- 1Department of Molecular Genetics, National Institute of Oncology, Budapest, Hungary
- 2Hereditary Tumors Research Group, Eötvös Loránd Research Network—Semmelweis University, Budapest, Hungary
- 3Department of Laboratory Medicine, Semmelweis University, Budapest, Hungary
The ongoing COVID-19 pandemic caused by the novel coronavirus, SARS-CoV-2 has affected all aspects of human society with a special focus on healthcare. Although older patients with preexisting chronic illnesses are more prone to develop severe complications, younger, healthy individuals might also exhibit serious manifestations. Previous studies directed to detect genetic susceptibility factors for earlier epidemics have provided evidence of certain protective variations. Following SARS-CoV-2 exposure, viral entry into cells followed by recognition and response by the innate immunity are key determinants of COVID-19 development. In the present review our aim was to conduct a thorough review of the literature on the role of single nucleotide polymorphisms (SNPs) as key agents affecting the viral entry of SARS-CoV-2 and innate immunity. Several SNPs within the scope of our approach were found to alter susceptibility to various bacterial and viral infections. Additionally, a multitude of studies confirmed genetic associations between the analyzed genes and autoimmune diseases, underlining the versatile immune consequences of these variants. Based on confirmed associations it is highly plausible that the SNPs affecting viral entry and innate immunity might confer altered susceptibility to SARS-CoV-2 infection and its complex clinical consequences. Anticipating several COVID-19 genomic susceptibility loci based on the ongoing genome wide association studies, our review also proposes that a well-established polygenic risk score would be able to clinically leverage the acquired knowledge.
Introduction
Severe acute respiratory syndrome coronavirus-2 (SARS-CoV-2), the virus responsible for the ongoing pandemic COVID-19 has yet infected more than 108 million people worldwide with a reported mortality rate between 0.5 and 10% in different countries (1). SARS-CoV-2 is a novel coronavirus originally detected in China. The specific mechanism by which it infects humans and effects human health is not fully understood. The clinical characteristics of COVID-19 usually incorporates fever, fatigue, dry cough, and dyspnea, while severe infections may result in bilateral pneumonia, and life-threatening acute respiratory distress syndrome (ARDS). Although severe complications usually manifest in elder patients with concurrent chronic diseases (e.g., high blood pressure, diabetes) young, healthy individuals might also suffer from critical consequences of the disease, requiring intensive care. The wide range of disease susceptibility especially in younger patients suggests that difference in genetic background of individuals might contribute to these alterations. In fact, the analysis of previous, unrelated infectious diseases provides clear evidence that specific protective genetic variations are enriched in populations where certain infections are endemic. For instance, sickle cell trait and carrying specific HLA antigens in African populations confer diminished susceptibility against malaria infection (2, 3). Another example, Δ32, a 32-base pair deletion of the CCR5 gene prevents cellular viral entry of human immunodeficiency virus (HIV) resulting in effective resistance against HIV infection in individuals homozygous regarding this variation (4).
In the present review we aim to summarize previously published genotype-phenotype studies of genes which might play a role in the susceptibility to COVID-19. The associations between various single nucleotide polymorphisms (SNPs) and certain traits were studied using targeted and genome-wide approaches. In the case of targeted approach, hypothesis-driven selection of specific genes/SNPs were analyzed in cases and controls while during genome-wide association studies (GWASs) detection of novel genomic loci with susceptibility to various traits/diseases are possible. Our examination focuses on genetic variants of 2 key processes in the initiation of the disease: viral entry and recognition and response by the innate immune system. Also, as several international collaborations are ongoing to provide large-scale genomic susceptibility data, we propose that a well-established polygenic risk score would be able to optimally leverage the acquired knowledge.
Viral Entry
Large emphasis has been directed to decipher how SARS-CoV-2 is incorporated in human cells. Key data in this regard originate from studies focusing on SARS-CoV, responsible for the SARS epidemic of 2002–2003, which shares 79.6% sequence identity with SARS-CoV-2 (5). In fact, the spike protein of SARS-CoV binds to angiotensin-converting enzyme 2 (ACE2) that serves as a receptor for the virus (6), and recent data confirmed that SARS-CoV-2 also binds ACE2 in vitro (7–9). Further analyses revealed that the spike protein of SARS-CoV-2 is cleaved by transmembrane protease serine 2 (TMPRSS2) (7), facilitating viral entry. Also of note, both ACE2 and TMPRSS2 are primarily expressed in bronchial transient secretory cells (10), elucidating the predilection of the lower airways. Additionally, proprotein convertase FURIN was shown to pre-activate the viral entry of SARS-CoV-2 (11), while additional factors as PIKfyve, TPCN2 and cathepsin L (CTSL) are also critical in this process (12).
Table 1 summarizes genetic variants of the aforementioned genes with suggested genotype-phenotype findings. The main physiological function of ACE2 is catalyzing the hydrolysis of angiotensin I and angiotensin II into angiotensin (1–9) and angiotensin (1–7), respectively, contributing to blood pressure regulation (34). Therefore, numerous SNP association studies were directed to ascertain the role of ACE2 genetic variants on certain cardiovascular and metabolic traits. Throughout several populations, ACE2 polymorphisms have been associated with susceptibility to cardiovascular and metabolic diseases including hypertension and type 2 diabetes mellitus underlining the potential functional impact of these SNPs on ACE2 expression and/or function (13–21). A TMPRSS2 SNP has been linked to TMPRSS2-ERG genetic fusion which is a frequent molecular event in prostate cancer (22, 23). More importantly, a study examining patients of the 2009 swine flu pandemic caused by the H1N1 influenza virus found that TMPRSS2 SNP rs2070788 is associated with severity of the disease (24). Additionally, genotype-specific TMPRSS2 expression was confirmed in human lung tissues regarding rs2070788 and rs383510, the latter being tagged to the former polymorphism. Mechanistically, rs383510 was found to enhance the transcription of TMPRSS2 mRNA, and these 2 SNPs were also found to associate with susceptibility to the H7N9 influenza virus (24).
Certain high throughput screening studies identified rs4702, a common genetic variant of proprotein convertase FURIN as susceptibility factor for schizophrenia and hypertension (25, 26), while other studies correlated another SNP rs17514846 with other various traits including coronary artery disease, metabolic syndrome and longevity (27–29).
While we found no SNP association studies for PIKfyve, certain variants of the TPCN2 gene coding for cation-selective ion channel were found to be associated with type 2 diabetes mellitus (T2DM) and hair color (30, 31). In the case of CTSL, two studies performed on different populations confirmed that a promoter polymorphism correlates with hypertension in Asian and American populations (32, 33).
Innate Immunity
After SARS-CoV-2 successfully infected cells, a complex immune response initiates, in which the rapid and coordinated response of the innate immunity is pre-requisite (35). Following infection, the innate immune system recognizes viral antigens mainly by RIG-I-Like Receptors (RLRs) and Toll-Like Receptors (TLRs) (35). In the first step in RLR-dependent immune response, cytoplasmic RNA sensors RIG-I and MDA5 recognize viral RNA, after which interaction with mitochondrial antiviral signaling protein (MAVS) initiate signaling changes activating interferon regulatory factor IRF3 and IRF7, resulting in type I IFN (IFN-α and IFN-β) production and antiviral response (35–38).
Supplementary Table 1 summarizes the SNP association studies concerning the agents implicated in viral recognition and response by the innate immune system. Several RIG-I SNPs were found to be associated with neutralizing antibody levels after measles and rubella vaccinations while other studies found RIG-I SNPs to be associated with nasopharyngeal carcinoma and EV71-induced hand, foot, and mouth disease (39–43). MDA5 genetic variants were thoroughly investigated in relation to autoimmunity with several associations being found with psoriasis, systemic lupus erythematosus (SLE), type 1 diabetes mellitus (T1DM), hypothyroidism and multiple sclerosis (MS) (44–53). Polymorphisms in MAVS were analyzed regarding inflammatory response finding that rs7269320 associated with osteoarthritis (54). Moreover, in an African American cohort, where rs11905552 of the MAVS gene was much more frequent compared to European Americans, this SNP associated with low type I IFN production in patients with SLE (55). Studies focusing on genetic variants of IRF3 and IRF7 found associations with SLE and systemic sclerosis (56–59), while IFN-α genetic variants were found to be associated with mixed connective tissue disease and prognosis in glioma patients (60, 61).
During TLR-mediated immune response, TLR3, TLR7, TLR8, and TLR9 sense intracellular, while TLR2 and TLR4 detect extracellular, cell surface-associated viral antigens (35, 62). TLRs transduce the signal by binding MyD88 and TRIF, which in turn stimulate IRF3, IRF7, and NF-κB enhancing type I IFN response (35, 63–65).
A multitude of case-control studies analyzed the role of TLR-associated SNPs in health and disease. TLR3 SNPs were associated with infectious, autoimmune, and neoplastic diseases. Certain studies demonstrated association of TLR3 polymorphisms with hepatitis B and C virus (HBV and HCV), herpes simplex virus (HSV), HIV infections (66–72), while SNPs rs3775291, rs3775292, rs5743312, and rs7657186 were associated with vaccine-induced immunity to serogroup C meningococcal vaccine as defined by virus-specific IgG persistence (73). rs3775291 was also associated with various autoimmune disorders including SLE, rheumatoid arthritis (RA), and sarcoidosis (74–76). Regarding neoplastic diseases, TLR3 genetic variants were linked to breast, colorectal and nasopharyngeal cancers, while also serving as prognostic factors in colorectal cancer (CRC) and melanoma malignum (MM) (42, 77–81). Several lines of evidence supported the association between SLE and TLR7 SNPs in various populations (82–85), while additional studies found correlation between TLR7 polymorphisms and susceptibility to HCV and chikungunya virus infection, asthma, and age-related macular degeneration (86–89). The association between rs3764880 of TLR8 and tuberculosis susceptibility in males has been confirmed in European, Russian, and Chinese populations (90–92) and other SNPs of TLR8 were associated with asthma, SLE, and chikungunya virus infection (84, 87, 88). With regard to the fourth TLR sensing intracellular viral antigens, TLR9 has 4 SNPs which were found to be associated with several infectious, autoimmune, and neoplastic diseases. Confirmed associations with infectious diseases include malaria, cytomegalovirus (CMV), and tuberculosis (90, 93–97), while individuals with certain TLR9 polymorphisms are more susceptible to post-infectious irritable bowel syndrome, SLE and lupus nephritis, Graves' disease-related ophthalmopathy, and RA (98–104). With respect to neoplastic diseases, acute myeloid leukemia (AML), and cervical cancer were also associated with TLR9 genetic variants (105–107), while rs187084 is proposed to be a prognostic factor in patients with prostate cancer (108).
TLR2 and TLR4 SNPs are probably the most widely investigated genetic variants in the scope of our review. Similarly to studies conducted in other TLR genes, TLR2 polymorphisms were also found to be associated with tuberculosis and CMV infection (90, 109–111), and additional pathogenic role concerning bacterial vaginosis in HIV-infected patients, recurrent vulvovaginal candidiasis, aggressive periodontitis, neonatal sepsis, Lyme disease, pneumonia, and reactive arthritis were also proposed (112–119). SNP rs3804100 has been linked to measles-specific antibody levels following immunization, while rs5743708 associated with nasal Staphylococcus aureus carriage (120, 121). Autoimmune disorders linked to TLR2 SNPs incorporate psoriasis and T1DM (122, 123), while hepatocellular carcinoma (HCC), marginal zone lymphoma, oral, and laryngeal squamous cell carcinoma and prognosis of women with breast cancer have also been linked to certain genetic variants of TLR2 (124–127).
