- 1Department of Translational Research & Cellular Therapeutics, Arthur Riggs Diabetes & Metabolism Research Institute, Beckman Research Institute, City of Hope National Medical Center, Duarte, CA, United States
- 2Division of Microbiology, University of Osnabrück, Osnabrück, Germany
- 3Faculty of Pharmacy, Zagazig University, Zagazig, Egypt
A therapy that includes an oral vaccine for type 1 diabetes (T1D) using live attenuated Salmonella MvP728 (ΔhtrA/ΔpurD), cytokines (IL10 and TGFβ) and preproinsulin (PPI) antigen in combination with a sub-therapeutic dose of anti-CD3 mAb was developed by our team. The vaccine combination therapy reduced insulitis and prevented and reversed diabetes in non-obese diabetic (NOD) mice. Here, we show the effectiveness of an alternative Salmonella mutant (ΔmsbB) as a carrier strain, which is anticipated to have lower risks of an inflammatory response and septicemia as a result of modification in the lipopolysaccharide (LPS) via detoxification of lipid A. This mutant strain proved to have highly reduced pathogenic side effects. Salmonella strain ΔmsbB expressed autoantigens and in combination with cytokines and anti-CD3 mAb, successfully prevented and reversed T1D to levels comparable to the previously used carrier strain ΔhtrA/ΔpurD. Additionally, the Salmonella msbB mutant resulted in higher rates of host cell infection. These results further demonstrate the potential of an oral Salmonella-based combined therapy in the treatment of early T1D.
Introduction
Oral delivery of antigens directly to the gut-associated lymphoid tissue (GALT) is an effective means of inducing tolerance (1–3). We have developed an oral Salmonella-based delivery system using the Salmonella Pathogenicity Island 2-encoded type III secretion system (SPI2-T3SS) effective in delivering an autoantigen (preproinsulin, PPI), in combination with plasmids encoding cytokines that are known to support the immune suppression (TGFβ and IL-10), to prevent and reverse type 1 diabetes (T1D) in non-obese diabetic (NOD) mice (4–6). The co-administration of a short-course of anti-CD3 monoclonal antibody along with a reduced dose of Salmonella-based vaccine and PPI/TGFβ/IL10 preserved insulin-positive cells, reduced insulitis and prevented/reversed T1D in NOD mice (5, 6).
The delivery system exploits Salmonella’s natural ability to survive and thrive in the vacuoles of GALT phagosomes (7–9). The Salmonella-containing vacuoles (SCV) within antigen-presenting cells (APCs) allow for self-propagation of the bacteria. From this location, the bacteria safely deliver a recombinant antigen into the host cell cytosol or deliver vectors that are expressed by the host cell directly, while bypassing the intestinal degradation (7, 9–11). This delayed delivery, or in vivo expression method utilizing the SPI2-T3SS (12, 13) increases the safety and efficacy of treatment by ensuring that the antigen expression only occurs after bacterial uptake by the APCs. The recombinant antigen is then processed and presented by the host APCs to other GALT cells, which then migrate to distant organs (14). The flexibility of the system allows rapid development of new immunotherapies which provides a robust delivery of multiple antigens in a safe and inexpensive manner. Such vaccines were very effective in eliciting CD8 and CD4 T cell-mediated immune responses in animal models of infection and cancer (7, 11, 15, 16).
A suitable Salmonella strain requires a balance between immune stimulation and safety. Wild type bacteria can cause fatal septicemia, yet overly attenuated bacteria will not stimulate the immune system. Previously, the double mutant strain MvP728 of Salmonella (ΔhtrA/ΔpurD) (4–7) was employed as a carrier. This strain was effective in the prevention and reversal of T1D in NOD mice (4–6). Yet there is some risk with this strain due to the presence of endotoxic lipopolysaccharide (LPS) in the cell envelope.
In Gram-negative bacteria like Salmonella, the LPS-containing cell envelope serves as a vital defense mechanism for Salmonella to survive in the host (17, 18). Modification of Salmonella LPS is a logical way to develop a safer vector (17, 19) by balancing the function with attenuation. Lipid A is a conserved LPS moiety composed of core oligosaccharide and acyl chains (20, 21). Wild-type LPS contains a mixture of hexa- and hepta-acylated lipid A, which strongly stimulates the Toll-like receptor-4 (TLR4) to induce an inflammatory response (22). Modifying the amount of lipid A acylation may limit its ability to activate TLR4. The Salmonella enzyme myristoyl transferase (MsbB) is known to modify LPS by adding myristic acid residues to lipid A, resulting in a hexa-acylated lipid (22). Lipid A is also modified by acyltransferase adding a palmitic acid to yield hepta-acylated lipid A (20). Mutating msbB resulted in one fewer acyl chains on lipid A and created a mixture of penta- and hexa-acylated lipid A. The ΔmsbB altered the LPS, while maintaining an agonist effect on TLR4 induces weaker proinflammatory signaling than wild-type LPS (20, 21).
In this study Salmonella ΔmsbB (18, 20, 22) virulence and its use as a potential delivery system for autoantigens was assessed as an alternative to the Salmonella double mutant ΔhtrA/ΔpurD. Also, the combination therapy of ΔmsbB (PI+TGFβ+IL10) with anti-CD3 mAb to prevent and revert new-onset diabetes in NOD mice was tested.
Materials and Methods
Preparation of Salmonella Vaccines
The Salmonella strains and plasmids used in this study are listed in Table 1. The msbB deletion strain was generated as previously described (10, 23, 25) by λ Red-meditated deletion and insertion of an aph cassette using primers listed in Table 2. After confirmation of the resulting mutant strain, the aph cassette was deleted by flippase (FLP)-mediated site-specific recombination target (FRT) (26–28) to yield ST55 (ΔmsbB, Table 1). The expression plasmids were prepared and transformed into an attenuated strain of S. typhimurium MvP728 (ΔhtrA/ΔpurD) (7), MvP1553 (ΔrfaD) and ΔmsbB as described (4). Bacteria were cultured in Luria-Bertani (LB). If required for selection, ampicillin (100μg/ml), kanamycin and/or carbenicillin (50µg/ml) was added. The Salmonella mutant ΔmsbB was cultured in LB media without sodium chloride as the strain is sensitive to high osmolarity (29). The mouse preproinsulin (mPPI) and mouse proinsulin (mPI) were expressed by the Salmonella SPI2-T3SS as fusion to effector protein (SseF) under the control of intracellular-activated promoter (PsseA) to mediate the translocation into host cell cytoplasm. The cytokine TGFβ and IL10 were expressed and secreted by host cells under the control of cytomegalovirus (CMV) immediate early promoter (4).
Plasmid Construction
All PCR amplifications were performed using a polymerase with proof-reading activity (Thermo Fisher Scientific, MA). Plasmid manipulations were performed according to standard procedures and the resulting plasmid constructs were confirmed by DNA sequencing (9, 23). The recombinant plasmids were transformed into DH5α E. coli TOP 10 competent cells (Invitrogen, CA) using standard procedure. Confirmed plasmid constructs were transformed into Salmonella strains by electroporation using the micro pulsar 2.5 kV, 200 Ω and 25 μF (Bio-Rad) (9, 23).
Plasmid Construction of gfp Reporter
To test the expression level of SPI2-T3SS genes in different mutants of S. typhimurium, green fluorescent protein (GFP) was employed and expressed under promoter control of an operon in SPI2-T3SS of Salmonella. The DNA sequence encoding GFP was amplified from pFPV25.1 using the primers GFP-EcoRV-For/GFP-XbaI-Rev (Table 2). The gfp sequence was digested with EcoRV/XbaI and subcloned into EcoRV/XbaI digested p2810 resulting in pMH 531 (Table 1). In the plasmid pMH 531, the expression of gfp gene was under the control of the SPI2 promoter. GFP can then be produced as a fusion protein with SseF (11).
