- 1Adult Bone Marrow Transplantation (BMT) Service, Division of Hematologic Malignancies, Memorial Sloan Kettering Cancer Center, New York, NY, United States
- 2Department of Medicine, Weill Cornell Medical College, New York, NY, United States
Older patients with hematologic malignancies are increasingly considered for allogeneic hematopoietic cell transplantation with encouraging outcomes. While aging-related thymic dysfunction remains a major obstacle to optimal and timely immune reconstitution post- transplantation, recent accumulating evidence has suggested that various aging hallmarks such as cellular senescence, inflamm-aging, and hematopoietic stem cell exhaustion, could also impact immune reconstitution post-transplantation in both thymic-dependent and independent manner. Here we review molecular and cellular aspects of immune senescence and immune rejuvenation related to allogeneic hematopoietic cell transplantation among older patients and discuss potential strategies for mechanism-based therapeutic intervention.
Introduction
In recent years, allogeneic hematopoietic cell transplantation (HCT), the original form of cellular immunotherapy, is increasingly utilized in older adults (1). This trend is likely due to the increasing incidence of hematologic malignancies in older adults; improved toxicity profiles of conditioning regimen, novel graft manipulation and graft-versus-host disease (GVHD) prevention strategies; as well as better selection, optimization, and supportive care of the geriatric patient (1–3). Importantly, outcomes for older patients in allogeneic HCT are improving gradually and many older patients are now routinely evaluated for and undergo the lifesaving procedure (1, 4–6).
One of the most important aspects of successful allogeneic HCT in older adults is the capacity for donor immune reconstitution, especially the optimal recovery of T-cell adaptive immunity critical for both anti-infection and anti-tumor activities (7, 8). However, in the context of an aging host, delayed immune reconstitution may be caused by age-related thymic atrophy/involution, suppressive inflammatory cytokine milieu, or HCT-associated complications such as infection or GVHD (9, 10). In this mini-review, we discuss immune reconstitution in the context of existing aging hallmarks and pathways, its clinical relevance to transplant outcomes, and opportunities for mechanism-based interventions to improve clinical outcomes for older patients.
Hallmarks of Aging and the Immune System
Recent development in the field of geroscience has provided a unique opportunity to examine the issue of immune reconstitution following HCT in older adults. The normal healthy aging process is defined by at least nine different and relatively conserved denominators of aging hallmarks including stem cell exhaustion, cellular senescence, mitochondrial dysfunction, epigenetic alterations, telomere attrition, genomic instability, altered intercellular communication, loss of proteostasis, and deregulated nutrient sensing (11). Perturbations or exacerbated responses in any of these pathways are known to cause pathologic states such as cancer, neurodegenerative disease, or cardiovascular disease (11, 12). We highlight here several of these hallmarks which are evident in the human immune system and more importantly, potential druggable targets for intervention to improve outcomes of older patients in allogeneic HCT.
Stem Cell Aging
There are many cell-intrinsic changes in hematopoietic stem cells (HSCs), innate and adaptive immune cells, as well as microenvironmental changes in the bone marrow, and primary and secondary lymphoid organs during aging (13). The first of which is the gradual age-related functional decline in the hematopoietic compartment, especially of HSCs. Aged HSCs exhibit impaired self-renewal and long-term reconstitution potential both in competitive and noncompetitive settings (14, 15), presumably due to the increased proliferative stress following HSC engraftment (16, 17). Furthermore, accumulating data indicate that decreased lymphoid production in the elderly is in part caused by cell-intrinsic alterations in lineage potential of HSCs and their committed progenitors as evident in numerous transcriptional and epigenetic profiling studies in aged HSCs from mice (17–20) and humans (21). At the molecular level, increased Wnt5a expression in HSCs induces elevated RhoGTPase Cdc42 signaling and alteration of histone H4K16 distribution, thereby mediating the aging phenotype (22, 23). Importantly, pharmacologic inhibition of Cdc42 activity, by Cdc42 activity-specific inhibitor (CASIN), can reverse the aging phenotype and reconstitute a “younger” immune system based on gene expression profiles and DNA vaccine responses in mouse transplantation models (24).
These preclinical studies have had significant implications in allogeneic HCT especially related to the age of donor HSCs. Several large registry studies have invariably demonstrated the positive impact of younger donor age on allo-HCT outcomes across matched unrelated donors and haploidentical donors (25, 26). Mechanistically, HCT from younger donors are associated with improved immune reconstitution post-HCT based on faster kinetics of CD4+ T cell (both naïve and memory), CD8+ T cell, B cell, and NK cell development, which translated to decreased non-relapse mortality and increased disease-free survival in several transplant platforms (27, 28). Interestingly, a recent study demonstrated that in the haploidentical transplantation setting, older donor age was associated with early alloreactivity and reduced numbers of CD45RA+ Treg in the graft and at immune recovery, suggesting an adverse impact of aging-related change in older donor HSCs (29). These clinical observations correlated well with early murine transplantation experiments demonstrating impaired immune reconstitution post-transplantation with older mice as donors (30, 31).
Cellular Senescence
A second important hallmark is cellular senescence, defined as a state of stable growth arrest once cells are subjected to significant stress and DNA damage. While this is an intrinsic host defensive mechanism to prevent cancer development, these senescent cells also accumulate in different organs and most importantly, secrete pro-inflammatory cytokine and adhesive molecules (32). This senescence-associated secretory phenotype (SASP) creates a pro-inflammatory milieu that is detrimental to the function of many host cells including the immune system (33). This is especially true for the bone marrow niche which is composed of several cell types that play important modulatory roles in both aging and the development of hematologic malignancies (34, 35). In an elegant murine study, Kusumbe et al., demonstrated a causal link between suppression of endothelial NOTCH signaling and the pro-inflammatory bone marrow milieu in aged mice (36). Restoration of NOTCH signaling was sufficient to ameliorate aging defects in the vascular niche essential for HSC maintenance. Moreover, both intrinsic and extrinsic mechanisms of aging are likely operating in the bone marrow niche. Kuribayashi et al. demonstrated that transplantation of aged HSCs in a young BM successfully recapitulated the transcriptional landscape of young HSCs but not their methylation profile, suggesting the presence of both instructive (BM milieu) and permissive (cell-intrinsic) contributors of HSC aging (37). In another study, infusion of young endothelial cells in aged or lethally irradiated mice enhanced HSC reconstitution and hematopoietic recovery by mitigating damage to the BM vascular microenvironment- exemplifying the role of BM microenvironment in HSC aging (38).
