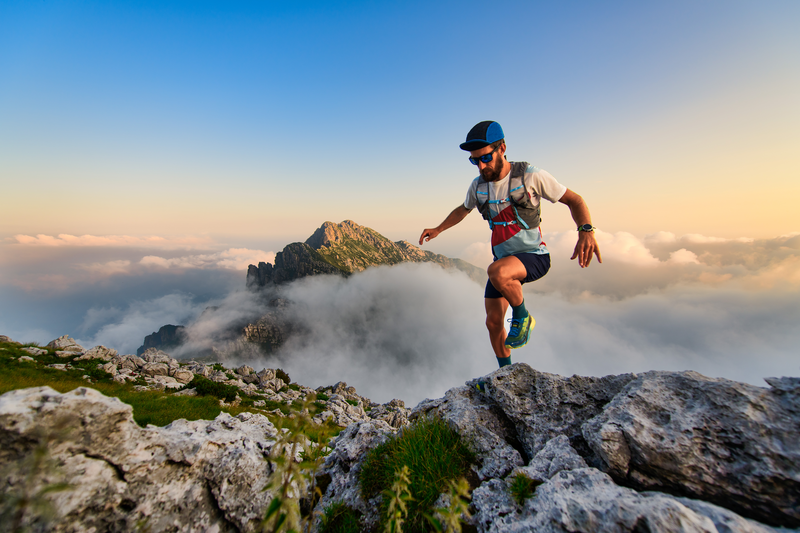
94% of researchers rate our articles as excellent or good
Learn more about the work of our research integrity team to safeguard the quality of each article we publish.
Find out more
MINI REVIEW article
Front. Immunol. , 09 April 2021
Sec. Autoimmune and Autoinflammatory Disorders
Volume 12 - 2021 | https://doi.org/10.3389/fimmu.2021.663102
Dopamine (DA) receptor, a significant G protein-coupled receptor, is classified into two families: D1-like (D1 and D5) and D2-like (D2, D3, and D4) receptor families, with further formation of homodimers, heteromers, and receptor mosaic. Increasing evidence suggests that the immune system can be affected by the nervous system and neurotransmitters, such as dopamine. Recently, the role of the DA receptor in inflammation has been widely studied, mainly focusing on NLRP3 inflammasome, NF-κB pathway, and immune cells. This article provides a brief review of the structures, functions, and signaling pathways of DA receptors and their relationships with inflammation. With detailed descriptions of their roles in Parkinson disease, inflammatory bowel disease, rheumatoid arthritis, systemic lupus erythematosus, and multiple sclerosis, this article provides a theoretical basis for drug development targeting DA receptors in inflammatory diseases.
Dopamine (DA), a member of a group of neurotransmitters called “catecholamines”, relies on the conversion of tyrosine to L-DOPA by tyrosine hydroxylase (TH). Chromaffin cells in suprarenal glands and the intestine are the main sources of plasma dopamine. Other sources of dopamine are immune cells, peripheral nervous system, and central nervous system.
Dopamine receptors (DRs) are mainly divided into D1-like (D1 and D5) and D2-like (D2, D3, and D4) receptors, which display different affinities for dopamine: D3R>D5R>D4R>D2R>D1R(Ki(nM) = 27, 228, 450, 1705, 2340, respectively) (1). As members of monoaminergic G protein-coupled receptor (GPCR) family, they not only regulate behavior, movement, and endocrine but are also important molecules connecting the nervous system and immune system. Integrating the knowledge acquired by those studies, evidences indicate that stimulation of low-affinity DRs are coupled to anti-inflammatory mechanisms, while high-affinity DRs have opposite effects (Table 1).
DRs and other GPCRs can form homodimers as well as heteromers with receptors from other superfamilies.
It is a general physical property of the class-A GPCRs to form transient homodimerization (47), which can be confirmed by the minimal single functional unit consist of D2R homodimers and a G protein, mediated by direct association among receptors (48).
Activation of the Gq-coupled D1-D2R heteromers in striatal neurons results in PLC-dependent intracellular calcium release, thus activating calmodulin-dependent protein kinase II (CaMKII)/Mutations of the methyl-CpG binding protein 2 (MeCP2)/brain-derived neurotrophic factor (BDNF) pathway (49).
D1-D3R heteromers, located in the ventral striatum, enhance D1R agonist affinity, the potency of D1R in activating adenylyl cyclase (AC), impair agonist-induced D1R internalization (20), and induce a switch from G protein-dependent to G protein-independent D1R-mediated signaling, including MAPK and AKT (50).
D2R can exist in a heterodimeric form with D4R, participating in dopamine-induced decrease of K+-induced glutamate release (51).
The C-terminal tail of the A2AR and the intracellular loop3 of the D2R form antagonistic heteromers through direct electrostatic interactions in striatopallidal GABAergic neurons and glial cells (52), resulting in a rapid switch from D2R-Gi coupling toward β-arrestin2/Akt/GSK-3 signaling (53, 54), and reducing the activity of the striatopallidal GABA/enkephalin-mediated inhibition of the excitatory glutamate thalamocortical pathway through Ca2+ and cAMP/PKA pathway whose activation involves the phosphorylation of different PKA substrates, including DARPP-32, CREB, and α-amino-3-hydroxy-5-methyl-4-isoxazole-propionic acid receptor (AMPAR) (55, 56). In addition, A2AR agonists and antagonists produce the same allosteric modulation of D2R agonist binding when individually administered, the effect of which is offset when co-administered (57).
A2AR activation reduces D3R agonist affinity and the ability of D3R to inhibit AC (58).
There exist antagonistic intramembrane A1-D1R interactions at the AC level in the dorsal, ventral striatum, and prefrontal cortex (59). A1R activation in the A1–D1R heteromers leads to an uncoupling of the D1 receptor to its Gs/olf protein, modulates D1R antagonist binding sites that cause a reduction of their affinity, and offsets D1R-induced GluA1 phosphorylation that facilitates AMPA glutamate transmission (60). In addition, A1R signaling inhibits excitatory synaptic transmission through the inhibition of glutamate release, ionotropic glutamate receptors, and neuronal excitability via inward rectifying, cAMP/PKA/DARPP-32 pathway, as well as calcium-activated potassium channels (61, 62).
H3-D1R and H3-D2R heteromers, in which H3R potentiates the D2R-induced inhibition of the indirect pathway and inhibits the D1R-induced excitation of the direct pathway in AC level, modulate DA and GABA release (63) and subsequent locomotor activation. H3-D1R heteromers also couple to Gi to direct histaminergic input towards β-arrestin/MAPK pathway within the GABAergic neurons (64) with an increased phosphorylation of rpS6 and transient phosphorylation of GSK3β (65), and affect both rapid receptors signaling like Ca2+ mobilization and longer cell signaling pathways like p38, involving neuronal cell death (66). While H3-D2R heteromers regulate Akt-GSK3β, producing a more slowly developing dephosphorylation (65).
Heteromers, consist of D1R and NMDAR, increase the phosphorylation of NR1 and NR2B subunits, surface insertion of NR2B, and NMDA-triggered Ca2+ upregulation via cAMP/PKA/RACK1-mediated Fyn activation (67, 68), and PKC/CAKβ/Src signaling (69), thus enhancing NMDAR-mediated currents. While D1R, coupled to NR2A subunit, inhibits NMDA receptor-gated currents through a reduction in cell surface receptor numbers (70, 71). In addition, NMDA-D1R heteromers participate in the attenuation of NMDA receptor-mediated excitotoxicity via PI-3K/Akt/GSK3β pathway instead of modulating Ca2+ influx (70, 72). Besides, D1R-mediated cAMP/PKA/DARPP-32 signaling, Ca2+ pathway, and tyrosine phosphorylation of NR2B subunit allow for the activation of NMDA-mediated ERK, a signal integrator for dopamine and glutamate neurotransmission (73).
NMDAR abolishes D1R internalization and enhances D1R-mediated cAMP accumulation via a SNARE-dependent mechanism (74, 75).
In glutamate synapses, NMDA-D2R heteromers, in which ICL3 of D2R interacts with the NR2B subunit, interfere with the binding of Ca2+/CaMKII to NR2B, reduce NR2B phosphorylation, and inhibit NMDA receptor-mediated currents (76).
CB1-DR heteromers in the striatum (32) modulate D1R and D2R function in an opposite fashion. Stimulation of either CB1 or D2R results in Gαi signaling, while simultaneous co-activation of both receptors switches coupling from Gαi to Gαs proteins (77).
D3R-nAChR heteromers in DA neurons are the molecular unit involved in the induction of neurotrophic effects, neuroprotection, and inhibition of α-syn accumulation (78).
A2AR and mGlu5 act synergistically to counteract the D2R signaling in striatopallidal neurons, reducing mGlu5 desensitization (79), and exciting the striatopallidal GABA neurons with firing and altered gene expression (80, 81).
A2A-CB1-D2 RM in striatopallidal neurons selectively couples to the mitogen-activated protein kinase pathway (82). The binding of A2AR and CB1R agonists decreases D2R agonist affinity (32).
NLRP3 inflammasome is a group of intracellular multi-protein complexes, participating in the pathogenesis of a variety of diseases, including inflammatory bowel disease, gout, atherosclerosis, and Alzheimer’s disease (83, 84). It was found that DA can inhibit lipopolysaccharide (LPS)-induced activation of NLRP3 inflammasome and subsequent production of caspase-1 and IL-1β in a time- and dose-dependent manner through G protein pathway and β-arrestin–dependent pathway (Figure 1).
Figure 1 Roles that dopamine receptors play in regulating inflammasome formation as well as NF-κB pathway. (A) Elevated cAMP, induced by D1 like receptors, directly binds to NLRP3, triggering the ubiquitination of NLRP3 NACHT and LRR domains with K48 ubiquitin chains by MARCH7, targeting NLRP3 to autophagy-mediated degradation. (B) β-arrestin2 recruited by D2R binds to NLRP3 to repress its activation. (C) D5R directly recruits TRAF6, ARRB2, TAK1, IKKs, and PP2A to form a multiprotein complex, impairing TRAF6-mediated activation of NF-κB. (D) Activated cAMP/PKA/CREB signaling inhibits p65/RelA and p50 activation as well as their DNA binding by delaying IKB-α degradation and competing for the KIX binding site on CREB binding protein. (E) D2R signaling increases the level of CRYAB, which combines with NF-κB p65, thus negatively regulating the NF-κB signaling pathway. (F) D2R activation increases the expression of PPP2R2C, leading to PP2A and Akt dephosphorylation, and to the inhibition of the IKKα/IκBα/NF-κB signaling pathway.
