- College of Medical and Dental Sciences, Institute for Immunology and Immunotherapy, University of Birmingham, Birmingham, United Kingdom
Persistent liver inflammation can lead to cirrhosis, which associates with significant morbidity and mortality worldwide. There are no curative treatments beyond transplantation, followed by long-term immunosuppression. The global burden of end stage liver disease has been increasing and there is a shortage of donor organs, therefore new therapies are desperately needed. Harnessing the power of the immune system has shown promise in certain autoimmunity and cancer settings. In the context of the liver, regulatory T cell (Treg) therapies are in development. The hypothesis is that these specialized lymphocytes that dampen inflammation may reduce liver injury in patients with chronic, progressive diseases, and promote transplant tolerance. Various strategies including intrinsic and extracorporeal expansion of Treg cells, aim to increase their abundance to suppress immune responses. We recently discovered that hepatocytes engulf and delete Treg cells by enclysis. Herein, we propose that inhibition of enclysis may potentiate existing regulatory T cell therapeutic approaches in patients with autoimmune liver diseases and in patients receiving a transplant. Moreover, in settings where the abundance of Treg cells could hinder beneficial immunity, such us in chronic viral infection or liver cancer, enhancement of enclysis could result in transient, localized reduction of Treg cell numbers and tip the balance towards antiviral and anti-tumor immunity. We describe enclysis as is a natural process of liver immune regulation that lends itself to therapeutic targeting, particularly in combination with current Treg cell approaches.
Introduction
The liver has a critical role in detoxification and hence it often becomes the site of cellular damage. Hepatocytes are liver epithelia that bear the worst of this process, and drive liver regeneration during injury. A healthy liver can cope with small amounts of tissue damage and repair itself as needed. Persistent liver injury, however, can lead to progressive liver damage, fibrosis, cirrhosis and end stage disease requiring a transplant.
Mortality due to liver disease is projected to overtake coronary heart disease by 2020 in the UK (1, 2). Globally, liver disease is estimated to account for 2 million deaths every year (3). Aside from preventable dietary injury, the major causes of liver failure are viral and autoimmune hepatitis. Autoimmune family liver diseases are T-cell-driven disorders that lead to hepatocyte (autoimmune hepatitis, AIH) or bile duct damage (primary biliary cholangitis, PBC, primary sclerosing cholangitis, PSC) that cause progressive liver failure. In some groups of patients that we are unable to predict, steroid or bile acid treatment respectively, can delay disease progression. Patients that fail to responsd to treatment rely on transplantation for survival. Viral hepatitis caused by hepatitis B (HBV) or hepatitis C virus (HCV), is the major indication for liver transplantation worldwide. In the UK, 180,000 patients have diagnosed chronic HBV (1) and 215,000 have persistent HCV infection (1). The WHO estimates that in 2015, HBV resulted in 887,000 deaths (4) and in 2016, HCV resulted in 339,000 deaths worldwide (5). Of these diseases that cause chronic liver inflammation, HCV is the only one where a curative treatment for the majority of patients is now available. However clinical disease progression continues in cirrhotic patients with severe disease even after virus eradication (6).
The increasing demand for liver transplantation and the decline in donor organs has highlighted the need for alternative novel therapies to prevent chronic hepatitis, which eventually leads to liver cirrhosis and increases the risk for liver cancer. The outcome of liver inflammation is determined by the balance of effector and regulatory immune cells activities: chronic hepatitis arises when effector cells persist, causing liver injury. Despite their prevalence, effector cells in chronic disease fail to control hepatocellular carcinoma, one of the two cancers with mortality projected to rise by 38% by 2035 (1). There is a pressing need to discover new approaches to toggle liver inflammation to prevent liver failure (7).
The liver plays a key role in immune tolerance (8) and it hosts a rich, specialised immune compartment (9, 10). Regulatory T cells (Treg) play a critical role in dampening overactive immune responses (11, 12). Treg cells suppress immune effector function by secreting immunosuppressive cytokines, by competing with effector T cells for costimulatory molecules by depriving effector cells of IL-2 and other direct and indirect processes recently reviewed in detail by Romano and colleagues (13).
Understanding how to control Treg cell frequencies in the liver is of increasing clinical interest in transplantation, in chronic liver inflammation from multiple etiologies, and in primary and metastatic liver cancer. The clinical goal is to increase the abundance of functional Treg cells in autoimmunity (14–16) and eliminate Tregs in early infection (16, 17) in order to clear the virus and potentiate immunotherapy (12, 18).
Enclysis in Liver Immune Regulation
CD4+ T cells are crucial cytokine-producing helper cells that orchestrate the tailoring of immune responses to fit the cause of injury. Hepatocytes comprise around 80% of the liver mass and perform vital functions including drug detoxification, clearance of dead cells (efferocytosis) and the remarkable ability of the liver to regenerate. We recently discovered that hepatocytes preferentially engulf live CD4+ T cells (19) in i) 2-D coculture and 3-D organoid models, ii) perfused human liver explants and iii) in healthy and end stage disease livers in vivo. Yet, not all T cells were treated equally: hepatocytes preferentially engulfed and deleted Treg cells, compared to effector T helper cells (Th), which promote immune responses (Figure 1). The incidence of Treg cells inside hepatocytes was higher in autoimmune hepatitis compared to viral hepatitis (19). We termed the engulfment and lysis of Treg cells Enclysis from the Greek ϵγκλϵίω (to enclose, to confine, to keep in captivity), and herein we explore how to target this process in different liver disease settings. The liver is the largest internal organ and it filters blood at a rate of ~ 1.4 L/min, enclysis could therefore have a major impact in Treg cell populations.
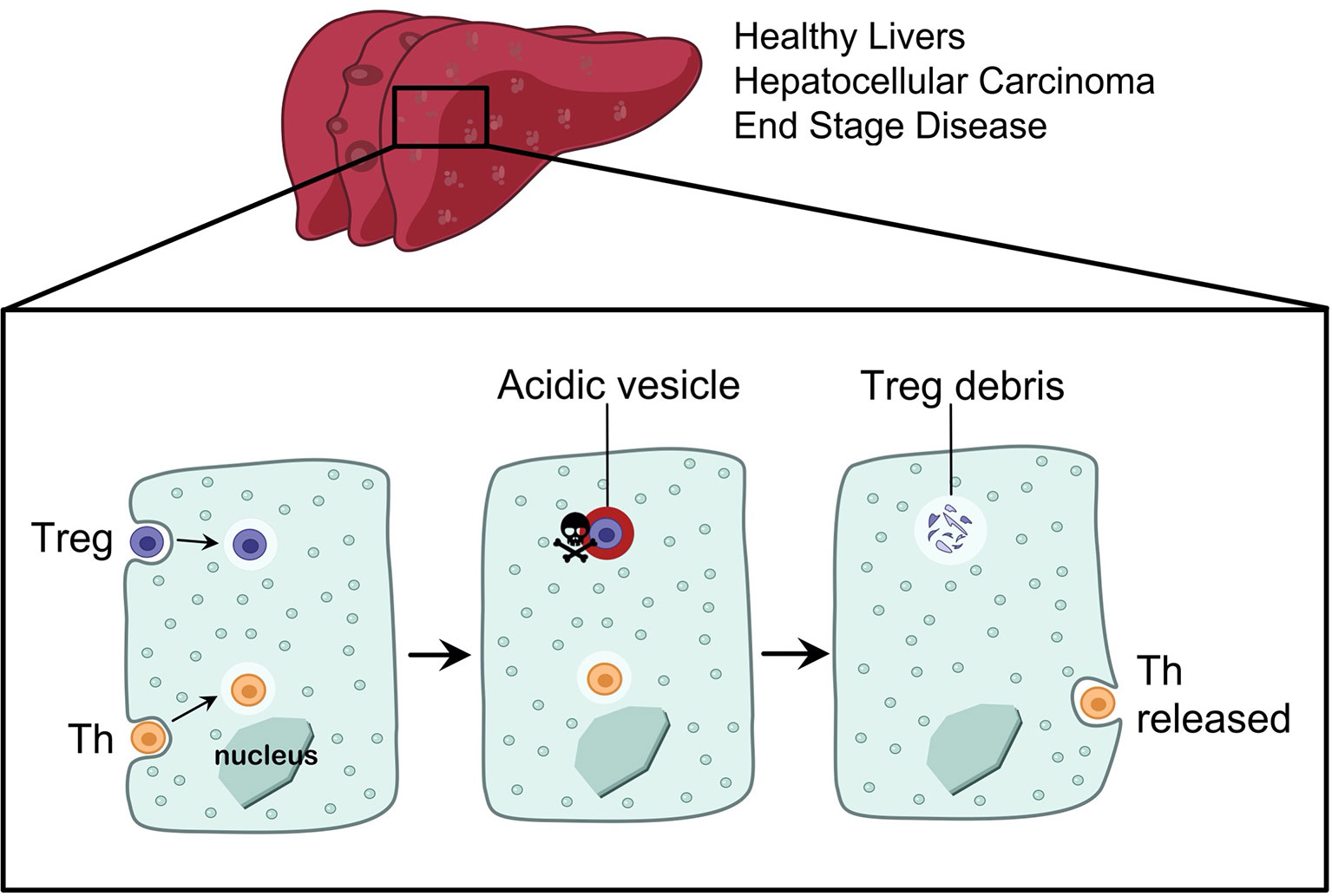
Figure 1 Enclysis is a CD4+ T cell engulfment process that leads to the deletion of regulatory T cells. Hepatocytes from non-cirrhotic donor livers, or from end stage disease explants, and hepatocellular carcinoma cells were all able to engulf CD4+ T cells by enclysis. T helper cells and Treg cells experience different fates inside hepatocytes, where T helper cells were preserved alive for hours or released and thus they survived enclytic capture, while Treg-containing vesicles quickly acidified.
We demonstrated that enclysis was distinct to known cell engulfment processes (19, 20), including dead cell efferocytosis (21), the elimination of autoreactive cytotoxic T cells by suicidal emperipolesis (22) and the homotypic internalization of cancer epithelia called entosis (23, 24), it is therefore possible to target it specifically.
Targeting Enclysis in Autoimmune Liver Disease
Loss of tolerance in the liver is observed in autoimmune family disorders such as autoimmune hepatitis (AIH), primary biliary cholangitis (PBC), and primary sclerosing cholangitis (PSC), where immune-mediated injury affects hepatocytes, small and large bile ducts respectively. The trigger for these diseases is not understood. Immunosuppression in AIH and PBC can be beneficial, however this is not the case for PSC (25). Current therapies are not curative and vary in efficacy in a manner that is presently challenging to predict for each patient, stressing the need for new treatments that effectively dampen inflammation in the liver to prevent the need for a transplant (26–29). Treg cell therapies are in various stages of development for AIH but not for cholangiopathies (25, 27). In autoimmunity, increasing the frequency of Tregs specifically in the liver by inhibiting enclysis may be useful alone or in combination with immunosuppressive regimens.