Cellular senescence also applies to the aging of T-cells, especially in the context of immune effector function (39). T-cell aging is characterized by the expression of senescence markers such as CD57 and the loss of CD28+, which is distinct from T-cell exhaustion characterized by the expression of checkpoint inhibitors such as PD-1 (40). These senescent T-cells have been associated with chronic viral infections, reduced IL-2 production, increased IL-6 production, and decreased anti-tumor effector activities (41, 42). Delayed T-cell immune reconstitution in older adults most likely results from age-associated thymic functional decline and the aging host environment in the bone marrow as well as other lymphoid organs (43, 44). Functionally, T-cell aging and delayed immune reconstitution affects T-cell immunity and in the context of allogeneic HCT, may affect the ability to defend against various infections and disease relapse.
Mitochondrial Dysfunction
Related to the aging of HSC and within the emerging field of immunometabolism lies another tenet of tissue aging: mitochondrial dysfunction. Although an association between these two processes has long been recognized (45, 46), the molecular pathways linking these cellular perturbations are poorly elucidated. Studies investigating bioenergetic utilization in aged HSCs have reported enhanced mitochondrial OXPHOS and increased ROS production (47, 48), thereby compromising HSC function. However, it remains unclear if high ROS levels are a trigger or a consequence of HSC dysfunction. Emerging studies have also attributed HSC aging to changes in the BM microenvironment, but the influence of niche factors on metabolic perturbations in HSCs, are yet to be explored. The integrity of mitochondrial DNA (mtDNA) can also influence HSC aging, as exemplified in DNA polymerase gamma (Polg) mutant mice deficient in proof-reading capacity, thereby harboring mutations in mtDNA in HSCs and exhibiting features of accelerated aging across numerous tissues including BM (49). Hematopoietic defects include progressive megaloblastic anemia, erythrodysplasia, and impaired de novo B and T cell lymphopoiesis, which are features characteristics of myelodysplastic syndromes (50). For further reading and a more comprehensive overview of the role of mitochondria in HSC function, please refer to the following reviews (51, 52).
Activated T cells undergo extensive intracellular metabolic rewiring thereby engaging/co-opting several mitochondrial processes (glycolysis, biogenesis, glutaminolysis, OXPHOS, one-carbon metabolism), to facilitate their high metabolic demand. Aged T cells (naïve CD4+) exhibit impaired mitochondrial adaptation and attenuation of cellular metabolism. Supplementation of glycine which restores one-carbon metabolism, was sufficient to partially rejuvenate aged T cells (53). In a more recent study (54), Tfam (encodes mitochondrial transcription factor) deficiency in mouse T cells disrupted mitochondrial integrity and induced inflammaging, resulting in increased senescence and recapitulating premature aging. Treatment with Nicotinamide riboside, a precursor of oxidized nicotinamide adenine dinucleotide (NAD+) and vitamin B3 analog, attenuated systemic inflammation and ameliorated multiple morbidities in mice with Tfam-deficient T cells (skeletal wasting, cardiovascular dysfunction, and restored normal physical activity). An emerging area of investigation of immunosenescence is the role of mitokines- mitochondrial stress-induced soluble factors (GDF15, FGF21, and human), which increase with aging and play an adaptive immunometabolic role to arrest the deleterious effects of aging (55). In the context of allo-HCT, it’s conceivable that primary and secondary effects of dysfunctional mitochondria in aged HSCs and lymphoid precursors confer reduced reconstitution potential, thereby delaying post-transplant immune reconstitution.
Epigenetic Aging and Telomeres
Finally, additional hallmarks of aging such as epigenetic alterations and telomere shortening have been implicated in post-transplant outcomes (56). Recently, several elegant clinical translational studies compared the biologic age of donor with that of the recipient age and showed that there was significant accelerated aging associated with HCT, manifested as a rapid increase of epigenetic age and telomere shortening (57, 58). Using a panel of DNA methylation-based, epigenetic aging biomarkers, Stolzerl et al. compared the biological age of transplant recipients with their donors and found that after a latency period of epigenetic rejuvenation in donor cells, accelerated epigenetic aging accounted for 2.4 years per chronologic year (59). Most importantly, both this study and an earlier study demonstrated that the donor age was the key determinant of DNA methylation-based, epigenetic aging, supporting again the benefit of using younger donors (59, 60). The mechanism of benefits from younger donors may be explained by their longer telomere length as shown in a CIBMTR study of allogeneic HCT recipients of severe aplastic anemia, where longer donor (rather than recipient) telomere length was associated with improved 5-year overall survival (61). The specific impact of epigenetic aging and telomere attrition on post-HCT immune reconstitution, however, has not been examined in these studies.
Immune Reconstitution Post-Transplant in Older Patients
Clinically speaking, immune reconstitution post allogeneic HCT in older patients follows similar pattern as younger patients, with both thymus-dependent and independent mechanisms (44, 62). However, the aging of the immune system especially the thymus and the bone marrow significantly impact the kinetics of immune reconstitution, resulting in suboptimal clinical outcomes among older patients post allogeneic HCT. For example, the effective reconstitution of adoptive T-cell immunity, especially a diverse CD4+ T-cell repertoire, requires a functional thymus (63). This is crucial in aging patients where thymic atrophy due to involution is common, resulting in impaired recovery and increased risks of infection among older patients. In contrast, CD8+ T-cells predominantly expand in the periphery thus may be less sensitive to the effect of aging (44, 62). Interestingly, a recent large-scale genome-wide association study has suggested that besides age and gender, a common variant (rs2204985) within the T cell receptor TCRA-TCRD locus is associated with increased thymopoiesis and T cell diversity in transplantation models (64). Moreover, infection with many human herpesviruses especially cytomegalovirus (CMV) has significant impact on the pattern and repertoire of overall T cell and CMV-specific immunity post-allogeneic HCT (65, 66). For further details of thymus-dependent mechanism underlying immune reconstitution and various enhancement strategies, the readers are referred to an excellent recent review (44).