D1-like DRs are coupled to the stimulatory G-subunit, Gαs, whereas D2-like DRs, are coupled to the inhibitory G-subunit, Gαi. D1-like DRs stimulate the activity of AC by activating Gαs/olf, thus promoting the production of cAMP, which directly binds to NLRP3, triggering the ubiquitination of NLRP3 NACHT and LRR domains with K48 ubiquitin chains by the E3 ubiquitin ligase membrane associated ring-CH-type finger 7, targeting NLRP3 to autophagy-mediated degradation (2, 85).
β-arrestin is involved in the negative feedback regulation of inflammatory process in sepsis, asthma, rheumatoid arthritis, and other inflammatory diseases (86). β-arrestin2 recruited by D2R functions as the downstream protein of GPR120 and GPR40 to repress inflammasome activation via binding to NLRP3 in a concentration-dependent manner (87, 88).
An inactive form of NF-κB consists of a three-subunit complex: two DNA-binding subunits of p50 and p65/RelA, and an inhibitory subunit called IκB (Figure 1). Activation of NF-κB requires the phosphorylation and degradation of IκBα by ubiquitin–proteosome pathway, contributing to translocation of NF-κB dimer into the nucleus and the transcription of inflammatory genes, including cyclooxygenase-2, inducible nitric oxide synthase, tumor necrosis factor-α (TNF-α), and interleukin-6 (IL-6) (89).
D1R participates in the regulation of inflammation through cAMP/PKA/NF-κB pathway. Elevated cAMP activates PKA and phosphorylates cAMP-response element binding protein (CREB), inhibiting p65/RelA and p50 activation as well as their DNA binding ability by delaying IKB-α degradation and competing for the KIX binding site on CREB binding protein (90).
D5R inhibits NF-κB signaling by mediating the negative regulation of ARRB2/PP2A on TRAF6-dependent signaling. A study found that D5R, via the EFD and IYX(X)I/L motifs in its CT and IC3 loop, respectively, can directly recruit TRAF6 and its negative regulator ARRB2, as well as downstream signaling proteins, such as TAK1, IKKs, and PP2A, to form a multiprotein complex, which impairs TRAF6-mediated activation of NF-κB (91).
D2R negatively regulates the NF-κB pathway. First, the pathway downstream D2R activation leads, via increasing the expression of PPP2R2C, to PP2A and Akt dephosphorylation, and the inhibition of the IKKα/IκBα/NF-κB pathway as well as the expression of NLRP3 mRNA (2, 92). Second, D2R signaling increases the level of αB-crystallin, also called CRYAB, which is known as a small heat-shock protein with neuroprotective and anti-inflammatory activities (93). D2R agonists were found to promote a direct combination between CRYAB and NF-κB p65 (92) and enhance the interaction between CRYAB and STAT3, blocking its DNA binding activity (94).
Dopamine dynamically regulates the immune response of T cells through DRs, depending on the concentrations of dopamine, the activation states of T cells, and the types and subtypes of T cells. Dopamine concentrations can be divided into three gradients: 10 nM, 1 μM, and 0.1 to 1 mM. Dopamine’s optimal concentration for inducing a physiological and specific effect on resting T cells turns out to be low: 10 nM, in which dopamine activates normal resting/primeval effector cells or improves the continuous important cell function, and inhibits activated T cells. Dopamine at a concentration range of 0.1 to 10 μM still affects T cells, but the potency and specificity are lower. At a very high concentration of 0.1 to 1 mM, dopamine’s effect is non-specific and even toxic (95).
Tregs are inhibitory T cells, mainly inhibiting the activity of Teffs. D1-like DRs on the surface of Tregs reduce their inhibitory activity, as well as the production of IL-10 and TGF–β (96), the effects of which are significantly attenuated in activated Tregs (97). Instead, activation of D1-like DRs in Teffs does not lead to self-inhibition.
According to cytokines produced during T cell activation, naive CD4+ T cells undergo differentiation into specific effector phenotypes, including Th1, Th2, and Th17. IFN-γ and IL-12 induce Th1 differentiation, while IL-4 is the primary inducer of differentiation into Th2, and IL-6 and TGF-β together induce Th17 phenotype (98). In activated CD4+ T cells, D3R signaling, preferentially activated at lower dopamine concentrations, enhances the production of IFN-γ (99) and reduces the synthesis of IL-4 and IL-10 and the expression of SOCS5 (100), a side-regulator of Th2 differentiation. Besides, D3R stimulating reduces cAMP level and extracellular signal-regulated kinase 1/2 (ERK1/2) phosphorylation, resulting in enhanced activation of CD4+ T cells and Th1 differentiation (101).
Dendritic cells (DCs) have been shown to synthesize and store dopamine, which is released to the original CD4 + T cells during DC-T cell interaction, thus affecting the differentiation of CD4+ T cells. The expression of D1R and D5R is more than that of D2R and D3R on the DC surface. D1-like DRs-mediated increase in cAMP promotes TH phosphorylation, thus boosting the synthesis of dopamine and Th2 differentiation, while D2-like DRs play an opposite role (95).
However, D5R and D2R have different effects. D5R signaling significantly enhances the production of LPS-induced IL-23 and IL-12 (44), inducing Th1/Th17 differentiation and the activity of B cell-activating transcription factor, increasing the expression of Th17 transcription factors, like ROR-γt (102). Besides, stimulation of D2R induces a significant human monocyte-derived DC-mediated Th2 differentiation and suppresses the secretion of inflammatory cytokines (103).
D2-like DRs participate in a cAMP-independent pathway to regulate macrophage phagocytic function, thus reducing the secretion of IL-2, IL-4, and IFN-γ (104), the effect of which is concentration-dependent. Studies demonstrated that D2-like DRs decrease the production of TNF-α, IL-6, and CCL2 at 10−8 M concentration (105), while increase the production of IL-6 significantly at 2 and 20 × 10−6 M (106) concentration. Conversely, Gomez et al. proposed that another mechanism is to increase the expression of the Fcγ receptor, thus increasing the phagocytosis of macrophages (107) (Figure 2).
Figure 2 Roles that dopamine receptors play in macrophages. (A)The activation of D2-like DRs can regulate the phagocytic activity of macrophages through β-arrestin2 pathway, and reduce the secretion of IL-2, IL-4, and IFN-γ. (B) LPS increases TNF - α production via TLRs and mediates activation of STAT3, which can be inhibited by D5R signaling. (C) Dopamine reduces the production of anti-inflammatory factor IL-10 through D5R. (D) D1R/cAMP/C/EBPϵ signaling increases IL-10 production, thus inhibiting LPS-mediated production of TNF-α.
A study showed that D1R signaling blocks the function of LPS-activated macrophages and monocytes and production of inflammatory cytokines (108). Also, the elevated cAMP can indirectly activate CCAAT/enhancer-binding protein, together with which CREB/activating transcription factors are believed to be the major cause of IL-10 production by monocytes (109). However, D5R in monocytes obtained from MS patients involves the reduction of STAT3 activation, a transcription factor that limits the production of IL-12 and IL-23 (110). A study in HIV patients demonstrated that dopamine promotes the inflammatory phenotype of macrophages through D5R at physiological concentration and reduces the production of IL-10 (105).
Parkinson disease (PD) is the second most prevalent central nervous system degenerative disease, characterized by slow and progressive loss of midbrain substantia nigra dopamine with the accumulation of α-synuclein in Lewy bodies and neuritis (111).
DRs expressed on astrocytes and microglia have been confirmed to participate in the pathogenesis of chronic nervous system inflammatory diseases. Increases in D1R and D4R, and decreases in D3R, D5R mRNA expression are showed in a study analyzing the mRNA expression of all five DRs in BV2 microglial cells in response to LPS (112). It is noteworthy that these anti-inflammatory effects exerted by dopaminergic signaling in astrocytes are mediated by D1R and D2R, while D3R mediates the pro-inflammatory effects (8).
D1R signaling participates in the negative regulation of the activation of NLRP3 inflammasome, which can be assembled upon stimulation with accumulated endogenous metabolites such as fibrillar amyloid β and 25-hydroxycholesterol (113), the subsequent secretion of caspase-1 and IL-1β contributes to the destruction of dopaminergic neurons (114). A study reported that caspase-1 can process α-synuclein into a truncated, aggregation-prone form that facilitates its aggregation (115), thus participating in activation of NF-κB and expression of Toll-like receptors (TLRs).
Microglia can be divided into M1 type and M2 type phenotypes. M2 microglia promotes the release of anti-inflammatory factors, such as TGF-β and IL-10, while M1 microglia plays an opposite role (116). Studies have shown that D1R signaling inhibits the activation of M1 phenotype by cAMP/PKA/NF-κB pathway. Besides, cAMP, along with IL-4, activates CCAAT/enhancer-binding protein (C/EBP) to induce the expression of M2 regulatory genes (such as arginase-1), thus reducing the expression of M1-related inflammatory factors and increasing the expression of M2-related anti-inflammatory mediators (such as FIZZ1) (117).
Several lines of evidences suggest that D2R signaling alleviates neuroinflammatory injury by CRYAB/STAT3 pathway, β-arrestin2/NLRP3 pathway, and its regulation of macrophage phagocytic activity (4, 5, 94, 118). A2AR inhibition of D2R signaling regulates striatal glutamatergic transmission dysfunction via increasing the extracellular glutamate levels (119) and promotes microglia-mediated neuroinflammation (120). Besides, D2R modulates astroglial and microglial activity via decreasing the microglial AT1/AT2 ratio, thus inhibiting AT1/NADPH-oxidase/superoxide axis, based on AT1-D2R heteromers (6).