Plasmid Construction of tgfβ1 and 1l10 in pVAX1
Mouse cytokines TGFβ and IL10 were used as immunomodulators and were expressed by host cells under the control of the CMV promoter (4–6). The mammalian expression vector pVAX1 (Invitrogen, CA) was used to be consistent with the Food and Drug Administration (FDA) guidelines. For the construction of recombinant mouse TGFβ and mouse IL10 into pVAX1, PCR amplification of mouse tgfβ1 and il10 (from Origen, Table 1) sequences using the primers TGFb-HindII-For/TGFb-XhoI-Rev and mIL10-HindII-For/mIL10-XhoI-Rev was undertaken (Table 2). During the digestion with HindII/XhoI, the products were subcloned into HindII/XhoI that was digested by pVAX1 to produce pMH 524 and pMH 525 (Table 1).
Plasmid Construction of Mouse Proinsulin
The ORF of mouse proinsulin (mPI) was amplified from pMH 509 (Table 1) (4) using the primers mPI-HpaI-For/MycDDK-XbaI-Rev (Table 2) and digested with HpaI/XbaI. This is followed by sub-cloning into plasmid p2810 that was digested with EcoRV/XbaI to produce plasmid pMH 532 (Table 1). The plasmid expression vector of mouse proinsulin was under the control of the SPI2 promoter PsseA and fused with SseF, which itself mediates the translocation to the host cell cytoplasm.
Animal Vaccination
Seven-week-old female NOD/ShiLtJ mice were obtained from The Jackson Laboratory (Bar Harbor, ME) and received high-quality care at the animal facility at the City of Hope (COH) (4–6). The study was approved by the COH Institutional Animal Care and Use Committee (IACUC# 18017).
Normoglycemic or diabetic NOD mice were vaccinated by gavage as published (4–6) with 107 CFU/mouse of Salmonella delivering plasmids for the expression of TGFβ+IL10 plus 105 CFU/mouse of Salmonella expressing sseF-PPI. The total volume delivered bacteria per animal was 200µl of a 5% sodium bicarbonate solution. Animals have received the vaccine on days 0 and 7. Vehicle control mice were gavaged with 200µl of a 5% sodium bicarbonate solution. Mice were treated for 5 consecutive days i.p. (days -1 to 3 start of vaccination; 2.5 μg/mouse) with hamster anti-CD3 mAb as published (4–6). Blood glucose was measured every 3-4 days with a One Touch Ultra glucometer (LifeScan, Milpitas, CA). Mice were considered diabetic when two consecutive blood glucose values were more than 200mg/dL (11.1 mM).
In Vitro Infection
Murine RAW264.7 macrophages were obtained from the American Type Culture Collection (ATCC no.-TIB-71) and maintained according to the provider’s instructions. Cultures were treated with Salmonella expressing GFP (pMH531) or Salmonella expressing mPPI (pMH509) at a multiplicity of infection of 10 for 25 min, washed, and cultured for 16–20 hours prior to expression analysis (11). The cells were immune-stained as previously described (7). Briefly, after infection, the cells were fixed with BD Cyofix/Cytoperm buffer at room temperature for 20 min and then washed 3 times with BD Cyofix/Cytoperm washing buffer. The slides containing Salmonella expressing mPPI were incubated with mouse anti-LPS of S. typhimurium O-4 (1E6) (Santa Cruz Biotechnology, CA, 1:50) and Guinea Pig anti-insulin (Abcam, 1:50) for 1 hour. Goat anti-mouse Alex-Flour-488 (Biolegend, 1/100) and anti-Guinea Pig-Cy3 (Abcam, 1/50) were used as secondary antibodies to detect Salmonella and PPI, respectively. The slides were mounted on VECTASHIELD antifade reagent containing DAPI (VECTOR Laboratories) and sealed with Nail polish (Sally Hansen). Fluorescent images were acquired on a Zeiss observer II fluorescence microscope equipped with a digital imaging camera (Zeiss AxioCam 506). Images were captured using ZEN Blue light software. Salmonella expressing GFP or PPI were quantified from 10 to 18 infected cells obtained from 5 to 8 fields using ImageJ software.
Virulence and Fecal Shedding of ΔmsbB Strain
Seven-week-old female NOD mice were orally administered with 105, 107, and 109 CFU/mouse of the Salmonella msbB mutant. Mice were weighed every 48 hours and stool were collected every 48-72 h over 3 weeks. To analyze the shedding of Salmonella strains in vaccinated mice, approximately 100 mg of fresh stool was homogenized in 1 ml of PBS. LB agar plates deficient in sodium chloride and containing selective antibiotics were swabbed and the bacteria were characterized.
Statistical Analysis
Kaplan-Meier survival curves were used to evaluate the incidence of diabetes between different groups of mice. The significance of the differences was determined by Mantel-Cox log-rank test analysis. For analysis of GFP- or PPI-expression between different Salmonella mutants, one-way ANOVA for unpaired values was used. All data were reported as mean ± SEM. Statistical calculations were performed using GraphPad Prism 9 software and a p value of <0.05 was considered significant.
Results
In Vitro Assessment of Salmonella-Based Expression
The expression of Salmonella SPI2-T3SS system as a vaccine carrier using Salmonella mutated in LPS such as MvP1553 (ΔrfaD) and ΔmsbB along with the previously tested MvP728 (ΔhtrA/ΔpurD) was compared. All Salmonella mutants were transformed with a plasmid for expression of GFP under the control of SPI2 promoter and were used to infect RAW264.7 macrophages. After 20 hours of infection, significantly greater numbers of GFP-expressing Salmonella ΔmsbB were noted in macrophages compared to GFP-expressing Salmonella ΔrfaD and ΔhtrA/purD (Figure 1A). Quantification of GFP intensity per infected host cell indicated increased signal intensities for the ΔmsbB strain compared to ΔrfaD and ΔhtrA/purD strains (Figure 1B).