Thymus-independent, peripheral T-cell expansion predominates at least during the first year when mature T cells from the graft are triggered to expand either via stimulation by host allo-antigens or through homeostatic proliferation in response to lymphopenia (62). As soon as a graft is infused into a recipient, mature T cells from the graft will encounter and respond to host allo-antigens, such as incompatible major and minor histocompatibility leukocyte antigens, as well as tumor-associated and tumor-specific antigens, which results in both GVHD and graft-versus-tumor (GvT) effect (62). However, the T-cell repertoire generated through a thymic-independent mechanism is limited in diversity and skewed (67). The effect of older age and the SASP environment on thymus-independent immune reconstitution post-HCT has not been specifically studied. However, some studies of early T-cell senescence post-HCT have suggested a potential modulatory role in immune reconstitution. For example, T lymphocytes express p16INK4a exponentially with increasing age, a tumor suppressor involved in cell cycle regulation. Wood et al. measured the expression of p16INK4a in hematopoietic stem cell–derived T cells as well as their cellular functioning both before and after allo-HCT. After transplantation, allogeneic HCT recipients demonstrated a significant increase (1.9-fold) in p16INK4a expression, equivalent to a decade of aging. Importantly, RNA sequencing of T cells both before and after HCT revealed changes in T cell subsets mimicking the changes that occur with normal aging (68).
The type of conditioning regimen and graft resource also play major role in immune reconstitution especially in older adults. Total body irradiation (TBI) is particularly damaging to the thymus in experimental models, and its use in older patients has been associated with increased mortality and treatment-related complications (7). Likewise, different graft sources are associated with different kinetics of immune reconstitution which leads to differential impact on relapse and non-relapsed mortality (69, 70). Both studies demonstrated that allografts from peripheral blood have faster overall kinetics of immune reconstitution than either bone marrow or cord blood, and that faster immune reconstitution of T-cells is associated with better survival outcomes. In addition, faster NK T-cell reconstitution is associated with a reduced incidence of relapse (69, 70).
Targeting Aging Host for Therapeutic Intervention
Strategies to improve immune reconstitution and HCT outcomes for older patients, we should start with an appropriate selection of younger donors, appropriate transplant platforms, and meticulous supportive care targeting geriatrics related issues peri-transplant. Traditionally, strategies aimed to enhance thymic function have been investigated to enhance post-transplant immune reconstitution, with molecules such as sex hormone ablation, KGF, interleukin-7, growth hormone, IL22, BMP4, RANKL under investigation (10, 44). Recent discoveries in geroscience, however, have stimulated additional interest in exploring therapeutic targets in aging pathways to improve outcomes in cancer therapy.
The contribution of age-related changes in the bone marrow microenvironment on HSC aging has been well studied (71). However, it was not until recently that its therapeutic potential was explored. In an endothelial transplantation experiment, Poulos et al. demonstrated that bone marrow endothelial cells (EC) supported HSC development and infusion of young ECs enhanced hematopoietic recovery following myelosuppressive therapy. Importantly, co-infusion of young ECs augmented aged HSC engraftment and enhanced survival of lethally irradiated mice by mitigating damage to the BM vascular microenvironment (38). These results have significant implications for older patients and immune reconstitution post-HCT. Early phase clinical trials are underway to explore the clinic impact of third party, genetically engineered endothelial cells in mitigating side effects of high dose chemotherapy and to promote HSC recovery post-HCT (NCT03925935).
Senolytics is a group of compounds with selective activity against senescence cells, with the potential to reverse the aging process (72). These compounds are yet to be tested in the setting of human or murine model of allo-HCT or immune reconstitution, although in a landmark study, Chang et al. showed that ABT263, a selective apoptosis inducer of senescence cells in culture when orally administrated to either sublethally irradiated or normally aged mice effectively depleted senescent bone marrow HSCs and mitigated TBI-induced premature aging of the hematopoietic system and rejuvenated the aged HSCs (73, 74). This study suggested that senolytic drugs especially ABT263 may represent a new class of radiation protectors while also stimulate immune reconstitution via rejuvenation of HSC post allo-HCT. Other senolytics drugs are in development as well, including some repurposed drugs and even cell therapy using chimeric antigen receptor T-cells (75, 76). One such combination is dasatinib plus quercertin (D+Q) which is clinically safe and has shown potential efficacy in a pulmonary fibrosis model of aging. Kirkland et al. launched the first clinical trial with D+Q to be given to survivors of HCT, a population prone to premature aging (NCT02652052).
Another possible target is SASP and its associated cytokine milieu (72). Notably, some agents which target SASP components are already used for certain disease applications and are potential candidates for drug repurposing. An IL‐1 receptor antagonist, anakinra, an anti‐IL‐6‐receptor antibody, tocilizumab, and tumor necrosis factor‐α inhibitors, such as etanercept and infliximab, are currently used to treat rheumatoid arthritis. As the IL‐1 receptor is a key inducer of many other SASP factors such as IL‐6 and IL‐8, these drugs may be useful for selectively blocking the deleterious impact of SASP (32). Targeted inhibition of the IL‐6 or IL‐8 signaling axis weakens the inflammatory response in murine models of senescence (77).
Another class of agents that could be useful in the allogeneic HCT setting is mTOR inhibitors, which has antiaging and immunomodulatory properties. In a classic murine model of ex vivo HSC expansion, mTOR inhibitor rapamycin promoted long term expansion and hematopoietic reconstitution via inhibition of HSC senescence (78, 79). The implication of the study in HCT remains to be explored, as the mTOR inhibitor sirolimus is a commonly used GVHD prevention medication. Even more interestingly, in randomized early-phase trials of older adults, combinatorial inhibition of the mTOR pathway using two synthetic inhibitors was effective in reducing rates of infections and enhancing immune functions as measured by influenza vaccination response and immune regulatory gene upregulation. On the cellular level, mTOR inhibition led to reduced numbers of CD4+ CD8+ T-cells expressing the exhaustion marker program-death 1 receptor (80, 81). Therefore, these agents are attractive compounds to stimulate immune reconstitution post HCT in older adults.