Genetic deficiency of D3R, attenuated neuroinflammation and subsequent neurodegeneration on a murine model of PD induced by acute intoxication with 1-methyl-4-phenyl-1,2,3,6-tetrahydropyridine (121), related to the limited basal production of Fizz1 (8) and the acquisition of M1 phenotype. Besides, the high levels of IFN-γ and TNF-α, secreted by D3R signaling-induced Th1 and Th17 differentiation, lead to M1 phenotype (122), confirmed by a study that compared with the control group, PD patients have increased Th1 cells and Th17 cells but decreased Tregs (123).
However, experimental results showed decreased D3R expression on CD4+ T cells in the peripheral circulation of PD patients, which might be due to a compensatory mechanism attempting to reduce the inflammatory effect (124, 125). Another plausible explanation was that CD4+ T cells with high D3R expression were specific for α-synuclein, thus these cells could only be detected at the site of inflammatory infiltration, instead of the peripheral circulation (124).
D3R expressed on striatal neurons can raise dopamine concentration, decrease α-Syn accumulation, enhance secretion of BDNF, ameliorate neuroinflammation, alleviate oxidative stress, and promote neurogenesis in the nigrostriatal pathway.
D3R increases the content of DA in the synaptic cleft by impeding DAT’s reuptake of DA, inhibiting MAO to reduce DA decomposition, and promoting the release of DA by VMAT2. Further, D3R activation hinders the phosphorylation of α-Syn to inhibit fibril formation (126). Besides, D3R activation enhances autophagy-dependent degradation of toxic fibrils by modulating autophagy constituent proteins LC3 and autophagy-related protein Beclin1 (15). It was found that D1, D2, and possible D1-D2 receptor heteromers can activate BDNF receptors in striatal neurons (127). Also, D3R can jointly protect striatal neurons through its bi-directional regulation with BDNF, a high level of which may ameliorate symptoms in PD patients (128). Experiments have shown that D3R agonists can normalize glutathione (GSH) and GSH peroxidase levels in animal models of PD (17), thereby reducing ROS-induced damage, however, some studies showed no association.
Evidences for the existence of DR heteromers in the striatum (see Structure) provide novel targets in treating PD and other brain disorders. This section is a supplement to clinical implications of heteromers on treatment response and prognosis of PD.
Striatal D1-D3R heteromers are closely correlated with age of onset, PD stage, dopamine responsiveness, and survival time (129). Besides, the expression of D3R induced by long-term L-DOPA treatment aggravates D1R oversensitivity and is correlated with the severity of LID, via activating D1R/Shp-2/Erk1/2 pathway (130).
Chronic administration of the histamine H3 receptor agonist immepip decreases L-Dopa-induced dyskinesias (28), while a combination of D2 agonists and inhibitors of endocannabinoid degradation improves parkinsonian motor deficits (131).
NMDAR antagonist MK-801 aggravates D1R-induced dyskinesias, while effectively reduces D2R-induced dyskinesias, the degree of which is of the same magnitude as the reduction of L-DOPA-induced dyskinesias (31).
There is an increase in the therapeutic index and locomotor improvement of L-DOPA with adenosine A2AR antagonists, like istradefylline (25, 132) and tozadenant (26), and/or D2R agonists, based on the existence of A2A–D2R heteromers (24, 133), which function also as a biomarker to monitor PD (134). Besides, adenosine A1 receptor stimulation reduces D1 receptor-mediated GABAergic transmission from striato-nigral terminals and attenuates L-DOPA-induced dyskinesia in dopamine-denervated mice (27).
In addition, simultaneous blockade of both mGlu5 and A2AR in A2A–mGlu5-D2R RM increases their efficacy in reversing parkinsonian deficits (29). While a study showed that A2A-CB1-D2 RM expression attenuates in L-Dopa-treated PD monkeys with abolished negative cross-talk (32).
DRs are widely expressed in the hippocampus. D1R can upregulate Wnt/β-catenin signaling in the hippocampus of PD rats, leading to the enhanced NSC proliferation, long-term survival, and neuronal differentiation (33). Besides, D2R-dependent cross-talk modulates Wnt3a expression via Wnt/β-catenin signaling and an evolutionarily conserved TCF/LEF site within the WNT3A promoter, thus modulating cell proliferation (135).
Inflammatory bowel disease (IBD) is a group of chronic gastrointestinal inflammatory diseases including Crohn’s disease (CD) and ulcerative colitis (UC). Dopamine in IBD can be produced from enteric nervous system, the intestinal epithelial layer, and certain immune cells. Interestingly, inflamed mucus from IBD patients show a significant reduction of dopamine, mainly related to reduced dopamine uptake and the number of sympathetic fibers interacting with the intestinal wall (136).
Reduced intestinal dopamine levels [≈140 pg/ml in healthy individuals;≈45 pg/ml in CD and UC patients (137)] play a pro-inflammatory role by activating D3R and D5R. On one hand, D3R signaling depresses the immunosuppressive potency of Tregs, attenuates IL-10 production, and limits the acquisition of gut-tropism (34). On the other hand, Th1/Th17 differentiation, induced by increased D3R expression on intestinal CD4+ T cells, the induction of ROR-γt expression by D5R signaling in dendritic cells, and the increased IL-23 and IL-12 resulting from D5R signaling, contributes to the persistence of chronic inflammation (138).
Conversely, high concentrations of dopamine in the intestine of healthy people can stimulate D2R, promoting the production of IL-10, inhibiting intestinal motility and ulcer development (139), as well as playing a role of the negative regulator of VEGF–VEGFR2-mediated increase in vascular permeability (35, 140, 141), thus controlling the development of IBD.
Rheumatoid arthritis (RA) is a systemic inflammatory autoimmune disease characterized by persistent inflammation of the joint synovium (142). Dysregulated immune signals, such as dopamine, control bone remodeling via affecting osteoclasts differentiation or the secretion of pro-inflammatory cytokines.
Dopamine released by DCs contributes to the Th17/Treg imbalance via the IL-6-Th17 axis and causes aggravation of synovial inflammation. A study showed that D2-like DRs agonist improves Th17/Treg imbalance by downregulating the expression of Th17-related pro-inflammatory cytokines but upregulating Treg-related anti-inflammatory cytokine expression (143), the effect of which can be suppressed by selective D2-like DRs antagonist.
D3R on bone marrow-derived mast cells may negatively regulate LPS-induced TLR4 expression and its downstream production of TNF and other cytokines (38), thus effectively inhibiting the production of ROS and reducing joint inflammation in RA patients. With the increase in RA severity degree, D3R-positive MCs in the synovial fluid are gradually reduced, led by ROS production, reduced antioxidant capacity, reduced cell membrane stability, and increased sensitivity of membrane components to a damaging agent, which are negatively correlated with the level of MDA and protein carbonylation (144).
B cells have unique bone action properties. D2R expression on B cells in RA patients is negatively correlated with disease activity (145), concerning the descending TNF-α level.
Synovial fibroblasts (SF) are resident cells of the intimal lining layer of synovial tissue. SFs have an intact endogenous dopamine system in which D1R is overexpressed, promoting the migration of RASF cells, leading to a strong increase of SF migration in young patients (146), and decreased release of IL-6 and IL-8. However, some experiments demonstrated that the inhibitory effect on IL-8 release is not significant (146). These findings suggest that DRs expressed on synovial fibroblasts in RA patients may mainly participate in cell migration rather than inflammatory processes.
Osteoclasts are tissue-specific macrophage polykaryons that arise from the differentiation of monocyte/macrophage precursor cells at or near the bone surface, whose maturation and activation are mainly related to the activation of the RANK signaling (147). It was found that dopamine significantly inhibits the formation of osteoclast in a dose-dependent manner, mainly related to the restraint of RANKL-mediated expression of c-Fos and NFATc1 in the preosteoclast by D2R-induced cAMP/PKA/CREB pathway (41).
Systemic lupus erythematosus (SLE) is an autoimmune disease characterized by the involvement of kidneys and brain, with under-expressed D2R and overexpressed D4R on peripheral blood mononuclear cells (PBMCs) (148).
D2R promotes the activation and differentiation of CD4+T cells by regulating the polarization of Treg (149). D2R agonist, such as bromocriptine, suppresses PRL secretion to decrease HPRL and to normalize the dopaminergic system in SLE, through the pertussis toxin (PTX)-sensitive Gi/o and PTX-insensitive Gz proteins, as well as a G protein-independent, β-arrestin/glycogen synthase kinase-3-dependent pathway (150, 151).
Studies have shown that the stimulation of D4R on human T cells promotes quiescence (152), and overexpression of D4R in SLE patients may act as a compensatory mechanism to inhibit uncontrolled T cell proliferation, an important link in the pathogenesis of SLE.
Multiple sclerosis (MS) is an inflammatory autoimmune disorder of central nervous system (CNS), correlated with Tregs dysfunction, enhanced Th1 and Th17 responses, and autoreactive B cell overactivity.
There is a decreased expression of D5R in PBMCs in untreated MS patients (153), and an increase in patients treated with IFN-β. Th cell subsets involved in the pathogenesis of MS include Th1 and Th17 lymphocytes (154). D5R expressed on DCs plays a role in MS by regulating Th1 and Th17 differentiation, γδT cell functions, and GM-CSF-producing CD4+T cells via STAT3/NF-κB/IL-6/12/23 pathway, and is correlated with disease severity (155).
D5R, which functions as a negative immunomodulator of TH and Tregs’ inhibitory activity, is up-regulated in Tregs from untreated MS patients, resulting in neuronal damage and neuroinflammation (46). Besides, D3R expression in Tregs is unaltered in untreated MS patients but significantly decreases after IFN-β treatment. A recent study showed that increased D3R and D5R mRNA expression in Tregs may be associated with the risk of MS at twelve months (156).
Dopamine receptor, a significant G protein-coupled receptor, is classified into two families: D1-like DRs and D2-like DRs, with further formation of homodimers, heteromers, and receptor mosaic. Dysfunction of the systemic or local dopaminergic system during inflammation has been found in animal models and patients with various inflammatory diseases, such as Parkinson disease, inflammatory bowel disease, rheumatoid arthritis, systemic lupus erythematosus, and multiple sclerosis, indicating an important role that DRs play in inflammatory diseases. As described in this review, DRs regulate the release of inflammatory mediators and subsequent pathological processes by interacting with inflammasomes, inflammatory pathways, and immune cells, depending on different immune cells, receptor subtypes, and disease models. In conclusion, a comprehensive understanding of the relationship between DRs and inflammation will provide new insights into the inhibition of inflammatory responses by targeting dopamine receptors and ultimately contribute to the development of drugs to treat inflammatory diseases.
