- 1Respiratory and Critical Care Unit, 1st Medical Center of Chinese Chinese People’s Liberation Army (PLA) General Hospital, Beijing, China
- 2Department of Internal Medicine, Hubei Province Corps Hospital of The Chinese Armed Police Force (CAPF), Wuhan, China
- 3Pulmonary and Critical Care Medicine College of Chinese PLA General Hospital, 8th Medical Center of Chinese PLA General Hospital, Beijing, China
- 4Department of Internal Medicine, Tianjin Municipal Corps Hospital of CAPF, Tianjin, China
- 5Department of Dermatology, 1st Medical Center of Chinese PLA General Hospital, Beijing, China
- 6Department of Pathology, 8th Medical Center of Chinese PLA General Hospital, Beijing, China
Eosinophilic asthma (EA) is a common subtype of asthma and often progresses to severe disease. In order to understand its pathogenesis, targeted next-generation gene sequencing was performed on 77 Chinese EA patients and 431 Chinese healthy controls to obtain differential genomic variations. Among the 41 Single Nucleotide Polymorphisms (SNPs) screened for mutation sites in more than 3 patients, filaggrin gene FLG rs192116923 T>G and FLG rs75235053 C>G were newly found to be associated with EA patients with atopic dermatitis (AD) (P <0.001) and severe EA (P=0.032), respectively. Filaggrin has been shown to be mainly expressed in epithelial cells and plays an important role in formation of an effective skin barrier. Bioinformatic analysis indicated FLG rs192116923 T>G may increase the binding of Smad3 to transmit TGF-β1 signaling, and thereby inhibit filaggrin expression, and FLG rs75235053 C>G may add new splicing sites to reduce filaggrin monomers. It has been known that the level of Th2 cytokine IL-4 is increased in EA patients, and IL-4 increases airway epithelial permeability and enhances inflammatory response through some unclear mechanisms. To figure out whether filaggrin is involved in immune responses in asthma, we have treated human respiratory epithelial cell line BEAS-2B cells with IL-4 and found that the expression levels of filaggrin and E-cadherin decreased significantly in a time and dose-dependent manner, suggesting that IL-4 increased airway epithelial permeability by reducing filaggrin and adhesion molecule. In addition, in our study, IL-4 increased the expression of epithel-derived inflammatory cytokines IL-33 and TSLP which further enhanced the Th2 inflammatory response. To investigate the role of filaggrin in development of EA, knockdown filaggrin with siRNA revealed a decrease in E-cadherin levels, which were further down-regulated by IL-4 stimulation. Knockdown of filaggrin alone did not affect the levels of IL-33 and TSLP, but further exacerbated the decrease of IL-33/TSLP caused by IL-4, suggesting that filaggrin may involve in IL-4R signaling pathway to regulate the level of IL-33/TSLP. In conclusion, in the Th2 cytokine milieu of asthma, FLG deficient mutation in airway epithelial cells may increase the epithelial permeability and the expression of IL-33/TSLP which positively feedback the Th2 inflammation response.
Introduction
Asthma is a heterogeneous disease in which genetics and the environment work together. In previous reports, it has been proved that epigenetics, respiratory infections, diet and other factors affect the onset of asthma. Genetic component is substantial, with estimates of heritability ranging from 35% to 95% in asthma (1). As of 2006, prior to publication of the first asthma genome-wide association study (GWAS), candidate gene studies had implicated more than 100 genes in the etiology of asthma (2). There are 38 loci or genomic regions available in GWAS Catalog associated with asthma nowadays (3). In the post-GWAS era, more studies focused on genomics related to asthma sub-phenotypes (4–6) or a certain clinical feature or asthma pharmacogenetics (7, 8). These studies can reduce heterogeneity, identify new asthma risk loci, even in smaller samples (9), and be more useful for elucidating the genetics and etiology of asthma.
Eosinophilic asthma (EA) refers to a clinical inflammatory phenotype of asthma with a significant number of sputum, airway, and/or blood eosinophils, and approximately half of asthmatics in this category. Recognizing EA is not only helpful for identifying patients who will benefit from inhaled corticosteroids (ICS), but also for identifying patients who will be suitable for the biologic therapies targeted eosinophilia and/or related inflammatory pathways in severe asthma patients (10). In our previous report, we described the clinical characteristics and biomarkers in different asthma subtypes. However, genomic analysis on EA has rarely been reported until now which need further exploration. Targeted next-generation sequencing (targeted region sequencing, TRS) technology combined microarray technology with next-generation sequencing has advantages of efficiency, accuracy, low-cost, high-coverage, and capability to discover new mutation sites. This technology has high clinical application values and is suitable for the detection of large number of clinical samples, and has been extensively used in tumor researches and clinical trials (11). Thus, we performed TRS on the peripheral blood of EA patients to determine the genomic variations [mainly including SNPs and Insertion-Deletions (InDels)] by comparing with healthy controls, analyzed their association with clinical characteristics and regulatory mechanisms to explore the molecular basis of the pathogenesis of EA. Among these screened SNPs, FLG rs192116923 T>G allele was found for the first time to be associated with EA patients with atopic dermatitis (AD). In addition, FLG rs75235053 C>G allele and GSTM1 rs412302 G>A allele were associated with severe EA.
Filaggrin (FLG) mainly expresses in epidermal tissues and plays an important role in the formation of the stratum corneum and the maintenance of epithelial barrier. FLG loss-of-function mutations firstly found in ichthyosis vulgaris patients were related to AD or eczema, food allergy, asthma and hay fever (12). Recent GWAS reports found FLG loss-of-function mutations were closely related to childhood-onset and severity of asthma (13). It has been reported that filaggrin can be depressed by Th2 cytokines such as IL-4 which play an important role in the pathogenesis of asthma and AD (14). Besides, filaggrin deficiency has been proved being correlated with the increasing of the epithelial cell–derived cytokines, thymic stromal lymphopoietin (TSLP) and IL-33, which are critical mediators of T helper type 2 inflammation (15–17). In this report we demonstrated that filaggrin constitutively expressed in the human bronchial epithelial cell line BEAS-2B cells. To evaluate the function of filaggrin in bronchial cells upon the on-set of asthma, we simulated the cytokine environment in asthma with Th2 cytokine IL-4. Then we found that the expressions of filaggrin and E-cadherin were reduced while IL-33 and TSLP were increased by IL-4 inducing. Furthermore, filaggrin deficiency probably enhanced Th2 inflammatory response by reducing the expressing of E-cadherin and inducing the expression of IL-33 and TSLP with IL-4 inducing. Corresponding to the genetics analysis of EA patient, we conjectured that the loss-of-function mutation of FLG probably results in the reduction of the adhesion molecule E-cadherin and the secretion of type 2 cytokines (TSLP and IL-33) in bronchial epithelial cells which may result in the on-set or exacerbation of asthma.
Methods and Materials
Patient Selection
From November 2016 to May 2019, 77 EA patients were recruited from the Department of Respiratory, Chinese PLA general hospital, Beijing. In addition to meeting the asthma criteria in Global Initiative for Asthma (GINA) 2020 (18), patients should also meet the following inclusion criteria: The percentage of eosinophils in induced sputum is ≥ 3%, and the percentage of neutrophils in induced sputum is ≤61% (19); and/or the blood eosinophils count is ≥0.3×109/L; and/or fractional exhaled nitric oxide (FeNO) is ≥35ppb (20). Patients with acute infections, other respiratory diseases, tumors, liver and kidney dysfunction, etc. should be excluded. The present study was approved by the Human Research Ethics Committee of the Chinese PLA general hospital, and all participants provided written informed consent.
Observable Clinical Characteristics and Definitions
(i) Early-onset (<12 years) and late-onset (≥12 years) EA (21). (ii) Allergic [allergic sensitization and symptoms in response to allergen exposure (allergic sensitization: specific IgE demonstrated by skin prick-puncture tests or specific IgE in vitro tests to at least one of an appropriate panel of allergens)] (22) and non-allergic EA. (iii) Frequent exacerbations (with 2 or more asthma exacerbations leading to emergency room visit or hospitalization within the last 12 months) (23) and infrequent exacerbations of EA. (iv) Combined AD [also known as atopic eczema, eczema, which often occur in families with atopic diseases (atopic dermatitis, asthma, and/or allergic rhino-conjunctivitis). The clinical dermatologists confirmed the diagnosis of AD] (24). (v) Severe [high dose of ICS plus a second controller (and/or systemic corticosteroids) required to prevent asthma from becoming uncontrolled or still uncontrolled] (25) and mild-moderate eosinophilic asthma [well controlled with GINA (18) Step 1-3 treatment with as-needed reliever medication monotherapy or low dose of controller treatment, such as ICS, leuko-triene receptor antagonists (LTRA)].
Target Region Sequencing
Target Region Sequencing (TRS) is a technique of next generation sequencing (NGS) which captures targeted genes or specific regions of the genome. In this study, genomic DNA samples were extracted from peripheral blood of 77 EA patients. An asthma disease probe (Agilent, USA) is 491.178 kb, designed to capture the exon regions (including the 10 bp flanking on either side) and upstream regions (1 kb) of 109 asthma candidate genes (Table S1) frequently involved in literatures including several large-scale GWAS studies. Samples with index tag were pair-end sequence simultaneously on Illumina HiSeq 2500 Sequencer (Illumina, San Diego, CA) for 150 cycles. FASTQ sequencing files were aligned with human genome assembly GRCh37 using Burrows-Wheeler Aligner (BWA,26), processed using Picard to mark duplicates package and utilizing GATK 3.0 Haplotype Caller to call the raw variants. The data received had an average read depth of more than 300-fold coverage in more than 98% of the targeted regions.
Variation Filtering and Annotation
Based on the alignment results, SAM tools were applied to identify SNP sites. SNPs were annotated using ANNOVAR software. The public databases, including the dbSNP147 database, 1000 Genome Project, NHLBI-ESP Project (esp6500si), ClinVar and GnomAD (The Genome Aggregation Database), were used to screen the variants. The functional effect prediction was evaluated using SIFT, Polyphen 2, Mutation Taster, CADD score. InDel mutations in gene coding or splicing regions were likely to alter protein translation. Non-integer three-fold frameshift InDel mutations may cause changes in the entire reading frame and have an impact on gene function. Filtering and annotation methods are roughly the same as SNP. All variants were assigned in American College of Medical Genetics and Genomics standards and guidelines (26).
Bioinformatics Analysis
Polyphen 2, SIFT score, Mutation Taster, and CADD were used to predict whether the SNP was harmful mutation. HaploReg v4.1 (https://pubs.broadinstitute.org/mammals/haploreg/haploreg.php), Regulome RB online analysis (http://www.regulomedb.org/), SNPinfo (https://snpinfo.niehs.nih.gov/) and SNP2TFBS (https://ccg.epfl.ch/snp2tfbs/snpviewer.php) were used to predict the gene regulation or biological function of SNPs. Alamut Visual 2.12 software was used to predict the effect of SNPs on splicing of precursor mRNA.
Cell Culture and Cell Induction Conditions
Immortalized human keratinocyte HaCaT cells and BEAS-2B (human bronchial epithelium cells transformed with Ad12-SV40 2B) cells (Fenghui Bio Inc., Hunan, China) were cultured in DMEM medium (HyClone, China) containing 10% fetal bovine serum (Gibco, USA), 100 U/ml penicillin, and 100 μg/ml streptomycin (Solarbio, China), at 37°C with 5% CO2 and 95% humidity. The experimental cells were divided into the control group (phosphate buffer saline (PBS), Solarbio), IL-4 (R&D Systems China) 25 ng/ml, 50 ng/ml, 100 ng/ml induction group and the induction duration was 12h, 24 h and 48h, respectively (27). In the stimulation experiments, cells were cultured in serum-free medium.
