- 1Division of Hematology, Department of Internal Medicine, Medical School, University of Patras, Patras, Greece
- 2Division of Gastroenterology, Department of Internal Medicine, Medical School, University of Patras, Patras, Greece
Through food intake, humans obtain a variety of nutrients that are essential for growth, cellular function, tissue development, energy, and immune defense. A special interaction between nutrients and gut-associated lymphoid tissue occurs in the intestinal tract. Enterocytes of the intestinal barrier act as sensors for antigens from nutrients and the intestinal microbiota, which they deliver to the underlying immune system of the lamina propria, triggering an immune response. Studies investigating the mechanism of influence of nutrition on immunological outcomes have highlighted an important role of macronutrients (proteins, carbohydrates, fatty acids) and micronutrients (vitamins, minerals, phytochemicals, antioxidants, probiotics) in modulating immune homeostasis. Nutrients exert their role in innate immunity and inflammation by regulating the expression of TLRs, pro- and anti-inflammatory cytokines, thus interfering with immune cell crosstalk and signaling. Chemical substrates derived from nutrient metabolism may act as cofactors or blockers of enzymatic activity, influencing molecular pathways and chemical reactions associated with microbial killing, inflammation, and oxidative stress. Immune cell function appears to be influenced by certain nutrients that form parts of the cell membrane structure and are involved in energy production and prevention of cytotoxicity. Nutrients also contribute to the initiation and regulation of adaptive immune responses by modulating B and T lymphocyte differentiation, proliferation and activation, and antibody production. The purpose of this review is to present the available data from the field of nutritional immunology to elucidate the complex and dynamic relationship between nutrients and the immune system, the delineation of which will lead to optimized nutritional regimens for disease prevention and patient care.
Introduction
Nutrition is critical to maintaining the health and vitality of all living organisms. Nutrients ingested in the diet are essential for growth, cellular function and tissue development, energy supply, and immune defense (1). The diet of omnivores, including humans, consists of animal and plant products; these are divided into macronutrients (proteins, carbohydrates, fatty acids), micronutrients (vitamins, minerals, phytochemicals, antioxidants, probiotics), and dietary fiber, all of which have important biological functions (2).
The main goal of nutritional immunology is to study in detail the effects of nutrients on the immune system (3). An unhealthy diet or malnutrition characterized by macro- and micronutrient deficiencies can lead to ineffective immune responses and leave the organism unprotected from pathogens. In addition, many diseases are associated with a loss of essential nutrients, leading to nutrient deficiencies (4). Nutrients per se can mediate pro- and anti-inflammatory responses and modulate chronic inflammatory and autoimmune diseases (2, 3).
A field called nutrigenetics studies nutrition as a target for preventing and reversing disease progression. Nutrigenetics aims to develop personalized dietary patterns, taking into account that genetic predisposition characterizes some types of chronic diseases and that gene expression is directly influenced by environmental factors, including food metabolites (5).
The Interaction of Nutrients and Gut-Associated Lymphoid Tissue
A schematic representation of the interplay between nutrients, gut microbiota, and immune system is shown in Figure 1. The gastrointestinal tract represents a major component of the immune system; it contains its own lymphoid tissue called gut-associated lymphoid tissue (GALT), which protects the gastrointestinal tract from invading pathogens. GALT is found in an extensive area of the intestine, organized in lymphoid follicles in the lamina propria known as Peyer’s patches (PPs), and scattered within the intestinal epithelium and in the lamina propria below the intestinal epithelium as B cells, T cells, dendritic cells (DCs), and macrophages. GALT forms the center of mucosal immunity in the intestine, where absorption of nutrients occurs and antigens from nutrients and the intestinal microbiota (bacteria, archaea, viruses, fungi, and protozoa) can elicit an immune response. The intestinal epithelial barrier, composed mainly of enterocytes (Paneth cells, goblet cells, microfold (M) cells), is a crucial tissue structure that prevents pathogen invasion (6).
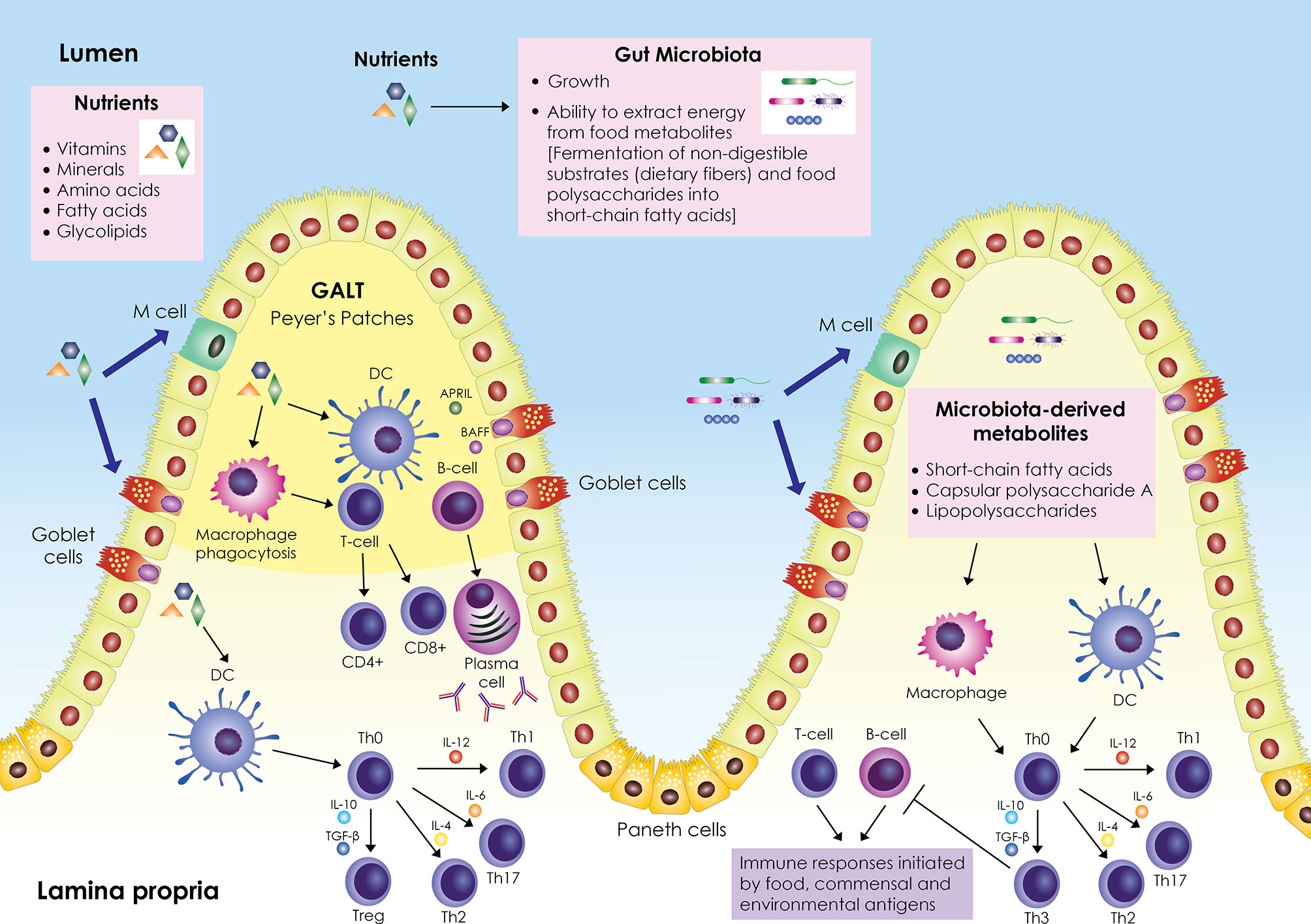
Figure 1 Schematic representation of the interplay between nutrients, gut microbiota and immune system. The gut-associated lymphoid tissue (GALT) occupies a large area of the gut; it is scattered within the intestinal epithelium and is also organized into lymphoid follicles in the lamina propria called Peyer’s patches. GALT consists mainly of B and T cells, macrophages, and dendritic cells (DCs). Enterocytes (Paneth cells, goblet cells, microfold (M) cells) are responsible for the active transport or passive diffusion of antigens from food during digestion and microbial components. M cells, located in Peyer’s patches, take up luminal antigens by transcytosis and present them to underlying DCs in the lamina propria, which in turn interact with B and T cells either in Peyer’s patches or in mesenteric lymph nodes. DCs secrete cytokines and induce differentiation of T helper cell precursors (Th0) into effector Th cells (Th1, Th2, Th17) or Tregs. Antigen impingement on intestinal epithelial cells and subsequent activation of DCs induces differentiation of B cells into IgA-secreting plasma cells. In parallel, nutrients modulate the gut microbiota by promoting or inhibiting its growth and affecting its ability to derive energy from dietary chemicals. Microbiota-derived metabolites (short-chain fatty acids, capsular polysaccharide A, lipopolysaccharides) stimulate and modulate DCs and macrophages to create an antigen presentation environment that favors the differentiation of Th0 cells into Th3 Tregs that inhibit T- and B-cell inflammatory responses triggered by food, commensal, and environmental antigens. Adapted from refs (6–16).
Enterocytes are responsible for the active transport or passive diffusion of antigens from food during digestion and microbial components. Goblet cells secrete mucus that forms the epithelial barrier against pathogen invasion and have been found to present antigens to CD103+ DCs in the lamina propria (7). Intestinal DCs represent a crucial regulatory cell population of intestinal immunity; depending on environmental stimuli, they secrete cytokines and cell mediators, mainly IL-10, TGF-β, IL-6, IL-4, IL-12, B-cell activating factor (BAFF) and a proliferation-inducing ligand (APRIL), and induce the differentiation of T and B cells. IL-10, TGF-β, IL-6, IL-4, IL-12, induce the differentiation of T helper (Th) cell precursors (Th0) into effector Th cells (mainly Th1, Th2, Th17) or regulatory Th cells (Tregs, mainly Th3) (8–10), while BAFF and APRIL induce the differentiation of B cells into IgA-producing plasma cells in a T cell-independent manner (11). Enteroendocrine cells scattered in the intestinal tract play an important role in controlling nutrient uptake by recognizing nutrient-derived antigens in the lumen via microvilli structures and G protein-coupled receptors (17). M cells, located in the PPs, take up lumen antigens by transcytosis and present them to the underlying DCs in the lamina propria, which in turn can interact with B and T cells either in the PPs or the mesenteric lymph nodes (8, 12).
Nutrients exert an important role in gut immunity through their effect on microorganisms residing in the gastrointestinal tract. The gut microbiota ferments non-digestible substrates such as dietary fiber or metabolizes dietary polysaccharides into short-chain fatty acids for energy (18). In parallel, nutrients modulate the gut microbiota by promoting or inhibiting its growth and influencing its ability to obtain energy from dietary chemicals (13).
The gut microbiota shapes the development of specialized immune responses through molecular signaling that supports immune cell proliferation and communication (14, 15). Specifically, microbiota signaling regulates the expression of cytokines and antiviral mediators and mediates DNA modifications in immune cells, mainly macrophages, effector Th cells, and Tregs. Microbiota-derived metabolites and their components (short-chain fatty acids, capsular polysaccharide A, lipopolysaccharides) can stimulate and modulate antigen-presenting cells (APCs), mainly DCs and macrophages, to create an antigen presentation environment (composed mainly of cytokines IL-10, TGF-β) that favors the differentiation of Th0 cells into Th3 Tregs, which inhibit the inflammatory responses of T and B cells initiated by food, commensal and environmental antigens (14–16).
Diets enriched in saturated fatty acids, proteins, and carbohydrates have been associated with increased colonization of certain microbes at the expense of other species (19); it appears that changes in the composition of the microbiota due to a high-fat diet are associated with cardiometabolic disease (19). Studies on the effects of different dietary patterns on gut microbiota composition, short-chain fatty acid content, and inflammatory markers in healthy subjects compared to subjects with chronic diseases or metabolic syndrome showed that certain dietary components, mainly fiber and different types of fat, alter gut microbiota composition and inflammatory marker content such as c-reactive protein and IL-6 in the latter (20); therefore, modulation of microbiota composition by specific diet could lead to personalized therapeutic interventions in the future. Passive transfer of specific microorganisms, such as lactic acid-producing bacteria, Candida and Penicillium fungi, and plant viruses, into the gastrointestinal tract through diet has been previously reported (21).
Methodology
A comprehensive literature search was conducted by electronically searching the PubMed database in all domains and using the following search strategies, including the keywords: (1) (micronutrients) OR (macronutrients) OR (nutrients) AND (immune system) OR (immune function) OR (immunity) OR (immune responses), (2) (any micronutrient/macronutrient) AND (immune system) OR (immune function), including all article types, and (3) (any micronutrient/macronutrient) AND (immune system) OR (immune function), including clinical trial and randomized controlled trial (species: Human) to examine articles written in English and published between January 1990 and March 2021 (all article types) and between January 2016 and March 2021 (clinical trials and randomized controlled trials). Critical appraisal of the collected articles depended on the original studies, including animal and human data.