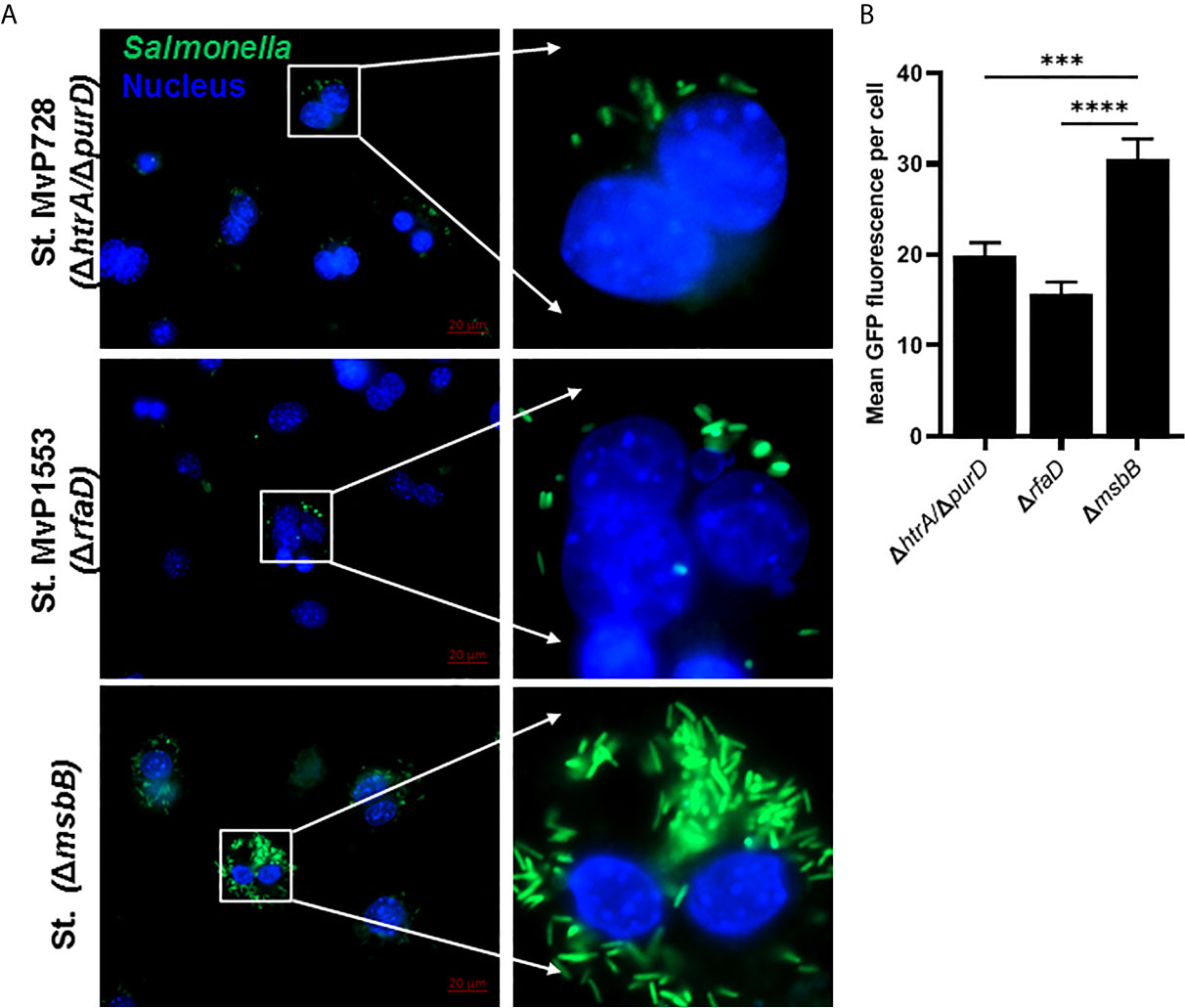
Figure 1 In vitro comparison of Salmonella SPI2-based protein expression in macrophage infected with different Salmonella mutants. RAW264.7 macrophages were infected with Salmonella mutants MvP728 (ΔhtrAΔ/purD), MvP1553(ΔrfaD) or ΔmsbB all of which were transformed with a plasmid encoding for GFP in frame with sseF under control of an intracellular promoter (pMH531, Table 1). Twenty hours after infection, cells were fixed and stained with DAPI for nuclei (blue) followed by analysis with Zeiss observer II fluorescence microscope to assess GFP within the host cells. Pictures were captured using ZEN Blue software. (A) A representative image (63X magnification, scale bar 20 µm) is shown. (B) Quantification of the mean fluorescence (green) of Salmonella expressing GFP per cell ± SEM. Data based on assessment of 15 to 18 infected cells in 5 to 8 fields. One-way ANOVA was used to determine significance (***p < 0.001 and ****p < 0.0001).
To evaluate the expression and translocation of the foreign protein into the host cell cytosol via the SPI2-T3SS of Salmonella, murine macrophages were infected with different Salmonella mutants carrying the mPPI construct, namely a fusion protein of SPI2-T3SS effector SseF and mPPI. To examine whether the attenuation was altered the SPI2-T3SS-mediated expression and translocation, we used Salmonella mutants ΔmsbB and ΔrfaD compared to ΔhtrA/ΔpurD. The mutant strain deficient in msbB translocated PPI into the cytosol of macrophages more than both Salmonella ΔrfaD and Salmonella ΔhtrA/ΔpurD (Figure 2A). After infection of macrophages, the mean green fluorescence (for Salmonella) and red fluorescence (for PPI) per cell was quantified (Figures 2B, C). A significant increase in the mean green and red fluorescence intensities was seen in the ΔmsbB compared to the ΔrfaD and ΔhtrA/ΔpurD strains (Supplementary Figure 1).
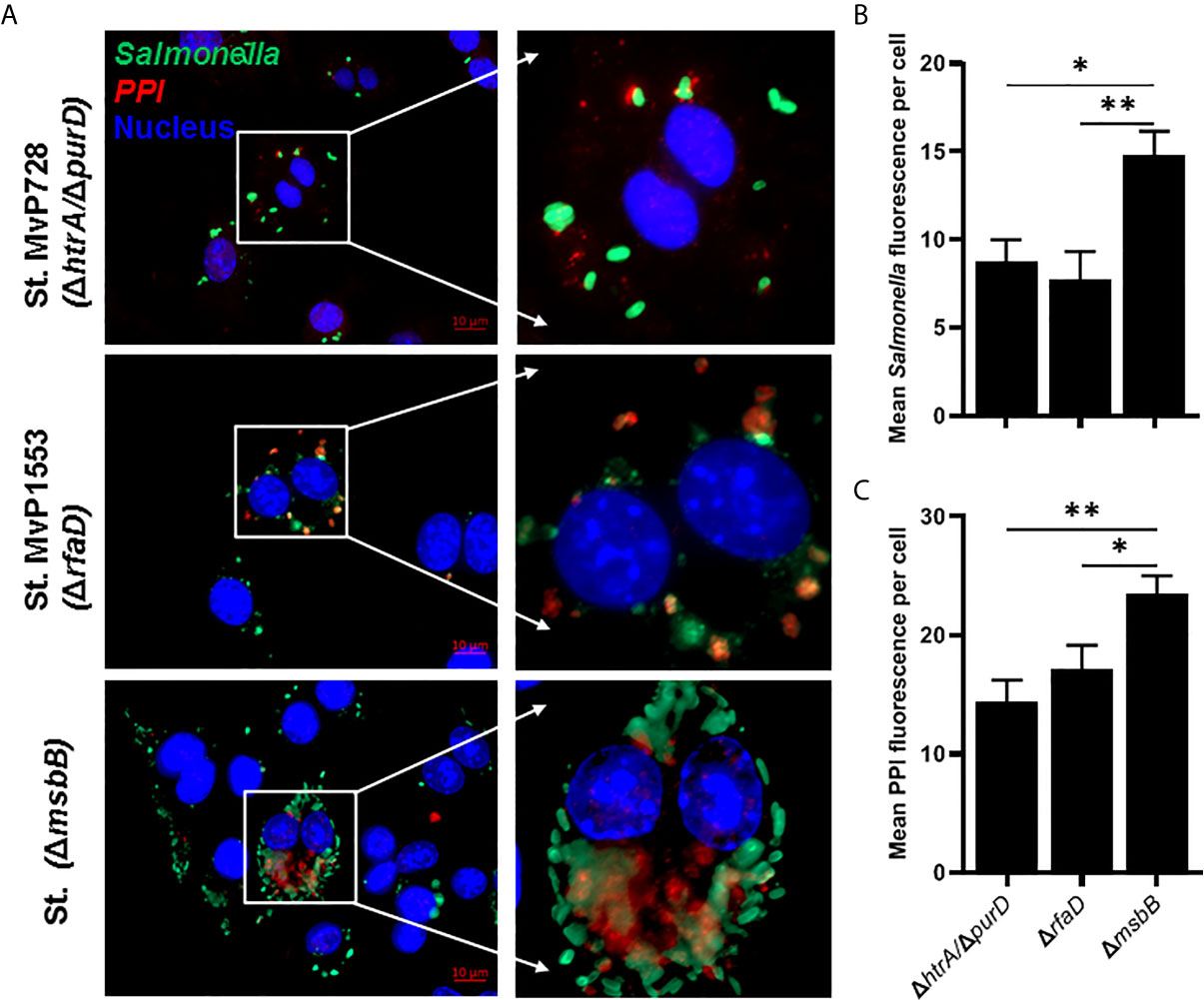
Figure 2 In vitro comparison of the translocation of PPI in a SPI2-T3SS-dependent manner between different Salmonella mutants. RAW264.7 macrophage cells were infected with Salmonella mutants MvP728 (ΔhtrA/ΔpurD), MvP1553(ΔrfaD) or ΔmsbB harboring a plasmid encoding for mPPI in the frame with SseF, and effector protein of the SPI2-T3SS, and expressed under control of an intracellular promoter (pMH509, Table 1). After infection for 20 hours, cells were fixed and stained with Guinea Pig anti-insulin polyclonal and anti-Salmonella monoclonal primary antibodies. Secondary antibodies were applied for detection of insulin (red), Salmonella (green), and DAPI was used for staining of nuclei (blue) followed by analysis as in Figure 1. Cells were then visualized for the presence of bacteria located within the cytosol of host cells with colocalized red clusters of PPI. (A) A representative image (63X magnification, scale bar 10 µm) is displayed. (B) Quantification of the mean Salmonella fluorescence (green) per cell ± SEM. (C) Quantification of the mean PPI fluorescence (red) per cell ± SEM. Salmonella expressing PPI was quantified from 12 to 16 infected cells in 5 to 8 fields and results displayed are representative of 2 independent experiments. Statistical analysis using one-way ANOVA. The significance level is indicated by asterisks (*p < 0.05 and **p < 0.01).