Knockdown of Filaggrin Using siRNA Transfection
Three sequences of filaggrin siRNA and a siRNA negative control were designed and synthetized, as follows: siRNA-307 (sense GCACAGUCAUCAUGAUAAACA, antisense UUUAUCAUGAUGACUGUG CUU), siRNA-485 (sense GGAUAUUCACCUACUCAUAGA, antisense UAUGAGUAGGUGAAUAUCCU U), siRNA-5581 (sense CAGAAGUGUCAGCAGACAAAC, antisense UUGUCUGCUGACACUUCUGGA), and siRNA-NC (sense UUCUCCGAACGUGUCACGUTT, antisense ACGUGACACGUUCGGAGAATT). BEAS-2B cells were selected with good growth status, and were inoculated into 6-well plate, adjusting the cells to 2×105 per well. A mixture of 5 μL lipofectamine 6000TM (Beyotime, China), 100 pmol siRNA and 125 μl DMEM serum-free medium (HyClone) were added and incubated for 20 min at room temperature. Then each well was added with 250 μl siRNA-Lipo6000TM mixed culture medium. Six hours after transfection, the medium in the wells was replaced with DMEM complete medium, and the culture was continued for 48 h. Then cells were incubated with IL-4 or control for another 24 hours and then collected for analysis.
Real-Time Quantitative RT-PCR Analysis
Real-time quantitative RT-PCR was performed to quantitatively estimate the mRNA expression of filaggrin and measure the filaggrin knock-down efficiency, and estimate the mRNA expression of TSLP, IL33, filaggrin, and E-cadherin. Total RNA from cultured HaCaT and BEAS-2B cells was isolated using Trizol reagent (Invitrogen) according to the published method. The purified RNA was reverse transcribed to cDNA by using the PrimeScript RT reagent Kit (Takara) according to the manufacturer’s instruction. MA-686 Real-time PCR Instrument (Molarray, Suzhou, China) was used to analyze quantitative RT-PCR according to the manufacturer’s instruction. Primer sequences were as follows: Filaggrin: forward: 5’-CTCATCACAGCCACACCACATCC-3’, reverse: 5’-GCCGTCTCCTGATTGTTCATCGT TA-3’; TSLP: forward: 5’-GCTACTCAGGCAATGAAGAAGAGGAG-3’, reverse: 5’-CCTTGTAATTGTGACAC TTGTTCCAGAC-3’; IL33: forward: 5’-GGCATTCTTACACGAGGAAGTGAAGT-3’, reverse: 5’-TGTCTCATC TAGGCTCTGGTAGGTT-3’; E-cadherin: forward: 5’-TCCAGCTTCACCATCAAAGTT-3’, reverse: 5’-TTCCACCA CGATCTCATACCT-3’; GAPDH: forward: 5’-TCAAGAAGGTGGTGAAGCAGG-3’, reverse: 5’-GCGTCAAA GGTGGAGGAGTG-3’. The reaction system was: 2× Real-time PCR Master Mix 10 μL, 20 μM primers 0.8 μL, cDNA template 2 μL, 5 U/μl rTaq DNA polymerase 0.4 μL and ddH2O 6.8 μL. The following PCR condition were used: 95°C for 3 min, 95°C for 30 s, 55°C for 20 s, 40 cycles, and 72°C for 20 s. This experiment was carried out in triplicate and independently repeated at least three times. The expression levels were normalized to an endogenous control, GAPDH. The formula RQ = 2-ΔΔCt was used to calculate, and 2-ΔΔCt was used to express the difference in gene transcript expression.
Western Blotting Analysis
Whole BEAS-2B cell extracts were prepared in RIPA lysis buffer (Beyotime) for Western blotting and centrifuged at 14,000 rpm for 15 min at 4°C. The supernatants were then obtained and protein concentrations were detected using the BCA protein quantification kit (Beyotime). The protein extracts were loaded and separated by SDS-PAGE and then transferred to PVDF membranes (Millipore). The membranes were incubated overnight at 4°C with specific primary antibodies. Then, membranes were incubated with secondary antibodies and visualized using the Tanon 5200 automatic chemiluminescence image analysis system (Science Technology Co., LTD). The primary antibodies used for immunoblotting were as follows: E-cadherin (1:500, Cell Signaling Technology), filaggrin (1:500, Affinity), IL-4R (1:500, Affinity)GAPDH (1:5000, Cell Signaling Technology).
Immunofluorescence Staining
Cells cultured were fixed with 4% paraformaldehyde and kept overnight at 4°C, permeabilized with PBS containing 10% goat serum for 1 h at room temperature to block non-specific protein interactions. Rabbit anti-filaggrin primary polyclonal antibody (1: 500, Abcam) was diluted with PBS at 4°C overnight. The cells were washed in PBS and then incubated with 594-conjugated AffiniPure donkey anti-rabbit secondary antibody IgG (H+L) (Jackson, 1:300) at 4°C overnight. After washing the cells with PBS, DAPI nuclear staining was performed for 3 min. After PBS was washed again, the cells were imaged using confocal microscopy (Leica Microsystems, Wetzlar, Germany).
Immunohistochemistry
The paraffin sections of human normal bronchia, lung and skin were provided by the department of pathology, 8th Medical Center of Chinese PLA General Hospital with the approval of the patients. The slices were processed as the protocol previously described (28) and then dipped into rabbit anti human filaggrin antibody (Affbiotech, Jiangsu, China) for over 2 hours at room temperature. Rabbit IgG before immunization and antigen absorbent immune serum were applied as negative controls. After three washes in PBS, the slices were incubated with HRP-conjugated goat anti-rabbit IgG (ZSGB-BIO, Beijing, China) for 30 min at room temperature. Then antibody complexes were visualized by incubation with DAB chromogen. As the protocol, sections were counterstained with Mayer’s hematoxylin for 10 sec, dehydrated through gradient ethanol, cleared in dimethyl benzene, mounted, and examined using light microscopy (Olympus,Tokyo,Japan).
ELISA
Concentrations of released IL-33 and TSLP in the cell supernatants were assessed. We used the human IL-33 ELISA Kit (Meimian Biology, China) and the human TSLP ELISA kit (Meimian Biology, China). Standard reagents were prepared according to the instructions, and the standard dilutions and cell culture supernatant were added to the detection wells. The diluted antibody was added and incubated at room temperature for 2 h. Labeled streptavidin (1:100 dilution) 100 μl and chromogenic substrate 100 μl were added to each well, and incubated in the dark for 30 min. Within 30 minutes after the color change, a microplate reader (BioTek Epoch, USA) was used to detect the dual wavelength and the optical density (OD) value at the reference wavelength was determined. Regression fitting was used to generate a standard curve, and the OD value was linearly analyzed.
Statistical Analysis
Statistical analysis was performed using SPSS 20.0(SPSS Inc., Chicago, IL, USA) and GraphPad Prism 8.0 software (GraphPad Software Inc., San Diego, CA, USA). On the SHEsis platform (http://analysis.bio-x.cn/myAnalysis.php), Hardy-Weinberg equilibrium test was performed on the gene distribution of each SNP locus in 77 patients and 431 healthy controls in Chinese internal database, and linkage disequilibrium between SNPs was also detected. Data were expressed as the mean ± standard deviation (SD) with normal distribution or median and interquartile range (IQR) without normal distribution. Significance of differences between the groups was evaluated with independent samples Student’t t -testand LSD analysis was used for multiple comparisons between the two groups. The Pearson chi-square test or Fisher’s exact test was used for the comparison between two categorical variables. All statistical tests were set to two-way test with a test level of α=0.05.
Results
Differential SNPs Detected by Case-Control Association Analysis of EA Patients
Clinical baseline data and classified clinical characteristics distribution of EA patients were shown in Table 1. The median age of EA patients was 48 (from 36 to 54) years-old and there were 38 (49.4%) female patients. There was no significant difference in the median age and sex ratio of EA group and healthy control group. The proportion of patients with a history of smoking was 42.9%. The percentage of patients with allergic rhinitis (AR) and AD was 68.8% and 37.7%, respectively. The median duration of asthma or wheezing at baseline was 9 (from 3 to 19) years. The number of early- and late-onset EA patients was 16 and 61, respectively. All of the early-onset EA patients were also allergic. The number of allergic and non-allergic EA patients was 70 and 7, respectively. And the number of mild-moderate and severe EA patients was 20 and 57, respectively. Moreover, the median frequency of asthma exacerbations in the past 1 year was 2, while the median asthma control test (ACT) score was 17. There were 32 and 45 EA patients with frequent and infrequent exacerbations, respectively. The number of EA patients with and without AD was 29 and 48, respectively. According to the laboratory data, the median FeNO and total serum IgE was 44 (25-75) ppb and 229 (88.7-590) IU/ml, respectively. The median percent of peripheral blood eosinophils was 4.8% (3.5%-8.9%). The median percent of induced sputum eosinophils was 3% (0.5%-8%).
In association analysis of allele frequencies of 77 EA patients and 431 healthy individuals in Chinese internal database excluding sites that did not meet the H-W equilibrium, we detected 63 single nucleotide variants (SNVs). Of these variants, we detected 28 promoter variants, 34 exonic variants, 1 splicing variant. Four of them were newly discovered SNPs. Meanwhile, seven missense SNPs (ADAM8 rs553707790, TGFB1 rs943475580, NLRP3 rs188623199, IL25 rs770223596, ATG7 rs555273544, IRF c.G628A, IL13 rs770488630) were most likely pathogenic variants. A small number of patients (less than 4) were selected out for mutation sites, and 41 SNPs were obtained (Table 2). These SNPs had linkage disequilibrium: HLA-G rs3823321 and rs14722477, HLA-G rs1130356 and rs1049033, CCL11 rs17735961 and rs1129844, SPINK5 rs2303063 and rs2303062, MS4A2 rs1441585, rs574700 and rs569108, CCL2 rs2857656 and rs4586 (r2≥0.8). After all, all the sequencing data of the 77 EA patients have been uploaded to GenBank with the Bioproject ac number PRJNA718105 (https://dataview.ncbi.nlm.nih.gov/object/PRJNA718105?reviewer=djfq3k2vpjeb5uvi8loaag0d3f).
Correlation Between Genomic Variations and Clinical Characteristics of EA Patients
Correlation analysis was performed between genomic variations and clinical characteristics of EA patients. There were several SNPs associated with clinical characteristics of categorical and continuous variables of EA patients, shown in Table 3. FLG rs75235053 C>G allele (P=0.032, OR 4.121 comparing CG and CC genotype) and GSTM1 rs412302 G>A allele (P=0.016, OR 9.500 comparing AA+AG and GG genotype) were associated with severe EA. Besides, another FLG mutation FLG rs192116923 T>G allele was associated with EA patients with AD (P <0.001, OR 5.983 comparing GT + GG and TT genotype). Besides, SPINK5 rs2303063 G>A allele was linked to early-onset allergic EA (P=0.011, OR 6.344 comparing GA and GG genotype), and TLR4 rs75048248 C>T allele was associated with frequent-exacerbation EA (P = 0.018, OR 10.154 comparing CT and CC genotype).
We also indentified statistically significant associations of some SNPs and blood eosiniphil count and IgE level. Among them, CTLA4 rs5742909 C>T allele was positively correlated with serum total IgE of EA patients (P =0.036), GSTM1 rs412302 G>A allele was positively correlated with blood eosinophil count of EA patients (P =0.047), and LTC4S rs3776944 G>A allele was negatively correlated with blood eosinophil percentage of EA patients (P =0.001).