Conclusion
Strategies to improve immune reconstitution and transplant outcomes for older patients could include: an appropriate selection of younger donors, appropriate transplant platforms, and meticulous supportive care targeting geriatrics-related issues peri-transplant. Advances in aging science have provided opportunities to examine their impact on immune reconstitution post-transplant, as well as potential mechanism-based, therapeutic targets to improve outcomes. In Figure 1, we summarize these important areas of consideration and their druggable targets. Not only we are learning to better care for the older patient in allo-HCT, but we are also beginning to translate and apply the findings in aging science to improve outcomes of immune recovery post-transplantation. This will hopefully result in better outcomes for older patients undergoing allo-HCT.
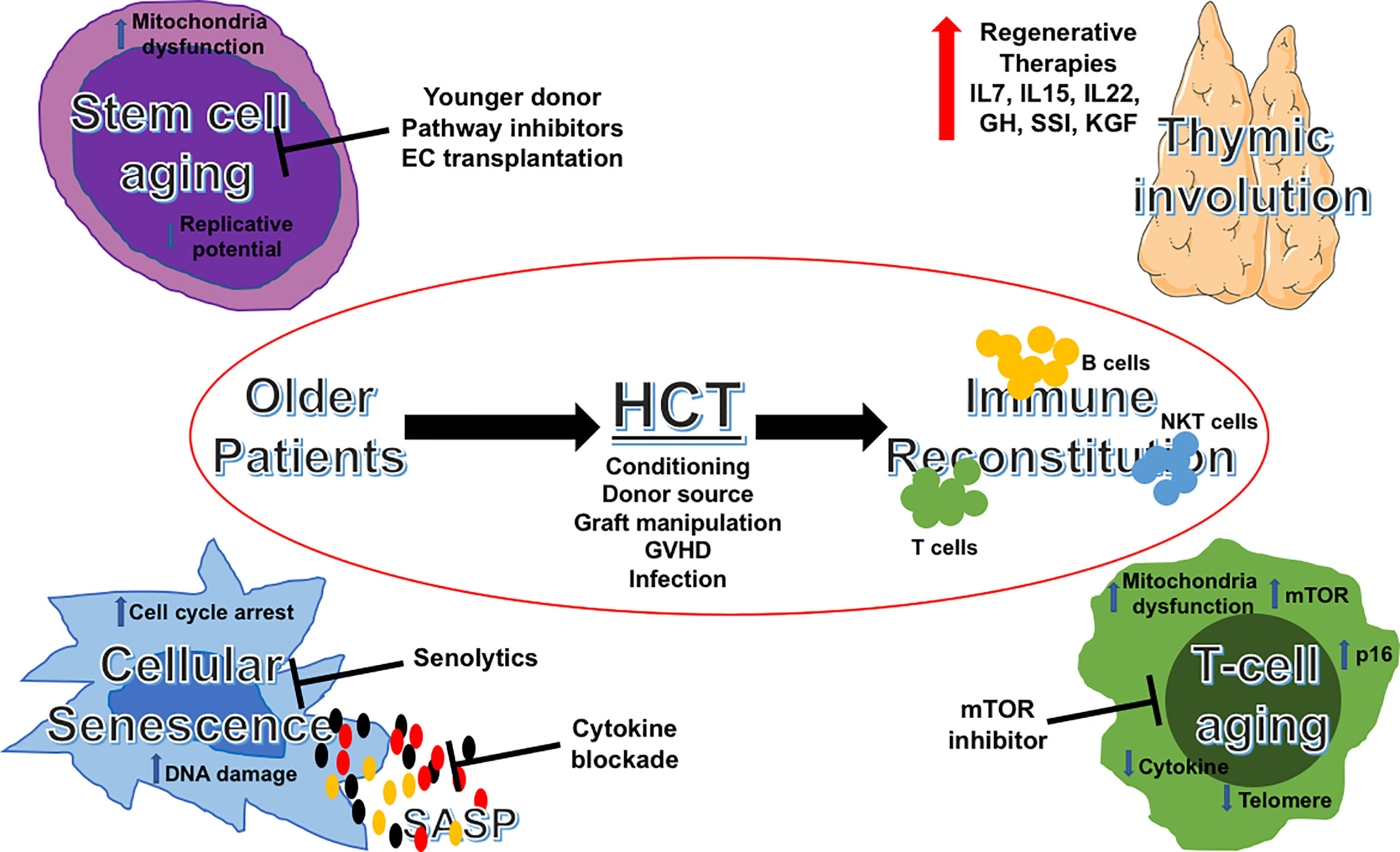
Figure 1 Aging pathways and potential therapeutic targets in allogeneic HCT for older patients. Schematic illustration of potential targetable aging pathways in allogeneic HCT for older patients. HCT, hematopoietic cell transplantation; IL, interleukins; GH, growth hormone; SSI, sex steroid inhibition; KGF, keratinocyte growth factor; GVHD, graft-versus-host disease; SASP, senescence-associated secretory phenotype; mTOR, mechanistic target of rapamycin.
Author Contributions
All authors contributed to the article and approved the submitted version.
Funding
This research was supported in part by the NIH/NCI Cancer Center Support Grant P30 CA008748 and the Program Project Grant P01 CA023766. The content is solely the responsibility of the authors and does not necessarily represent the official views of the National Institutes of Health.
Conflict of Interest
MB has received research support from Seres Therapeutics; has consulted, received honorarium from or participated in advisory boards for Seres Therapeutics, Flagship Ventures, Novartis, Evelo, Jazz Pharmaceuticals, Therakos, Amgen, Magenta Therapeutics, WindMIL Therapeutics, Merck & Co, Inc., Acute Leukemia Forum (ALF) and DKMS Medical Council (Board); has IP Licensing with Seres Therapeutics, and Juno Therapeutics. RL has served on the advisory board of Kite – a Gilead Company.
The remaining author declares that the research was conducted in the absence of any commercial or financial relationships that could be construed as a potential conflict of interest.
Acknowledgments
We thank Sheena Kapoor for administrative assistance.
