- Division of Rheumatology & Clinical Immunology, University of Pittsburgh, Pittsburgh, PA, United States
The invasive fungal infections (IFIs) are a major cause of mortality due to infectious disease worldwide. Majority of the IFIs are caused by opportunistic fungi including Candida, Aspergillus and Cryptococcus species. Lack of approved antifungal vaccines and the emergence of antifungal drug-resistant strains pose major constraints in controlling IFIs. A comprehensive understanding of the host immune response is required to develop novel fungal vaccines to prevent death from IFIs. In this review, we have discussed the challenges associated with the development of antifungal vaccines. We mentioned how host-pathogen interactions shape immunological memory and development of long-term protective immunity to IFIs. Furthermore, we underscored the contribution of long-lived innate and adaptive memory cells in protection against IFIs and summarized the current vaccine strategies.
Introduction
Every year around 1.5 million death occur due to invasive fungal infections (IFIs) worldwide (1). Approximately, 80% of this mortality are due to infections caused by opportunistic fungi (1). Majority of these patients die due to fungal sepsis caused by uncontrolled fungal growth. Additionally, IFIs can impact vital internal organs like lungs, heart, brain, kidneys, and liver leading to end-organ damage. Death due to fungal sepsis and end-organ damage are commonly seen in immunocompromised patients or individuals with inborn errors in immunity, who are unable to control IFIs (2). With the increase in the number of immunocompromised patients, there is an unmet clinical need to develop fungal vaccines to prevent these fatal infections.
One of the major IFIs is invasive Candidiasis or disseminated Candidiasis (2–4). The causative agent for invasive Candidiasis is Candida species, more commonly Candida albicans, responsible for causing around 46–75% of all IFIs. When Candida spp gain access to the bloodstream, it causes significantly high mortality (rates 40-60%), particularly in hospitalized patients and immunocompromised individuals (1, 5). Another cause of IFIs is invasive Aspergillosis, which is caused by a saprophytic bacteria A. fumigatus. Approximately, 200,000 people die due to invasive Aspergillosis each year, with mortality rates as high as 30–95% (1). Cryptococcosis, caused by Cryptococcus neoformans infects over a million individuals with 20–70% mortality rate annually (1, 6).
It is now well established that occurrence and severity of IFIs are associated to the immune status of the host (7). Majority of the these pathogenic fungi are opportunistic pathogens and cause disease when immune system is suppressed (1). Consequently, the prevalence of IFIs has significantly increased due to alarming rise in the number of HIV and cancer patients and use of immunosuppressive medications. Hence, treating IFIs in the face of compromised immune system is clinically daunting. Thus, to reduce the global incidence and mortality of IFIs, there is a serious need for the development of effective and safe fungal vaccines.
In this review, we systematically discussed the complex interactions between Candida, Aspergillus and Cryptococcus species and the host immune system. We emphasized the role of innate and adaptive immune cells in the context of long-term antifungal memory response and protective immunity. We also outlined the recent efforts in developing candidate antifungal vaccine strategies against IFIs.
Challenges Associated With the Development of Fungal Vaccines
Majority of the IFIs affect patients with severe immunodeficiency (8). Indeed, patients with compromised immune system and long-term hospitalized patients undergoing invasive medical interventions are at higher risk of developing these systemic infections (1, 8, 9). With the rise in the incidence of cancer and use of immunosuppressive and invasive medical therapies, the incidence and severity of IFIs are likely to increase with time. In the case of invasive Candidiasis, the crude mortality rate can reach as high as 60% in patients with immune deficiency (10). Similarly, C. neoformans cause severe meningoencephalitis in HIV patients (11). The mortality rates for invasive Aspergillosis can reach up to 80% in patients with compromised immune system (8, 12, 13).
Treatment with current antifungal drugs have several limitations. These include lack of early diagnostic tools, renal toxicity, development of drug resistance, narrow range of antifungal activity and major drug-drug interactions (14). Thus, there is a demanding clinical need to develop safe and effective antifungal vaccines to mitigate the risk of fatality in these patients. Currently, various research groups have devoted enormous resources to develop effective fungal vaccines. These research efforts are mainly directed against using novel vaccine formulations to generate long-term protective memory in both high-risk patients as well as in healthy individuals (8, 15). However, generation of vaccine-induced protective immunity in immunocompromised subjects is a key clinical challenge (16). Additionally, generating sterile immunity by vaccinating against commensal fungus (e.g., Candida spp) could be challenging and in worst case scenario may lead to autoimmunity. The potential high costs in preparing the fungal vaccines to vaccinate a small group of high-risk individuals does not motivate big pharmaceutical companies to develop vaccines against IFIs. Most importantly, designing an effective strategy for vaccination against IFIs is impeded by our lack of understanding of antifungal immunity. Despite these challenges, clinical trials of vaccines against these opportunistic fungi are ongoing and producing encouraging results, which have been described in this review in a systematic manner.
Characterization of Immunological Memory Response in IFIs
Vaccines trigger short and long-term protective immunity against infections. One of the hallmarks of vaccines is the development of anti-pathogen memory response in the host. The immunological memory against past infections aids in the rapid protection during subsequent assault by the same pathogen. Numerous studies have shown that both innate and adaptive immune cells can memorize previous infections and launch pathogen-specific immunity during secondary infections (17, 18). This is particularly evident in case of many IFIs that cause life threatening diseases. Here, we systematically outline the innate and adaptive immune components responsible for generating innate and adaptive antifungal memory response in mice and human settings.
Innate Immune Components of Functional Antifungal Memory Response
Neutrophils
Neutrophils are the first line of defense against infection and play important role in the clearance of the invading pathogens. This process is primarily mediated by two strategies: phagocytosis and secretion of anti-microbials. The role of neutrophils in controlling IFIs is emphasized by the fact that neutropenia is a risk factor for invasive Candidiasis (19, 20) and Aspergillosis (21), but not Cryptococcosis (22). There are multiple ways by which neutrophils control IFIs. The primary and secondary granules of neutrophil contain various antimicrobial peptides, proteolytic and nucleolytic enzymes; all of which are important for the fungicidal activity. Additionally, neutrophils produce reactive oxygen species (ROS) to kill phagocytosed fungi within the phagolysosome (20). Neutrophils facilitate the infiltration of other immune cells to the infection site via production of chemokines (23). The neutrophils also inhibit fungal growth through the deprivation of essential nutrients (24). Moreover, neutrophils produce neutrophil extracellular traps (NETs), which are meshwork of extracellular chromatin DNA, histones, and antimicrobial peptides to trap and kill pathogens (24, 25). Notably, the NETs play important role for the killing of the pathogenic fungal hyphae (24, 25).
Monocytes and Macrophages
Macrophages are scavenger cells, which are derived from blood monocytes. The tissue-resident macrophages reside within the tissues while others remain in the circulation and secondary lymphoid organs and migrate to the sites of infection (26, 27). Upon fungal recognition, macrophages undergo polarization into classical activated macrophages (M1) and alternative activated macrophages (M2) (26, 27). M1 macrophages contribute to fungus clearance by producing pro-inflammatory and fungicidal factors, whereas M2 macrophages support fungal persistence and take part in the resolution of inflammation and tissue healing process (28). On the other hand, tissue-resident macrophages act as a source of pro-inflammatory cytokines and chemokines, which facilitate the migration of other innate and adaptive immune cells to the infected tissue (29).
The classical monocytes, as defined by CD16 and CD14 expression, control fungal infection by inhibiting the conidial germination of A. fumigatus and C. albicans (30, 31). The monocytes drive the production of IL-1β and prostaglandin E2 production following C. albicans infection and facilitate protective T-helper 17 (Th17) differentiation (31). Similar antifungal activity of monocytes were also demonstrated in Cryptococcosis (32).
Natural Killer (NK) Cells
Unlike neutrophils and macrophages, the role of NK cells in fungal clearance in IFIs is disputed. This is particularly evident in mouse model of invasive Candidiasis, where depletion of NK cells is linked to either no effect or increased susceptibility to C. albicans in two separate studies, respectively (33, 34). Additionally, NK cells depleted SCID mice succumbed to invasive Candidiasis. However, NK cell depletion had minimal impact on antifungal immunity in animals with normal immune system (35). In sharp contrast, another report demonstrated the contribution NK-cells specific IL-17 receptor signaling in antifungal immunity in invasive Candidiasis (36).
Dendritic Cells (DCs)
DCs are less efficient in clearing fungal infections in comparison to neutrophils and macrophages (37). Rather, DCs orchestrate the primary immune response by acting as professional antigen presenting cells (APCs) (37). After fungal recognition, DCs process the exogenous fungal antigens and present it to the naïve T cells in context of major histocompatability complex II (MHC II). Additionally, DCs produce cytokines that aid in the differentiation of naïve CD4 T cells towards various T-helper (Th) subsets including Th1, Th2 and Th17 and T regulatory (T regs) cells (38) (Figure 1). Interestingly, DCs direct different Th subsets development based on the fungal recognition of yeast and hyphal forms of C. albicans (39). In addition to its role as APCs, DCs have been shown to protect mice and human from A. fumigatus infections by some unknown mechanisms (40).