YFF and YL contributed to the conceptual design, writing, editing, and generation of figures for this manuscript. All authors contributed to the article and approved the submitted version.
The authors acknowledge the financial support from Nanjing Medical University (Project approval No.81872541).
The authors declare that the research was conducted in the absence of any commercial or financial relationships that could be construed as a potential conflict of interest.
1. Van Tol HH, Bunzow JR, Guan HC, Sunahara RK, Seeman P, Niznik HB, et al. Cloning of the gene for a human dopamine D4 receptor with high affinity for the antipsychotic clozapine. Nature (1991) 350(6319):610–4. doi: 10.1038/350610a0
2. Yan YQ, Jiang W, Liu L, Wang XQ, Ding C, Tian ZG, et al. Dopamine Controls Systemic Inflammation through Inhibition of NLRP3 Inflammasome. Cell (2015) 160(1-2):62–73. doi: 10.1016/j.cell.2014.11.047
3. Shao W, Zhang SZ, Tang M, Zhang XH, Zhou Z, Yin YQ, et al. Suppression of neuroinflammation by astrocytic dopamine D2 receptors via alpha B-crystallin. Nature (2013) 494(7435):90–4. doi: 10.1038/nature11748
4. Zhu J, Hu Z, Han X, Wang D, Jiang Q, Ding J, et al. Dopamine D2 receptor restricts astrocytic NLRP3 inflammasome activation via enhancing the interaction of beta-arrestin2 and NLRP3. Cell Death Differ (2018) 25(11):2037–49. doi: 10.1038/s41418-018-0127-2
5. Espinosa-Oliva AM, de Pablos RM, Sarmiento M, Villaran RF, Carrillo-Jimenez A, Santiago M, et al. Role of dopamine in the recruitment of immune cells to the nigro-striatal dopaminergic structures. Neurotoxicology (2014) 41:89–101. doi: 10.1016/j.neuro.2014.01.006
6. Dominguez-Meijide A, Rodriguez-Perez AI, Diaz-Ruiz C, Guerra MJ, Labandeira-Garcia JL. Dopamine modulates astroglial and microglial activity via glial renin-angiotensin system in cultures. Brain Behav Immun (2017) 62:277–90. doi: 10.1016/j.bbi.2017.02.013
7. Cervetto C, Venturini A, Passalacqua M, Guidolin D, Genedani S, Fuxe K, et al. A2A-D2 receptor-receptor interaction modulates gliotransmitter release from striatal astrocyte processes. J Neurochem (2017) 140(2):268–79. doi: 10.1111/jnc.13885
8. Montoya A, Elgueta D, Campos J, Chovar O, Falcon P, Matus S, et al. Dopamine receptor D3 signalling in astrocytes promotes neuroinflammation. J Neuroinflamm (2019) 16(1):258. doi: 10.1186/s12974-019-1652-8
9. Barcia C, Ros CM, Annese V, Gomez A, Ros-Bernal F, Aguado-Llera D, et al. IFN-gamma signaling, with the synergistic contribution of TNF-alpha, mediates cell specific microglial and astroglial activation in experimental models of Parkinson’s disease. Cell Death Dis (2012) 3:e379. doi: 10.1038/cddis.2012.123
10. Castro-Hernandez J, Afonso-Oramas D, Cruz-Muros I, Salas-Hernandez J, Barroso-Chinea P, Moratalla R, et al. Prolonged treatment with pramipexole promotes physical interaction of striatal dopamine D3 autoreceptors with dopamine transporters to reduce dopamine uptake. Neurobiol Dis (2015) 74:325–35. doi: 10.1016/j.nbd.2014.12.007
11. Benitez A, Edens H, Fishman J, Moran K, Asgharnejad M. Rotigotine transdermal system: developing continuous dopaminergic delivery to treat Parkinson’s disease and restless legs syndrome. Ann N Y Acad Sci (2014) 1329:45–66. doi: 10.1111/nyas.12508
12. Elmer LW, Surmann E, Boroojerdi B, Jankovic J. Long-term safety and tolerability of rotigotine transdermal system in patients with early-stage idiopathic Parkinson’s disease: a prospective, open-label extension study. Parkinsonism Relat Disord (2012) 18(5):488–93. doi: 10.1016/j.parkreldis.2012.01.008
13. Zhang QS, Heng Y, Mou Z, Huang JY, Yuan YH, Chen NH. Reassessment of subacute MPTP-treated mice as animal model of Parkinson’s disease. Acta Pharmacol Sin (2017) 38(10):1317–28. doi: 10.1038/aps.2017.49
14. Luo D, Sharma H, Yedlapudi D, Antonio T, Reith MEA, Dutta AK. Novel multifunctional dopamine D2/D3 receptors agonists with potential neuroprotection and anti-alpha synuclein protein aggregation properties. Bioorg Med Chem (2016) 24(21):5088–102. doi: 10.1016/j.bmc.2016.08.021
15. Wang JD, Cao YL, Li Q, Yang YP, Jin M, Chen D, et al. A pivotal role of FOS-mediated BECN1/Beclin 1 upregulation in dopamine D2 and D3 receptor agonist-induced autophagy activation. Autophagy (2015) 11(11):2057–73. doi: 10.1080/15548627.2015.1100930
16. Saylor AJ, McGinty JF. An intrastriatal brain-derived neurotrophic factor infusion restores striatal gene expression in Bdnf heterozygous mice. Brain Struct Funct (2010) 215(2):97–104. doi: 10.1007/s00429-010-0282-9
17. Lieberknecht V, Junqueira SC, Cunha MP, Barbosa TA, de Souza LF, Coelho IS, et al. Pramipexole, a Dopamine D2/D3 Receptor-Preferring Agonist, Prevents Experimental Autoimmune Encephalomyelitis Development in Mice. Mol Neurobiol (2017) 54(2):1033–45. doi: 10.1007/s12035-016-9717-5
18. Salvi R, Steigleder T, Schlachetzki JC, Waldmann E, Schwab S, Winner B, et al. Distinct Effects of Chronic Dopaminergic Stimulation on Hippocampal Neurogenesis and Striatal Doublecortin Expression in Adult Mice. Front Neurosci (2016) 10:77. doi: 10.3389/fnins.2016.00077
19. Merlo S, Canonico PL, Sortino MA. Distinct effects of pramipexole on the proliferation of adult mouse sub-ventricular zone-derived cells and the appearance of a neuronal phenotype. Neuropharmacology (2011) 60(6):892–900. doi: 10.1016/j.neuropharm.2011.01.026
20. Marcellino D, Ferre S, Casado V, Cortes A, Le Foll B, Mazzola C, et al. Identification of dopamine D1-D3 receptor heteromers. Indications for a role of synergistic D1-D3 receptor interactions in the striatum. J Biol Chem (2008) 283(38):26016–25. doi: 10.1074/jbc.M710349200
21. Fiorentini C, Savoia P, Savoldi D, Barbon A, Missale C. Persistent activation of the D1R/Shp-2/Erk1/2 pathway in l-DOPA-induced dyskinesia in the 6-hydroxy-dopamine rat model of Parkinson’s disease. Neurobiol Dis (2013) 54:339–48. doi: 10.1016/j.nbd.2013.01.005
22. Wang T, Duan SJ, Wang SY, Lu Y, Zhu Q, Wang LJ, et al. Coadministration of hydroxysafflor yellow A with levodopa attenuates the dyskinesia. Physiol Behav (2015) 147:193–7. doi: 10.1016/j.physbeh.2015.04.038
23. Utsumi H, Okuma Y, Kano O, Suzuki Y, Iijima M, Tomimitsu H, et al. Evaluation of the efficacy of pramipexole for treating levodopa-induced dyskinesia in patients with Parkinson’s disease. Intern Med (2013) 52(3):325–32. doi: 10.2169/internalmedicine.52.8333
24. Fuxe K, Marcellino D, Genedani S, Agnati L. Adenosine A2A receptors, dopamine D2 receptors and their interactions in Parkinson’s disease. Mov Disord (2007) 22:1990–2017. doi: 10.1002/mds.21440
25. Pourcher E, Fernandez HH, Stacy M, Mori A, Ballerini R, Chaikin P. Istradefylline for Parkinson’s disease patients experiencing motor fluctuations: results of the KW-6002-US-018 study. Parkinsonism Relat Disord (2012) 18(2):178–84. doi: 10.1016/j.parkreldis.2011.09.023
26. Hauser RA, Olanow CW, Kieburtz KD, Pourcher E, Docu-Axelerad A, Lew M, et al. Tozadenant (SYN115) in patients with Parkinson’s disease who have motor fluctuations on levodopa: a phase 2b, double-blind, randomised trial. Lancet Neurol (2014) 13(8):767–76. doi: 10.1016/S1474-4422(14)70148-6
27. Mango D, Bonito-Oliva A, Ledonne A, Cappellacci L, Petrelli R, Nistico R, et al. Adenosine A1 receptor stimulation reduces D1 receptor-mediated GABAergic transmission from striato-nigral terminals and attenuates l-DOPA-induced dyskinesia in dopamine-denervated mice. Exp Neurol (2014) 261:733–43. doi: 10.1016/j.expneurol.2014.08.022
28. Avila-Luna A, Rios C, Galvez-Rosas A, Montes S, Arias-Montano JA, Bueno-Nava A. Chronic administration of the histamine H3 receptor agonist immepip decreases L-Dopa-induced dyskinesias in 6-hydroxydopamine-lesioned rats. Psychopharmacol (Berl) (2019) 236(6):1937–48. doi: 10.1007/s00213-019-5182-y
29. Coccurello R, Breysse N, Amalric M. Simultaneous blockade of adenosine A2A and metabotropic glutamate mGlu5 receptors increase their efficacy in reversing Parkinsonian deficits in rats. Neuropsychopharmacology (2004) 29(8):1451–61. doi: 10.1038/sj.npp.1300444