TLR4 polymorphisms have been associated with various infectious diseases. Manifest tuberculosis is associated with rs11536889, rs12377632, rs1927911, and rs7873784 (109, 110, 128), while additional associated infection-related diseases include sepsis and sepsis-related organ failure for rs11536889 and Chlamydia trachomatis infection in women with pelvic inflammatory disease for rs1927911 (129–131). The most intensively investigated TLR4 SNP, rs4986790 is associated with a wide range of infections including Gram-negative and Mycobacterium bacteria in high-risk populations, severe respiratory syncytial virus disease, clinical malaria, recurrent cystitis, chronic cavitary pulmonary aspergillosis, HCV infection, and prognosis of HBV-infected individuals (132–141). It also has a probable effect on IL-4 secretion after measles vaccination (142). Another SNP of TLR4, rs5030717 is associated with childhood otitis media (143).
With respect to autoimmune-related diseases, TLR4 SNPs associate with ankylosing spondylitis, RA, giant cell arteritis, and preeclampsia (144–147). In addition, rs10759932 and rs4986790 are linked to acute rejection following kidney and lung transplantation, respectively (148, 149). Several TLR4 polymorphisms (rs10759932, rs10983755, rs11536889, rs1927911, rs2149356, and rs4986790) are associated with gastric cancer susceptibility, where the risk-elevating Helicobacter pylori infection might have an important role (150–154). Other tumors linked to TLR4 genetic variants include HCC, prostate cancer, CRC, and non-Hodgkin lymphoma (NHL) (79, 155–160).
Genetic variants of adapter molecule MYD88 are associated with tuberculosis susceptibility, Buerger disease and treatment response in patients with RA (90, 161–163). SNPs of the other key adapter agent, TRIF are associated with pneumonia susceptibility and thyroid cancer (164, 165).
In addition to type I IFN response viral recognition in the innate immune system leads to NF-kB activation. NF-kB is a multiprotein complex consisting of NFKB1, NFKB2, RELA, RELB, and REL (166). Type I IFN response and NF-kB activation result in IL-6 and IL-8 production (35). The activation of these mediators contributes to inflammation and complex antiviral immune response (35).
As a key player in inflammatory response, NFKB1 genetic variants has also been associated with atherosclerotic manifestations including coronary artery disease, acute coronary syndrome, dilated cardiomyopathy, and ischaemic stroke (167–173). Promoter polymorphism rs28362491 is linked to HCV infection and autoimmune diseases including Behcet's disease and SLE (174–176), while rs3774937 is associated with acute rejection after renal transplantation (177). Neoplastic diseases associated with NFKB1 SNPs include CRC, Hodgkin lymphoma, NHL, cervical squamous cell carcinoma, liver, thyroid, breast, and lung cancer (178–186). rs11574851 of NFKB2 was found to be linked to RA susceptibility among anti-citrullinated protein antibodies-positive patients (187), while in healthy women rs1049728 of RELA associated with the concentration of soluble ICAM-1, which is an endothelium-derived inflammatory marker (188). Genetic variants of REL have been shown to be linked to various autoimmune diseases including RA, psoriasis, and celiac disease (189–193).
Polymorphisms of IL6 have been shown to pre-dispose to pulmonary tuberculosis, acute lung injury in patients with systemic inflammatory response syndrome and post-infectious irritable bowel syndrome (98, 194, 195). An association with RA has also been proposed (196). rs1800795 has been shown to have a role in the prognosis of patients following renal and lung transplantation (197–199). IL6 SNPs were also confirmed to have a role in the susceptibility of various cardiovascular disorders including hypertension and stroke (200–202).
IL-8 is coded by CXCL8 gene, SNPs of which have been shown to be linked to infectious, autoimmune, and neoplastic diseases. Acne vulgaris, chronic periodontitis, and invasive aspergillosis among immunocompromised patients have been shown to be associated with various variants (203–205). Autoimmune diseases including idiopathic pulmonary fibrosis, childhood IgA nephropathy, erosive oral lichen planus, childhood asthma, and Graves' disease have also been linked to genetic variants of CXCL8 (206–210). Concerning neoplastic diseases, non-small cell lung cancer, and gastric cancer have been proposed to be associated with CXCL8 SNPs (154, 211, 212).
In conclusion, large majority of the discussed SNPs present pleiotropic effects, among which the frequent presence of various autoimmune and infection-related traits highlights their putative involvement in the susceptibility and severity of COVID-19.
Toward Precision Risk Assessment: Predicting COVID-19 Susceptibility and Severity Based on a Polygenic Risk Score
As genetic susceptibility regarding COVID-19 is an ongoing topic of several large international collaborations we anticipate to acquire a large amount of evidence regarding susceptibility loci in the near future. Indeed, recent studies identified germline variants of TLR3- and IRF7-dependent type I IFN immunity to associate with more severe COVID-19 infection (213). In particular, disease-causing germline variants have been detected in TLR3, UNC93B1, TICAM1, TBK1, IRF3, IRF7, IFNAR1, and IFNAR2 in patients with life-threatening COVID-19 (213). Another recent study analyzing 1,610 COVID-19 patients and 2,205 control subjects from the first wave in heavily affected Italy and Spain found 2 chromosomal loci on chromosome three and nine with significant association with COVID-19 patients (214). On chromosome three the affected area includes several actors which might alter COVID-19 susceptibility including chemokine receptors, while on chromosome nine the association signal coincided with the AB0 blood group locus (214). AB0 blood group has independently been linked to COVID-19 susceptibility (214–216). Further studies are needed to confirm these associations in independent populations.
Applying this knowledge to detect individuals with elevated risk for severe disease might help to prioritize them for vaccination and stricter protection measures. As COVID-19 susceptibility and severity seem to have a polygenic background, we propose that a curated polygenic risk score (PGRS) might facilitate the detection of individuals with high risk for infection (Figure 1). Based on genome-wide analyses, polygenic risk scores are able to detect high-risk individuals in various diseases, fine-tuning the more widely used risk stratification dependent on baseline anthropometric and physiological characteristics (217, 218). A most recent GWAS on a cohort of COVID-19 patients from the U.K. found eight lead variants from independent genome-wide significant regions including rs2236757 in IFNAR2 coding for interferon α and β receptor subunit 2 (219). Though the individual odds ratio for each of the relatively frequent variants varies between 1.3 and 2.1, the combined odds ratio in the case of harboring all these susceptibility variants rises to 29.5, underlining the applicability of a polygenic risk score (219).
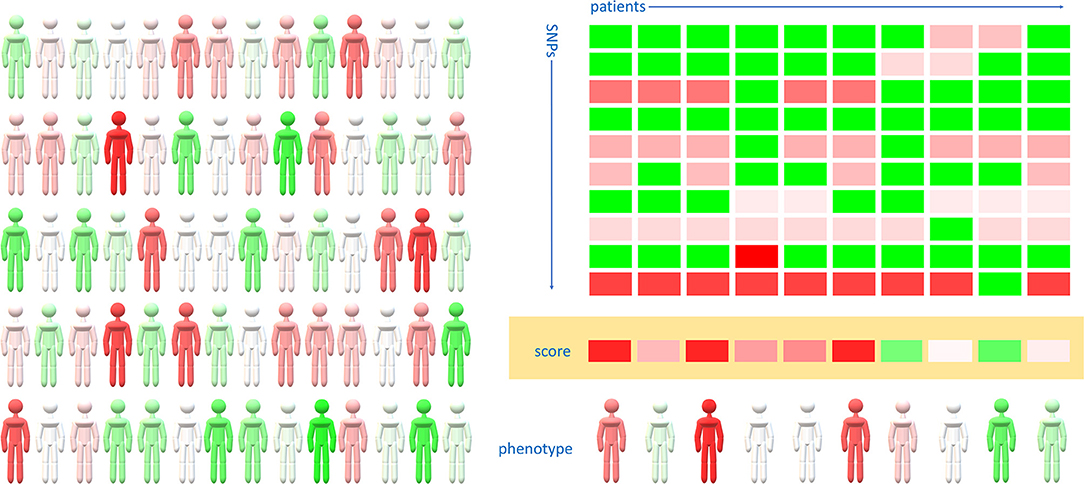
Figure 1. Polygenic risk scores might detect high-risk individuals regarding COVID-19 susceptibility and severity. The actual susceptibility and severity of COVID-19 varies widely within the population (left panel, redder individuals are more, greener individuals are less prone for severe COVID-19 disease). Genome-wide association studies might distinguish a group of SNPs from which a clinically relevant polygenic risk score can be built (right panel). Color-coded squares represent the presence of the risk allele (red) or the alternative allele (green) in each individual. The intensity of red corresponds to the odds ratio of the risk allele compared to the alternative allele. Resultant values of the odds ratios of each SNPs are color-coded as the polygenic risk score (orange background). Personalized risk scores correlate well with actual COVID-19 risk, however additional environmental, anthropometric factors and comorbidities also modify the phenotype.
In addition to COVID-19 susceptibility, inclusion of genetic predictors of disease severity and treatment response might also be included. In particular, based on the effectiveness of glucocorticoid administration confirmed by the randomized, controlled RECOVERY clinical trial (220), it would be interesting to see if pharmacogenetic modifiers of glucocorticoid action, sensitivity and metabolism contribute to the severity of COVID-19 infection and treatment response (221).
It is important to note that the majority of the observed associations in Table 1 and Supplementary Table 1 were only validated in specific populations. By analyzing the population-specific allelic frequencies of the reviewed viral entry and innate immunity-related SNPs reviewed (Supplementary Table 2) we can conclude that the large variations in SNP frequencies might heavily influence their association with various traits in select populations. Additionally, pronounced differences in risk allele frequencies of the 8 proposed lead COVID-19-related SNPs (219) are present in different populations (Supplementary Table 3). Moreover, these differences most probably alter epistatic interactions between genes, adding an additional layer of complexity (222).
Therefore, the observed population dependency of genotype-phenotype associations would probably result in population-specific PGRSs rather than a universal PGRS optimal for all populations. Dedicated efforts to perform population-specific GWASs regarding COVID-19 susceptibility and severity to build population-specific PGRSs are needed to address these differences.
Discussion
The disruption caused by the COVID-19 pandemic has yet unknown consequences on the whole human society and on each affected patient's health as well. Understanding the susceptibility toward this disease is important to detect high-risk individuals and also to decipher molecular mechanisms needed for the development of the clinical phenotype. Viral entry and innate immunity are key mechanisms in the initiation of SARS-CoV-2 infection. We performed a thorough literature review concerning genotype-phenotype association studies regarding agents of these mechanisms. Our results indicated that SNPs in the genes of these processes are frequently associated with susceptibility to various bacterial and viral infections. Additionally, several autoimmune diseases are also linked to these genes, underlining the versatile immune consequences of these genetic variants. Based on the confirmed associations it is highly plausible that the abovementioned SNPs might confer altered susceptibility to SARS-CoV-2 infection and its complex clinical consequences.
In addition to viral entry and innate immunity, other mechanisms including adaptive immunity are also of paramount importance regarding the susceptibility to COVID-19 (35). To better characterize putative genomic susceptibility loci, well-designed, international genome-wide association studies (GWAS) are needed.
As multiple GWASs on host genetic susceptibility are ongoing, several genomic susceptibility loci are proposed to be detected. Translating these individual susceptibility variants into clinically relevant polygenic risk scores would fully leverage this acquired knowledge to easily detect high-risk individuals prioritized for vaccination and stricter protective measures.