References
1. D’Souza A, Fretham C, Lee SJ, Arora M, Brunner J, Chhabra S, et al. Current Use of and Trends in Hematopoietic Cell Transplantation in the United States. Biol Blood Marrow Transplant (2020) 26(8):e177–e82. doi: 10.1016/j.bbmt.2020.04.013
2. Rosko A, Artz A. Aging: Treating the Older Patient. Biol Blood Marrow Transplant (2017) 23(2):193–200. doi: 10.1016/j.bbmt.2016.11.007
3. Jayani R, Rosko A, Olin R, Artz A. Use of Geriatric Assessment in Hematopoietic Cell Transplant. J Geriatr Oncol (2020) 11(2):225–36. doi: 10.1016/j.jgo.2019.09.012
4. Muffly L, Pasquini MC, Martens M, Brazauskas R, Zhu X, Adekola K, et al. Increasing Use of Allogeneic Hematopoietic Cell Transplantation in Patients Aged 70 Years and Older in the United States. Blood (2017) 130(9):1156–64. doi: 10.1182/blood-2017-03-772368
5. Sorror ML, Sandmaier BM, Storer BE, Franke GN, Laport GG, Chauncey TR, et al. Long-Term Outcomes Among Older Patients Following Nonmyeloablative Conditioning and Allogeneic Hematopoietic Cell Transplantation for Advanced Hematologic Malignancies. JAMA (2011) 306(17):1874–83. doi: 10.1001/jama.2011.1558
6. McClune BL, Weisdorf DJ, Pedersen TL, Tunes da Silva G, Tallman MS, Sierra J, et al. Effect of Age on Outcome of Reduced-Intensity Hematopoietic Cell Transplantation for Older Patients With Acute Myeloid Leukemia in First Complete Remission or With Myelodysplastic Syndrome. J Clin Oncol (2010) 28(11):1878–87. doi: 10.1200/JCO.2009.25.4821
7. Chaudhry MS, Velardi E, Malard F, van den Brink MR. Immune Reconstitution After Allogeneic Hematopoietic Stem Cell Transplantation: Time to T Up the Thymus. J Immunol (2017) 198(1):40–6. doi: 10.4049/jimmunol.1601100
8. Elfeky R, Lazareva A, Qasim W, Veys P. Immune Reconstitution Following Hematopoietic Stem Cell Transplantation Using Different Stem Cell Sources. Expert Rev Clin Immunol (2019) 15(7):735–51. doi: 10.1080/1744666X.2019.1612746
9. Gaballa A, Clave E, Uhlin M, Toubert A, Arruda LCM. Evaluating Thymic Function After Human Hematopoietic Stem Cell Transplantation in the Personalized Medicine Era. Front Immunol (2020) 11:1341. doi: 10.3389/fimmu.2020.01341
10. Simons L, Cavazzana M, Andre I. Concise Review: Boosting T-Cell Reconstitution Following Allogeneic Transplantation-Current Concepts and Future Perspectives. Stem Cells Transl Med (2019) 8(7):650–7. doi: 10.1002/sctm.18-0248
11. Lopez-Otin C, Blasco MA, Partridge L, Serrano M, Kroemer G. The Hallmarks of Aging. Cell (2013) 153(6):1194–217. doi: 10.1016/j.cell.2013.05.039
12. Campisi J, Kapahi P, Lithgow GJ, Melov S, Newman JC, Verdin E. From Discoveries in Ageing Research to Therapeutics for Healthy Ageing. Nature (2019) 571(7764):183–92. doi: 10.1038/s41586-019-1365-2
13. Sadighi Akha AA. Aging and the Immune System: An Overview. J Immunol Methods (2018) 463:21–6. doi: 10.1016/j.jim.2018.08.005
14. Elias HK, Bryder D, Park CY. Molecular Mechanisms Underlying Lineage Bias in Aging Hematopoiesis. Semin Hematol (2017) 54(1):4–11. doi: 10.1053/j.seminhematol.2016.11.002
15. de Haan G, Lazare SS. Aging of Hematopoietic Stem Cells. Blood (2018) 131(5):479–87. doi: 10.1182/blood-2017-06-746412
16. Wolf NS, Priestley GV, Averill LE. Depletion of Reserve in the Hemopoietic System: III. Factors Affecting the Serial Transplantation of Bone Marrow. Exp Hematol (1983) 11(8):762–71.
17. Rossi DJ, Bryder D, Zahn JM, Ahlenius H, Sonu R, Wagers AJ, et al. Cell Intrinsic Alterations Underlie Hematopoietic Stem Cell Aging. Proc Natl Acad Sci U S A (2005) 102(26):9194–9. doi: 10.1073/pnas.0503280102
18. Chambers SM, Shaw CA, Gatza C, Fisk CJ, Donehower LA, Goodell MA. Aging Hematopoietic Stem Cells Decline in Function and Exhibit Epigenetic Dysregulation. PloS Biol (2007) 5(8):e201. doi: 10.1371/journal.pbio.0050201
19. Sun D, Luo M, Jeong M, Rodriguez B, Xia Z, Hannah R, et al. Epigenomic Profiling of Young and Aged HSCs Reveals Concerted Changes During Aging That Reinforce Self-Renewal. Cell Stem Cell (2014) 14(5):673–88. doi: 10.1016/j.stem.2014.03.002
20. Abdel-Fatah TM, Russell R, Albarakati N, Maloney DJ, Dorjsuren D, Rueda OM, et al. Genomic and Protein Expression Analysis Reveals Flap Endonuclease 1 (FEN1) as a Key Biomarker in Breast and Ovarian Cancer. Mol Oncol (2014) 8(7):1326–38. doi: 10.1016/j.molonc.2014.04.009
21. Pang WW, Price EA, Sahoo D, Beerman I, Maloney WJ, Rossi DJ, et al. Human Bone Marrow Hematopoietic Stem Cells are Increased in Frequency and Myeloid-Biased With Age. Proc Natl Acad Sci U S A (2011) 108(50):20012–7. doi: 10.1073/pnas.1116110108
22. Florian MC, Dorr K, Niebel A, Daria D, Schrezenmeier H, Rojewski M, et al. Cdc42 Activity Regulates Hematopoietic Stem Cell Aging and Rejuvenation. Cell Stem Cell (2012) 10(5):520–30. doi: 10.1016/j.stem.2012.04.007