AIH is a chronic liver disease characterized by excessive immune responses associated with effector T cells (30, 31). In northern Europe, AIH affects approximately 1.9 per 100,000, with 25% of patients presenting as asymptomatic, and 40% showing signs of acute hepatitis before diagnosis (32–34). Approximately one third of patients show previous signs of increased fibrosis and liver cirrhosis (35). When treatment is readily available, a 10-year survival rate can be as high as 93%, however if no treatment is given, 40% of patients may die within six months of diagnosis (36) and current treatment shows measurable effects in up to 80% of patients (30, 37).
Various underlying liver damage mechanisms are associated with AIH. Predominantly, the presentation of self-antigens by antigen presenting cells to the T cell receptor of T-helper lymphocytes results in their activation and differentiation into more specified subsets of T-helper cells (38). For example, Th1 differentiation triggers IL-2 and interferon-gamma (IFN-γ) production, which in turn initiates activation of cytotoxic CD8+ T cells (39). Increased exposure of hepatocytes to IFN-γ increases the production of MHC class I complexes leading to increased T-cell activation and liver injury (13). Th2-type T cells secrete IL-4, IL-10 and IL-13, which are key cytokines for B cell maturation and the production of autoantibodies against hepatic auto-antigens, contributing further to cytotoxicity (40). Successful treatment of hepatic autoimmunity reduces hepatic inflammation and fibrosis. Our increased understanding of disease pathogenesis over the past few years suggests that a reduction in the dose and duration of steroid treatment can be considered, to minimize adverse effects associated with immunosuppression (41–43).
Evidence of Treg involvement in AIH have emerged in patients and murine models (44–46). Lapierre et al. demonstrated that severe AIH develops as a result of reduced numbers of functional Tregs, and that adoptive transfer of Tregs in mice with AIH was sufficient to restore peripheral tolerance to an ectopic liver autoantigen (FTCD), and induce remission (44). Various studies aimed to answer if Treg cell frequency or function were altered in AIH livers. Some showed numerical/functional impairment of regulatory cells in AIH (45, 47–54), and others found no deficiency in their patient cohorts (55, 56).
Although we noted no differences between FOXP3+ Treg cell numbers in liver tissue sections from AIH patients compared to other end stage diseases, we found that more FOXP3+ cells were engulfed by hepatocytes in AIH (19). Enclysis was also more frequent in FOXP3+ cells compared to Tbet+ helper cells, and this difference was increased in AIH patients compared to those with hepatitis B infection. Further investigation is needed to establish if the imbalance in enclysis observed with AIH would play a role in disease pathogenesis.
In patients with AIH (57, 58), as well as ten other autoimmune conditions (58), treatment with low dose IL-2 was safe, paving the way for phase II trials. Intriguingly, complexed IL-2/anti-IL-2 restored the balance between Treg and effector T cells (Teff) in the liver thereby improving the course of disease in experimental murine AIH (59). In mice with concanavalin-A-induced AIH, adoptive transfer of Tregs stimulated by allogeneic hepatic stellate cells alleviated injury (60). In CYP2D6-induced AIH mice and in AIH patients, Treg/Th17 imbalance associated with poor prognosis (61).
Supplementation with all-trans-retinoic acid (RA) or rapamycin (RP) agents enhanced Treg function and decreased up-regulation of Th1/Th2/Th17 transcription factors in cells from AIH patients (62). Further Treg conditioning experiments achieved skewing towards regulatory phenotype in cells from healthy volunteers and AIH patients, however AIH cells did not maintain suppressing function after stimulation (63). Together these data show that Treg therapies in AIH show promise, but we must carefully consider the preparation of Treg cells ex vivo or in vivo.
Ex vivo expansion of clinical grade regulatory T cells is laborious and costly, but it is certainly possible (64), and they can reach the liver following infusion (65). The persistence of these cells in inflamed livers and the duration of any benefit need further investigation, however in low dose IL-2-treated patients with refractory AIH, increase in Treg populations persisted until 28 days after treatment (57). Enclysis-targeting interventions could prolong efficacy of Treg therapies by preventing elimination of Treg cells in the liver parenchyma. Worryingly, immunosuppressive treatment with steroid and azathioprine diminished intrahepatic Treg cells (66). The role of immunosuppressive treatments on enclysis has not been studied. Table 1 summarizes current and novel AIH therapies and lists the potential of enclysis inhibitors in treating AIH.
Targeting Enclysis in Transplantation
As regulatory T cell therapies are in the forefront for transplantation (11, 67–69), targeting enclysis may also be useful to help prevent Graft Versus Host Disease (GVHD) and the rejection of donated organs, or to maintain remission profiles for patients post-transplant. Transplant rejection is limited solely through immunosuppressive drugs that are taken for the duration of a patient’s life, increasing risk of infection. In the liver, however, withdrawal of immunosuppression post-transplant is possible in some patients and must be managed carefully (70).
In the absence of frequent biopsies, we are unable to confirm complete absence of GVHD activity in liver patients, intervention success is therefore measured as operational tolerance. The first study to achieve operational tolerance in transplant patients was by Todo et al. where a cell product enriched for Treg cells was infused in ten patients who were weaned off immunosuppression after 18 months (71). The treatment failed to achieve operational tolerance in only three out of ten patients, all of whom suffered from autoimmune liver diseases. Furthermore, in recent phase 1 clinical trials blood Treg cells were isolated and infused into post-liver transplantation patients at 1-4.5 million/KG (68). Nine patients were administered GMP-Treg cells and it was shown that the therapy was safe and the overall Treg number increased. However, patients with autoimmune liver disease remained a challenge.
Regulatory T cell therapies are remarkably promising in transplantation and have been reviewed recently (11, 12, 72–78). To avoid broad immunosuppression and the risk of infection with polyclonal Tregs, one strategy is to focus on antigen-specific Treg cells, which induced tolerance in animal models and can also be expanded from patients (64). CAR-T cell technologies are also being explored to this end (79, 80). To aid these efforts, enclysis inhibition has the potential to prolong therapeutic Treg cell persistence in the liver by preventing their engulfment and degradation by donor liver hepatocytes. Table 2 summarizes current approaches of regulatory T cell therapies and shows how enclysis could act in preventing liver transplant rejection and GVHD.
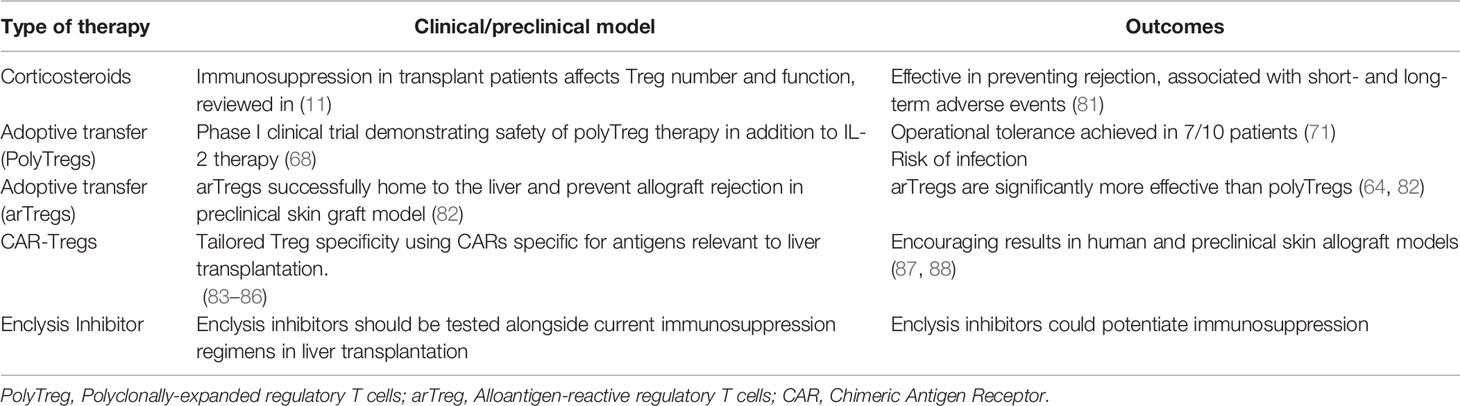
Table 2 Treg cell-focused approaches to prevent transplant rejection and graft versus host disease (GvHD).
Targeting Enclysis in Viral Infection
Hepatitis B virus (HBV) and hepatitis C virus (HCV) are hepatotropic viruses that are the leading causes of liver transplantation worldwide. They cause progressive liver damage after decades of infection that can promote a pro-tumorigenic environment and may lead to liver failure requiring a transplant (89, 90). Chronic infection with HBV and HCV account for approximately 80% of hepatocellular carcinoma (HCC) cases worldwide (91). Every year, 1.4 million people die from viral hepatitis-related cirrhosis and liver cancer (92). An early, broad, robust T cell response has been associated with viral clearance (93, 94), and regulatory T cell frequencies are thought to hamper antiviral responses and promote persistence (90).
Sprengers et al. showed a correlation between the levels of intrahepatic CD8+ T cells and the degree of liver damage in HBV, highlighting the concept that a balance between an immune response and tolerance is essential to ensure clearance of the virus, whilst limiting any liver cell injury leading to fibrosis (95). Although Treg cells suppress immune-mediated mechanisms of liver damage, the suppression of antiviral T cell responses, which are essential for the resolution of acute HBV infection, may promote viral persistence. Indeed, vigorous cytotoxic responses are crucial to control viral infections beyond the liver (96, 97), and transient depletion of Treg cells can boost cytotoxic T cell antiviral activity (98).
There are limited data on the immunological response to acute HBV and HCV infection in the liver because of the potential complications associated with standard liver biopsies and the difficulty in detecting an often-asymptomatic acute phase. Therefore, most studies of intrahepatic Treg cells in HBV and HCV have focused on the chronic phases of infection. Elevated Treg levels in chronic HCV infection were associated with limited immunopathological damage, suggesting a critical role for Tregs in controlling the chronic inflammatory response to HCV thus limiting hepatic damage (90, 99). However, Tregs have also been shown to inhibit CD8+ T cells in chronic infection in a nonspecific manner; as they were shown to also suppress CMV-, EBV-, and HCV-specific T cells (100, 101).