All things considered, genetic variants of genes of viral entry and innate immunity might alter susceptibility, and prognosis of COVID-19. Further GWASs are needed to better characterize susceptibility loci and to develop clinically relevant risk stratification strategies.
Author Contributions
VG contributed to the design, performed literature search, and drafted the manuscript. AB contributed to the literature search. JP contributed to the design and the literature search. AP conceived the review, contributed to the design, and literature search. All authors read and have agreed to the final manuscript.
Funding
The authors would like to acknowledge the financial support of the Ministry of Human Capacities (ÁEEK/41872-16/2020) and of the 2019 Thematic Excellence Program (TUDFO/51757/2019-ITM).
Conflict of Interest
The authors declare that the research was conducted in the absence of any commercial or financial relationships that could be construed as a potential conflict of interest.
Supplementary Material
The Supplementary Material for this article can be found online at: https://www.frontiersin.org/articles/10.3389/fimmu.2021.653489/full#supplementary-material
References
1. Dong E, Du H, Gardner L. An interactive web-based dashboard to track COVID-19 in real time. Lancet Infect Dis. (2020) 20:533–4. doi: 10.1016/S1473-3099(20)30120-1
2. Allison AC. Polymorphism and natural selection in human populations. Cold Spring Harb Symp Quant Biol. (1964) 29:137–49. doi: 10.1101/SQB.1964.029.01.018
3. Hill AV, Allsopp CE, Kwiatkowski D, Anstey NM, Twumasi P, Rowe PA, et al. Common west African HLA antigens are associated with protection from severe malaria. Nature. (1991) 352:595–600. doi: 10.1038/352595a0
4. Liu R, Paxton WA, Choe S, Ceradini D, Martin SR, Horuk R, et al. Homozygous defect in HIV-1 coreceptor accounts for resistance of some multiply-exposed individuals to HIV-1 infection. Cell. (1996) 86:367–77. doi: 10.1016/S0092-8674(00)80110-5
5. Zhou P, Yang XL, Wang XG, Hu B, Zhang L, Zhang W, et al. A pneumonia outbreak associated with a new coronavirus of probable bat origin. Nature. (2020) 579:270–3. doi: 10.1038/s41586-020-2012-7
6. Li W, Moore MJ, Vasilieva N, Sui J, Wong SK, Berne MA, et al. Angiotensin-converting enzyme 2 is a functional receptor for the SARS coronavirus. Nature. (2003) 426:450–4. doi: 10.1038/nature02145
7. Hoffmann M, Kleine-Weber H, Schroeder S, Kruger N, Herrler T, Erichsen S, et al. SARS-CoV-2 cell entry depends on ACE2 and TMPRSS2 and is blocked by a clinically proven protease inhibitor. Cell. (2020) 181:271–80.e8. doi: 10.1016/j.cell.2020.02.052
8. Walls AC, Park YJ, Tortorici MA, Wall A, McGuire AT, Veesler D. Structure, function, and antigenicity of the SARS-CoV-2 spike glycoprotein. Cell. (2020) 181:281–92.e6. doi: 10.1016/j.cell.2020.02.058
9. Yan R, Zhang Y, Li Y, Xia L, Guo Y, Zhou Q. Structural basis for the recognition of SARS-CoV-2 by full-length human ACE2. Science. (2020) 367:1444–8. doi: 10.1126/science.abb2762
10. Lukassen S, Chua RL, Trefzer T, Kahn NC, Schneider MA, Muley T, et al. SARS-CoV-2 receptor ACE2 and TMPRSS2 are primarily expressed in bronchial transient secretory cells. EMBO J. (2020) 39:e105114. doi: 10.15252/embj.20105114
11. Shang J, Wan Y, Luo C, Ye G, Geng Q, Auerbach A, et al. Cell entry mechanisms of SARS-CoV-2. Proc Natl Acad Sci USA. (2020) 117:11727–34. doi: 10.1073/pnas.2003138117
12. Ou X, Liu Y, Lei X, Li P, Mi D, Ren L, et al. Characterization of spike glycoprotein of SARS-CoV-2 on virus entry and its immune cross-reactivity with SARS-CoV. Nat Commun. (2020) 11:1620. doi: 10.1038/s41467-020-15562-9
13. Fan Z, Wu G, Yue M, Ye J, Chen Y, Xu B, et al. Hypertension and hypertensive left ventricular hypertrophy are associated with ACE2 genetic polymorphism. Life Sci. (2019) 225:39–45. doi: 10.1016/j.lfs.2019.03.059
14. Liu C, Li Y, Guan T, Lai Y, Shen Y, Zeyaweiding A, et al. ACE2 polymorphisms associated with cardiovascular risk in Uygurs with type 2 diabetes mellitus. Cardiovasc Diabetol. (2018) 17:127. doi: 10.1186/s12933-018-0771-3
15. Meng N, Zhang Y, Ma J, Li H, Zhou F, Qu Y. Association of polymorphisms of angiotensin I converting enzyme 2 with retinopathy in type 2 diabetes mellitus among Chinese individuals. Eye. (2015) 29:266–71. doi: 10.1038/eye.2014.254
16. Zhang Q, Cong M, Wang N, Li X, Zhang H, Zhang K, et al. Association of angiotensin-converting enzyme 2 gene polymorphism and enzymatic activity with essential hypertension in different gender: a case-control study. Medicine. (2018) 97:e12917. doi: 10.1097/MD.0000000000012917
17. Wang SX, Tao T, Fu ZQ, Xie XZ, Wang H, Wang YT. Polymorphisms of angiotensin-converting enzyme 2 gene confer a risk to lone atrial fibrillation in Chinese male patients. Chin Med J. (2013) 126:4608–11. doi: 10.3760/cma.j.issn.0366-6999.20131381
18. Vangjeli C, Dicker P, Tregouet DA, Shields DC, Evans A, Stanton AV, et al. A polymorphism in ACE2 is associated with a lower risk for fatal cardiovascular events in females: the MORGAM project. J Renin Angiotensin Aldosterone Syst. (2011) 12:504–9. doi: 10.1177/1470320311405557
19. Feng W, Sun L, Qu XF. Association of AGTR1 and ACE2 gene polymorphisms with structural atrial fibrillation in a Chinese Han population. Pharmazie. (2017) 72:17–21. doi: 10.1691/ph.2017.6752
20. Kumar A, Rani B, Sharma R, Kaur G, Prasad R, Bahl A, et al. ACE2, CALM3 and TNNI3K polymorphisms as potential disease modifiers in hypertrophic and dilated cardiomyopathies. Mol Cell Biochem. (2018) 438:167–74. doi: 10.1007/s11010-017-3123-9
21. Zhao Q, Gu D, Kelly TN, Hixson JE, Rao DC, Jaquish CE, et al. Association of genetic variants in the apelin-APJ system and ACE2 with blood pressure responses to potassium supplementation: the GenSalt study. Am J Hypertens. (2010) 23:606–13. doi: 10.1038/ajh.2010.36
22. Bhanushali A, Rao P, Raman V, Kokate P, Ambekar A, Mandva S, et al. Status of TMPRSS2-ERG fusion in prostate cancer patients from India: correlation with clinico-pathological details and TMPRSS2 Met160Val polymorphism. Prostate Int. (2018) 6:145–50. doi: 10.1016/j.prnil.2018.03.004
23. FitzGerald LM, Agalliu I, Johnson K, Miller MA, Kwon EM, Hurtado-Coll A, et al. Association of TMPRSS2-ERG gene fusion with clinical characteristics and outcomes: results from a population-based study of prostate cancer. BMC Cancer. (2008) 8:230. doi: 10.1186/1471-2407-8-230
24. Cheng Z, Zhou J, To KK, Chu H, Li C, Wang D, et al. Identification of TMPRSS2 as a susceptibility gene for severe 2009 pandemic a(H1N1) influenza and A(H7N9) influenza. J Infect Dis. (2015) 212:1214–21. doi: 10.1093/infdis/jiv246
25. Turpeinen H, Seppala I, Lyytikainen LP, Raitoharju E, Hutri-Kahonen N, Levula M, et al. A genome-wide expression quantitative trait loci analysis of proprotein convertase subtilisin/kexin enzymes identifies a novel regulatory gene variant for FURIN expression and blood pressure. Hum Genet. (2015) 134:627–36. doi: 10.1007/s00439-015-1546-5
26. Fromer M, Roussos P, Sieberts SK, Johnson JS, Kavanagh DH, Perumal TM, et al. Gene expression elucidates functional impact of polygenic risk for schizophrenia. Nat Neurosci. (2016) 19:1442–53. doi: 10.1038/nn.4399
27. Consortium CAD, Deloukas P, Kanoni S, Willenborg C, Farrall M, Assimes TL, et al. Large-scale association analysis identifies new risk loci for coronary artery disease. Nat Genet. (2013) 45:25–33. doi: 10.1038/ng.2480
28. Ueyama C, Horibe H, Yamase Y, Fujimaki T, Oguri M, Kato K, et al. Association of FURIN and ZPR1 polymorphisms with metabolic syndrome. Biomed Rep. (2015) 3:641–7. doi: 10.3892/br.2015.484
29. Pilling LC, Kuo CL, Sicinski K, Tamosauskaite J, Kuchel GA, Harries LW, et al. Human longevity: 25 genetic loci associated in 389,166 UK biobank participants. Aging. (2017) 9:2504–20. doi: 10.18632/aging.101334
30. Fan Y, Li X, Zhang Y, Fan X, Zhang N, Zheng H, et al. Genetic variants of TPCN2 associated with type 2 diabetes risk in the Chinese population. PLoS ONE. (2016) 11:e0149614. doi: 10.1371/journal.pone.0149614
31. Sulem P, Gudbjartsson DF, Stacey SN, Helgason A, Rafnar T, Jakobsdottir M, et al. Two newly identified genetic determinants of pigmentation in Europeans. Nat Genet. (2008) 40:835–7. doi: 10.1038/ng.160
32. Chen S, Wang Z, Zhang L, Lu G, Zhou C, Wang DW, et al. Association between polymorphism in the human cathepsin L (CTSL1) promoter with hypertension in the uygur, kazak and han populations in China. J Coll Phys Surg Pak. (2015) 25:640–3.