Bioinformatics Analysis of the Biological Function of FLG exon SNPs
Since FLG rs75235053 C>G and rs192116923 T>G were associated with severe EA and EA patients with AD, respectively, their biological functions were tested by bioinformatics. FLG encodes the filaggrin precursor protein profilaggrin, which contains 4061 amino acids. Its protein code is mainly in the third exon region, and mutations are also mainly distributed in this region. Rs192116923 T >G is a missense mutation p. Glu 2652 Asp, while rs75235053 C >G is a missense mutation p. Ser 3662 Thr, and both of them are anticipated by Polyphen 2, SIFT score, Mutation Taster, and CADD as non-harmful mutations. HaploReg v4.1 (https://pubs.broadinstitute.org/mammals/haploreg/haploreg.php) predicts that rs192116923-T mutation to -G can modify its binding motif to Smad3, and SNP2TFBS (https://ccg.epfl.ch/snp2tfbs/snpviewer.php) predicts that it can bind to Smad2_Smad3_Smad4, whereas PROMO suggests that rs75235053-C mutation to -G may reduce the binding to c-Jun.
IL-4 Decreased the Expression of Filaggrin and E-Cadherin, but Increased the Expression of IL-33 and TSLP in Time- and Dose-Dependent Manner
In this study, filaggrin has been proved constitutively expressed in human bronchial epithelial cell line BEAS-2B cells via RT-PCR and IF detection, and mainly distributed in the cytoplasm (Figure 1). Most importantly, in this study, it has been confirmed that filaggrin highly expressed in human normal bronchial epithelial cells and relatively lowerly expressed in alveolar epithelial cells in vivo (Figure 2).
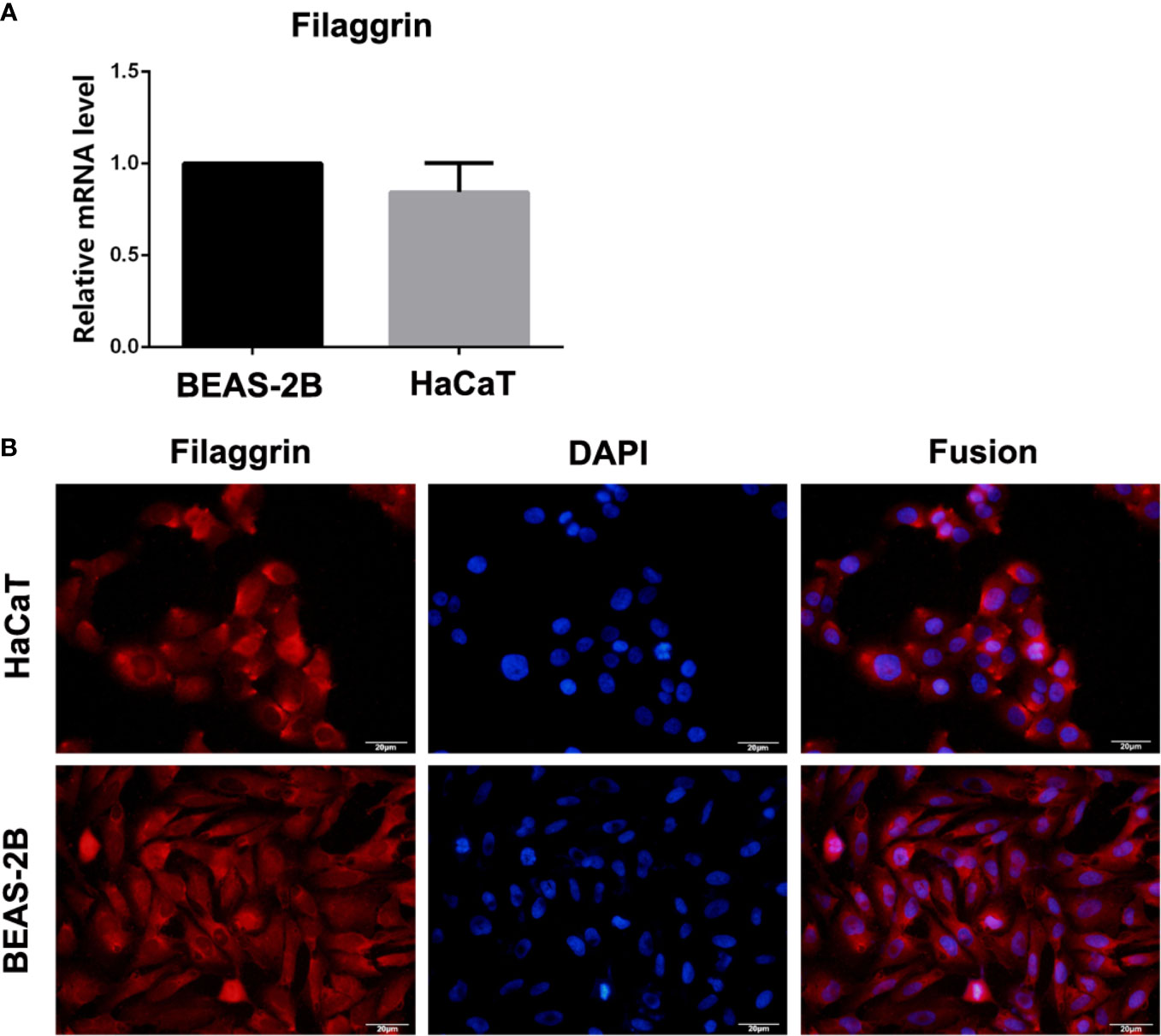
Figure 1 The mRNA and protein expression of filaggrin in BEAS-2B and HaCaT cells. (A) Filaggrin mRNA expression in HaCaT cells and BEAS-2B cells. (B) Immunofluorescence (IF) results of filaggrin in BEAS-2B and HaCaT cells. The photographs were magnified of 400 times and the bars represented 20μm.
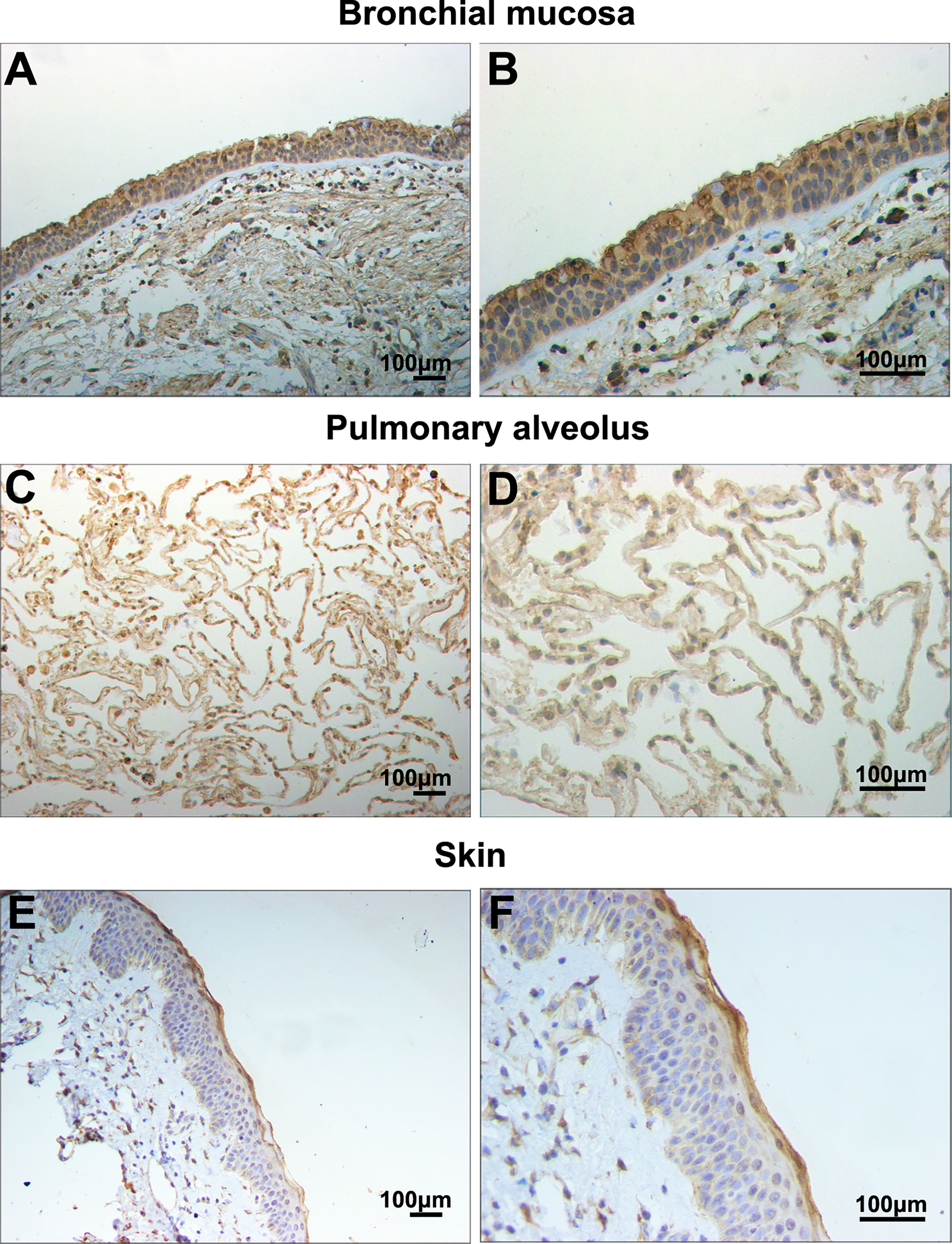
Figure 2 Filaggrin was expressed in normal human bronchial epithelial cells and alveolar epithelial cells. Identification of filaggrin expression in normal human bronchial mucosa (representative example of n=6), pulmonary alveolus (representative example of n=5) and skin tissues (representative example of n=3) by immunohistochemistry (IHC). Magnification of (A, C, E):100×; (B, D, F):200×. Bar=100μm.
Then we found that the Th2 cytokine IL-4 reduced the mRNA and protein expressions of filaggrin and E-cadherin in BEAS-2B cells. E-cadherin is the main adhesion junction protein of airway epithelium. Moreover, filaggrin and E-cadherin were gradually down-regulated as the stimulation period prolonged (Figures 3, 4) or the concentration of IL-4 increased (Figures 5 and 6). In Figures 3 and 4, it was shown that the expression of E-cadherin was not significantly increased until 24h incubation of IL-4 and the protein expression of filaggrin was not statistically change after 24h incubation of IL-4. Thus we chose 24h incubation period for the next step of experiments.