23. Florian MC, Nattamai KJ, Dorr K, Marka G, Uberle B, Vas V, et al. A Canonical to non-Canonical Wnt Signalling Switch in Haematopoietic Stem-Cell Ageing. Nature (2013) 503(7476):392–6. doi: 10.1038/nature12631
24. Leins H, Mulaw M, Eiwen K, Sakk V, Liang Y, Denkinger M, et al. Aged Murine Hematopoietic Stem Cells Drive Aging-Associated Immune Remodeling. Blood (2018) 132(6):565–76. doi: 10.1182/blood-2018-02-831065
25. Kollman C, Spellman SR, Zhang MJ, Hassebroek A, Anasetti C, Antin JH, et al. The Effect of Donor Characteristics on Survival After Unrelated Donor Transplantation for Hematologic Malignancy. Blood (2016) 127(2):260–7. doi: 10.1182/blood-2015-08-663823
26. Canaani J, Savani BN, Labopin M, Huang XJ, Ciceri F, Arcese W, et al. Donor Age Determines Outcome in Acute Leukemia Patients Over 40 Undergoing Haploidentical Hematopoietic Cell Transplantation. Am J Hematol (2018) 93(2):246–53. doi: 10.1002/ajh.24963
27. Baron F, Storer B, Maris MB, Storek J, Piette F, Metcalf M, et al. Unrelated Donor Status and High Donor Age Independently Affect Immunologic Recovery After Nonmyeloablative Conditioning. Biol Blood Marrow Transplant (2006) 12(11):1176–87. doi: 10.1016/j.bbmt.2006.07.004
28. Gonzalez-Vicent M, Molina B, Deltoro N, Sevilla J, Vicario JL, Castillo A, et al. Donor Age Matters in T-cell Depleted Haploidentical Hematopoietic Stem Cell Transplantation in Pediatric Patients: Faster Immune Reconstitution Using Younger Donors. Leuk Res (2017) 57:60–4. doi: 10.1016/j.leukres.2017.03.001
29. Jaiswal SR, Bhakuni P, Joy A, Murli N, Rajoreya A, Chakrabarti A, et al. Higher CD45RA(+) Regulatory T Cells in the Graft Improves Outcome in Younger Patients Undergoing T Cell-Replete Haploidentical Transplantation: Where Donor Age Matters. Biol Blood Marrow Transplant (2018) 24(10):2025–33. doi: 10.1016/j.bbmt.2018.06.003
30. Hirayama M, Azuma E, Jiang Q, Kobayashi M, Iwamoto S, Kumamoto T, et al. The Reconstitution of CD45RBhiCD4+ Naive T Cells is Inversely Correlated With Donor Age in Murine Allogeneic Haematopoietic Stem Cell Transplantation. Br J Haematol (2000) 111(2):700–7. doi: 10.1046/j.1365-2141.2000.02391.x
31. Azuma E, Hirayama M, Yamamoto H, Komada Y. The Role of Donor Age in Naive T-cell Recovery Following Allogeneic Hematopoietic Stem Cell Transplantation: The Younger the Better. Leuk Lymphoma (2002) 43(4):735–9. doi: 10.1080/10428190290016827
32. Gorgoulis V, Adams PD, Alimonti A, Bennett DC, Bischof O, Bishop C, et al. Cellular Senescence: Defining a Path Forward. Cell (2019) 179(4):813–27. doi: 10.1016/j.cell.2019.10.005
33. Chen Y, Liu S, Leng SX. Chronic Low-grade Inflammatory Phenotype (CLIP) and Senescent Immune Dysregulation. Clin Ther (2019) 41(3):400–9. doi: 10.1016/j.clinthera.2019.02.001
34. Lazzari E, Butler JM. The Instructive Role of the Bone Marrow Niche in Aging and Leukemia. Curr Stem Cell Rep (2018) 4(4):291–8. doi: 10.1007/s40778-018-0143-7
35. Kovtonyuk LV, Fritsch K, Feng X, Manz MG, Takizawa H. Inflamm-Aging of Hematopoiesis, Hematopoietic Stem Cells, and the Bone Marrow Microenvironment. Front Immunol (2016) 7:502. doi: 10.3389/fimmu.2016.00502
36. Kusumbe AP, Ramasamy SK, Itkin T, Mae MA, Langen UH, Betsholtz C, et al. Age-Dependent Modulation of Vascular Niches for Haematopoietic Stem Cells. Nature (2016) 532(7599):380–4. doi: 10.1038/nature17638
37. Kuribayashi W, Oshima M, Itokawa N, Koide S, Nakajima-Takagi Y, Yamashita M, et al. Limited Rejuvenation of Aged Hematopoietic Stem Cells in Young Bone Marrow Niche. J Exp Med (2021) 218(3). doi: 10.1084/jem.20192283
38. Poulos MG, Ramalingam P, Gutkin MC, Llanos P, Gilleran K, Rabbany SY, et al. Endothelial Transplantation Rejuvenates Aged Hematopoietic Stem Cell Function. J Clin Invest (2017) 127(11):4163–78. doi: 10.1172/JCI93940
39. Goronzy JJ, Weyand CM. Mechanisms Underlying T Cell Ageing. Nat Rev Immunol (2019) 19(9):573–83. doi: 10.1038/s41577-019-0180-1
40. Huff WX, Kwon JH, Henriquez M, Fetcko K, Dey M. The Evolving Role of CD8(+)CD28(-) Immunosenescent T Cells in Cancer Immunology. Int J Mol Sci (2019) 20(11):1–22. doi: 10.3390/ijms20112810
41. Vallejo AN, Weyand CM. Goronzy JJ. T-Cell Senescence: A Culprit of Immune Abnormalities in Chronic Inflammation and Persistent Infection. Trends Mol Med (2004) 10(3):119–24. doi: 10.1016/j.molmed.2004.01.002
42. Strioga M, Pasukoniene V, Characiejus D. Cd8+ CD28- and CD8+ Cd57+ T Cells and Their Role in Health and Disease. Immunology (2011) 134(1):17–32. doi: 10.1111/j.1365-2567.2011.03470.x
43. Small TN, Avigan D, Dupont B, Smith K, Black P, Heller G, et al. Immune Reconstitution Following T-cell Depleted Bone Marrow Transplantation: Effect of Age and Posttransplant Graft Rejection Prophylaxis. Biol Blood Marrow Transplant (1997) 3(2):65–75.