Virulence and Shedding of the msbB Mutant Strain
The Salmonella ΔmsbB strain was used as an anti-cancer vaccine (30, 31). However, this strain was not previously studied for vaccination in diabetic NOD mice. We determined the safety of ΔmsbB as a potential vaccine carrier. After oral administration of various doses of Salmonella msbB mutant, no mortality in NOD mice was observed. Mice tolerated up to 109 CFU/mouse for three weeks post-vaccination (Figure 3A). Animals displayed no changes in their weights before and after vaccination (Figure 3B and Supplementary Figure 2). Additionally, no changes in the activity of treated mice were noted. Analysis of the fecal shedding indicated that the recombinant vaccine strain was present in the gastrointestinal tract for up to 3 weeks post-vaccination (Figure 3C).
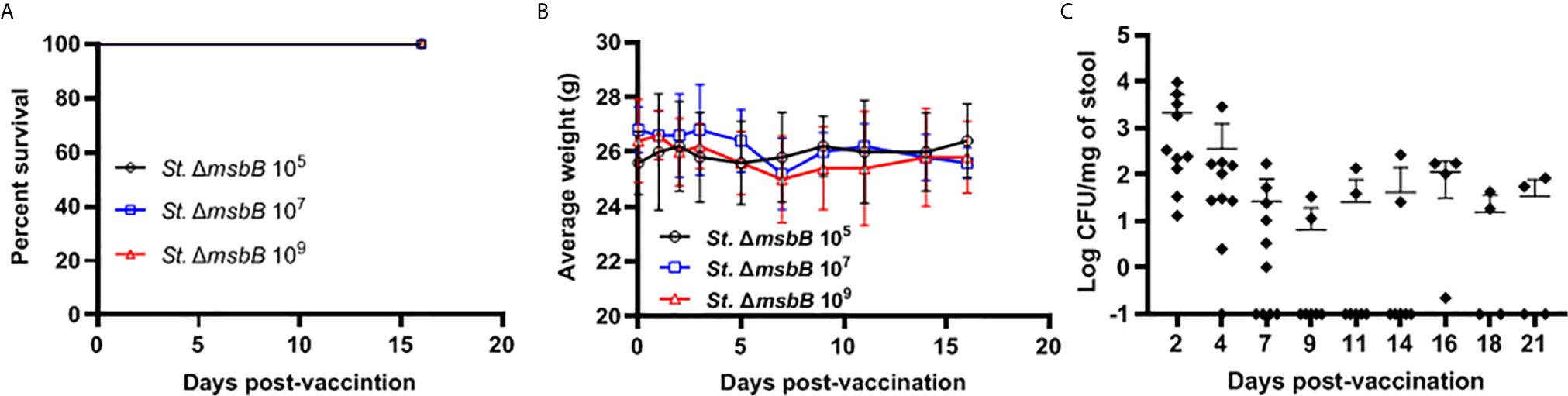
Figure 3 Virulence and shedding of msbB mutant strain. NOD mice were administered orally Salmonella ΔmsbB at CFU values of 105, 107, or 109 per mouse. (A) The survival of treated mice was followed for over 3 weeks post-vaccination (5 mice for each dose). (B) The side effects of ΔmsbB were monitored by tracking the average of body weights for each group of mice over the time of the experiment. The data at each time point is represented the mean ± SD. (C) The presence of the ΔmsbB in feces was determined over a period of 21 days (days 2, 4, 7, 9, 11, 14, 16, 18, and 21) post-vaccination. The means are indicated by horizontal bars ± SD (the number of mice ranged between 7-10 at each time point).
Salmonella msbB Mutant as a Vector for Prevention of Diabetes in NOD Mice
We showed that two doses of Salmonella-based combined therapy (PPI+TGFβ+IL10) with a sub-therapeutic dose of anti-CD3 mAb prevented diabetes in NOD mice (4–6). The orally administered ΔmsbB strain carrying both constructs for mPPI, mIL10, and/or mTGFβ was given in two doses at days 0 and 7 to normoglycemic NOD mice. Mice were injected with anti-CD3 antibody for five consecutive days (-1 to 3) (Figure 4A). Control mice were given the vehicle alone. Following administration, effects on blood glucose were assessed for 100 days (Figure 4A). A majority (80%) of the vaccinated NOD mice did not develop diabetes during the post-vaccination interval (Figure 4B) (Log-rank Mantel-Cox, p = 0.0064). In addition, the combination therapy-maintained blood glucose levels within a normal range in 80% of animals, compared with only 20% of animals given the vehicle (Figure 4C).