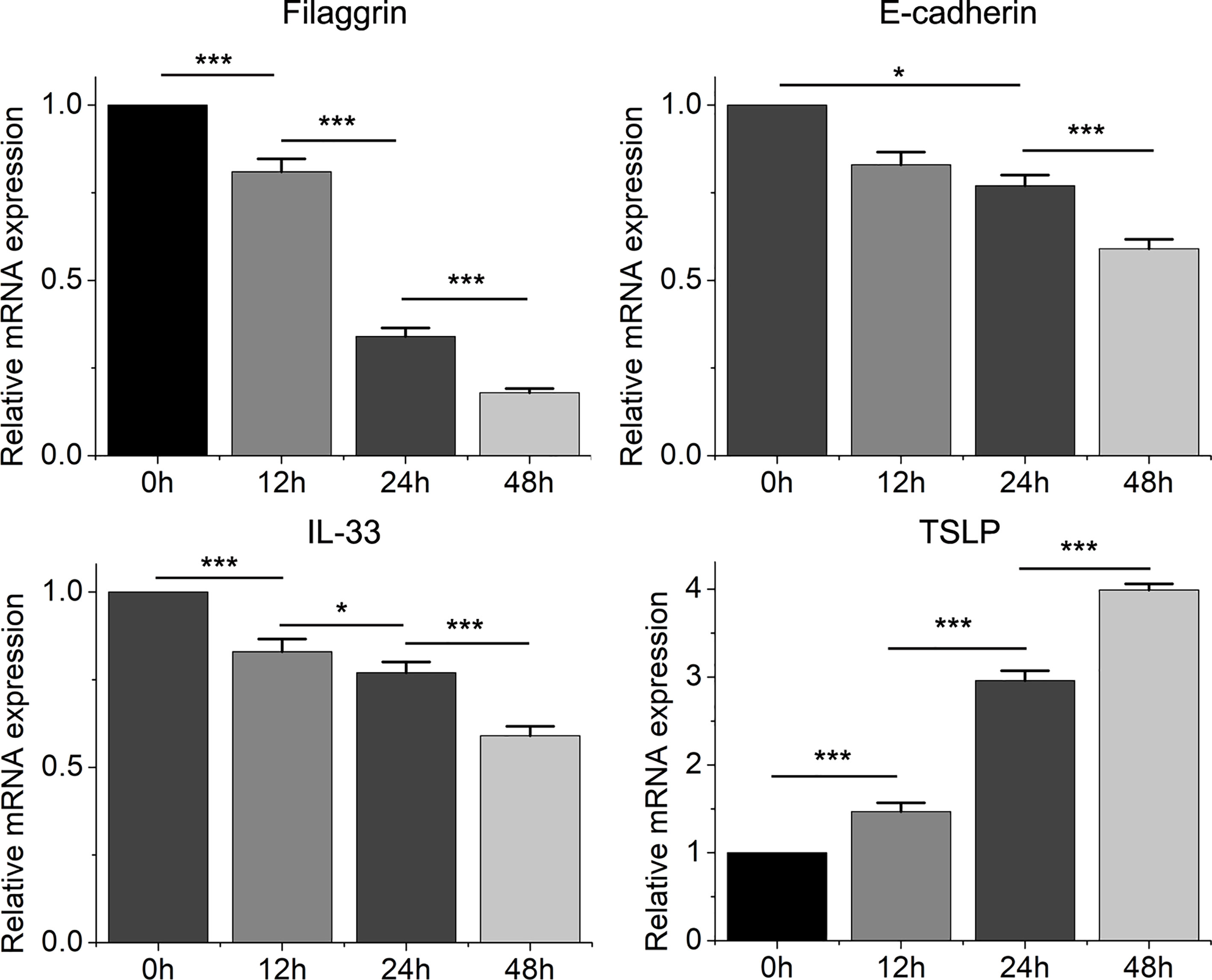
Figure 3 The mRNA expression of filaggrin, E-cadherin, IL-33 and TSLP in BEAS-2B cells with IL-4 treatment for different periods. Quantitative data are presented as the mean ± standard deviation (n=3). The Student’s t-test was used to analyze the difference between groups. *P < 0.05 was considered as statistically significant. ***P < 0.001.
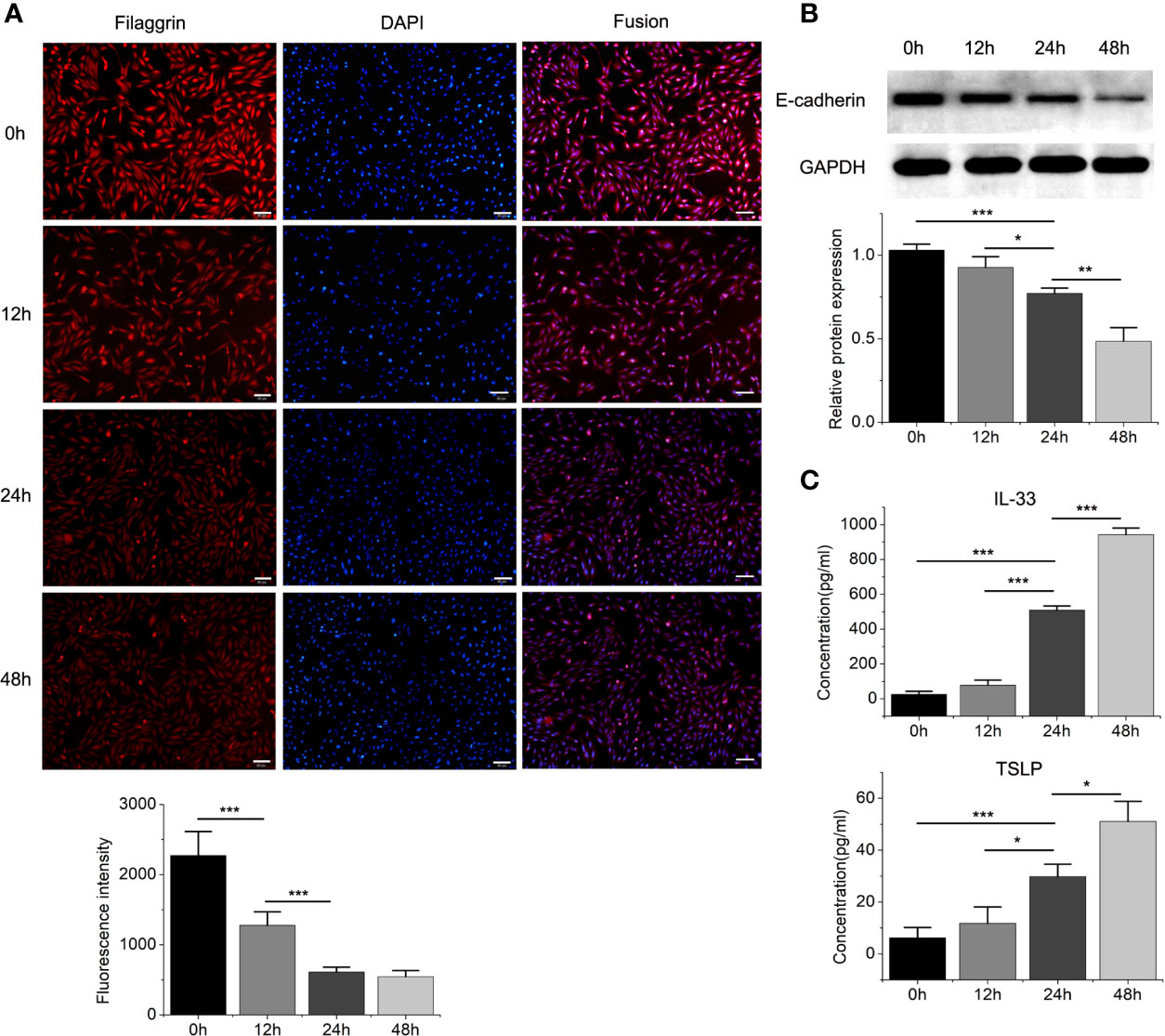
Figure 4 The protein expressions of filaggrin, E-cadherin, TSLP and IL-33 of BEAS-2B cells with IL-4 incubating for different periods. (A) The protein expression of filaggrin in BEAS-2B cells treated with IL-4 for different periods was detected by Immunofluorescence. The photographs were magnified of 200 times and the bars represented 50μm. (B) The protein expression of E-cadherin in BEAS-2B cells treated with IL-4 for different periods was detected by Western-blot. (C) IL-33 and TSLP in the cell culture supernatants with IL-4 treatment for different periods were detected by ELISA. Each experiment has been repeated for 3 times and presented as the mean ± SD (n=3). The Student’s t-test was used to analyze the difference between groups. *P < 0.05 was considered as statistically significant. **P < 0.01, ***P < 0.005.
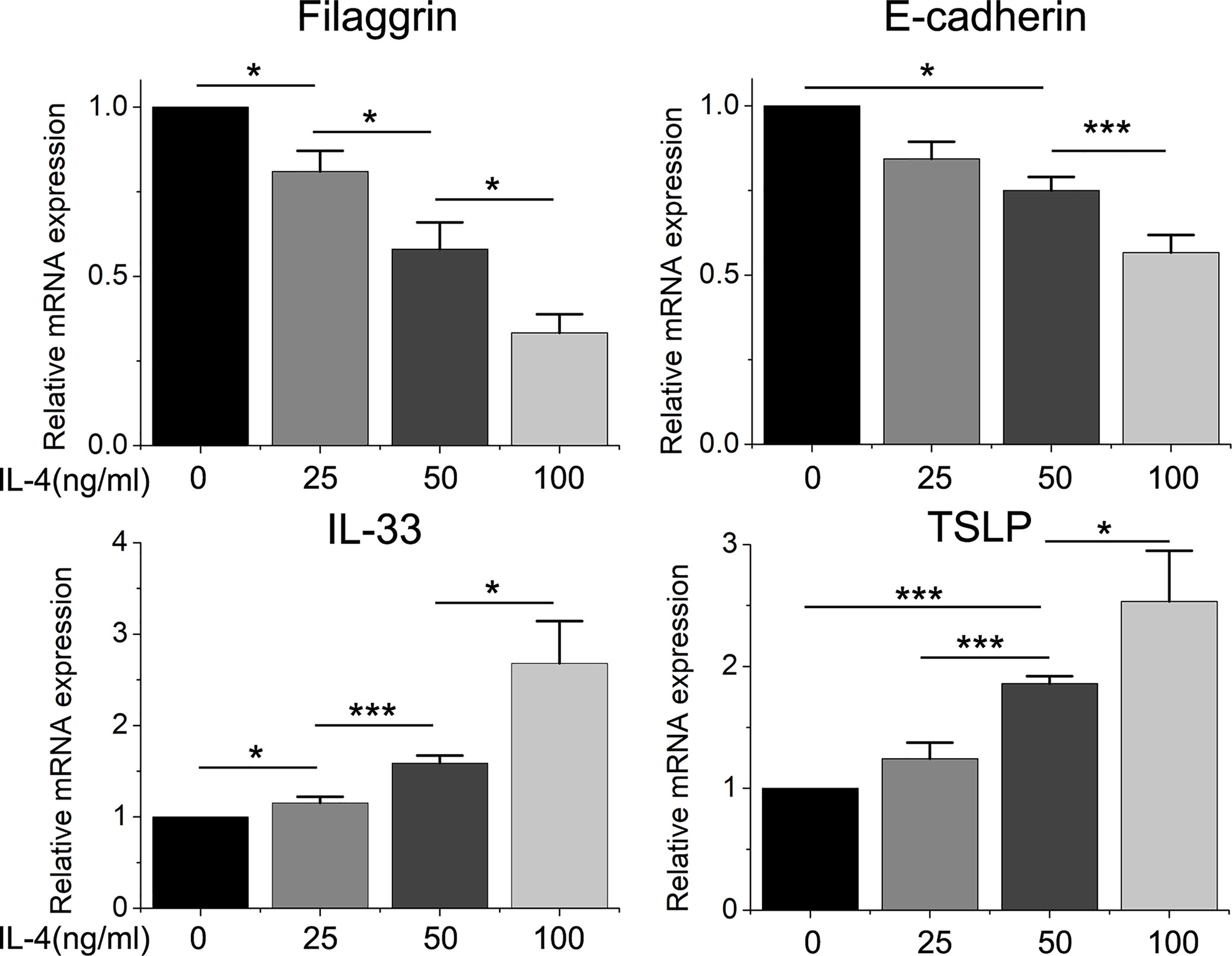
Figure 5 The mRNA expression of filaggrin, E-cadherin, IL-33 and TSLP in BEAS-2B cells treated with different concentration of IL-4. Quantitative data are presented as the mean ± standard deviation (n=3). The Student’s t-test was used to analyze the difference between groups. *P < 0.05 was considered as statistically significant. ***P < 0.005.