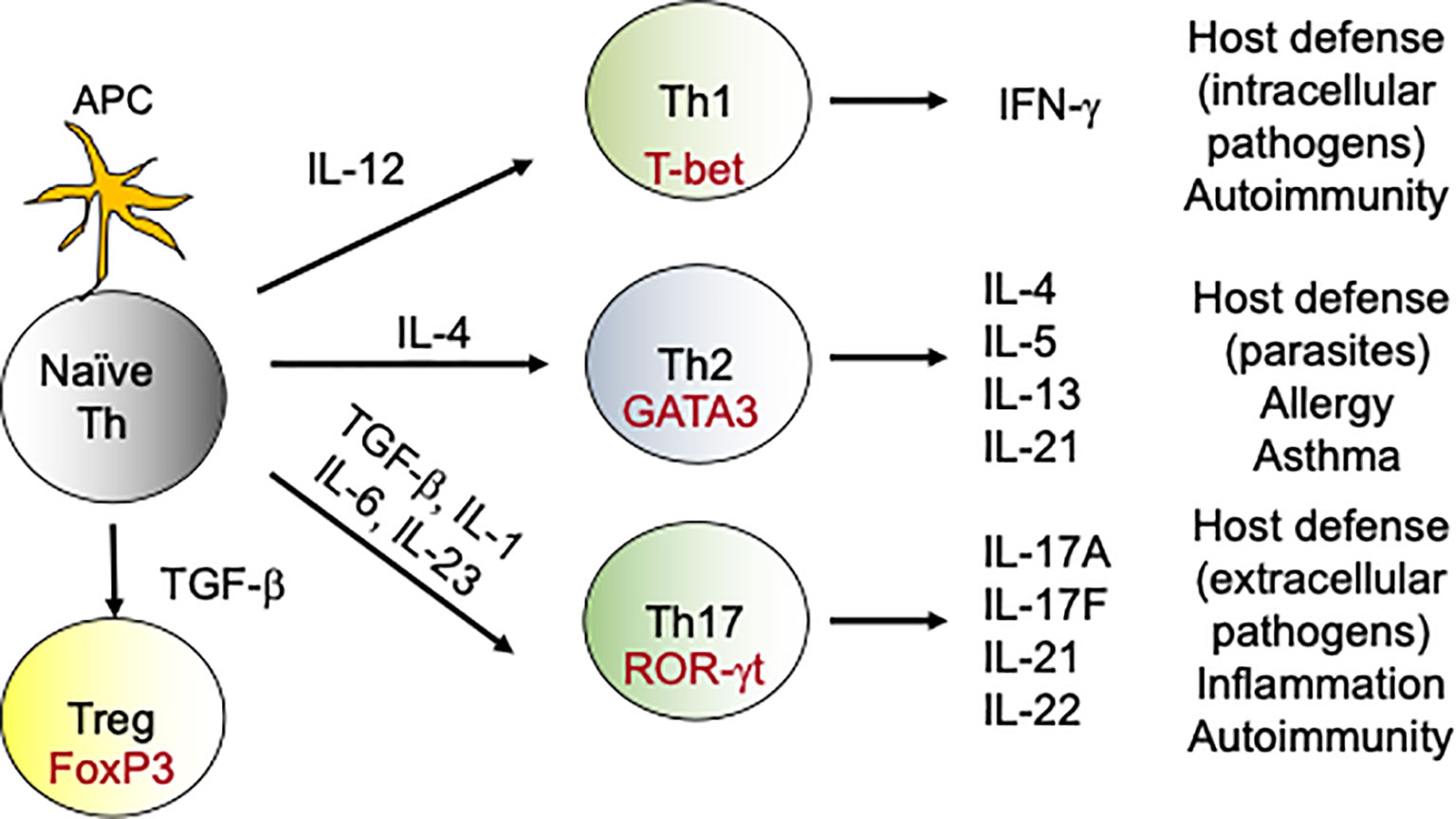
Figure 1 Differentiation of T-helper cells: During fungal infections, DCs present antigens to naive CD4 T cells. During DCs:T cells interaction, DCs produce cytokines which drives the differentiation of various Th subsets. For example, naïve CD4 T cells in the presence of IL-12 upregulate transcription factor T-bet and become Th1 cells. Similarly, IL-6, IL-1 and IL-23 aid in the differentiation of Th17 cells, which produce IL-17 family of cytokines. These subsets (Th1, Th2, Th17 and Tregs) by virtue of their cytokine production exert various effector functions, which modulates the immune response against the fungus. In the context to antifungal immunity, activated Th1 and Th17 cells migrate to the site of infection from secondary lymphoid organs and help in the fungal clearance.
Complement System
In disseminated candidiasis, the activation of complement occurs via alternative pathway in a galactomannan/β‐glucan dependent manner. Complement-depleted animals and C5-deficient mice showed increased susceptibility following systemic infection (41, 42). However, no correlation was found between occurrence of disseminated candidiasis and single complement deficiencies in humans (43). Similarly, C5 deficient mice also demonstrated exaggerated susceptibility systemic infection with A. fumigatus (44). The protective effect of C5a in Aspergillosis is mainly mediated by its strong chemotactic properties to recruit inflammatory cells in the infected lungs. The complement receptors CR1, CR3 and CR4 on innate cells drive phagocytosis of the opsonized C. neoformans (45, 46). Additionally, C3a and C5a have been shown to drive the expression of cytokines and chemokines during infection including IL-8 and TNFα (47, 48). For B dermatitidis infection, glucan deficiency is linked diminished C3‐binding, indicating β‐glucan as a critical player of complement activation (49).
Innate Sensing of the Fungal Pathogens
The interaction between immune cells and fungi is crucial for the generation of functional memory response in IFIs. The recognition of the fungal pathogen associated molecular patterns (PAMPs) by pathogen recognition receptors (PRRs) of the host initiates the antifungal host defense and subsequent development of memory response. The toll-like receptors (TLR; TLR-2, TLR-4, TLR-9), C-type lectin receptors (CLRs; Dectin-1, 2, Galectin, mannose receptor, macrophage-inducible C-type lectin [mincle], dendritic cells specific intercellular adhesion molecule grabbing nonintegrin [DC-SIGN]), nucleotide-binding oligomerization domain (NOD)-like receptors (NLRs) recognize fungal cell wall components such as glucans, mannans, peptidoglycan-associated proteins and phospholipomannan to initiate the antifungal activity (12, 50, 51). The recognition of fungal PAMPs by specific PRRs drives an intracellular signaling cascade in the responding cells that leads to not only the activation of innate and adaptive immune defense but also programs cells to develop and survive as memory cells (52).
Trained Immunity in Response to IFIs
Innate immune cells can be trained by fungi and fungal components. Recent mouse model studies demonstrated the innate memory response in monocytes/macrophages and NK cells against C. albicans (53–55). In this system, mice infected with an attenuated C. albicans strain showed protection against invasive Candidiasis (56, 57). The protection is mediated by a T cell-independent but macrophage-dependent manner, popularly known as innate memory response or trained immunity (58). Mechanistically, trained immunity is mediated by epigenetic reprogramming and metabolic shift in innate immune cells (52, 58, 59). Upon recognition of C. albicans or β-glucans by monocytes and macrophages, dectin 1-mediated activation of RAF1 signaling cause stable alterations in histone methylation and acetylation. Trained monocytes and macrophages show increased ability to secret cytokines and more efficient and rapid control of fungus following secondary infection (59). Moreover, trained immunity relies on AKT, mammalian target of rapamycin (mTOR) and hypoxia-inducible factor 1α (HIF1α), activation and on a shift of glucose utilization from oxidative phosphorylation to glycolysis (52). Interestingly, BCG and measles vaccinated children showed evidence of memory innate immune response (60, 61). These observations suggest that these vaccines would provide cross-protection to other infections including IFIs (62). Interestingly, defect in trained immunity has also been observed in patients with STAT1 deficiency and suffering from chronic mucocutaneous candidiasis, thus highlighting the clinical importance of this least understood innate defense mechanisms in control of fungal infections (63). Although trained immunity in macrophages and NK cells has been described, the concept of long-term immunological memory development in short-lived neutrophils is difficult to prove.
Adaptive Immune Components of Functional Antifungal Memory Response
During infection, professional APCs process and present microbial antigen to naive T cells in the context of MHC II. Naïve T cells via T cell receptor (TCR) recognize the antigen-MHC II complex and undergo activation and proliferation (17, 64). This event leads to the generation of short-lived effector T cells that clear the pathogen. The activated T cells will also help B cells to produce antibody secreting plasma cells. The generation of short-lived effector T and B cells precedes the development of memory T and B-cells population, respectively. In most cases, the memory T and B cells survive for years, even in the absence of antigens (17, 64). The memory T cells can be divided into two functionally distinct subsets such as T central memory (Tcm) and T effector memory (Tem) cells (65). Upon secondary exposure, the memory T and B cells will respond quickly and clear the pathogen via cell and humoral-mediated immunity, respectively.