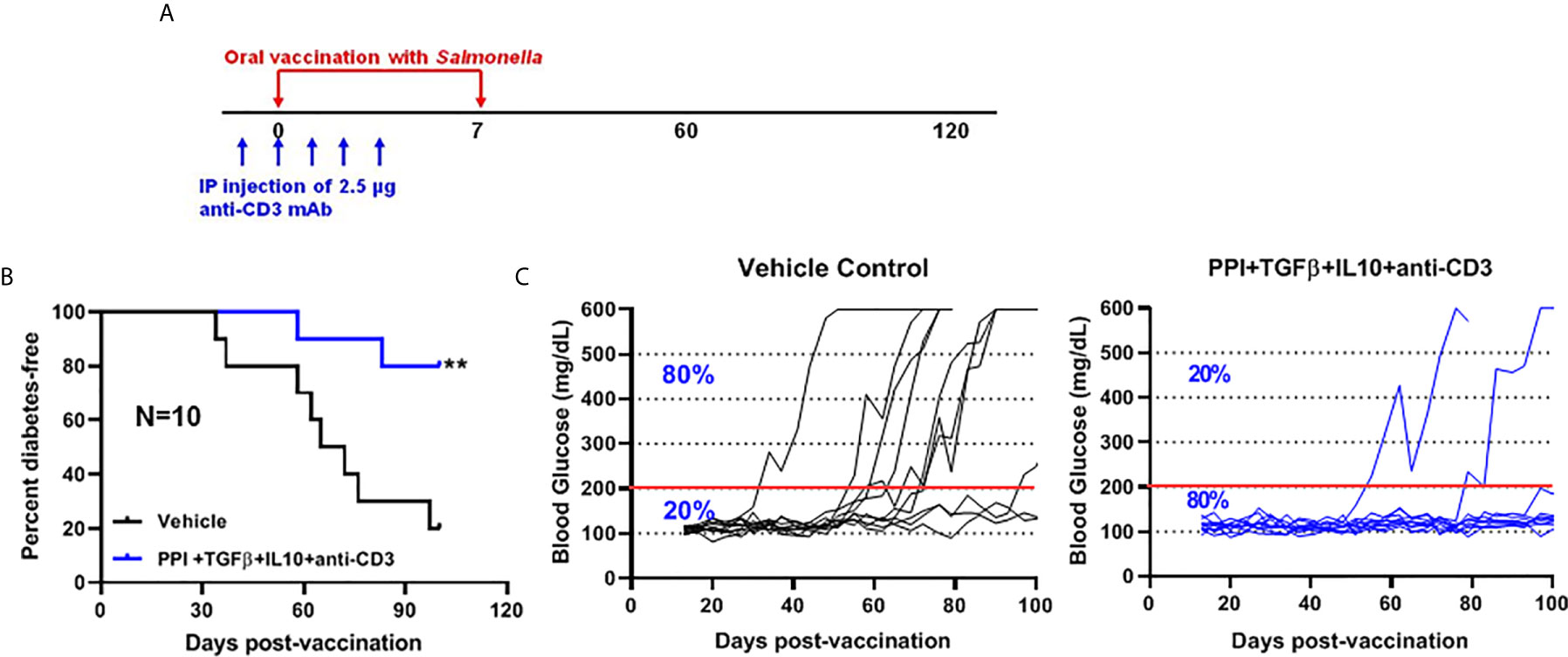
Figure 4 The oral Salmonella msbB mutant reduced the incidence of diabetes and prevented hyperglycemia. (A) NOD mice were vaccinated orally with a cocktail of Salmonella ΔmsbB cultures transformed with plasmids encoding mPPI, mTGFβ, and mIL10 at days 0 and 7. Mice received anti-CD3 mAb for 5 consecutive days (-1 to 3). Blood glucose levels were measured every 3-4 days for 100 days. (B) Combined vaccine therapy using ΔmsbB decreased the incidence of diabetes in NOD mice. Data is presented as the Log-rank plot of the percentage of NOD mice that remained diabetes-free. The difference between the group of mice vaccinated with combined therapy and the vehicle group was significant (**p < 0.01) by the log-rank (Mantel-Cox) test. (C) Combined vaccine using ΔmsbB prevented hyperglycemia and stabilized blood glucose levels in NOD mice. N represents the number of the mice per treatment group. The red line indicates the threshold blood glucose level of 200 mg/dL.
Salmonella-Based Combination Therapy Using an Oral Live msbB Mutant Prevents Diabetes in NOD Mice
The role of combination therapy (PPI+TFGβ+IL10) with anti-CD3 for the prevention of diabetes in NOD mice, vaccinated with Salmonella ΔhtrAΔ/purD mutant, was reported (5, 6, 32). Normoglycemic NOD mice were administered orally with Salmonella ΔmsbB expressing autoantigen proinsulin (PI) combined with or without TGFβ and IL10 combined with a sub-therapeutic dose (2.5 mg/mouse) of anti-CD3 mAb. Animals administered with Salmonella ΔmsbB along with the combination of PI+TGFβ+IL10 and anti-CD3 antibody were prevented from developing diabetes (Log-rank Mantel-Cox, p = 0.006) for more than 100 days post-treatment compared to control mice (Figure 5A). Interestingly, 60% of NOD mice were prevented from developing diabetes (Log-rank Mantel-Cox, p = 0.03) when treated with either PI+TGFβ or PI+IL10 plus anti-CD3 mAb compared to vehicle (Figure 5B). Further, orally administered Salmonella ΔmsbB in combination with PI+TGFβ+IL10 and anti-CD3 mAb prevented the hyperglycemia and normalized blood glucose levels in 80% of mice for more than 100 days post-vaccination (Figure 5C) compared to 20% of mice, given vehicle alone, and 60% of the mice treated with either PI+TGFβ or PI+IL10 and anti-CD3 mAb (Figure 5C).
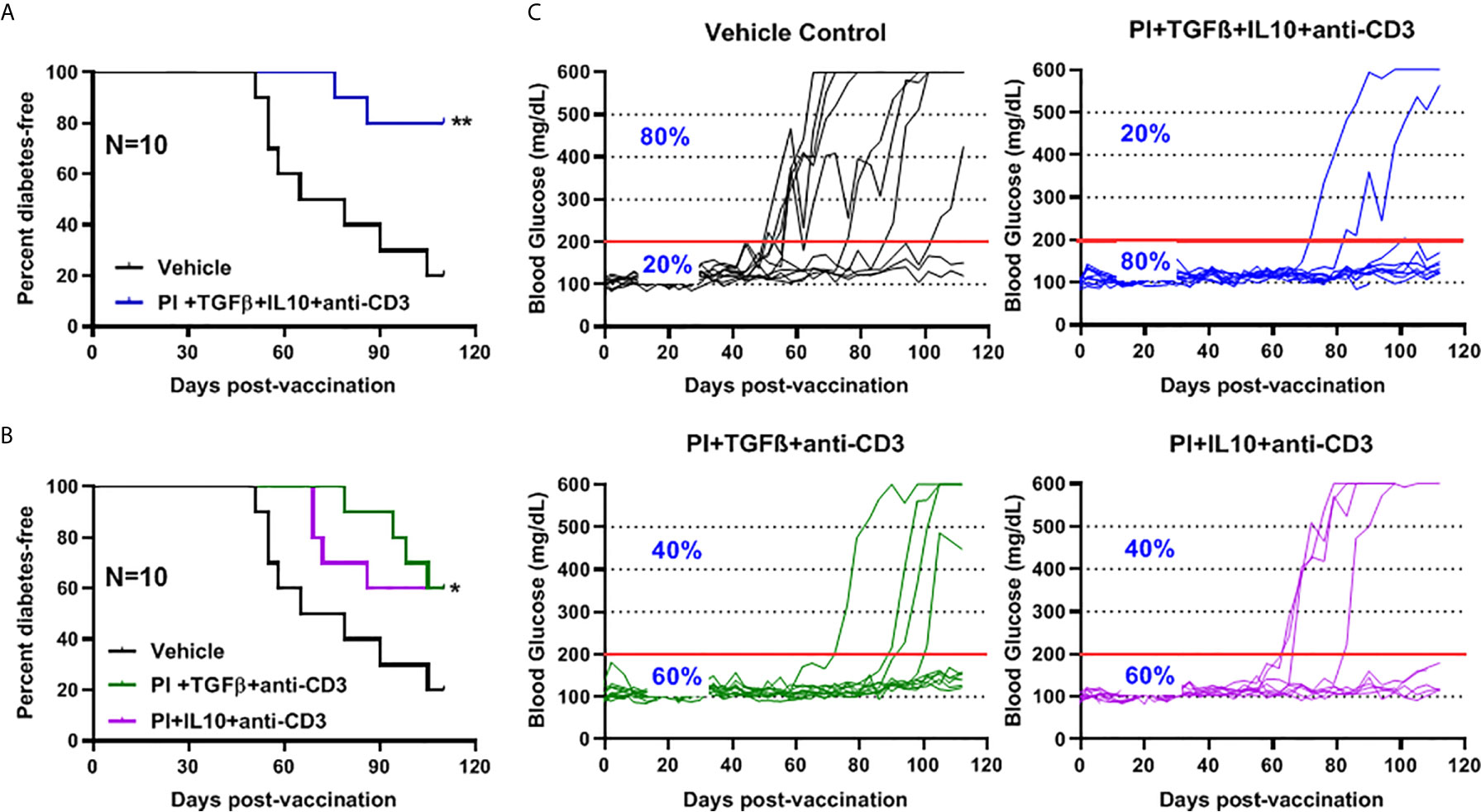
Figure 5 Combination therapy further reduced the incidence of diabetes and prevented hyperglycemia in NOD mice. NOD mice were orally vaccinated with a combination of Salmonella ΔmsbB transformed with mPI+TGFβ+IL10, mPI+TGFβ or, mPI+IL10 and anti-CD3 mAb. Blood glucose levels were measured every 3-4 days for 100 days post-vaccination. (A) Combined vaccine therapy (mPI+TGFβ+IL10) decreased the incidence of diabetes in NOD mice compared to vehicle treated mice. (B) Combined vaccine therapy (mPI+TGFβ) or (mPI+IL10) decreased the incidence of diabetes in NOD mice compared to control mice. Data are presented as the Log-rank plot of the percentage of NOD mice that remained diabetes-free over the time course of the study. The difference between the group of mice vaccinated with combined therapy and the vehicle group was significant (*p < 0.05 and **p < 0.01) by the log-rank (Mantel-Cox) test. (C) Combined vaccine using msbB mutant prevented hyperglycemia and stabilized blood glucose levels in NOD mice. N represents the number of the mice per treatment group. The red line indicates the threshold blood glucose level of 200 mg/dL.