The Role of Micronutrients in Immune System Function in Vitro and in Vivo
A variety of studies, including in vitro culture systems of immune cells and animal models, have been performed to evaluate the immunological effects of specific micronutrients in host defense and immune homeostasis. The precise mechanisms of micronutrient involvement in the coordination of complex immune responses are still under investigation, which will provide pertinent information on their clinical role in pathological conditions. The effects of micronutrients on the immune system are summarized in Table 1.
Vitamin A
Vitamin A is a dietary micronutrient that is present in the form of carotenoids and retinyl esters and is associated with the maintenance of robust immune functions. Vitamin A binds to retinoic acid receptors (RARs), which form heterodimers and act as transcription factors to regulate the expression of several genes involved in cell growth and differentiation, lipid homeostasis, and insulin responses (139). Carotenoids are universal stimulators of immunity; metabolic cleavage of β-carotene generates retinaldehyde molecules that activate the RXR receptor (140), with resultant regulation of many immune-related genes across species. Experiments with invertebrates showed that carotenoids regulate the expression of immune-related genes such as TLRs, heat shock proteins HSP70 and HSP90, thioredoxin-like protein (TRX), and peptidoglycan recognition receptor proteins (PGRPs) (22), possibly having a positive impact on the innate immune response against pathogens. Experiments with rabbits showed that carotenoids can enhance the humoral immune response by increasing serum IgM, IgG and IgA levels (23). A metabolite of vitamin A (all-trans-retinoic acid, ATRA) binds to the RAR in the nucleus of neutrophils isolated from human blood and induces the mTOR pathway, increasing the cytotoxic activity and extracellular trap release of neutrophils (24). Retinoid supplementation has also been shown to control an inflammatory response in vitro by downregulating TLR expression and secretion of the proinflammatory cytokines TNF-α and IL-6 by macrophages when they phagocytose pathogens (25). The effect of vitamin A on adaptive immunity depends on the expression of RARs by T and B cells, with signaling induced by ATRA binding to RAR affecting cell development, proliferation and differentiation, and Ig class switching (26). Retinoid supplementation in normal B cell cultures resulted in modulation of B lymphocyte activation, differentiation and cytokine production (27). In parallel, retinoic acid was shown to induce IgA secretion (28), suppress IgE secretion, and accelerate allograft rejection by enhancing T cell migration into the graft in vivo, a finding that could be considered in studies of factors determining successful transplantation (29, 30). A role of vitamin A in immunomodulation, especially in autoimmune and inflammatory diseases, could be proposed, since vitamin A has been shown to have a tolerogenic effect on immune cells in vitro; in particular, it promotes the development of Th2 cells (31), inhibits the IL-6-driven generation of proinflammatory Th17 cells, and induces the differentiation of Th0 cells into Tregs (32). Moreover, vitamin A induces the differentiation of human immature DCs into tolerogenic DCs, which in turn induce the differentiation of naive B cells into B cells expressing vitamin A receptors and having immunosuppressive/regulatory activity (33).
Vitamin B2 (Riboflavin)
Riboflavin is a micronutrient with antioxidant and anti-inflammatory activity. Vitamin B2 is converted by riboflavin kinase into two active forms, flavin adenine dinucleotide and flavin mononucleotide, which are cofactors involved in redox reactions in the tricarboxylic acid cycle and fatty acid oxidation (34). These metabolic pathways are involved in T cell activation, differentiation and proliferation (35). An anti-inflammatory role of vitamin B2 through down-regulation of the NF-κB pathway and production of proinflammatory cytokines has also been suggested, as vitamin B2 has an inhibitory effect on proteasome activity, which blocks the activity of NF-κB components in the cytoplasm (36). Culture with vitamin B2 altered the responses of murine macrophages to lipopolysaccharide (LPS) stimulation: expression of TLR4 and secretion of the proinflammatory cytokine TNF-α were reduced, while expression of the anti-inflammatory cytokine IL-10 and production of the antibacterial/antiviral agent nitric oxide (NO) were increased (37). B2 supplementation in mice increased the phagocytic activity of Staphylococcus aureus-infected macrophages and decreased the levels of the cytokines IFN-γ, IL-6, and IL-1β, possibly by regulating the NF-κB pathway (36). A possible role of B2 supplementation in attenuating insulin resistance and the development of the metabolic syndrome was highlighted in an in vitro adipocyte-macrophage co-culture system that mimics obesity-related inflammation. Specifically, riboflavin supplementation resulted in a reduction in proinflammatory factors (TNF-α, IL-6, MCP-1, HMGB1) and an increase in anti-inflammatory factors adiponectin and IL-10 (38), highlighting the need for further evaluation of this micronutrient as a promising target in the treatment of inflammatory conditions associated with obesity and metabolic syndrome.
Vitamin C
Vitamin C is a water-soluble micronutrient of high molecular importance as it is a cofactor of hydroxylases, enzymes involved in the regulation of gene transcription, and cell signaling pathways (39). An anti-inflammatory effect of vitamin C has been reported through its antioxidant activity in the cytoplasm, where it quenches ROS and is oxidized to dehydroascorbic acid (DHA) with the release of electrons. DHA directly inhibits the kinase IKKβ, blocking NF-κB activation and the expression of proinflammatory cytokines (40). Moreover, culture of LPS-stimulated peripheral blood mononuclear cells with vitamin C resulted in decreased secretion of TNF-α and IFN-γ and increased secretion of IL-10 by the cells (41). Enhancement of innate immunity appears to be achieved by vitamin C, as it has been shown to promote microbial killing by increasing the migration of neutrophils to sites of infection in response to chemotactic signals and the release of ROS (42). Addition of vitamin C to NK cell cultures resulted in increased NK cell proliferation (43), indicating a potent role of this micronutrient in studies testing NK cell-based immunotherapy against malignancies. High-dose administration of vitamin C in murine cancer models has also highlighted the beneficial effect of this vitamin on the inhibition of tumor growth in a T cell-dependent manner, as it appears to modulate tissue infiltration by adaptive immune cells and increase the cytotoxic activity of adoptively transferred CD8 T cells in mice (44). In addition, vitamin C has been shown to help maintain the integrity of tissue epithelial barriers and accelerate wound healing by promoting collagen synthesis, proliferation, and migration of fibroblasts (45, 46). Vitamin C has also been shown to improve adaptive immunity by promoting T and B cell differentiation and proliferation (47, 48) and increasing antibody production (49).
Vitamin D
Vitamin D is an important micronutrient for bone and mineral metabolism by promoting intestinal absorption of calcium and phosphate and stimulating osteoclast differentiation and calcium absorption from bone, thus maintaining calcium balance in the skeleton (50). Vitamin D exerts its role by binding to its receptor (VDR), which is expressed in many cell types including B and T cells (51). In activated and proliferating B and T cells, the expression of VDR is increased (52, 53), indicating an important involvement of vitamin D in adaptive immune responses. The vitamin D-VDR complex forms heterodimers with the retinoid X receptor (RXR), a nuclear receptor that forms heterodimers with other steroid hormone receptors that act as transcription factors and regulate gene expression (54). The vitamin D-VDR-RXR complexes enter the nucleus, bind to the vitamin D response element and regulate the expression of vitamin D-responsive genes (55). An immunomodulatory effect of vitamin D on Th cell responses has been highlighted in vitro; vitamin D has been shown to exert a potent anti-inflammatory effect by down-regulating Th1-mediated immune responses and inhibiting the expression of the proinflammatory cytokines IL-2, IFN-γ, IL-6, TNF-α and IL-17 in Th cells (56, 57), and by enhancing Th2 activity by promoting the production of the cytokines IL-4, IL-5 and IL-10 (58). A decrease in Th17 cell number and an increase in Treg cell number have also been noted (59, 60). In terms of innate immunity, vitamin D has been shown to inhibit the expression of IL-6 and TLRs 2, 4 and 9 in monocytes, resulting in hyporesponsiveness to pathogen-associated molecular patterns, a fact that could have a negative impact on effectiveness in encountering pathogens, or a positive impact on acute pathological conditions, including sepsis and autoimmunity, in which TLR activity is increased (61). Vitamin D has also been shown to increase chemotaxis and phagocytosis as well as the expression of antimicrobial peptides in cultured monocytes and myeloid cells (56, 62, 63). An important activity of vitamin D in immune tolerance has also been highlighted, as it is one of the biological factors (others are IL-10, TGF-β, apoptotic cells) that induce differentiation of human immature DCs into tolerogenic DCs in vitro, which in turn induce differentiation of naive T cells into Tregs (64).
Vitamin E and Vitamin B9
Vitamin E is a fat-soluble compound found in the cell membrane where it scavenges peroxyl radicals, preventing oxidation of polyunsaturated fatty acids and oxidative damage to the cell. A balanced redox state in the cell can influence signal transduction, as the activity of signaling enzymes is regulated by free radicals (65). Thus, vitamin E exerts an important role in modulating membrane integrity, signal transduction, and oxidative stress in immune cells, as their cell membranes are enriched in vitamin E isoforms (α- and γ-tocopherol) (65). Experiments using cell-free and cell-based systems have shown significant anti-inflammatory activity of endogenous metabolites of vitamin E targeting target 5-lipoxygenase, a key enzyme in the biosynthesis of inflammatory lipid mediators, including chemoattractant and vasoactive leukotrienes (66). Vitamin E has been associated with the regulation of cellular immunity and protection against infection (67), it increases the interaction of APCs with T cells with subsequent T cell activation, and it can alter the function of DCs by influencing their migration and decreasing IL-12 production; it also increases the activity of NK cells (67) and acts as a modulator of Th0 cell differentiation into Th1 or Th2 cells (68, 69).
Folic acid (vitamin B9) is a micronutrient that affects DNA synthesis and cell cycle, DNA methylation, and regulation of gene expression. Substrates from the metabolism of B9 can interfere with enzymatic reactions in these processes and act as co-factors (70). Vitamin B9 also contributes to the maintenance of Tregs in the colon; mice fed a folic acid-deficient diet were more prone to intestinal inflammation (71).
Selenium, Copper, Zinc, Iron and Folic Acid Trace Elements
Trace elements are micronutrients that are present in small amounts in the living organism, performing important biological functions as they participate in chemical and molecular processes in the form of cofactors of many enzymes and antioxidant molecules.
Selenium in the form of the amino acid selenocysteine forms part of the catalytic active site of peroxidases that catalyze oxygen-reactive species and may regulate metabolic reactions that induce the synthesis of lipoxygenases, enzymes involved in the synthesis of inflammatory mediators (72, 73). Selenium upregulates cellular immunity by enhancing TCR-induced activation of T cells and Th0 differentiation into Th1 cells in mice (74) and is involved in redox signaling, an important mechanism for the killing of microorganisms by phagocytes (72, 75).
Copper plays a major role in chemical reactions important for energy production and prevention of oxidative stress in the cell, since enzymes (cytochrome c oxidase, copper-zinc superoxide dismutase) involved in electron transfer during the mitochondrial transport chain and in catalytic free radical reactions are copper-containing (76). An interesting role of the copper trace element in the regulation of gene transcription has also been highlighted in yeast species, in which the level of gene expression changed in response to copper availability (77).
Zinc is involved in TLR4 signaling by inhibiting the activation of the transcription factor NF-κB (78) and decreasing the production of the proinflammatory cytokines IL-1β, TNF-α, and IL-6 (79). In vitro, zinc mediates the differentiation of CD34+ progenitor cells into NK cells (32), and in vivo, zinc supplementation results in increased numbers of IFN-γ-producing NK cells (80).
Iron can affect cell cycle and proliferation by acting as a co-factor in a variety of enzymes involved in DNA synthesis and repair (81). Iron is necessary for the regulation of immune cell proliferation and innate immune response. Transferrin receptors responsible for iron uptake are expressed in immune cells (monocytes, macrophages, T cells) (82). Iron is also an important substrate for pathogen growth, and a variety of genes and proteins involved in iron homeostasis have been linked to immunomodulatory effects, including TLR signaling, regulation of T-cell differentiation and activity, and resistance to infection (82).
Effects of Micronutrient Supplementation on Immunity and Disease: Clinical Data
The supply of necessary micronutrients is crucial for the normal development of the immune system during the different stages of human growth (gestation, neonatal maturation, weaning). Inadequate maternal nutrition and micronutrient deficiencies, especially vitamins A, D, E, B2, B6, B12, and folic acid, are associated with an increased risk of infant morbidity due to respiratory infections, intestinal inflammation, allergies, asthma, and neurodevelopmental disorders (83–87). Prenatal IgE antibody formation is influenced by food metabolites and predicts the development of food allergies in infancy (88). Diet continues to influence immune system function during aging. Diet and nutritional status can influence immunosenescence, a gradual deterioration of the immune system with age that results from continuous antigenic challenges, infections, and chronic inflammation (89).