CD4 T Cells
Upon antigen encounter, naïve CD4 T cells differentiate into various flavors of Th cells. This include Th1, Th2, Th9, Th17, Th22, regulatory T cells, and follicular helper T cells (Figure 1). The most important Th cells in the antifungal immune response against IFIs are the Th1 and Th17 cells (66, 67).
(i) Th1 Cells
The Th1 response is characterized by the production of pro-inflammatory cytokines such as IFN-γ, which plays important role in host defense against intracellular pathogens. The Th1 cells also offer a protective immune response to the host against IFIs (68). For example, IFN-γ or IFN-γ receptor-deficient mice succumb to invasive Candidiasis and pulmonary Cryptococcosis, respectively (69, 70). In line with the mouse model studies, individuals with invasive Candidiasis showed better prognosis after recombinant IFN-γ therapy (71). IFN-γ therapy also lowered Cryptococcus burden in the cerebrospinal fluid of patients with HIV associated Cryptococcal meningitis (72). Additionally, cell-based therapy with IFN-γ producing A. fumigatus specific CD4 T cells protected mice with bonemarrow transplantation and humans following invasive Aspergillosis (73, 74).
(ii) Th17 Cells
The discovery of Th17 subset has revolutionized our knowledge of the antifungal host defense. APC-derived cytokines like IL-6, IL-1β and IL-23 facilitate Th17 differentiation (75, 76) (Figure 1). Th17 cells produce IL-17 family of cytokines (IL-17A-F) that bind to IL-17 receptor subfamilies (IL-17RA-RE) on target cells (77). The target cells of IL-17 are mostly non-hematopoietic cells. Th17 cells via the production of IL-17 stimulates the expression of cytokines, chemokines and antimicrobial peptides in target cells, which in turn promote the recruitment of innate cells and clearance of the fungi. Considerable data implicate Th17 cells in immunity to Candida and other fungi (19, 78). Indeed, humans with genetic polymorphisms in IL-17 pathway are at increased risk of fungal infections. For example, mutations in genes controlling IL-17 signaling (ACT1, IL17RA, IL17F), genes that drive Th17 development (DECTIN1, CARD9, STAT3, STAT1, IL12RB) or in individuals with naturally-occurring anti-IL-17 Abs (AIRE deficiency) showed increased susceptibility to mucosal Candidiasis, but not invasive Candidiasis (79). Thus, the role of IL-17 or Th17 cells in the protection against IFIs remains to be clarified. We and others have shown that IL-17- and IL-17R-deficient mice are highly susceptible to DC (36, 80–82). At mucosal surfaces and skin, IL-17 protects against C. albicans by inducing the expression of anti-microbial peptides (AMP) that limit infection and chemokines that mediate recruitment of neutrophils with antifungal activities (19, 78). However, we discovered a surprisingly kidney tissue protective role for IL-17 in invasive Candidiasis (81, 82). Supporting these findings, immunization with Als3 containing vaccine conferred protection against invasive Candidiasis by inducing a strong Th1 and Th17 response (83). Additionally, Th1/Th17 paradigm has also been shown to be critical for protection against Cryptococcosis in mice (84).
CD8 T Cells
The APCs cross-present exogenous fungal antigens to CD8 T cells to activate and differentiate them into Tc1, Tc2, Tc3 subtypes (85). Once activated, CD8 T cells proliferate to become effector cells that control the fungal infection by mediating specific effector functions. These include activating macrophages via production of IFN-γ and direct killing of pathogenic fungus. Indeed, mice depleted of CD8 T cells showed increased susceptibility to Cryptococcal infection and CD8 T cells restricted C. neoformans growth in infected macrophages by secreting IFN-γ (86, 87). While Tc1 cells secret cytotoxic molecules such as perforin and granzymes to lyse fungal cells, Tc2 cells secrete IL-4 and IL-10 and play important role in immune regulation (88). Interestingly, Tc3 cells secrete IL-17 cytokines and express transcription factor RORγ and CCR6, hallmarks of classical Th17 cells (88). IL-17 produced from Tc3 cells drives the infiltration of innate immune effectors and upregulates the expression of antimicrobial peptides, defensins from the epithelial cells, which are critical for controlling fungal burden in the infected organs (88).
B Cells
While the most important function of B cells is to produce antibodies during infection, it also contributes to antigen presentation to T cells and cytokine production. During IFIs, antifungal antibodies directed against fungal cell wall components confer host defense (89). Accordingly, passive transfer of serum from vaccinated mice were able to protect against fungal infection (90). Antibodies control fungal agents by multiple mechanisms including preventing fungal entry, activation of classical complement pathway, inhibition of fungal replication, suppression of germ tube formation and inhibiting the formation of fungal biofilms. For example, antibodies against mannoprotein of C. albicans showed candidacidal function by suppressing adherence and germination (91). It has also been demonstrated that antibody-mediated iron starvation is an effective way of controlling fungal growth (89). The anti-β-glucans antibodies directly inhibit the growth of C. albicans and C. neoformans (92). Finally, antibodies against C. neoformans capsular antigen interfere with the biofilm formation.
T and B Cells Memory Response
For antifungal vaccines, it is critical to develop and maintain long-lasting memory response both in immunocompetent and immunocompromised patients. Recently, some of the antifungal vaccine candidates have been tested for various IFIs. These include C. albicans, Aspergillus spp, Cryptococcus spp, Blastomyces spp, Paracoccidioides brasiliensis and Sporothrix spp. However, our comprehensive understanding of the memory response in providing long-term protective immunity against IFIs is currently lacking (93).
The various subsets of effector CD4 and CD8 T cells such as Th1, Th17, Tc1 and Tc17 develop into memory cells and confer long term protective immunity (88). A recent study by Nanjappan et al. demonstrated that Tc17 cells persist as long-lasting memory cells by the production of GM-CSF and TNF-α and confers protection against secondary fungal infections (88). Interestingly, Tc17 cells-mediated antifungal resistance was demonstrated in the absence of CD4 T cells, indicating that CD8 T cells can play a dominant role in controlling fungal load in the absence of CD4 T cells (88). This observation is of high clinical relevance since HIV patients who lack CD4-mediated immunity and are at high risk of IFIs (88).
The formation of germinal centers in the secondary lymphoid organs precedes the formation of memory B cells (MBCs) following primary infection (Figure 2). Once formed, the MBCs survive for long time and repeatedly produce antibody in the case of re-infections. Earlier studies from the mouse models elucidated that many therapeutic vaccines mediate antifungal protection by generating the antibody (89, 91, 94). Interestingly, some of the anti-β-glucan antibodies cross-react with several other fungal pathogens, indicating that single antibody may protect against multiple fungal pathogens (92). In contrast, antibodies generated during primary IFIs and naturally acquired antibodies at the stage of early childhood infection was unable to protect against subsequent IFIs (95). This data indicates that anti-fungus antibodies is not sufficient to prevent future fungal infections and T cell-mediated immune response plays a significant role in the long-term.