Salmonella msbB Mutant as Therapeutic Vaccination Vector for Reversal of Diabetes in NOD Mice
To further investigate the safety and efficacy of the msbB, we assessed its potential to reverse diabetes. Early-stage diabetic NOD mice received the mutant vaccine carrying the PPI+TGFβ+IL10 constructs by gavage on days 0 and 7 and anti-CD3 mAb for five consecutive days (-1 to 3) post-vaccination (Figure 6A). Over 60% of vaccinated diabetic NOD mice that showed blood glucose levels < 200 mg/dL were persisted during the entire post-vaccination interval (Figure 6B). Interestingly, 75% of diabetic NOD mice with blood glucose levels less than 400 mg/dL were reversed to normal blood levels (less than 200 mg/dL) after vaccination (Figure 6C).
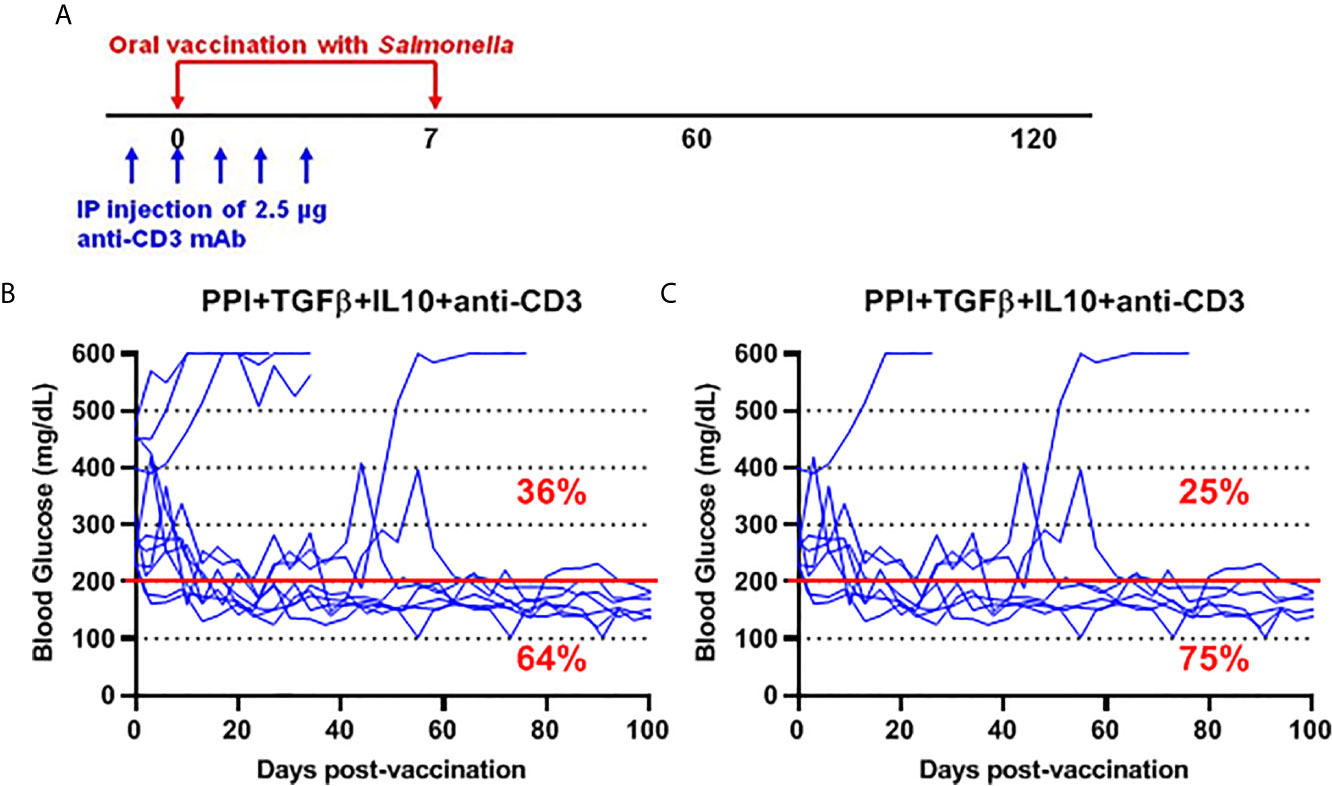
Figure 6 Salmonella-based combination therapy using the msbB mutant reversed diabetes in NOD mice. (A) New-onset diabetic NOD mice were vaccinated orally with a cocktail of ΔmsbB transformed with plasmids encoding mPPI+mTGFβ+mIL10 at days 0 and 7. Mice received anti-CD3 mAb for 5 consecutive days at days (-1 to 3) post-vaccination. (B) Reversal of diabetes in mice after vaccination. (C) Reversal of diabetes in vaccinated mice with blood glucose less than 400 mg/dL. Data displayed as the blood glucose levels in NOD mice for 100 days. The red line indicates the threshold blood glucose level of 200 mg/dL.
Discussion
Although the Salmonella ΔhtrA/ΔpurD strain is attenuated, it retains outer membrane LPS that could, in theory, promote endotoxic shock. The goal of this study was to investigate the use of less toxic Salmonella strains as an oral Salmonella-based vaccine to prevent and revert diabetes in NOD mice. Our established Salmonella-based vaccine uses diabetic autoantigen expressed by the SPI2-T3SS construct inside APCs. It was not known how the SPI2-T3SS would function in Salmonella with mutated LPS like ΔrfaD and ΔmsbB. We used GFP as a reporter protein that was expressed under control of a promoter in SPI2-T3SS of Salmonella. Elevated green fluorescence was noted in macrophages carrying the Salmonella ΔmsbB which decreased for cells harboring the ΔrfaD and ΔhtrA/ΔpurD strains.
Salmonella ΔmsbB was considered a potential therapeutic vaccine for cancer treatment (30, 31). Salmonella strain with a mutation in msbB (strain VNP20009) was used in a phase I trial in individuals with cancer (33). While the study did not show therapeutic effect, it did show the safety of the strain. The msbB mutation employed results in a modified version of LPS on the outer membrane that resulted in decreased TLR4 signaling and virulence in vivo (20, 22). Additionally, deletion of the Salmonella msbB gene resulted in decreased induction of TNFα when bacteria were administered to animals (20, 29).