In a study of 36 healthy Bangladeshi men with low vitamin A stores vitamin A supplementation resulted in increased numbers of NK cells, production of reactive oxygen species (ROS) by monocytes, decreased serum cytokines IL-6 and IL-17, limited inflammatory responses to pathogens mediated by Th1 and Th17 cells, and increased levels of IFN-γ-inducible protein 10 (IP-10, CXCL10); the latter recruits activated T cells to sites of tissue inflammation (90, 91). A clinical study of 306 Bangladeshi infants examined the effects of vitamin A administration on Treg development, mucosal targeting of immune cells in response to chemokine receptor 9 (CCR9) expression, and systemic endotoxin burden as indicated by altered plasma concentrations of soluble CD14 (sCD14), a marker of systemic bacterial endotoxin. In low birth weight infants receiving vitamin A supplementation, the expression of CCR9 on Tregs was increased, indicating an increase in their numbers in the intestinal mucosa with a consequent decrease in intestinal inflammation. The decrease in sCD14 concentration after vitamin A supplementation at 2 years of age showed an important role of vitamin A in improving intestinal integrity, also considering the better vitamin A status at the same age (92). Considering the possible positive role of vitamin A in intestinal immunity and homeostasis, it could be proposed as a therapeutic factor in patients with inflammatory bowel disease.
A beneficial role of B2 was demonstrated in a prospective clinical intervention study that included 70 patients with Crohn’s disease. B2 supplementation (oral dose 100 mg daily for 3 weeks) showed anti-inflammatory effects, decreased systemic oxidative stress, and symptom improvement, represented by decreased serum levels of inflammatory markers, increased plasma free thiols, and decreased clinical disease activity (Harvey-Bradshaw Index), respectively (93).
Together with B2, vitamin B12 has shown promising results in controlling inflammation-associated disease symptoms. Patients with Alzheimer’s disease who received antipsychotic drugs plus vitamin B12 had decreased expression of the proinflammatory cytokines IL-8 and TNF-α and increased expression of the anti-inflammatory cytokine TGF-β and exhibited milder psychotic symptoms (94). A prospective clinical trial involving 30 healthy Brazilian adults examined the effect of a daily dose (5 mg) of folic acid on immunity and showed a folate-associated significant reduction in the number and cytotoxicity of NK cells, as well as higher levels of the cytokines IL-8 and TNF-α (95). The association of folic acid with reduced NK responses has also been suggested as a potent factor in the virus-mediated pathology of autoimmune type 1 diabetes, whereby defects in the folate pathway may lead to NK dysfunction and thus enhance the negative influence of viral infections in the initiation of autoimmune responses (96).
Vitamin C also plays an important role in the prevention of infections and inflammation (97, 98). Intravenous infusion of vitamin C in patients with allergic diseases resulted in decreased serum histamine levels (99). Vitamin C supplementation of 0.2 g/day or more was associated with decreased severity and duration of the common cold (100).
A cohort observational study that included hospitalized patients with COVID-19 aged >50 years showed a beneficial effect of combining vitamin D, magnesium and vitamin B12 (DMB) on clinical outcomes of the disease. DMB supplementation resulted in a reduction in the number of patients requiring oxygen and/or intensive care support, suggesting an ameliorative role of these micronutrients on disease severity (101). Other clinical studies have also shown a protective role of vitamin D supplementation against acute respiratory infections and symptom relief in vitamin D-deficient children and immunodeficient patients (102, 103), also considering that it affects the balance of type 1/type 2 cytokines; specifically, it causes a decrease in the production of type 1 cytokines TNF-α, IFN-γ, IL-6, IL-8, IL-9, IL-12, IL-17, and an increase in the production of type 2 cytokines IL-4, IL-5, IL-10 (104, 105). A positive effect of vitamin D on the augmentation of salivary immune responses was also found during stressful military training in healthy subjects, who showed higher salivary secretory immunoglobulin A and cathelicidin secretion rates (indices of mucosal immunity) after vitamin D supplementation (106). A possible therapeutic role of vitamin D in autoimmune diseases was highlighted in a study of patients with Hashimoto’s thyroiditis, in which vitamin D supplementation resulted in a significant reduction in anti-thyroglobulin antibodies (107), and in patients with type 1 diabetes, where vitamin D supplementation was shown to lower the levels of Th17 inflammatory cells (108). An anti-inflammatory effect of vitamin D was also found in patients with ulcerative colitis, who showed a significant decrease in serum proinflammatory cytokine levels (TNF-α, IFN-γ, and IL12p70) (109). Vitamin D supplementation has also been suggested as a potent adjuvant therapy to antiretroviral drugs in HIV infection after a decrease in markers of T cell activation/exhaustion and monocyte activation was shown in HIV-infected adolescents receiving a maximum dose of 120,000 IU/month (110).
Vitamin E is another micronutrient with potent immunomodulatory activity. Supplementation of vitamin E and a tocotrienol-rich fraction in healthy subjects has been associated with regulation of gene expression and molecular signaling pathways related to immune response, response to stress, stimuli, hypoxia, bacteria, and complement. A possible antioxidant and anti-inflammatory activity of vitamin E through the downregulation of signaling pathways related to apoptosis, NF-κB kinase, the cascade of extracellular signal-regulated kinase-1 and extracellular signal-regulated kinase-2 has been indicated (111). A beneficial effect of co-administration of nutrients and therapeutic medications in diseases was suggested in a randomized controlled trial investigating the effect of vitamin E on liver histology in patients with nonalcoholic steatohepatitis (NASH) and type 2 diabetes, where the combined treatment of vitamin E and pioglitazone improved steatosis and inflammation in patients leading to resolution of NASH (112).
Supplementation of B9 in patients with mild cognitive impairment improved cognitive function, which was associated with a reduction in peripheral inflammatory cytokines (113).
Regarding the effects of trace element supplementation on immune function and disease, zinc supplementation in patients with HIV showed potency in lowering markers of systemic inflammation, monocyte activation, and microbial translocation (114). Serum zinc and selenium levels have also been reported as predictive markers of survival in COVID-19 patients, with reference ranges of these trace elements associated with high-survival probability, highlighting the need for further evaluation of their supplementation effects in the disease (115). Prenatal and postnatal zinc supplementation has been shown to improve T cell-dependent antibody responses to hepatitis B vaccination in infants (116). An anticancer role of zinc supplementation was demonstrated in type 2 diabetic patients with metabolic syndrome who received 30 mg elemental zinc/day or placebo for eight weeks and exhibited increased proportion of monocytes expressing transmembrane TNF-α (117). Co-administration of zinc and magnesium in patients with polycystic ovary syndrome significantly decreased serum high-sensitivity C-reactive protein, a marker of inflammation, and downregulated proinflammatory IL-1 and TNF-α cytokines (118). Moreover, selenium supplementation in healthy individuals improves their response to vaccination and increases their antibody titers (119, 120), indicating a potent role of micronutrients as cofactors in vaccine-mediated immunity. An anti-inflammatory effect of selenium was also demonstrated in infertile women with polycystic ovary syndrome who were candidates for in vitro fertilization, who showed a decrease in gene expression of the proinflammatory cytokines IL-1 and TNF-α after selenium supplementation (121). A positive influence of selenium administration on immunity in cancer was observed, as patients with lymphomas and solid tumors who received selenium showed a significant increase in neutrophils and IgG and IgA antibody titers (122).
Micronutrient Deficiencies Can Impair Immune Function
Deficiencies in micronutrients such as vitamins A, B12, C, folic acid, riboflavin, iron, zinc, and selenium can increase immunosenescence, particularly susceptibility to infection and progression of inflammation (123).
Vitamin A deficiency has been shown to decrease the number of NK cells and impair their activity, impair the ability of neutrophils and macrophages to undergo phagocytosis, impair the growth and differentiation of B cells, decrease the number of T cells and limit their distribution (124).
Vitamin B2 deficiency has been associated with obesity-associated chronic inflammation. Experiments with mouse adipocytes showed that B2 deficiency resulted in stimulation of the proinflammatory NF-κB signaling pathway and increased levels of IL-1, TNF-α and ROS (125).
Vitamin B12 plays an immunomodulatory role in cellular immunity (126, 127) and its deficiency has been associated with upregulation of proinflammatory TNF-α in macrophages from B12-deficient mice and a decrease in IL-6 levels in B12-deficient rats, conditions that reversed in both animal models after increasing B12 supplementation (128–130). Vitamin B12-deficient anemic patients exhibit lower numbers of all lymphocytes, Tc cells, a change in Th/Tc cell ratio, and suppressed NK cell activity, all of which are reversed after B12 supplementation (126).
Deficiencies of certain trace elements have also been shown to have negatively affect the number and function of immune cells, leading to dysregulation of immune homeostasis.
Zinc deficiency leads to thymic atrophy, decreased number and activity of lymphocytes, altered cytokine production, increased oxidative stress, and inflammation (131) and is also associated with autoimmune and inflammatory diseases by affecting Th cell polarization by promoting differentiation of Th0 cells into proinflammatory Th17 cells, accompanied by loss of Treg function (132).
In mice, it has been shown that selenium deficiency leads to a decrease in B cell count (133). However, excessive selenium intake can be counterproductive, as infectious microorganisms can utilize this nutrient in their biochemical processes (134).
Copper deficiency is associated with a reduction in the number and antimicrobial function of neutrophils and also with a reduction in the IL-2 secretion of T cells with consequent reduction in T cell proliferation (135).
Abnormalities in Th and Tc cell numbers and function have been reported in patients with hereditary hemochromatosis, which is characterized by low iron stores (136). Iron deficiency is associated with weakened cellular immunity, decreased numbers of neutrophils and their phagocytic activity, lower levels of IL-6 and IgG antibodies (137, 138).
Effects of Macronutrients on Immunity and Disease
The effects of macronutrients on the immune system are summarized in Table 2. Macronutrients, including proteins, carbohydrates, and fatty acids, provide tissues with the energy necessary for their development and function (180). Immunomodulation by macronutrients has been studied in experimental animals and in human intervention studies by testing the effect of their intake on immunological outcomes.
Proteins
Proteins represent important macronutrients for the immune system, considering that amino acids are essential for the synthesis of immune proteins, including cytokines and antibodies that mediate immune responses (141). Metabolic degradation of certain amino acids (tryptophan, arginine) leads to the production of chemical substrates involved in biological processes (142). The metabolism of tryptophan contributes to the synthesis of the cofactor NAD+, which is involved in redox reactions and electron transfer. The degradation of arginine has been associated with the induction of the non-canonical NF-κB pathway and the regulation of gene transcription in the context of immune tolerance (142). Metabolism of arginine and methionine also leads to the synthesis of polyamines, which are organic compounds that regulate cell proliferation through their role in maintaining DNA, mRNA, and cell membrane stability; polyamines have been linked to the induction and regulation of inflammation and pathogen recognition by affecting receptor-ligand binding. Degradation of methionine leads to the synthesis of glutathione, an antioxidant organic compound involved in the prevention of oxidative stress, regulation of NK and T cell cytotoxicity and macrophage activity, and activation of T cells (143).
Amino acids exert multiple roles in the immune system, including regulation of the activation of adaptive and innate immune cells (B and T cells, NK cells, macrophages), proliferation of lymphocytes, and production of antibodies, cytokines, and cytotoxic factors (141, 144). Hydrolysates or bioactive peptides released by gastrointestinal digestion have been reported to have antioxidant activities, increase killing activity of NK cells, increase phagocytic activity of macrophages, and increase the size of lymphocyte populations, antibody and cytokine production (145).
Studies in pigs and the fruit fly Drosophila melanogaster, showed that high dietary protein intake has a positive effect on intestinal cellular and humoral immunity, associated with increased expression of antimicrobial peptides (146). On the other hand, low protein diet has been reported to induce immunity against tumor cells in mice. Specifically, a decrease in protein content and the associated decrease in amino acids cause ER stress, which leads to the activation of T cells that secrete proinflammatory cytokines (147).
A positive effect of a high-protein diet on the modulation of inflammatory immune responses was demonstrated in an intervention study of type 2 diabetics who were fed a diet high in animal or plant protein for 6 weeks. After this period, they had reduced levels of the proinflammatory adipokines chemerin and progranulin regardless of protein source (148). Overweight and obese adults who followed an energy-restricted diet with either normal or high protein achieved weight loss accompanied by significant decreases in proinflammatory monocyte subpopulations, plasma lipids, and lipoproteins (149).