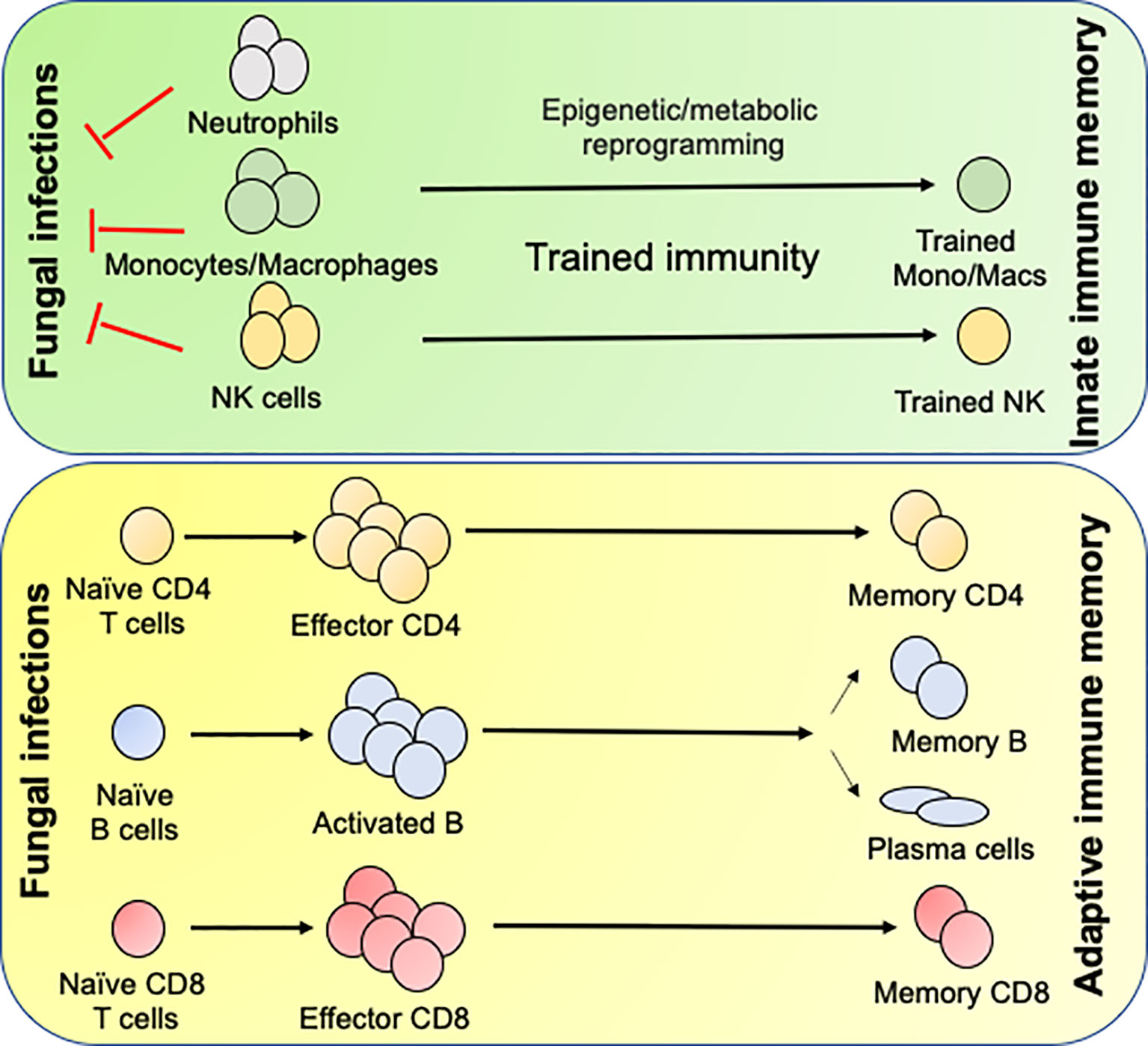
Figure 2 Generation of innate and adaptive antifungal memory response: Following fungal infections, innate cells like neutrophils, monocytes/macrophages and NK cells infiltrate the infected organs and clear the fungi. During this process the monocytes/macrophages and NK cells undergo epigenetic and metabolic reprogramming to form innate memory cells in a process known as Trained immunity. Following initial innate response, naïve CD4 and CD8 T cells recognize fungal antigens in context to APCs and form effector cells, which aid in the clearance of infection. Once the infection is cleared most of the effector cells die leaving few CD4 and CD8 T cells to become long lived memory cells. The memory CD4 and CD8 T cells either reside in the secondary lymphoid organs or tissues and confer rapid protection following secondary fungal infections. B cells recognize antigen by the B cell receptor and signals derived from the antigen specific CD4 T cells in the T/B zone of the secondary lymphoid organs. Some activated B cells form short-lived plasmablasts while others enter germinal center (GC). In the GC, the B cells undergo clonal expansion, somatic hypermutation and class-switch recombination. Antigen selected B cells eventually differentiate into memory B cells or antibody secreting long lived plasma cells, which migrate to the bone marrow.
Efforts to Develop New Antifungal Vaccines
The goal of an efficient fungal vaccine is to generate long-lasting immunological memory and provide sterile immunity against a recurrent exposure to the fungi (93). To achieve these objectives, several vaccination strategies with different vaccine candidates have been tested in pre-clinical animal models and clinical trials, which are outlined below (Figure 3).
C. albicans
Over the past decade, numerous groups have assessed multiple candidate vaccines to develop sterilizing and long-term antifungal immunity against C. albicans. These candidates include polysaccharides from fungal cell wall, fungal protein subunits and live attenuated strains of C. albicans (96). The most promising vaccine strategy ever tested is the use of N-terminal portion of the agglutinin like sequence 3 protein (Als3p) of C. albicans (97). Mice and non-human primates were immunized with purified Alsp3 protein mixed with aluminum hydroxide as adjuvant. Following encouraging results in animal models, the vaccine was further subjected to clinical trials in human. Seventy-three adults were vaccinated with two doses and placebo as control. All the subjects generated robust anti-Als3p IgG antibody after the first dose. The second dose elicited a strong IgA1 antibody titer in addition to IgG response. The vaccine successfully elicited T-cell response, as evident by the production of T cells cytokine such as IL-17 and IFN-γ, which were critical for anti-Candida immunity. One of the major limitations of this study is that it has not been tested in individuals receiving corticosteroids and antibiotics. In a separate study, a recombinant version of the secreted aspartyl proteinase 2 (Sap-2) protein of C. albicans was used to vaccinate mice and assess susceptibility to vaginitis (98). The Sap-2 protein along with virosome as adjuvant were vaccinated by intravaginal route. The vaccination resulted in the generation of Sap-2 specific protective antibodies, which also cross reacted with different Saps (99). Additionally, a live attenuated strain of C. albicans and has also been tested in the mouse models (98–100). Although these vaccine strategies showed promising results, they have not been tested in humans (98). This is primarily due to the high risk of introducing attenuated live fungus in humans particularly in the immunocompromised individuals.
Aspergillus spp
The pulmonary Aspergillosis is a serious infection in immunocompromised patients. However, it is also observed in immunocompetent individuals, indicating that a vaccine against Aspergillus spp should be capable of inducing protective immunity in both immunosuppressed and immunocompetent subjects. A study by Cenci et al. showed that intranasal immunization of crude Aspergillus antigens was effective in generating Th1 immunity and protected mice from pulmonary aspergillosis (73). Notably, a follow-up study using sonicated fungal antigen was successful to generate protective immunity in corticosteroid treated immunosuppressed mice. This data indicates that it is possible to protect against pulmonary Aspergillosis in the face of immunosuppression, which is a major clinical problem in hospital settings (101). In a separate report, epitope p41 from the cell wall glucanase of A. fumigatus was used to vaccinate mice against Aspergillosis (102). The antigenic epitope was presented by professional APCs through to generate Th1 response. Interestingly, the antifungal CD4 T cells response generated by p41 epitope can cross-react with C. albicans. Additionally, a pan-fungal vaccine using β-glucans of S. cerevisiae generated optimal protection against several pathogenic fungi, including A. fumigatus (103). This vaccine can generate protective immunity in the absence of adjuvants, which is an added advantage. Since, this study was performed in immunocompetent animals, it is not known whether this immunization strategy will work similarly in immunocompromised individuals. Nevertheless, the idea of a pan-fungal vaccine to protect against these multiple deadly fungal diseases is interesting and warrants careful consideration in the near future (103, 104).
Cryptococcus spp
Cryptococcosis is the most common cause of HIV-related meningitis. Hence, vaccines against Cryptococcus spp need to be efficient in the absence of functional CD4 T cells, which are primary cells in helping B cells to produce antibodies (8). Following an asymptomatic acute phase infection, the fungus enters into latent state. In the face of immunosuppression, the latent fungus reactivates to cause disease in HIV infected individuals (105). Hence, a successful vaccine should be able to restrain both the acute and recurrent infection (105). The first vaccine designed to immunize mice against C. neoformans comprised of an antiphagocytic antigen from the capsule of C. neoformans, known as glucuronoxylomannann (GMX) (106). Importantly, GMX does not require T cell help for the generation of antibody response. To enhance the antigenicity of the vaccine, GMX was mixed with tetanus toxoid and injected in mice (106). Although vaccinated mice developed strong anti-Cryptococcus-specific antibody response, most of these antibodies are non-protective (105, 107). A separate group vaccinated T cells depleted mice with an strain of C. neoformans that were engineered to express IFN-γ (108). Vaccination protected the T cells deficient mice following a secondary pulmonary infection using a pathogenic strain. This data for the first time showed the feasibility of mounting a protective immune response to Cryptococcus in the absence of normal immune system. Subsequently, a study utilized a live attenuated strain to immunize mice and assess protection against virulent Cryptococcus strains (109). In this setting, the mutant lacked the sterol glucosidase enzyme (Δsgl1), leading to an increased production of sterol glucosides in the cells. Infection with the Δsgl1 fungus enable mice withstand a secondary infection with virulent strains of C. neoformans and C. gattii.