Herein, we showed that a recombinant Salmonella msbB mutant combined with anti-CD3 mAb provided protection from diabetes in NOD mice. No side effects or unwanted reactions such as increased mortality, weight loss or change in activity was noted even after a high dose of the vaccine was given. However, we observed shedding of the Salmonella ΔmsbB strain for at least 3 weeks. The msbB mutant was able to express and translocate high amounts of protein in vitro. Interestingly, large numbers of Salmonella ΔmsbB were found inside infected cells and this was more than for MvP728 (ΔhtrA/ΔpurD) and MvP1553 (ΔrfaD). This finding suggests better survival and replication of the msbB mutant compared to other strains. Furthermore, the mutant bacteria delivered recombinant antigen into the host cell cytosol. The lesser effectiveness of the MvP1553 (ΔrfaD) mutant could be due to the high attenuation and/or the type of LPS modification, although this remains to be determined. The rfaD mutation affects assembly of the polymeric O-antigen of LPS and the mutant strains have a ‘rough’ phenotype. This results in loss of virulence due to fast clearance by antimicrobial effectors. This safety comes as the price of lower immune stimulation (18). In contrast, the ΔmsbB strain possesses polymeric O-antigen but a detoxified LPS lipid A (18).
We observed fecal shedding of the ΔmsbB strain for up to 9 days post-vaccination for most of the vaccinated mice. However, a small number of mice showed prolonged fecal shedding. This indicates the need for further attenuating mutations to ensure clearance of the vaccine. Examples of attenuating mutations include deletion of lppAB which encodes Braun’s lipoprotein (34) or the purD mutation present in VNP20009 (35). Another strategy for attenuation is based on conditional auxotrophy of the carrier strain (18). However, for therapeutic vaccines applied under close clinical supervision, less attenuation but efficient vaccine carrier strains can be considered as the strains can be controlled and eliminated by antibiosis.
The data herein indicated that the msbB mutant is suitable carrier for diabetes prevention (80%) in NOD mice. Still, enhanced infectivity may account for some of the better outcomes seen with the msbB mutant. Mice vaccinated with oral Salmonella msbB mutant showed persistence of the organisms for at least 3 weeks. This is long enough to allow expression and translocation of SPI2-T3SS effector proteins and presentation to immune cells (11). Interestingly, using mouse proinsulin (mPI) instead of mouse preproinsulin (mPPI) autoantigen in combination therapy prevents diabetes in 60% of vaccinated NOD mice compared to 20% in vehicle treated mice. The prevention of the incidence of diabetes is increased to 80% after vaccination with ΔmsbB and PI+TGFβ+IL10+anti-CD3. This result highlights the importance of combination therapy for maximum effect.
Furthermore, the data demonstrate that the Salmonella msbB mutant reverted new onset diabetes (65%) in NOD mice. Interestingly, 75% of diabetic NOD mice with initial blood glucose levels less than 400 mg/dL demonstrated normal blood levels after vaccination. This suggests that combination therapy can reverse new onset diabetes when given after disease onset. Importantly, new onset disease is associated with the residual beta cell function. However, in instances of long-standing T1D there is a paucity of residual beta cell mass likely adversely impacting the vaccine effectiveness.
In summary, the Salmonella ΔmsbB mutant is a potential vaccine for prevention and reversal of new onset diabetes. Further, the Salmonella msbB mutant poses less inflammatory risk. These results support the development of a Salmonella msbB mutant as a vaccine vector for clinical application.
Data Availability Statement
The raw data supporting the conclusions of this article will be made available by the corresponding author, without undue reservation.
Ethics Statement
The study was approved by the City of Hope Institutional Animal Care and Use Committee (IACUC# 18017).
Author Contributions
MIH planned the experiments. MIH, JC, JR, and NG performed the experiments and collected data. MH generated plasmids and bacterial strains. JC, MIH, and FK analyzed the data. JC, MIH, and FK reviewed the data and wrote the manuscript. MIH and MH wrote and edited the submitted manuscript. MIH submitted the manuscript and was responsible for the accuracy of the data. All authors contributed to the article and approved the submitted version.
Funding
This work was supported by the Wanek Family Project to Cure Type 1 Diabetes (award to MIH).
Conflict of Interest
The authors declare that the research was conducted in the absence of any commercial or financial relationships that could be construed as a potential conflict of interest.
Supplementary Material
The Supplementary Material for this article can be found online at: https://www.frontiersin.org/articles/10.3389/fimmu.2021.667897/full#supplementary-material
References
1. Cheminay C, Hensel M. Rational Design of Salmonella Recombinant Vaccines. Int J Med Microbiol (2008) 298(1-2):87–98. doi: 10.1016/j.ijmm.2007.08.006
2. Holmgren J, Czerkinsky C. Mucosal Immunity and Vaccines. Nat Med (2005) 11(4 Suppl):S45–53. doi: 10.1038/nm1213
3. Weiner HL, da Cunha AP, Quintana F, Wu H. Oral Tolerance. Immunol Rev (2011) 241(1):241–59. doi: 10.1111/j.1600-065X.2011.01017.x
4. Husseiny MI, Rawson J, Kaye A, Nair I, Todorov I, Hensel M, et al. An Oral Vaccine for Type 1 Diabetes Based on Live Attenuated. Salmonella Vaccine (2014) 32(20):2300–7. doi: 10.1016/j.vaccine.2014.02.070
5. Mbongue JC, Rawson J, Garcia PA, Gonzalez N, Cobb J, Kandeel F, et al. Reversal of New Onset Type 1 Diabetes by Oral Salmonella-Based Combination Therapy and Mediated by Regulatory T-Cells in NOD Mice. Front Immunol (2019) 10:320. doi: 10.3389/fimmu.2019.00320
6. Husseiny MI, Du W, Mbongue J, Lenz A, Rawson J, Kandeel F, et al. Factors Affecting Salmonella-based Combination Immunotherapy for Prevention of Type 1 Diabetes in non-Obese Diabetic Mice. Vaccine (2018) 36(52):8008–18. doi: 10.1016/j.vaccine.2018.10.101
7. Xiong G, Husseiny MI, Song L, Erdreich-Epstein A, Shackleford GM, Seeger RC, et al. Novel Cancer Vaccine Based on Genes of Salmonella Pathogenicity Island 2. Int J Cancer (2010) 126(11):2622–34. doi: 10.1002/ijc.24957
8. Xu X, Husseiny MI, Goldwich A, Hensel M. Efficacy of Intracellular Activated Promoters for Generation of Salmonella-based Vaccines. Infect Immun (2010) 78(11):4828–38. doi: 10.1128/IAI.00298-10
9. Husseiny MI, Hensel M. Evaluation of an Intracellular-Activated Promoter for the Generation of Live Salmonella Recombinant Vaccines. Vaccine (2005) 23(20):2580–90. doi: 10.1016/j.vaccine.2004.11.035
10. Husseiny MI, Hensel M. Evaluation of Salmonella Live Vaccines With Chromosomal Expression Cassettes for Translocated Fusion Proteins. Vaccine (2009) 27(28):3780–7. doi: 10.1016/j.vaccine.2009.03.053