44. Velardi E, Tsai JJ, van den Brink MRM. T Cell Regeneration After Immunological Injury. Nat Rev Immunol (2020) 23:1–15. doi: 10.1038/s41577-020-00457-z
45. Trifunovic A, Wredenberg A, Falkenberg M, Spelbrink JN, Rovio AT, Bruder CE, et al. Premature Ageing in Mice Expressing Defective Mitochondrial DNA Polymerase. Nature (2004) 429(6990):417–23. doi: 10.1038/nature02517
46. Kujoth GC, Hiona A, Pugh TD, Someya S, Panzer K, Wohlgemuth SE, et al. Mitochondrial DNA Mutations, Oxidative Stress, and Apoptosis in Mammalian Aging. Science (2005) 309(5733):481–4. doi: 10.1126/science.1112125
47. Ito K, Hirao A, Arai F, Takubo K, Matsuoka S, Miyamoto K, et al. Reactive Oxygen Species Act Through P38 MAPK to Limit the Lifespan of Hematopoietic Stem Cells. Nat Med (2006) 12(4):446–51. doi: 10.1038/nm1388
48. Mohrin M, Shin J, Liu Y, Brown K, Luo H, Xi Y, et al. Stem Cell Aging. A Mitochondrial UPR-mediated Metabolic Checkpoint Regulates Hematopoietic Stem Cell Aging. Science (2015) 347(6228):1374–7. doi: 10.1126/science.aaa2361
49. Norddahl GL, Pronk CJ, Wahlestedt M, Sten G, Nygren JM, Ugale A, et al. Accumulating Mitochondrial DNA Mutations Drive Premature Hematopoietic Aging Phenotypes Distinct From Physiological Stem Cell Aging. Cell Stem Cell (2011) 8(5):499–510. doi: 10.1016/j.stem.2011.03.009
50. Chen ML, Logan TD, Hochberg ML, Shelat SG, Yu X, Wilding GE, et al. Erythroid Dysplasia, Megaloblastic Anemia, and Impaired Lymphopoiesis Arising From Mitochondrial Dysfunction. Blood (2009) 114(19):4045–53. doi: 10.1182/blood-2008-08-169474
51. Nakamura-Ishizu A, Ito K, Suda T. Hematopoietic Stem Cell Metabolism During Development and Aging. Dev Cell (2020) 54(2):239–55. doi: 10.1016/j.devcel.2020.06.029
52. Filippi MD, Ghaffari S. Mitochondria in the Maintenance of Hematopoietic Stem Cells: New Perspectives and Opportunities. Blood (2019) 133(18):1943–52. doi: 10.1182/blood-2018-10-808873
53. Ron-Harel N, Notarangelo G, Ghergurovich JM, Paulo JA, Sage PT, Santos D, et al. Defective Respiration and One-Carbon Metabolism Contribute to Impaired Naive T Cell Activation in Aged Mice. Proc Natl Acad Sci U S A (2018) 115(52):13347–52. doi: 10.1073/pnas.1804149115
54. Desdin-Mico G, Soto-Heredero G, Aranda JF, Oller J, Carrasco E, Gabande-Rodriguez E, et al. T Cells With Dysfunctional Mitochondria Induce Multimorbidity and Premature Senescence. Science (2020) 368(6497):1371–6. doi: 10.1126/science.aax0860
55. Conte M, Martucci M, Chiariello A, Franceschi C, Salvioli S. Mitochondria, Immunosenescence and Inflammaging: A Role for Mitokines? Semin Immunopathol (2020) 42(5):607–17. doi: 10.1007/s00281-020-00813-0
56. Cupit-Link MC, Arora M, Wood WA, Hashmi SK. Relationship Between Aging and Hematopoietic Cell Transplantation. Biol Blood Marrow Transplant (2018) 24(10):1965–70. doi: 10.1016/j.bbmt.2018.08.015
57. Thornley I, Sutherland R, Wynn R, Nayar R, Sung L, Corpus G, et al. Early Hematopoietic Reconstitution After Clinical Stem Cell Transplantation: Evidence for Stochastic Stem Cell Behavior and Limited Acceleration in Telomere Loss. Blood (2002) 99(7):2387–96. doi: 10.1182/blood.V99.7.2387
58. Wynn RF, Cross MA, Hatton C, Will AM, Lashford LS, Dexter TM, et al. Accelerated Telomere Shortening in Young Recipients of Allogeneic Bone-Marrow Transplants. Lancet (1998) 351(9097):178–81. doi: 10.1016/S0140-6736(97)08256-1
59. Stolzel F, Brosch M, Horvath S, Kramer M, Thiede C, von Bonin M, et al. Dynamics of Epigenetic Age Following Hematopoietic Stem Cell Transplantation. Haematologica (2017) 102(8):e321–e3. doi: 10.3324/haematol.2016.160481
60. Weidner CI, Ziegler P, Hahn M, Brummendorf TH, Ho AD, Dreger P, et al. Epigenetic Aging Upon Allogeneic Transplantation: The Hematopoietic Niche Does Not Affect Age-Associated DNA Methylation. Leukemia (2015) 29(4):985–8. doi: 10.1038/leu.2014.323
61. Gadalla SM, Wang T, Haagenson M, Spellman SR, Lee SJ, Williams KM, et al. Association Between Donor Leukocyte Telomere Length and Survival After Unrelated Allogeneic Hematopoietic Cell Transplantation for Severe Aplastic Anemia. JAMA (2015) 313(6):594–602. doi: 10.1001/jama.2015.7
62. Ogonek J, Kralj Juric M, Ghimire S, Varanasi PR, Holler E, Greinix H, et al. Immune Reconstitution After Allogeneic Hematopoietic Stem Cell Transplantation. Front Immunol (2016) 7:507. doi: 10.3389/fimmu.2016.00507
63. Miller J. The Function of the Thymus and its Impact on Modern Medicine. Science (2020) 369(6503). doi: 10.1126/science.aba2429
64. Clave E, Araujo IL, Alanio C, Patin E, Bergstedt J, Urrutia A, et al. Human Thymopoiesis is Influenced by a Common Genetic Variant Within the TCRA-TCRD Locus. Sci Transl Med (2018) 10(457). doi: 10.1126/scitranslmed.aao2966