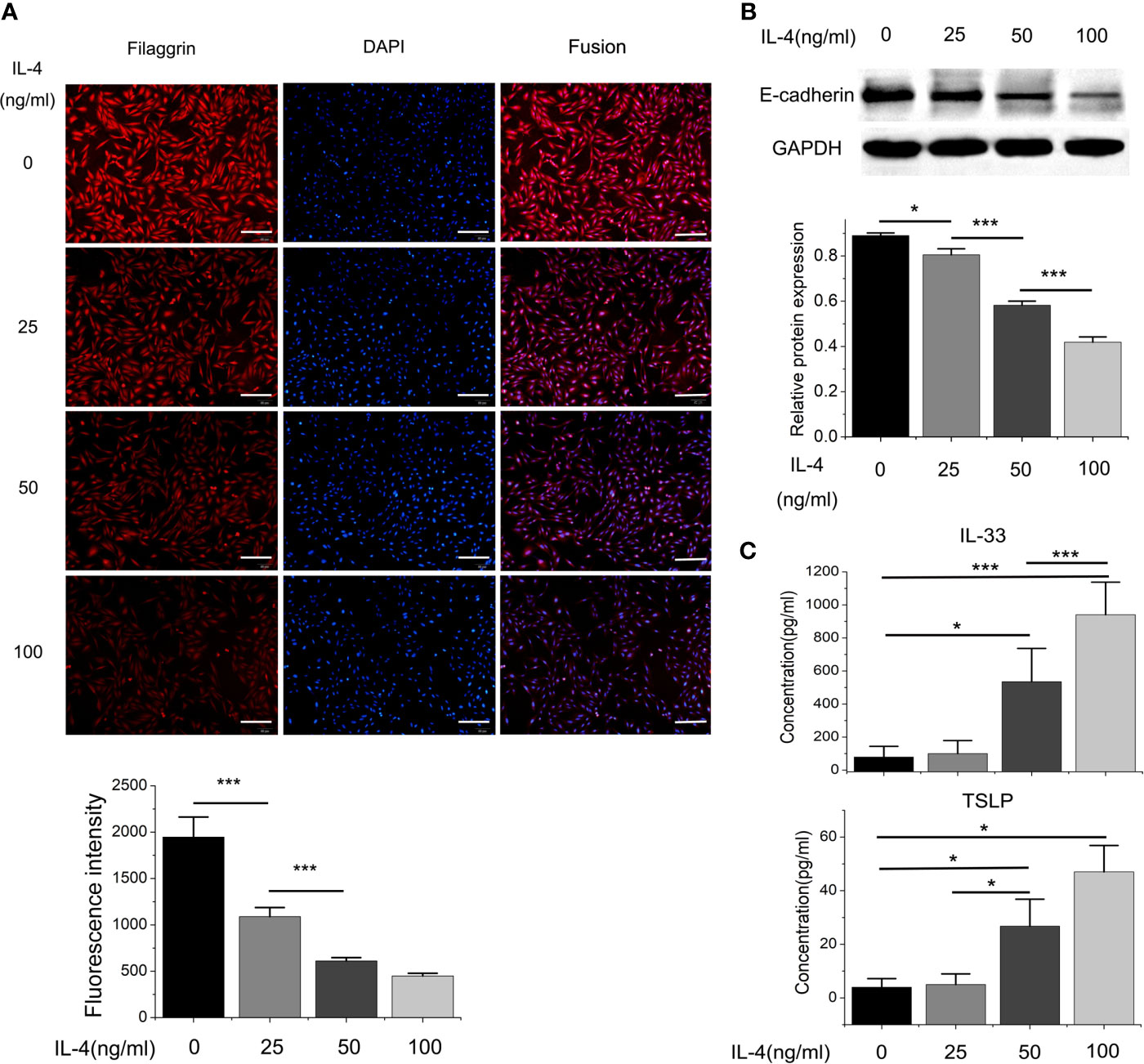
Figure 6 The protein expressions of filaggrin, E-cadherin, TSLP and IL-33 of BEAS-2B cells with different concentration of IL-4 incubation. (A) The protein expression of filaggrin in BEAS-2B cells treated with different concentration of IL-4 was detected by Immunofluorescence. The photographs were magnified of 200 times and the bars represented 50μm. (B) The protein expression of E-cadherin BEAS-2B cells treated with different concentration of IL-4 was detected by Western-blot. (C) IL-33 and TSLP in the cell culture supernatants with different concentration of IL-4 treatment were detected by ELISA. Each experiment has been repeated for 3 times and presented as the mean ± SD (n=3). The Student’s t-test was used to analyze the difference between groups. *P < 0.05 was considered as statistically significant. **P < 0.01, ***P < 0.005.
Different dose of IL-4 incubation also led to different results. In Figures 5 and 6, the protein expression of IL-33 and TSLP were statistically up-regulated until the IL-4 concentration rose to 50ng/ml, while there was no significant difference between the 50ng/ml IL-4 group and 100ng/ml IL-4 group, so that we chose the 50ng/ml of IL-4 in further researches.
On the other hand, the mRNA levels of epithelial cytokines IL-33 and TSLP, which are key regulators of Th2 inflammatory response, in the BEAS-2B cells with IL-4 inducing were obviously higher than those in the control group. Furthermore, IL-4 also increased the concentrations of soluble IL-33 and TSLP in BEAS-2B cells culture medium, and the regulation of IL-4 to IL-33 and TSLP was also dose- and time-dependent (Figures 3–6).
Knockdown of Filaggrin Further Down-Regulated the Expression of E-Cadherin in BEAS-2B Cells, and Also Further Up-Regulated the Expression of IL-33 and TSLP After IL-4 Inducing
We successfully knocked down filaggrin with designed siRNA (Figures S1, 2) and then evaluated the effect for related molecules. Knock-down filaggrin not only suppressed the filaggrin expression (Figure 7) but also suppressed the expression of E-cadherin (Figure 8). Moreover, filaggrin deficiency further decreased the expression of E-cadherin in BEAS-2B cells with IL-4 inducing. Interestingly, filaggrin deficiency did not affect the expression of IL-33 and TSLP in BEAS-2B cells apparently (Figure 8). However, when the BEAS-2B cells were incubated with IL-4, the expression levels of IL-33 and TSLP were elevated notably by knock-down filaggrin with specific siRNA which suggested that filaggrin was probably involved in the regulation mechanism of IL-4 to IL-33 and TSLP.
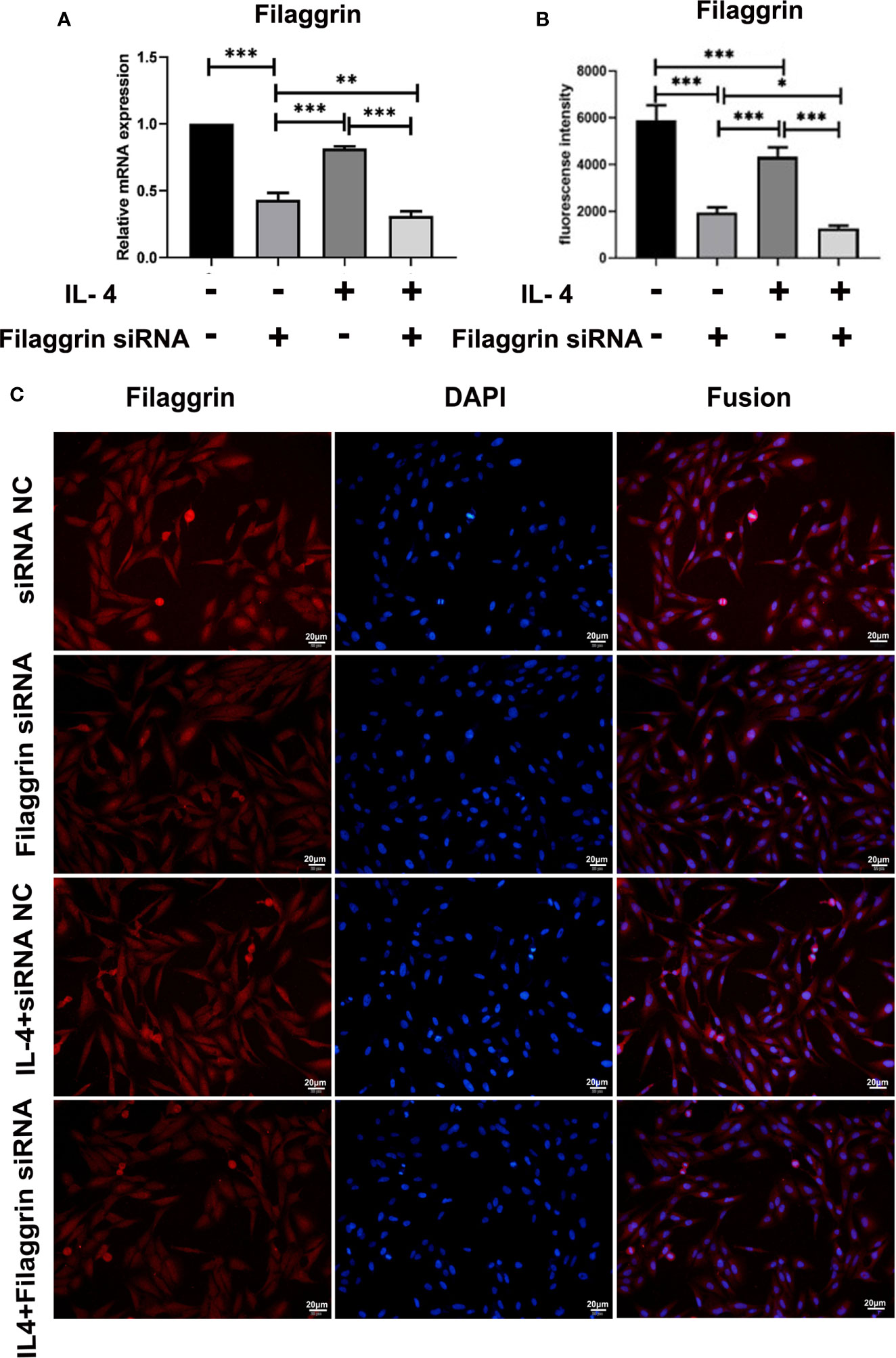
Figure 7 Filaggrin was more significantly decreased by IL-4 treatment after knock-down of filaggrin with specific siRNA. BEAS-2B cells were divided into four groups: siRNA NC group (control group), filaggrin siRNA group, IL-4 inducted group and filaggrin siRNA + IL-4 inducted group. (A) The mRNA expression of filaggrin among the four groups detected with RT-PCR. (B, C) The protein levels of filaggrin in the four groups were detected with IF. The photographs were magnified of 400 times and the bars represented 20μm. Each experiment has been repeated for 3 times and presented as the mean ± SD (n=3). The Student’s t-test was used to analyze the difference between groups. *P < 0.05 was considered as statistically significant. **P < 0.01, ***P < 0.005.