Endemic Mycoses
Mice depleted of CD4 T cells and immunized with an attenuated strain of B. dermatitidis (lacking the gene for the adhesin Bad-1) showed protection against the infection by a wild type virulent strain of B. dermatitidis (110). This observation highlights the importance of CD4 T cell independent immune pathways to generate protective immunity against endemic blastmycosis. Another interesting study showed that immunization with P10, a modified peptide derived from the antigen gp43 of P. brasiliensis, protected both immunocompetent and immunocompromised mice against this pathogen (111). Moreover, immunization with S. cerevisiae expressing gp43 conferred resistance in mice, as evident by lower fungal burden and production of IL-12 and IFN-γ in the lungs (112). Finally, de Almeida et al. showed that treatment of mice with antibodies against the glycoprotein 70 (gp70) from S. schenkii showed protection against infection with different strains of S. schenkii and S. brasiliensis (113). This result suggest that passive antibody therapies can be a treatment option in immunocompromised patients against endemic mycoses (96).
Conclusions
The biomedical science has been blessed with many successful vaccines that have prevented millions of deaths and irradicated many infectious diseases. Even now, there are no approved vaccines against any fungal pathogens and the IFIs continue to be a major threat to human health especially among the immunocompromised patients. Limitations associated with conventional antifungal diagnosis and drugs further aggravated the scenario. Extensive research over the past decades have shown that vaccination against one fungus can confer protection against the other, thus laying the groundwork for the development and validation of universal antifungal vaccine concept. Thus, the research for the generation of universal fungal vaccines have gained momentum and popularity in the research community, which may pave the path for the development of fungal vaccines against wide array of fungal pathogens. These efforts may not only prove beneficial in discovering fungal vaccine candidates from unexpected and distant species, but also may provide information on re-purposing some of the successful vaccines used against other pathogens in fungal diseases.
Author Contributions
The author confirms being the sole contributor of this work and has approved it for publication.
Funding
This work was supported by a Rheumatology Research Foundation grant to PB and NIH grants AI145242, AI142354 and DK104680 to PB.
Conflict of Interest
The author declares that the research was conducted in the absence of any commercial or financial relationships that could be construed as a potential conflict of interest.
The handling editor declared a past co-authorship with the author.
Acknowledgments
The author thanks his lab members for helpful discussions and suggestions.
References
1. Brown GD, Denning DW, Gow NA, Levitz SM, Netea MG, White TC. Hidden Killers: Human Fungal Infections. Sci Transl Med (2012) 4(165):165rv13. doi: 10.1126/scitranslmed.3004404
2. Constantine GM, Lionakis MS. Lessons From Primary Immunodeficiencies: Autoimmune Regulator and Autoimmune Polyendocrinopathy-Candidiasis-Ectodermal Dystrophy. Immunol Rev (2019) 287(1):103–20. doi: 10.1111/imr.12714
3. Pappas PG, Lionakis MS, Arendrup MC, Ostrosky-Zeichner L, Kullberg BJ. Invasive Candidiasis. Nat Rev Dis Primers (2018) 4:18026–31. doi: 10.1038/nrdp.2018.26
4. van de Veerdonk FL, Kullberg BJ, Netea MG. Pathogenesis of Invasive Candidiasis. Curr Opin Crit Care (2010) 16(5):453–9. doi: 10.1097/MCC.0b013e32833e046e
5. Pfaller MA, Diekema DJ. Epidemiology of Invasive Candidiasis: A Persistent Public Health Problem. Clin Microbiol Rev (2007) 20(1):133–63. doi: 10.1128/CMR.00029-06
6. Park BJ, Wannemuehler KA, Marston BJ, Govender N, Pappas PG, Chiller TM. Estimation of the Current Global Burden of Cryptococcal Meningitis Among Persons Living With HIV/AIDS. AIDS (2009) 23(4):525–30. doi: 10.1097/QAD.0b013e328322ffac
7. Netea MG, Latz E, Mills KH, O’Neill LA. Innate Immune Memory: A Paradigm Shift in Understanding Host Defense. Nat Immunol (2015) 16(7):675–9. doi: 10.1038/ni.3178
8. Spellberg B. Vaccines for Invasive Fungal Infections. F1000 Med Rep (2011) 3:13–9. doi: 10.3410/M3-13
9. Cassone A, Cauda R. Candida and Candidiasis in HIV-infected Patients: Where Commensalism, Opportunistic Behavior and Frank Pathogenicity Lose Their Borders. AIDS (2012) 26(12):1457–72. doi: 10.1097/QAD.0b013e3283536ba8
10. Moriyama B, Gordon LA, McCarthy M, Henning SA, Walsh TJ, Penzak SR. Emerging Drugs and Vaccines for Candidemia. Mycoses (2014) 57(12):718–33. doi: 10.1111/myc.12265
11. Rittershaus PC, Kechichian TB, Allegood JC, Merrill AH Jr., Hennig M, Luberto C, et al. Glucosylceramide Synthase is an Essential Regulator of Pathogenicity of Cryptococcus Neoformans. J Clin Invest (2006) 116(6):1651–9. doi: 10.1172/JCI27890
12. Bourgeois C, Kuchler K. Fungal Pathogens-a Sweet and Sour Treat for Toll-Like Receptors. Front Cell Infect Microbiol (2012) 2:142. doi: 10.3389/fcimb.2012.00142
13. Perlroth J, Choi B, Spellberg B. Nosocomial Fungal Infections: Epidemiology, Diagnosis, and Treatment. Med Mycol (2007) 45(4):321–46. doi: 10.1080/13693780701218689
14. Denning DW, Bromley MJ. Infectious Disease. How to Bolster the Antifungal Pipeline. Science (2015) 347(6229):1414–6. doi: 10.1126/science.aaa6097
15. Cassone A, Casadevall A. Recent Progress in Vaccines Against Fungal Diseases. Curr Opin Microbiol (2012) 15(4):427–33. doi: 10.1016/j.mib.2012.04.004
16. Medici NP, Del Poeta M. New Insights on the Development of Fungal Vaccines: From Immunity to Recent Challenges. Mem Inst Oswaldo Cruz (2015) 110(8):966–73. doi: 10.1590/0074-02760150335
17. MacLeod MK, Kappler JW, Marrack P. Memory CD4 T Cells: Generation, Reactivation and Re-Assignment. Immunology (2010) 130(1):10–5. doi: 10.1111/j.1365-2567.2010.03260.x
18. Bekkering S, Dominguez-Andres J, Joosten LAB, Riksen NP, Netea MG. Trained Immunity: Reprogramming Innate Immunity in Health and Disease. Annu Rev Immunol (2021) 39. doi: 10.1146/annurev-immunol-102119-073855
19. Horn DL, Neofytos D, Anaissie EJ, Fishman JA, Steinbach WJ, Olyaei AJ, et al. Epidemiology and Outcomes of Candidemia in 2019 Patients: Data From the Prospective Antifungal Therapy Alliance Registry. Clin Infect Dis (2009) 48(12):1695–703. doi: 10.1086/599039
20. Desai JV, Lionakis MS. The Role of Neutrophils in Host Defense Against Invasive Fungal Infections. Curr Clin Microbiol Rep (2018) 5(3):181–9. doi: 10.1007/s40588-018-0098-6
21. Feldmesser M. Prospects of Vaccines for Invasive Aspergillosis. Med Mycol (2005) 43(7):571–87. doi: 10.1080/13693780500402138
22. Hirai Y, Ainoda Y, Shoji T, Fujita T, Yoshinaga K, Shiseki M, et al. Disseminated Cryptococcosis in a non-Hodgkin’s Lymphoma Patient With Late-Onset Neutropenia Following rituximab-CHOP Chemotherapy: A Case Report and Literature Review. Mycopathologia (2011) 172(3):227–32. doi: 10.1007/s11046-011-9423-9
23. Lin L, Ibrahim AS, Baquir B, Palosaari A, Spellberg B. Luminescent-Activated Transfected Killer Cells to Monitor Leukocyte Trafficking During Systemic Bacterial and Fungal Infection. J Infect Dis (2012) 205(2):337–47. doi: 10.1093/infdis/jir725
24. Urban CF, Ermert D, Schmid M, Abu-Abed U, Goosmann C, Nacken W, et al. Neutrophil Extracellular Traps Contain Calprotectin, a Cytosolic Protein Complex Involved in Host Defense Against Candida Albicans. PloS Pathog (2009) 5(10):e1000639. doi: 10.1371/journal.ppat.1000639
25. Urban CF, Reichard U, Brinkmann V, Zychlinsky A. Neutrophil Extracellular Traps Capture and Kill Candida Albicans Yeast and Hyphal Forms. Cell Microbiol (2006) 8(4):668–76. doi: 10.1111/j.1462-5822.2005.00659.x
26. Martinez FO, Helming L, Gordon S. Alternative Activation of Macrophages: An Immunologic Functional Perspective. Annu Rev Immunol (2009) 27:451–83. doi: 10.1146/annurev.immunol.021908.132532
27. Varin A, Gordon S. Alternative Activation of Macrophages: Immune Function and Cellular Biology. Immunobiology (2009) 214(7):630–41. doi: 10.1016/j.imbio.2008.11.009
