications also have duplications of KIR3DL1S1 and KIR3DP1. Based on our observations, including the fact that cB04~tB03 are always in phase, we propose that a single deletion of KIR2DS3*00103~KIR2DP1~KIR2DL1*00401~ KIR3DP1~KIR2DL4*00501~KIR3DS1*01301~KIR2DL5A*00501 from the haplotype cB01~tB01 originated the cB04~tB03 haplotype. This seven-locus fragment was possibly inserted into cA01~tA01, which would explain the novel full-length haplotype that we identified in multiple individuals. The large cohort that we analyzed allowed us to phase the alleles of the seven-locus indel at high-resolution, providing a unique opportunity to infer the origin of these haplotypic variants.
Previous studies described KIR haplotypes at lower genotyping resolution and in smaller sample sizes. For example, Vierra-Green et al. (52) described KIR haplotypes at 3-digit resolution in 506 Euro-Americans while Hou et al. (20) analyzed most KIR genes at higher resolution but in a small cohort. Here, we present the first study to show 5-digit allelic haplotypes of all KIR genes for a large sample of the European-descent U.S population. Notably, the most common centromeric haplotype in our study cohort, KIR3DL3*00901~ KIR2DL3*00101~KIR2DL1*00302 (f = 0.10), is also the most common in four African populations (Datoonga, f = 0.11; Baka, f = 0.15; Dogon, f = 0.18; and Fulani, f = 0.14) (18). This haplotype is likely the same reported as the most frequent (f = 0.23) in a smaller European American cohort that was not analyzed for all KIR genes at high resolution (20). Interestingly, two of the low-frequency telomeric haplotypes present in our sample (tA02 and KIR3DL2) have not been reported in other European populations, but were observed in African populations from Mali (3DL2, f = 0.01), Democratic Republic of Congo (tA02, f = 0.08) and Tanzania (3DL2, f = 0.01; tA02, f = 0.01) (18). A limitation of determining haplotypes without family segregation studies or confirmation by long-range sequencing is the impossibility to identify unknown haplotype structures or to precisely infer those haplotypes observed in lower frequencies. For this reason, we were not able to confidently identify the less common haplotypes, therefore, presenting data only for the most common ones. However, our large population sample coupled with the curated high-quality data allow us to identify the haplotypic diversity that represent most of the KIR diversity in Europeans.
Our well-powered analysis of LD across the KIR region shows that some alleles are clearly associated with specific structural haplotypes. Because KIR2DL4*00501 and KIR3DL2*00701 are present in tB01 and are associated with other tB01-associated alleles, such as KIR3DS1*01301, it was possible to verify that the insertion of fragments containing KIR3DS1 occurred on the cA01~tA01 haplotype. In some cases, specific alleles also associate with uncommon haplotypes, which may be used as markers for these unique haplotypes. These examples highlight how our detailed LD information for high-resolution KIR sequencing constitutes a significant resource, yielding valuable information that will facilitate the comprehension and identification of KIR haplotypes in future studies.
With the development of robust high-resolution KIR typing methods, the discovery of novel KIR variants has become more achievable. However, short read misalignment is a major confounding factor in discovering novel variants, particularly those differing from known variants by only one nucleotide. Because the identification of possible novel SNVs is overall not likely in comparison to the possibility of being artifacts due to misalignment of highly similar sequence reads, our pipeline is initially set to exclude them from the primary analysis but flag them for further detailed inspection. This is a limitation intrinsic to short-sequence data analysis in the context of the KIR sequence homology, and rare novel variants might be missed. Aiming for an overview of the extent of this methodological limitation, we applied Sanger method to re-sequence all possible novel variants in two highly polymorphic genes, regardless of their frequencies. Although we confirmed approximately one-third of the possible novel SNVs for KIR3DL1S1 and KIR2DL1, most of these variants were observed in only one or a few individuals. In other words, even though our pipeline can possibly miss some of the novel variation, the new SNVs do not represent significant overall distortions in our dataset. In fact, our method has been proven to show an overall high performance for determining accurate genotypes (median 96.5%), and only 1% to 3% of unresolved genotypes (50).