Carbohydrates
An important aspect of the effect of macronutrients on the immune system is their involvement in immune recognition. Carbohydrates represent common cell surface molecules that can be recognized as antigens by TLRs. Glycolipids, zwitterionic polysaccharides and glycopeptides can be presented to γδ and αβ T-cells either through endosomal pathways or extracellular receptors (150). Glycoproteins and glycolipids can bind to glycan-binding proteins such as lectins and antibodies, regulating cell adhesion during leukocyte migration and immunity to infection through recognition of carbohydrates contained in the membrane of pathogens (151). In addition, carbohydrates participate in enzymatic processes of protein glycosylation that generate important functional biopolymers. Glycosylated peptides act as glycoantigens that influence the binding of antigen presentation proteins of the HLA-I and HLA-II systems and subsequent T-cell recognition of antigenic peptides. Glycoantigens processed through intracellular pathways in APCs are presented to Th, Tc and NKT cells and modulate their activation and cytokine production (152).
An anti-inflammatory role of carbohydrate intake was highlighted in a study that reported that consumption of a carbohydrate-containing beverage resulted in a decrease in the expression of TLR4 receptors in monocytes of obese/overweight children compared to normal weight children (153). Another study reported that carbohydrate intake by athletes was associated with a more balanced number of immune cells in the blood, decreased phagocytic activity of monocytes and granulocytes, and decreased ROS and inflammatory cytokine levels (154).
A low-carbohydrate, high-fat diet with or without after-meal walks in patients with type 2 diabetes improved glucose control and fasting pro-insulin levels and significantly decreased phosphorylated c-Jun N-terminal kinase in peripheral blood mononuclear cells, which is a marker of cellular inflammation (155).
A hydrolyzed polysaccharide, a rice bran arabinoxylan compound, administered as a dietary supplement in HIV patients resulted in a significant decrease in peripheral blood Tc cells and a significant increase in the Th/Tc cell ratio (156).
An anti-inflammatory effect of a polysaccharide-based diet was also observed in patients with multiple sclerosis, in whom a 12-month polysaccharide-based multinutrient diet contributed to a significant decrease in IL-2, TNF-α, EGF, and CD95 + CD34+ cell subsets (157).
Fatty Acids
Fatty acids provide an important source of energy, are components of the cell membrane, and modulate cell function by acting as signaling molecules that can regulate gene expression (158). They can also influence immune cell functions by serving as precursors for the synthesis of lipid compounds involved in the regulation of immune responses and inflammatory pathways (158, 159). Metabolic derivatives of fatty acids, including eicosapentaenoic acid (EPA) and docosahexaenoic acid (DHA), are precursors for anti-inflammatory molecules that contribute to monocyte recruitment to sites of inflammation, where they engulf and remove apoptotic neutrophils (160). Fatty acids regulate the phagocytic activity of macrophages, infiltration of DCs into lymph nodes and activation of mast cells (161, 162). They also cross-react with the peroxisome proliferation activation receptor and TLRs (163, 164).
Fatty acids can have a dual effect on the regulation of inflammation, depending on whether or not double bonds are present between the individual carbon atoms (unsaturated and saturated fatty acids). Saturated fatty acids have been shown to stimulate in vitro and in vivo the intracellular macromolecular complex Nod-like receptor protein 3 (NLRP3) inflammasome, which promotes the production of the proinflammatory cytokines IL-1β and IL-18, whereas unsaturated fatty acids exert an inhibitory effect on the NLRP3 inflammasome by limiting the activity of the transcription factor NF-κB (165), an observation that could be taken into account in clinical nutrition studies to attenuate inflammatory conditions in diseases.
The concentration of fatty acids is a factor that may influence their effect on immune cells. Low concentrations of free fatty acids induce T cell proliferation and cytokine production, whereas high concentrations of free fatty acids cause mitochondrial membrane dysfunction, leading to activation of apoptotic pathways and cell death (166).
Fatty acids, as components of lipid rafts in the cell membrane, can modulate cell signaling, which affects immune cell function, considering that lipid rafts have been shown to contribute to Th cell activation (167). Metabolic derivatives of omega-3 fatty acids have also been shown to prevent the differentiation of Th0 cells into proinflammatory Th cells and decrease the secretion of proinflammatory cytokines (IL-2, IFN-γ, TNF-α, IL-17) by Th and Tc cells (168).
Contradictions exist regarding the effects of omega-3 fatty acids on B cell activation, as in vitro cultures of B cells isolated from the spleens of mice showed no change in the expression of B cell activation markers, whereas in vivo assessment of B cell activation in mice fed a fatty acid-enriched diet showed increased levels of the activation markers CD69, MHC-II, and CD11c (169–171). However, DHA and EPA stimulate IgM production by B cells by increasing the number of antibody-producing cells (172, 173)
An anti-atherosclerotic effect of omega-3 fatty acids has been suggested, as they have been shown to decrease the expression of adhesion molecules by endothelial cells, thereby affecting the migration of leukocytes from the bloodstream into tissues and the subsequent formation of inflammatory foam cells (174).
In a study of 940 children, polyunsaturated fatty acids were shown to reduce the risk of allergic diseases (asthma, rhinitis, and aeroallergen sensitization) (175).
It has been shown that intake of short-chain fatty acids in multiple sclerosis patients or healthy controls promotes the differentiation of Th0 cells into Tregs with increased suppressive capacity (176).
A positive effect of fatty acids on immunity and inflammation has also been demonstrated in breast cancer patients who followed an enriched fish oil diet, including DHA and EPA, resulting in the maintenance of Th cell and serum hsCRP levels, indicating a positive contribution of fatty acids to immune system function and inflammatory response (177). Omega-3 fatty acids have shown a positive effect on attenuating inflammation in patients with chronic kidney disease, as their supplementation resulted in increased production of LTB5 and specialized proresolving lipid mediators, which mediate the resolution of inflammation, and decreased levels of myeloperoxidase, an inflammatory mediator secreted by neutrophils (178).
A significant decrease in the levels of proinflammatory cytokines IL-17A and TNF-α was observed in patients with asthma who received a daily capsule of DHA and EPA, indicating a potent role of fatty acids as a complementary approach in the treatment of this disease (179).
Future Perspectives
Macro- and micronutrients exert critical and diverse roles in both innate and adaptive immunity by regulating the proliferation, function, and activity of various types of immune cells, as well as their interactions and signal transduction associated with inflammatory responses. Micronutrient deficiencies and malnutrition are common in most countries of the world, highlighting the need for well-organized nutritional care for the general population to prevent disease. Specific guidelines for nutritional care and assessment of patients’ nutritional status are published to guide physicians on the best therapeutic strategies. However, medical nutritional therapy of critically ill patients requires further investigation to describe the relationship between nutrients and patients’ immune physiology (181, 182). Nutritional care of cancer patients undergoing chemotherapy or radiotherapy is a challenge to improve the efficacy and outcomes of cancer treatment and limit disease progression (183). A detailed study of the effect of nutrients on the immune system is an important goal of nutritional immunology (184). Molecular signaling of specific micronutrients is being studied to find drug targets for specific diseases associated with micronutrient deficiency, such as targeting the zinc transporter to treat insulin resistance in type 2 diabetes (185). Studies are being conducted to investigate the effects of specific dietary interventions on human disease progression and chronic inflammation (186). Diets containing nutrients with anti-inflammatory properties have been shown to reduce the risk of depression and attenuate the severity of depression symptoms (187). The study of food consumption in overweight adolescents has revealed the existence of a unique dietary profile in this group associated with obesity (188). The role of nutrition in the management of the severity of COVID-19 and the recovery of surviving patients is currently under investigation; in particular, supplementation of vitamins C, D and zinc to improve patient health, restore immune homeostasis, and reduce the risk of infection for healthy individuals, and probiotics to improve gastrointestinal symptoms in COVID-19 patients (189).
The field is open for further studies that will help to clarify the biological role of food metabolites in the physiology of the organism and especially the immune system, as well as the association of specific dietary nutrients with the pathogenesis of diseases.
Author Contributions
AM and CT conceived and coordinated the study. ET and AM did the literature search and analysis and wrote the manuscript. AM and CT were responsible for the revision of the manuscript for important intellectual content. All authors contributed to the article and approved the submitted version.
Funding
ET is a recipient of KARATHEODORIS grant #80672 from the University of Patras. Τhe publication of this article has been financed by the Research Committee of the University of Patras.
Conflict of Interest
The authors declare that the research was conducted in the absence of any commercial or financial relationships that could be construed as a potential conflict of interest.
Abbreviations
APC, antigen-presenting cell; APRIL, a proliferation-inducing ligand; ATRA, all-trans-retinoic acid; BAFF, B-cell activating factor; CCR9, chemokine receptor 9; DC, dendritic cell; DHA, dehydroascorbic acid or docosahexaenoic acid; DMB, vitamin D, magnesium and vitamin B12; EPA, eicosapentaenoic acid; GALT, gut-associated lymphoid tissue; LPS, lipopolysaccharide; M cell, microfold cell; NASH, nonalcoholic steatohepatitis; NK cell, natural killer cell; NLRP, Nod-like receptor protein; NO, nitric oxide; PGRP, peptidoglycan recognition receptor protein; ROS, reactive oxygen species; PPs, Peyer’s patches; RAR, retinoic acid receptor; RXR, retinoid X receptor; sCD14, soluble CD14; Tc, T cytotoxic; Th, T helper; TLR, Toll-like receptor; Treg, regulatory T cell; TRX, thioredoxin-like protein;
VDR, vitamin D receptor.