28. Reales-Calderon JA, Aguilera-Montilla N, Corbi AL, Molero G, Gil C. Proteomic Characterization of Human Proinflammatory M1 and Anti-Inflammatory M2 Macrophages and Their Response to Candida Albicans. Proteomics (2014) 14(12):1503–18. doi: 10.1002/pmic.201300508
29. Lionakis MS, Swamydas M, Fischer BG, Plantinga TS, Johnson MD, Jaeger M, et al. CX3CR1-Dependent Renal Macrophage Survival Promotes Candida Control and Host Survival. J Clin Invest (2013) 123(12):5035–51. doi: 10.1172/JCI71307
30. Serbina NV, Cherny M, Shi C, Bleau SA, Collins NH, Young JW, et al. Distinct Responses of Human Monocyte Subsets to Aspergillus Fumigatus Conidia. J Immunol (2009) 183(4):2678–87. doi: 10.4049/jimmunol.0803398
31. Smeekens SP, van de Veerdonk FL, Joosten LA, Jacobs L, Jansen T, Williams DL, et al. The Classical CD14(+) CD16(-) Monocytes, But Not the Patrolling CD14(+) CD16(+) Monocytes, Promote Th17 Responses to Candida Albicans. Eur J Immunol (2011) 41(10):2915–24. doi: 10.1002/eji.201141418
32. Hardison SE, Herrera G, Young ML, Hole CR, Wozniak KL, Wormley FL Jr. Protective Immunity Against Pulmonary Cryptococcosis is Associated With STAT1-mediated Classical Macrophage Activation. J Immunol (2012) 189(8):4060–8. doi: 10.4049/jimmunol.1103455
33. Romani L, Mencacci A, Cenci E, Spaccapelo R, Schiaffella E, Tonnetti L, et al. Natural Killer Cells do Not Play a Dominant Role in CD4+ Subset Differentiation in Candida Albicans-Infected Mice. Infect Immun (1993) 61(9):3769–74. doi: 10.1128/IAI.61.9.3769-3774.1993
34. Whitney PG, Bar E, Osorio F, Rogers NC, Schraml BU, Deddouche S, et al. Syk Signaling in Dendritic Cells Orchestrates Innate Resistance to Systemic Fungal Infection. PloS Pathog (2014) 10(7):e1004276. doi: 10.1371/journal.ppat.1004276
35. Quintin J, Voigt J, van der Voort R, Jacobsen ID, Verschueren I, Hube B, et al. Differential Role of NK Cells Against Candida Albicans Infection in Immunocompetent or Immunocompromised Mice. Eur J Immunol (2014) 44(8):2405–14. doi: 10.1002/eji.201343828
36. Bar E, Whitney PG, Moor K, Reis e Sousa C, LeibundGut-Landmann S. Il-17 Regulates Systemic Fungal Immunity by Controlling the Functional Competence of NK Cells. Immunity (2014) 40(1):117–27. doi: 10.1016/j.immuni.2013.12.002
37. Netea MG, Gijzen K, Coolen N, Verschueren I, Figdor C, Van der Meer JW, et al. Human Dendritic Cells are Less Potent At Killing Candida Albicans Than Both Monocytes and Macrophages. Microbes Infect (2004) 6(11):985–9. doi: 10.1016/j.micinf.2004.05.013
38. Borghi M, Renga G, Puccetti M, Oikonomou V, Palmieri M, Galosi C, et al. Antifungal Th Immunity: Growing Up in Family. Front Immunol (2014) 5:506–13. doi: 10.3389/fimmu.2014.00506
39. d’Ostiani CF, Del Sero G, Bacci A, Montagnoli C, Spreca A, Mencacci A, et al. Dendritic Cells Discriminate Between Yeasts and Hyphae of the Fungus Candida Albicans. Implications for Initiation of T Helper Cell Immunity In Vitro and In Vivo. J Exp Med (2000) 191(10):1661–74. doi: 10.1084/jem.191.10.1661
40. Ramirez-Ortiz ZG, Lee CK, Wang JP, Boon L, Specht CA, Levitz SM. A Nonredundant Role for Plasmacytoid Dendritic Cells in Host Defense Against the Human Fungal Pathogen Aspergillus Fumigatus. Cell Host Microbe (2011) 9(5):415–24. doi: 10.1016/j.chom.2011.04.007
41. Gelfand JA, Hurley DL, Fauci AS, Frank MM. Role of Complement in Host Defense Against Experimental Disseminated Candidiasis. J Infect Dis (1978) 138(1):9–16. doi: 10.1093/infdis/138.1.9
42. Fulurija A, Ashman RB, Papadimitriou JM. Early Inflammatory Responses to Candida Albicans Infection in Inbred and Complement-Deficient Mice. FEMS Immunol Med Microbiol (1996) 14(2-3):83–94. doi: 10.1111/j.1574-695X.1996.tb00274.x
43. Figueroa JE, Densen P. Infectious Diseases Associated With Complement Deficiencies. Clin Microbiol Rev (1991) 4(3):359–95. doi: 10.1128/CMR.4.3.359
44. Martinez FO, Helming M, Gordon S. Alternative Activation of Macrophages: An Immunologic Functional Perspective. Annu Rev Immunol (2009) 27:451–83. doi: 10.1146/annurev.immunol.021908.132532
45. Levitz SM, Tabuni A. Binding of Cryptococcus Neoformans by Human Cultured Macrophages. Requirements for Multiple Complement Receptors and Actin. J Clin Invest (1991) 87(2):528–35. doi: 10.1172/JCI115027
46. Levitz SM, Tabuni A, Kozel TR, MacGill RS, Ingalls RR, Golenbock DT. Binding of Cryptococcus Neoformans to Heterologously Expressed Human Complement Receptors. Infect Immun (1997) 65(3):931–5. doi: 10.1128/IAI.65.3.931-935.1997
47. Vecchiarelli A, Retini C, Casadevall A, Monari C, Pietrella D, Kozel TR. Involvement of C3a and C5a in Interleukin-8 Secretion by Human Polymorphonuclear Cells in Response to Capsular Material of Cryptococcus Neoformans. Infect Immun (1998) 66(9):4324–30. doi: 10.1128/.66.9.4324-4330.1998
48. Delfino D, Cianci L, Migliardo M, Mancuso G, Cusumano V, Corradini C, et al. Tumor Necrosis Factor-Inducing Activities of Cryptococcus Neoformans Components. Infect Immun (1996) 64(12):5199–204. doi: 10.1128/IAI.64.12.5199-5204.1996
49. Zhang MX, Klein B. Activation, Binding, and Processing of Complement Component 3 (C3) by Blastomyces Dermatitidis. Infect Immun (1997) 65(5):1849–55. doi: 10.1128/IAI.65.5.1849-1855.1997
50. Hardison SE. Brown GD. C-Type Lectin Receptors Orchestrate Antifungal Immunity. Nat Immunol (2012) 13(9):817–22. doi: 10.1038/ni.2369
51. Pietrella D, Bistoni G, Corbucci C, Perito S, Vecchiarelli A. Candida Albicans Mannoprotein Influences the Biological Function of Dendritic Cells. Cell Microbiol (2006) 8(4):602–12. doi: 10.1111/j.1462-5822.2005.00651.x
52. Cheng SC, Quintin J, Cramer RA, Shepardson KM, Saeed S, Kumar V, et al. mTOR- and HIF-1alpha-mediated Aerobic Glycolysis as Metabolic Basis for Trained Immunity. Science (2014) 345(6204):1250684. doi: 10.1126/science.1250684
53. Quintin J, Cheng SC, van der Meer JW, Netea MG. Innate Immune Memory: Towards a Better Understanding of Host Defense Mechanisms. Curr Opin Immunol (2014) 29:1–7. doi: 10.1016/j.coi.2014.02.006
54. Levy O, Netea MG. Innate Immune Memory: Implications for Development of Pediatric Immunomodulatory Agents and Adjuvanted Vaccines. Pediatr Res (2014) 75(1-2):184–8. doi: 10.1038/pr.2013.214
55. Kleinnijenhuis J, Quintin J, Preijers F, Joosten LA, Jacobs C, Xavier RJ, et al. BCG-Induced Trained Immunity in NK Cells: Role for non-Specific Protection to Infection. Clin Immunol (2014) 155(2):213–9. doi: 10.1016/j.clim.2014.10.005
56. Bistoni F, Vecchiarelli A, Cenci E, Puccetti P, Marconi P, Cassone A. Evidence for Macrophage-Mediated Protection Against Lethal Candida Albicans Infection. Infect Immun (1986) 51(2):668–74. doi: 10.1128/IAI.51.2.668-674.1986
57. Bistoni F, Verducci G, Perito S, Vecchiarelli A, Puccetti P, Marconi P, et al. Immunomodulation by a Low-Virulence, Agerminative Variant of Candida Albicans. Further Evidence for Macrophage Activation as One of the Effector Mechanisms of Nonspecific Anti-Infectious Protection. J Med Vet Mycol (1988) 26(5):285–99. doi: 10.1080/02681218880000401
58. Netea MG, Quintin J, van der Meer JW. Trained Immunity: A Memory for Innate Host Defense. Cell Host Microbe (2011) 9(5):355–61. doi: 10.1016/j.chom.2011.04.006
59. Saeed S, Quintin J, Kerstens HH, Rao NA, Aghajanirefah A, Matarese F, et al. Epigenetic Programming of Monocyte-to-Macrophage Differentiation and Trained Innate Immunity. Science (2014) 345(6204):1251086. doi: 10.1126/science.1251086
60. Sun JC, Ugolini S, Vivier E. Immunological Memory Within the Innate Immune System. EMBO J (2014) 33(12):1295–303. doi: 10.1002/embj.201387651
61. Aaby P, Benn CS. Saving Lives by Training Innate Immunity With Bacille Calmette-Guerin Vaccine. Proc Natl Acad Sci USA (2012) 109(43):17317–8. doi: 10.1073/pnas.1215761109
62. Benn CS, Netea MG, Selin LK, Aaby P. A Small Jab - a Big Effect: Nonspecific Immunomodulation by Vaccines. Trends Immunol (2013) 34(9):431–9. doi: 10.1016/j.it.2013.04.004
63. Ifrim DC, Quintin J, Meerstein-Kessel L, Plantinga TS, Joosten LA, van der Meer JW, et al. Defective Trained Immunity in Patients With STAT-1-dependent Chronic Mucocutaneaous Candidiasis. Clin Exp Immunol (2015) 181(3):434–40. doi: 10.1111/cei.12642
64. Stemberger C, Neuenhahn M, Buchholz VR, Busch DH. Origin of CD8+ Effector and Memory T Cell Subsets. Cell Mol Immunol (2007) 4(6):399–405.