Low frequent variants may, however, be of particular interest especially if they may cause impact on the receptors’ function. It is remarkable that most confirmed novel variants cause moderate to radical non-synonymous substitutions, ultimately leading to functional protein variation. To provide new insights to the functional significance of describing novel variants, we focused on variant rs200879366*G, which was previously reported with a frequency of 0.01 in the Finnish population (84) but had not previously been associated with a specific KIR allele. Although residue 151 is in the D2 domain it does not make direct contact with the HLA-C ligand. Nevertheless, polymorphism at the neighboring residue, position 154, has been implicated in differential avidity for the HLA-C ligand (85). Our prediction shows that Asp135, which is directly engaged in HLA binding, forms a bond with Arg151, allowing us to speculate that rs200879366*G may result in reduced binding to HLA. This example demonstrates the likelihood that many other functional variants will be identified as we interrogate KIR allelic diversity in worldwide populations.
According to the recently updated IPD-KIR database (Release 2.10.0, 16 December 2020), the variant rs200879366*G marks two unconfirmed alleles, KIR2DL1*044 and KIR2DL1*046, corroborating our findings. These unconfirmed allele sequences were freshly submitted by the same group that genotyped KIR in over a million European samples from the DKMS donor registry (86). Although a remarkable effort in its scale and importance to the field, that study targeted specific exons of each KIR, resulting in a 3-digit resolution genotyping with substantial ambiguities. In contrast, our study sought to analyze all KIR exons and introns of each gene (5-digit resolution). While smaller than the DKMS study by orders of magnitude, our study is nevertheless the largest sample to-date to comprehensively analyze all aspects of KIR variation at this resolution, including copy number, allele-haplotype associations, pairwise LD, and functional consequences of novel variation.
For decades, most KIR studies in populations were limited to analyzing the presence and absence of genes (5, 7, 9, 62, 87–89). The study of KIR gene content laid the basis of the field and suggested that KIR diversity and plasticity were and may still be ahead of our technical capabilities. Here, we aimed to set new ground for exploring KIR diversity by providing the first large-scale study to deeply analyze copy number variation and high-resolution allelic variation of all genes in a large population sample from the United States. Our results show a large proportion of multi-locus deletions and duplications of genes that were until recently considered rare, in addition to unusual gene-content haplotypes and a high frequency of novel alleles. We argue that as we continue to interrogate KIR at high-resolution, we will continue to uncover more layers of this region’s complexity, discovering frequent novel variants with functional relevance that have been previously missed due to technical limitations.
Data Availability Statement
The original contributions presented in the study are included in the article/Supplementary Material. Further inquiries can be directed to the corresponding author.
Author Contributions
JH, PN, and DA designed the study. NN-G, GM-M, and DA performed NGS and Sanger sequencing. LA and DA analyzed the data. WM and RD contributed with bioinformatics analysis. HS performed molecular dynamics simulations. SC managed and organized the samples. PP, MF-V, JO, PN, and JH contributed with samples and/or reagents. LA, DA, and JH drafted the manuscript. All authors contributed to the article and approved the submitted version.
Funding
This work was supported by grants U19NS095774 and U01 AI090905 from the U.S. National Institutes of Health (NIH). The UCSF DNA biorepository is supported by Si-2001-35701 from the National Multiple Sclerosis Society.
Conflict of Interest
The authors declare that the research was conducted in the absence of any commercial or financial relationships that could be construed as a potential conflict of interest.
Acknowledgments
LA acknowledges the scholarship from the Coordenação de Aperfeiçoamento de Pessoal de Nível Superior – Brasil (CAPES) – Finance Code 001 and the Doctorate Dissertation Research Award scholarship from Fulbright Program.
Supplementary Material
The Supplementary Material for this article can be found online at: https://www.frontiersin.org/articles/10.3389/fimmu.2021.674778/full#supplementary-material
Supplementary Table 1 | KIR gene-content frequencies in European-Americans.
Supplementary Table 2 | High-resolution allelic frequencies of the KIR genes in European-Americans.
Supplementary Table 3 | Multiple configurations of the haplotype cB04~tB03 in European-Americans.
Supplementary Table 4 | Pairwise linkage disequilibrium between KIR alleles. Only pairs with r2 > 0.2 and D’ > 0.7 are shown.