11. Husseiny MI, Wartha F, Hensel M. Recombinant Vaccines Based on Translocated Effector Proteins of Salmonella Pathogenicity Island 2. Vaccine (2007) 25(1):185–93. doi: 10.1016/j.vaccine.2005.11.020
12. Abrahams GL, Hensel M. Manipulating Cellular Transport and Immune Responses: Dynamic Interactions Between Intracellular Salmonella enterica and its Host Cells. Cell Microbiol (2006) 8(5):728–37. doi: 10.1111/j.1462-5822.2006.00706.x
13. Hensel M. Salmonella Pathogenicity Island 2. Mol Microbiol (2000) 36(5):1015–23. doi: 10.1046/j.1365-2958.2000.01935.x
14. Coombes JL, Powrie F. Dendritic Cells in Intestinal Immune Regulation. Nat Rev Immunol (2008) 8(6):435–46. doi: 10.1038/nri2335
15. Evans DT, Chen LM, Gillis J, Lin KC, Harty B, Mazzara GP, et al. Mucosal Priming of Simian Immunodeficiency Virus-Specific Cytotoxic T-lymphocyte Responses in Rhesus Macaques by the Salmonella Type III Secretion Antigen Delivery System. J Virol (2003) 77(4):2400–9. doi: 10.1128/jvi.77.4.2400-2409.2003
16. Nishikawa H, Sato E, Briones G, Chen LM, Matsuo M, Nagata Y, et al. In Vivo Antigen Delivery by a Salmonella typhimurium Type III Secretion System for Therapeutic Cancer Vaccines. J Clin Invest (2006) 116(7):1946–54. doi: 10.1172/JCI28045
17. Kong Q, Six DA, Roland KL, Liu Q, Gu L, Reynolds CM, et al. Salmonella Synthesizing 1-Dephosphorylated [Corrected] Lipopolysaccharide Exhibits Low Endotoxic Activity While Retaining its Immunogenicity. J Immunol (2011) 187(1):412–23. doi: 10.4049/jimmunol.1100339
18. Frahm M, Felgner S, Kocijancic D, Rohde M, Hensel M, Curtiss R 3rd, et al. Efficiency of Conditionally Attenuated Salmonella enterica Serovar Typhimurium in Bacterium-Mediated Tumor Therapy. mBio (2015) 6(2):1–11. doi: 10.1128/mBio.00254-15
19. Kong Q, Yang J, Liu Q, Alamuri P, Roland KL, Curtiss R 3. Effect of Deletion of Genes Involved in Lipopolysaccharide Core and O-antigen Synthesis on Virulence and Immunogenicity of Salmonella enterica Serovar Typhimurium. Infect Immun (2011) 79(10):4227–39. doi: 10.1128/IAI.05398-11
20. Khan SA, Everest P, Servos S, Foxwell N, Zahringer U, Brade H, et al. A Lethal Role for Lipid A in Salmonella Infections. Mol Microbiol (1998) 29(2):571–9. doi: 10.1046/j.1365-2958.1998.00952.x
21. Rietschel ET, Kirikae T, Schade FU, Mamat U, Schmidt G, Loppnow H, et al. Bacterial Endotoxin: Molecular Relationships of Structure to Activity and Function. FASEB J (1994) 8(2):217–25. doi: 10.1096/fasebj.8.2.8119492
22. Claes AK, Steck N, Schultz D, Zahringer U, Lipinski S, Rosenstiel P, et al. Salmonella enterica Serovar Typhimurium Delta msbB Triggers Exacerbated Inflammation in Nod2 Deficient Mice. PLoS One (2014) 9(11):e113645. doi: 10.1371/journal.pone.0113645
23. Husseiny MI, Hensel M. Rapid Method for the Construction of Salmonella enterica Serovar Typhimurium Vaccine Carrier Strains. Infect Immun (2005) 73(3):1598–605. doi: 10.1128/IAI.73.3.1598-1605.2005
24. Valdivia RH, Falkow S. Fluorescence-Based Isolation of Bacterial Genes Expressed Within Host Cells. Science (1997) 277(5334):2007–11. doi: 10.1126/science.277.5334.2007
25. Husseiny MI, Hensel M. Construction of Highly Attenuated Salmonella enterica Serovar Typhimurium Live Vectors for Delivering Heterologous Antigens by Chromosomal Integration. Microbiol Res (2008) 163(6):605–15. doi: 10.1016/j.micres.2006.10.003
26. Datsenko KA, Wanner BL. One-Step Inactivation of Chromosomal Genes in Escherichia coli K-12 Using PCR Products. Proc Natl Acad Sci U S A (2000) 97(12):6640–5. doi: 10.1073/pnas.120163297
27. Ellermeier CD, Janakiraman A, Slauch JM. Construction of Targeted Single Copy Lac Fusions Using Lambda Red and FLP-mediated Site-Specific Recombination in Bacteria. Gene (2002) 290(1-2):153–61. doi: 10.1016/s0378-1119(02)00551-6
28. Yamada Y, Maeda M, Alshahni MM, Monod M, Staib P, Yamada T. Flippase (FLP) Recombinase-Mediated Marker Recycling in the Dermatophyte Arthroderma Vanbreuseghemii. Microbiol (Reading) (2014) 160(Pt 10):2122–35. doi: 10.1099/mic.0.076562-0
29. Low KB, Ittensohn M, Le T, Platt J, Sodi S, Amoss M, et al. Lipid A Mutant Salmonella With Suppressed Virulence and TNFalpha Induction Retain Tumor-Targeting In Vivo. Nat Biotechnol (1999) 17(1):37–41. doi: 10.1038/5205
30. Liu T, Chopra AK. An Enteric Pathogen Salmonella Enterica Serovar Typhimurium Suppresses Tumor Growth by Downregulating CD44high and CD4T Regulatory (Treg) Cell Expression in Mice: The Critical Role of Lipopolysaccharide and Braun Lipoprotein in Modulating Tumor Growth. Cancer Gene Ther (2010) 17(2):97–108. doi: 10.1038/cgt.2009.58
31. Kong Q, Six DA, Liu Q, Gu L, Roland KL, Raetz CR, et al. Palmitoylation State Impacts Induction of Innate and Acquired Immunity by the Salmonella enterica Serovar Typhimurium msbB Mutant. Infect Immun (2011) 79(12):5027–38. doi: 10.1128/IAI.05524-11
32. Mbongue JC, Alhoshani A, Rawson J, Garcia PA, Gonzalez N, Ferreri K, et al. Tracking of an Oral Salmonella-Based Vaccine for Type 1 Diabetes in Non-obese Diabetic Mice. Front Immunol (2020) 11:712. doi: 10.3389/fimmu.2020.00712
33. Toso JF, Gill VJ, Hwu P, Marincola FM, Restifo NP, Schwartzentruber DJ, et al. Phase I Study of the Intravenous Administration of Attenuated Salmonella typhimurium to Patients With Metastatic Melanoma. J Clin Oncol (2002) 20(1):142–52. doi: 10.1200/JCO.2002.20.1.142
34. Erova TE, Kirtley ML, Fitts EC, Ponnusamy D, Baze WB, Andersson JA, et al. Protective Immunity Elicited by Oral Immunization of Mice With Salmonella enterica Serovar Typhimurium Braun Lipoprotein (Lpp) and Acetyltransferase (MsbB) Mutants. Front Cell Infect Microbiol (2016) 6:148. doi: 10.3389/fcimb.2016.00148
Keywords: type 1 diabetes, autoantigens, immunotherapy, immunomodulator, Salmonella msbB mutant, oral vaccination, diabetes reversal and prevention
Citation: Cobb J, Rawson J, Gonzalez N, Hensel M, Kandeel F and Husseiny MI (2021) Oral Salmonella msbB Mutant as a Carrier for a Salmonella-Based Vaccine for Prevention and Reversal of Type 1 Diabetes. Front. Immunol. 12:667897. doi: 10.3389/fimmu.2021.667897
Received: 15 February 2021; Accepted: 06 May 2021;
Published: 24 May 2021.
Edited by:
Xuguang (Sean) Li, Health Canada, CanadaReviewed by:
Camille Locht, Institut National de la Santé et de la Recherche Médicale (INSERM), FranceSathya Narayanan Thulasi Raman, Health Canada, Canada
Copyright © 2021 Cobb, Rawson, Gonzalez, Hensel, Kandeel and Husseiny. This is an open-access article distributed under the terms of the Creative Commons Attribution License (CC BY). The use, distribution or reproduction in other forums is permitted, provided the original author(s) and the copyright owner(s) are credited and that the original publication in this journal is cited, in accordance with accepted academic practice. No use, distribution or reproduction is permitted which does not comply with these terms.
*Correspondence: Mohamed I. Husseiny, bWVsc2F5ZWRAY29oLm9yZw==