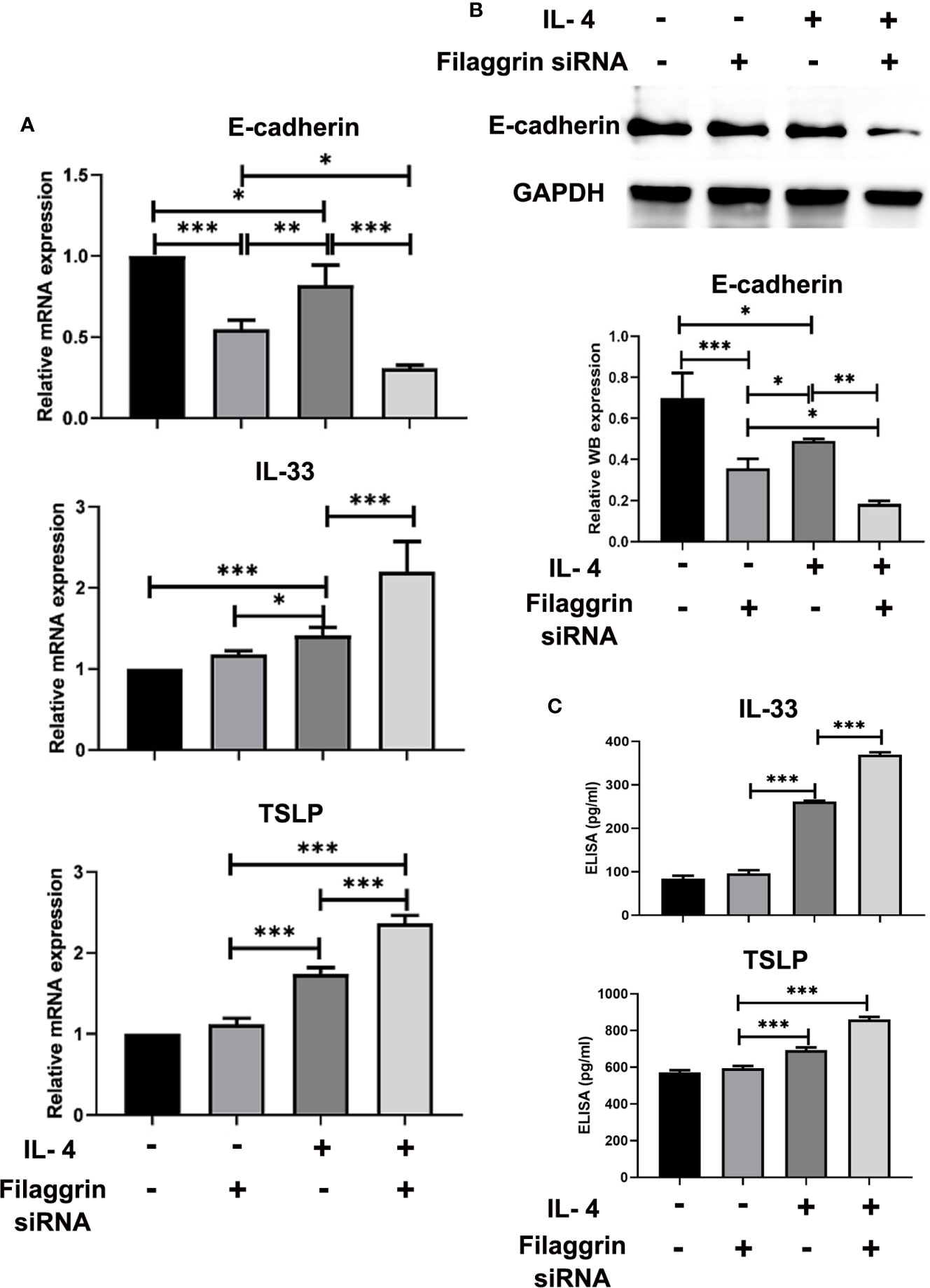
Figure 8 The expressions of E-cadherin, TSLP and IL-33 were more significantly modulated by IL-4 treatment after knock-down of filaggrin with specific siRNA. As same as the description in previous figure legend, BEAS-2B cells were divided into four groups: siRNA NC group (control group), filaggrin siRNA group, IL-4 inducted group and filaggrin siRNA + IL-4 inducted group. (A) The mRNA expression of E-cadherin, IL-33 and TSLP among the four groups detected with RT-PCR. (B) The protein levels of E-cadherin in the four groups were detected with Western-blot. (C) Levels of IL-33 and TSLP in the cell culture supernatant of the four groups were measured by ELISA. Each experiment has been repeated for 3 times and presented as the mean ± standard deviation (n=3). And the Student’s t-test was used to analyze the difference between groups. *P < 0.05; **P < 0.01; ***P < 0.001.
Disscusion
Asthma is a chronic inflammatory disease of the airways that affects more than 300 million people worldwide (29). It has been known that airway eosinophilia is associated with an increasing risk of asthma exacerbations (30). As the previous studies, asthma can be divided into eosinophilic asthma (EA), neutrophilic asthma (NA), paucigranulocytic asthma (PGA) and mixed-granulocytic asthma (MGA) based on the relative cell counts of induced sputum (1). Among the four inflammatory phenotypes, EA is found in 17-36% of asthma patients who have severe airflow obstruction despite optimal treatment and more frequent exacerbations (31). In our previous research, 36.9% asthmatic patients were defined as EA in 176 asthma subjects (1). It is known that the eosinophilic airway inflammation is highly correlated with childhood-onset and severe asthma, so we focused on this specific group and explored the pathogenic mechanisms of EA.
Since asthma is well-known as a heterogeneous polygenic disease, plenty of genetic studies of asthma in different populations have documented an association with polymorphisms of IL4, IL13, ADRB2, TNF, HLA-DRB1, FCER1B, L4RA, CD14, HLA-DQB1 and ADAM33 genes (2). These genes implicate abnormalities in epithelial barrier function and innate and adaptive immune responses which reveal the pathogenic mechanism of asthma. Although a series of genetic loci have been identified with involvement of asthma in different population groups, so far, the genomic background of EA has not been clarified yet. To analyze the genomic variations of EA patients, and their correlation with clinical characteristics, in this study targeted next-generation sequencing (including the promoter and exon regions of 109 asthma candidate genes) was used to detect the peripheral blood of 77 Chinese EA patients, and compared with 431 Chinese healthy controls to obtain differential genomic variations. We identified differential genomic variations in EA patients included 63 single nucleotide variants (SNVs) and 14 Insertion-Deletions (InDels) (Table S2) affecting protein translation. There were 41 differential SNPs screened with mutant patients more than 3. And among these screened SNPs, FLG rs192116923-G allele was found for the first time to be associated with EA patients with AD. On the other hand, FLG rs75235053-G allele and GSTM1 rs412302-A allele were associated with severe EA. These finding suggested that filaggrin was implicated in the pathogenesis of EA.
Filaggrin is a crucial epidermal protein encoded by FLG gene that is important for the formation of the corneocyte, as well as the generation of its intracellular metabolites, which contribute to stratum corneum hydration and pH (32). Loss-of-function mutations in 1 or both alleles of FLG result in reduced or completely absent levels of epidermal filaggrin, respectively. Recent GWAS reports found FLG loss-of-function mutations were closely related to childhood-onset and severity of asthma (33). FLG R501X and 2282del4 account for about 48% of AD patients and 9% of European and American Caucasians, but were hardly seen in Asian and African people (34, 35). Since the genetic association of SNPs in FLG gene and asthma has been concerned in previous researches, we analyzed the biological function of FLG rs75235053 C>G and rs192116923 T>G by bioinformatics tools. Interestingly, FLG rs75235053 C>G appeared to be a new splicing site, suggesting that it may cause mRNA truncation and reduce filaggrin monomers, thereby affecting the epidermal barrier function. FLG rs192116923 T >G may increase the binding to Smad3 or Smad2_Smad3_Smad4 complex. Smad3 is a nuclear transcription factor necessary for TGF-β1 signaling and can be triggered by TGF-β1. Activated and phosphorylated Smad3, Smad2 and Smad4 form a trimeric complex, which can enter the nucleus and induce gene transcription after binding to DNA under the action of DNA-binding cofactors (36). Recent evidence indicated that TGF-β1 could decrease filaggrin protein expression in the late stage of epidermal differentiation (37), so the combination of Smad3 may have an inhibitory effect on filaggrin expression.
Furthermore, it was found that FLG gene deficiency can affect epidermal cell migration, adhesion and proliferation, promote apoptosis, and interfere with the cell cycle (37). Considering the critical roles of filaggrin in epithelial cells, whether the respiratory epithelial cells expressed filaggrin and the further regulatory mechanisms still needs further exploration. Although some studies proposed that filaggrin expression was absent in the upper airway epithelial cells in both healthy controls and asthma patients (38), in our study it showed opposite results. In this article, it was firstly reported that human airway epithelial cell line BEAS-2B cells constitutively expressed filaggrin, and its expression is not less than human immortalized epidermal cell line HaCaT cells. More importantly, we have proved that filaggrin is also highly expressed in human normal bronchial epithelial cells in vivo. This finding created the basis for further investigation of filaggrin in asthma.
On the other hand, asthma is generally divided into T helper type 2(Th2/T2)-high and T2-low endotypes according to the cytokines involved in the pathogenesis of asthma (39). EA patients usually exhibit the secretion of typical Th2 cytokines, such as IL-4, IL-5 and IL-13, and induce Th2 immune response, which then generates a series of cascades downstream, including IgE-stimulated hypersensitivity, airway epithelial cell activation, effector cell (including mast cells, eosinophils and basophils) chemotaxis and epithelial and subepithelial matrix remodeling (10). It has been proved that AD skin is characterized by the over-expression of IL-4 and IL-13 while these Th2 cytokines significantly reduced filaggrin gene expression in keratinocytes which indicated that filaggrin was downregulated by Th2 milieu (14). Since Th2 cytokines also play vital roles in the pathogenesis of asthma, to exploring how filaggrin expression was regulated in the bronchial epithelial microenvironment by Th2 cytokines and whether the influenced filaggrin level elicited any downstream reaction to maintain the inflammatory response in asthma, we mimicked the Th2 cytokine environment with IL-4 and demonstrated that IL-4 suppressed the expression of filaggrin in bronchial epithelial cell line BEAS-2B cells.
In this study, it was found that IL-4 not only suppressed filaggrin expression but also disrupted the expression of E-cadherin. E-cadherin is the main adhesion junction protein of airway epithelium and an important part of the airway epithelial connection complex (40). E-cadherin establishes the connection between cells, promotes cell polarization, and regulates the transport of macromolecules and ions around cells, thereby maintains the normal function of airway epithelial barrier. Early epithelial-mesenchymal transition (EMT) is related to the loss of E-cadherin, and the reduction of E-cadherin can promote the transformation of M2-type macrophage (41, 42). They all play an important role in the pathogenesis of asthma.
Consistent with previous studies in vivo in AD (43), we found that IL-4 remarkably upregulated IL-33 and TSLP in BEAS-2B cells. Researchers also found that IL-4 increased the expression of IL-33 in keratinocytes and they conjected that there may be a positive feedback loop connected IL-4 and IL-33 in AD (44, 45). It has been known that the epithelial cytokines IL-33 and TSLP are warning factors for external stimuli and key regulators of Th2 inflammatory response, and play a role in tissue repair after injury (46). In the Th-2 environment in AD, epithelial IL-25, IL-33, and TSLP are upregulated. IL-33 can activate group 2 innate lymphoid cells (ILC2s) to produce Th2-type cytokines, such as IL-5, IL-13, and up-regulate the expression of OX40 and PD-L1 on the cell surface (47, 48). On the other hand, in the presence of IL-33, antigens can activate Th2-polarized CD4+T cells to participate in Th2 immune responses (49). Moreover, it has been reported that both IL-33 in human keratinocytes can downregulate the expression of filaggrin through phosphorylation of STAT3 and ERK (50). TSLP is also an endogenous mediator in patients with AD and a master regulator of Th2-driven inflammation (43). More importantly, TSLP is a direct trigger factor of T cell migration in FLG-deficient skin analogs (51).
However, the role of filaggrin in the regulation of IL-4 to E-cadherin and the epithelial-derived cytokines is still indistinct. Thus, we designed the specific filaggrin siRNA and successfully knocked down filaggrin expression in BEAS-2B cells. Then it was proved that the deficiency of filaggrin only effected E-cadherin but did not significantly impact IL-33 or TSLP. Moreover, E-cadherin was more observably reduced by filaggrin deficiency when induced with IL-4. And simultaneously, the expressions of IL-33 and TSLP were more significantly enhanced in effect of IL-4 when filaggrin expression was suppressed. These findings raised the possibility that the destruction of airway epithelial barrier and the promotion of Th2 immune response caused by FLG mutations was also part of the mechanism of asthma.
The molecular mechanism of IL-4 regulating filaggrin and other molecules was not clear yet and we are still working on this issue. We have proved that the filaggrin siRNA did not change the IL-4R expression in BEAS-2B cells. Therefore, filaggrin knockdown did not affect the responses of BEAS-2B cells to IL-4 incubation via regulation of IL-4R. Previous studies have shown that increased apoptosis of bronchial epithelial cells also played important roles in asthma pathogenesis. Recently, Jiapeng Hu and his colleagues proved that IL-4 or IL-13 stimulation induced the overexpression of fructose-1,6- bisphosphatase (Fbp1) which aggravated the oxidative stress and apoptosis in bronchial epithelial cells (52). In our study, we aslo found that IL-4 treatment and filaggrin siRNA transfection suppressed the viability of BEAS-2B cells. We speculated that knock-down of filaggrin may also lead to the oxidative stress induced apoptosis as the same as IL-4 treatment, which still needs further exploration.