References
1. Martinet L, Smyth MJ. Balancing Natural Killer Cell Activation Through Paired Receptors. Nat Rev Immunol (2015) 15:243–54. doi: 10.1038/nri3799
2. Lanier LL. NK Cell Receptors. Annu Rev Immunol (1998) 16:359–93. doi: 10.1146/annurev.immunol.16.1.359
3. Wende H, Colonna M, Ziegler A, Volz A. Organization of the Leukocyte Receptor Cluster (LRC) on Human Chromosome 19q13.4. Mamm Genome (1999) 10:154–60. doi: 10.1007/s003359900961
4. Wilson MJ, Torkar M, Trowsdale J. Genomic Organization of a Human Killer Cell Inhibitory Receptor Gene. Tissue Antigens (1997) 49:574–9. doi: 10.1111/j.1399-0039.1997.tb02804.x
5. Norman PJ, Chandanayingyong D, Vaughan RW, Verity DH, Stephens HAF. Distribution of Natural Killer Cell Immunoglobulin-Like Receptor Sequences in Three Ethnic Groups. Immunogenetics (2001) 52:195–205. doi: 10.1007/s002510000281
6. Uhrberg M, Valiante NM, Shum BP, Shilling HG, Lienert-Weidenbach K, Corliss B, et al. Human Diversity in Killer Cell Inhibitory Receptor Genes. Immunity (1997) 7:753–63. doi: 10.1016/S1074-7613(00)80394-5
7. Hsu KC, Liu X-R, Selvakumar A, Mickelson E, O’Reilly RJ, Dupont B. Killer Ig-Like Receptor Haplotype Analysis by Gene Content: Evidence for Genomic Diversity With a Minimum of Six Basic Framework Haplotypes, Each With Multiple Subsets. J Immunol (2002) 169:5118–29. doi: 10.4049/jimmunol.169.9.5118
8. Gonzalez-Galarza FF, McCabe A, Santos EJMD, Jones J, Takeshita L, Ortega-Rivera ND, et al. Allele Frequency Net Database (AFND) 2020 Update: Gold-Standard Data Classification, Open Access Genotype Data and New Query Tools. Nucleic Acids Res (2020) 48:D783–8. doi: 10.1093/nar/gkz1029
9. Hollenbach JA, Nocedal I, Ladner MB, Single RM, Trachtenberg EA. Killer Cell Immunoglobulin-Like Receptor (KIR) Gene Content Variation in the HGDP-CEPH Populations. Immunogenetics (2012) 64:719–37. doi: 10.1007/s00251-012-0629-x
10. Single RM, Martin MP, Gao X, Meyer D, Yeager M, Kidd JR, et al. Global Diversity and Evidence for Coevolution of KIR and HLA. Nat Genet (2007) 39:1114–9. doi: 10.1038/ng2077
11. Misra MK, Augusto DG, Martin GM, Nemat-Gorgani N, Sauter J, Hofmann JA, et al. Report From the Killer-cell Immunoglobulin-Like Receptors (KIR) Component of the 17th International HLA and Immunogenetics Workshop. Hum Immunol (2018) 79:825–33. doi: 10.1016/j.humimm.2018.10.003
12. Robinson J, Barker DJ, Georgiou X, Cooper MA, Flicek P, Marsh SGE. Ipd-IMGT/HLA Database. Nucleic Acids Res (2020) 48:D948–55. doi: 10.1093/nar/gkz950
13. Roe D, Vierra-Green C, Pyo C-W, Eng K, Hall R, Kuang R, et al. Revealing Complete Complex KIR Haplotypes Phased by Long-Read Sequencing Technology. Genes Immun (2017) 18:127–34. doi: 10.1038/gene.2017.10
14. Traherne JA, Martin M, Ward R, Ohashi M, Pellett F, Gladman D, et al. Mechanisms of Copy Number Variation and Hybrid Gene Formation in the KIR Immune Gene Complex. Hum Mol Genet (2010) 19:737–51. doi: 10.1093/hmg/ddp538
15. Martin MP, Bashirova A, Traherne J, Trowsdale J, Carrington M. Cutting Edge: Expansion of the KIR Locus by Unequal Crossing Over. J Immunol (2003) 171:2192. doi: 10.4049/jimmunol.171.5.2192
16. Norman PJ, Abi-Rached L, Gendzekhadze K, Hammond JA, Moesta AK, Sharma D, et al. Meiotic Recombination Generates Rich Diversity in NK Cell Receptor Genes, Alleles, and Haplotypes. Genome Res (2009) 19:757–69. doi: 10.1101/gr.085738.108
17. Pyo C-W, Wang R, Vu Q, Cereb N, Yang SY, Duh F-M, et al. Recombinant Structures Expand and Contract Inter and Intragenic Diversification At the KIR Locus. BMC Genomics (2013) 14:89. doi: 10.1186/1471-2164-14-89