Patients who spontaneously cleared HCV infection had reduced Treg cell frequencies in their blood compared to chronically infected patients after clearance, however there is a paucity of information regarding liver Treg populations in these cohorts (100–103). In chimpanzees, the sole animal model for HCV immunity, Treg cells persisted after viral clearance and aided the maintenance of HCV-specific memory T cells by regulating proliferation, ex vivo effector functions, and activation-induced death of HCV-specific memory T cells (104).
Another important study in this field was the low dose IL-2 treatment of ten patients with HCV-induced vasculitis (105). Extrahepatic manifestations present in some HCV-infected people and these include mixed cryoglobulinaemia with reduced peripheral blood Treg cells (106). Low dose IL-2 restored blood Treg frequencies in these patients without increasing viraemia or liver enzymes, and 8/10 showed clinical improvement (105). In this setting, increase in functional peripheral Treg numbers did not induce a viral flare; it would be interesting to investigate if hepatic Treg frequencies were affected.
In the case of HBV, it is hypothesised that persistent infection leads to transforming growth factor β (TGF-β) production from hepatic stellate cells which contributes to the differentiation of conventional CD4+ T cells into induced Treg cells (107). In chronic HCV infection, elevated serum IL-10 levels are thought to play a role in the induction of Tregs (108, 109). To address whether viral infection would offer measurable immune suppression in the context of transplantation, Bohne et al. conducted a clinical trial of immunosuppression withdrawal in 34 HCV patients, 50% of whom achieved operational tolerance. The magnitude of HCV-induced proinflammatory gene expression and the breadth of anti-HCV effector T cell responses in these patients did not influence drug withdrawal outcome (110). The authors describe an overall restrained alloreactive immune landscape in HCV patients, and viral infection did not hinder the establishment of operational tolerance.
To clear infection in patients with chronic hepatitis, reducing Treg cell frequencies in the liver in a specific and transient way may provide a sufficient boost to antiviral immunity. Increasing enclysis with enhancer compounds may reduce Treg cell numbers in the liver without depleting Tregs from the circulation. Indeed, depletion of Tregs has been shown to increase the immune control of acute HBV early in infection as the hepatitis B antigens HBeAg and HBsAg were cleared considerably faster in the serum of Treg-depleted mice compared to control. Further, early elimination of Tregs in acute HBV infection was shown to improve the recruitment of macrophages and DCs into HBV-infected livers, aiding viral clearance (16, 111). Table 3 lists current therapies for viral hepatitis, and highlights how enclysis might be of benefit.
Targeting Enclysis in Liver Cancer
Primary liver cancer is the sixth most common type of cancer and is responsible for over 700,000 deaths annually, making it the fourth leading cause of cancer-related death worldwide (117). Hepatocellular carcinoma (HCC) is the most common malignancy in the liver, accounting for over 75% of all primary liver cancer cases (118). Malignancies in the liver, and particularly HCC, are characterized by high rates of recurrence and poor prognosis, owing mostly to the late presentation and thus late diagnosis of disease (119). As aforementioned, HBV and HCV infections are a substantial risk factor for HCC (120). Other risk factors include alcohol consumption leading to alcoholic liver disease and steatohepatitis as well as non-alcoholic fatty liver disease, associated with obesity and other metabolic disorders (121). Most HCC cases attributed to these risk factors develop in the context of cirrhosis in the liver, with up to 90% of HCC patients displaying liver cirrhosis pre-diagnosis (122).
The main treatments available for HCC, namely local ablation, surgical resection, or liver transplantation, are only viable if implemented at early stages of the disease. Thus, 80% of patients with HCC are not eligible for these treatments (123). For such patients, the first-line strategy for palliative treatment involves multi-kinase inhibitors. One such inhibitor is sorafenib, which is associated with severe adverse events (122, 124). Overall, there is a considerable unmet clinical need for patients that continue to progress or do not respond to systemic treatment. This emphasizes the need for new therapies for HCC.
It is well-established that chronic pathological inflammation is a key driver of HCC tumourigenesis in cirrhotic livers (125). Repeated and unresolved injury to hepatic tissues leads to the constitutive activation of a local immune response, resulting in the enhanced secretion of proinflammatory and mitogenic cytokines such as IL-6 and tumor necrosis factor α (TNF-α) (126). This, coupled with the subsequent recruitment of effector immune cells, results in a proinflammatory tissue microenvironment. The hypoxic environment caused by impaired blood flow to the liver further exacerbates inflammation in the cirrhotic liver. Such hostile environments drive hepatocyte apoptosis and increase the production of reactive oxygen species, driving hepatocellular mutagenesis and genomic instability, further potentiating the carcinogenic phenotype (122, 127).
The creation of a pro-inflammatory environment also stimulates the recruitment of regulatory immune cells. In HCC, tumor-infiltrating Tregs are recruited to the neoplastic site following enhanced secretion of the chemokines CCL17 and CCL2 by tumor associated macrophages (128). Once activated, these cells promote immune evasion through a variety of proposed immunosuppressive mechanisms, including the secretion of TGF-β and IL-10, the inhibition of dendritic cell activation and antigen presentation, and granzyme-dependent cytotoxicity (129). The constitutive expression of CTLA-4 is important for Treg immunosuppressive functionality, as its interaction with CD80 and CD86 ligands on the surface of dendritic cells impedes the antigen presenting cells’ activation by costimulatory molecules (130).
Different stages of HCC pathogenesis have been linked to distinct signatures of tumor-infiltrating immune cell populations (131). A large-scale analysis of tumor-infiltrating immune cells in 1090 HCC tumors revealed that greater intratumoural Treg populations were strongly correlated with poorer patient outcomes (132). This finding is consistent with several studies that also report an association between high levels of Treg infiltration and poor prognosis and response to treatment in a variety of different cancer types, including breast cancer, cervical cancer, and melanomas (133–135). In 2020, Yu et al. also reported a greater-than-3-fold increase in Treg populations in tumor tissues compared to adjacent healthy tissues, further implicating Tregs in HCC development (132). In another study, a higher proportion of Treg tumor infiltration in HCC tumors was also associated with poor tissue differentiation and advanced stages of hepatic fibrosis (136). Overall, it appears that elevated Treg numbers are associated with poor patient outcome. Thus, we propose that harnessing hepatocytes’ capacity for enclysis could provide a novel and specific mechanism of reducing Treg populations in HCC. Enhancing enclysis in the context of HCC could restrict Treg frequencies and alleviate local immunosuppression within the tumor microenvironment, aiming to reinstate tumor immunogenicity and suppress HCC tumourigenesis.
Treg cells may also augment immune dysfunction by inducing effector T cell exhaustion, characterised by inhibition of intratumoural CD8+ T cell expansion and activation (137). This exhaustive effect synergizes with neoplastic cells’ overexpression of programmed death-ligand 1 (PD-L1), activating effector T cells’ programmed cell death protein 1 (PD-1) pathway and further eliminating CD8+ T cells at tumor site (138). The various immunomodulatory mechanisms employed by Tregs contribute to the inhibition of effector immune cells in the tumor stroma, consequently depleting anti-tumor immunity and promoting immune evasion and tumor progression. Accordingly, enhanced Treg tumor infiltration was found to impede both the activation and recruitment of effector CD8+ T cells in patients with HCC (139). Tumor-infiltrating CD8+ T cells from advanced HCC tumor samples displayed significantly impaired secretion of the cytolytic enzymes perforin, granzyme A, and granzyme B. Specifically, Tregs extracted from patients with HCC were capable of significantly suppressing CD8+ T cells’ production of these cytolytic enzymes, in addition to inhibiting the secretion of the key anti-tumor cytokines IFN-γ and TNF-α in vitro (139). These findings support a direct role for Tregs in dampening anti-tumoral immune responses by diminishing effector T cell activation in patients with HCC.
Treg cells have been explored as a therapeutic target in a variety of different cancer types (128). These approaches mainly aim to restore and replenish intrinsic anti-tumor immune responses by eliminating the suppressive effect of Tregs within the tumor stroma. For example, in the context of melanoma, antibody blockade of CTLA-4 results in both a strong increase in both CD8+ and CD4+ T cell infiltration and a reduced proportion of intratumoural Tregs in vivo (140). Additionally, co-culture of CD8+ T cells extracted from HCC patients in vitro with Treg cells showed that Treg cells treated with anti-PD1 and anti-PD-L1 resulted in restoration of IFN-γ secretion compared to control Treg cells, which inhibited IFN-γ secretion and cytotoxicity of CD8+ T cells (141). Despite these findings, clinical trials of immune checkpoint monotherapy in patients with advanced HCC exhibited only modest improvements in patient outcomes. Treatment with the anti-CTLA-4 monoclonal antibody tremelimumab produced a less than 20% partial response rate in HCC patients (142). Furthermore, a phase III clinical study concluded that the anti PD-1 antibody nivolumab showed no superiority over sorafenib treatment in improving overall survival in patients with HCC (143), demonstrating that check point inhibition may not be sufficient to transcend liver intrinsic immune tolerance mechanisms.
Pharmacological enhancement of enclysis could be used as an adjuvant to checkpoint blockade immunotherapy, further depleting tumor-infiltrating Treg populations and thereby improving the efficacy of CTLA-4 and PD-1/PD-L1 blockade in patients with advanced HCC. As a natural liver-restricted process, targeting enclysis locally and transiently may boost anti-tumor responses in the presence or absence of check point inhibition or CAR-T cell therapy. Table 4 lists current HCC therapies that target regulatory T cells, and highlights how enclysis enhancement might potentiate their effects to eliminate tumors in the liver.
Pharmacological Interventions to Modify Enclysis
To target enclysis specifically, we must elucidate the mechanisms by which T cells are engulfed by hepatocytes, how these are specific for CD4+ T cells, and how Treg cells suffer a different fate to Teff cells (Figure 2). It is important to note that when considering ways to target enclysis, we must take into account housekeeping functions of hepatocytes such as phagocytosis, and prevent the modulation of these functions as a side-effect. To this end, broad endocytosis inhibitors would not be suitable to modulate enclysis.