Moreover, whether this positive feedback mechanism occurred in normal human bronchial cells is still unclear. We tried to perform these experiments in primary normal human bronchial epithelial (NHBE) cells, but the isolated bronchial epithelial cells were probably contaminated with fibroblasts and other stroma cells and were hard to identify. Further explorations are needed to answer this question.
In conclusion, we proposed that human normal airway epithelial cells in vitro and in vivo constitutively expressed filaggrin. The Th2 cytokines, IL-4, could reduce the expression of filaggrin and E-cadherin and induce the expression of epithelial-derived T2 cytokines in airway epithelial cells. Furthermore, filaggrin deficiency enhanced Th2 inflammatory response via upregulating the expression of IL-33 and TSLP in the presence of Th2 cytokines. After all, we conjected that the loss of function mutations of FLG gene we found in previous study in EA patients may destruct the integrity of airway epithelia not only via deficiency of filaggrin but also via suppression of E-cadherin. Furthermore, filaggrin deficiency could intensify the Th2 responses by induction of inflammatory molecules IL-33 and TSLP under the Th2 condition, which forms a positive feedback regulatory loop to amplify the immune response and promotes the progression of asthma (Figure 9).
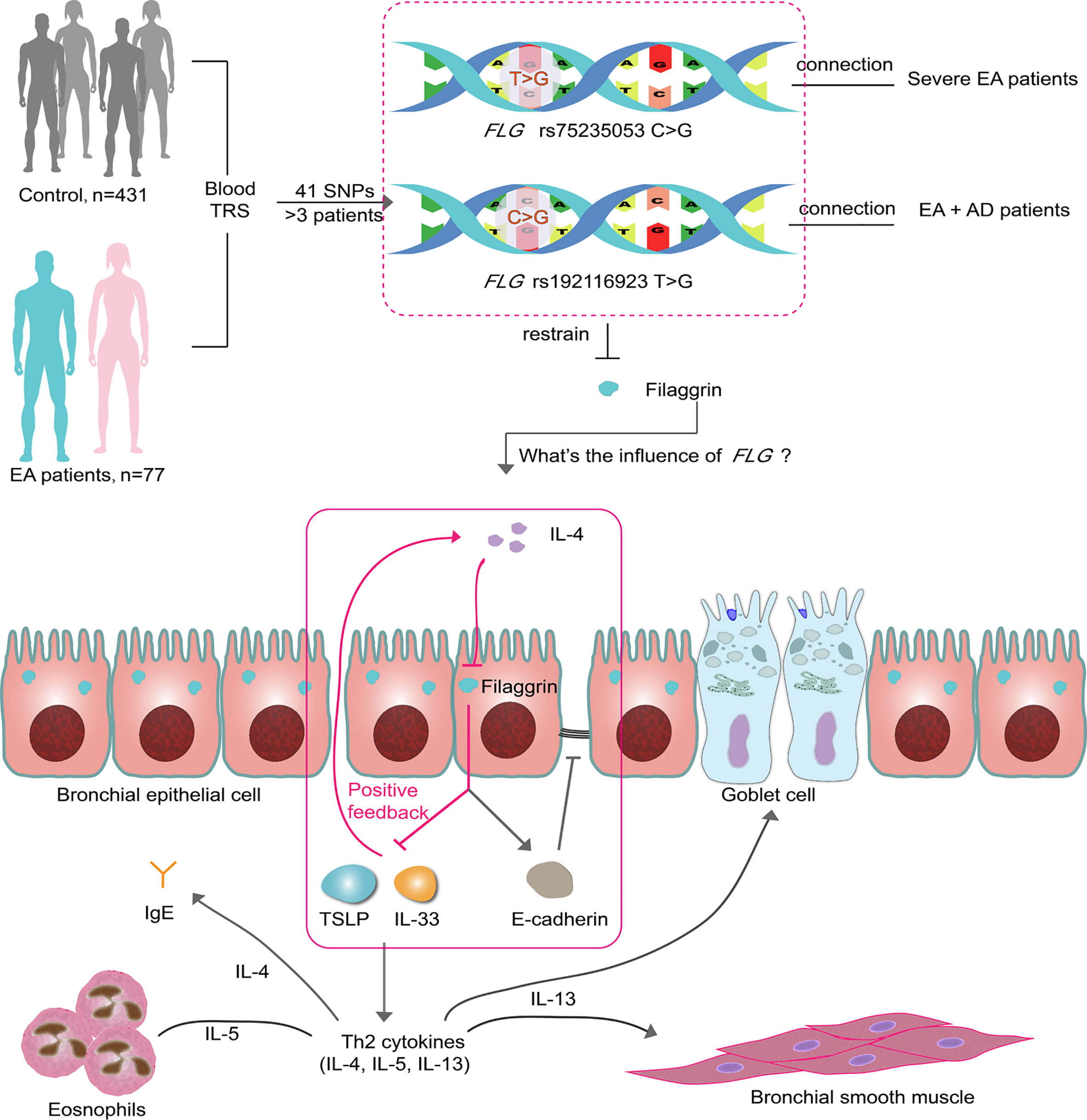
Figure 9 Two SNPs may influence EA patients via filaggrin through a positive feedback mechanism. There are 41 SNPs indentified from 77 EA patients by TRS. Two of them in FLG gene are associated with the clinical characteristics of EA patients. In this article, it has been proven that filaggrin expression is inhibited by the Th2 cytokine IL-4 in human bronchial epithelial cells, while the reductive filaggrin increases the expression of epithelial derived cytokines TSLP and IL-33 which induce the releasing of Th2 cytokines such as IL-4 and form a positive feedback loop (red box). The declining filaggrin also intensifies the reduction of E-cadherin induced by IL-4. EA, Eosinophilic asthma; AD, atopic dermatitis; TRS, target region sequencing.
Data Availability Statement
The datasets presented in this study can be found in online repositories. The names of the repository/repositories and accession number(s) can be found below: BioProject accession PRJNA718105.
Ethics Statement
The studies involving human participants were reviewed and approved by the Human Research Ethics Committee of the Chinese PLA general hospital. The patients/participants provided their written informed consent to participate in this study. Written informed consent was obtained from the individual(s) for the publication of any potentially identifiable images or data included in this article.
Author Contributions
WG, JG, HH and PB contributed to conception and design of the study. WG performed the major parts of the experiments. JG organized the database. WG and JG performed the statistical analysis. PB contributed to critical parts of the study implementation. WG wrote the first draft of the manuscript. JG, MM, YZ, and PB wrote sections of the manuscript. All authors contributed to manuscript revision, read, and approved the submitted version.
Funding
This study was supported by Logistic Support Department of CMC Health Care Project (No.21BJZ42), National Natural Science Foundation of Beijing (No.7212104) and the Special Program for Innovation of the National Defence Science and Technology of China(No.20-163-12-ZD-029-006-01).
Conflict of Interest
The authors declare that the research was conducted in the absence of any commercial or financial relationships that could be construed as a potential conflict of interest.
Publisher’s Note
All claims expressed in this article are solely those of the authors and do not necessarily represent those of their affiliated organizations, or those of the publisher, the editors and the reviewers. Any product that may be evaluated in this article, or claim that may be made by its manufacturer, is not guaranteed or endorsed by the publisher.
Supplementary Material
The Supplementary Material for this article can be found online at: https://www.frontiersin.org/articles/10.3389/fimmu.2021.672312/full#supplementary-material
References
1. Gao W, Han GJ, Zhu YJ, Mao D, Hu H. Clinical Characteristics and Biomarkers Analysis of Asthma Inflammatory Phenotypes. Biomarkers Med (2020) 14:211–22. doi: 10.2217/bmm-2019-0487
2. Ober C, Hoffjan S. Asthma Genetics 2006: The Long and Winding Road to Gene Discovery. Genes Immun (2006) 7:95–100. doi: 10.1038/sj.gene.6364284
3. Willis-Owen SAG, Cookson WOC, Moffatt MF. The Genetics and Genomics of Asthma. Annu Rev Genomics Hum Genet (2018) 19:223–46. doi: 10.1146/annurev-genom-083117-021651
4. Hirai K, Shirai T, Suzuki M, Shimomura T, Itoh K. Association Between (CCTTT)n Repeat Polymorphism in NOS2 Promoter and Asthma Exacerbations. J Allergy Clin Immunol (2018) 142:663–665.e3. doi: 10.1016/j.jaci.2018.02.023
5. Zhao LP, Di ZH, Zhang LL, Wang L, Ma L, Lv Y, et al. Shared and Distinct Genetic Risk Factors for Childhood-Onset and Adult-Onset Asthma: Genome-Wide and Transcriptome-Wide Studies. J Allergy Clin Immunol (2019) 26:1–8. doi: 10.1016/j.jdermsci.2016.01.011
6. Turner S, Francis B, Wani N, Vijverberg S, Pino-Yanes M, Mukhopadhyay S, et al. Variants in Genes Coding for Glutathione S-Transferases and Asthma Outcomes in Children. Pharmacogenomics (2018) 19:707–13. doi: 10.2217/pgs-2018-0027
7. Zuurhout MJ, Vijverberg SJ, Raaijmakers JA, Koenderman L, Postma DS, Koppelman GH, et al. Arg16 ADRB2 Genotype Increases the Risk of Asthma Exacerbation in Children With a Reported Use of Long- Acting b2 -Agonists: Results of the PACMAN Cohort. Pharmacogenomics (2013) 14:1965–71. doi: 10.2217/pgs.13.200
8. Farzan N, Vijverberg SJ, Hernandez-Pacheco N, Bel EHD, Berce V, Bønnelykke K, et al. 17q21 Variant Increases the Risk of Exacerbations in Asthmatic Children Despite Inhaled Corticosteroids Use. Allergy: Eur J Allergy Clin Immunol (2018) 73:2083–8. doi: 10.1111/all.13499
9. Kim KW, Ober C. Lessons Learned From GWAS of Asthma. Allergy Asthma Immunol Res (2019) 11:170–87. doi: 10.4168/aair.2019.11.2.170
10. Carr TF, Zeki AA, Kraft M. Eosinophilic and Noneosinophilic Asthma. Am J Respir Crit Care Med (2018) 197:22–37. doi: 10.1164/rccm.201611-2232PP
11. Bewicke-Copley F, Arjun Kumar E, Palladino G, Korfi K, Wang J. Applications and Analysis of Targeted Genomic Sequencing in Cancer Studies. Comput Struct Biotechnol J (2019) 17:1348–59. doi: 10.1016/j.csbj.2019.10.004
12. McLean WHI. Filaggrin Failure – From Ichthyosis Vulgaris to Atopic Eczema and Beyond. Br J Dermatol (2016) 175:4–7. doi: 10.1111/bjd.14997
13. Pividori M, Schoettler N, Nicolae DL, Ober C, Im HK. Shared and Distinct Genetic Risk Factors for Childhood-Onset and Adult-Onset Asthma: Genome-Wide and Transcriptome-Wide Studies. Lancet Respir Med (2019) 7:509–22. doi: 10.1016/S2213-2600(19)30055-4
14. Howell MD, Kim BE, Gao P, V A, Boguniewicz M, Debenedetto A, et al. Cytokine Modulation of AD Filaggrin Skin Expression. PRism (2009) 120:150–5. doi: 10.1016/j.jaci.2007.04.031
15. Hönzke S, Wallmeyer L, Ostrowski A, Radbruch M, Mundhenk L, Schäfer-Korting M, et al. Influence of Th2 Cytokines on the Cornified Envelope, Tight Junction Proteins, and β-Defensins in Filaggrin-Deficient Skin Equivalents. J Invest Dermatol (2016) 136:631–9. doi: 10.1016/j.jid.2015.11.007
16. Lee KH, Cho KA, Kim JY, Kim JY, Baek JH, Woo SY, et al. Filaggrin Knockdown and Toll-Like Receptor 3 (TLR3) Stimulation Enhanced the Production of Thymic Stromal Lymphopoietin (TSLP) From Epidermal Layers. Exp Dermatol (2011) 20:149–51. doi: 10.1111/j.1600-0625.2010.01203.x
17. Renert-Yuval Y, Del Duca E, Pavel AB, Fang M, Lefferdink R, Wu J, et al. The Molecular Features of Normal and Atopic Dermatitis Skin in Infants, Children, Adolescents and Adults. J Allergy Clin Immunol (2021) 148(1):148–63. doi: 10.1016/j.jaci.2021.01.001
18. GINA: Interim Guidance About COVID-19 & Asthma - Updated 26 April 2021. (2021). Available at: https://ginasthma.org/ [Accessed July 12, 2021].