65. Klebanoff CA, Gattinoni L, Torabi-Parizi P, Kerstann K, Cardones AR, Finkelstein SE, et al. Central Memory Self/Tumor-Reactive CD8+ T Cells Confer Superior Antitumor Immunity Compared With Effector Memory T Cells. Proc Natl Acad Sci USA (2005) 102(27):9571–6. doi: 10.1073/pnas.0503726102
66. Cutler JE, Deepe GS Jr., Klein BS. Advances in Combating Fungal Diseases: Vaccines on the Threshold. Nat Rev Microbiol (2007) 5(1):13–28. doi: 10.1038/nrmicro1537
67. Chen K. Kolls Jk. T Cell-Mediated Host Immune Defenses in the Lung. Annu Rev Immunol (2013) 31:605–33. doi: 10.1146/annurev-immunol-032712-100019
68. Herring AC, Lee J, McDonald RA, Toews GB, Huffnagle GB. Induction of interleukin-12 and Gamma Interferon Requires Tumor Necrosis Factor Alpha for Protective T1-cell-mediated Immunity to Pulmonary Cryptococcus Neoformans Infection. Infect Immun (2002) 70(6):2959–64. doi: 10.1128/IAI.70.6.2959-2964.2002
69. Balish E, Wagner RD, Vazquez-Torres A, Pierson C, Warner T. Candidiasis in Interferon-Gamma Knockout (IFN-Gamma-/-) Mice. J Infect Dis (1998) 178(2):478–87. doi: 10.1086/515645
70. Chen GH, McDonald RA, Wells JC, Huffnagle GB, Lukacs NW, Toews GB. The Gamma Interferon Receptor is Required for the Protective Pulmonary Inflammatory Response to Cryptococcus Neoformans. Infect Immun (2005) 73(3):1788–96. doi: 10.1128/IAI.73.3.1788-1796.2005
71. Delsing CE, Gresnigt MS, Leentjens J, Preijers F, Frager FA, Kox M, et al. Interferon-Gamma as Adjunctive Immunotherapy for Invasive Fungal Infections: A Case Series. BMC Infect Dis (2014) 14(166):2334–46. doi: 10.1186/1471-2334-14-166
72. Jarvis JN, Meintjes G, Rebe K, Williams GN, Bicanic T, Williams A, et al. Adjunctive Interferon-Gamma Immunotherapy for the Treatment of HIV-associated Cryptococcal Meningitis: A Randomized Controlled Trial. AIDS (2012) 26(9):1105–13. doi: 10.1097/QAD.0b013e3283536a93
73. Cenci E, Mencacci A, Bacci A, Bistoni F, Kurup VP, Romani L. T Cell Vaccination in Mice With Invasive Pulmonary Aspergillosis. J Immunol (2000) 165(1):381–8. doi: 10.4049/jimmunol.165.1.381
74. Perruccio K, Tosti A, Burchielli E, Topini F, Ruggeri L, Carotti A, et al. Transferring Functional Immune Responses to Pathogens After Haploidentical Hematopoietic Transplantation. Blood (2005) 106(13):4397–406. doi: 10.1182/blood-2005-05-1775
75. McGeachy MJ, Chen Y, Tato CM, Laurence A, Joyce-Shaikh B, Blumenschein WM, et al. The Interleukin 23 Receptor is Essential for the Terminal Differentiation of Interleukin 17-Producing Effector T Helper Cells In Vivo. Nat Immunol (2009) 10(3):314–24. doi: 10.1038/ni.1698
76. Zielinski CE, Mele F, Aschenbrenner D, Jarrossay D, Ronchi F, Gattorno M, et al. Pathogen-Induced Human TH17 Cells Produce IFN-gamma or IL-10 and are Regulated by IL-1beta. Nature (2012) 484(7395):514–8. doi: 10.1038/nature10957
77. McGeachy MJ, Cua DJ, Gaffen SL. The IL-17 Family of Cytokines in Health and Disease. Immunity (2019) 50(4):892–906. doi: 10.1016/j.immuni.2019.03.021
78. Conti HR, Gaffen SL. Il-17-Mediated Immunity to the Opportunistic Fungal Pathogen Candida Albicans. J Immunol (2015) 195(3):780–8. doi: 10.4049/jimmunol.1500909
79. Milner JD, Holland SM. The Cup Runneth Over: Lessons From the Ever-Expanding Pool of Primary Immunodeficiency Diseases. Nat Rev Immunol (2013) 13(9):635–48. doi: 10.1038/nri3493
80. Huang W, Na L, Fidel PL, Schwarzenberger P. Requirement of interleukin-17A for Systemic anti-Candida Albicans Host Defense in Mice. J Infect Dis (2004) 190(3):624–31. doi: 10.1086/422329
81. Ramani K, Garg AV, Jawale CV, Conti HR, Whibley N, Jackson EK, et al. The Kallikrein-Kinin System: A Novel Mediator of IL-17-Driven Anti-Candida Immunity in the Kidney. PloS Pathog (2016) 12(11):e1005952. doi: 10.1371/journal.ppat.1005952
82. Ramani K, Jawale CV, Verma AH, Coleman BM, Kolls JK, Biswas PS. Unexpected Kidney-Restricted Role for IL-17 Receptor Signaling in Defense Against Systemic Candida Albicans Infection. JCI Insight (2018) 3(9):e98241. doi: 10.1172/jci.insight.98241
83. Lin L, Ibrahim AS, Xu X, Farber JM, Avanesian V, Baquir B, et al. Th1-Th17 Cells Mediate Protective Adaptive Immunity Against Staphylococcus Aureus and Candida Albicans Infection in Mice. PloS Pathog (2009) 5(12):e1000703. doi: 10.1371/journal.ppat.1000703
84. Kleinschek MA, Muller U, Brodie SJ, Stenzel W, Kohler G, Blumenschein WM, et al. Il-23 Enhances the Inflammatory Cell Response in Cryptococcus Neoformans Infection and Induces a Cytokine Pattern Distinct From IL-12. J Immunol (2006) 176(2):1098–106. doi: 10.4049/jimmunol.176.2.1098
85. Mittrucker HW, Visekruna A, Huber M. Heterogeneity in the Differentiation and Function of CD8(+) T Cells. Arch Immunol Ther Exp (Warsz) (2014) 62(6):449–58. doi: 10.1007/s00005-014-0293-y
86. Mody CH, Chen GH, Jackson C, Curtis JL, Toews GB. In Vivo Depletion of Murine CD8 Positive T Cells Impairs Survival During Infection With a Highly Virulent Strain of Cryptococcus Neoformans. Mycopathologia (1994) 125(1):7–17. doi: 10.1007/BF01103969
87. Lindell DM, Moore TA, McDonald RA, Toews GB, Huffnagle GB. Generation of Antifungal Effector CD8+ T Cells in the Absence of CD4+ T Cells During Cryptococcus Neoformans Infection. J Immunol (2005) 174(12):7920–8. doi: 10.4049/jimmunol.174.12.7920
88. Nanjappa SG, Heninger E, Wuthrich M, Gasper DJ, Klein BS. Tc17 Cells Mediate Vaccine Immunity Against Lethal Fungal Pneumonia in Immune Deficient Hosts Lacking CD4+ T Cells. PloS Pathog (2012) 8(7):e1002771. doi: 10.1371/journal.ppat.1002771
89. Brena S, Cabezas-Olcoz J, Moragues MD, Fernandez de Larrinoa I, Dominguez A, Quindos G, et al. Fungicidal Monoclonal Antibody C7 Interferes With Iron Acquisition in Candida Albicans. Antimicrob Agents Chemother (2011) 55(7):3156–63. doi: 10.1128/AAC.00892-10
90. Xin H, Cutler JE. Vaccine and Monoclonal Antibody That Enhance Mouse Resistance to Candidiasis. Clin Vaccine Immunol (2011) 18(10):1656–67. doi: 10.1128/CVI.05215-11
91. Moragues MD, Omaetxebarria MJ, Elguezabal N, Sevilla MJ, Conti S, Polonelli L, et al. A Monoclonal Antibody Directed Against a Candida Albicans Cell Wall Mannoprotein Exerts Three anti-C. Albicans Activities. Infect Immun (2003) 71(9):5273–9. doi: 10.1128/IAI.71.9.5273-5279.2003