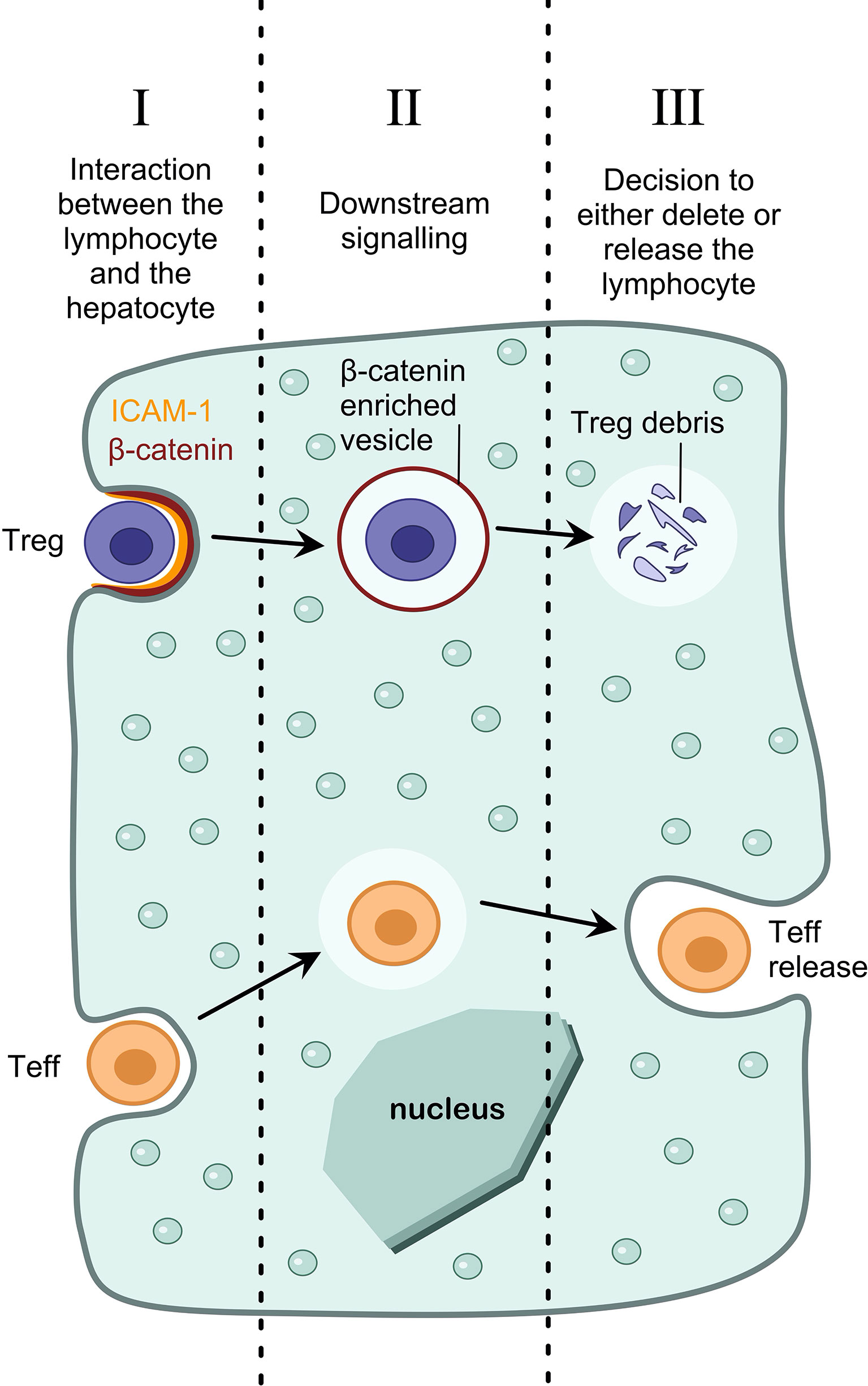
Figure 2 Pharmacological interventions to modulate enclysis. Understanding the mechanisms of enclysis reveals opportunities for therapeutic targeting. For example, understanding the interaction between the lymphocyte and the hepatocyte (I), the downstream signaling pathways that lead to T cell internalization (II), and the mechanism by which a hepatocyte decides the fate of the T cell (either deletion of regulatory T cells or survival and release of non-regulatory T cells) (III).
We have shown that ICAM-1 plays a role in early adhesion of T cells to hepatocytes, and inhibiting ICAM-1 using blocking antibodies reduces enclysis (19). Despite ICAM-1 playing a role in lymphocyte adhesion, it is unlikely that it mediates enclysis specificity, since ligands for ICAM-1 (e.g. β2 integrins), are also present on other lymphocytes such as CD8+ T cells and B cells, which are not subjected to enclytic capture. Enclysis receptor interactions between T cells and hepatocytes can be inhibited using blocking antibodies, or enhanced by treatment with soluble factors that upregulate surface expression of the putative receptor(s). By targeting molecules on hepatocytes, rather than T cells, we can avoid causing broad T cell dysfunction, which would echo functionally outside the liver resulting in extra-hepatic implications.
Additionally, by understanding the signaling cascade of events after Treg cell adhesion, which result in internalization, we could target enclysis specifically. For example, we have demonstrated that enclytic vesicles are enriched in beta-catenin, and not E-cadherin, although both are important for epithelial cell-in-cell structures in cancer cells (19, 24). It would be important to understand if Wnt/β-catenin signaling pathway (147) molecules play a role in the formation of the enclytic vesicle. Cell-permeable small molecules or growth factors are ideal to modulate signaling pathways.
Finally, we have demonstrated that hepatocytes are able to make a distinction between regulatory T cells (which results in their degradation) and helper T cells (which results in survival and release) (19). Understanding the mechanisms by which hepatocytes make this distinction will reveal new molecular targets for therapeutic intervention. For example, to increase the frequency of regulatory T cells in the liver, we could manipulate the hepatocyte such that it blocks Treg cell degradation in enclytic vesicles, and rather directs the cells towards a pathway reserved for the release of T helper cells.
Future Perspectives and Concluding Remarks
As Mujal and Krummel elegantly discussed (148), it is simplistic to consider immunity as an on/off switch between activation and tolerance. The outcome of complex immune responses is best described as a continuum, where synergistic effects from multiple cell types and processes protect from autoimmunity while permitting efficient viral clearance and tumor elimination. Regulatory T cell activity is part of this continuum, and we are beginning to understand how these cells can be employed most effectively in the tolerising microenvironment of the liver.
In viral infection, the liver needs to deal with abundant Treg populations swiftly to mount an effective immune response, thus Tregs cross the sinusoidal endothelial layers into the parenchyma. Hepatocytes engulf them by enclysis to rapidly tip the balance towards inflammation when needed. At the resolution of infection, the endothelial barriers are restored and Treg populations increase again to restore tolerance.
We described how inhibition of enclysis could increase frequencies of Tregs in AIH and in transplantation, where regulatory cell-targeting approaches have started to yield promising results. Conversely, we propose use of enclysis-enhancing drugs to promote Treg deletion in a well-controlled transient and liver-specific setting, to boost antiviral and anti-tumor responses where necessary (Figure 3). Small interventions such as enclysis modulation may be sufficient to restore healthy immune regulation in livers where chronic inflammation has caused immune dysfunction. This fits well with the “accommodation archetypes” described by Mujal and Krummel (148).
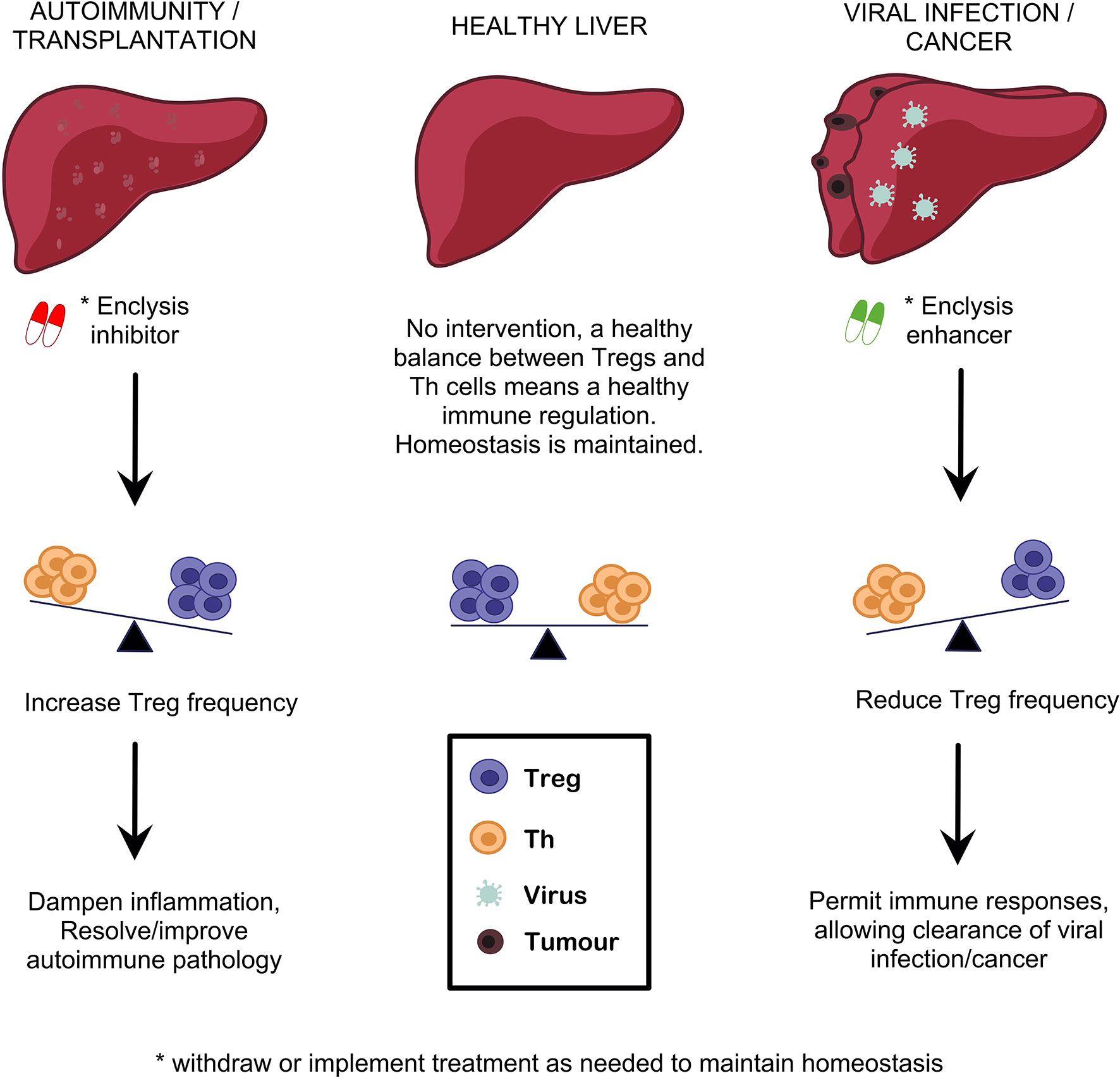
Figure 3 Targeting enclysis to restore immune regulation in autoimmune liver disease, transplantation, viral infection and liver cancer. Enclysis is a natural process with the potential to alter regulatory T cell frequencies specifically in the liver, and potentiate existing Treg therapies. We propose that enclysis inhibition may augment Treg abundance where most needed, to dampen overactive immune responses in autoimmunity or transplantation. Conversely, enclysis-enhancing compounds would aid local, transient elimination of Treg cells to jump-start exhausted antiviral or anti-tumor responses.