References
1. Koithan M, Devika J. New Approaches to Nutritional Therapy. J Nurse Pract (2010) 6:805–6. doi: 10.1016/j.nurpra.2010.07.001
2. Walls J, Sinclair L, Finlay D. Nutrient Sensing, Signal Transduction and Immune Responses. Semin Immunol (2016) 28:396–407. doi: 10.1016/j.smim.2016.09.001
3. Wu D, Lewis ED, Pae M, Meydani SN. Nutritional Modulation of Immune Function: Analysis of Evidence, Mechanisms, and Clinical Relevance. Front Immunol (2018) 9:3160. doi: 10.3389/fimmu.2018.03160
4. Oz HS. Nutrients, Infectious and Inflammatory Diseases. Nutrients (2017) 9:1085. doi: 10.3390/nu9101085
5. Sharma P, Dwivedi S. Nutrigenomics and Nutrigenetics: New Insight in Disease Prevention and Cure. Indian J Clin Biochem (2017) 32:371–3. doi: 10.1007/s12291-017-0699-5
6. Shi HN, Walker WA. Development and Physiology of the Intestinal Mucosal Defense. Mucosal Immunol (2015) 1:9–29. doi: 10.1016/B978-0-12-415847-4.00002-1
7. McDole JR, Wheeler LW, McDonald KG, Wang B, Konjufca V, Knoop KA, et al. Goblet Cells Deliver Luminal Antigen to CD103+ Dendritic Cells in the Small Intestine. Nature (2012) 483:345–9. doi: 10.1038/nature10863
8. Stagg AJ. Intestinal Dendritic Cells in Health and Gut Inflammation. Front Immunol (2018) 9:2883. doi: 10.3389/fimmu.2018.02883
9. Kadowaki N. Dendritic Cells: A Conductor of T Cell Differentiation. Allergol Int (2007) 56:193–9. doi: 10.2332/allergolint.R-07-146
10. Coombes JL, Siddiqui KR, Arancibia-Cárcamo CV, Hall J, Sun C-M, Belkaid Y, et al. A Functionally Specialized Population of Mucosal CD103+ Dcs Induces Foxp3+ Regulatory T Cells Via a TGF-β–and Retinoic Acid–Dependent Mechanism. J Exp Med (2007) 204:1757–64. doi: 10.1084/jem.20070590
11. Ohteki T, Tezuka H. Regulation of IgA Production by Intestinal Dendritic Cells and Related Cells. Front Immunol (2019) 10:1891. doi: 10.3389/fimmu.2019.01891
12. Kobayashi N, Takahashi D, Takano S, Kimura S, Hase K. The Roles of Peyer’s Patches and Microfold Cells in the Gut Immune System: Relevance to Autoimmune Diseases. Front Immunol (2019) 10:2345. doi: 10.3389/fimmu.2019.02345
13. Korem T, Zeevi D, Suez J, Weinberger A, Avnit-Sagi T, Pompan-Lotan M, et al. Growth Dynamics of Gut Microbiota in Health and Disease Inferred From Single Metagenomic Samples. Science (2015) 349:1101–6. doi: 10.1126/science.aac4812
14. Levy M, Thaiss CA, Elinav E. Metagenomic Cross-Talk: The Regulatory Interplay Between Immunogenomics and the Microbiome. Genome Med (2015) 7:120. doi: 10.1186/s13073-015-0249-9
15. Rooks MG, Garrett WS. Gut Microbiota, Metabolites and Host Immunity. Nat Rev Immunol (2016) 16:341–52. doi: 10.1038/nri.2016.42
16. Belkaid Y, Hand TW. Role of the Microbiota in Immunity and Inflammation. Cell (2014) 157:121–41. doi: 10.1016/j.cell.2014.03.011
17. Spreckley E, Murphy KG. The L-Cell in Nutritional Sensing and the Regulation of Appetite. Front Nutr (2015) 2:23. doi: 10.3389/fnut.2015.00023
18. Den Besten G, van Eunen K, Groen AK, Venema K, Reijngoud D-J, Bakker BM. The Role of Short-Chain Fatty Acids in the Interplay Between Diet, Gut Microbiota, and Host Energy Metabolism. J Lipid Res (2013) 54:2325–40. doi: 10.1194/jlr.R036012
19. Wan Y, Wang F, Yuan J, Li J, Jiang D, Zhang J, et al. Effects of Dietary Fat on Gut Microbiota and Faecal Metabolites, and Their Relationship With Cardiometabolic Risk Factors: A 6-Month Randomised Controlled-Feeding Trial. Gut (2019) 68:1417–29. doi: 10.1136/gutjnl-2018-317609
20. Telle-Hansen VH, Holven KB, Ulven SM. Impact of a Healthy Dietary Pattern on Gut Microbiota and Systemic Inflammation in Humans. Nutrients (2018) 10:1783. doi: 10.3390/nu10111783
21. David LA, Maurice CF, Carmody RN, Gootenberg DB, Button JE, Wolfe BE, et al. Diet Rapidly and Reproducibly Alters the Human Gut Microbiome. Nature (2014) 505:559–63. doi: 10.1038/nature12820
22. Tan K, Zhang H, Lim L-S, Ma H, Li S, Zheng H. Roles of Carotenoids in Invertebrate Immunology. Front Immunol (2020) 10:3041. doi: 10.3389/fimmu.2019.03041
23. Ghodratizadeh S, Kanbak G, Beyramzadeh M, Dikmen ZG, Memarzadeh S, Habibian R. Effect of Carotenoid β-Cryptoxanthin on Cellular and Humoral Immune Response in Rabbit. Vet Res Commun (2014) 38:59–62. doi: 10.1007/s11259-013-9584-8
24. Shrestha S, Kim SY, Yun YJ, Kim JK, Lee JM, Shin M, et al. Retinoic Acid Induces Hypersegmentation and Enhances Cytotoxicity of Neutrophils Against Cancer Cells. Immunol Lett (2017) 182:24–9. doi: 10.1016/j.imlet.2017.01.001
25. Kim SY, Koo JE, Song MR, Lee JY. Retinol Suppresses the Activation of Toll-like Receptors in MyD88- and STAT1-Independent Manners. Inflammation (2013) 36:426–33. doi: 10.1007/s10753-012-9562-2
26. Larange A, Cheroutre H. Retinoic Acid and Retinoic Acid Receptors as Pleiotropic Modulators of the Immune System. Annu Rev Immunol (2016) 34:369–94. doi: 10.1146/annurev-immunol-041015-055427
27. Blomhoff H, Smeland EB, Erikstein B, Rasmussen AM, Skrede B, Skjønsberg C, et al. Vitamin A Is a Key Regulator for Cell Growth, Cytokine Production, and Differentiation in Normal B Cells. J Biol Chem (1992) 267:23988–92. doi: 10.1016/S0021-9258(18)35934-9
28. Pantazi E, Marks E, Stolarczyk E, Lycke N, Noelle RJ, Elgueta R. Cutting Edge: Retinoic Acid Signaling in B Cells Is Essential for Oral Immunization and Microflora Composition. J Immunol (2015) 195:1368–71. doi: 10.4049/jimmunol.1500989
29. Pino-Lagos K, Guo Y, Brown C, Alexander MP, Elgueta R, Bennett KA, et al. A Retinoic Acid–Dependent Checkpoint in the Development of CD4+ T Cell-Mediated Immunity. J Exp Med (2011) 208:1767–75. doi: 10.1084/jem.20102358
30. Seo G-Y, Lee J-M, Jang Y-S, Kang SG, S-i Y, Ko H-J, et al. Mechanism Underlying the Suppressor Activity of Retinoic Acid on IL4-Induced Ige Synthesis and Its Physiological Implication. Cell Immunol (2017) 322:49–55. doi: 10.1016/j.cellimm.2017.10.001
31. Stephensen CB, Rasooly R, Jiang X, Ceddia MA, Weaver CT, Chandraratna RA, et al. Vitamin A Enhances In Vitro Th2 Development Via Retinoid X Receptor Pathway. J Immunol (2002) 168:4495–503. doi: 10.4049/jimmunol.168.9.4495
32. Mucida D, Park Y, Kim G, Turovskaya O, Scott I, Kronenberg M, et al. Reciprocal TH17 and Regulatory T Cell Differentiation Mediated by Retinoic Acid. Science (2007) 317:256–60. doi: 10.1126/science.1145697
33. Di Caro V, Phillips B, Engman C, Harnaha J, Trucco M, Giannoukakis N. Retinoic Acid-Producing, Ex-Vivo-Generated Human Tolerogenic Dendritic Cells Induce the Proliferation of Immunosuppressive B Lymphocytes. Clin Exp Immunol (2013) 174:302–17. doi: 10.1111/cei.12177
34. Udhayabanu T, Manole A, Rajeshwari M, Varalakshmi P, Houlden H, Ashokkumar B. Riboflavin Responsive Mitochondrial Dysfunction in Neurodegenerative Diseases. J Clin Med (2017) 6:52. doi: 10.3390/jcm6050052
35. Almeida L, Lochner M, Berod L, Sparwasser T. Metabolic Pathways in T Cell Activation and Lineage Differentiation. Sem Immunol (2016) 28:514–24. doi: 10.1016/j.smim.2016.10.009
36. Dey S, Bishayi B. Riboflavin Along With Antibiotics Balances Reactive Oxygen Species and Inflammatory Cytokines and Controls Staphylococcus Aureus Infection by Boosting Murine Macrophage Function and Regulates Inflammation. J Inflammation (Lond) (2016) 13:36. doi: 10.1186/s12950-016-0145-0
37. Mazur-Bialy AI, Pochec E, Plytycz B. Immunomodulatory Effect of Riboflavin Deficiency and Enrichment - Reversible Pathological Response Versus Silencing of Inflammatory Activation. J Physiol Pharmacol (2015) 66:793–802.
38. Mazur-Bialy AI, Pocheć E. Riboflavin Reduces Proinflammatory Activation of Adipocyte-Macrophage Co-Culture. Potential Application of Vitamin B2 Enrichment for Attenuation of Insulin Resistance and Metabolic Syndrome Development. Molecules (2016) 21:1724. doi: 10.3390/molecules21121724
39. Kuiper C, Vissers M. Ascorbate as a Co-Factor for Fe-and 2-Oxoglutarate Dependent Dioxygenases: Physiological Activity in Tumor Growth and Progression. Front Oncol (2014) 4:359. doi: 10.3389/fonc.2014.00359
40. Cárcamo JM, Pedraza A, Bórquez-Ojeda O, Zhang B, Sanchez R, Golde DW. Vitamin C Is a Kinase Inhibitor: Dehydroascorbic Acid Inhibits IκBα Kinase β. Mol Cell Biol (2004) 24:6645–52. doi: 10.1128/MCB.24.15.6645-6652.2004
41. Molina N, Morandi AC, Bolin AP, Otton R. Comparative Effect of Fucoxanthin and Vitamin C on Oxidative and Functional Parameters of Human Lymphocytes. Int Immunopharmacol (2014) 22:41–50. doi: 10.1016/j.intimp.2014.06.026
42. Bozonet SM, Carr AC, Pullar JM, Vissers M. Enhanced Human Neutrophil Vitamin C Status, Chemotaxis and Oxidant Generation Following Dietary Supplementation With Vitamin C-Rich SunGold Kiwifruit. Nutrients (2015) 7:2574–88. doi: 10.3390/nu7042574
43. Huijskens MJ, Walczak M, Sarkar S, Atrafi F, Senden-Gijsbers BL, Tilanus MG, et al. Ascorbic Acid Promotes Proliferation of Natural Killer Cell Populations in Culture Systems Applicable for Natural Killer Cell Therapy. Cytotherapy (2015) 17:613–20. doi: 10.1016/j.jcyt.2015.01.004
44. Magrì A, Germano G, Lorenzato A, Lamba S, Chilà R, Montone M, et al. High-Dose Vitamin C Enhances Cancer Immunotherapy. Sci Transl Med (2020) 12:eaay8707. doi: 10.1126/scitranslmed.aay8707
45. Kishimoto Y, Saito N, Kurita K, Shimokado K, Maruyama N, Ishigami A. Ascorbic Acid Enhances the Expression of Type 1 and Type 4 Collagen and SVCT2 in Cultured Human Skin Fibroblasts. Biochem Biophys Res Commun (2013) 430:579–84. doi: 10.1016/j.bbrc.2012.11.110
46. Mohammed BM, Fisher BJ, Kraskauskas D, Ward S, Wayne JS, Brophy DF, et al. Vitamin C Promotes Wound Healing Through Novel Pleiotropic Mechanisms. Int Wound J (2016) 13:572–84. doi: 10.1111/iwj.12484
47. Huijskens MJ, Walczak M, Koller N, Briedé JJ, Senden-Gijsbers BL, Schnijderberg MC, et al. Technical Advance: Ascorbic Acid Induces Development of Double-Positive T Cells From Human Hematopoietic Stem Cells in the Absence of Stromal Cells. J Leukoc Biol (2014) 96:1165–75. doi: 10.1189/jlb.1TA0214-121RR
48. Kouakanou L, Yan X, Peters C, He J, Wu Y, Yin Z, et al. Vitamin C Promotes the Proliferation and Effector Functions of Human γδ T Cells. Cell Mol Immunol (2020) 17:462–73. doi: 10.1038/s41423-019-0247-8
49. Qi T, Sun M, Zhang C, Chen P, Xiao C, Chang X. Ascorbic Acid Promotes Plasma Cell Differentiation Through Enhancing TET2/3-mediated DNA Demethylation. Cell Rep (2020) 33:108452. doi: 10.1016/j.celrep.2020.108452
50. Pike JW, Christakos S. Biology and Mechanisms of Action of the Vitamin D Hormone. Endocrinol Metab Clin North Am (2017) 46:815–43. doi: 10.1016/j.ecl.2017.07.001
51. Coleman LA, Mishina M, Thompson M, Spencer SM, Reber AJ, Davis WG, et al. Age, Serum 25-Hydroxyvitamin D and Vitamin D Receptor (VDR) Expression and Function in Peripheral Blood Mononuclear Cells. Oncotarget (2016) 7:35512–21. doi: 10.18632/oncotarget.9398
52. Chen S, Sims GP, Chen XX, Gu YY, Chen S, Lipsky PE. Modulatory Effects of 1, 25-Dihydroxyvitamin D3 on Human B Cell Differentiation. J Immunol (2007) 179:1634–47. doi: 10.4049/jimmunol.179.3.1634
53. Mahon BD, Wittke A, Weaver V, Cantorna MT. The Targets of Vitamin D Depend on the Differentiation and Activation Status of CD4 Positive T Cells. J Cell Biochem (2003) 89:922–32. doi: 10.1002/jcb.10580
54. Gil A, Plaza-Diaz J, Mesa MD. Vitamin D: Classic and Novel Actions. Ann Nutr Metab (2018) 72:87–95. doi: 10.1159/000486536
55. Ryan JW, Anderson PH, Morris HA. Pleiotropic Activities of Vitamin D Receptors–Adequate Activation for Multiple Health Outcomes. Clin Biochem Rev (2015) 36:53–61.