30. Ferrada C, Ferré S, Casadó V, Cortés A, Franco R. Interactions between histamine H3 and dopamine D2 receptors and the implications for striatal function. Neuropharmacology (2008) 55(2):190–7. doi: 10.1016/j.neuropharm.2008.05.008
31. Flores AJ, Bartlett MJ, So LY, Laude ND, Parent KL, Heien ML, et al. Differential effects of the NMDA receptor antagonist MK-801 on dopamine receptor D1- and D2-induced abnormal involuntary movements in a preclinical model. Neurosci Lett (2014) 564:48–52. doi: 10.1016/j.neulet.2014.02.004
32. Bonaventura J, Rico AJ, Moreno E, Sierra S, Sanchez M, Luquin N, et al. L-DOPA-treatment in primates disrupts the expression of A(2A) adenosine-CB(1) cannabinoid-D(2) dopamine receptor heteromers in the caudate nucleus. Neuropharmacology (2014) 79:90–100. doi: 10.1016/j.neuropharm.2013.10.036
33. Mishra A, Singh S, Tiwari V, Parul, Shukla S. Dopamine D1 receptor activation improves adult hippocampal neurogenesis and exerts anxiolytic and antidepressant-like effect via activation of Wnt/beta-catenin pathways in rat model of Parkinson’s disease. Neurochem Int (2019) 122:170–86. doi: 10.1016/j.neuint.2018.11.020
34. Ugalde V, Contreras F, Prado C, Chovar O, Espinoza A, Pacheco R. Dopaminergic signalling limits suppressive activity and gut homing of regulatory T cells upon intestinal inflammation. Mucosal Immunol (2020) 1–15. doi: 10.1038/s41385-020-00354-7
35. Tolstanova G, Deng X, Ahluwalia A, Paunovic B, Prysiazhniuk A, Ostapchenko L, et al. Role of Dopamine and D2 Dopamine Receptor in the Pathogenesis of Inflammatory Bowel Disease. Dig Dis Sci (2015) 60(10):2963–75. doi: 10.1007/s10620-015-3698-5
36. Nakano K, Yamaoka K, Hanami K, Saito K, Sasaguri Y, Yanagihara N, et al. Dopamine induces IL-6-dependent IL-17 production via D1-like receptor on CD4 naive T cells and D1-like receptor antagonist SCH-23390 inhibits cartilage destruction in a human rheumatoid arthritis/SCID mouse chimera model. J Immunol (2011) 186(6):3745–52. doi: 10.4049/jimmunol.1002475
37. Lu JH, Liu YQ, Deng QW, Peng YP, Qiu YH. Dopamine D2 Receptor Is Involved in Alleviation of Type II Collagen-Induced Arthritis in Mice. BioMed Res Int (2015) 2015:1–9. doi: 10.1155/2015/496759
38. Xue L, Geng Y, Li M, Jin YF, Ren HX, Li X, et al. The effects of D3R on TLR4 signaling involved in the regulation of METH-mediated mast cells activation. Int Immunopharmacol (2016) 36:187–98. doi: 10.1016/j.intimp.2016.04.030
39. Capellino S, Cosentino M, Luini A, Bombelli R, Lowin T, Cutolo M, et al. Increased Expression of Dopamine Receptors in Synovial Fibroblasts From Patients With Rheumatoid Arthritis. Arthritis Rheumatol (2014) 66(10):2685–93. doi: 10.1002/art.38746
40. van Nie L, Salinas-Tejedor L, Dychus N, Fasbender F, Hulser ML, Cutolo M, et al. Dopamine induces in vitro migration of synovial fibroblast from patients with rheumatoid arthritis. Sci Rep (2020) 10(1):1–13. doi: 10.1038/s41598-020-68836-z
41. Hanami K, Nakano K, Saito K, Okada Y, Yamaoka K, Kubo S, et al. Dopamine D2-like receptor signaling suppresses human osteoclastogenesis. Bone (2013) 56(1):1–8. doi: 10.1016/j.bone.2013.04.019
42. Saha S, Tieng A, Pepeljugoski KP, Zandamn-Goddard G, Peeva E. Prolactin, systemic lupus erythematosus, and autoreactive B cells: lessons learnt from murine models. Clin Rev Allergy Immunol (2011) 40(1):8–15. doi: 10.1007/s12016-009-8182-6
43. McMurray RW, Weidensaul D, Allen SH, Walker SE. Efficacy of bromocriptine in an open label therapeutic trial for systemic lupus erythematosus. J Rheumatol (1995) 22(11):2084–91.
44. Prado C, Contreras F, Gonzalez H, Diaz P, Elgueta D, Barrientos M, et al. Stimulation of dopamine receptor D5 expressed on dendritic cells potentiates Th17-mediated immunity. J Immunol (2012) 188(7):3062–70. doi: 10.4049/jimmunol.1103096
45. Nakano K, Higashi T, Hashimoto K, Takagi R, Tanaka Y, Matsushita S. Antagonizing dopamine D1-like receptor inhibits Th17 cell differentiation: preventive and therapeutic effects on experimental autoimmune encephalomyelitis. Biochem Biophys Res Commun (2008) 373(2):286–91. doi: 10.1016/j.bbrc.2008.06.012
46. Cosentino M, Zaffaroni M, Trojano M, Giorelli M, Pica C, Rasini E, et al. Dopaminergic modulation of CD4+CD25(high) regulatory T lymphocytes in multiple sclerosis patients during interferon-beta therapy. Neuroimmunomodulation (2012) 19(5):283–92. doi: 10.1159/000336981
47. Kasai RS, Ito SV, Awane RM, Fujiwara TK, Kusumi A. The Class-A GPCR Dopamine D2 Receptor Forms Transient Dimers Stabilized by Agonists: Detection by Single-Molecule Tracking. Cell Biochem Biophys (2018) 76(1-2):29–37. doi: 10.1007/s12013-017-0829-y
48. Han Y, Moreira IS, Urizar E, Weinstein H, Javitch JA. Allosteric communication between protomers of dopamine class A GPCR dimers modulates activation. Nat Chem Biol (2009) 5(9):688–95. doi: 10.1038/nchembio.199
49. Perreault ML, Hasbi A, O’Dowd BF, George SR. Heteromeric Dopamine Receptor Signaling Complexes: Emerging Neurobiology and Disease Relevance. Neuropsychopharmacology (2014) 39(1):156–68. doi: 10.1038/npp.2013.148
50. Guitart X, Moreno E, Rea W, Sanchez-Soto M, Cai NS, Quiroz C, et al. Biased G Protein-Independent Signaling of Dopamine D1-D3 Receptor Heteromers in the Nucleus Accumbens. Mol Neurobiol (2019) 56(10):6756–69. doi: 10.1007/s12035-019-1564-8
51. González S, Rangel-Barajas C, Peper M, Lorenzo R, Moreno E, Ciruela F, et al. Dopamine D4 receptor, but not the ADHD-associated D4.7 variant, forms functional heteromers with the dopamine D2S receptor in the brain. Mol Psychiatry (2012) 17(6):650–62. doi: 10.1038/mp.2011.93
52. Pelassa S, Guidolin D, Venturini A, Averna M, Frumento G, Campanini L, et al. A2A-D2 Heteromers on Striatal Astrocytes: Biochemical and Biophysical Evidence. Int J Mol Sci (2019) 20(10):2457. doi: 10.3390/ijms20102457
53. Sahlholm K, Gomez-Soler M, Valle-Leon M, Lopez-Cano M, Taura JJ, Ciruela F, et al. Antipsychotic-Like Efficacy of Dopamine D-2 Receptor-Biased Ligands is Dependent on Adenosine A(2A) Receptor Expression. Mol Neurobiol (2018) 55(6):4952–8. doi: 10.1007/s12035-017-0696-y
54. Borroto-Escuela DO, Romero-Fernandez W, Tarakanov AO, Ciruela F, Agnati LF, Fuxe K. On the existence of a possible A2A-D2-beta-Arrestin2 complex: A2A agonist modulation of D2 agonist-induced beta-arrestin2 recruitment. J Mol Biol (2011) 406(5):687–99. doi: 10.1016/j.jmb.2011.01.022
55. Fuxe K, Marcellino D, Borroto-Escuela DO, Guescini M, Fernández-Dueas V, Tanganelli S, et al. Adenosine–Dopamine Interactions in the Pathophysiology and Treatment of CNS Disorders. CNS Drug Rev (2010) 16(3):e18-42. doi: 10.1111/j.1755-5949.2009.00126.x
56. Beggiato S, Antonelli T, Tomasini MC, Borelli AC, Agnati LF, Tanganelli S, et al. Adenosine A2A-D2 receptor-receptor interactions in putative heteromers in the regulation of the striato-pallidal gaba pathway: possible relevance for parkinson’s disease and its treatment. Curr Protein Pept Sci (2014) 15(7):673–80. doi: 10.2174/1389203715666140901103205
57. Bonaventura J, Navarro G, Casado-Anguera V, Azdad K, Rea W, Moreno E, et al. Allosteric interactions between agonists and antagonists within the adenosine A2A receptor-dopamine D2 receptor heterotetramer. Proc Natl Acad Sci USA (2015) 112(27):E3609–18. doi: 10.1073/pnas.1507704112
58. Torvinen M, Marcellino D, Canals M, Agnati LF, Lluis C, Franco R, et al. Adenosine A2A receptor and dopamine D3 receptor interactions: evidence of functional A2A/D3 heteromeric complexes. Mol Pharmacol (2005) 67(2):400–7. doi: 10.1124/mol.104.003376
59. Franco R, Lluis C, Canela EI, Mallol J, Agnati L, Casado V, et al. Receptor-receptor interactions involving adenosine A1 or dopamine D1 receptors and accessory proteins. J Neural Transm (Vienna) (2007) 114(1):93–104. doi: 10.1007/s00702-006-0566-7
60. Hobson BD, O’Neill CE, Levis SC, Monteggia LM, Neve RL, Self DW, et al. Adenosine A1 and dopamine d1 receptor regulation of AMPA receptor phosphorylation and cocaine-seeking behavior. Neuropsychopharmacology (2013) 38(10):1974–83. doi: 10.1038/npp.2013.96
61. Cunha RA. Neuroprotection by adenosine in the brain: From A(1) receptor activation to A (2A) receptor blockade. Purinergic Signal (2005) 1(2):111–34. doi: 10.1007/s11302-005-0649-1