33. Mbewe-Campbell N, Wei Z, Zhang K, Friese RS, Mahata M, Schork AJ, et al. Genes and environment: novel, functional polymorphism in the human cathepsin L (CTSL1) promoter disrupts a xenobiotic response element (XRE) to alter transcription and blood pressure. J Hypertens. (2012) 30:1961–9. doi: 10.1097/HJH.0b013e328356b86a
34. Vickers C, Hales P, Kaushik V, Dick L, Gavin J, Tang J, et al. Hydrolysis of biological peptides by human angiotensin-converting enzyme-related carboxypeptidase. J Biol Chem. (2002) 277:14838–43. doi: 10.1074/jbc.M200581200
35. Li K, Hao Z, Zhao X, Du J, Zhou Y. SARS-CoV-2 infection-induced immune responses: friends or foes? Scand J Immunol. (2020) 92:e12895. doi: 10.1111/sji.12895
36. Nikonov A, Molder T, Sikut R, Kiiver K, Mannik A, Toots U, et al. RIG-I and MDA-5 detection of viral RNA-dependent RNA polymerase activity restricts positive-strand RNA virus replication. PLoS Pathog. (2013) 9:e1003610. doi: 10.1371/journal.ppat.1003610
37. Lee NR, Ban J, Lee NJ, Yi CM, Choi JY, Kim H, et al. Activation of RIG-I-mediated antiviral signaling triggers autophagy through the MAVS-TRAF6-beclin-1 signaling axis. Front Immunol. (2018) 9:2096. doi: 10.3389/fimmu.2018.02096
38. Xie T, Chen T, Li C, Wang W, Cao L, Rao H, et al. RACK1 attenuates RLR antiviral signaling by targeting VISA-TRAF complexes. Biochem Biophys Res Commun. (2019) 508:667–74. doi: 10.1016/j.bbrc.2018.11.203
39. Ovsyannikova IG, Haralambieva IH, Dhiman N, O'Byrne MM, Pankratz VS, Jacobson RM, et al. Polymorphisms in the vitamin A receptor and innate immunity genes influence the antibody response to rubella vaccination. J Infect Dis. (2010) 201:207–13. doi: 10.1086/649588
40. Ovsyannikova IG, Salk HM, Larrabee BR, Pankratz VS, Poland GA. Single-nucleotide polymorphism associations in common with immune responses to measles and rubella vaccines. Immunogenetics. (2014) 66:663–9. doi: 10.1007/s00251-014-0796-z
41. Haralambieva IH, Ovsyannikova IG, Umlauf BJ, Vierkant RA, Shane Pankratz V, Jacobson RM, et al. Genetic polymorphisms in host antiviral genes: associations with humoral and cellular immunity to measles vaccine. Vaccine. (2011) 29:8988–97. doi: 10.1016/j.vaccine.2011.09.043
42. Moumad K, Lascorz J, Bevier M, Khyatti M, Ennaji MM, Benider A, et al. Genetic polymorphisms in host innate immune sensor genes and the risk of nasopharyngeal carcinoma in North Africa. G3. (2013) 3:971–7. doi: 10.1534/g3.112.005371
43. Li YP, Li M, Jia XL, Deng HL, Wang WJ, Wu FP, et al. Association of gene polymorphisms of pattern-recognition receptor signaling pathway with the risk and severity of hand, foot, and mouth disease caused by enterovirus 71 in Chinese Han population. J Med Virol. (2018) 90:692–8. doi: 10.1002/jmv.25000
44. Li Y, Liao W, Cargill M, Chang M, Matsunami N, Feng BJ, et al. Carriers of rare missense variants in IFIH1 are protected from psoriasis. J Invest Dermatol. (2010) 130:2768–72. doi: 10.1038/jid.2010.214
45. Molineros JE, Maiti AK, Sun C, Looger LL, Han S, Kim-Howard X, et al. Admixture mapping in lupus identifies multiple functional variants within IFIH1 associated with apoptosis, inflammation, and autoantibody production. PLoS Genet. (2013) 9:e1003222. doi: 10.1371/journal.pgen.1003222
46. Jermendy A, Szatmari I, Laine AP, Lukacs K, Horvath KH, Korner A, et al. The interferon-induced helicase IFIH1 Ala946Thr polymorphism is associated with type 1 diabetes in both the high-incidence finnish and the medium-incidence Hungarian populations. Diabetologia. (2010) 53:98–102. doi: 10.1007/s00125-009-1561-y
47. Smyth DJ, Cooper JD, Bailey R, Field S, Burren O, Smink LJ, et al. A genome-wide association study of nonsynonymous SNPs identifies a type 1 diabetes locus in the interferon-induced helicase (IFIH1) region. Nat Genet. (2006) 38:617–9. doi: 10.1038/ng1800
48. Emdin CA, Khera AV, Chaffin M, Klarin D, Natarajan P, Aragam K, et al. Analysis of predicted loss-of-function variants in UK Biobank identifies variants protective for disease. Nat Commun. (2018) 9:1613. doi: 10.1038/s41467-018-03911-8
49. Nejentsev S, Walker N, Riches D, Egholm M, Todd JA. Rare variants of IFIH1, a gene implicated in antiviral responses, protect against type 1 diabetes. Science. (2009) 324:387–9. doi: 10.1126/science.1167728
50. Chistiakov DA, Voronova NV, Savost'Anov KV, Turakulov RI. Loss-of-function mutations E6 27X and I923V of IFIH1 are associated with lower poly(I:C)-induced interferon-beta production in peripheral blood mononuclear cells of type 1 diabetes patients. Hum Immunol. (2010) 71:1128–34. doi: 10.1016/j.humimm.2010.08.005
51. Enevold C, Oturai AB, Sorensen PS, Ryder LP, Koch-Henriksen N, Bendtzen K. Multiple sclerosis and polymorphisms of innate pattern recognition receptors TLR1-10, NOD1-2, DDX58, and IFIH1. J Neuroimmunol. (2009) 212:125–31. doi: 10.1016/j.jneuroim.2009.04.008
52. Yang H, Wang Z, Xu K, Gu R, Chen H, Yu D, et al. IFIH1 gene polymorphisms in type 1 diabetes: genetic association analysis and genotype-phenotype correlation in Chinese Han population. Autoimmunity. (2012) 45:226–32. doi: 10.3109/08916934.2011.633134
53. Wang C, Ahlford A, Laxman N, Nordmark G, Eloranta ML, Gunnarsson I, et al. Contribution of IKBKE and IFIH1 gene variants to SLE susceptibility. Genes Immun. (2013) 14:217–22. doi: 10.1038/gene.2013.9
54. Liu J, Tang LY, Wang YG, Lu SY, Zhang EN, Wang ZG, et al. Identification of MAVS as a novel risk factor for the development of osteoarthritis. Aging Dis. (2018) 9:40–50. doi: 10.14336/AD.2017.0308
55. Pothlichet J, Niewold TB, Vitour D, Solhonne B, Crow MK, Si-Tahar M. A loss-of-function variant of the antiviral molecule MAVS is associated with a subset of systemic lupus patients. EMBO Mol Med. (2011) 3:142–52. doi: 10.1002/emmm.201000120
56. Santana-de Anda K, Gomez-Martin D, Monsivais-Urenda AE, Salgado-Bustamante M, Gonzalez-Amaro R, Alcocer-Varela J. Interferon regulatory factor 3 as key element of the interferon signature in plasmacytoid dendritic cells from systemic lupus erythematosus patients: novel genetic associations in the Mexican mestizo population. Clin Exp Immunol. (2014) 178:428–37. doi: 10.1111/cei.12429
57. Lin LH, Ling P, Liu MF. The potential role of interferon-regulatory factor 7 among Taiwanese patients with systemic lupus erythematosus. J Rheumatol. (2011) 38:1914–9. doi: 10.3899/jrheum.101004
58. Fu Q, Zhao J, Qian X, Wong JL, Kaufman KM, Yu CY, et al. Association of a functional IRF7 variant with systemic lupus erythematosus. Arthritis Rheum. (2011) 63:749–54. doi: 10.1002/art.30193
59. Carmona FD, Gutala R, Simeon CP, Carreira P, Ortego-Centeno N, Vicente-Rabaneda E, et al. Novel identification of the IRF7 region as an anticentromere autoantibody propensity locus in systemic sclerosis. Ann Rheum Dis. (2012) 71:114–9. doi: 10.1136/annrheumdis-2011-200275
60. Paradowska-Gorycka A, Wajda A, Stypinska B, Walczuk E, Walczyk M, Felis-Giemza A, et al. Interferons (IFN-A/-B/-G) genetic variants in patients with mixed connective tissue disease (MCTD). J Clin Med. (2019) 8:2046. doi: 10.3390/jcm8122046
61. Fujita M, Scheurer ME, Decker SA, McDonald HA, Kohanbash G, Kastenhuber ER, et al. Role of type 1 IFNs in antiglioma immunosurveillance–using mouse studies to guide examination of novel prognostic markers in humans. Clin Cancer Res. (2010) 16:3409–19. doi: 10.1158/1078-0432.CCR-10-0644
62. Arpaia N, Barton GM. Toll-like receptors: key players in antiviral immunity. Curr Opin Virol. (2011) 1:447–54. doi: 10.1016/j.coviro.2011.10.006
63. Barton GM, Medzhitov R. Toll-like receptor signaling pathways. Science. (2003) 300:1524–5. doi: 10.1126/science.1085536
64. Spiegel M, Pichlmair A, Martinez-Sobrido L, Cros J, Garcia-Sastre A, Haller O, et al. Inhibition of Beta interferon induction by severe acute respiratory syndrome coronavirus suggests a two-step model for activation of interferon regulatory factor 3. J Virol. (2005) 79:2079–86. doi: 10.1128/JVI.79.4.2079-2086.2005
65. Kostoula C, Shaker T, Cerovic M, Craparotta I, Marchini S, Butti E, et al. TLR3 preconditioning induces anti-inflammatory and anti-ictogenic effects in mice mediated by the IRF3/IFN-beta axis. Brain Behav Immun. (2019) 81:598–607. doi: 10.1016/j.bbi.2019.07.021
66. Al-Qahtani A, Al-Ahdal M, Abdo A, Sanai F, Al-Anazi M, Khalaf N, et al. Toll-like receptor 3 polymorphism and its association with hepatitis B virus infection in Saudi Arabian patients. J Med Virol. (2012) 84:1353–9. doi: 10.1002/jmv.23271
67. Huang X, Li H, Wang J, Huang C, Lu Y, Qin X, et al. Genetic polymorphisms in Toll-like receptor 3 gene are associated with the risk of hepatitis B virus-related liver diseases in a Chinese population. Gene. (2015) 569:218–24. doi: 10.1016/j.gene.2015.05.054