18. Nemat-Gorgani N, Guethlein LA, Henn BM, Norberg SJ, Chiaroni J, Sikora M, et al. Diversity of KIR, Hla Class I, and Their Interactions in Seven Populations of Sub-Saharan Africans. JI (2019) 202:2636–47. doi: 10.4049/jimmunol.1801586
19. Alicata C, Ashouri E, Nemat-Gorgani N, Guethlein LA, Marin WM, Tao S, et al. Kir Variation in Iranians Combines High Haplotype and Allotype Diversity With an Abundance of Functional Inhibitory Receptors. Front Immunol (2020) 11:556. doi: 10.3389/fimmu.2020.00556
20. Hou L, Chen M, Ng J, Hurley CK. Conserved KIR Allele-Level Haplotypes are Altered by Microvariation in Individuals With European Ancestry. Genes Immun (2012) 13:47–58. doi: 10.1038/gene.2011.52
21. Tao S, Kichula KM, Harrison GF, Farias TDJ, Palmer WH, Leaton LA, et al. The Combinatorial Diversity of KIR and HLA Class I Allotypes in Peninsular Malaysia. Immunology (2020) 162:389–404. doi: 10.1111/imm.13289
22. Long EO, Rajagopalan S. HLA Class I Recognition by Killer Cell Ig-like Receptors. Semin Immunol (2000) 12:101–8. doi: 10.1006/smim.2000.0212
23. Norman PJ, Parham P. Complex Interactions: The Immunogenetics of Human Leukocyte Antigen and Killer Cell Immunoglobulin-Like Receptors. Semin Hematol (2005) 42:65–75. doi: 10.1053/j.seminhematol.2005.01.007
24. Trowsdale J, Barten R, Haude A, Stewart CA, Beck S, Wilson MJ. The Genomic Context of Natural Killer Receptor Extended Gene Families: Genomics of NK Receptor Gene Families. Immunol Rev (2001) 181:20–38. doi: 10.1034/j.1600-065X.2001.1810102.x
25. Norman PJ, Hollenbach JA, Nemat-Gorgani N, Guethlein LA, Hilton HG, Pando MJ, et al. Co-Evolution of Human Leukocyte Antigen (Hla) Class I Ligands With Killer-Cell Immunoglobulin-Like Receptors (KIR) in a Genetically Diverse Population of Sub-Saharan Africans. PloS Genet (2013) 9:e1003938. doi: 10.1371/journal.pgen.1003938
26. Augusto DG, Petzl-Erler ML. KIR and HLA Under Pressure: Evidences of Coevolution Across Worldwide Populations. Hum Genet (2015) 134:929–40. doi: 10.1007/s00439-015-1579-9
27. Gendzekhadze K, Norman PJ, Abi-Rached L, Graef T, Moesta AK, Layrisse Z, et al. Co-Evolution of KIR2DL3 With HLA-C in a Human Population Retaining Minimal Essential Diversity of KIR and HLA Class I Ligands. PNAS (2009) 106:18692–7. doi: 10.1073/pnas.0906051106
28. Moffett A, Colucci F. Co-Evolution of NK Receptors and HLA Ligands in Humans is Driven by Reproduction. Immunol Rev (2015) 267:283–97. doi: 10.1111/imr.12323
29. Kulkarni S, Martin MP, Carrington M. The Yin and Yang of HLA and KIR in Human Disease. Semin Immunol (2008) 20:343–52. doi: 10.1016/j.smim.2008.06.003
30. Williams AP, Bateman AR, Khakoo SI. Hanging in the Balance. KIR and Their Role in Disease. Mol Interv (2005) 5:226–40. doi: 10.1124/mi.5.4.6
31. Boudreau JE, Hsu KC. Natural Killer Cell Education in Human Health and Disease. Curr Opin Immunol (2018) 50:102–11. doi: 10.1016/j.coi.2017.11.003
32. Augusto DG. The Impact of KIR Polymorphism on the Risk of Developing Cancer: Not as Strong as Imagined? Front Genet (2016) 7:121. doi: 10.3389/fgene.2016.00121
33. Anderson KM, Augusto DG, Dandekar R, Shams H, Zhao C, Yusufali T, et al. Killer Cell Immunoglobulin-Like Receptor Variants Are Associated With Protection From Symptoms Associated With More Severe Course in Parkinson Disease. J Immunol (2020) 205:1323–30. doi: 10.4049/jimmunol.2000144