62. Acevedo J, Santana-Almansa A, Matos-Vergara N, Marrero-Cordero LR, Cabezas-Bou E, Diaz-Rios M. Caffeine stimulates locomotor activity in the mammalian spinal cord via adenosine A1 receptor-dopamine D1 receptor interaction and PKA-dependent mechanisms. Neuropharmacology (2016) 101:490–505. doi: 10.1016/j.neuropharm.2015.10.020
63. Alfaro-Rodriguez A, Alonso-Spilsbury M, Arch-Tirado E, Gonzalez-Pina R, Arias-Montano JA, Bueno-Nava A. Histamine H3 receptor activation prevents dopamine D1 receptor-mediated inhibition of dopamine release in the rat striatum: a microdialysis study. Neurosci Lett (2013) 552:5–9. doi: 10.1016/j.neulet.2013.07.026
64. Valjent E, Biever A, Gangarossa G, Puighermanal E. Dopamine signaling in the striatum. Adv Protein Chem Struct Biol (2019) 116:375–96. doi: 10.1016/bs.apcsb.2019.01.004
65. Rapanelli M, Frick LR, Horn KD, Schwarcz RC, Pogorelov V, Nairn AC, et al. The Histamine H3 Receptor Differentially Modulates Mitogen-activated Protein Kinase (MAPK) and Akt Signaling in Striatonigral and Striatopallidal Neurons. J Biol Chem (2016) 291(40):21042–52. doi: 10.1074/jbc.M116.731406
66. Moreno-Delgado D, Puigdellivol M, Moreno E, Rodriguez-Ruiz M, Botta J, Gasperini P, et al. Modulation of dopamine D1 receptors via histamine H3 receptors is a novel therapeutic target for Huntington’s disease. Elife (2020) 9:e51093. doi: 10.7554/eLife.51093
67. Dai WL, Bao YN, Fan JF, Ma B, Li SS, Zhao WL, et al. Blockade of spinal dopamine D1/D2 receptor suppresses activation of NMDA receptor through Galphaq and Src kinase to attenuate chronic bone cancer pain. J Adv Res (2021) 28:139–48. doi: 10.1016/j.jare.2020.08.005
68. Trepanier CH, Jackson MF, MacDonald JF. Regulation of NMDA receptors by the tyrosine kinase Fyn. FEBS J (2012) 279(1):12–9. doi: 10.1111/j.1742-4658.2011.08391.x
69. Li YC, Liu G, Hu JL, Gao WJ, Huang YQ. Dopamine D(1) receptor-mediated enhancement of NMDA receptor trafficking requires rapid PKC-dependent synaptic insertion in the prefrontal neurons. J Neurochem (2010) 114(1):62–73. doi: 10.1111/j.1471-4159.2010.06720.x
70. Lee FJ, Xue S, Pei L, Vukusic B, Chery N, Wang Y, et al. Dual regulation of NMDA receptor functions by direct protein-protein interactions with the dopamine D1 receptor. Cell (2002) 111(2):219–30. doi: 10.1016/S0092-8674(02)00962-5
71. Varela JA, Hirsch SJ, Chapman D, Leverich LS, Greene RW. D1/D5 modulation of synaptic NMDA receptor currents. J Neurosci (2009) 29(10):3109–19. doi: 10.1523/JNEUROSCI.4746-08.2009
72. Lei G, Xia Y, Johnson KM. The role of Akt-GSK-3beta signaling and synaptic strength in phencyclidine-induced neurodegeneration. Neuropsychopharmacology (2008) 33(6):1343–53. doi: 10.1038/sj.npp.1301511
73. Beaulieu JM, Gainetdinov RR. The physiology, signaling, and pharmacology of dopamine receptors. Pharmacol Rev (2011) 63(1):182–217. doi: 10.1124/pr.110.002642
74. Fiorentini C, Gardoni F, Spano P, Di Luca M, Missale C. Regulation of dopamine D1 receptor trafficking and desensitization by oligomerization with glutamate N-methyl-D-aspartate receptors. J Biol Chem (2003) 278(22):20196–202. doi: 10.1074/jbc.M213140200
75. Pei L, Lee FJ, Moszczynska A, Vukusic B, Liu F. Regulation of dopamine D1 receptor function by physical interaction with the NMDA receptors. J Neurosci (2004) 24(5):1149–58. doi: 10.1523/JNEUROSCI.3922-03.2004
76. Liu T, Whitten ST, Hilser VJ. Ensemble-based signatures of energy propagation in proteins: a new view of an old phenomenon. Proteins (2006) 62(3):728–38. doi: 10.1002/prot.20749
77. Bagher AM, Laprairie RB, Toguri JT, Kelly MEM, Denovan-Wright EM. Bidirectional allosteric interactions between cannabinoid receptor 1 (CB1) and dopamine receptor 2 long (D-2L) heterotetramers. Eur J Pharmacol (2017) 813:66–83. doi: 10.1016/j.ejphar.2017.07.034
78. Bono F, Mutti V, Fiorentini C, Missale C. Dopamine D3 Receptor Heteromerization: Implications for Neuroplasticity and Neuroprotection. Biomolecules (2020) 10(7):1016. doi: 10.3390/biom10071016
79. Fuxe K, Ferré S, Woods A, Lluis C, Franco R, Agnati L. L.01 Novel strategies for treatment of schizophrenia and Parkinson’s disease. Focus on receptor-receptor interactions in the basal ganglia. Eur Neuropsychopharmacol (2003) 13(03):S93–4. doi: 10.1016/S0924-977X(03)91596-3
80. Morin N, Di Paolo T. Interaction of Adenosine Receptors with Other Receptors from Therapeutic Perspective in Parkinson’s Disease. Adenosine Recept Neurol Psychiatry (2014) 119:151–67. doi: 10.1016/B978-0-12-801022-8.00007-6
81. Morelli M, Di Paolo T, Wardas J, Calon F, Xiao D, Schwarzschild MA. Role of adenosine A2A receptors in parkinsonian motor impairment and l-DOPA-induced motor complications. Prog Neurobiol (2007) 83(5):293–309. doi: 10.1016/j.pneurobio.2007.07.001
82. Navarro G, Ferre S, Cordomi A, Moreno E, Mallol J, Casado V, et al. Interactions between Intracellular Domains as Key Determinants of the Quaternary Structure and Function of Receptor Heteromers. J Biol Chem (2010) 285(35):27346–59. doi: 10.1074/jbc.M110.115634
83. Heneka MT, Kummer MP, Stutz A, Delekate A, Schwartz S, Vieira-Saecker A, et al. NLRP3 is activated in Alzheimer’s disease and contributes to pathology in APP/PS1 mice. Nature (2013) 493(7434):674–8. doi: 10.1038/nature11729
84. Wen H, Gris D, Lei Y, Jha S, Zhang L, Huang MT, et al. Fatty acid-induced NLRP3-ASC inflammasome activation interferes with insulin signaling. Nat Immunol (2011) 12(5):408–15. doi: 10.1038/ni.2022
85. Lee GS, Subramanian N, Kim AI, Aksentijevich I, Goldbach-Mansky R, Sacks DB, et al. The calcium-sensing receptor regulates the NLRP3 inflammasome through Ca2+ and cAMP. Nature (2012) 492(7427):123–7. doi: 10.1038/nature11588
86. Fan H. beta-Arrestins 1 and 2 are critical regulators of inflammation. Innate Immun (2014) 20(5):451–60. doi: 10.1177/1753425913501098
87. Song N, Fang Y, Sun X, Jiang Q, Song C, Chen M, et al. Salmeterol, agonist of beta2-aderenergic receptor, prevents systemic inflammation via inhibiting NLRP3 inflammasome. Biochem Pharmacol (2018) 150:245–55. doi: 10.1016/j.bcp.2018.02.009
88. Yan Y, Jiang W, Spinetti T, Tardivel A, Castillo R, Bourquin C, et al. Omega-3 fatty acids prevent inflammation and metabolic disorder through inhibition of NLRP3 inflammasome activation. Immunity (2013) 38(6):1154–63. doi: 10.1016/j.immuni.2013.05.015
89. Lilienbaum A, Israel A. From calcium to NF-kappa B signaling pathways in neurons. Mol Cell Biol (2003) 23(8):2680–98. doi: 10.1128/MCB.23.8.2680-2698.2003
90. Neumann M, Grieshammer T, Chuvpilo S, Kneitz B, Lohoff M, Schimpl A, et al. RelA/p65 is a molecular target for the immunosuppressive action of protein kinase A. EMBO J (1995) 14(9):1991–2004. doi: 10.1002/j.1460-2075.1995.tb07191.x
91. Wu Y, Hu Y, Wang B, Li S, Ma C, Liu X, et al. Dopamine Uses the DRD5-ARRB2-PP2A Signaling Axis to Block the TRAF6-Mediated NF-kappaB Pathway and Suppress Systemic Inflammation. Mol Cell (2020) 78(1):42–56 e6. doi: 10.1016/j.molcel.2020.01.022
92. Zhang Y, Chen Y, Wu J, Manaenko A, Yang P, Tang J, et al. Activation of Dopamine D2 Receptor Suppresses Neuroinflammation Through alphaB-Crystalline by Inhibition of NF-kappaB Nuclear Translocation in Experimental ICH Mice Model. Stroke (2015) 46(9):2637–46. doi: 10.1161/STROKEAHA.115.009792
93. Hagemann TL, Boelens WC, Wawrousek EF, Messing A. Suppression of GFAP toxicity by alphaB-crystallin in mouse models of Alexander disease. Hum Mol Genet (2009) 18(7):1190–9. doi: 10.1093/hmg/ddp013
94. Qiu J, Yan Z, Tao K, Li Y, Li Y, Li J, et al. Sinomenine activates astrocytic dopamine D2 receptors and alleviates neuroinflammatory injury via the CRYAB/STAT3 pathway after ischemic stroke in mice. J Neuroinflamm (2016) 13(1):263. doi: 10.1186/s12974-016-0739-8
95. Levite M. Dopamine and T cells: dopamine receptors and potent effects on T cells, dopamine production in T cells, and abnormalities in the dopaminergic system in T cells in autoimmune, neurological and psychiatric diseases. Acta Physiol (Oxf) (2016) 216(1):42–89. doi: 10.1111/apha.12476