68. Qian F, Bolen CR, Jing C, Wang X, Zheng W, Zhao H, et al. Impaired toll-like receptor 3-mediated immune responses from macrophages of patients chronically infected with hepatitis C virus. Clin Vaccine Immunol. (2013) 20:146–55. doi: 10.1128/CVI.00530-12
69. Al-Anazi MR, Matou-Nasri S, Abdo AA, Sanai FM, Alkahtani S, Alarifi S, et al. Association of toll-like receptor 3 single-nucleotide polymorphisms and hepatitis C virus infection. J Immunol Res. (2017) 2017:1590653. doi: 10.1155/2017/1590653
70. Svensson A, Tunback P, Nordstrom I, Padyukov L, Eriksson K. Polymorphisms in Toll-like receptor 3 confer natural resistance to human herpes simplex virus type 2 infection. J Gen Virol. (2012) 93:1717–24. doi: 10.1099/vir.0.042572-0
71. Sironi M, Biasin M, Cagliani R, Forni D, De Luca M, Saulle I, et al. A common polymorphism in TLR3 confers natural resistance to HIV-1 infection. J Immunol. (2012) 188:818–23. doi: 10.4049/jimmunol.1102179
72. Huik K, Avi R, Pauskar M, Kallas E, Jogeda EL, Karki T, et al. Association between TLR3 rs3775291 and resistance to HIV among highly exposed caucasian intravenous drug users. Infect Genet Evol. (2013) 20:78–82. doi: 10.1016/j.meegid.2013.08.008
73. Moore CE, Hennig BJ, Perrett KP, Hoe JC, Lee SJ, Fletcher H, et al. Single nucleotide polymorphisms in the Toll-like receptor 3 and CD44 genes are associated with persistence of vaccine-induced immunity to the serogroup C meningococcal conjugate vaccine. Clin Vaccine Immunol. (2012) 19:295–303. doi: 10.1128/CVI.05379-11
74. Laska MJ, Troldborg A, Hansen B, Stengaard-Pedersen K, Junker P, Nexo BA, et al. Polymorphisms within Toll-like receptors are associated with systemic lupus erythematosus in a cohort of Danish females. Rheumatology. (2014) 53:48–55. doi: 10.1093/rheumatology/ket316
75. Laska MJ, Hansen B, Troldborg A, Lorenzen T, Stengaard-Pedersen K, Junker P, et al. A non-synonymous single-nucleotide polymorphism in the gene encoding toll-like receptor 3 (TLR3) is associated with sero-negative rheumatoid arthritis (RA) in a Danish population. BMC Res Notes. (2014) 7:716. doi: 10.1186/1756-0500-7-716
76. Ikezoe K, Handa T, Tanizawa K, Kubo T, Ito I, Sokai A, et al. A toll-like receptor 3 single nucleotide polymorphism in Japanese patients with sarcoidosis. Tissue Antigens. (2015) 85:204–8. doi: 10.1111/tan.12535
77. Yeyeodu ST, Kidd LR, Oprea-Ilies GM, Burns BG, Vancleave TT, Shim JY, et al. IRAK4 and TLR3 sequence variants may alter breast cancer risk among African-American women. Front Immunol. (2013) 4:338. doi: 10.3389/fimmu.2013.00338
78. Fan L, Zhou P, Chen AX, Liu GY, Yu KD, Shao ZM. Toll-like receptor 3−926T>A increased the risk of breast cancer through decreased transcriptional activity. Oncoimmunology. (2019) 8:e1673126. doi: 10.1080/2162402X.2019.1673126
79. Slattery ML, Herrick JS, Bondurant KL, Wolff RK. Toll-like receptor genes and their association with colon and rectal cancer development and prognosis. Int J Cancer. (2012) 130:2974–80. doi: 10.1002/ijc.26314
80. Castro FA, Forsti A, Buch S, Kalthoff H, Krauss C, Bauer M, et al. TLR-3 polymorphism is an independent prognostic marker for stage II colorectal cancer. Eur J Cancer. (2011) 47:1203–10. doi: 10.1016/j.ejca.2010.12.011
81. Park JY, Amankwah EK, Anic GM, Lin HY, Walls B, Park H, et al. Gene variants in angiogenesis and lymphangiogenesis and cutaneous melanoma progression. Cancer Epidemiol Biomarkers Prev. (2013) 22:827–34. doi: 10.1158/1055-9965.EPI-12-1129
82. Kawasaki A, Furukawa H, Kondo Y, Ito S, Hayashi T, Kusaoi M, et al. TLR7 single-nucleotide polymorphisms in the 3' untranslated region and intron 2 independently contribute to systemic lupus erythematosus in Japanese women: a case-control association study. Arthritis Res Ther. (2011) 13:R41. doi: 10.1186/ar3277
83. Shen N, Fu Q, Deng Y, Qian X, Zhao J, Kaufman KM, et al. Sex-specific association of X-linked Toll-like receptor 7 (TLR7) with male systemic lupus erythematosus. Proc Natl Acad Sci USA. (2010) 107:15838–43. doi: 10.1073/pnas.1001337107
84. Enevold C, Nielsen CH, Jacobsen RS, Hermansen ML, Molbo D, Avlund K, et al. Single nucleotide polymorphisms in genes encoding toll-like receptors 7, 8 and 9 in Danish patients with systemic lupus erythematosus. Mol Biol Rep. (2014) 41:5755–63. doi: 10.1007/s11033-014-3447-4
85. Raafat, II, El Guindy N, Shahin RMH, Samy LA, El Refai RM. Toll-like receptor 7 gene single nucleotide polymorphisms and the risk for systemic lupus erythematosus: a case-control study. Z Rheumatol. (2018) 77:416–20. doi: 10.1007/s00393-017-0283-7
86. Yue M, Gao CF, Wang JJ, Wang CJ, Feng L, Wang J, et al. Toll-like receptor 7 variations are associated with the susceptibility to HCV infection among Chinese females. Infect Genet Evol. (2014) 27:264–70. doi: 10.1016/j.meegid.2014.07.034
87. Dutta SK, Tripathi A. Association of toll-like receptor polymorphisms with susceptibility to chikungunya virus infection. Virology. (2017) 511:207–13. doi: 10.1016/j.virol.2017.08.009
88. Moller-Larsen S, Nyegaard M, Haagerup A, Vestbo J, Kruse TA, Borglum AD. Association analysis identifies TLR7 and TLR8 as novel risk genes in asthma and related disorders. Thorax. (2008) 63:1064–9. doi: 10.1136/thx.2007.094128
89. Edwards AO, Chen D, Fridley BL, James KM, Wu Y, Abecasis G, et al. Toll-like receptor polymorphisms and age-related macular degeneration. Invest Ophthalmol Vis Sci. (2008) 49:1652–9. doi: 10.1167/iovs.07-1378
90. Varzari A, Deyneko IV, Vladei I, Grallert H, Schieck M, Tudor E, et al. Genetic variation in TLR pathway and the risk of pulmonary tuberculosis in a Moldavian population. Infect Genet Evol. (2019) 68:84–90. doi: 10.1016/j.meegid.2018.12.005
91. Davila S, Hibberd ML, Hari Dass R, Wong HE, Sahiratmadja E, Bonnard C, et al. Genetic association and expression studies indicate a role of toll-like receptor 8 in pulmonary tuberculosis. PLoS Genet. (2008) 4:e1000218. doi: 10.1371/journal.pgen.1000218
92. Wang MG, Zhang MM, Wang Y, Wu SQ, Zhang M, He JQ. Association of TLR8 and TLR9 polymorphisms with tuberculosis in a Chinese Han population: a case-control study. BMC Infect Dis. (2018) 18:561. doi: 10.1186/s12879-018-3485-y
93. Esposito S, Molteni CG, Zampiero A, Baggi E, Lavizzari A, Semino M, et al. Role of polymorphisms of toll-like receptor (TLR) 4, TLR9, toll-interleukin 1 receptor domain containing adaptor protein (TIRAP) and FCGR2A genes in malaria susceptibility and severity in Burundian children. Malar J. (2012) 11:196. doi: 10.1186/1475-2875-11-196
94. Omar AH, Yasunami M, Yamazaki A, Shibata H, Ofori MF, Akanmori BD, et al. Toll-like receptor 9 (TLR9) polymorphism associated with symptomatic malaria: a cohort study. Malar J. (2012) 11:168. doi: 10.1186/1475-2875-11-168
95. Paradowska E, Jablonska A, Studzinska M, Skowronska K, Suski P, Wisniewska-Ligier M, et al. TLR9-1486T/C and 2848C/T SNPs are associated with human cytomegalovirus infection in infants. PLoS ONE. (2016) 11:e0154100. doi: 10.1371/journal.pone.0154100
96. Kobayashi K, Yuliwulandari R, Yanai H, Naka I, Lien LT, Hang NT, et al. Association of TLR polymorphisms with development of tuberculosis in Indonesian females. Tissue Antigens. (2012) 79:190–7. doi: 10.1111/j.1399-0039.2011.01821.x
97. Torres-Garcia D, Cruz-Lagunas A, Garcia-Sancho Figueroa MC, Fernandez-Plata R, Baez-Saldana R, Mendoza-Milla C, et al. Variants in toll-like receptor 9 gene influence susceptibility to tuberculosis in a Mexican population. J Transl Med. (2013) 11:220. doi: 10.1186/1479-5876-11-220
98. Villani AC, Lemire M, Thabane M, Belisle A, Geneau G, Garg AX, et al. Genetic risk factors for post-infectious irritable bowel syndrome following a waterborne outbreak of gastroenteritis. Gastroenterology. (2010) 138:1502–13. doi: 10.1053/j.gastro.2009.12.049
99. dos Santos BP, Valverde JV, Rohr P, Monticielo OA, Brenol JC, Xavier RM, et al. TLR7/8/9 polymorphisms and their associations in systemic lupus erythematosus patients from southern Brazil. Lupus. (2012) 21:302–9. doi: 10.1177/0961203311425522
100. Zhang J, Zhu Q, Meng F, Lei H, Zhao Y. Association study of TLR-9 polymorphisms and systemic lupus erythematosus in northern Chinese Han population. Gene. (2014) 533:385–8. doi: 10.1016/j.gene.2013.08.051
101. Huang CM, Huang PH, Chen CL, Lin YJ, Tsai CH, Huang WL, et al. Association of toll-like receptor 9 gene polymorphism in Chinese patients with systemic lupus erythematosus in Taiwan. Rheumatol Int. (2012) 32:2105–9. doi: 10.1007/s00296-011-1925-8
102. Zhou XJ, Lv JC, Cheng WR, Yu L, Zhao MH, Zhang H. Association of TLR9 gene polymorphisms with lupus nephritis in a Chinese Han population. Clin Exp Rheumatol. (2010) 28(3):397–400.