92. Torosantucci A, Bromuro C, Chiani P, De Bernardis F, Berti F, Galli C, et al. A Novel Glyco-Conjugate Vaccine Against Fungal Pathogens. J Exp Med (2005) 202(5):597–606. doi: 10.1084/jem.20050749
93. Nanjappa SG, Klein BS. Vaccine Immunity Against Fungal Infections. Curr Opin Immunol (2014) 28:27–33. doi: 10.1016/j.coi.2014.01.014
94. Sandini S, La Valle R, Deaglio S, Malavasi F, Cassone A, De Bernardis F. A Highly Immunogenic Recombinant and Truncated Protein of the Secreted Aspartic Proteases Family (rSap2t) of Candida Albicans as a Mucosal Anticandidal Vaccine. FEMS Immunol Med Microbiol (2011) 62(2):215–24. doi: 10.1111/j.1574-695X.2011.00802.x
95. Goldman DL, Khine H, Abadi J, Lindenberg DJ, Pirofski L, Niang R, et al. Serologic Evidence for Cryptococcus Neoformans Infection in Early Childhood. Pediatrics (2001) 107(5):E66. doi: 10.1542/peds.107.5.e66
96. Wang XJ, Sui X, Yan L, Wang Y, Cao YB, Jiang YY. Vaccines in the Treatment of Invasive Candidiasis. Virulence (2015) 6(4):309–15. doi: 10.4161/21505594.2014.983015
97. Schmidt CS, White CJ, Ibrahim AS, Filler SG, Fu Y, Yeaman MR, et al. Ndv-3, a Recombinant Alum-Adjuvanted Vaccine for Candida and Staphylococcus Aureus, is Safe and Immunogenic in Healthy Adults. Vaccine (2012) 30(52):7594–600. doi: 10.1016/j.vaccine.2012.10.038
98. Cassone A. Vulvovaginal Candida Albicans Infections: Pathogenesis, Immunity and Vaccine Prospects. BJOG (2015) 122(6):785–94. doi: 10.1111/1471-0528.12994
99. Edwards JE. Fungal Cell Wall Vaccines: An Update. J Med Microbiol (2012) 61(Pt 7):895–903. doi: 10.1099/jmm.0.041665-0
100. Saville SP, Lazzell AL, Chaturvedi AK, Monteagudo C, Lopez-Ribot JL. Efficacy of a Genetically Engineered Candida Albicans tet-NRG1 Strain as an Experimental Live Attenuated Vaccine Against Hematogenously Disseminated Candidiasis. Clin Vaccine Immunol (2009) 16(3):430–2. doi: 10.1128/CVI.00480-08
101. Ito JI, Lyons JM. Vaccination of Corticosteroid Immunosuppressed Mice Against Invasive Pulmonary Aspergillosis. J Infect Dis (2002) 186(6):869–71. doi: 10.1086/342509
102. Stuehler C, Khanna N, Bozza S, Zelante T, Moretti S, Kruhm M, et al. Cross-Protective TH1 Immunity Against Aspergillus Fumigatus and Candida Albicans. Blood (2011) 117(22):5881–91. doi: 10.1182/blood-2010-12-325084
103. Liu M, Clemons KV, Bigos M, Medovarska I, Brummer E, Stevens DA. Immune Responses Induced by Heat Killed Saccharomyces Cerevisiae: A Vaccine Against Fungal Infection. Vaccine (2011) 29(9):1745–53. doi: 10.1016/j.vaccine.2010.12.119
104. Stevens DA, Clemons KV, Liu M. Developing a Vaccine Against Aspergillosis. Med Mycol (2011) 49:170–6. doi: 10.3109/13693786.2010.497775
105. Datta K, Pirofski LA. Towards a Vaccine for Cryptococcus Neoformans: Principles and Caveats. FEMS Yeast Res (2006) 6(4):525–36. doi: 10.1111/j.1567-1364.2006.00073.x
106. Devi SJ, Schneerson R, Egan W, Ulrich TJ, Bryla D, Robbins JB, et al. Cryptococcus Neoformans Serotype A Glucuronoxylomannan-Protein Conjugate Vaccines: Synthesis, Characterization, and Immunogenicity. Infect Immun (1991) 59(10):3700–7. doi: 10.1128/IAI.59.10.3700-3707.1991
107. Casadevall A, Pirofski L. Insights Into Mechanisms of Antibody-Mediated Immunity From Studies With Cryptococcus Neoformans. Curr Mol Med (2005) 5(4):421–33. doi: 10.2174/1566524054022567
108. Wozniak KL, Young ML, Wormley FL Jr. Protective Immunity Against Experimental Pulmonary Cryptococcosis in T Cell-Depleted Mice. Clin Vaccine Immunol (2011) 18(5):717–23. doi: 10.1128/CVI.00036-11
109. Rella A, Mor V, Farnoud AM, Singh A, Shamseddine AA, Ivanova E, et al. Role of Sterylglucosidase 1 (Sgl1) on the Pathogenicity of Cryptococcus Neoformans: Potential Applications for Vaccine Development. Front Microbiol (2015) 6:836–43. doi: 10.3389/fmicb.2015.00836
110. Wuthrich M, Filutowicz HI, Warner T, Deepe GS Jr., Klein BS. Vaccine Immunity to Pathogenic Fungi Overcomes the Requirement for CD4 Help in Exogenous Antigen Presentation to CD8+ T Cells: Implications for Vaccine Development in Immune-Deficient Hosts. J Exp Med (2003) 197(11):1405–16. doi: 10.1084/jem.20030109
111. Munoz JE, Luft VD, Amorim J, Magalhaes A, Thomaz L, Nosanchuk JD, et al. Immunization With P10 Peptide Increases Specific Immunity and Protects Immunosuppressed BALB/c Mice Infected With Virulent Yeasts of Paracoccidioides Brasiliensis. Mycopathologia (2014) 178(3-4):177–88. doi: 10.1007/s11046-014-9801-1
112. Assis-Marques MA, Oliveira AF, Ruas LP, dos Reis TF, Roque-Barreira MC, Coelho PS. Saccharomyces Cerevisiae Expressing Gp43 Protects Mice Against Paracoccidioides Brasiliensis Infection. PloS One (2015) 10(3):e0120201. doi: 10.1371/journal.pone.0120201
113. Nascimento RC, Espindola NM, Castro RA, Teixeira PA, Loureiro y Penha CV, Lopes-Bezerra LM, et al. Passive Immunization With Monoclonal Antibody Against a 70-kDa Putative Adhesin of Sporothrix Schenckii Induces Protection in Murine Sporotrichosis. Eur J Immunol (2008) 38(11):3080–9. doi: 10.1002/eji.200838513
Keywords: fungus, invasive infections, immunity, memory response, vaccine
Citation: Biswas PS (2021) Vaccine-Induced Immunological Memory in Invasive Fungal Infections – A Dream so Close yet so Far. Front. Immunol. 12:671068. doi: 10.3389/fimmu.2021.671068
Received: 22 February 2021; Accepted: 01 April 2021;
Published: 21 April 2021.
Edited by:
Jigar V. Desai, National Institutes of Health (NIH), United StatesReviewed by:
Javier Carbone, Gregorio Marañón Hospital, SpainSusana Magadan, University of Vigo, Spain
Copyright © 2021 Biswas. This is an open-access article distributed under the terms of the Creative Commons Attribution License (CC BY). The use, distribution or reproduction in other forums is permitted, provided the original author(s) and the copyright owner(s) are credited and that the original publication in this journal is cited, in accordance with accepted academic practice. No use, distribution or reproduction is permitted which does not comply with these terms.
*Correspondence: Partha S. Biswas, psb13@pitt.edu