34. Augusto DG, Lobo-Alves SC, Melo MF, Pereira NF, Petzl-Erler ML. Activating KIR and HLA Bw4 Ligands are Associated to Decreased Susceptibility to Pemphigus Foliaceus, an Autoimmune Blistering Skin Disease. PloS One (2012) 7:e39991. doi: 10.1371/journal.pone.0039991
35. Suzuki Y, Hamamoto Y, Ogasawara Y, Ishikawa K, Yoshikawa Y, Sasazuki T, et al. Genetic Polymorphisms of Killer Cell Immunoglobulin-Like Receptors are Associated With Susceptibility to Psoriasis Vulgaris. J Invest Dermatol (2004) 122:1133–6. doi: 10.1111/j.0022-202X.2004.22517.x
36. Hollenbach JA, Pando MJ, Caillier SJ, Gourraud P-A, Oksenberg JR. The Killer Immunoglobulin-Like Receptor KIR3DL1 in Combination With HLA-Bw4 is Protective Against Multiple Sclerosis in African Americans. Genes Immun (2016) 17:199–202. doi: 10.1038/gene.2016.5
37. Kim H-J, Choi H-B, Jang J-P, Baek I-C, Choi E-J, Park M, et al. Hla-Cw Polypmorphism and Killer Cell Immunoglobulin-Like Receptor (KIR) Gene Analysis in Korean Colorectal Cancer Patients. Int J Surg (2014) 12:815–20. doi: 10.1016/j.ijsu.2014.06.012
38. Middleton D, Vilchez JR, Cabrera T, Meenagh A, Williams F, Halfpenny I, et al. Analysis of KIR Gene Frequencies in HLA Class I Characterised Bladder, Colorectal and Laryngeal Tumours. Tissue Antigens (2007) 69:220–6. doi: 10.1111/j.1399-0039.2006.00792.x
39. Al Omar S, Middleton D, Marshall E, Porter D, Xinarianos G, Raji O, et al. Associations Between Genes for Killer Immunoglobulin-Like Receptors and Their Ligands in Patients With Solid Tumors. Hum Immunol (2010) 71:976–81. doi: 10.1016/j.humimm.2010.06.019
40. Auer ED, Tong HV, Amorim LM, Malheiros D, Hoan NX, Issler HC, et al. Natural Killer Cell Receptor Variants and Chronic Hepatitis B Virus Infection in the Vietnamese Population. Int J Infect Dis (2020) 96:541–7. doi: 10.1016/j.ijid.2020.05.033
41. Martin MP, Qi Y, Gao X, Yamada E, Martin JN, Pereyra F, et al. Innate Partnership of HLA-B and KIR3DL1 Subtypes Against HIV-1. Nat Genet (2007) 39:733–40. doi: 10.1038/ng2035
42. Podhorzer A, Dirchwolf M, Machicote A, Belen S, Montal S, Paz S, et al. The Clinical Features of Patients With Chronic Hepatitis C Virus Infections are Associated With Killer Cell Immunoglobulin-Like Receptor Genes and Their Expression on the Surface of Natural Killer Cells. Front Immunol (2017) 8:1912. doi: 10.3389/fimmu.2017.01912
43. Alves HV, de Moraes AG, Pepineli AC, Tiyo BT, de Lima Neto QA, Santos T da S, et al. The Impact of KIR/HLA Genes on the Risk of Developing Multibacillary Leprosy. PloS Negl Trop Dis (2019) 13:e0007696. doi: 10.1371/journal.pntd.0007696
44. Trowsdale J, Moffett A. NK Receptor Interactions With MHC Class I Molecules in Pregnancy. Semin Immunol (2008) 20:317–20. doi: 10.1016/j.smim.2008.06.002
45. Moffett A, Chazara O, Colucci F, Johnson MH. Variation of Maternal KIR and Fetal HLA-C Genes in Reproductive Failure: Too Early for Clinical Intervention. Reprod BioMed Online (2016) 33:763–9. doi: 10.1016/j.rbmo.2016.08.019
46. Colucci F. The Role of KIR and HLA Interactions in Pregnancy Complications. Immunogenetics (2017) 69:557–65. doi: 10.1007/s00251-017-1003-9
47. Hiby SE, Apps R, Sharkey AM, Farrell LE, Gardner L, Mulder A, et al. Maternal Activating KIRs Protect Against Human Reproductive Failure Mediated by Fetal HLA-C2. J Clin Invest (2010) 120:4102–10. doi: 10.1172/JCI43998
48. Hollenbach JA, Norman PJ, Creary LE, Damotte V, Montero-Martin G, Caillier S, et al. A Specific Amino Acid Motif of HLA-DRB1 Mediates Risk and Interacts With Smoking History in Parkinson’s Disease. Proc Natl Acad Sci USA (2019) 116:7419–24. doi: 10.1073/pnas.1821778116