56. Baeke F, Takiishi T, Korf H, Gysemans C, Mathieu C. Vitamin D: Modulator of the Immune System. Curr Opin Pharmacol (2010) 10:482–96. doi: 10.1016/j.coph.2010.04.001
57. Xie Z, Chen J, Zheng C, Wu J, Cheng Y, Zhu S, et al. 1,25-Dihydroxyvitamin D(3)-Induced Dendritic Cells Suppress Experimental Autoimmune Encephalomyelitis by Increasing Proportions of the Regulatory Lymphocytes and Reducing T Helper Type 1 and Type 17 Cells. Immunology (2017) 152:414–24. doi: 10.1111/imm.12776
58. Boonstra A, Barrat FJ, Crain C, Heath VL, Savelkoul HF, O’Garra A. 1alpha,25-Dihydroxyvitamin D3 has a Direct Effect on Naive CD4(+) T Cells to Enhance the Development of Th2 Cells. J Immunol (2001) 167:4974–80. doi: 10.4049/jimmunol.167.9.4974
59. Fawaz L, Mrad MF, Kazan JM, Sayegh S, Akika R, Khoury SJ. Comparative Effect of 25(OH)D3 and 1,25(OH)2D3 on Th17 Cell Differentiation. Clin Immunol (2016) 167:59–71. doi: 10.1016/j.clim.2016.02.011
60. Şıklar Z, Karataş D, Doğu F, Hacıhamdioğlu B, İkincioğulları A, Berberoğlu M. Regulatory T Cells and Vitamin D Status in Children With Chronic Autoimmune Thyroiditis. J Clin Res Pediatr Endocrinol (2016) 8:276–81. doi: 10.4274/jcrpe.2766
61. Sadeghi K, Wessner B, Laggner U, Ploder M, Tamandl D, Friedl J, et al. Vitamin D3 Down-Regulates Monocyte TLR Expression and Triggers Hyporesponsiveness to Pathogen-Associated Molecular Patterns. Eur J Immunol (2006) 36:361–70. doi: 10.1002/eji.200425995
62. Onwuneme C, Blanco A, O’Neill A, Watson B, Molloy EJ. Vitamin D Enhances Reactive Oxygen Intermediates Production in Phagocytic Cells in Term and Preterm Infants. Pediatr Res (2016) 79:654–61. doi: 10.1038/pr.2015.268
63. Gombart AF, Borregaard N, Koeffler HP. Human Cathelicidin Antimicrobial Peptide (CAMP) Gene Is a Direct Target of the Vitamin D Receptor and Is Strongly Up-Regulated in Myeloid Cells by 1, 25-Dihydroxyvitamin D3. FASEB J (2005) 19:1067–77. doi: 10.1096/fj.04-3284com
64. Ferreira GB, Vanherwegen AS, Eelen G, Gutierrez ACF, Lommel LV, Marchal K, et al. Vitamin D3 Induces Tolerance in Human Dendritic Cells by Activation of Intracellular Metabolic Pathways. Cell Rep (2015) 10:711–25. doi: 10.1016/j.celrep.2015.01.013
65. Traber MG. Vitamin E Regulatory Mechanisms. Annu Rev Nutr (2007) 27:347–62. doi: 10.1146/annurev.nutr.27.061406.093819
66. Pein H, Ville A, Pace S, Temml V, Garscha U, Raasch M, et al. Endogenous Metabolites of Vitamin E Limit Inflammation by Targeting 5-Lipoxygenase. Nat Commun (2018) 9:018–06158. doi: 10.1038/s41467-018-06158-5
67. Lee GY, Han SN. The Role of Vitamin E in Immunity. Nutrients (2018) 10:1614. doi: 10.3390/nu10111614
68. Li-Weber M, Weigand MA, Giaisi M, Süss D, Treiber MK, Baumann S, et al. Vitamin E Inhibits CD95 Ligand Expression and Protects T Cells From Activation-Induced Cell Death. J Clin Invest (2002) 110:681–90. doi: 10.1172/JCI0215073
69. Malmberg K-J, Lenkei R, Petersson M, Ohlum T, Ichihara F, Glimelius B, et al. A Short-Term Dietary Supplementation of High Doses of Vitamin E Increases T Helper 1 Cytokine Production in Patients With Advanced Colorectal Cancer. Clin Cancer Res (2002) 8:1772–8.
70. Crider KS, Yang TP, Berry RJ, Bailey LB. Folate and DNA Methylation: A Review of Molecular Mechanisms and the Evidence for Folate’s Role. Adv Nutr (2012) 3:21–38. doi: 10.3945/an.111.000992
71. Kinoshita M, Kayama H, Kusu T, Yamaguchi T, Kunisawa J, Kiyono H, et al. Dietary Folic Acid Promotes Survival of Foxp3+ Regulatory T Cells in the Colon. J Immunol (2012) 189:2869–78. doi: 10.4049/jimmunol.1200420
72. Safir N, Wendel A, Saile R, Chabraoui L. The Effect of Selenium on Immune Functions of J774. 1 Cells. Clin Chem Lab Med (2003) 41:1005–11. doi: 10.1515/CCLM.2003.154
73. Weitzel F, Wendel A. Selenoenzymes Regulate the Activity of Leukocyte 5-Lipoxygenase Via the Peroxide Tone. J Biol Chem (1993) 268:6288–92. doi: 10.1016/S0021-9258(18)53251-8
74. Hoffmann FW, Hashimoto AC, Shafer LA, Dow S, Berry MJ, Hoffmann PR. Dietary Selenium Modulates Activation and Differentiation of CD4+ T Cells in Mice Through a Mechanism Involving Cellular Free Thiols. J Nutr (2010) 140:1155–61. doi: 10.3945/jn.109.120725
75. Zhang Y, Roh YJ, Han SJ, Park I, Lee HM, Ok YS, et al. Role of Selenoproteins in Redox Regulation of Signaling and the Antioxidant System: A Review. Antioxidants (Basel) (2020) 9:383. doi: 10.3390/antiox9050383
76. Kardos J, Héja L, Simon Á, Jablonkai I, Kovács R, Jemnitz K. Copper Signalling: Causes and Consequences. Cell Commun Signal (2018) 16:1–22. doi: 10.1186/s12964-018-0292-4
77. González M, Reyes-Jara A, Suazo M, Jo WJ, Vulpe C. Expression of Copper-Related Genes in Response to Copper Load. Am J Clin Nutr (2008) 88:830S–4S. doi: 10.1093/ajcn/88.3.830S
78. Liu M-J, Bao S, Gálvez-Peralta M, Pyle CJ, Rudawsky AC, Pavlovicz RE, et al. ZIP8 Regulates Host Defense Through Zinc-Mediated Inhibition of NF-κb. Cell Rep (2013) 3:386–400. doi: 10.1016/j.celrep.2013.01.009
79. Brieger A, Rink L, Haase H. Differential Regulation of TLR-dependent MyD88 and TRIF Signaling Pathways by Free Zinc Ions. J Immunol (2013) 191:1808–17. doi: 10.4049/jimmunol.1301261
80. Metz CH, Schröder AK, Overbeck S, Kahmann L, Plümäkers B, Rink L. T-Helper Type 1 Cytokine Release Is Enhanced by In Vitro Zinc Supplementation Due to Increased Natural Killer Cells. Nutrition (2007) 23:157–63. doi: 10.1016/j.nut.2006.10.007
81. Puig S, Ramos-Alonso L, Romero AM, Martínez-Pastor MT. The Elemental Role of Iron in DNA Synthesis and Repair. Metallomics (2017) 9:1483–500. doi: 10.1039/C7MT00116A
82. Ward RJ, Crichton RR, Taylor DL, Della Corte L, Srai SK, Dexter DT. Iron and the Immune System. J Neural Transm (2011) 118:315–28. doi: 10.1007/s00702-010-0479-3
83. Saeed F, Nadeem M, Ahmed RS, Tahir Nadeem M, Arshad MS, Ullah A. Studying the Impact of Nutritional Immunology Underlying the Modulation of Immune Responses by Nutritional Compounds-a Review. Food Agr Immunol (2016) 27:205–29. doi: 10.1080/09540105.2015.1079600
84. Lotto V, Choi S-W, Friso S. Vitamin B6: A Challenging Link Between Nutrition and Inflammation in CVD. Br J Nutr (2011) 106:183–95. doi: 10.1017/S0007114511000407
85. Gaffney-Stomberg E, McClung JP. Inflammation and Diminished Iron Status: Mechanisms and Functional Outcomes. Curr Opin Clin Nutr Metab Care (2012) 15:605–13. doi: 10.1097/MCO.0b013e328357f63b
86. Marques AH, O’Connor TG, Roth C, Susser E, Bjorke-Monsen AL. The Influence of Maternal Prenatal and Early Childhood Nutrition and Maternal Prenatal Stress on Offspring Immune System Development and Neurodevelopmental Disorders. Front Neurosci (2013) 7:120. doi: 10.3389/fnins.2013.00120
87. Purandare C. Maternal Nutritional Deficiencies and Interventions. J Obstet Gynaecol India (2012) 62:621–3. doi: 10.1007/s13224-013-0347-9
88. Kondo N, Kobayashi Y, Shinoda S, Kasahara K, Kameyama T, Iwasa S, et al. Cord Blood Lymphocyte Responses to Food Antigens for the Prediction of Allergic Disorders. Arch Dis Child (1992) 67:1003–7. doi: 10.1136/adc.67.8.1003
89. Maijo M, Clements SJ, Ivory K, Nicoletti C, Carding SR. Nutrition, Diet and Immunosenescence. Mech Ageing Dev (2014) 136:116–28. doi: 10.1016/j.mad.2013.12.003
90. Ahmad SM, Haskell MJ, Raqib R, Stephensen CB. Markers of Innate Immune Function Are Associated With Vitamin a Stores in Men. J Nutr (2009) 139:377–85. doi: 10.3945/jn.108.100198
91. Lee JH, Kim B, Jin WJ, Kim HH, Ha H, Lee ZH. Pathogenic Roles of CXCL10 Signaling Through CXCR3 and TLR4 in Macrophages and T Cells: Relevance for Arthritis. Arthritis Res Ther (2017) 19:163. doi: 10.1186/s13075-017-1353-6
92. Ahmad SM, Huda MN, Raqib R, Qadri F, Alam MJ, Afsar MNA, et al. High-Dose Neonatal Vitamin A Supplementation to Bangladeshi Infants Increases the Percentage of CCR9-positive Treg Cells in Infants With Lower Birthweight in Early Infancy, and Decreases Plasma sCD14 Concentration and the Prevalence of Vitamin A Deficiency at Two Years of Age. J Nutr (2020) 150:3005–12. doi: 10.1093/jn/nxaa260
93. von Martels JZH, Bourgonje AR, Klaassen MAY, Alkhalifah HAA, Sadaghian Sadabad M, Vich Vila A, et al. Riboflavin Supplementation in Patients With Crohn’s Disease [The RISE-UP Study]. J Crohns Colitis (2020) 14:595–607. doi: 10.1093/ecco-jcc/jjz208
94. Vakilian A, Razavi-Nasab SM, Ravari A, Mirzaei T, Moghadam-Ahmadi A, Jalali N, et al. Vitamin B12 in Association With Antipsychotic Drugs can Modulate the Expression of Pro-/Anti-Inflammatory Cytokines in Alzheimer Disease Patients. Neuroimmunomodulation (2017) 24:310–9. doi: 10.1159/000486597
95. Paniz C, Bertinato JF, Lucena MR, De Carli E, Amorim P, Gomes GW, et al. A Daily Dose of 5 mg Folic Acid for 90 Days Is Associated With Increased Serum Unmetabolized Folic Acid and Reduced Natural Killer Cell Cytotoxicity in Healthy Brazilian Adults. J Nutr (2017) 147:1677–85. doi: 10.3945/jn.117.247445
96. Bayer AL, Fraker CA. The Folate Cycle as a Cause of Natural Killer Cell Dysfunction and Viral Etiology in Type 1 Diabetes. Front Endocrinol (2017) 8:315. doi: 10.3389/fendo.2017.00315
98. Carr AC, Maggini S. Vitamin C and Immune Function. Nutrients (2017) 9:1211. doi: 10.3390/nu9111211
99. Hagel AF, Layritz CM, Hagel WH, Hagel H-J, Hagel E, Dauth W, et al. Intravenous Infusion of Ascorbic Acid Decreases Serum Histamine Concentrations in Patients With Allergic and Non-Allergic Diseases. Naunyn Schmiedebergs Arch Pharmacol (2013) 386:789–93. doi: 10.1007/s00210-013-0880-1
100. Bucher A, White N. Vitamin C in the Prevention and Treatment of the Common Cold. Am J Lifestyle Med (2016) 10:181–3. doi: 10.1177/1559827616629092
101. Tan CW, Ho LP, Kalimuddin S, Cherng BPZ, Teh YE, Thien SY, et al. Cohort Study to Evaluate the Effect of Vitamin D, Magnesium, and Vitamin B(12) in Combination on Progression to Severe Outcomes in Older Patients With Coronavirus (COVID-19). Nutrition (2020) 79-80:111017. doi: 10.1016/j.nut.2020.111017
102. Camargo CA, Ganmaa D, Frazier AL, Kirchberg FF, Stuart JJ, Kleinman K, et al. Randomized Trial of Vitamin D Supplementation and Risk of Acute Respiratory Infection in Mongolia. Pediatrics (2012) 130:e561–7. doi: 10.1542/peds.2011-3029
103. Bergman P, Norlin A-C, Hansen S, Rekha RS, Agerberth B, Björkhem-Bergman L, et al. Vitamin D3 Supplementation in Patients With Frequent Respiratory Tract Infections: A Randomised and Double-Blind Intervention Study. BMJ Open (2012) 2:e001663. doi: 10.1136/bmjopen-2012-001663
104. Lemire JM, Archer DC, Beck L, Spiegelberg HL. Immunosuppressive Actions of 1, 25-Dihydroxyvitamin D3: Preferential Inhibition of Th1 Functions. J Nutr (1995) 125:1704S–8S. doi: 10.1016/0960-0760(95)00106-A
105. Zhang Y, Leung DY, Richers BN, Liu Y, Remigio LK, Riches DW, et al. Vitamin D Inhibits Monocyte/Macrophage Proinflammatory Cytokine Production by Targeting MAPK Phosphatase-1. J Immunol (2012) 188:2127–35. doi: 10.4049/jimmunol.1102412