Author Contributions
YA, AY, JK, AB and ZS researched and composed the review. YA prepared the figures. SD provided helpful critique of the manuscript. YA and ZS finalized the review. All authors contributed to the article and approved the submitted version.
Funding
This work is supported by an MIBTP studentship to YA (BBSRC, UKRI, grant number BB/T00746X/1), an NC3R trainee postdoctoral fellowship to SD (UKRI) and an MRF intermediate career fellowship to ZS (UKRI, grant number NC/R002061/1) and an MRF intermediate career fellowship to ZS (UKRI, grant number MRF-169-0001-F-STAM-C0826).
Conflict of Interest
The authors declare that the research was conducted in the absence of any commercial or financial relationships that could be construed as a potential conflict of interest.
References
1. Liver Disease Crisis, British Liver Trust (2019). Available at: https://britishlivertrust.org.uk/about-us/media-centre/statistics/.
2. Williams R, Alexander G, Armstrong I, Baker A, Bhala N, Camps-Walsh G, et al. Disease burden and costs from excess alcohol consumption, obesity, and viral hepatitis: fourth report of the Lancet Standing Commission on Liver Disease in the UK. Lancet (2018) 391:1097–107. doi: 10.1016/S0140-6736(17)32866-0
3. Asrani SK, Devarbhavi H, . Eaton J, Kamath PS. Burden of liver diseases in the world. J Hepatol (2019) 70:151–71. doi: 10.1016/j.jhep.2018.09.014
4. Hepatitis B. World Health Organisation (2020). Available at: https://www.who.int/news-room/fact-sheets/detail/hepatitis-b.
5. Hepatitis C. World Health Organisation (2020). Available at: https://www.who.int/news-room/fact-sheets/detail/hepatitis-c.
6. Krassenburg LAP, Maan R, Ramji A, Manns MP, Cornberg M, Wedemeyer H, et al. Clinical outcomes following DAA therapy in patients with HCV-related cirrhosis depend on disease severity. J Hepatol (2020) 20:33805–8. doi: 10.1016/j.jhep.2020.11.021
7. Del Campo JA, . Gallego P, Grande L. Role of inflammatory response in liver diseases: Therapeutic strategies. World J Hepatol (2018) 10:1–7. doi: 10.4254/wjh.v10.i1.1
8. Richardson N, . Ng STH, Wraith DC. Antigen-Specific Immunotherapy for Treatment of Autoimmune Liver Diseases. Front Immunol (2020) 11:1586. doi: 10.3389/fimmu.2020.01586
9. Robinson MW, Harmon C, O’Farrelly C. Liver immunology and its role in inflammation and homeostasis. Cell Mol Immunol (2016) 13:267–76. doi: 10.1038/cmi.2016.3
10. Kubes P, Jenne C. Immune Responses in the Liver. Annu Rev Immunol (2018) 36:247–77. doi: 10.1146/annurev-immunol-051116-052415
11. Ferreira LMR, Muller YD, Bluestone JA, Tang Q. Next-generation regulatory T cell therapy. Nat Rev Drug Discovery (2019) 18:749–69. doi: 10.1038/s41573-019-0041-4
12. Bluestone JA, Tang Q. Treg cells-the next frontier of cell therapy. Science (2018) 362:154–5. doi: 10.1126/science.aau2688
13. Romano M, Fanelli G, Albany CJ, Giganti G, Lombardi G. Past, Present, and Future of Regulatory T Cell Therapy in Transplantation and Autoimmunity. Front Immunol (2019) 10:43. doi: 10.3389/fimmu.2019.00043
14. Esensten JH, Muller YD, Bluestone JA, Tang Q. Regulatory T-cell therapy for autoimmune and autoinflammatory diseases: The next frontier. J Allergy Clin Immunol (2018) 142:1710–8. doi: 10.1016/j.jaci.2018.10.015
15. Jeffery HC, Braitch MK, Brown S, Oo YH. Clinical Potential of Regulatory T Cell Therapy in Liver Diseases: An Overview and Current Perspectives. Front Immunol (2016) 7:334. doi: 10.3389/fimmu.2016.00334
16. Li W, Han J, Wu H. Regulatory T-cells promote hepatitis B virus infection and hepatocellular carcinoma progression. Chronic Dis Transl Med (2016) 2:67–80. doi: 10.1016/j.cdtm.2016.09.001
17. Ghazal K, Morales O, Barjon C, Dahlqvist G, Aoudjehane L, Ouaguia L, et al. Early high levels of regulatory T cells and T helper 1 may predict the progression of recurrent hepatitis C after liver transplantation. Clin Res Hepatol Gastroenterol (2019) 43:273–81. doi: 10.1016/j.clinre.2018.10.005
18. Azimi CS, Tang Q, Roybal KT, Bluestone JA. NextGen cell-based immunotherapies in cancer and other immune disorders. Curr Opin Immunol (2019) 59:79–87. doi: 10.1016/j.coi.2019.03.007
19. Davies SP, Reynolds GM, Wilkinson AL, Li X, Rose R, Leekha M, et al. Hepatocytes delete regulatory T cells by enclysis, a CD4+ T cell engulfment process. Cell Rep (2019) 29(6):1610–20.e4. doi: 10.1016/j.celrep.2019.09.068
20. Davies SP, Terry LV, Wilkinson AL, Stamataki Z. Cell-in-Cell Structures in the Liver: A Tale of Four E’s. Front Immunol (2020) 11:650. doi: 10.3389/fimmu.2020.00650
21. Davies SP, . Reynolds GM, Stamataki Z. Clearance of Apoptotic Cells by Tissue Epithelia: A Putative Role for Hepatocytes in Liver Efferocytosis. Front Immunol (2018) 9:44. doi: 10.3389/fimmu.2018.00044
22. Benseler V, Warren A, Vo M, Holz LE, Tay SS, Le Couteur DG, et al. Hepatocyte entry leads to degradation of autoreactive CD8 T cells. Proc Natl Acad Sci USA (2011) 108:16735–40. doi: 10.1073/pnas.1112251108
23. Overholtzer M, Brugge JS. The cell biology of cell-in-cell structures. Nat Rev Mol Cell Biol (2008) 9:796–809. doi: 10.1038/nrm2504
24. Overholtzer M, Mailleux AA, Mouneimne G, Normand G, Schnitt SJ, King RW, et al. A nonapoptotic cell death process, entosis, that occurs by cell-in-cell invasion. Cell (2007) 131:966–79. doi: 10.1016/j.cell.2007.10.040
25. Vesterhus M, Karlsen TH. Emerging therapies in primary sclerosing cholangitis: pathophysiological basis and clinical opportunities. J Gastroenterol (2020) 55:588–614. doi: 10.1007/s00535-020-01681-z
26. Carbone M, Milani C, Gerussi A, Ronca V, Cristoferi L, Invernizzi P. Primary biliary cholangitis: a multifaceted pathogenesis with potential therapeutic targets. J Hepatol (2020) 73:965–6. doi: 10.1016/j.jhep.2020.05.041
27. Gerussi A, Luca M, Cristoferi L, Ronca V, Mancuso C, Milani C, et al. New Therapeutic Targets in Autoimmune Cholangiopathies. Front Med (Lausanne) (2020) 7:117. doi: 10.3389/fmed.2020.00117
28. Webb GJ, Hirschfield GM, Krawitt EL, Gershwin ME. Cellular and Molecular Mechanisms of Autoimmune Hepatitis. Annu Rev Pathol (2018) 13:247–92. doi: 10.1146/annurev-pathol-020117-043534
29. Jeffery HC, Jeffery LE, Lutz P, Corrigan M, Webb GJ, Hirschfield GM, et al. Low-dose interleukin-2 promotes STAT-5 phosphorylation, T(reg) survival and CTLA-4-dependent function in autoimmune liver diseases. Clin Exp Immunol (2017) 188:394–411. doi: 10.1111/cei.12940
30. Trivedi PJ, Hubscher SG, Heneghan M, Gleeson D, Hirschfield GM. Grand round: Autoimmune hepatitis. J Hepatol (2019) 70:773–84. doi: 10.1016/j.jhep.2018.11.006
31. Grant CR, Holder BS, Liberal R, Heneghan MA, Ma Y, Mieli-Vergani G, et al. Immunosuppressive drugs affect interferon (IFN)-γ and programmed cell death 1 (PD-1) kinetics in patients with newly diagnosed autoimmune hepatitis. Clin Exp Immunol (2017) 189:71–82. doi: 10.1111/cei.12956
32. Heneghan MA, Yeoman AD, Verma S, . Smith AD, Longhi MS. Autoimmune hepatitis. Lancet (2013) 382:1433–44. doi: 10.1016/S0140-6736(12)62163-1
33. Liberal R, Vergani D, Mieli-Vergani G. Update on Autoimmune Hepatitis. J Clin Transl Hepatol (2015) 3:42–52. doi: 10.1002/cld.296
34. Pimpin L, Cortez-Pinto H, Negro F, Corbould E, Lazarus JV, Webber L, et al. Burden of liver disease in Europe: Epidemiology and analysis of risk factors to identify prevention policies. J Hepatol (2018) 69:718–35. doi: 10.1016/j.jhep.2018.05.011
36. Feld JJ, Dinh H, Arenovich T, Marcus VA, Wanless IR, Heathcote EJ. Autoimmune hepatitis: effect of symptoms and cirrhosis on natural history and outcome. Hepatology (2005) 42:53–62. doi: 10.1002/hep.20732
37. Kirstein MM, Metzler F, Geiger E, Heinrich E, Hallensleben M, . Manns MP, et al. Prediction of short- and long-term outcome in patients with autoimmune hepatitis. Hepatology (2015) 62:1524–35. doi: 10.1002/hep.27983
38. Crispe IN. Liver antigen-presenting cells. J Hepatol (2011) 54:357–65. doi: 10.1016/j.jhep.2010.10.005
39. Ichiki Y, Aoki CA, Bowlus CL, Shimoda S, Ishibashi H, Gershwin ME. T cell immunity in autoimmune hepatitis. Autoimmun Rev (2005) 4:315–21. doi: 10.1016/j.autrev.2005.01.005
40. Longhi MS, Ma Y, Mieli-Vergani G, Vergani D. Aetiopathogenesis of autoimmune hepatitis. J Autoimmun (2010) 34:7–14. doi: 10.1016/j.jaut.2009.08.010
41. Doycheva I, Watt KD, Gulamhusein AF. Autoimmune hepatitis: Current and future therapeutic options. Liver Int (2019) 39:1002–13. doi: 10.1111/liv.14062