103. Liao WL, Chen RH, Lin HJ, Liu YH, Chen WC, Tsai Y, et al. Toll-like receptor gene polymorphisms are associated with susceptibility to Graves' ophthalmopathy in Taiwan males. BMC Med Genet. (2010) 11:154. doi: 10.1186/1471-2350-11-154
104. Etem EO, Elyas H, Ozgocmen S, Yildirim A, Godekmerdan A. The investigation of toll-like receptor 3, 9 and 10 gene polymorphisms in Turkish rheumatoid arthritis patients. Rheumatol Int. (2011) 31:1369–74. doi: 10.1007/s00296-010-1472-8
105. Rybka J, Gebura K, Wrobel T, Wysoczanska B, Stefanko E, Kuliczkowski K, et al. Variations in genes involved in regulation of the nuclear factor - kappaB pathway and the risk of acute myeloid leukaemia. Int J Immunogenet. (2016) 43:101–6. doi: 10.1111/iji.12255
106. Roszak A, Lianeri M, Sowinska A, Jagodzinski PP. Involvement of Toll-like Receptor 9 polymorphism in cervical cancer development. Mol Biol Rep. (2012) 39:8425–30. doi: 10.1007/s11033-012-1695-8
107. Lai ZZ, Ni Z, Pan XL, Song L. Toll-like receptor 9 (TLR9) gene polymorphisms associated with increased susceptibility of human papillomavirus-16 infection in patients with cervical cancer. J Int Med Res. (2013) 41:1027–36. doi: 10.1177/0300060513483398
108. Stark JR, Wiklund F, Gronberg H, Schumacher F, Sinnott JA, Stampfer MJ, et al. Toll-like receptor signaling pathway variants and prostate cancer mortality. Cancer Epidemiol Biomark Prev. (2009) 18:1859–63. doi: 10.1158/1055-9965.EPI-08-0981
109. Xue X, Qiu Y, Jiang D, Jin T, Yan M, Zhu X, et al. The association analysis of TLR2 and TLR4 gene with tuberculosis in the Tibetan Chinese population. Oncotarget. (2017) 8:113082–9. doi: 10.18632/oncotarget.22996
110. Wu L, Hu Y, Li D, Jiang W, Xu B. Screening toll-like receptor markers to predict latent tuberculosis infection and subsequent tuberculosis disease in a Chinese population. BMC Med Genet. (2015) 16:19. doi: 10.1186/s12881-015-0166-1
111. Taniguchi R, Koyano S, Suzutani T, Goishi K, Ito Y, Morioka I, et al. Polymorphisms in TLR-2 are associated with congenital cytomegalovirus (CMV) infection but not with congenital CMV disease. Int J Infect Dis. (2013) 17:e1092–7. doi: 10.1016/j.ijid.2013.06.004
112. Royse KE, Kempf MC, McGwin G, Wilson CM, Tang J, Shrestha S. Toll-like receptor gene variants associated with bacterial vaginosis among HIV-1 infected adolescents. J Reprod Immunol. (2012) 96:84–9. doi: 10.1016/j.jri.2012.08.002
113. Mackelprang RD, Scoville CW, Cohen CR, Ondondo RO, Bigham AW, Celum C, et al. Toll-like receptor gene variants and bacterial vaginosis among HIV-1 infected and uninfected African women. Genes Immun. (2015) 16:362–5. doi: 10.1038/gene.2015.13
114. Rosentul DC, Delsing CE, Jaeger M, Plantinga TS, Oosting M, Costantini I, et al. Gene polymorphisms in pattern recognition receptors and susceptibility to idiopathic recurrent vulvovaginal candidiasis. Front Microbiol. (2014) 5:483. doi: 10.3389/fmicb.2014.00483
115. Takahashi M, Chen Z, Watanabe K, Kobayashi H, Nakajima T, Kimura A, et al. Toll-like receptor 2 gene polymorphisms associated with aggressive periodontitis in Japanese. Open Dent J. (2011) 5:190–4. doi: 10.2174/1874210601105010190
116. Abu-Maziad A, Schaa K, Bell EF, Dagle JM, Cooper M, Marazita ML, et al. Role of polymorphic variants as genetic modulators of infection in neonatal sepsis. Pediatr Res. (2010) 68:323–9. doi: 10.1203/PDR.0b013e3181e6a068
117. Schroder NW, Diterich I, Zinke A, Eckert J, Draing C, von Baehr V, et al. Heterozygous Arg753Gln polymorphism of human TLR-2 impairs immune activation by Borrelia burgdorferi and protects from late stage Lyme disease. J Immunol. (2005) 175:2534–40. doi: 10.4049/jimmunol.175.4.2534
118. Fischer M, Spies-Weisshart B, Schrenk K, Gruhn B, Wittig S, Glaser A, et al. Polymorphisms of dectin-1 and TLR2 predispose to invasive fungal disease in patients with acute myeloid leukemia. PLoS ONE. (2016) 11:e0150632. doi: 10.1371/journal.pone.0150632
119. Tsui FW, Xi N, Rohekar S, Riarh R, Bilotta R, Tsui HW, et al. Toll-like receptor 2 variants are associated with acute reactive arthritis. Arthritis Rheum. (2008) 58:3436–8. doi: 10.1002/art.23967
120. Ovsyannikova IG, Haralambieva IH, Vierkant RA, Pankratz VS, Jacobson RM, Poland GA. The role of polymorphisms in Toll-like receptors and their associated intracellular signaling genes in measles vaccine immunity. Hum Genet. (2011) 130:547–61. doi: 10.1007/s00439-011-0977-x
121. Zukowski M, Taryma-Lesniak O, Kaczmarczyk M, Kotfis K, Szydlowski L, Ciechanowicz A, et al. Relationship between toll-like receptor 2 R753Q and T16934A polymorphisms and Staphylococcus aureus nasal carriage. Anaesthesiol Intensive Ther. (2017) 49:110–5. doi: 10.5603/AIT.a2017.0027
122. Shi G, Wang T, Li S, Cheng Y, Sheng P, Fan Y, et al. TLR2 and TLR4 polymorphisms in Southern Chinese psoriasis vulgaris patients. J Dermatol Sci. (2016) 83:145–7. doi: 10.1016/j.jdermsci.2016.04.014
123. Sabah-Ozcan S, Gurel G. The polymorphism rs4696480 in the TLR2 gene is associated with psoriasis patients in the Turkish population. Immunol Lett. (2019) 211:28–32. doi: 10.1016/j.imlet.2019.05.008
124. Junjie X, Songyao J, Minmin S, Yanyan S, Baiyong S, Xiaxing D, et al. The association between Toll-like receptor 2 single-nucleotide polymorphisms and hepatocellular carcinoma susceptibility. BMC Cancer. (2012) 12:57. doi: 10.1186/1471-2407-12-57
125. Purdue MP, Lan Q, Wang SS, Kricker A, Menashe I, Zheng TZ, et al. A pooled investigation of Toll-like receptor gene variants and risk of non-Hodgkin lymphoma. Carcinogenesis. (2009) 30:275–81. doi: 10.1093/carcin/bgn262
126. de Barros Gallo C, Marichalar-Mendia X, Setien-Olarra A, Acha-Sagredo A, Bediaga NG, Gainza-Cirauqui ML, et al. Toll-like receptor 2 rs4696480 polymorphism and risk of oral cancer and oral potentially malignant disorder. Arch Oral Biol. (2017) 82:109–14. doi: 10.1016/j.archoralbio.2017.06.003
127. Lee J, Choi J, Chung S, Park J, Kim JE, Sung H, et al. Genetic predisposition of polymorphisms in HMGB1-related genes to breast cancer prognosis in Korean women. J Breast Cancer. (2017) 20:27–34. doi: 10.4048/jbc.2017.20.1.27
128. Zaki HY, Leung KH, Yiu WC, Gasmelseed N, Elwali NE, Yip SP. Common polymorphisms in TLR4 gene associated with susceptibility to pulmonary tuberculosis in the Sudanese. Int J Tuberc Lung Dis. (2012) 16:934–40. doi: 10.5588/ijtld.11.0517
129. Wang H, Wei Y, Zeng Y, Qin Y, Xiong B, Qin G, et al. The association of polymorphisms of TLR4 and CD14 genes with susceptibility to sepsis in a Chinese population. BMC Med Genet. (2014) 15:123. doi: 10.1186/s12881-014-0123-4
130. Mansur A, von Gruben L, Popov AF, Steinau M, Bergmann I, Ross D, et al. The regulatory toll-like receptor 4 genetic polymorphism rs11536889 is associated with renal, coagulation and hepatic organ failure in sepsis patients. J Transl Med. (2014) 12:177. doi: 10.1186/1479-5876-12-177
131. Taylor BD, Darville T, Ferrell RE, Kammerer CM, Ness RB, Haggerty CL. Variants in toll-like receptor 1 and 4 genes are associated with Chlamydia trachomatis among women with pelvic inflammatory disease. J Infect Dis. (2012) 205:603–9. doi: 10.1093/infdis/jir822
132. Agnese DM, Calvano JE, Hahm SJ, Coyle SM, Corbett SA, Calvano SE, et al. Human toll-like receptor 4 mutations but not CD14 polymorphisms are associated with an increased risk of gram-negative infections. J Infect Dis. (2002) 186:1522–5. doi: 10.1086/344893
133. Sampath V, Mulrooney NP, Garland JS, He J, Patel AL, Cohen JD, et al. Toll-like receptor genetic variants are associated with Gram-negative infections in VLBW infants. J Perinatol. (2013) 33:772–7. doi: 10.1038/jp.2013.80
134. Wagner J, Skinner NA, Catto-Smith AG, Cameron DJ, Michalski WP, Visvanathan K, et al. TLR4, IL10RA, and NOD2 mutation in paediatric Crohn's disease patients: an association with Mycobacterium avium subspecies paratuberculosis and TLR4 and IL10RA expression. Med Microbiol Immunol. (2013) 202:267–76. doi: 10.1007/s00430-013-0290-5
135. Tal G, Mandelberg A, Dalal I, Cesar K, Somekh E, Tal A, et al. Association between common Toll-like receptor 4 mutations and severe respiratory syncytial virus disease. J Infect Dis. (2004) 189:2057–63. doi: 10.1086/420830
136. da Silva Santos S, Clark TG, Campino S, Suarez-Mutis MC, Rockett KA, Kwiatkowski DP, et al. Investigation of host candidate malaria-associated risk/protective SNPs in a Brazilian amazonian population. PLoS ONE. (2012) 7:e36692. doi: 10.1371/journal.pone.0036692
137. Hawn TR, Scholes D, Li SS, Wang H, Yang Y, Roberts PL, et al. Toll-like receptor polymorphisms and susceptibility to urinary tract infections in adult women. PLoS ONE. (2009) 4:e5990. doi: 10.1371/journal.pone.0005990
138. Carvalho A, Pasqualotto AC, Pitzurra L, Romani L, Denning DW, Rodrigues F. Polymorphisms in toll-like receptor genes and susceptibility to pulmonary aspergillosis. J Infect Dis. (2008) 197:618–21. doi: 10.1086/526500
139. Al-Qahtani AA, Al-Anazi MR, Al-Zoghaibi F, Abdo AA, Sanai FM, Khan MQ, et al. The association of toll-like receptor 4 polymorphism with hepatitis C virus infection in Saudi Arabian patients. Biomed Res Int. (2014) 2014:357062. doi: 10.1155/2014/357062
140. Cussigh A, Fabris C, Fattovich G, Falleti E, Cmet S, Bitetto D, et al. Toll like receptor 4 D299G associates with disease progression in caucasian patients with chronic HBV infection: relationship with gender. J Clin Immunol. (2013) 33:313–6. doi: 10.1007/s10875-012-9822-9
141. Wu JF, Chen CH, Ni YH, Lin YT, Chen HL, Hsu HY, et al. Toll-like receptor and hepatitis B virus clearance in chronic infected patients: a long-term prospective cohort study in Taiwan. J Infect Dis. (2012) 206:662–8. doi: 10.1093/infdis/jis420
142. Dhiman N, Ovsyannikova IG, Vierkant RA, Ryan JE, Pankratz VS, Jacobson RM, et al. Associations between SNPs in toll-like receptors and related intracellular signaling molecules and immune responses to measles vaccine: preliminary results. Vaccine. (2008) 26:1731–6. doi: 10.1016/j.vaccine.2008.01.017