96. Cosentino M, Fietta AM, Ferrari M, Rasini E, Bombelli R, Carcano E, et al. Human CD4+CD25+ regulatory T cells selectively express tyrosine hydroxylase and contain endogenous catecholamines subserving an autocrine/paracrine inhibitory functional loop. Blood (2007) 109(2):632–42. doi: 10.1182/blood-2006-01-028423
97. Kipnis J, Cardon M, Avidan H, Lewitus GM, Mordechay S, Rolls A, et al. Dopamine, through the extracellular signal-regulated kinase pathway, downregulates CD4+CD25+ regulatory T-cell activity: implications for neurodegeneration. J Neurosci (2004) 24(27):6133–43. doi: 10.1523/JNEUROSCI.0600-04.2004
98. Zhang Y, Zhang YG, Gu WP, Sun B. Th1/Th2 Cell Differentiation and Molecular Signals. T Helper Cell Differ Their Funct (2014) 841:E1–2. doi: 10.1007/978-94-017-9487-9_9
99. Ilani T, Strous RD, Fuchs S. Dopaminergic regulation of immune cells via D3 dopamine receptor: a pathway mediated by activated T cells. FASEB J (2004) 18(13):1600–2. doi: 10.1096/fj.04-1652fje
100. Seki Y, Hayashi K, Matsumoto A, Seki N, Tsukada J, Ransom J, et al. Expression of the suppressor of cytokine signaling-5 (SOCS5) negatively regulates IL-4-dependent STAT6 activation and Th2 differentiation. Proc Natl Acad Sci USA (2002) 99(20):13003–8. doi: 10.1073/pnas.202477099
101. Tripathi P, Sahoo N, Ullah U, Kallionpaa H, Suneja A, Lahesmaa R, et al. A novel mechanism for ERK-dependent regulation of IL4 transcription during human Th2-cell differentiation. Immunol Cell Biol (2012) 90(7):676–87. doi: 10.1038/icb.2011.87
102. Gong S, Li J, Ma L, Li K, Zhang L, Wang G, et al. Blockade of dopamine D1-like receptor signalling protects mice against OVA-induced acute asthma by inhibiting B-cell activating transcription factor signalling and Th17 function. FEBS J (2013) 280(23):6262–73. doi: 10.1111/febs.12549
103. Kawano M, Takagi R, Saika K, Matsui M, Matsushita S. Dopamine regulates cytokine secretion during innate and adaptive immune responses. Int Immunol (2018) 30(12):591–606. doi: 10.1093/intimm/dxy057
104. Gaskill PJ, Calderon TM, Coley JS, Berman JW. Drug induced increases in CNS dopamine alter monocyte, macrophage and T cell functions: implications for HAND. J Neuroimmune Pharmacol (2013) 8(3):621–42. doi: 10.1007/s11481-013-9443-y
105. Nolan RA, Muir R, Runner K, Haddad EK, Gaskill PJ. Role of Macrophage Dopamine Receptors in Mediating Cytokine Production: Implications for Neuroinflammation in the Context of HIV-Associated Neurocognitive Disorders. J Neuroimmune Pharmacol (2019) 14(1):134–56. doi: 10.1007/s11481-018-9825-2
106. Gaskill PJ, Carvallo L, Eugenin EA, Berman JW. Characterization and function of the human macrophage dopaminergic system: implications for CNS disease and drug abuse. J Neuroinflamm (2012) 9:203. doi: 10.1186/1742-2094-9-203
107. Gomez F, Ruiz P, Briceno F, Rivera C, Lopez R. Macrophage Fcgamma receptors expression is altered by treatment with dopaminergic drugs. Clin Immunol (1999) 90(3):375–87. doi: 10.1006/clim.1998.4665
108. Bone NB, Liu Z, Pittet JF, Zmijewski JW. Frontline Science: D1 dopaminergic receptor signaling activates the AMPK-bioenergetic pathway in macrophages and alveolar epithelial cells and reduces endotoxin-induced ALI. J Leukoc Biol (2017) 101(2):357–65. doi: 10.1189/jlb.3HI0216-068RR
109. Brenner S, Prosch S, Schenke-Layland K, Riese U, Gausmann U, Platzer C. cAMP-induced Interleukin-10 promoter activation depends on CCAAT/enhancer-binding protein expression and monocytic differentiation. J Biol Chem (2003) 278(8):5597–604. doi: 10.1074/jbc.M207448200
110. Prado C, Gaiazzi M, Gonzalez H, Ugalde V, Figueroa A, Osorio-Barrios FJ, et al. Dopaminergic Stimulation of Myeloid Antigen-Presenting Cells Attenuates Signal Transducer and Activator of Transcription 3-Activation Favouring the Development of Experimental Autoimmune Encephalomyelitis. Front Immunol (2018) 9:571. doi: 10.3389/fimmu.2018.00571
111. Jankovic J. Parkinson’s disease: clinical features and diagnosis. J Neurol Neurosurg Psychiatry (2008) 79(4):368–76. doi: 10.1136/jnnp.2007.131045
112. Broome ST, Louangaphay K, Keay KA, Leggio GM, Musumeci G, Castorina A. Dopamine: an immune transmitter. Neural Regen Res (2020) 15(12):2173–85. doi: 10.4103/1673-5374.284976
113. Wang X, Chi J, Huang D, Ding L, Zhao X, Jiang L, et al. alpha-synuclein promotes progression of Parkinson’s disease by upregulating autophagy signaling pathway to activate NLRP3 inflammasome. Exp Ther Med (2020) 19(2):931–8. doi: 10.3892/etm.2019.8297
114. Litteljohn D, Mangano E, Clarke M, Bobyn J, Moloney K, Hayley S. Inflammatory mechanisms of neurodegeneration in toxin-based models of Parkinson’s disease. Parkinsons Dis (2010) 2011:713517. doi: 10.4061/2011/713517
115. Wang W, Nguyen LT, Burlak C, Chegini F, Guo F, Chataway T, et al. Caspase-1 causes truncation and aggregation of the Parkinson’s disease-associated protein alpha-synuclein. Proc Natl Acad Sci USA (2016) 113(34):9587–92. doi: 10.1073/pnas.1610099113
116. Kirkley KS, Popichak KA, Afzali MF, Legare ME, Tjalkens RB. Microglia amplify inflammatory activation of astrocytes in manganese neurotoxicity. J Neuroinflamm (2017) 14(1):99. doi: 10.1186/s12974-017-0871-0
117. Ghosh M, Xu Y, Pearse DD. Cyclic AMP is a key regulator of M1 to M2a phenotypic conversion of microglia in the presence of Th2 cytokines. J Neuroinflamm (2016) 13(1):9. doi: 10.1186/s12974-015-0463-9
118. Sharma D, Parameswaran N. Multifaceted role of beta-arrestins in inflammation and disease. Genes Immun (2015) 16(8):499–513. doi: 10.1038/gene.2015.37
119. Cervetto C, Venturini A, Guidolin D, Maura G, Passalacqua M, Tacchetti C, et al. Homocysteine and A2A-D2 Receptor-Receptor Interaction at Striatal Astrocyte Processes. J Mol Neurosci (2018) 65(4):456–66. doi: 10.1007/s12031-018-1120-4
120. Meng F, Guo Z, Hu Y, Mai W, Zhang Z, Zhang B, et al. CD73-derived adenosine controls inflammation and neurodegeneration by modulating dopamine signalling. Brain (2019) 142(3):700–18. doi: 10.1093/brain/awy351
121. Chen Y, Ni YY, Liu J, Lu JW, Wang F, Wu XL, et al. Dopamine receptor 3 might be an essential molecule in 1-methyl-4-phenyl-1,2,3,6-tetrahydropyridine-induced neurotoxicity. BMC Neurosci (2013) 14:76. doi: 10.1186/1471-2202-14-76
122. Barcia C, Ros CM, Annese V, Gomez A, Ros-Bernal F, Aguado-Llera D, et al. IFN-gamma signaling, with the synergistic contribution of TNF-alpha, mediates cell specific microglial and astroglial activation in experimental models of Parkinson’s disease. Cell Death Dis (2012) 2(4):e142. doi: 10.1038/cddis.2011.17
123. Chen Y, Qi B, Xu W, Ma B, Li L, Chen Q, et al. Clinical correlation of peripheral CD4+cell subsets, their imbalance and Parkinson’s disease. Mol Med Rep (2015) 12(4):6105–11. doi: 10.3892/mmr.2015.4136
124. Gonzalez H, Contreras F, Prado C, Elgueta D, Franz D, Bernales S, et al. Dopamine receptor D3 expressed on CD4+ T cells favors neurodegeneration of dopaminergic neurons during Parkinson’s disease. J Immunol (2013) 190(10):5048–56. doi: 10.4049/jimmunol.1203121
125. Nagai Y, Ueno S, Saeki Y, Soga F, Hirano M, Yanagihara T. Decrease of the D3 dopamine receptor mRNA expression in lymphocytes from patients with Parkinson’s disease. Neurology (1996) 46(3):791–5. doi: 10.1212/WNL.46.3.791
126. Chau KY, Cooper JM, Schapira AH. Pramipexole reduces phosphorylation of alpha-synuclein at serine-129. J Mol Neurosci (2013) 51(2):573–80. doi: 10.1007/s12031-013-0030-8
127. Barbeau A, Godin AG, Swift JL, De Koninck Y, Wiseman PW, Beaulieu JM. Quantification of receptor tyrosine kinase activation and transactivation by G-protein-coupled receptors using spatial intensity distribution analysis (SpIDA). Methods Enzymol (2013) 522:109–31. doi: 10.1016/B978-0-12-407865-9.00007-8
128. Jiang L, Zhang H, Wang C, Ming F, Shi X, Yang M. Serum level of brain-derived neurotrophic factor in Parkinson’s disease: a meta-analysis. Prog Neuropsychopharmacol Biol Psychiatry (2019) 88:168–74. doi: 10.1016/j.pnpbp.2018.07.010
129. Yang P, Knight WC, Li H, Guo Y, Xu J. Dopamine D1 + D3 receptor density may correlate with parkinson disease clinical features. Ann Clin Trans Neurol (2021) 8(1):224–37. doi: 10.1002/acn3.51274