42. Weiler-Normann C, Lohse AW. Autoimmune hepatitis: from immunopathogenesis to diagnostic and therapeutic innovation. Curr Opin Gastroenterol (2021) 37:86–90. doi: 10.1097/MOG.0000000000000701
43. Terry LV, Oo YH. The Next Frontier of Regulatory T Cells: Promising Immunotherapy for Autoimmune Diseases and Organ Transplantations. Front Immunol (2020) 11:565518. doi: 10.3389/fimmu.2020.565518
44. Lapierre P, Béland K, Yang R, Alvarez F. Adoptive transfer of ex vivo expanded regulatory T cells in an autoimmune hepatitis murine model restores peripheral tolerance. Hepatology (2013) 57:217–27. doi: 10.1002/hep.26023
45. Grant CR, Liberal R, Holder BS, Cardone J, Ma Y, Robson SC, et al. Dysfunctional CD39(POS) regulatory T cells and aberrant control of T-helper type 17 cells in autoimmune hepatitis. Hepatology (2014) 59:1007–15. doi: 10.1002/hep.26583
46. Wang H, Feng X, Yan W, Tian D. Regulatory T Cells in Autoimmune Hepatitis: Unveiling Their Roles in Mouse Models and Patients. Front Immunol (2020) 11:575572. doi: 10.3389/fimmu.2020.575572
47. Longhi MS, Hussain MJ, Mitry RR, Arora SK, Mieli-Vergani G, Ma Y. Functional study of CD4+CD25+ regulatory T cells in health and autoimmune hepatitis. J Immunol (2006) 176:4484–91. doi: 10.4049/jimmunol.176.7.4484
48. Liberal R, Grant CR, Holder BS, Cardone J, Martinez-Llordella M, Ma Y, et al. In autoimmune hepatitis type 1 or the autoimmune hepatitis-sclerosing cholangitis variant defective regulatory T-cell responsiveness to IL-2 results in low IL-10 production and impaired suppression. Hepatology (2015) 62:863–75. doi: 10.1002/hep.27884
49. Ferri S, Longhi MS, De Molo C, Lalanne C, Muratori P, Granito A, et al. A multifaceted imbalance of T cells with regulatory function characterizes type 1 autoimmune hepatitis. Hepatology (2010) 52:999–1007. doi: 10.1002/hep.23792
50. Longhi MS, Ma Y, Mitry RR, Bogdanos DP, Heneghan M, Cheeseman P, et al. Effect of CD4+ CD25+ regulatory T-cells on CD8 T-cell function in patients with autoimmune hepatitis. J Autoimmun (2005) 25:63–71. doi: 10.1016/j.jaut.2005.05.001
51. Longhi MS, Ma Y, Bogdanos DP, Cheeseman P, Mieli-Vergani G, Vergani D. Impairment of CD4(+)CD25(+) regulatory T-cells in autoimmune liver disease. J Hepatol (2004) 41:31–7. doi: 10.1016/j.jhep.2004.03.008
52. Liberal R, Grant CR, Holder BS, Ma Y, Mieli-Vergani G, Vergani D, et al. The impaired immune regulation of autoimmune hepatitis is linked to a defective galectin-9/tim-3 pathway. Hepatology (2012) 56:677–86. doi: 10.1002/hep.25682
53. Okumura A, Ishikawa T, Sato S, Yamauchi T, Oshima H, Ohashi T, et al. Deficiency of forkhead box P3 and cytotoxic T-lymphocyte-associated antigen-4 gene expressions and impaired suppressor function of CD4(+)CD25(+) T cells in patients with autoimmune hepatitis. Hepatol Res (2008) 38:896–903. doi: 10.1111/j.1872-034X.2008.00349.x
54. Vuerich M, Harshe R, Frank LA, Mukherjee S, Gromova B, Csizmadia E, et al. Altered aryl-hydrocarbon-receptor signalling affects regulatory and effector cell immunity in autoimmune hepatitis. J Hepatol (2021) 74:48–57. doi: 10.1016/j.jhep.2020.06.044
55. John K, Hardtke-Wolenski M, Jaeckel E, Manns MP, Schulze-Osthoff K, Bantel H. Increased apoptosis of regulatory T cells in patients with active autoimmune hepatitis. Cell Death Dis (2017) 8:3219. doi: 10.1038/s41419-017-0010-y
56. Peiseler M, Sebode M, Franke B, Wortmann F, Schwinge D, Quaas A, et al. FOXP3+ regulatory T cells in autoimmune hepatitis are fully functional and not reduced in frequency. J Hepatol (2012) 57:125–32. doi: 10.1016/j.jhep.2012.02.029
57. Lim TY, Martinez-Llordella M, Kodela E, Gray E, . Heneghan MA, Sanchez-Fueyo A. Low-Dose Interleukin-2 for Refractory Autoimmune Hepatitis. Hepatology (2018) 68:1649–52. doi: 10.1002/hep.30059
58. Rosenzwajg M, Lorenzon R, Cacoub P, Pham HP, Pitoiset F, El Soufi K, et al. Immunological and clinical effects of low-dose interleukin-2 across 11 autoimmune diseases in a single, open clinical trial. Ann Rheum Dis 78 (2019) 78(2):209–17. doi: 10.1136/annrheumdis-2018-214229
59. Buitrago-Molina LE, Pietrek J, Noyan F, Schlue J, Manns MP, Wedemeyer H, et al. Treg-specific IL-2 therapy can reestablish intrahepatic immune regulation in autoimmune hepatitis. J Autoimmun (2021) 117:102591. doi: 10.1016/j.jaut.2020.102591
60. Huang H, Deng Z. Adoptive transfer of regulatory T cells stimulated by Allogeneic Hepatic Stellate Cells mitigates liver injury in mice with concanavalin A-induced autoimmune hepatitis. Biochem Biophys Res Commun (2019) 512:14–21. doi: 10.1016/j.bbrc.2019.02.147
61. Liu Y, Yan W, Yuan W, Wang P, Huang D, . Luo X, et al. Treg/Th17 imbalance is associated with poor autoimmune hepatitis prognosis. Clin Immunol (2019) 198:79–88. doi: 10.1016/j.clim.2018.11.003
62. Holder BS, Grant CR, Liberal R, Ma Y, Heneghan MA, Mieli-Vergani G, et al. Retinoic acid stabilizes antigen-specific regulatory T-cell function in autoimmune hepatitis type 2. J Autoimmun (2014) 53:26–32. doi: 10.1016/j.jaut.2014.02.001
63. Liberal R, Grant CR, Yuksel M, Graham J, Kalbasi A, Ma Y, et al. D. Vergani, and Regulatory T-cell conditioning endows activated effector T cells with suppressor function in autoimmune hepatitis/autoimmune sclerosing cholangitis. Hepatology (2017) 66:1570–84. doi: 10.1002/hep.29307
64. Putnam AL, Safinia N, Medvec A, Laszkowska M, Wray M, Mintz MA, et al. Clinical grade manufacturing of human alloantigen-reactive regulatory T cells for use in transplantation. Am J Transplant (2013) 13:3010–20. doi: 10.1111/ajt.12433
65. Oo YH, Ackrill S, Cole R, Jenkins L, Anderson P, Jeffery HC, et al. Liver homing of clinical grade Tregs after therapeutic infusion in patients with autoimmune hepatitis. JHEP Rep (2019) 1:286–96. doi: 10.1016/j.jhepr.2019.08.001
66. Taubert R, Hardtke-Wolenski M, Noyan F, Wilms A, Baumann AK, Schlue J, et al. Intrahepatic regulatory T cells in autoimmune hepatitis are associated with treatment response and depleted with current therapies. J Hepatol (2014) 61:1106–14. doi: 10.1016/j.jhep.2014.05.034
67. Tang Q, Bluestone JA, Kang SM. CD4(+)Foxp3(+) regulatory T cell therapy in transplantation. J Mol Cell Biol (2012) 4:11–21. doi: 10.1093/jmcb/mjr047
68. Sanchez-Fueyo A, Whitehouse G, Grageda N, Cramp ME, Lim TY, Romano M, et al. Applicability, safety, and biological activity of regulatory T cell therapy in liver transplantation. Am J Transplant (2020) 20:1125–36. doi: 10.1111/ajt.15700
69. Oo YH, Sakaguchi S. Regulatory T-cell directed therapies in liver diseases. J Hepatol (2013) 59:1127–34. doi: 10.1016/j.jhep.2013.05.034
70. Thomson AW, Vionnet J, Sanchez-Fueyo A. Understanding, predicting and achieving liver transplant tolerance: from bench to bedside. Nat Rev Gastroenterol Hepatol (2020) 17:719–39. doi: 10.1038/s41575-020-0334-4
71. Todo S, Yamashita K, Goto R, Zaitsu M, Nagatsu A, Oura T, et al. A pilot study of operational tolerance with a regulatory T-cell-based cell therapy in living donor liver transplantation. Hepatology (2016) 64:632–43. doi: 10.1002/hep.28459
72. Whitehouse GP, Hope A, Sanchez-Fueyo A. Regulatory T-cell therapy in liver transplantation. Transpl Int (2017) 30:776–84. doi: 10.1111/tri.12998
73. Tang Q, Vincenti F. Transplant trials with Tregs: perils and promises. J Clin Invest (2017) 127:2505–12. doi: 10.1172/JCI90598
74. Sakaguchi S, Mikami N, Wing JB, Tanaka A, . Ichiyama K, Ohkura N. Regulatory T Cells and Human Disease. Annu Rev Immunol (2020) 38:541–66. doi: 10.1146/annurev-immunol-042718-041717
75. Raffin C, . Vo LT, Bluestone JA. Treg cell-based therapies: challenges and perspectives. Nat Rev Immunol (2020) 20:158–72. doi: 10.1038/s41577-019-0232-6
76. Giganti G, Atif M, Mohseni Y, Mastronicola D, Grageda N, Povoleri GA, et al. Treg cell therapy: How cell heterogeneity can make the difference. Eur J Immunol (2020) 51(1):39–55. doi: 10.1002/eji.201948131
77. Atif M, Conti F, Gorochov G, . Oo YH, Miyara M. Regulatory T cells in solid organ transplantation. Clin Transl Immunol (2020) 9:e01099. doi: 10.1002/cti2.1099
78. Gauthier JM, Harrison MS, Krupnick AS, Gelman AE, Kreisel D. The emerging role of regulatory T cells following lung transplantation. Immunol Rev (2019) 292:194–208. doi: 10.1111/imr.12801
79. Rosado-Sanchez I, Levings MK. Building a CAR-Treg: Going from the basic to the luxury model. Cell Immunol (2020) 358:104220. doi: 10.1016/j.cellimm.2020.104220
80. Dawson NAJ, Levings MK. Antigen-specific regulatory T cells: are police CARs the answer? Transl Res (2017) 187:53–8. doi: 10.1016/j.trsl.2017.06.009