143. Hafren L, Einarsdottir E, Kentala E, Hammaren-Malmi S, Bhutta MF, MacArthur CJ, et al. Predisposition to childhood otitis media and genetic polymorphisms within the toll-like receptor 4 (TLR4) locus. PLoS ONE. (2015) 10:e0132551. doi: 10.1371/journal.pone.0132551
144. Sode J, Bank S, Vogel U, Andersen PS, Sorensen SB, Bojesen AB, et al. Genetically determined high activities of the TNF-alpha, IL23/IL17, and NFkB pathways were associated with increased risk of ankylosing spondylitis. BMC Med Genet. (2018) 19:165. doi: 10.1186/s12881-018-0680-z
145. Yang H, Wei C, Li Q, Shou T, Yang Y, Xiao C, et al. Association of TLR4 gene non-missense single nucleotide polymorphisms with rheumatoid arthritis in Chinese Han population. Rheumatol Int. (2013) 33:1283–8. doi: 10.1007/s00296-012-2536-8
146. Palomino-Morales R, Torres O, Vazquez-Rodriguez TR, Morado IC, Castaneda S, Callejas-Rubio JL, et al. Association between toll-like receptor 4 gene polymorphism and biopsy-proven giant cell arteritis. J Rheumatol. (2009) 36:1501–6. doi: 10.3899/jrheum.081286
147. van Rijn BB, Franx A, Steegers EA, de Groot CJ, Bertina RM, Pasterkamp G, et al. Maternal TLR4 and NOD2 gene variants, pro-inflammatory phenotype and susceptibility to early-onset preeclampsia and HELLP syndrome. PLoS ONE. (2008) 3:e1865. doi: 10.1371/journal.pone.0001865
148. Hwang YH, Ro H, Choi I, Kim H, Oh KH, Hwang JI, et al. Impact of polymorphisms of TLR4/CD14 and TLR3 on acute rejection in kidney transplantation. Transplantation. (2009) 88:699–705. doi: 10.1097/TP.0b013e3181b2f34a
149. Palmer SM, Burch LH, Davis RD, Herczyk WF, Howell DN, Reinsmoen NL, et al. The role of innate immunity in acute allograft rejection after lung transplantation. Am J Respir Crit Care Med. (2003) 168:628–32. doi: 10.1164/rccm.200303-447OC
150. Castano-Rodriguez N, Kaakoush NO, Pardo AL, Goh KL, Fock KM, Mitchell HM. Genetic polymorphisms in the Toll-like receptor signalling pathway in Helicobacter pylori infection and related gastric cancer. Hum Immunol. (2014) 75:808–15. doi: 10.1016/j.humimm.2014.06.001
151. Fan YF, Wu YM, Liu H, Yu Y, Jiang YY, Xue YZ, et al. TLR4 polymorphisms associated with developing gastric pre-cancer lesions in a Chinese Han population. Hum Immunol. (2014) 75:176–81. doi: 10.1016/j.humimm.2013.11.002
152. Li P, He CY, Xu Q, Sun LP, Ha MW, Yuan Y. Effect of the−2081G/A polymorphism of the TLR4 gene and its interaction with Helicobacter pylori infection on the risk of gastric cancer in Chinese individuals. Genet Test Mol Biomarkers. (2014) 18:610–5. doi: 10.1089/gtmb.2014.0047
153. Huang L, Yuan K, Liu J, Ren X, Dong X, Tian W, et al. Polymorphisms of the TLR4 gene and risk of gastric cancer. Gene. (2014) 537:46–50. doi: 10.1016/j.gene.2013.12.030
154. de Oliveira JG, Rossi AF, Nizato DM, Miyasaki K, Silva AE. Profiles of gene polymorphisms in cytokines and Toll-like receptors with higher risk for gastric cancer. Dig Dis Sci. (2013) 58:978–88. doi: 10.1007/s10620-012-2460-5
155. Jiang ZC, Tang XM, Zhao YR, Zheng L. A functional variant at miR-34a binding site in toll-like receptor 4 gene alters susceptibility to hepatocellular carcinoma in a Chinese Han population. Tumor Biol. (2014) 35:12345–52. doi: 10.1007/s13277-014-2547-z
156. Cheng I, Plummer SJ, Casey G, Witte JS. Toll-like receptor 4 genetic variation and advanced prostate cancer risk. Cancer Epidemiol Biomark Prev. (2007) 16:352–5. doi: 10.1158/1055-9965.EPI-06-0429
157. Kim HJ, Bae JS, Chang IH, Kim KD, Lee J, Shin HD, et al. Sequence variants of Toll-like receptor 4 (TLR4) and the risk of prostate cancer in Korean men. World J Urol. (2012) 30:225–32. doi: 10.1007/s00345-011-0690-3
158. Song J, Kim DY, Kim CS, Kim HJ, Lee DH, Lee HM, et al. The association between Toll-like receptor 4 (TLR4) polymorphisms and the risk of prostate cancer in Korean men. Cancer Genet Cytogenet. (2009) 190:88–92. doi: 10.1016/j.cancergencyto.2008.12.011
159. Tsilidis KK, Helzlsouer KJ, Smith MW, Grinberg V, Hoffman-Bolton J, Clipp SL, et al. Association of common polymorphisms in IL10, and in other genes related to inflammatory response and obesity with colorectal cancer. Cancer Causes Control. (2009) 20:1739–51. doi: 10.1007/s10552-009-9427-7
160. Gu X, Shen Y, Fu L, Zuo HY, Yasen H, He P, et al. Polymorphic variation of inflammation-related genes and risk of non-hodgkin lymphoma for Uygur and Han Chinese in Xinjiang. Asian Pac J Cancer Prev. (2014) 15:9177–83. doi: 10.7314/APJCP.2014.15.21.9177
161. Capparelli R, De Chiara F, Di Matteo A, Medaglia C, Iannelli D. The MyD88 rs6853 and TIRAP rs8177374 polymorphic sites are associated with resistance to human pulmonary tuberculosis. Genes Immun. (2013) 14:504–11. doi: 10.1038/gene.2013.48
162. Chen Z, Nakajima T, Inoue Y, Kudo T, Jibiki M, Iwai T, et al. A single nucleotide polymorphism in the 3'-untranslated region of MyD88 gene is associated with Buerger disease but not with takayasu arteritis in Japanese. J Hum Genet. (2011) 56:545–7. doi: 10.1038/jhg.2011.44
163. Potter C, Cordell HJ, Barton A, Daly AK, Hyrich KL, Mann DA, et al. Association between anti-tumour necrosis factor treatment response and genetic variants within the TLR and NF{kappa}B signalling pathways. Ann Rheum Dis. (2010) 69:1315–20. doi: 10.1136/ard.2009.117309
164. Yang Y, Yang S, Chen Z, Liu L. Correlation between TICAM1 gene polymorphisms and community-acquired pneumonia in children. J Biochem Mol Toxicol. (2020) 34:e22503. doi: 10.1002/jbt.22503
165. Sigurdson AJ, Brenner AV, Roach JA, Goudeva L, Muller JA, Nerlich K, et al. Selected single-nucleotide polymorphisms in FOXE1, SERPINA5, FTO, EVPL, TICAM1 and SCARB1 are associated with papillary and follicular thyroid cancer risk: replication study in a German population. Carcinogenesis. (2016) 37:677–84. doi: 10.1093/carcin/bgw047
166. Nabel GJ, Verma IM. Proposed NF-kappa B/I kappa B family nomenclature. Genes Dev. (1993) 7:2063. doi: 10.1101/gad.7.11.2063
167. Lai HM, Li XM, Yang YN, Ma YT, Xu R, Pan S, et al. Genetic Variation in NFKB1 and NFKBIA and susceptibility to coronary artery disease in a Chinese Uygur population. PLoS ONE. (2015) 10:e0129144. doi: 10.1371/journal.pone.0129144
168. Lai H, Chen Q, Li X, Ma Y, Xu R, Zhai H, et al. Association between genetic polymorphism in NFKB1 and NFKBIA and coronary artery disease in a Chinese Han population. Int J Clin Exp Med. (2015) 8:21487–96.
169. Guo XL, Liu XC, Su GB, Zhou CY, Cui QT. Association of NF-kappaB1 gene polymorphisms with coronary artery disease in a Han Chinese population. Genet Mol Res. (2016) 15:1–7. doi: 10.4238/gmr.15038072
170. Yang YN, Zhang JY, Ma YT, Xie X, Li XM, Liu F, et al.−94 ATTG insertion/deletion polymorphism of the NFKB1 gene is associated with coronary artery disease in Han and Uygur women in China. Genet Test Mol Biomarkers. (2014) 18:430–8. doi: 10.1089/gtmb.2013.0431
171. Jin SY, Luo JY, Li XM, Liu F, Ma YT, Gao XM, et al. NFKB1 gene rs28362491 polymorphism is associated with the susceptibility of acute coronary syndrome. Biosci Rep. (2019) 39:BSR20182292. doi: 10.1042/BSR20182292
172. Zhou B, Rao L, Peng Y, Wang Y, Li Y, Gao L, et al. Functional polymorphism of the NFKB1 gene promoter is related to the risk of dilated cardiomyopathy. BMC Med Genet. (2009) 10:47. doi: 10.1186/1471-2350-10-47
173. Kim SK, Jang HM, Kim DY. The promoter polymorphism of NFKB1 gene contributes to susceptibility of ischemic stroke in Korean population. J Exerc Rehabil. (2018) 14:1096–100. doi: 10.12965/jer.1836592.296
174. Fan HZ, Huang P, Shao JG, Tian T, Li J, Zang F, et al. Genetic variation on the NFKB1 genes associates with the outcomes of HCV infection among Chinese Han population. Infect Genet Evol. (2018) 65:210–5. doi: 10.1016/j.meegid.2018.07.031
175. Oner T, Yenmis G, Tombulturk K, Cam C, Kucuk OS, Yakicier MC, et al. Association of Pre-miRNA-499 rs3746444 and Pre-miRNA-146a rs2910164 polymorphisms and susceptibility to Behcet's disease. Genet Test Mol Biomarkers. (2015) 19:424–30. doi: 10.1089/gtmb.2015.0016
176. Gao M, Wang CH, Sima X, Han XM. NFKB1−94 insertion/deletion ATTG polymorphism contributes to risk of systemic lupus erythematosus. DNA Cell Biol. (2012) 31:611–5. doi: 10.1089/dna.2011.1389
177. Vu D, Tellez-Corrales E, Sakharkar P, Kissen MS, Shah T, Hutchinson I, et al. Impact of NF-kappaB gene polymorphism on allograft outcome in hispanic renal transplant recipients. Transpl Immunol. (2013) 28:18–23. doi: 10.1016/j.trim.2012.11.001
178. Mohd Suzairi MS, Tan SC, Ahmad Aizat AA, Mohd Aminudin M, Siti Nurfatimah MS, Andee ZD, et al. The functional-94 insertion/deletion ATTG polymorphism in the promoter region of NFKB1 gene increases the risk of sporadic colorectal cancer. Cancer Epidemiol. (2013) 37:634–8. doi: 10.1016/j.canep.2013.05.007
179. Andersen V, Christensen J, Overvad K, Tjonneland A, Vogel U. Polymorphisms in NFkB, PXR, LXR and risk of colorectal cancer in a prospective study of Danes. BMC Cancer. (2010) 10:484. doi: 10.1186/1471-2407-10-484
180. Chang ET, Birmann BM, Kasperzyk JL, Conti DV, Kraft P, Ambinder RF, et al. Polymorphic variation in NFKB1 and other aspirin-related genes and risk of hodgkin lymphoma. Cancer Epidemiol Biomark Prev. (2009) 18:976–86. doi: 10.1158/1055-9965.EPI-08-1130
181. Cerhan JR, Liu-Mares W, Fredericksen ZS, Novak AJ, Cunningham JM, Kay NE, et al. Genetic variation in tumor necrosis factor and the nuclear factor-kappaB canonical pathway and risk of non-hodgkin's lymphoma. Cancer Epidemiol Biomark Prev. (2008) 17:3161–9. doi: 10.1158/1055-9965.EPI-08-0536
182. Zhou B, Qie M, Wang Y, Yan L, Zhang Z, Liang A, et al. Relationship between NFKB1−94 insertion/deletion ATTG polymorphism and susceptibility of cervical squamous cell carcinoma risk. Ann Oncol. (2010) 21:506–11. doi: 10.1093/annonc/mdp507
183. Gao J, Xu HL, Gao S, Zhang W, Tan YT, Rothman N, et al. Genetic polymorphism of NFKB1 and NFKBIA genes and liver cancer risk: a nested case-control study in Shanghai, China. BMJ Open. (2014) 4:e004427. doi: 10.1136/bmjopen-2013-004427
184. Wang X, Peng H, Liang Y, Sun R, Wei T, Li Z, et al. A functional insertion/deletion polymorphism in the promoter region of the NFKB1 gene increases the risk of papillary thyroid carcinoma. Genet Test Mol Biomarkers. (2015) 19:167–71. doi: 10.1089/gtmb.2014.0271