49. Norman PJ, Hollenbach JA, Nemat-Gorgani N, Marin WM, Norberg SJ, Ashouri E, et al. Defining KIR and HLA Class I Genotypes At Highest Resolution Via High-Throughput Sequencing. Am J Hum Genet (2016) 99:375–91. doi: 10.1016/j.ajhg.2016.06.023
50. Marin W, Dandekar R, Augusto DG, Yusufali T, Heyn B, Hofmann J, et al. High-Throughput Interpretation of Killer-cell Immunoglobulin-Like Receptor Short-Read Sequencing Data With PING. bioRxiv [Preprint] (2021). doi: 10.1101/2021.03.24.436770
51. Pyo C-W, Guethlein LA, Vu Q, Wang R, Abi-Rached L, Norman PJ, et al. Different Patterns of Evolution in the Centromeric and Telomeric Regions of Group A and B Haplotypes of the Human Killer Cell Ig-Like Receptor Locus. PloS One (2010) 5:e15115. doi: 10.1371/journal.pone.0015115
52. Vierra-Green C, Roe D, Hou L, Hurley CK, Rajalingam R, Reed E, et al. Allele-Level Haplotype Frequencies and Pairwise Linkage Disequilibrium for 14 KIR Loci in 506 European-American Individuals. PloS One (2012) 7:e47491. doi: 10.1371/journal.pone.0047491
53. Peakall R, Smouse PE. GenAlEx 6.5: Genetic Analysis in Excel. Population Genetic Software for Teaching and Research—an Update. Bioinformatics (2012) 28:2537–9. doi: 10.1093/bioinformatics/bts460
54. Excoffier L, Lischer HEL. Arlequin Suite Ver 3.5: A New Series of Programs to Perform Population Genetics Analyses Under Linux and Windows. Mol Ecol Resour (2010) 10:564–7. doi: 10.1111/j.1755-0998.2010.02847.x
55. Sanger F, Nicklen S, Coulson AR. DNA Sequencing With Chain-Terminating Inhibitors. PNAS (1977) 74:5463–7. doi: 10.1073/pnas.74.12.5463
56. Vargas L de B, Dourado RM, Amorim LM, Ho B, Calonga-Solís V, Issler HC, et al. Single Nucleotide Polymorphism in KIR2DL1 is Associated With HLA-C Expression in Global Populations. Front Immunol (2020) 11:1881. doi: 10.3389/fimmu.2020.01881
57. Fan QR, Long EO, Wiley DC. Crystal Structure of the Human Natural Killer Cell Inhibitory Receptor KIR2DL1-HLA-Cw4 Complex. Nat Immunol (2001) 2:452–60. doi: 10.1038/87766
58. Grant BJ, Rodrigues APC, ElSawy KM, McCammon JA, Caves LSD. Bio3d: An R Package for the Comparative Analysis of Protein Structures. Bioinformatics (2006) 22:2695–6. doi: 10.1093/bioinformatics/btl461
59. Phillips JC, Hardy DJ, Maia JDC, Stone JE, Ribeiro JV, Bernardi RC, et al. Scalable Molecular Dynamics on CPU and GPU Architectures With NAMD. J Chem Phys (2020) 153:044130. doi: 10.1063/5.0014475
60. Jorgensen WL, Chandrasekhar J, Madura JD, Impey RW, Klein ML. Comparison of Simple Potential Functions for Simulating Liquid Water. J Chem Phys (1983) 79:926–35. doi: 10.1063/1.445869
61. Pugh J, Nemat-Gorgani N, Djaoud Z, Guethlein LA, Norman PJ, Parham P. In Vitro Education of Human Natural Killer Cells by KIR3DL1. Life Sci Alliance (2019) 2:e201900434. doi: 10.26508/lsa.201900434
62. Wilson MJ, Torkar M, Haude A, Milne S, Jones T, Sheer D, et al. Plasticity in the Organization and Sequences of Human KIR/ILT Gene Families. Proc Natl Acad Sci USA (2000) 97:4778. doi: 10.1073/pnas.080588597
63. Vilches C, Parham P. K IR : Diverse, Rapidly Evolving Receptors of Innate and Adaptive Immunity. Annu Rev Immunol (2002) 20:217–51. doi: 10.1146/annurev.immunol.20.092501.134942
64. Sherry ST, Ward MH, Kholodov M, Baker J, Phan L, Smigielski EM, et al. dbSNP: The NCBI Database of Genetic Variation. Nucleic Acids Res (2001) 29:308–11. doi: 10.1093/nar/29.1.308