81. Hussaini T, Turgeon RD, Partovi N, Erb SR, Scudamore CH, Yoshida EM. Immunosuppression Practices in Liver Transplantation: A Survey of North American Centers. Exp Clin Transplant (2018) 16:550–3. doi: 10.6002/ect.2017.0096
82. Sagoo P, Ali N, Garg G, Nestle FO, . Lechler RI, Lombardi G. Human regulatory T cells with alloantigen specificity are more potent inhibitors of alloimmune skin graft damage than polyclonal regulatory T cells. Sci Transl Med (2011) 3:83ra42. doi: 10.1126/scitranslmed.3002076
83. Kim YC, Zhang AH, Yoon J, Culp WE, Lees JR, . Wucherpfennig KW, et al. Engineered MBP-specific human Tregs ameliorate MOG-induced EAE through IL-2-triggered inhibition of effector T cells. J Autoimmun (2018) 92:77–86. doi: 10.1016/j.jaut.2018.05.003
84. Kim YC, Zhang AH, Su Y, Rieder SA, Rossi RJ, Ettinger RA, et al. Engineered antigen-specific human regulatory T cells: immunosuppression of FVIII-specific T- and B-cell responses. Blood (2015) 125:1107–15. doi: 10.1182/blood-2014-04-566786
85. Yeh W-I, Seay HR, Newby B, Posgai AL, Moniz FB, Michels A, et al. Avidity and Bystander Suppressive Capacity of Human Regulatory T Cells Expressing De Novo Autoreactive T-Cell Receptors in Type 1 Diabetes. Front Immunol (2017) 8. doi: 10.3389/fimmu.2017.01313
86. Brusko TM, Koya RC, Zhu S, Lee MR, Putnam AL, McClymont SA, et al. Human antigen-specific regulatory T cells generated by T cell receptor gene transfer. PloS One (2010) 5:e11726. doi: 10.1371/journal.pone.0011726
87. Boardman DA, Philippeos C, Fruhwirth GO, Ibrahim MA, Hannen RF, Cooper D, et al. Expression of a Chimeric Antigen Receptor Specific for Donor HLA Class I Enhances the Potency of Human Regulatory T Cells in Preventing Human Skin Transplant Rejection. Am J Transplant (2017) 17:931–43. doi: 10.1111/ajt.14185
88. Sicard A, Lamarche C, Speck M, Wong M, Rosado-Sanchez I, Blois M, et al. Donor-specific chimeric antigen receptor Tregs limit rejection in naive but not sensitized allograft recipients. Am J Transplant (2020) 20:1562–73. doi: 10.1111/ajt.15787
89. Rehermann B, Bertoletti A. Immunological aspects of antiviral therapy of chronic hepatitis B virus and hepatitis C virus infections. Hepatology (2015) 61:712–21. doi: 10.1002/hep.27323
90. Jung MK, Shin EC. Regulatory T Cells in Hepatitis B and C Virus Infections. Immune Netw (2016) 16:330–6. doi: 10.4110/in.2016.16.6.330
91. Yang JD, Hainaut P, Gores GJ, Amadou A, Plymoth A, Roberts LR. A global view of hepatocellular carcinoma: trends, risk, prevention and management. Nat Rev Gastroenterol Hepatol (2019) 16:589–604. doi: 10.1038/s41575-019-0186-y
92. Wiktor SZ, Hutin YJ. The global burden of viral hepatitis: better estimates to guide hepatitis elimination efforts. Lancet (2016) 388:1030–1. doi: 10.1016/S0140-6736(16)31018-2
93. Thimme R, Wieland S, Steiger C, Ghrayeb J, Reimann KA, Purcell RH, et al. CD8(+) T cells mediate viral clearance and disease pathogenesis during acute hepatitis B virus infection. J Virol (2003) 77:68–76. doi: 10.1128/JVI.77.1.68-76.2003
94. Shoukry NH, Grakoui A, Houghton M, Chien DY, Ghrayeb J, . Reimann KA, et al. Memory CD8+ T cells are required for protection from persistent hepatitis C virus infection. J Exp Med (2003) 197:1645–55. doi: 10.1084/jem.20030239
95. Sprengers D, van der Molen RG, Kusters JG, De Man RA, Niesters HG, Schalm SW, et al. Analysis of intrahepatic HBV-specific cytotoxic T-cells during and after acute HBV infection in humans. J Hepatol (2006) 45(2):182–9. doi: 10.1016/j.jhep.2005.12.022
96. Day CL, Kaufmann DE, Kiepiela P, Brown JA, Moodley ES, Reddy S, et al. PD-1 expression on HIV-specific T cells is associated with T-cell exhaustion and disease progression. Nature (2006) 443:350–4. doi: 10.1038/nature05115
97. Allen TM, Altfeld M, Geer SC, Kalife ET, Moore C, O’Sullivan K M, et al. Selective escape from CD8+ T-cell responses represents a major driving force of human immunodeficiency virus type 1 (HIV-1) sequence diversity and reveals constraints on HIV-1 evolution. J Virol (2005) 79:13239–49. doi: 10.1128/JVI.79.21.13239-13249.2005
98. Dietze KK, Zelinskyy G, Gibbert K, Schimmer S, Francois S, Myers L, et al. Transient depletion of regulatory T cells in transgenic mice reactivates virus-specific CD8+ T cells and reduces chronic retroviral set points. Proc Natl Acad Sci USA (2011) 108:2420–5. doi: 10.1073/pnas.1015148108
99. Xu D, Fu J, Jin L, Zhang H, Zhou C, Zou Z, et al. Circulating and liver resident CD4+CD25+ regulatory T cells actively influence the antiviral immune response and disease progression in patients with hepatitis B. J Immunol (2006) 177:739–47. doi: 10.4049/jimmunol.177.1.739
100. Rushbrook SM, Ward SM, Unitt E, Vowler SL, Lucas M, Klenerman P, et al. Regulatory T cells suppress in vitro proliferation of virus-specific CD8+ T cells during persistent hepatitis C virus infection. J Virol (2005) 79:7852–9. doi: 10.1128/JVI.79.12.7852-7859.2005
101. Boettler T, Spangenberg HC, Neumann-Haefelin C, Panther E, Urbani S, Ferrari C, et al. T cells with a CD4+CD25+ regulatory phenotype suppress in vitro proliferation of virus-specific CD8+ T cells during chronic hepatitis C virus infection. J Virol 79 (2005) 79(12):7860–7. doi: 10.1128/JVI.79.12.7860-7867.2005
102. Cabrera R, Tu Z, Xu Y, Firpi RJ, Rosen HR, Liu C, et al. An immunomodulatory role for CD4(+)CD25(+) regulatory T lymphocytes in hepatitis C virus infection. Hepatology (2004) 40:1062–71. doi: 10.1002/hep.20454
103. Sugimoto K, Ikeda F, Stadanlick J, Nunes FA, . Alter HJ, Chang KM. Suppression of HCV-specific T cells without differential hierarchy demonstrated ex vivo in persistent HCV infection. Hepatology (2003) 38:1437–48. doi: 10.1016/j.hep.2003.09.026
104. Manigold T, Shin EC, Mizukoshi E, Mihalik K, Murthy KK, Rice CM, et al. Foxp3+CD4+CD25+ T cells control virus-specific memory T cells in chimpanzees that recovered from hepatitis C. Blood (2006) 107:4424–32. doi: 10.1182/blood-2005-09-3903
105. Saadoun D, Rosenzwajg M, Joly F, Six A, Carrat F, Thibault V, et al. Regulatory T-cell responses to low-dose interleukin-2 in HCV-induced vasculitis. N Engl J Med (2011) 365:2067–77. doi: 10.1056/NEJMoa1105143
106. Boyer O, Saadoun D, Abriol J, Dodille M, Piette JC, Cacoub P, et al. CD4+CD25+ regulatory T-cell deficiency in patients with hepatitis C-mixed cryoglobulinemia vasculitis. Blood (2004) 103:3428–30. doi: 10.1182/blood-2003-07-2598
107. Yang G, Liu A, Xie Q, Guo TB, Wan B, Zhou B, et al. Association of CD4+CD25+Foxp3+ regulatory T cells with chronic activity and viral clearance in patients with hepatitis B. Int Immunol (2007) 19:133–40. doi: 10.1093/intimm/dxl130
108. MacDonald AJ, Duffy M, Brady MT, McKiernan S, Hall W, Hegarty J, et al. CD4 T helper type 1 and regulatory T cells induced against the same epitopes on the core protein in hepatitis C virus-infected persons. J Infect Dis (2002) 185:720–7. doi: 10.1086/339340
109. Cusick MF, Schiller JJ, Gill JC, Eckels DD. Hepatitis C virus induces regulatory T cells by naturally occurring viral variants to suppress T cell responses. Clin Dev Immunol (2011) 2011:806061. doi: 10.1155/2011/806061
110. Bohne F, Londono MC, Benitez C, Miquel R, Martinez-Llordella M, Russo C, et al. HCV-induced immune responses influence the development of operational tolerance after liver transplantation in humans. Sci Transl Med (2014) 6:242ra81. doi: 10.1126/scitranslmed.3008793
111. Stross L, Günther J, Gasteiger G, Asen T, Graf S, Aichler M, et al. Foxp3+ regulatory T cells protect the liver from immune damage and compromise virus control during acute experimental hepatitis B virus infection in mice. Hepatology (2012) 56:873–83. doi: 10.1002/hep.25765
112. Fanning GC, Zoulim F, Hou J, Bertoletti A. Therapeutic strategies for hepatitis B virus infection: towards a cure. Nat Rev Drug Discovery (2019) 18:827–44. doi: 10.1038/s41573-019-0037-0
113. Sung PS, Shin EC. Immunological Mechanisms for Hepatocellular Carcinoma Risk after Direct-Acting Antiviral Treatment of Hepatitis C Virus Infection. J Clin Med (2021) 10. doi: 10.3390/jcm10020221
114. Boni C, Janssen HLA, Rossi M, Yoon SK, Vecchi A, Barili V, et al. Combined GS-4774 and Tenofovir Therapy Can Improve HBV-Specific T-Cell Responses in Patients With Chronic Hepatitis. Gastroenterology (2019) 157:227–241 e7. doi: 10.1053/j.gastro.2019.03.044