106. Scott JM, Kazman JB, Palmer J, McClung JP, Gaffney-Stomberg E, Gasier HG. Effects of Vitamin D Supplementation on Salivary Immune Responses During Marine Corps Basic Training. Scand J Med Sci Sports (2019) 29:1322–30. doi: 10.1111/sms.13467
107. Chahardoli R, Saboor-Yaraghi AA, Amouzegar A, Khalili D, Vakili AZ, Azizi F. Can Supplementation With Vitamin D Modify Thyroid Autoantibodies (Anti-TPO Ab, Anti-Tg Ab) and Thyroid Profile (T3, T4, TSH) in Hashimoto’s Thyroiditis? A Double Blind, Randomized Clinical Trial. Horm Metab Res (2019) 51:296–301. doi: 10.1055/a-0856-1044
108. Savastio S, Cadario F, D’Alfonso S, Stracuzzi M, Pozzi E, Raviolo S, et al. Vitamin D Supplementation Modulates ICOS+ and ICOS- Regulatory T Cell in Siblings of Children With Type 1 Diabetes. J Clin Endocrinol Metab (2020) 105:dgaa588. doi: 10.1210/clinem/dgaa588
109. Sharifi A, Vahedi H, Nedjat S, Rafiei H, Hosseinzadeh-Attar MJ. Effect of Single-Dose Injection of Vitamin D on Immune Cytokines in Ulcerative Colitis Patients: A Randomized Placebo-Controlled Trial. Apmis (2019) 127:681–7. doi: 10.1111/apm.12982
110. Eckard AR, O’Riordan MA, Rosebush JC, Lee ST, Habib JG, Ruff JH, et al. Vitamin D Supplementation Decreases Immune Activation and Exhaustion in HIV-1-infected Youth. Antivir Ther (2018) 23:315–24. doi: 10.3851/IMP3199
111. Ghani SMA, Goon JA, Azman N, Zakaria SNA, Hamid Z, Ngah WZW. Comparing the Effects of Vitamin E Tocotrienol-Rich Fraction Supplementation and α-Tocopherol Supplementation on Gene Expression in Healthy Older Adults. Clinics (2019) 7:e688. doi: 10.6061/clinics/2019/e688
112. Bril F, Biernacki DM, Kalavalapalli S, Lomonaco R, Subbarayan SK, Lai J, et al. Role of Vitamin E for Nonalcoholic Steatohepatitis in Patients With Type 2 Diabetes: A Randomized Controlled Trial. Diabetes Care (2019) 42:1481–8. doi: 10.2337/dc19-0167
113. Ma F, Wu T, Zhao J, Song A, Liu H, Xu W, et al. Folic Acid Supplementation Improves Cognitive Function by Reducing the Levels of Peripheral Inflammatory Cytokines in Elderly Chinese Subjects With MCI. Sci Rep (2016) 6:37486. doi: 10.1038/srep37486
114. Dirajlal-Fargo S, Yu J, Kulkarni M, Sattar A, Funderburg N, Barkoukis H, et al. Brief Report: Zinc Supplementation and Inflammation in Treated HIV. J Acquir Immune Defic Syndr (2019) 82:275–80. doi: 10.1097/QAI.0000000000002129
115. Heller RA, Sun Q, Hackler J, Seelig J, Seibert L, Cherkezov A, et al. Prediction of Survival Odds in COVID-19 by Zinc, Age and Selenoprotein P as Composite Biomarker. Redox Biol (2021) 38:101764. doi: 10.1016/j.redox.2020.101764
116. Ahmad SM, Hossain MB, Monirujjaman M, Islam S, Huda MN, Kabir Y, et al. Maternal Zinc Supplementation Improves Hepatitis B Antibody Responses in Infants But Decreases Plasma Zinc Level. Eur J Nutr (2016) 55:1823–9. doi: 10.1007/s00394-015-0999-6
117. Meksawan K, Sermsri U, Chanvorachote P. Zinc Supplementation Improves Anticancer Activity of Monocytes in Type-2 Diabetic Patients With Metabolic Syndrome. Anticancer Res (2014) 34:295–9.
118. Afshar Ebrahimi F, Foroozanfard F, Aghadavod E, Bahmani F, Asemi Z. The Effects of Magnesium and Zinc Co-Supplementation on Biomarkers of Inflammation and Oxidative Stress, and Gene Expression Related to Inflammation in Polycystic Ovary Syndrome: A Randomized Controlled Clinical Trial. Biol Trace Elem Res (2018) 184:300–7. doi: 10.1007/s12011-017-1198-5
119. Hawkes WC, Kelley DS, Taylor PC. The Effects of Dietary Selenium on the Immune System in Healthy Men. Biol Trace Elem (2001) 81:189–213. doi: 10.1385/BTER:81:3:189
120. Ivory K, Prieto E, Spinks C, Armah CN, Goldson AJ, Dainty JR, et al. Selenium Supplementation has Beneficial and Detrimental Effects on Immunity to Influenza Vaccine in Older Adults. Clin Nutr (2017) 36:407–15. doi: 10.1016/j.clnu.2015.12.003
121. Heidar Z, Hamzepour N, Zadeh Modarres S, Mirzamoradi M, Aghadavod E, Pourhanifeh MH, et al. The Effects of Selenium Supplementation on Clinical Symptoms and Gene Expression Related to Inflammation and Vascular Endothelial Growth Factor in Infertile Women Candidate for In Vitro Fertilization. Biol Trace Elem Res (2020) 193:319–25. doi: 10.1007/s12011-019-01715-5
122. Rocha KC, Vieira ML, Beltrame RL, Cartum J, Alves SI, Azzalis LA, et al. Impact of Selenium Supplementation in Neutropenia and Immunoglobulin Production in Childhood Cancer Patients. J Med Food (2016) 19:560–8. doi: 10.1089/jmf.2015.0145
123. El-Zayat SR SH, Mannaa FA. Micronutrients and Many Important Factors That Affect the Physiological Functions of Toll-Like Receptors. Bull Natl Res Cent (2019) 43:123. doi: 10.1186/s42269-019-0165-z
124. Huang Z, Liu Y, Qi G, Brand D, Zheng S. Role of Vitamin A in the Immune System. J Clin Med (2018) 7:258. doi: 10.3390/jcm7090258
125. Mazur-Bialy AI, Pochec E. Vitamin B2 Deficiency Enhances the Proinflammatory Activity of Adipocyte, Consequences for Insulin Resistance and Metabolic Syndrome Development. Life Sci (2017) 178:9–16. doi: 10.1016/j.lfs.2017.04.010
126. Tamura J, Kubota K, Murakami H, Sawamura M, Matsushima T, Tamura T, et al. Immunomodulation by Vitamin B12: Augmentation of CD8+ T Lymphocytes and Natural Killer (NK) Cell Activity in Vitamin B12-deficient Patients by methyl-B12 Treatment. Clin Exp Immunol (1999) 116:28–32. doi: 10.1046/j.1365-2249.1999.00870.x
127. Erkurt MA, Aydogdu I, Dikilitaş M, Kuku I, Kaya E, Bayraktar N, et al. Effects of Cyanocobalamin on Immunity in Patients With Pernicious Anemia. Med Princ Pract (2008) 17:131–5. doi: 10.1159/000112967
128. Kuroishi T, Endo Y, Muramoto K, Sugawara S. Biotin Deficiency Up-Regulates TNF-Alpha Production in Murine Macrophages. J Leukoc Biol (2008) 83:912–20. doi: 10.1189/jlb.0607428
129. Nemazannikova N, Mikkelsen K, Stojanovska L, Blatch GL, Apostolopoulos V. Is There a Link Between Vitamin B and Multiple Sclerosis? Med Chem (2018) 14:170–80. doi: 10.2174/1573406413666170906123857
130. Scalabrino G, Corsi MM, Veber D, Buccellato FR, Pravettoni G, Manfridi A, et al. Cobalamin (Vitamin B(12)) Positively Regulates Interleukin-6 Levels in Rat Cerebrospinal Fluid. J Neuroimmunol (2002) 127:37–43. doi: 10.1016/S0165-5728(02)00095-4
131. Wessels I, Maywald M, Rink L. Zinc as a Gatekeeper of Immune Function. Nutrients (2017) 9:1286. doi: 10.3390/nu9121286
132. Kulik L, Maywald M, Kloubert V, Wessels I, Rink L. Zinc Deficiency Drives Th17 Polarization and Promotes Loss of Treg Cell Function. J Nutr Biochem (2019) 63:11–8. doi: 10.1016/j.jnutbio.2018.09.011
133. Vega L, Rodríguez-Sosa M, García-Montalvo EA, Del Razo LM, Elizondo G. Non-Optimal Levels of Dietary Selenomethionine Alter Splenocyte Response and Modify Oxidative Stress Markers in Female Mice. Food Chem Toxicol (2007) 45:1147–53. doi: 10.1016/j.fct.2006.12.021
134. Hoffmann PR, Berry MJ. The Influence of Selenium on Immune Responses. Mol Nutr Food Res (2008) 52:1273–80. doi: 10.1002/mnfr.200700330
135. Percival SS. Copper and Immunity. Am J Clin Nutr (1998) 67:1064S–8S. doi: 10.1093/ajcn/67.5.1064S
136. Arosa F, Da Silva A, Godinho I, Ter Steege J, Porto G, Rudd C, et al. Decreased CD8-p561ck Activity in Peripheral Blood T-lymphocytes From Patients With Hereditary Haemochromatosis. Scand J Immunol (1994) 39:426–32. doi: 10.1111/j.1365-3083.1994.tb03396.x
137. Ekiz C, Agaoglu L, Karakas Z, Gurel N, Yalcin I. The Effect of Iron Deficiency Anemia on the Function of the Immune System. Hematol J (2005) 5:579–83. doi: 10.1038/sj.thj.6200574
138. Hassan TH, Badr MA, Karam NA, Zkaria M, El Saadany HF, Abdel Rahman DM, et al. Impact of Iron Deficiency Anemia on the Function of the Immune System in Children. Medicine (2016) 95:e5395. doi: 10.1097/MD.0000000000005395
139. Al Tanoury Z, Piskunov A, Rochette-Egly C. Vitamin A and Retinoid Signaling: Genomic and Nongenomic Effects. J Lip Res (2013) 54:1761–75. doi: 10.1194/jlr.R030833
140. Rodriguez-Concepcion M, Avalos J, Bonet ML, Boronat A, Gomez-Gomez L, Hornero-Mendez D, et al. A Global Perspective on Carotenoids: Metabolism, Biotechnology, and Benefits for Nutrition and Health. Prog Lipid Res (2018) 70:62–93. doi: 10.1016/j.plipres.2018.04.004
141. Li P, Yin Y-L, Li D, Kim SW, Wu G. Amino Acids and Immune Function. Br J Nutr (2007) 98:237–52. doi: 10.1017/S000711450769936X
142. Grohmann U, Mondanelli G, Belladonna ML, Orabona C, Pallotta MT, Iacono A, et al. Amino-Acid Sensing and Degrading Pathways in Immune Regulation. Cytokine Growth Factor Rev (2017) 35:37–45. doi: 10.1016/j.cytogfr.2017.05.004
143. Whelan R. The Role of Amino Acids in the Immune System-a Special Focus on Broilers (2019). Available at: https://en.engormix.com/poultry-industry/articles/the-role-amino-acids-t43647.htm (Accessed November 2020).
144. Ruth MR, Field CJ. The Immune Modifying Effects of Amino Acids on Gut-Associated Lymphoid Tissue. J Anim Sci Biotechno (2013) 4:1–10. doi: 10.1186/2049-1891-4-27
145. Chalamaiah M, Yu W, Wu J. Immunomodulatory and Anticancer Protein Hydrolysates (Peptides) From Food Proteins: A Review. Food Chem (2018) 245:205–22. doi: 10.1016/j.foodchem.2017.10.087
146. Tang Y, Li J, Liao S, Qi M, Kong X, Tan B, et al. The Effect of Dietary Protein Intake on Immune Status in Pigs of Different Genotypes. Food Agr Immunol (2018) 29:776–84. doi: 10.1080/09540105.2018.1455812
147. Rubio-Patino C, Bossowski JP, De Donatis GM, Mondragon L, Villa E, Aira LE, et al. Low-Protein Diet Induces IRE1alpha-Dependent Anticancer Immunosurveillance. Cell Metab (2018) 27:828–42. doi: 10.1016/j.cmet.2018.02.009
148. Markova M, Koelman L, Hornemann S, Pivovarova O, Sucher S, Machann J, et al. Effects of Plant and Animal High Protein Diets on Immune-Inflammatory Biomarkers: A 6-Week Intervention Trial. Clin Nutr (2019) 39:862–9. doi: 10.1016/j.clnu.2019.03.019
149. Kim JE, Lin G, Zhou J, Mund JA, Case J, Campbell WW. Weight Loss Achieved Using an Energy Restriction Diet With Normal or Higher Dietary Protein Decreased the Number of CD14(++)CD16(+) Proinflammatory Monocytes and Plasma Lipids and Lipoproteins in Middle-Aged, Overweight, and Obese Adults. Nutr Res (2017) 40:75–84. doi: 10.1016/j.nutres.2017.02.007
150. Cobb BA, Kasper DL. Coming of Age: Carbohydrates and Immunity. Eur J Immunol (2005) 35:352–6. doi: 10.1002/eji.200425889
151. Cummings RD. Stuck on Sugars–How Carbohydrates Regulate Cell Adhesion, Recognition, and Signaling. Glycocon J (2019) 36:241–57. doi: 10.1007/s10719-019-09876-0
152. Sun L, Middleton DR, Wantuch PL, Ozdilek A, Avci FY. Carbohydrates as T-cell Antigens With Implications in Health and Disease. Glycobiology (2016) 26:1029–40. doi: 10.1093/glycob/cww062
153. Niemiro GM, Chiarlitti NA, Khan NA, De Lisio M. A Carbohydrate Beverage Reduces Monocytes Expressing TLR4 in Children With Overweight or Obesity. J Nutr (2019) 150:616–22. doi: 10.1093/jn/nxz294
154. Nieman DC. Influence of Carbohydrate on the Immune Response to Intensive, Prolonged Exercise. Exerc Immunol Rev (1998) 4:64–76.