65. Stewart CA, Laugier-Anfossi F, Vély F, Saulquin X, Riedmuller J, Tisserant A, et al. Recognition of Peptide–MHC Class I Complexes by Activating Killer Immunoglobulin-Like Receptors. PNAS (2005) 102:13224–9. doi: 10.1073/pnas.0503594102
66. Hilton HG, Guethlein LA, Goyos A, Nemat-Gorgani N, Bushnell DA, Norman PJ, et al. Polymorphic HLA-C Receptors Balance the Functional Characteristics of KIR Haplotypes. J Immunol (2015) 195:3160–70. doi: 10.4049/jimmunol.1501358
67. Bari R, Bell T, Leung W-H, Vong QP, Chan WK, Das Gupta N, et al. Significant Functional Heterogeneity Among KIR2DL1 Alleles and a Pivotal Role of Arginine245. Blood (2009) 114:5182–90. doi: 10.1182/blood-2009-07-231977
68. Béziat V, Traherne JA, Liu LL, Jayaraman J, Enqvist M, Larsson S, et al. Influence of KIR Gene Copy Number on Natural Killer Cell Education. Blood (2013) 121:4703–7. doi: 10.1182/blood-2012-10-461442
69. Le Luduec J-B, Boudreau JE, Freiberg JC, Hsu KC. Novel Approach to Cell Surface Discrimination Between KIR2DL1 Subtypes and KIR2DS1 Identifies Hierarchies in NK Repertoire, Education, and Tolerance. Front Immunol (2019) 10:734. doi: 10.3389/fimmu.2019.00734
70. Norman PJ, Abi-Rached L, Gendzekhadze K, Korbel D, Gleimer M, Rowley D, et al. Unusual Selection on the KIR3DL1/S1 Natural Killer Cell Receptor in Africans. Nat Genet (2007) 39:1092–9. doi: 10.1038/ng2111
71. Augusto DG, Norman PJ, Dandekar R, Hollenbach JA. Fluctuating and Geographically Specific Selection Characterize Rapid Evolution of the Human Kir Region. Front Immunol (2019) 10:989. doi: 10.3389/fimmu.2019.00989
72. Vivian JP, Duncan RC, Berry R, O’Connor GM, Reid HH, Beddoe T, et al. Killer Cell Immunoglobulin-Like Receptor 3DL1-Mediated Recognition of Human Leukocyte Antigen B. Nature (2011) 479:401–5. doi: 10.1038/nature10517
73. Pymm P, Illing PT, Ramarathinam SH, O’Connor GM, Hughes VA, Hitchen C, et al. MHC-I Peptides Get Out of the Groove and Enable a Novel Mechanism of HIV-1 Escape. Nat Struct Mol Biol (2017) 24:387–94. doi: 10.1038/nsmb.3381
74. Saunders PM, MacLachlan BJ, Pymm P, Illing PT, Deng Y, Wong SC, et al. The Molecular Basis of How Buried Human Leukocyte Antigen Polymorphism Modulates Natural Killer Cell Function. PNAS (2020) 117:11636–47. doi: 10.1073/pnas.1920570117
75. Fan QR, Mosyak L, Winter CC, Wagtmann N, Long EO, Wiley DC. Structure of the Inhibitory Receptor for Human Natural Killer Cells Resembles Haematopoietic Receptors. Nature (1997) 389:96–100. doi: 10.1038/38028
76. Stewart-Jones GBE, di Gleria K, Kollnberger S, McMichael AJ, Jones EY, Bowness P. Crystal Structures and KIR3DL1 Recognition of Three Immunodominant Viral Peptides Complexed to HLA-B*2705. Eur J Immunol (2005) 35:341–51. doi: 10.1002/eji.200425724
77. Grantham R. Amino Acid Difference Formula to Help Explain Protein Evolution. Science (1974) 185:862–4. doi: 10.1126/science.185.4154.862
78. Vilches C, Rajalingam R, Uhrberg M, Gardiner CM, Young NT, Parham P. KIR2DL5, a Novel Killer-Cell Receptor With a D0-D2 Configuration of Ig-Like Domains. J Immunol (2000) 164:5797–804. doi: 10.4049/jimmunol.164.11.5797
79. Barten R, Torkar M, Haude A, Trowsdale J, Wilson MJ. Divergent and Convergent Evolution of NK-cell Receptors. Trends Immunol (2001) 22:52–7. doi: 10.1016/S1471-4906(00)01802-0
80. Gómez-Lozano N, Estefanía E, Williams F, Halfpenny I, Middleton D, Solís R, et al. The Silent KIR3DP1 Gene (CD158c) is Transcribed and Might Encode a Secreted Receptor in a Minority of Humans, in Whom the KIR3DP1, KIR2DL4 and KIR3DL1/KIR3DS1 Genes are Duplicated. Eur J Immunol (2005) 35:16–24. doi: 10.1002/eji.200425493