19. Baines KJ, Simpson JL, Wood LG, Scott RJ, Gibson PG. Systemic Upregulation of Neutrophil α-Defensins and Serine Proteases in Neutrophilic Asthma. Thorax (2011) 66:942–7. doi: 10.1136/thx.2010.157719
20. Silkoff PE, Laviolette M, Singh D, FitzGerald JM, Kelsen S, Backer V, et al. Identification of Airway Mucosal Type 2 Inflammation by Using Clinical Biomarkers in Asthmatic Patients. J Allergy Clin Immunol (2017) 140:710–9. doi: 10.1016/j.jaci.2016.11.038
21. Tan DJ, Walters EH, Perret JL, Burgess JA, Johns DP, Lowe AJ, et al. Clinical and Functional Differences Between Early-Onset and Late-Onset Adult Asthma: A Population-Based Tasmanian Longitudinal Health Study. Thorax (2016) 71:981–7. doi: 10.1136/thoraxjnl-2015-208183
22. Schatz M, Rosenwasser L. The Allergic Asthma Phenotype. J Allergy Clin Immunol: In Pract (2014) 2:645–8. doi: 10.1016/j.jaip.2014.09.004
23. Jalota L, Allison DR, Prajapati V, Vempilly JJ, Jain VV. Ability of Exhaled Nitric Oxide to Discriminate for Airflow Obstruction Among Frequent Exacerbators of Clinically Diagnosed Asthma. Lung (2018) 196:455–62. doi: 10.1007/s00408-018-0132-8
24. Liu P, Zhao Y, Mu ZL, Lu QJ, Zhang L, Yao X, et al. Clinical Features of Adult/Adolescent Atopic Dermatitis and Chinese Criteria for Atopic Dermatitis. Chin Med J (2016) 129:757–62. doi: 10.4103/0366-6999.178960
25. Chung KF, Wenzel SE, Brozek JL, Bush A, Castro M, Sterk PJ, et al. International ERS/ATS Guidelines on Definition, Evaluation and Treatment of Severe Asthma. Eur Respir J (2014) 43:343–73. doi: 10.1183/09031936.00202013
26. Li H, Durbin R. Fast and Accurate Short Read Alignment With Burrows-Wheeler Transform. Bioinformatics (2009) 25:1754–60. doi: 10.1093/bioinformatics/btp324
27. Matoso A, Mukkada VA, Lu S, Monahan R, Cleveland K, Noble L, et al. Expression Microarray Analysis Identifies Novel Epithelial-Derived Protein Markers in Eosinophilic Esophagitis. Modern Pathol (2013) 26:665–76. doi: 10.1038/modpathol.2013.41
28. Gong J, Zhu C, Zhuang R, Song C, Li Q, Xu Z, et al. Establishment of an Enzyme-Linked Immunosorbent Assay System for Determining Soluble CD96 and its Application in the Measurement of Scd96 in Patients With Viral Hepatitis B and Hepatic Cirrhosis. Clin Exp Immunol (2009) 155:207–15. doi: 10.1111/j.1365-2249.2008.03829.x
29. Reinhart L, Knight W, Roberts L, Mendes C. HHS Public Access Global Burden of Disease Study 2010. Lancet (2012) 380:2163–96. doi: 10.1016/S0140-6736(12)61729-2
30. Papi A, Brightling C, Pedersen SE, Reddel HK. Asthma. Lancet (2018) 391:783–800. doi: 10.1016/S0140-6736(17)33311-1.
31. Chung KF. New Treatments for Severe Treatment-Resistant Asthma: Targeting the Right Patient. Lancet Respir Med (2013) 1:639–52. doi: 10.1016/S2213-2600(13)70128-0
32. Rahrig S, Dettmann JM, Brauns B, Lorenz VN, Buhl T, Kezic S, et al. Transient Epidermal Barrier Deficiency and Lowered Allergic Threshold in Filaggrin-Hornerin (FlgHrnr–/–) Double-Deficient Mice. Allergy: Eur J Allergy Clin Immunol (2019) 74:1327–39. doi: 10.1111/all.13756
33. Basu K, Palmer CNA, Lipworth BJ, Irwin McLean WH, Terron-Kwiatkowski A, Zhao Y, et al. Filaggrin Null Mutations Are Associated With Increased Asthma Exacerbations in Children and Young Adults. Eur J Allergy Clin Immunol (2008) 63:1211–7. doi: 10.1111/j.1398-9995.2008.01660.x
34. Sandilands A, Terron-Kwiatkowski A, Hull PR, O’Regan GM, Clayton TH, Watson RM, et al. Comprehensive Analysis of the Gene Encoding Filaggrin Uncovers Prevalent and Rare Mutations in Ichthyosis Vulgaris and Atopic Eczema. Nat Genet (2007) 39:650–4. doi: 10.1038/ng2020
35. Akiyama M. FLG Mutations in Ichthyosis Vulgaris and Atopic Eczema: Spectrum of Mutations and Population Genetics. Br J Dermatol (2010) 162:472–7. doi: 10.1111/j.1365-2133.2009.09582.x
36. Hu HH, Chen DQ, Wang YN, Feng YL, Cao G, Vaziri ND, et al. New Insights Into TGF-β/Smad Signaling in Tissue Fibrosis. Chemico-Biol Interact (2018) 292:76–83. doi: 10.1016/j.cbi.2018.07.008
37. Elias MS, Wright SC, Nicholson WV, Morrison KD, Prescott AR, Ten Have S, et al. Functional and Proteomic Analysis of a Full Thickness Filaggrin-Deficient Skin Organoid Model. Wellcome Open Res (2019) 4:134. doi: 10.12688/wellcomeopenres.15405.2
38. De Benedetto A, Qualia CM, Baroody FM, Beck LA. Filaggrin Expression in Oral, Nasal, and Esophageal Mucosa. J Invest Dermatol (2008) 128:1594–7. doi: 10.1038/sj.jid.5701208
39. Woodruff PG, Boushey HA, Dolganov GM, Barker CS, Yee HY, Donnelly S, et al. Genome-Wide Profiling Identifies Epithelial Cell Genes Associated With Asthma and With Treatment Response to Corticosteroids. Proc Natl Acad Sci USA (2007) 104:15858–63. doi: 10.1073/pnas.0707413104
40. Georas SN, Rezaee F. Immunology and Allergic Airway Inflammation. J Allergy Clin Immunol (2015) 134:509–20. doi: 10.1016/j.jaci.2014.05.049
41. Nieto MA. The Ins and Outs of the Epithelial to Mesenchymal Transition in Health and Disease. Annu Rev Cell Dev Biol (2011) 27:347–76. doi: 10.1146/annurev-cellbio-092910-154036
42. Hackett TL. Epithelial-Mesenchymal Transition in the Pathophysiology of Airway Remodelling in Asthma. Curr Opin Allergy Clin Immunol (2012) 12:53–9. doi: 10.1097/ACI.0b013e32834ec6eb
43. Werfel T, Allam JP, Biedermann T, Eyerich K, Gilles S, Guttman-Yassky E, et al. Cellular and Molecular Immunologic Mechanisms in Patients With Atopic Dermatitis. J Allergy Clin Immunol (2016) 138:336–49. doi: 10.1016/j.jaci.2016.06.010
44. Tsuji G, Hashimoto-hachiya A, Yen VH, Takemura M, Mitamura Y, Ito T, et al. Aryl Hydrocarbon Receptor Activation Downregulates IL-33 Expression in Keratinocytes via. J Clin Med (2020) 9:1–15. doi: 10.3390/jcm9030891
45. Qiao Y, Wang Q. The Expression and Regulation of Interleukin-33 in Human Epidermal Keratinocytes: A New Mediator of Atopic Dermatitis and Its Possible Signaling Pathway. J Interferon Cytokine Res (2016) 00:1–11. doi: 10.1089/jir.2015.0159
46. Roan F, Obata-Ninomiya K, Ziegler SF. Epithelial Cell–Derived Cytokines: More Than Just Signaling the Alarm. J Clin Invest (2019) 129:1441–51. doi: 10.1172/JCI124606
47. Halim TYF, Rana BMJ, Walker JA, Kerscher B, Knolle MD, Jolin HE, et al. Tissue-Restricted Adaptive Type 2 Immunity Is Orchestrated by Expression of the Costimulatory Molecule OX40L on Group 2 Innate Lymphoid Cells. Immunity (2018) 48:1195–1207.e6. doi: 10.1016/j.immuni.2018.05.003
48. Schwartz C, Khan AR, Floudas A, Saunders SP, Hams E, Rodewald HR, et al. ILC2s Regulate Adaptive Th2 Cell Functions via PD-L1 Checkpoint Control. J Exp Med (2017) 214:2507–21. doi: 10.1084/jem.20170051
49. Imai Y. Interleukin-33 in Atopic Dermatitis. J Dermatol Sci (2019) 96:2–7. doi: 10.1016/j.jdermsci.2019.08.006
50. Ryu WI, Lee H, Bae HC, Ryu HJ, Son SW. IL-33 Down-Regulates Filaggrin Expression by Inducing STAT3 and ERK Phosphorylation in Human Keratinocytes. J Dermatol Sci (2016) 82:131–4. doi: 10.1016/j.jdermsci.2016.01.011
51. Wallmeyer L, DIetert K, Sochorová M, Gruber AD, Kleuser B, Vávrová K, et al. TSLP Is a Direct Trigger for T Cell Migration in Filaggrin-Deficient Skin Equivalents. Sci Rep (2017) 7:1–12. doi: 10.1038/s41598-017-00670-2
Keywords: eosinophilic asthma, single nucleotide polymorphisms (SNP), FLG, Th2 immune response, IL-33, TSLP
Citation: Gao W, Gong J, Mu M, Zhu Y, Wang W, Chen W, Han G, Hu H and Bao P (2021) The Pathogenesis of Eosinophilic Asthma: A Positive Feedback Mechanism That Promotes Th2 Immune Response via Filaggrin Deficiency. Front. Immunol. 12:672312. doi: 10.3389/fimmu.2021.672312
Received: 25 February 2021; Accepted: 14 July 2021;
Published: 13 August 2021.
Edited by:
Shengjun Wang, Jiangsu University Affiliated People’s Hospital, ChinaReviewed by:
Norihiro Harada, Juntendo University, JapanJulie Worrell, University of Glasgow, United Kingdom
Copyright © 2021 Gao, Gong, Mu, Zhu, Wang, Chen, Han, Hu and Bao. This is an open-access article distributed under the terms of the Creative Commons Attribution License (CC BY). The use, distribution or reproduction in other forums is permitted, provided the original author(s) and the copyright owner(s) are credited and that the original publication in this journal is cited, in accordance with accepted academic practice. No use, distribution or reproduction is permitted which does not comply with these terms.
*Correspondence: Pengtao Bao, YmFvMDNAMTYzLmNvbQ==; Hong Hu, aHVob25nX2RyQGFsaXl1bi5jb20=
†These authors have contributed equally to this work and share first authorship