115. Saadoun D, Pol S, Ferfar Y, Alric L, Hezode C, Si Ahmed SN, et al. Efficacy and Safety of Sofosbuvir Plus Daclatasvir for Treatment of HCV-Associated Cryoglobulinemia Vasculitis. Gastroenterology (2017) 153:49–52 e5. doi: 10.1053/j.gastro.2017.03.006
116. Tian Z, Cao X, . Chen Y, Lyu Q. Regional immunity in tissue homeostasis and diseases. Sci China Life Sci (2016) 59:1205–9. doi: 10.1007/s11427-016-0351-y
117. Bray F, Ferlay J, Soerjomataram I, Siegel RL, Torre LA, Jemal A. Global cancer statistics 2018: GLOBOCAN estimates of incidence and mortality worldwide for 36 cancers in 185 countries. CA Cancer J Clin (2018) 68:394–424. doi: 10.3322/caac.21492
118. Affo S, . Yu LX, Schwabe RF. The Role of Cancer-Associated Fibroblasts and Fibrosis in Liver Cancer. Annu Rev Pathol (2017) 12:153–86. doi: 10.1146/annurev-pathol-052016-100322
119. Patel N, . Yopp AC, Singal AG. Diagnostic delays are common among patients with hepatocellular carcinoma. J Natl Compr Canc Netw (2015) 13:543–9. doi: 10.6004/jnccn.2015.0074
120. Plummer M, de Martel C, Vignat J, Ferlay J, Bray F, Franceschi S. Global burden of cancers attributable to infections in 2012: a synthetic analysis. Lancet Glob Health (2016) 4:e609–16. doi: 10.1016/S2214-109X(16)30143-7
121. Pocha C, Xie C. Hepatocellular carcinoma in alcoholic and non-alcoholic fatty liver disease-one of a kind or two different enemies? Transl Gastroenterol Hepatol (2019) 4:72. doi: 10.21037/tgh.2019.09.01
122. O’Rourke JM, Sagar VM, Shah T, Shetty S. Carcinogenesis on the background of liver fibrosis: Implications for the management of hepatocellular cancer. World J Gastroenterol (2018) 24:4436–47. doi: 10.3748/wjg.v24.i39.4436
123. Davila JA, Duan Z, McGlynn KA, El-Serag HB. Utilization and outcomes of palliative therapy for hepatocellular carcinoma: a population-based study in the United States. J Clin Gastroenterol (2012) 46:71–7. doi: 10.1097/MCG.0b013e318224d669
124. Likhitsup A, Razumilava N, Parikh ND. Treatment for Advanced Hepatocellular Carcinoma: Current Standard and the Future. Clin Liver Dis (Hoboken) (2019) 13:13–9. doi: 10.1002/cld.782
125. Tarao K, Nozaki A, Ikeda T, Sato A, Komatsu H, Komatsu T, et al. Real impact of liver cirrhosis on the development of hepatocellular carcinoma in various liver diseases-meta-analytic assessment. Cancer Med (2019) 8:1054–65. doi: 10.1002/cam4.1998
126. Ramakrishna G, Rastogi A, Trehanpati N, Sen B, Khosla R, Sarin SK. From cirrhosis to hepatocellular carcinoma: new molecular insights on inflammation and cellular senescence. Liver Cancer (2013) 2:367–83. doi: 10.1159/000343852
127. Takaki A, Yamamoto K. Control of oxidative stress in hepatocellular carcinoma: Helpful or harmful? World J Hepatol (2015) 7:968–79. doi: 10.4254/wjh.v7.i7.968
128. Pinato DJ, Guerra N, Fessas P, Murphy R, Mineo T, Mauri FA, et al. Immune-based therapies for hepatocellular carcinoma. Oncogene (2020) 39:3620–37. doi: 10.1038/s41388-020-1249-9
129. Wang Y, Ma Y, Fang Y, Wu S, Liu L, Fu D, et al. Regulatory T cell: a protection for tumour cells. J Cell Mol Med (2012) 16:425–36. doi: 10.1111/j.1582-4934.2011.01437.x
130. Qureshi OS, Zheng Y, Nakamura K, Attridge K, Manzotti C, Schmidt EM, et al. Trans-endocytosis of CD80 and CD86: a molecular basis for the cell-extrinsic function of CTLA-4. Science (2011) 332:600–3. doi: 10.1126/science.1202947
131. Budhu A, Forgues M, Ye QH, Jia HL, He P, Zanetti KA, et al. Prediction of venous metastases, recurrence, and prognosis in hepatocellular carcinoma based on a unique immune response signature of the liver microenvironment. Cancer Cell (2006) 10:99–111. doi: 10.1016/j.ccr.2006.06.016
132. Yu S, Wang Y, Hou J, Li W, Wang X, Xiang L, et al. Tumor-infiltrating immune cells in hepatocellular carcinoma: Tregs is correlated with poor overall survival. PloS One (2020) 15:e0231003. doi: 10.1371/journal.pone.0231003
133. Zhou Y, Shao N, Aierken N, Xie C, Ye R, Qian X, et al. Prognostic value of tumor-infiltrating Foxp3+ regulatory T cells in patients with breast cancer: a meta-analysis. J Cancer (2017) 8:4098–105. doi: 10.7150/jca.21030
134. Shang B, Liu Y, . Jiang S-j, Liu Y. Prognostic value of tumor-infiltrating FoxP3+ regulatory T cells in cancers: a systematic review and meta-analysis. Sci Rep (2015) 5:15179. doi: 10.1038/srep15179
135. Leslie C, Bowyer SE, White A, Grieu-Iacopetta F, Trevenen M, Iacopetta B, et al. FOXP3+ T regulatory lymphocytes in primary melanoma are associated with BRAF mutation but not with response to BRAF inhibitor. Pathology (2015) 47:557–63. doi: 10.1097/PAT.0000000000000314
136. Kurebayashi Y, Ojima H, Tsujikawa H, Kubota N, Maehara J, Abe Y, et al. Landscape of immune microenvironment in hepatocellular carcinoma and its additional impact on histological and molecular classification. Hepatology (2018) 68:1025–41. doi: 10.1002/hep.29904
137. Lugade AA, Kalathil S, Miller A, . Iyer R, Thanavala Y. High immunosuppressive burden in advanced hepatocellular carcinoma patients: Can effector functions be restored? Oncoimmunology (2013) 2:e24679. doi: 10.4161/onci.24679
138. Penaloza-MacMaster P. CD8 T-cell regulation by T regulatory cells and the programmed cell death protein 1 pathway. Immunology (2017) 151:146–53. doi: 10.1111/imm.12739
139. Fu J, Xu D, Liu Z, Shi M, Zhao P, Fu B, et al. Increased regulatory T cells correlate with CD8 T-cell impairment and poor survival in hepatocellular carcinoma patients. Gastroenterology (2007) 132:2328–39. doi: 10.1053/j.gastro.2007.03.102
140. Curran MA, Montalvo W, Yagita H, Allison JP. PD-1 and CTLA-4 combination blockade expands infiltrating T cells and reduces regulatory T and myeloid cells within B16 melanoma tumors. Proc Natl Acad Sci (2010) 107:4275–80. doi: 10.1073/pnas.0915174107
141. Langhans B, Nischalke HD, Krämer B, Dold L, Lutz P, Mohr R, et al. Role of regulatory T cells and checkpoint inhibition in hepatocellular carcinoma. Cancer Immunol Immunother (2019) 68:2055–66. doi: 10.1007/s00262-019-02427-4
142. Sangro B, Gomez-Martin C, de la Mata M, Iñarrairaegui M, Garralda E, Barrera P, et al. A clinical trial of CTLA-4 blockade with tremelimumab in patients with hepatocellular carcinoma and chronic hepatitis C. J Hepatol (2013) 59:81–8. doi: 10.1016/j.jhep.2013.02.022
143. Sangro B, Park J-W, Cruz CMD, Anderson J, Lang L, Neely J, et al. A randomized, multicenter, phase 3 study of nivolumab vs sorafenib as first-line treatment in patients (pts) with advanced hepatocellular carcinoma (HCC): CheckMate-459. J Clin Oncol (2016) 34:TPS4147–TPS4147. doi: 10.1200/JCO.2016.34.15_suppl.TPS4147
144. Kalathil SG, Hutson A, Barbi J, Iyer R, Thanavala Y. Augmentation of IFN-gamma+ CD8+ T cell responses correlates with survival of HCC patients on sorafenib therapy. JCI Insight (2019) 4. doi: 10.1172/jci.insight.130116
145. Saleh R, Elkord E. Treg-mediated acquired resistance to immune checkpoint inhibitors. Cancer Lett (2019) 457:168–79. doi: 10.1016/j.canlet.2019.05.003
146. Srivastava S, Riddell SR. Chimeric Antigen Receptor T Cell Therapy: Challenges to Bench-to-Bedside Efficacy. J Immunol (2018) 200:459–68. doi: 10.4049/jimmunol.1701155
147. Li X, Xiang Y, Li F, Yin C, Li B, . Ke X. WNT/beta-Catenin Signaling Pathway Regulating T Cell-Inflammation in the Tumor Microenvironment. Front Immunol (2019) 10:2293. doi: 10.3389/fimmu.2019.02293
Keywords: enclysis, hepatitis, transplantation, liver autoimmunity, regulatory T cells (Treg), immune regulation, tolerance, liver cancer
Citation: Aghabi YO, Yasin A, Kennedy JI, Davies SP, Butler AE and Stamataki Z (2021) Targeting Enclysis in Liver Autoimmunity, Transplantation, Viral Infection and Cancer. Front. Immunol. 12:662134. doi: 10.3389/fimmu.2021.662134
Received: 31 January 2021; Accepted: 22 March 2021;
Published: 19 April 2021.
Edited by:
Marco Carbone, University of Milano-Bicocca, ItalyReviewed by:
Gregor Ebert, Walter and Eliza Hall Institute of Medical Research, AustraliaKathrin Sutter, University of Duisburg-Essen, Germany
Copyright © 2021 Aghabi, Yasin, Kennedy, Davies, Butler and Stamataki. This is an open-access article distributed under the terms of the Creative Commons Attribution License (CC BY). The use, distribution or reproduction in other forums is permitted, provided the original author(s) and the copyright owner(s) are credited and that the original publication in this journal is cited, in accordance with accepted academic practice. No use, distribution or reproduction is permitted which does not comply with these terms.
*Correspondence: Zania Stamataki, ei5zdGFtYXRha2lAYmhhbS5hYy51aw==