185. Sapcharoen K, Sanguansermsri P, Yasothornsrikul S, Muisuk K, Srikummool M. Gene combination of CD44 rs187116, CD133 rs2240688, NF-kappaB1 rs28362491 and GSTM1 deletion as a potential biomarker in risk prediction of breast cancer in lower Northern Thailand. Asian Pac J Cancer Prev. (2019) 20:2493–502. doi: 10.31557/APJCP.2019.20.8.2493
186. Shiels MS, Engels EA, Shi J, Landi MT, Albanes D, Chatterjee N, et al. Genetic variation in innate immunity and inflammation pathways associated with lung cancer risk. Cancer. (2012) 118:5630–6. doi: 10.1002/cncr.27605
187. Manuel Sanchez-Maldonado J, Martinez-Bueno M, Canhao H, Ter Horst R, Munoz-Pena S, Moniz-Diez A, et al. NFKB2 polymorphisms associate with the risk of developing rheumatoid arthritis and response to TNF inhibitors: results from the REPAIR consortium. Sci Rep. (2020) 10:4316. doi: 10.1038/s41598-020-61331-5
188. Pare G, Ridker PM, Rose L, Barbalic M, Dupuis J, Dehghan A, et al. Genome-wide association analysis of soluble ICAM-1 concentration reveals novel associations at the NFKBIK, PNPLA3, RELA, and SH2B3 loci. PLoS Genet. (2011) 7:e1001374. doi: 10.1371/journal.pgen.1001374
189. Gregersen PK, Amos CI, Lee AT, Lu Y, Remmers EF, Kastner DL, et al. REL, encoding a member of the NF-kappaB family of transcription factors, is a newly defined risk locus for rheumatoid arthritis. Nat Genet. (2009) 41:820–3. doi: 10.1038/ng.395
190. Eyre S, Hinks A, Flynn E, Martin P, Wilson AG, Maxwell JR, et al. Confirmation of association of the REL locus with rheumatoid arthritis susceptibility in the UK population. Ann Rheum Dis. (2010) 69:1572–3. doi: 10.1136/ard.2009.122887
191. Bowes J, Ho P, Flynn E, Ali F, Marzo-Ortega H, Coates LC, et al. Comprehensive assessment of rheumatoid arthritis susceptibility loci in a large psoriatic arthritis cohort. Ann Rheum Dis. (2012) 71:1350–4. doi: 10.1136/annrheumdis-2011-200802
192. Ali FR, Barton A, Smith RL, Bowes J, Flynn E, Mangino M, et al. An investigation of rheumatoid arthritis loci in patients with early-onset psoriasis validates association of the REL gene. Br J Dermatol. (2013) 168:864–6. doi: 10.1111/bjd.12106
193. Trynka G, Zhernakova A, Romanos J, Franke L, Hunt KA, Turner G, et al. Coeliac disease-associated risk variants in TNFAIP3 and REL implicate altered NF-kappaB signalling. Gut. (2009) 58:1078–83. doi: 10.1136/gut.2008.169052
194. He S, Yang S, Zhao Q, Wang L, Liu H, Sheng Y, et al. Association of IL4, IL6, and IL10 polymorphisms with pulmonary tuberculosis in a Tibetan Chinese population. Oncotarget. (2018) 9:16418–26. doi: 10.18632/oncotarget.23995
195. O'Mahony DS, Glavan BJ, Holden TD, Fong C, Black RA, Rona G, et al. Inflammation and immune-related candidate gene associations with acute lung injury susceptibility and severity: a validation study. PLoS ONE. (2012) 7:e51104. doi: 10.1371/journal.pone.0051104
196. Li F, Xu J, Zheng J, Sokolove J, Zhu K, Zhang Y, et al. Association between interleukin-6 gene polymorphisms and rheumatoid arthritis in Chinese Han population: a case-control study and a meta-analysis. Sci Rep. (2014) 4:5714. doi: 10.1038/srep05714
197. Bamoulid J, Courivaud C, Deschamps M, Mercier P, Ferrand C, Penfornis A, et al. IL-6 promoter polymorphism−174 is associated with new-onset diabetes after transplantation. J Am Soc Nephrol. (2006) 17:2333–40. doi: 10.1681/ASN.2006010066
198. Lu KC, Jaramillo A, Lecha RL, Schuessler RB, Aloush A, Trulock EP, et al. Interleukin-6 and interferon-gamma gene polymorphisms in the development of bronchiolitis obliterans syndrome after lung transplantation. Transplantation. (2002) 74:1297–302. doi: 10.1097/00007890-200211150-00017
199. Muller-Steinhardt M, Hartel C, Muller B, Kirchner H, Fricke L. The interleukin-6−174promoter polymorphism is associated with long-term kidney allograft survival. Kidney Int. (2002) 62:1824–7. doi: 10.1046/j.1523-1755.2002.00609.x
200. Jeng JR, Wang JH, Liu WS, Chen SP, Chen MY, Wu MH, et al. Association of interleukin-6 gene G-174C polymorphism and plasma plasminogen activator inhibitor-1 level in Chinese patients with and without hypertension. Am J Hypertens. (2005) 18:517–22. doi: 10.1016/j.amjhyper.2004.10.028
201. Grocott HP, White WD, Morris RW, Podgoreanu MV, Mathew JP, Nielsen DM, et al. Genetic polymorphisms and the risk of stroke after cardiac surgery. Stroke. (2005) 36:1854–8. doi: 10.1161/01.STR.0000177482.23478.dc
202. Cole JW, Brown DW, Giles WH, Stine OC, O'Connell JR, Mitchell BD, et al. Ischemic stroke risk, smoking, and the genetics of inflammation in a biracial population: the stroke prevention in young women study. Thromb J. (2008) 6:11. doi: 10.1186/1477-9560-6-11
203. Hussain S, Iqbal T, Sadiq I, Feroz S, Shafique Satti H. Polymorphism in the IL-8 gene promoter and the risk of acne vulgaris in a Pakistani population. Iran J Allergy Asthma Immunol. (2015) 14:443–9.
204. Scarel-Caminaga RM, Kim YJ, Viana AC, Curtis KM, Corbi SC, Sogumo PM, et al. Haplotypes in the interleukin 8 gene and their association with chronic periodontitis susceptibility. Biochem Genet. (2011) 49:292–302. doi: 10.1007/s10528-010-9407-3
205. Lupianez CB, Canet LM, Carvalho A, Alcazar-Fuoli L, Springer J, Lackner M, et al. Polymorphisms in host immunity-modulating genes and risk of invasive aspergillosis: results from the AspBIOmics consortium. Infect Immun. (2015) 84:643–57. doi: 10.1128/IAI.01359-15
206. Ahn MH, Park BL, Lee SH, Park SW, Park JS, Kim DJ, et al. A promoter SNP rs4073T>A in the common allele of the interleukin 8 gene is associated with the development of idiopathic pulmonary fibrosis via the IL-8 protein enhancing mode. Respir Res. (2011) 12:73. doi: 10.1186/1465-9921-12-73
207. Suh JS, Hahn WH, Cho BS. Polymorphisms of CXCL8 and its receptor CXCR2 contribute to the development and progression of childhood IgA nephropathy. J Interferon Cytokine Res. (2011) 31:309–15. doi: 10.1089/jir.2010.0031
208. Dan H, Liu W, Zhou Y, Wang J, Chen Q, Zeng X. Association of interleukin-8 gene polymorphisms and haplotypes with oral lichen planus in a Chinese population. Inflammation. (2010) 33:76–81. doi: 10.1007/s10753-009-9160-0
209. Charrad R, Kaabachi W, Rafrafi A, Berraies A, Hamzaoui K, Hamzaoui A. IL-8 gene variants and expression in childhood Asthma. Lung. (2017) 195:749–57. doi: 10.1007/s00408-017-0058-6
210. Gu LQ, Jia HY, Zhao YJ, Liu N, Wang S, Cui B, et al. Association studies of interleukin-8 gene in Graves' disease and Graves' ophthalmopathy. Endocrine. (2009) 36:452–6. doi: 10.1007/s12020-009-9240-9
211. Rafrafi A, Chahed B, Kaabachi S, Kaabachi W, Maalmi H, Hamzaoui K, et al. Association of IL-8 gene polymorphisms with non small cell lung cancer in tunisia: a case control study. Hum Immunol. (2013) 74:1368–74. doi: 10.1016/j.humimm.2013.06.033
212. Gonzalez-Hormazabal P, Romero S, Musleh M, Bustamante M, Stambuk J, Pisano R, et al. IL-8-251T>A (rs4073) Polymorphism is associated with prognosis in gastric cancer patients. Anticancer Res. (2018) 38:5703–8. doi: 10.21873/anticanres.12907
213. Zhang Q, Bastard P, Liu Z, Le Pen J, Moncada-Velez M, Chen J, et al. Inborn errors of type I IFN immunity in patients with life-threatening COVID-19. Science. (2020) 370. doi: 10.1126/science.abd4570
214. Ellinghaus D, Degenhardt F, Bujanda L, Buti M, Albillos A, Invernizzi P, et al. Genomewide association study of severe Covid-19 with respiratory failure. N Engl J Med. (2020) 383:1522–34. doi: 10.1056/NEJMoa2020283
215. Zhao J, Yang Y, Huang H, Li D, Gu D, Lu X, et al. Relationship between the ABO blood group and the COVID-19 susceptibility. Clin Infect Dis. (2020) ciaa1150. doi: 10.1093/cid/ciaa1150
216. Zietz M, Tatonetti NP. Testing the association between blood type and COVID-19 infection, intubation, and death. medRxiv. (2020). doi: 10.1101/2020.04.08.20058073
217. Torkamani A, Wineinger NE, Topol EJ. The personal and clinical utility of polygenic risk scores. Nat Rev Genet. (2018) 19:581–90. doi: 10.1038/s41576-018-0018-x
218. Lambert SA, Abraham G, Inouye M. Towards clinical utility of polygenic risk scores. Hum Mol Genet. (2019) 28:R133–R42. doi: 10.1093/hmg/ddz187
219. Pairo-Castineira E, Clohisey S, Klaric L, Bretherick AD, Rawlik K, Pasko D, et al. Genetic mechanisms of critical illness in Covid-19. Nature. (2020). doi: 10.1038/s41586-020-03065-y. [Epub ahead of print].
220. Group RC, Horby P, Lim WS, Emberson JR, Mafham M, Bell JL, et al. Dexamethasone in hospitalized patients with Covid-19 - preliminary report. N Engl J Med. (2020). NEJMoa2021436. doi: 10.1056/NEJMoa2021436
221. Young MJ, Clyne CD, Chapman KE. Endocrine aspects of ACE2 regulation: RAAS, steroid hormones and SARS-CoV-2. J Endocrinol. (2020) 247:R45–62. doi: 10.1530/JOE-20-0260
Keywords: SARS-CoV-2, COVID-19, genetic susceptibility, genotype-phenotype association studies, viral entry, innate immunity, polygenic risk score, risk stratification
Citation: Grolmusz VK, Bozsik A, Papp J and Patócs A (2021) Germline Genetic Variants of Viral Entry and Innate Immunity May Influence Susceptibility to SARS-CoV-2 Infection: Toward a Polygenic Risk Score for Risk Stratification. Front. Immunol. 12:653489. doi: 10.3389/fimmu.2021.653489
Received: 14 January 2021; Accepted: 16 February 2021;
Published: 08 March 2021.
Edited by:
Jeane E. L. Visentainer, State University of Maringá, BrazilReviewed by:
Paulo Rodrigues-Santos, University of Coimbra, PortugalAna Maria Sell, State University of Maringá, Brazil
Sokratis A. Apostolidis, Hospital of the University of Pennsylvania, United States
Copyright © 2021 Grolmusz, Bozsik, Papp and Patócs. This is an open-access article distributed under the terms of the Creative Commons Attribution License (CC BY). The use, distribution or reproduction in other forums is permitted, provided the original author(s) and the copyright owner(s) are credited and that the original publication in this journal is cited, in accordance with accepted academic practice. No use, distribution or reproduction is permitted which does not comply with these terms.
*Correspondence: Attila Patócs, cGF0b2NzLmF0dGlsYUBtZWQuc2VtbWVsd2Vpcy11bml2Lmh1