130. Azkona G, Sagarduy A, Aristieta A, Vazquez N, Zubillaga V, Ruiz-Ortega JA, et al. Buspirone anti-dyskinetic effect is correlated with temporal normalization of dysregulated striatal DRD1 signalling in L-DOPA-treated rats. Neuropharmacology (2014) 79:726–37. doi: 10.1016/j.neuropharm.2013.11.024
131. Kreitzer AC, Malenka RC. Endocannabinoid-mediated rescue of striatal LTD and motor deficits in Parkinson’s disease models. Nature (2007) 445(7128):643–7. doi: 10.1038/nature05506
132. Iijima M, Orimo S, Terashi H, Suzuki M, Hayashi A, Shimura H, et al. Efficacy of istradefylline for gait disorders with freezing of gait in Parkinson’s disease: A single-arm, open-label, prospective, multicenter study. Expert Opin Pharmacother (2019) 20(11):1405–11. doi: 10.1080/14656566.2019.1614167
133. Armentero MT, Pinna A, Ferre S, Lanciego JL, Muller CE, Franco R. Past, present and future of A(2A) adenosine receptor antagonists in the therapy of Parkinson’s disease. Pharmacol Ther (2011) 132(3):280–99. doi: 10.1016/j.pharmthera.2011.07.004
134. Bhattacharjee AK, Lang LX, Jacobson O, Shinkre B, Ma Y, Niu G, et al. Striatal adenosine A(2A) receptor-mediated positron emission tomographic imaging in 6-hydroxydopamine-lesioned rats using [F-18]-MRS5425. Nucl Med Biol (2011) 38(6):897–906. doi: 10.1016/j.nucmedbio.2011.01.009
135. Han F, Konkalmatt P, Mokashi C, Kumar M, Zhang Y, Ko A, et al. Dopamine D2 receptor modulates Wnt expression and control of cell proliferation. Sci Rep (2019) 9(1):16861. doi: 10.1038/s41598-019-52528-4
136. Straub RH, Grum F, Strauch U, Capellino S, Bataille F, Bleich A, et al. Anti-inflammatory role of sympathetic nerves in chronic intestinal inflammation. Gut (2008) 57(7):911–21. doi: 10.1136/gut.2007.125401
137. Magro F, Vieira-Coelho MA, Fraga S, Serrao MP, Veloso FT, Ribeiro T, et al. Impaired synthesis or cellular storage of norepinephrine, dopamine, and 5-hydroxytryptamine in human inflammatory bowel disease. Dig Dis Sci (2002) 47(1):216–24. doi: 10.1023/a:1013256629600
138. Contreras F, Prado C, Gonzalez H, Franz D, Osorio-Barrios F, Osorio F, et al. Dopamine Receptor D3 Signaling on CD4+ T Cells Favors Th1- and Th17-Mediated Immunity. J Immunol (2016) 196(10):4143–9. doi: 10.4049/jimmunol.1502420
139. Miyazawa T, Matsumoto M, Kato S, Takeuchi K. Dopamine-induced protection against indomethacin-evoked intestinal lesions in rats–role of anti-intestinal motility mediated by D2 receptors. Med Sci Monit (2003) 9(2):BR71–7.
140. Basu S, Nagy JA, Pal S, Vasile E, Eckelhoefer IA, Bliss VS, et al. The neurotransmitter dopamine inhibits angiogenesis induced by vascular permeability factor/vascular endothelial growth factor. Nat Med (2001) 7(5):569–74. doi: 10.1038/87895
141. Basu S, Sarkar C, Chakroborty D, Nagy J, Mitra RB, Dasgupta PS, et al. Ablation of peripheral dopaminergic nerves stimulates malignant tumor growth by inducing vascular permeability factor/vascular endothelial growth factor-mediated angiogenesis. Cancer Res (2004) 64(16):5551–5. doi: 10.1158/0008-5472.CAN-04-1600
142. Smolen JS, Aletaha D, Barton A, Burmester GR, Emery P, Firestein GS, et al. Rheumatoid arthritis. Nat Rev Dis Primers (2018) 4:18001. doi: 10.1038/nrdp.2018.1
143. Lu JH, Liu YQ, Deng QW, Peng YP, Qiu YH. Dopamine D2 Receptor Is Involved in Alleviation of Type II Collagen-Induced Arthritis in Mice. BioMed Res Int (2015) 2015:496759. doi: 10.1155/2015/496759
144. Xue L, Li XY, Chen QP, He JT, Dong YY, Wang J, et al. Associations between D3R expression in synovial mast cells and disease activity and oxidant status in patients with rheumatoid arthritis. Clin Rheumatol (2018) 37(10):2621–32. doi: 10.1007/s10067-018-4168-1
145. Wei L, Zhang C, Chen HY, Zhang ZJ, Ji ZF, Yue T, et al. Dopamine receptor DR2 expression in B cells is negatively correlated with disease activity in rheumatoid arthritis patients. Immunobiology (2015) 220(3):323–30. doi: 10.1016/j.imbio.2014.10.016
146. van Nie L, Salinas-Tejedor L, Dychus N, Fasbender F, Hulser ML, Cutolo M, et al. Dopamine induces in vitro migration of synovial fibroblast from patients with rheumatoid arthritis. Sci Rep (2020) 10(1):11928. doi: 10.1038/s41598-020-68836-z
147. Boyle WJ, Simonet WS, Lacey DL. Osteoclast differentiation and activation. Nature (2003) 423(6937):337–42. doi: 10.1038/nature01658
148. Jafari M, Ahangari G, Saberi M, Samangoui S, Torabi R, Zouali M. Distorted expression of dopamine receptor genes in systemic lupus erythematosus. Immunobiology (2013) 218(7):979–83. doi: 10.1016/j.imbio.2012.11.002
149. Jafari M, Ahangari G, Saberi M, Samangoui S, Torabi R, Zouali M. Distorted expression of dopamine receptor genes in systemic lupus erythematosus. Immunobiology (2013) 218(7):979–83. doi: 10.1016/j.imbio.2012.11.002
150. Gonzalez-Iglesias AE, Murano T, Li S, Tomic M, Stojilkovic SS. Dopamine inhibits basal prolactin release in pituitary lactotrophs through pertussis toxin-sensitive and -insensitive signaling pathways. Endocrinology (2008) 149(4):1470–9. doi: 10.1210/en.2007-0980
151. Jara LJ, Medina G, Saavedra MA, Vera-Lastra O, Torres-Aguilar H, Navarro C, et al. Prolactin has a pathogenic role in systemic lupus erythematosus. Immunol Res (2017) 65(2):512–23. doi: 10.1007/s12026-016-8891-x
152. Sarkar C, Das S, Chakroborty D, Chowdhury UR, Basu B, Dasgupta PS, et al. Cutting Edge: Stimulation of dopamine D4 receptors induce T cell quiescence by up-regulating Kruppel-like factor-2 expression through inhibition of ERK1/ERK2 phosphorylation. J Immunol (2006) 177(11):7525–9. doi: 10.4049/jimmunol.177.11.7525
153. Giorelli M, Livrea P, Trojano M. Dopamine fails to regulate activation of peripheral blood lymphocytes from multiple sclerosis patients: effects of IFN-beta. J Interferon Cytokine Res (2005) 25(7):395–406. doi: 10.1089/jir.2005.25.395
154. Tzartos JS, Friese MA, Craner MJ, Palace J, Newcombe J, Esiri MM, et al. Interleukin-17 production in central nervous system-infiltrating T cells and glial cells is associated with active disease in multiple sclerosis. Am J Pathol (2008) 172(1):146–55. doi: 10.2353/ajpath.2008.070690
155. Pacheco R, Contreras F, Zouali M. The dopaminergic system in autoimmune diseases. Front Immunol (2014) 5:117. doi: 10.3389/fimmu.2014.00117
156. Cosentino M, Zaffaroni M, Legnaro M, Bombelli R, Schembri L, Baroncini D, et al. Dopaminergic receptors and adrenoceptors in circulating lymphocytes as putative biomarkers for the early onset and progression of multiple sclerosis. J Neuroimmunol (2016) 298:82–9. doi: 10.1016/j.jneuroim.2016.07.008
Keywords: dopamine, inflammation, inflammatory bowel disease, Parkinson disease, rheumatoid arthritis, systemic lupus erythematosus, multiple sclerosis
Citation: Feng YF and Lu Y (2021) Immunomodulatory Effects of Dopamine in Inflammatory Diseases. Front. Immunol. 12:663102. doi: 10.3389/fimmu.2021.663102
Received: 03 February 2021; Accepted: 12 March 2021;
Published: 09 April 2021.
Edited by:
Mariele Gatto, University of Gothenburg, SwedenReviewed by:
Alessandro Salvalaggio, University Hospital of Padua, ItalyCopyright © 2021 Feng and Lu. This is an open-access article distributed under the terms of the Creative Commons Attribution License (CC BY). The use, distribution or reproduction in other forums is permitted, provided the original author(s) and the copyright owner(s) are credited and that the original publication in this journal is cited, in accordance with accepted academic practice. No use, distribution or reproduction is permitted which does not comply with these terms.
*Correspondence: Yan Lu, bHV5YW42Mjg5QDE2My5jb20=
Disclaimer: All claims expressed in this article are solely those of the authors and do not necessarily represent those of their affiliated organizations, or those of the publisher, the editors and the reviewers. Any product that may be evaluated in this article or claim that may be made by its manufacturer is not guaranteed or endorsed by the publisher.
Research integrity at Frontiers
Learn more about the work of our research integrity team to safeguard the quality of each article we publish.