65. Itzykson R, Robin M, Moins-Teisserenc H, Delord M, Busson M, Xhaard A, et al. Cytomegalovirus Shapes Long-Term Immune Reconstitution After Allogeneic Stem Cell Transplantation. Haematologica (2015) 100(1):114–23. doi: 10.3324/haematol.2014.113415
66. Annaloro C, Serpenti F, Saporiti G, Galassi G, Cavallaro F, Grifoni F, et al. Viral Infections in HSCT: Detection, Monitoring, Clinical Management, and Immunologic Implications. Front Immunol (2020) 11:569381. doi: 10.3389/fimmu.2020.569381
67. Mackall CL, Fleisher TA, Brown MR, Andrich MP, Chen CC, Feuerstein IM, et al. Age, Thymopoiesis, and CD4+ T-Lymphocyte Regeneration After Intensive Chemotherapy. N Engl J Med (1995) 332(3):143–9. doi: 10.1056/NEJM199501193320303
68. Wood WA, Krishnamurthy J, Mitin N, Torrice C, Parker JS, Snavely AC, et al. Chemotherapy and Stem Cell Transplantation Increase P16(INK4a) Expression, a Biomarker of T-cell Aging. EBioMedicine (2016) 11:227–38. doi: 10.1016/j.ebiom.2016.08.029
69. Waller EK, Logan BR, Fei M, Lee SJ, Confer D, Howard A, et al. Kinetics of Immune Cell Reconstitution Predict Survival in Allogeneic Bone Marrow and G-CSF-mobilized Stem Cell Transplantation. Blood Adv (2019) 3(15):2250–63. doi: 10.1182/bloodadvances.2018029892
70. Ando T, Tachibana T, Tanaka M, Suzuki T, Ishiyama Y, Koyama S, et al. Impact of Graft Sources on Immune Reconstitution and Survival Outcomes Following Allogeneic Stem Cell Transplantation. Blood Adv (2020) 4(2):408–19. doi: 10.1182/bloodadvances.2019001021
71. Zhang L, Mack R, Breslin P, Zhang J. Molecular and Cellular Mechanisms of Aging in Hematopoietic Stem Cells and Their Niches. J Hematol Oncol (2020) 13(1):157. doi: 10.1186/s13045-020-00994-z
72. Birch J, Gil J. Senescence and the SASP: Many Therapeutic Avenues. Genes Dev (2020) 34(23-24):1565–76. doi: 10.1101/gad.343129.120
73. Chang J, Wang Y, Shao L, Laberge RM, Demaria M, Campisi J, et al. Clearance of Senescent Cells by ABT263 Rejuvenates Aged Hematopoietic Stem Cells in Mice. Nat Med (2016) 22(1):78–83. doi: 10.1038/nm.4010
74. Tchkonia T, Zhu Y, van Deursen J, Campisi J, Kirkland JL. Cellular Senescence and the Senescent Secretory Phenotype: Therapeutic Opportunities. J Clin Invest (2013) 123(3):966–72. doi: 10.1172/JCI64098
75. Kirkland JL, Tchkonia T. Cellular Senescence: A Translational Perspective. EBioMedicine (2017) 21:21–8. doi: 10.1016/j.ebiom.2017.04.013
76. Amor C, Feucht J, Leibold J, Ho YJ, Zhu C, Alonso-Curbelo D, et al. Senolytic CAR T Cells Reverse Senescence-Associated Pathologies. Nature (2020) 583(7814):127–32. doi: 10.1038/s41586-020-2403-9
77. Watanabe S, Kawamoto S, Ohtani N, Hara E. Impact of Senescence-Associated Secretory Phenotype and its Potential as a Therapeutic Target for Senescence-Associated Diseases. Cancer Sci (2017) 108(4):563–9. doi: 10.1111/cas.13184
78. Luo Y, Li L, Zou P, Wang J, Shao L, Zhou D, et al. Rapamycin Enhances Long-Term Hematopoietic Reconstitution of Ex Vivo Expanded Mouse Hematopoietic Stem Cells by Inhibiting Senescence. Transplantation (2014) 97(1):20–9. doi: 10.1097/TP.0b013e3182a7fcf8
79. Chen C, Liu Y, Liu Y, Zheng P. mTOR Regulation and Therapeutic Rejuvenation of Aging Hematopoietic Stem Cells. Sci Signal (2009) 2(98):ra75. doi: 10.1126/scisignal.2000559
80. Mannick JB, Del Giudice G, Lattanzi M, Valiante NM, Praestgaard J, Huang B, et al. mTOR Inhibition Improves Immune Function in the Elderly. Sci Transl Med (2014) 6(268):268ra179. doi: 10.1126/scitranslmed.3009892
Keywords: aging, cellular senescence, mitochondrial dysfunction, immune reconstitution, allogeneic hematologic stem cell transplantation
Citation: Lin RJ, Elias HK and van den Brink MRM (2021) Immune Reconstitution in the Aging Host: Opportunities for Mechanism-Based Therapy in Allogeneic Hematopoietic Cell Transplantation. Front. Immunol. 12:674093. doi: 10.3389/fimmu.2021.674093
Received: 28 February 2021; Accepted: 30 March 2021;
Published: 19 April 2021.
Edited by:
John R. Greenland, University of California, San Francisco, United StatesReviewed by:
Antoine Toubert, Université Paris Diderot, FranceMichael Uhlin, Karolinska Institutet (KI), Sweden
Copyright © 2021 Lin, Elias and van den Brink. This is an open-access article distributed under the terms of the Creative Commons Attribution License (CC BY). The use, distribution or reproduction in other forums is permitted, provided the original author(s) and the copyright owner(s) are credited and that the original publication in this journal is cited, in accordance with accepted academic practice. No use, distribution or reproduction is permitted which does not comply with these terms.
*Correspondence: Marcel R. M. van den Brink, dmFuZGVuYm1AbXNrY2Mub3Jn