155. Myette-Côté É, Durrer C, Neudorf H, Bammert TD, Botezelli JD, Johnson JD, et al. The Effect of a Short-Term Low-Carbohydrate, High-Fat Diet With or Without Postmeal Walks on Glycemic Control and Inflammation in Type 2 Diabetes: A Randomized Trial. Am J Physiol Regul Integr Comp Physiol (2018) 315:R1210–9. doi: 10.1152/ajpregu.00240.2018
156. Lewis JE, Atlas Bsn SE, Abbas MH, Rasul A, Farooqi A, Lantigua LA, et al. The Novel Effects of a Hydrolyzed Polysaccharide Dietary Supplement on Immune, Hepatic, and Renal Function in Adults With HIV in a Randomized, Double-Blind, Placebo-Control Trial. J Diet Suppl (2020) 17:429–41. doi: 10.1080/19390211.2019.1619010
157. Reginald McDaniel H, LaGanke C, Bloom L, Goldberg S, Lages LC, Lantigua LA, et al. The Effect of a Polysaccharide-Based Multinutrient Dietary Supplementation Regimen on Infections and Immune Functioning in Multiple Sclerosis. J Diet Suppl (2020) 17:184–99. doi: 10.1080/19390211.2018.1495675
158. Yaqoob P, Calder PC. Fatty Acids and Immune Function: New Insights Into Mechanisms. Br J Nutr (2007) 98:S41–5. doi: 10.1017/S0007114507832995
159. Nicolaou A, Mauro C, Urquhart P, Marelli-Berg F. Polyunsaturated Fatty Acid-Derived Lipid Mediators and T Cell Function. Front Immunol (2014) 5:75. doi: 10.3389/fimmu.2014.00075
160. Serhan CN, Chiang N, Van Dyke TE. Resolving Inflammation: Dual Anti-Inflammatory and Pro-Resolution Lipid Mediators. Nat Rev Immunol (2008) 8:349–61. doi: 10.1038/nri2294
161. Kumar NG, Contaifer D, Madurantakam P, Carbone S, Price ET, Van Tassell B, et al. Dietary Bioactive Fatty Acids as Modulators of Immune Function: Implications on Human Health. Nutrients (2019) 11:2974. doi: 10.3390/nu11122974
162. Radzikowska U, Rinaldi AO, Çelebi Sözener Z, Karaguzel D, Wojcik M, Cypryk K, et al. The Influence of Dietary Fatty Acids on Immune Responses. Nutrients (2019) 11:2990. doi: 10.3390/nu11122990
163. Huang S, Rutkowsky JM, Snodgrass RG, Ono-Moore KD, Schneider DA, Newman JW, et al. Saturated Fatty Acids Activate TLR-Mediated Proinflammatory Signaling Pathways. J Lipid Res (2012) 53:2002–13. doi: 10.1194/jlr.D029546
164. Liu M, Montgomery MK, Fiveash CE, Osborne B, Cooney GJ, Bell-Anderson K, et al. Pparα-Independent Actions of Omega-3 PUFAs Contribute to Their Beneficial Effects on Adiposity and Glucose Homeostasis. Sci Rep (2014) 4:5538. doi: 10.1038/srep05538
165. Sui Y-H, Luo W-J, Xu Q-Y, Hua J. Dietary Saturated Fatty Acid and Polyunsaturated Fatty Acid Oppositely Affect Hepatic NOD-like Receptor Protein 3 Inflammasome Through Regulating Nuclear Factor-Kappa B Activation. World J Gastroenterol (2016) 22:2533–44. doi: 10.3748/wjg.v22.i8.2533
166. de Jong AJ, Kloppenburg M, Toes RE, Ioan-Facsinay A. Fatty Acids, Lipid Mediators, and T-Cell Function. Front Immunol (2014) 5:483. doi: 10.3389/fimmu.2014.00483
167. Schieffer D, Naware S, Bakun W, Bamezai AK. Lipid Raft-Based Membrane Order Is Important for Antigen-Specific Clonal Expansion of CD4(+) T Lymphocytes. BMC Immunol (2014) 15:58. doi: 10.1186/s12865-014-0058-8
168. Chiurchiù V, Leuti A, Dalli J, Jacobsson A, Battistini L, Maccarrone M, et al. Proresolving Lipid Mediators Resolvin D1, Resolvin D2, D Maresin 1 Are Critical in Modulating T Cell Responses. Sci Transl Med (2016) 8:353ra111. doi: 10.1126/scitranslmed.aaf7483
169. Rockett BD, Teague H, Harris M, Melton M, Williams J, Wassall SR, et al. Fish Oil Increases Raft Size and Membrane Order of B Cells Accompanied by Differential Effects on Function. J Lipid Res (2012) 53:674–85. doi: 10.1194/jlr.M021782
170. Gurzell EA, Teague H, Harris M, Clinthorne J, Shaikh SR, Fenton JI. DHA-Enriched Fish Oil Targets B Cell Lipid Microdomains and Enhances Ex Vivo and In Vivo B Cell Function. J Leukoc Biol (2013) 93:463–70. doi: 10.1189/jlb.0812394
171. Rockett BD, Salameh M, Carraway K, Morrison K, Shaikh SR. N-3 PUFA Improves Fatty Acid Composition, Prevents Palmitate-Induced Apoptosis, and Differentially Modifies B Cell Cytokine Secretion In Vitro and Ex Vivo. J Lipid Res (2010) 51:1284–97. doi: 10.1194/jlr.M000851
172. Teague H, Fhaner CJ, Harris M, Duriancik DM, Reid GE, Shaikh SR. N-3 PUFAs Enhance the Frequency of Murine B-cell Subsets and Restore the Impairment of Antibody Production to a T-independent Antigen in Obesity. J Lipid Res (2013) 54:3130–8. doi: 10.1194/jlr.M042457
173. Ramon S, Gao F, Serhan CN, Phipps RP. Specialized Proresolving Mediators Enhance Human B Cell Differentiation to Antibody-Secreting Cells. J Immunol (2012) 189:1036–42. doi: 10.4049/jimmunol.1103483
174. Baker EJ, Yusof MH, Yaqoob P, Miles EA, Calder PC. Omega-3 Fatty Acids and Leukocyte-Endothelium Adhesion: Novel Anti-Atherosclerotic Actions. Mol Aspects Med (2018) 64:169–81. doi: 10.1016/j.mam.2018.08.002
175. Magnusson J, Ekström S, Kull I, Håkansson N, Nilsson S, Wickman M, et al. Polyunsaturated Fatty Acids in Plasma at 8 Years and Subsequent Allergic Disease. J Allergy Clin Immunol (2018) 142:510–516.e6. doi: 10.1016/j.jaci.2017.09.023
176. Duscha A, Jörg S, Berg J, Linker R, Gold R, Haghikia A. The Effect of the Orally Applied Short Chain Fatty Acid Propionate on the Immune Regulation in MS: Results of a Human Proof-of-Concept Study. Neurology (2017) 88:P3.388.
177. Paixão E, Oliveira ACM, Pizato N, Muniz-Junqueira MI, Magalhães KG, Nakano EY, et al. The Effects of EPA and DHA Enriched Fish Oil on Nutritional and Immunological Markers of Treatment Naïve Breast Cancer Patients: A Randomized Double-Blind Controlled Trial. Nutr J (2017) 16:71. doi: 10.1186/s12937-017-0295-9
178. Barden AE, Shinde S, Burke V, Puddey IB, Beilin LJ, Irish AB, et al. The Effect of N-3 Fatty Acids and Coenzyme Q10 Supplementation on Neutrophil Leukotrienes, Mediators of Inflammation Resolution and Myeloperoxidase in Chronic Kidney Disease. Prostaglandins Other Lipid Mediat (2018) 136:1–8. doi: 10.1016/j.prostaglandins.2018.03.002
179. Farjadian S, Moghtaderi M, Kalani M, Gholami T, Hosseini Teshnizi S. Effects of Omega-3 Fatty Acids on Serum Levels of T-helper Cytokines in Children With Asthma. Cytokine (2016) 85:61–6. doi: 10.1016/j.cyto.2016.06.002
180. Lean ME. Principles of Human Nutrition. Medicine (2019) 47:140–4. doi: 10.1016/j.mpmed.2018.12.014
181. Magee PJ, McCann MT. Micronutrient Deficiencies: Current Issues. Proc Nutr Soc (2019) 78:147–9. doi: 10.1017/S0029665118002677
182. Singer P, Blaser AR, Berger MM, Alhazzani W, Calder PC, Casaer MP, et al. ESPEN Guideline on Clinical Nutrition in the Intensive Care Unit. Clin Nutr (2019) 38:48–79. doi: 10.1016/j.clnu.2018.08.037
183. Cotogni P, Pedrazzoli P, De Waele E, Aprile G, Farina G, Stragliotto S, et al. Nutritional Therapy in Cancer Patients Receiving Chemoradiotherapy: Should We Need Stronger Recommendations to Act for Improving Outcomes? J Cancer (2019) 10:4318–25. doi: 10.7150/jca.31611
184. Ponton F, Wilson K, Cotter SC, Raubenheimer D, Simpson SJ. Nutritional Immunology: A Multi-Dimensional Approach. PloS Pathog (2011) 7:e1002223. doi: 10.1371/journal.ppat.1002223
185. Adulcikas J, Sonda S, Norouzi S, Sohal SS, Myers S. Targeting the Zinc Transporter ZIP7 in the Treatment of Insulin Resistance and Type 2 Diabetes. Nutrients (2019) 11:408. doi: 10.3390/nu11020408
186. Lankinen M, Uusitupa M, Schwab U. Nordic Diet and Inflammation-a Review of Observational and Intervention Studies. Nutrients (2019) 11:1369. doi: 10.3390/nu11061369
187. Tolkien K, Bradburn S, Murgatroyd C. An Anti-Inflammatory Diet as a Potential Intervention for Depressive Disorders: A Systematic Review and Meta-Analysis. Clin Nutr (2019) 38:2045–52. doi: 10.1016/j.clnu.2018.11.007
188. Andrade V, de Santana ML, Fukutani KF, Queiroz AT, Arriaga MB, Conceição-Machado MEP, et al. Multidimensional Analysis of Food Consumption Reveals a Unique Dietary Profile Associated With Overweight and Obesity in Adolescents. Nutrients (2019) 11:1946. doi: 10.3390/nu11081946
Keywords: micronutrients, macronutrients, microbiota, GALT, APC, lymphocytes, cytokines, antibodies
Citation: Tourkochristou E, Triantos C and Mouzaki A (2021) The Influence of Nutritional Factors on Immunological Outcomes. Front. Immunol. 12:665968. doi: 10.3389/fimmu.2021.665968
Received: 09 February 2021; Accepted: 05 May 2021;
Published: 31 May 2021.
Edited by:
Reinaldo B. Oria, Federal University of Ceara, BrazilReviewed by:
Michael Kogut, United States Department of Agriculture, United StatesMaria Vittoria Barone, University of Naples Federico II, Italy
Copyright © 2021 Tourkochristou, Triantos and Mouzaki. This is an open-access article distributed under the terms of the Creative Commons Attribution License (CC BY). The use, distribution or reproduction in other forums is permitted, provided the original author(s) and the copyright owner(s) are credited and that the original publication in this journal is cited, in accordance with accepted academic practice. No use, distribution or reproduction is permitted which does not comply with these terms.
*Correspondence: Athanasia Mouzaki, bW91emFraUB1cGF0cmFzLmdy