81. Norman PJ, Carrington CVF, Byng M, Maxwell LD, Curran MD, Stephens HAF, et al. Natural Killer Cell Immunoglobulin-Like Receptor (KIR) Locus Profiles in African and South Asian Populations. Genes Immun (2002) 3:86–95. doi: 10.1038/sj.gene.6363836
82. Williams F, Maxwell LD, Halfpenny IA, Meenagh A, Sleator C, Curran MD, et al. Multiple Copies of KIR 3DL/S1 and KIR 2DL4 Genes Identified in a Number of Individuals. Hum Immunol (2003) 64:729–32. doi: 10.1016/s0198-8859(03)00089-2
83. Solloch UV, Schefzyk D, Schäfer G, Massalski C, Kohler M, Pruschke J, et al. Estimation of German Kir Allele Group Haplotype Frequencies. Front Immunol (2020) 11:429. doi: 10.3389/fimmu.2020.00429
84. Auton A, Abecasis GR, Altshuler DM, Durbin RM, Abecasis GR, Bentley DR, et al. A Global Reference for Human Genetic Variation. Nature (2015) 526:68–74. doi: 10.1038/nature15393
85. Hilton HG, Vago L, Older Aguilar A, Moesta AK, Graef T, Abi Rached L, et al. Mutation At Positively Selected Positions in the Binding Site for HLA-C Shows KIR2DL1 is a More Refined But Less Adaptable NK Cell Receptor Than KIR2DL3. J Immunol (2012) 189:1418–30. doi: 10.4049/jimmunol.1100431
86. Wagner I, Schefzyk D, Pruschke J, Schöfl G, Schöne B, Gruber N, et al. Allele-Level KIR Genotyping of More Than a Million Samples: Workflow, Algorithm, and Observations. Front Immunol (2018) 9:2843. doi: 10.3389/fimmu.2018.02843
87. Crum KA, Logue SE, Curran MD, Middleton D. Development of a PCR-SSOP Approach Capable of Defining the Natural Killer Cell Inhibitory Receptor (KIR) Gene Sequence Repertoires. Tissue Antigens (2000) 56:313–26. doi: 10.1034/j.1399-0039.2000.560403.x
88. Hollenbach JA, Augusto DG, Alaez C, Bubnova L, Fae I, Fischer G, et al. 16(Th) IHIW: Population Global Distribution of Killer Immunoglobulin-Like Receptor (KIR) and Ligands. Int J Immunogenet (2013) 40:39–45. doi: 10.1111/iji.12028
Keywords: NK receptors, alleles, high resolution, NGS, sequencing, population
Citation: Amorim LM, Augusto DG, Nemat-Gorgani N, Montero-Martin G, Marin WM, Shams H, Dandekar R, Caillier S, Parham P, Fernández-Viña MA, Oksenberg JR, Norman PJ and Hollenbach JA (2021) High-Resolution Characterization of KIR Genes in a Large North American Cohort Reveals Novel Details of Structural and Sequence Diversity. Front. Immunol. 12:674778. doi: 10.3389/fimmu.2021.674778
Received: 01 March 2021; Accepted: 20 April 2021;
Published: 07 May 2021.
Edited by:
Jeane E. L. Visentainer, State University of Maringá, BrazilReviewed by:
Christiane Ayo, Faculty of Medicine of São José do Rio Preto, BrazilLuís Cristóvão Porto, Rio de Janeiro State University, Brazil
Copyright © 2021 Amorim, Augusto, Nemat-Gorgani, Montero-Martin, Marin, Shams, Dandekar, Caillier, Parham, Fernández-Viña, Oksenberg, Norman and Hollenbach. This is an open-access article distributed under the terms of the Creative Commons Attribution License (CC BY). The use, distribution or reproduction in other forums is permitted, provided the original author(s) and the copyright owner(s) are credited and that the original publication in this journal is cited, in accordance with accepted academic practice. No use, distribution or reproduction is permitted which does not comply with these terms.
*Correspondence: Jill A. Hollenbach, jill.hollenbach@ucsf.edu
†These authors have contributed equally to this work and share first authorship